- 1Department of Clinical Oncology, Li Ka Shing Faculty of Medicine, The University of Hong Kong, Hong Kong, Hong Kong SAR, China
- 2Department of Clinical Oncology, The University of Hong Kong-Shenzhen Hospital, Shenzhen, China
- 3State Key Laboratory of Oncology in Southern China, Sun Yat-sen University Cancer Center, Guangzhou, China
Esophageal squamous cell carcinoma (ESCC) is one of the most common cancers in the world, with a high rate of morbidity. The invasion and metastasis of ESCC is the main reason for high mortality. More and more evidence suggests that metastasized cancer cells require cellular elements that contribute to ESCC tumor microenvironment (TME) formation. TME contains many immune cells and stromal components, which are critical to epithelial–mesenchymal transition, immune escape, angiogenesis/lymphangiogenesis, metastasis niche formation, and invasion/metastasis. In this review, we will focus on the mechanism of different microenvironment cellular elements in ESCC invasion and metastasis and discuss recent therapeutic attempts to restore the tumor-suppressing function of cells within the TME. It will represent the whole picture of TME in the metastasis and invasion process of ESCC.
1 Introduction
Esophageal squamous cell carcinoma (ESCC) is one of the most common cancers in the world, with high rates of morbidity and mortality (1). More than half of the ESCC patients are in advanced stages when they are first diagnosed. Extensive metastases prevent patients from having radical surgery, which is the only clinical method of curing ESCC currently (2). The Food and Drug Administration (FDA) has approved a number of new immune and targeted drugs, such as programmed cell death protein 1 (PD-1) inhibitors and human epidermal growth factor receptor-2 (Her-2) inhibitors for advanced ESCC treatment, but the survival rate of those advanced patients is still low (3, 4). It is reported that the 5-year survival rate for advanced esophageal cancer (19%) was on par with lung cancer (19%) and next only to liver cancer (18%) and pancreatic cancer (9%) (5, 6). Local invasion and distant metastasis of ESCC are the main reasons for the failure of treating these advanced patients. Therefore, further molecular research of the ESCC landscape has the potential to ascertain new biomarkers and molecular targets that affect ESCC progression and enable the design of new therapeutic strategies (7).
Recently, the central role of the tumor microenvironment (TME) in the invasion and metastasis of de novo ESCC has been identified. TME includes immune cells, fibroblasts, endothelial cells, perivascular cells, neurons, and extracellular matrix. There is increasing evidence that TME plays an important role in cell proliferation, cell survival, epithelial–mesenchymal transition (EMT), angiogenesis/lymphangiogenesis immunosuppression, invasion, and metastasis (8, 9). TME is a dynamic environment constantly reshaped by tumor and tumor-associated cells to make tumor cells survive well (10). Thus, TME is now regarded as a target-rich environment for the development of novel anticancer drugs in ESCC. Actually, many drugs that focus on diverse components of TME, including vascular endothelial growth factor (VEGF) and immune checkpoints, have been approved for clinical use (11, 12).
In this review, we summarize recent advances in how ESCC cells recruit and modify cells in the immune microenvironment to make them more conducive to metastasize and how those factors in the TME support the ESCC invasion and metastasis. Also, we discuss the regulation of abnormal molecular signaling pathways and networks stimulated by tumor and TME interactions, which might provide new diagnostic, prognostic, or therapeutic opportunities.
2 Invasion and Metastasis Process of Esophageal Squamous Cell Carcinoma
Metastasis is the process by which circulating tumor cells colonize in other tissues or organs and become diffuse tumor cells. However, only 0.01% of circulating tumor cells have been reported to successfully colonize and grow into diffuse tumor cells (13, 14). It is because the circulating tumor cells are seriously influenced by the human local microenvironment. The “seed and soil” hypothesis raised by Paget can be used to well characterize this process (15). Tumor cells in situ (“seeds”) tend to stay on some specific target organs (“soil”), which have TME beneficial to the survival of tumor cells. At present, it is supposed that there are three main steps for the formation of a metastasis niche: first, the primary tumors secrete some factors around them (invasion), exosomes, and micro-vesicles (metastasis) to create the pre-metastatic niche (16, 17). Then, those factors induce immune cells, such as marrow-derived suppressor cells (MDSCs), macrophages, dendritic cells (DCs), neutrophils (18, 19), and regulatory T cells (Tregs) to polarize into tumor-promoting cells. Also, some stromal components such as cancer-associated fibroblasts (CAFs) promoting angiogenesis, secreting cytokines, inducing EMT, recombining matrix components, recruiting inflammatory cells to help ESCC cells invade and metastasize (20, 21), and other factors (hypoxia, etc.) (17). Finally, all those factors remodel the microenvironment into TME, and invasion and metastasis occur (Figure 1).
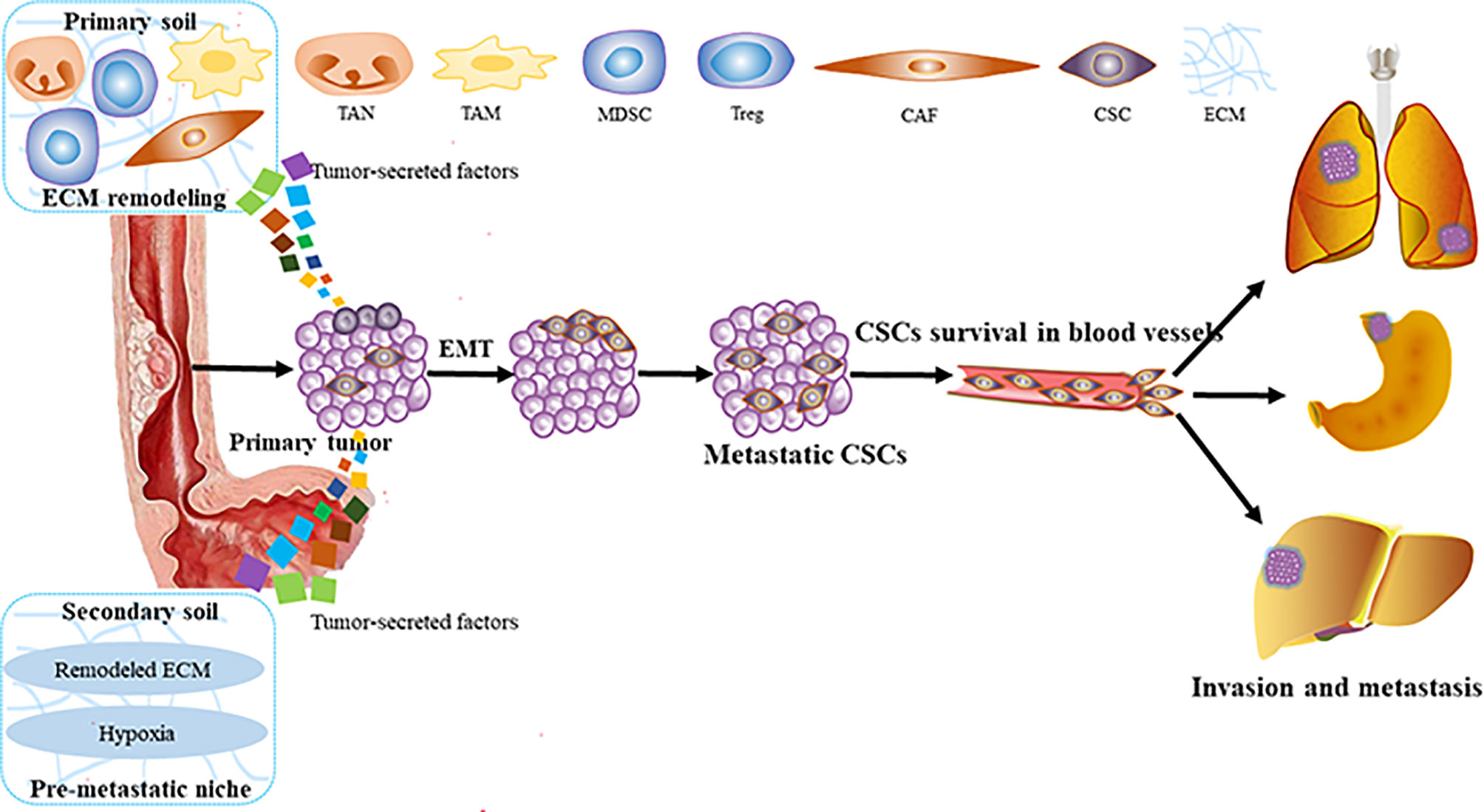
Figure 1 The process of ESCC invasion and metastasis. ESCC, esophageal squamous carcinoma; MDSC, marrow-derived suppressor cell; Tregs, regulatory T cells; CAFS, cancer associated fibroblasts; EMT, epithelial—mesenchymal transition; TAM, tumor-associated macrophage; TAN, tumor-related neutrophil; ECM, extracellular matrix; CSCs, cancer stem cells.
Lymphatic metastasis is the most common way of ESCC metastasis, which is determined by the characteristics of lymphatic reflux in the esophageal wall (22, 23). Also, lymph node metastasis is the most important prognostic factor of ESCC. As to the “seeds and soil” hypothesis, lymph node metastasis is not a simple process of direct migration of ESCC cells. Many kinds of literature have reported that the niche of ESCC lymph nodes has changed significantly before metastasis (24, 25). It has been shown that the lymph node immune status of pN0 and pN1 patients is completely different. There is an obvious activated pattern of immune response in the pN0 patients. On the contrary, pN1 patients show a distinct pattern of inhibition, such as reduced immune response, immune cell proliferation, and increased immune cell apoptosis (26, 27). It means that in the early stage of ESCC metastasis, drainage of tumor antigens to lymph nodes results in the antitumor status. However, as time goes on, more and more tumor secretory factors and immunosuppressive cells will accumulate. Then, the immune state of lymph nodes will change from antitumor to pro-tumor mode until the tumor cells first colonize and metastasize (28). Therefore, an in-depth study of the interaction between tumor cells and the immune microenvironment and how it promotes the ESCC invasion and metastasis will guide the development of future diagnosis and treatment strategies.
3 The Role of Tumor Microenvironment in Esophageal Squamous Cell Carcinoma Invasion and Metastasis
3.1 Immune Modulation Promotes Esophageal Squamous Cell Carcinoma Invasion and Metastasis
Tumors escaping from the immune system are the key to tumor invasion and metastasis. Tumor cells can form specific TME that inhibits antitumor immune response by recruiting various alternative tumor-associated immune cells or expressing inhibitory molecular factors (Figure 2). Specific immune cell types and influencing factors in ESCC will be discussed below.
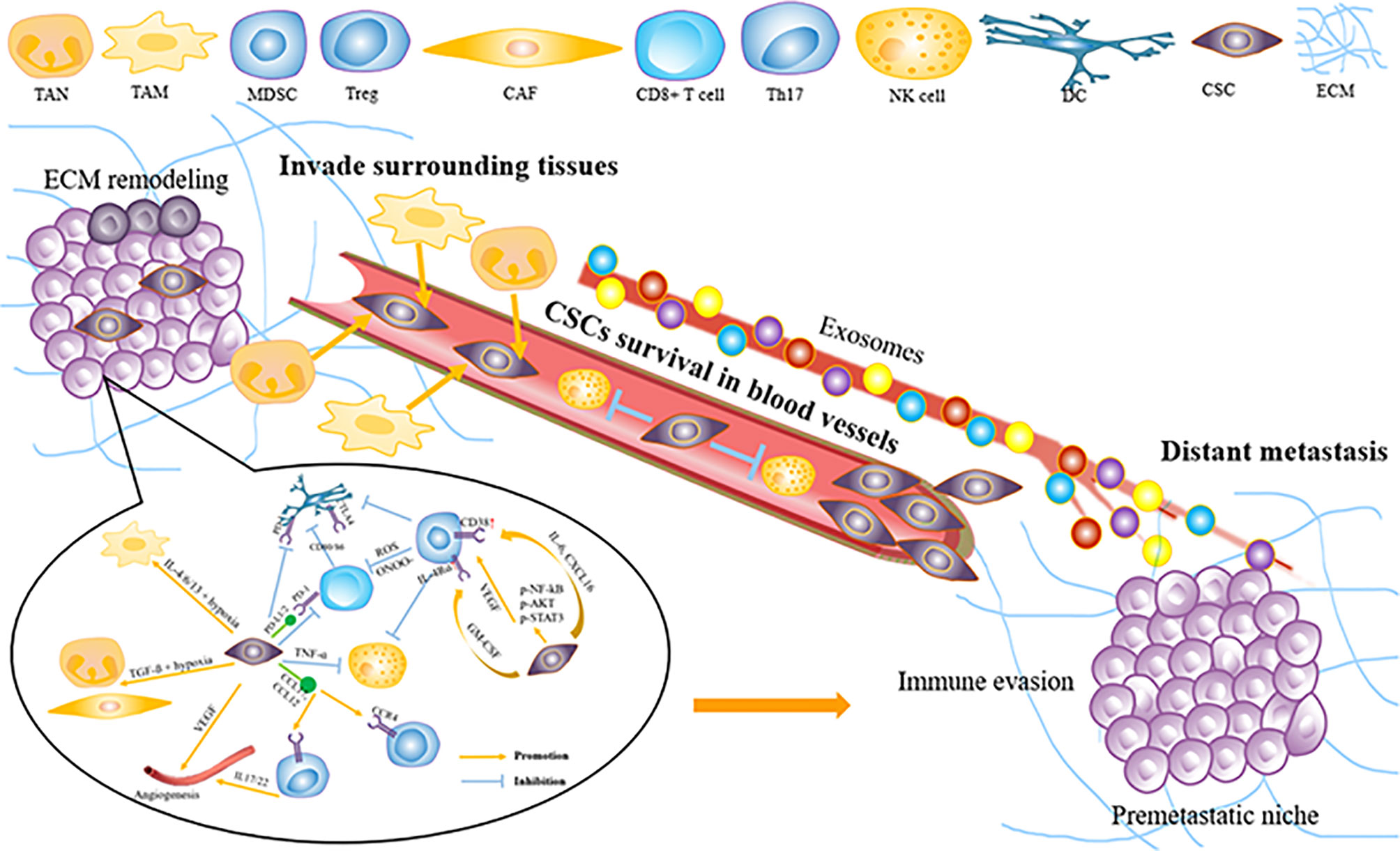
Figure 2 How tumor microenvironment support ESCC invasion and metastasis. ESCC, esophageal squamous carcinoma; MD.SC, man-our-derived suppressor cell; DC, dendritic cell; Treg, regulatory T cell; CAE, Cancer associated fibroblast; VEGF, vascular endothelial growth factor; NK cell, natural killer cell; TAM, tumor-associated macrophage; TAN, tumor-related neutrophil; ECM, extracellular matrix; CSCs, cancer Stem cells.
3.1.1 Myeloid-Derived Suppressor Cells
Myeloid-derived suppressor cells (MDSCs) are the suppressive cell population of the immune system, which play a pivotal role in the TME (29). MDSCs can greatly inhibit the cytotoxic function of T cells and NK cells during circulation and support ESCC progression (30). The specific markers of MDSCs are most often identified by the expression of CD11b and lack of HLA-DR expression (31). In ESCC, MDSCs produce reactive oxygen species (ROS) and peroxynitrite (ONOO−), which block the activation and proliferation of T cells to disrupt immune responses (32). Also, MDSCs inhibit the proliferation of CD8+ T cells by phosphorylating T-cell receptor (TCR) and CD8 molecules during direct interaction with T cells, which results in the downregulation of immune activity (33, 34). In addition, VEGF produced by MDSCs promotes tumor angiogenesis, creates a pre-metastasis environment, and prolongs immunosuppression (35, 36). Furthermore, it has been demonstrated recently that MDSCs could paralyze T cells by cell–cell transfer of the metabolite methylglyoxal, which would reduce the antitumor immunity of T cells and promote invasion and metastasis (37). Further research into the biology of MDSCs, especially the functions of specific population cells, will provide directions for therapeutic development.
3.1.2 Regulatory T Cells
Tregs, a subgroup of CD4+ helper T cells identified by CD25 and Foxp3 expression, play an immunosuppressive role in cancer. Tregs attenuate antitumor immunity by secreting immunosuppressive cytokines, interfering with tumor-associated antigen presentation, and inhibiting cytotoxic cell function (38, 39). It has been demonstrated that Foxp3 expression in ESCC means a poor prognosis (40, 41). It is reported that FOXP3 might directly inhibit the IL-2 and promote cytotoxic T lymphocyte-associated antigen 4 (CTLA4) and CD25 expression (42). In ESCC, increased recruitment of Tregs is mediated, at least in part, by chemokines CCL17 and CCL22, secreted by tumor cells and macrophages (43). It has been reported that IL-33, which has a high expression in ESCC, could promote CCL2 expression via the NF-κB pathway and then recruit Tregs to promote ESCC migration (44, 45). Treg infiltration has been found to be prognostic, and more Tregs are often associated with deeper tumor invasion, extensive metastasis, and reduced survival (46, 47). Tregs have several context-dependent functions that are not well described, which poses challenges for ESCC invasion and migration.
3.1.3 Tumor-Associated Macrophages
Tumor-associated macrophages (TAMs) promote various pro-tumor mechanisms. Macrophages are classified into M1 and M2 types, of which M2 macrophages secreted type II cytokines to facilitate various pro-tumorigenic mechanisms (48). The specific markers of TAMs are most often identified by expression of iNOS for M1 type and CD163 for M2 type. Hypoxia can induce M2 polarization, and then TAMs will produce growth factors and proteases that promote tumorigenesis and inhibit the immune system, angiogenesis, invasion, and metastasis (49, 50). CD68+ PD-1+ TAMs in ESCC TME tend to be of M2 phenotype, which can result in the upregulation of PD-L1 expression in tumor cells and promote ESCC invasion and migration (51, 52). Activation of the AKT/ERK pathway is a driving force for ESCC cell invasion and migration, and this pathway can be triggered by a variety of factors produced by TAMs or cancer cells themselves (53, 54). CD163+ TAMs can also promote ESCC cell invasion and migration by releasing thymidine phosphorylase (TP) to augment angiogenesis and produce IL-1β to enhance EMT (55, 56). The M2/M1 macrophage ratio of ESCC patients has also been used as a predictor of lymph node metastasis (57). All of these suggest potential intervention and immunotherapy strategies for TAMs in the invasion and migration of ESCC patients.
3.1.4 Tumor-Associated Neutrophils
Tumor-associated neutrophils (TANs) are completely different from circulating neutrophils (58). Transforming growth factor-β (TGF-β) in TME promotes the transformation of neutrophils from antitumor N1 to pro-tumor N2 (59). Unlike M1 and M2, there is no suitable marker to indicate the N1 and N2 neutrophils in the tumor (60). The study of TANs mainly focuses on the neutrophil-to-lymphocyte ratio (NLR) (60). It has been reported that preoperative NLR elevation was associated with lymph node metastasis, deeper tumor invasion, and advanced TNM stage (61). Neutrophils will undergo apoptosis after activation, forming neutrophil extracellular traps (NETs), which have been shown to predict the lymph node and distant metastasis (62, 63). All of these indicate that TANs can be a good predictor of ESCC invasion and migration.
3.1.5 Mast Cells and Eosinophils
Mast cells (MCs) and eosinophils often co-participate in response to parasitic infections and allergic diseases (64). In the TME of ESCC, high MC density has been found to be closely associated with tumor angiogenesis, invasion, and metastasis and predicts poor survival in ESCC patients (65, 66). It is reported that trypsin release from MCs promotes tumor cell metastasis through exosomes (67). Yet the high expression of eosinophils has been reported to be positively associated with low rates of metastasis in early ESCC patients (68). Also, it has been reported recently that metastasis-entrained eosinophils could promote lymphocyte-mediated antitumor immunity (69). A large number of new studies are needed for the mechanism of eosinophil in ESCC, which will provide new ideas for the ESCC invasion and metastasis and eosinophil-based immunotherapy.
3.1.6 Th17 Lymphocytes
Th17 lymphocytes are a branch of CD4+ helper T cells, and IL-17 is its main effector molecule. IL-17A expressed by Th17 cells can induce the production of chemokines in ESCC cells, such as CCL20, CXCL-9, CXCL-10, and CXCL13 (70, 71). These chemokines could promote the proliferation and differentiation of Th17 lymphocytes in ESCC TME (72). Also, increased Th17 lymphocytes are positively associated with more lymph node metastasis (73). It has been reported that IL-17A can activate MMP-2 and MMP-9 through the ROS/NF-κB signaling pathway (74), while matrix metalloproteinases (MMPs) could catalyze the degradation of extracellular matrix and promote ESCC migration and metastasis (75, 76). The role of Th17 lymphocytes in ESCC invasion and metastasis needs to be further investigated.
3.2 Stromal Components Facilitate Esophageal Squamous Cell Carcinoma Invasion and Metastasis
In addition to immune cells, stromal components and CAFs play a critical role in ESCC invasion and metastasis (77) (Figure 2). Fibroblast activation protein-α (FAP) and α-smooth muscle actin (α-SMA) are often used as the markers for the activated phenotype of CAFs, of which the process is induced by ESCC secreting TGF-β (78, 79).
CAFs have been proved to promote ESCC invasion and metastasis by secretion of cytokines, induction of EMT, recruitment of immune cells, and other mechanisms to reconstruct TME (80). IL-6 secreted by FAP+ CAFs not only can promote ESCC cell invasion and EMT but also can recruit FoxP3+ T cells and induce TAM M2 polarization to promote metastasis (81, 82). The presence of CAFs in ESCC patients is associated with increased micro-vessel density, TAMs, and EMT, which are critical for ESCC invasion and metastasis (83, 84). A number of genes have been shown to promote ESCC invasion and metastasis via the CAF transformation and EMT process (85, 86). Also, it has been demonstrated that CAFs promote ESCC invasion by secreting hepatocyte growth factor (HGF) and infiltrating MDSCs (87, 88). Also, CAFs have been reported to be associated with low 3-year survival and ESCC progression after chemoradiotherapy (89). FAP-α has been reported to be an important regulator in ESCC lymph node metastasis (90). HGF and TGF-β are closely related to tumor invasion and metastasis (91). It has been demonstrated that CAFs could express HGF and TGF-β1 and then promote ESCC invasion and metastasis via the HGF/Met and TGFβ1/Smad pathways, respectively (92, 93). It has been confirmed that infiltrating MDSCs activate CAFs to promote ESCC invasion (94). Interaction between CAFs and immune cells to promote ESCC invasion and metastasis needs further research.
Due to the high heterogeneity of ESCC, traditional genomic and transcriptome analyses tend to ignore some signals displayed by specific cell populations or cell states. However, with the development of single-cell sequencing technology, several single-cell studies about ESCC and TME have been published in recent years. It has been reported that single-cell transcriptome sequencing was performed in 11 ESCC patients to analyze the TME. Heterogeneity was found in most ESCC interstitial cell types, particularly between fibroblasts and immune cells. Also, tumor-specific CST1+ myofibroblast subpopulations had been identified to have prognostic values and potential biological significance (95). Also, the main association framework between cancer cells and various non-cancer cells in TME has been established via single-cell transcriptome sequencing, which contributes to the further investigation of ESCC progression and prognosis (96). Furthermore, a comparison between esophagus non-malignant tissues and ESCC tissues via single-cell transcriptome network analysis has shown that energy supply-related pathways are pivotal in cancer metabolic reprogramming for TME. Immune checkpoints, which are potential targets for ESCC immunotherapy, have been found to be significantly overexpressed in ESCC, including LAG3 and HAVCR2 (97). At present, there are no single-cell studies specifically for ESCC invasion and metastasis, which needs further investigation.
4 The Role of Cellular Communication in Esophageal Squamous Cell Carcinoma Invasion and Metastasis
4.1 Tumor Cells Remodel Tumor Microenvironment to Promote Esophageal Squamous Cell Carcinoma Invasion and Metastasis
4.1.1 Cytokine/Chemokine Network
Metastasis is a multistep process that requires tumor cells to separate from the primary tumor and migrate through the lymphatic or blood circulatory system to target distant organs (98). There is increasing evidence that primary tumors can prepare the cytokine/chemokine network for invasion and metastasis (99, 100) (Figure 2).
CXCL12 is a chemokine that functions through CXCR4 and plays an important role in ESCC invasion and metastasis (101). It is noteworthy that CXCR4 is expressed only in ESCC tissues but not in the normal esophageal epithelium (102). Expression of CXCL12 or CXCR4 in ESCC patients is significantly related to ESCC invasion, lymph node metastasis, and poor survival (103, 104). It has been shown that ESCC cells could secrete large amounts of CXCL12 via an autocrine way and increase their receptor CXCR4 expression compared with normal cells (105). Also, ESCC cells could enhance the activation of the p-ERK1/2 pathway via the CXCL12/CXCR4 axis to promote ESCC invasion and metastasis (106).
It has been reported that CCR7, combined with CCL21, supports a metastatic niche directly (107). A number of studies have shown that high levels of CCR7 are related to ESCC metastasis and poor survival (108). It has been investigated that co-expressed CCR7 and MUC1 could facilitate ESCC invasion and metastasis via the ERK1/2 pathway (109, 110). Some studies have also demonstrated that there is an interaction between CCR7 and VEGF-C, and their expression can be used as the predictor for ESCC lymphatic metastasis (111).
Many studies have indicated that high levels of CXCL8 and CXCR2 in ESCC patients are associated with metastasis and poor prognosis (112). It has been shown that CXCL8 is upregulated in TAMs and promotes ESCC invasion and metastasis via CXCR1/CXCR2 receptors to activate AKT and ERK1/2 signaling pathways (52). Also, a clinical study has shown that CXCL8 expression is significantly associated with metastasis and the increase of CXCR2- and CD204-positive macrophages (108, 113). It is necessary to further investigate the biological significance of cytokine/chemokine networks in ESCC and their potential use as future drug targets.
4.1.2 Exosome
Exosomes are nanovesicles (30–150-nm diameter) that are secreted by various cell types (114). Recently, it has been shown that exosomes play important roles in ESCC invasion and metastasis (115). It is reported that exosomes released by ESCC can enrich miR-320b and promote ESCC lymph node metastasis via programmed cell death 4 (PDCD4) through the AKT signaling pathway (116). Exosome-shuttling miR-21 has been shown to promote ESCC invasion and metastasis by targeting PDCD4 via the c-Jun N-terminal kinase (JNK) signaling pathway (117). Clinical data have also displayed that serum exosomal hsa_circ_0026611 expression is significantly upregulated with ESCC lymph node metastasis (118). Exosome long non-coding RNA (lncRNA) LINC01711 promotes ESCC invasion via FSCN1 upregulation and miR-326 downregulation (119). Also, it has been reported that T cell-derived exosomes promote ESCC metastasis via promoting EMT by β-catenin and NF-κB/snail signaling pathways upregulation (120). However, there is still a long way to the mechanisms of how these exosomes are involved in ESCC invasion and metastasis.
4.1.3 Vascular Endothelial Growth Factor
VEGF is the key mediator of angiogenesis, which has the function of triggering endothelial cell proliferation, migration, and breakdown of the extracellular matrix for new blood vessels. It has been reported that when tumor cells overexpressing HMGB1 co-cultured with B cells, the proliferating B cells can be induced to express VEGF and then elevate angiogenesis (121). A significant decrease in VEGF-C has been found in high tumor lymphocytic infiltration (122). It is reported that low expression of CD80 can be associated with VEGF overexpression. CD80 impairment in the ESCC tissues is correlated with poor survival, which indicates the dysfunction of the immune system and promotes the ESCC progression (123). Some studies have confirmed that VEGF-C, a lymphangiogenic factor, is associated with survival, tumor depth, stage, and lymph node metastasis of ESCC (124, 125). Also, many genes have been reported to promote ESCC invasion and metastasis via VEGF-related pathways or axis (126, 127). Development of new angiogenesis inhibitors and regulation of tumor vascular microenvironment are still possible ways to treat ESCC invasion and metastasis.
4.2 The Interaction Between Immune Cells Promotes Esophageal Squamous Cell Carcinoma Invasion and Metastasis
In addition to the interaction between various immune cells and ESCC cells, there is an important interaction among various immune cells, which indirectly promotes ESCC invasion and metastasis. For example, Th-2 could secrete many cytokines (IL-6 and IL-13) to recruit MDSCs in the ESCC TME (128, 129). Also, IL-4 and IL-13 derived from Th-2 could promote macrophages polarizing into M2 macrophages (130). MDSCs with high CD38 levels have been reported to inhibit the cytotoxic effect of ESCC-activated T cells (131). MDSCs could also induce Tregs and CAFs to inhibit the antigen-presenting cells (APCs) and indirectly inhibit the cytotoxic effect of ESCC-activated T cells (42, 132). In addition, ESCC cells could produce RCAS1 to induce DC, promote tumor-infiltrating lymphocyte apoptosis, and inhibit CD8+ T-cell activity (133). IL-17A-producing cells could enhance CD1a+ DC infiltration of TME via the release of CCL2 or CCL20, which is associated with better survival in ESCC patients (134). Th17 cells and MCs in ESCC TME have been shown to secrete IL-17 to promote ESCC cells to release CXCL9/10, CXCL2/3, and CCL2/20, which could facilitate NK cell infiltration and activity (66). PD-1, a member of the CD28 family, is mainly expressed on activated T cells (135). When PD-1 is combined with its ligand (PD-L1 or PD-L2), which can be expressed by tumor cells, immune cells (i.e., macrophages), and endothelial cells, then T-cell activation will be inhibited (136, 137). TME contains a variety of immune cells, which form a complex regulatory network through receptor-ligand binding or the release of various immune factors, thus affecting the invasion and metastasis of ESCC.
5 Targeting Tumor Microenvironment for Esophageal Squamous Cell Carcinoma Invasion and Metastasis
Targeting approaches using different methods to remodel the TME and then inhibit ESCC invasion and metastasis are discussed as follows (Figure 3).
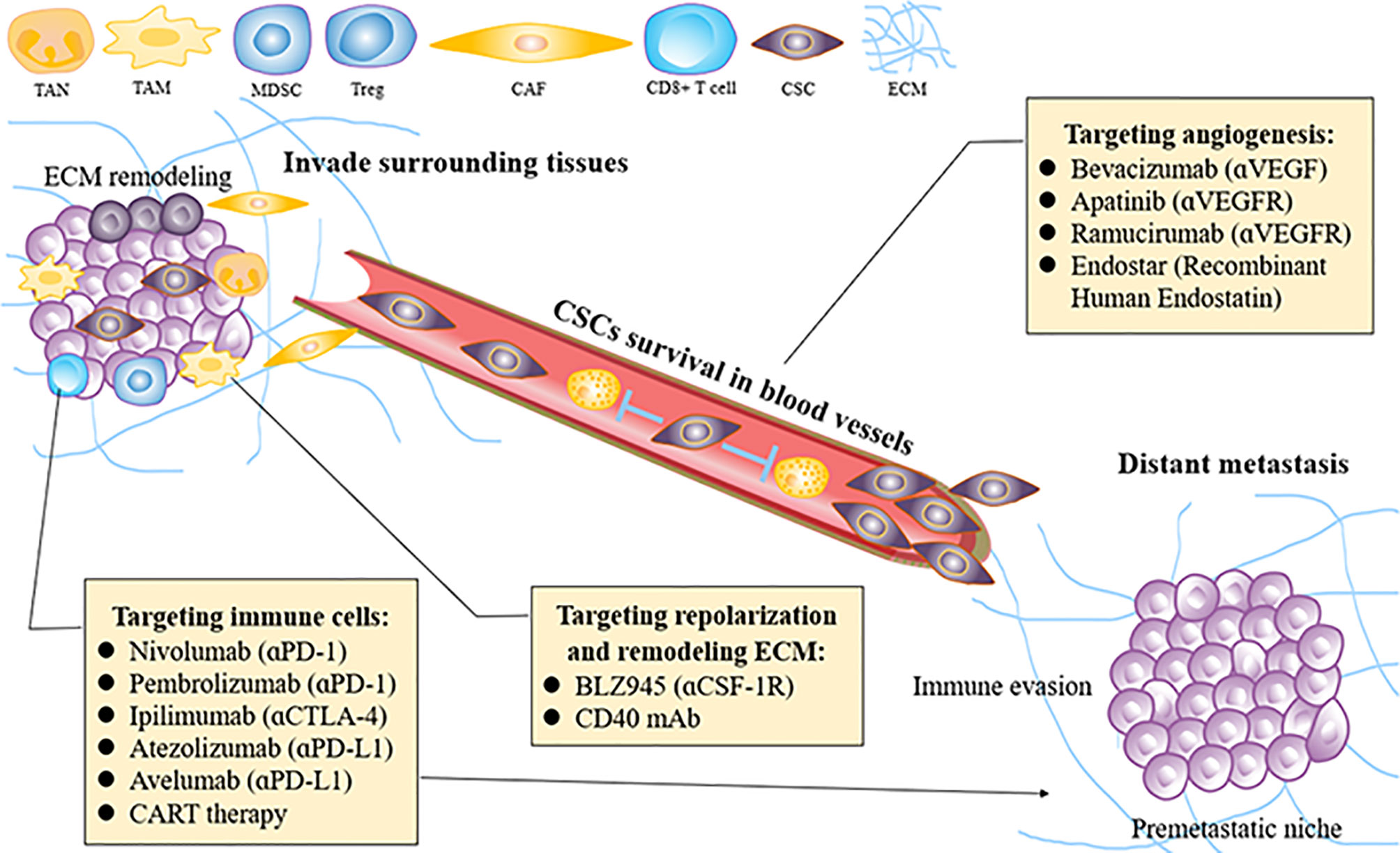
Figure 3 How to re-educate the tumor microenvironment for treating ESCC invasion and metastasis. ESCC, esophageal squamous carcinoma; MDSC, mallow-derived suppressor cell; Treg, regulatory T cell; CAF, cancer associated fibroblast; VEGF, vascular endothelial growth factor; TAM, tumor-associated macrophage; TAN, tumor-related neutrophil; ECM, extracellular matrix; CSCs, cancer stem cells; PID-1, programmed cell death protein 1; CTLA4, T lymphocyte-associated antigen 4; CSF-1, colony-stimulating factor-1.
5.1 Targeting Angiogenesis for Esophageal Squamous Cell Carcinoma Invasion and Metastasis
Angiogenesis plays a crucial role in the development of ESCC, by delivering oxygen and nutrients to tumors, and its key mediator is VEGF (138). Distant vascular metastasis is another way of tumor progression. Many VEGF/VEGFR inhibitors have been developed to induce vascular normalization and make patients more sensitive to chemotherapy (139). It has been found that low doses of VEGF inhibitor (apatinib) could regulate the TME, relieve hypoxia, and increase the number of T cells at the tumor site, thereby enhancing the efficacy of PD-1/PD-L1 inhibitors, while excessive doses do not produce such an effect (140). However, this theory has not been tested in ESCC. The development of new angiogenesis inhibitors and regulation of vascular TME are still possible ways to avoid ESCC invasion and metastasis.
5.2 Targeting Immune Markers for Esophageal Squamous Cell Carcinoma Invasion and Metastasis
5.2.1 Immune Checkpoint Inhibitors
PD-1 is an immune checkpoint that inactivates T-cell immune function. Its two ligands, PD-L1 and PD-L2, combined with the PD-1 receptor, could induce depletion of PD-1 signaling pathways and associated T cells and inhibit T-cell activation and proliferation reversibly (141). Many studies have reported that the expression of both PD-L1 and PD-L2 is elevated in ESCC. In fact, in ESCC patients, increased PD-L1 or PD-L2 expression in ESCC cells is correlated with reduced survival, while increased PD-L1 expression is associated with increased depth of tumor invasion and worse survival (142, 143). In addition, the expression of PD-L2 is related to decreased CD8+ T-cell infiltration. The increased PD-L2 expression is induced by tumor-promoting Th2 cytokines such as IL-13 or IL-4 (144).
The expression of CTLA4 is another immune checkpoint that inactivates by inhibiting TCR signaling (145). CTLA4 is expressed not only in tumor-infiltrating immune cells but also in cancer cells, which is a key part of immune escape (146). Existing evidence already suggests that PD-1 inhibitors show therapeutic promise in lung cancer and melanoma and might also be used in ESCC (147). Also, many studies are targeted at how to regulate other immune cells in TME to improve the efficacy of immunotherapy (148, 149).
5.2.2 Other Immune Cells
TAMs can produce a variety of tumor-promoting factors, such as colony-stimulating factor-1 (CSF-1), so they might be attractive targets for remodeling immune responses within TME (150). In recent years, targeting TAM therapies such as CSF-1 or CSF-1R blockade have attracted extensive attention in tumor research. The combination of CSF-1R blockade and PD-1/PD-L1 inhibitors is underway (NCT02323191) (151). IL-6 secreted by FAP+ CAFs not only can promote ESCC cell invasion and EMT but also can recruit FoxP3+ T cells and induce TAM M2 polarization to promote metastasis (81, 82). Using CAF-targeted NIR-PIT to eliminate CAFs could interfere with ESCC invasion and metastasis effectively. The combination of the CAF-targeted NIR-PIT with traditional anticancer drugs might be a promising choice (152).
5.3 T-Cell Modification for Esophageal Squamous Cell Carcinoma Invasion and Metastasis
Chimeric antigen receptor (CAR) T-cell therapy means that T cells are modified into CAR T cells by genetic engineering to specifically recognize and attack tumor cells (153). Ephrin type A receptor 2 (EphA2) and HER-2, highly expressed in ESCC, are common targets of CAR T-cell therapy and have been verified to effectively kill esophageal cancer cells (154, 155). Enhanced MUC1-CAR T cells have been shown to have better antitumor activity because they can survive longer in vivo, which means they have long-lasting antitumor effects (156). Also, it has been recently reported that IDO1 inhibitor-loaded nanosheets could enhance CAR T-cell effectiveness in ESCC and CD276 suppress CAR T-cell function (157, 158). The selection of different solid tumor-specific antigens and the delivery of CAR T cells are still the disadvantages of CAR T-cell therapy (159).
6 Conclusion
In this review, we have summarized how ESCC invasion and metastasis occur and discussed how the major cell populations, stromal components, and their interaction in the TME promote ESCC invasion and metastasis. Also, we summarized recent therapies targeting TME for ESCC invasion and metastasis. Looking forward, it is critical to further investigate how cancer cells transfer to the new environment and adapt surrounding cells and components into a suitable environment for tumor invasion and metastasis. At present, there are few diagnostic methods and new drugs targeted for ESCC invasion and metastasis. Advances in these areas promise improved treatment options and better outcomes for this deadly disease.
Author Contributions
XYG and BLL supervised and reviewed the manuscript. SYZ conducted the literature review and wrote the manuscript. All authors contributed to the article and approved the submitted version.
Funding
This work was supported by grants from the Hong Kong Research Grant Council (RGC) grants including Collaborative Research Funds (C7065-18GF, C7026-18GF, and C4039-19GF), Research Impact Funds (R4017-18, R1020-18F, and R7022-20), Shenzhen Fundamental Research Program (JCYJ20180508153249223), The Shenzhen Science and Technology program (KQTD20180411185028798), and the Program for Guangdong Introducing Innovative and Entrepreneurial Teams (2019BT02Y198). XYG is the Sophie YM Chan Professor in Cancer Research.
Conflict of Interest
The authors declare that the research was conducted in the absence of any commercial or financial relationships that could be construed as a potential conflict of interest.
Publisher’s Note
All claims expressed in this article are solely those of the authors and do not necessarily represent those of their affiliated organizations, or those of the publisher, the editors and the reviewers. Any product that may be evaluated in this article, or claim that may be made by its manufacturer, is not guaranteed or endorsed by the publisher.
References
1. Bray F, Ferlay J, Soerjomataram I, Siegel RL, Torre LA, Jemal A, et al. Global Cancer Statistics 2018: GLOBOCAN Estimates of Incidence and Mortality Worldwide for 36 Cancers in 185 Countries. CA Cancer J Clin (2018) 68(6):394–424. doi: 10.3322/caac.21492
2. Ajani JA, D'Amico TA, Bentrem DJ, Chao J, Corvera C, Das P, et al. Esophageal and Esophagogastric Junction Cancers, Version 2.2019, NCCN Clinical Practice Guidelines in Oncology. J Natl Compr Canc Netw (2019) 17(7):855–83. doi: 10.6004/jnccn.2019.0033
3. Creemers A, Ebbing EA, Hooijer GKJ, Stap L, Jibodh-Mulder RA, Gisbertz SS, et al. The Dynamics of HER2 Status in Esophageal Adenocarcinoma. Oncotarget (2018) 9(42):26787–99. doi: 10.18632/oncotarget.25507
4. Barsouk A, Rawla P, Hadjinicolaou AV, Aluru JS, Barsouk A. Targeted Therapies and Immunotherapies in the Treatment of Esophageal Cancers. Med Sci (Basel) (2019) 7(10):100. doi: 10.3390/medsci7100100
5. Siegel RL, Miller KD, Jemal A. Cancer Statistics, 2019. CA Cancer J Clin (2019) 69(1):7–34. doi: 10.3322/caac.21551
6. Jin Z, Chen D, Chen M, Wang C, Zhang B, Zhang J, et al. Neoadjuvant Chemoradiotherapy Is Beneficial to the Long-Term Survival of Locally Advanced Esophageal Squamous Cell Carcinoma: A Network Meta-Analysis. World J Surg (2022) 46(1):136–46. doi: 10.1007/s00268-021-06301-2
7. Guccione C, Yadlapati R, Shah S, Shah S, Knight R, Curtius K, et al. Challenges in Determining the Role of Microbiome Evolution in Barrett's Esophagus and Progression to Esophageal Adenocarcinoma. Microorganisms (2021) 9(10):2003. doi: 10.3390/microorganisms9102003
8. Klein S, Duda DG. Machine Learning for Future Subtyping of the Tumor Microenvironment of Gastro-Esophageal Adenocarcinomas. Cancers (Basel) (2021) 13(19):4919. doi: 10.3390/cancers13194919
9. Nienhüser H, Wirsik N, Schmidt T. Esophageal Tumor Microenvironment. Adv Exp Med Biol (2020) 1296:103–16. doi: 10.1007/978-3-030-59038-3_6
10. Han P, Cao P, Hu S, Kong K, Deng Y, Zhao B, et al. Esophageal Microenvironment: From Precursor Microenvironment to Premetastatic Niche. Cancer Manag Res (2020) 12:5857–79. doi: 10.2147/CMAR.S258215
11. Wang L, Han H, Wang Z, Shi L, Yang M, Qin Y, et al. Targeting the Microenvironment in Esophageal Cancer. Front Cell Dev Biol (2021) 9:684966. doi: 10.3389/fcell.2021.684966
12. Lv X, Xu G. Regulatory Role of the Transforming Growth Factor-β Signaling Pathway in the Drug Resistance of Gastrointestinal Cancers. World J Gastrointest Oncol (2021) 13(11):1648–67. doi: 10.4251/wjgo.v13.i11.1648
13. Cheung KJ, Ewald AJ. A Collective Route to Metastasis: Seeding by Tumor Cell Clusters. Science (2016) 352(6282):167–9. doi: 10.1126/science.aaf6546
14. Doglioni G, Parik S, Fendt SM. Interactions in the (Pre)metastatic Niche Support Metastasis Formation. Front Oncol (2019) 9:219. doi: 10.3389/fonc.2019.00219
15. Akhtar M, Haider A, Rashid S, Al-Nabet ADMH. Paget's “Seed and Soil” Theory of Cancer Metastasis: An Idea Whose Time has Come. Adv Anat Pathol (2019) 26(1):69–74. doi: 10.1097/PAP.0000000000000219
16. Wortzel I, Dror S, Kenific CM, Lyden D. Exosome-Mediated Metastasis: Communication From a Distance. Dev Cell (2019) 49(3):347–60. doi: 10.1016/j.devcel.2019.04.011
17. Sun H, Meng Q, Shi C, et al. Hypoxia-Inducible Exosomes Facilitate Liver-Tropic Premetastatic Niche in Colorectal Cancer. Hepatology (2021) 74(5):2633–51. doi: 10.1002/hep.32009
18. Tang F, Tie Y, Hong W, Wei Y, Tu C, Wei X, et al. Targeting Myeloid-Derived Suppressor Cells for Premetastatic Niche Disruption After Tumor Resection. Ann Surg Oncol (2021) 28(7):4030–48. doi: 10.1245/s10434-020-09371-z
19. Paiva AE, Lousado L, Guerra DAP, Azevedo PO, Sena IFG, Andreotti JP, et al. Pericytes in the Premetastatic Niche. Cancer Res (2018) 78(11):2779–86. doi: 10.1158/0008-5472.CAN-17-3883
20. Maughan BL, Pal SK, Gill D, Boucher K, Martin C, Salgia M, et al. Modulation of Premetastatic Niche by the Vascular Endothelial Growth Factor Receptor Tyrosine Kinase Inhibitor Pazopanib in Localized High-Risk Prostate Cancer Followed by Radical Prostatectomy: A Phase II Randomized Trial. Oncologist (2018) 23(12):1413–e151. doi: 10.1634/theoncologist.2018-0652
21. Kwa MQ, Herum KM, Brakebusch C. Cancer-Associated Fibroblasts: How Do They Contribute to Metastasis? Clin Exp Metastasis (2019) 36(2):71–86. doi: 10.1007/s10585-019-09959-0
22. Chen D, Mao Y, Zheng Y, Wen J, Song P, Xue Y, et al. Extracapsular Lymph Node Involvement Is a Robust Survival Predictor in Esophageal Cancer Patients: A Pooled Analysis. Eur J Surg Oncol (2021) 47(8):1875–82. doi: 10.1016/j.ejso.2021.03.247
23. Rani P, Gupta AJ, Mehrol C, Singh M, Khurana N, Passey JC, et al. Clinicopathological Correlation of Tumor-Stroma Ratio and Inflammatory Cell Infiltrate With Tumor Grade and Lymph Node Metastasis in Squamous Cell Carcinoma of Buccal Mucosa and Tongue in 41 Cases With Review of Literature. J Cancer Res Ther (2020) 16(3):445–51. doi: 10.4103/0973-1482.193113
24. Patel S, Raghavan S, Prem A. Prognostic Impact of Pathological Response in Lymph Nodes in Esophageal Squamous Cell Cancers: Is It Over-Rated? Eur J Surg Oncol (2021) 47(11):2959. doi: 10.1016/j.ejso.2021.07.031
25. Zhong J, Wang K, Fang S, Fu J. Prognostic Impact of Sterilized Lymph Nodes in Esophageal Squamous Cell Carcinomas After Neoadjuvant Chemoradiotherapy. Eur J Surg Oncol (2021) 47(12):3074–80. doi: 10.1016/j.ejso.2021.04.026
26. Otto B, Koenig AM, Tolstonog GV, Jeschke A, Klaetschke K, Vashist YK, et al. Molecular Changes in Pre-Metastatic Lymph Nodes of Esophageal Cancer Patients. PloS One (2014) 9(7):e102552. doi: 10.1371/journal.pone.0102552
27. Wakita A, Motoyama S, Sato Y, Kawakita Y, Nagaki Y, Terata K, et al. Evaluation of Metastatic Lymph Nodes in Cn0 Thoracic Esophageal Cancer Patients With Inconsistent Pathological Lymph Node Diagnosis. World J Surg Oncol (2020) 18(1):111. doi: 10.1186/s12957-020-01880-1
28. Zhou L, Zhao Y, Zheng Y, Wang M, Tian T, Lin S, et al. The Prognostic Value of the Number of Negative Lymph Nodes Combined With Positive Lymph Nodes in Esophageal Cancer Patients: A Propensity-Matched Analysis. Ann Surg Oncol (2020) 27(6):2042–50. doi: 10.1245/s10434-019-08083-3
29. Ma T, Renz BW, Ilmer M, Koch D, Yang Y, Werner J, et al. Myeloid-Derived Suppressor Cells in Solid Tumors. Cells (2022) 11(2):310. doi: 10.3390/cells11020310
30. Xu J, Peng Y, Yang M, Guo N, Liu H, Gao H, et al. Increased Levels of Myeloid-Derived Suppressor Cells in Esophageal Cancer Patients is Associated With the Complication of Sepsis. BioMed Pharmacother (2020) 125:109864. doi: 10.1016/j.biopha.2020.109864
31. Hegde S, Leader AM, Merad M. MDSC: Markers, Development, States, and Unaddressed Complexity. Immunity (2021) 54(5):875–84. doi: 10.1016/j.immuni.2021.04.004
32. Li R, Jia Z, Trush MA. Defining ROS in Biology and Medicine. React Oxyg Species (Apex) (2016) 1(1):9–21. doi: 10.20455/ros.2016.803
33. Zhou H, Yang P, Li H, Zhang L, Li J, Zhang T, et al. Carbon Ion Radiotherapy Boosts Anti-Tumour Immune Responses by Inhibiting Myeloid-Derived Suppressor Cells in Melanoma-Bearing Mice. Cell Death Discovery (2021) 7(1):332. doi: 10.1038/s41420-021-00731-6
34. Wang Y, Sun H, Zhu N, Wu X, Sui Z, Gong L, et al. Myeloid-Derived Suppressor Cells in Immune Microenvironment Promote Progression of Esophagogastric Junction Adenocarcinoma. Front Oncol (2021) 11:640080. doi: 10.3389/fonc.2021.640080
35. Ren Y, Dong X, Zhao H, Feng J, Chen B, Zhou Y, et al. Myeloid-Derived Suppressor Cells Improve Corneal Graft Survival Through Suppressing Angiogenesis and Lymphangiogenesis. Am J Transpl (2021) 21(2):552–66. doi: 10.1111/ajt.16291
36. Horikawa N, Abiko K, Matsumura N, Hamanishi J, Baba T, Yamaguchi K, et al. Expression of Vascular Endothelial Growth Factor in Ovarian Cancer Inhibits Tumor Immunity Through the Accumulation of Myeloid-Derived Suppressor Cells. Clin Cancer Res (2017) 23(2):587–99. doi: 10.1158/1078-0432.CCR-16-0387
37. Baumann T, Dunkel A, Schmid C, Schmitt S, Hiltensperger M, Lohr K, et al. Regulatory Myeloid Cells Paralyze T Cells Through Cell-Cell Transfer of the Metabolite Methylglyoxal. Nat Immunol (2020) 21(5):555–66. doi: 10.1038/s41590-020-0666-9
38. Huppert LA, Green MD, Kim L, Chow C, Leyfman Y, Daud AI, et al. Tissue-Specific Tregs in Cancer Metastasis: Opportunities for Precision Immunotherapy. Cell Mol Immunol (2022) 19(1):33–45. doi: 10.1038/s41423-021-00742-4
39. Yan S, Zhang Y, Sun B. The Function and Potential Drug Targets of Tumour-Associated Tregs for Cancer Immunotherapy. Sci China Life Sci (2019) 62(2):179–86. doi: 10.1007/s11427-018-9428-9
40. Xue L, Lu HQ, He J, Zhao XW, Zhong L, Zhang ZZ, et al. Expression of FOXP3 in Esophageal Squamous Cell Carcinoma Relating to the Clinical Data. Dis Esophagus (2010) 23(4):340–6. doi: 10.1111/j.1442-2050.2009.01013.x
41. Wang G, Liu G, Liu Y, Liu Y, Li X, Su Z, et al. FOXP3 Expression in Esophageal Cancer Cells Is Associated With Poor Prognosis in Esophageal Cancer. Hepatogastroenterology (2012) 59(119):2186–91. doi: 10.5754/hge11961
42. Shitara K, Nishikawa H. Regulatory T Cells: A Potential Target in Cancer Immunotherapy. Ann N Y Acad Sci (2018) 1417(1):104–15. doi: 10.1111/nyas.13625
43. Chen BJ, Zhao JW, Zhang DH, Zheng AH, Wu GQ. Immunotherapy of Cancer by Targeting Regulatory T Cells. Int Immunopharmacol (2022) 104:108469. doi: 10.1016/j.intimp.2021.108469
44. Yue Y, Lian J, Wang T, Luo C, Yuan Y, Qin G, et al. Interleukin-33-Nuclear Factor-κb-CCL2 Signaling Pathway Promotes Progression of Esophageal Squamous Cell Carcinoma by Directing Regulatory T Cells. Cancer Sci (2020) 111(3):795–806. doi: 10.1111/cas.14293
45. Cui G, Li Z, Ren J, Yuan A. IL-33 in the Tumor Microenvironment is Associated With the Accumulation of FoxP3-Positive Regulatory T Cells in Human Esophageal Carcinomas. Virchows Arch (2019) 475(5):579–86. doi: 10.1007/s00428-019-02579-9
46. Nabeki B, Ishigami S, Uchikado Y, Sasaki K, Kita Y, Okumura H, et al. Interleukin-32 Expression and Treg Infiltration in Esophageal Squamous Cell Carcinoma. Anticancer Res (2015) 35(5):2941–7.
47. Lian J, Liu S, Yue Y, Yang Q, Zhang Z, Yang S, et al. Eomes Promotes Esophageal Carcinoma Progression by Recruiting Treg Cells Through the CCL20-CCR6 Pathway. Cancer Sci (2021) 112(1):144–54. doi: 10.1111/cas.14712
48. Zhu S, Yi M, Wu Y, Dong B, Wu K, et al. Roles of Tumor-Associated Macrophages in Tumor Progression: Implications on Therapeutic Strategies. Exp Hematol Oncol (2021) 10(1):60. doi: 10.1186/s40164-021-00252-z
49. Zou Y, Zhang J, Xu J, Fu L, Xu Y, Wang X, et al. SIRT6 Inhibition Delays Peripheral Nerve Recovery by Suppressing Migration, Phagocytosis and M2-Polarization of Macrophages. Cell Biosci (2021) 11(1):210. doi: 10.1186/s13578-021-00725-y
50. Quail DF, Joyce JA. Microenvironmental Regulation of Tumor Progression and Metastasis. Nat Med (2013) 19(11):1423–37. doi: 10.1038/nm.3394
51. Kodaira H, Koma YI, Hosono M, Higashino N, Suemune K, Nishio M, et al. ANXA10 Induction by Interaction With Tumor-Associated Macrophages Promotes the Growth of Esophageal Squamous Cell Carcinoma. Pathol Int (2019) 69(3):135–47. doi: 10.1111/pin.12771
52. Hosono M, Koma YI, Takase N, Urakawa N, Higashino N, Suemune K, et al. CXCL8 Derived From Tumor-Associated Macrophages and Esophageal Squamous Cell Carcinomas Contributes to Tumor Progression by Promoting Migration and Invasion of Cancer Cells. Oncotarget (2017) 8(62):106071–88. doi: 10.18632/oncotarget.22526
53. Yokozaki H, Koma YI, Shigeoka M, Nishio M. Cancer as a Tissue: The Significance of Cancer-Stromal Interactions in the Development, Morphogenesis and Progression of Human Upper Digestive Tract Cancer. Pathol Int (2018) 68(6):334–52. doi: 10.1111/pin.12674
54. Okamoto M, Koma YI, Kodama T, Nishio M, Shigeoka M, Yokozaki H, et al. Growth Differentiation Factor 15 Promotes Progression of Esophageal Squamous Cell Carcinoma via TGF-β Type II Receptor Activation. Pathobiology (2020) 87(2):100–13. doi: 10.1159/000504394
55. Hu JM, Liu K, Liu JH, Jiang XL, Wang XL, Yang L, et al. The Increased Number of Tumor-Associated Macrophage is Associated With Overexpression of VEGF-C, Plays an Important Role in Kazakh ESCC Invasion and Metastasis. Exp Mol Pathol (2017) 102(1):15–21. doi: 10.1016/j.yexmp.2016.12.001
56. Liu J, Li C, Zhang L, Liu K, Jiang X, Wang X, et al. Association of Tumor-Associated Macrophages With Cancer Cell EMT, Invasion, and Metastasis of Kazakh Esophageal Squamous Cell Cancer. Diagn Pathol (2019) 14(1):55. doi: 10.1186/s13000-019-0834-0
57. Cao W, Peters JH, Nieman D, Sharma M, Watson T, Yu J, et al. Macrophage Subtype Predicts Lymph Node Metastasis in Esophageal Adenocarcinoma and Promotes Cancer Cell Invasion In Vitro. Br J Cancer (2015) 113(5):738–46. doi: 10.1038/bjc.2015.292
58. Hurt B, Schulick R, Edil B, El Kasmi KC, Barnett C Jr. Cancer-Promoting Mechanisms of Tumor-Associated Neutrophils. Am J Surg (2017) 214(5):938–44. doi: 10.1016/j.amjsurg.2017.08.003
59. Qin F, Liu X, Chen J, Huang S, Wei W, Zou Y, et al. Anti-TGF-β Attenuates Tumor Growth via Polarization of Tumor Associated Neutrophils Towards an Anti-Tumor Phenotype in Colorectal Cancer. J Cancer (2020) 11(9):2580–92. doi: 10.7150/jca.38179
60. Wu L, Saxena S, Awaji M, Singh RK. Tumor-Associated Neutrophils in Cancer: Going Pro. Cancers (Basel) (2019) 11(4):564. doi: 10.3390/cancers11040564
61. Sun Y, Zhang L. The Clinical Use of Pretreatment NLR, PLR, and LMR in Patients With Esophageal Squamous Cell Carcinoma: Evidence From a Meta-Analysis. Cancer Manag Res (2018) 10:6167–79. doi: 10.2147/CMAR.S171035
62. Liew PX, Kubes P. The Neutrophil's Role During Health and Disease. Physiol Rev (2019) 99(2):1223–48. doi: 10.1152/physrev.00012.2018
63. Rayes RF, Mouhanna JG, Nicolau I, Bourdeau F, Giannias B, Rousseau S, et al. Primary Tumors Induce Neutrophil Extracellular Traps With Targetable Metastasis Promoting Effects. JCI Insight (2019) 5(16):e128008. doi: 10.1172/jci.insight.128008
64. Noto CN, Hoft SG, DiPaolo RJ. Mast Cells as Important Regulators in Autoimmunity and Cancer Development. Front Cell Dev Biol (2021) 9:752350. doi: 10.3389/fcell.2021.752350
65. Fakhrjou A, Niroumand-Oscoei SM, Somi MH, Ghojazadeh M, Naghashi S, Samankan S, et al. Prognostic Value of Tumor-Infiltrating Mast Cells in Outcome of Patients With Esophagus Squamous Cell Carcinoma. J Gastrointest Cancer (2014) 45(1):48–53. doi: 10.1007/s12029-013-9550-2
66. Wang B, Li L, Liao Y, Li J, Yu X, Zhang Y, et al. Mast Cells Expressing Interleukin 17 in the Muscularis Propria Predict a Favorable Prognosis in Esophageal Squamous Cell Carcinoma. Cancer Immunol Immunother (2013) 62(10):1575–85. doi: 10.1007/s00262-013-1460-4
67. Xiao H, He M, Xie G, Liu Y, Zhao Y, Ye X, et al. The Release of Tryptase From Mast Cells Promote Tumor Cell Metastasis via Exosomes. BMC Cancer (2019) 19(1):1015. doi: 10.1186/s12885-019-6203-2
68. Ohashi Y, Ishibashi S, Suzuki T, Shineha R, Moriya T, Satomi S, et al. Significance of Tumor Associated Tissue Eosinophilia and Other Inflammatory Cell Infiltrate in Early Esophageal Squamous Cell Carcinoma. Anticancer Res (2000) ;20(5A):3025–30.
69. Grisaru-Tal S, Dulberg S, Beck L, Zhang C, Itan M, Hediyeh-Zadeh S, et al. Metastasis-Entrained Eosinophils Enhance Lymphocyte-Mediated Antitumor Immunity. Cancer Res (2021) 81(21):5555–71. doi: 10.1158/0008-5472.CAN-21-0839
70. Nicola S, Rolla G, Bucca C, Geronazzo G, Ridolfi I, Ferraris A, et al. Gastric Juice Expression of Th-17 and T-Reg Related Cytokines in Scleroderma Esophageal Involvement. Cells (2020) 9(9):2106. doi: 10.3390/cells9092106
71. Wang Y, Xu G, Wang J, Li XH, Sun P, Zhang W, et al. Relationship of Th17/Treg Cells and Radiation Pneumonia in Locally Advanced Esophageal Carcinoma. Anticancer Res (2017) 37(8):4643–7. doi: 10.21873/anticanres.11866
72. Chen D, Jiang R, Mao C, Shi L, Wang S, Yu L, et al. Chemokine/chemokine Receptor Interactions Contribute to the Accumulation of Th17 Cells in Patients With Esophageal Squamous Cell Carcinoma. Hum Immunol (2012) 73(11):1068–72. doi: 10.1016/j.humimm.2012.07.333
73. Chen D, Hu Q, Mao C, Jiao Z, Wang S, Yu L, et al. Increased IL-17-Producing CD4(+) T Cells in Patients With Esophageal Cancer. Cell Immunol (2012) 272(2):166–74. doi: 10.1016/j.cellimm.2011.10.015
74. Liu D, Zhang R, Wu J, Pu Y, Yin X, Cheng Y, et al. Interleukin-17A Promotes Esophageal Adenocarcinoma Cell Invasiveness Through ROS-Dependent, NF-κb-Mediated MMP-2/9 Activation. Oncol Rep (2017) 37(3):1779–85. doi: 10.3892/or.2017.5426
75. García-Varona A, Fernández-Vega I, Santos-Juanes J. Immunohistochemical Expression Analysis of MMP-1, TIMP-2 and P53 in Barrett's Esophagus, Dysplasia and Esophageal Adenocarcinoma. Pol J Pathol (2021) 72(1):48–56. doi: 10.5114/pjp.2021.106443
76. Chen N, Zhang G, Fu J, Wu Q. Matrix Metalloproteinase-14 (MMP-14) Downregulation Inhibits Esophageal Squamous Cell Carcinoma Cell Migration, Invasion, and Proliferation. Thorac Cancer (2020) 11(11):3168–74. doi: 10.1111/1759-7714.13636
77. Mao X, Xu J, Wang W, Liang C, Hua J, Liu J, et al. Crosstalk Between Cancer-Associated Fibroblasts and Immune Cells in the Tumor Microenvironment: New Findings and Future Perspectives. Mol Cancer (2021) 20(1):131. doi: 10.1186/s12943-021-01428-1
78. Peng L, Wang D, Han Y, Huang T, He X, Wang J, et al. Emerging Role of Cancer-Associated Fibroblasts-Derived Exosomes in Tumorigenesis. Front Immunol (2022) 12:795372. doi: 10.3389/fimmu.2021.795372
79. Kalluri R, Zeisberg M. Fibroblasts in Cancer. Nat Rev Cancer (2006) 6(5):392–401. doi: 10.1038/nrc1877
80. Wang J, Zhang G, Wang J, Wang L, Huang X, Cheng Y, et al. The Role of Cancer-Associated Fibroblasts in Esophageal Cancer. J Transl Med (2016) 14:30. doi: 10.1186/s12967-016-0788-x
81. Kato T, Noma K, Ohara T, Kashima H, Katsura Y, Sato H, et al. Cancer-Associated Fibroblasts Affect Intratumoral CD8+ and FoxP3+ T Cells Via IL6 in the Tumor Microenvironment. Clin Cancer Res (2018) 24(19):4820–33. doi: 10.1158/1078-0432.CCR-18-0205
82. Higashino N, Koma YI, Hosono M, Takase N, Okamoto M, Kodaira H, et al. Fibroblast Activation Protein-Positive Fibroblasts Promote Tumor Progression Through Secretion of CCL2 and Interleukin-6 in Esophageal Squamous Cell Carcinoma. Lab Invest (2019) 99(6):777–92. doi: 10.1038/s41374-018-0185-6
83. Shimizu M, Koma YI, Sakamoto H, Tsukamoto S, Kitamura Y, Urakami S, et al. Metallothionein 2a Expression in Cancer-Associated Fibroblasts and Cancer Cells Promotes Esophageal Squamous Cell Carcinoma Progression. Cancers (Basel) (2021) 13(18):4552. doi: 10.3390/cancers13184552
84. Du X, Xu Q, Pan D, Xu D, Niu B, Hong W, et al. HIC-5 in Cancer-Associated Fibroblasts Contributes to Esophageal Squamous Cell Carcinoma Progression. Cell Death Dis (2019) 10(12):873. doi: 10.1038/s41419-019-2114-z
85. Fang L, Che Y, Zhang C, Huang J, Lei Y, Lu Z, et al. PLAU Directs Conversion of Fibroblasts to Inflammatory Cancer-Associated Fibroblasts, Promoting Esophageal Squamous Cell Carcinoma Progression via uPAR/Akt/NF-κb/IL8 Pathway. Cell Death Discovery (2021) 7(1):32. doi: 10.1038/s41420-021-00410-6
86. Cai R, Wang P, Zhao X, Lu X, Deng R, Wang X, et al. LTBP1 Promotes Esophageal Squamous Cell Carcinoma Progression Through Epithelial-Mesenchymal Transition and Cancer-Associated Fibroblasts Transformation. J Transl Med (2020) 18(1):139. doi: 10.1186/s12967-020-02310-2
87. Grugan KD, Miller CG, Yao Y, Michaylira CZ, Ohashi S, Klein-Szanto AJ, et al. Fibroblast-Secreted Hepatocyte Growth Factor Plays a Functional Role in Esophageal Squamous Cell Carcinoma Invasion. Proc Natl Acad Sci U S A (2010) 107(24):11026–31. doi: 10.1073/pnas.0914295107
88. Stairs DB, Bayne LJ, Rhoades B, Vega ME, Waldron TJ, Kalabis J, et al. Deletion of P120-Catenin Results in a Tumor Microenvironment With Inflammation and Cancer That Establishes it as a Tumor Suppressor Gene. Cancer Cell (2011) 19(4):470–83. doi: 10.1016/j.ccr.2011.02.007
89. Chen Y, Li X, Yang H, Xia Y, Guo L, Wu X, et al. Expression of Basic Fibroblast Growth Factor, CD31, and α-Smooth Muscle Actin and Esophageal Cancer Recurrence After Definitive Chemoradiation. Tumor Biol (2014) 35(7):7275–82. doi: 10.1007/s13277-014-1987-9
90. Li F, Wu X, Sun Z, Cai P, Wu L, Li D, et al. Fibroblast Activation Protein-α Expressing Fibroblasts Promote Lymph Node Metastasis in Esophageal Squamous Cell Carcinoma. Onco Targets Ther (2020) 13:8141–8. doi: 10.2147/OTT.S257529
91. Labib PL, Goodchild G, Pereira SP. Molecular Pathogenesis of Cholangiocarcinoma. BMC Cancer (2019) 19(1):185. doi: 10.1186/s12885-019-5391-0
92. Xu Z, Wang S, Wu M, Zeng W, Wang X, Dong Z, et al. Tgfβ1 and HGF Protein Secretion by Esophageal Squamous Epithelial Cells and Stromal Fibroblasts in Oesophageal Carcinogenesis. Oncol Lett (2013) 6(2):401–6. doi: 10.3892/ol.2013.1409
93. Ozawa Y, Nakamura Y, Fujishima F, Felizola SJ, Takeda K, Okamoto H, et al. C-Met in Esophageal Squamous Cell Carcinoma: An Independent Prognostic Factor and Potential Therapeutic Target. BMC Cancer (2015) 15:451. doi: 10.1186/s12885-015-1450-3
94. Xiang H, Ramil CP, Hai J, Zhang C, Wang H, Watkins AA, et al. Cancer-Associated Fibroblasts Promote Immunosuppression by Inducing ROS-Generating Monocytic MDSCs in Lung Squamous Cell Carcinoma. Cancer Immunol Res (2020) 8(4):436–50. doi: 10.1158/2326-6066.CIR-19-0507
95. Dinh HQ, Pan F, Wang G, Huang QF, Olingy CE, Wu ZY, et al. Integrated Single-Cell Transcriptome Analysis Reveals Heterogeneity of Esophageal Squamous Cell Carcinoma Microenvironment. Nat Commun (2021) 12(1):7335. doi: 10.1038/s41467-021-27599-5
96. Zhang X, Peng L, Luo Y, Zhang S, Pu Y, Chen Y, et al. Dissecting Esophageal Squamous-Cell Carcinoma Ecosystem by Single-Cell Transcriptomic Analysis. Nat Commun (2021) 12(1):5291. doi: 10.1038/s41467-021-25539-x
97. Chen Z, Zhao M, Liang J, Hu Z, Huang Y, Li M, et al. Dissecting the Single-Cell Transcriptome Network Underlying Esophagus non-Malignant Tissues and Esophageal Squamous Cell Carcinoma. EBioMedicine (2021) 69:103459. doi: 10.1016/j.ebiom.2021.103459
98. Fares J, Fares MY, Khachfe HH, Salhab HA, Fares Y, et al. Molecular Principles of Metastasis: A Hallmark of Cancer Revisited. Signal Transduct Target Ther (2020) 5(1):28. doi: 10.1038/s41392-020-0134-x
99. Bhat AA, Nisar S, Maacha S, Carneiro-Lobo TC, Akhtar S, Siveen KS, et al. Cytokine-Chemokine Network Driven Metastasis in Esophageal Cancer; Promising Avenue for Targeted Therapy. Mol Cancer (2021) 20(1):2. doi: 10.1186/s12943-020-01294-3
100. Yao M, Brummer G, Acevedo D, Cheng N. Cytokine Regulation of Metastasis and Tumorigenicity. Adv Cancer Res (2016) 132:265–367. doi: 10.1016/bs.acr.2016.05.005
101. Wu X, Zhang H, Sui Z, Wang Y, Yu Z, et al. The Biological Role of the CXCL12/CXCR4 Axis in Esophageal Squamous Cell Carcinoma. Cancer Biol Med (2021) 18(2):401–10. doi: 10.20892/j.issn.2095-3941.2020.0140
102. Yang X, Lu Q, Xu Y, Liu C, Sun Q, et al. Clinicopathologic Significance of CXCR4 Expressions in Patients With Esophageal Squamous Cell Carcinoma. Pathol Res Pract (2020) 216(1):152787. doi: 10.1016/j.prp.2019.152787
103. Loh CY, Chai JY, Tang TF, Wong WF, Sethi G, Shanmugam MK, et al. The E-Cadherin and N-Cadherin Switch in Epithelial-To-Mesenchymal Transition: Signaling, Therapeutic Implications, and Challenges. Cells (2019) 8(10):1118. doi: 10.3390/cells8101118
104. Fedele M, Sgarra R, Battista S, Cerchia L, Manfioletti G. The Epithelial-Mesenchymal Transition at the Crossroads Between Metabolism and Tumor Progression. Int J Mol Sci (2022) 23(2):800. doi: 10.3390/ijms23020800
105. Wang X, Cao Y, Zhang S, Chen Z, Fan L, Shen X, et al. Stem Cell Autocrine CXCL12/CXCR4 Stimulates Invasion and Metastasis of Esophageal Cancer. Oncotarget (2017) 8(22):36149–60. doi: 10.18632/oncotarget.15254
106. Mortezaee K. CXCL12/CXCR4 Axis in the Microenvironment of Solid Tumors: A Critical Mediator of Metastasis. Life Sci (2020) 249:117534. doi: 10.1016/j.lfs.2020.117534
107. Zhang M, Zhou S, Zhang L, Ye W, Wen Q, Wang J, et al. Role of Cancer-Related Inflammation in Esophageal Cancer. Crit Rev Eukaryot Gene Expr (2013) 23(1):27–35. doi: 10.1615/CritRevEukarGeneExpr.2013006033
108. Cai QY, Liang GY, Zheng YF, Tan QY, Wang RW, Li K, et al. CCR7 Enhances the Angiogenic Capacity of Esophageal Squamous Carcinoma Cells In Vitro via Activation of the NF-κb/VEGF Signaling Pathway. Am J Transl Res (2017) 9(7):3282–92.
109. Wang Q, Zou H, Wang Y, Shang J, Yang L, Shen J, et al. CCR7-CCL21 Axis Promotes the Cervical Lymph Node Metastasis of Tongue Squamous Cell Carcinoma by Up-Regulating MUC1. J Craniomaxillofac Surg (2021) 49(7):562–9. doi: 10.1016/j.jcms.2021.02.027
110. Shi M, Chen D, Yang D, Liu XY. CCL21-CCR7 Promotes the Lymph Node Metastasis of Esophageal Squamous Cell Carcinoma by Up-Regulating MUC1. J Exp Clin Cancer Res (2015) 34:149. doi: 10.1186/s13046-015-0268-9
111. Song Y, Wang Z, Liu X, Liu X, Jiang W, Shi M., et al. CCR7 and VEGF-C: Molecular Indicator of Lymphatic Metastatic Recurrence in Pn0 Esophageal Squamous Cell Carcinoma After Ivor-Lewis Esophagectomy? Ann Surg Oncol (2012) 19(11):3606–12. doi: 10.1245/s10434-012-2419-y
112. Liu Q, Li A, Tian Y, Wu JD, Liu Y, Li T, et al. The CXCL8-CXCR1/2 Pathways in Cancer. Cytokine Growth Factor Rev (2016) 25(2):61–71. doi: 10.1016/j.cytogfr.2016.08.002
113. Waugh DJ, Wilson C. The Interleukin-8 Pathway in Cancer. Clin Cancer Res (2008) 14(21):6735–41. doi: 10.1158/1078-0432.CCR-07-4843
114. Mathieu M, Martin-Jaular L, Lavieu G, Théry C, et al. Specificities of Secretion and Uptake of Exosomes and Other Extracellular Vesicles for Cell-to-Cell Communication. Nat Cell Biol (2019) 21(1):9–17. doi: 10.1038/s41556-018-0250-9
115. Jing Z, Chen K, Gong L. The Significance of Exosomes in Pathogenesis, Diagnosis, and Treatment of Esophageal Cancer. Int J Nanomed (2021) 16:6115–27. doi: 10.2147/IJN.S321555
116. Liu T, Li P, Li J, Qi Q, Sun Z, Shi S, et al. Exosomal and Intracellular miR-320b Promotes Lymphatic Metastasis in Esophageal Squamous Cell Carcinoma. Mol Ther Oncol (2021) 23:163–80. doi: 10.1016/j.omto.2021.09.003
117. Liao J, Liu R, Shi YJ, Yin LH, Pu YP. Exosome-Shuttling microRNA-21 Promotes Cell Migration and Invasion-Targeting PDCD4 in Esophageal Cancer. Int J Oncol (2016) 48(6):2567–79. doi: 10.3892/ijo.2016.3453
118. Liu S, Lin Z, Rao W, Zheng J, Xie Q, Lin Y, et al. Upregulated Expression of Serum Exosomal Hsa_Circ_0026611 is Associated With Lymph Node Metastasis and Poor Prognosis of Esophageal Squamous Cell Carcinoma. J Cancer (2021) 12(3):918–26. doi: 10.7150/jca.50548
119. Xu ML, Liu TC, Dong FX, Meng LX, Ling AX, Liu S, et al. Exosomal lncRNA LINC01711 Facilitates Metastasis of Esophageal Squamous Cell Carcinoma via the miR-326/FSCN1 Axis. Aging (Albany NY) (2021) 13(15):19776–88. doi: 10.18632/aging.203389
120. Min H, Sun X, Yang X, Zhu H, Liu J, Wang Y, et al. Exosomes Derived From Irradiated Esophageal Carcinoma-Infiltrating T Cells Promote Metastasis by Inducing the Epithelial-Mesenchymal Transition in Esophageal Cancer Cells. Pathol Oncol Res (2018) 24(1):11–8. doi: 10.1007/s12253-016-0185-z
121. Kam NW, Wu KC, Dai W, Wang Y, Yan LYC, Shakya R, et al. Peritumoral B Cells Drive Proangiogenic Responses in HMGB1-Enriched Esophageal Squamous Cell Carcinoma. Angiogenesis (2021), 1–23.doi: 10.1007/s10456-021-09819-0
122. Donlon NE, Sheppard A, Davern M, O'Connell F, Phelan JJ, Power R, et al. Linking Circulating Serum Proteins With Clinical Outcomes in Esophageal Adenocarcinoma-An Emerging Role for Chemokines. Cancers (Basel) (2020) 12(11):3356. doi: 10.3390/cancers12113356
123. Yang W, Zhang Y, Yu J, Li S, et al. The Low Expression of CD80 Correlated With the Vascular Endothelial Growth Factor in Esophageal Cancer Tissue. Eur J Surg Oncol (2010) 36(5):501–6. doi: 10.1016/j.ejso.2010.01.007
124. Wang M, Li Y, Xiao Y, Yang M, Chen J, Jian Y, et al. Nicotine-Mediated OTUD3 Downregulation Inhibits VEGF-C mRNA Decay to Promote Lymphatic Metastasis of Human Esophageal Cancer. Nat Commun (2021) 12(1):7006. doi: 10.1038/s41467-021-27348-8
125. Li J, Xie Y, Wang X, Jiang C, Yuan X, Zhang A, et al. Overexpression of VEGF-C and MMP-9 Predicts Poor Prognosis in Kazakh Patients With Esophageal Squamous Cell Carcinoma. Peer J (2019) 7:e8182. doi: 10.7717/peerj.8182
126. Ma S, Lu CC, Yang LY, Wang JJ, Wang BS, Cai HQ, et al. ANXA2 Promotes Esophageal Cancer Progression by Activating MYC-HIF1A-VEGF Axis. J Exp Clin Cancer Res (2018) 37(1):183. doi: 10.1186/s13046-018-0851-y
127. Mohammadi F, Javid H, Afshari AR, Mashkani B, Hashemy SI. Substance P Accelerates the Progression of Human Esophageal Squamous Cell Carcinoma via MMP-2, MMP-9, VEGF-A, and VEGFR1 Overexpression. Mol Biol Rep (2020) 47(6):4263–72. doi: 10.1007/s11033-020-05532-1
128. Chen MF, Kuan FC, Yen TC, Lu MS, Lin PY, Chung YH, et al. IL-6-Stimulated CD11b+ CD14+ HLA-DR- Myeloid-Derived Suppressor Cells, are Associated With Progression and Poor Prognosis in Squamous Cell Carcinoma of the Esophagus. Oncotarget (2014) 5(18):8716–28. doi: 10.18632/oncotarget.2368
129. Li J, Zhang BZ, Qin YR, Bi J, Liu HB, Li Y, et al. CD68 and Interleukin 13, Prospective Immune Markers for Esophageal Squamous Cell Carcinoma Prognosis Prediction. Oncotarget (2016) 7(13):15525–38. doi: 10.18632/oncotarget.6900
130. Van Dyken SJ, Locksley RM. Interleukin-4- and Interleukin-13-Mediated Alternatively Activated Macrophages: Roles in Homeostasis and Disease. Annu Rev Immunol (2013) 31:317–43. doi: 10.1146/annurev-immunol-032712-095906
131. Karakasheva TA, Waldron TJ, Eruslanov E, Kim SB, Lee JS, O'Brien S, et al. CD38-Expressing Myeloid-Derived Suppressor Cells Promote Tumor Growth in a Murine Model of Esophageal Cancer. Cancer Res (2015) 75(19):4074–85. doi: 10.1158/0008-5472.CAN-14-3639
132. Paluskievicz CM, Cao X, Abdi R, Zheng P, Liu Y, Bromberg JS, et al. T Regulatory Cells and Priming the Suppressive Tumor Microenvironment. Front Immunol (2019) 10:2453. doi: 10.3389/fimmu.2019.02453
133. Kato H, Nakajima M, Masuda N, Faried A, Sohda M, Fukai Y, et al. Expression of RCAS1 in Esophageal Squamous Cell Carcinoma is Associated With a Poor Prognosis. J Surg Oncol (2005) 90(2):89–94. doi: 10.1002/jso.20249
134. Lu L, Pan K, Zheng HX, Li JJ, Qiu HJ, Zhao JJ, et al. IL-17A Promotes Immune Cell Recruitment in Human Esophageal Cancers and the Infiltrating Dendritic Cells Represent a Positive Prognostic Marker for Patient Survival. J Immunother (2013) 36(8):451–8. doi: 10.1097/CJI.0b013e3182a802cf
135. Yi M, Zheng X, Niu M, Zhu S, Ge H, Wu K, et al. Combination Strategies With PD-1/PD-L1 Blockade: Current Advances and Future Directions. Mol Cancer (2022) 21(1):28. doi: 10.1186/s12943-021-01489-2
136. Siddiqui AZ, Almhanna K. Beyond Chemotherapy, PD-1, and HER-2: Novel Targets for Gastric and Esophageal Cancer. Cancers (Basel) (2021) 13(17):4322. doi: 10.3390/cancers13174322
137. Hong Y, Ding ZY. PD-1 Inhibitors in the Advanced Esophageal Cancer. Front Pharmacol (2019) 10:1418. doi: 10.3389/fphar.2019.01418
138. Wang Y, Chen T, Li K, Mu W, Liu Z, Shi A, et al. Recent Advances in the Mechanism Research and Clinical Treatment of Anti-Angiogenesis in Biliary Tract Cancer. Front Oncol (2021) 11:777617. doi: 10.3389/fonc.2021.777617
139. Wang K, Chen Q, Liu N, Zhang J, Pan X, et al. Recent Advances in, and Challenges of, Anti-Angiogenesis Agents for Tumor Chemotherapy Based on Vascular Normalization. Drug Discovery Today (2021) 26(11):2743–53. doi: 10.1016/j.drudis.2021.07.024
140. Zhao S, Ren S, Jiang T, Zhu B, Li X, Zhao C, et al. Low-Dose Apatinib Optimizes Tumor Microenvironment and Potentiates Antitumor Effect of PD-1/PD-L1 Blockade in Lung Cancer. Cancer Immunol Res (2019) 7(4):630–43. doi: 10.1158/2326-6066.CIR-17-0640
141. Zou W, Wolchok JD, Chen L. PD-L1 (B7-H1) and PD-1 Pathway Blockade for Cancer Therapy: Mechanisms, Response Biomarkers, and Combinations. Sci Transl Med (2016) 8(328):328rv4. doi: 10.1126/scitranslmed.aad7118
142. Ohigashi Y, Sho M, Yamada Y, Tsurui Y, Hamada K, Ikeda N, et al. Clinical Significance of Programmed Death-1 Ligand-1 and Programmed Death-1 Ligand-2 Expression in Human Esophageal Cancer. Clin Cancer Res (2005) 11(8):2947–53. doi: 10.1158/1078-0432.CCR-04-1469
143. Chen L, Deng H, Lu M, Xu B, Wang Q, Jiang J, et al. B7-H1 Expression Associates With Tumor Invasion and Predicts Patient's Survival in Human Esophageal Cancer. Int J Clin Exp Pathol (2014) 7(9):6015–23.
144. Derks S, Nason KS, Liao X, Stachler MD, Liu KX, Liu JB, et al. Epithelial PD-L2 Expression Marks Barrett's Esophagus and Esophageal Adenocarcinoma. Cancer Immunol Res (2015) 3(10):1123–9. doi: 10.1158/2326-6066.CIR-15-0046
145. Zhang H, Dai Z, Wu W, Wang Z, Zhang N, Zhang L, et al. Regulatory Mechanisms of Immune Checkpoints PD-L1 and CTLA-4 in Cancer. J Exp Clin Cancer Res (2021) 40(1):184. doi: 10.1186/s13046-021-01987-7
146. Huang TX, Fu L. The Immune Landscape of Esophageal Cancer. Cancer Commun (Lond) (2019) 39(1):79. doi: 10.1186/s40880-019-0427-z
147. Ri MH, Ma J, Jin X. Development of Natural Products for Anti-PD-1/PD-L1 Immunotherapy Against Cancer. J Ethnopharmacol (2021) 281:114370. doi: 10.1016/j.jep.2021.114370
148. Zhulai G, Oleinik E. Targeting Regulatory T Cells in Anti-PD-1/PD-L1 Cancer Immunotherapy. Scand J Immunol (2022) 95(3):e13129. doi: 10.1111/sji.13129
149. Tashireva LA, Muravyova DT, Popova NO, Goldberg VE, Vtorushin SV, Perelmuter VM., et al. Parameters of Tumor Microenvironment Determine Effectiveness of Anti-PD-1/PD-L1 Therapy. Biochem (Mosc) (2021) 86(11):1461–8. doi: 10.1134/S0006297921110092
150. Mantovani A, Marchesi F, Malesci A, Laghi L, Allavena P., et al. Tumour-Associated Macrophages as Treatment Targets in Oncology. Nat Rev Clin Oncol (2017) 14(7):399–416. doi: 10.1038/nrclinonc.2016.217
151. Conte E. Targeting Monocytes/Macrophages in Fibrosis and Cancer Diseases: Therapeutic Approaches. Pharmacol Ther (2021) 108031. doi: 10.1016/j.pharmthera.2021.108031
152. Katsube R, Noma K, Ohara T, Nishiwaki N, Kobayashi T, Komoto S, et al. Fibroblast Activation Protein Targeted Near Infrared Photoimmunotherapy (NIR PIT) Overcomes Therapeutic Resistance in Human Esophageal Cancer. Sci Rep (2021) 11(1):1693. doi: 10.1038/s41598-021-81465-4
153. Cui X, Liu R, Duan L, Cao D, Zhang Q, Zhang A, et al. CAR-T Therapy: Prospects in Targeting Cancer Stem Cells. J Cell Mol Med (2021) 25(21):9891–904. doi: 10.1111/jcmm.16939
154. Shi H, Yu F, Mao Y, Ju Q, Wu Y, Bai W, et al. EphA2 Chimeric Antigen Receptor-Modified T Cells for the Immunotherapy of Esophageal Squamous Cell Carcinoma. J Thorac Dis (2018) 10(5):2779–88. doi: 10.21037/jtd.2018.04.91
155. Yu F, Wang X, Shi H, Jiang M, Xu J, Sun M, et al. Development of Chimeric Antigen Receptor-Modified T Cells for the Treatment of Esophageal Cancer. Tumori (2021) 107(4):341–52. doi: 10.1177/0300891620960223
156. Zhang H, Zhao H, He X, He X, Xi F, Liu J, et al. JAK-STAT Domain Enhanced MUC1-CAR-T Cells Induced Esophageal Cancer Elimination. Cancer Manag Res (2020) 12:9813–24. doi: 10.2147/CMAR.S264358
157. Shao J, Hou L, Liu J, Liu Y, Ning J, Zhao Q, et al. Indoleamine 2,3-Dioxygenase 1 Inhibitor-Loaded Nanosheets Enhance CAR-T Cell Function in Esophageal Squamous Cell Carcinoma. Front Immunol (2021) 12:661357. doi: 10.3389/fimmu.2021.661357
158. Yue G, Tang J, Zhang L, Niu H, Li H, Luo S, et al. CD276 Suppresses CAR-T Cell Function by Promoting Tumor Cell Glycolysis in Esophageal Squamous Cell Carcinoma. J Gastrointest Oncol (2021) 12(1):38–51. doi: 10.21037/jgo-21-50
Keywords: esophageal squamous cell carcinoma, tumor microenvironment, invasion, metastasis, immune regulation
Citation: Zheng S, Liu B and Guan X (2022) The Role of Tumor Microenvironment in Invasion and Metastasis of Esophageal Squamous Cell Carcinoma. Front. Oncol. 12:911285. doi: 10.3389/fonc.2022.911285
Received: 02 April 2022; Accepted: 18 May 2022;
Published: 22 June 2022.
Edited by:
Nathaniel Weygant, Fujian University of Traditional Chinese Medicine, ChinaReviewed by:
Zhong-Xi Huang, Southern Medical University, ChinaTing Zhuang, Xinxiang Medical University, China
Copyright © 2022 Zheng, Liu and Guan. This is an open-access article distributed under the terms of the Creative Commons Attribution License (CC BY). The use, distribution or reproduction in other forums is permitted, provided the original author(s) and the copyright owner(s) are credited and that the original publication in this journal is cited, in accordance with accepted academic practice. No use, distribution or reproduction is permitted which does not comply with these terms.
*Correspondence: Xinyuan Guan, eHlndWFuQGhrdS5oaw==