- 1Department of Medical Oncology and Hematology, University Hospital Zurich, Zurich, Switzerland
- 2Department of Medical Oncology, Inselspital, University Hospital of Bern, Bern, Switzerland
- 3Department for BioMedical Research, University of Bern, Bern, Switzerland
- 4Department of Pathology and Molecular Pathology, University Hospital Zurich, Zurich, Switzerland
- 5Institute of Pathology, Stadtspital Zurich Triemli, Zurich, Switzerland
- 6Department of Internal Medicine–Oncology, See Spital Horgen, Horgen, Switzerland
Background: Anaplastic lymphoma kinase (ALK) rearrangements are known oncogenic drivers in non-small cell lung cancer (NSCLC). Few case reports described the occurrence of such rearrangements in large cell neuroendocrine carcinomas (LCNECs) of the lung without information on clinical responses to ALK tyrosine kinase inhibitors (TKIs) in these cases. Currently, neuroendocrine tumors of the lungs are not screened for ALK rearrangements.
Methods: To illustrate the clinical impact of molecular characterization in LCNECs, we report the disease course in three patients with ALK-rearranged metastatic LCNEC from our clinical routine, as well as their treatment response to ALK TKIs (index cases). To gain insight into the prevalence of ALK rearrangements in neuroendocrine tumors of the lung, we analyzed a retrospective cohort of 436 tumor biopsies including LCNEC (n = 61), small cell lung cancer (SCLC) (n = 206), typical (n = 91) and atypical (n = 69) carcinoids, and mixed histology (n = 9) for the presence of ALK rearrangements using a sequential diagnostic algorithm. ALK immunohistochemistry (IHC) was evaluable in 362 cases; fluorescence in situ hybridization (FISH) was evaluable in 28 out of the 35 IHC-positive cases, followed by next-generation sequencing (NGS) that was available in 12 cases.
Results: Within the retrospective cohort, ALK IHC was positive in 35 out of 362 (9.7%) evaluable samples. FISH was positive in 3 out of the 28 (10.7%) evaluable cases: 2 with atypical carcinoids and 1 with LCNEC. Additionally, the 3 index cases showed positive ALK IHC, which was confirmed by NGS. Within the retrospective cohort, NGS confirmed the presence of an ALK genomic rearrangement in one FISH-positive atypical carcinoid where material was sufficient for sequencing. Two out of three patients with metastatic ALK-rearranged LCNEC received up-front treatment with the ALK TKI alectinib and showed rapid tumor response at all metastatic sites, including multiple brain metastases.
Conclusions: ALK rearrangements represent rare but targetable oncogenic driver alterations in LCNEC. Contrarily to NSCLC, the detection of ALK rearrangements in neuroendocrine tumors of the lung is challenging, since ALK IHC can lead to false-positive results and therefore needs confirmation by FISH or NGS. Up-front comprehensive molecular profiling with NGS should be performed in metastatic LCNEC in order not to miss actionable genomic alterations.
Introduction
Large cell neuroendocrine carcinomas (LCNECs) constitute a rare subtype of pulmonary malignancies, accounting for approximately 3% of all primary lung cancers (LCs) (1). LCNECs usually course aggressively and exhibit a very poor prognosis in advanced disease stages (2–4). Molecular profiling of LCNEC shows profound genomic heterogeneity, harboring common features to small cell lung cancer (SCLC) and to non-small cell lung cancer (NSCLC). Frequent genomic alterations concern TP53, RB1, STK11, KEAP1, and KRAS (5–7). Genomic and transcriptomic analyses of a cohort of 75 LCNECs suggested the subclassification into two major molecular subtypes: type I LCNEC (37%), characterized by biallelic TP53 and STK11 or KEAP1 alterations and neuroendocrine phenotype, and type II LCNEC (42%), harboring biallelic TP53 and RB1 inactivation. Type II LCNECs, contrary to type I, show reduced levels of neuroendocrine markers and enrichment of immune signaling pathways (8, 9).
While the majority of LCNECs develop de novo, more rarely, histological transformation from a distinct subtype of NSCLC has been reported (10–12). This histological transformation can occur as an acquired resistance mechanism to targeted treatment with epidermal growth factor receptor (EGFR) or anaplastic lymphoma kinase (ALK) tyrosine kinase inhibitors (TKIs) (13–17). However, the most frequent histologic transformation as resistance mechanism to targeted therapies is into SCLC (12).
Due to LCNEC genomic heterogeneity, efforts have been done to identify molecular predictors of therapeutic vulnerability. For instance, the presence of wild-type RB1 has been associated with better survival outcome and better response to gemcitabine or taxane-containing chemotherapy regimens as compared to platin–etoposide combinations. On the other hand, no differences in chemotherapeutic sensitivities were detected for tumors harboring RB1 loss (6). In a single-center retrospective review by Zhou et al. (18) , histopathological and clinical features from 126 patients with central (n = 45) and peripheral (n = 81) LCNEC were analyzed. In 9 out of 81 peripheral tumors (18.8%), EGFR alterations could be detected. One additional tumor (2.1%) harbored a ROS1 fusion. No central LCNEC showed molecular alterations in EGFR or ROS1, and no ALK or BRAF alterations were reported. Patients with central tumors had a significantly worse survival outcome, further illustrating the biological and clinical heterogeneity of LCNEC (18).
ALK rearrangements drive 5%–6% of NSCLCs (19, 20). For LCNEC, very few data regarding the presence of ALK rearrangements are reported in the literature (21). Most larger molecular profiling studies in LCNEC, with 65 or 35 cases, respectively, did not detect any ALK rearrangements using comprehensive molecular profiling, including mutational, fusion, and copy number analysis (7, 22).
Additionally, ALK fusions also have been rarely found in other neuroendocrine tumors of the lung, such as typical (TCs) and atypical pulmonary carcinoids (ACs) (21), as well as in SCLC (23).
In this study, we investigated the prevalence of ALK alterations in a large cohort of neuroendocrine tumors of the lung, including LCNEC, SCLC, TC, and AC (n = 439) by immunohistochemistry (IHC), fluorescence in situ hybridization (FISH), and next-generation sequencing (NGS). Additionally, we report three clinical cases from our institution, which illustrate the clinical impact of up-front comprehensive profiling of pulmonary LCNEC.
Materials and Methods
Patient Cohort
Four tissue microarrays (TMAs) were constructed employing formalin-fixed paraffin-embedded (FFPE) tissue samples comprising SCLC, LCNEC, TC, AC, and mixed histology. The TMA cohort consisted of tumor samples from 436 patients with neuroendocrine lung tumors biopsied or resected between 1991 and 2012. Two cores for each patient have been included. All samples were retrieved from the Department of Pathology and Molecular Pathology, University Hospital Zurich, Switzerland. We further included tumor samples from three additional patients to this study, with identified metastatic ALK-positive LCNEC (index cases). In these cases, the negative smoking history led the clinician to perform molecular characterization as for NSCLC. Our study was approved by the local ethics committee (KEK ZH-Nr. 29-2009) and was conducted in accordance with local laws and regulations.
ALK Immunohistochemistry
ALK IHC on 2-µm-thick TMA sections was performed using a mouse monoclonal antibody for ALK (Clone 5A4; Leica, Wetzlar, Germany) at 1:10 dilution. A cell line with confirmed ALK translocation served as a positive control. ALK expression was evaluated by two experienced pulmonary pathologists (JR, MH) in a blinded manner. In case of a discrepancy in IHC scoring, a consensus score was established. The cytoplasmic staining intensity was semiquantitatively scored as follows: 0 (negative), 1+ (weak), 2+ (moderate), or 3+ (strong). Cases with any positivity (scores 1+, 2+, and 3+) were considered positive (Figure 1).
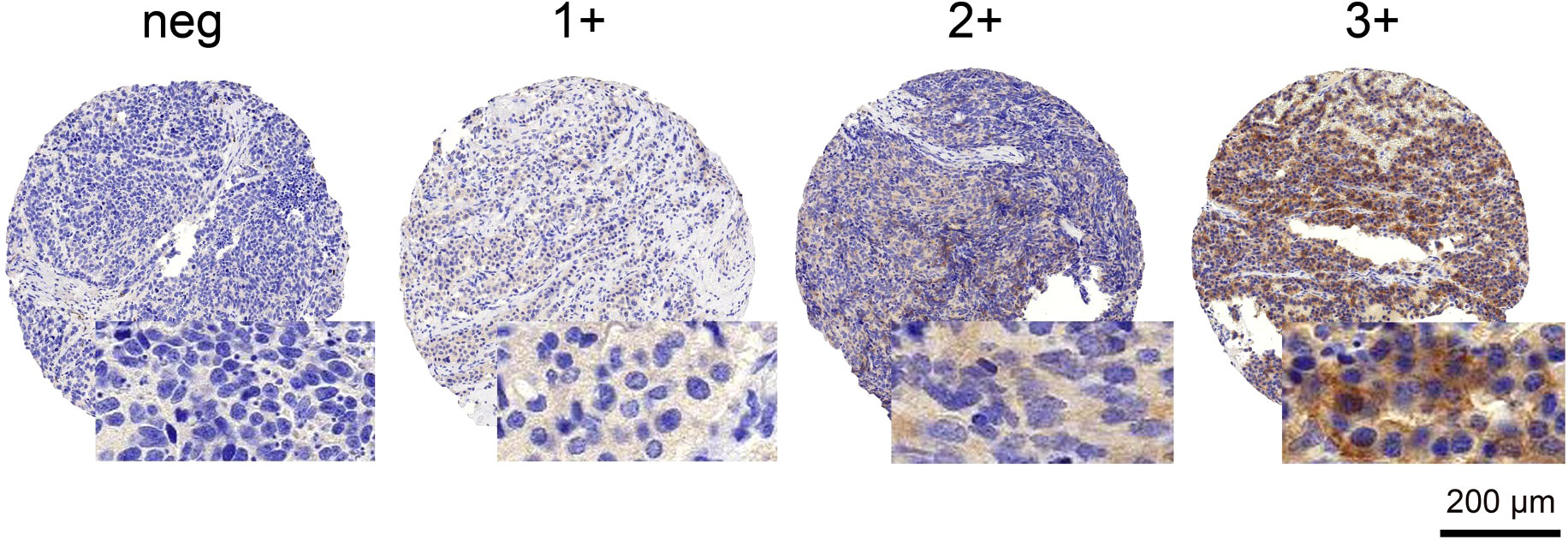
Figure 1 ALK IHC staining patterns. Examples of negative and positive (1+, 2+, and 3+) staining patterns are illustrated. Scale bar 200 µm. neg, negative.
ALK Fluorescence In Situ Hybridization
FISH for the detection of ALK rearrangements was performed using a commercially available ALK assay (Vysis LSI ALK Dual Colour, Break-Apart Rearrangement Probe; Abbott Molecular, IL, USA). FISH analyses were performed on all IHC-positive samples (scores 1+, 2+, and 3+) according to the manufacturer’s recommendations (24). Signals were counted in at least 50 tumor nuclei per case using an epifluorescence microscope (Zeiss, Oberkochen, Germany). FISH for ALK locus rearrangement was considered positive if at least 15% of analyzed cells showed either a split of one set of red and green signals greater than two signal widths apart and/or if loss of one green signal (5′ probe) had occurred as per Abbott Molecular scoring criteria (24–26).
Next-Generation Sequencing
NGS was performed on the 2+ and 3+ IHC-positive cases, where enough tumor material was available. In total, 15 samples were sequenced using Oncomine™ Focus Assay (OFA) Panel (RNA-part), Archer® FusionPlex® Sarcoma kit or FoundationOne®CDx (Foundation Medicine, Cambridge, MA, USA) assay.
For OFA sequencing, RNA was isolated using Maxwell 16 LEV RNA FFPE Purification Kit (Promega). The RNA concentration was measured with Qubit. For the OFA panel, enrichment and chip loading were done on the Ion Chef with the Ion 540 Kit. The S5 platform was used for sequencing with the Ion S5 Sequencing Kit. The protocols from Life Technologies/Thermo Fisher Scientific were followed in all steps. Alignment, variant calling, and annotation were done with the Ion Reporter software 5.10 (Oncomine™ Focus v2.4-Fusions-Single Sample).
Sequencing using the Archer® FusionPlex® Sarcoma panel was performed using the NextSeq550 platform and the NextSeq550 High Output Kit v3 chemistry. The protocols from Archer were followed in all steps. Alignment, variant calling, and annotation were done with the Archer software Version 6.2.1.
Isolated DNA was analyzed by the hybrid capture-based NGS platform (FoundationOne®CDx) at the Department of Pathology and Molecular Pathology, University Hospital Zurich, Switzerland. The methods of F1CDx have been previously described (27). The current F1CDx gene panel (https://www.foundationmedicine.com) analyzes 324 genes, including ALK rearrangements. Additionally, microsatellite instability (MSI) and tumor mutational burden (TMB) are assessed.
Results
Patient Cohort
Clinicopathological characteristics of the analyzed cohort are illustrated in Table 1.
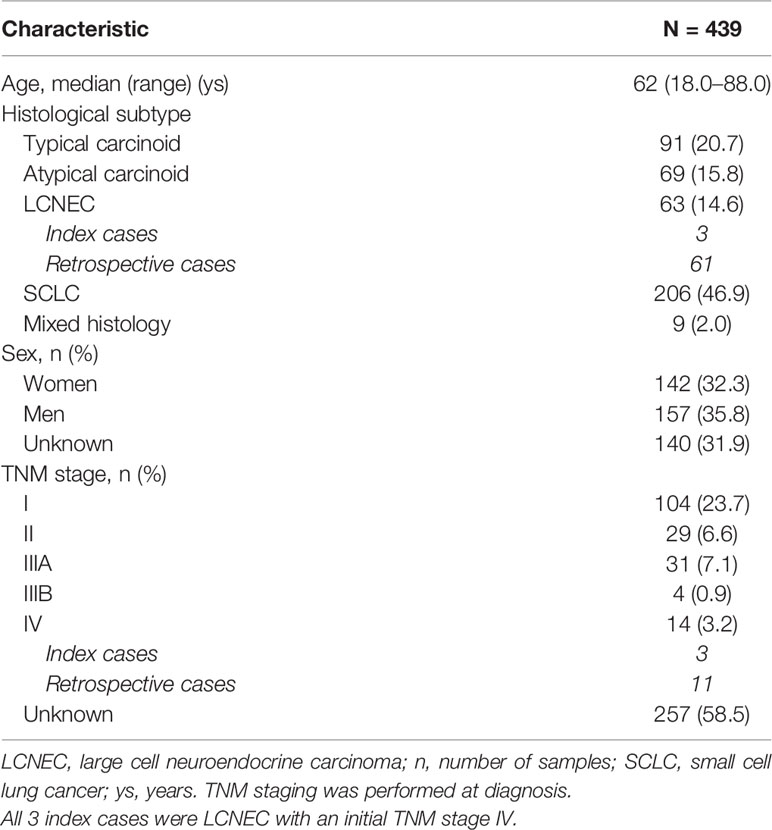
Table 1 Clinicopathological characteristics of the global cohort [retrospective cases (n = 436) and patient index cases (n = 3)].
ALK Immunohistochemistry
Within the retrospective cohort, a positive cytoplasmatic ALK expression (1+, 2+, and 3+) was observed in 35 out of the evaluable 362 (9.7%) cases. These cases comprised 10 TCs, 13 ACs, three LCNECs, and nine SCLCs. No samples with a mixed histology showed a positive IHC staining (Table 2). Not all cores within the TMA were suitable for immunohistochemical analysis, as some cores had missing tumor tissue (~15%). The three index cases had a positive ALK IHC (3+).
ALK Fluorescence In Situ Hybridization
In three out of 28 (10.7%) evaluable cases from the retrospective cohort, an ALK rearrangement detected by FISH was observed. The positive cases showed either a split of one set of red and green signals or loss of one green signal (5′ probe). The positive cases consisted of two AC and one LCNEC (Figure 2 and Table 2).
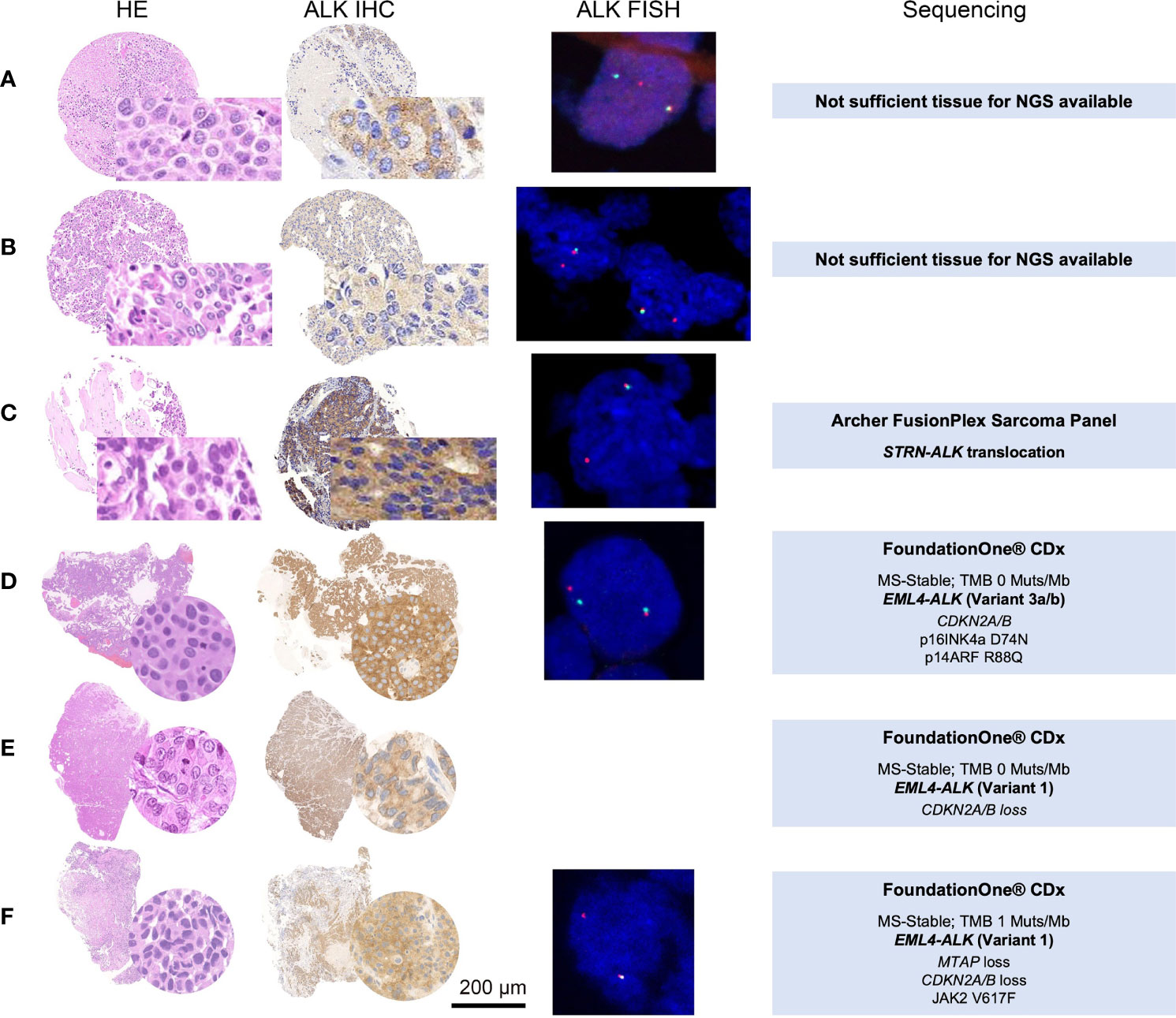
Figure 2 HE, ALK IHC, ALK FISH, and NGS sequencing data of the five ALK FISH-positive cases. Panels (A, D, E, F) were diagnosed as LCNEC, whereas (B, C) were evaluated as (AC). IHC was scored as 2+ (A), 1+ (B), and 3+ (C–F). ALK FISH break-apart probes revealed aberrant staining patterns (A, D) one fused and one split red and green signal; (B, D, E) one fused and one single red signal) consistent with ALK rearrangements. In three cases (C–F), the material was sufficient to perform NGS, confirming two times an EML4-ALK translocation (D) Variant 3a/b; (E, F) Variant 1) and one time an STRN-ALK translocation (C). HE, hematoxylin–eosin; ALK, anaplastic lymphoma kinase; IHC, immunohistochemistry; FISH, fluorescence in situ hybridization; NGS, next-generation sequencing. Scale bar 200 µm.
Next-Generation Sequencing
Within the retrospective cohort, 12 out of the 35 cases with 2+ and 3+ ALK staining by IHC had enough material to perform an NGS. Only 1 AC out of the 12 sequenced cases showed an ALK rearrangement. This case demonstrated a rare STRN-ALK fusion (breakpoint: chr2:37143221, chr2:29446394). Of the three LCNEC index cases, two harbored the typical EML4-ALK rearrangement, variant 1 (breakpoint chr2:42522656,chr2:29446394) and variant 3a/b (breakpoint: chr2:42492091,chr2:29446394). The three LCNEC cases showed low TMB (0, 0, and 1) and were microsatellite stable (Table 2).
Clinical and Histological Characteristics of ALK-Positive Tumors
The three EML4-ALK-rearranged cases corresponded to biopsies from patients with LCNEC stage IV, never smokers, and with brain metastatic disease (Table 2). Disease course data and response to ALK TKIs are exposed under Clinical Case Reports. The fourth positive case by NGS, showing a rare STRN-ALK fusion, was an archive case and did not receive targeted treatment, therefore was not included as a clinical case report.
Clinical Case Reports
Case 1
A 37-year-old male patient was referred to our department with advanced LCNEC, presenting with multiple (more than 50) brain metastases, cT1 cN3 cM1c, The Union for International Cancer Control (UICC) Stage IVB, according to TNM Staging System 8th Edition (2017). The patient had never smoked. Due to symptomatic bleeding of one of the largest brain metastases, primary resection was indicated. Molecular profiling performed on the resected brain metastases showed IHC 3+ positivity for ALK, with no further alterations in EGFR, KRAS, BRAF, or ROS1. Programmed death-ligand 1 (PD-L1) staining (Klon E1L3N) showed expression on tumor cells of <1% (TC0) and expression on immune cells of ≥1% and <5% (IC1).
The patient started first-line treatment with alectinib, showing good clinical and radiographical partial response of the brain metastases already 4 weeks after treatment initiation (Figures 3A, B). Since neurological symptoms rapidly improved after treatment initiation, no whole-brain radiation therapy (WBRT) was required. Under treatment with alectinib, a nearly complete response of the primary tumor, as well as of the lymph node metastasis, was observed. After 10 months on alectinib, disease progression was observed, and treatment was switched to third-generation ALK TKI lorlatinib following current clinical standards, with ongoing tumor response for already 12 months.
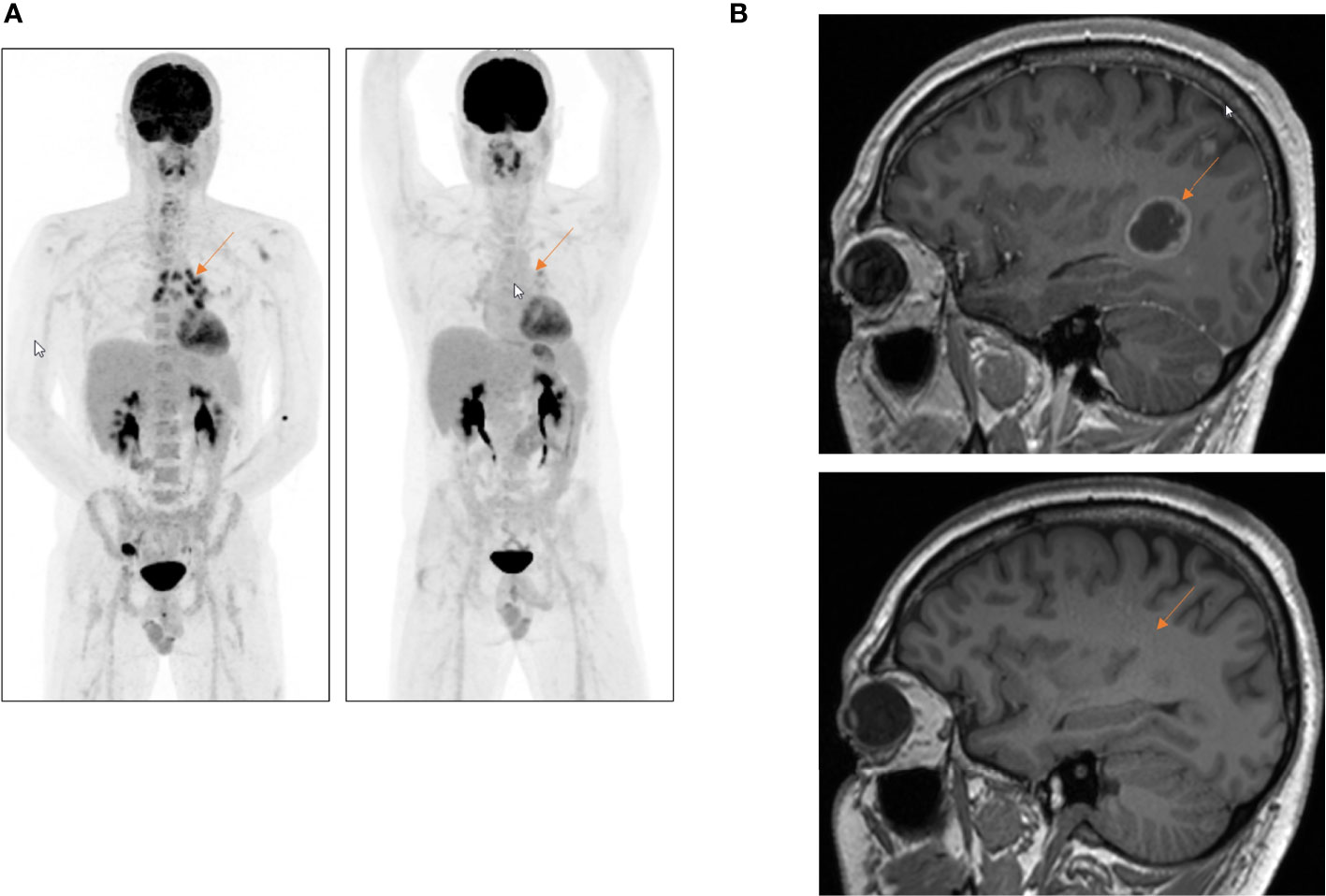
Figure 3 First-line treatment with alectinib in a 37-year-old patient with a metastatic ALK-rearranged LCNEC. (A) FDG-PET scan, whole-body overview, before (left) and 12 weeks after (right) initiation of first-line treatment with alectinib. (B) Complete response of the multiple brain metastases 10 weeks after the start of alectinib. Whole-brain radiation could be avoided. Red arrow shows excellent tumor response under treatment with alectinib.
Case 2
A 32-year-old female never smoker presented with metastatic LCNEC of the lung, with multiple small brain metastases, a single hilar lymph node metastasis, and a symptomatic metastasis to the ovary (cT2 cN1 pM1c, UICC Stage IVB, according to TNM Staging System 8th Edition, 2017). The patient underwent palliative ovariectomy and stereotactic radiosurgery of all brain metastases based on individual decision after discussion with the patient. The patient was asymptomatic regarding brain metastases at that time point. A first cycle of chemotherapy with carboplatin–etoposide was administered, while up-front NGS was pending. NGS unexpectedly showed an EML4-ALK rearrangement. Fluorodeoxyglucose (FDG)-PET/CT scan was performed, showing no metabolic or morphological response (stable disease) 4 weeks after the first chemotherapy application, and first-line treatment was switched to alectinib. The patient showed a rapid excellent tumor response to alectinib, with complete metabolic remission at all metastatic sites 6 weeks after treatment initiation (Figure 4). The patient is currently on treatment with alectinib since 5 months.
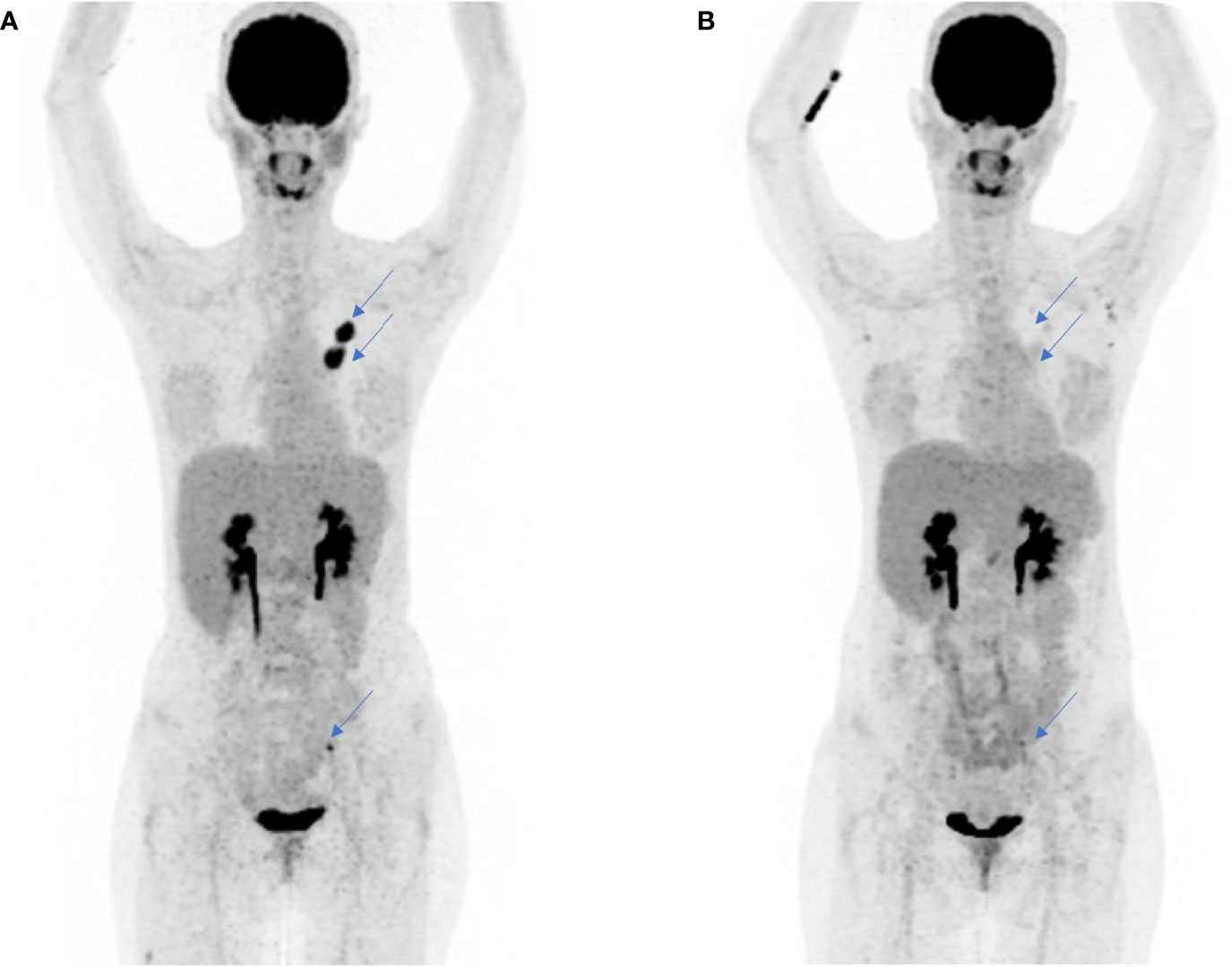
Figure 4 Complete metabolic and subtotal morphologic response to molecularly targeted treatment with alectinib in a 32-year-old patient diagnosed with a metastatic ALK-rearranged LCNEC. (A) FDG-PET scan, whole-body overview, before initiation of first-line treatment with alectinib. No metabolic or morphological response had been observed after one cycle of platin-based chemotherapy administered before obtaining the results of tumor molecular profiling showing ALK rearrangement. (B) FDG-PET scan performed 8 weeks after treatment start with alectinib showing complete metabolic and subtotal morphological response of the primary tumor and the lymph node metastases (blue arrows).
Case 3
A 68-year-old female patient was diagnosed with LCNEC, cT3 cN3 cM1a (pleural), UICC Stage IVA. First-line chemotherapy with carboplatin and etoposide was initiated, and a very good partial response was observed after 3 cycles. After multidisciplinary discussion on thoracic tumor board, since a single pleural metastasis was initially observed and given the good tumor response, radiotherapy to the primary tumor and lymph nodes was indicated, as well as continuation of chemotherapy. The patient completed 6 cycles of chemotherapy and received 66 Gy on the primary tumor. Three months later, the patient presented with diffuse bone pain and clinical deterioration. Radiographical assessment confirmed the presence of multiple bone, hepatic, lymph node, and brain metastases. Because the patient had never smoked, a molecular profiling by NGS was performed, showing a 3+ positive staining for ALK, which was confirmed by NGS. Treatment with alectinib was initiated, but unfortunately, after a few days, the patient presented rapid clinical deterioration, ultimately succumbing to her disease.
Discussion
LCNECs constitute a rare group of pulmonary malignancies clinically characterized by an aggressive disease course and limited benefit from conventional platin-based polychemotherapy regimens (28–30). The molecular landscape of LCNEC is heterogeneous and dominated by yet not actionable genomic alterations such as TP53, RB1, and STK11/KEAP1 (8).
Based on the 3 illustrated ALK-rearranged index cases out of our clinical routine, we aimed to analyze the prevalence of ALK rearrangements in a retrospective cohort of 436 neuroendocrine tumors of the lung, including LCNEC, SCLC, TCs, and ACs. To our knowledge, this is one of the largest published series reviewing the prevalence of ALK rearrangements in neuroendocrine pulmonary tumors. In our series, 35/362 (9.7%) of all screened tumors, evaluable by IHC, showed any positive staining for ALK (score 1+, 2+, or 3+). FISH for ALK rearrangement was positive uniquely in three cases (1 LCNEC and 2 ACs). NGS confirmed the rearrangement in the FISH-positive AC where enough material was available for sequencing. In the global cohort (retrospective and index patients), the four positive LCNEC cases showed IHC scores of 3+, 3+, 3+, and 2+, whereas the AC cases revealed scores of 1+ and 3+. This supports previously published data from NSCLC, where ALK IHC 2+ or 3+ shows the best concordance with the presence of ALK genomic rearrangements (31, 32). Still, the majority of analyzed cases with ALK positivity by IHC showed no genomic rearrangements in ALK when performing FISH or NGS. Relevantly, all SCLCs and TCs with a 2+ or 3+ score in our cohort had no ALK rearrangement by NGS. Previous work has shown that ALK positivity by IHC is an insufficient surrogate of the presence of genomic rearrangements in SCLC and LCNEC. For example, Kondoh et al. (23) evaluated the immunohistochemical expression of ALK in 142 SCLCs, which was positive in 11.3% of the cases and all negative by FISH or real-time PCR. In another study, 27 LCNECs were analyzed, and although four cases were initially considered positive per IHC, only one genomic rearrangement was confirmed (33).
Our study shows that, unlike in NSCLC, IHC is an insufficient tool for the diagnosis of ALK rearrangement in neuroendocrine tumors of the lung due to the high prevalence of false-positive cases and low specificity. Also in other tumor entities, false-positive ALK expression has been reported, e.g., in neuroblastoma mediated by MYCN amplification (34). For NSCLC, on the contrary, ALK positivity by IHC is usually considered diagnostic and leads to treatment indication with ALK TKIs, despite that also some false-positive and discordant cases with FISH and NGS have been reported (35). These false-positive cases have been related to the presence of ALK amplifications or activating mutations (35, 36).
In our cohort, all three ALK-rearranged LCNECs harbored the classical EML4-ALK fusion and are further characterized by low TMB and microsatellite stability (Figure 2). The ALK-rearranged AC showed an STRN-ALK rearrangement. This is a rare fusion and has only been described in a few case reports across different tumor entities (37, 38). In NSCLC, two case reports of late-stage adenocarcinomas harboring this rearrangement exhibited an excellent response to treatment with alectinib and crizotinib, respectively (39, 40). The patient with STRN-ALK-rearranged AC from our cohort presented with an initial stage pT2 pN2 cM0 but developed bone and brain metastases, receiving a platin-based chemotherapy and no targeted treatment. One limitation of our study is the fact that for all IHC-positive cases, not enough tumor material was available for further confirmation of ALK rearrangement by either FISH or NGS.
We identified ALK rearrangements in our 3 index cases and one additional case out of the 61 (1.6%) retrospectively reviewed LCNEC and in 2 out of 69 (2.9%) analyzed AC cases. Clinically, the three reported index patients with ALK-rearranged LCNEC presented with an aggressive course of metastatic disease and multiple brain metastases. The two patients who underwent up-front molecular profiling with consequent early detection of ALK rearrangement showed a profound and rapid response to ALK TKIs. Additionally, due to up-front detection of ALK rearrangement, WBRT could be avoided in a 37-year-old patient (Case 1), who is still responding to second-line targeted treatment with lorlatinib. The illustrated index cases underline the clinical relevance of up-front screening for actionable genomic alterations in patients with advanced LCNEC. Kim et al. (41) performed comprehensive molecular characterization (NGS, whole-transcriptome sequencing, and IHC) of a cohort of 467 LCNECs, and ALK fusions were identified in 1.7% of the cases. In a case series, published by Zheng et al. (21) , the authors identified 4 cases of pulmonary neuroendocrine tumors (2 LCNECs, 1 AC, and 1 high-grade neuroendocrine carcinoma). One LCNEC was uniquely identified by ALK-positive IHC. An LCNEC and AC showed an EML4-ALK fusion, and the high-grade neuroendocrine carcinoma, a KLC1-ALK fusion. Interestingly, 2 out of these 4 patients, one LCNEC and one atypical metastatic carcinoid, developed brain metastases. Both tumors presenting an EML4-ALK fusion responded to treatment with the ALK TKI crizotinib (21). No treatment response data were available for the case harboring the KLC1-ALK fusion. Further case reports have shown efficacy of ALK TKIs in LCNEC with confirmed ALK rearrangement (42). Importantly, all 3 index ALK-rearranged cases were metastatic at diagnosis. On the contrary, most of the patients in the retrospective cohort had a localized disease stage at diagnosis and time point of the biopsy or tumor resection. Due to the limitation of the sample size, our study cannot answer the question whether ALK rearrangement occurs more frequently in metastatic LCNEC.
The updated molecular testing guidelines for the selection of lung cancer patients for treatment with targeted TKIs, reviewed in 2018 by The College of American Pathologists (CAP) and the International Association for the Study of Lung Cancer (IASLC), do not recommend the systematic molecular profiling of neuroendocrine tumors of the lung (43). Despite that targetable alterations are rare in LCNEC, our work shows the high clinical relevance of screening for ALK alterations in these tumors.
In summary, we show that ALK rearrangements are rare but highly actionable targets in LCNEC and that up-front molecular profiling can dramatically impact the disease course of patients with metastatic ALK-driven LCNEC.
Conclusions
ALK rearrangements may oncogenically drive LCNEC, a rare and clinically aggressive subtype of lung cancer, sensitizing this tumor to treatment with ALK inhibitors. Given the genomic heterogeneity of LCNEC, up-front comprehensive molecular profiling should be offered to patients with advanced LCNEC. Moreover, our study shows that ALK IHC alone is an insufficient diagnostic method to assess the ALK status in neuroendocrine tumors of the lung, requiring integration of FISH and NGS into the diagnostic algorithm.
Data Availability Statement
Data supporting the findings of this study are available within the article and its supplementary information files or from the corresponding author upon request. In order to minimize the risk of re-identification, individual-level data are not publicly available. However, requests from accredited researchers for access to de-identified individual-level or aggregate data relevant to this manuscript, can be made available upon request by contacting the corresponding author. Accredited researchers should provide contact information, affiliation/organization, and research rationale.
Ethics Statement
The studies involving human participants were reviewed and approved by Kantonale Ethikkomission, Kanton Zürich, Switzerland (KEK ZH-Nr. 29-2009). The patients/participants provided their written informed consent to participate in this study.
Author Contributions
DA contributed to data collection, data analysis, and article writing. MH contributed to data collection, data analysis, and article editing. RF contributed to data collection and data analysis. SH contributed to data analysis and article editing. MR contributed to data collection, data analysis, and article editing. JR contributed to data collection, data analysis, and article editing. AC-F contributed to study conception and coordination and article editing. All authors contributed to the article and approved the submitted version.
Conflict of Interest
SH reports consulting and advisory fees from Bayer outside the submitted work. RF reports grants and consulting and advisory fees from Pierre Fabre, Bayer, Merck, MSD, Astra Zeneca, Novartis, and BMS outside the submitted work. MK reports ownership relationship with Novavax, Biontech, and Mol. Partners outside the submitted work. AC-F reports patent relationship with Astra Zeneca, Bristol Meyer Squibb, Boehringer Ingelheim, Pfizer, Roche, Takeda, MSD, and Janssen-Cilag outside the submitted work.
The remaining authors declare that the research was conducted in the absence of any commercial or financial relationships that could be construed as a potential conflict of interest.
Publisher’s Note
All claims expressed in this article are solely those of the authors and do not necessarily represent those of their affiliated organizations, or those of the publisher, the editors and the reviewers. Any product that may be evaluated in this article, or claim that may be made by its manufacturer, is not guaranteed or endorsed by the publisher.
Supplementary Material
The Supplementary Material for this article can be found online at: https://www.frontiersin.org/articles/10.3389/fonc.2022.911294/full#supplementary-material
Supplementary Figure 1 | Hematoxylin-eosin (H-E), synaptophysin (SYN) and Ki-67 IHC stainings of neuroendocrine tumors of the lung. (A) H-E, SYN and Ki-67 staining of selected TMA cases comprising typical carcinoid, atypical carcinoid, SCLC and LCNEC. (B) H-E, SYN and Ki-67 staining of three ALK rearranged LCNEC cases. AC, atypical carcinoid; LCNEC, large cell neuroendocrine carcinoma; SCLC, small cell lung cancer; TC, typical carcinoid; TMA, tissue microarray. Scale bars 200 µm and 2 mm.
Supplementary Figure 2 | Schematic diagram illustrating workflow for ALK diagnostics per IHC, FISH and NGS in the retrospective cohort. IHC was assessed first on all evaluable samples (n=365/439). FISH was scored on 28 samples positive by ALK IHC. NGS was performed on 12 samples positive per IHC and with enough available material. ALK, anaplastic lymphoma kinase; IHC, immunohistochemistry, FISH, fluorescence in situ hybridization; NGS, next-generation sequencing.
Supplementary Figure 3 | Schematic diagram illustrating workflow for ALK diagnostics per IHC, FISH and NGS in 3 index cases. IHC was assessed and was evaluated as positive (3+) on all 3 samples (n=3/3). FISH was scored and resulted positive on 2/2 evaluable samples. NGS confirmed ALK rearrangement in all 3 cases. ALK, anaplastic lymphoma kinase; IHC, immunohistochemistry; FISH, fluorescence in situ hybridization; NGS, next-generation sequencing
References
1. Fasano M, Della Corte CM, Papaccio F, Ciardiello F, Morgillo F. Pulmonary Large-Cell Neuroendocrine Carcinoma: From Epidemiology to Therapy. J Thorac Oncol (2015) 10(8):1133–41. doi: 10.1097/JTO.0000000000000589
2. Takei H, Asamura H, Maeshima A, Suzuki K, Kondo H, Niki T, et al. Large Cell Neuroendocrine Carcinoma of the Lung: A Clinicopathologic Study of Eighty-Seven Cases. J Thorac Cardiovasc surg (2002) 124(2):285–92. doi: 10.1067/mtc.2002.122523
3. Battafarano RJ, Fernandez FG, Ritter J, Meyers BF, Guthrie TJ, Cooper JD, et al. Large Cell Neuroendocrine Carcinoma: An Aggressive Form of non-Small Cell Lung Cancer. J Thorac Cardiovasc surg (2005) 130(1):166–72. doi: 10.1016/j.jtcvs.2005.02.064
4. Veronesi G, Morandi U, Alloisio M, Terzi A, Cardillo G, Filosso P, et al. Large Cell Neuroendocrine Carcinoma of the Lung: A Retrospective Analysis of 144 Surgical Cases. Lung Cancer (2006) 53(1):111–5. doi: 10.1016/j.lungcan.2006.03.007
5. Rekhtman N, Pietanza MC, Hellmann MD, Naidoo J, Arora A, Won H, et al. Next-Generation Sequencing of Pulmonary Large Cell Neuroendocrine Carcinoma Reveals Small Cell Carcinoma-Like and Non-Small Cell Carcinoma-Like Subsets. Clin Cancer Res (2016) 22(14):3618–29. doi: 10.1158/1078-0432.CCR-15-2946
6. Derks JL, Leblay N, Thunnissen E, van Suylen RJ, den Bakker M, Groen HJM, et al. Molecular Subtypes of Pulmonary Large-Cell Neuroendocrine Carcinoma Predict Chemotherapy Treatment Outcome. Clin Cancer Res (2018) 24(1):33–42. doi: 10.1158/1078-0432.CCR-17-1921
7. Miyoshi T, Umemura S, Matsumura Y, Mimaki S, Tada S, Makinoshima H, et al. Genomic Profiling of Large-Cell Neuroendocrine Carcinoma of the Lung. Clin Cancer Res (2017) 23(3):757–65. doi: 10.1158/1078-0432.CCR-16-0355
8. George J, Walter V, Peifer M, Alexandrov LB, Seidel D, Leenders F, et al. Integrative Genomic Profiling of Large-Cell Neuroendocrine Carcinomas Reveals Distinct Subtypes of High-Grade Neuroendocrine Lung Tumors. Nat Commun (2018) 9(1):1048–. doi: 10.1038/s41467-018-03099-x
9. Lantuejoul S, Fernandez-Cuesta L, Damiola F, Girard N, McLeer A. New Molecular Classification of Large Cell Neuroendocrine Carcinoma and Small Cell Lung Carcinoma With Potential Therapeutic Impacts. Transl Lung Cancer Res (2020) 9(5):2233–44. doi: 10.21037/tlcr-20-269
10. Moriya R, Hokari S, Shibata S, Koizumi T, Tetsuka T, Ito K, et al. Histological Transformation to Large Cell Neuroendocrine Carcinoma From Lung Adenocarcinoma Harboring an EGFR Mutation: An Autopsy Case Report. Intern Med (2017) 56(15):2013–7. doi: 10.2169/internalmedicine.56.7452
11. Belluomini L, Caliò A, Giovannetti R, Motton M, Mazzarotto R, Micheletto C, et al. Molecular Predictors of EGFR-Mutant NSCLC Transformation Into LCNEC After Frontline Osimertinib: Digging Under the Surface. ESMO Open (2021) 6(1):1–2. doi: 10.1016/j.esmoop.2020.100028
12. Rekhtman N. Lung Neuroendocrine Neoplasms: Recent Progress and Persistent Challenges. Modern Pathol (2022) 35(1):36–50. doi: 10.1038/s41379-021-00943-2
13. Lim JU, Woo IS, Jung YH, Byeon JH, Park CK, Kim TJ, et al. Transformation Into Large-Cell Neuroendocrine Carcinoma Associated With Acquired Resistance to Erlotinib in Nonsmall Cell Lung Cancer. Korean J Internal Med (2014) 29(6):830–3. doi: 10.3904/kjim.2014.29.6.830
14. Baglivo S, Ludovini V, Sidoni A, Metro G, Ricciuti B, Siggillino A, et al. Large Cell Neuroendocrine Carcinoma Transformation and EGFR-T790M Mutation as Coexisting Mechanisms of Acquired Resistance to EGFR-TKIs in Lung Cancer. Mayo Clinic Proc (2017) 92(8):1304–11. doi: 10.1016/j.mayocp.2017.03.022
15. Fares AF, Lok BH, Zhang T, Cabanero M, Lau SCM, Stockley T, et al. ALK-Rearranged Lung Adenocarcinoma Transformation Into High-Grade Large Cell Neuroendocrine Carcinoma: Clinical and Molecular Description of Two Cases. Lung Cancer (2020) 146:350–4. doi: 10.1016/j.lungcan.2020.06.005
16. Lee M, Patel D, Jofre S, Fidvi S, Suhrland M, Cohen P, et al. Large Cell Neuroendocrine Carcinoma Transformation as a Mechanism of Acquired Resistance to Osimertinib in Non-Small Cell Lung Cancer: Case Report and Literature Review. Clin Lung Cancer (2021) 23(3):E276–82. doi: 10.1016/j.cllc.2021.08.002
17. Miyazaki S, Kuno Y, Hayai S, Teramachi R, Yamashita R, Saito Y, et al. An EGFR T790M-Mutated Lung Adenocarcinoma Undergoing Large-Cell Neuroendocrine Carcinoma Transformation After Osimertinib Therapy: A Case Report. J Med Case Rep (2020) 14(1):122. doi: 10.1186/s13256-020-02447-0
18. Zhou F, Hou L, Ding T, Song Q, Chen X, Su C, et al. Distinct Clinicopathologic Features, Genomic Characteristics and Survival of Central and Peripheral Pulmonary Large Cell Neuroendocrine Carcinoma: From Different Origin Cells? Lung Cancer (2018) 116:30–7. doi: 10.1016/j.lungcan.2017.12.009
19. Devarakonda S, Morgensztern D, Govindan R. Genomic Alterations in Lung Adenocarcinoma. Lancet Oncol (2015) 16(7):e342–51. doi: 10.1016/S1470-2045(15)00077-7
20. Du X, Shao Y, Qin H-F, Tai Y-H, Gao H-J. ALK-Rearrangement in non-Small-Cell Lung Cancer (NSCLC). Thorac Cancer (2018) 9(4):423–30. doi: 10.1111/1759-7714.12613
21. Zheng Q, Zheng M, Jin Y, Shen X, Shan L, Shen L, et al. ALK-Rearrangement Neuroendocrine Carcinoma of the Lung: A Comprehensive Study of a Rare Case Series and Review of Literature. Onco Targets Ther (2018) 11:4991–8. doi: 10.2147/OTT.S172124
22. Karlsson A, Brunnström H, Lindquist KE, Jirström K, Jönsson M, Rosengren F, et al. Mutational and Gene Fusion Analyses of Primary Large Cell and Large Cell Neuroendocrine Lung Cancer. Oncotarget (2015) 6(26):22028–37. doi: 10.18632/oncotarget.4314
23. Kondoh C, Horio Y, Hayashi Y, Ebi H, Hida T, Hasegawa Y, et al. Anaplastic Lymphoma Kinase Expression in Small-Cell Lung Cancer. Histopathology (2019) 75(1):20–8. doi: 10.1111/his.13842
24. Camidge DR, Kono SA, Flacco A, Tan AC, Doebele RC, Zhou Q, et al. Optimizing the Detection of Lung Cancer Patients Harboring Anaplastic Lymphoma Kinase (ALK) Gene Rearrangements Potentially Suitable for ALK Inhibitor Treatment. Clin Cancer Res (2010) 16(22):5581–90. doi: 10.1158/1078-0432.CCR-10-0851
25. Mino-Kenudson M, Chirieac LR, Law K, Hornick JL, Lindeman N, Mark EJ, et al. A Novel, Highly Sensitive Antibody Allows for the Routine Detection of ALK-Rearranged Lung Adenocarcinomas by Standard Immunohistochemistry. Clin Cancer Res (2010) 16(5):1561–71. doi: 10.1158/1078-0432.CCR-09-2845
26. Marchetti A, Ardizzoni A, Papotti M, Crinò L, Rossi G, Gridelli C, et al. Recommendations for the Analysis of ALK Gene Rearrangements in non-Small-Cell Lung Cancer: A Consensus of the Italian Association of Medical Oncology and the Italian Society of Pathology and Cytopathology. J Thorac Oncol (2013) 8(3):352–8. doi: 10.1097/JTO.0b013e31827d5280
27. Frampton GM, Fichtenholtz A, Otto GA, Wang K, Downing SR, He J, et al. Development and Validation of a Clinical Cancer Genomic Profiling Test Based on Massively Parallel DNA Sequencing. Nat Biotechnol (2013) 31(11):1023–31. doi: 10.1038/nbt.2696
28. Le Treut J, Sault MC, Lena H, Souquet PJ, Vergnenegre A, Le Caer H, et al. Multicentre Phase II Study of Cisplatin-Etoposide Chemotherapy for Advanced Large-Cell Neuroendocrine Lung Carcinoma: The GFPC 0302 Study. Ann Oncol (2013) 24(6):1548–52. doi: 10.1093/annonc/mdt009
29. Niho S, Kenmotsu H, Sekine I, Ishii G, Ishikawa Y, Noguchi M, et al. Combination Chemotherapy With Irinotecan and Cisplatin for Large-Cell Neuroendocrine Carcinoma of the Lung: A Multicenter Phase II Study. J Thorac Oncol (2013) 8(7):980–4. doi: 10.1097/JTO.0b013e31828f6989
30. Shah MH, Goldner WS, Halfdanarson TR, Bergsland E, Berlin JD, Halperin D, et al. NCCN Guidelines Insights: Neuroendocrine and Adrenal Tumors, Version 22018. J Natl Compr Cancer Net JNCCN (2018) 16(6):693–702. doi: 10.6004/jnccn.2018.0056
31. Zhou J, Zhao J, Sun K, Wang B, Wang L, Chen X, et al. Accurate and Economical Detection of ALK Positive Lung Adenocarcinoma With Semiquantitative Immunohistochemical Screening. PloS One (2014) 9(3):e92828. doi: 10.1371/journal.pone.0092828
32. Yi ES, Boland JM, Maleszewski JJ, Roden AC, Oliveira AM, Aubry MC, et al. Correlation of IHC and FISH for ALK Gene Rearrangement in non-Small Cell Lung Carcinoma: IHC Score Algorithm for FISH. J Thorac Oncol (2011) 6(3):459–65. doi: 10.1097/JTO.0b013e318209edb9
33. Manrique TG, Benítez AMV, Zarco ER, Escudero AG, Baz DV. P3.02-047 Testing EGFR and ALK in Large Cell Neuroendocrine Carcinoma of the Lung. Looking for Biological Features in Rare Tumors. J Thorac Oncol (2017) 12(11, Supplement 2):S2254. doi: 10.1016/j.jtho.2017.09.1576
34. Hasan MK, Nafady A, Takatori A, Kishida S, Ohira M, Suenaga Y, et al. ALK is a MYCN Target Gene and Regulates Cell Migration and Invasion in Neuroblastoma. Sci Rep (2013) 3:3450. doi: 10.1038/srep03450
35. Ilie MI, Bence C, Hofman V, Long-Mira E, Butori C, Bouhlel L, et al. Discrepancies Between FISH and Immunohistochemistry for Assessment of the ALK Status are Associated With ALK ‘Borderline’&-Positive Rearrangements or a High Copy Number: A Potential Major Issue for Anti-ALK Therapeutic Strategies. Ann Oncol (2015) 26(1):238–44. doi: 10.1093/annonc/mdu484
36. Togashi Y, Mizuuchi H, Kobayashi Y, Hayashi H, Terashima M, Sakai K, et al. An Activating ALK Gene Mutation in ALK IHC-Positive/FISH-Negative Nonsmall-Cell Lung Cancer. Ann Oncol (2015) 26(8):1800–1. doi: 10.1093/annonc/mdv240
37. Singhi AD, Ali SM, Lacy J, Hendifar A, Nguyen K, Koo J, et al. Identification of Targetable ALK Rearrangements in Pancreatic Ductal Adenocarcinoma. J Natl Compr Canc Netw (2017) 15(5):555–62. doi: 10.6004/jnccn.2017.0058
38. Lai AZ, Schrock AB, Erlich RL, Ross JS, Miller VA, Yakirevich E, et al. Detection of an ALK Fusion in Colorectal Carcinoma by Hybrid Capture-Based Assay of Circulating Tumor DNA. Oncologist (2017) 22(7):774–9. doi: 10.1634/theoncologist.2016-0376
39. Su C, Jiang Y, Jiang W, Wang H, Liu S, Shao Y, et al. STRN-ALK Fusion in Lung Adenocarcinoma With Excellent Response Upon Alectinib Treatment: A Case Report and Literature Review. Onco Targets Ther (2020) 13:12515–9. doi: 10.2147/OTT.S282933
40. Yang Y, Qin SK, Zhu J, Wang R, Li YM, Xie ZY, et al. A Rare STRN-ALK Fusion in Lung Adenocarcinoma Identified Using Next-Generation Sequencing-Based Circulating Tumor DNA Profiling Exhibits Excellent Response to Crizotinib. Mayo Clin Proc Innov Qual Outcomes (2017) 1(1):111–6. doi: 10.1016/j.mayocpiqo.2017.04.003
41. Kim C, McGrath JE, Xiu J, Nagasaka M, Ma PC, Nieva JJ, et al. Genomic and Immunologic Characterization of Large-Cell Neuroendocrine Carcinoma of the Lung. J Clin Oncol (2021) 39(15_suppl):8535. doi: 10.1200/JCO.2021.39.15_suppl.8535
42. Hayashi N, Fujita A, Saikai T, Takabatake H, Sotoshiro M, Sekine K, et al. Large Cell Neuroendocrine Carcinoma Harboring an Anaplastic Lymphoma Kinase (ALK) Rearrangement With Response to Alectinib. Internal Med (Tokyo Japan) (2018) 57(5):713–6. doi: 10.2169/internalmedicine.9368-17
43. Lindeman NI, Cagle PT, Aisner DL, Arcila ME, Beasley MB, Bernicker EH, et al. Updated Molecular Testing Guideline for the Selection of Lung Cancer Patients for Treatment With Targeted Tyrosine Kinase Inhibitors: Guideline From the College of American Pathologists, the International Association for the Study of Lung Cancer, and the Association for Molecular Pathology. Arch Pathol Lab Med (2018) 142(3):321–46. doi: 10.5858/arpa.2017-0388-CP
Keywords: ALK rearrangements, neuroendocrine tumors of the lung, large cell neuroendocrine carcinoma, ALK inhibitors, targeted treatment
Citation: Akhoundova D, Haberecker M, Fritsch R, Höller S, Kiessling MK, Rechsteiner M, Rüschoff JH and Curioni-Fontecedro A (2022) Targeting ALK in Neuroendocrine Tumors of the Lung. Front. Oncol. 12:911294. doi: 10.3389/fonc.2022.911294
Received: 02 April 2022; Accepted: 02 May 2022;
Published: 07 June 2022.
Edited by:
Petros Christopoulos, Heidelberg University Hospital, GermanyReviewed by:
Fabrizio Tabbò, University of Turin, ItalyGiulio Metro, Hospital of Santa Maria della Misericordia in Perugia, Italy
Copyright © 2022 Akhoundova, Haberecker, Fritsch, Höller, Kiessling, Rechsteiner, Rüschoff and Curioni-Fontecedro. This is an open-access article distributed under the terms of the Creative Commons Attribution License (CC BY). The use, distribution or reproduction in other forums is permitted, provided the original author(s) and the copyright owner(s) are credited and that the original publication in this journal is cited, in accordance with accepted academic practice. No use, distribution or reproduction is permitted which does not comply with these terms.
*Correspondence: Alessandra Curioni-Fontecedro, alessandra.curioni@usz.ch
†These authors have contributed equally to this work
‡These authors have contributed equally to this work