- 1Institute of Toxicology, University Medical Center, Mainz, Germany
- 2Department of Neurology, Sozialstiftung, Bamberg, Germany
Radiation concomitant with the DNA methylating drug temozolomide (TMZ) is the gold standard in the treatment of glioblastoma. In this adjuvant setting, TMZ is regarded to be a radiation sensitizer. However, similar to ionising radiation, TMZ induces DNA double-strand breaks and is itself a potent trigger of apoptosis, cellular senescence and autophagy, suggesting that radiation and TMZ act independently. Although cell culture experiments yielded heterogeneous results, some data indicate that the cytotoxic effect of radiation was only enhanced when TMZ was given before radiation treatment. Based on the molecular mechanism of action of TMZ, the importance of specific TMZ and radiation-induced DNA lesions, their repair as well as their interactions, possible scenarios for an additive or synergistic effect of TMZ and radiation are discussed, and suggestions for an optimal timing of radio-chemical treatments are proposed.
Introduction
Temozolomide (TMZ), an orally deliverable alkylating chemotherapeutic agent, is essential part of the standard treatment of glioblastoma (GBM). It is applied after tumor resection in a dose of 75 mg/m2 once per day, concomitantly with fractionated ionizing radiation (IR), with 2 Gy per fraction, cumulating up to 60 Gy (1). After radio-chemotherapy, adjuvant treatment consists of TMZ at higher dose (150-200 mg/m2, six cycles day 1-5/28) (1). At relapse, several modified protocols are applied that deviate from this classic “Stupp” regimen (2). Dose-dense schedules consist of TMZ at lower doses, i.e. 150 mg/m2 day 1-7/14, 100 mg/m2 day 1-21/28, 100 mg/m2 day 1-5/7 or 50 mg/m2 administered continuously (3). Even at these low dose levels, TMZ is effective, as indicated by clinical studies (4, 5). Moreover, at least in O6-methylguanine-DNA methyltransferase (MGMT)-deficient tumors, repeated TMZ treatments very likely lead to an accumulation of critical DNA damages that trigger cell death, which is supported by our studies on glioblastoma cells in vitro (6). The concurrent administration of TMZ with IR results in a prolongation of overall survival (OS) and progression free survival (PFS) by a few months (1).
An alternative therapy regimen is based on lomustine (CCNU), a chloroethylating agent that induces O6-chloroethylguanine and subsequently interstrand-crosslinks in the DNA (7). It is administered at day 1, followed by TMZ day 2-6 of the 6-week cycles. In this setting, the PFS is identical to the Stupp protocol, but the OS is increased, albeit at the expense of additional side effects (8). In this setting, IR is not included even though it is conceivable that IR together with lomustine ameliorates glioblastoma cell death, given the cell death pathway triggered by chloroethylating agents (7). However, it is expected that the side effects in the irradiation field are more severe since the toxicity of lomustine and IR is likely not restricted to the proliferating tumor cell pool, but also to the non-proliferating healthy brain tissue. In contrast, the toxic effect of TMZ is strictly limited on replicating cells (9) and, therefore, specifically affects the proliferating tumor cell population. Nearly nothing is known about the complex interaction of TMZ, IR and lomustine-induced DNA damage and signaling.
Tmz and Radiation
In chemoradiation, TMZ is believed to be a radiosensitizer (10). This view is based on a series of experimental studies which, taken together, led to the conclusion that pretreatment with TMZ or simultaneous treatment with TMZ and IR increases cytotoxicity above the additive effect. Actually, these studies are quite heterogeneous, ranging from additive to near-synergistic effects (11–15). Thus, treatment with a low dose of TMZ 2 h before irradiation exacerbated the cytotoxic effects that were described as supra-additive, which was not the case when TMZ was administered after IR (11). We repeated this experiment and were able to confirm that TMZ pretreatment enhances the level of IR-induced apoptosis. However, this was only the case if a high dose of TMZ was used (100 µM instead of 20 µM). It is important to note that in this setting IR (6 Gy) and TMZ alone did not enhance apoptosis (which was measured 5 h after IR treatment) above the control level (Figure 1A).
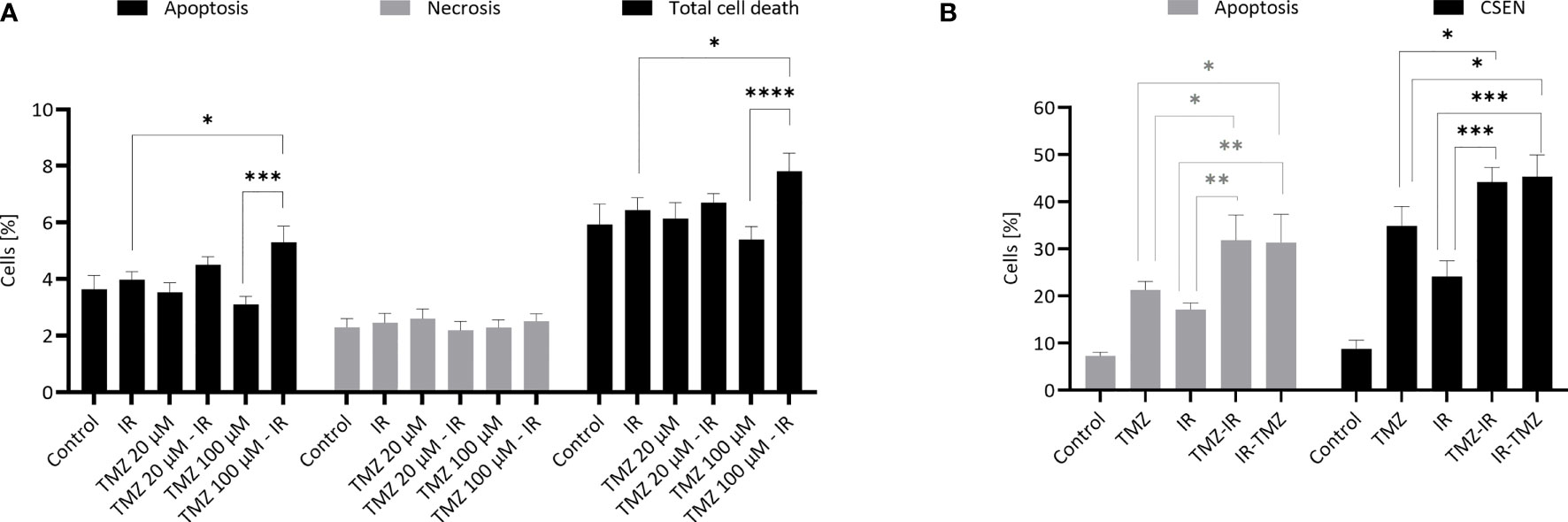
Figure 1 TMZ and IR- induced apoptosis and senescence. (A) Effect of TMZ pretreatment on radiation-induced apoptosis. Human LN229 glioblastoma cells in the exponential growth phase were treated with TMZ (20 or 100 µM) and 3 h later irradiated with γ-rays (6 Gy). Apoptosis (annexin V+, PI-), late apoptosis/necrosis (annexin V+, PI+) and total cell death were measured 5 h later by flow cytometry. Data are the mean of 4 independent experiments +/- SEM. *p < 0.05; ***< 0.001. (B) Effect of IR pre- and post-treatment on TMZ-induced apoptosis and cellular senescence. Human LN229 glioblastoma cells in the exponential growth phase were treated with TMZ (20 µM) and 3 h later irradiated with γ-rays (6 Gy) (TMZ-IR). In a parallel setting, they were irradiated (6 Gy) and 6 h later treated with TMZ (20 µM) (IR-TMZ). Apoptosis (early apoptosis: annexin V+/PI-; late apoptosis: annexin V+/PI+) and senescence (C12FDG+) were measured 7 d later by flow cytometry. Data are the mean of 3-4 independent experiments +/- SEM. *p < 0.05; **< 0.01; ***< 0.001 ; ****<0.0001. Experiments were essentially performed as previously described (16).
Apoptosis induced by TMZ in glioblastoma cells is a late response, starting 3 d after TMZ treatment and reaching a maximum 5 d later, while senescence reaches a plateau after 7 d (17). To see whether IR has an impact on TMZ-induced cell death, we treated glioblastoma cells with a therapeutically relevant low dose of 20 µM TMZ 2 h before or 6 h after IR (6 Gy) and measured apoptosis and cellular senescence (CSEN) 7 days later. Under these conditions, both TMZ and IR alone induced significant apoptosis. Interestingly, TMZ pre- and post-treatment were effective in enhancing the apoptosis level (Figure 1B). For the endpoint cellular senescence we also observed, similar to apoptosis, a significant increase (Figure 1B). However, the effects were less than additive, indicating that IR provokes rather an inhibiting effect on TMZ-induced genotoxic pathways. Overall, our data support the notion that the timing (pre-treatment) and the TMZ dose are critical as to the amelioration of the cytotoxic effect of IR.
Tmz - a Radiosensitizer?
Whether TMZ is a real radiosensitizer has not yet been critically questioned. As we know the mechanism of action of TMZ quite precisely (7, 18), it is pertinent to answer this question anew. A genuine radiosensitizer is characterized by provoking an increase in the radiation effect, whereby the radiosensitizer itself is not toxic (19). In tumor therapy, the intended endpoint of the biological radiation effect is death of the tumor cell, with the number of unrepaired DNA double-strand breaks (DSBs) being the critical, decisive factor (20). Theoretically, radiosensitization can occur through the following mechanisms: a) increase in the number of radiation-induced DSBs, b) reduced repair of the induced breaks resulting in an enhanced level of critical breaks, c) amelioration of the DNA damage response that triggers cell death, d) stimulation of pro-apoptotic pathways, e) inhibition of antiapoptotic pathways, f) inhibition of autophagy and cellular senescence, which counteract the cell death response.
Does TMZ meet these requirements? TMZ does not impact the repair of radiation-induced DSBs. However, it is conceivable that the genotoxic properties of TMZ itself contribute to an increase in the amount of toxic DSBs. How this can occur requires a closer look at the mode of action of TMZ. Chemically, the drug is a triazene derivative that does not need metabolic activation. It decomposes spontaneously in the cell yielding 3-methyl-(triazen-1-yl)imidazole-4-carboxamide and, in a second step, 5-aminoimidazole-4-carboxamide and monomethyl hydrazine, which finally methylates all nucleophilic centers in the cell. However, as revealed by studies with DNA repair mutants and isogenic cell lines (21), the main cytotoxic target of TMZ is the nuclear DNA. Similar to other SN1 alkylating agents, TMZ alkylates the DNA at 12 nucleophilic sites (22). The major methylation products are N-methylpurines such as N7-methylguanine, N3-methylguanine, and N3-methyladenine (comprising about 80% of total alkylation), whereas base oxygen methylations are less frequent. Thus, O6-methylguanine (O6MeG) accounts for only 7% of the total DNA alkylation. Although produced in minor amounts, O6MeG is the main mutagenic, carcinogenic, genotoxic, and cytotoxic lesion (23). It is also responsible for autophagy and cellular senescence, which are induced by TMZ concomitant to apoptosis and which counteract cell death (17, 24).
TMZ has a short half-life (about 2 h in serum) and therefore exposures can be considered pulse-treatments. O6MeG in the DNA arrives a peak level within 3-4 h after TMZ treatment of glioblastoma cells (16). In cells with the DNA repair protein MGMT the damage is repaired within minutes and therefore cannot develop any toxic effects. However, in tumor cells without MGMT, which are classified as promoter-methylated (25), O6MeG remains and accumulates following repeated treatments in the tumor cell DNA.
Molecular Mechanism of Tmz and Ir
The mechanisms of O6MeG-triggered cell death responses have been thoroughly investigated (26). O6MeG itself is not a cytotoxic DNA damage, but in proliferating cells the lesion results in O6MeG/thymine mismatches during DNA replication. These are recognized by the cell’s mismatch repair (MMR) system. The MMR proteins (MSH2, MSH6, MLH1 and PMS2) repair the damage by removing thymine, but the mispairing properties of O6MeG results in restoring the mismatch; the repair is on the spot, leading to futile repair cycles. This finally causes gaps in the DNA and, in a subsequent round of replication, inhibition of the DNA synthesis and open replication forks with vulnerable single-stranded DNA, whose cleavage by nucleases inevitably leads to DSBs. This occurs in the post-treatment cell cycle (i.e. two DNA replication cycles after induction of O6MeG are required), which is compatible with the time-course of apoptosis and DSB formation (27). Results obtained with synchronized cells confirmed this model (28). It was further shown that DSBs, induced by the processing of O6MeG/thymine, trigger complex DNA damage response pathways, which are activated primarily by ATR, and secondary by ATM, and downstream by CHK1 and CHK2, respectively (29), as well as activation of the SIAH1-HIPK2–p53 axis (30). In this scenario, the following factors determine drug resistance: MGMT, MMR, the proliferation level, DNA damage response (DDR) activation, and DSB repair by homologous recombination (HR). Repair of TMZ-induced DSBs by non-homologous end-joining (NHEJ) plays only a marginal role (31, 32). In contrast to TMZ, IR induces DSBs replication independently and the main pathway of DSB repair following IR in G1 is NHEJ (33). Non-repaired IR-induced DSB activate the DDR mainly via ATM, while O6MeG mediated replication blocks and DSBs activate both ATR and ATM, which trigger downstream cell death pathways (7, 29).
In view of this scenario, it is conceivable that the DSB rate in the tumor cells is increased, and cell death pathways are activated to a greater extent if TMZ is administered together with IR (see Figure 2A). This presupposes, however, that a) the tumor cells are MGMT-deficient, b) the tumor cells proliferate (since the conversion of O6MeG into DSB is strictly replication-dependent) and c) MMR as well as the DDR are not affected by IR. It should be noted that IR is a potent inducer of genes, which has also been discussed for the MGMT gene (34). However, MGMT silencing is caused by promoter methylation and IR would therefore have to change the promoter methylation status, which according to available data is not the case. Moreover, we have not been able to demonstrate any MGMT induction by IR in glioblastoma cells in vitro (35).
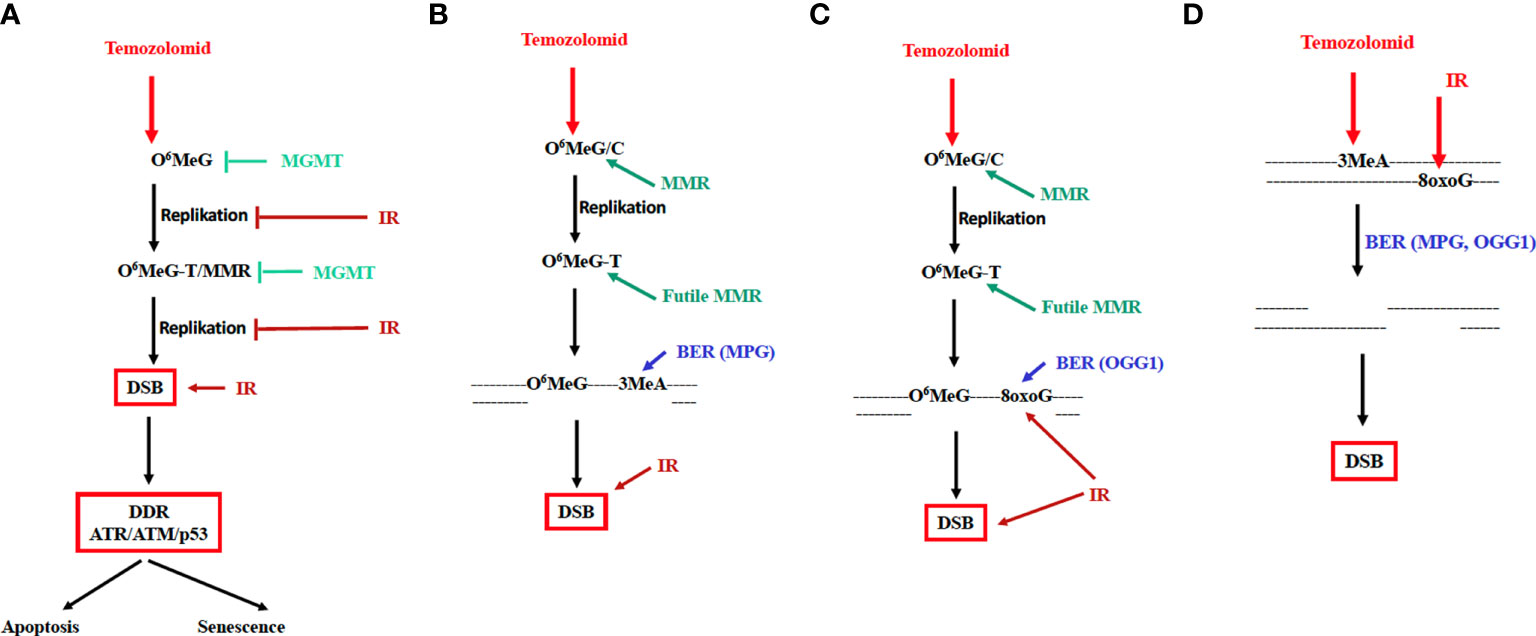
Figure 2 Mechanism of TMZ-induced cytotoxicity and cellular senescence and possible mode of interaction of TMZ and IR. MGMT and IR inhibiting the cytotoxic pathway are indicated. DDR: DNA damage response involving the kinases ATR, ATM and the transcription factor p53. (A) Genotoxic pathway triggered by O6MeG; futile MMR cycle model. (B) MMR and BER of N-methylation lesions cooperate in inducing DSBs. (C) MMR and BER of oxidative lesions cooperate in inducing DSBs. (D) DSBs are formed in overlapping base excision repair patches of lesions induced by TMZ and radiation. BER, long-patch base excision repair; MPG, N-methylpurine-DNA glycosylase; OGG1, 8-oxo-guanine-DNA glycosylase.
The possible influence of IR on mismatch repair, DNA synthesis and tumor cell proliferation is critical. In vitro, the MMR genes MSH2 and MSH6 were shown to be downregulated by IR (17). Whether this also occurs in vivo is unknown. Radiation inhibits cell cycle progression, arresting cells in the G1/S (36), and dose response curves do not display a no-effect threshold for DNA synthesis inhibition (37). It is therefore conceivable that DNA synthesis is inhibited already by a single therapeutic dose of 2 Gy, and even more so by repeated doses. Under these conditions TMZ will inevitably become ineffective since the conversion of O6MeG into DSB cannot take place. According to these considerations, radiation prior to the administration of TMZ would be counterproductive, at least if the radiation dose is sufficiently high to cause DNA synthesis inhibition through G1 blockage (Figure 2A). It is conceivable that concurrent daily treatments with 2 Gy plus TMZ may lead to even stronger inhibition of replication and therefore to a significant attenuation of the TMZ-induced cytotoxic response.
Possible Interactions Between Radiation- and Tmz-Induced Lesions
As already mentioned, experimental evidence suggests that treatment of GBM cells with TMZ + IR can have both synergistic and additive effects. A detailed study showed that dosage and timing are crucial. Using very low doses of TMZ, radiosensitization was only recorded when TMZ was given to the cells before irradiation; TMZ post-treatment had no effect (11). It was concluded that toxic N-alkylations induced by TMZ (such as 3-methyladenine) and O6MeG interact, leading to an increase in the DSB rate. A model based on this supposition is shown in Figure 2B. This model claims that base excision repair in O6MeG/T mismatch repair patches may lead to DSB formation. If one additionally assumes that following irradiation oxidative base damages (such as 8-oxoG or thymine glycol) were generated in the immediate vicinity of O6MeG, it is conceivable that MMR of O6MeG/T and, concomitantly, BER of oxidative damage (e.g. through the repair enzyme OGG1) in the O6MeG harboring strand lead to the formation of DSB (Figure 2C).
This model applies to radiation exposure in the TMZ post-treatment cell cycle. It does not explain, however, why pretreatment with TMZ has a radio-sensitizing effect (Figure 1A). An explanation for this finding rests on the supposition that base damage through alkylation (N7-MeG, N3-MeG, N3MeA) and oxidation (e.g. 8-oxoguanine) in overlapping BER patches may lead to DSB, similar to what was proposed for extensive alkylation damage in overlapping repair patches (38). Given a patch size of about 25 nucleotides during long-patch BER (39) this likely happens at high DNA alkylation and oxidation levels. Since BER peaks immediately after damage induction, DSB formation in overlapping BER patches is anticipated to occur in the first hours after treatment. This is entirely different from DSBs resulting from O6MeG/T processing, which results in a wave of DSB >3 days after TMZ treatment (own unpublished data). Interestingly, apoptosis induced by IR in TMZ pretreated glioblastoma cells can be observed already 5 h after irradiation. It is also important to note that a low dose of TMZ is insufficient; only with a high dose of 100 µM the yield of radiation-induced apoptosis was significantly enhanced (Figure 1A). This data confirms what was reported by Bobola et al. (11), and is compatible with the model shown in Figure 2D. The observation that TMZ pretreatment leads to radio-sensitization in both MGMT proficient and deficient cells, i.e. independent of O6MeG (11), further supports this model. In summary, TMZ appears to be a radiosensitizer when TMZ treatment occurs prior to irradiation.
It is important to note that apart from being a radiosensitizer, TMZ itself is a powerful cytotoxic agent that induces not only apoptosis but also autophagy and cellular senescence (24). Importantly, dose-response studies revealed that O6MeG adducts in the DNA, DSBs, apoptosis and senescence increase linearly with dose, without a clear threshold (16, 40). This supports the notion that low doses of TMZ are effective in MGMT lacking tumors. The cytotoxic potency of TMZ leads to the question of whether, conversely, IR can cause an amplification of the TMZ effect, i.e. acting as drug sensitizer. This is conceivable given the cell death cascade evoked by O6MeG. In this scenario, blocked replication forks that activate ATR, and DSBs generated through O6MeG/T-MMR activating ATM (29) are the decisive downstream events triggering the DDR (Figure 2A). If in this window additional DSBs were induced by radiation treatment, it is conceivable that the toxic effect of TMZ is ameliorated. However, own unpublished data showed that no synergistic, but at best only additive effects were recorded under these treatment conditions.
Conclusions
What are the conclusions regarding dose and timing in TMZ-radiotherapy? If we translate these considerations into the clinical application, we would like to suggest modification of the therapy protocol in a following way.
a) Given the facts that IR inhibits DNA synthesis and TMZ requires DNA replication, it is reasonable to conclude that the TMZ treatment should initially be carried out for 3 days without radiotherapy. This TMZ boost is anticipated to be most effective as it targets the proliferating glioblastoma cell population. For this boost a high dose (200 mg/m2/day), which is usually used for maintenance therapy, is recommended since it is expected to be effective also in cancer cells expressing MGMT at a very low level.
b) We further recommend starting the radio-chemotherapy cycle with a high dose of TMZ (200 mg/m2 + 2 Gy) for a short period (3 days) to enable DSBs to be formed according to the model in Figure 2D. After this TMZ-IR boost, therapy according to the classical scheme (TMZ 75 mg/m2 + RT 2 Gy) should be continued. With this boost TMZ-radiotherapy it is anticipated that in the initial phase of treatment the tumor cell cytotoxicity through TMZ can fully be expressed.
c) Given the fact that TMZ pretreatment is most effective in exerting radiosensitization, TMZ should be administered 2-4 hours before irradiation (the peak plasma level after oral TMZ is reached after about 2 hours and DNA alkylation is at its maximum after 3-4 hours).
d) Not only IR, but also TMZ causes cell cycle arrest, which limits apoptosis induction. If this immediate-early effect is transient (in contrast to senescence), it is anticipated that therapy-free intervals (of 2 days) may enhance the tumor-cytotoxic effect because of replication recovery. At the same time limiting side effects (notably hematotoxicity) might be reduced because of MGMT restoration in the stem cell compartment. Of note, CD35+ hematopoetic stem cells contain very low MGMT levels (41).
e) A benefit of concomitant TMZ-radiotherapy was recorded not only for promoter methylated, but also unmethylated (MGMT+) cases (42). This supports the view that an MGMT-independent minor pathway does exist, which is stimulated in the concurrent TMZ-radiotherapy setting (outlined in Figure 2D). Thus, on the basis of this model, a benefit of concurrent TMZ-radiotherapy is expected independent of the MGMT status.
f) Both IR and TMZ are strong inducers of cellular senescence, causing an irreversible proliferation arrest. This will necessarily negate the cytotoxicity of TMZ, although attenuating (transiently) tumor growth. Thus, the role of senescence-associated secretory phenotype, exhibited by glioblastoma cells upon TMZ treatment (17), for the progression of the disease remains to be established and the use of senolytic drugs in glioblastoma radio-chemo therapy a reasonable challenge.
Data Availability Statement
The original contributions presented in the study are included in the article, further inquiries can be directed to the corresponding author.
Author Contributions
BK: conceptualization, data curation, supervision, funding acquisition, and writing of the original draft. LB: experiments, quantification of apoptosis and senescence. HS: discussion, critical reading and improvements. All authors read and approved the submitted version of the manuscript.
Conflict of Interest
The authors declare that the research was conducted in the absence of any commercial or financial relationships that could be construed as a potential conflict of interest.
Publisher’s Note
All claims expressed in this article are solely those of the authors and do not necessarily represent those of their affiliated organizations, or those of the publisher, the editors and the reviewers. Any product that may be evaluated in this article, or claim that may be made by its manufacturer, is not guaranteed or endorsed by the publisher.
Acknowledgments
Work of BK is supported by Deutsche Forschungsgemeinschaft DFG KA724/31-1.
References
1. Stupp R, Hegi ME, Mason WP, van den Bent MJ, Taphoorn MJ, Janzer RC, et al. Effects of Radiotherapy With Concomitant and Adjuvant Temozolomide Versus Radiotherapy Alone on Survival in Glioblastoma in a Randomised Phase III Study: 5-Year Analysis of the EORTC-NCIC Trial. Lancet Oncol (2009) 10:459–66. doi: 10.1016/S1470-2045(09)70025-7
2. Wick W, Steinbach JP, Kuker WM, Dichgans J, Bamberg M, Weller M. One Week on/One Week Off: A Novel Active Regimen of Temozolomide for Recurrent Glioblastoma. Neurology (2004) 62:2113–5. doi: 10.1212/01.WNL.0000127617.89363.84
3. Strik HM, Marosi C, Kaina B, Neyns B. Temozolomide Dosing Regimens for Glioma Patients. Curr Neurol Neurosci Rep (2012) 12:286–93. doi: 10.1007/s11910-012-0262-y
4. Kong DS, Lee JI, Kim WS, Son MJ, Lim DH, Kim ST, et al. A Pilot Study of Metronomic Temozolomide Treatment in Patients With Recurrent Temozolomide-Refractory Glioblastoma. Oncol Rep (2006) 16:1117–21. doi: 10.3892/or.16.5.1117
5. Zustovich F, Landi L, Lombardi G, Porta C, Galli L, Fontana A, et al. Sorafenib Plus Daily Low-Dose Temozolomide for Relapsed Glioblastoma: A Phase II Study. Anticancer Res (2013) 33:3487–94.
6. Beltzig L, Stratenwerth B, Kaina B. Accumulation of Temozolomide-Induced Apoptosis, Senescence and DNA Damage by Metronomic Dose Schedule: A Proof-Of-Principle Study With Glioblastoma Cells. Cancers (Basel) (2021) 13:1–9. doi: 10.3390/cancers13246287
7. Kaina B, Christmann M. DNA Repair in Personalized Brain Cancer Therapy With Temozolomide and Nitrosoureas. DNA Repair (Amst) (2019) 78:128–41. doi: 10.1016/j.dnarep.2019.04.007
8. Herrlinger U, Tzaridis T, Mack F, Steinbach JP, Schlegel U, Sabel M, et al. Lomustine-Temozolomide Combination Therapy Versus Standard Temozolomide Therapy in Patients With Newly Diagnosed Glioblastoma With Methylated MGMT Promoter (CeTeG/NOA-09): A Randomised, Open-Label, Phase 3 Trial. Lancet (2019) 393:678–88. doi: 10.1016/S0140-6736(18)31791-4
9. Roos W, Baumgartner M, Kaina B. Apoptosis Triggered by DNA Damage O6-Methylguanine in Human Lymphocytes Requires DNA Replication and Is Mediated by P53 and Fas/CD95/Apo-1. Oncogene (2004) 23:359–67. doi: 10.1038/sj.onc.1207080
10. Corso CD, Bindra RS, Mehta MP. The Role of Radiation in Treating Glioblastoma: Here to Stay. J Neurooncol (2017) 134:479–85. doi: 10.1007/s11060-016-2348-x
11. Bobola MS, Kolstoe DD, Blank A, Silber JR. Minimally Cytotoxic Doses of Temozolomide Produce Radiosensitization in Human Glioblastoma Cells Regardless of MGMT Expression. Mol Cancer Ther (2010) 9:1208–18. doi: 10.1158/1535-7163.MCT-10-0010
12. Chakravarti A, Erkkinen MG, Nestler U, Stupp R, Mehta M, Aldape K, et al. Temozolomide-Mediated Radiation Enhancement in Glioblastoma: A Report on Underlying Mechanisms. Clin Cancer Res (2006) 12:4738–46. doi: 10.1158/1078-0432.CCR-06-0596
13. Kil WJ, Cerna D, Burgan WE, Beam K, Carter D, Steeg PS, et al. In Vitro and In Vivo Radiosensitization Induced by the DNA Methylating Agent Temozolomide. Clin Cancer Res (2008) 14:931–8. doi: 10.1158/1078-0432.CCR-07-1856
14. van Nifterik KA, van den Berg J, Stalpers LJ, Lafleur MV, Leenstra S, Slotman BJ, et al. Differential Radiosensitizing Potential of Temozolomide in MGMT Promoter Methylated Glioblastoma Multiforme Cell Lines. Int J Radiat Oncol Biol Phys (2007) 69:1246–53. doi: 10.1016/j.ijrobp.2007.07.2366
15. van Rijn J, Heimans JJ, van den Berg J, van der Valk P, Slotman BJ. Survival of Human Glioma Cells Treated With Various Combination of Temozolomide and X-Rays. Int J Radiat Oncol Biol Phys (2000) 47:779–84. doi: 10.1016/S0360-3016(99)00539-8
16. Stratenwerth B, Geisen SM, He Y, Beltzig L, Sturla SJ, Kaina B. Molecular Dosimetry of Temozolomide: Quantification of Critical Lesions, Correlation to Cell Death Responses, and Threshold Doses. Mol Cancer Ther (2021) 20:1789–99. doi: 10.1158/1535-7163.MCT-21-0228
17. Aasland D, Gotzinger L, Hauck L, Berte N, Meyer J, Effenberger M, et al. Temozolomide Induces Senescence and Repression of DNA Repair Pathways in Glioblastoma Cells via Activation of ATR-CHK1, P21, and NF-Kappab. Cancer Res (2019) 79:99–113. doi: 10.1158/0008-5472.CAN-18-1733
18. Kaina B. Temozolomide in Glioblastoma Therapy: Role of Apoptosis, Senescence and Autophagy. Comment on Strobel Et Al., Temozolomide and Other Alkylating Agents in Glioblastoma Therapy. Biomedicines (2019) 7:69. doi: 10.3390/biomedicines7040090
19. Tenzer A, Zingg D, Riesterer O, Vuong V, Bodis S, Pruschy M. Signal Transduction Inhibitors as Radiosensitizers. Curr Med Chem Anti-Canc Agents (2002) 2:727–42. doi: 10.2174/1568011023353651
20. Mladenov E, Magin S, Soni A, Iliakis G. DNA Double-Strand Break Repair as Determinant of Cellular Radiosensitivity to Killing and Target in Radiation Therapy. Front Oncol (2013) 3:113. doi: 10.3389/fonc.2013.00113
21. Kaina B, Fritz G, Mitra S, Coquerelle T. Transfection and Expression of Human O6-Methylguanine-DNA Methyltransferase (MGMT) cDNA in Chinese Hamster Cells: The Role of MGMT in Protection Against the Genotoxic Effects of Alkylating Agents. Carcinogenesis (1991) 12:1857–67. doi: 10.1093/carcin/12.10.1857
22. Beranek DT. Distribution of Methyl and Ethyl Adducts Following Alkylation With Monofunctional Alkylating Agents. Mutat Res (1990) 231:11–30. doi: 10.1016/0027-5107(90)90173-2
23. Kaina B, Christmann M, Naumann S, Roos WP. MGMT: Key Node in the Battle Against Genotoxicity, Carcinogenicity and Apoptosis Induced by Alkylating Agents. DNA Repair (Amst) (2007) 6:1079–99. doi: 10.1016/j.dnarep.2007.03.008
24. Knizhnik AV, Roos WP, Nikolova T, Quiros S, Tomaszowski KH, Christmann M, et al. Survival and Death Strategies in Glioma Cells: Autophagy, Senescence and Apoptosis Triggered by a Single Type of Temozolomide-Induced DNA Damage. PloS One (2013) 8:e55665. doi: 10.1371/journal.pone.0055665
25. Christmann M, Nagel G, Horn S, Krahn U, Wiewrodt D, Sommer C, et al. MGMT Activity, Promoter Methylation and Immunohistochemistry of Pretreatment and Recurrent Malignant Gliomas: A Comparative Study on Astrocytoma and Glioblastoma. Int J Cancer (2010) 127:2106–18. doi: 10.1002/ijc.25229
26. Roos WP, Kaina B. DNA Damage-Induced Cell Death: From Specific DNA Lesions to the DNA Damage Response and Apoptosis. Cancer Lett (2013) 332:237–48. doi: 10.1016/j.canlet.2012.01.007
27. Ochs K, Kaina B. Apoptosis Induced by DNA Damage O6-Methylguanine Is Bcl-2 and Caspase-9/3 Regulated and Fas/caspase-8 Independent. Cancer Res (2000) 60:5815–24.
28. Quiros S, Roos WP, Kaina B. Processing of O6-Methylguanine Into DNA Double-Strand Breaks Requires Two Rounds of Replication Whereas Apoptosis Is Also Induced in Subsequent Cell Cycles. Cell Cycle (2010) 9:168–78. doi: 10.4161/cc.9.1.10363
29. Eich M, Roos WP, Nikolova T, Kaina B. Contribution of ATM and ATR to the Resistance of Glioblastoma and Malignant Melanoma Cells to the Methylating Anticancer Drug Temozolomide. Mol Cancer Ther (2013) 12:2529–40. doi: 10.1158/1535-7163.MCT-13-0136
30. He Y, Roos WP, Wu Q, Hofmann TG, Kaina B. The SIAH1-HIPK2-P53ser46 Damage Response Pathway Is Involved in Temozolomide-Induced Glioblastoma Cell Death. Mol Cancer Res (2019) 17:1129–41. doi: 10.1158/1541-7786.MCR-18-1306
31. Roos WP, Batista LFZ, Naumann S, Wick W, Weller M, Menck CFM, et al. Apoptosis in Malignant Glioma Cells Triggered by the Temozolomide-Induced DNA Lesion O6-Methylguanine. Oncogene (2007) 26:186–97. doi: 10.1038/sj.onc.1209785
32. Roos WP, Nikolova T, Quiros S, Naumann SC, Kiedron O, Zdzienicka MZ, et al. Brca2/Xrcc2 Dependent HR, But Not NHEJ, Is Required for Protection Against O(6)-Methylguanine Triggered Apoptosis, DSBs and Chromosomal Aberrations by a Process Leading to SCEs. DNA Repair (Amst) (2009) 8:72–86. doi: 10.1016/j.dnarep.2008.09.003
33. Rothkamm K, Kruger I, Thompson LH, Lobrich M. Pathways of DNA Double-Strand Break Repair During the Mammalian Cell Cycle. Mol Cell Biol (2003) 23:5706–15. doi: 10.1128/MCB.23.16.5706-5715.2003
34. Christmann M, Kaina B. Epigenetic Regulation of DNA Repair Genes and Implications for Tumor Therapy. Mutat Res (2019) 780:15–28. doi: 10.1016/j.mrrev.2017.10.001
35. Aasland D, Reich TR, Tomicic MT, Switzeny OJ, Kaina B, Christmann M. Repair Gene O(6) -Methylguanine-DNA Methyltransferase Is Controlled by SP1 and Up-Regulated by Glucocorticoids, But Not by Temozolomide and Radiation. J Neurochem (2018) 144:139–51. doi: 10.1111/jnc.14262
36. Kuerbitz SJ, Plunkett BS, Walsh WV, Kastan MB. Wild-Type P53 is a Cell Cycle Checkpoint Determinant Following Irradiation. Proc Natl Acad Sci USA (1992) 89:7491–5. doi: 10.1073/pnas.89.16.7491
37. Painter RB. Inhibition of Mammalian Cell DNA Synthesis by Ionizing Radiation. Int J Radiat. Biol (1986) 49:771–81.
38. Coquerelle T, Dosch J, Kaina B. Overexpression of N-Methylpurine-DNA Glycosylase in Chinese Hamster Ovary Cells Renders Them More Sensitive to the Production of Chromosomal Aberrations by Methylating Agents–A Case of Imbalanced DNA Repair. Mutat Res (1995) 336:9–17. doi: 10.1016/0921-8777(94)00035-5
39. Christmann M, Tomicic MT, Roos WP, Kaina B. Mechanisms of Human DNA Repair: An Update. Toxicology (2003) 193:3–34. doi: 10.1016/S0300-483X(03)00287-7
40. He Y, Kaina B. Are There Thresholds in Glioblastoma Cell Death Responses Triggered by Temozolomide? Int J Mol Sci (2019) 20(1562):1–20. doi: 10.3390/ijms20071562
41. Jansen M, Sorg UR, Ragg S, Flasshove M, Seeber S, Williams DA, et al. Hematoprotection and Enrichment of Transduced Cells In Vivo After Gene Transfer of MGMT(P140K) Into Hematopoietic Stem Cells. Cancer Gene Ther (2002) 9:737–46. doi: 10.1038/sj.cgt.7700490
Keywords: glioblastoma, temozolomide, radiotherapy, MGMT, DNA repair, apoptosis, treatment protocols
Citation: Kaina B, Beltzig L and Strik H (2022) Temozolomide – Just a Radiosensitizer? Front. Oncol. 12:912821. doi: 10.3389/fonc.2022.912821
Received: 04 April 2022; Accepted: 05 May 2022;
Published: 16 June 2022.
Edited by:
Enrico Franceschi, Institute of Neurological Sciences of Bologna (ISNB) (IRCCS), ItalyReviewed by:
Franz Rödel, University Hospital Frankfurt, GermanyCopyright © 2022 Kaina, Beltzig and Strik. This is an open-access article distributed under the terms of the Creative Commons Attribution License (CC BY). The use, distribution or reproduction in other forums is permitted, provided the original author(s) and the copyright owner(s) are credited and that the original publication in this journal is cited, in accordance with accepted academic practice. No use, distribution or reproduction is permitted which does not comply with these terms.
*Correspondence: Bernd Kaina, a2FpbmFAdW5pLW1haW56LmRl