- 1Unidad de Tumores Sólidos Infantiles, Instituto de Investigación de Enfermedades Raras (IIER), Instituto de Salud Carlos III (ISCIII), Madrid, Spain
- 2Centro de Investigación Biomédica en Red de Enfermedades Raras, Instituto de Salud Carlos III (U758; CB06/07/1009; CIBERER-ISCIII), Madrid, Spain
- 3Universidad Nacional de Educación a Distancia (UNED), Madrid, Spain
It is widely accepted that the tumor microenvironment, particularly the extracellular matrix, plays an essential role in the development of tumors through the interaction with specific protein-membrane receptors. One of the most relevant proteins in this context is the transmembrane protein CD44. The role of CD44 in tumor progression, invasion, and metastasis has been well established in many cancers, although a comprehensive review concerning its role in sarcomas has not been published. CD44 is overexpressed in most sarcomas and several in vitro and in vivo experiments have shown a direct effect on tumor progression, dissemination, and drug resistance. Moreover, CD44 has been revealed as a useful marker for prognostic and diagnostic (CD44v6 isoform) in osteosarcoma. Besides, some innovative treatments such as HA-functionalized liposomes therapy have become an excellent CD44-mediated intracellular delivery system for osteosarcoma. Unfortunately, the reduced number of studies deciphering the prognostic/diagnostic value of CD44 in other sarcoma subgroups, neither than osteosarcoma, in addition to the low number of patients involved in those studies, have produced inconclusive results. In this review, we have gone through the information available on the role of CD44 in the development, maintenance, and progression of sarcomas, analyzing their implications at the prognostic, therapeutic, and mechanistic levels. Moreover, we illustrate how research involving the specific role of CD44 in the different sarcoma subgroups could suppose a chance to advance towards a more innovative perspective for novel therapies and future clinical trials.
1 Introduction
Sarcomas are a heterogeneous group of tumors originating from mesenchymal cells. These rare malignancies account for approximately 10% of childhood solid tumors arising in soft and bone tissues (1), and 2% of cancers diagnosed in adult individuals (representing an overall annual incidence of approximately six adult cases per 100,000 people in Europe) (2, 3). Sarcomas are mostly detected in the extremities (approximately 60%) and the trunk (around 18%) (4), but they can arise from any part of the body (retroperitoneum-12%, head and neck-9%, and mediastinum-1%). Metastases, when detected, are located predominantly in the lung, although are rarely present at the time of diagnosis (around 10% of the cases) (5–7).
According to the World Health Organization (WHO), there are more than 100 histological subtypes of sarcomas with different clinical characteristics (8). This classification considers the histology but also key genetic alterations, which are found within specific sarcomas. During the last decades, our understanding of the molecular mechanisms that underlie most of the sarcomas has been largely determined, contributing to improving diagnosis and treatment. The genetic alterations more frequently detected in sarcomas are: tyrosine-kinase activating mutations (9), gene fusions of growth factors or kinases (e.g., involving ALK, ROS1 or NTRK family) (10–12), gene fusions involving transcriptions factors (e.g., EWSR1-FLI1, PAX3-FOXO1) (13, 14), inactivation of tumor suppressor genes (e.g., NF1, PTEN or TP53) (15–17), gene amplification (e.g. MDM2 and MDM2/CDK4 co-amplification) (18) and epigenetic dysregulation (19, 20).
In addition to genetic alterations, other factors are also involved in the initiation, maintenance, and progression of cancer cells (21). Thereby, it is now widely accepted that the tumor microenvironment plays an essential role in tumor maintenance and progression. Particularly, the extracellular matrix (ECM) regulates many aspects related to the processes of cancer cell invasion, cancer cell dissemination, and the establishment of distant foci of metastasis. The components of the ECM interact with specific protein membrane receptors activating signaling pathways involved in migration, epithelial-mesenchymal transition (EMT), mesenchymal-epithelial transition (MET), and stemness (22). Collagens, proteoglycans, laminins, fibronectins, glycosaminoglycans, as the hyaluronic acid, or matricellular proteins, as periostin, are extracellular components present in the ECM. All of them are able to interact with specific receptors modulating the malignant phenotype, the metastatic processes, or the resistance to drugs (23, 24). One of the more relevant actors in this scenario is the transmembrane protein CD44, which interacts with many ECM components, triggering multiple signaling cascades within tumor cells. The role of CD44 in tumor progression and particularly in the processes of invasion and metastasis has been well established in many cancers mainly of epithelial origin, which have been the subject of excellent reviews (25–27). However, as far as we know, no comprehensive review compiling the data concerning the role of CD44 in sarcomas has been published. In this review, we have gone through the information available on the role of CD44 in the development, maintenance, and progression of sarcomas and analyzed their implications at the prognostic, therapeutic, and mechanistic levels.
2 CD44: Structure, Ligands, and Signaling
2.1 CD44 Structure
The gene encoding human CD44 protein is located on the short arm of chromosome 11 and it is composed of 18 exons (HGNC:1681). Several exons (exons 6-14) undergo alternative splicing generating different isoforms (CD44v2-v10) (Figure 1) (25, 28). The isoform CD44v1 is not present in humans due to the absence of one exon in comparison to the CD44 gene in mice, which is composed of 19 exons instead 18 (28). The CD44 standard isoform, called CD44s (29), is composed of exons 1-5 (N-terminal and ligand binding domain) and exons 15-18 (C-terminal domain), thus excluding all the alternatively spliced exons. There are three differentiated regions in this complex cell-surface glycoprotein (Figure 2): i) the ectodomain, constituted by exons 1-16. It includes the alternatively spliced regions and thus their length is variable. This domain interacts with numerous extracellular ligands; ii) the transmembrane domain (TM, exon 17) and iii) the intracellular domain (ICD, exon 18) which interacts with kinases and other signaling molecules (27, 30) (UniProt accession number: P16070).
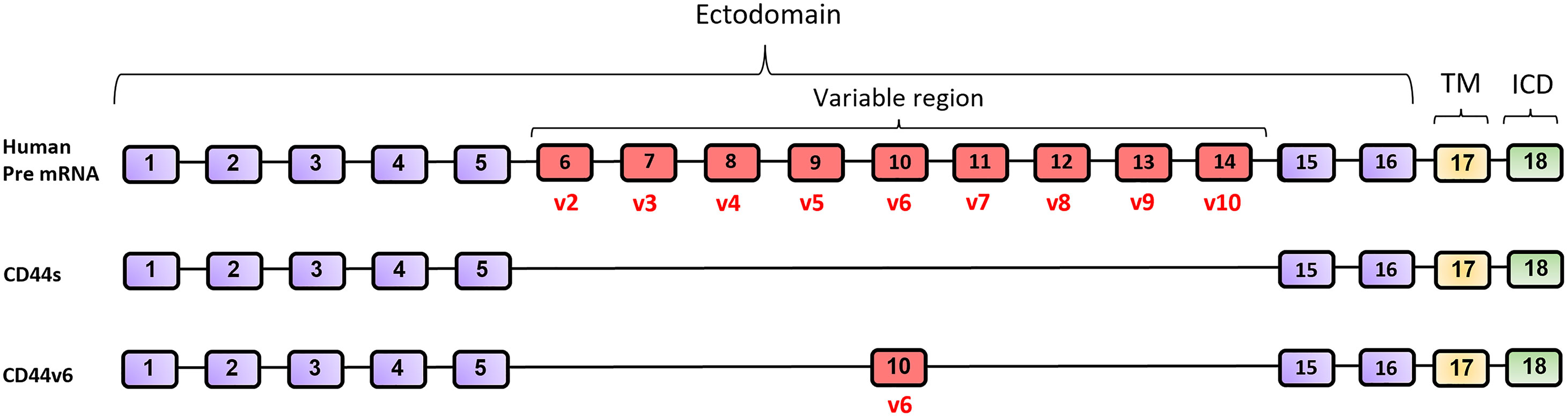
Figure 1 Diagram of full-length CD44 pre-mRNA, CD44s and CD44v6 mRNA in human. CD44 is encoded by 18 exons (ENSEMBL accession number #ENSG00000026508. https://www.ensembl.org/). CD44 isoform 1 (CD44v1) is not present in humans. Exons marked in purple color are always expressed in the ectodomain of all CD44 isoforms. Up to nine exon variants can be inserted by alternative splicing to compose the variable region (Exons 6-14 in red). Exon 17 (yellow) codes for the transmembrane domain (TM) and exon 18 (green) codes for the intracellular domain (ICD).
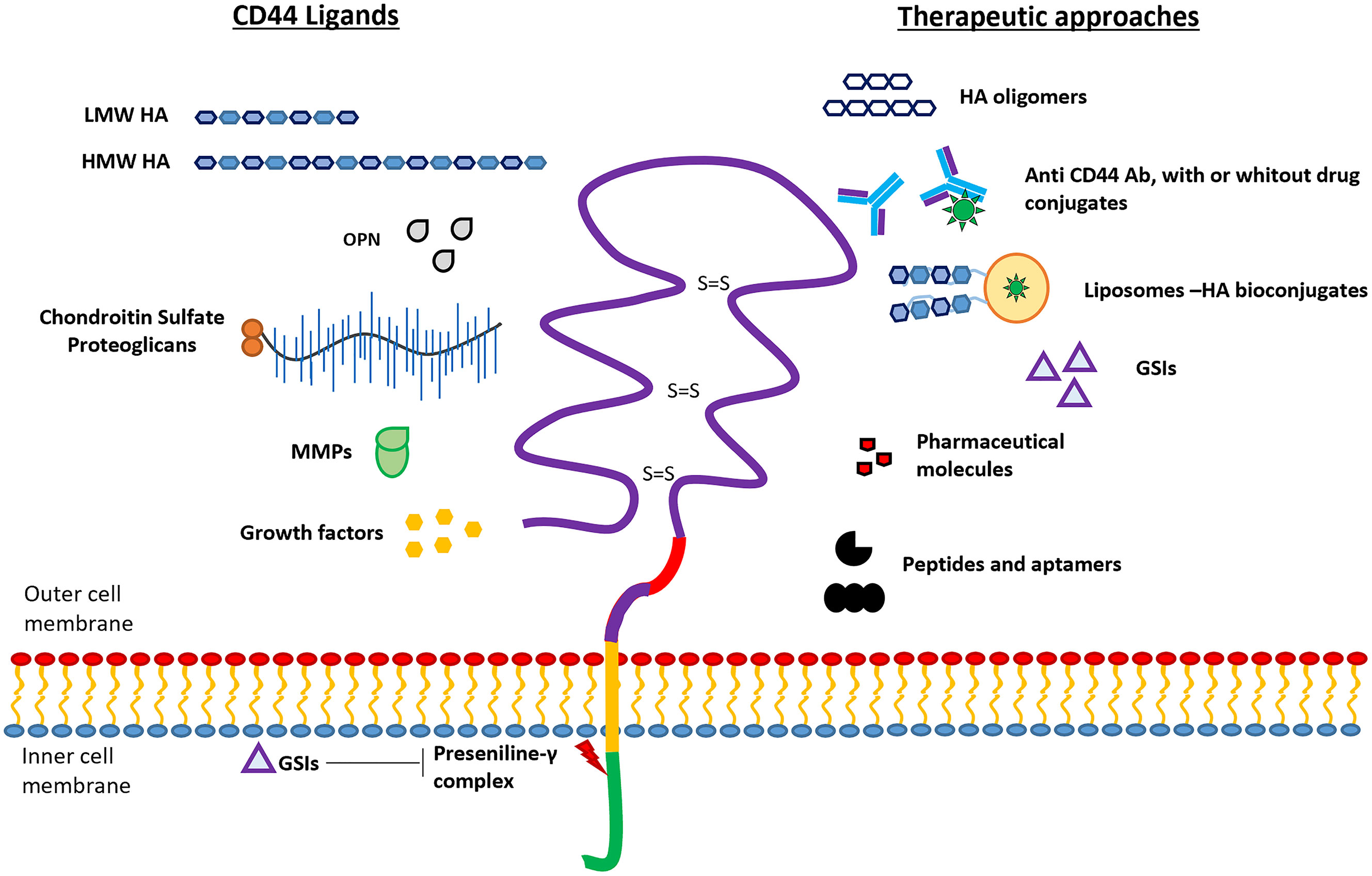
Figure 2 Schematic representation of CD44 protein structure. The four domains of CD44 glycoprotein are represented with different colors: ectodomain (purple), variable domain (red), transmembrane domain (yellow), and intracellular domain (green). Ligands interacting with the ectodomain (left), and the different therapeutic approaches (right) are schematically represented. Presenilin‐γ complex promotes the ICD cleavage which can be blocked by using γ-secretase inhibitors (GSIs).
2.2 CD44 Ligands
The extracellular domain of CD44 has been shown to interact with several ligands as for example hyaluronic acid (HA), osteopontin (OPN), serglycin/sulfated proteoglycans, fibronectin, collagen, and matrix metalloproteases (MMPs) (Figure 2). The binding of these ligands to CD44 triggers diverse cellular signaling cascades, some of which have been studied in sarcomas (see below) (25, 27, 31–37). The bioavailability of these biomolecules at the tumor or metastatic sites can modulate cancer progression through the activation of specific pathways. In this section, we analyzed briefly the effects triggered by CD44-ligand interactions.
2.2.1 Hyaluronic Acid (HA)
HA is a primary ECM constituent (commonly found in connective tissue and bone marrow) and the major ligand for CD44 (36). HA is a linear polymer of disaccharide units of β-1,3-N-acetyl glucosamine and β-1,4-glucuronic acid that is synthesized by the hyaluronan synthase proteins (HAS1-3) (38). The CD44/HA binding region resides in the N-terminal domain, located in residues 21-178, and is stabilized by three cysteine disulfide cross-links (39) (Figure 2). Interestingly, the molecular weight of HA, which can vary between dozens (≈5 KDa) and thousands of units (2,000 KDa), affects the binding of HA to CD44 and consequently to the effects that it causes in tumor cells (35). High molecular weight HA (HMW-HA, >500kDa) causes cell cycle arrest and reduces proliferation, neo-angiogenesis, and inflammation (35, 40), while low molecular weight HA (LMW-HA, 1-500 kDa) induces proliferation, ECM degradation processes (mediated by metalloproteases) and cell migration and invasion (35, 41). Ras, MAPK, PI3K, Nanog‐Stat3, Oct4‐Sox2‐Nanog, c-Met or c‐Src kinase signaling are some of the pathways directly modulated through CD44-HA binding (42–45). Merlin-ERM (ezrin-radixin-moesin) family of proteins, acts as a tumor suppressor by inhibiting the CD44-HA signaling (46).
2.2.2 Osteopontin (OPN)
OPN is an acidic phosphoprotein mainly secreted in plasma by macrophages and activated T cells and into the bone matrix by osteoblasts, among other cell types. This protein has been shown to be of predictive value in several types of cancers (47) and to be specifically upregulated in primary cultures from giant cell tumors of bone and desmoplastic fibroma samples (48). The CD44 residues implied in OPN binding involve the region containing amino acids 121 to 140 (47). The CD44/OPN interaction (32) supports tumor progression through the activation of the phosphatidylinositol 3-kinase/Akt signaling pathway (49), increasing consequently cell survival. Several cells, such as MDA-MB-435 or LCC15-MB among other breast cancer cell lines produce increased levels of this protein (50), which provide them with a growth advantage. HIF‐2α (Hypoxia-Inducible Factor 2α) expression is also regulated by CD44‐OPN interaction through a CBP/p300‐dependent mechanism (mediated by CD44-ICD) and it has been demonstrated to promote stem-like properties and tumoral aggressiveness in glioma cells (51).
2.2.3 Proteoglycans
Several proteoglycans are being shown to interact with CD44. For example, serglycin a proteoglycan characterized by repeats of Serine-Glycine dipeptide, is primarily expressed in hematopoietic tissue (52). However, this proteoglycan can be secreted by many cancer cells (e.g., nasopharyngeal carcinoma cells, myeloma cells, non-small cell lung cancers) (53, 54). CD44-Serglycin interaction maintains tumoral cell stemness through CD44 upregulation (as part of a positive feedback loop) mediated by MAPK/β-catenin pathway activation in nasopharyngeal carcinoma cells (55). Another proteoglycan, the versican, which is secreted by tumor stromal fibroblasts and cancer cells, binds to HA and generates HA/versican aggregations through its N-terminal region that contains a G1 domain (56), composed of an immunoglobulin-like domain (57, 58). The macromolecular complexes CD44/HA/versican promoted the invasion events in ovarian cancer cells (59). Another proteoglycan, aggrecan, a major component of cartilage, interacts with CD44, mediates cell adhesion, and induces CD44 oligomerization, which may lead to the triggering of the phosphorylation of Src kinases, activation of Rho-like GTPases or NF-κB activation cascades (60).
2.2.4 Fibronectin and Collagen
Fibronectin and collagens are abundantly present in the ECM and are mostly found in connective tissues. The interaction of fibronectin with CD44 facilitates the extravasation of cells and the adhesion processes, allowing cancer cells to adhere more efficiently to ECM within a metastatic microenvironment (61). On the other hand, collagens are the main components of the ECM which must be degraded so that tumor cells can colonize other tissues and organs. CD44 can upregulate serine protease and collagen-degrading enzymatic expression and its activity to achieve it (62) (see the paragraph below matrix metalloproteinases). Additionally, CD44 and type IV collagen interactions have been involved to participate in cell adhesion processes in colorectal carcinoma cell lines (KM-12c, CCL 188, and MIP-101) (63).
2.2.5 Matrix Metalloproteinases (MMPs)
MMPs are a group of calcium-dependent zinc-containing endopeptidases, which are responsible for tissue remodeling and degradation of ECM components, including collagens, elastins, gelatin, matrix glycoproteins, and proteoglycans (64). Malignant cells produce high levels of these proteins, which allow them to degrade ECM components faster than normal cells (65). The tumor invasiveness promoted by MMP-9 seems to be mediated by the binding of the proteolytically active metalloprotease to the CD44 ectodomain (66). Alternatively, the migration in rounded-amoeboid cells is supported through a non-catalytic mechanism based on actomyosin contractility modifications via CD44-MMP9 interaction (65). The metalloproteinase MT1-MMP, also known as MMP14, colocalizes with CD44 forming a complex through the hemopexin-like (PEX) domain in the MT1-MMP and the CD44-ICD, whose interaction is indispensable for degrading the extracellular matrix barrier during cancer invasion and is also involved in the CD44 ectodomain cleavage (see below) (67, 68).
2.3 Signaling Pathways Activated Through CD44
CD44-dependent signalling pathway activation takes place through two different mechanisms: i) the interaction between adaptor molecules and the intracellular domain (CD44-ICD) (28, 37) and ii) through the direct translocation of the CD44-ICD to the nucleus (37, 69, 70).
i) The CD44-ICD domain lacks kinase activity but it can interact with adaptor molecules like ankyrin and ERM (ezrin-radixin-moesin protein family) (Figure 3), which link CD44 to the actin‐cytoskeleton network. These interactions trigger changes in the tumor cell cytoskeletal architecture and cell signaling (e.g., c-Met activation, Ras-MAPK cascade, Snail/β-catenin translocation, or PI3K-AKT pathway activation), playing a role in the regulation of epithelial‐mesenchymal transition mechanisms (EMT) and the activation of angiogenesis, proliferation, and invasion mechanisms (70, 71).
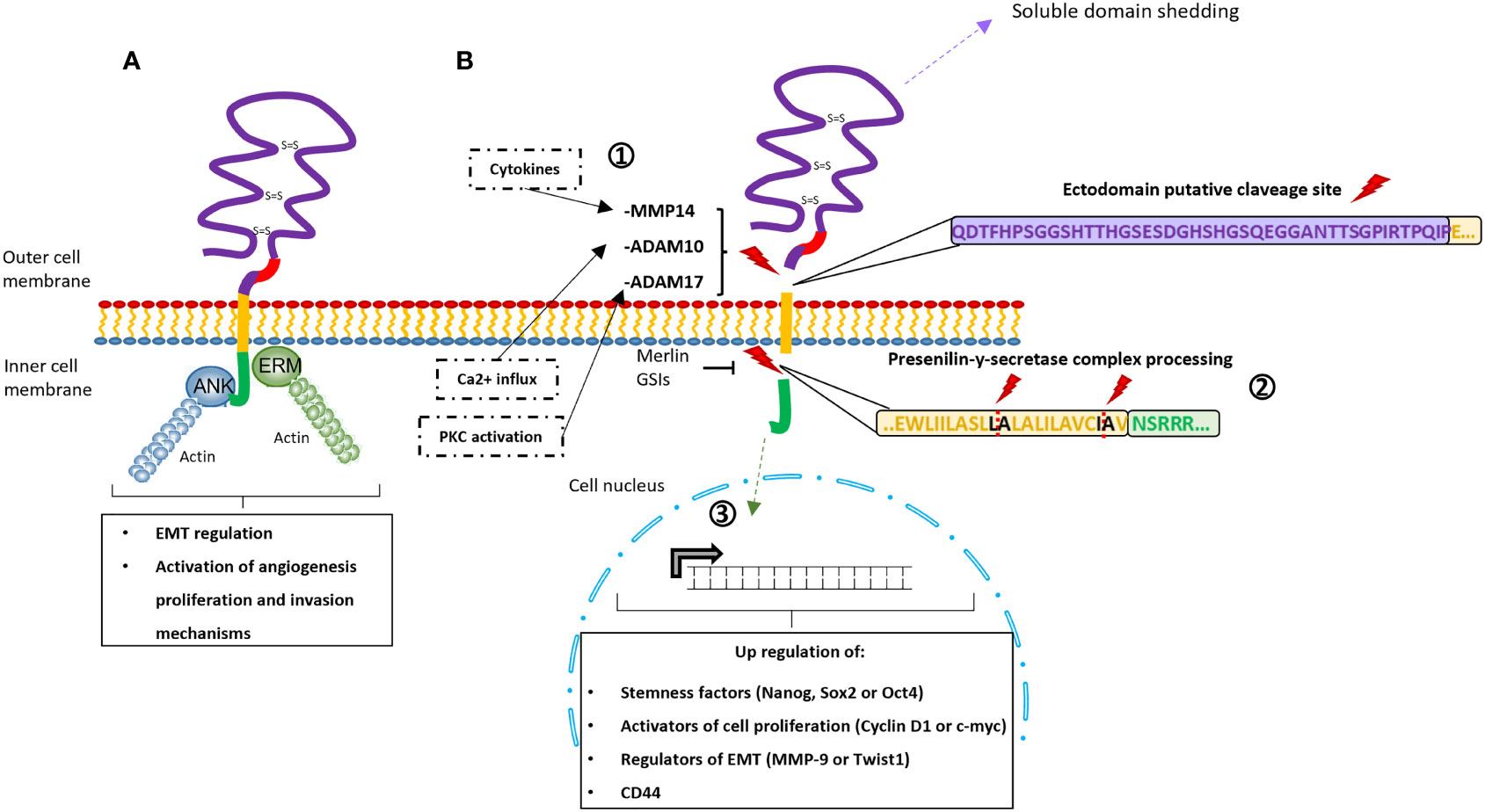
Figure 3 Schematic representation of the signaling pathways activated through CD44. (A) Interaction between adaptor molecules Ankyrin (blue) and Ezrin-Radixin-Moesin (green) with the intracellular domain CD44-ICD. This interaction triggers modifications in the cytoskeletal disposition and the activation of specific pathways (e.g., c-Met activation, Ras-MAPK cascade, Snail/β-catenin translocation, or PI3K-AKT pathway activation). As consequence, this via is involved in epithelial‐mesenchymal transition mechanisms (EMT) and the activation of angiogenesis, proliferation, and invasion mechanisms. (B) Schematic representations of CD44-ICD signaling via, including the sequential proteolytic cleavage of the CD44 protein. 1) The ectodomain shedding is induced by PKC, Ca2+ influx or cytokines that activate the MMPs (ADAM17, ADAM10 and MMP14, respectively). 2) The presenilin-y-secretase complex is activated and processes the ICD through the specific cleavage sites in the transmembrane domain (residues L-A and residues I-A). GSIs (γ-secretase inhibitors) and the merlin protein can inhibit this second proteolytic processing. 3) The ICD is released and translocated to the nucleus upregulating stemness factors, activators of cell proliferation, epithelial‐mesenchymal transition mechanisms (EMT) regulators and CD44 itself.
ii) On the other hand, CD44-ICD can translocate to the cell nucleus thanks to the transportin1-specific nuclear localization signal (residues DRKPS) present at its N-terminal region and there (Figure 3) (71, 72). At the nucleus, CD44-ICD regulates the expression of some genes through the formation of different complexes with CBP/p300, Runx2, or Stat3 (42, 70, 73–76). Among the genes that have been shown to be regulated by CD44-ICD are some stemness factors (e.g., Nanog, Sox2, or Oct4), activators of cell proliferation (e.g. Cyclin D1 or c‐myc), regulators of EMT (e.g., MMP‐9 or Twist1) or CD44 itself (51, 69, 70, 73, 74, 76, 77).
The translocation of CD44-ICD to the nucleus is preceded by a sequential cleavage (Figure 3) of the CD44 protein (69). The ectodomain cleavage produced between the variable region and the transmembrane domain could be activated by three different pathways; i) the protein kinase C (PKC) which activates the ADAM Metallopeptidase Domain 17 (ADAM17); ii) the calcium influx which activates the ADAM10; iii) the interleukin 1, TGFβ1, or interferon γ which induces the expression of MT1-MMP (MMP14) activating proMMP-2 (78, 79), and promoting cell migration and invasiveness (80–82). Subsequently, a mechanism called regulated intramembrane proteolysis (RIP), which is driven by the presenilin‐γ‐secretases is activated. The presenilin‐γ‐secretase complex, constituted by presenilin 1 and presenilin 2 γ‐secretases, recognizes the proteolytic cleavage sites at the CD44 transmembrane domain (residues Ala278-Leu279 and Ile287-Ala288) and detaches the intracellular domain from the rest of the protein (83), releasing the CD44-ICD domain to the cytoplasm (70, 73, 84). Interestingly, this sequential proteolytic cleavage can be promoted by CD44-ligand interactions (26, 35, 79, 83, 85), while the CD44-ICD releasing can be inhibited by γ-secretase inhibitors (GSI) (69, 77) or by merlin protein. Merlin recognizes the same motif recognized by ERM (71) and can provide a tumor suppressor state by blocking the cleavage site (46).
3 MSCs, CSCs, AND DIFFERENTIATED TISSUES: CD44 EXPRESSION AND INFLUENCE DURING CELL DIFFERENTIATION AND SARCOMAGENESIS
Sarcomas are thought to arise from mesenchymal stem cells (MSCs) or derived progenitors, from which normal differentiated cells are also produced (for example adipo-, chondro-, myogenic, and osteocytic cells) (86). Therefore, the study of the similarities and differences among different grades of cell differentiation, that is, mesenchymal stem cells, sarcoma cells, and normal differentiated cells, is important to understand the role of CD44 in sarcomas.
3.1 CD44 Expression in MSCs, Sarcoma (CSCs), and Differentiated Tissues
MSCs presents different surface markers including STRO-1, CD166, CD146, CD106, CD105, CD90, CD73, CD54, CD44, CD34, CD29 and CD13 (87, 88). CD44 shows high expression in MSCs as well as in sarcomas (89–92). Interestingly, CD44 has been shown to be expressed in the subpopulation of cancer stem cells (CSCs), which can reconstitute the tumor mass. CSCs and MSCs share similar stem-like properties and features. These similitudes among the characteristics of MSC (stemness, healing, regeneration processes, and cell recruitment) and cancer stem cells CSCs (potential for tumor initiation, EMT, tumoral progression, and stemness) with increased CD44 expression have already been broadly described in different cancers (28, 70, 93–97). Concordantly, CD44 is expressed at high levels in most sarcomas (Figure 4). The available data from the public database (DEPMAP Portal, https://depmap.org/portal/) confirms that CD44 expression was elevated in all sarcoma cell lines available in the dataset, with the unique notable exception of Ewing sarcoma, which expresses low levels of CD44 in comparison to the rest of sarcoma cell lines. Controversially, Skubitz et al., performed a CSCs characterization of stem cell markers in 31 soft tissue sarcoma from patients and determined that neither CD44, ALDH1, or CD133 were useful for sarcoma (CSCs) identification in these samples (98).
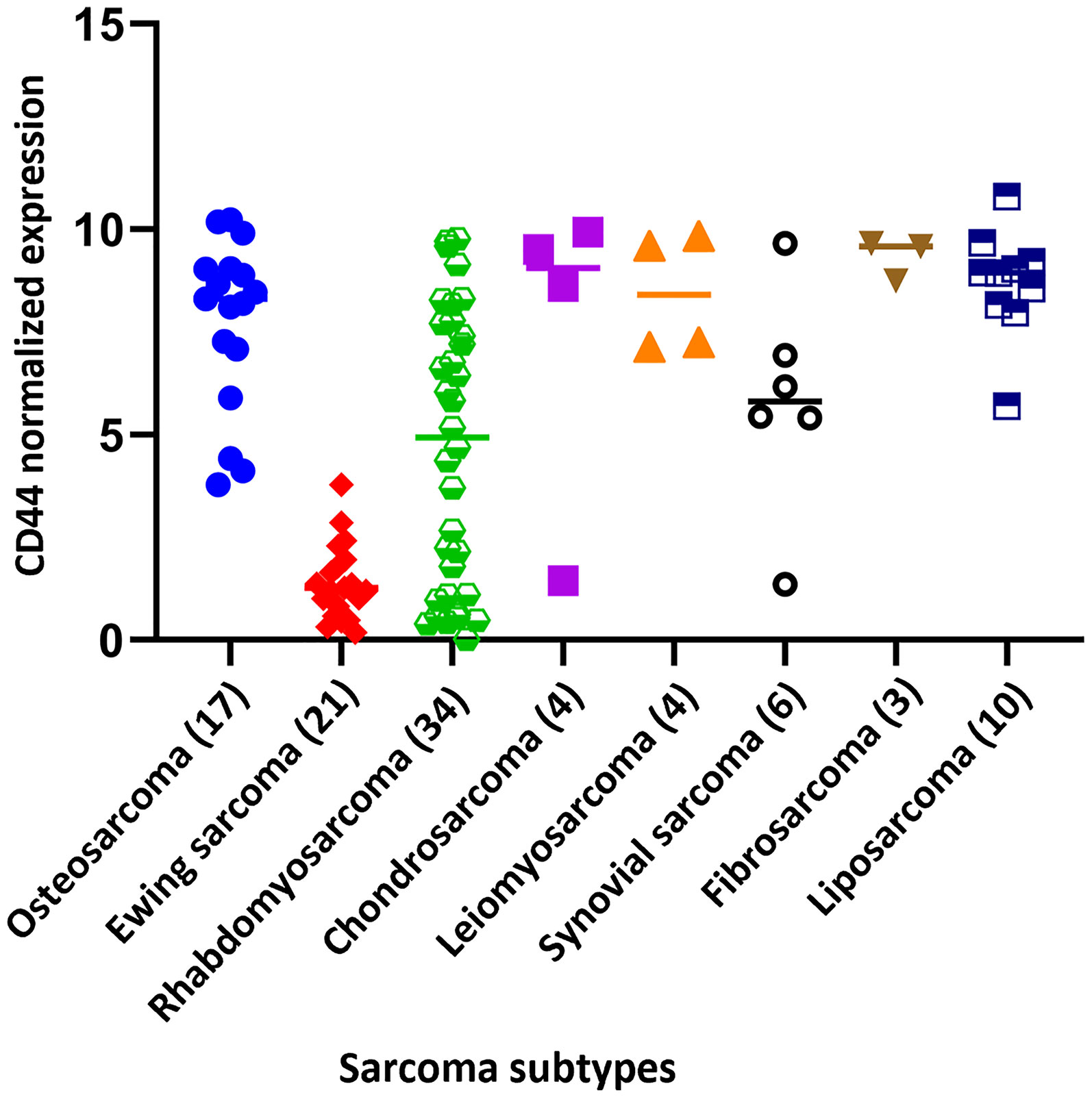
Figure 4 CD44 normalized expression of the different cell line sarcoma subtypes. Data extracted from DEPMAP Portal (https://depmap.org/portal/). The number of cell lines in each sarcoma subtype is indicated in brackets.
On the other hand, the CD44 protein is detected in the most differentiated tissues according to the Human Protein Atlas database (https://www.proteinatlas.org/). In consequence, CD44 shows a reduced tissue specificity due to its ubiquitous nature. Despite its ubiquity, CD44 is predominantly found in epithelial cells, bone marrow, and lymphoid tissue. Mesenchymal-derived tissues such as chondrocytes and bone cells have been demonstrated to express CD44 and modulate its expression in specific biological processes (cell-cell and/or cell-matrix attachment, morphological changes observed from osteoblasts to osteocytes, osteoclastogenesis, regulatory function in chondrocytes) (99–101). Additionally, CD44 seems to be involved in the processes of MSCs differentiation (101–105). Interestingly, CD44 expression was suggested to be reduced during the process of differentiation from MSCs to differentiated mature cells (103, 106). Thus, Huynh et al., monitored the process of chondrogenic differentiation from MSCs derived from bone marrow. They analysed the changes in the mRNA profile during differentiation and described several MSC markers, such as CD44, ENG, NT5E, or THY1, that progressively decreased during the differentiation process, while well-established chondrogenic markers (e.g., COL2A1, ACAN, COL9A1, COL11A1, COMP) were up regulated as the process of cell differentiation progressed (106). Moroes et al., studied the effect of CD90 and CD44 on differentiation processes induced in mesenchymal stem cells. The silencing of CD90 decreased the expression of CD44 which promoted the osteogenic and adipogenic differentiation of MSCs in vitro, thus associating the expression of high levels of CD44 and CD90 to the stemness state of MSCs (103, 107). Alternatively, the impairments in maturation processes involving high levels of CD44 were tested in neural differentiation experiments from MSCs and astrocytes precursor cells. Increased expression of CD44 inhibited oligodendrocytes differentiation and arrested the cells at the glial progenitor state. The in vivo research performed in a CD44 misexpression transgenic mouse model (CNP-CD44 mouse), supported that the CD44 overexpression blocked the oligodendrocyte maturation (108, 109). Complementary, Naruse et al., demonstrated that despite the widespread expression of CD44 in progenitor cells at early embryonic stages, the CD44 expression in adults was restricted to a specific mature subtype of cells (granule neurons). Thus, supporting the role of CD44 in the maintenance of stemness properties, but being able to promote differentiation of specific subtypes of neurons and astrocytes (110).
In summary, there is a relatively high expression level of CD44 in MSCs and sarcomas according to different studies and publicly available datasets. The differences in CD44 expression among the stem-like cells (MSCs and CSCs/sarcomas) and differentiated tissues suggest a down-regulation event of CD44 upon differentiation into mature cells, acquiring a new basal level of CD44, corresponding to its novel biological functions, which is susceptible of up/down-regulation.
3.2 CD44 and Tumor Microenvironment
The mesenchymal cell microenvironment has been demonstrated to be crucial for the development of multiple functions of mesenchymal stem cells (MSCs). This microenvironment provides components for cell regeneration and self‐renewal potential maintenance (111, 112). For instance, abundant quantities of HA are produced by MSCs to maintain their stemness (70). Injure healing processes and tissue regeneration are typical MSCs-driven processes, for which MSCs recruitment is mandatory, and CD44s-HA interaction seems to be a determinant mechanism to promote cell migration (113). Therefore, CD44 and the microenvironment play an important role in the processes performed by these cells and as consequence in their derived tumors.
Functionally, the high expression of CD44 benefits CSCs in several situations. CD44 works as a co-receptor for growth factors (CD44v3 co-receipts FGF and VEFG, while CD44v6 co-receipts EGF and HGF) and cytokines in the tumoral niche, potentiating receptor tyrosine kinase or CD44‐mediated signalling (37, 114) and triggering TGF-β up-regulation and the induction of Snail and Twist (115, 116). These two genes are considered key regulators of EMT and cancer progression (117–119). Snail has also been associated with cell cycle regulation, apoptosis evasion, cell adhesion, neuroendocrine differentiation, and chemoresistance (118–120). Twist is thought to promote metastasis, although the mechanisms implied are poorly understood (121, 122). Additionally, CD44-regulated transcription factors related to stem cell expression programs (Sox2, Klf4, Oct4, and Nanog), are commonly identified in MSCs and tumors (115), when CD44 has been knocked-down the expression of these markers (Oct4, Nanog, and Sox2) are notably reduced (123). Consequently, the transcriptional networks mediated by these factors are crucial for stemness properties observed during tissue development (70, 124, 125).
The hypoxic low-ROS (reactive oxygen species) environment is also associated with proliferation and the maintenance of self‐renewal capacities in cancer stem cells (CSCs) and solid tumors (126, 127). In this situation, CD44 has a specific function. HIF‐1α induced by hypoxia promotes glycolytic metabolism, angiogenesis (128), and CD44, CD44v6/v7/v8 expression (129). CD44 regulates ROS-mediated cytotoxicity in tumors through two different mechanisms (70). The interaction of CD44‐ICD-PKM2 (pyruvate kinase isoenzyme type M2) increases PKM2 phosphorylation (suppressing its activity) which promotes the activation of glycolytic metabolism, driving the CSCs to a pro-antioxidant status (130). Low PKM2 activity increases the levels of reduced NADPH which favors the regeneration of reduced glutathione GSH (131, 132). Alternatively, the interaction CD44-XCT (subunit of the cystine‐glutamate transporter Xc), promotes cystine uptake for the synthesis of GSH (133). As consequence, oxidative stress is controlled and reduced, providing a favorable environment for CSCs. The relevance of this mechanism has supposed its targeting through xCT inhibitors, like the sulfasalazine. This molecule has been tested in a dose-escalation clinical study with advanced gastric cancer patients, showing the inhibition of the proliferation in CD44v-positive cancer cells, both in vitro and in vivo (134).
4 Functional Characterization of CD44 in Sarcoma Cell Lines
Most of the studies carried out to understand the functionality of CD44 in cancer have been performed in epithelial tumors, although some relevant works focused specifically on sarcomas.
4.1 Chondrosarcoma
Some studies performed in chondrosarcoma cell lines have contributed to understanding the role of CD44 in these tumors and clarifying the signal transduction cascade triggered upon CD44 stimulation. Suzuki et al., reported that LMW-HA (molecular weight 3.5 kDa) can stimulate CD44 and subsequently, increase c-Met expression and c-Met phosphorylation in the chondrosarcoma cell line HCS-2/8 (45). In a similar study, using the same chondrosarcoma cell line, Kobayashi et al., demonstrated that the mRNA and protein of the urokinase-type plasminogen activator (uPA) and theurokinase-type plasminogen activator receptor (uPAR) were upregulated after CD44 stimulation with LMW-HA. Moreover, it was shown that CD44 stimulation with LMW-HA induced MAPK pathway activation, via phosphorylation of MEK1/2, ERK1/2, and c-Jun. Additionally, the signalling cascade derived from the interaction of HA with CD44 could be disrupted at different points; by using anti-CD44 antibodies, MAP kinase inhibitors, neutralizing anti-uPAR pAb, anti-catalytic anti-uPA mAb, or amiloride (135). These results provide a novel therapeutical alternative and suggest that there could exist an autocrine loop; CD44 stimulation - MAP kinase cascade activation (including c-Met) - uPA/uPAR overexpression, in chondrosarcoma cells that can boost their invasiveness.
4.2 Osteosarcoma
Gene silencing and CRISPR/Cas9-mediated knockout have been used to elucidate the role of CD44 in osteosarcoma pathogenesis, particularly in relation to resistance to treatment and metastasis formation. Knocking-out of CD44 using CRISPR/Cas9 system in the drug-resistant osteosarcoma cell lines KHOSR2 and U-2OSR2 (both resistant to Doxorubicin) showed significant inhibition of migration, invasion, proliferation, and resistance to doxorubicin (136). In another study, CRISPR/Cas9 was also used to knocking-out CD44 in the human osteosarcoma cell lines MNNG/HOS and 143B, both highly metastatic. This study showed the inhibition of cell proliferation and tumor-sphere formation in 3D cultures upon CD44 inactivation (137). CD44 inactivation, through a siRNA strategy, performed by Kong et al., in MG63 and U2OS cells showed a reduction in proliferation rate and inhibited the cell migration and the invasive capability (138). It was suggested that this effect could be a consequence of the downregulation of cathepsin S (a lysosomal cysteine protease of papain subfamily) upon CD44 knocking out. Concordantly, a similar study performed by Gvozdenovic et al., in 143B osteosarcoma cell line using an shRNA-silencing approach to downregulate CD44 expression, resulted in a decrease in the adhesion of osteosarcoma cells to HA, migration, and a diminished capacity to growth anchorage independent in soft agar. However, CD44 silencing favored the growth of the primary tumor and the appearance of pulmonary metastasis in an intratibial xenograft model (139), highlighting the existence of controversial results between in vitro and in vivo situations. Immunohistochemistry analysis of intratibial primary tumor and lung metastasis showed notably lower levels of merlin, a tumor suppressor protein, compared to the same cell lines in in vitro conditions. Therefore, this enhanced malignant phenotype in vivo could be associated with the impairment of the adhesion properties to ECM due to CD44 depletion or the reduced expression of merlin in in vivo conditions. The authors explained that since 143-B cells were obtained through Ki-Ras transformation (140), the enhanced Ras-driven metastatic behavior is evidenced because of merlin protein expression lacked in vivo. Although the mechanism involved in merlin downregulation in osteosarcoma cells has not been yet elucidated, a possible explanation could be deduced from studies carried out in breast cancer cells. In breast cancer cells, the phosphorylation of merlin which promotes its proteasomal degradation is a process initiated by OPN and mediated by the Akt pathway (141), thus suppressing completely the antitumoral activity of merlin. Interestingly, the CD44 expression in osteosarcoma CSCs has been determined to be affected by pimozide treatment (a STAT5 inhibitor). This drug reduced considerably the expression of CD44 among other stem cell markers (CD133, Oct-4, and ABCG2) in KHOS/NP and SJSA-1 cell lines and, in consequence, impaired the growth and stemness of CSCs (142). The available data from the depmap portal (https://depmap.org/portal/) showed some differences in CD44 expression among osteosarcoma cell lines. Thus, MG63, OS252, C396, and HS888T cell lines showed the highest levels of CD44 expression, while NOS1 and HSOS1 had the lowest levels.
In addition to the experimental strategies described above, the study of miRNAs and long non-coding mRNAs that can downregulate CD44 levels have been also useful to determine the role of CD44 in osteosarcoma pathogenesis. For example, ectopic expression of miR-34a represses the expression of CD44 in two osteosarcoma cell sublines (F5M2 with highly metastatic potential and F4 with a low metastatic potential), inhibiting their migration and invasive ability (143). Another miRNA, the miR-199a-3p that targets directly the 3′-UTR region of CD44, produces a reduction in the levels of CD44 mRNA. Interestingly, miR-199a-3p levels are notably reduced in osteosarcoma cells and tumors compared to normal osteoblast. The overexpression of miR-199a-3p in KHOS and U-2OS inhibited CD44 mRNA levels and significantly increased the sensitivity of these cells to doxorubicin (144). In other studies, in vitro knockdown of the oncogenic long non-coding RNA GAPLINC (Gastric adenocarcinoma predictive long intergenic non-coding) in HOS and Saos-2 osteosarcoma cell lines, reduced migration and invasion by inhibiting considerably CD44 expression, although it does not impair cell proliferation (145). In summary, these studies suggest that the CD44-miR-199a-3p axis and GAPLINC regulation are relevant regulators in the development of metastasis, tumor recurrence, and drug resistance in osteosarcoma cells mediated by CD44.
4.3 Ewing Sarcoma
Ewing sarcoma, an extremely rare sarcoma that arises mainly during childhood and adolescence, presents a notably reduced expression of CD44 compared to the rest of the sarcoma cell lines (Figure 4). The explanation for this difference could rely on the CD44 gene regulation, which contains an element recognized by the ETS-1 (v-ets erythroblastosis virus E26 oncogene homolog 1) family transcription factors. Ewing sarcoma is genetically characterized by a balanced chromosomal translocation, in which a member of the FET gene family (FUS, EWSR1, or TAF15) is fused with an ETS transcription factor (FLI1, ERG, ETV1, ETV4, or FEV, among others) (146). The EWSR1–FLI1 chimeric protein can recognize the domain on the CD44 promotor and regulates its expression. An assay performed in the A673 cell line with an shRNA silencing approach for EWSR1-FLI1 showed that EWSR1-FLI1 inhibition induces CD44, CD59, CD73, CD29, and CD54 surface antigen expression, among others (147). Therefore, the lower CD44 levels find in this rare cancer are due to the high basal expression levels of the EWSR1-ETS1. This phenomenon, regarding the downregulation of CD44 in comparison to other sarcomas, could be determinant for the tumor growth, dissemination, and drug resistance in this sarcoma subtype. Thus, further research is required to define the role of CD44 in Ewing sarcoma.
Beyond this observation, the role of CD44 in the progression, metastasis, or drug resistance of Ewing sarcoma remains unclear. Recently, Paulis et al., showed that CD44 was involved in a phenomenon known as ‘vasculogenic mimicry’, defined as the ability of some cells to transdifferentiate into cells with endothelial characteristics and constitute vasculogenic networks independent of angiogenesis, which is associated with tumor aggressiveness and poor prognosis. The authors used two derived Ewing sarcoma cell lines (EW7 and SIM.EW27) and two breast cancer cell lines (MDA-MB-231 and MCF-7) to study key molecules involved in the vasculogenic mimicry. The authors concluded that CD44/c-Met signalling cascade, highly overexpressed in the aggressive cell lines (EW7 and MDA-MB-231), is crucial for vasculogenic mimicry (148). Overexpression of CD44 (CD44s and CD44v6 isoform) in the EW7 and MDA-MB-231 cell lines (highly metastatic) compared to the low-invasive cell lines (SIM.EW27 and MCF-7) was related to increased aggressiveness and to the promotion of the formation of vasculogenic structures in vitro. Accordingly, knocking-out CD44 by siRNA reduced the CD44 RNA level and the protein expression to approximately 40% in EW7 cells. As result, the migration capacity was markedly reduced, as well as the vascular-like networks. Tumor samples from patients analyzed in this study also supported the association among vasculogenic structures and ‘blood lakes’ formation and CD44 expression. CD44 was detected by immunochemistry in 14 out of 15 Ewing sarcoma tissues whose score was positive for the presence of blood lakes (149).
4.4 Fibrosarcoma
Several studies involving fibrosarcoma cell lines have been performed to elucidate the role of the receptors for hyaluronic acid-mediated motility (RHAMM, another HA receptor) and HA interactions in fibrosarcoma. Kouvidi et al., determined the effect of HA/RHAMM signalling on the ability of HT1080 fibrosarcoma cell line to adhere to fibronectin. On one hand, the adhesion properties of HT1080 cells significantly increased (p ≤ 0.01) with LMW-HA treatment, while HMW-HA inhibited them. On the other hand, the HT1080 RHAMM-deficient cells had a diminished adherence capacity compared with control cells. Additionally, in vitro experiments established that the activation of FAK and ERK1/2 signalling pathways, triggered through the RHAMM/HA interaction, regulated cell adhesion properties in HT1080 fibrosarcoma cells (150). Hatano et al., reported that, in in vitro conditions, RHAMM interacted with ERK (as previously explained), increasing the proliferative ability of fibroma cells. However, this process involved the interaction of CD44 with the epidermal growth factor receptor (EGFR) (151). Therefore, the axis CD44/RHAMM seems to mediate proliferation and metastatic dissemination due to the modification of adhesiveness to ECM components.
The specific contribution of CD44 in metastatic dissemination was studied in murine models (152). Culp et al., overexpressed the human CD44s (standard isoform) on the nonmetastatic cell lines: sis-transformed Balb/c 3T3 cells and ras-revertant IIIA4 cells. Afterward, the cells were implanted in athymic nude mice. The result of the experiment demonstrated that the increased expression of human CD44s in the mice model promoted micrometastasis events of the lungs (152).
The functional studies performed in different sarcomas demonstrate that CD44 plays a relevant role in sarcomagenesis and tumor progression. Ligand-dependent processes, proliferation events, migration/adhesion capacities, or drug resistance were shown to be regulated through specific mechanisms in which CD44, ECM components, and microenvironment have a direct or indirect effect. Although several studies have shown a relevant role of CD44 in a reduced number of sarcomas, there is a clear lack of studies in other types of sarcomas such as myxofibrosarcoma, synovial sarcoma, or rhabdomyosarcoma, among others. This highlights the existence of a poorly developed field of research that needs to be completed to define in detail the role that CD44 plays in these types of cancer.
5 Prognostic Value of CD44 Expression in Sarcomas
Several studies have analyzed the prognostic value of CD44 expression in sarcomas (Table 1). Given that sarcomas are rare cancers, and therefore it may be difficult to accumulate a relevant number of cases, several of these studies have been carried out with a series of patients consisting of various tumor types. Henderson et al., analyzed the expression of CD44 by immunohistochemistry in a series of 23 soft tissue sarcomas (8 liposarcomas, 4 myxofibrosarcoma, 4 undifferentiated pleiomorphic sarcomas, 1 alveolar soft parts sarcoma, 1 extraskeletal mesenchymal chondrosarcoma, 1 leiomyosarcoma, 1 malignant peripheral nerve sheath tumor, 1 extraskeletal osteosarcoma, 1 synovial sarcoma, and 1 primitive neuro-ectodermal tumor. Their results suggest that increased CD44 expression was associated with a worse disease specific survival (p=0.056, univariant analysis). In the same article, the authors analyzed by multivariate analysis 74 cases of soft tissue sarcoma from The Cancer Genome Atlas program (TCGA) that included clinical and genomic data (https://www.cancer.gov/about-nci/organization/ccg/research/structural-genomics/tcga). It was shown that CD44 copy number variations predicted worse disease specific survival (p=0.007). Thus, the authors concluded that increased expression of CD44 was correlated with worse outcomes in soft tissue sarcomas (153). In another study, Kahara et al., analyzed by immunohistochemistry the expression of several CD44 isoforms in a series of 47 sarcomas (18 malignant fibrous histiocytomas, 13 synovial sarcomas, 7 malignant schwannomas, and 9 liposarcomas). Isoforms CD44v3, v4, v5, v6, v7, and v9 were frequently detected, whereas CD44v10 was not expressed in any of the samples analyzed. CD44v6 isoform was commonly detected in high-grade tumors and both CD44v6 and v9 expression correlated negatively with metastasis-free survival (154). Kebudi et al., also studied the relevance of CD44 levels in serum (measured by ELISA), in the context of pediatric sarcomas (18 rhabdomyosarcomas, 22 Ewing sarcoma, and 15 osteosarcomas). In this study, the differences observed between the serum levels of CD44 in children with sarcoma and healthy controls were not significant (p>0.05), as result, a non-diagnostic or prognosis value was established (155).
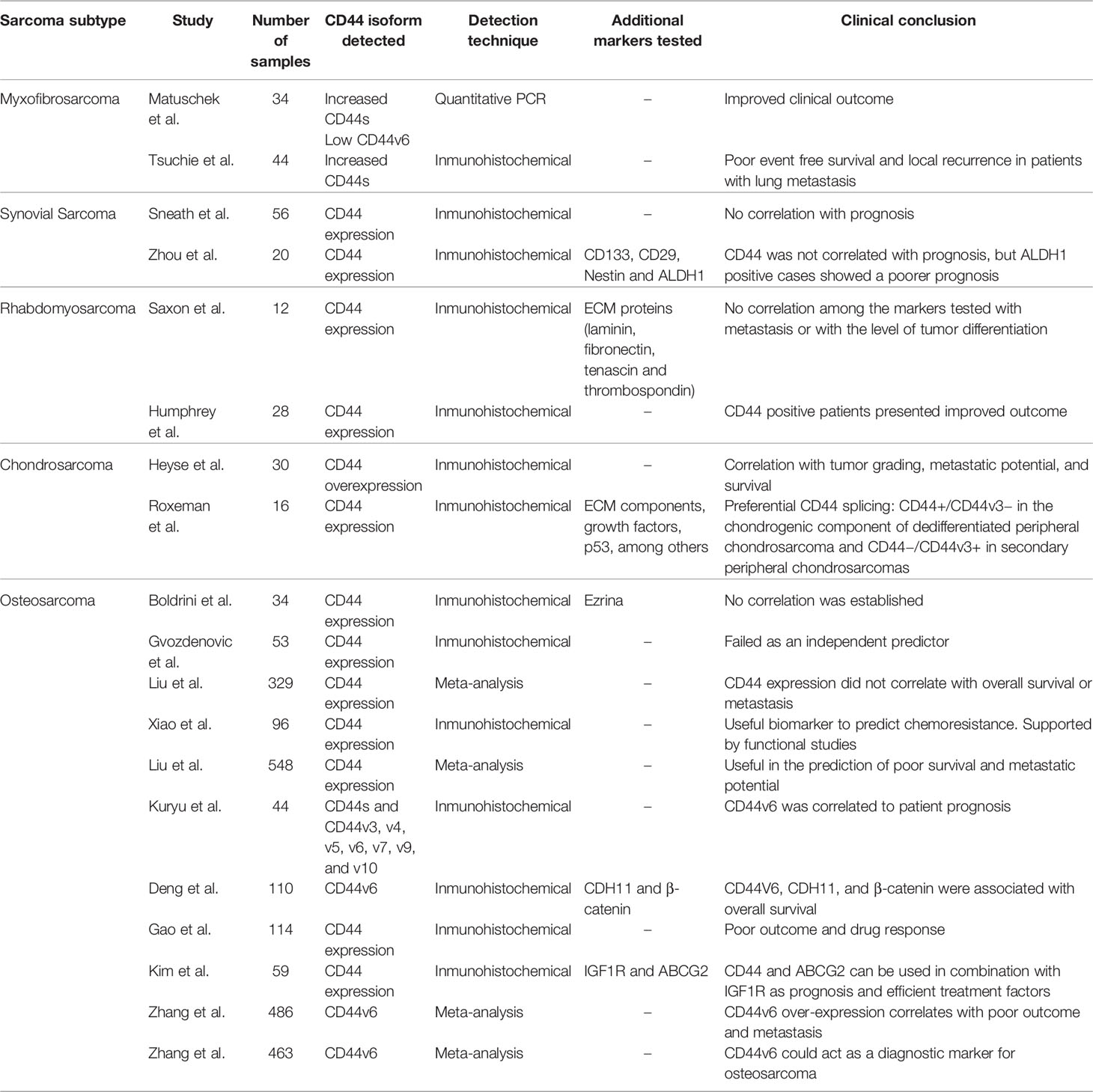
Table 1 Summary of sarcoma subtype studies analyzing CD44 expression alone or in combination with other biomarkers in patient samples.
5.1 Studies Performed on Specific Sarcoma Subtypes
5.1.1 Myxofibrosarcoma
Myxofibrosarcoma is one of the most common and aggressive soft tissue sarcomas, commonly developed in the limbs. A multivariate study focused on the analysis of CD44 isoforms in 34 adult patients with myxofibrosarcoma showed that increased expression of CD44s and reduced expression of CD44v6 isoform correlated significantly with an improved outcome (p<0.05 and p<0.02, respectively) (156). Additionally, a recent multivariate analysis designed to identify possible relationships between CD44s expression and clinic-pathological factors carried out in 44 retrospectively enrolled myxofibrosarcoma patients, showed that increased expression of CD44s correlated negatively with event-free survival and local recurrence. Additionally, in the subgroup of patients with distant metastasis, the CD44 expression was significantly elevated in patients with lung metastasis compared to patients with lymph node metastasis (p=0.044) (157).
5.1.2 Synovial Sarcoma
Synovial sarcoma is an aggressive soft tissue sarcoma, whose initiation and progression rely on the fusion gene SYT-SSX (158). This tumor has a low incidence, and it is predominantly detected in the lower extremities of young adults (8). This tumor is formed by mesenchymal spindle cells, but it is not related to the synovial membrane. Studies of immunohistochemistry determined that CD44 expression did not correlate with prognosis (survival, local recurrence, or metastasis) in synovial sarcoma (159). The uselessness of CD44 levels in synovial sarcoma prognosis has also been supported by Zhou et al., where stem cell-associated markers (CD133, CD29, CD44, nestin) and ALDH1 were characterized immunohistochemically in 20 synovial sarcomas. No relationship was settled down between these markers and clinical parameters (age, gender, sites, tumor size, histological type, tumor stage, and distant metastases). However, this study confirmed that ALDH1 positive synovial sarcomas had a significantly poorer prognosis compared to ALDH1 negative synovial sarcomas (160), providing an alternative prognosis marker to CD44.
5.1.3 Rhabdomyosarcoma
Rhabdomyosarcoma is a malignant soft tissue tumor that arises from muscle cells. It commonly arises during childhood. There are two main types of rhabdomyosarcomas that differ in their molecular and clinical characteristics. Alveolar rhabdomyosarcomas are characterized by the presence of reciprocal translocations that result in chimeric proteins that fusion the PAX3 or PAX7 genes with the transcription factor FOXO1. By contrast, embryonal rhabdomyosarcomas are characterized by chromosomal losses and gains (161). Humphrey et al., analyzed by immunohistochemistry, a series of 28 rhabdomyosarcomas (8 alveolar and 20 embryonal) and showed that CD44 positive patients had improved outcomes compared with CD44 negative tumors (p=0.001, Fisher test) (162). By contrast, a study performed by Saxon et al., in 12 pediatric rhabdomyosarcoma tumor-derived cells (five alveolars, six embryonal, and one botryoid subtype) reported no association between the expression of ECM proteins (laminin, fibronectin, thrombospondin, tenascin) and CD44 with metastatic events at clinical presentation (163).
5.1.4 Chondrosarcoma
Chondrosarcoma is a type of sarcoma that develops from chondrocyte progenitors, primarily affecting the cartilage of the femur, arm, pelvis, or knee. Heyse et al., determined that the levels of CD44s increased with the malignant grade of the tumor and that the overexpression of CD44 correlated with metastatic potential and survival (164). This study included 22 conventional chondrosarcomas, two dedifferentiated chondrosarcomas, two extraskeletal chondrosarcomas, and one periostal, mesenchymal, clear cell, and myxoid chondrosarcoma each. In another study, Rozeman et al., analyzed sixteen chondrosarcomas by immunohistochemistry for CD44, different ECM components, and growth factors. The study suggested the existence of a differential pattern of CD44 isoform. Thus, CD44 positive and CD44v3 negative expressions were found in the chondrogenic component of dedifferentiated peripheral chondrosarcoma, whereas in secondary peripheral chondrosarcomas was detected the expression of CD44 negative− and CD44v3 positive isoforms (165). Dedifferentiated peripheral chondrosarcoma is a rare subtype of chondrosarcoma arising superimposed on the cartilage cap of a pre-existing osteochondroma (8). These findings suggest that different CD44 isoforms can be associated with different grades of chondrogenic differentiation in chondrosarcomas. No data about the correlation of clinical parameters with CD44 expression were present in this study.
5.1.5 Osteosarcoma
Osteosarcoma is one of the most common sarcomas observed during childhood and adolescence (8). Approximately 30% of patients develop pulmonary metastasis (166). Some parameters well-established that affect the patient outcome are the anatomic location of the primary tumor, tumor size, dissemination processes, and response to induction chemotherapy (167). These tumors are the sarcomas in which more studies on CD44 have been carried out although the role of CD44 as a prognostic marker in osteosarcoma patients is controversial. Some studies, including one meta-analysis of 329 patients from six different studies (168), conclude that there is no significant association between prognostic or metastatic recurrence with CD44 expression levels (168–170). However, recent studies, including other meta-analyses of 548 osteosarcoma patients from nine studies, have concluded that CD44 expression may predict survival, metastasis recurrence, and drug resistance (136, 137). This agrees with in vitro studies carried out in different osteosarcoma cell lines (KHOS doxorubicin resistant, U2-OS doxorubicin resistant, MNNG/HOS, and 143B) that demonstrated that CD44 promotes cell migration, cell invasiveness, and drug resistance (136, 137, 139, 170).
Other studies focused on osteosarcoma have revealed that overexpression of CD44v6 (171, 172), CD133 (173), CD133/CD44 combination (174), CDH11 (172), β-catenin (172), GAPLINC (145), CD44-miR-199a-3p axis (144) and the combination CD44/IGF1R/ABCG2 (175) could provide valuable information as prognostic markers in this sarcoma. Supporting the role of the CD44v6 marker in osteosarcoma, two meta-analyses (both with more than 460 osteosarcoma cases) focused on the prognostic utility of this specific CD44 isoform revealed that its overexpression is associated with the overall survival rate and the occurrence of metastatic events and could be used as a complementary diagnostic marker in osteosarcoma (176, 177). However, the authors explained that the diagnostic utility of CD44v6 presented some limitations due to the low number of articles involved in the meta-analysis and the heterogeneity among them (because of the cut-off values, control groups, assay kits, and other aspects). Interestingly, the CD44v6 variant is one of the CD44 isoforms that more frequently correlates with tumor progression in other malignancies. For example, poor prognosis, presence of metastasis, or disease progression have been correlated with CD44v6 expression changes (alone or in combination with other isoforms) in gastric carcinoma (178, 179), non-small cell lung carcinoma (180, 181), hepatocarcinoma (182), acute myeloid leukemia (183), non-Hodgkin’s lymphoma (184, 185), pancreatic adenocarcinoma (186), primary pancreatic cancer (187) and uterine cervical carcinoma (188). Therefore, the CD44v6 variant seems to play a critical role in disease progression and could provide useful prognostic information in osteosarcoma, among other malignancies.
The combination of CD44 with other surface markers can provide additional information about the malignant potential of osteosarcoma cells. For example, in nude mice intratibial xenograft model performed with Saos-2 osteosarcoma cell line, CD133+/CD44+ cells were potentially more metastatic (174). A multivariate analysis performed on 90 osteosarcomas and 20 osteochondromas, indicated the clinical stage, metastasis status, and the relationship between the expression of CD44V6, CDH11, and β-catenin correlated with improved outcome (172). GAPLINC showed an indirect regulation of CD44 due to both mRNAs are negatively regulated by the same microRNA, the miR211-3p. GAPLINC overexpression in osteosarcoma was shown to correlate with advanced Enneking stage, distant metastasis, and poor outcome (145), providing an additional novel biomarker. The expression of CD44/IGF1R/ABCG2, tumoral markers related to prognosis and drug resistance, have also been analyzed, alone and in combination, in order to obtain information about their contribution to the patient prognosis. Thus, the Insulin Growth Factor 1 receptor (IGFR1) has been shown to be a prognostic factor for metastasis in osteosarcoma (189). The analysis of the expression pattern in 59 osteosarcomas showed that IGF1R expression is highly correlated with ABCG2 expression and with CD44 expression in osteosarcoma patients under 10 years old (175).
6 Therapeutic Approaches Targeting CD44
CD44 can be considered a suitable therapeutic target in many malignancies by blocking the activation of the signalling pathways in which this protein is involved, or by using its overexpression to drive, specifically, the pharmacological treatment. In fact, several strategies targeting CD44 have already been developed which include small molecular inhibitors, peptides, aptamers, blocking antibodies, secretase inhibitors, drug delivery systems, CD44 decoys, and HA oligomers (Table 2).
6.1 Inhibition of CD44 Expression
Since CD44 is expressed at high levels in most types of sarcoma, inhibition of CD44 expression could have therapeutic usefulness. In this sense, several compounds have been shown to regulate the expression of CD44, both directly and indirectly (218). Silibinin, a flavonolignan composed of two diastereomers (silibinin A and B), strongly inhibited the activity of the CD44 promoter in the prostate (PC-3M) and pancreatic cancer cell lines (BxPC-3 and PANC-1) (190, 191). Zerumbone, a sesquiterpenoid and cyclic ketone, suppressed EGF-dependent CD44 expression through inhibition of the STAT3 pathway in breast cancer cell lines (SKBR3 and MDA-MB468) (192). Additionally, the inhibition of the STAT3 pathway (through the selective blocking of STAT3 phosphorylation) was obtained with the combination of curcumin and epigallocatechin gallate in a pharmacological treatment, which reduced the CD44+ population of CSCs in MDA-MB-231 and MCF7 breast cancer cell lines (193). Therefore, the use of Sibilin, Zerumbone, or curcumin/epigallocatechin notably reduced the population of CD44+ tumor cells (breast cancer, pancreatic cancer, and prostate cancer), suggesting a novel approach for the treatment of sarcomas.
6.2 Inhibition of γ-Secretases
As described above, CD44-ICD is released upon cleavage of CD44 by γ‐secretases and, subsequently, it is translocated to the nucleus where upregulates the expression of specific target genes implied in stemness, migration/invasiveness, or cell proliferation. The cleavage mechanism requires, inexorably, the activity of the presenilin‐γ‐secretases (aspartyl proteases). Thus, direct inhibition of these enzymes with γ-secretase inhibitors (GSIs) is a particularly interesting approach to block CD44-mediated signalling because these inhibitors can block CD44-ICD release and, consequently, interfere with the gene regulatory functions of CD44-ICD. The γ-secretase complex processes the precursor of amyloid protein (APP) and cleavages the intracellular domain of NOTCH signaling protein (NOTCH-ICD), analogously, to CD44-ICD. Several γ-secretase complex inhibitors (GSIs) have been developed and deployed for the treatment of Alzheimer’s with variable success (219) and there has been a repositioning of these molecules as candidate drugs against cancer and immune diseases. Since GSIs are also active inhibiting CD44-ICD cleavage (220), the CD44-ICD downstream signalling could be effectively affected and consequently, CD44-mediated cell migration and invasion mechanisms. Many of these inhibitors have been extensively tested in clinical trials and currently, it is an active development field. For example, the GSI PF-3084014 (Nirogacestat) was well tolerated in patients with advanced cancer and demonstrated promising clinical benefit in patients with refractory, progressive desmoid tumors who receive long-term treatment (NCT01981551) (194). Another of these GSIs, LY450139 (Semagacestat), was also well-tolerated and demonstrated evidence of clinical activity in heavily pretreated patients with breast cancer and leiomyosarcoma (NCT01695005) (195). In preclinical studies, the potent GSI BMS-906024 showed enhanced antitumor activity in combination with paclitaxel versus either drug alone, decreasing cell proliferation and increasing apoptosis in in vivo studies using cell line- and patient-derived lung adenocarcinoma xenografts (196). However, this approach has not been tested to date in sarcomas and may represent an attractive treatment strategy.
6.3 Blocking CD44 With Antibodies
Antibodies targeting CD44 are being tested in preclinical and clinical trials for many types of cancer. These antibodies can be conjugated with anti-tumor drugs to target cells overexpressing CD44 to favor the antitumoral drug uptake or act as blockers of CD44-mediated signalling. There have been developed several molecule-conjugated antibodies. The Roche radioimmunoconjugate RO5429083 (RG7356) antibody targets a conformational-dependent epitope of CD44 (in the constant region of the extracellular domain), and it is being tested in clinical trials to treat neoplasms (NCT01358903) and acute myelogenous leukemia (NCT01641250) (197, 198). Another anti-CD44 monoclonal antibody, called U36, labeled with indium-111, was tested in head and neck carcinoma xenografts that expressed CD44v6 isoform. The authors of this study suggested the possible use of this antibody as a cancer detection tool (199). Bivatuzumab mertansine is the combination of a humanized monoclonal antibody against CD44v6 and a cytotoxic agent (mertansine), which has been tested in a phase I trial in head and neck carcinoma (200, 201). However, the therapeutic response in these trials was variable and severe skin toxicity was detected due to the binding of the antibody to the CD44v6 isoform located on the keratinocyte’s membrane.
Alternatively, CD44 blocking antibodies have also been developed. H4C4, a monoclonal antibody that recognizes the extracellular domain of CD44 (from residue 69 to residue 90), reduced tumor growth, metastasis, and post-radiation tumor recurrence in human pancreatic mice xenografts (202). This antibody affected both bulk tumor cells and tumor initiating cells, decreasing the capacity of self-renewal and tumor initiation through STAT3 signalling inhibition and the downregulation of the stemness gene Nanog (202). The CD44 blocking monoclonal antibodies IM7 (that recognizes the constant region of the ECD, specifically residues 145-186) and KM201 (that binds to a region that is close to the HA binding domain) abrogate the VEGF secretion mechanism through the inhibition of the HA-CD44 signalling in human umbilical vein endothelial cells (203). Additionally, Uchino et al., demonstrated that the IM7 antibody decreased cell migration and invasion capacities in breast cancer cells (MCF-7-14 cells and its clone CL6, and MDA-MB-231 cells) using in vitro conditions (204). Therefore, there is an untested group of anti-CD44 blocking antibodies, which may have therapeutic potential in the context of sarcomas, but further research is needed.
6.4 Blocking CD44 With Peptides and Aptamers
Another therapeutic strategy could be based on the use of peptides and aptamers to block CD44 interactions or reduce CD44 expression. These molecules have a great affinity and specificity to target proteins and thus are excellent tools to inhibit their functions. Several peptides and RNA aptamers directed against CD44 have already been tested in many cancer models (221). The peptide PEP-1 (a short amphipathic peptide that blocks the interaction CD44/HA) reduced the CD44 expression levels in mice models of gastric cancer (205). PCK3145, a synthetic peptide corresponding to amino acids 31-45 of prostate secretory protein 94 (PSP94), was demonstrated to reduce bone metastases and prostate tumor growth tumor in rats injected with MAT-Ly-Lu-B-2 cell line (206). The mechanism of action of this antitumoral peptide is not completely understood, but it seems the peptide interferes with the tyrosine kinase activity associated with the VEGF signalling axis in endothelial cells inhibiting angiogenesis processes (in vivo testing with Mat Ly Lu rat prostate cancer cells) (207) and reduces the levels of plasma matrix metalloproteinase MMP-9 (a CD44 ligand). Annabi et al., studied the antitumoral effect of this peptide in the fibrosarcoma cell line HT1080 and showed that it inhibited cell adhesiveness to HA, laminin-1, and type-I collagen. PCK3145 inhibited the secretion of MMP-9 and triggered the shedding of the ectodomain of CD44 from the cell surface preventing the interaction of CD44 with MMP-9 (208). The RNA aptamer (Apt1) was used to functionalize the surface of PEGylated liposomes. This combination, Apt1-Liposome, allowed a better cellular uptake of the conjugated molecule in CD44 positive cell lines A549 (lung cancer) and MDA-MB-231 (breast cancer) (209). Finally, CD44-EpCAM (Epithelial Cell Adhesion Molecule) aptamer is a double-stranded RNA adaptor which acts blocking both, CD44 and EpCAM, simultaneously reducing tumor progression and promoting apoptosis, both in vitro and in vivo xenograft models of ovarian tumor cells (OVCAR8) (210).
6.5 CD44-Ligand Chemotherapy Delivery Systems
In addition, several strategies exploit the ability of CD44 to interact with a variety of extracellular proteins (25). For example, the interaction of CD44 with HA, its principal ligand, can be used to maximize the uptake of chemotherapy delivery systems on cells overexpressing CD44. Chemotherapeutic agent-encapsulated liposomes, or covalently bound HA-bioconjugates, were selectively taken up by tumor cells after systemic delivery and reduced specific gene expression in several xenograft models (222–224). Several of these innovative tools based on HA-functionalized liposomes have become an excellent CD44-mediated intracellular delivery system for doxorubicin (DOX) or photodynamic anticancer therapy in osteosarcomas: Chol-SS-mPEG/HA-L (211), HA-LsDOX (212), ALN–HA–SS–L–L/DOX (213), and HA-es-ZnPP (214). Chol-SS-mPEG/HA-L is an HA-coated redox responsive liposome, whose cytoplasmic drug release system is triggered by GSH. The HA-LsDOX liposomes mechanism consists in the release of the drug into the endoplasmic reticulum, promoting protein sulfhydration and ubiquitination, and activating CHOP-mediated pro-apoptotic signalling. ALN-HA-SS-L-L/DOX is a liposome with dual bone and CD44 targeting abilities and redox sensitivity whose efficacy appears to be increased by the co-administration of internalizing RGD (Arg-Gly-Asp motif). HA-es-ZnPP, a hyaluronan conjugated zinc protoporphyrin via an ester bond with a tumor environment-responsive mechanism, was tested in vitro and in a mouse sarcoma S180 solid tumor model. This nanoprobe for photodynamic anticancer approach combined with a xenon light source, demonstrated prolonged circulation time and enhanced cell permeability and retention. Consequently, an improved anticancer therapy effect without apparent side effects.
The micelles with HA functionalization have been used in other drug–gene combinations therapies to co-delivery the antitumor treatments (e.g., siRNA against overexpressed cancerous genes, anticancer drugs-Paclitaxel, doxorubicin, superparamagnetic iron oxide nanoparticles-SPIONs…) (225–228). The synergistic effect of these micelles on cell cytotoxicity was tested in the MDA-MB-231 breast cancer cell (229) and OVCAR8TR ovarian cancer cells (230, 231), both overexpressing CD44. Additionally, a dual-targeting platform for CD44 has already been tested in hepatic tumors (e.g., HA- glycyrrhetinic acid -conjugated polymer to target liver) (232, 233) and breast cancer (e.g., HA-folic acid micelles for cells overexpressing the folic acid receptor) (234). These multiple targeting strategies showed better consistency and increased cellular uptake, thus enhanced cytotoxicity. In the study performed by Xi Y et al. curcumin was used in a dual-targeting delivery therapy combining the active bone accumulating ability with tumor CD44 targeting capacity in alendronate-hyaluronic acid-octadecanoic acid (ALN-HA-C18) micelles (215). The curcumin loaded in ALN-HA-C18 micelles showed increased cytotoxic activity against MG-63 cells and reduced tumor growth in the osteosarcoma mouse model compared to the free drug. Nanoparticles labeled with CD133-EGFR aptamers were determined to inhibit tumor growth in the osteosarcoma mouse model (235). Thereby, novel combinations of dual-targeting such as CD133-CD44 aptamers, both overexpressed in osteosarcoma CSCs, are potentially susceptible to being tested in these malignancies. Overall, these innovative delivery packages showed an increased tissue specificity, enhanced anticancer effect and great potential in clinical developments and delivery systems, supposing a therapeutic alternative superficially explored in sarcomas.
In summary, these studies indicate that treatment with HA-functionalized liposomes or photodynamic therapy reduced tumor growth and increased survival time in xenograft models of osteosarcoma, becoming a promising osteosarcoma-targeted therapy. Beyond its biological properties, HA nanomedicine is limited by the reduced methods for chemical conjugation, the fluctuant cellular uptake caused by the chemical characteristics of HA, and the unavoidable biological degradation when it enters in vivo (228). Therefore, the effects of these limitations need to be further studied to ensure minimum adverse responses and maximum clinical efficacy.
6.6 Blocking CD44 With CD44 Ligands
Finally, another way to block the CD44 function is by blocking CD44-HA interactions. The soluble CD44 ectodomain has been demonstrated to compete with the CD44-HA interaction on malignant cells, inhibiting tumor progression and invasiveness (236). Conversely, another strategy is to use HA-oligomers to block CD44-HA interactions. HA oligomers are small molecule chains composed of a variable number of units (6-12 mers). These molecules have been shown to suppress signalling pathways involved in drug resistance against different therapeutic agents (237, 238). Several studies have shown that HA disaccharides bind CD44, competing by and displacing the biological HA polymer (239, 240) and can inhibit HA synthesis (241). Despite its therapeutic potential there are few studies addressing the therapeutic possibilities of these molecules in sarcomas. Hosono et al., studied the effect of hyaluronan oligosaccharides on tumor growth in murine (LM-8) and human (MG-63) osteosarcoma cells. Hyaluronan octamers promoted apoptosis and reduced both cell viability, cell proliferation and cell motility, and decreased the retention of endogenous HA. The intratumoral injection of these molecules in xenograft models produced a reduction of HA accumulation in the tumor, suppressing the dissemination of tumor cells to the lung (216). A similar effect has been observed in other cancers such as melanoma, carcinoma, or glioma (94, 217). HA oligomers therapies provide a viable alternative for blocking the CD44-HA interactions in sarcomas which has been only superficially explored, so that clinical trials should be performed to confirm this antitumoral approach.
7 Conclusions
CD44 has a key role, along with ECM components, in sarcoma tumor development, metastasis, and drug resistance. Understanding CD44 signalling is crucial to comprise the multifactorial role of this protein in several processes, such as activation of different pathways, upregulation of several genes, or modification of cytoskeletal structures that promote EMT mechanisms, among others.
MSCs are thought to give rise to sarcomas. As consequence, several molecular mechanisms, transcription factors, metabolic pathways, and cell markers are shared among MSCs, CSCs and sarcomas. CD44, in this context, provides the elements to maintain the stemness and to take advantage of the tumoral microenvironment in hypoxia conditions. Studies focused on MSCs, and sarcomas have shown CD44 to be highly expressed in these tissues compared to mature cells. Thus, it has been proposed that during cell differentiation, expression of CD44 could be downregulated, and conversely, high levels of CD44 are necessary to maintain the cells in a stemness state.
Further research involving the sarcoma functional mechanisms could be really enriching in the characterization context of sarcoma, as well as, in the therapeutic approaches targeting CD44. Some of the innovative treatment tools such as peptides, aptamers, HA oligomers, pharmacological substances, and chemotherapy delivery systems, among others, have been developed from the discovery and well-understanding of the CD44 structure, the signalling pathways and the interactions involved.
The data from the characterization of osteosarcomas have revealed that CD44 (concretely CD44v6 isoform) is a potential and promising marker for prognosis and diagnosis. There is a low number of detailed studies deciphering the prognostic/diagnostic value of CD44 in sarcomas (excluding osteosarcoma which is one of the most studied and common sarcomas). In addition, sarcoma-specific studies involving a greater number of patients are necessary to confirm the influence of CD44 variant expression on the patients’ prognosis (regardless of the utility of CD44v6 as a prognostic marker in osteosarcoma). The complexity and distinct molecular origin of the different sarcoma subgroups have made impossible to apply a unified characterization or prognosis criterion, and therefore they should be studied separately. In addition, the reduced number of studies focused on CD44, and the low number of patients involved in the different sarcoma subgroups have produced inconclusive results. As consequence, the combination of different characterization markers will be more precise and will provide relevant information to determine patients’ outcomes, neither than the use of CD44 individually.
To conclude, more research about the contribution of CD44 in sarcomas could contribute to understand the pathogenesis of these rare cancers, and evolve towards novel therapies and future clinical trials.
Author Contributions
Writing original draft preparation, EF-T; writing - review and editing, EF-T, RM-FM, and JA; supervision, JA; funding acquisition, JA. All authors have read and agreed to the published version of the manuscript.
Funding
This review was funded by the Instituto de Salud Carlos III, grant numbers PI20CIII/00020, DTS18CIII/00005, Asociacion Pablo Ugarte, grant numbers TRPV205/18; Asociación Candela Riera, Asociación Todos Somos Iván & Fundación Sonrisa de Alex, grant numbers TVP333-19, TVP-1324/15; ASION, grant number TVP141/17. R.M.M-FdM is supported for a grant of the Spanish Center for Biomedical Network Research on Rare Diseases (CIBERER).
Conflict of Interest
The authors declare that the research was conducted in the absence of any commercial or financial relationships that could be construed as a potential conflict of interest.
Publisher’s Note
All claims expressed in this article are solely those of the authors and do not necessarily represent those of their affiliated organizations, or those of the publisher, the editors and the reviewers. Any product that may be evaluated in this article, or claim that may be made by its manufacturer, is not guaranteed or endorsed by the publisher.
References
1. Williams RF, Fernandez-Pineda I, Gosain A. Pediatric Sarcomas. Surg Clinics North America. W.B. Saunders; (2016) 96:1107–25. doi: 10.1016/j.suc.2016.05.012
2. Stiller CA, Trama A, Serraino D, Rossi S, Navarro C, Chirlaque MD, et al. Descriptive Epidemiology of Sarcomas in Europe: Report From the RARECARE Project. Eur J Cancer (2013) 49(3):684–95. doi: 10.1016/j.ejca.2012.09.011
3. Dufresne A, Brahmi M, Karanian M, Blay JY. Using Biology to Guide the Treatment of Sarcomas and Aggressive Connective-Tissue Tumours. Nat Rev Clin Oncol (2018) 15:443–58. doi: 10.1038/s41571-018-0012-4
4. Lawrence W, Donegan WL, Natarajan N, Mettlin C, Beart R, Winchester D. Adult Soft Tissue Sarcomas: A Pattern of Care Survey of the American College of Surgeons. Ann Surg (1987) 205(4):349–59. doi: 10.1097/00000658-198704000-00003
5. Christie-Large M, James SLJ, Tiessen L, Davies AM, Grimer RJ. Imaging Strategy for Detecting Lung Metastases at Presentation in Patients With Soft Tissue Sarcomas. Eur J Cancer (2008) 44(13):1841–5. doi: 10.1016/j.ejca.2008.06.004
6. Vezeridis MP, Moore R, Karakousis CP. Metastatic Patterns in Soft-Tissue Sarcomas. Arch Surg (1983) 118(8):915–8. doi: 10.1001/archsurg.1983.01390080023007
7. Potter DA, Glenn J, Kinsella T, Glatstein E, Lack EE, Restrepo C, et al. Patterns of Recurrence in Patients With High-Grade Soft-Tissue Sarcomas. J Clin Oncol (2016) 3(3):353–66. doi: 10.1200/JCO.1985.3.3.353
8. Fletcher CDM, Bridge JA, Hogendoorn PCW, Mertens F. WHO Classification of Tumours of Soft Tissue and Bone, 4th ed, Vol. 5. World Health Organization, 2013. ISBN-13: 978-92-832-2434-1
9. Hyman DM, Puzanov I, Subbiah V, Faris JE, Chau I, Blay J-Y, et al. Vemurafenib in Multiple Nonmelanoma Cancers With BRAF V600 Mutations. New Engl J Med (2015) 373(8):726–36. doi: 10.1056/NEJMoa1502309
10. Simon MP, Pedeutour F, Sirvent N, Grosgeorge J, Minoletti F, Coindre JM, et al. Deregulation of the Platelet-Derived Growth Factor B-Chain Gene via Fusion With Collagen Gene COL1A1 in Dermatofibrosarcoma Protuberans and Giant-Cell Fibroblastoma. Nat Genet (1997) 15(1):95–8. doi: 10.1038/ng0197-95
11. Hyman DM, Laetsch TW, Kummar S, DuBois SG, Farago AF, Pappo AS, et al. The Efficacy of Larotrectinib (LOXO-101), a Selective Tropomyosin Receptor Kinase (TRK) Inhibitor, in Adult and Pediatric TRK Fusion Cancers. J Clin Oncol (2017) 35(15_suppl):LBA2501–LBA2501. doi: 10.1200/JCO.2017.35.18_suppl.LBA2501
12. Mariño-Enríquez A, Wang WL, Roy A, Lopez-Terrada D, Lazar AJF, Fletcher CDM, et al. Epithelioid Inflammatory Myofibroblastic Sarcoma: An Aggressive Intra-Abdominal Variant of Inflammatory Myofibroblastic Tumor With Nuclear Membrane or Perinuclear Alk. Am J Surg Pathol (2011) 35(1):135–44. doi: 10.1097/PAS.0b013e318200cfd5
13. Delattre O, Zucman J, Melot T, Garau XS, Zucker J-M, Lenoir GM, et al. The Ewing Family of Tumors – A Subgroup of Small-Round-Cell Tumors Defined by Specific Chimeric Transcripts. New Engl J Med (1994) 331(5):294–9. doi: 10.1056/NEJM199408043310503
14. Prieur A, Tirode F, Cohen P, Delattre O. EWS/FLI-1 Silencing and Gene Profiling of Ewing Cells Reveal Downstream Oncogenic Pathways and a Crucial Role for Repression of Insulin-Like Growth Factor Binding Protein 3. Mol Cell Biol (2004) 24(16):7275–83. doi: 10.1128/MCB.24.16.7275-7283.2004
15. Kawaguchi KI, Oda Y, Saito T, Takahira T, Yamamoto H, Tamiya S, et al. Genetic and Epigenetic Alterations of the PTEN Gene in Soft Tissue Sarcomas. Hum Pathol (2005) 36(4):357–63. doi: 10.1016/j.humpath.2005.01.017
16. Kenerson H, Folpe AL, Takayama TK, Yeung RS. Activation of the mTOR Pathway in Sporadic Angiomyolipomas and Other Perivascular Epithelioid Cell Neoplasms. Hum Pathol (2007) 38(9):1361–71. doi: 10.1016/j.humpath.2007.01.028
17. Gibault L, Ferreira C, Pérot G, Audebourg A, Chibon F, Bonnin S, et al. From PTEN Loss of Expression to RICTOR Role in Smooth Muscle Differentiation: Complex Involvement of the mTOR Pathway in Leiomyosarcomas and Pleomorphic Sarcomas. Modern Pathol (2012) 25(2):197–211. doi: 10.1038/modpathol.2011.163
18. Coindre JM, Pédeutour F, Aurias A. Well-Differentiated and Dedifferentiated Liposarcomas. Virchows Archiv. Springer Verlag; (2010) 456:167–79. doi: 10.1007/s00428-009-0815-x
19. Huang L, Xu J, Wood DJ, Zheng MH. Gene Expression of Osteoprotegerin Ligand, Osteoprotegerin, and Receptor Activator of NF-κb in Giant Cell Tumor of Bone: Possible Involvement in Tumor Cell-Induced Osteoclast-Like Cell Formation. Am J Pathol (2000) 156(3):761–7. doi: 10.1016/S0002-9440(10)64942-5
20. Kadoch C, Copeland RA, Keilhack H. PRC2 and SWI/SNF Chromatin Remodeling Complexes in Health and Disease. Vol. 55 Biochem Am Chem Society; (2016) :1600–14. doi: 10.1021/acs.biochem.5b01191
21. Hanahan D, Weinberg RA. Hallmarks of Cancer: The Next Generation. Cell Cell Press; (2011) 144:646–74. doi: 10.1016/j.cell.2011.02.013
22. Avnet S, Cortini M. Role of Pericellular Matrix in the Regulation of Cancer Stemness. Stem Cell Rev Rep (2016) 12:464–75. doi: 10.1007/s12015-016-9660-x
23. Winkler J, Abisoye-Ogunniyan A, Metcalf KJ, Werb Z. Concepts of Extracellular Matrix Remodelling in Tumour Progression and Metastasis. Nat Commun Nat Rese (2020) 11:1–19. doi: 10.1038/s41467-020-18794-x
24. González-González L, Alonso J. Periostin: A Matricellular Protein With Multiple Functions in Cancer Development and Progression. Front Oncol (2018) 8:225. doi: 10.3389/fonc.2018.00225
25. Chen C, Zhao S, Karnad A, Freeman JW. The Biology and Role of CD44 in Cancer Progression: Therapeutic Implications. J Hematol Oncol (2018) 11:1–23. doi: 10.1186/s13045-018-0605-5
26. Senbanjo LT, Chellaiah MA. CD44: A Multifunctional Cell Surface Adhesion Receptor Is a Regulator of Progression and Metastasis of Cancer Cells. Front Cell Dev Biol (2017) 5(MAR):18. doi: 10.3389/fcell.2017.00018
27. Xu H, Tian Y, Yuan X, Wu H, Liu Q, Pestell RG, et al. The Role of CD44 in Epithelial–Mesenchymal Transition and Cancer Development. OncoTargets Ther (2015) 8:3783–92. doi: 10.2147/OTT.S95470
28. Xu H, Niu M, Yuan X, Wu K, Liu A. CD44 as a Tumor Biomarker and Therapeutic Target. Exp Hematol Oncol (2020) 9:1–14. doi: 10.1186/s40164-020-00192-0
29. Orian-Rousseau V, Sleeman J. CD44 is a Multidomain Signaling Platform That Integrates Extracellular Matrix Cues With Growth Factor and Cytokine Signals. In: Adv Cancer Res (2014), 231–54. doi: 10.1016/B978-0-12-800092-2.00009-5
30. Idzerda RL, Carter WG, Nottenburg C, Wayner EA, Gallatin WM, John TS. Isolation and DNA Sequence of a cDNA Clone Encoding a Lymphocyte Adhesion Receptor for High Endothelium. Proc Natl Acad Sci U S A. (1989) 86(12):4659–63. doi: 10.1073/pnas.86.12.4659
31. Faassen AE, Schrager JA, Klein DJ, Oegema TR, Couchman JR, McCarthy JB. A Cell Surface Chondroitin Sulfate Proteoglycan, Immunologically Related to CD44, is Involved in Type I Collagen-Mediated Melanoma Cell Motility and Invasion. J Cell Biol (1992) 116(2):521–31. doi: 10.1083/jcb.116.2.521
32. Weber GF, Ashkar S, Glimcher MJ, Cantor H. Receptor-Ligand Interaction Between CD44 and Osteopontin (Eta-1). Science (1996) 271(5248):509–12. doi: 10.1126/science.271.5248.509
33. Toyama-Sorimachi N, Sorimachi H, Tobita Y, Kitamura F, Yagita H, Suzuki K, et al. A Novel Ligand for CD44 is Serglycin, a Hematopoietic Cell Lineage- Specific Proteoglycan. Possible involvement lymphoid Cell adherence activation J Biol Chem (1995) 270(13):7437–44. doi: 10.1074/jbc.270.13.7437
34. Knutson JR, Iida J, Fields GB, McCarthy JB. CD44/chondroitin Sulfate Proteoglycan and α2β1 Integrin Mediate Human Melanoma Cell Migration on Type IV Collagen and Invasion of Basement Membranes. Mol Biol Cell (1996) 7(3):383–96. doi: 10.1091/mbc.7.3.383
35. Liu M, Tolg C, Turley E. Dissecting the Dual Nature of Hyaluronan in the Tumor Microenvironment. Front Immunol Front Media S.A.; (2019) 10:947. doi: 10.3389/fimmu.2019.00947
36. Misra S, Heldin P, Hascall VC, Karamanos NK, Skandalis SS, Markwald RR, et al. Hyaluronan-CD44 Interactions as Potential Targets for Cancer Therapy. FEBS J (2011) 278:1429–43. doi: 10.1111/j.1742-4658.2011.08071.x
37. Ponta H, Sherman L, Herrlich PA. CD44: From Adhesion Molecules to Signalling Regulators. Nat Rev Mol Cell Biol (2003) 4, 33–45. doi: 10.1038/nrm1004
38. Weigel PH. Hyaluronan Synthase: The Mechanism of Initiation at the Reducing End and a Pendulum Model for Polysaccharide Translocation to the Cell Exterior. Int J Cell Biol (2015) 2015:15. doi: 10.1155/2015/367579
39. Takeda M, Ogino S, Umemoto R, Sakakura M, Kajiwara M, Sugahara KN, et al. Ligand-Induced Structural Changes of the CD44 Hyaluronan-Binding Domain Revealed by NMR. J Biol Chem (2006) 281(52):40089–95. doi: 10.1074/jbc.M608425200
40. Enegd B, King JAJ, Stylli S, Paradiso L, Kaye AH, Novak U, et al. Overexpression of Hyaluronan Synthase-2 Reduces the Tumorigenic Potential of Glioma Cells Lacking Hyaluronidase Activity. Neurosurgery (2002) 50(6):1311–8. doi: 10.1097/00006123-200206000-00023
41. Schwertfeger KL, Cowman MK, Telmer PG, Turley EA, McCarthy JB. Hyaluronan, Inflammation, and Breast Cancer Progression. Front Immunol Front Media S.A. (2015) 6. doi: 10.3389/fimmu.2015.00236
42. Bourguignon LYW, Spevak CC, Wong G, Xia W, Gilad E. Hyaluronan-CD44 Interaction With Protein Kinase Cϵ Promotes Oncogenic Signaling by the Stem Cell Marker Nanog and the Production of microRNA-21, Leading to Down-Regulation of the Tumor Suppressor Protein PDCD4, Anti-Apoptosis, and Chemotherapy Resistance in Breast Tumor Cells. J Biol Chem (2009) 284(39):26533–46. doi: 10.1074/jbc.M109.027466
43. Lv L, Liu HG, Dong SY, Yang F, Wang QX, Guo GL, et al. Upregulation of CD44v6 Contributes to Acquired Chemoresistance via the Modulation of Autophagy in Colon Cancer SW480 Cells. Tumor Biol (2016) 37(7):8811–24. doi: 10.1007/s13277-015-4755-6
44. Ju SY, Chiou SH, Su Y. Maintenance of the Stemness in CD44+ HCT-15 and HCT-116 Human Colon Cancer Cells Requires miR-203 Suppression. Stem Cell Res (2014) 12(1):86–100. doi: 10.1016/j.scr.2013.09.011
45. Suzuki M, Kobayashi H, Kanayama N, Nishida T, Takigawa M, Terao T. CD44 Stimulation by Fragmented Hyaluronic Acid Induces Upregulation and Tyrosine Phosphorylation of C-Met Receptor Protein in Human Chondrosarcoma Cells. Biochim Biophys Acta - Mol Cell Res (2002) 1591(1–3):37–44. doi: 10.1016/S0167-4889(02)00246-X
46. Bai Y, Liu YJ, Wang H, Xu Y, Stamenkovic I, Yu Q. Inhibition of the Hyaluronan-CD44 Interaction by Merlin Contributes to the Tumor-Suppressor Activity of Merlin. Oncogene (2007) 26(6):836–50. doi: 10.1038/sj.onc.1209849
47. Marroquin CE, Downey L, Guo H, Kuo PC. Osteopontin Increases CD44 Expression and Cell Adhesion in RAW 264.7 Murine Leukemia Cells. Immunol Lett (2004) 95(1):109–12.
48. de Vita A, Vanni S, Miserocchi G, Fausti V, Pieri F, Spadazzi C, et al. A Rationale for the Activity of Bone Target Therapy and Tyrosine Kinase Inhibitor Combination in Giant Cell Tumor of Bone and Desmoplastic Fibroma: Translational Evidences. Biomedicines (2022) 10(2):372. doi: 10.3390/biomedicines10020372
49. Lin YH, Yang-Yen HF. The Osteopontin-CD44 Survival Signal Involves Activation of the Phosphatidylinositol 3-Kinase/Akt Signaling Pathway. J Biol Chem (2001) 276(49):46024–30. doi: 10.1074/jbc.M105132200
50. Sharp JA, Sung V, Slavin J, Thompson EW, Henderson MA. Tumor Cells are the Source of Osteopontin and Bone Sialoprotein Expression in Human Breast Cancer. Lab Investigation; J Tech Methods Pathol (1999) 79(7):869–77.
51. Pietras A, Katz AM, Ekström EJ, Wee B, Halliday JJ, Pitter KL, et al. Osteopontin-CD44 Signaling in the Glioma Perivascular Niche Enhances Cancer Stem Cell Phenotypes and Promotes Aggressive Tumor Growth. Cell Stem Cell (2014) 14(3):357–69. doi: 10.1016/j.stem.2014.01.005
52. Kolset SO, Tveit H. Serglycin - Structure and Biology. Cell Mol Life Sci Cell Mol Life Sci; (2008) 65:1073–85. doi: 10.1007/s00018-007-7455-6
53. Li XJ, Qian CN. Serglycin in Human Cancers. Chin J Cancer (2011) 30(9):585. doi: 10.5732/cjc.011.10314
54. Guo JY, Hsu HS, Tyan SW, Li FY, Shew JY, Lee WH, et al. Serglycin in Tumor Microenvironment Promotes non-Small Cell Lung Cancer Aggressiveness in a CD44-Dependent Manner. Oncogene (2017) 36:17. doi: 10.1038/onc.2016.404
55. Chu Q, Huang H, Huang T, Cao L, Peng L, Shi S, et al. Extracellular Serglycin Upregulates the CD44 Receptor in an Autocrine Manner to Maintain Self-Renewal in Nasopharyngeal Carcinoma Cells by Reciprocally Activating the MAPK/β-Catenin Axis. Cell Death Dis (2016) 7(11):e2456–e2456. doi: 10.1038/cddis.2016.287
56. Merrilees MJ, Zuo N, Evanko SP, Day AJ, Wight TN. G1 Domain of Versican Regulates Hyaluronan Organization and the Phenotype of Cultured Human Dermal Fibroblasts. J Histochem Cytochem (2016) 64(6):353. doi: 10.1369/0022155416643913
57. LeBaron RG, Zimmermann DR, Ruoslahti E. Hyaluronate Binding Properties of Versican. J Biol Chem (1992) 267(14):10003–10. doi: 10.1016/S0021-9258(19)50191-0
58. Mauri P, Scarpa A, Nascimbeni AC, Benazzi L, Parmagnani E, Mafficini A, et al. Identification of Proteins Released by Pancreatic Cancer Cells by Multidimensional Protein Identification Technology: A Strategy for Identification of Novel Cancer Markers. FASEB J (2005) 19(9):1125–7. doi: 10.1096/fj.04-3000fje
59. Ween MP, Oehler MK, Ricciardelli C. Role of Versican, Hyaluronan and CD44 in Ovarian Cancer Metastasis. Int J Mol Sci MDPI AG; (2011) 12:1009–29. doi: 10.3390/ijms12021009
60. Fujimoto T, Kawashima H, Tanaka T, Hirose M, Toyama-Sorimachi N, Matsuzawa Y, et al. CD44 Binds a Chondroitin Sulfate Proteoglycan, Aggrecan. Int Immunol (2001) 13(3):359–66. doi: 10.1093/intimm/13.3.359
61. Mcfarlane S, Mcfarlane C, Montgomery N, Hill A, Waugh DJJ. CD44-Mediated Activation of α5β1-Integrin, Cortactin and Paxillin Signaling Underpins Adhesion of Basal-Like Breast Cancer Cells to Endothelium and Fibronectin-Enriched Matrices. Oncotarget (2015) 6(34):36762. doi: 10.18632/oncotarget.5461
62. Montgomery N, Hill A, McFarlane S, Neisen J, O’Grady A, Conlon S, et al. CD44 Enhances Invasion of Basal-Like Breast Cancer Cells by Upregulating Serine Protease and Collagen-Degrading Enzymatic Expression and Activity. Breast Cancer Res (2012) 14:3. doi: 10.1186/bcr3199
63. Ishii S, Ford R, Thomas P, Nachman A, Steele G, Jessup JM. CD44 Participates in the Adhesion of Human Colorectal Carcinoma Cells to Laminin and Type IV Collagen. Surg Oncol (1993) 2(4):255–64. doi: 10.1016/0960-7404(93)90015-Q
64. Verma RP, Hansch C. Matrix Metalloproteinases (MMPs): Chemical-Biological Functions and (Q)SARs. Bioorganic Medicinal Chem Bioorg Med Chem; (2007) 15:2223–68. doi: 10.1016/j.bmc.2007.01.011
65. Orgaz JL, Pandya P, Dalmeida R, Karagiannis P, Sanchez-Laorden B, Viros A, et al. Diverse Matrix Metalloproteinase Functions Regulate Cancer Amoeboid Migration. Nat Commun (2014) 5(1):1–13. doi: 10.1038/ncomms5255
66. Yu Q, Stamenkovic I. Localization of Matrix Metalloproteinase 9 to the Cell Surface Provides a Mechanism for CD44-Mediated Tumor Invasion. Genes Dev (1999) 13(1):35–48. doi: 10.1101/gad.13.1.35
67. Mori H, Tomari T, Koshikawa N, Kajita M, Itoh Y, Sato H, et al. CD44 Directs Membrane-Type 1 Matrix Metalloproteinase to Lamellipodia by Associating With its Hemopexin-Like Domain. EMBO J (2002) 21(15):3949. doi: 10.1093/emboj/cdf411
68. Nagano O, Saya H. Mechanism and Biological Significance of CD44 Cleavage. Cancer Sci (2004) 95(12):930–5. doi: 10.1111/j.1349-7006.2004.tb03179.x
69. Okamoto I, Kawano Y, Murakami D, Sasayama T, Araki N, Miki T, et al. Proteolytic Release of CD44 Intracellular Domain and its Role in the CD44 Signaling Pathway. J Cell Biol (2001) 155(5):755–62. doi: 10.1083/jcb.200108159
70. Yan Y, Zuo X, Wei D. Concise Review: Emerging Role of CD44 in Cancer Stem Cells: A Promising Biomarker and Therapeutic Target. Stem Cells Trans Med (2015) 4(9):1033–43. doi: 10.5966/sctm.2015-0048
71. Tsukita S, Oishi K, Sato N, Sagara J, Kawai A, Tsukita S. ERM Family Members as Molecular Linkers Between the Cell Surface Glycoprotein CD44 and Actin-Based Cytoskeletons. J Cell Biol (1994) 126(2):391–401. doi: 10.1083/jcb.126.2.391
72. Janiszewska M, de Vito C, Le Bitoux MA, Fusco C, Stamenkovic I. Transportin Regulates Nuclear Import of CD44. J Biol Chem (2010) 285(40):30548. doi: 10.1074/jbc.M109.075838
73. Su YJ, Lai HM, Chang YW, Chen GY, Lee JL. Direct Reprogramming of Stem Cell Properties in Colon Cancer Cells by CD44. EMBO J (2011) 30(15):3186–99. doi: 10.1038/emboj.2011.211
74. Bourguignon LYW, Earle C, Wong G, Spevak CC, Krueger K. Stem Cell Marker (Nanog) and Stat-3 Signaling Promote MicroRNA-21 Expression and Chemoresistance in Hyaluronan/CD44-Activated Head and Neck Squamous Cell Carcinoma Cells. Oncogene (2012) 31(2):149–60. doi: 10.1038/onc.2011.222
75. Senbanjo LT, Aljohani H, Majumdar S, Chellaiah MA. Characterization of CD44 Intracellular Domain Interaction With RUNX2 in PC3 Human Prostate Cancer Cells. Cell Commun Signaling (2019) 17(1):1–13. doi: 10.1186/s12964-019-0395-6
76. Senbanjo LT, AlJohani H, AlQranei M, Majumdar S, Ma T, Chellaiah MA. Identification of Sequence-Specific Interactions of the CD44-Intracellular Domain With RUNX2 in the Transcription of Matrix Metalloprotease-9 in Human Prostate Cancer Cells. Cancer Drug Resist (2020) 3(3):586. doi: 10.20517/cdr.2020.21
77. Cho Y, Lee HW, Kang HG, Kim HY, Kim SJ, Chun KH. Cleaved CD44 Intracellular Domain Supports Activation of Stemness Factors and Promotes Tumorigenesis of Breast Cancer. Oncotarget (2015) 6(11):8709–21. doi: 10.18632/oncotarget.3325
78. Medrano-González PA, Rivera-Ramírez O, Montaño LF, Rendón-Huerta EP. Proteolytic Processing of CD44 and Its Implications in Cancer. Stem Cells Int (2021) 2021. doi: 10.1155/2021/6667735
79. Okamoto I, Kawano Y, Tsuiki H, Sasaki JI, Nakao M, Matsumoto M, et al. CD44 Cleavage Induced by a Membrane-Associated Metalloprotease Plays a Critical Role in Tumor Cell Migration. Oncogene (1999) 18(7):1435–46. doi: 10.1038/sj.onc.1202447
80. Kajita M, Itoh Y, Chiba T, Mori H, Okada A, Kinoh H, et al. Membrane-Type 1 Matrix Metalloproteinase Cleaves CD44 and Promotes Cell Migration. J Cell Biol (2001) 153(5):893–904. doi: 10.1083/jcb.153.5.893
81. Rao JS. Molecular Mechanisms of Glioma Invasiveness: The Role of Proteases. Nat Rev Cancer (2003) 3:7. doi: 10.1038/nrc1121
82. Takahashi K, Eto H, Tanabe KK. Involvement of CD44 in Matrix Metalloproteinase-2 Regulation in Human Melanoma Cells. Int J Cancer. (1999) 80:387–95. doi: 10.1002/(SICI)1097-0215(19990129)80:3<387::AID-IJC9>3.0.CO;2-T
83. Lammich S, Okochi M, Takeda M, Kaether C, Capell A, Zimmer AK, et al. Presenilin-Dependent Intramembrane Proteolysis of CD44 Leads to the Liberation of Its Intracellular Domain and the Secretion of an Abeta-LikePeptide. J Biol Chem (2002) 277(47):44754–9. doi: 10.1074/jbc.M206872200
84. Pelletier L, Guillaumot P, Frêche B, Luquain C, Christiansen D, Brugière S, et al. γ-Secretase-Dependent Proteolysis of CD44 Promotes Neoplastic Transformation of Rat Fibroblastic Cells. Cancer Res (2006) 66(7):3681–7. doi: 10.1158/0008-5472.CAN-05-3870
85. Sugahara KN, Murai T, Nishinakamura H, Kawashima H, Saya H, Miyasaka M. Hyaluronan Oligosaccharides Induce CD44 Cleavage and Promote Cell Migration in CD44-Expressing Tumor Cells. J Biol Chem (2003) 278(34):32259–65. doi: 10.1074/jbc.M300347200
86. Heo JS, Choi Y, Kim HS, Kim HO. Comparison of Molecular Profiles of Human Mesenchymal Stem Cells Derived From Bone Marrow, Umbilical Cord Blood, Placenta and Adipose Tissue. Int J Mol Med (2016) 37(1):115–25. doi: 10.3892/ijmm.2015.2413
87. Mafi P, Hindocha S, Mafi R, Griffin M, Khan WS. Adult Mesenchymal Ste Cells and Cell Surface Characterization: A Systematic Review of the Literature. Open Orthop J (2011) 5(Suppl 2):253–60. doi: 10.2174/1874325001105010253
88. Lv FJ, Tuan RS, Cheung KMC, Leung VYL. Concise Review: The Surface Markers and Identity of Human Mesenchymal Stem Cells. Stem Cells (2014) 32(6):1408–19. doi: 10.1002/stem.1681
89. Genadry KC, Pietrobono S, Rota R, Linardic CM. Soft Tissue Sarcoma Cancer Stem Cells: An Overview. Front Oncol Front Media S.A.; (2018) 8:475. doi: 10.1007/BF00307793
90. Gibbs CP, Kukekov VG, Reith JD, Tchigrinova O, Suslov ON, Scott EW, et al. Stem-Like Cells in Bone Sarcomas: Implications for Tumorigenesis. Neoplasia (2005) 7(11):967–76. doi: 10.1593/neo.05394
91. di Fiore R, Santulli A, Drago Ferrante R, Giuliano M, de Blasio A, Messina C, et al. Identification and Expansion of Human Osteosarcoma-Cancer-Stem Cells by Long-Term 3-Aminobenzamide Treatment. J Cell Physiol (2009) 219(2):301–13. doi: 10.1002/jcp.21667
92. Martínez-Delgado P, Lacerenza S, Obrador-Hevia A, Lopez-Alvarez M, Mondaza-Hernandez JL, Blanco-Alcaina E, et al. Cancer Stem Cells in Soft-Tissue Sarcomas. Cells (2020) 9(6):1449. doi: 10.3390/cells9061449
93. Geng S, Guo Y, Wang Q, Li L, Wang J. Cancer Stem-Like Cells Enriched With CD29 and CD44 Markers Exhibit Molecular Characteristics With Epithelial-Mesenchymal Transition in Squamous Cell Carcinoma. Arch Dermatol Res (2013) 305(1):35–47. doi: 10.1007/s00403-012-1260-2
94. Thapa R, Wilson GD. The Importance of CD44 as a Stem Cell Biomarker and Therapeutic Target in Cancer. Stem Cells Int (2016) 2016:15. doi: 10.1155/2016/2087204
95. Morath I, Hartmann TN, Orian-Rousseau V. CD44: More Than a Mere Stem Cell Marker. Int J Biochem Cell Biol (2016) 81(Pt A):166–73. doi: 10.1016/j.biocel.2016.09.009
96. Skandalis SS, Karalis TT, Chatzopoulos A, Karamanos NK. Hyaluronan-CD44 Axis Orchestrates Cancer Stem Cell Functions. Cell Signalling. (2019) 63:109377. doi: 10.1016/j.cellsig.2019.109377
97. Williams K, Motiani K, Giridhar PV, Kasper S. CD44 Integrates Signaling in Normal Stem Cell, Cancer Stem Cell and (Pre)Metastatic Niches. Exp Biol Med Exp Biol Med (Maywood); (2013) 238:324–38. doi: 10.1177/1535370213480714
98. Skubitz KM, Wilson JD, Cheng EY, Lindgren BR, Boylan KLM, Skubitz APN. Effect of Chemotherapy on Cancer Stem Cells and Tumor-Associated Macrophages in a Prospective Study of Preoperative Chemotherapy in Soft Tissue Sarcoma. J Transl Med (2019) 17(1):1–13. doi: 10.1186/s12967-019-1883-6
99. Nakamura H, Kenmotsu SI, Sakai H, Ozawa H. Localization of CD44, the Hyaluronate Receptor, on the Plasma Membrane of Osteocytes and Osteoclasts in Rat Tibiae. Cell Tissue Res(1995) 280(2):225–33. doi: 10.1007/BF00307793
100. Li Y, Zhong G, Sun W, Zhao C, Zhang P, Song J, et al. CD44 Deficiency Inhibits Unloading-Induced Cortical Bone Loss Through Downregulation of Osteoclast Activity. Sci Rep (2015) 5:1. doi: 10.1038/srep16124
101. Albrecht C, Schlegel W, Eckl P, Jagersberger T, Sadeghi K, Berger A, et al. Alterations in CD44 Isoforms and HAS Expression in Human Articular Chondrocytes During the De- and Re-Differentiation Processes. Int J Mol Med (2009) 23(2):253–9. doi:10.3892/ijmm_00000124
102. Xu Y, Wang YQ, Wang AT, Yu CY, Luo Y, Liu RM, et al. Effect of CD44 on Differentiation of Human Amniotic Mesenchymal Stem Cells Into Chondrocytes via Smad and ERK Signaling Pathways. Mol Med Rep (2020) 21(6):2357. doi: 10.3892/mmr.2020.11044
103. Moraes D. What the Relationship Between CD90 E CD44 in Mesenchymal Stem Cells? Cytotherapy (2018) 20(5):S47. doi: 10.1016/j.jcyt.2018.02.124
104. Hughes DE, Salter DM, Simpson R. CD44 Expression in Human Bone: A Novel Marker of Osteocytic Differentiation. J Bone Mineral Res (1994) 9(1):39–44. doi: 10.1002/jbmr.5650090106
105. Wu SC, Chen CH, Chang JK, Fu YC, Wang CK, Eswaramoorthy R, et al. Hyaluronan Initiates Chondrogenesis Mainly via CD44 in Human Adipose-Derived Stem Cells. J Appl Physiol (2013) 114(11):1610–8. doi: 10.1152/japplphysiol.01132.2012
106. Huynh NP, Zhang B, Guilak F. High-Depth Transcriptomic Profiling Reveals the Temporal Gene Signature of Human Mesenchymal Stem Cells During Chondrogenesis. FASEB J (2019) 33(1):358–72. doi: 10.1096/fj.201800534R
107. Moraes DA, Sibov TT, Pavon LF, Alvim PQ, Bonadio RS, da Silva JR, et al. A Reduction in CD90 (THY-1) Expression Results in Increased Differentiation of Mesenchymal Stromal Cells. Stem Cell Res Ther (2016) 7:1. doi: 10.1186/s13287-016-0359-3
108. Okolicsanyi RK, Camilleri ET, Oikari LE, Yu C, Cool SM, van Wijnen AJ, et al. Human Mesenchymal Stem Cells Retain Multilineage Differentiation Capacity Including Neural Marker Expression After Extended In Vitro Expansion. PloS One (2015) 10(9):e0137255. doi: 10.1371/journal.pone.0137255
109. Liu Y, Han SS, Wu Y, Tuohy TM, Xue H, Cai J. CD44 Expression Identifies Astrocyte-Restricted Precursor Cells. Dev Biol (2004) 276(1):31–46. doi: 10.1016/j.ydbio.2004.08.018
110. Naruse M, Shibasaki K, Yokoyama S, Kurachi M, Ishizaki Y. Dynamic Changes of CD44 Expression From Progenitors to Subpopulations of Astrocytes and Neurons in Developing Cerebellum. PloS One (2013) 8(1):e53109. doi: 10.1371/journal.pone.0053109
111. Jensen T, Vadasz S, Phoenix K, Claffey K, Parikh N, Finck C. Descriptive Analysis of Tumor Cells With Stem Like Phenotypes in Metastatic and Benign Adrenal Tumors. J Pediatr Surg (2015) 50(9):1493–501. doi: 10.1016/j.jpedsurg.2015.04.012
112. Vega FM, Colmenero-Repiso A, Gómez-Muñoz MA, Rodríguez-Prieto I, Aguilar-Morante D, Ramírez G, et al. CD44-High Neural Crest Stem-Like Cells are Associated With Tumour Aggressiveness and Poor Survival in Neuroblastoma Tumours. EBioMedicine (2019) 49:82–95. doi: 10.1016/j.ebiom.2019.10.041
113. Zhu H, Mitsuhashi N, Klein A, Barsky LW, Weinberg K, Barr ML, et al. The Role of the Hyaluronan Receptor CD44 in Mesenchymal Stem Cell Migration in the Extracellular Matrix. Stem Cells (2006) 24(4):928–35. doi: 10.1634/stemcells.2005-0186
114. Zhao P, Damerow MS, Stern P, Liu AH, Sweet-Cordero A, Siziopikou K, et al. CD44 Promotes Kras-Dependent Lung Adenocarcinoma. Oncogene (2013) 32(43):5186–90. doi: 10.1038/onc.2012.542
115. Chanmee T, Ontong P, Kimata K, Itano N. Key Roles of Hyaluronan and its CD44 Receptor in the Stemness and Survival of Cancer Stem Cells. Front Oncol Front Media S.A.; (2015) 5:1. doi: 10.3389/fonc.2015.00180
116. Chanmee T, Ontong P, Mochizuki N, Kongtawelert P, Konno K, Itano N. Excessive Hyaluronan Production Promotes Acquisition of Cancer Stem Cell Signatures Through the Coordinated Regulation of Twist and the Transforming Growth Factor β (TGF-β)-Snail Signaling Axis. J Biol Chem (2014) 289(38):26038–56. doi: 10.1074/jbc.M114.564120
117. Kidan N, Khamaisie H, Ruimi N, Roitman S, Eshel E, Dally N, et al. Ectopic Expression of Snail and Twist in Ph+ Leukemia Cells Upregulates CD44 Expression and Alters Their Differentiation Potential. J Cancer (2017) 8(19):3952–68. doi: 10.7150/jca.19633
118. Guarino M, Rubino B, Ballabio G. The Role of Epithelial-Mesenchymal Transition in Cancer Pathology. Pathol (2007) 39(3):305–18. doi: 10.1080/00313020701329914
119. Emadi Baygi M, Soheili ZS, Schmitz I, Sameie S, Schulz WA. Snail Regulates Cell Survival and Inhibits Cellular Senescence in Human Metastatic Prostate Cancer Cell Lines. Cell Biol Toxicol (2010) 26(6):553–67. doi: 10.1007/s10565-010-9163-5
120. Fontana F, Raimondi M, Marzagalli M, Sommariva M, Limonta P, Gagliano N. Epithelial-To-Mesenchymal Transition Markers and CD44 Isoforms Are Differently Expressed in 2D and 3D Cell Cultures of Prostate Cancer Cells. Cells (2019) 8(2):143. doi: 10.3390/cells8020143
121. Yang J, Mani SA, Donaher JL, Ramaswamy S, Itzykson RA, Come C, et al. Twist, a Master Regulator of Morphogenesis, Plays an Essential Role in Tumor Metastasis. Cell (2004) 117(7):927–39. doi: 10.1016/j.cell.2004.06.006
122. Matsuo N, Shiraha H, Fujikawa T, Takaoka N, Ueda N, Tanaka S, et al. Twist Expression Promotes Migration and Invasion in Hepatocellular Carcinoma. BMC Cancer (2009) 9(1):1–12. doi: 10.1186/1471-2407-9-240
123. Shigeishi H, Biddle A, Gammon L, Emich H, Rodini CO, Gemenetzidis E, et al. Maintenance of Stem Cell Self-Renewal in Head and Neck Cancers Requires Actions of GSK3b Influenced by CD44 and RHAMM. Stem Cells (2013) 31(10):2073–83. doi: 10.1002/stem.1418
124. Marangoni E, Lecomte N, Durand L, de Pinieux G, Decaudin D, Chomienne C, et al. CD44 Targeting Reduces Tumour Growth and Prevents Post-Chemotherapy Relapse of Human Breast Cancers Xenografts. Br J Cancer (2009) 100(6):918–22. doi: 10.1038/sj.bjc.6604953
125. Jin L, Hope KJ, Zhai Q, Smadja-Joffe F, Dick JE. Targeting of CD44 Eradicates Human Acute Myeloid Leukemic Stem Cells. Nat Med (2006) 12(10):1167–74. doi: 10.1038/nm1483
126. Jang YY, Sharkis SJ. A Low Level of Reactive Oxygen Species Selects for Primitive Hematopoietic Stem Cells That may Reside in the Low-Oxygenic Niche. Blood (2007) 110(8):3056–63. doi: 10.1182/blood-2007-05-087759
127. Zhou D, Shao L, Spitz DR. Reactive Oxygen Species in Normal and Tumor Stem Cells. In: Adv Cancer Res (2014) 122:1–67. doi:10.1016/B978-0-12-420117-0.00001-3
128. Mohyeldin A, Garzón-Muvdi T, Quiñones-Hinojosa A. Oxygen in Stem Cell Biology: A Critical Component of the Stem Cell Niche. Cell Stem Cell Cell Stem Cell; (2010) 7:150–61. doi: 10.1016/j.stem.2010.07.007
129. Krishnamachary B, Penet MF, Nimmagadda S, Mironchik Y, Raman V, Solaiyappan M, et al. Hypoxia Regulates CD44 and Its Variant Isoforms Through HIF-1α in Triple Negative Breast Cancer. PloS One (2012) 7(8):e44078. doi: 10.1371/journal.pone.0044078
130. Tamada M, Nagano O, Tateyama S, Ohmura M, Yae T, Ishimoto T, et al. Modulation of Glucose Metabolism by CD44 Contributes to Antioxidant Status and Drug Resistance in Cancer Cells. Cancer Res (2012) 72(6):1438–48. doi: 10.1158/0008-5472.CAN-11-3024
131. Christofk HR, vander Heiden MG, Harris MH, Ramanathan A, Gerszten RE, Wei R, et al. The M2 Splice Isoform of Pyruvate Kinase is Important for Cancer Metabolism and Tumour Growth. Nat (2008) 452(7184):230–3. doi: 10.1038/nature06734
132. Tamada M, Suematsu M, Saya H. Pyruvate Kinase M2: Multiple Faces for Conferring Benefits on Cancer Cells. Clin Cancer Res Clin Cancer Res; (2012) 18:5554–61. doi: 10.1158/1078-0432.CCR-12-0859
133. Ishimoto T, Nagano O, Yae T, Tamada M, Motohara T, Oshima H, et al. CD44 Variant Regulates Redox Status in Cancer Cells by Stabilizing the xCT Subunit of System Xc- and Thereby Promotes Tumor Growth. Cancer Cell (2011) 19(3):387–400. doi: 10.1016/j.ccr.2011.01.038
134. Shitara K, Doi T, Nagano O, Imamura CK, Ozeki T, Ishii Y, et al. Dose-Escalation Study for the Targeting of CD44v+ Cancer Stem Cells by Sulfasalazine in Patients With Advanced Gastric Cancer (EPOC1205). Gastric Cancer (2017) 20(2):341–9. doi: 10.1007/s10120-016-0610-8
135. Kobayashi H, Suzuki M, Kanayama N, Nishida T, Takigawa M, Terao T. CD44 Stimulation by Fragmented Hyaluronic Acid Induces Upregulation of Urokinase-Type Plasminogen Activator and its Receptor and Subsequently Facilitates Invasion of Human Chondrosarcoma Cells. Int J Cancer. (2002) 102(4):379–89. doi: 10.1002/ijc.10710
136. Xiao Z, Wan J, Nur AA, Dou P, Mankin H, Liu T, et al. Targeting CD44 by CRISPR-Cas9 in Multi-Drug Resistant Osteosarcoma Cells. Cell Physiol Biochem (2018) 51(4):1879–93. doi: 10.1159/000495714
137. Liu T, Yan Z, Liu Y, Choy E, Hornicek FJ, Mankin H, et al. CRISPR-Cas9-Mediated Silencing of CD44 in Human Highly Metastatic Osteosarcoma Cells. Cell Physiol Biochem (2018) 46(3):1218–30. doi: 10.1159/000489072
138. Kong L, Ji H, Gan X, Cao S, Li Z, Jin Y. Knockdown of CD44 Inhibits Proliferation, Migration and Invasion of Osteosarcoma Cells Accompanied by Downregulation of Cathepsin s. J Orthopaedic Surg Res (2022) 17(1):154. doi: 10.1186/s13018-022-03048-x
139. Gvozdenovic A, Arlt MJE, Campanile C, Brennecke P, Husmann K, Born W, et al. Silencing of CD44 Gene Expression in Human 143-B Osteosarcoma Cells Promotes Metastasis of Intratibial Tumors in SCID Mice. PloS One (2013) 8(4):e60329. doi: 10.1371/journal.pone.0060329
140. Rhim JS, Cho HY, Huebner RJ. Non-Producer Human Cells Induced by Murine Sarcoma Virus. Int J Cancer (1975) 15(1):23–9. doi: 10.1002/ijc.2910150104
141. Morrow KA, Das S, Metge BJ, Ye K, Mulekar MS, Tucker JA, et al. Loss of Tumor Suppressor Merlin in Advanced Breast Cancer is Due to Post-Translational Regulation. J Biol Chem (2011) 286(46):40376–85. doi: 10.1074/jbc.M111.250035
142. Subramaniam D, Angulo P, Ponnurangam S, Dandawate P, Ramamoorthy P, Srinivasan P, et al. Suppressing STAT5 Signaling Affects Osteosarcoma Growth and Stemness. Cell Death Dis (2020) 11(2):1–15. doi: 10.1038/s41419-020-2335-1
143. Zhao H, Ma B, Wang Y, Han T, Zheng L, Sun C, et al. miR-34a Inhibits the Metastasis of Osteosarcoma Cells by Repressing the Expression of CD44. Oncol Rep (2013) 29(3):1027–36. doi: 10.3892/or.2013.2234
144. Gao Y, Feng Y, Shen JK, Lin M, Choy E, Cote GM, et al. CD44 is a Direct Target of miR-199a-3p and Contributes to Aggressive Progression in Osteosarcoma. Sci Rep (2015) 5:1–9. doi: 10.1038/srep11365
145. Liao S, Zhou S, Wang C. GAPLINC is a Predictor of Poor Prognosis and Regulates Cell Migration and Invasion in Osteosarcoma. Biosci Rep (2018) 38(5): BSR20181171. doi: 10.1042/BSR20181171
146. Grünewald TGP, Cidre-Aranaz F, Surdez D, Tomazou EM, de Álava E, Kovar H, et al. Ewing Sarcoma. Nat Rev Dis Primers (2018) 4(1): 5. doi: 10.1038/s41572-018-0003-x
147. Tirode F, Laud-Duval K, Prieur A, Delorme B, Charbord P, Delattre O. Mesenchymal Stem Cell Features of Ewing Tumors. Cancer Cell (2007) 11(5):421–9. doi: 10.1016/j.ccr.2007.02.027
148. Paulis YWJ, Huijbers EJM, van der Schaft DWJ, Soetekouw PMMB, Pauwels P, Tjan-Heijnen VCG, et al. CD44 Enhances Tumor Aggressiveness by Promoting Tumor Cell Plasticity. Oncotarget (2015) 6(23):19634–46. doi: 10.18632/oncotarget.3839
149. Van Der Schaft DW, Hillen F, Pauwels P, Kirschmann DA, Castermans K, oude Egbrink MGA, et al. Tumor Cell Plasticity in Ewing Sarcoma, an Alternative Circulatory System Stimulated by Hypoxia. Cancer Res (2005) 65(24):11520–8. doi: 10.1158/0008-5472.CAN-05-2468
150. Kouvidi K, Berdiaki A, Nikitovic D, Katonis P, Afratis N, Hascall VC, et al. Role of Receptor for Hyaluronic Acid-Mediated Motility (RHAMM) in Low Molecular Weight Hyaluronan (LMWHA)-Mediated Fibrosarcoma Cell Adhesion. J Biol Chem (2011) 286(44):38509. doi: 10.1074/jbc.M111.275875
151. Hatano H, Shigeishi H, Kudo Y, Higashikawa K, Tobiume K, Takata T, et al. RHAMM/ERK Interaction Induces Proliferative Activities of Cementifying Fibroma Cells Through a Mechanism Based on the CD44–EGFR. Lab Invest (2011) 91:3. doi: 10.1038/labinvest.2010.176
152. Kogerman P, Sy MS, Culp LA. Counter-Selection for Over-Expressed Human CD44s in Primary Tumors Versus Lung Metastases in a Mouse Fibrosarcoma Model. Oncogene (1997) 15(12):1407–16. doi: 10.1038/sj.onc.1201306
153. Henderson T, Chen M, Darrow MA, Li CS, Chiu CL, Monjazeb AM, et al. Alterations in Cancer Stem-Cell Marker CD44 Expression Predict Oncologic Outcome in Soft-Tissue Sarcomas. J Surg Res (2018) 223:207–14. doi: 10.1016/j.jss.2017.11.016
154. Kahara N, Ozaki T, Doi T, Nishida K, Kawai A, Shibahara M, et al. CD44 Expression in Soft Tissue Sarcomas. Virchows Archiv. (2000) 436(6):574–8. doi: 10.1007/s004289900159
155. Kebudi R, Ayan I, Yasasever V, Demokan S, Görgün Ö. Are Serum Levels of CD44 Relevant in Children With Pediatric Sarcomas? Pediatr Blood Cancer. (2006) 46(1):62–5. doi: 10.1002/pbc.20554
156. Matuschek C, Lehnhardt M, Gerber PA, Poremba C, Hamilton J, Lammering G, et al. Increased CD44s and Decreased CD44v6 RNA Expression are Associated With Better Survival in Myxofibrosarcoma Patients: A Pilot Study. Eur J Med Res (2014) 19(1):1–7. doi: 10.1186/2047-783X-19-6
157. Tsuchie H, Emori M, Miyakoshi N, Nagasawa H, Okada K, Nanjyo H, et al. Prognostic Impact of CD44 Expression in Patients With Myxofibrosarcoma. In Vivo. (2019) 33(6):2095–102. doi: 10.21873/invivo.11709
158. Fligman I, Lonardo F, Jhanwar SC, Gerald WL, Woodruff J, Ladanyi M. Molecular Diagnosis of Synovial Sarcoma and Characterization of a Variant SYT-SSX2 Fusion Transcript. Am J Pathol (1995) 147(6):1592–9.
159. Sneath RJ, Mangham DC. CD44 Isoform Expression in Synovial Sarcoma Correlates With Epitheliogenesis But Not Prognosis. Histopathology (2000) 37(2):166–74. doi: 10.1046/j.1365-2559.2000.00953.x
160. Zhou Y, Chen D, Qi Y, Liu R, Li S, Zou H, et al. Evaluation of Expression of Cancer Stem Cell Markers and Fusion Gene in Synovial Sarcoma: Insights Into Histogenesis and Pathogenesis. Oncol Rep (2017) 37(6):3351–60. doi: 10.3892/or.2017.5617
161. Parham DM, Barr FG. Classification of Rhabdomyosarcoma and its Molecular Basis. Adv Anatomic Pathol Adv Anat Pathol; (2013) 20:387–97. doi: 10.1097/PAP.0b013e3182a92d0d
162. Humphrey G, Hazel DL, MacLennan K, Lewis I. Expression of CD44 by Rhabdomyosarcoma: A New Prognostic Marker? Br J Cancer. (1999) 80(5–6):918–21. doi: 10.1038/sj.bjc.6690442
163. Saxon BR, Byard RW, Han P. Cellular Expression of Adhesion Factors in Childhood Rhabdomyosarcoma. Pediatr Pathol Lab Med (1997) 17(2):259–66. doi: 10.1080/15513819709168570
164. Heyse TJ, Malcherczyk D, Moll R, Timmesfeld N, Wapelhorst J, Fuchs-Winkelmann S, et al. CD44: Survival and Metastasis in Chondrosarcoma. Osteoarthr Cartil (2010) 18(6):849–56. doi: 10.1016/j.joca.2010.02.007
165. Rozeman LB, de Bruijn IHB, Bacchini P, Staals EL, Bertoni F, Bovée JVMG, et al. Dedifferentiated Peripheral Chondrosarcomas: Regulation of EXT-Downstream Molecules and Differentiation-Related Genes. Modern Pathol (2009) 22(11):1489–98. doi: 10.1038/modpathol.2009.120
166. Misaghi A, Goldin A, Awad M, Kulidjian AA. Osteosarcoma: A Comprehensive Review. SICOT J (2018) 4:12. doi: 10.1051/sicotj/2017028
167. Mankin HJ, Hornicek FJ, Rosenberg AE, Harmon DC, Gebhardt MC. Survival Data for 648 Patients With Osteosarcoma Treated at One Institution. Clin Orthopaedics Related Res (2004) 429):286–91. doi: 10.1097/01.blo.0000145991.65770.e6
168. Liu Y, Wu Y, Gu S, Sun Z, Rui Y, Wang J, et al. Prognostic Role of CD44 Expression in Osteosarcoma: Evidence From Six Studies. Diagn Pathol (2014) 9(1):1–5. doi: 10.1186/1746-1596-9-140
169. Boldrini E, Peres SV, Morini S, de Camargo B. Immunoexpression of Ezrin and CD44 in Patients With Osteosarcoma. J Pediatr Hematology/Oncology. (2010) 32(6):e213–7. doi: 10.1097/MPH.0b013e3181e5e247
170. Gvozdenovic A, Arlt MJE, Campanile C, Brennecke P, Husmann K, Li Y, et al. CD44 Enhances Tumor Formation and Lung Metastasis in Experimental Osteosarcoma and is an Additional Predictor for Poor Patient Outcome. J Bone Mineral Res (2013) 28(4):838–47. doi: 10.1002/jbmr.1817
171. Kuryu M, Ozaki T, Nishida K, Shibahara M, Kawai A, Inoue H. Expression of CD44 Variants in Osteosarcoma. J Cancer Res Clin Oncol (1999) 125(11):646–52. doi: 10.1007/s004320050329
172. Deng Z, Niu G, Cai L, Wei R, Zhao X. The Prognostic Significance of CD44V6, CDH11, and β -Catenin Expression in Patients With Osteosarcoma. BioMed Res Int (2013) 2013:9. doi: 10.1155/2013/496193
173. Tirino V, Desiderio V, Paino F, de Rosa A, Papaccio F, Fazioli F, et al. Human Primary Bone Sarcomas Contain CD133 + Cancer Stem Cells Displaying High Tumorigenicity In Vivo. FASEB J (2011) 25(6):2022–30. doi: 10.1096/fj.10-179036
174. He A, Yang X, Huang Y, Feng T, Wang Y, Sun Y, et al. CD133+CD44+ Cells Mediate in the Lung Metastasis of Osteosarcoma. J Cell Biochem (2015) 116(8):1719–29. doi: 10.1002/jcb.25131
175. Kim CK, Oh S, Kim SJ, Leem SH, Heo J, Chung SH. Correlation of IGF1R Expression With ABCG2 and CD44 Expressions in Human Osteosarcoma. Genes Genomics (2018) 40(4):381–8. doi: 10.1007/s13258-017-0639-z
176. Zhang Y, Ding C, Wang J, Sun G, Cao Y, Xu L, et al. Prognostic Significance of CD44V6 Expression in Osteosarcoma: A Meta-Analysis. J Orthop Surg Res (2015) 10:187. doi: 10.1186/s13018-015-0328-z
177. Zhang Y, Lun L, Zhu B, Wang Q, Ding C, Hu Y, et al. Diagnostic Accuracy of CD44V6 for Osteosarcoma: A Meta-Analysis. J Orthopaedic Surg Res (2016) 11(1):1–9. doi: 10.1186/s13018-016-0470-2
178. Martin TA, Harrison G, Mansel RE, Jiang WG. The Role of the CD44/ezrin Complex in Cancer Metastasis. Crit Rev Oncol Hematol (2003) 46(2):165–86. doi: 10.1016/s1040-8428(02)00172-5.
179. Castellà EM, Ariza A, Pellicer I, Fernández-Vasalo A, Ojanguren I. Differential Expression of CD44v6 in Metastases of Intestinal and Diffuse Types of Gastric Carcinoma. J Clin Pathol (1998) 51(2):134–7. doi: 10.1136/jcp.51.2.134
180. Zhao S, He JL, Qiu ZX, Chen NY, Luo Z, Chen BJ, et al. Prognostic Value of CD44 Variant Exon 6 Expression in non-Small Cell Lung Cancer: A Meta-Analysis. Asian Pacific J Cancer Prev (2014) 15(16):6761–6. doi: 10.7314/APJCP.2014.15.16.6761
181. Tran TA, Kallakury BVS, Sheehan CE, Ross JS. Expression of CD44 Standard Form and Variant Isoforms in non-Small Cell Lung Carcinomas. Hum Pathol (1997) 28(7):809–14. doi: 10.1016/S0046-8177(97)90154-4
182. Endo K, Terada T. Protein Expression of CD44 (Standard and Variant Isoforms) in Hepatocellular Carcinoma: Relationships With Tumor Grade, Clinicopathologic Parameters, P53 Expression, and Patient Survival. J Hepatol (2000) 32(1):78–84. doi: 10.1016/S0168-8278(00)80192-0
183. Legras S, Günthert U, Stauder R, Curt F, Oliferenko S, Kluin-Nelemans HC, et al. A Strong Expression of CD44-6v Correlates With Shorter Survival of Patients With Acute Myeloid Leukemia. Blood (1998) 91(9):3401–13. doi: 10.1182/blood.V91.9.3401
184. Stauder R, Eisterer W, Thaler J, Günthert U. CD44 Variant Isoforms in non-Hodgkin’s Lymphoma: A New Independent Prognostic Factor. Blood (1995) 85(10):2885–99. doi: 10.1182/blood.V85.10.2885.bloodjournal85102885
185. Ristamäki R, Joensuu H, Söderström K -O, Jalkanen S. CD44v6 Expression in non-Hodgkin’s Lymphoma: An Association With Low Histological Grade and Poor Prognosis. J Pathol (1995) 176(3):259–67. doi: 10.1002/path.1711760308
186. Gansauge F, Gansauge S, Zobywalski A, Scharnweber C, Link KH, Nussler AK, et al. Differential Expression of CD44 Splice Variants in Human Pancreatic Adenocarcinoma and in Normal Pancreas. Cancer Res (1995) 55(23):5499–503.
187. Gotoda T, Matsumura Y, Kondo H, Saitoh D, Shimada Y, Kosuge T, et al. Expression of CD44 Variants and its Association With Survival in Pancreatic Cancer. Jpn J Cancer Res (1998) 89(10):1033–40. doi: 10.1111/j.1349-7006.1998.tb00493.x
188. Ayhan A, Baykal C, Al A, Ayhan A. Altered CD44 Variant 6 Expression in FIGO Stage IB Cervical Carcinoma. Gynecologic Oncol (2001) 83(3):569–74. doi: 10.1006/gyno.2001.6406
189. Cao Y, Roth M, Piperdi S, Montoya K, Sowers R, Rao P, et al. Insulin-Like Growth Factor 1 Receptor and Response to Anti-IGF1R Antibody Therapy in Osteosarcoma. PloS One (2014) 9(8):e106249. doi: 10.1371/journal.pone.0106249
190. Handorean AM, Yang K, Robbins EW, Flaig TW, Iczkowski KA. Silibinin Suppresses CD44 Expression in Prostate Cancer Cells. Am J Transl Res (2009) 1(1):80–6.
191. Nambiar D, Prajapati V, Agarwal R, Singh RP. In Vitro and In Vivo Anticancer Efficacy of Silibinin Against Human Pancreatic Cancer BxPC-3 and PANC-1 Cells. Cancer Lett (2013) 334(1):109–17. doi: 10.1016/j.canlet.2012.09.004
192. Kim S, Kil WH, Lee J, Oh SJ, Han J, Jeon M, et al. Zerumbone Suppresses EGF-Induced CD44 Expression Through the Inhibition of STAT3 in Breast Cancer Cells. Oncol Rep (2014) 32(6):2666–72. doi: 10.3892/or.2014.3514
193. Chung SS, Vadgama JV. Curcumin and Epigallocatechin Gallate Inhibit the Cancer Stem Cell Phenotype via Down-Regulation of STAT3-Nfκb Signaling. Anticancer Res (2015) 35(1):39–46.
194. Kummar S, Coyne GOS, Do KT, Turkbey B, Meltzer PS, Polley E, et al. Clinical Activity of the γ-Secretase Inhibitor PF-03084014 in Adults With Desmoid Tumors (Aggressive Fibromatosis). J Clin Oncol (2017) 35(14):1561–9. doi: 10.1200/JCO.2016.71.1994
195. Massard C, Azaro A, Soria JC, Lassen U, le Tourneau C, Sarker D, et al. First-In-Human Study of LY3039478, an Oral Notch Signaling Inhibitor in Advanced or Metastatic Cancer. Ann Oncol (2018) 29(9):1911–7. doi: 10.1093/annonc/mdy244
196. Morgan KM, Fischer BS, Lee FY, Shah JJ, Bertino JR, Rosenfeld J, et al. Gamma Secretase Inhibition by BMS-906024 Enhances Efficacy of Paclitaxel in Lung Adenocarcinoma. Mol Cancer Ther (2017) 16(12):2759–69. doi: 10.1158/1535-7163.MCT-17-0439
197. Menke-van der Houven van Oordt CW, Gomez-Roca C, van Herpen C, Coveler AL, Mahalingam D, Verheul HMW, et al. First-In-Human Phase I Clinical Trial of RG7356, an Anti-CD44 Humanized Antibody, in Patients With Advanced, CD44-Expressing Solid Tumors. . Oncotarget (2016) 7(48):80046–58. doi: 10.18632/oncotarget.11098
198. Vey N, Delaunay J, Martinelli G, Fiedler W, Raffoux E, Prebet T, et al. Phase I Clinical Study of RG7356, an Anti-CD44 Humanized Antibody, in Patients With Acute Myeloid Leukemia. Oncotarget (2016) 7(22):32532–42. doi: 10.18632/oncotarget.8687
199. Sandström K, Nestor M, Ekberg T, Engström M, Anniko M, Lundqvist H. Targeting CD44v6 Expressed in Head and Neck Squamous Cell Carcinoma: Preclinical Characterization of an 111In-Labeled Monoclonal Antibody. Tumor Biol (2008) 29(3):137–44. doi: 10.1159/000143399
200. Riechelmann H, Sauter A, Golze W, Hanft G, Schroen C, Hoermann K, et al. Phase I Trial With the CD44v6-Targeting Immunoconjugate Bivatuzumab Mertansine in Head and Neck Squamous Cell Carcinoma. Oral Oncol (2008) 44(9):823–9. doi: 10.1016/j.oraloncology.2007.10.009
201. Tijink BM, Buter J, de Bree R, Giaccone G, Lang MS, Staab A, et al. A Phase I Dose Escalation Study With Anti-CD44v6 Bivatuzumab Mertansine in Patients With Incurable Squamous Cell Carcinoma of the Head and Neck or Esophagus. Clin Cancer Res (2006) 12(20 PART 1):6064–72. doi: 10.1158/1078-0432.CCR-06-0910
202. Li L, Hao X, Qin J, Tang W, He F, Smith A, et al. Antibody Against CD44s Inhibits Pancreatic Tumor Initiation and Postradiation Recurrence in Mice. Gastroenterol (2014) 146(4):1108–1118.e12. doi: 10.1053/j.gastro.2013.12.035
203. Murphy JF, Lennon F, Steele C, Kelleher D, Fitzgerald D, Long A. Engagement of CD44 Modulates Cyclooxygenase Induction, VEGF Generation, and Cell Proliferation in Human Vascular Endothelial Cells. FASEB J (2005) 19(3):1–17. doi: 10.1096/fj.03-1376fje
204. Uchino M, Kojima H, Wada K, Imada M, Onoda F, Satofuka H, et al. Nuclear β-Catenin and CD44 Upregulation Characterize Invasive Cell Populations in non-Aggressive MCF-7 Breast Cancer Cells. BMC Cancer (2010) 10:414. doi: 10.1186/1471-2407-10-414
205. Khurana SS, Riehl TE, Moore BD, Fassan M, Rugge M, Romero-Gallo J, et al. The Hyaluronic Acid Receptor CD44 Coordinates Normal and Metaplastic Gastric Epithelial Progenitor Cell Proliferation. J Biol Chem (2013) 288(22):16085–97. doi: 10.1074/jbc.M112.445551
206. Shukeir N, Arakelian A, Chen G, Garde S, Ruiz M, Panchal C, et al. A Synthetic 15-Mer Peptide (PCK3145) Derived From Prostate Secretory Protein can Reduce Tumor Growth, Experimental Skeletal Metastases, and Malignancy-Associated Hypercalcemia. Cancer Res (2004) 64(15):5370–7. doi: 10.1158/0008-5472.CAN-04-0788
207. Lamy S, Ruiz MT, Wisniewski J, Garde S, Rabbani SA, Panchal C, et al. A Prostate Secretory Protein94-Derived Synthetic Peptide PCK3145 Inhibits VEGF Signalling in Endothelial Cells: Implication in Tumor Angiogenesis. Int J Cancer (2006) . 118(9):2350–8. doi: 10.1002/ijc.21615
208. Annabi B, Bouzeghrane M, Currie JC, Hawkins R, Dulude H, Daigneault L, et al. A PSP94-Derived Peptide PCK3145 Inhibits MMP-9 Secretion and Triggers CD44 Cell Surface Shedding: Implication in Tumor Metastasis. Clin Exp Metastasis (2005) 22(5):429–39. doi: 10.1007/s10585-005-2669-1
209. Alshaer W, Hillaireau H, Vergnaud J, Ismail S, Fattal E. Functionalizing Liposomes With Anti-CD44 Aptamer for Selective Targeting of Cancer Cells. Bioconjugate Chem (2015) 26(7):1307–13. doi: 10.1021/bc5004313
210. Zheng J, Zhao S, Yu X, Huang S, Liu HY. Simultaneous Targeting of CD44 and EpCAM With a Bispecific Aptamer Effectively Inhibits Intraperitoneal Ovarian Cancer Growth. Theranostics (2017) 7(5):1373–88. doi: 10.7150/thno.17826
211. Chi Y, Yin X, Sun K, Feng S, Liu J, Chen D, et al. Redox-Sensitive and Hyaluronic Acid Functionalized Liposomes for Cytoplasmic Drug Delivery to Osteosarcoma in Animal Models. J Controlled Release. (2017) 261:113–25. doi: 10.1016/j.jconrel.2017.06.027
212. Gazzano E, Buondonno I, Marengo A, Rolando B, Chegaev K, Kopecka J, et al. Hyaluronated Liposomes Containing H2S-Releasing Doxorubicin are Effective Against P-Glycoprotein-Positive/Doxorubicin-Resistant Osteosarcoma Cells and Xenografts. Cancer Letters. (2019) 456:29–39. doi: 10.1016/j.canlet.2019.04.029
213. Feng S, Wu ZX, Zhao Z, Liu J, Sun K, Guo C, et al. Engineering of Bone- and CD44-Dual-Targeting Redox-Sensitive Liposomes for the Treatment of Orthotopic Osteosarcoma. ACS Appl Mater Interfaces (2019) 11(7):7357–68. doi: 10.1021/acsami.8b18820
214. Gao S, Islam R, Fang J. Tumor Environment-Responsive Hyaluronan Conjugated Zinc Protoporphyrin for Targeted Anticancer Photodynamic Therapy. J Pers Med (2021) 11(2):1–15. doi: 10.3390/jpm11020136
215. Xi Y, Jiang T, Yu Y, Yu J, Xue M, Xu N, et al. Dual Targeting Curcumin Loaded Alendronate-Hyaluronan- Octadecanoic Acid Micelles for Improving Osteosarcoma Therapy. Int J Nanomedicine (2019) 14:6425. doi: 10.2147/IJN.S211981
216. Hosono K, Nishida Y, Knudson W, Knudson CB, Naruse T, Suzuki Y, et al. Hyaluronan Oligosaccharides Inhibit Tumorigenicity of Osteosarcoma Cell Lines MG-63 and LM-8 In Vitro and In Vivo via Perturbation of Hyaluronan-Rich Pericellular Matrix of the Cells. Am J Pathol (2007) 171(1):274–86. doi: 10.2353/ajpath.2007.060828
217. Gilg AG, Tye SL, Tolliver LB, Wheeler WG, Visconti RP, Duncan JD, et al. Targeting hyaluronan interactions in malignant gliomas and their drug-resistant multipotent progenitors. Clin Cancer Res (2008) 14(6):1804–13. doi: 10.1158/1078-0432.CCR-07-1228
218. Hu Y, Fu L. Targeting Cancer Stem Cells: A New Therapy to Cure Cancer Patients. Am J Cancer Res (2012) 2(3):340–56.
219. Xia W. γ-Secretase and its Modulators: Twenty Years and Beyond. Neurosci Letters. Elsevier Ireland Ltd; (2019) 701:162–9. doi: 10.1016/j.neulet.2019.02.011
220. Ran Y, Hossain F, Pannuti A, Lessard CB, Ladd GZ, Jung JI, et al. γ-Secretase Inhibitors in Cancer Clinical Trials are Pharmacologically and Functionally Distinct. EMBO Mol Med (2017) 9(7):950–66. doi: 10.15252/emmm.201607265
221. Orian-Rousseau V, Ponta H. Perspectives of CD44 Targeting Therapies. Arch Toxicol (2015) 89(1):3–14. doi:10.1007/s00204-014-1424-2
222. Dalla Pozza E, Lerda C, Costanzo C, Donadelli M, Dando I, Zoratti E, et al. Targeting Gemcitabine Containing Liposomes to CD44 Expressing Pancreatic Adenocarcinoma Cells Causes an Increase in the Antitumoral Activity. Biochim Biophys Acta - Biomembranes (2013) 1828(5):1396–404. doi: 10.1016/j.bbamem.2013.01.020
223. Peer D, Margalit R. Loading Mitomycin C Inside Long Circulating Hyaluronan Targeted Nano-Liposomes Increases its Antitumor Activity in Three Mice Tumor Models. Int J Cancer (2004) 108(5):780–9. doi: 10.1002/ijc.11615
224. Peer D, Margalit R. Tumor-Targeted Hyaluronan Nanoliposomes Increase the Antitumor Activity of Liposomal Doxorubicin in Syngeneic and Human Xenograft Mouse Tumor Models. Neoplasia (2004) 6(4):343–53. doi: 10.1593/neo.03460
225. Cheng D, Cao N, Chen J, Yu X, Shuai X. Multifunctional Nanocarrier Mediated Co-Delivery of Doxorubicin and siRNA for Synergistic Enhancement of Glioma Apoptosis in Rat. Biomater (2012) 33(4):1170–9. doi: 10.1016/j.biomaterials.2011.10.057
226. Šmejkalová D, Nešporová K, Huerta-Angeles G, Syrovátka J, Jirák D, Gálisová A, et al. Selective In Vitro Anticancer Effect of Superparamagnetic Iron Oxide Nanoparticles Loaded in Hyaluronan Polymeric Micelles. Biomacromolecules (2014) 15(11):4012–20. doi: 10.1021/bm501065q
227. Wickens JM, Alsaab HO, Kesharwani P, Bhise K, Amin MCIM, Tekade RK, et al. Recent Advances in Hyaluronic Acid-Decorated Nanocarriers for Targeted Cancer Therapy. Drug Discovery Today (2017) 22(4):665–80. doi: 10.1016/j.drudis.2016.12.009
228. Cai J, Fu J, Li R, Zhang F, Ling G, Zhang P. A Potential Carrier for Anti-Tumor Targeted Delivery-Hyaluronic Acid Nanoparticles. Carbohydr Polym (2019) 208:356–64. doi: 10.1016/j.carbpol.2018.12.074
229. Yin T, Wang L, Yin L, Zhou J, Huo M. Co-Delivery of Hydrophobic Paclitaxel and Hydrophilic AURKA Specific siRNA by Redox-Sensitive Micelles for Effective Treatment of Breast Cancer. Biomater (2015) 61:10–25. doi: 10.1016/j.biomaterials.2015.05.022
230. Yang X, Iyer AK, Singh A, Milane L, Choy E, Hornicek FJ, et al. Cluster of Differentiation 44 Targeted Hyaluronic Acid Based Nanoparticles for MDR1 siRNA Delivery to Overcome Drug Resistance in Ovarian Cancer. Pharm Res (2015) 32(6):2097–109. doi: 10.1007/s11095-014-1602-1
231. Yang X, Lyer AK, Singh A, Choy E, Hornicek FJ, Amiji MM, et al. MDR1 siRNA Loaded Hyaluronic Acid-Based CD44 Targeted Nanoparticle Systems Circumvent Paclitaxel Resistance in Ovarian Cancer. Sci Rep (2015) 5:1–9. doi: 10.1038/srep08509
232. Ahmed M, Narain R. Carbohydrate-Based Materials for Targeted Delivery of Drugs and Genes to the Liver. Nanomedicine (Lond) (2015) 10(14):2263–88. doi: 10.2217/nnm.15.58
233. Wang Y, Du H, Zhai G. Recent Advances in Active Hepatic Targeting Drug Delivery System. Curr Drug Targets (2014) 15(6):573–99. doi: 10.2174/1389450115666140309232100
234. Liu Y, Sun J, Cao W, Yang J, Lian H, Li X, et al. Dual Targeting Folate-Conjugated Hyaluronic Acid Polymeric Micelles for Paclitaxel Delivery. Int J Pharm (2011) 421(1):160–9. doi: 10.1016/j.ijpharm.2011.09.006
235. Chen F, Zeng Y, Qi X, Chen Y, Ge Z, Jiang Z, et al. Targeted Salinomycin Delivery With EGFR and CD133 Aptamers Based Dual-Ligand Lipid-Polymer Nanoparticles to Both Osteosarcoma Cells and Cancer Stem Cells. Nanomedicine (2018) 14(7):2115–27. doi: 10.1016/j.nano.2018.05.015
236. Ahrens T, Sleeman JP, Schempp CM, Howells N, Hofmann M, Ponta H, et al. Soluble CD44 Inhibits Melanoma Tumor Growth by Blocking Cell Surface CD44 Binding to Hyaluronic Acid. Oncogene (2001) 20(26):3399–408. doi: 10.1038/sj.onc.1204435
237. Toole BP, Slomiany MG. Hyaluronan, CD44 and Emmprin: Partners in Cancer Cell Chemoresistance. Drug Resist Updates (2008) 11(3):110–21. doi: 10.1016/j.drup.2008.04.002
238. Toole B, Ghatak S, Misra S. Hyaluronan Oligosaccharides as a Potential Anticancer Therapeutic. Curr Pharm Biotechnol (2008) 9(4):249–52. doi: 10.2174/138920108785161569
239. Lesley J, Hascall VC, Tammi M, Hyman R. Hyaluronan Binding by Cell Surface CD44. J Biol Chem (2000) 275(35):26967–75. doi: 10.1016/S0021-9258(19)61467-5
240. Underhill CB, Toole BP. Binding of Hyaluronate to the Surface of Cultured Cells. J Cell Biol (1979) 82(2):475–84. doi: 10.1083/jcb.82.2.475
241. Slomiany MG, Dai L, Bomar PA, Knackstedt TJ, Kranc DA, Tolliver L, et al. Abrogating Drug Resistance in Malignant Peripheral Nerve Sheath Tumors by Disrupting Hyaluronan-CD44 Interactions With Small Hyaluronan Oligosaccharides. Cancer Res (2009) 69(12):4992–8. doi: 10.1158/0008-5472.CAN-09-0143
Keywords: CD44, sarcoma, cancer therapy, biomarker, CD44-ICD, signalling transduction, epithelial mesenchymal transition (EMT), extracellular matrix (ECM)
Citation: Fernández-Tabanera E, Melero-Fernández de Mera RM and Alonso J (2022) CD44 In Sarcomas: A Comprehensive Review and Future Perspectives. Front. Oncol. 12:909450. doi: 10.3389/fonc.2022.909450
Received: 31 March 2022; Accepted: 02 May 2022;
Published: 17 June 2022.
Edited by:
Alberto Passi, University of Insubria, ItalyReviewed by:
Alessandro De Vita, Scientific Institute of Romagna for the Study and Treatment of Tumors (IRCCS), ItalyHashem Obaid Alsaab, Taif University, Saudi Arabia
Copyright © 2022 Fernández-Tabanera, Melero-Fernández de Mera and Alonso. This is an open-access article distributed under the terms of the Creative Commons Attribution License (CC BY). The use, distribution or reproduction in other forums is permitted, provided the original author(s) and the copyright owner(s) are credited and that the original publication in this journal is cited, in accordance with accepted academic practice. No use, distribution or reproduction is permitted which does not comply with these terms.
*Correspondence: Javier Alonso, ZmphbG9uc29AaXNjaWlpLmVz