- 1Institute of Hematology, Union Hospital, Tongji Medical College, Huazhong University of Science and Technology, Wuhan, China
- 2Department of Hematology, Tongji Hospital, Tongji Medical College, Huazhong University of Science and Technology, Wuhan, China
Exosomes are membrane limited structures which derive from cell membranes and cytoplasm. When released into extracellular space, they circulate through the extracellular fluid, including the peripheral blood and tissue fluid. Exosomes surface molecules mediate their targeting to specific recipient cells and deliver their contents to recipient cells by receptor-ligand interaction and/or phagocytosis and/or endocytosis or direct fusion with cell membrane. Exosomes contain many functional molecules, including nucleic acids (DNAs, mRNAs, non-coding RNAs), proteins (transcription factors, enzymes), and lipids which have biological activity. By passing these cargos, exosomes can transfer information between cells. In this way, exosomes are extensively involved in physiological and pathological processes, such as angiogenesis, matrix reprogramming, coagulation, tumor progression. In recent years, researcher have found that exosomes from malignant tumors can mediate information exchange between tumor cells or between tumor cells and non-tumor cells, thereby promoting tumor survival, progression, and resistance to therapy. In this review, we discuss the pro-tumor and anti-therapeutic effects of exosomes in hematological malignancies, hoping to contribute to the early conquest of hematological malignancy.
Introduction
Exosomes are particles (30-150 nm in diameter) secreted by cells that are wrapped in lipid bilayer and contain various cargo on and within the membrane molecule surface. And because they have no functional nucleus, they can’t replicate themselves (1). Since Johnstone et al. discovered them in 1983 during transferrin receptor recycling in maturation of sheep reticulocytes (2) and subsequently named them exosomes (3), exosomes have been extensively studied about their biogenesis, their role in tumor progression and therapy resistance. Exosomes (sometimes only identified as small extracellular vesicles) can also transmit information through the bioactive nucleic acids (DNA, mRNA, miRNAs, CircRNAs), proteins (transcription factors, enzymes), and lipids that they contain (4–6). In addition, unlike conventional information molecules, exosomes have specific integrin profiles on their membranes, enabling them to target specific tissues and cells (7). After reaching their target tissue or target cell, by receptor-ligand interaction and/or phagocytosis and/or endocytosis and/or direct fusion with cell membrane, exosome cargos can directly regulate the function of recipient cells or alter their gene expression. So far, it has been found that the amount and contents of exosomes or other extracellular vesicles in tumor patients are quite different from those in normal patients (8, 9). In addition, the exosomes of tumor patients are different before and after treatment. Researchers found that, in both adult and pediatric acute myelogenous leukemia (AML), the amount of double-stranded DNA in the extracellular vesicles (EVs), which conclude exosomes, was consistent with the disease state. The amount of DNA in EVs in plasma decreases after treatment and increases after relapse (10–12). However, there are also some data suggesting fluctuations in plasma EVs levels in AML patients, with an initial sharp decline and a gradual increase during treatment. Whether this is due to increased resistance of tumor cells to treatment is unclear (11). In conclusion, these results suggest that exosomes are involved in the progression of tumor diseases. And many more specific studies have shown that exosomes promote tumor cell survival and disease progression in the following ways. 1. Promoting angiogenesis to supply nutrition, inhibiting apoptosis, and delivering pro-survival cytokines. 2. Promoting matrix reprogramming into a pro-tumor niche. 3. Inhibiting anti-tumor immune cells such as NK cells, CTL cells, and promoting the function of immunomodulatory cells. 4. Promoting resistance therapy by efflux drugs, and directly sequester drugs. The role of exosomes in hematological malignancies will be described in detail in the following sections. In addition, there have been reviews on topics similar to this review. But there is still a certain novelty in our paper (13–15). 1. Most of the reviews on similar topics describe that EVs secretion by tumor cells affects stromal cells and immune cells. But our manuscript also shows that tumor cells support each other by exchanging exosomes. 2. The evidence for our opinion mainly comes from the research of hematological tumors, and the evidence from solid tumors is rarely used, which makes this paper more reliable for researchers in the field of hematologic tumor. The diseases involved in this paper are briefly introduced in Table 1. All the molecules covered in this paper are shown in Table 2. In addition, for the convenience of readers, we added the mechanism diagram of exosome's influence on tumors. These two figures are presented in the paper in the form of Figure 1 and Figure 2.
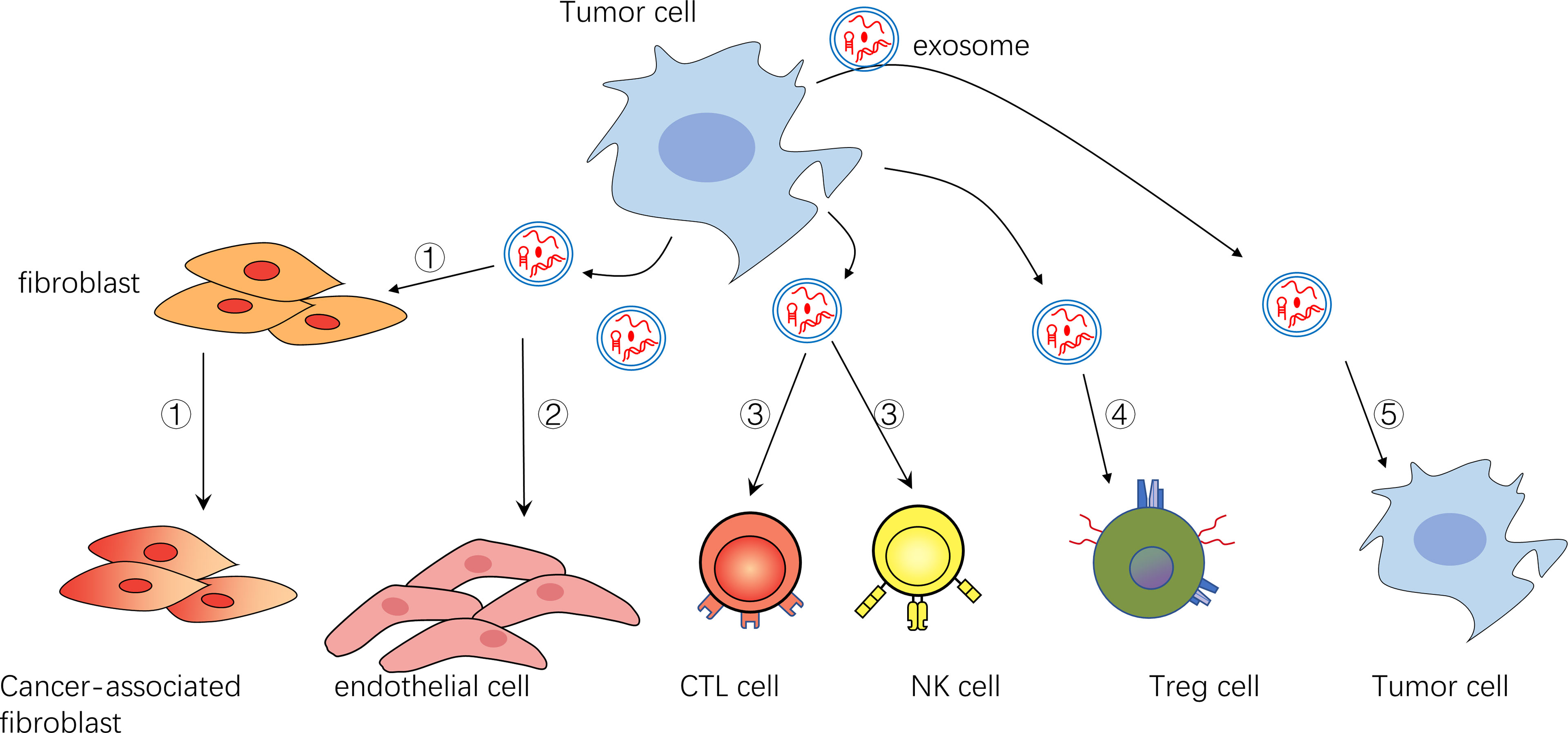
Figure 1 ①Exosomes can transform fibroblasts into tumor-associated fibroblasts ②Exosomes can promote endothelial cell growth and vascular formation ③Exosomes can inhibit NK cells and CTL cells ④Exosomes can activate immunomodulatory cells such as Treg cells ⑤Exosomes can act on themselves or their surrounding tumor cells to promote their survival and inhibit their apoptosis.
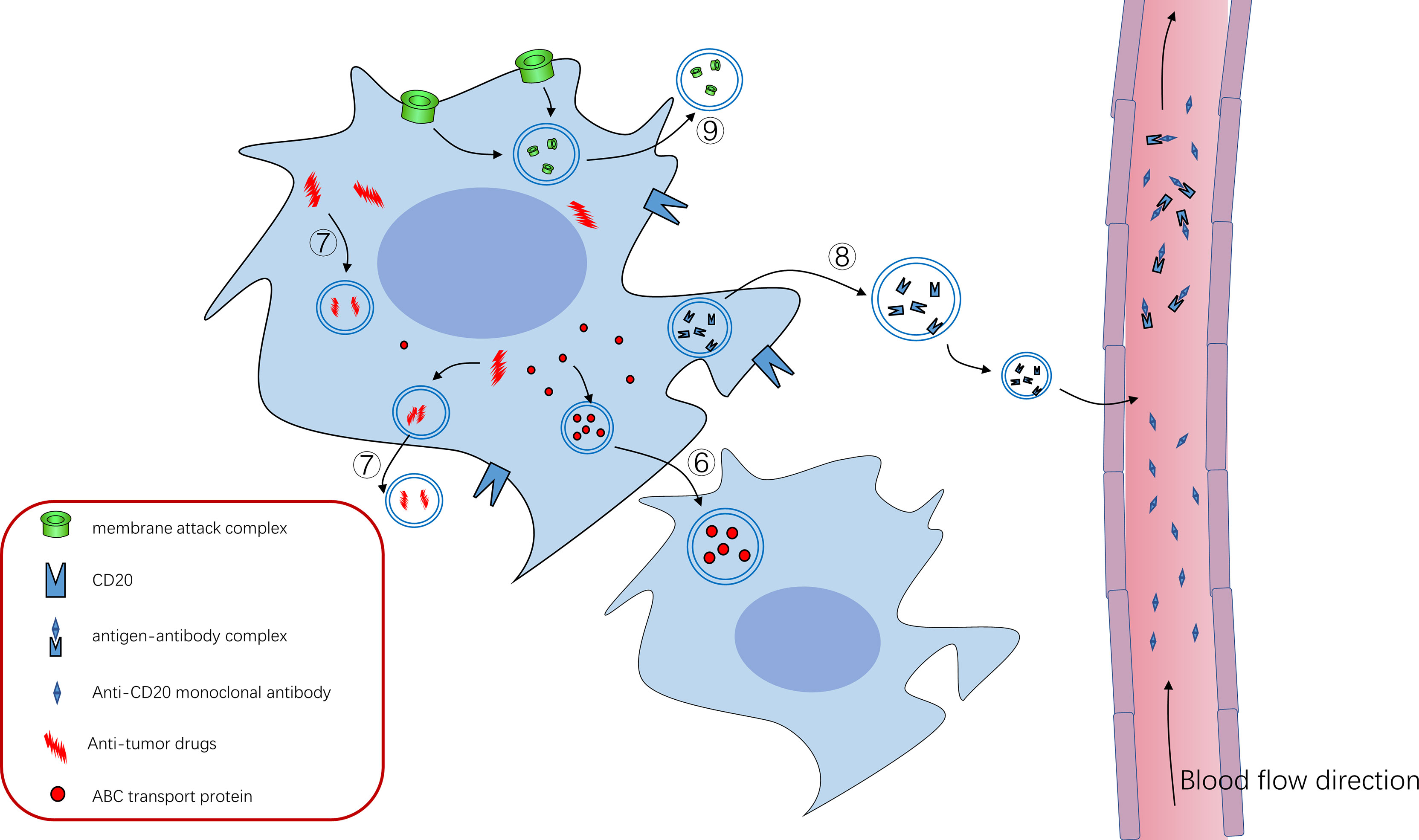
Figure 2 ⑥ABC transporter can mediate the formation of exosomes and drug resistance of tumor cells, and ABC transporter can be “shared” among tumor cells through exosomes to promote tumor progression ⑦Tumor cells can use exosomes to isolate or excrete intracellular antitumor drugs to achieve therapeutic resistance ⑧CD20 exists on the cell membrane of B cells, and anti-CD20 Rituximab can bind to CD20 to kill tumor cells. Tumor cells use exosomes to package some CD20 as decoy to release cells, which combine with anti-CD20 Rituximab to reduce their bioavailability and protect tumor cells from killing effects ⑨Tumor cells use exosomes to expel the membrane attack complex (MAC) inserted into the cell membrane to the outside of the cell, reducing its killing effect.
Exosomes Have the Function of Promoting the Survival and Progression of Tumor Cells
Promoting Angiogenesis
Tumor angiogenesis is closely related to disease progression. Tumor cells secrete angiogenic factors such as vascular endothelial growth factor (VEGF), fibroblast growth factor (FGF), transforming growth factor-β(TGF-β), hypoxia inducible factor-1 (HIF-1) and Interleukin-8 (IL-8), which promote the proliferation and growth of endothelial cells, and the new born blood vessels provide nutrition and oxygen for tumor tissue (36, 48). This exists in the whole process of tumor disease progression. In the field of hematological tumors, researchers have found that exosomes (or small extracellular vesicles) secreted by a variety of hematological malignancies can promote angiogenesis in the bone marrow (26). In malignant myeloma, the proliferation of plasma cells leads to hypoxia. In this case, tumor cells secrete exosomes that contain miR-135b. And then miR-135b exert its function to suppress its molecule target factor-inhibiting hypoxia-inducible factor 1 (FIH-1) after it is transported to the target cell vascular endothelial cell. In this way, exosomes secreted by multiple myeloma cells can enhance HIF-1 bioactivity, thereby promoting angiogenesis (34). In chronic myelogenous leukemia (CML), exosomes derived from K562 cells line are internalized by human umbilical endothelial cells (HUVECs), activating the Src signaling pathway. These endothelial cells have an increased tendency to form tubular structures, ultimately promoting angiogenesis (49). In another study, exosomes derived from K562 cells containing miR-92a have the same effect as to promote the formation of vascular tubular structures (50). Besides, exosomes derived from chronic lymphocytic leukemia (CLL) promote endothelial cell migration and angiogenesis in vitro and in vivo (51, 52).
Activating Pro-Survival Signal Pathway
Through paracrine and/or autocrine, exosomes derived from tumor cells or stromal cells deliver growth factors, extracellular enzymes, or other factors necessary for tumor growth to promote tumor progression, such as transforming growth factor-β1 (TGF-β1), Caveolin-1, and Wnt (27). These factors can promote the survival of tumor cells and increase their invasiveness and migration ability (37, 53). For example, CML cell-derived exosomes contain TGF-β1, which interacts with TGF-β1 receptors on the surface of tumor cells to promote cells survival and proliferation (38). Treatment with TGF-β1 inhibitors significantly reduced exosome-stimulated cell proliferation and colony formation (54). In vivo multiple myeloma mouse model, bone marrow stromal cell (BMSC)-derived exosomes contain oncoproteins, cytokines, adhesion molecules, such as IL-6, CCL2, and fibronectin, which can induce the survival, proliferation, and migration of multiple myeloma cells. Whereas normal BMSC-derived exosomes significantly reduced myeloma cell proliferation (16). In addition, researchers have found that exosomes obtained from myeloma patients’ BMSC, when taken by tumor cells, can affect several signaling pathways related to cell proliferation, differentiation, and apoptosis, including c-Jun N-terminal kinase (JNK), p38, p53, and Akt pathway (28). In diffuse large B-cell lymphoma, Koch et al. found that Wnt signaling activated by exosomes can induce non-side population cells to exhibit a side population (SP) phenotype. This side population of cells has characteristics like those of tumor stem cells, which involve in the occurrence, progression, metastasis, and recurrence of tumor diseases (55).
Suppressing Apoptosis of Tumor Cells
When the immune surveillance function of the body is normal, the malignant cells will initiate programmed cell death, avoiding the progression into malignant tumors. The apoptotic ability of cells depends to a large extent on the relative expression of anti-apoptotic protein (namely Bcl-2, Bcl-xl etc.) and pro-apoptotic protein (namely Bax, Caspases etc.). When the anti-apoptotic protein system gains the advantage, tumor cells exhibit an anti-apoptotic phenotype, these cells eventually develop into malignant tumors. In hematological malignancies, exosomes secreted by tumor cells or stromal cells can mediate the anti-apoptotic process. Researcher have found that, in the murine multiple myeloma model, tumor cells treated with exosomes derived from BMSCs show a unique protein expression profile. Antiapoptotic protein Bcl-2 increases whereas proapoptotic protein Bax don’t change. Besides, Full-length caspase-9 increases, cleaved caspase-9 and caspase-3 reduce, which means reduced pro-apoptotic activity of the caspase (28). In another research, exosomes (or small extracellular vesicles) derived from apoptotic-resistant AML cells containing proteins with apoptotic regulatory functions. When apoptotic-sensitive AML cells are co-cultured with exosomes of apoptotic-resistant AML cells, the expression of anti-apoptotic protein Bcl-2 will up-regulate. Accordingly, such AML cells exhibit anti-apoptotic, growth-promoting properties (56).
Researchers found that exosomes derived from imatinib-resistant CML cells contain high level of miR-365, and they can be internalized into drug-sensitive CML cells and imbibe resistance characteristics. In addition, imatinib-sensitive CML cells transfected with pre-miR-365 showed lower chemotherapy sensitivity and apoptosis rate, which also confirmed the anti-apoptosis function of miR-365. Further studies show that miR-365 induce drug resistance by inhibiting the expression of pro-apoptotic protein (Caspase3) in CML cells. In conclusion, these studies suggests that exosomes (or other extracellular vesicles) can mediate drug-resistant horizontal transfer between CML cells (57, 58).
Promoting the Transformation of Stromal Cells Into Pro-Tumor Phenotype, Forming a Pro-Tumor Niche
Fibroblasts, as the main producers of collagenous fiber, participate in the formation and remodeling of extracellular matrix. Cancer-associated fibroblasts (CAF) are derived from fibroblasts and mesenchymal stem cells and have been shown to generate extracellular matrix components that contribute to the growth and progression of the tumor cells (59). Tumor-derived exosomes (or other extracellular vesicles) induce many types of cells to differentiate into CAF, thereby participating in extracellular matrix remodeling and reprogramming of the tumor microenvironment (17, 29, 60). Researchers have found that, in CLL, exosomes derived from tumor cells promote disease progression by transforming surrounding BMSCs into CAF, prompting proliferation of stromal cells and secretion of inflammatory cytokines of stromal cells, thereby promoting disease progression (51). Notably, exosomes derived from K562 cells, namely the CML cells line, containing human telomerase reverse transcriptase (hTERT) mRNA. These exosomes are transported into telomerase negative fibroblasts, then these mRNAs are translated into active enzymes in the recipient cell. Then these fibroblasts proliferate and live longer, becoming CAF that promote leukemia cells survival and disease progression (61, 62).
In AML, tumor-derived exosomes transfer multiple RNAs related to the pathogenesis of AML into mouse BMSC, including the mRNA of IGF-IR, CXCR4, and MMP9. The transfer of these transcripts promotes the proliferation of receptor cells and causes stromal cells to secrete growth factors that promote the growth of leukemia cells, leading to matrix remodeling (30, 63). Bone marrow stromal cells (BMSCs) treated with AML-derived exosomes increase the expression of genes that support the growth of tumor cells, such as IL-6 and CCL3, decrease the expression of genes that support normal hematopoiesis, such as CXCL12, KITL and IGF1 (31). Thus, through exosome-mediated information exchange, stromal cells increase their proliferation and survival, secrete more pro-leukemic cytokines, inhibit normal hematopoiesis, and promote disease progression.
Interleukin-8 (IL-8) belongs to the chemokine family, whose primary function is thought to be activation and chemotaxis of neutrophils. Corrado et al. found that, in chronic myelogenous leukemia (CML), tumor-derived exosomes containing amphiregulin (AREG), thereby activating epidermal growth factor receptor (EGFR) signaling in bone marrow stromal cells (BMSC), then the recipient stromal cells increase IL-8 expression. In addition, multiple myeloma-derived exosomes are also rich in AREG (64). Researchers also proved that IL-8 can promote leukemia cells survival in vitro and tumor growth of leukemia cells in vivo (39, 41).
Heparinase degrades heparin sulfate, a major component of the extracellular matrix, which results in extracellular matrix remodeling. In recent research, the role of heparinase in tumor progression has been gradually recognized (32). During therapy of myeloma, exosomes derived from tumor cells contain high levels of heparinase. Exposure of untreated tumor cells to these heparinase-positive exosomes activates ERK signaling, thereby regulating tumor cell survival. Besides, macrophages exposed to these heparinase-containing exosomes enhance their ability to secrete tumor necrosis factor-α (TNF-α), an important growth factor that promotes survival of myeloma cells (65).
Other Mechanisms
Many tumor cells produce signature oncoproteins that play an important role in their own malignant proliferation. It has been found that tumor cells can package this signature oncoprotein (or oncoprotein-associated mRNA) into exosomes and deliver it to non-tumor cells, making it a more progressive phenotype and promoting the progression of tumor disease. Burkitt lymphoma cells are closely associated with Epstein-Barr virus (EBV) infection, and the infected tumor cells produce the latent membrane protein1 (LMP1) encoded by EBV. This protein mimics the CD40 signal and stimulates the growth, proliferation, and differentiation of B cells to a plasmacytoid phenotype, which is associated with an increased degree of malignancy. Researchers found that exosomes from Burkitt lymphoma cell lines contain LMP1, and primary B cells can bind and internalize these exosomes to induce cell proliferation and exhibit a malignant phenotype (66). In addition, the macrovesicles (exosome analogs) secreted by CML cells line K562 contain BCR-ABL1 mRNA, which can promote the transformation of bone marrow mesenchymal stem cells (BM-MSC) into a pro-tumor phenotype. These transformed cells can promote the proliferation of K562 cells and the expression of BCR-ABL (18).
Exosomes Have the Function of Impressing Anti-Tumor Immune System
Suppressing Anti-Tumor Immune Cells Directly
Under normal circumstances, natural killer cells (NK) and cytotoxic T lymphocytes (CTL) can recognize and kill malignant cells, and there are also artificially modified immune cells such as CAR-T and CAR-NK cells to kill tumor cells. However, tumor cells can evade the killing effect of immune cells through a variety of mechanisms to achieve the effect of immune evasion. Tumor cells secrete exosomes (sometimes only identified as small extracellular vesicles) containing a variety of immunosuppressive molecules, which can directly inhibit anti-tumor immune cells, such as NK, CTL cells (19, 42). For instance, exosomes obtained from plasma of acute myelogenous leukemia (AML) patients have been found to contain various immunosuppressive membrane molecules, including FasL, PD-L1, TGF-β (67).
For NK cells, activatory killer receptors that recognize non-MHC1 ligand molecules on their surfaces include NKG2D homodimer and natural cytotoxicity receptors (NCR). After NKG2D binds to its ligand, MHC class I chain-related molecules A/B (MICA/B), which expressed on the surface of target cells (namely tumor cells), NK cells can kill target cells and exert their immune surveillance function. Researchers have found that exosomes (or just small extracellular vesicles) derived from AML patients contain high levels of TGF-β1, which can down-regulate the expression level of NKG2D on NK cells, thereby inhibiting NK cell function (12, 68). Correspondingly, the number of NK cells and cytotoxicity in peripheral blood of AML patients are significantly reduced, as well as the expression level of NKG2D. Most importantly, the cytotoxicity of NK cells is restored after neutralization with the addition of anti-TGF-β1 antibodies (8). Interestingly, in T-and B cell leukemia/lymphoma, tumor cell increases MICA/B-containing exosome (or just small extracellular vesicles) secretion under oxidative stress, thereby preventing NKG2D from binding to the corresponding ligand on target cells by providing a decoy to bind NKG2D. This reduces the cytotoxic effect of NK cells on target cells, thereby promoting the immune escape of tumor cells (69).
Natural cytotoxicity receptors (NCR) include NKp46, NKp30 and NKp44, which can activate the killing effect of NK cells by transduction of activation signals (70). The ligand recognized by NCR hasn’t been thoroughly studied, but recent studies have found that BCL2-related athanogene-6 (BAG-6) is the ligand of NKp30 (71). Interestingly, binding of NKp30 with the soluble BAG-6 in plasma inactivates NK cells. In contrast, BAG-6-containing-exosomes can activate NK cells, enhance the cytotoxicity of NK cells, to better kill tumor cells. The level of BAG-6-containing exosomes in the plasma of patients with chronic lymphocytic leukemia (CLL) is decreased, and the level of soluble BAG6 is increased, which leads to the reduction of NK cells mediated killing of tumor cells. This suggests that the imbalance between BAG-6-containing exosomes and soluble BAG6 may mediate the immune escape of tumor cells (20).
For CTL, (namely CD8+T cells) they can express FasL, the ligand of death receptor Fas, to kill their target cells through the Fas/FasL pathway, and express the death receptor Fas to accept death signal. After CTL cells are activated and the immune effect is exerted, Fas on CTL cells binds with FasL on other immunosuppressive cells and mediates its own apoptosis, terminating the immune response in time, thus maintaining the immune balance of the body. However, tumor cells can also take advantage of this mechanism by secreting exosomes or other extracellular vesicles containing FasL to induce apoptosis of CTL cells, thus inhibiting the immune response to tumor cells (21, 72). Researchers have found that AML-derived exosomes mediate CD8+T cell apoptosis through Fas/FasL pathway, down-regulate the expression of CD3ζ, which is critical for transduction of activation signals, and down-regulate the expression of Janus kinase 3 (JAK3) in activated T cells. By these mechanisms, these exosomes inhibit the function of CD8+T cell (40). Besides, exosomes (or small extracellular vesicles) derived from tumor cells can inhibit CD8+T cells function through TGF-β (35).
Activating Immunomodulatory Cells
CD4+CD25+Foxp3+T cells, also known as Treg cells, play an important role in immune regulation by suppressing the immune response through direct contact or by secreting inhibitory cytokines such as IL-10 and TGF-β. In patients with hematological malignancies, such as AML, the number of Tregs in peripheral blood and tumor tissues is increased (73). Researchers have found that exosomes in supernatant and serum of tumor cells from AML patients contain IL-10 and TGF-β1, and these exosomes promote CD4+CD25- T cells proliferation and transformation into CD4+CD25+Foxp3+T cells. In addition, neutralizing antibodies specific to TGFβ1 and/or IL-10 inhibit the ability of these exosomes to amplify Treg cells (40).
MDSCs, namely Myeloid-derived suppressor cells, can produce immunosuppressive molecules to directly inhibit lymphocytes, and can also induce Treg production to indirectly inhibit immune response. It’s found that murine MM exosomes can regulate the signal transducer and activator of transcription 3 (STAT3), promote the growth of MDSCs and enhance its immunosuppressive activity in vivo (43).
M2-type macrophages can suppress the immune response by secreting inhibitory cytokines or direct contact, and participate in immune regulation. Plasma exosomes of CLL patients are rich in non-coding Y RNA hY4. Transfer of this exosome or hY4 alone to monocytes can facilitate their transformation into an immunosuppressive phenotype, namely M2-type macrophages. These transformed macrophages increase their expression of PD-L1 to inhibit immune cells. Besides, further studies show that hY4 transmits information through Toll-like receptors 7 (TLR7) (33). In addition, researchers found that diffuse large B-cell lymphoma-derived exosomes also promote the transformation of macrophages into the M2 phenotype (74).,
Suppressing Anti-Tumor Immune Molecules
The immune system can rely on complement-dependent lysis to kill tumor cells. However, tumor-derived exosomes have been shown to contain casein kinase 2 (CK2), which phosphorylates C9 in the complement system and protects B-lymphoma cells from complement-mediated lysis. In addition, culturing Burkitt lymphoma cells with DRB (a selective inhibitor of protein kinase CK2) enhanced the killing effect of rituximab and complement for tumor cells (24). The complement treated K562 cells can package the membrane attack complex (MAC) inserted into their cell membrane surface into vesicles and release it out of the cell to protect themselves from killing. Mortalin has been shown to promote the secretion of MAC-laden vesicles in K562 cells (22). Whereas Mortalin inhibitor restore K562 cells sensitivity to complement-dependent cytotoxicity (75).
Exosomes Have the Function of Favoring Therapy Resistance of Tumor Cells
Exosomes or Exosome-Like Membrane Structures Can Sequester Intracellular Drugs or Excrete Them From Tumor Cells
Chemotherapeutic drugs can kill most tumor cells, but some tumor cells can use exosomes or similar membrane structures to evade killing through multiple mechanisms. For example, some tumor cells can actively enrich drugs in intracellular vesicles, and then secrete such membrane structure out of the cell, to maintain a low concentration of drugs in tumor cells. Or tumor cells may simply accumulate the drug in intracellular vesicles, isolating the drug from other components in the cytoplasm. Then the tumor cells acquire resistance to drug therapy.
In acute lymphoblastic leukemia (ALL), for example, p-glycoproteins have been found to be incorporated into exosome-like membrane structure, microparticles, and these transport proteins can promote the influx of anthracyclines in tumor cells into the microparticles, thereby trapping the drugs in such membranes. So, the chemotherapeutic drugs can’t kill the tumor cells, and the tumor cells develop therapy resistance (44). In addition, ABCA3 locates on the lysosome and intracellular multivesicular bodies in drug-resistant tumor cells in AML, realizing subcellular drug isolation and endows tumor cells with drug resistance (23, 25).
Diffuse large B-cell lymphoma cells treated with chemotherapeutic agents can expel the chemotherapeutic drugs doxorubicin and pixantrone from the cell by secreting drugs-containing exosomes. Treatment of lymphoma cells with the indomethacin can reduce expression levels of ABCA3, which is important for exosome biosynthesis, and thus inhibiting exosomes biogenesis. This causes doxorubicin and pixantrone drugs to accumulate longer in tumor cells and increases the sensitivity of tumors to these drugs (76).
In addition to these drugs, tumor cells may also excrete other endogenous substances that are not conducive to their survival by secreting extracellular vesicles (EVs). For example, the expression levels of miR-196 and miR-20a are higher in drug-sensitive AML cell line HL60, but lower in drug-resistant cells HL60/AR, and higher levels of miR-196 and miR-20a are found in small extracellular vesicle (sEV) secreted by drug-resistant cells. This selective expulsion of specific miRNAs from cells may be a way for drug-resistant cells to maintain drug resistance. However, this still needs further research (46).
Exosomes and ATP-Binding Cassette Transporter
ABC transporters, namely ATP-binding cassette transporters (ABC), are transmembrane proteins which contains an ATP-binding cassette domain. They are encoded by a large gene family and is highly conserved in long-term evolution. Under normal physiological conditions, ABC protein exists on the plasma membrane and mediates transmembrane transport of sugars, amino acids, and peptides. A variety of drug-resistant tumor cells express ABC protein, which can transport anticancer drugs or endogenous anticancer molecules to the extracellular by means of energy generated by ATP hydrolysis, resulting in drug resistance of tumor cells. Recently, researchers found that exosomes mediate the horizontal transfer of drug resistance properties between tumor cells by transporting ABC-related molecules, such as proteins and mRNAs.
ABCB1 encodes p-glycoprotein (p-gp), namely multidrug resistance protein-1 (MDR-1). Drug-resistant ALL cells line VLB100 (high ABCB1 expression) can directly deliver functional P-gp protein to drug-sensitive ALL cells line CCRF-CEM via membrane-coated particles. CCRF-CEM cells integrate P-gp into their cell membrane and become a drug-resistant phenotype (47). In addition, exosomes from the multidrug-resistant osteosarcoma cell line MG63/DXR30 contain high levels of MDR-1 mRNA. After incubating these exosomes with parental MG-63 cells, MDR-1 mRNA and P-gp in parental MG-63 cells increase, and the sensitivity of parental MG-63 cells to adriamycin also decrease. This suggests that drug resistance can be transmitted between tumor cells not only through protein levels but also through mRNA levels (45).
ABCC1, namely multidrug resistance associated protein 1 (MRP1), is another ABC transporters associated with multidrug resistance. In acute promyelocytic leukemia (APL), APL cells line HL60/AR shows high expression of MRP-1, and they are highly resistant to daunorubicin. Researches treated drug-sensitive HL60 cells with extracellular vesicles produced by HL60/AR, and they found that HL60 cell line begin to express MRP-1, and increase resistance to daunorubicin. This establishes a transfer of drug resistance between the two strains (46, 77, 78).
Exosomes That Carrying Decoy Can Reduce the Effectiveness of Monoclonal Antibodies
CD20 is expressed on the surface of B cells at all stages of development and differentiation except plasma cells, and plays an important regulatory role in the proliferation and differentiation of B cells. Anti-CD20 monoclonal antibodies such as rituximab can treat B-cell lymphoma and chronic lymphocytic leukemia by killing tumor cells through mechanisms such as antibody-dependent cytotoxicity, complement-dependent cytotoxicity, and inducing apoptosis. However, invasive B-cell lymphoma cells can release exosomes containing CD20 to combine with rituximab, reducing their bioavailability, depleting complement, and protecting target cells from antibody attack. Researchers have now discovered that exosome biogenesis is regulated by ATP-binding cassette protein A3 (ABCA3). Pharmacological blocking and/or silencing of ABCA3 inhibits exosome release, thereby enhancing the sensitivity of lymphoma cells to rituximab (79).
Future Perspectives
So far, we have discussed the role of exosomes (sometimes small extracellular vesicles) in various hematological malignancies. And all that is known is for applications, and we have to talk about exosomes applications in oncology. The applications I see as promising fall into two categories. First, since tumor cells can promote the progression of disease through exosomes, we can use drugs to inhibit the synthesis of exosomes or other extracellular vesicles, so as to achieve the purpose of inhibiting tumors (80). Of course, in this process, attention should be paid to the problem that while inhibiting the synthesis and secretion of exosomes in tumor cells, drugs will definitely affect the synthesis of exosomes in normal cells. While using drugs to achieve this goal, we should maximize the impact on tumor cells while minimizing the impact on normal cells. This requires us to distinguish tumor exosomes from normal exosomes as much as possible, and it is best to enable drugs to target tumor exosomes. Second, with the current technical level, synthetic exosomes or other extracellular vesicles can be realized (81). We already know that there is a specific integrin profile on the surface of exosomes, which can enable exosomes to target and bind to corresponding cells. Can we consider synthetic exosomes, whose surface has specific membrane molecules that can target and bind to tumor cells. Besides various tumor suppressor molecules, such as cytotoxins, antibodies and cytokines, can be inserted into synthetic exosomes to achieve precise delivery of anticancer drugs. In addition, using exosomes to exert antitumor effect should have a broader prospect in hematological malignancies. Because exosomes are always transported through body fluids, and hematological malignancies contain most of their tumor cells in the blood, this makes it more likely that these methods will be used in hematological malignancies first.
Conclusion
In some respects, tumor cells embody Darwinian evolution theory- not fit to die, fit to live. Tumor cells survive in their own microenvironment through a variety of mechanisms. For example, these tumor cells disable the immune cells that were supposed to kill them, making immune-regulatory cells more powerful. Not only do they secrete pro-survival factors that promote their own survival and inhibit apoptosis, but they also recruit otherwise neutral stromal cells to help them survive. They can reprogram their microenvironment to produce more blood vessels to supply them with nutrients and oxygen, and more pro-survival cytokines to help them survive, develop, and metastasize. When drugs are used to kill them, they can fight for survival through various mechanism. Tumor cells can use molecular pumps to flush drugs out of their cells, or sequester drugs in vesicles inside cells, reducing drugs impact on tumor cells themselves. They can even release decoys that bind to drugs and protect themselves from damage. Exosomes and other similar membrane structures can be involved in all these processes, on the one hand promoting tumor cell survival, on the other hand reducing the therapeutic effect. In this review, we introduce in detail the relevant studies on the role of exosomes in hematological malignancies in recent years, hoping to promote in-depth research in this area and conquer hematological malignancies as soon as possible.
Author Contributions
HW made the figures; XZ and HW wrote the paper; XZ and YY reviewed the paper. All authors contributed to the article and approved the submitted version.
Conflict of Interest
The authors declare that the research was conducted in the absence of any commercial or financial relationships that could be construed as a potential conflict of interest.
Publisher’s Note
All claims expressed in this article are solely those of the authors and do not necessarily represent those of their affiliated organizations, or those of the publisher, the editors and the reviewers. Any product that may be evaluated in this article, or claim that may be made by its manufacturer, is not guaranteed or endorsed by the publisher.
Abbreviations
AML, acute myelogenous leukemia; APL, acute promyelocytic leukemia; BM-MSC, bone marrow mesenchymal stem cells; BMSC, bone marrow stromal cell; CAF, cancer-associated fibroblast; CK2, casein kinase 2; CLL, chronic lymphocytic leukemia; CML, chronic myelogenous leukemia; EGFR, epidermal growth factor receptor; EVs, extracellular vesicles; FGF, fibroblast growth factor; FIH-1, factor-inhibiting hypoxia-inducible factor 1; HIF-1, hypoxia inducible factor-1; hTERT, human telomerase reverse transcriptase; HUVECs, human umbilical vein endothelial cells; IL-8, Interleukin-8; LMP1, latent membrane protein1; MAC, membrane attack complex; MDSC, myeloid-derived suppressor cell; MDR-1, multidrug resistance protein-1; MICA/B, MHC class I chain-related molecules A/B; MRP1, multidrug resistance associated protein 1; NCR, natural cytotoxicity receptors; P-gp, p-glycoprotein; sEV, small extracellular vesicles; STAT3, signal transducer and activator of transcription 3; TGF-β, transforming growth factor-β; VEGF, vascular endothelial growth factor.
References
1. Théry C, Witwer KW, Aikawa E, Alcaraz MJ, Anderson JD, Andriantsitohaina R, et al. Minimal Information for Studies of Extracellular Vesicles 2018 (Misev2018): A Position Statement of the International Society for Extracellular Vesicles and Update of the Misev2014 Guidelines. J Extracell Vesicles (2018) 7(1):1535750. doi: 10.1080/20013078.2018.1535750
2. Pan BT, Johnstone RM. Fate of the Transferrin Receptor During Maturation of Sheep Reticulocytes In Vitro: Selective Externalization of the Receptor. Cell (1983) 33(3):967–78. doi: 10.1016/0092-8674(83)90040-5
3. Johnstone RM, Adam M, Hammond JR, Orr L, Turbide C. Vesicle Formation During Reticulocyte Maturation. Association of Plasma Membrane Activities With Released Vesicles (Exosomes). J Biol Chem (1987) 262(19):9412–20. doi: 10.1016/S0021-9258(18)48095-7
4. Chevillet JR, Kang Q, Ruf IK, Briggs HA, Vojtech LN, Hughes SM, et al. Quantitative and Stoichiometric Analysis of the Microrna Content of Exosomes. Proc Natl Acad Sci USA (2014) 111(41):14888–93. doi: 10.1073/pnas.1408301111
5. Rezaie J, Rahbarghazi R, Pezeshki M, Mazhar M, Yekani F, Khaksar M, et al. Cardioprotective Role of Extracellular Vesicles: A Highlight on Exosome Beneficial Effects in Cardiovascular Diseases. J Cell Physiol (2019) 234(12):21732–45. doi: 10.1002/jcp.28894
6. Vahabi A, Rezaie J, Hassanpour M, Panahi Y, Nemati M, Rasmi Y, et al. Tumor Cells-Derived Exosomal Circrnas: Novel Cancer Drivers, Molecular Mechanisms, and Clinical Opportunities. Biochem Pharmacol (2022) 200:115038. doi: 10.1016/j.bcp.2022.115038
7. Hoshino A, Costa-Silva B, Shen TL, Rodrigues G, Hashimoto A, Tesic Mark M, et al. Tumour Exosome Integrins Determine Organotropic Metastasis. Nature (2015) 527(7578):329–35. doi: 10.1038/nature15756
8. Szczepanski MJ, Szajnik M, Welsh A, Whiteside TL, Boyiadzis M. Blast-Derived Microvesicles in Sera From Patients With Acute Myeloid Leukemia Suppress Natural Killer Cell Function Via Membrane-Associated Transforming Growth Factor-Beta1. Haematologica (2011) 96(9):1302–9. doi: 10.3324/haematol.2010.039743
9. Wieckowski E, Whiteside TL. Human Tumor-Derived Vs Dendritic Cell-Derived Exosomes Have Distinct Biologic Roles and Molecular Profiles. Immunol Res (2006) 36(1-3):247–54. doi: 10.1385/ir:36:1:247
10. Bernardi S, Zanaglio C, Farina M, Polverelli N, Malagola M, Russo D. Dsdna From Extracellular Vesicles (Evs) in Adult Aml. Ann Hematol (2021) 100(5):1355–6. doi: 10.1007/s00277-020-04109-z
11. Kontopoulou E, Strachan S, Reinhardt K, Kunz F, Walter C, Walkenfort B, et al. Evaluation of Dsdna From Extracellular Vesicles (Evs) in Pediatric Aml Diagnostics. Ann Hematol (2020) 99(3):459–75. doi: 10.1007/s00277-019-03866-w
12. Hong CS, Muller L, Whiteside TL, Boyiadzis M. Plasma Exosomes as Markers of Therapeutic Response in Patients With Acute Myeloid Leukemia. Front Immunol (2014) 5:160. doi: 10.3389/fimmu.2014.00160
13. Bernardi S, Farina M. Exosomes and Extracellular Vesicles in Myeloid Neoplasia: The Multiple and Complex Roles Played by These “Magic Bullets”. Biol (Basel) (2021) 10(2):105. doi: 10.3390/biology10020105
14. Trino S, Lamorte D, Caivano A, De Luca L, Sgambato A, Laurenzana I. Clinical Relevance of Extracellular Vesicles in Hematological Neoplasms: From Liquid Biopsy to Cell Biopsy. Leukemia (2021) 35(3):661–78. doi: 10.1038/s41375-020-01104-1
15. Abbaszade Dibavar M, Pourbagheri-Sigaroodi A, Asemani Y, Salari S, Bashash D. Extracellular Vesicles (Evs): What We Know of the Mesmerizing Roles of These Tiny Vesicles in Hematological Malignancies? Life Sci (2021) 271:119177. doi: 10.1016/j.lfs.2021.119177
16. Roccaro AM, Sacco A, Maiso P, Azab AK, Tai YT, Reagan M, et al. Bm Mesenchymal Stromal Cell-Derived Exosomes Facilitate Multiple Myeloma Progression. J Clin Invest (2013) 123(4):1542–55. doi: 10.1172/jci66517
17. Rezaie J, Ahmadi M, Ravanbakhsh R, Mojarad B, Mahbubfam S, Shaban SA, et al. Tumor-Derived Extracellular Vesicles: The Metastatic Organotropism Drivers. Life Sci (2022) 289:120216. doi: 10.1016/j.lfs.2021.120216
18. Fu FF, Zhu XJ, Wang HX, Zhang LM, Yuan GL, Chen ZC, et al. Bcr-Abl1-Positive Microvesicles Malignantly Transform Human Bone Marrow Mesenchymal Stem Cells in Vitro. Acta Pharmacol Sin (2017) 38(11):1475–85. doi: 10.1038/aps.2017.116
19. Whiteside TL. Tumor-Derived Exosomes and Their Role in Cancer Progression. Adv Clin Chem (2016) 74:103–41. doi: 10.1016/bs.acc.2015.12.005
20. Reiners KS, Topolar D, Henke A, Simhadri VR, Kessler J, Sauer M, et al. Soluble Ligands for Nk Cell Receptors Promote Evasion of Chronic Lymphocytic Leukemia Cells From Nk Cell Anti-Tumor Activity. Blood (2013) 121(18):3658–65. doi: 10.1182/blood-2013-01-476606
21. Wieckowski EU, Visus C, Szajnik M, Szczepanski MJ, Storkus WJ, Whiteside TL. Tumor-Derived Microvesicles Promote Regulatory T Cell Expansion and Induce Apoptosis in Tumor-Reactive Activated Cd8+ T Lymphocytes. J Immunol (2009) 183(6):3720–30. doi: 10.4049/jimmunol.0900970
22. Pilzer D, Fishelson Z. Mortalin/Grp75 Promotes Release of Membrane Vesicles From Immune Attacked Cells and Protection From Complement-Mediated Lysis. Int Immunol (2005) 17(9):1239–48. doi: 10.1093/intimm/dxh300
23. Chapuy B, Koch R, Radunski U, Corsham S, Cheong N, Inagaki N, et al. Intracellular Abc Transporter A3 Confers Multidrug Resistance in Leukemia Cells by Lysosomal Drug Sequestration. Leukemia (2008) 22(8):1576–86. doi: 10.1038/leu.2008.103
24. Bohana-Kashtan O, Pinna LA, Fishelson Z. Extracellular Phosphorylation of C9 by Protein Kinase Ck2 Regulates Complement-Mediated Lysis. Eur J Immunol (2005) 35(6):1939–48. doi: 10.1002/eji.200425716
25. Hekmatirad S, Moloudizargari M, Moghadamnia AA, Kazemi S, Mohammadnia-Afrouzi M, Baeeri M, et al. Inhibition of Exosome Release Sensitizes U937 Cells to Pegylated Liposomal Doxorubicin. Front Immunol (2021) 12:692654. doi: 10.3389/fimmu.2021.692654
26. Ohyashiki JH, Umezu T, Ohyashiki K. Exosomes Promote Bone Marrow Angiogenesis in Hematologic Neoplasia: The Role of Hypoxia. Curr Opin Hematol (2016) 23(3):268–73. doi: 10.1097/moh.0000000000000235
27. Gross JC, Chaudhary V, Bartscherer K, Boutros M. Active Wnt Proteins Are Secreted on Exosomes. Nat Cell Biol (2012) 14(10):1036–45. doi: 10.1038/ncb2574
28. Wang J, Hendrix A, Hernot S, Lemaire M, De Bruyne E, Van Valckenborgh E, et al. Bone Marrow Stromal Cell-Derived Exosomes as Communicators in Drug Resistance in Multiple Myeloma Cells. Blood (2014) 124(4):555–66. doi: 10.1182/blood-2014-03-562439
29. Webber J, Steadman R, Mason MD, Tabi Z, Clayton A. Cancer Exosomes Trigger Fibroblast to Myofibroblast Differentiation. Cancer Res (2010) 70(23):9621–30. doi: 10.1158/0008-5472.Can-10-1722
30. Rezaie J, Aslan C, Ahmadi M, Zolbanin NM, Kashanchi F, Jafari R. The Versatile Role of Exosomes in Human Retroviral Infections: From Immunopathogenesis to Clinical Application. Cell Biosci (2021) 11(1):19. doi: 10.1186/s13578-021-00537-0
31. Kumar B, Garcia M, Weng L, Jung X, Murakami JL, Hu X, et al. Acute Myeloid Leukemia Transforms the Bone Marrow Niche Into a Leukemia-Permissive Microenvironment Through Exosome Secretion. Leukemia (2018) 32(3):575–87. doi: 10.1038/leu.2017.259
32. Masola V, Zaza G, Gambaro G, Franchi M, Onisto M. Role of Heparanase in Tumor Progression: Molecular Aspects and Therapeutic Options. Semin Cancer Biol (2020) 62:86–98. doi: 10.1016/j.semcancer.2019.07.014
33. Haderk F, Schulz R, Iskar M, Cid LL, Worst T, Willmund KV, et al. Tumor-Derived Exosomes Modulate Pd-L1 Expression in Monocytes. Sci Immunol (2017) 2(13):eaah5509. doi: 10.1126/sciimmunol.aah5509
34. Umezu T, Tadokoro H, Azuma K, Yoshizawa S, Ohyashiki K, Ohyashiki JH. Exosomal Mir-135b Shed From Hypoxic Multiple Myeloma Cells Enhances Angiogenesis by Targeting Factor-Inhibiting Hif-1. Blood (2014) 124(25):3748–57. doi: 10.1182/blood-2014-05-576116
35. Valenti R, Huber V, Filipazzi P, Pilla L, Sovena G, Villa A, et al. Human Tumor-Released Microvesicles Promote the Differentiation of Myeloid Cells With Transforming Growth Factor-Beta-Mediated Suppressive Activity on T Lymphocytes. Cancer Res (2006) 66(18):9290–8. doi: 10.1158/0008-5472.Can-06-1819
36. Nishida N, Yano H, Nishida T, Kamura T, Kojiro M. Angiogenesis in Cancer. Vasc Health Risk Manag (2006) 2(3):213–9. doi: 10.2147/vhrm.2006.2.3.213
37. Hassanpour M, Rezabakhsh A, Rezaie J, Nouri M, Rahbarghazi R. Exosomal Cargos Modulate Autophagy in Recipient Cells Via Different Signaling Pathways. Cell Biosci (2020) 10:92. doi: 10.1186/s13578-020-00455-7
38. Raimondo S, Saieva L, Corrado C, Fontana S, Flugy A, Rizzo A, et al. Chronic Myeloid Leukemia-Derived Exosomes Promote Tumor Growth Through an Autocrine Mechanism. Cell Commun Signal (2015) 13:8. doi: 10.1186/s12964-015-0086-x
39. Corrado C, Raimondo S, Saieva L, Flugy AM, De Leo G, Alessandro R. Exosome-Mediated Crosstalk Between Chronic Myelogenous Leukemia Cells and Human Bone Marrow Stromal Cells Triggers an Interleukin 8-Dependent Survival of Leukemia Cells. Cancer Lett (2014) 348(1-2):71–6. doi: 10.1016/j.canlet.2014.03.009
40. Whiteside TL. Immune Modulation of T-Cell and Nk (Natural Killer) Cell Activities by Texs (Tumour-Derived Exosomes). Biochem Soc Trans (2013) 41(1):245–51. doi: 10.1042/bst20120265
41. Corrado C, Saieva L, Raimondo S, Santoro A, De Leo G, Alessandro R. Chronic Myelogenous Leukaemia Exosomes Modulate Bone Marrow Microenvironment Through Activation of Epidermal Growth Factor Receptor. J Cell Mol Med (2016) 20(10):1829–39. doi: 10.1111/jcmm.12873
42. Hong CS, Sharma P, Yerneni SS, Simms P, Jackson EK, Whiteside TL, et al. Circulating Exosomes Carrying an Immunosuppressive Cargo Interfere With Cellular Immunotherapy in Acute Myeloid Leukemia. Sci Rep (2017) 7(1):14684. doi: 10.1038/s41598-017-14661-w
43. Wang J, De Veirman K, Faict S, Frassanito MA, Ribatti D, Vacca A, et al. Multiple Myeloma Exosomes Establish a Favourable Bone Marrow Microenvironment With Enhanced Angiogenesis and Immunosuppression. J Pathol (2016) 239(2):162–73. doi: 10.1002/path.4712
44. Gong J, Luk F, Jaiswal R, George AM, Grau GE, Bebawy M. Microparticle Drug Sequestration Provides a Parallel Pathway in the Acquisition of Cancer Drug Resistance. Eur J Pharmacol (2013) 721(1-3):116–25. doi: 10.1016/j.ejphar.2013.09.044
45. Torreggiani E, Roncuzzi L, Perut F, Zini N, Baldini N. Multimodal Transfer of Mdr by Exosomes in Human Osteosarcoma. Int J Oncol (2016) 49(1):189–96. doi: 10.3892/ijo.2016.3509
46. Bouvy C, Wannez A, Laloy J, Chatelain C, Dogné JM. Transfer of Multidrug Resistance Among Acute Myeloid Leukemia Cells Via Extracellular Vesicles and Their Microrna Cargo. Leuk Res (2017) 62:70–6. doi: 10.1016/j.leukres.2017.09.014
47. Bebawy M, Combes V, Lee E, Jaiswal R, Gong J, Bonhoure A, et al. Membrane Microparticles Mediate Transfer of P-Glycoprotein to Drug Sensitive Cancer Cells. Leukemia (2009) 23(9):1643–9. doi: 10.1038/leu.2009.76
48. Khaksar M, Sayyari M, Rezaie J, Pouyafar A, Montazersaheb S, Rahbarghazi R. High Glucose Condition Limited the Angiogenic/Cardiogenic Capacity of Murine Cardiac Progenitor Cells in In Vitro and In Vivo Milieu. Cell Biochem Funct (2018) 36(7):346–56. doi: 10.1002/cbf.3354
49. Mineo M, Garfield SH, Taverna S, Flugy A, De Leo G, Alessandro R, et al. Exosomes Released by K562 Chronic Myeloid Leukemia Cells Promote Angiogenesis in a Src-Dependent Fashion. Angiogenesis (2012) 15(1):33–45. doi: 10.1007/s10456-011-9241-1
50. Umezu T, Ohyashiki K, Kuroda M, Ohyashiki JH. Leukemia Cell to Endothelial Cell Communication Via Exosomal Mirnas. Oncogene (2013) 32(22):2747–55. doi: 10.1038/onc.2012.295
51. Paggetti J, Haderk F, Seiffert M, Janji B, Distler U, Ammerlaan W, et al. Exosomes Released by Chronic Lymphocytic Leukemia Cells Induce the Transition of Stromal Cells Into Cancer-Associated Fibroblasts. Blood (2015) 126(9):1106–17. doi: 10.1182/blood-2014-12-618025
52. Yang Y, Li J, Geng Y. Exosomes Derived From Chronic Lymphocytic Leukaemia Cells Transfer Mir-146a to Induce the Transition of Mesenchymal Stromal Cells Into Cancer-Associated Fibroblasts. J Biochem (2020) 168(5):491–8. doi: 10.1093/jb/mvaa064
53. Syn N, Wang L, Sethi G, Thiery JP, Goh BC. Exosome-Mediated Metastasis: From Epithelial-Mesenchymal Transition to Escape From Immunosurveillance. Trends Pharmacol Sci (2016) 37(7):606–17. doi: 10.1016/j.tips.2016.04.006
54. Zhou J, Wang S, Sun K, Chng WJ. The Emerging Roles of Exosomes in Leukemogeneis. Oncotarget (2016) 7(31):50698–707. doi: 10.18632/oncotarget.9333
55. Koch R, Demant M, Aung T, Diering N, Cicholas A, Chapuy B, et al. Populational Equilibrium Through Exosome-Mediated Wnt Signaling in Tumor Progression of Diffuse Large B-Cell Lymphoma. Blood (2014) 123(14):2189–98. doi: 10.1182/blood-2013-08-523886
56. Wojtuszkiewicz A, Schuurhuis GJ, Kessler FL, Piersma SR, Knol JC, Pham TV, et al. Exosomes Secreted by Apoptosis-Resistant Acute Myeloid Leukemia (Aml) Blasts Harbor Regulatory Network Proteins Potentially Involved in Antagonism of Apoptosis. Mol Cell Proteomics (2016) 15(4):1281–98. doi: 10.1074/mcp.M115.052944
57. Min QH, Wang XZ, Zhang J, Chen QG, Li SQ, Liu XQ, et al. Exosomes Derived From Imatinib-Resistant Chronic Myeloid Leukemia Cells Mediate a Horizontal Transfer of Drug-Resistant Trait by Delivering Mir-365. Exp Cell Res (2018) 362(2):386–93. doi: 10.1016/j.yexcr.2017.12.001
58. Hrdinova T, Toman O, Dresler J, Klimentova J, Salovska B, Pajer P, et al. Exosomes Released by Imatinib−Resistant K562 Cells Contain Specific Membrane Markers, Ifitm3, Cd146 and Cd36 and Increase the Survival of Imatinib−Sensitive Cells in the Presence of Imatinib. Int J Oncol (2021) 58(2):238–50. doi: 10.3892/ijo.2020.5163
59. Chen Y, McAndrews KM, Kalluri R. Clinical and Therapeutic Relevance of Cancer-Associated Fibroblasts. Nat Rev Clin Oncol (2021) 18(12):792–804. doi: 10.1038/s41571-021-00546-5
60. Ringuette Goulet C, Bernard G, Tremblay S, Chabaud S, Bolduc S, Pouliot F. Exosomes Induce Fibroblast Differentiation Into Cancer-Associated Fibroblasts Through Tgfβ Signaling. Mol Cancer Res (2018) 16(7):1196–204. doi: 10.1158/1541-7786.Mcr-17-0784
61. Gutkin A, Uziel O, Beery E, Nordenberg J, Pinchasi M, Goldvaser H, et al. Tumor Cells Derived Exosomes Contain Htert Mrna and Transform Nonmalignant Fibroblasts Into Telomerase Positive Cells. Oncotarget (2016) 7(37):59173–88. doi: 10.18632/oncotarget.10384
62. Gao X, Wan Z, Wei M, Dong Y, Zhao Y, Chen X, et al. Chronic Myelogenous Leukemia Cells Remodel the Bone Marrow Niche Via Exosome-Mediated Transfer of Mir-320. Theranostics (2019) 9(19):5642–56. doi: 10.7150/thno.34813
63. Huan J, Hornick NI, Shurtleff MJ, Skinner AM, Goloviznina NA, Roberts CT Jr., et al. Rna Trafficking by Acute Myelogenous Leukemia Exosomes. Cancer Res (2013) 73(2):918–29. doi: 10.1158/0008-5472.Can-12-2184
64. Raimondo S, Saieva L, Vicario E, Pucci M, Toscani D, Manno M, et al. Multiple Myeloma-Derived Exosomes Are Enriched of Amphiregulin (Areg) and Activate the Epidermal Growth Factor Pathway in the Bone Microenvironment Leading to Osteoclastogenesis. J Hematol Oncol (2019) 12(1):2. doi: 10.1186/s13045-018-0689-y
65. Bandari SK, Purushothaman A, Ramani VC, Brinkley GJ, Chandrashekar DS, Varambally S, et al. Chemotherapy Induces Secretion of Exosomes Loaded With Heparanase That Degrades Extracellular Matrix and Impacts Tumor and Host Cell Behavior. Matrix Biol (2018) 65:104–18. doi: 10.1016/j.matbio.2017.09.001
66. Gutzeit C, Nagy N, Gentile M, Lyberg K, Gumz J, Vallhov H, et al. Exosomes Derived From Burkitt’s Lymphoma Cell Lines Induce Proliferation, Differentiation, and Class-Switch Recombination in B Cells. J Immunol (2014) 192(12):5852–62. doi: 10.4049/jimmunol.1302068
67. Hong CS, Muller L, Boyiadzis M, Whiteside TL. Isolation and Characterization of Cd34+ Blast-Derived Exosomes in Acute Myeloid Leukemia. PloS One (2014) 9(8):e103310. doi: 10.1371/journal.pone.0103310
68. Clayton A, Mitchell JP, Court J, Linnane S, Mason MD, Tabi Z. Human Tumor-Derived Exosomes Down-Modulate Nkg2d Expression. J Immunol (2008) 180(11):7249–58. doi: 10.4049/jimmunol.180.11.7249
69. Hedlund M, Nagaeva O, Kargl D, Baranov V, Mincheva-Nilsson L. Thermal- and Oxidative Stress Causes Enhanced Release of Nkg2d Ligand-Bearing Immunosuppressive Exosomes in Leukemia/Lymphoma T and B Cells. PloS One (2011) 6(2):e16899. doi: 10.1371/journal.pone.0016899
70. Raulet DH, Guerra N. Oncogenic Stress Sensed by the Immune System: Role of Natural Killer Cell Receptors. Nat Rev Immunol (2009) 9(8):568–80. doi: 10.1038/nri2604
71. Binici J, Koch J. Bag-6, a Jack of All Trades in Health and Disease. Cell Mol Life Sci (2014) 71(10):1829–37. doi: 10.1007/s00018-013-1522-y
72. Abusamra AJ, Zhong Z, Zheng X, Li M, Ichim TE, Chin JL, et al. Tumor Exosomes Expressing Fas Ligand Mediate CD8+ T-Cell Apoptosis. Blood Cells Mol Dis (2005) 35(2):169–73. doi: 10.1016/j.bcmd.2005.07.001
73. Szczepanski MJ, Szajnik M, Czystowska M, Mandapathil M, Strauss L, Welsh A, et al. Increased Frequency and Suppression by Regulatory T Cells in Patients With Acute Myelogenous Leukemia. Clin Cancer Res (2009) 15(10):3325–32. doi: 10.1158/1078-0432.Ccr-08-3010
74. Ling HY, Yang Z, Wang PJ, Sun Y, Ju SG, Li J, et al. Diffuse Large B-Cell Lymphoma-Derived Exosomes Push Macrophage Polarization Toward M2 Phenotype Via Gp130/Stat3 Signaling Pathway. Chem Biol Interact (2022) 352:109779. doi: 10.1016/j.cbi.2021.109779
75. Pilzer D, Saar M, Koya K, Fishelson Z. Mortalin Inhibitors Sensitize K562 Leukemia Cells to Complement-Dependent Cytotoxicity. Int J Cancer (2010) 126(6):1428–35. doi: 10.1002/ijc.24888
76. Koch R, Aung T, Vogel D, Chapuy B, Wenzel D, Becker S, et al. Nuclear Trapping Through Inhibition of Exosomal Export by Indomethacin Increases Cytostatic Efficacy of Doxorubicin and Pixantrone. Clin Cancer Res (2016) 22(2):395–404. doi: 10.1158/1078-0432.Ccr-15-0577
77. Namee NM, O’Driscoll L. Extracellular Vesicles and Anti-Cancer Drug Resistance. Biochim Biophys Acta Rev Cancer (2018) 1870(2):123–36. doi: 10.1016/j.bbcan.2018.07.003
78. Lu JF, Luk F, Gong J, Jaiswal R, Grau GE, Bebawy M. Microparticles Mediate Mrp1 Intercellular Transfer and the Re-Templating of Intrinsic Resistance Pathways. Pharmacol Res (2013) 76:77–83. doi: 10.1016/j.phrs.2013.07.009
79. Aung T, Chapuy B, Vogel D, Wenzel D, Oppermann M, Lahmann M, et al. Exosomal Evasion of Humoral Immunotherapy in Aggressive B-Cell Lymphoma Modulated by Atp-Binding Cassette Transporter A3. Proc Natl Acad Sci USA (2011) 108(37):15336–41. doi: 10.1073/pnas.1102855108
80. Rezaie J, Akbari A, Rahbarghazi R. Inhibition of Extracellular Vesicle Biogenesis in Tumor Cells: A Possible Way to Reduce Tumorigenesis. Cell Biochem Funct (2022) 40(3):248–62. doi: 10.1002/cbf.3695
Keywords: exosome, hematological malignancies, therapy resistance, immunosuppression, tumor progression
Citation: Wang H, You Y and Zhu X (2022) The Role of Exosomes in the Progression and Therapeutic Resistance of Hematological Malignancies. Front. Oncol. 12:887518. doi: 10.3389/fonc.2022.887518
Received: 01 March 2022; Accepted: 28 April 2022;
Published: 26 May 2022.
Edited by:
Anjali Mishra, Sidney Kimmel Cancer Center, United StatesReviewed by:
Simona Bernardi, University of Brescia, ItalyMarc Poirot, U1037 Centre de Recherche en Cancérologie de Toulouse (INSERM), France
Jafar Rezaie, Urmia University of Medical Sciences, Iran
Copyright © 2022 Wang, You and Zhu. This is an open-access article distributed under the terms of the Creative Commons Attribution License (CC BY). The use, distribution or reproduction in other forums is permitted, provided the original author(s) and the copyright owner(s) are credited and that the original publication in this journal is cited, in accordance with accepted academic practice. No use, distribution or reproduction is permitted which does not comply with these terms.
*Correspondence: Xiaojian Zhu, zhuxiaojian@hust.edu.cn