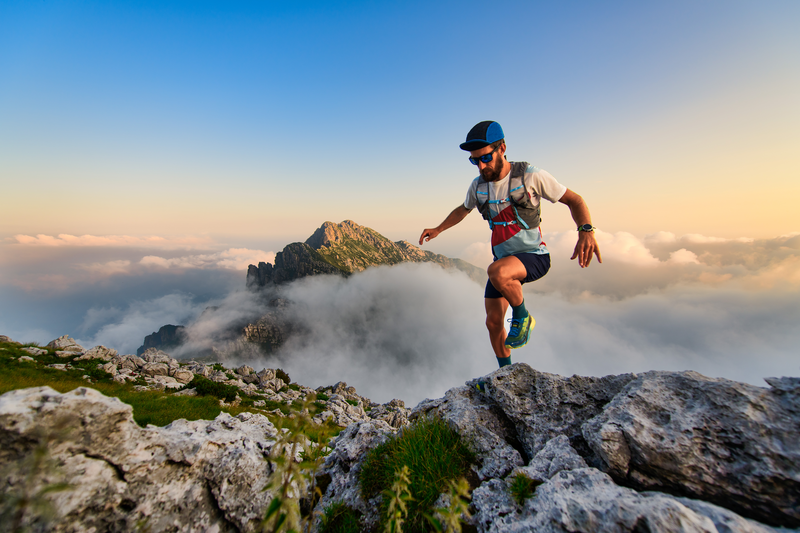
94% of researchers rate our articles as excellent or good
Learn more about the work of our research integrity team to safeguard the quality of each article we publish.
Find out more
REVIEW article
Front. Oncol. , 08 August 2022
Sec. Molecular and Cellular Oncology
Volume 12 - 2022 | https://doi.org/10.3389/fonc.2022.882896
This article is part of the Research Topic Diagnostic, Prognostic and Predictive Factors of Response in the Era of Precision Oncology in Breast Cancer View all 33 articles
Adaptive and innate immune cells play a crucial role as regulators of cancer development.
Inflammatory cells in blood flow seem to be involved in pro-tumor activities and contribute to breast cancer progression. Circulating lymphocyte ratios such as the platelet-lymphocytes ratio (PLR), the monocyte-lymphocyte ratio (MLR) and the neutrophil-lymphocyte ratio (NLR) are new reproducible, routinely feasible and cheap biomarkers of immune response. These indexes have been correlated to prognosis in many solid tumors and there is growing evidence on their clinical applicability as independent prognostic markers also for breast cancer.
In this review we give an overview of the possible value of lymphocytic indexes in advanced breast cancer prognosis and prediction of outcome. Furthermore, targeting the immune system appear to be a promising therapeutic strategy for breast cancer, especially macrophage-targeted therapies. Herein we present an overview of the ongoing clinical trials testing systemic inflammatory cells as therapeutic targets in breast cancer.
Over the past years, the role of the immune system in cancer development and progression has gained increasing attention. The immune system has a paradoxical behavior during cancer development; some immune cells are able to recognize tumor cells and defend the host (immunosurveillance), whereas other cells can contribute to activating immune escape mechanisms (1). A condition of persistent smoldering inflammation, determined by oncogenic mutations in tumors, creates an inflammatory microenvironment typical of cancer tissue (2). This “low grade” inflammation leads to the proliferation and survival of malignant cells, promotes angiogenesis, subverts adaptive immune responses and leads the immune cells towards an immunosuppressive phenotype (3). Tumor-associated chronic inflammation is definitely a hallmark of cancer that fosters progression to a metastatic stage (4).
Immune cells in tumor microenvironment (TME) and in the peripheral blood are significantly involved in breast cancer (BC) diffusion (5). Circulating inflammatory cells are characterized by pro-tumor activities such as enhanced angiogenesis, chemokine production or immune-surveillance and promote the metastatic potential of tumor cells (6).
BC is linked to modifications in systemic inflammatory indexes. Platelet, neutrophil, lymphocyte, monocyte counts, inflammatory cytokines and acute phase proteins (like C-reactive protein or PCR) are considered potentially new prognostic parameters. Combined indexes have been determined to define the condition of systemic inflammation as the platelet-lymphocytes ratio (PLR), monocyte-lymphocyte ratio (MLR), neutrophil-lymphocyte ratio (NLR) and systemic immune-inflammation index (SII) (7). These lymphocyte indexes have been correlated to prognosis in many solid tumors and are considered applicable in clinical practice as reliable independent prognostic markers (8–12).
It is plausible that imbalances in the ratio of immune cellular counts may provide an insight into underlying tumor progression and prognosis also in patients with BC. The availability and non-invasive nature of these indexes makes them affordable biological markers.
One of the major questions is whether cancer-related inflammation can be exploited into useful approaches in treating advanced/metastatic BC (aBC).
In this review, we will provide an overview of the potential prognostic value of lymphocytic indexes in aBC and discuss the therapeutic potential of targeting the immune system in this context.
Platelets have a crucial role as regulators of inflammation and are involved in various stages in BC development and dissemination (13). Tumor-activated platelets further contribute to cancer progression by promoting critical processes such as angiogenesis and metastasis. Platelets modulate innate immunity (antigen presentation by dendritic cells, monocyte recruitment and differentiation or neutrophil extracellular trap formation) and also promote thrombosis and metastasis (for example with the mechanism of lysophosphatidic acid-dependent (LPA) metastasization or formation of platelet clots) (Figure 1) (14). Moreover, the adaptive immune responses can be modulated also by platelets inducing the differentiation of T-helper 17 cells (13).
Figure 1 Circulating inflammatory cells in blood flow in breast cancer. Inflammatory cells are involved in many ways in promoting cancer cells invasiveness. Evasion of tumor cells from the primary site into circulation is partially permitted by tumor associated macrophages and other immune cell responsible for an immunouppressive microenvironment. Immune cells are also attracted by tumor factors from the blood flows. Circulating tumor cells (CTCs) in blood flow are accompanied in cluster with macrophages and monocytes. Neutrophils release neutrophils extracellular traps (NETs) that determines aggregation of CTCs and other immune cells guaranteeing their survival and a favorable microenvironment in circulation. Platelets, activated by tumor promoting factors, trigger hemostasis mechanisms that catch CTCs cells favoring the adhesion to vessel walls. Lysophosphatidic acid (LPA) dependent mechanism, platelet derived growth factors (PDGFs), interleukin-8 (IL-8) and platelet-derived extracellular vesicles (PEVs) contribute to the formation of platelet clots that include and protect CTCs. CTCs that are not included in aggregates are unlikely to survive in the bloodstream. Immune cells are also important in the formation of the metastatic-niche. Macrophages associated to metastasis (MAMs) derive from the bloodstream and are recruited in the process of metastasis. Adapted from “Breast Cancer to Brain Metastasis”, by BioRender.com (2022). Retrieved from https://app.biorender.com/biorender-templates.
Platelet-derived growth factors (PDGFs) contribute to sustaining proliferative signals. Among them we recognize PDGF, transforming growth factor –beta (TGF-beta) and platelet -derived endothelial cell growth factor (PD-ECGF) that are often produced by BC cells and enhance their progression and aggressiveness (15). Platelet-derived extracellular vesicles (PEVs) are also considered potential mediators in the activation of signaling connected to migration in metastatic BC cell lines (16).
Furthermore, the link between hemostasis and BC assumes that platelets have a central role in disease progression (17). In peripheral blood, tumor cell interaction with adherent platelets arrest tumor cells thanks to adhesion proteins and crosslinking plasma protein ligands that support platelets to adhere to the vessel wall. Tumor cells that fail to attach are rapidly cleared from the circulation and undergo apoptosis. To facilitate adhesion to platelets, some cancer cells can upregulate aberrant surface proteins. The binding to platelets helps metastatic cells to arrest within the microvessels of their target organs, where then they extravasate, start to proliferate at the attachment site, or remain dormant for extended periods of time (18). PDGFs support the proliferation and extravasation of invading metastatic cells in the metastatic niche (Figure 1) (19).
Furthermore, BC cells secrete high levels of interleukin-8 (IL-8) in response to platelets that may activate their AKT pathway promoting an invasive capacity. Patients with BC receiving aspirin had lower circulating IL-8, and their platelets did not increase tumor cell invasion compared with patients not receiving aspirin (20, 21).
In BC, elevated platelet-related markers may be associated with poor prognosis. The meta-analysis of 17,079 individuals conducted by Guo et al. confirms that an high PLR is associated with poor overall survival (OS) as well as high risk of recurrence for BC patients (22). However the metanalisis includes few studies about aBC patients, therefore the specific relationship between PLR and aBC need to be better explored.
Neutrophils have a central role in inflammatory response; patients with various cancer types, including BC, often exhibit increased numbers of circulating neutrophils (9).
Neutrophils with an immature phenotype have been observed in the blood stream of cancer patients. Increased levels of tumor-induced granulocyte-colony stimulating factor (G-CSF) and granulocyte–macrophage-colony stimulating factor (GM-CSF) enhance hematopoiesis towards the production of myeloid cells, granulocyte–monocyte progenitors (GMPs) and neutrophil progenitors (23).
Neutrophils seem to be involved in BC progression promoting metastasis-initiating cells that drive cancer spread (24, 25). They can secrete immunosuppressive mediators and angiogenic factors such as reactive oxygen species, vascular endothelial growth factor (VEGF) and matrix metalloproteinase 9 (MMP-9) contributing to a pro-tumor microenvironment (26). Neutrophil-secreted factors alter the heterogeneity of cancer cells, favoring breast metastasis-initiating cells (27). In a BC model, neutrophils induced by tumor cells showed to suppress CD8+ T lymphocytes promoting metastasis through immunosuppression (28). Furthermore, it has been observed that neutrophils may support the metastatic potential of circulating tumor cells (CTCs) in patients with metastatic disease (29).
In BC, the formation of neutrophils extracellular traps (NET), web-like structures formed by DNA and intracellular contents expelled by these cells, has been linked to increased invasiveness and risk of venous thromboembolism (30). The tumor releases pro-inflammatory factors, pro-NETotic factors and extracellular vesicles into the circulation that can activate platelets and the endothelium causing NET release. NETs can capture CTCs, promote the formation of metastases and also the extravasation in the damaged endothelium and generate a highly inflammatory microenvironment for the pre-metastatic niche (Figure 1) (30). NETosis seems to be more frequently produced by morphologically circulating immature neutrophils that express a pro-metastatic behavior, as observed in an in vitro model of BC liver metastasis (31). IL-8 is able to cause neutrophils NETs release and at the same time has an important chemoattractant effect for these cells in the BC microenvironment (32).
NLR is the most widely evaluated inflammatory index. Its elevation is associated with poor prognosis in several cancers and showed to be an independent factor of outcome prediction (9, 33). The prognostic value of the NLR index has been studied in BC (34). A systematic review of fifteen studies analyzing a total of 8563 patients highlighted that a high NLR is associated with a poor OS and DFS in patients with BC especially in triple negative disease and HER-2 positive (HER2+) BC population rather than hormone receptor-positive (HR+) BC patients (35). In a retrospective study, that had the aim to determine the prognostic implications of NLR in the peripheral blood of patients with malignant bone metastasis collected from a prospective cohort, the ratio was significantly associated with tumor type (P<0.0001, included BC) (36).
The combination of NLR/PLR can be considered a more stable marker to changes as compared to single ratios, which may be influenced by concomitant drugs or conditions (e.g. infections or corticosteroids). Combined indexes may reflect also the immune balance and the patients’ immunogenic phenotype as a worse independent prognostic indicator from common prognostic factors such as grading, Ki-67, and molecular subtypes (37). However, conflicting data persist regarding the utility of NLR in predicting prognosis in patients with metastatic disease.
Similarly to NLR and PLR, lymphocyte-to-monocyte ratio (LMR) reflects the imbalance between adaptive and innate immune system in patients with advanced neoplasia and with an inadequate anti-tumor activity (38–40). Lower LMR has been associated with poor survival in BC (41–44). Few studies showed how lymphopenia can be a predictor of poor outcome in aBC patients with increased risk of disease progression and worse long-term survival, assuming a link to a weak anti-tumor response and lower tumor-infiltrating lymphocytes (TILs) (45, 46).
TILs are an emerging tissutal predictive biomarker for BC and their phenotype influences the TME. Infiltration of type 2 (CD4+ T-helper cells or Th2), including Forkhead box P3 (FOXP3) CD4+ regulatory T-cells, inhibits cytotoxic T-cells (CTL) function, supports proliferation and promotes an adaptive anti-inflammatory immune response that is responsible for tumor growth. Especially TNBCs may present a lymphocytic infiltrate >50% and are consequently termed “lymphocyte predominant BCs” (47).
The circulating lymphocyte count and lymphocytes characteristics, especially T-cell receptor diversity, have been investigated, either alone or in combination, as prognostic factors at diagnosis in aBC patients (48). It was observed that the severe restriction of TCR diversity (≤ 33%) was independently associated with shorter OS (48). In addition, the quantitative alteration of lymphocytes in the peripheral blood, and mainly the CD4+ lymphopenia, resulted to be strongly associated with aBC progression (46). In a study from Trédan et al., the cohort of patients with aBC treated in first line showed a median OS of 1.2 months for severe CD4+ lymphopaenic patients, 14.7 months for patients with mild CD4+ lymphopaenia and 24.9 months for non-CD4+ lymphopaenic patients (log rank p-value < 10−4) (46). Importantly, the relative majority of immunosuppressive cytokines (IL-6 and IL-10) and immunosuppressive circulating lymphocytes, like CD8+CD28- suppressor T lymphocytes, in peripheral blood of aBC patients have been associated with a shortened PFS (49).
Immune myeloid cells, such as myeloid-derived suppressor cells (MDSCs), macrophages and monocytes also showed to play a major role in BC. Tumor-induced systemic immune changes might be reflected by some peripheral blood immune cells alterations (50). For example, monocytes are attracted to tumors by many chemokines and motility factors released by the same BC cells, including interferon-γ (INFγ) (51), and lower IFNγ signaling responses in peripheral monocytes tend to correlate to an increased tumor macrophages infiltration.
Circulating monocytes are recruited at the tumor level and induced to differentiate into macrophages that have a central role in the TME. These cells are also directly associated with CTCs in peripheral blood of aBC patients, especially in TNBC (52), and might be involved in guiding CTCs migration in the peripheral circulation to the metastatic niches (Figure 1) (53).
An imbalanced ratio between monocytes and lymphocytes (MLR) underlines the alteration in immune defense against cancer evasion. In a study involving more than 500 patients with aBC, among various immune indexes, only MLR was able to independently predict OS, especially in TNBCs, implying a substantial difference between biological subtypes (52). In the same study, among other predictors of the outcome, CTC (≧̸5 versus <5), metastatic sites, and tumor subtypes (TNBC versus HER2-/ER+ tumors) remained significant. However, several unanswered biological questions remain, such as what determines the tropism of these inflammatory cells or CTCs at a specific metastatic site (e.g. bone) (54) and, in TNBC, which biological characteristic and which different treatment could have a major impact on the metastatic potential of these single cells (55, 56). Another study corroborated these findings showing in the univariate analysis that MLR-high patients with aBC experienced poor prognosis (HR 1.77, 95% CI: 1.24–2.54, p=0.002) (57). MLR was also significantly associated with the extension of the metastatic disease at presentation. The prognostic impact has been also evaluated analyzing the variation of MLR (and also PLR, NLR) during treatment. The reduction or stability of the ratios was associated to better OS (MLR p = 0.028, NLR p = 0.034 and PLR p = 0.003) (57).
The outcome of metastatic BC seems to be also affected by the type of circulating macrophages. Aberrant macrophage polarization has been observed in BC patients. Polarized macrophages are usually classified as M1 or M2 macrophages. M1 subtypes are characterized by intracellular killing and tumor resistance. M2 macrophages instead are associated with immunosuppressive phenotype and are further categorized into other three subtypes: M2a, induced by interleukin-4 (IL-4) or interleukin-13 (IL-13); M2b, induced by immune complexes and agonists of toll-like receptors or interleukin-1 receptors (IL-1R); and M2c, induced by interleukin-10 (IL-10) and glucocorticoid hormones (58). M2a macrophages, differentiated in vitro with IL-4/IL-13, significantly increase the migratory and invasive potential of BC cells compared to M2b or M2c macrophages (59). Some studies observed that the percentages of M2-macrophages are high in BC patients, especially a higher percentage of M2c subtype was observed in patients with advanced disease, highlighting the role of IL-10 in facilitating tumor progression (60). The M2 population has also been associated with clinical parameters such as lymph node metastasis, advanced stages, histological differentiation (p<0.05). The authors also observed that ER negative (ER-) patients show higher levels of M2-like monocytes (61).
The importance of phenotype of circulating monocytes has also been highlighted by high gene expression of MMP-1 and MMP-11 in peripheral mononuclear cells of BC patients correlating to an increased hematogenous diffusion stimulated by interaction with BC cells and cancer associated fibroblasts (CAF) (62).
Analyzing the specific monocyte sub-populations has defined also a link between high levels of systemic CD14+CD16++ monocytes and better OS and PFS in ER-positive and ER-negative BC patients respectively (63). This suggests the potential therapeutic targeting of circulating immune cells.
In TME, tumor-associated macrophages (TAM) are involved in advanced tumor development, progression and dissemination. They contribute to matrix specific formation or degradation and immunosuppression (64). Tumor derived stimuli (anti-inflammatory cytokines IL-4, IL-10, IL-13 and TGF-B), contribute to polarizing TAMs toward an immunosuppressive function as observed in peripheral blood. M2-macrophages increase the expression of specific receptors (some of them are CD68, CD163, CD206, CD204 and macrophage receptor with collagenous structure or MARCO), and the production of VEGF and IL-10, favoring an immunosuppressive environment (64, 65).
Macrophages can also be differently influenced by various breast tumor histotypes due to a specific crosstalk between them and cancer cells. The TNBC-educated macrophages down-regulate citrulline metabolism and differentiate into M2-like macrophages with increased macrophage mannose receptor (MMR) expression, a commonly used marker to define M2 (66). In the TME macrophages enhance the inhibition of T cell response and the recruitment of immunosuppressive leukocytes reducing the tumoricidal function. Macrophages promote angiogenesis (through the secretion of VEGF by perivascular TAMs) and the production of matrix metalloproteinase (MMP) enzymes that remodel the tumor stroma facilitating migration and intravasation (64). In inflammatory BC (IBC), TAMs contribute to its metastatic phenotype, due to a production of cytokines (IL−6, IL−8, and IL−10) that are sufficient to develop the migration effect. In IBCs cells the Ras homology GTPase RhoC is necessary for the enhanced migration response after TAMs signals (67). In general a high infiltration of TAMs is associated with unfavorable features in patients with aBC.
Macrophages also work on the tumor cell seeding of metastatic sites, constituting metastasis associated macrophages (MAMs). Measurements of the monocyte trafficking from TME in a metastatic BC preclinical mouse model showed that MAMs are derived from inflammatory monocytes that are specifically early recruited in the process of pulmonary metastasis, before other immune cells and resident macrophages (68). The recruitment of inflammatory monocytes, which express CCR2 (the receptor for chemokine CCL2), as well as the subsequent recruitment of MAMs, is dependent on CCL2 synthesized by both the tumor and the stroma (69). MAMs are abundant in BC bone metastases (prevalent form of metastasis in BC patients) (70) and derive in large part from recruited inflammatory monocytes. The recruitment of these cells is mostly mediated by the CCL2-CCR2 signaling and CSF1-CSF1 receptor pathways, which are critical for BC metastasis outgrowth and are considered a potential new therapeutic target (71).
The presence of TAMs has been associated with resistance to classical treatments in BC. TAM-mediated chemoresistance has been observed preclinically after paclitaxel infusion. The high recruitment of TAMs due to the CSF1-CSF1R signaling suppresses the mitotic-arrest induced by the taxane (72, 73). It has also been observed resistance to immunotherapy and anti-HER2 agents, especially due to the ability of TAMs to reduce the presence of cytotoxic lymphocytes (74).
Myeloid-derived suppressor cells (MDSCs) are commonly related with tumor progression, angiogenesis and poor prognosis in different cancer types, due to their capacity to elude immune-surveillance. MDSCs are a heterogeneous group of immature myeloid cells (IMCs) with strong immunosuppressive patterns and functions. In physiological conditions, IMCs quickly differentiate into mature leukocytes which play essential roles in host defense against pathogens (75). However, in some conditions such as cancer or inflammation, IMCs fail their normal differentiation and acquire the features of an immature and dysfunctional myeloid population, namely MDSCs with the capacity to suppress cytotoxic T cell responses (76). According to surface antigen expression, MDSCs can be differentiated in granulocytic-MDSCs (G-MDSCs; including neutrophils, eosinophils, basophils, and mast cells) and monocytic-MDSCs (Mo-MDSCs; including monocytes, macrophages, and dendritic cells) (77, 78).
In BC patients, MDSCs seem to be enriched in peripheral blood and can correlate with a poor prognosis, clinical stage and metastatic extension (79, 80). The enrichment of MDSCs is related to an immunoregulatory switch that facilitates the transition to a systemic and more aggressive disease (81). Bergenfeltz et al. observed that an increased level of Mo-MDSCs is detectable in peripheral blood of aBC patients (82). A study by the same authors shows how high levels of Mo-MDSCs are significantly associated with ER- tumors, disease progression, worse progression-free survival, liver and bone metastasis. The inflammatory stimuli, typical of ER- BC (as GM-CSF produced by tumor cells), induces Mo-MDSCs accumulation (83). The same study observed an interesting association between MDSCs and CTCs, supposing a possible clusterization of CTCs with leukocytes including MDSCs capable of enhancing tumoral cells dissemination and metastasization. Besides their known immunosuppressive functions, MDSCs also have direct effects on BC cells contributing to invasiveness and metastasis through the activation of the intracellular phosphatase and tensin homolog (PTEN)/Akt pathway that results in an increased expression of MMP and promotion of invasion and metastasis (75). The phosphoinositide 3-kinase gamma (PI3K γ) signaling plays a crucial role in the activation and migration of myeloid cells, and its expression in MDSCs facilitates tumor growth (84).
The wide involvement of MDSCs, macrophages and monocytes in the mechanisms of BC progression makes them an interesting biomarker to be studied in depth as a potential therapeutic target. Prospective studies are required to define the real effectiveness of circulating inflammatory biomarkers in aBC (Table 1).
In the metastatic setting, more predictive markers for therapeutic efficacy, as well as prognostic biomarkers, are urgently needed.
High NLR, MLR and PLR showed a significant association with shorter progression free survival (PFS) in metastatic ER- BC patients treated with eribulin based regimen hypothesizing that the histological subtype and high NLR (the only independent factors at the final analysis) might be related to low responsiveness to this treatment (85). NLR and PLR are also predictive of benefit from platinum-containing chemotherapy specifically in metastatic TNBC patients. In the study conducted by Vernier et al. patients receiving carboplatin based chemotherapy with higher PLR and NLR experienced a worse PFS compared to ER+/HER2− patients treated with the same regimens (86). These feasible indexes could also be combined with germline or somatic BRCA 1/2 gene mutation and TILs that are actually considered strong predictive and prognostic biomarkers in TNBC (87). Further research is needed to evaluate a potential correlation existing between these biomarkers.
NLR and PLR may also represent a predictive marker for response to endocrine therapy in stage IV BC (12, 88, 89). Lymphocytic indexes have been studied in patients with ER+ aBC in correlation to response to new treatments with contrasting results. To date, Cyclin Dependent Kinase 4 and 6 (CDK 4/6) inhibitors are the first line treatment for this histological subtype associated with aromatase inhibitors or fulvestrant, and preclinical evidence indicates that these new treatments have the ability to stimulate antitumor immunity (90). A retrospective study showed an independent association between high NLR or PLR and lower PFS after three cycles of CDK4/6 inhibitors treatment (p = 0.007 and p = 0.005, respectively) (91). Also Weiner et al. at SABCS 2020 presented a study where PLR at baseline resulted to be associated with worse PFS of patients treated with first line CDK4/6 inhibitors (92). The same association between NLR and PFS has been observed in a retrospective study involving patients treated with everolimus-based treatments (p=0.01) (12).
The impact of lymphocytic indexes was also evaluated in HER2+ aBC patients receiving dual anti-HER2 blockade. In a cohort of 57 patients only the Pan-Immune-Inflammatory Value (PIV), (defined as the product of peripheral blood neutrophil, platelet, and monocyte counts divided by lymphocyte counts) was statistically significantly associated with worse OS at multivariable analysis (93). In the same population, the single indexes (MLR, NLR, and PLR) did not demonstrate a significant association to prognosis, but correlated with worse outcomes. The effects of these monoclonal antibodies might be mediated by systemic peripheral inflammatory cells, especially circulating lymphocytes, in association to TILs present in the TME (94).
There is an urgent need to identify effective biomarkers for predicting survival benefits from ICIs in patients with TNBC after the demonstration of the efficacy of atezolizumab and pembrolizumab in this category of patients (95). The 20% of this BC histological subtype expresses Programmed cell Death protein-1 (PD-1), an immune checkpoint receptor that limits T-cell effectors function within tissues interacting with its specific ligand PD-L1 (96). PD-L1 is expressed on the membrane of BC cells and recognized by the specific receptor on CD8 + T cells. Immune checkpoint inhibitors (ICIs) may influence systemic inflammation in patients and in conditions of low lymphocyte counts the efficacy of these drugs may be invalidated (97). Studies among various malignancies (including aBC) demonstrated that higher NLR is significantly associated with poorer OS and PFS, lower rates of response and clinical benefit (26). The combination of NLR and tumor mutational burden (TMB) increased the capacity of predicting the outcome after an ICIs treatment; indeed, the category of patients with NLR-low/TMB-high showed higher response rate (26).
Although the increasing evidence available suggests a relationship between lymphocytic ratios and prognosis in aBC (Table 2) several issues persist about the feasible clinical application. First of all there is lack of consensus regarding a shared cut-off value, secondarily the sensibility and specificity of these ratios varies among different studies and almost the totality of the studies are retrospective. Finally large prospective studies with a rigorous methodology are mandatory to determine the real clinical value and applicability of inflammatory indexes.
Table 2 Prognostic role of circulating biomarkers in response to treatments in aBC, available results from retrospective analysis.
Circulating inflammatory cells are considered a useful target in the therapeutic strategy for aBC due to their pro-tumor involvement. However, the delicate balance between the tumor-inhibitory and tumor-promoting properties of immune cells implies the need for adequately targeted therapeutic approaches.
The clinical benefit of targeting tumor-cell platelets interaction in aBC is still under question. Many studies support the idea of utilizing targeted platelet therapies to inhibit the platelet’s role in the malignancy. Platelets exposed to tamoxifen or ticagrelor release significantly lower amounts of pro-angiogenic VEGF and have less interaction with BC cells (98, 99). However the concomitant use of anti-platelet therapy in cancer patients has a rationale but carries many risks as the declining platelet function and counts as a consequence of disease progression or myelosuppressive effects of treatments.
As previously reported, TANs are particularly involved in tumor progression and studies on new drugs are evaluating therapeutic strategies on several fronts: inhibition of neutrophils recruitment in tumors, depletion of neutrophils in TME, targeting tumor-promoting TAN polarization (100). Targeting neutrophils as a treatment option has been investigated in many preclinical models with discouraging results due to the short life span of these cells (nearly 24 h in blood) (101). Low toxicities strategies to inhibit protumor neutrophils are warranted and expected as promising approaches. Treatments that target the mechanism of interaction between tumor cells and neutrophils are more encouraging.
INF-β and TGF-β are cytokines with a role in switching neutrophils polarization from N1 to N2. In a BC mouse model the blockade of TGF-β increased the percentage of N1 and the activity of CD8+ T cells (102). A phase I trial enrolling patients with solid tumors (including BC patients) has the objective to evaluate the efficacy of a selective and orally active TGF-β receptor 1 inhibitor (NCT03685591). This new TGF-β receptor 1 inhibitor combined with palbociclib in a xenograft BC model led to a significant increase in OS, suggesting the potential for such combination (103).
Neutrophils are considered the major productors of pro-angiogenic factors and the presence of a rich neutrophils infiltrate in TME has been associated with resistance to anti-VEGF therapies (104). Tumors enriched in neutrophils are also more likely resistant to ICIs. Consequently, there are many ongoing studies (phase I/II) evaluating the association between ICIs and new compounds against neutrophils in solid tumors, but results are still awaited (Table 3) (104). Chemokines and interleukins involved in the TAN recruitment (like CXCL1, 2, 5, 6, 8, IL-6, IL17) and their signaling are possible new targets for inhibitory drugs associated with ICIs enhancing their activity (105). The inhibition of enzymes involved in the protumor phenotype as nicotinamide phosphoribosyl transferase (NAMPT) or CXCR2 signaling resulted in an effective reduction of tumor growth and polarization to N1 (106).
Targeting MDSCs may also become a potential strategy to enhance antitumor activity of current treatments (Table 3). Entinostat, a selective HDAC1/3 inhibitor, can decrease the populations of MDSCs and FOXP3+ Tregs in murine models of mammary carcinoma (107). Combination of entinostat with nivolumab and ipilimumab is currently under evaluation in a phase I trial in patients with invasive and metastatic BC (NCT02453620) (Table 1). Entinostat showed promising preclinical and clinical data in HR+ endocrine-resistant BC. G-MDSCs and Mo-MDSCs manifested a reduction (14.67 vs +20.56%; p = 0.03 and -62.3 vs +1.97%; p = 0.002 respectively) in a post hoc analysis of samples from entinostat treated patients in ENCORE301 trial (108). CD40 was also significantly downregulated in the majority of MDSC subsets (109). Other drugs like IPI-549 (eganelisib) transported by liposomes, can inhibit PI3Kγ in MDSCs, resulting in downregulation of arginase 1 (Arg-1) that conduces to MDSCs apoptosis and reduction of their immunosuppressive activity to CD8+ T cells. This strategy synergizes with ICIs and inhibits tumor growth via facilitating the dendritic cell maturation and tumor infiltration of CD8+ T cells while decreasing the tumor infiltration of immunosuppressive regulatory T cells, MDSCs, and M2-like TAMs in solid tumors (81). Some trials targeting PI3Kγ involving BC patients are currently ongoing (Table 3).
Sitravatinib is an oral spectrum-selective tyrosine kinase inhibitor that targets the TYRO3/AXL/MERTK pathways and split the VEGFR2/KIT family receptor tyrosine kinases (RTKs). Inhibition of this pathway may promote the depletion of MDSCs in the TME and at the same time repolarize TAMs towards the M1 phenotype (110). The NCT04123704 trial is evaluating sitravatinib in aBC.
The myeloid lineage in solid tumors can also be targeted by MTL-CEBPA, a novel immunotherapy constituted by a small activating RNA (saRNA) that upregulates C/EBPα, a master regulator of myeloid cell differentiation with anticancer properties. Furthermore, this saRNA restores CEBPA gene transcription, and increases both CEBPA mRNA levels and protein expression at tumor cell level activating the expression of suppressor genes that are downregulated in certain types of cancer. MTL-CEBPA has been evaluated in a phase I trial including BC patients (111). The NCT04105335 trial is now recruiting patients with solid tumors and evaluating MTL-CEBPA in combination with pembrolizumab.
MDSCs are also depleted by other new drugs like ORIN1001 that targets and binds to the RNase domain of the Inositol-requiring enzyme 1 (IRE1) involved in stress adaptation mechanisms in tumoral cells and TME (112).
Macrophage-targeted treatment strategies instead are showing more promising results and are currently being evaluated in many clinical trials. These strategies include: inhibition of macrophage and macrophage precursors recruitment, depletion of TAMs, repolarization of TAMs to an antitumor phenotype, inhibition of tumorigenic factors and mechanisms promoted by TAM and enhancement of macrophage-mediated tumor cell killing or phagocytosis (Figure 2).
Figure 2 Macrophage-targeted treatment strategies on study. Macrophage-targeted treatment strategies include: inhibition of macrophage and macrophage precursors recruitment targeting the CSF1-CSFR and CCL2-CCR2 pathways, depletion of tumor associated macrophages (TAMs) (like biphosphonates), repolarization of TAMs to an antitumor phenotype, inhibition of tumorigenic factors and mechanisms promoted by TAM and enhancement of macrophage-mediated tumor cell killing or phagocytosis. The repolarization of TAMs is mediated by stimulating the costimulatory receptor CD40, Toll-like receptor 7 (TLR7) or administrating anti-CD47 drugs. Anti CR3 factors enhance the innate activity of macrophages, favoring the antitumoral phenotypes. Ang2 and the respective receptor TIE2 constitute another druggable pathway favoring antitumor responses and inhibiting the functions of TAMs.
The disruption of macrophage recruitment is currently being exploited targeting the CSF1-CSFR and CCL2-CCR2 pathways with specific antibodies (Table 4). The CCL2 blockade showed to sequester monocytes in the bone marrow, instead the inhibition of CSF1 signaling can reduce monocyte development (64, 113). Trials with novel CSF1 inhibitors showed contrasting results. The NCT01596751 trial evaluated the tolerability of PLX3397 (pexidartinib), an anti CSF1, associated with eribulin on 67 aBC patients (phase I part) and then the effect on PFS in a TNBC cohort (phase II part), but results are still awaited. Pexidartinib showed tumor response associated with paclitaxel in the BC patient group of the NCT01525602 trial (114). Conversely, lacnotuzumab, another anti CSF1, when combined to carboplatin-gemcitabine did not show a greater antitumor activity with a worse tolerability profile (115). Emactuzumab, in phase I trials including advanced BC patients, showed a specific reduction of immunosuppressive TAMs, but did not result in clinically relevant antitumor activity (116, 117). In contrast, in the NCT02265536 trial a meaningful stable disease >9 months in two patients with aBC was obtained with LY3022855 (118). However, the interruption of these treatments seems to induce a rebound effect, with abnormal elevated circulating monocytes or accelerated metastases (119).
Table 4 Clinical trials enrolling breast cancer patients involving new treatments targeting macrophages.
Other treatment options that can deplete TAMs are potentially constituted by antibodies targeting antigens expressed by TAMs such as the scavenger receptor A, CD52 and folate receptor β (120, 121). However, these targets have not been studied in breast cancer models.
Bisphosphonates are also under evaluation for their capacity to induce apoptosis in monocytic cells (122). They significantly reduce complications of breast cancer bone metastasis by inhibiting resident macrophages or osteoclasts, and recent clinical trials indicate additional anti-metastatic effects outside the bone microenvironment (123). In vitro, bisphosphonates cause increased macrophage death whereas in vivo inhibit the production of pro-angiogenic factors, such as MMP-9, other evidence suggests a shifting in TAMs to a pro-tumoricidal phenotype (122).
TAMs reprogramming to a M1 phenotype can be achieved by stimulating the costimulatory receptor CD40, complement receptor 3 (CR3), administrating Toll-like receptor 7 (TLR7) and 8 agonists, inhibiting IL10 or delivering IL-12 (124). For example imiquimod, a TLR agonist, can induce the production of proinflammatory cytokines by macrophages, therefore restoring the ability to attack BC cells (125). The topical application of imiquimod showed to reduce skin metastasis in aBC patients in association to nab-paclitaxel, but responses were fleeting (126). The subcutaneous administration of a TLR agonist has been experimented in a phase II trial involving also heavily pretreated aBC patients, showing modest results and a considerable risk of cardiac toxicity (127).
Other strategies that stimulate TAMs include agonistic anti-CD40 or inhibitory anti-CD47 antibodies. The co-stimulatory receptor CD40 is expressed on macrophages and usually binds the CD154 on T cells. The agonist action of specific antibodies can reverse immune suppression and drive antitumor T cell responses (128). A first-in-human study completed in 2017 showed that the injection of a CD40 agonist antibody into superficial lesions was well tolerated and associated with pharmacodynamic responses (129). Selicrelumab, a fully human CD40 agonist, is being experimented in some phase I trials including BC patients, both alone and in association to other drugs as vanacizumab, a bispecific antibody directed to Angiopoietin 2 (Ang2) and VEGF-A (NCT02665416). Other studies are evaluating the effect of this agonist especially in TNBC, for example in association to a FMS-like tyrosine kinase 3 ligand (FLT3) inhibitor or classical chemo/immunotherapy treatments (Table 4). New biotechnologies targeting CD40 are also in study, such as oncolytic adenoviral vectors or designed ankyrin repeat proteins (DARPins), which are genetically engineered antibody mimetic proteins typically exhibiting a highly specific and high-affinity target protein binding. MP0317 is a DARPin intravenously administered drug targeting fibroblast activation protein (FAP) and CD40 that is currently being evaluated in a phase I trial including also aBC patients (NCT05098405).
Regarding CD47, blocking the interaction between it and the signal-regulatory protein alpha (SIRP-alfa), a “don’t eat me” signal can re-activate the phagocytic activity of TAMs (130). Many tumors overexpress CD47, enabling immune escape from the innate immune system such as macrophages binding SIRPα and compromising the antigen presentation and T cell infiltration (131). Targeting CD47 can also enhance the anti-tumor effect of other therapeutic strategies. The combination of anti-CD47 with trastuzumab, significantly suppressed the growth of antibody-dependent cellular cytotoxicity (ADCC)-tolerant HER2+ BC via Fc-dependent antibody-dependent cellular phagocytosis (ADCP) (132). The ASPEN-01 open-label, multicentre, phase I dose-escalation and dose-expansion study, evaluated the association of evorpacept (an anti-CD47) plus either intravenous pembrolizumab or trastuzumab. The safety findings support the use of evorpacept and preliminary data on the antitumor activity suggest future investigation. However, this trial included only one patient with aBC (133). Many other phase I and II trials are ongoing, evaluating the CD47 blocking effect on solid tumors also in aBC patients (Table 4). The tolerability of anti-CD47 has been successfully evaluated in a trial including five aBC patients (134).
The CD47-SIRPα axis may also be targeted using the SIRPα factor. TTI-621 (SIRPαFc) is a soluble recombinant fusion protein that acts by binding human CD47 evaluated in the. NCT02663518 trial in various solid tumors.
The macrophage-1 antigen, also called CR3, is a complement receptor consisting of CD11b (integrin αM) and CD18 (integrin β2). CR3 is a pattern recognition receptor, capable of recognizing and binding to many molecules found on the surfaces of foreign cells enabling phagocytosis (135). The clinical trial NCT02981303 have evaluated the capacity of a new molecule constituted by a pathogen-associated molecular pattern (PAMP) to enhance innate immune cell killing and the maturation of antigen presenting cells when combined to ICIs in TNBC, meeting both safety and efficacy requirements (136). CR3 is the principal β2 integrin known to contribute to PAMPs recognition (137).
There is also evidence that targeting the Ang2-TIE2 may inhibit the functions of TIE2-expressing macrophages, a TAM subset endowed with proangiogenic activity in mouse tumor models (138). Ang2 is a ligand of the TIE2 receptor and modulates endothelial cell biology facilitating angiogenesis. Ang2 inhibition, by monoclonal antibodies, peptibodies, or CovX-Bodies, may determine antitumor responses and also inhibit the functions of TAMs (139). In BC the expression of Ang2 is correlated to more aggressiveness. The intravasation occurs in sites where a TIE2-expressing macrophage and an endothelial cell are in direct contact. Ablation of the activity of these macrophages blocks intravasation after Ang2-TIE2 axis inhibition (140).
Trebananib, a peptibody that inhibits the binding of angiopoietin 1 and 2 to TIE2 showed potential anticancer effect in a phase Ib and phase II studies, with manageable AEs (141, 142). Vanucizumab is another novel bispecific antibody inhibiting VEGF-A and Ang2 that demonstrated safety and anti-tumor activity in a phase I study of 42 patients with advanced solid tumors (143). Other two trials have evaluated the tolerability of nesvacumab (an antiAng2 antibody) in advanced neoplasms (NCT01688960, NCT01271972) showing a preliminary antitumor activity (144). Rebastinib, instead, is a TIE2 inhibitor that blocks the assembly of macrophages and endothelial cells involved in metastasization at the peripheral site (tumor microenvironment of metastasis) (145). The NCT02824575 trial hypothesizes that rebastinib combined with antitubulin therapy could improve clinical outcomes in BC by preventing intravasation. The nonsteroidal anti-inflammatory drug celecoxib showed an interesting activity in BC increasing the presence of M1 like macrophages, but the real effect is in doubt (146, 147). In this perspective, the NCT00075673 trial has evaluated the weekly administration of oral vinorelbine in combination with celecoxib in aBC. Results are still awaited.
In view of the central role of innate and adaptive immune systems in cancer development, immune cells are not only considered potential therapeutic targets, but also innovative vehicles for treatments. The genetic engineering to deliver, correct or enhance immune cells demonstrated to be successful. Chimeric antigen receptor (CAR) T-cell treatment has provided notable results in hematological tumors (148). Unfortunately, the same evidence has not been demonstrated in solid tumor, where T-cells encounter substantial difficulties in penetrating and surviving in the TME (149, 150). The extracellular matrix (ECM) is one of the major parts of TME, and it is a physical barrier to various kinds of anticancer therapies. MMPs can degrade almost all ECM components, and macrophages are an important source of MMPs (151). Amongst the cell types used in engineered cell immunotherapies, macrophages have recently emerged as prominent candidates for the treatment of solid tumors, including BC (Figure 3) (152). In a preclinical study, macrophages engineered with specific CARs (CAR-M), activated after the detection of the HER2 antigen on tumor cell surface (153). The activation of these engineered macrophages triggered by the internal signaling of CD147 determines the production of MMPs. The infusion of CAR-147 macrophages reduces the tumor collagen deposition (153). The initial in vitro tests failed to show strong antitumor activity of the CAR-147, however infusion of CAR-147 cells into the aggressive HER2-4T1 bearing mouse model showed significant tumor growth inhibition (153).
Figure 3 CAR-M activity in breast cancer. (A) Macrophages modified with Chimeric antigen receptor (CAR-M) present an improved phagocytic activity and antigen presentation capacity against tumors. CAR-M therapy is developed by the transfer of an edited specific CAR gene into macrophages withdrawn from patient peripheric blood. (B) These genetically modified cells are then more effective in binding to the tumor cell surface via specific antigen identification and active against tumor cells when reinfused into the patient. Furthermore CAR-M are able to produce metalloproteinases (MMPs) that can degrade part of the extracellular matrix (ECM) components in the tumor stroma. This activity facilitate penetration of anti-tumor immune cells into the tumor. Adapted from “Car T Cell Therapy Overview”, by BioRender.com (2022). Retrieved from https://app.biorender.com/biorender-templates.
One first in human phase I trial is currently active and recruiting patients with HER2+ advanced solid tumors experimenting with engineered CAR-M (NCT04660929). Another active protocol has the objective to collect tumor samples to develop patients’ derived organoids from HER2-, HER2-low and HER2+ BCs to test the antitumor activity of newly developed CAR-M (NCT05007379).
In vitro studies showed that CAR-M infused in tumor models increased intratumoral T-cell infiltration, NK cell infiltration, dendritic cell infiltration/activation, and TILs activation and at the same time can reduce tumor growth (152).
The combination of CAR-M treatment with other anti-tumor therapies such as CAR-T cells, ICIs and chemotherapy may synergize and provide an optimal tumor control. Nevertheless, toxicity remains an important concern and further optimization of CAR products is required (150).
Finally, preliminary outcomes about the use of mesenchimal stem cells (MSCs) in BC are interesting. Genetically modified MSCs with the insertion of tumor suppressor genes, proapoptotic genes, immune involved genes can inhibit cancer cell growth. Moreover modified MSCs delivering anticancer agents into tumor tissue have been studied in several cancer types, results in BC are awaited (154).
Definitely the immune system has a very important role in cancer biology and must be taken into account when trying to understand the complexity of tumor behavior.
Increasing evidence suggests a close relationship in particular between neutrophils and macrophages with BC treatment, prognosis and outcome. Lymphocytic indexes are attractive as new potential prognostic and predictive factors for aBC treatment, mainly because they are easily detectable and applicable in daily clinical practice. Wider prospective studies are needed to unveil their real effectiveness.
The clinical efficacy of targeting immune cells (especially macrophages) in BC still needs to get official validation, but preclinical results are encouraging. Drug combination strategies seem to be the most appropriate to reduce the immunosuppressive action of immune cells in TME (155–157). The association of new compounds to classical chemotherapy, anti-HER2 agents or ICIs is currently tested in the majority of ongoing clinical trials (Tables 3, 4). Combination approaches may overcome resistance mechanisms. Ongoing trials’ results are eagerly awaited to refine the optimal timing and better define treatment sequentiality to maximize therapeutic benefit.
Conceptualization, CG and UD. Methodology, CG, UD and FS. Validation, UD and FS. Writing—original draft preparation, CG. Writing—review and editing, UD and FS. Supervision, UD, MP, GS, FM, CC, SB, MS, GD, SS and LC. All authors contributed to the article and approved the submitted version.
This work was partly supported thanks to the contribution of Ricerca Corrente by the Italian Ministry of Health within the research line L2 (Innovative therapies, phase I-III clinical trials).
This research received no external funding. MP has received advisory board fees from Novartis. UD has received advisory board or consultant fees from Merck Sharp and Dohme, Bristol Myers Squibb, Janssen, Astellas, Sanofi, Bayer, Pfizer, Ipsen, Novartis, and Pharmamar and institutional research grants from Astrazeneca, Sanofi, and Roche.
The remaining authors declare that the research was conducted in the absence of any commercial or financial relationships that could be construed as a potential conflict of interest.
All claims expressed in this article are solely those of the authors and do not necessarily represent those of their affiliated organizations, or those of the publisher, the editors and the reviewers. Any product that may be evaluated in this article, or claim that may be made by its manufacturer, is not guaranteed or endorsed by the publisher.
1. De Visser KE, Eichten A, Coussens LM. Paradoxical roles of the immune system during cancer development. Nat Rev Cancer (2006) 6(1):24–37. doi: 10.1038/NRC1782
2. Hanahan D, Weinberg RA. The hallmarks of cancer. Cell (2000) 100(1):57–70. doi: 10.1016/S0092-8674(00)81683-9
3. Mantovani A, Allavena P, Sica A, Balkwill F. Cancer-related inflammation. Nat (2008) 454(7203):436–44. doi: 10.1038/nature07205
4. Diakos CI, Charles KA, McMillan DC, Clarke SJ. Cancer-related inflammation and treatment effectiveness. Lancet Oncol (2014) 15(11):e493–503. doi: 10.1016/S1470-2045(14)70263-3
5. Mego M, Gao H, Cohen EN, Anfossi S, Giordano A, Sanda T, et al. Circulating tumor cells (CTC) are associated with defects in adaptive immunity in patients with inflammatory breast cancer. J Cancer (2016) 7(9):1095–104. doi: 10.7150/jca.13098
6. Koh CH, Bhoo-Pathy N, Ng KL, Jabir R S, Tan G-H, See M-H, et al. Utility of pre-treatment neutrophil-lymphocyte ratio and platelet-lymphocyte ratio as prognostic factors in breast cancer. Br J Cancer (2015) 113(1):150–8. doi: 10.1038/BJC.2015.183
7. Cho U, Park HS, Im SY, Yoo CY, Jung JH, Suh YJ, et al. Prognostic value of systemic inflammatory markers and development of a nomogram in breast cancer. PloS One (2018) 13(7):e0200936. doi: 10.1371/journal.pone.0200936
8. Dolan RD, McSorley ST, Horgan PG, Laird B, McMillan DC. The role of the systemic inflammatory response in predicting outcomes in patients with advanced inoperable cancer: Systematic review and meta-analysis. Crit Rev Oncol Hematol (2017) 116:134–46. doi: 10.1016/j.critrevonc.2017.06.002
9. Templeton AJ, Ace O, McNamara MG, Vera-Badillo FE, Hermanns T, Seruga B., et al. Prognostic role of platelet to lymphocyte ratio in solid tumors: a systematic review and meta-analysis. Cancer Epidemiol Biomarkers Prev (2014) 23(7):1204–12. doi: 10.1158/1055-9965.EPI-14-0146
10. Teng JJ, Zhang J, Zhang TY, Zhang S, Li BS. Prognostic value of peripheral blood lymphocyte-to-monocyte ratio in patients with solid tumors: a meta-analysis. Onco Targets Ther (2015) 9:37–47. doi: 10.2147/OTT.S94458
11. Zheng K, Liu X, Ji W, Lu J, Cui J, Li W. The efficacy of different inflammatory markers for the prognosis of patients with malignant tumors. J Inflammation Res (2021) 14:5769–85. doi: 10.2147/jir.s334941
12. Schettini F, Sobhani N, Ianza A, Triulzi T, Molteni A, Lazzari MC, et al. Immune system and angiogenesis-related potential surrogate biomarkers of response to everolimus-based treatment in hormone receptor-positive breast cancer: an exploratory study. Breast Cancer Res Treat (2020) 184(2):421–31. doi: 10.1007/s10549-020-05856-3
13. Stoiber D, Assinger A. Platelet-leukocyte interplay in cancer development and progression. Cells (2020) 9(4):855. doi: 10.3390/CELLS9040855
14. Leblanc R, Lee SC, David M, Bordet JC, Norman DD, Patil R, et al. Interaction of platelet-derived autotaxin with tumor integrin αVβ3 controls metastasis of breast cancer cells to bone. Blood (2014) 124(20):3141–50. doi: 10.1182/blood-2014-04-568683
15. Kang DW, Min DS. Platelet derived growth factor increases phospholipase D1 but not phospholipase D2 expression via NFkappaB signaling pathway and enhances invasion of breast cancer cells. Cancer Lett (2010) 294(1):125–33. doi: 10.1016/j.canlet.2010.01.031
16. Zuo XX, Yang Y, Zhang Y, Zhang ZG, Wang XF, Shi YG. Platelets promote breast cancer cell MCF-7 metastasis by direct interaction: surface integrin α2β1-contacting-mediated activation of wnt-β-catenin pathway. Cell Commun Signal (2019) 17(1):142. doi: 10.1186/S12964-019-0464-X
17. Lal I, Dittus K, Holmes CE. Platelets, coagulation and fibrinolysis in breast cancer progression. Breast Cancer Res (2013) 15(4):207. doi: 10.1186/BCR3425
18. Elaskalani O, Berndt MC, Falasca M, Metharom P. Targeting platelets for the treatment of cancer. Cancers (Basel) (2017) 9(7):94. doi: 10.3390/cancers9070094
19. Felding-Habermann B. Targeting tumor cell-platelet interaction in breast cancer metastasis. Pathophysiol Haemost Thromb (2003) 33(Suppl. 1):S56–58. doi: 10.1159/000073295
20. Johnson KE, Ceglowski JR, Roweth HG, Forward JA, Tippy MD, El-Husayni S, et al. Aspirin inhibits platelets from reprogramming breast tumor cells and promoting metastasis. Blood Adv (2019) 3(2):198–211. doi: 10.1182/bloodadvances.2018026161
21. Boucharaba A, Serre C-M, Grès S, Saulnier-Blache JS, Bordet JC, et al. Platelet-derived lysophosphatidic acid supports the progression of osteolytic bone metastases in breast cancer. J Clin Invest (2004) 114(12):1714–25. doi: 10.1172/JCI22123
22. Guo W, Lu X, Liu Q, Zhang T, Li P, Qiao W, et al. Prognostic value of neutrophil-to-lymphocyte ratio and platelet-to-lymphocyte ratio for breast cancer patients: An updated meta-analysis of 17079 individuals. Cancer Med (2019) 8(9):4135–48. doi: 10.1002/CAM4.2281
23. Coffelt SB, Wellenstein MD, De Visser KE. Neutrophils in cancer: neutral no more. Nat Rev Cancer (2016) 16(7):431–46. doi: 10.1038/NRC.2016.52
24. Patel S, Fu S, Mastio J, Dominguez GA, Purohit A, Kossenkov A, et al. Unique pattern of neutrophil migration and function during tumor progression. Nat Immunol (2018) 19(11):1236–47. doi: 10.1038/S41590-018-0229-5
25. Tüting T, De Visser KE. CANCER. How neutrophils promote metastasis Sci (2016) 352(6282):145–6. doi: 10.1126/science.aaf7300
26. Valero C, Lee M, Hoen D, Weiss K, Kelly DW, Adusumilli PS, et al. Pretreatment neutrophil-to-lymphocyte ratio and mutational burden as biomarkers of tumor response to immune checkpoint inhibitors. Nat Commun (2021) 12(1):729. doi: 10.1038/S41467-021-20935-9
27. Wculek SK, Malanchi I. Neutrophils support lung colonization of metastasis-initiating breast cancer cells. Nat (2015) 528(7582):413–7. doi: 10.1038/nature16140
28. Coffelt SB, Kersten K, Doornebal CW, Weiden J, Vrijland K, Hau CS, et al. IL-17-producing γδ T cells and neutrophils conspire to promote breast cancer metastasis. Nat (2015) 522(7556):345–8. doi: 10.1038/nature14282
29. Szczerba BM, Castro-Giner F, Vetter M, Krol I, Gkountela S, Landin J, et al. Neutrophils escort circulating tumour cells to enable cell cycle progression. Nat (2019) 566(7745):553–7. doi: 10.1038/S41586-019-0915-Y
30. Snoderly HT, Boone BA, Bennewitz MF. Neutrophil extracellular traps in breast cancer and beyond: current perspectives on NET stimuli, thrombosis and metastasis, and clinical utility for diagnosis and treatment. Breast Cancer Res (2019) 21(1):145. doi: 10.1186/S13058-019-1237-6
31. Hsu BE, Tabariès S, Johnson RM, Andrzejewski S, Senecal J, Lehuédé C, et al. Immature low-density neutrophils exhibit metabolic flexibility that facilitates breast cancer liver metastasis. Cell Rep (2019) 27(13):3902–15.e6. doi: 10.1016/j.celrep.2019.05.091
32. Disis ML, Stanton SE. Immunotherapy in breast cancer: An introduction. Breast (2018) 37:196–9. doi: 10.1016/J.breast.2017.01.013
33. Hirahara T, Arigami T, Yanagita S, Matsushita D, Uchikado Y, Kita Y, et al. Combined neutrophil-lymphocyte ratio and platelet-lymphocyte ratio predicts chemotherapy response and prognosis in patients with advanced gastric cancer. BMC Cancer (2019) 19(1):672. doi: 10.1186/S12885-019-5903-Y
34. Wei B, Yao M, Xing C, Wang W, Yao J, Hong Y, et al. The neutrophil lymphocyte ratio is associated with breast cancer prognosis: an updated systematic review and meta-analysis. Onco Targets Ther (2016) 9:5567–75. doi: 10.2147/OTT.S108419
35. Ethier JL, Desautels D, Templeton A, Shah PS, Amir E. Prognostic role of neutrophil-to-lymphocyte ratio in breast cancer: a systematic review and meta-analysis. Breast Cancer Res (2017) 19(1):2. doi: 10.1186/S13058-016-0794-1
36. Shouyu W, Zhen Z, Fang F, Xue G, Wei S, Huanran L. The neutrophil/lymphocyte ratio is an independent prognostic indicator in patients with bone metastasis. Oncol Lett (2011) 2(4):735–40. doi: 10.3892/OL.2011.304
37. Graziano V, Grassadonia A, Iezzi L, Vici P, Pizzuti L, Barba M, et al. Combination of peripheral neutrophil-to-lymphocyte ratio and platelet-to-lymphocyte ratio is predictive of pathological complete response after neoadjuvant chemotherapy in breast cancer patients. Breast (2019) 44:33–8. doi: 10.1016/j.breast.2018.12.014
38. Gu L, Li H, Chen L, Ma X, Li X, Gao Y, et al. Prognostic role of lymphocyte to monocyte ratio for patients with cancer: evidence from a systematic review and meta-analysis. Oncotarget (2016) 7(22):31926–42. doi: 10.18632/oncotarget.7876
39. Nishijima TF, Muss HB, Shachar SS, Tamura K, Takamatsu Y. Prognostic value of lymphocyte-to-monocyte ratio in patients with solid tumors: A systematic review and meta-analysis. Cancer Treat Rev (2015) 41(10):971–8. doi: 10.1016/J.CTRV.2015.10.003
40. Li W, Ma G, Wu Q, Deng Y, Liu Y, Wang J. Prognostic value of lymphocyte-to-monocyte ratio among Asian lung cancer patients: a systematic review and meta-analysis. Oncotarget (2017) 8(66):110606–13. doi: 10.18632/ONCOTARGET.20574
41. Goto W, Kashiwagi S, Asano Y, Takada K, Takahashi K, Hatano T, et al. Predictive value of lymphocyte-to-monocyte ratio in the preoperative setting for progression of patients with breast cancer. BMC Cancer (2018) 18(1):1137. doi: 10.1186/S12885-018-5051-9
42. Marín Hernández C, Piñero Madrona A, Gil Vázquez PJ, Galindo Fernández PJ, Ruiz Merino G, Alonso Romero JL, et al. Usefulness of lymphocyte-to-monocyte, neutrophil-to-monocyte and neutrophil-to-lymphocyte ratios as prognostic markers in breast cancer patients treated with neoadjuvant chemotherapy. Clin Transl Oncol (2018) 20(4):476–83. doi: 10.1007/S12094-017-1732-0
43. Ji H, Xuan Q, Yan C, Liu T, Nanding A, Zhang Q. The prognostic and predictive value of the lymphocyte to monocyte ratio in luminal-type breast cancer patients treated with CEF chemotherapy. Oncotarget (2016) 7(23):34881–9. doi: 10.18632/ONCOTARGET.8993
44. Ni XJ, Zhang XL, Ou-Yang QW, Qian GW, Wang L, Chen S, et al. An elevated peripheral blood lymphocyte-to-monocyte ratio predicts favorable response and prognosis in locally advanced breast cancer following neoadjuvant chemotherapy. PloS One (2014) 9(11):e111886. doi: 10.1371/journal.pone.0111886
45. Che YQ, Zhang Y, Wang D, Liu HY, Shen D, Luo Y. Baseline lymphopenia: A predictor of poor outcomes in HER2 positive metastatic breast cancer treated with trastuzumab. Drug Des Devel Ther (2019) 13:3727–34. doi: 10.2147/DDDT.S212610
46. Trédan O, Manuel M, Clapisson G, Bachelot T, Chabaud S, Bardin-dit-Courageot C, et al. Patients with metastatic breast cancer leading to CD4+ T cell lymphopaenia have poor outcome. Eur J Cancer (2013) 49(7):1673–82. doi: 10.1016/J.EJCA.2012.11.028
47. Salgado R, Denkert C, Demaria S, Sirtaine N, Klauschen F, Pruneri G, et al. The evaluation of tumor-infiltrating lymphocytes (TILs) in breast cancer: recommendations by an international TILs working group 2014. Ann Oncol Off J Eur Soc Med Oncol (2015) 26(2):259–71. doi: 10.1093/annonc/mdu450
48. Manuel M, Trédan O, Bachelot T, Clapisson G, Courtier A, Parmentier G, et al. Lymphopenia combined with low TCR diversity (divpenia) predicts poor overall survival in metastatic breast cancer patients. Oncoimmunology (2012) 1(4):432–40. doi: 10.4161/ONCI.19545
49. Song G, Wang X, Jia J, Yuan Y, Wan F, Zhou X, et al. Elevated level of peripheral CD8(+)CD28(-) T lymphocytes are an independent predictor of progression-free survival in patients with metastatic breast cancer during the course of chemotherapy. Cancer Immunol Immunother (2013) 62(6):1123–30. doi: 10.1007/S00262-013-1424-8
50. Hiam-Galvez KJ, Allen BM, Spitzer MH. Systemic immunity in cancer. Nat Rev Cancer (2021) 21(6):345–59. doi: 10.1038/S41568-021-00347-Z
51. Wang L, Simons DL, Lu X, Tu TY, Avalos C, Chang AY, et al. Breast cancer induces systemic immune changes on cytokine signaling in peripheral blood monocytes and lymphocytes. EBioMedicine (2020) 52:102631. doi: 10.1016/J.EBIOM.2020.102631
52. De Giorgi U, Mego M, Scarpi E, Giordano A, Giuliano M, Valero V, et al. Association between circulating tumor cells and peripheral blood monocytes in metastatic breast cancer. Ther Adv Med Oncol (2019) 11:1758835919866065. doi: 10.1177/1758835919866065
53. Adams DL, Martin SS, Alpaugh RK, Charpentier M, Tsai S, Bergan RC, et al. Circulating giant macrophages as a potential biomarker of solid tumors. Proc Natl Acad Sci USA (2014) 111(9):3514–9. doi: 10.1073/pnas.1320198111
54. De Giorgi U, Mego M, Rohren EM, Liu P, Handy BC, Reuben JM, et al. 18F-FDG PET/CT findings and circulating tumor cell counts in the monitoring of systemic therapies for bone metastases from breast cancer. J Nucl Med (2010) 51(8):1213–8. doi: 10.2967/jnumed.110.076455
55. Pietri E, Conteduca V, Andreis D, Massa I, Melegari E, Sarti S, et al. Androgen receptor signaling pathways as a target for breast cancer treatment. Endocr Relat Cancer (2016) 23(10):R485–98. doi: 10.1530/ERC-16-0190
56. Pedrazzoli P, Ferrante P, Kulekci A, Schiavo R, De Giorgi U, Carminati O, et al. Autologous hematopoietic stem cell transplantation for breast cancer in Europe: critical evaluation of data from the European group for blood and marrow transplantation (EBMT) registry 1990-1999. Bone Marrow Transplant (2003) 32(5):489–94. doi: 10.1038/sj.bmt.1704153
57. Gerratana L, Basile D, Toffoletto B, Bulfoni M, Zago S, Magini A, et al. Biologically driven cut-off definition of lymphocyte ratios in metastatic breast cancer and association with exosomal subpopulations and prognosis. Sci Rep (2020) 10(1):7010. doi: 10.1038/S41598-020-63291-2
58. Wang Lx, Zhang S, Wu Hj, Rong X, Guo J. M2b macrophage polarization and its roles in diseases. J Leukoc Biol (2019) 106(2):345–58. doi: 10.1002/JLB.3RU1018-378RR
59. Rahal OM, Wolfe AR, Mandal PK, Larson R, Tin S, Jimenez C, et al. Blocking interleukin (IL)4- and IL13-mediated phosphorylation of STAT6 (Tyr641) decreases M2 polarization of macrophages and protects against macrophage-mediated radioresistance of inflammatory breast cancer. Int J Radiat Oncol Biol Phys (2018) 100(4):1034–43. doi: 10.1016/J.IJROBP.2017.11.043
60. Hung CH, Chen FM, Lin YC, Tsai ML, Wang SL, Chen YC, et al. Altered monocyte differentiation and macrophage polarization patterns in patients with breast cancer. BMC Cancer (2018) 18(1):366. doi: 10.1186/S12885-018-4284-Y
61. Zhang B, Cao M, He Y, Liu Y, Zhang G, Yang C, et al. Increased circulating M2-like monocytes in patients with breast cancer. Tumour Biol (2017) 39(6):1010428317711571. doi: 10.1177/1010428317711571
62. Eiro N, Cid S, Aguado N, Fraile M, de Pablo N, Fernández B, et al. MMP1 and MMP11 expression in peripheral blood mononuclear cells upon their interaction with breast cancer cells and fibroblasts. Int J Mol Sci (2021) 22(1):371. doi: 10.3390/ijms22010371
63. Larsson AM, Nordström O, Johansson A, Rydén L, Leandersson K, Bergenfelz C, et al. Peripheral blood mononuclear cell populations correlate with outcome in patients with metastatic breast cancer. Cells (2022) 11(10):1639. doi: 10.3390/cells11101639
64. Williams CB, Yeh ES, Soloff AC. Tumor-associated macrophages: unwitting accomplices in breast cancer malignancy. NPJ Breast cancer (2016) 2(1):15025. doi: 10.1038/npjbcancer.2015.25
65. Leek RD, Hunt NC, Landers RJ, Lewis CE, Royds JA, Harris AL. Macrophage infiltration is associated with VEGF and EGFR expression in breast cancer. J Pathol (2000) 190(4):430–6. doi: 10.1002/(SICI)1096-9896(200003)190:4<430::AID-PATH538>3.0.CO;2-6
66. Hollmén M, Roudnicky F, Karaman S, Detmar M. Characterization of macrophage–cancer cell crosstalk in estrogen receptor positive and triple-negative breast cancer. Sci Rep (2015) 5:9188. doi: 10.1038/SREP09188
67. Allen SG, Chen YC, Madden JM, Fournier CL, Altemus MA, Hiziroglu AB, et al. Macrophages enhance migration in inflammatory breast cancer cells. via RhoC GTPase Signaling Sci Rep (2016) 6:39190. doi: 10.1038/SREP39190
68. Qian BZ, Li J, Zhang H, Kitamura T, Zhang J, Campion LR, et al. CCL2 recruits inflammatory monocytes to facilitate breast-tumour metastasis. Nat (2011) 475(7355):222–5. doi: 10.1038/nature10138
69. Kitamura T, Doughty-Shenton D, Cassetta L, Fragkogianni S, Brownlie D, Kato Y, et al. Monocytes differentiate to immune suppressive precursors of metastasis-associated macrophages in mouse models of metastatic breast cancer. Front Immunol (2018) 8:2004. doi: 10.3389/FIMMU.2017.02004
70. Coleman RE. Metastatic bone disease: clinical features, pathophysiology and treatment strategies. Cancer Treat Rev (2001) 27(3):165–76. doi: 10.1053/CTRV.2000.0210
71. Ma RY, Zhang H, Li XF, Zhang CB, Selli C, Tagliavini G, et al. Monocyte-derived macrophages promote breast cancer bone metastasis outgrowth. J Exp Med (2020) 217(11):e20191820. doi: 10.1084/JEM.20191820
72. Olson OC, Kim H, Quail DF, Foley EA, Joyce JA. Tumor-associated macrophages suppress the cytotoxic activity of antimitotic agents. Cell Rep (2017) 19(1):101–13. doi: 10.1016/j.celrep.2017.03.038
73. Yang C, He L, He P, Liu Y, Wang W, He Y, et al. Increased drug resistance in breast cancer by tumor-associated macrophages through IL-10/STAT3/bcl-2 signaling pathway. Med Oncol (2015) 32(2):352. doi: 10.1007/S12032-014-0352-6
74. Xu M, Liu M, Du X, Li S, Li H, Li X, et al. Intratumoral delivery of IL-21 overcomes anti-Her2/Neu resistance through shifting tumor-associated macrophages from M2 to M1 phenotype. J Immunol (2015) 194(10):4997–5006. doi: 10.4049/jimmunol.1402603
75. De Cicco P, Ercolano G, Ianaro A. The new era of cancer immunotherapy: Targeting myeloid-derived suppressor cells to overcome immune evasion. Front Immunol (2020) 11:1680. doi: 10.3389/fimmu.2020.01680
76. Ohki S, Shibata M, Gonda K, Machida T, Shimura T, Nakamura I, et al. Circulating myeloid-derived suppressor cells are increased and correlate to immune suppression, inflammation and hypoproteinemia in patients with cancer. Oncol Rep (2012) 28(2):453–8. doi: 10.3892/OR.2012.1812
77. Yang Y, Li C, Liu T, Dai X, Bazhin AV. Myeloid-derived suppressor cells in tumors: From mechanisms to antigen specificity and microenvironmental regulation. Front Immunol (2020) 11:1371. doi: 10.3389/fimmu.2020.01371
78. Millrud CR, Bergenfelz C, Leandersson K. On the origin of myeloid-derived suppressor cells. Oncotarget (2017) 8(2):3649–65. doi: 10.18632/oncotarget.12278
79. Markowitz J, Wesolowski R, Papenfuss T, Brooks TR, Carson WE. Myeloid-derived suppressor cells in breast cancer. Breast Cancer Res Treat (2013) 140(1):13–21. doi: 10.1007/S10549-013-2618-7
80. Yu J, Du W, Yan F, Wang Y, Li H, Cao S, et al. Myeloid-derived suppressor cells suppress antitumor immune responses through IDO expression and correlate with lymph node metastasis in patients with breast cancer. J Immunol (2013) 190(7):3783–97. doi: 10.4049/JIMMUNOL.1201449
81. Toor SM, Syed Khaja AS, El Salhat H, Faour I, Kanbar J, Quadri AA, et al. Myeloid cells in circulation and tumor microenvironment of breast cancer patients. Cancer Immunol Immunother (2017) 66(6):753–64. doi: 10.1007/S00262-017-1977-Z
82. Bergenfelz C, Larsson AM, Von Stedingk K, Aaltonen K, Jansson S, Jernström H, et al. Systemic monocytic-MDSCs are generated from monocytes and correlate with disease progression in breast cancer patients. PloS One (2015) 10(5):e0127028. doi: 10.1371/journal.pone.0127028
83. Bergenfelz C, Roxå A, Mehmeti M, Leandersson K, Larsson AM. Clinical relevance of systemic monocytic-MDSCs in patients with metastatic breast cancer. Cancer Immunol Immunother (2020) 69(3):435–48. doi: 10.1007/S00262-019-02472-Z
84. Ding D, Zhong H, Liang R, Lan T, Zhu X, Huang S, et al. Multifunctional nanodrug mediates synergistic photodynamic therapy and MDSCs-targeting immunotherapy of colon cancer. Adv Sci (Weinheim Baden-Wurttemberg Ger (2021) 8(14):e2100712. doi: 10.1002/advs.202100712
85. Myojin M, Horimoto Y, Ito M, Kitano S, Ishizuka Y, Sasaki R, et al. Neutrophil-to-lymphocyte ratio and histological type might predict clinical responses to eriburin-based treatment in patients with metastatic breast cancer. Breast Cancer (2020) 27(4):732–8. doi: 10.1007/S12282-020-01069-0
86. Vernieri C, Mennitto A, Prisciandaro M, Huber V, Milano M, Rinaldi L, et al. The neutrophil-to-lymphocyte and platelet-to-lymphocyte ratios predict efficacy of platinum-based chemotherapy in patients with metastatic triple negative breast cancer. Sci Rep (2018) 8(1):8703. doi: 10.1038/S41598-018-27075-Z
87. Lusho S, Durando X, Mouret-Reynier MA, Kossai M, Lacrampe N, Molnar I, et al. Platelet-to-Lymphocyte ratio is associated with favorable response to neoadjuvant chemotherapy in triple negative breast cancer: A study on 120 patients. Front Oncol (2021) 11:678315. doi: 10.3389/fonc.2021.678315
88. Iimori N, Kashiwagi S, Asano Y, Goto W, Takada K, Takahashi K, et al. Clinical significance of the neutrophil-to-Lymphocyte ratio in endocrine therapy for stage IV breast cancer. In Vivo (2018) 32(3):669–75. doi: 10.21873/invivo.11292
89. Takada K, Kashiwagi S, Asano Y, Goto W, Takahashi K, Shibutani M, et al. Clinical evaluation of dynamic monitoring of neutrophil-to-lymphocyte and platelet-to-lymphocyte ratios in primary endocrine therapy for advanced breast cancer. Anticancer Res (2019) 39(10):5581–8. doi: 10.21873/anticanres.13752
90. Ameratunga M, Kipps E, Okines AFC, Lopez JS. To cycle or fight–CDK4/6 inhibitors at the crossroads of anticancer immunity. Clin Cancer Res (2019) 25(1):21–8. doi: 10.1158/1078-0432.CCR-18-1999
91. Zattarin E, Fabbroni C, Ligorio F, Nichetti F, Lobefaro R, Rivoltini L, et al. 300P association between the neutrophil-to-lymphocyte and platelet-to-lymphocyte ratios and efficacy of CDK 4/6 inhibitors in advanced breast cancer: The observational multicenter Italian PALMARES study. Ann Oncol (2020) 31(4):S362–3. doi: 10.1016/j.annonc.2020.08.402
92. Weiner A, Munoz-Arcos LS, Ko H, Anampa J. Abstract PS4-30: Neutrophil-to-lymphocyte ratio or platelet-to-lymphocyte ratio as prognostic biomarkers in patients with metastatic breast cancer treated with CDK4/6 inhibitors. Cancer Res (2021) 81(Suppl. 4):PS4–30. doi: 10.1158/1538-7445.SABCS20-PS4-30
93. Ligorio F, Fucà G, Zattarin E, Lobefaro R, Zambelli L, Leporati R, et al. The pan-Immune-Inflammation-Value predicts the survival of patients with human epidermal growth factor receptor 2 (HER2)–positive advanced breast cancer treated with first-line taxane-Trastuzumab-Pertuzumab. Cancers (2021) 13(8):1964. doi: 10.3390/cancers13081964
94. Araki K, Ito Y, Fukada I, Kobayashi K, Miyagawa Y, Imamura M, et al. Predictive impact of absolute lymphocyte counts for progression-free survival in human epidermal growth factor receptor 2-positive advanced breast cancer treated with pertuzumab and trastuzumab plus eribulin or nab-paclitaxel. BMC Cancer (2018) 18(1):982. doi: 10.1186/S12885-018-4888-2
95. Voutsadakis IA. Prediction of immune checkpoint inhibitors benefit from routinely measurable peripheral blood parameters. Chin Clin Oncol (2020) 9(2):19. doi: 10.21037/CCO.2020.03.03
96. Mittendorf EA, Philips AV, Meric-Bernstam F, Qiao N, Wu Y, Harrington S, et al. PD-L1 expression in triple-negative breast cancer. Cancer Immunol Res (2014) 2(4):361–70. doi: 10.1158/2326-6066.CIR-13-0127
97. Kartolo A, Holstead R, Khalid S, Emack J, Hopman W, Robinson A, et al. Serum neutrophil-to-lymphocyte ratio and platelet-to-lymphocyte ratio in prognosticating immunotherapy efficacy. Immunother (2020) 12(11):785–98. doi: 10.2217/IMT-2020-0105
98. Johnson KE, Forward JA, Tippy MD, Ceglowski JR, El-Husayni S, Kulenthirarajan R, et al. Tamoxifen directly inhibits platelet angiogenic potential and platelet-mediated metastasis. Arterioscler Thromb Vasc Biol (2017) 37(4):664–74. doi: 10.1161/atvbaha.116.308791
99. Gareau AJ, Brien C, Gebremeskel S, Liwski RS, Johnston B, Bezuhly M. Ticagrelor inhibits platelet-tumor cell interactions and metastasis in human and murine breast cancer. Clin Exp Metastasis (2018) 35(1-2):25–35. doi: 10.1007/S10585-018-9874-1
100. Acharyya S, Massague J. Arresting supporters: targeting neutrophils in metastasis. Cell Res (2016) 26(3):273–4. doi: 10.1038/CR.2016.17
101. McCracken JM, Allen LAH. Regulation of human neutrophil apoptosis and lifespan in health and disease. J Cell Death (2014) 7(1):15. doi: 10.4137/JCD.S11038
102. Wu L, Saxena S, Awaji M, Singh RK. Tumor-associated neutrophils in cancer: Going pro. Cancers (Basel) (2019) 11(4):564. doi: 10.3390/cancers11040564
103. Pernasetti FM, inventor; PFIZER Inc., applicant. Combinations of tgfb inhibitors and cdk i nhibitors for the treatment of breast cancer. International publication number WO2020058820A1. World Intellectual Property Organization (2020).
104. Faget J, Peters S, Quantin X, Meylan E, Bonnefoy N. Neutrophils in the era of immune checkpoint blockade. J Immunother Cancer (2021) 9(7):e002242. doi: 10.1136/JITC-2020-002242
105. Wu L, Awaji M, Saxena S, Varney ML, Sharma B, Singh RK. IL-17-CXC chemokine receptor 2 axis facilitates breast cancer progression by up-regulating neutrophil recruitment. Am J Pathol (2020) 190(1):222–33. doi: 10.1016/j.ajpath.2019.09.016
106. Zhang W, Shen Y, Huang H, Pan S, Jiang J, Chen W, et al. A Rosetta stone for breast cancer: Prognostic value and dynamic regulation of neutrophil in tumor microenvironment. Front Immunol (2020) 11:1779. doi: 10.3389/fimmu.2020.01779
107. Truong AS, Zhou M, Krishnan B, Utsumi T, Manocha U, Stewart KG, et al. Entinostat induces antitumor immune responses through immune editing of tumor neoantigens. J Clin Invest (2021) 131(16):e138560. doi: 10.1172/JCI138560
108. Connolly RM, Rudek MA, Piekarz R. Entinostat: a promising treatment option for patients with advanced breast cancer. Future Oncol (2017) 13(13):1137–48. doi: 10.2217/fon-2016-0526
109. Tomita Y, Lee MJ, Lee S, Tomita S, Chumsri S, Cruickshank S, et al. The interplay of epigenetic therapy and immunity in locally recurrent or metastatic estrogen receptor-positive breast cancer: Correlative analysis of ENCORE 301, a randomized, placebo-controlled phase II trial of exemestane with or without entinostat. Oncoimmunology (2016) 5(11):e1219008. doi: 10.1080/2162402X.2016.1219008
110. Yokoyama Y, Lew ED, Seelige R, Tindall EA, Walsh C, Fagan PC, et al. Immuno-oncological efficacy of RXDX-106, a novel TAM (TYRO3, AXL, MER) family small-molecule kinase inhibitor. Cancer Res (2019) 79(8):1996–2008. doi: 10.1158/0008-5472.can-18-2022
111. Plummer R, Sodergren M, Pinato D, Sarker D, Reebye V, Spalding D, et al. 515 a phase 1 study of myeloid modulating agent MTL-CEBPA in combination with pembrolizumab in adult patients with advanced solid tumours. J Immunother Cancer (2021) 9(Suppl. 2):A545–5. doi: 10.1136/jitc-2021-SITC2021.515
112. Gabrail NY, Hamilton EP, Elias AD, Rimawi M F, Li C, Corvez MM, et al. A phase 1/2 trial of ORIN1001, a first-in-class IRE1 inhibitor, in patients with advanced solid tumors. J Clin Oncol (2021) 15(Suppl.):3080–0. doi: 10.1200/jco.2021.39.15_suppl.3080
113. Lu X, Kang Y. Chemokine (C-c motif) ligand 2 engages CCR2+ stromal cells of monocytic origin to promote breast cancer metastasis to lung and bone. J Biol Chem (2009) 284(42):29087–96. doi: 10.1074/jbc.m109.035899
114. Wesolowski R, Sharma N, Reebel L, Rodal MB, Peck A, West BL, et al. Phase ib study of the combination of pexidartinib (PLX3397), a CSF-1R inhibitor, and paclitaxel in patients with advanced solid tumors. Ther Adv Med Oncol (2019) 11:1758835919854238. doi: 10.1177/1758835919854238
115. Kuemmel S, Campone M, Loirat D, Lopez RL, Beck JT, De Laurentiis M, et al. A randomized phase II study of anti-CSF1 monoclonal antibody lacnotuzumab (MCS110) combined with gemcitabine and carboplatin in advanced triple-negative breast cancer. Clin Cancer Res (2022) 28(1):106–15. doi: 10.1158/1078-0432.CCR-20-3955
116. Gomez-Roca CA, Italiano A, Le Tourneau C, Cassier PA, Toulmonde M, D'Angelo SP, et al. Phase I study of emactuzumab single agent or in combination with paclitaxel in patients with advanced/metastatic solid tumors reveals depletion of immunosuppressive M2-like macrophages. Ann Oncol (2019) 30(8):1381–92. doi: 10.1093/annonc/mdz163
117. MacHiels JP, Gomez-Roca C, Michot JM, Zamarin D, Mitchell T, Catala G, et al. Phase ib study of anti-CSF-1R antibody emactuzumab in combination with CD40 agonist selicrelumab in advanced solid tumor patients. J Immunother cancer (2020) 8(2):e001153. doi: 10.1136/jitc-2020-001153
118. Autio KA, Klebanoff CA, Schaer D, Kauh JSW, Slovin SF, Adamow M, et al. Immunomodulatory activity of a colony-stimulating factor-1 receptor inhibitor in patients with advanced refractory breast or prostate cancer: A phase I study. Clin Cancer Res (2020) 26(21):5609–20. doi: 10.1158/1078-0432.CCR-20-0855
119. Bonapace L, Coissieux MM, Wyckoff J, Mertz KD, Varga Z, Junt T, et al. Cessation of CCL2 inhibition accelerates breast cancer metastasis by promoting angiogenesis. Nat (2014) 515(7525):130–3. doi: 10.1038/nature13862
120. Puig-Kröger A, Sierra-Filardi E, Domínguez-Soto A, Samaniego R, Corcuera MT, Gómez-Aguado F, et al. Folate receptor beta is expressed by tumor-associated macrophages and constitutes a marker for M2 anti-inflammatory/regulatory macrophages. Cancer Res (2009) 69(24):9395–403. doi: 10.1158/0008-5472.can-09-2050
121. Bak SP, Walters JJ, Takeya M, Conejo-Garcia JR, Berwin BL. Scavenger receptor-a-targeted leukocyte depletion inhibits peritoneal ovarian tumor progression. Cancer Res (2007) 67(10):4783–9. doi: 10.1158/0008-5472.CAN-06-4410
122. Rogers TL, Holen I. Tumour macrophages as potential targets of bisphosphonates. J Transl Med (2011) 9(1):177. doi: 10.1186/1479-5876-9-177
123. Rietkötter E, Menck K, Bleckmann A, et al. Zoledronic acid inhibits macrophage/microglia-assisted breast cancer cell invasion. Oncotarget (2013) 4(9):1449–60. doi: 10.18632/oncotarget.1201
124. Qiu SQ, Waaijer SJH, Zwager MC, de Vries EGE, van der Vegt B, Schröder CP. Tumor-associated macrophages in breast cancer: Innocent bystander or important player? Cancer Treat Rev (2018) 70:178–89. doi: 10.1016/j.ctrv.2018.08.010
125. Adams S, Kozhaya L, Martiniuk F, Meng TC, Chiriboga L, Liebes L, et al. Topical TLR7 agonist imiquimod can induce immune-mediated rejection of skin metastases in patients with breast cancer. Clin Cancer Res (2012) 18(24):6748–57. doi: 10.1158/1078-0432.CCR-12-1149
126. Salazar LG, Lu H, Reichow JL, Childs JS, Coveler AL, Higgins DM, et al. Topical imiquimod plus nab-paclitaxel for breast cancer cutaneous metastases: A phase 2 clinical trial. JAMA Oncol (2017) 3(7):969–73. doi: 10.1001/jamaoncol.2016.6007
127. Geller MA, Cooley S, Argenta PA, Downs LS, Carson LF, Judson PL, et al. Toll-like receptor-7 agonist administered subcutaneously in a prolonged dosing schedule in heavily pretreated recurrent breast, ovarian, and cervix cancers. Cancer Immunol Immunother (2010) 59(12):1877–84. doi: 10.1007/S00262-010-0914-1
128. Djureinovic D, Wang M, Kluger HM. Agonistic CD40 antibodies in cancer treatment. Cancers (Basel) (2021) 13(6):1–18. doi: 10.3390/cancers13061302
129. Irenaeus SMM, Nielsen D, Ellmark P, Yachnin J, Deronic A, Nilsson A, et al. First-in-human study with intratumoral administration of a CD40 agonistic antibody, ADC-1013, in advanced solid malignancies. Int J cancer (2019) 145(5):1189–99. doi: 10.1002/IJC.32141
130. Jiang Z, Sun H, Yu J, Tian W, Song Y. Targeting CD47 for cancer immunotherapy. J Hematol Oncol (2021) 14(1). doi: 10.1186/S13045-021-01197-W
131. Dizman N, Buchbinder EI. Cancer therapy targeting CD47/SIRPα. Cancers (Basel) (2021) 13(24):6229. doi: 10.3390/cancers13246229
132. Upton R, Banuelos A, Feng D, Biswas T, Kao K, McKenna K, et al. Combining CD47 blockade with trastuzumab eliminates HER2-positive breast cancer cells and overcomes trastuzumab tolerance. Proc Natl Acad Sci USA (2021) 118(29):e2026849118. doi: 10.1073/pnas.2026849118
133. Lakhani NJ, Chow LQM, Gainor JF, LoRusso P, Lee KW, Chung HC, et al. Evorpacept alone and in combination with pembrolizumab or trastuzumab in patients with advanced solid tumours (ASPEN-01): a first-in-human, open-label, multicentre, phase 1 dose-escalation and dose-expansion study. Lancet Oncol (2021) 22(12):1740–51. doi: 10.1016/S1470-2045(21)00584-2
134. Sikic BI, Lakhani N, Patnaik A, Shah SA, Chandana SR, Rasco D, et al. First-in-Human, first-in-Class phase I trial of the anti-CD47 antibody Hu5F9-G4 in patients with advanced cancers. J Clin Oncol (2019) 37(12):946–53. doi: 10.1200/JCO.18.02018
135. Brown GD. Innate antifungal immunity: the key role of phagocytes. Annu Rev Immunol (2011) 29:1–21. doi: 10.1146/annurev-immunol-030409-101229
136. Iglesias JL, Prathikanti R, Ma B, Mattson P, Kedrowski D, Lowe J, et al. A multicenter, open-label, phase II study of PGG beta-glucan and pembrolizumab in patients (pts) with advanced melanoma (MEL) following progression on treatment with checkpoint inhibitors (CPI) or triple negative breast cancer (TNBC) failing front-line chemotherapy for metastatic disease. JCO (2017) 15(Suppl.):TPS3105–TPS3105. doi: 10.1200/jco.2017.35.15_suppl.tps3105
137. Huang JH, Lin CY, Wu SY, Chen WY, Chu CL, Brown GD, et al. CR3 and dectin-1 collaborate in macrophage cytokine response through association on lipid rafts and activation of syk-JNK-AP-1 pathway. PloS Pathog (2015) 11(7):e1004985. doi: 10.1371/journal.ppat.1004985
138. De Palma M, Naldini L. Angiopoietin-2 TIEs up macrophages in tumor angiogenesis. Clin Cancer Res (2011) 17(16):5226–32. doi: 10.1158/1078-0432.CCR-10-0171
139. Mazzieri R, Pucci F, Moi D, Zonari E, Ranghetti A, Berti A, et al. Targeting the ANG2/TIE2 axis inhibits tumor growth and metastasis by impairing angiogenesis and disabling rebounds of proangiogenic myeloid cells. Cancer Cell (2011) 19(4):512–26. doi: 10.1016/J.CCR.2011.02.005
140. Sfiligoi C, De Luca A, Cascone I, Sorbello V, Fuso L, Ponzone R, et al. Angiopoietin-2 expression in breast cancer correlates with lymph node invasion and short survival. Int J cancer (2003) 103(4):466–74. doi: 10.1002/ijc.10851
141. Diéras V, Wildiers H, Jassem J, Dirix LY, Guastalla JP, Bono P, et al. Trebananib (AMG 386) plus weekly paclitaxel with or without bevacizumab as first-line therapy for HER2-negative locally recurrent or metastatic breast cancer: A phase 2 randomized study. Breast (2015) 24(3):182–90. doi: 10.1016/j.breast.2014.11.003
142. Kaufman PA, Wildiers H, Freyer G, Kemeny M, Gonçalves A, Jerusalem G, et al. Phase 1b study of trebananib plus paclitaxel and trastuzumab in patients with HER2-positive locally recurrent or metastatic breast cancer. Clin Breast Cancer (2019) 19(1):47–57. doi: 10.1016/j.clbc.2018.09.012
143. Hidalgo M, Martinez-Garcia M, Le Tourneau C, Massard C, Garralda E, Boni V, et al. First-in-Human phase I study of single-agent vanucizumab, a first-in-Class bispecific anti-Angiopoietin-2/Anti-VEGF-A antibody, in adult patients with advanced solid tumors. Clin Cancer Res (2018) 24(7):1536–45. doi: 10.1158/1078-0432.ccr-17-1588
144. Papadopoulos KP, Kelley RK, Tolcher AW, Razak AR, Van Loon K, Patnaik A, et al. A phase I first-in-Human study of nesvacumab (REGN910), a fully human anti–Angiopoietin-2 (Ang2) monoclonal antibody, in patients with advanced solid tumors. Clin Cancer Res (2016) 22(6):1348–55. doi: 10.1158/1078-0432.ccr-15-1221
145. Harney AS, Karagiannis GS, Pignatelli J, Smith BD, Kadioglu E, Wise SC, et al. The selective Tie2 inhibitor rebastinib blocks recruitment and function of Tie2Hi macrophages in breast cancer and pancreatic neuroendocrine tumors. Mol Cancer Ther (2017) 16(11):2486–501. doi: 10.1158/1535-7163.mct-17-0241
146. Brandão RD, Veeck J, Van de Vijver KK, Lindsey P, de Vries B, van Elssen CH, et al. A randomised controlled phase II trial of pre-operative celecoxib treatment reveals anti-tumour transcriptional response in primary breast cancer. Breast Cancer Res (2013) 15(2):R29. doi: 10.1186/BCR3409
147. Bundred NJ, Cramer A, Morris J, Renshaw L, Cheung KL, Flint P, et al. Cyclooxygenase-2 inhibition does not improve the reduction in ductal carcinoma in situ proliferation with aromatase inhibitor therapy: results of the ERISAC randomized placebo-controlled trial. Clin Cancer Res (2010) 16(5):1605–12. doi: 10.1158/1078-0432.CCR-09-1623
148. Gill S, Brudno JN. CAR T-cell therapy in hematologic malignancies: Clinical role, toxicity, and unanswered questions. Am Soc Clin Oncol Educ book Am Soc Clin Oncol Annu Meet (2021) 41(41):e246–65. doi: 10.1200/EDBK_320085
149. Marofi F, Motavalli R, Safonov VA, Thangavelu L, Yumashev AV, Alexander M, et al. CAR T cells in solid tumors: challenges and opportunities. Stem Cell Res Ther (2021) 12(1):81. doi: 10.1186/S13287-020-02128-1
150. Schettini F, Barbao P, Brasó-Maristany F, Galván P, Martínez D, Paré L, et al. Identification of cell surface targets for CAR-T cell therapies and antibody-drug conjugates in breast cancer. ESMO Open (2021) 6(3):100102. doi: 10.1016/j.esmoop.2021.100102
151. Elkington PT, Green JA, Friedland JS. Analysis of matrix metalloproteinase secretion by macrophages. Methods Mol Biol (2009) 531:253–65. doi: 10.1007/978-1-59745-396-7_16
152. Sloas C, Gill S, Klichinsky M. Engineered CAR-macrophages as adoptive immunotherapies for solid tumors. Front Immunol (2021) 12:783305. doi: 10.3389/fimmu.2021.783305
153. Zhang W, Liu L, Su HF, Liu Q, Shen J, Dai H, et al. Chimeric antigen receptor macrophage therapy for breast tumours mediated by targeting the tumour extracellular matrix. Br J Cancer (2019) 121(10):837–45. doi: 10.1038/S41416-019-0578-3
154. Borzone FR, Giorello MB, Sanmartin MC, Yannarelli G, Martinez LM, Chasseing NA. Mesenchymal stem cells and cancer-associated fibroblasts as a therapeutic strategy for breast cancer. Br J Pharmacol (2022) 1–19. doi: 10.1111/bph.15861
155. Conti M, Tazzari V, Baccini C, Pertici G, Serino LP, De Giorgi U. Anticancer drug delivery with nanoparticles. In Vivo (2006) 20(6A):697–701.
156. Dempke WCM, Fenchel K, Uciechowski P, Dale SP. Second- and third-generation drugs for immuno-oncology treatment-the more the better? Eur J Cancer (2017) 74:55–72. doi: 10.1016/j.ejca.2017.01.001
Keywords: metastatic breast cancer, biomarker, inflammatory cells, NLR, prognostic, new treatments, macrophages, predictive
Citation: Gianni C, Palleschi M, Schepisi G, Casadei C, Bleve S, Merloni F, Sirico M, Sarti S, Cecconetto L, Di Menna G, Schettini F and De Giorgi U (2022) Circulating inflammatory cells in patients with metastatic breast cancer: Implications for treatment. Front. Oncol. 12:882896. doi: 10.3389/fonc.2022.882896
Received: 24 February 2022; Accepted: 05 July 2022;
Published: 08 August 2022.
Edited by:
Jawed Akhtar Siddiqui, University of Nebraska Medical Center, United StatesReviewed by:
Marcus Vetter, University Hospital of Basel, SwitzerlandCopyright © 2022 Gianni, Palleschi, Schepisi, Casadei, Bleve, Merloni, Sirico, Sarti, Cecconetto, Di Menna, Schettini and De Giorgi. This is an open-access article distributed under the terms of the Creative Commons Attribution License (CC BY). The use, distribution or reproduction in other forums is permitted, provided the original author(s) and the copyright owner(s) are credited and that the original publication in this journal is cited, in accordance with accepted academic practice. No use, distribution or reproduction is permitted which does not comply with these terms.
*Correspondence: Caterina Gianni, Y2F0ZXJpbmEuZ2lhbm5pQGlyc3QuZW1yLml0
Disclaimer: All claims expressed in this article are solely those of the authors and do not necessarily represent those of their affiliated organizations, or those of the publisher, the editors and the reviewers. Any product that may be evaluated in this article or claim that may be made by its manufacturer is not guaranteed or endorsed by the publisher.
Research integrity at Frontiers
Learn more about the work of our research integrity team to safeguard the quality of each article we publish.