- Department of Dermatology, Weill Cornell Medical College of Cornell University, New York, NY, United States
Melanin is synthesized in melanocytes and is transferred into keratinocytes to block the effects of ultraviolet (UV) radiation and is important for preventing skin cancers including melanoma. However, it is known that after melanomagenesis and melanoma invasion or metastases, melanin synthesis still occurs. Since melanoma cells are no longer involved in the sun tanning process, it is unclear why melanocytes would maintain melanin synthesis after melanomagenesis has occurred. Aside from blocking UV-induced DNA mutation, melanin may provide other metabolic functions that could benefit melanoma. In addition, studies have suggested that there may be a selective advantage to melanin synthesis in melanoma; however, mechanisms regulating melanin synthesis outside the epidermis or hair follicle is unknown. We will discuss how melanosomal pH controls melanin synthesis in melanocytes and how melanosomal pH control of melanin synthesis might function in melanoma. We will also discuss potential reasons why melanin synthesis might be beneficial for melanoma cellular metabolism and provide a rationale for why melanin synthesis is not limited to benign melanocytes.
Canonical regulation of melanogenesis
Ultraviolet (UV) radiation leads to numerous effects on distinct cells within the skin including keratinocytes, melanocytes, and fibroblasts. Many of the effects of UV lead to the release of paracrine factors which have both local and systemic effects (1). Melanin synthesis is an evolutionarily conserved metabolic process that has evolved in higher organisms to protect tissues from UV radiation (2). There are two types of melanin in the skin: eumelanin and pheomelanin (3). Both types of melanin are synthesized from the amino acid tyrosine via both enzymatic and non-enzymatic reactions (Figure 1) (4). Pheomelanin is favored when the melanin synthetic enzymes TYRP1/TRP1 and TYRP2/TRP2/DCT have low activity since pheomelanin is largely synthesized via non-enzymatic reactions and mainly relies on the concentration of dopaquinone and cysteine (Figure 1) (5–8). Upon the upregulation of TYRP1 and TYRP2, dopaquinone is diverted to eumelanin. High eumelanin is thought to be protective and high pheomelanin is associated with carcinogenesis (9). This is because eumelanin absorbs UV light and reactive oxygen species (ROS) whereas pheomelanin consumes cysteine and can generate ROS on its own (Figure 1) (10–12). Therefore, the regulation of the eumelanin to pheomelanin ratio is important for the control of UV-induced DNA damage and metabolically produced ROS.
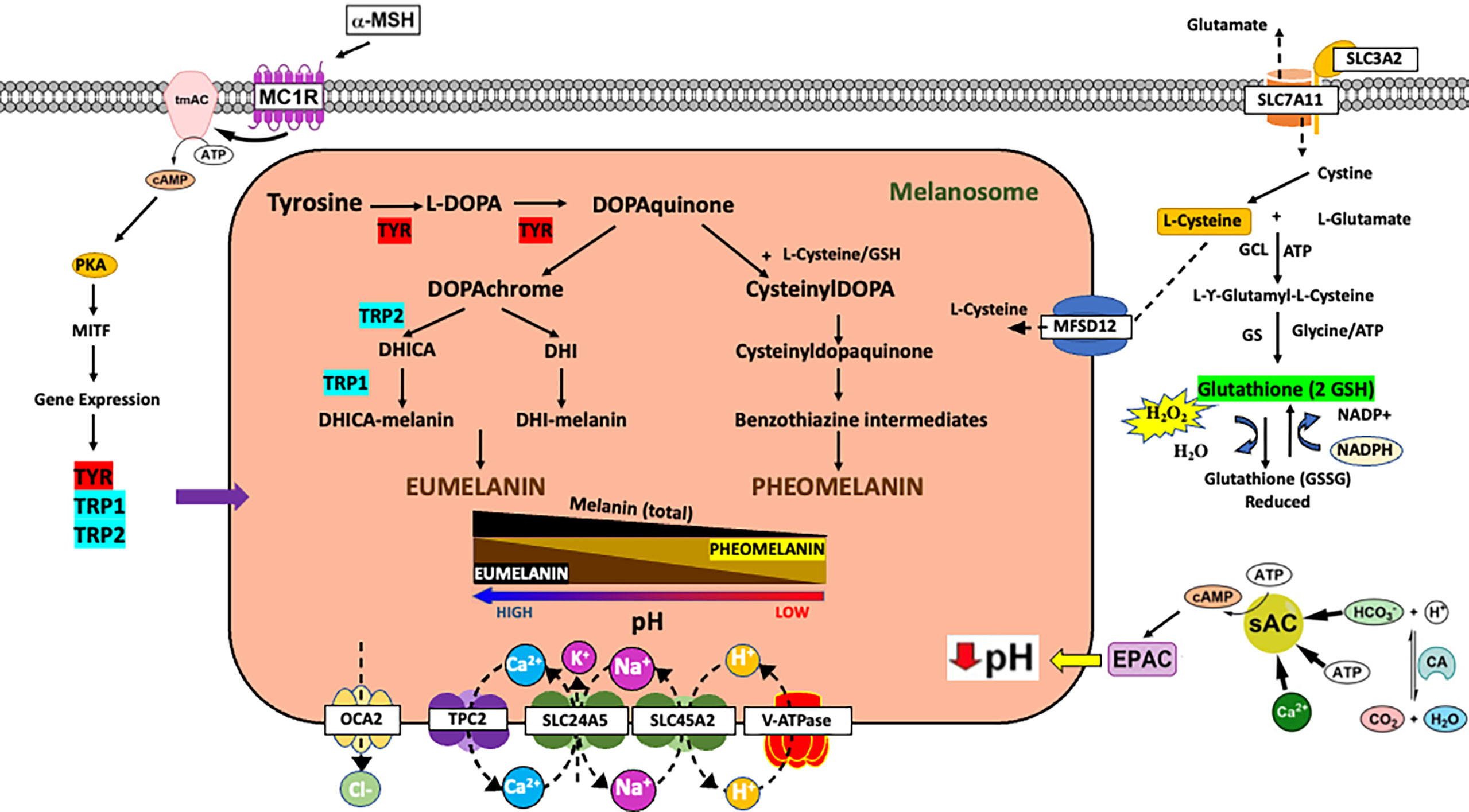
Figure 1 Impact of melanin metabolism on oxidative stress in melanoma. Overview of mechanisms of controlling melanin synthesis via gene expression or control of melanosome pH. Schematic of tyrosine metabolism in the cell highlighting the impact of melanin metabolism on oxidative stress. a-MSH, alpha melanocortin stimulating hormone; MC1R, melanocortin 1 receptor; tmAC, transmembrane adenylyl cyclase; PKA, protein kinase A; TYR, tyrosinase; TRP1, TYRP1/tyrosinase related protein 1; TRP2, TYRP2/DCT/tyrosinase related protein 2; EPAC, exchange protein activated by cAMP; sAC, soluble adenylyl cyclase; CA, carbonic anhydrase.
There are a variety of external stimuli and genetic factors that influence melanin synthesis (13, 14). First and foremost, melanin synthesis in the skin is associated with polymorphisms in numerous genes (15), which can impact both baseline melanin synthesis and the ability of the skin to respond to external signals such as UV radiation. In the canonical response of skin to UV radiation, keratinocytes in the skin respond to UV radiation induced DNA damage by secreting α-melanocyte-stimulating hormone (α-MSH) which activates the melanocortin 1 receptor (MC1R), a G protein-coupled receptor on the surface of melanocytes, leading to activation of a cAMP signaling cascade and an increase in eumelanin production (2) (Figure 1). The increase in eumelanin leads to photoprotection against UV-induced DNA damage, thereby lowering the risk of sun-induced carcinogenesis. The MC1R gene is known to be highly polymorphic in Caucasian populations, and those deficient in MC1R activity are characterized by red hair, fair skin, poor tanning, and an increased risk of melanoma and skin cancer. There are also other paracrine factors and hormones that can impact melanin synthesis by altering intracellular cAMP (14). However, there are other genes critical for skin pigmentation which encode for proteins that do not control pigmentation via signal transduction cascades but instead have a defined function at the melanosome, the organelle responsible for making melanin (16). For example, polymorphisms in genes such as OCA2, SLC45A2, and TPC2 affect baseline pigmentation and when the proteins they encode are non-functional, diseases of pigmentation such as albinism occur (17, 18). The proteins encoded by these genes are membrane channels and are present on the melanosome. These proteins mainly function to control melanosome pH (4). Melanosomal pH regulates melanin synthesis because tyrosinase, the rate limiting enzyme in melanin synthesis, is pH sensitive (Figure 1) (4, 19–21). Tyrosinase requires an acidic pH for the hydroxylation of tyrosine into L-DOPA (20). Once L-DOPA is formed it can act as a co-factor for additional tyrosine hydroxylation. Thus, tyrosinase can be active at low pH; however, L-DOPA oxidation is favored in more alkaline pH. Therefore, overall melanin synthesis is accelerated as melanosome pH approaches neutral (19, 22, 23). It should further be noted that under circumstances where complete tyrosine hydroxylation and/or L-DOPA oxidation is not possible these metabolites have the potential to be released and possibly induce inter- and intracellular biology (24–26). Polymorphisms and loss of function mutations in these genes are associated with skin cancer and melanoma risk most likely due to a reduction in eumelanin synthesis (27, 28). In addition to melanosome proteins important for the control of melanosome pH, there are other melanosome proteins critical for cysteine (e.g., MFSD12) and metal ion import/export that have the potential to impact both melanin synthesis and global cellular metabolism. It is also important to note that melanosomal pH may be reflective of intracellular pH which is impacted by changes in extracellular pH. Extracellular pH varies greatly in the epidermis with the cornified layer having a recorded pH of 5.0 at the surface of the skin and a range of pHs from 6.3 to 6.9 at the stratum granulosum depending on the method used for pH measurement (29, 30). Regardless of measured pHs at the stratum granulosum, the proton concentration surrounding melanocytes is much higher than intracellular levels. Thus, the pH microenvironment around the melanocyte may also impact melanosomal pH and melanin synthesis.
Non-canonical regulation of melanin synthesis via alteration of melanosomal pH
There are two cAMP pathways that can regulate melanogenesis: a canonical and a non-canonical pathway. In the canonical pathway, the activation of the melanocortin 1 receptor (MC1R) leads to the stimulation of the transmembrane adenylyl cyclase (tmAC) and production of cAMP which induces a gene expression program that increases the expression of MITF, TYR, TYRP1, and TYRP2; genes critical for melanogenesis (31, 32). In contrast, there is a non-canonical cAMP signaling pathway in melanocytes that is defined by the soluble adenylyl cyclase (sAC). sAC activation acidifies melanosomal pH and inhibits tyrosinase activity; whereas inhibition of sAC leads to the elevation of melanosome pH and the activation of tyrosinase (Figure 1) (21, 23). Melanosomal pH directly affects melanin metabolism because the rate-limiting enzyme tyrosinase is pH-sensitive (Figure 1). Melanosomes, depending on the stage of development and other factors, have variable pHs (19). When melanosomes mature their intra-organellar pH increases from 5 to 6.8 and pigment production increases (33). In addition, melanosomes from white/fair skin tend to be relatively more acidic as compared to melanosomes from black/dark skin, which tend to have a more neutral pH (19, 34). In addition to affecting the activity of tyrosinase, melanosomal pH, in cooperation with cysteine levels, can control the EM to PM ratio (Figure 1) (5, 21, 22, 35–37). Alkaline melanosomal pH favors EM over PM formation and thus promotes an anti-oxidant environment. Changes in the extracellular concentration of cystine are known to increase eumelanin levels presumably due to a decrease in intracellular cysteine levels (38). However, the mechanisms that control cystine/cysteine levels to affect melanin synthesis are poorly understood. Mouse models suggest that the subtle gray phenotype occurs because of a mutation in Slc7a11, which encodes for the plasma membrane cystine/glutamate exchanger xCT (Figure 1) (39). In the absence of SLC7A11 activity, cystine is not transported into the cell leading to a decrease in PM synthesis with little to no effect on EM. Recently, it was determined that the MFSD12 (major facilitator superfamily domain-containing protein 12) is important for the import of cysteine/cystine into the melanosome (Figure 1) (40), which may explain why polymorphisms in MFSD12 are associated with darker pigmentation in mice and humans (41, 42). Thus, differences in melanosomal pH can have significant effects on melanin metabolism.
Association between genetic defects affecting melanosomal pH and melanomagenesis
The association between MC1R polymorphisms and melanoma risk is well established and is explained by defects in UV-induced tanning and protective eumelanin pigmentation (2). However, there are several polymorphisms that affect melanosomal channels and transporters which alter melanosomal pH and increase the risk of melanoma (18). Oculocutaneous albinism type 2 (OCA2) is caused by mutations in the OCA2 gene. Individuals with OCA2 mutation are at a higher risk of developing UV-induced skin cancers including melanoma. The OCA2 channel is normally incorporated into melanosomes early during melanosome maturation, and functions to neutralize melanosomal pH for optimal tyrosinase function and eumelanin production (Figure 1) (43, 44). Therefore, genetic mutations of the OCA2 channel lead to acidic melanosomal pH, hypopigmentation due to decreased eumelanin synthesis, and increased risk for melanoma. The SLC45A2 (solute carrier family 45 member 2) gene encodes a H+/sugar co-transporter protein on the melanosomal membrane (Figure 1). SLC45A2 is required for melanosomes to progress from stage III to IV. Mutations in the SLC45A2 gene have been associated with oculocutaneous albinism type 4, a condition characterized by strong melanin deficiency. It has also been found that the SLC45A2 transporter expressed ectopically in HeLa cells localizes to lysosomes and plays a role in raising lysosomal pH. This suggests that SLC45A2 expression in melanocytes can potentially plays a role in modulating melanosomal pH to support melanin production. According to genome-wide association studies on European populations, DNA variants at the OCA2 and SLC45A2 genes have been associated with increased susceptibility to cutaneous malignant melanoma (45). Two pore channel 2 (TPC2/TPCN2) is an ion channel not exclusively expressed on melanosomes or in melanocytes and is a voltage-independent cation channel that responds to ligands instead of membrane potential (17, 46–48). Unlike OCA2 and SLC45A2, loss of TPC2 activity leads to an increase in melanin synthesis (17, 43, 49, 50). TPC2 is not associated with pigment diseases but polymorphisms are associated with hair color and UV sensitivity mostly in Dutch and Icelandic individuals (51–53). Whereas proteins that control melanosomal pH can impact the risk of developing melanoma, these same proteins are also differentially expressed during invasion and metastasis (54, 55). Thus, it is possible that changes in melanosomal pH may impact melanin synthesis in metastatic melanoma.
Potential roles of melanin during melanomagenesis
Whereas melanin in the skin has a clear role in skin cancer protection, it is unclear why melanoma cells would maintain the production of this molecule. Since melanin synthesis generates toxic intermediates and can be an energetic process, it stands to reason that melanoma maintains this metabolic process because melanin supports the growth of melanoma cells after transformation. Eumelanin (EM) possesses antioxidant properties and pheomelanin (PM) is pro-oxidant. Both pigment types are derived from the common precursor dopaquinone, which is formed from the oxidation of L-tyrosine by the enzyme tyrosinase (4) (Figure 1). EM scavenges free radicals produced from UV induced damage due to its paramagnetic, redox, and ion exchange antioxidant properties. These properties neutralize reactive oxygen species (ROS) (56–58). PM is a yellow to reddish-brown pigment produced when cellular L-cysteine binds with dopaquinone to produce cysteinyldopa isomers (Figure 1) (4). The oxidation of this thiol-dopa produces PM. During the production of PM ROS is generated which can lead to cellular damage (59).
Cellular ROS is normally controlled by the enzyme glutathione-S-transferase (GST), which catalyzes the binding of GSH with dopaquinone and glutathione reductase (GR), which reduces the oxidized GSH in cells to a usable form (Figure 1) (60). GSH is a multifunctional molecule and is the main antioxidant used by cells to neutralize ROS. GSH is a ubiquitous compound that consists of the amino acids cysteine, glycine, and glutamate. It has a biologically active sulfhydryl (SH) group, which allows it to neutralize ROSs such as H2O2 (61). GSH is a non-enzymatic antioxidant and an endogenous redox buffer that donates electrons to peroxidases (62). GSH is also involved in other biochemical pathways such as maintaining the SH groups of proteins and other molecules, serving as a coenzyme for certain enzymes, and during detoxification processes within cells (61). GSH can be regenerated by NADPH which reduces its oxidized form (62). Thus, any cellular stress that leads to movement of cysteine into GSH could decrease cellular concentrations of L-cysteine and induce a eumelanotic shift in melanogenesis (38, 63). Since EM protects against ROS and PM produces ROS (e.g., H2O2) the balance between these two melanin synthetic pathways would affect ROS levels during melanomagenesis (Figure 1) (64).
ROS can affect tumor biology via many distinct mechanisms. Examples include modifying DNA or upregulating nitric oxide synthase (NOS) synthesis (65). ROS produced as byproducts of enzymatic and non-enzymatic metabolic processes include superoxide radicals (O2.-), hydroxyl radicals (OH.), and hydrogen peroxide (H2O2). At high levels, these exemplary species lead to the oxidation of cellular lipids, proteins, and DNA inducing cellular damage and mutation important for melanoma initiation and progression (62, 66). In contrast, lower levels of ROS promote activation of vital signaling pathways such as cellular proliferation and survival (67).
Melanoma cells promoting EM production at the expense of PM production preserve GSH for cellular ROS reduction because PM production requires L-cysteine. However, the mechanisms by which melanoma might alter melanin synthesis are not well established. Of note, the production of PM in the presence of L-cysteine is increased around pH 5.8-6.3, while the generation of EM is suppressed at pH 5.8 (68). This suggests that there may be a role for melanosomal pH in the control of EM/PM ratio and GSH synthesis. By increasing melanosomal pH, melanocytes could increase antioxidant EM and preserve antioxidant GSH levels to combat the effects of ROS. Aside from regulating ROS, melanogenesis can impact melanoma by altering the expression of stress related genes such as HIF-1a and metabolic regulatory proteins such as GLUT-1 (69).
Potential roles of melanin during melanoma metastasis
The presence of melanin in cutaneous melanomas is associated with a higher risk of metastasis, aggressiveness of cancer, and death (70–73). In individuals with uveal melanomas, differential melanin synthesis is associated with a higher risk of metastasis and death (74). Independent of its effects on growth, melanomas with high levels of melanin appear to attenuate the efficacy of radiotherapy; patients with amelanotic melanomas had longer survival times when compared to pigmented melanomas (70). Thus, these reports suggest that melanin synthesis may play an important role in melanoma well beyond tumor initiation.
Metastasizing melanoma can appear as gray-black or blue-black suggesting a robust eumelanin synthetic pathway. In addition, increased ROS in metastatic melanoma cells can prevent metastasis. Oxidative stress can impair cancer progression by suppressing protein translation (75). Epithelial melanoma cells upon invasion and metastasis require antioxidant protection to successfully travel in the bloodstream to form secondary tumors; therefore, balancing ROS is critical for metastasizing tumors (76).
To manage ROS, metastatic cells have developed alternative mechanisms to overcome the toxic effects of ROS, such as producing NADPH to regenerate GSH reserves. Melanotic melanoma cells contain high amounts of reduced GSH and glutathione-S-transferase (GST) in the cytosol as suggested by the increased activity of glutathione reductase in these cells as compared to amelanotic melanoma cells (77). It is reported that detached cancer cells will increase their glucose uptake and upregulate the pentose phosphate pathway as well as other metabolic pathways to generate more NADPH (78–80) for the regeneration of reduced GSH. Alternatively, melanoma can also increase reduced GSH reserves by increasing serine and glycine synthesis (81).
Melanogenesis would be an excellent mechanism for balancing the cell’s overall redox levels. To restore the cell’s redox balance, the melanotic melanoma cells could produce more GSH (77) which could be achieved by diverting melanin synthesis away from pheomelanin. In addition, it has been suggested that melanin possesses some immunosuppressive properties and the ability to have other paracrine signaling effects via the production of certain melanin intermediates such as DOPA (82–84). Furthermore, since melanin metabolism affects tyrosine levels, there is a potential impact of altered melanin metabolism on the production of tyrosine-derived signal molecules. In addition, there is a potential impact of melanosomal metabolism on the cellular microenvironment which might enhance melanoma mestastasis (85). Thus, balancing melanosomal metabolism may be a critical mechanism during melanoma progression.
Conclusions
Melanin has an established role in the protection of the epidermis from UV radiation. However, melanin synthesis continues in melanoma following invasion and metastasis. Given the potentially toxic effects of melanin synthesis, it is unclear why melanoma would maintain this metabolic process. Melanoma is very sensitive to ROS during invasion and metastasis. Since melanin metabolism can affect ROS both positively and negatively, it is possible that melanoma cells harness melanin metabolism to balance ROS. In addition, it appears that melanin levels can affect the melanoma therapeutic response. Whereas traditional methods of regulating melanin metabolism (e.g., MC1R) are not significantly altered in melanoma, genes important for the regulation of melanosomal pH are altered in melanoma. We propose that altering melanosomal pH may be an effective mechanism for the regulation of melanin synthesis. Specifically, modulating melanosomal pH could alter the eumelanin to pheomelanin ratio which has a dramatic effect on cellular ROS. Currently there is a paucity of studies focused on understanding melanosome metabolism (23, 40). We predict that additional investigation of melanosomal pH and melanosome metabolism in melanoma may reveal new mechanisms that affect melanoma metastasis or therapeutic response.
Author contributions
JY, MY, and JZ all contributed to the writing and editing of the manuscript. All authors read and approved the final manuscript.
Funding
This study was funded by the National Institute of Arthritis and Musculoskeletal and Skin Diseases (NIAMS), grant number 1 R01 AR077664-01A1.
Conflict of interest
The authors declare that the research was conducted in the absence of any commercial or financial relationships that could be construed as a potential conflict of interest.
Publisher’s note
All claims expressed in this article are solely those of the authors and do not necessarily represent those of their affiliated organizations, or those of the publisher, the editors and the reviewers. Any product that may be evaluated in this article, or claim that may be made by its manufacturer, is not guaranteed or endorsed by the publisher.
References
1. Slominski AT, Zmijewski MA, Plonka PM, Szaflarski JP, Paus R. How UV light touches the brain and endocrine system through skin, and why. endocrinology. 159 (2018), 159:1992–2007. doi: 10.1210/en.2017-03230
2. Abdel-Malek ZA, Knittel J, Kadekaro AL, Swope VB, Starner R. The melanocortin 1 receptor and the UV response of human melanocytes–a shift in paradigm. Photochem Photobiol (2008) 84:501–8. doi: 10.1111/j.1751-1097.2008.00294.x
3. Thody AJ, Higgins EM, Wakamatsu K, Ito S, Burchill SA, Marks JM. Pheomelanin as well as eumelanin is present in human epidermis. J Invest Dermatol (1991) 97:340–4. doi: 10.1111/1523-1747.ep12480680
4. Wakamatsu K, Zippin JH, Ito S. Chemical and biochemical control of skin pigmentation with special emphasis on mixed melanogenesis. Pigment Cell Melanoma Res (2021) 34:730–47. doi: 10.1111/pcmr.12970
5. Ito S, Wakamatsu K. Chemistry of mixed melanogenesis–pivotal roles of dopaquinone. Photochem Photobiol (2008) 84:582–92. doi: 10.1111/j.1751-1097.2007.00238.x
6. Ozeki H, Ito S, Wakamatsu K, Ishiguro I. Chemical characterization of pheomelanogenesis starting from dihydroxyphenylalanine or tyrosine and cysteine. effects of tyrosinase and cysteine concentrations and reaction time. Biochim Biophys Acta (1997) 1336:539–48. doi: 10.1016/s0304-4165(97)00068-8
7. Potterf SB, Virador V, Wakamatsu K, Furumura M, Santis C, Ito S, et al. Cysteine transport in melanosomes from murine melanocytes. Pigment Cell Res (1999) 12:4–12. doi: 10.1111/j.1600-0749.1999.tb00502.x
8. Wakamatsu K, Nagao A, Watanabe M, Nakao K, Ito S. Pheomelanogenesis is promoted at a weakly acidic pH. Pigment Cell Melanoma Res (2017), 372–7. doi: 10.1111/pcmr.12587
9. Brenner M, Hearing VJ. The protective role of melanin against UV damage in human skin. Photochem Photobiol (2008) 84:539–49. doi: 10.1111/j.1751-1097.2007.00226.x
10. Bustamante J, Bredeston L, Malanga G, Mordoh J. Role of melanin as a scavenger of active oxygen species. Pigment Cell Res (1993) 6:348–53. doi: 10.1111/j.1600-0749.1993.tb00612.x
11. Hachinohe M, Matsumoto H. Involvement of reactive oxygen species generated from melanin synthesis pathway in phytotoxicty of l-DOPA. J Chem Ecol (2005) 31:237–46. doi: 10.1007/s10886-005-1338-9
12. Jenkins NC, Grossman D. Role of melanin in melanocyte dysregulation of reactive oxygen species. BioMed Res Int (2013) 2013:908797. doi: 10.1155/2013/908797
13. Costin GE, Hearing VJ. Human skin pigmentation: melanocytes modulate skin color in response to stress. FASEB J (2007) 21:976–94. doi: 10.1096/fj.06-6649rev
14. Slominski A, Tobin DJ, Shibahara S, Wortsman J. Melanin pigmentation in mammalian skin and its hormonal regulation. Physiol Rev (2004) 84:1155–228. doi: 10.1152/physrev.00044.2003
15. Sturm RA, Larsson M. Genetics of human iris colour and patterns. Pigment Cell Melanoma Res (2009) 22:544–62. doi: 10.1111/j.1755-148X.2009.00606.x
16. Wiriyasermkul P, Moriyama S, Nagamori S. Membrane transport proteins in melanosomes: Regulation of ions for pigmentation. Biochim Biophys Acta Biomembr (2020), 183318. doi: 10.1016/j.bbamem.2020.183318
17. Ambrosio AL, Boyle JA, Aradi AE, Christian KA, Di Pietro SM. TPC2 controls pigmentation by regulating melanosome pH and size. Proc Natl Acad Sci U.S.A. (2016) 113:5622–7. doi: 10.1073/pnas.1600108113
18. Duffy DL, Zhao ZZ, Sturm RA, Hayward NK, Martin NG, Montgomery GW. Multiple pigmentation gene polymorphisms account for a substantial proportion of risk of cutaneous malignant melanoma. J Invest Dermatol (2010) 130:520–8. doi: 10.1038/jid.2009.258
19. Ancans J, Tobin DJ, Hoogduijn MJ, Smit NP, Wakamatsu K, Thody AJ. Melanosomal pH controls rate of melanogenesis, eumelanin/phaeomelanin ratio and melanosome maturation in melanocytes and melanoma cells. Exp Cell Res (2001) 268:26–35. doi: 10.1006/excr.2001.5251
20. Tripathi RK, Chaya Devi C, Ramaiah A. pH-dependent interconversion of two forms of tyrosinase in human skin. Biochem J (1988) 252:481–7. doi: 10.1042/bj2520481
21. Zhou D, Ota K, Nardin C, Feldman M, Widman A, Wind O, et al. Mammalian pigmentation is regulated by a distinct cAMP-dependent mechanism that controls melanosome pH. Sci Signal (2018) 11:eaau7987. doi: 10.1126/scisignal.aau7987
22. Ancans J, Hoogduijn MJ, Thody AJ. Melanosomal pH, pink locus protein and their roles in melanogenesis. J Invest Dermatol (2001) 117:158–9. doi: 10.1046/j.0022-202x.2001.01397.x
23. Chen Q, Zhou D, Abdel-Malek Z, Zhang F, Goff PS, Sviderskaya EV, et al. Measurement of melanin metabolism in live cells by [U-(13)C]-L-Tyrosine fate tracing using liquid chromatography-mass spectrometry. J Invest Dermatol (2021) 141:1810–8:e1816. doi: 10.1016/j.jid.2021.01.007
24. Slominski A, Moellmann G, Kuklinska E. L-tyrosine, l-dopa, and tyrosinase as positive regulators of the subcellular apparatus of melanogenesis in bomirski ab amelanotic melanoma cells. Pigment Cell Res (1989) 2:109–16. doi: 10.1111/j.1600-0749.1989.tb00170.x
25. Slominski A, Paus R. Are l-tyrosine and l-dopa hormone-like bioregulators? J Theor Biol (1990) 143:123–38. doi: 10.1016/s0022-5193(05)80292-9
26. Slominski A, Paus R, Schadendorf D. Melanocytes as “sensory” and regulatory cells in the epidermis. J Theor Biol (1993) 164:103–20. doi: 10.1006/jtbi.1993.1142
27. Fernandez LP, Milne RL, Pita G, Avilés JA, Lázaro P, Benítez J, et al. SLC45A2: a novel malignant melanoma-associated gene. Hum Mutat (2008) 29:1161–7. doi: 10.1002/humu.20804
28. Hawkes JE, Cassidy PB, Manga P, Boissy RE, Goldgar D, Cannon-Albright L, et al. Report of a novel OCA2 gene mutation and an investigation of OCA2 variants on melanoma risk in a familial melanoma pedigree. J Dermatol Sci (2013) 69:30–7. doi: 10.1016/j.jdermsci.2012.09.016
29. Behne MJ, Meyer JW, Hanson KM, Barry NP, Murata S, Crumrine D, et al. NHE1 regulates the stratum corneum permeability barrier homeostasis. microenvironment acidification assessed with fluorescence lifetime imaging. J Biol Chem (2002) 277:47399–406. doi: 10.1074/jbc.M204759200
30. Ohman H, Vahlquist A. In vivo studies concerning a pH gradient in human stratum corneum and upper epidermis. Acta Derm Venereol (1994) 74:375–9. doi: 10.2340/0001555574375379
31. Abdel-Malek Z, Swope VB, Suzuki I, Akcali C, Harriger MD, Boyce ST, et al. Mitogenic and melanogenic stimulation of normal human melanocytes by melanotropic peptides. Proc Natl Acad Sci U.S.A. (1995) 92:1789–93. doi: 10.1073/pnas.92.5.1789
32. Swope VB, Jameson JA, McFarland KL, Supp DM, Miller WE, McGraw DW, et al. Defining MC1R regulation in human melanocytes by its agonist alpha-melanocortin and antagonists agouti signaling protein and beta-defensin 3. J Invest Dermatol (2012) 132:2255–62. doi: 10.1038/jid.2012.135
33. Schallreuter KU, Kothari S, Chavan B, Spencer JD. Regulation of melanogenesis–controversies and new concepts. Exp Dermatol (2008) 17:395–404. doi: 10.1111/j.1600-0625.2007.00675.x
34. Smith DR, Spaulding DT, Glenn HM, Fuller BB. The relationship between Na(+)/H(+) exchanger expression and tyrosinase activity in human melanocytes. Exp Cell Res (2004) 298:521–34. doi: 10.1016/j.yexcr.2004.04.033
35. Ito S, Wakamatsu K. Quantitative analysis of eumelanin and pheomelanin in humans, mice, and other animals: a comparative review. Pigment Cell Res (2003) 16:523–31. doi: 10.1034/j.1600-0749.2003.00072.x
36. Lamoreux ML, Wakamatsu K, Ito S. Interaction of major coat color gene functions in mice as studied by chemical analysis of eumelanin and pheomelanin. Pigment Cell Res (2001) 14:23–31. doi: 10.1034/j.1600-0749.2001.140105.x
37. Land EJ, Ito S, Wakamatsu K, Riley PA. Rate constants for the first two chemical steps of eumelanogenesis. Pigment Cell Res (2003) 16:487–93. doi: 10.1034/j.1600-0749.2003.00082.x
38. del Marmol V, Ito S, Bouchard B, Libert A, Wakamatsu K, Ghanem G, et al. Cysteine deprivation promotes eumelanogenesis in human melanoma cells. J Invest Dermatol (1996) 107:698–702. doi: 10.1111/1523-1747.ep12365591
39. Chintala S, Li W, Lamoreux ML, Ito S, Wakamatsu K, Sviderskaya EV, et al. Slc7a11 gene controls production of pheomelanin pigment and proliferation of cultured cells. Proc Natl Acad Sci U.S.A. (2005) 102:10964–9. doi: 10.1073/pnas.0502856102
40. Adelmann CH, Traunbauer AK, Chen B, Condon KJ, Chan SH, Kunchok T, et al. MFSD12 mediates the import of cysteine into melanosomes and lysosomes. Nature (2020) 588:699–704. doi: 10.1038/s41586-020-2937-x
41. Adhikari K, Mendoza-Revilla J, Sohail A, Fuentes-Guajardo M, Lampert J, Chacon-Duque JC, et al. A GWAS in Latin americans highlights the convergent evolution of lighter skin pigmentation in Eurasia. Nat Commun (2019) 10:358. doi: 10.1038/s41467-018-08147-0
42. Crawford NG, Kelly DE, Hansen MEB, Beltrame MH, Fan S, Bowman SL, et al. Loci associated with skin pigmentation identified in African populations. Science (2017) 358(6365):eaan8433. doi: 10.1126/science.aan8433
43. Bellono NW, Escobar IE, Lefkovith AJ, Marks MS, Oancea E. An intracellular anion channel critical for pigmentation. Elife (2014) 3:e04543. doi: 10.7554/eLife.04543
44. Le L, Escobar IE, Ho T, Lefkovith AJ, Latteri E, Haltaufderhyde KD, et al. SLC45A2 protein stability and regulation of melanosome pH determine melanocyte pigmentation. Mol Biol Cell (2020) 31:2687–702. doi: 10.1091/mbc.E20-03-0200
45. Visconti A, Duffy DL, Liu F, Zhu G, Wu W, Chen Y, et al. Genome-wide association study in 176,678 europeans reveals genetic loci for tanning response to sun exposure. Nat Commun (2018) 9:1684. doi: 10.1038/s41467-018-04086-y
46. Calcraft PJ, Ruas M, Pan Z, Cheng X, Arredouani A, Hao X, et al. NAADP mobilizes calcium from acidic organelles through two-pore channels. Nature (2009) 459:596–600. doi: 10.1038/nature08030
47. She J, Zeng W, Guo J, Chen Q, Bai XC, Jiang Y. Structural mechanisms of phospholipid activation of the human TPC2 channel. Elife (2019) 8:e45222. doi: 10.7554/eLife.45222
48. Zong X, Schieder M, Cuny H, Fenske S, Gruner C, Rotzer K, et al. The two-pore channel TPCN2 mediates NAADP-dependent Ca(2+)-release from lysosomal stores. Pflugers Arch (2009) 458:891–9. doi: 10.1007/s00424-009-0690-y
49. Bellono NW, Escobar IE, Oancea E. A melanosomal two-pore sodium channel regulates pigmentation. Sci Rep (2016) 6:26570. doi: 10.1038/srep26570
50. Bellono NW, Oancea EV. Ion transport in pigmentation. Arch Biochem Biophys (2014) 563:35–41. doi: 10.1016/j.abb.2014.06.020
51. Bock J, Krogsaeter E, Passon M, Chao YK, Sharma S, Grallert H, et al. Human genome diversity data reveal that L564P is the predominant TPC2 variant and a prerequisite for the blond hair associated M484L gain-of-function effect. PloS Genet (2021) 17:e1009236. doi: 10.1371/journal.pgen.1009236
52. Chao YK, Schludi V, Chen CC, Butz E, Nguyen ONP, Muller M, et al. TPC2 polymorphisms associated with a hair pigmentation phenotype in humans result in gain of channel function by independent mechanisms. Proc Natl Acad Sci U.S.A. (2017) 114:E8595–602. doi: 10.1073/pnas.1705739114
53. Sulem P, Gudbjartsson DF, Stacey SN, Helgason A, Rafnar T, Jakobsdottir M, et al. Two newly identified genetic determinants of pigmentation in europeans. Nat Genet (2008) 40:835–7. doi: 10.1038/ng.160
54. Falkenius J, Lundeberg J, Johansson H, Tuominen R, Frostvik-Stolt M, Hansson J, et al. High expression of glycolytic and pigment proteins is associated with worse clinical outcome in stage III melanoma. Melanoma Res (2013) 23:452–60. doi: 10.1097/CMR.0000000000000027
55. Netanely D, Leibou S, Parikh R, Stern N, Vaknine H, Brenner R, et al. Classification of node-positive melanomas into prognostic subgroups using keratin, immune, and melanogenesis expression patterns. Oncogene (2021) 40:1792–805. doi: 10.1038/s41388-021-01665-0
56. Al Khatib M, Costa J, Baratto MC, Basosi R, Pogni R. Paramagnetism and relaxation dynamics in melanin biomaterials. J Phys Chem B (2020) 124:2110–5. doi: 10.1021/acs.jpcb.9b11785
57. Debing I, Ijzerman AP, Vauquelin G. Melanosome binding and oxidation-reduction properties of synthetic l-dopa-melanin as in vitro tests for drug toxicity. Mol Pharmacol (1988) 33:470–6.
58. Rozanowska M, Sarna T, Land EJ, Truscott TG. Free radical scavenging properties of melanin interaction of eu- and pheo-melanin models with reducing and oxidising radicals. Free Radic Biol Med (1999) 26:518–25. doi: 10.1016/s0891-5849(98)00234-2
59. Mitra D, Luo X, Morgan A, Wang J, Hoang MP, Lo J, et al. An ultraviolet-radiation-independent pathway to melanoma carcinogenesis in the red hair/fair skin background. Nature (2012) 491:449–53. doi: 10.1038/nature11624
60. Villarama CD, Maibach HI. Glutathione as a depigmenting agent: an overview. Int J Cosmet Sci (2005) 27:147–53. doi: 10.1111/j.1467-2494.2005.00235.x
61. Meister A, Tate SS. Glutathione and related gamma-glutamyl compounds: biosynthesis and utilization. Annu Rev Biochem (1976) 45:559–604. doi: 10.1146/annurev.bi.45.070176.003015
62. Gill JG, Piskounova E, Morrison SJ. Cancer, oxidative stress, and metastasis. Cold Spring Harbor Symp Quantitative Biol (2016) 81:163–75. doi: 10.1101/sqb.2016.81.030791
63. Smit NP, van der Meulen H, Koerten HK, Kolb RM, Mommaas AM, Lentjes EG, et al. Melanogenesis in cultured melanocytes can be substantially influenced by l-tyrosine and l-cysteine. J Invest Dermatol (1997) 109:796–800. doi: 10.1111/1523-1747.ep12340980
64. Tanaka H, Yamashita Y, Umezawa K, Hirobe T, Ito S, Wakamatsu K. The pro-oxidant activity of pheomelanin is significantly enhanced by UVA irradiation: Benzothiazole moieties are more reactive than benzothiazine moieties. Int J Mol Sci (2018) 19(10):2889. doi: 10.3390/ijms19102889
65. de Jager TL, Cockrell AE, Du Plessis SS. Ultraviolet light induced generation of reactive oxygen species. Adv Exp Med Biol (2017) 996:15–23. doi: 10.1007/978-3-319-56017-5_2
66. Floyd RA. Role of oxygen free radicals in carcinogenesis and brain ischemia. FASEB J (1990) 4:2587–97. doi: 10.1096/fasebj.4.9.2189775
67. Rhee SG. Cell signaling. H2O2, a necessary evil for cell signaling. Science (2006) 312:1882–3. doi: 10.1126/science.1130481
68. Ito S, Suzuki N, Takebayashi S, Commo S, Wakamatsu K. Neutral pH and copper ions promote eumelanogenesis after the dopachrome stage. Pigment Cell Melanoma Res (2013) 26:817–25. doi: 10.1111/pcmr.12137
69. Slominski A, Kim TK, Brozyna AA, Janjetovic Z, Brooks DL, Schwab LP, et al. The role of melanogenesis in regulation of melanoma behavior: melanogenesis leads to stimulation of HIF-1alpha expression and HIF-dependent attendant pathways. Arch Biochem Biophys (2014) 563:79–93. doi: 10.1016/j.abb.2014.06.030
70. Brozyna AA, Jozwicki W, Carlson JA, Slominski AT. Melanogenesis affects overall and disease-free survival in patients with stage III and IV melanoma. Hum Pathol (2013) 44:2071–4. doi: 10.1016/j.humpath.2013.02.022
71. Brozyna AA, Jozwicki W, Roszkowski K, Filipiak J, Slominski AT. Melanin content in melanoma metastases affects the outcome of radiotherapy. Oncotarget (2016) 7:17844–53. doi: 10.18632/oncotarget.7528
72. Slominski A, Zbytek B, Slominski R. Inhibitors of melanogenesis increase toxicity of cyclophosphamide and lymphocytes against melanoma cells. international journal of cancer. J Int du Cancer (2009) 124:1470–7. doi: 10.1002/ijc.24005
73. Slominski RM, Zmijewski MA, Slominski AT. The role of melanin pigment in melanoma. Exp Dermatol (2015) 24:258–9. doi: 10.1111/exd.12618
74. Jha J, Singh MK, Singh L, Pushker N, Lomi N, Meel R, et al. Association of TYRP1 with hypoxia and its correlation with patient outcome in uveal melanoma. Clin Transl Oncol (2021) 23:1874–84. doi: 10.1007/s12094-021-02597-7
75. Chio IIC, Jafarnejad SM, Ponz-Sarvise M, Park Y, Rivera K, Palm W, et al. NRF2 promotes tumor maintenance by modulating mRNA translation in pancreatic cancer. Cell (2016) 166:963–76. doi: 10.1016/j.cell.2016.06.056
76. Piskounova E, Agathocleous M, Murphy MM, Hu Z, Huddlestun SE, Zhao Z, et al. Oxidative stress inhibits distant metastasis by human melanoma cells. Nature (2015) 527:186–91. doi: 10.1038/nature15726
77. Benathan M, Alvero-Jackson H, Mooy AM, Scaletta C, Frenk E. Relationship between melanogenesis, glutathione levels and melphalan toxicity in human melanoma cells. Melanoma Res (1992) 2:305–14. doi: 10.1097/00008390-199212000-00003
78. Anastasiou D, Poulogiannis G, Asara JM, Boxer MB, Jiang JK, Shen M, et al. Inhibition of pyruvate kinase M2 by reactive oxygen species contributes to cellular antioxidant responses. Science (2011) 334:1278–83. doi: 10.1126/science.1211485
79. Patra KC, Hay N. The pentose phosphate pathway and cancer. Trends Biochem Sci (2014) 39:347–54. doi: 10.1016/j.tibs.2014.06.005
80. Schafer ZT, Grassian AR, Song L, Jiang Z, Gerhart-Hines Z, Irie HY, et al. Antioxidant and oncogene rescue of metabolic defects caused by loss of matrix attachment. Nature (2009) 461:109–13. doi: 10.1038/nature08268
81. DeNicola GM, Chen PH, Mullarky E, Sudderth JA, Hu Z, Wu D, et al. NRF2 regulates serine biosynthesis in non-small cell lung cancer. Nat Genet (2015) 47:1475–81. doi: 10.1038/ng.3421
82. Slominski A, Paus R, Mihm MC. Inhibition of melanogenesis as an adjuvant strategy in the treatment of melanotic melanomas: selective review and hypothesis. Anticancer Res (1998) 18:3709–15.
83. Slominski A, Zmijewski MA, Pawelek J. L-tyrosine and l-dihydroxyphenylalanine as hormone-like regulators of melanocyte functions. Pigment Cell Melanoma Res (2012) 25:14–27. doi: 10.1111/j.1755-148X.2011.00898.x
84. Slominski RM, Sarna T, Plonka PM, Raman C, Brozyna AA, Slominski AT. Melanoma, melanin, and melanogenesis: The yin and yang relationship. Front Oncol (2022) 12:842496. doi: 10.3389/fonc.2022.842496
Keywords: melanoma, melanosome, pH, reactive oxygen (ROS), melanin, cAMP
Citation: You J, Yusupova M and Zippin JH (2022) The potential impact of melanosomal pH and metabolism on melanoma. Front. Oncol. 12:887770. doi: 10.3389/fonc.2022.887770
Received: 02 March 2022; Accepted: 08 August 2022;
Published: 22 November 2022.
Edited by:
Laura Poliseno, Department of Biomedical Sciences, (CNR), ItalyReviewed by:
Andrzej T. Slominski, University of Alabama at Birmingham, United StatesMingxing Lei, Chongqing University, China
Copyright © 2022 You, Yusupova and Zippin. This is an open-access article distributed under the terms of the Creative Commons Attribution License (CC BY). The use, distribution or reproduction in other forums is permitted, provided the original author(s) and the copyright owner(s) are credited and that the original publication in this journal is cited, in accordance with accepted academic practice. No use, distribution or reproduction is permitted which does not comply with these terms.
*Correspondence: Jonathan H. Zippin, amh6aXBwaW5AbWVkLmNvcm5lbGwuZWR1
†These authors have contributed equally to this work