- 1Department of Radiation Oncology, The James Comprehensive Cancer Center, Ohio State University, Columbus, OH, United States
- 2Department of Radiation Oncology, The University of Texas MD Anderson Cancer Center, Houston, TX, United States
- 3Department of Radiation Oncology, Loyola University, Chicago, IL, United States
- 4Department of Radiation Oncology, Virginia Commonwealth University, Richmond, VA, United States
- 5Department of Pediatrics, Nationwide Children’s Hospital, Columbus, OH, United States
- 6Department of Radiation Oncology, University Hospitals Seidman Cancer Center, Case Western Reserve School of Medicine, Cleveland, OH, United States
- 7Department of Radiation Oncology, Mayo Clinic, Jacksonville, FL, United States
Background: Central nervous system tumors are now the most common primary neoplasms seen in children, and radiation therapy is a key component in management. Secondary malignant neoplasms (SMNs) are rare, but dreaded complications. Proton beam therapy (PBT) can potentially minimize the risk of SMNs compared to conventional photon radiation therapy (RT), and multiple recent studies with mature data have reported the risk of SMNs after PBT. We performed this systematic review and meta-analysis to characterize and compare the incidence of SMNs after proton and photon-based radiation for pediatric CNS tumors.
Methods: A systematic search of literature on electronic (PubMed, Cochrane Central, and Embase) databases was conducted in accordance with the Preferred Reporting Items for Systematic Reviews and Meta-Analyses (PRISMA) method. We included studies reporting the incidence and nature of SMNs in pediatric patients with primary CNS tumors. The crude incidence of SMNs and all secondary neoplasms were separately extracted, and the random-effects model was used for pooled analysis and subgroup comparison was performed between studies using photons vs. protons.
Results: Twenty-four studies were included for analysis. A total of 418 SMNs were seen in 38,163 patients. The most common SMN were gliomas (40.6%) followed by meningiomas (38.7%), sarcomas (4.8%), and thyroid cancers (4.2%). The median follow-up was 8.8 years [3.3–23.2].The median latency to SMN for photons and protons were 11.9 years [5-23] and 5.9 years [5-6.7], respectively. The pooled incidence of SMNs was 1.8% (95% CI: 1.1%–2.6%, I2 = 94%) with photons and 1.5% (95% CI: 0%–4.5%, I2 = 81%) with protons. The pooled incidence of all SNs was not different [photons: 3.6% (95% CI: 2.5%–4.8%, I2 = 96%) vs. protons: 1.5% (95% CI: 0–4.5%, I2 = 80%); p = 0.21].
Conclusion: We observed similar rates of SMN with PBT at 1.5% compared to 1.8% with photon-based RT for pediatric CNS tumors. We observed a shorter latency to SMN with PBT compared to RT. With increasing use of pencil beam scanning PBT and VMAT, further studies are warranted to evaluate the risk of secondary cancers in patients treated with these newer modalities.
Introduction
Central nervous system (CNS) tumors are now the most common neoplasms seen in children and adolescents, comprising about one-fourth of all childhood cancers, and remain the leading cause of cancer-related deaths in this population (1–3). Of these, the most common tumor types seen are glioma, medulloblastoma, and germ cell tumors. Radiation therapy (RT) is a key component in multimodal management of most of these tumors. Although survival outcomes in children have improved with advances in radiation techniques, treatment-related late effects continue to be a concern. Secondary malignant neoplasms (SMNs) are one of the most dreaded complications, particularly in the pediatric population given significantly higher rates of SMNs noted than in adults following RT (4). The cumulative risk of secondary malignant brain tumors after therapeutic photon-based cranial irradiation has been reported to be 0.5%–3.7% at 10–15 years (5, 6). Recent strategies to decrease the integral dose and subsequently minimize late toxicities in the pediatric population include the use of more conformal RT techniques such as intensity-modulated radiation therapy (IMRT). However, even with more targeted photon-based therapy, higher integral doses due to more monitor units, leakage radiation, and higher volume of tissues exposed to low-dose radiation can result in more SMNs (7).
An emerging area of interest to minimize the risk of SMNs after RT is proton beam therapy (PBT). The lack of an exit dose with proton therapy due to the Bragg peak phenomenon may reduce the risk of SMNs in the pediatric population (8). This is especially useful in patients with medulloblastoma who often receive craniospinal irradiation leading to increased exposure of thoracic and abdominopelvic structures to RT. Although protons theoretically should be associated with a lower risk of SMNs, secondary neutrons resulting from various apparatuses along the proton beamline as well as the radiobiologic uncertainties have been concerning (9). Secondary cancers commonly occur >5–10 years after RT (10). The widespread use of proton therapy in the United States started at the beginning of the 21st century and only recently have mature data been available with adequate follow-up to estimate late effects of protons. Hence, there are limited data comparing the incidence of SMNs following photon based RT vs. PBT.
It becomes difficult to establish the true incidence of SMN due to the fact that in addition to radiation exposure, the genetic abnormalities (e.g., Li-Fraumeni syndrome) and risk factors associated with primary tumors (e.g., smoking) could predispose the individuals to develop a second cancer. Given that radiation is often the standard of care or an important salvage treatment for most CNS tumors, it becomes challenging to have randomized or matched cohorts comparing the risk of secondary cancers in irradiated and non-irradiated patients. Thus, we undertook this systematic review to evaluate the current literature on secondary cancers and to characterize and compare the incidence of SMNs after proton and photon-based radiation for pediatric CNS tumors.
Methods
A systematic search of literature was conducted according to the Preferred Reporting Items for Systematic Reviews and Meta-Analyses (PRISMA) method. Supplementary Table 1 shows the PRISMA checklist. The PubMed (National Institutes of Health), Cochrane Central (Cochrane collaboration), and Embase (Elsevier) databases were queried with the Medical Subject Heading (MeSH) terms—”secondary malignant neoplasm or second primary or radiation-induced”, “radiotherapy or radiation or irradiation”, “child or pediatric or adolescent”, and “brain or nervous system neoplasm”. Appropriate synonyms were determined and searched for as text in article titles, abstracts, and keywords. The search did not have a language filter. After removing duplicates, a total of 1,168 articles were identified. RU and JP did the search independently and any disagreements were resolved by mutual discussion.
Eligibility criteria for articles
The inclusion criteria were as follows: (i) any prospective, retrospective, or cohort study reporting the incidence and nature of SMNs in a cohort of at least 10 pediatric patients with primary CNS tumors; (ii) study should include patients with age <21 years; (iii) original article in English language or an available translated version.
The exclusion criteria were as follows: (i) review articles and isolated case reports; (ii) preclinical and modeling studies without clinical information; (iii) studies describing only benign secondary tumors such as meningiomas and pituitary adenomas, or secondary skin cancers alone; (iv) papers with incomplete, missing, or duplicated data. Studies reporting the incidence of SMN in a cohort inclusive of significant number of patients not receiving RT were excluded as well (11–14). We did not require a minimum median follow-up duration as most proton literature have less long-term follow-up data available.
Article review
The search process was performed consistent with the PRISMA flow diagram shown in Figure 1. The articles from the initial search were imported into Reference Manager Software. The duplicates were excluded, and the titles of articles were evaluated. The articles were independently reviewed by two authors (RU and JP), and relevant studies were identified. The articles found to be relevant to the topic of interest were shortlisted, and the full-length paper of these articles was assessed for the eligibility criteria. The included study references were cross-searched for additional studies. SMNs were defined based on Cahan’s criteria described by a classic study examining sarcomas developed in irradiated bone (15): (1) history of radiation exposure and presence of tumor in a previously irradiated region, (2) sufficient latency time between the original and new tumors, (3) histology of the new tumor must be distinct from the original that is typically seen as a second neoplasm, and (4) the tissue in which the alleged induced tumor arose must have been normal (i.e., metabolically and genetically) prior to the radiation exposure. For eligible studies, data were extracted as available, including the total number of patients, pediatric patients, radiation modality, median follow-up, incidence and cumulative incidence of SMNs, initial and secondary tumor types, median interval between RT and occurrence of SMNs, and the location of SMNs with respect to the radiation target. Secondary “malignant” neoplasms and all secondary neoplasms (SNs) were separately assessed and analyzed. SMNs included neoplasms like high-grade gliomas, sarcomas, thyroid cancers, and basal cell carcinoma, while benign neoplasms like meningioma, thyroid adenoma, osteoma, and desmoid tumors were included as SNs but not SMNs.
Statistical analysis
Crude incidence of SMNs and all secondary malignancies were separately tabulated along with 95% confidence intervals, and the random-effects model described by DerSimonian and Laird was used for pooled analysis of incidence. The study-specific incidence rates were standardized to a cumulative incidence over 10 years for each study. The forest plot illustrating this information was plotted by the generic inverse variance method, the Jackson method for confidence intervals of tau and tau (2). Study heterogeneity was assessed using the inconsistency index (I2 statistic) with values of 0%–30%, 31%–60%, 61%–75%, and 76–100% indicating low, moderate, substantial, and considerable heterogeneity, respectively. A subgroup comparison was performed between studies using photons and protons. Meta-analyses were performed using R 3.6.1 (https://www.r-project.org/; Vienna, Austria), R package meta was used for meta-analysis, and statistical significance was set at p < 0.05 (16).
Results
An electronic search of PubMed, Embase, and Cochrane Central identified 1,744 articles. Figure 1 describes the PRISMA flow diagram of the search strategy. A cross-reference search of included studies further identified 11 new studies. After removal of duplicates, 1,168 abstracts were assessed. After assessing the title and/or abstracts, 71 articles were found to be relevant to the research question. The full-length articles of these studies were assessed, and 24 studies were found to fit the inclusion criteria (5, 16–38). Notable exclusions were case reports, review articles, and studies in preclinical models. Studies reporting the incidence of SMNs in a non-pediatric population were also reviewed for systematic review but not for meta-analysis. In studies reporting SMNs in all age groups and other tumor types, data for only pediatric patients with primary CNS tumors were extracted from the available segregated data. The studies with possible overlap were also excluded. Among the included studies, there was a potential overlap between patient populations only between two studies by Neglia et al. (2006) and Armstrong et al. (2009), who reported second cancers on the St. Jude’s Childhood Cancer Survivor Study (CCSS). The Newcastle–Ottawa scale assessment was used to assess the quality of the studies included in the systematic review and is presented in the supplementary data (Supplementary Table 2).
A total of 418 SMNs and 645 any secondary neoplasms were seen in 38,163 patients overall. The most common secondary neoplasms seen were gliomas (40.6%) followed by meningioma (38.7%), sarcomas (4.8%), thyroid cancer (4.2%), and basal cell carcinoma (1.3%) (Table 1). The overall median follow-up was 8.8 years (range: 3.3 to 23.2 years), while the median latency to a secondary cancer was 9.8 years (range: 5 to 23 years). Twenty-one studies (n = 36,763) reported the incidence of SMNs with photons (6, 17–36), while four studies reported the incidence of SMNs after protons (36–39) (n = 1,400). Table 1 summarizes the details of these studies. The median follow-up for photons and protons was 8.8 years (range: 3.5 to 23.2 years) and 6.9 years (range: 3.3 to 12.8 years), respectively, while the median latency to a secondary cancer was 11.9 years (range: 5 to 23 years) and 5.9 years (range: 5 to 6.7 years), respectively.
The pooled random-effects incidence of SMNs with photons was 1.8% (95% CI: 1.1%–2.6%, I2 = 94%) while with protons it was 1.5% (95% CI: 0%–4.5%, I2 = 81%) (Figure 2). There was no significant difference among the two subgroups (p = 0.91). The pooled random-effects incidence of all secondary malignancies was also slightly lower with protons compared to photons, but the difference was not statistically different among the two groups [photons: 3.6% (95% CI: 2.5%–4.8%, I2 = 96%) vs. protons: 1.5% (95% CI: 0–4.5%, I2 = 80%); p = 0.21] (Figure 3). Supplementary Figure 1 shows the funnel plots demonstrating publication bias for the respective forest plots.
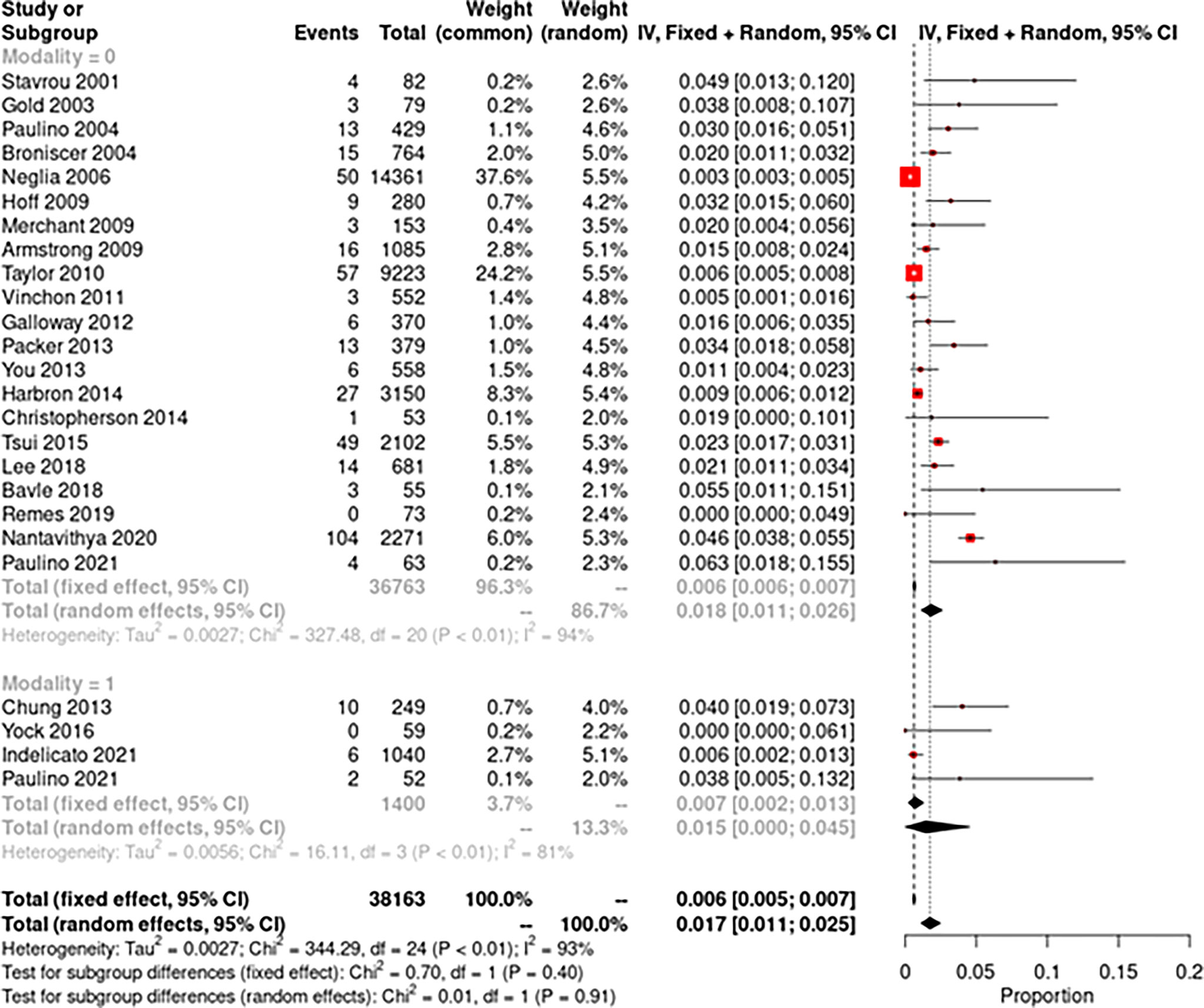
Figure 2 Forest plot demonstrating pooled analysis of secondary malignant neoplasms with photons (modality = 0) and protons (modality = 1).
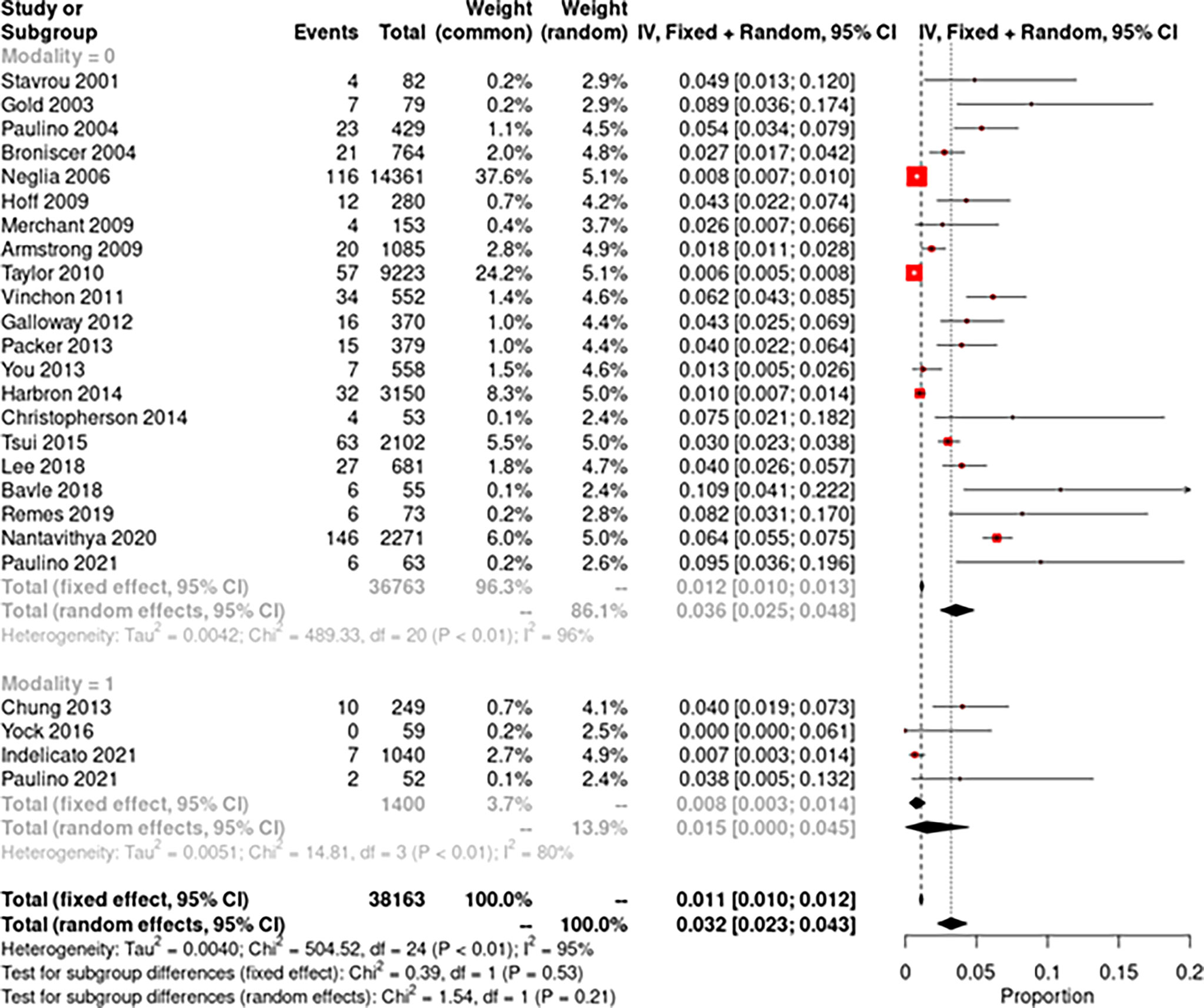
Figure 3 Forest plot demonstrating pooled analysis of all secondary neoplasms with photons (modality = 0) and protons (modality = 1).
Incidence of SMNs with photon therapy
Early studies described radiation-induced neoplasms with low-dose RT in children who received RT for tinea capitis (mean dose 1.5 Gy) (40, 41). Meningiomas were the most common tumors seen with a relative risk (RR) of 9.5 compared to the general population, while that for glioma was 2.6. More recently, Packer et al. assessed the incidence of secondary tumors in 379 children with medulloblastoma treated per the COG-A9961 trial and estimated a 10-year cumulative incidence of 4.2% for SMNs (28). Bavle et al. found a 10-year cumulative incidence of 6.1% for all secondary neoplasms, 3.1% for secondary benign neoplasms, and 3.7% for SMNs in a retrospective study and meta-analysis including 1,114 patients (6). Similar studies in ependymoma survivors have shown a 7-year cumulative incidence of 2.3% (23), and those in germinoma survivors have shown an 11-year cumulative incidence of 9% (42). However, in the latter study, only one out of five second cancers were malignant, while the other four were benign meningiomas.
Incidence of SMNs with proton therapy
Indelicato et al. assessed the risk of SMNs in 1,713 pediatric patients (1,040 CNS tumors) treated with passive scatter proton therapy and found a 5- and 10-year cumulative incidence of SMNs to be 0.8% and 3.1%, respectively. Paulino et al. (36) analyzed 115 children with medulloblastomas <18 years of age who received craniospinal irradiation with either photon craniospinal irradiation (CSI) and an intensity-modulated RT boost (n = 63) or passively scattered proton CSI and a boost (n = 52). They observed a 5- and 10-year risk of SMN to be 1% and 6.9%, respectively, without a difference in incidence by RT group (p = 0.74). There was no difference in the distribution of SMNs according to sex, age at RT (≤7 or >7 years), risk category, CSI dose (18.0–23.4 vs. 30.6–39.6 Gy), RT modality, or type of chemotherapy (SJMB vs. COG and other). Interestingly, SMNs after PBT occurred earlier at 2.5–5.5 years after RT, while SMNs after photon therapy occurred later at 6–12 years after RT. Of note, two SMNs occurred in the clinical target volume in the brain (malignant glioneuronal tumor, glioblastoma), two occurred in the exit dose region from the photon spinal field (papillary thyroid cancer, cardiac tumor), one occurred in the entrance path of a proton beam (parotid mucoepidermoid cancer), and one occurred outside the radiation field (testicular germ cell tumor).
Chung et al. (37) have compared the incidence of second cancers in patients who underwent proton therapy for a variety of diagnoses with a population-based cohort of matched patients treated with photon radiation. They matched 558 proton patients with 558 photon patients from the Surveillance, Epidemiology and End Results registry; one-third of the patients evaluated had a primary tumor of the central nervous system. The median duration of follow-up/latency time to second malignancy was 6.7/6.0 years for proton patients and 6.0/4.75 years for photon patients. Of note, the patients in the proton cohort often received a portion of their treatment with photons. The 10-year cumulative incidence rates for second malignancies were 5.4% for proton patients and 8.6% for photon patients. After adjusting for age, sex, year of diagnosis, and primary site, proton therapy was not related with an increased risk of second tumor. Second malignancies were seen up to 23 years after proton therapy, again emphasizing the need for a longer follow-up to determine the true incidence.
Discussion
In our meta-analysis of 24 studies, the pooled incidence of SMNs in childhood cancers treated with photon-based radiation techniques was 1.8%, compared to 1.5% with protons. The reported overall cumulative incidence of SMN at 10 years across studies ranged from 1.4% to 8.9% for photons, versus 0% to 5.4% with protons. We did not find a statistically significant difference between the two groups with respect to SMNs or any SNs. The median follow-up for studies reporting outcomes with proton therapy was slightly shorter at 6.9 years compared to 8.8 years with photons. Also, the secondary cancers seem to occur after a shorter latency after RT with protons, with a median of 5.9 years, compared to 11.9 years with photons. Similar results have been reported by Paulino et al., who compared the risk of secondary cancers after proton- or photon-based CSI for pediatric medulloblastoma patients (36). It has also been previously reported that radiation-induced large-vessel vasculopathy is observed earlier after proton therapy for pediatric CNS tumors at a median of 1.5 years than with photon therapy where the median time to development of vasculopathy is 5 years (43). One reason for this finding could be exposure to secondary neutrons with proton therapy (9). Neutrons have different radiobiological properties with a higher linear energy transfer and may cause earlier onset of late effects.
Several preclinical studies have modeled the risk of SMNs after proton therapy. Arvold et al. calculated second tumor rates in 10 patients with benign intracranial meningiomas treated with PBT at Massachusetts General Hospital based on dosimetric comparisons between proton RT and photon RT treatment plans. They observed that the relative risk of second tumors was significantly lower for PBT compared to photon plans (1.3 vs. 2.8 per 10,000 patients per year, p < 0.002) (44). The excess risk of an intracranial RT-associated second tumor was calculated using the method proposed by Schneider et al., based on the concept of organ equivalent dose (45). Several other modeling studies have demonstrated a similar reduced risk of second tumors after PBT compared to photon radiation (46, 47). In another modeling study, PBT decreased the expected incidence of radiation-induced secondary cancers for rhabdomyosarcoma by a factor of 2 and for medulloblastoma by a factor of 8 to 15 when compared to intensity-modulated RT and conventional photon-based RT, respectively (48). Despite these modeling studies suggesting dosimetric advantages of protons in preventing SMNs, an important caveat is that in order to achieve this benefit protons must be delivered by pencil beam scanning rather than passive scattering technique. The passive modulation proton technique can potentially expose the patient to an even higher dose of radiation distant from the target due to secondary neutron production from the scattering foil (7). Neutrons can lead to considerable contribution to the integral dose in particular, since neutrons have a large quality factor and thus even a small physical dose can result in considerable biological effects (49). This at least in part may negate the Bragg peak effect of PBT and balances the overall integral dose. This is a potential reason for similar rates of SMN seen in our study in the two groups. In contrast, active scanning sweeps a fine pencil beam through the target and fewer neutrons are produced in the patient itself.
One of the major risk factors for development of SMNs is younger age at the time of RT. Age at RT of <7 years and craniospinal irradiation significantly increased the risk of a secondary tumor (p <.05) in a cohort of 681 patients (33). Also in this study, secondary tumors developed in 11 of 128 patients (8.6%) with primary medulloblastomas, which was higher than the overall cumulative incidence (33). This is suggestive of the impact of radiation volume, which is significantly higher for medulloblastomas, on incidence of SMN as well. Another major factor associated with SMNs is genetic predisposition, for example Li-Fraumeni syndrome. A majority of patients developing basal cell carcinoma had underlying Gorlin syndrome or multiple-nevus syndrome. There is heterogeneity among several studies in defining SMNs, as some studies exclude genetically predisposed patients who develop SMNs while most others do not.
There is strong evidence that secondary tumors are more frequent in patients who receive both radiotherapy and chemotherapy (50). Although we excluded the studies reporting incidence of SMN in patients treated with chemotherapy alone, some of the secondary cancers seen in these studies especially hematological malignancies could be related to chemotherapy use, which was not separately analyzed, and could be one of the potential confounding factors on this study.
The natural history of the primary tumor affects the incidence of SBT; patients need to live sufficiently long to develop a SMN. The latency for benign neoplasms such as meningiomas is usually much longer than for malignant neoplasms such as high-grade glioma and sarcomas. The latency period for secondary tumors ranges from 5.5 to 30 years, with gliomas developing in 5–10 years and meningiomas developing around 15–20 years after radiation (51). Paulino et al. observed that male gender, cranial irradiation for leukemia, and use of craniospinal or whole-brain radiation were associated with a shorter latent time from RT to development of a meningioma, while patients receiving lower doses of RT had a longer latent time (51).
Among radiation-induced CNS neoplasms in adults, meningiomas are about 70%, gliomas about 20%, and sarcomas less than 10%. In children, the most common secondary neoplasm is malignant glioma, comprising around 40%–50% of all SMNs (52). We found a similar distribution in our study. For adult patients, a rough estimate of secondary malignancy of 0.1%–1% per decade after radiation is often quoted, extrapolated from long-term follow-up of patients treated for prostate (53) and cervical cancer (54) where surgical controls were compared to their radiation counterparts. Xiang et al. compared the risk of second cancers in 450,373 pediatric and adult patients who received 3D-CRT, IMRT, or PBT from the National Cancer Database (NCDB) (55). The overall incidence of SMN was 1.55 per 100 patient-years. There was no difference in the risk of second cancers between IMRT vs. 3D-CRT (adjusted OR = 1; 95% CI = 0.97–1.02), but PBT had an overall significantly lower risk compared to IMRT (adjusted OR = 0.31; 95% CI = 0.26–0.36). They also verified the consistency of results in propensity score-matched analyses, and overall, this study represents strong evidence suggesting a lower risk of SMNs with PBT, albeit with limitations of NCDB data completeness.
There are several limitations of this systematic review and meta-analysis, the most prominent of which is the high heterogeneity evident by the high I2 statistic. This is likely due to more and larger studies demonstrating the incidence of SMNs after photon therapy compared to studies reporting outcomes after PBT. Also, there is a heterogenous patient population across different studies, and heterogeneity in the treatment regimen with regard to chemotherapy, radiation modality, doses, and volumes. The overall follow-up with PBT is shorter than most of the photon cohorts, which may bias the true incidence of SMNs. Another major limitation is the different time periods of comparison. Assessment of SMNs often requires a long-term follow-up, which means our oldest study ranges from 1999 to 2021. The natural history of primary tumor can affect the incidence of SMNs as patients need to live sufficiently long enough to develop an SMN. In this context, improved survival with modern systemic drugs and surgical and RT techniques can impact the incidence of SMNs. In addition, among patients treated in recent eras of improved survivorship care including routine surveillance imaging, it is possible that this may lead to perhaps earlier or increased detection of SMNs. Finally, another important factor associated with SMNs is genetic predisposition. Nearly 10% children with pediatric cancers harbor cancer-predisposing genes (56), and this has not been clearly reported in these studies.
Future recommendations
It has been conventionally suggested that secondary cancers often develop in tissues that receive a lower radiation dose or “low dose spill-off” receiving <2.5 Gy (11). This is also supported by the incidence of brain tumors after only diagnostic x-ray exposure (57). However, in a recent study by Galloway et al., the most common location of the second tumor was in the whole-brain field (57%) and in the moderate-dose range receiving 20–36 Gy (81%) (27). These findings suggest that along with reducing the low-dose area, decreasing the volume of brain receiving moderate radiation doses can substantially decrease the second tumor rates. This can be potentially accomplished by more conformal radiation techniques such as VMAT and PBS proton therapy. It has been predicted that IMRT can increase the risk of SMN due to more volume of irradiated tissue, especially low-dose bath and increased total monitor units delivered (7, 58), but clinical studies have not demonstrated a similar increased risk (55, 59). Also, limited studies have evaluated the use of volumetric arc modulated radiotherapy (VMAT), which is being used increasingly for medulloblastoma patients. VMAT is able to confine the CSI dose to the spine with less dose anteriorly in the thorax, abdomen, and pelvis. Treatment times are also faster compared with older methods of IMRT, with less leakage radiation expected, although there remains the disadvantage of a larger volume of normal tissue receiving low-dose RT. Similarly with proton therapy, the lower risk of SMNs is expected with pencil beam scanning proton therapy (PBS) and not passively scattered PBT because of an increased total body dose due to secondary neutrons in the latter (7, 60). A more frequent use of VMAT and PBS proton therapy, both of which decrease the irradiated tissue volume outside the clinical target volume, is recommended. In particular, for patients with medulloblastoma, treatment plans and protocols should focus on minimizing the amount of normal tissue receiving low to medium doses in the range of 20–36 Gy, by decreasing the craniospinal dose while keeping a posterior fossa boost dose similar. Continued avoidance of whole-brain RT for pediatric leukemia patients is recommended. Another approach to decrease low–medium-dose radiation exposure is to reduce the CTV margins and minimize PTV expansions with image guidance. In this regard, recent data from the ACNS0331 trial suggest changing the boost CTV volume from the entire posterior fossa to the tumor bed only and the ACNS 0831 study suggests decreasing boost margins in ependymoma without compromising local control and survival (61, 62). This aims to further decrease the risk of secondary cancers along with other normal tissue toxicities (63).
Conclusion
Despite advances in radiation techniques, the risk of late secondary malignancies remains a concern, especially in the pediatric population. We observed similar rates of SMN with PBT at 1.5% compared to 1.8% with photon-based RT for pediatric CNS tumors in our meta-analysis of 24 studies, and the difference was not statistically significant. The risk of all secondary cancers was also lower, but the difference was not statistically significant. We observed a shorter latency to secondary cancers with proton therapy compared to photon-based radiation, which may be related to secondary neutron exposure. With increasing use of techniques that decrease the irradiated tissue volume outside the clinical target volume, like pencil beam scanning proton therapy and VMAT, further studies with a longer follow-up are warranted to evaluate the risk of secondary cancers in patients treated with these newer modalities.
Data availability statement
The original contributions presented in the study are included in the article/Supplementary Material. Further inquiries can be directed to the corresponding author.
Author contributions
RU, DY, BV and JP all conducted the literature search, data analysis. All authors performed data interpretation, manuscript writing and editing. All authors reviewed and accept the final manuscript.
Conflict of interest
The authors declare that the research was conducted in the absence of any commercial or financial relationships that could be construed as a potential conflict of interest.
Publisher’s note
All claims expressed in this article are solely those of the authors and do not necessarily represent those of their affiliated organizations, or those of the publisher, the editors and the reviewers. Any product that may be evaluated in this article, or claim that may be made by its manufacturer, is not guaranteed or endorsed by the publisher.
Supplementary material
The Supplementary Material for this article can be found online at: https://www.frontiersin.org/articles/10.3389/fonc.2022.893855/full#supplementary-material
References
1. American Cancer Society. Cancer facts & figures 2019. Atlanta, Ga: American Cancer Society (2019).
2. Scheurer ME, Lupo PJ, Bondy ML. Chapter 1: Epidemiology of childhood cancer. In: Pizzo PA, Poplack DG, editors. Principles and practice of pediatric oncology, 7th ed. Philadelphia, Pa: Lippincott Williams & Wilkins (2016).
3. Ostrom QT, Patil N, Cioffi G, Waite K, Kruchko C, Barnholtz-Sloan JS. CBTRUS statistical report: Primary brain and other central nervous system tumors diagnosed in the united states in 2013–2017. Neuro Oncol (2020) 22(Suppl 1):iv1–iv96. doi: 10.1093/neuonc/noaa200
4. Smith H, Published on behalf of the lnternational Commission on Radiological Protection. International commission on radiological protection. recommendations Vol. 211. . Ottawa, Canada: Annals of the ICRP Publication (1990). p. 60.
5. Paulino AC, Mai WY, Chintagumpala M, Taher A, Teh BS. Radiation-induced malignant gliomas: is there a role for reirradiation? Int J Radiat Oncol Biol Phys (2008) 71(5):1381–7. doi: 10.1016/j.ijrobp.2007.12.018
6. Bavle A, Tewari S, Sisson A, Chintagumpala M, Anderson M, Paulino AC. Meta-analysis of the incidence and patterns of second neoplasms after photon craniospinal irradiation in children with medulloblastoma. Pediatr Blood Cancer (2018) 65(8):e27095. doi: 10.1002/pbc.27095
7. Hall EJ. Intensity-modulated radiation therapy, protons, and the risk of second cancers. Int J Radiat Oncol Biol Phys (2006) 65(1):1–7. doi: 10.1016/j.ijrobp.2006.01.027
8. Hall EJ. The impact of protons on the incidence of second malignancies in radiotherapy. Technol Cancer Res Treat (2007) 6(4 Suppl):31–4. doi: 10.1177/15330346070060S405
9. Hälg RA, Schneider U. Neutron dose and its measurement in proton therapy-current state of knowledge. Br J Radiol (2020) 93(1107):20190412. doi: 10.1259/bjr.20190412
10. Yonehara S, Brenner AV, Kishikawa M, Inskip PD, Preston DL, Ron E, et al. Clinical and epidemiologic characteristics of first primary tumors of the central nervous system and related organs among atomic bomb survivors in Hiroshima and Nagasaki, 1958-1995. Cancer (2004) 101(7):1644–54. doi: 10.1002/cncr.20543
11. Diallo I, Haddy N, Adjadj E, Samand A, Quiniou E, Chavaudra J, et al. Frequency distribution of second solid cancer locations in relation to the irradiated volume among 115 patients treated for childhood cancer. Int J Radiat Oncol Biol Phys (2009) 74(3):876–83. doi: 10.1016/j.ijrobp.2009.01.040
12. de Vathaire F, Hawkins M, Campbell S, Oberlin O, Raquin MA, Schlienger JY, et al. Second malignant neoplasms after a first cancer in childhood: temporal pattern of risk according to type of treatment. Br J Cancer (1999) 79(11):1884–93. doi: 10.1038/sj.bjc.6690300
13. Little MP, de Vathaire F, Shamsaldin A, Oberlin O, Campbell S, Grimaud E, et al. Risks of brain tumour following treatment for cancer in childhood: Modification by genetic factors, radiotherapy and chemotherapy. Int J Cancer (1998) 78(3):269–75. doi: 10.1002/(SICI)1097-0215(19981029)78:3<269::AID-IJC1>3.0.CO;2-T
14. Friedman DL, Whitton J, Leisenring W, Mertens AC, Hammond S, Stovall M, et al. Subsequent neoplasms in 5-year survivors of childhood cancer: the childhood cancer survivor study. J Natl Cancer Inst (2010) 102(14):1083–95. doi: 10.1093/jnci/djq238
15. Cahan WG, Woodard HQ, Higinbotham NL, Stewart FW, Coley BL. Sarcoma arising in irradiated bone: report of eleven cases. 1948 Cancer (1998) 82(1):8–34. doi: 10.1002/(sici)1097-0142(19980101)82:1<8::aid-cncr3>3.0.co;2-w
16. R: The r project for statistical computing. Available at: https://www.r-project.org/ (Accessed December 28, 2021).
17. Stavrou T, Bromley CM, Nicholson HS, Byrne J, Packer RJ, Goldstein AM, et al. Prognostic factors and secondary malignancies in childhood medulloblastoma. J Pediatr Hematology/Oncol (2001) 23(7):431–6. doi: 10.1097/00043426-200110000-00008
18. Gold DG, Neglia JP, Dusenbery KE. Second neoplasms after megavoltage radiation for pediatric tumors. Cancer (2003) 97(10):2588–96. doi: 10.1002/cncr.11356
19. Paulino AMC, Fowler BZ. Secondary neoplasms after radiotherapy for a childhood solid tumor. Pediatr Hematol Oncol (2005) 22(2):89–101. doi: 10.1080/08880010590896459
20. Broniscer A, Ke W, Fuller CE, Wu J, Gajjar A, Kun LE. Second neoplasms in pediatric patients with primary central nervous system tumors: The st. Jude children’s research hospital experience. Cancer (2004) 100(10):2246–52. doi: 10.1002/cncr.20253
21. Neglia JP, Robison LL, Stovall M, Liu Y, Packer RJ, Hammond S, et al. New primary neoplasms of the central nervous system in survivors of childhood cancer: a report from the childhood cancer survivor study. J Natl Cancer Inst (2006) 98(21):1528–37. doi: 10.1093/jnci/djj411
22. von Hoff K, Hinkes B, Gerber NU, Deinlein F, Mittler U, Urban C, et al. Long-term outcome and clinical prognostic factors in children with medulloblastoma treated in the prospective randomised multicentre trial HIT’91. Eur J Cancer (2009) 45(7):1209–17. doi: 10.1016/j.ejca.2009.01.015
23. Merchant TE, Li C, Xiong X, Kun LE, Boop FA, Sanford RA. Conformal radiotherapy after surgery for paediatric ependymoma: a prospective study. Lancet Oncol (2009) 10(3):258–66. doi: 10.1016/s1470-2045(08)70342-5
24. Armstrong GT, Liu Q, Yasui Y, Huang S, Ness KK, Leisenring W, et al. Long-term outcomes among adult survivors of childhood central nervous system malignancies in the childhood cancer survivor study. J Natl Cancer Inst (2009) 101(13):946–58. doi: 10.1093/jnci/djp148
25. Taylor AJ, Little MP, Winter DL, Sugden E, Ellison DW, Stiller CA, et al. Population-based risks of CNS tumors in survivors of childhood cancer: the British childhood cancer survivor study. J Clin Oncol (2010) 28(36):5287–93. doi: 10.1200/JCO.2009.27.0090
26. Vinchon M, Leblond P, Caron S, Delestret I, Baroncini M, Coche B. Radiation-induced tumors in children irradiated for brain tumor: a longitudinal study. Childs Nerv Syst (2011) 27(3):445–53. doi: 10.1007/s00381-011-1390-4
27. Galloway TJ, Indelicato DJ, Amdur RJ, Morris CG, Swanson EL, Marcus RB. Analysis of dose at the site of second tumor formation after radiotherapy to the central nervous system. Int J Radiat Oncol Biol Phys (2012) 82(1):90–4. doi: 10.1016/j.ijrobp.2010.10.062
28. Packer RJ, Zhou T, Holmes E, Vezina G, Gajjar A. Survival and secondary tumors in children with medulloblastoma receiving radiotherapy and adjuvant chemotherapy: results of children’s oncology group trial A9961. Neuro Oncol (2013) 15(1):97–103. doi: 10.1093/neuonc/nos267
29. You SH, Lyu CJ, Kim DS, Suh CO. Second primary brain tumors following cranial irradiation for pediatric solid brain tumors. Childs Nerv Syst (2013) 29(10):1865–70. doi: 10.1007/s00381-013-2098-4
30. Harbron RW, Feltbower RG, Glaser A, Lilley J, Pearce MS. Secondary malignant neoplasms following radiotherapy for primary cancer in children and young adults. Pediatr Hematol Oncol (2014) 31(3):259–67. doi: 10.3109/08880018.2013.838723
31. Christopherson KM, Rotondo RL, Bradley JA, Pincus DW, Wynn TT, Fort JA, et al. Late toxicity following craniospinal radiation for early-stage medulloblastoma. Acta Oncol (2014) 53(4):471–80. doi: 10.3109/0284186X.2013.862596
32. Tsui K, Gajjar A, Li C, Srivastava D, Broniscer A, Wetmore C, et al. Subsequent neoplasms in survivors of childhood central nervous system tumors: risk after modern multimodal therapy. Neuro-Oncology (2015) 17(3):448–56. doi: 10.1093/neuonc/nou279
33. Lee CY, Chen YW, Lee YY, Chang FC, Chen HH, Lin SC, et al. Irradiation-induced secondary tumors following pediatric central nervous system tumors: Experiences of a single institute in Taiwan (1975-2013). Int J Radiat Oncol Biol Phys (2018) 101(5):1243–52. doi: 10.1016/j.ijrobp.2018.04.032
34. Remes TM, Suo-Palosaari MH, Heikkilä VP, Sutela AK, Koskenkorva PKT, Toiviainen-Salo SM, et al. Radiation-induced meningiomas after childhood brain tumor: A magnetic resonance imaging screening study. J Adolesc Young Adult Oncol (2019) 8(5):593–601. doi: 10.1089/jayao.2019.0010
35. Nantavithya C, Paulino AC, Liao K, McGovern SL, Grosshans DR, McAleer MF, et al. Development of second primary tumors and outcomes in medulloblastoma by treatment modality: A surveillance, epidemiology, and end results analysis. Pediatr Blood Cancer (2020) 67(8):e28373. doi: 10.1002/pbc.28373
36. Paulino AC, Ludmir EB, Grosshans DR, Su JM, McGovern SL, Okcu MF, et al. Overall survival and secondary malignant neoplasms in children receiving passively scattered proton or photon craniospinal irradiation for medulloblastoma. Cancer (2021) 127(20):3865–71. doi: 10.1002/cncr.33783
37. Chung CS, Yock TI, Nelson K, Xu Y, Keating NL, Tarbell NJ. Incidence of second malignancies among patients treated with proton versus photon radiation. Int J Radiat Oncol Biol Phys (2013) 87(1):46–52. doi: 10.1016/j.ijrobp.2013.04.030
38. Yock TI, Yeap BY, Ebb DH, Weyman E, Eaton BR, Sherry NA, et al. Long-term toxic effects of proton radiotherapy for paediatric medulloblastoma: a phase 2 single-arm study. Lancet Oncol (2016) 17(3):287–98. doi: 10.1016/S1470-2045(15)00167-9
39. Indelicato DJ, Bates JE, Mailhot Vega RB, Rotondo RL, Hoppe BS, Morris CG, et al. Second tumor risk in children treated with proton therapy. Pediatr Blood Cancer (2021) 68(7):e28941. doi: 10.1002/pbc.28941
40. Ron E, Modan B, Boice JD, Alfandary E, Stovall M, Chetrit A, et al. Tumors of the brain and nervous system after radiotherapy in childhood. N Engl J Med (1988) 319(16):1033–9. doi: 10.1056/NEJM198810203191601
41. Sadetzki S, Flint-Richter P, Ben-Tal T, Nass D. Radiation-induced meningioma: a descriptive study of 253 cases. J Neurosurg (2002) 97(5):1078–82. doi: 10.3171/jns.2002.97.5.1078
42. Jabbour SK, Zhang Z, Arnold D, Wharam MD. Risk of second tumor in intracranial germinoma patients treated with radiation therapy: the johns Hopkins experience. J Neurooncol (2009) 91(2):227–32. doi: 10.1007/s11060-008-9703-5
43. Kralik SF, Watson GA, Shih CS, Ho CY, Finke W, Buchsbaum J. Radiation-induced Large vessel cerebral vasculopathy in pediatric patients with brain tumors treated with proton radiation therapy. Int J Radiat Oncol Biol Phys (2017) 99(4):817–24. doi: 10.1016/j.ijrobp.2017.07.009
44. Arvold ND, Niemierko A, Broussard GP, Adams J, Fullerton B, Loeffler JS, et al. Projected second tumor risk and dose to neurocognitive structures after proton versus photon radiotherapy for benign meningioma. Int J Radiat OncologyBiologyPhysics (2012) 83(4):e495–500. doi: 10.1016/j.ijrobp.2011.10.056
45. Schneider U, Zwahlen D, Ross D, Kaser-Hotz B. Estimation of radiation-induced cancer from three-dimensional dose distributions: Concept of organ equivalent dose. Int J Radiat OncologyBiologyPhysics (2005) 61(5):1510–5. doi: 10.1016/j.ijrobp.2004.12.040
46. Brodin NP, Munck Af Rosenschöld P, Aznar MC, Kiil-Berthelsen A, Vogelius IR, Nilsson P, et al. Radiobiological risk estimates of adverse events and secondary cancer for proton and photon radiation therapy of pediatric medulloblastoma. Acta Oncol (2011) 50(6):806–16. doi: 10.3109/0284186X.2011.582514
47. Zhang R, Howell RM, Giebeler A, Taddei PJ, Mahajan A, Newhauser WD. Comparison of risk of radiogenic second cancer following photon and proton craniospinal irradiation for a pediatric medulloblastoma patient. Phys Med Biol (2013) 58(4):807–23. doi: 10.1088/0031-9155/58/4/807
48. Miralbell R, Lomax A, Cella L, Schneider U. Potential reduction of the incidence of radiation-induced second cancers by using proton beams in the treatment of pediatric tumors. Int J Radiat Oncol Biol Phys (2002) 54(3):824–9. doi: 10.1016/s0360-3016(02)02982-6
49. Schneider U. Modeling the risk of secondary malignancies after radiotherapy. Genes (Basel) (2011) 2(4):1033–49. doi: 10.3390/genes2041033
50. Niranjan A, Kondziolka D, Lunsford LD. Neoplastic transformation after radiosurgery or radiotherapy: risk and realities. Otolaryngol Clin North Am (2009) 42(4):717–29. doi: 10.1016/j.otc.2009.04.005
51. Paulino AC, Ahmed IM, Mai WY, Teh BS. The influence of pretreatment characteristics and radiotherapy parameters on time interval to development of radiation-associated meningioma. Int J Radiat Oncol Biol Phys (2009) 75(5):1408–14. doi: 10.1016/j.ijrobp.2009.01.052
52. Pettorini BL, Park YS, Caldarelli M, Massimi L, Tamburrini G, Di Rocco C. Radiation-induced brain tumours after central nervous system irradiation in childhood: a review. Childs Nerv Syst (2008) 24(7):793–805. doi: 10.1007/s00381-008-0631-7
53. Brenner DJ, Curtis RE, Hall EJ, Ron E. Second malignancies in prostate carcinoma patients after radiotherapy compared with surgery. Cancer (2000) 88(2):398–406. doi: 10.1002/(sici)1097-0142(20000115)88:2<398::aid-cncr22>3.0.co;2-v
54. Boice JD, Engholm G, Kleinerman RA, Blettner M, Stovall M, Lisco H, et al. Radiation dose and second cancer risk in patients treated for cancer of the cervix. Radiat Res (1988) 116(1):3–55. doi: 10.2307/3577477
55. Xiang M, Chang DT, Pollom EL. Second cancer risk after primary cancer treatment with three-dimensional conformal, intensity-modulated, or proton beam radiation therapy. Cancer (2020) 126(15):3560–8. doi: 10.1002/cncr.32938
56. Zhang J, Walsh MF, Wu G, Edmonson MN, Gruber TA, Easton J, et al. Germline mutations in predisposition genes in pediatric cancer. N Engl J Med (2015) 373(24):2336–46. doi: 10.1056/NEJMoa1508054
57. Linet MS, Kim KP, Rajaraman P. Children’s exposure to diagnostic medical radiation and cancer risk: epidemiologic and dosimetric considerations. Pediatr Radiol (2009) 39 Suppl 1:S4–26. doi: 10.1007/s00247-008-1026-3
58. Patil VM, Kapoor R, Chakraborty S, Ghoshal S, Oinam AS, Sharma SC. Dosimetric risk estimates of radiation-induced malignancies after intensity modulated radiotherapy. J Cancer Res Ther (2010) 6(4):442–7. doi: 10.4103/0973-1482.77082
59. Paulino AC, Mazloom A, Terashima K, Su J, Adesina AM, Okcu MF, et al. Intensity-modulated radiotherapy (IMRT) in pediatric low-grade glioma. Cancer (2013) 119(14):2654–9. doi: 10.1002/cncr.28118
60. Bekelman JE, Schultheiss T, Berrington De Gonzalez A. Subsequent malignancies after photon versus proton radiation therapy. Int J Radiat Oncol Biol Phys (2013) 87(1):10–2. doi: 10.1016/j.ijrobp.2013.05.016
61. Michalski JM, Janss A, Vezina G, Gajjar A, Pollack I, Merchant TE, et al. Results of COG ACNS0331: A phase III trial of involved-field radiotherapy (IFRT) and low dose craniospinal irradiation (LD-CSI) with chemotherapy in average-risk medulloblastoma: A report from the children’s oncology group. Int J Radiat Oncol Biol Phys (2016) 96(5):937–8. doi: 10.1016/j.ijrobp.2016.09.046
62. Children’s Oncology Group. Phase III randomized trial of post-radiation chemotherapy in patients with newly diagnosed ependymoma ages 1 to 21 years (2021). clinicaltrials.gov. Available at: https://clinicaltrials.gov/ct2/show/NCT01096368 (Accessed November 4, 2021).
Keywords: secondary Malignant Neoplasms after proton therapy vs photon therapy secondary cancer, proton therapy, CNS radiation, pediatric cancer, photon
Citation: Upadhyay R, Yadav D, Venkatesulu BP, Singh R, Baliga S, Raval RR, Lazow M, Salloum R, Fouladi M, Mardis E, Zaorsky NG, Trifiletti DM, Paulino AC and Palmer JD (2022) Risk of secondary malignant neoplasms in children following proton therapy vs. photon therapy for primary CNS tumors: A systematic review and meta-analysis. Front. Oncol. 12:893855. doi: 10.3389/fonc.2022.893855
Received: 10 March 2022; Accepted: 21 July 2022;
Published: 12 August 2022.
Edited by:
Chi-kong Li, The Chinese University of Hong Kong, ChinaReviewed by:
Shidong Li, Temple University, United StatesAnthony Pak-Yin Liu, The University of Hong Kong, Hong Kong SAR, China
Copyright © 2022 Upadhyay, Yadav, Venkatesulu, Singh, Baliga, Raval, Lazow, Salloum, Fouladi, Mardis, Zaorsky, Trifiletti, Paulino and Palmer. This is an open-access article distributed under the terms of the Creative Commons Attribution License (CC BY). The use, distribution or reproduction in other forums is permitted, provided the original author(s) and the copyright owner(s) are credited and that the original publication in this journal is cited, in accordance with accepted academic practice. No use, distribution or reproduction is permitted which does not comply with these terms.
*Correspondence: Joshua D. Palmer, am9zaHVhLnBhbG1lckBvc3VtYy5lZHU=