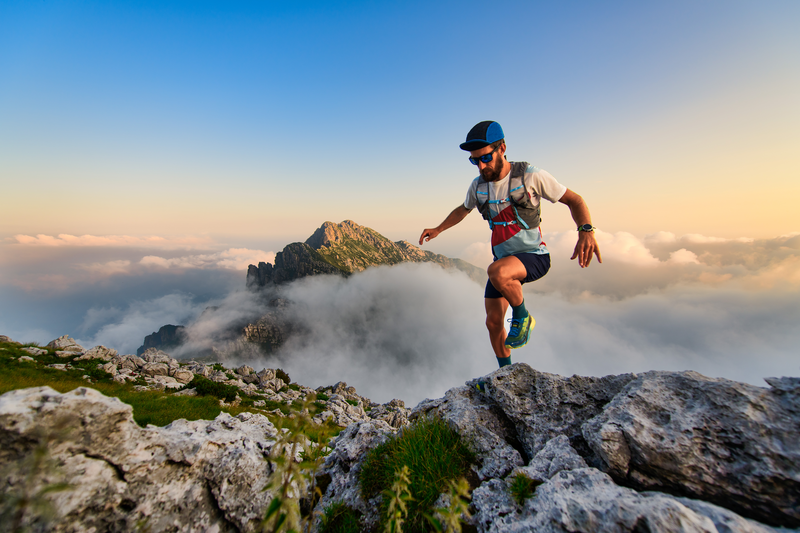
94% of researchers rate our articles as excellent or good
Learn more about the work of our research integrity team to safeguard the quality of each article we publish.
Find out more
ORIGINAL RESEARCH article
Front. Oncol. , 21 June 2022
Sec. Gynecological Oncology
Volume 12 - 2022 | https://doi.org/10.3389/fonc.2022.905900
This article is part of the Research Topic Women in Gynecological Oncology, volume II, 2022 View all 11 articles
hnRNP E1 (heterogeneous nuclear ribonucleoprotein E1) is an important RNA-binding protein (RBPs) that plays a vital role in tumor development. Human papillomavirus 16 (HPV16) contains numerous sites that can bind to RNA/DNA and may be modified by multiple RBPs, which contribute to HPV gene expression and HPV-associated cancer development. However, the effects of hnRNP E1 on HPV16 oncogenes in the development of cervical lesions remain unclear. A total of 816 participants with different grades of cervical lesions were enrolled in a community-based cohort established in Shanxi Province, China. The Gene Expression Omnibus (GEO) and The Cancer Genome Atlas (TCGA) databases were used to analyze the association between hnRNP E1 mRNA expression and cervical lesions. Cells with up_ and down_regulated hnRNP E1 were established. hnRNP E1 functions were evaluated using cell counting kit-8, flow cytometry analyses, and chromatin immunoprecipitation sequencing. Our results showed that hnRNP E1 expression was linearly dependent on the severity of the cervical lesions. Low expression of HPV16 E2, high expression of E6, and a low ratio of E2 to E6 could increase the risk of cervical lesions. hnRNP E1 expression was correlated with HPV16 oncogene expression. hnRNP E1-relevant genes were involved in the dopaminergic synapses, Wnt signaling pathway, gnRH secretion, and mTOR signaling pathway. hnRNP E1 significantly inhibited cell proliferation, induced apoptosis, arrested the cell cycle at the G0/G1 stage, and decreased HPV16 E6 expression. Our results indicate that hnRNP E1 could downregulate HPV16 E6 oncogene expression and inhibit cervical cancerization, which sheds new light on preventing the carcinogenicity of HPV across a range of diseases by regulating RNA-binding proteins.
Cervical cancer is the fourth most frequently diagnosed cancer in women globally (1). In contrast to the downward trends in developed countries, the incidence of cervical cancer in China has increased significantly. It is estimated that by 2020, there will be 0.11 million new cases and 0.06 million deaths in China (2). Shanxi Province, China has a notably high incidence of cervical cancer cases, with 5.42 associated deaths per 100,000 women in 2014 (3), which was about two times more than the national average (4).
High-risk human papillomavirus (HPV) persistent infection, especially HPV16 infection, accounts for more than 50% of HPV cases (5) and plays an important role in cervical carcinogenesis (6). The HPV16 genome comprises approximately 8000 bp of double-stranded circular DNA that can be divided into early genes (E1, E2, E4, E5, E6, and E7), late genes (L1 and L2), and long control regions. HPV16 E6, which induces and maintains cellular transformation, is an important oncogenic protein. Specifically, E6 and E7 expressions are necessary to drive proliferation in infected cells and for progression to high-grade lesions and cancer development. HPV16 E2 is considered to be the main inhibitor of E6 and E7 oncogene expression (7, 8). Integration of the HPV16 genome into host chromosomes is a vital event in cervical carcinogenesis, which usually causes disruption of E2, loss of regulation of E6, and subsequently E6 overexpression (9). Therefore, E2 and E6 play an important role in HPV integration and carcinogenesis and have attracted extensive attention as key genes for integration. However, the regulation of HPV gene expression depends on intracellular RNA processing and is usually modified by RNA binding proteins (RBPs) (10).
As RBPs, heterogeneous nuclear ribonucleoproteins (hnRNPs), contribute multiple functions to nucleic acid metabolism through post-transcriptional regulation (11). hnRNP E1 is a member of the hnRNP family and contains three K homology (KH) domains. It is required to achieve greater RNA/DNA binding affinity and specificity (12). HPV16 has two characteristic promoters, early promoter p97, and late promoter p670. In addition, the HPV16 genome covers 5′splice sites, 3′splice sites, and two polyadenylation sites. These sites provide the structural basis for specific binding to a variety of RBPs and their protein complexes (13). Studies have shown that hnRNP E1 is involved in multiple pathological processes (14, 15). hnRNP E1 expression is associated with numerous tumor types, such as liver cancer (16), pancreatic cancer (17), gastrointestinal adenocarcinomas (18), prostate cancer (14), and thyroid carcinoma (19). However, to the best of our knowledge, the association between hnRNP E1 expression and cervical cancerization remains unclear. Pillai et al. (20) used an immunohistochemical method to detect hnRNP E1 in cervical tissue with a small sample size. The results showed that the expression level of hnRNP E1 decreased gradually with the increase in the severity of the disease. Our previous study showed that high expression of hnRNP K, which is similar to hnRNP E1 in structure and function, could increase the risk of cervical lesions (21). Collier et al. (22) found that hnRNP E1 inhibited the translation of L2 mRNA of the HPV16 late gene. However, the association between hnRNP E1 and HPV16 and the progression of cervical carcinogenesis has not been reported.
Based on the specificity with which the unique KH structural domains of hnRNP E1 bind with RNA/DNA, considering that HPV16 provides RNA/DNA binding sites, we hypothesized that hnRNP E1 may be crucial to HPV16 oncogenes expression and cervical carcinogenesis. Our previous population-based results showed that hnRNP E1, HPV16 E2, and E6 are closely linked to cervical cancer development (23). In the present study, we analyzed the expression changes of hnRNP E1 in different cervical pathological stages. We further explored the potential function and mechanism of hnRNP E1 in cervical lesions in vitro. Our findings may serve as a foundation for elucidating novel molecular targets against HPV16 and new prognostic and predictive biomarkers for cervical cancer.
There were 11984 participants, including 11938 women from the community cohort established in Jiexiu City and Yangqv County, Shanxi Province, China from June to September 2014, and 46 women suspected of cervical cancer from the Shanxi Cancer Hospital. All participants followed inclusion criteria: a) married, b)18-65 years, c) had resided in Shanxi for at least 1 year, d) volunteered to participate in the study, and the exclusion criteria were: a) pregnancy, b) history of hysterectomy or cervical conization, current or prior malignancy, c) had other tumors, d) received chemotherapy or radiotherapy. After completing the ThinPrep cytologic test (TCT) of 11,938 women, 858 women were diagnosed with atypical squamous cells of undetermined significance and above (ASC-US+). Then, after 77 women were excluded, 781 women with ASC-US+ underwent HPV genotyping and histopathological examination. Of the 781 women, 469 were diagnosed by pathology as normal cervical (NC), 236 as low-grade cervical intraepithelial neoplasm (CIN I), and 71 as high-grade cervical intraepithelial neoplasm (CIN II/III) and 5 as squamous cell carcinoma of the cervix (SCC). Meantime, we collected 35 SCC patients diagnosed by pathology from the hospital. Ultimately, 816 participants were involved in the study (Figure 1). All participants signed informed consent, and the study was approved by the institutional review committee of Shanxi Medical University (2013–003). No potentially identifiable human images or data are presented in this study.
Figure 1 Flowchart of participants in the study. TCT, ThinPrep cytologic test; NILM, negative for intraepithelial lesion or malignancy; ASC-US+, atypical squamous cells of undetermined significance and above; NC, normal cervical; CIN I, low-grade cervical intraepithelial neoplasm; CIN II/III, high-grade cervical intraepithelial neoplasm; SCC, squamous cell carcinoma; HPV, human papillomavirus.
All participants were interviewed face-to-face to collect information about sociodemographic characteristics, personal hygiene behavior, lifestyle, menstrual state, sexual life, and history of the personal disease through a structured questionnaire. Cervical swabs were collected with a cervical brush and stored at 4°C and completed HPV typing within 24 hours. Cervical biopsy specimens were collected and stored in a -80°C refrigerator immediately.
HPV16 infection assay by flow-through hybridization technology, DNA extraction, and HPV genotyping have previously been described in detail (24, 25). Briefly, HPV-DNA in cervical swabs was extracted by HPV-DNA extraction kit and amplified by PCR. HPV genotyping was performed using an HPV Geno Array Test Kit (HybriBio Ltd, Chaozhou, China) according to the manufacturer’s instructions. Twenty-one HPV genotypes can be identified. In the study, HPV16 positive was defined as the participants with HPV 16 single infection or multiple infections with other HPV genotypes.
Cervical cancer cell lines (SiHa and C33A) were obtained from the Chinese Academy of Sciences (Beijing, China). SiHa cells were cultured in high glucose Dulbecco’s modified eagle medium (Hyclone, USA), and the minimum essential medium (Boster Inc, China) was used to culture C33A cells. All the cell lines were supplemented with 10% fetal bovine serum of 5% CO2 at 37°C.
hnRNP E1 cDNA plasmids (Genechem Co., Ltd., Shanghai, China) and hnRNP E1 shRNA (Sangon Biotech, Co., Ltd., Shanghai, China) were used to up_ and down_ regulate the expression of hnRNP E1, respectively. When the cell confluence reached 75%, the transfection complex composed of plasmid, transfection reagent (Roche Applied Science, Germany), and culture medium were added to the 6-well plates. DNA plasmids encoding Green fluorescent protein (GFP) were performed to establish the best transfection efficiencies and conditions.
Proteins were extracted from cells or tissues and added to the RIPA buffer. Cracking on ice for 30_60 minutes. The supernatant was collected by centrifugation at 4°C and 12000 rpm for 15 minutes. BCA protein assay kit (Boster Inc, China) was used for protein quantification. SDS-PAGE gel electrophoresis was performed. Then, the protein was transferred to the nitrocellulose membrane and 5% skimmed milk powder blocked the nonspecific antigen. The primary antibody was added and incubated at 4°C overnight. After washing the membrane, horseradish peroxidase-labeled IgG (secondary antibody) was added at 37 °C for 1h. Densitometric analysis was performed by Image Lab. In the assay, antibodies included rabbit anti-hnRNP E1 (Abcam, UK. ab74793, 1:1000), mouse anti-HPV16 E2 (Abcam, UK. ab17185, 1:1000), mouse anti-HPV16 E6 (Abcam, UK. Ab70, 1:1000), mouse anti-β-actin (Boster Inc, China, 1:300).
TRIzol Reagent (Invitrogen, CA, USA) was used for extracting total RNA from cultured cells. cDNA was prepared by reverse transcription using TransScript one-step gDNA Removal and cDNA Synthesis SuperMix kit (Transgen Biotech, China). Quantitative real-time RT-PCR (RT-qPCR) was carried out using the QuantiNova SYBR Green PCR kit (QIAGEN GmbH, Germany). PCR was carried out following the manufacturer’s protocol. The PCR primer information was shown in Table S1. β-actin was used as the internal control. The relative mRNA levels were defined by using the 2-△△Ct method.
10μl CCK8 stock solution (Dojindo Laboratories, Japan) were added to each cultured 96-well plate at the stages of post-transfection 12h, 24h, 36h, 48h, and 72h, further incubated for 2h at the 37°C. The absorbance value at 450 nm was measured by a microplate reader. To analyze the cell cycle, cultured cells were collected, counted, and added 500µl 70% pre-cooling ethanol to centrifugated cells, overnight at 4°C. The next day, centrifugated cells were treated with 500μl RNaseA/Propidium Iodide and kept away from light at 25°C for 30 min. Subsequently, the cell cycle was detected by flow cytometry (FCM). Cell apoptosis was detected using an Annexin V-APC/Propidium Iodide apoptosis detection kit (KGA1030, KeyGEN Biotech, China), then, cell proliferation indexes (PI) were evaluated by a formula of (S+G2/M)÷(G0/1+S+G2/M)×100%.
Chromatin immunoprecipitation (ChIP) experiments were performed on SiHa cells that had not been treated with any plasmid. The SimpleChIP Enzymatic Chromatin IP Kit (no. 9003; Cell Signaling Technology, Danvers, MA, USA) was used to prepare cross-linked chromatin for ChIP. Briefly, the cells were fixed with 1% formaldehyde for 10 min, followed by cell and nuclear lysis. Cross-linked DNA was sonicated and ranged in size from 200 to 900 bp. DNA/protein complexes were immunoprecipitated overnight using anti-hnRNP E1 (#8534, Cell Signaling Technology, Danvers, MA, USA) antibodies. After reverse cross-linking and DNA purification, enriched DNA was detected by PCR. The primers used were listed in Table S1. The quality control analysis of the ChIP experiment was presented in Figure S1. Sequencing was performed at BGI-Shenzhen (Shenzhen, China) using an BGISeq-500 system. The authors acknowledge that the data presented in this study must be deposited and made publicly available in an acceptable repository, prior to publication. Frontiers cannot accept a manuscript that does not adhere to our open data policies.
The raw data were aligned to hg19 using SOAPaligner/SOAP2 (26). Peak calling was conducted by MACS (Model-based Analysis of ChIP-Seq) (27). The Gene Ontology (GO) terms (28) and Kyoto Encyclopedia of Genes and Genomes (KEGG) pathway analyses (29) were performed in R software using the “clusterProfiler” to show enrichment closely related to hnRNP E1_relevant genes. A two‐sided Fisher’s exact test and the “enrichplot” and “ggplot2” packages were used to visualize the top 10 enriched terms and KEGG pathways.
Analyses were performed using SPSS 22.0, R 4.0 and graphics were generated using GraphPad Prism 7.0. Significance testing between groups was performed by analysis of variance (ANOVA), Bonferroni’s multiple comparison tests, The post hoc Least Significant Difference (LSD) test, the Kruskal-Wallis H test, and the Chi-square test. Possible associations were analyzed using Spearman’s rank-order correlation. Kaplan-Meier survival analyses were performed in R 4.0 software using the “survival” and “survminer” to assess the relevance of hnRNP E1 expression and prognosis of the patient with cervical cancer. All analyses were two-sided and α=0.05.
The raw gene expression profiles of GSE9750 and GSE75132 were downloaded from the Gene Expression Omnibus (GEO) and used to analyze the expression of hnRNP E1 in cervical cancer and its relationship with HPV. GSE9750, containing 24 normal cervical samples and 33 cervical cancer samples. GSE75132 included data from six participants with persistent HPV16 infection and participants without HPV. The mRNA expression and clinical information of 304 patients with cervical cancer were downloaded from The Cancer Genome Atlas (TCGA) portal (https://portal.gdc.cancer.gov/).
We analyzed the demographic characteristics and factors related to cervical lesions of 816 participants. The average age of 816 women was 47 ± 12 years (range 19_65 years). Of all participants, 79.5% had received education in junior high school and above. The distribution of marital status, age, education level, and occupation among different cervical lesions groups was not significant (P>0.05); however, early age of first sexual intercourse, multiple gravidities, low bathing frequency, and low vaginal cleaning frequency were found to be associated with increased risk of CIN and cervical cancer(P<0.05).
The prevalence of HPV16 in NC, CIN I, CIN II/III, and SCC were 8.53%, 14.41%, 40.85%, and 67.50%, respectively, with significant differences, and showed an upward trend with the aggravation of cervical lesions (χ2trend=113.560, P<0.001). The results were displayed in Table 1. Subsequent results showed that HPV16 E2 protein levels in the NC and CIN I groups were significantly higher than those in the CIN II/III and SCC groups. The HPV16 E6 protein levels in the NC and CIN I groups was significantly lower than those in the CIN II/III and SCC groups (Table 1). There were significant differences in the ratio of E2 to E6 in different groups (H=71.392, P<0.001), showing a decreasing trend from NC to CIN I, CIN II/III, and SCC (Table 1).
To explore hnRNP E1 expression patterns in cervical lesions, hnRNP E1 protein expression was detected using western blotting. With the progress of cervical lesions, hnRNP E1 protein expression levels gradually decreased (Figure 2A). The average hnRNP E1 expression level in NC (2.081 ± 1.708, n=469) was 2.30 times higher than that in SCC (0.906 ± 0.844, n=40). Moreover, data from the GEO dataset (GSE9750) showed that hnRNP E1 mRNA was more highly expressed in NC samples than in SCC samples (Figure 2B). We further investigated the associations between hnRNP E1 and HPV in cervical lesions and found that the expression levels of hnRNP E1 protein or mRNA in the HPV-negative group were significantly higher than those in the HPV-positive group (P<0.05), as shown in Figures 2C, D. Spearman’s rank correlation analysis showed positive correlations between hnRNP E1 expression and HPV16 E2 (rs=0.397, P<0.001) and the ratio of HPV16 E2 to E6 (rs=0.584, P<0.001) and a negative correlation between hnRNP E1 and HPV16 E6 (rs =-0.584, P<0.001), as shown in Figures 2E, F.
Figure 2 hnRNP E1 expression and correlations with HPV16 E2 and E6 in cervical lesions. (A) hnRNP E1 protein expression levels in the clinical samples. (B) The hnRNP E1 mRNA expression levels in NC and SCC samples, data were obtained from GEO dataset. (C) The hnRNP E1 protein expression levels in HPV+ and HPV- samples. (D) The hnRNP E1 mRNA expression levels in HPV+ and HPV- samples, data were available from GEO dataset. (E) hnRNP E1, HPV16 E2 and E6 expression levels was detected using western blotting in cervix tissues. β-actin was used as loading control. (F) Correlations between hnRNP E1, HPV16 E2 and E6 in cervical lesions. a/b/c/d, different letters indicate significant differences at least P<α’(α’=0.05/6 = 0.0083). HPV+ represents HPV-positive samples, and HPV- represents HPV-negative samples.
We further analyzed the relationship between hnRNP E1 expression and clinicopathological characteristics in cervical cancer based on the TCGA database. The Kruskal-Wallis rank-sum test showed that there was no significant correlation between hnRNP E1 expression and T stage (P=0.609), N stage (P=0.078), M stage (P=0.203), International Federation of Gynecology and Obstetrics (FIGO) stage (P=0.178), pathological type (P=0.602) and therapy (P=0.448) of cervical cancer (Figures 3A–F). To better understand the relevance of hnRNP E1 expression and the prognosis of the patient with cervical cancer, we performed Kaplan-Meier survival analysis. Patients were divided into high-hnRNP E1 and low-hnRNP E1 groups based on the median value. Results showed that the overall survival rate was not significant between the high-hnRNP E1 and low-hnRNP E1 groups (P>0.05, Figure 3G). Although our results have revealed the subtle prognostic value of hnRNP E1, compared with the low expression of hnRNP E1, high expression of hnRNP E1 has a better prognosis.
Figure 3 Correlations between hnRNP E1 and prognosis of the patient with cervical cancer based on TCGA database. hnRNP E1 mRNA expression levels in the different T stage (A), N stage (B), M stage (C), International Federation of Gynecology and obstetrics (FIGO) stage (D), Pathological type (E) and Therapy (F). (G) Kaplan-Meier survival curves were performed for the overall survival probability.
Based on the main purpose of this study was to explore the role of hnRNP E1 on the regulation of HPV16 oncogene expression in cervical cancerization, we conducted ChIP-seq in the untreated SiHa cell line. A total of 121 potential targets for hnRNP E1 across the human genome in SiHa cells were identified, including 357 binding sites (peaks). The average peak length was 161 bp, and the length was mainly distributed between 100_500 bp. To further analyze the potential biological functions of annotated genes related to hnRNP E1, GO and KEGG enrichment analyses were performed, and the screening criteria was P-value<0.3. According to the functional annotation in the GO database, the most significant biological process (BP) terms were peptidyl-threonine phosphorylation, peptidyl-serine phosphorylation, peptidyl-threonine modification, and peptidyl-serine modification, and Hippo signaling. When focusing on cellular components (CC), the most highly represented categories were the cell cortex, protein phosphatase type 2A complex, histone deacetylase complex, presynaptic active zone, and magnesium-dependent protein serine/threonine phosphatase complex. The main functional categories of molecular function (MF) were related to protein serine kinase activity, on-membrane spanning protein tyrosine phosphatase activity, heme transmembrane transporter activity, flap endonuclease activity, and inositol 1,4,5 trisphosphate binding. GO enrichment terms of BP, CC, and MF for hnRNP E1 annotated genes are shown in Figures 4A, C, E. Based on the KEGG pathway enrichment analysis, hnRNP E1_relevant genes were involved in the dopaminergic synapse, Wnt signaling pathway, gnRH secretion, mTOR signaling pathway, pathways of neurodegenerative diseases, sphingolipid signaling pathway, AMPK signaling pathway, proteoglycans in cancer, and long-term depression. (Figure 4G). The top 10 most enriched functions were obtained to construct a network (Figures 4B, D, F, H). It was worth noting that we found that hnRNP E1 relevant genes were enriched in HPV infection pathway. These results suggested that hnRNP E1 may play a key role in HPV-induced cervical lesions. Detailed information was listed in Table S2.
Figure 4 GO and KEGG pathway enrichment analyses of hnRNP E1 related genes. Note: BP, biological process. CC, cellular components. MF, molecular function. GO, Gene Ontology. KEGG, Kyoto Encyclopedia of Genes and Genomes.The top 10 significant BP (A), CC (C), MF (E), and KEGG pathways (G). Construction of the enrichment BP_genes network (B), CC_genes network (D), MF_genes network (F), and pathways_genes network (H). (A, C, E, G) The x axis reflects gene ratio, and the y axis represents the KEGG and GO terms. The node size represents the gene count. The node colors represent adjusted P values. (B, D, F, H) The size of the circle indicates the number of enriched genes. Lines represent linkages of genes and enriched terms.
To further explore the biological role of hnRNP E1 in cervical cancer, cultured SiHa, and C33A cell lines were transduced with hnRNP E1 overexpression plasmid or shRNA. OE indicates the hnRNP E1 overexpression group and NC-OE indicates the relative control group. KD indicates the hnRNP E1 knockdown group and NC-KD indicates the relative control group. Overexpression and knockdown efficiencies of hnRNP E1 were confirmed by RT-qPCR and western blotting (Figure 5A). As shown in Figure 5B, compared with the control group, overexpression of hnRNP E1 (OE) in SiHa and C33A cells resulted in decreased cell viability (NC-OE, P<0.05), and it was found that the inhibition rate of viability in hnRNP E1 overexpression was significantly higher than that of the control cells after transfection (0.36% ± 0.02% vs. 0.05% ± 0.04%, P<0.05; 0.28% ± 0.03% vs. 0.10% ± 0.03%, P<0.05). Conversely, knockdown of hnRNP E1 in SiHa and C33A cells significantly promoted cell growth with an increase in the cell proliferation rate at 48 h after transfection (0.23% ± 0.01% vs. 0.03% ± 0.03%, P<0.05; 0.22% ± 0.02% vs.0.03% ± 0.02%, P<0.05).
Figure 5 Effects of hnRNP E1 on cell biological function in cervical cancer cell lines. Note: OE indicates hnRNP E1 overexpression group and NC-OE indicates the relative control group. KD indicates hnRNP E1 knockdown group and NC-KD indicates the relative control group. *P < 0.05. (A) Stably transfected hnRNP E1-modified cervical cancer cells were identified by RT-qPCR and western blotting. (B) hnRNP E1 overexpression strongly suppressed cell viabilities in SiHa and C33A cells. hnRNP E1 knockdown markedly enhanced cell viabilities in SiHa and C33A cells. (C) hnRNP E1 arrests the cell cycle from the G0/G1 to the S phase. (D) hnRNP E1 promotes cell apoptosis.
Next, the cell cycles were analyzed by FCM. As shown in Figure 5C, overexpression of hnRNP E1 increased the percentage of G0/G1 phase cells in SiHa and C33A cells (77.33% ± 1.00% vs. 69.38% ± 1.09%, P<0.05; 69.31% ± 0.90% vs.63.82% ± 0.41%, P<0.05) and reduced the proportion of S/G2/M cells and PI (22.68% ± 0.83% vs.30.62% ± 1.09%, P<0.05; 30.73% ± 0.87% vs.36.18% ± 0.41%, P<0.05). Conversely, hnRNP E1 knockdown in SiHa and C33A cells markedly attenuated the percentage of G0/G1 cells (62.71% ± 0.47% vs. 71.87% ± 0.67%, P<0.05; 60.52% ± 0.28% vs. 65.34% ± 0.22%, P<0.05), but increased the proportion of S/G2/M phase and PI (37.20% ± 0.50% vs. 28.13% ± 0.67%, P<0.05; 40.32% ± 0.21% vs. 34.63% ± 0.24%, P<0.05). These results suggest that hnRNP E1 affects cell cycle progression and G0/G1 phase arrest. Additionally, we compared the changes in cell cycles and proliferation index of SiHa and C33A cells modified by hnRNP E1 and found that the changes in PI in SiHa cells were higher than those in C33A cells (Figure S2A). To understand hnRNP E1-induced cell apoptosis, we performed FCM on hnRNP E1-intervened SiHa and C33A cell lines. These results indicated that the total apoptotic rate was significantly increased after the upregulation of hnRNP E1 in SiHa and C33A cell lines (11.81% ± 0.77% vs. 5.52% ± 0.11%, P<0.05; 7.57% ± 0.53%, vs. 5.16% ± 0.88%, P<0.05), as shown in Figure 5D. Conversely, knockdown of hnRNP E1 in SiHa and C33A cells considerably reduced the total apoptotic rate (3.64% ± 0.95% vs. 6.33% ± 0.67%, P<0.05; 2.25% ± 0.27% vs.4.92% ± 0.25%, P<0.05). These results were consistent with our work in this population and indicated that hnRNP E1 played a tumor suppressor role in cervical cancer. Furthermore, we analyzed changes in the apoptotic rate of SiHa and C33A cells modified by hnRNP E1 and found that the changes in the early and late apoptotic rate of SiHa cells were superior to those of C33A (Figure S2B).
Our population-based study identified a negative correlation between hnRNP E1 and HPV16 E6 and a positive correlation between hnRNP E1 and HPV16 E2 protein expression. We confirmed that hnRNP E1 induced different biological changes in SiHa and C33A cells in vitro. Next, to test whether hnRNP E1 regulated HPV16 oncogene expression, HPV16 E2 and HPV16 E6 were assessed in SiHa cells. As shown in Figure 6A, hnRNP E1 overexpression downregulated HPV16 E6 expression at both the mRNA and protein levels but increased the ratio of HPV16 E2 to E6 at the mRNA level. Conversely, hnRNP E1 knockdown markedly enhanced HPV16 E6 expression at both the mRNA and protein levels but decreased the HPV16 E2 to E6 ratio at the mRNA level (Figure 6A).
Figure 6 hnRNP E1 effects on HPV16 oncogene and subsequent changes in cell biological function. Note: OE indicates hnRNP E1 overexpression group and NC-OE indicates the relative control group. KD indicates hnRNP E1 knockdown group and NC-KD indicates the relative control group. *P < 0.05. (A) The expression of HPV16 E2, and E6 were detected in SiHa cells by RT-qPCR and western blotting. (B) The relationship between HPV16 E6 expression, cell proliferation, and apoptosis in hnRNP E1-modified cells. The expression of HPV16 E2, and E6 were detected in SiHa cells by RT-qPCR and western blotting. The PI and total apoptosis rate were evaluated using flow cytometry analyses. As HPV16 E6 mRNA and protein expression levels decreased, the proliferation indices of SiHa cells decreased, while the total apoptosis rate increased. As HPV16 E6 expression increased, the proliferation indices of SiHa cells increased, while the total apoptosis rate decreased.
We further analyzed the relationship between HPV16 E6 expression, cell proliferation, and apoptosis. The expression of HPV16 E2 and E6 were detected in SiHa cells by RT-qPCR and western blotting. The PI and total apoptosis rate were evaluated using FCM. Our results indicated that as HPV16 E6 expression decreased, the proliferation indices of SiHa cells were reduced (P<0.05), while the total apoptosis rate increased (P<0.05). Conversely, as HPV16 E6 expression increased, the PI of SiHa cells increased (P<0.05), while the total apoptosis rate was attenuated (P<0.05), especially in hnRNP E1-modified cells (Figure 6B). Taken together, these findings indicated that hnRNP E1 may act as a tumor suppressor by downregulating HPV16 E6 expression.
In this study, we reviewed and compared hnRNP E1 expression in participants with and without HPV and explored the relationship between hnRNP E1 expression levels and cervical lesion development. Mechanistically, hnRNP E1 expression may be a significant factor affecting HPV carcinogenicity by downregulating HPV16 oncogene expression and mitigating cancerization.
In the past decade, the contribution of HPV16 persistent infection to invasive cervical cancer has been well-documented (30). The HPV16 prevalence was 3.7% in women residing in Shanxi Province, which exceeded the national average (0.78%) in 2014 (31). We observed that HPV16 infection rates were 8.53%, 14.41%, 40.85%, and 67.50% in the NC, CIN I, CIN II/III, and SCC groups, respectively. This further corroborated that HPV16 infection is a crucial etiological factor for cervical lesions, especially CIN II/III and SCC. Therefore, it is essential to carry out HPV screening to prevent the occurrence and progression of cervical cancer. HPV16 E2 is crucial for transcriptional regulation, DNA replication, viral genome tethering, and viral DNA packaging (32). Xue et al. (33) found that E2 was highly expressed in CINI compared with that in CIN II/III through immunohistochemistry. The present study revealed that HPV16 E2 low expression was closely related to the development of cervical lesions, especially CIN II/III and SCC. HPV16 E6 is a multifunctional oncoprotein that mediates various biological events, such as promoting the degradation of p53, regulating the transcription of cell cycle-associated genes, activating telomerase, and contributing to immune response and cell communication (34, 35). Wang et al. (36) suggested that HPV16 E6 could promote the migration and invasion of cervical cancer cells. Our previous study combined with this study indicated that HPV16 E6 increases the risk of cervical cancerization (37). BPV E2 expression results in specific inhibition of HPV E6 gene expression in cells and considerable growth inhibition8. HPV16 DNA integration and virus E2 gene damage often occur in cervical carcinogenesis, suggesting that inactivation of the HPV E2 gene allows high expression of the E6 gene, which can promote the growth of cervical epithelial cells (38). Our study showed that the ratios of HPV16 E2 to E6 in CIN II/III and SCC groups were significantly lower than those in the NC and CIN I groups. This observation was supported by Choi et al. (39) who discovered that the mean HPV16 E2/E6 ratio decreased significantly following a linear trend from CIN II/III to SCC. The ratio of E2 to E6 may be a sign of cancer progression.
hnRNP E1 was downregulated in numerous tumors (40). Zhang et al. (14) reported that hnRNP E1 functions as a tumor suppressor in gastric cancer. The expression of hnRNP E1 and miRNA-3978 in peritoneal metastasis of gastric cancer was inhibited (15). Pillai et al. (20) discovered that hnRNP E1 expression decreased from 86% in CIN I to 68% in CIN II/III and 40% in cervical cancer. Pathak et al. (41) also found that hnRNP E1 expression decreased progressively from the normal cervix (100%) to squamous intraepithelial lesions (75%) and cervical cancer (52.6%). The present study showed that with the development of cervical lesions, hnRNP E1 expression decreased from NC to CIN and SCC. Although the survival analysis based on the TCGA database revealed a subtle prognosis value of hnRNP E1. These results indicated that low hnRNP E1 expression contributed to the risk of cervical lesions and promoted the progression of cervical cancerization. hnRNP E1 may be considered a biomarker for the early detection of cervical carcinogenesis.
To understand the binding capacity of hnRNP E1 at the genome-wide level, we conducted ChIP-seq in the untreated SiHa cell line. Our data further supported that hnRNP E1 relevant genes were associated with a series of GO terms related to cell proliferation, metabolism, and apoptosis. KEGG pathway enrichment analysis revealed that hnRNP E1 relevant genes were mainly involved in the Wnt signaling pathway, GnRH secretion, dopaminergic synapse, mTOR signaling pathway, pathways of neurodegeneration-multiple diseases, sphingolipid signaling pathway, and AMPK signaling pathway. The aberrant Wnt/β-catenin, mTOR, and MAPK signaling pathways facilitate cancer cell proliferation and differentiation (42–44). GnRH is known primarily as a neuroendocrine decapeptide that is essential for maintaining the reproductive state (45). Most notably, we found that hnRNP E1 relevant genes were enriched in HPV infection pathway, although the effect was not significant. These results suggested that hnRNP E1 may play a key role in HPV-induced cervical lesions.
To better understand the biological mechanism of hnRNP E1 in cervical lesions, we performed in vitro plasmid transfection. Our results demonstrated that hnRNP E1 inhibited cervical cancer cell proliferation, promoted apoptosis, and arrested the cell cycle in the G0/G1 phase. In vitro research has shown that the deletion of hnRNP E1 reduced the expression of p27, a key regulator of the cell cycle, and promoted carcinogenesis (46). Overexpression of hnRNP E1 reduced the expression of p53 (47) and played a vital role in the cell’s biological function and DNA damage response (48). In addition, we confirmed that hnRNP E1 induced different biological changes in SiHa and C33A cells in vitro. The changes in biological function in SiHa cells were greater than those in C33A cells. Given the key tumor-suppressive role of hnRNP E1 in cervical cancerization, it may have great therapeutic potential for cervical cancer. hnRNP E1 can inhibit the translation of HPV16 L2 mRNA in vitro (22). However, it remains unclear whether hnRNP E1 can regulate the HPV16 early gene. Our results demonstrated that hnRNP E1 was positively correlated with HPV16 E2 or E2/E6 ratio and negatively correlated with HPV16 E6. In addition, in vitro experiments verified that hnRNP E1 overexpression diminished the expression of HPV16 E6 but improved the ratio of HPV16 E2 to E6. In contrast, hnRNP E1 knockdown significantly increased HPV16 E6 expression but attenuated the ratio of HPV16 E2 to E6. The relationship between hnRNP E1 and HPV16 E2 protein in SiHa cells was not found in this study, which may be related to the fact that during HPV integration, E2 is destroyed in SiHa and may not produce functional E2 (49). E6 is one of the earliest-expressed genes following HPV infection, containing the selective binding site for hnRNP E1 (50). The KH domain in hnRNP E1 may reduce the expression of HPV16 E6 by binding with its regulatory element. Interestingly, our results showed that with a decrease in HPV16 E6 expression, the proliferation index of SiHa cells decreased, and the total apoptosis rate increased. Conversely, with the increase in HPV16 E6 expression, the proliferation indices of SiHa cells increased while the total apoptosis rate decreased, and this effect was more significant when hnRNP E1 was modified. Our findings suggest that HPV16 E6 low expression inhibits the proliferation and promotes cervical cancer cell apoptosis, and this effect may be regulated by hnRNP E1.
Our study does, however, have several limitations. First, our study was based on a cross-sectional study, prospective cohort studies can be conducted in the future to further explore the effect of hnRNP E1 on the prognosis of cervical lesions and HPV16 gene expression. Second, we only selected two cervical cancer cell lines for in vitro experiments, the conclusions will be further demonstrated in a variety of cervical cancer cell lines. Lastly, the in vivo effect of hnRNPE1 overexpression in the xenograft of cervical cancer cell lines was unclear, future relevant animal experiments will need to be done.
In conclusion, our study indicated that abnormally low expression of hnRNP E1 could promote cervical lesion development and was closely linked with HPV16 E2, E6 expression, and the E2 to E6 ratio. hnRNP E1 suppresses tumor growth in vitro by inhibiting proliferation and promoting apoptosis. hnRNP E1 relevant genes were significantly enriched in cervical cancer-related pathways. Moreover, hnRNP E1 significantly downregulated the expression of HPV16 E6. Upregulating hnRNP E1, particularly incorporating the control patterns of HPV16 infection, may offer advances in the control of cervical cancer. Owing to the complexity of HPV gene expression and post-transcriptional regulation, in-depth studies are needed to understand the underlying mechanisms of hnRNP E1.
The datasets presented in this study can be found in online repositories. The names of the repository/repositories and accession number(s) can be found below: GEO with accession GSE203023: (https://www.ncbi.nlm.nih.gov/geo/query/acc.cgi?acc=GSE203023).
The studies involving human participants were reviewed and approved by the institutional review committee of Shanxi Medical University (2013–003). The patients/participants provided their written informed consent to participate in this study.
JTW conceived the project. RM, LD, and ZT designed the experiments. MZ and JHW performed the experiments. MW and YL contributed reagents, materials, and analysis tools. CL, MF, and HJ integrated, analyzed, and interpreted all data. JTW contributed to the supervision of the work. LS wrote the manuscript with the assistance and final approval of all authors.
This subject is supported by the National Natural Science Foundation of China (No. 81872705, No. 81473060), the National Health and Family Planning Commission of the People’s Republic of China (No. 201402010), and the Fundamental Research Program of Shanxi Province (No.202103021224354).
The authors declare that the research was conducted in the absence of any commercial or financial relationships that could be construed as a potential conflict of interest.
All claims expressed in this article are solely those of the authors and do not necessarily represent those of their affiliated organizations, or those of the publisher, the editors and the reviewers. Any product that may be evaluated in this article, or claim that may be made by its manufacturer, is not guaranteed or endorsed by the publisher.
The Supplementary Material for this article can be found online at: https://www.frontiersin.org/articles/10.3389/fonc.2022.905900/full#supplementary-material
1. Sung H, Ferlay J, Siegel RL, Laversanne M, Soerjomataram I, Jemal A, et al. Global Cancer Statistics 2020: GLOBOCAN Estimates of Incidence and Mortality Worldwide for 36 Cancers in 185 Countries. CA Cancer J Clin (2021) 71(3):209–49. doi: 10.3322/caac.21660
2. Cao W, Chen HD, Yu YW, Li N, Chen WQ. Changing Profiles of Cancer Burden Worldwide and in China: A Secondary Analysis of the Global Cancer Statistics 2020. (Engl) (2021) 134(7):783–91. doi: 10.1097/cm9.0000000000001474
3. Ma ZH, Gao QS, Cao L, Wang XZ, Guo XR, Wang XC, et al. Analysis of Cancer Incidence and Mortality in Registration Areas of Shanxi Province in 2014. Cancer Res Clinic Cancer Res Clinic (in Chinese) (2020) 32(3):186–91. doi: 10.3760/cma.j.cn115355-20191030-00492
4. Gu XY, Zheng RS, Sun KX, Zhang SW, Zeng HM, Zou XN, et al. Incidence and Mortality of Cervical Cancer in China, 2014. Chin J Oncology (in Chinese) (2018) 040(004):241–6. doi: 10.3760/cma.j.issn.0253-3766.2018.04.001
5. Xu HH, Wang K, Feng XJ, Dong SS, Lin A, Zheng LZ, et al. Prevalence of Human Papillomavirus Genotypes and Relative Risk of Cervical Cancer in China: A Systematic Review and Meta-Analysis. Oncotarget (2018) 9(20):15386–97. doi: 10.18632/oncotarget.24169
6. Luria L, Cardoza-Favarato G. Human Papillomavirus. StatPearls. Treasure Island (FL: StatPearls Publishing Copyright © 2022, StatPearls Publishing LLC (2022).
7. Graham SV. Human Papillomavirus E2 Protein: Linking Replication, Transcription, and RNA Processing. J Virol (2016) 90(19):8384–8. doi: 10.1128/jvi.00502-16
8. Taghizadeh E, Jahangiri S, Rostami D, Taheri F, Renani PG, Taghizadeh H, et al. Roles of E6 and E7 Human Papillomavirus Proteins in Molecular Pathogenesis of Cervical Cancer. Curr Protein Pept Sci (2019) 20(9):926–34. doi: 10.2174/1389203720666190618101441
9. Balasubramaniam SD, Balakrishnan V, Oon CE, Kaur G. Key Molecular Events in Cervical Cancer Development. Medicina (Kaunas) (2019) 55(7):384–96. doi: 10.3390/medicina55070384
10. Kajitani N, Schwartz S. Role of Viral Ribonucleoproteins in Human Papillomavirus Type 16 Gene Expression. Viruses (2020) 12(10):1110–39. doi: 10.3390/v12101110
11. Geuens T, Bouhy D, Timmerman V. The hnRNP Family: Insights Into Their Role in Health and Disease. Hum Genet (2016) 135(8):851–67. doi: 10.1007/s00439-016-1683-5
12. Valverde R, Edwards L, Regan L. Structure and Function of KH Domains. FEBS J (2008) 275(11):2712–26. doi: 10.1111/j.1742-4658.2008.06411.x
13. Johansson C, Schwartz S. Regulation of Human Papillomavirus Gene Expression by Splicing and Polyadenylation. Nat Rev Microbiol (2013) 11(4):239–51. doi: 10.1038/nrmicro2984
14. Zhang Y, Meng L, Xiao L, Liu R, Li Z, Wang YL. The RNA-Binding Protein PCBP1 Functions as a Tumor Suppressor in Prostate Cancer by Inhibiting Mitogen Activated Protein Kinase 1. Cell Physiol Biochem (2018) 48(4):1747–54. doi: 10.1159/000492315
15. Ji FJ, Wu YY, An Z, Liu XS, Jiang JN, Chen FF, et al. Expression of Both Poly R(C) Binding Protein 1 (PCBP1) and miRNA-3978 Is Suppressed in Peritoneal Gastric Cancer Metastasis. Sci Rep (2017) 7(1):15488. doi: 10.1038/s41598-017-15448-9
16. Huang S, Luo K, Jiang L, Zhang XD, Lv YH, Li RF. PCBP1 Regulates the Transcription and Alternative Splicing of Metastasis−Related Genes and Pathways in Hepatocellular Carcinoma. Sci Rep (2021) 11(1):23356. doi: 10.1038/s41598-021-02642-z
17. Jiang P, Li Z, Tian F, Li X, Yang J. Fyn/heterogeneous Nuclear Ribonucleoprotein E1 Signaling Regulates Pancreatic Cancer Metastasis by Affecting the Alternative Splicing of Integrin β1. Int J Oncol (2017) 51(1):169–83. doi: 10.3892/ijo.2017.4018
18. Liu Y, Sethi NS, Hinoue T, Schneider BG, Cherniack AD, Sanchez-Vega F, et al. Comparative Molecular Analysis of Gastrointestinal Adenocarcinomas. Cancer Cell (2018) 33(4):721–735.e728. doi: 10.1016/j.ccell.2018.03.010
19. Zhang MP, Zhang WS, Tan J, Zhao MH, Lian LJ, Cai J. Poly R(C) Binding Protein (PCBP) 1 Expression is Regulated at the Post-Translation Level in Thyroid Carcinoma. Am J Transl Res (2017) 9(2):708–14. doi: 10.3390/ijerph181910369
20. Pillai MR, Chacko P, Kesari LA, Jayaprakash PG, Jayaram HN, Antony AC. Expression of Folate Receptors and Heterogeneous Nuclear Ribonucleoprotein E1 in Women With Human Papillomavirus Mediated Transformation of Cervical Tissue to Cancer. J Clin Pathol (2003) 56(8):569–74. doi: 10.1136/jcp.56.8.569
21. Yang Q. Relationship Between hnRNP K and Cervical Carcinogenesis and Analysis of Whole Genomic Binding and Function of hnRNP K in Siha Cell (in Chinese) [D]. Taiyuan, Shanxi: Shanxi Medical University (2018).
22. Collier B, Goobar-Larsson L, Sokolowski M, Schwartz S. Translational Inhibition In Vitro of Human Papillomavirus Type 16 L2 mRNA Mediated Through Interaction With Heterogenous Ribonucleoprotein K and Poly(Rc)-Binding Proteins 1 and 2. J Biol Chem (1998) 273(35):22648–56. doi: 10.1074/jbc.273.35.22648
23. Lyu YJ, Ding L, Li QL, Li L, Wang M, Han Y, et al. Effects of hnRNP E1 and Both Early Genes E2 and E6 of HPV16 Together With Their Interactions on Cervical Carcinogenesis. Zhonghua Liu Xing Bing Xue Za Zhi (2019) 40(4):466–70. doi: 10.3760/cma.j.issn.0254-6450.2019.04.018
24. Li X, Ding L, Song L, Gao W, Wang L, Wang J. Effects of Exposure to Polycyclic Aromatic Hydrocarbons Combined With High-Risk Human Papillomavirus Infection on Cervical Intraepithelial Neoplasia: A Population Study in Shanxi Province, China. Int J Cancer (2020) 146(9):2406–12. doi: 10.1002/ijc.32562
25. Song L, Lyu Y, Ding L, Li X, Gao W, Wang M, et al. Prevalence and Genotype Distribution of High-Risk Human Papillomavirus Infection in Women With Abnormal Cervical Cytology: A Population-Based Study in Shanxi Province, China. Cancer Manag Res (2020) 12:12583–91. doi: 10.2147/cmar.S269050
26. Li R, Li Y, Kristiansen K, Wang J. SOAP: Short Oligonucleotide Alignment Program. Bioinformatics (2008) 24(5):713–4. doi: 10.1093/bioinformatics/btn025
27. Zhang Y, Liu T, Meyer CA, Eeckhoute J, Johnson DS, Bernstein BE, et al. Model-Based Analysis of ChIP-Seq (MACS). Genome Biol (2008) 9(9):R137. doi: 10.1186/gb-2008-9-9-r137
28. Gene Ontology Consortium Gene Ontology Consortium: Going Forward. Nucleic Acids Res (2015) 43:D1049–56. doi: 10.1093/nar/gku1179
29. Kanehisa M, Goto S. KEGG: Kyoto Encyclopedia of Genes and Genomes. Nucleic Acids Res (2000) 28(1):27–30. doi: 10.1093/nar/28.1.27
30. Comprehensive Cancer Information – National Cancer Institute . Available at: https://www.cancer.gov/.
31. Xu MY, Cao B, Chen Y, Du J, Yin J, Liu L, et al. Prevalence and Type Distribution of Human Papillomavirus in a Chinese Urban Population Between 2014 and 2018: A Retrospective Study. PeerJ (2020) 8:e8709. doi: 10.7717/peerj.8709
32. McBride AA. The Papillomavirus E2 Proteins. Virology (2013) 445(1-2):57–79. doi: 10.1016/j.virol.2013.06.006
33. Xue Y, Bellanger S, Zhang W, Lim D, Low J, Lunny D, et al. HPV16 E2 is an Immediate Early Marker of Viral Infection, Preceding E7 Expression in Precursor Structures of Cervical Carcinoma. Cancer Res (2010) 70(13):5316–25. doi: 10.1158/0008-5472.Can-09-3789
34. Wallace NA, Galloway DA. Novel Functions of the Human Papillomavirus E6 Oncoproteins. Annu Rev Virol (2015) 2(1):403–23. doi: 10.1146/annurev-virology-100114-055021
35. Aco-Tlachi M, Carreño-López R, Martínez-Morales PL, Maycotte P, Aguilar-Lemarroy A, Jave-Suárez LF, et al. Glycogene Expression Profiles Based on Microarray Data From Cervical Carcinoma HeLa Cells With Partially Silenced E6 and E7 HPV Oncogenes. Infect Agent Cancer (2018) 13:25–36. doi: 10.1186/s13027-018-0197-2
36. Wang Q, Song R, Zhao C, Liu H, Yang Y, Gu S, et al. HPV16 E6 Promotes Cervical Cancer Cell Migration and Invasion by Downregulation of NHERF1. Int J Cancer (2019) 144(7):1619–32. doi: 10.1002/ijc.31876
37. Wang JT, Ding L, Gao ES, Cheng YY. Analysis on the Expression of Human Papillomavirus Type 16 E2 and E6 Oncogenes and Disruption of E2 in Cervical Cancer. Zhonghua Liu Xing Bing Xue Za Zhi (2007) 28(10):968–71. doi: 10.3760/j.issn:0254-6450.2007.10.008
38. Cripe TP, Haugen TH, Turk JP, Tabatabai F, Schmid PG 3rd, Dürst M, et al. Transcriptional Regulation of the Human Papillomavirus-16 E6-E7 Promoter by a Keratinocyte-Dependent Enhancer, and by Viral E2 Trans-Activator and Repressor Gene Products: Implications for Cervical Carcinogenesis. EMBO J (1987) 6(12):3745–53. doi: 10.1002/j.1460-2075.1987.tb02709.x
39. Choi YJ, Lee A, Kim TJ, Jin HT, Seo YB, Park JS, et al. E2/E6 Ratio and L1 Immunoreactivity as Biomarkers to Determine HPV16-Positive High-Grade Squamous Intraepithelial Lesions (CIN2 and 3) and Cervical Squamous Cell Carcinoma. J Gynecol Oncol (2018) 29(3):e38. doi: 10.3802/jgo.2018.29.e38
40. Zhang X, Di C, Chen Y, Wang J, Su R, Huang G, et al. Multilevel Regulation and Molecular Mechanism of Poly (Rc)-Binding Protein 1 in Cancer. FASEB J (2020) 34(12):15647–58. doi: 10.1096/fj.202000911R
41. Pathak S, Bajpai D, Banerjee A, Bhatla N, Jain SK, Jayaram HN, et al. Serum One-Carbon Metabolites and Risk of Cervical Cancer. Nutr Cancer (2014) 66(5):818–24. doi: 10.1080/01635581.2014.916318
42. Zhang Y, Wang X. Targeting the Wnt/β-Catenin Signaling Pathway in Cancer. J Hematol Oncol (2020) 13(1):165. doi: 10.1186/s13045-020-00990-3
43. Che Y, Li Y, Zheng F, Zou K, Li Z, Chen M, et al. TRIP4 Promotes Tumor Growth and Metastasis and Regulates Radiosensitivity of Cervical Cancer by Activating MAPK, PI3K/AKT, and hTERT Signaling. Cancer Lett (2019) 452:1–13. doi: 10.1016/j.canlet.2019.03.017
44. Popova NV, Jücker M. The Role of mTOR Signaling as a Therapeutic Target in Cancer. Int J Mol Sci (2021) 22(4):1743–69. doi: 10.3390/ijms22041743
45. Whitlock KE, Postlethwait J, Ewer J. Neuroendocrinology of Reproduction: Is Gonadotropin-Releasing Hormone (GnRH) Dispensable? Front Neuroendocrinol (2019) 53:100738. doi: 10.1016/j.yfrne.2019.02.002
46. Shi H, Li H, Yuan R, Guan W, Zhang X, Zhang S, et al. PCBP1 Depletion Promotes Tumorigenesis Through Attenuation of P27(Kip1) mRNA Stability and Translation. J Exp Clin Cancer Res (2018) 37(1):187. doi: 10.1186/s13046-018-0840-1
47. Huo LR, Zhong N. Identification of Transcripts and Translatants Targeted by Overexpressed PCBP1. Biochim Biophys Acta (2008) 1784(11):1524–33. doi: 10.1016/j.bbapap.2008.06.017
48. Wawryk-Gawda E, Chylińska-Wrzos P, Lis-Sochocka M, Chłapek K, Bulak K, Jędrych M, et al. P53 Protein in Proliferation, Repair and Apoptosis of Cells. Protoplasma (2014) 251(3):525–33. doi: 10.1007/s00709-013-0548-1
49. Raybould R, Fiander A, Wilkinson GW, Hibbitts S. HPV Integration Detection in CaSki and SiHa Using Detection of Integrated Papillomavirus Sequences and Restriction-Site PCR. J Virol Methods (2014) 206:51–4. doi: 10.1016/j.jviromet.2014.05.017
Keywords: hnRNP E1, cervical cancerization, HPV16 E6, HPV16 E2/E6 ratio, regulation
Citation: Song L, Mao R, Ding L, Tian Z, Zhang M, Wang J, Wang M, Lyu Y, Liu C, Feng M, Jia H and Wang J (2022) hnRNP E1 Regulates HPV16 Oncogene Expression and Inhibits Cervical Cancerization. Front. Oncol. 12:905900. doi: 10.3389/fonc.2022.905900
Received: 28 March 2022; Accepted: 24 May 2022;
Published: 21 June 2022.
Edited by:
Elena Ioana Braicu, Charité Universitätsmedizin Berlin, GermanyReviewed by:
N. Sanjib Banerjee, University of Alabama at Birmingham, United StatesCopyright © 2022 Song, Mao, Ding, Tian, Zhang, Wang, Wang, Lyu, Liu, Feng, Jia and Wang. This is an open-access article distributed under the terms of the Creative Commons Attribution License (CC BY). The use, distribution or reproduction in other forums is permitted, provided the original author(s) and the copyright owner(s) are credited and that the original publication in this journal is cited, in accordance with accepted academic practice. No use, distribution or reproduction is permitted which does not comply with these terms.
*Correspondence: Jintao Wang, wangjt59@163.com
Disclaimer: All claims expressed in this article are solely those of the authors and do not necessarily represent those of their affiliated organizations, or those of the publisher, the editors and the reviewers. Any product that may be evaluated in this article or claim that may be made by its manufacturer is not guaranteed or endorsed by the publisher.
Research integrity at Frontiers
Learn more about the work of our research integrity team to safeguard the quality of each article we publish.