- 1Laboratory of Gynecological Oncology, Department of Gynecology, Fujian Maternity and Child Health Hospital, Affiliated Hospital of Fujian Medical University, Fuzhou, China
- 2Fujian Key Laboratory of Women and Children’s Critical Diseases Research, Fuzhou, China
Oxygen is critical to energy metabolism, and tumors are often characterized by a hypoxic microenvironment. Owing to the high metabolic energy demand of malignant tumor cells, their survival is promoted by metabolic reprogramming in the hypoxic microenvironment, which can confer tumor cell resistance to pyroptosis. Pyroptosis resistance can inhibit anti-tumor immunity and promote the development of malignant tumors. Hypoxia inducible factor-1α (HIF-1α) is a key regulator of metabolic reprogramming in tumor cells, and estrogen-related receptor α (ERRα) plays a key role in regulating cellular energy metabolism. Therefore, the close interaction between HIF-1α and ERRα influences the metabolic and functional changes in cancer cells. In this review, we summarize the reprogramming of tumor metabolism involving HIF-1α/ERRα. We review our understanding of the role of HIF-1α/ERRα in promoting tumor growth adaptation and pyroptosis resistance, emphasize its key role in energy homeostasis, and explore the regulation of HIF-1α/ERRα in preventing and/or treating endometrial carcinoma patients. This review provides a new perspective for the study of the molecular mechanisms of metabolic changes in tumor progression.
Introduction
Endometrial carcinoma (EC) ranks first among malignant tumors of the female reproductive system in developed countries and second in China (1). Over the past decade, the EC morbidity and mortality rates have gradually increased (2), and the age of patients is younger than previously observed (3). According to the statistics of the National Cancer Center, in 2019, the incidence of EC in China was 10.28/100,000, and the mortality rate was 1.9/100000. The mortality rate of uterine cancer is twice as high as its morbidity rate (4). This may be due to the fact that specific pathological types of EC, such as serous carcinoma or EC with high risk factors (such as distant metastasis or deep myometrial invasion), are resistant to pyroptosis after radiotherapy/chemotherapy, resulting in the risk of tumor recurrence and metastasis, and a significantly increased mortality rate (5). Although recent progress has been made in the diagnosis and treatment of EC, and better clinical outcomes have been achieved, the quality of life of patients is affected by the side effects of treatment. These can include infertility, surgical menopause, lower limb lymphedema, sexual dysfunction, depression, and fatigue, which pose a serious threat to women’s health (6, 7).
In 1983, Bokhman (8) classified EC into type I and type II according to the presence or absence of estrogen stimulation. The diagnosis and prognosis of type I EC are related to mutations in the genes PTEN, PI3KCA, POLE, CTNNB1, and TP53; deletions of the DNA mismatch repair protein; and the expression of estrogen receptor and progesterone receptor. The prognosis of type II EC is related to the overexpression of human epidermal growth factor receptor 2 (HER2) and mutations in the TP53 gene. The activation of PI3K/Akt, P53, mitogen-activated protein kinase, and Wnt/β-catenin signaling pathways is closely related to the pathogenesis of EC (9). In 2013, a molecular genetic analysis of 373 EC patient samples was carried out based on the Cancer Genome Atlas (TCGA), and cases were divided into four subgroups as follows: POLE mutation, microsatellite-instable (MSI), copy number low (CNL), and copy number high (CNH). Among them, patients with POLE mutations have the best prognosis, those with MSI and CNL have a moderate prognosis, and those with the CNH-mutant type have the worst prognosis (10). Therefore, it is necessary to better understand the molecular changes in the progression of EC and develop new biomarkers or targeted therapy to further improve the overall prognosis of patients with EC.
Despite the obvious heterogeneity of EC, only the standardized treatment is still used for all subtypes. Since the Bokhman classification cannot explain the high morphological and molecular heterogeneity of EC, the histopathological classification method based on tumor morphology and tumor grade needs to be further improved. Santoro et al. (11) proposed the distribution and prognosis of different histopathological types of EC in a molecular classification based on TCGA, put forward the improvement in risk stratification based on molecules, and discussed the corresponding treatment methods as well. These observations need to be evaluated by experienced gynecologic oncologists, pathologists, and geneticists. Histopathology and TCGA molecular typing combined with diagnosis and treatment methods can be used to accurately treat EC and predict the susceptibility and prognosis of EC to specific therapeutic drugs (12). However, according to the current diagnosis and treatment guidelines and the medical situation, it is impossible to perform a detailed molecular typing in every EC patient. This requires a multi-disciplinary team (MDT) (molecular biologists, clinicians, oncologists, geneticists, and other professionals, as well as paramedical units, such as pathology, ultrasound, and imaging) to decide the treatment method that is most likely to benefit the patients, by integrating medical history, complications, clinical, histomorphology, immunohistochemistry and molecular data. Through cross-cooperation in the MDT, a dynamic assessment of the condition, treatment, and regular reexamination can be carried out in the form of joint and inter-disciplinary consultations, so that the diagnosis and treatment strategy can be adjusted in time and a more reasonable treatment plan can be provided. MDT mode can be applied to the whole process of prevention, diagnosis, preoperative evaluation, postoperative treatment, and follow-up of EC outpatient service, which can improve the quality of life and prognosis of cancer patients (13).
Hypertension, obesity, and diabetes are the triple risk factors of EC; in fact, EC can be regarded as a metabolic disease (14). Inflammation may be activated in EC patients due to oxidative stress and increased systemic inflammation caused by metabolic syndrome, and tumor cells reprogram their metabolic pathways in this inflammatory environment to maintain a higher proliferation rate; promote tumor growth, invasion, and neovascularization; and resist cell death signals (15–17). Hypoxia inducible factor-1α (HIF-1α) is a key regulator of tumor invasion and a key promoter of energy adaptation. Increased HIF-1α activity promotes angiogenesis, metabolic reprogramming, metabolic adaptation, extracellular matrix remodeling, epithelial mesenchymal transformation (EMT), invasion, metastasis, resistance to radiotherapy and chemotherapy, maintenance of cancer stem cell phenotype, immune escape, and protein expression affecting changes in the tumor immune microenvironment during early carcinogenesis (18, 19). HIF-1α is a prognostic marker related to the overall poor prognosis of tumor patients (20). Estrogen-related receptor α (ERRα) subtypes are highly expressed in malignant tumors, especially in hormone-dependent/related tumors, such as breast cancer (BRCA) (21), prostate cancer (PCa) (22), ovarian cancer (23), and endometrial cancer (24–26). At the same time, ERRα, acting as a transcription factor, regulates the process of energy metabolism in the body via the tricarboxylic acid cycle (27), oxidative phosphorylation (OXPHOS) (28), and glucose and lipid metabolism (29). As a result, a variety of biological effects are observed, such as tumor cell proliferation, differentiation, and apoptosis; tumor angiogenesis; and control of systemic inflammation. These processes are related to the prognosis and progression of the tumor. At present, clarifying how tumor cells resist pyroptosis and adapt to hypoxia through metabolic reprogramming, is a hot research topic aimed at overcoming metastatic/recurrent tumors using anti-metabolic therapy. We hypothesized that metabolic reprogramming intervention of tumor cells may be an important approach for the treatment of metastatic tumors. Based on this hypothesis, this review summarizes the role of metabolic changes caused by HIF-1α/ERRα in the metabolic reprogramming of tumor cells in the growth, invasion, and metastasis of EC (Figure 1). These areas are very important for understanding the occurrence, progression, and treatment stratification of EC.
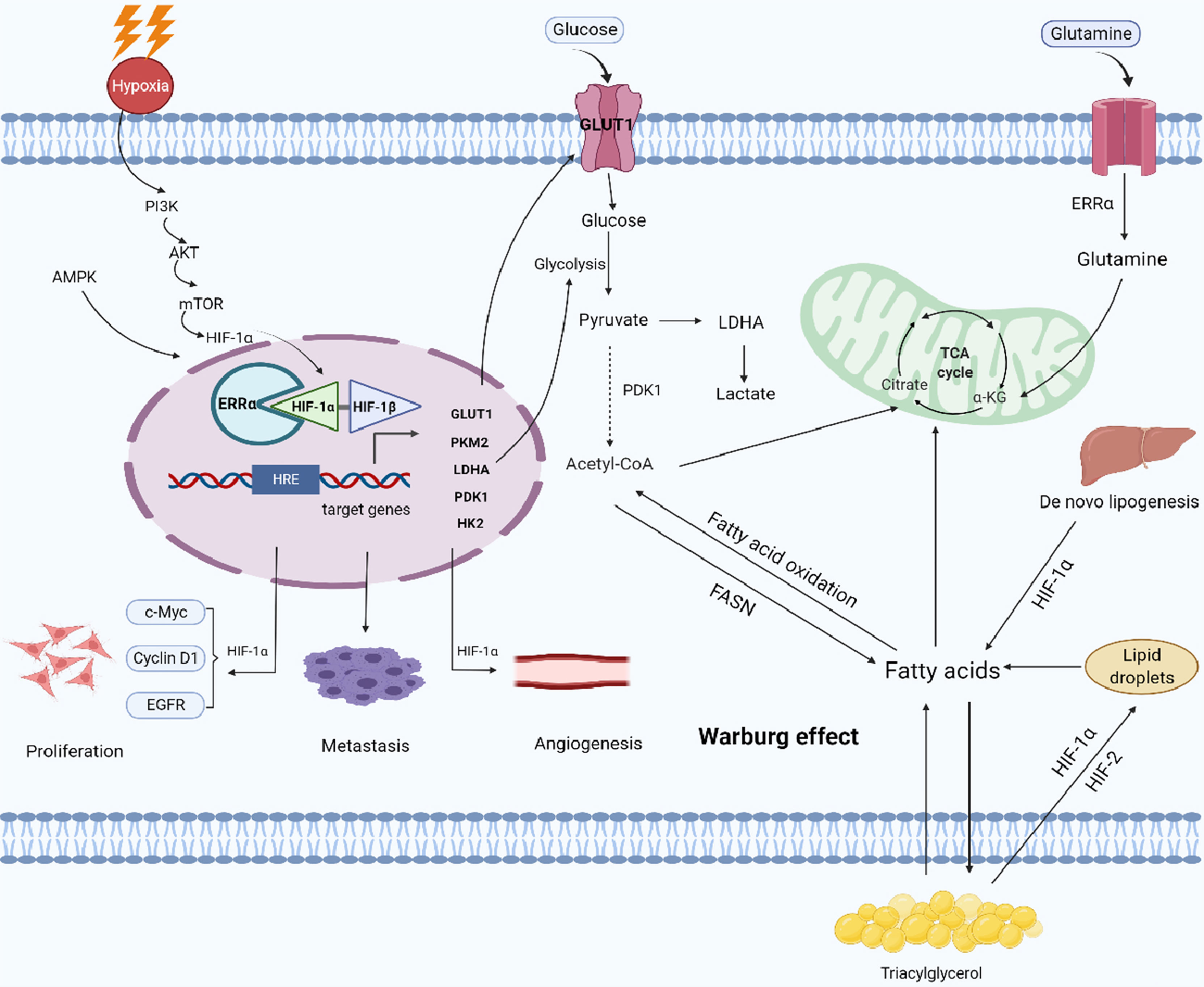
Figure 1 Working model of the role of HIF-1α/ERRα in cancer cell metabolism. Cancer cells can adapt to low oxygen conditions through the PI3K/AKT/mTOR and AMPK signaling pathways, which regulate metabolic reprogramming. ERRα interacts with HIF-1α, enhancing the transcriptional activity of HIF-1α and promoting the remodeling of glucose and lipid metabolism (formation of lipid droplets) in cancer cells. ERRα also enhances glutamine metabolism and lipid de novo synthesis, promoting metabolic adaptation in cancer cells. Together, these activities stimulate tumor proliferation, metastasis, and angiogenesis. Images were made in BioRender (Biorender.com).
HIF-1α Participates in Regulated Glucose and Lipid Metabolism in Cancer Cells
The metabolic phenotype of cancer cells varies in different types of cancer; for example, some malignant tumors mainly rely on glycolysis, whereas others exhibit an OXPHOS-mediated phenotype (30). In general, coordinated catabolism and anabolism are essential for tumor cells to maintain an energy supply and biosynthesis (31). The metabolic adaptation process of tumors is driven by several key carcinogenic signal cascades or kinase signals, including specific genes such as cMYC (32, 33), adenosine monophosphate-activated protein kinase (AMPK) (34), and PI3K/Akt/mTOR (35). Myc directly activates the transcription of glycolytic enzymes, namely GLUT1, LDHA, PKM2, and HK2; coordinates cell metabolism and cell proliferation; and promotes cell malignant transformation (32, 33). These key rate-limiting enzymes involved in glycolysis promote the development of drug resistance in tumor cells, which proves the importance and effectiveness of targeted therapy in the regulation of energy metabolism in malignant tumor cells. AMPK restores the cellular ATP pool by promoting catabolism and inhibiting anabolism and plays a central role in regulating the reprogramming of cellular energy metabolism to adapt to metabolic stress (36, 37). Inhibition of the PI3K/AKT/mTOR/HIF-1α signaling pathway can reduce the expression of the key glycolytic enzymes PKM2 and LDHA, thus inducing cell death and improving the cytotoxicity of cisplatin (38). PTEN can inhibit the activity of PI3K/AKT/mTOR pathway, and PTEN dysfunction is the most common genetic change in EC (39). Therefore, targeting PI3K may improve the personalized treatment of EC patients with PTEN mutation (40). Energy for tumor cells is provided by the high activity of aerobic glycolysis stimulated by HIF-1α. This creates an acidic microenvironment that promotes EMT and leads to more invasive phenotypes, such as radiotherapy and chemotherapy resistance or tumor metastasis, which are closely related to poor clinical prognosis (41). It is suggested that the regulation of HIF-1α may be a new approach to overcome the drug resistance of tumor cells. Hence, further exploration of the malignant progression of tumors driven by HIF-1α through the above carcinogenic pathway will contribute to the development of better treatment methodologies for tumors.
The reprogramming of lipid metabolism has become a marker of malignant tumors (42, 43). Lipids make up the basic components of the cell plasma membrane and they are also important signal molecules and energy sources. Lipid biosynthesis plays an important role in highly proliferative malignant tumor cells (44) (such as prostate, breast, liver, and kidney cancers) supporting the production of cell membrane and the regulation of its fluidity; promoting the formation of triacylglycerides and energy storage; promoting resistance to reactive oxygen species (ROS) damage (45, 46); and producing signal molecules involved in cancer cell migration, inflammation, and survival (47). These changes promote the further development of cancer. HIF-1α-dependent changes in lipid metabolism can promote the survival and growth of cancer cells. Lipid droplets can promote cancer cell proliferation and tumor growth, and there is a positive correlation between the level of lipid droplets in tumors and a poor prognosis (48). The accumulation of lipid droplets has been confirmed in different types of cancers, including breast (49, 50), cervical (51), prostate (52), and ovarian (53) cancers. Under hypoxic conditions, because oxygen-dependent stearoyl-CoA desaturase is inhibited (54), the ratio of saturated/unsaturated fatty acids changes, thus affecting the integrity of cell membranes and cell function. HIF-1α inhibits the accumulation of saturated lipids and reduces the toxicity induced by saturated fatty acids by promoting the expression of fatty acid synthase, triggering fatty acid synthesis and activation, which promote the production of unsaturated fatty acids (55, 56). These findings emphasize the importance of changes in lipid metabolism in promoting tumor development and suggest that targeting lipid metabolism in tumor cells may be a new approach for the treatment of patients with malignant tumors (Table 1). However, there are still few studies on the effects of HIF-1α on the distribution of lipid metabolism and the reprogramming of lipid metabolism in tumor cells.
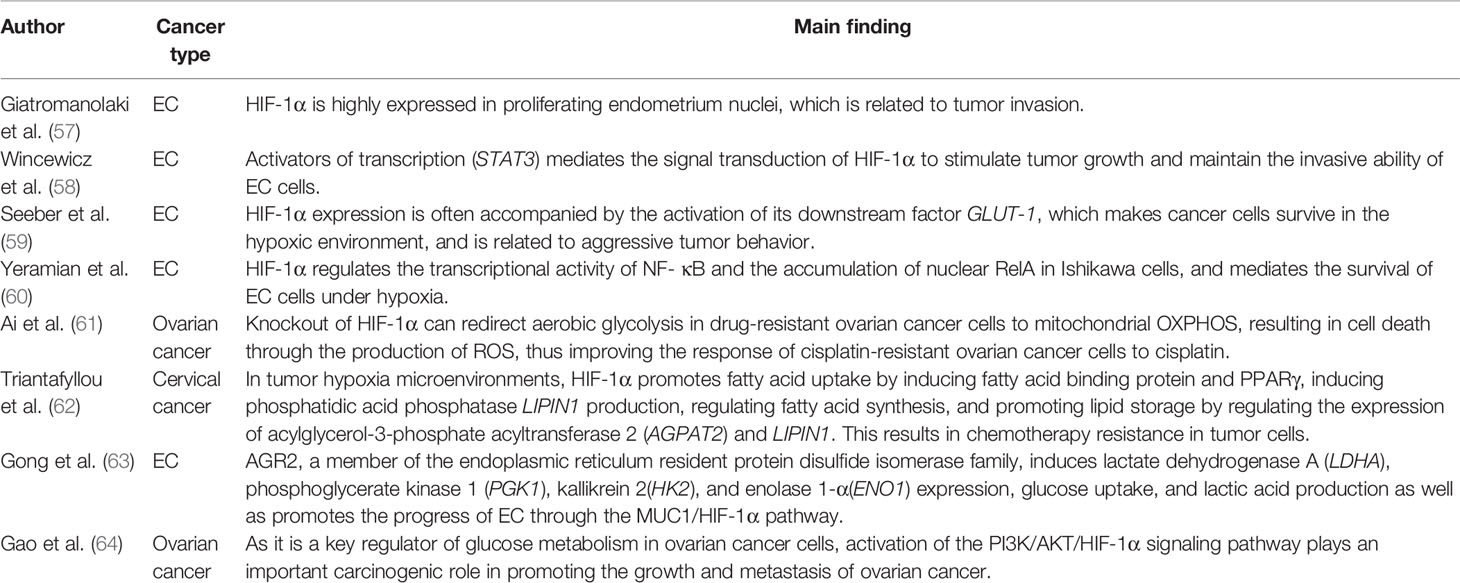
Table 1 Studies on HIF-1α regulation of glucose and lipid metabolism and promotion of gynecological malignant tumor progression.
ERRα Participates in Regulated Glucose and Lipid Metabolism in Cancer Cells
ERRα can control key metabolic processes by transcriptional regulation of different metabolic genes during cell differentiation (65). ERRα inhibits glucose oxidation by transcriptionally activating pyruvate dehydrogenase kinase 4 (PDK4) (66). Inhibition of ERRα can block mitochondrial respiration and enhance the effect of tumor chemotherapy (67). HIF-1α can improve oxygen-dependent energy metabolism through mitochondrial biogenesis, mainly through the signal transduction pathway of the PGC-1α/HIF-1α/ERRα axis. This, in turn, promotes the reprogramming of tumor metabolism and leads to tumor metastasis and progression. Therefore, we infer that ERRα is a key regulator of metabolic reprogramming under glucose stress and hypoxia.
ERRα plays an important role as a nuclear transcription factor in lipid metabolism. Increased activity of ERRα can increase the oxidation rate of fatty acids. Genetic or pharmacological inhibition of ERRα can reduce fat weight and lipid accumulation and resist high fat-diet-induced obesity (68). ERRα can also stimulate adipogenesis by increasing the accumulation of triglycerides (69). From a metabolic standpoint, lipid metabolism is significantly upregulated in EC, affecting the treatment outcome and/or disease progression of patients (70). The ERRα axis is a central regulator of metabolism in malignant tumors, especially in BRCA (71). High levels of ERRα activity maximize energy production by promoting mitochondrial metabolism and angiogenesis, thus meeting the energy metabolic needs of rapid cell proliferation (72, 73). These observations provide a causal relationship to explain how ERRα regulates tumor metabolism (Table 2).
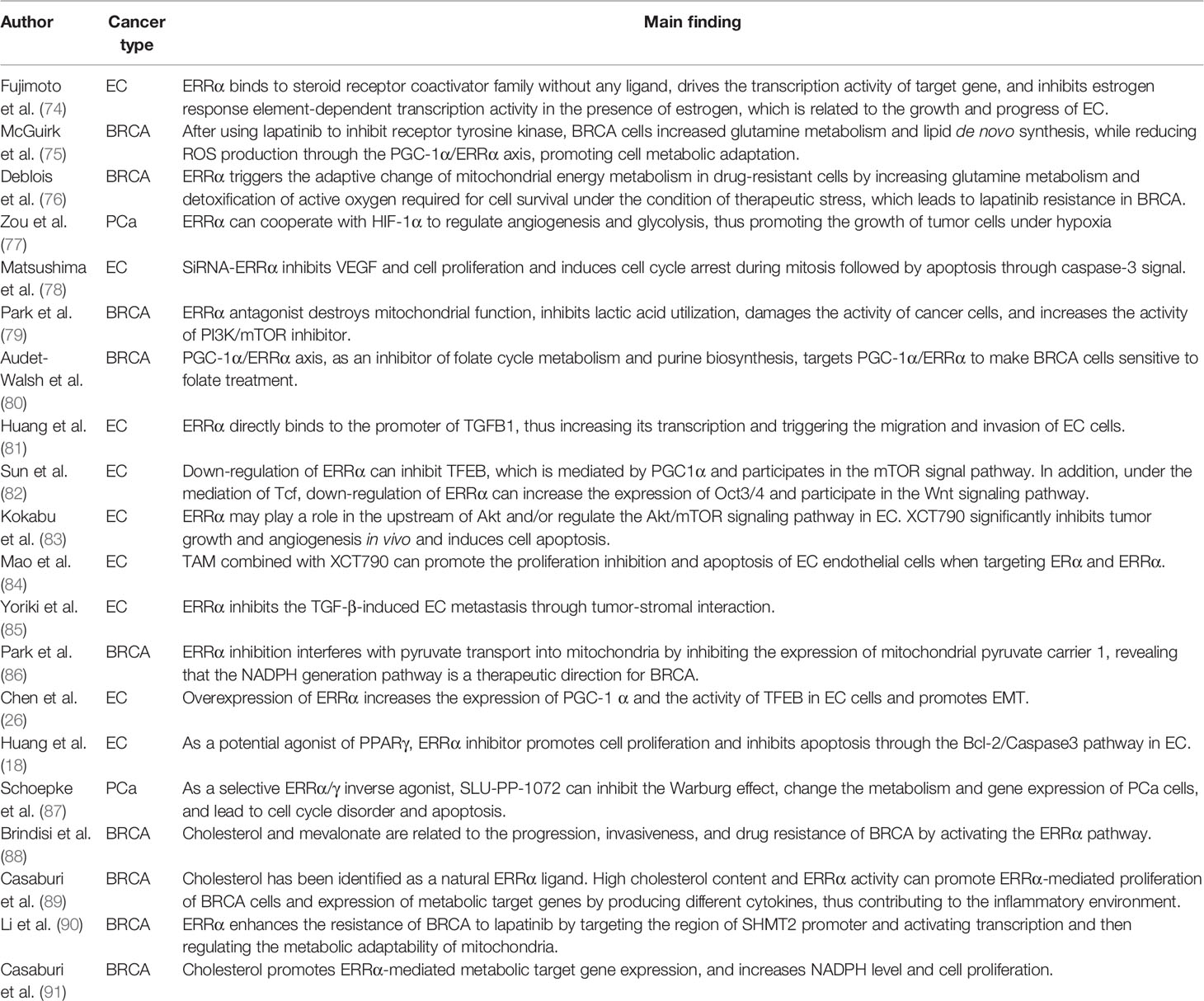
Table 2 Studies on ERRα regulation of glucose and lipid metabolism and promotion of hromone-related tumor progression.
HIF-1α and ERRα Crosstalk in Cancer, Especially in Endometrial Carcinoma
Metabolic reprogramming is essential for the survival of cancer cells in hypoxia and nutrient deficient environments. The surviving cancer cells have higher metabolic plasticity (92) and show more malignant biological behaviors such as invasiveness and chemotherapy resistance. The glycolytic activity of EC cells is considerably higher than that of non-tumor cells and contributes to the progression of EC (93). Therefore, it is very important to screen metabolic genes related to the progression of EC and select targeted treatments to improve the prognosis of EC patients. Jiang et al. (94) used The Cancer Genome Atlas database to analyze the expression of metabolism-related genes (MRGs). In this way, they could screen for differentially expressed MRGs (DE-MRGs) significantly related to the prognosis of EC patients. Functional enrichment analysis of DE-MRGs showed that most of these MRGs were enriched in amino acid, glycolysis, and glycerol phospholipid metabolism.
It is known that HIF-1α regulates a large group of genes/proteins involved in cell metabolism, pH, and EMT, thus making tumor cells more aggressive than that of other phenotypes (95). Hypoxia is related to angiogenesis in EC in which the transition from metabolism to aerobic glycolysis (Warburg effect) and tumor cell resistance to pyroptosis are very important for tumor growth (96). EC patients with tumors highly expressing HIF-1α exhibit a decreased disease-free survival. The expression of HIF-1α is an important prognostic factor in patients with EC and is related to myometrial invasion, histological grade, and recurrence after radiotherapy (59, 60). However, at present, no studies have examined the effect of differences in HIF-1α expression in relation to the different molecular types of EC. Furthermore, the exact mechanism underlying the role of HIF-1α in the malignant progression of EC is not clear. Byrne et al. (97) also found that glycolysis and lipogenesis are highly related to the malignant phenotype of EC. Inhibition of GLUT6 expression can inhibit glycolysis and the survival of EC cells, which reflects the key role of energy metabolism in tumor progression.
ERR is composed of ERRα, ERRβ, and ERRγ and is referred to as the central regulator of energy metabolism; its natural ligand has not been determined (98). An imbalance in ERRα activity can significantly affect cell metabolic homeostasis, causing metabolic disorders and cancer. In 2006, Sun et al. proposed that an imbalance in ERRα expression may be an important reason for the carcinogenesis of EC (99). Subsequently, when a lentiviral vector overexpressing ERRα was constructed and transfected into EC cells, the proliferation of EC cells was promoted (84, 100). XCT790 can effectively inhibit the expression of ERRα in EC. The expression of ERRα is closely related to the proliferation and apoptosis of EC cells (82). In 2018, it was proposed that ERRα is a key regulator of cell metabolism and plays an important role in gynecological endocrine-related tumors and energy metabolism (101). A knockout of ERRα can inhibit the invasion, metastasis, and angiogenesis of EC and promote apoptosis (83, 102). Endogenous or exogenous inhibition of the expression and function of ERRα has obvious anticancer effects (82). ERRα is also expressed in BRCA, PCa, ovarian cancer, and other malignant tumors, and the increase in ERRα expression is positively correlated with the late progression of malignant tumors (103), indicating that ERRα plays a regulatory role in tumor growth. Xia et al. (104) used in vivo and in vitro experiments to regulate the activity of ERRα. Through integration of gene expression profiles and genome-wide chromatin immunoprecipitation techniques, they determined the key role of ERRα in lipid and carbohydrate metabolism and mitochondrial function under physiological and pathological conditions. Furthermore, they were able to clarify the targeting of ERRα as a new way to treat metabolic disorders and other related diseases. Although a high expression level of ERRα in EC indicates a poor prognosis, the specific mechanism of the role of ERRα in the progression of EC has not been elucidated.
Functional studies in BRCA cells showed that ERRα can promote cancer growth through a variety of transcriptional regulatory networks or mechanisms, including enhancing HIF-1-dependent hypoxic cell growth (86), activating vascular endothelial growth factor promoting tumor angiogenesis (105), and enhancing glycolysis producing the Warburg effect (79). Studies by Zou et al. (77) showed that the regulation of carcinogenicity by ERRα in PCa is a key hypoxic growth regulator and an important cofactor of HIF-1α in hypoxic microenvironments. ERRα enhances HIF-1α signal transduction by interacting with HIF-1α, which makes ERRα-overexpressing PCa cells better adapted to a hypoxic microenvironment.
The activation of ERRs involves genes related to mitochondrial biogenesis and OXPHOS. However, this enhanced mitochondrial oxidation capacity is mainly targeted at fatty-acid metabolism because ERRs upregulate PDK4 preventing glucose oxidation. Since HIF-1α can activate PDK1 (106), it is essential for the transformation from glucose oxidation via the tricarboxylic acid cycle to glycolytic metabolism under hypoxic conditions. This shared function of inhibiting glucose respiratory metabolism may represent the internal relationship between ERRα and HIF-1α. The change in glucose and lipid metabolism is an important feature of the occurrence and development of EC. Combined with the regulation of glucose and lipid metabolism by ERRα and HIF-1α, the regulation of HIF-1α/ERRα may be a means to inhibit malignant progression, such as invasion and metastasis, of EC.
The Involvement of Pyroptosis in Endometrial Carcinoma
Cell resistance to pyroptosis is very important for tumor growth. Pyroptosis is widely involved in the occurrence and development of tumors, infectious diseases, metabolic diseases, nervous system-related diseases, and atherosclerotic diseases, but its specific regulatory mechanism is not clear (107). NOD-like receptor family, pyrin domain-containing protein 3 (NLRP3) activation of inflammatory bodies can activate caspase-1, which in turn, mediates the conversion of gasdermin D (GSDMD) and pro-IL-1β into active forms. GSDMD is a key effector of pyroptosis, forming pores in the plasma membrane that eventually leads to cell expansion and membrane dissolution. Pyroptosis resistance can inhibit anti-tumor immunity and promote the development of many types of cancers. Pyroptosis induces the production of inflammatory factors, such as IL-1 β, which act as alarm signals to activate and recruit immune cells to mediate the immune response. The upregulation of GSDMD protein induces the infiltration of M1 macrophages, CD4+, CD8+T lymphocytes, and other immune cells, and increases the sensitivity of cells to anti-PD-1 monoclonal antibody (108).
EC patients with POLE mutations and MSI exhibited high expression of PD-1 and PD-L1, accompanied by a large number of tumor-infiltrating lymphocytes, which indicates their suitability for immunotherapy (109). Liang et al. (110) found pyroptosis-related lncRNAs in TCGA database, which can be used to predict the prognosis and suitability for immunotherapy in EC. Pyroptosis-related genes in EC, such as GSDMD, are involved in the Wnt signaling and the substance metabolism pathways (111). Pyroptosis is closely related to the proliferation, invasion, and metastasis of cancer cells and can affect the efficacy of chemotherapy (112). However, the mechanisms by which tumor cells promote pyroptosis resistance by remodeling the energy metabolism need more exploration and confirmation, both in vivo and in vitro.
At present, there are various targeted therapies for EC. For example, anti-angiogenic drugs, such as bevacizumab, combined with vascular endothelial growth factor (VEGF), are used to inhibit tumor growth and metastasis (113). Abnormal activation of the PI3K/AKt/mTOR pathway is related to tumor metabolism, cell growth, invasion and migration, and angiogenesis. As a rapamycin analogue, ridaforolimus can effectively inhibit mTOR, thus inhibiting tumor growth and improving the progression-free survival of EC patients (114, 115). Immunotherapy, such as the pembrolizumab anti-PD-1 monoclonal antibody, can inhibit tumor progression (116). Some scholars have suggested that tumors with a mutation in the POLE gene can exhibit the differential regulation of tumor cell metabolism through glucose metabolism (117). Targeted therapy for EC provides a new treatment direction for advanced and recurrent EC. The clinical effects of chemotherapy in EC patients are different. Chemotherapy resistance is mediated by bypassing pyroptosis (108). The inhibition of ERRα enhances the chemotherapeutic effect in tumors (67). However, whether ERRα inhibition can promote chemotherapy resistance in tumor cells by blocking mitochondrial respiration, increasing ROS production, and then inducing cell pyroptosis needs further research. By adding targeted therapy, especially targeting a specific cell metabolic process, to chemotherapy, the survival of EC can be improved.
Hypoxia can lead to malignant progression of tumor cells through the following mechanisms: inhibiting the activity of immune cells, providing energy support for tumor cells, improving immune resistance of tumor cells, inhibiting apoptosis of tumor cells, and promoting metastasis of tumor cells (118, 119). ERRα cooperates with HIF-1α to regulate angiogenesis and glycolysis, thus promoting the growth of tumor cells under hypoxia, while stable HIF-1α further increases the expression of ERRα at the transcriptional level (77). HIF-1α/ERRα interaction promotes the adaptation of tumor cells to hypoxia, suggesting that there is a positive circuit among HIF-1α, ERRα, and mitochondrial biogenesis. However, in regard to the hypoxic microenvironment formed by the rapid proliferation of tumor cells, there have been studies on the effects of HIF-1α/ERRα interactions with glycolysis and angiogenesis. Whether EC cells with high expression of ERRα show enhanced anti-pyroptosis properties through interactions with HIF-1α, or whether they can show an anti-pyroptosis effect independent of HIF-1α, remains to be further studied. The effect of HIF-1α and ERRα on tumor microenvironment remodeling and chemotherapy resistance in EC is not clear, and their interaction may be an important mechanism for EC cells to resist pyroptosis (Figure 2).
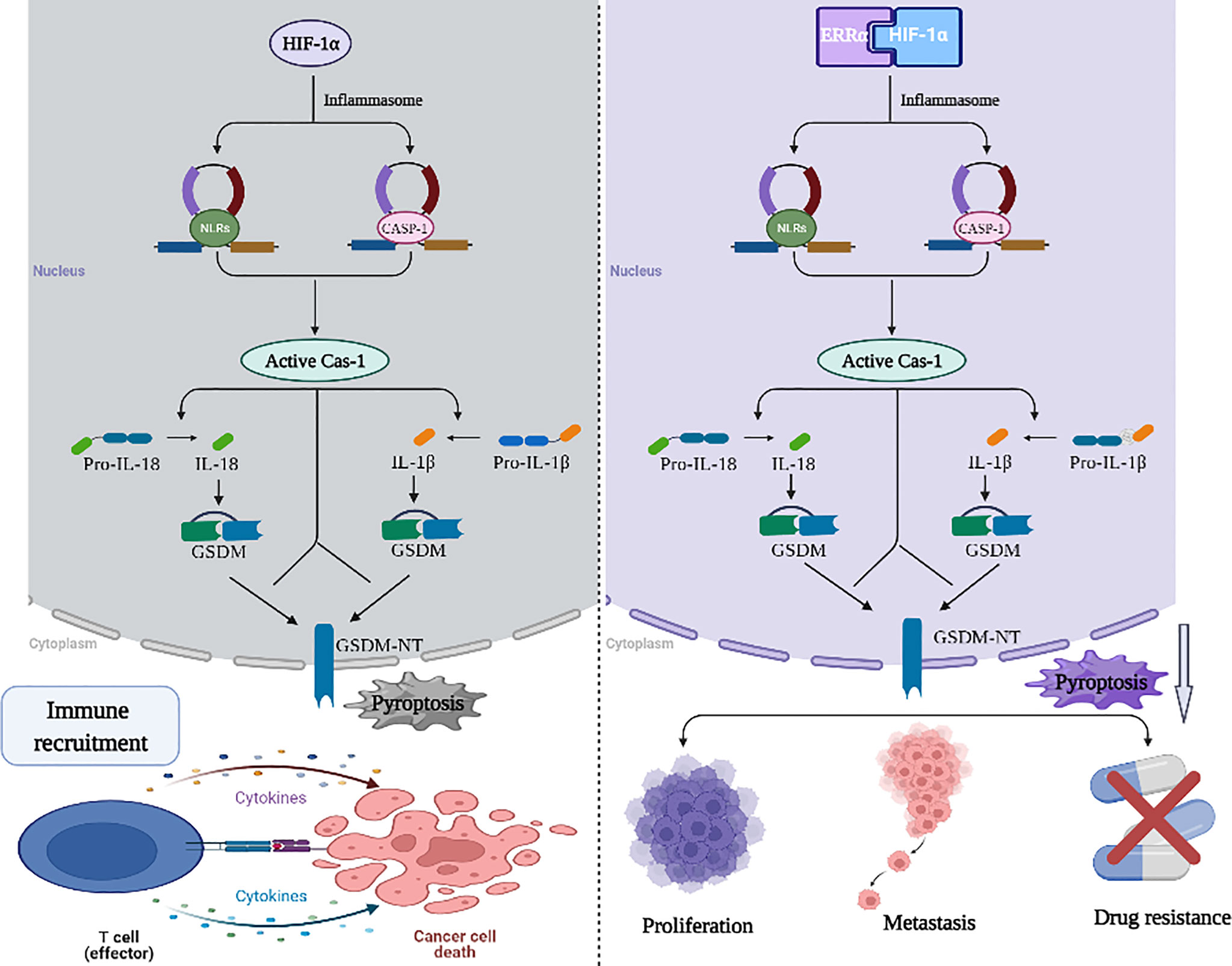
Figure 2 Working model of the role of HIF-1α/ERRα in pyroptosis resistance. HIF-1 α is a component of the molecular mechanism of pyroptosis mediated by NLRP3 inflammatory bodies, which can recruit immune cells to mediate the immune response. HIF-1α/ERRα interaction promotes the adaptation of tumor cells to hypoxia and promotes cancer cell resistance to pyroptosis. These activities stimulate tumor proliferation, metastasis, and drug resistance. Images were made in BioRender (Biorender.com).
Conclusion
EC is a gynecological disease accompanied by metabolic impairment, which requires management to improve the glucose and lipid metabolism of the patients, in addition to cancer treatment. Complications in EC patients can be resolved through a combined treatment strategy with inputs from gynecological oncology, nutrition, endocrinology, cardiology, and other disciplines. Tumor cells metabolically adapt to their microenvironment through metabolic reprogramming to meet their energy needs for proliferation and differentiation. Metabolic dysfunction in the cancer microenvironment can cause different outcomes. MRGs can be used as prognostic markers of tumors. Inhibition of hypoxia-induced metabolic pathways may be a new and promising therapeutic strategy. In this review, we presented evidence for the regulatory effect of HIF-1α and ERRα on MRGs through various signaling pathways in malignant tumors, thus changing the energy metabolism of tumor cells and causing tumor cell resistance to pyroptosis, clarifying the role of key genes in metabolic pathways. With continued exploration of natural endogenous ERRα ligands and understanding of the regulation of HIF-1α, the key goal in the future is to develop drugs that regulate the transcriptional activity of HIF-1α/ERRα to prevent and treat metabolism-related malignant tumors and other diseases. A future challenge for cancer researchers is to transform basic research into clinical application by designing clinical trials based on the molecular characteristics of EC, to study novel drugs for targeted monotherapy, or in combination with existing cytotoxic drugs.
Author Contributions
PPS carried out the primary literature search, drafted and revised the manuscript, tables, figures, and participated in discussions. PMS conceived the idea and edited the manuscript. LY helped edited the figures. XM helped modify the manuscript. All authors have read and agreed to the published version of the manuscript.
Funding
This research was supported by the Fund of Natural ScienceFoundation of Fujian Province, grant number 2020J02059 and 2021J01404, and Joint Funds for the Innovation of Science and Technology, Fujian Province (Grant no. 2020Y9160).
Conflict of Interest
The authors declare that the research was conducted in the absence of any commercial or financial relationships that could be construed as a potential conflict of interest.
Publisher’s Note
All claims expressed in this article are solely those of the authors and do not necessarily represent those of their affiliated organizations, or those of the publisher, the editors and the reviewers. Any product that may be evaluated in this article, or claim that may be made by its manufacturer, is not guaranteed or endorsed by the publisher.
Acknowledgments
The authors would thank Guanyu Ruan and Lixiang Huang for comments and advice on the manuscript.
Abbreviations
HIF-1α, Hypoxia inducible factor-1α; ERRα, Estrogen-related receptors α; EC, Endometrial carcinoma; MSI, Microsatellite-instable, CNL, Copy number low; CNH, Copy number high; EMT, Epithelial mesenchymal transformation; BRCA, Breast cancer; PCa, Prostate cancer; OXPHOS, Oxidative phosphorylation; NSCLC, Non-small cell lung cancer cells; EGFR, Epidermal growth factor receptor; HCC, Hepatocellular carcinoma; LDHA, Lactate dehydrogenase A; PGK1, Phosphoglycerate kinase 1; HK2, Kallikrein 2; ENO1, Enolase 1-α; PDK4, Pyruvate dehydrogenase kinase 4; PGC-1α, Peroxisome proliferator-activated receptor gamma coactivator 1-alpha; ROS, Reactive oxygen species metabolism-related genes; DE-MRGs, Differentially expressed metabolism-related genes; FABP, Fatty Acid Binding Protein; NLRP3, NOD-like receptor family, pyrin domain-containing protein 3; GSDMD, Gasdermin D; VEGF, Vascular endothelial growth factor.
References
1. Bray F, Ferlay J, Soerjomataram I, Siegel RL, Torre LA, Jemal A, et al. Global Cancer Statistics 2018: GLOBOCAN Estimates of Incidence and Mortality Worldwide for 36 Cancers in 185 Countries[J]. CA: A Cancer J Clin (2018) 68(6):394–424. doi: 10.3322/caac.21492
2. McAlpine JN, Temkin SM, Mackay HJ. Endometrial Cancer: Not Your Grandmother’s Cancer: Progress and Challenges Moving Forward[J]. Cancer (2016) 122(18):2787–98. doi: 10.1002/cncr.30094
3. Siegel RL, Miller KD, Jemal A. Cancer Statistics, 2020[J]. CA: A Cancer J Clin (2020) 70(1):7–30. doi: 10.3322/caac.21590
4. Siegel RL, Miller KD, Fuchs HE, Jemal A. Cancer Statistics, 2021[J]. CA: A Cancer J Clin (2021) 71(1):7–33. doi: 10.3322/caac.21654
5. Yang Y, Liu PY, Bao W, Chen SJ, Wu FS, Zhu PY, et al. Hydrogen Inhibits Endometrial Cancer Growth via a ROS/NLRP3/caspase-1/GSDMD-Mediated Pyroptotic Pathway[J]. BMC Cancer (2020) 20(1):28. doi: 10.1186/s12885-019-6491-6
6. Sanjida S, Obermair A, Gebski V, Armfield N, Janda M. Long-Term Quality of Life Outcomes of Women Treated for Early-Stage Endometrial Cancer[J]. Int J Gynecologic Cancer (2021) 31(4):530–6. doi: 10.1136/ijgc-2020-002145
7. Cianci S, Rosati A, Capozzi VA, Tarascio M, Uccella S, Palumbo M, et al. Quality of Life and Sexual Functioning of Patient Affected by Endometrial Cancer[J]. Minerva Med (2021) 112(1):81–95. doi: 10.23736/S0026-4806.20.07081-0
8. Bokhman JV. Two Pathogenetic Types of Endometrial Carcinoma[J]. Gynecologic Oncol (1983) 15(1):10–7. doi: 10.1016/0090-8258(83)90111-7
9. Lu KH, Broaddus RR. Endometrial Cancer[J]. D.L. Longo. New Engl J Med (2020) 383(21):2053–64. doi: 10.1056/NEJMra1514010
10. Liu Q, Chen C-Y, Chen G-L. High APOBEC1 Complementation Factor Expression Positively Modulates the Proliferation, Invasion, and Migration of Endometrial Cancer Cells Through Regulating P53/P21 Signaling Pathway[J]. Cancer Biotherapy Radiopharmaceuticals (2020):cbr.2020.3957. doi: 10.1089/cbr.2020.3957
11. Santoro A, Angelico G, Travaglino A, Inzani F, Arciuolo D, Valente M, et al. New Pathological and Clinical Insights in Endometrial Cancer in View of the Updated ESGO/ESTRO/ESP Guidelines[J]. Cancers (2021) 13(11):2623. doi: 10.3390/cancers13112623
12. Dondi G, Coluccelli S, De Leo A, Ferrari S, Gruppioni E, Bovicelli A, et al. An Analysis of Clinical, Surgical, Pathological and Molecular Characteristics of Endometrial Cancer According to Mismatch Repair Status. A Multidisciplinary Approach[J]. Int J Mol Sci (2020) 21(19):7188. doi: 10.3390/ijms21197188
13. Falzone L, Scandurra G, Lombardo V, Gattuso G, Lavoro A, Distefano A, et al. A Multidisciplinary Approach Remains the Best Strategy to Improve and Strengthen the Management of Ovarian Cancer (Review)[J]. Int J Oncol (2021) 59(1):53. doi: 10.3892/ijo.2021.5233
14. Yang X, Wang J. The Role of Metabolic Syndrome in Endometrial Cancer: A Review[J]. Front Oncol (2019) 9:744. doi: 10.3389/fonc.2019.00744
15. Wang Y, Dong C, Zhou BP. Metabolic Reprogram Associated With Epithelial-Mesenchymal Transition in Tumor Progression and Metastasis[J]. Genes Dis (2020) 7(2):172–84. doi: 10.1016/j.gendis.2019.09.012
16. Ohshima K, Morii E. Metabolic Reprogramming of Cancer Cells During Tumor Progression and Metastasis[J]. Metabolites (2021) 11(1):28. doi: 10.3390/metabo11010028
17. Hipólito A, Martins F, Mendes C, Lopes-Coelho F, Serpa J. Molecular and Metabolic Reprogramming: Pulling the Strings Toward Tumor Metastasis[J]. Front Oncol (2021) 11:656851. doi: 10.3389/fonc.2021.656851
18. Schito L, Semenza GL. Hypoxia-Inducible Factors: Master Regulators of Cancer Progression[J]. Trends Cancer (2016) 2(12):758–70. doi: 10.1016/j.trecan.2016.10.016
19. Miranda-Galvis M, Teng Y. Targeting Hypoxia-Driven Metabolic Reprogramming to Constrain Tumor Progression and Metastasis[J]. Int J Mol Sci (2020) 21(15):5487. doi: 10.3390/ijms21155487
20. Pezzuto A, Carico E. Role of HIF-1 in Cancer Progression: Novel Insights. A Review[J]. Curr Mol Med (2019) 18(6):343–51. doi: 10.2174/1566524018666181109121849
21. Ma J-H, Qi J, Lin S-Q, Zhang CY, Liu F, Xie WD, et al. STAT3 Targets ERR-α to Promote Epithelial–Mesenchymal Transition, Migration, and Invasion in Triple-Negative Breast Cancer Cells[J]. Mol Cancer Res (2019) 17(11):2184–95. doi: 10.1158/1541-7786.MCR-18-1194
22. Huang H, Li J, Shen J, Lin L, Wu X, Xiang S, et al. Increased ABCC4 Expression Induced by Errα Leads to Docetaxel Resistance via Efflux of Docetaxel in Prostate Cancer[J]. Front Oncol (2020) 10:1474. doi: 10.3389/fonc.2020.01474
23. Huang X, Ruan G, Liu G, Gao Y, Sun P. Immunohistochemical Analysis of PGC-1α and Errα Expression Reveals Their Clinical Significance in Human Ovarian Cancer[J]. OncoTargets Ther (2020) 13:13055–62. doi: 10.2147/OTT.S288332
24. Huang M, Chen L, Mao X, Liu G, Gao Y, You X, et al. ERRα Acts as a Potential Agonist of PPARγ to Induce Cell Apoptosis and Inhibit Cell Proliferation in Endometrial Cancer[J]. Aging (2020) 12(22):23029–046. doi: 10.18632/aging.104049
25. Gao. Different Biological Effect of Estrogen Receptor-Related Receptor α in Estrogen Receptor-Positive and -Negative Endometrial Carcinoma[J]. Mol Med Rep (2008) 1(6):917–24. doi: 10.3892/mmr_00000050
26. Chen L, Mao X, Huang M, Lei H, Xue L, Sun P. PGC-1α and Errα in Patients With Endometrial Cancer: A Translational Study for Predicting Myometrial Invasion[J]. Aging (2020) 12(17):16963–80. doi: 10.18632/aging.103611
27. De Vitto H, Bode AM, Dong Z. The PGC-1/ERR Network and its Role in Precision Oncology[J]. NPJ Precis Oncol (2019) 3(1):9. doi: 10.1038/s41698-019-0081-6
28. Victorino VJ, Barroso WA, Assunção AKM, Cury V, Jeremias IC, Petroni R, et al. PGC-1β Regulates HER2-Overexpressing Breast Cancer Cells Proliferation by Metabolic and Redox Pathways[J]. Tumor Biol (2016) 37(5):6035–44. doi: 10.1007/s13277-015-4449-0
29. Kida YS, Kawamura T, Wei Z, Sogo T, Jacinto S, Shigeno A, et al. ERRs Mediate a Metabolic Switch Required for Somatic Cell Reprogramming to Pluripotency[J]. Cell Stem Cell (2015) 16(5):547–55. doi: 10.1016/j.stem.2015.03.001
30. Raggi C, Taddei ML, Sacco E, Navari N, Correnti M, Piombanti B, et al. Mitochondrial Oxidative Metabolism Contributes to a Cancer Stem Cell Phenotype in Cholangiocarcinoma[J]. J Hepatol (2021) 74(6):1373–85. doi: 10.1016/j.jhep.2020.12.031
31. Sun L, Suo C, Li S, Zhang H, Gao P. Metabolic Reprogramming for Cancer Cells and Their Microenvironment: Beyond the Warburg Effect[J]. Biochim Biophys Acta (BBA) - Rev Cancer (2018) 1870(1):51–66. doi: 10.1016/j.bbcan.2018.06.005
32. Yang L, Li J, Li Y, Zhou Y, Wang Z, Zhang D, et al. Diclofenac Impairs the Proliferation and Glucose Metabolism of Triple−Negative Breast Cancer Cells by Targeting the C−Myc Pathway[J]. Exp Ther Med (2021) 21(6):584.
33. Gao S, Chen M, Wei W, Zhang X, Zhang M, Yao Y, et al. Crosstalk of mTOR/PKM2 and STAT3/c-Myc Signaling Pathways Regulate the Energy Metabolism and Acidic Microenvironment of Gastric Cancer[J]. J Cell Biochem (2019) 120(2):1193–202. doi: 10.1002/jcb.26915
34. Chen Z, Tian D, Liao X, Zhang Y, Xiao J, Chen W, et al. Apigenin Combined With Gefitinib Blocks Autophagy Flux and Induces Apoptotic Cell Death Through Inhibition of HIF-1α, C-Myc, P-EGFR, and Glucose Metabolism in EGFR L858R+T790M-Mutated H1975 Cells[J]. Front Pharmacol (2019) 10:260. doi: 10.3389/fphar.2019.00260
35. Alzahrani AS. PI3K/Akt/mTOR Inhibitors in Cancer: At the Bench and Bedside[J]. Semin Cancer Biol (2019) 59:125–32. doi: 10.1016/j.semcancer.2019.07.009
36. Jiang S, Shi F, Lin H, Ying Y, Luo L, Huang D, et al. Inonotus Obliquus Polysaccharides Induces Apoptosis of Lung Cancer Cells and Alters Energy Metabolism via the LKB1/AMPK Axis[J]. Int J Biol Macromol (2020) 151:1277–86. doi: 10.1016/j.ijbiomac.2019.10.174
37. Zheng Y, Jin J, Gao Y, Luo C, Wu X, Liu J. Phospholipase Cϵ Regulates Prostate Cancer Lipid Metabolism and Proliferation by Targeting AMP-Activated Protein Kinase (AMPK)/Sterol Regulatory Element-Binding Protein 1 (SREBP-1) Signaling Pathway[J]. Med Sci Monitor (2020) 26:e924328.
38. Sun Y, Liu W, Zhao Q, Zhang R, Wang J, Pan P, et al. Down-Regulating the Expression of miRNA-21 Inhibits the Glucose Metabolism of A549/DDP Cells and Promotes Cell Death Through the PI3K/AKT/mTOR/HIF-1α Pathway[J]. Front Oncol (2021) 11:653596. doi: 10.3389/fonc.2021.653596
39. Akiyama-Abe A, Minaguchi T, Nakamura Y, Michikami H, Shikama A, Nakao S, et al. Loss of PTEN Expression Is an Independent Predictor of Favourable Survival in Endometrial Carcinomas[J]. Br J Cancer (2013) 109(6):1703–10. doi: 10.1038/bjc.2013.455
40. Philip C-A, Laskov I, Beauchamp M-C, Marques M, Amin O, Bitharas J, et al. Inhibition of PI3K-AKT-mTOR Pathway Sensitizes Endometrial Cancer Cell Lines to PARP Inhibitors[J]. BMC Cancer (2017) 17(1):638. doi: 10.1186/s12885-017-3639-0
41. Sun Y, Chen Y, Xu M, Liu C, Shang H, Wang C. Shenmai Injection Supresses Glycolysis and Enhances Cisplatin Cytotoxicity in Cisplatin-Resistant A549/DDP Cells via the AKT-mTOR-C-Myc Signaling Pathway[J]. BioMed Res Int (2020) 2020:1–10. doi: 10.1155/2020/9243681
42. Broadfield LA, Pane AA, Talebi A, Swinnen JV, Fendt SM. Lipid Metabolism in Cancer: New Perspectives and Emerging Mechanisms[J]. Dev Cell (2021) 56(10):1363–93. doi: 10.1016/j.devcel.2021.04.013
43. Ligorio F, Pellegrini I, Castagnoli L, Vingiani A, Lobefaro R, Zattarin E, et al. Targeting Lipid Metabolism is an Emerging Strategy to Enhance the Efficacy of Anti-HER2 Therapies in HER2-Positive Breast Cancer[J]. Cancer Lett (2021) 511:77–87. doi: 10.1016/j.canlet.2021.04.023
44. Peck B, Schulze A. Lipid Desaturation - the Next Step in Targeting Lipogenesis in Cancer?[J]. FEBS J (2016) 283(15):2767–78. doi: 10.1111/febs.13681
45. Bensaad K, Favaro E, Lewis CA, Peck B, Lord S, Collins JM, et al. Fatty Acid Uptake and Lipid Storage Induced by HIF-1α Contribute to Cell Growth and Survival After Hypoxia-Reoxygenation[J]. Cell Rep (2014) 9(1):349–65. doi: 10.1016/j.celrep.2014.08.056
46. Welte MA, Gould AP. Lipid Droplet Functions Beyond Energy Storage[J]. Biochim Biophys Acta (BBA) - Mol Cell Biol Lipids (2017) 1862(10):1260–72. doi: 10.1016/j.bbalip.2017.07.006
47. Röhrig F, Schulze A. The Multifaceted Roles of Fatty Acid Synthesis in Cancer[J]. Nat Rev Cancer (2016) 16(11):732–49. doi: 10.1038/nrc.2016.89
48. Li Z, Liu H, Luo X. Lipid Droplet and its Implication in Cancer Progression[J]. Am J Cancer Res (2020) 10(12):4112–22.
49. Wright HJ, Hou J, Xu B, Cortez M, Potma EO, Tromberg BJ, et al. CDCP1 Drives Triple-Negative Breast Cancer Metastasis Through Reduction of Lipid-Droplet Abundance and Stimulation of Fatty Acid Oxidation[J]. Proc Natl Acad Sci (2017) 114(32):E6556–65. doi: 10.1073/pnas.1703791114
50. Blücher C, Zilberfain C, Venus T, Spindler N, Dietrich A, Burkhardt R, et al. Single Cell Study of Adipose Tissue Mediated Lipid Droplet Formation and Biochemical Alterations in Breast Cancer Cells[J]. Analyst (2019) 144(18):5558–70. doi: 10.1039/C9AN00816K
51. Guštin E, Jarc E, Kump A, Petan T. Lipid Droplet Formation in HeLa Cervical Cancer Cells Depends on Cell Density and the Concentration of Exogenous Unsaturated Fatty Acids[J]. Acta Chimica Slovenica (2017), 549–54.
52. Mitra R, Le TT, Gorjala P, Goodman OB. Positive Regulation of Prostate Cancer Cell Growth by Lipid Droplet Forming and Processing Enzymes DGAT1 and ABHD5[J]. BMC Cancer (2017) 17(1):631. doi: 10.1186/s12885-017-3589-6
53. Mukherjee A, Chiang C-Y, Daifotis HA, Nieman KM, Fahrmann JF, Lastra RR, et al. Adipocyte-Induced FABP4 Expression in Ovarian Cancer Cells Promotes Metastasis and Mediates Carboplatin Resistance[J]. Cancer Res (2020) 80(8):1748–61. doi: 10.1158/0008-5472.CAN-19-1999
54. Kamphorst JJ, Cross JR, Fan J, de Stanchina E, Mathew R, White EP, et al. Hypoxic and Ras-Transformed Cells Support Growth by Scavenging Unsaturated Fatty Acids From Lysophospholipids[J]. Proc Natl Acad Sci (2013) 110(22):8882–7. doi: 10.1073/pnas.1307237110
55. Xie H, Simon MC. Oxygen Availability and Metabolic Reprogramming in Cancer[J]. J Biol Chem (2017) 292(41):16825–32. doi: 10.1074/jbc.R117.799973
56. Ackerman D, Tumanov S, Qiu B, Michalopoulou E, Spata M, Azzam A, et al. Triglycerides Promote Lipid Homeostasis During Hypoxic Stress by Balancing Fatty Acid Saturation[J]. Cell Rep (2018) 24(10):2596–2605.e5. doi: 10.1016/j.celrep.2018.08.015
57. Giatromanolaki A, Koukourakis MI, Sivridis E, Gatter KC, Harris AL, Banham AH. Loss of Expression and Nuclear/Cytoplasmic Localization of the FOXP1 Forkhead Transcription Factor are Common Events in Early Endometrial Cancer: Relationship With Estrogen Receptors and HIF-1α Expression[J]. Modern Pathol (2006) 19(1):9–16. doi: 10.1038/modpathol.3800494
58. Wincewicz A, Koda M, Sulkowska M, Kanczuga-Koda L, Sulkowski S. Comparison of STAT3 With HIF-1alpha, Ob and ObR Expressions in Human Endometrioid Adenocarcinomas[J]. Tissue Cell (2008) 40(6):405–10. doi: 10.1016/j.tice.2008.04.004
59. Seeber LM, Horrée N, van der Groep P, van derWall E, Verheijen RH, van Diest PJ. Necrosis Related HIF-1α Expression Predicts Prognosis in Patients With Endometrioid Endometrial Carcinoma[J]. BMC Cancer (2010) 10(1):307.
60. Yeramian A, Santacana M, Sorolla A, Llobet D, Encinas M, Velasco A, et al. Nuclear Factor-κb2/P100 Promotes Endometrial Carcinoma Cell Survival Under Hypoxia in a HIF-1α Independent Manner[J]. Lab Invest (2011) 91(6):859–71. doi: 10.1038/labinvest.2011.58
61. Ai Z, Lu Y, Qiu S, Ai Z, Lu Y, Qiu S, et al. Overcoming Cisplatin Resistance of Ovarian Cancer Cells by Targeting HIF-1-Regulated Cancer Metabolism[J]. Cancer Lett (2016) 373(1):36–44. doi: 10.1016/j.canlet.2016.01.009
62. Triantafyllou E-A, Georgatsou E, Mylonis I, Triantafyllou E-A, Georgatsou E, Mylonis I, et al. Expression of AGPAT2, an Enzyme Involved in the Glycerophospholipid/Triacylglycerol Biosynthesis Pathway, is Directly Regulated by HIF-1 and Promotes Survival and Etoposide Resistance of Cancer Cells Under Hypoxia[J]. Biochim Biophys Acta (BBA) - Mol Cell Biol Lipids (2018) 1863(9):1142–52. doi: 10.1016/j.bbalip.2018.06.015
63. Gong W, Ekmu B, Wang X, Lu Y, Wan L. AGR2-Induced Glucose Metabolism Facilitated the Progression of Endometrial Carcinoma via Enhancing the MUC1/HIF-1α Pathway[J]. Hum Cell (2020) 33(3):790–800. doi: 10.1007/s13577-020-00356-4
64. Gao T, Zhang X, Zhao J, Zhou F, Wang Y, Zhao Z, et al. SIK2 Promotes Reprogramming of Glucose Metabolism Through PI3K/AKT/HIF-1α Pathway and Drp1-Mediated Mitochondrial Fission in Ovarian Cancer[J]. Cancer Lett (2020) 469:89–101. doi: 10.1016/j.canlet.2019.10.029
65. Bernatchez G, Giroux V, Lassalle T, Carpentier AC, Rivard N, Carrier JC. Errα Metabolic Nuclear Receptor Controls Growth of Colon Cancer Cells[J]. Carcinogenesis (2013) 34(10):2253–61. doi: 10.1093/carcin/bgt180
66. Zhang Y, Ma K, Sadana P, Chowdhury F, Gaillard S, Wang F, et al. Estrogen-Related Receptors Stimulate Pyruvate Dehydrogenase Kinase Isoform 4 Gene Expression[J]. J Biol Chem (2006) 281(52):39897–906. doi: 10.1074/jbc.M608657200
67. Zhou S, Xia H, Xu H, Tang Q, Nie Y, Gong QY, et al. Errα Suppression Enhances the Cytotoxicity of the MEK Inhibitor Trametinib Against Colon Cancer Cells[J]. J Exp Clin Can R: CR (2018) 37(1):218.
68. Patch RJ, Huang H, Patel S, Cheung W, Xu G, Zhao BP, et al. Indazole-Based Ligands for Estrogen-Related Receptor α as Potential Anti-Diabetic Agents[J]. Eur J Medicinal Chem (2017) 138:830–53. doi: 10.1016/j.ejmech.2017.07.015
69. Chen C, Li Y, Zeng N, He L, Zhang X, Tu T, et al. Inhibition of Estrogen-Related Receptor α Blocks Liver Steatosis and Steatohepatitis and Attenuates Triglyceride Biosynthesis[J]. Am J Pathol (2021) 191(7):1240–54. doi: 10.1016/j.ajpath.2021.04.007
70. Yao L, Chen S, Li W. Fatostatin Inhibits the Development of Endometrial Carcinoma in Endometrial Carcinoma Cells and a Xenograft Model by Targeting Lipid Metabolism[J]. Arch Biochem Biophysics (2020) 684:108327. doi: 10.1016/j.abb.2020.108327
71. Deblois G, Giguère V. Oestrogen-Related Receptors in Breast Cancer: Control of Cellular Metabolism and Beyond[J]. Nat Rev Cancer (2013) 13(1):27–36. doi: 10.1038/nrc3396
72. Audet-walsh É, Giguére V. The Multiple Universes of Estrogen-Related Receptor α and γ in Metabolic Control and Related Diseases[J]. Acta Pharmacologica Sin (2015) 36(1):51–61. doi: 10.1038/aps.2014.121
73. Deblois G, St-Pierre J, Giguère V. The PGC-1/ERR Signaling Axis in Cancer[J]. Oncogene (2013) 32(30):3483–90. doi: 10.1038/onc.2012.529
74. Fujimoto J, Sato E. Clinical Implication of Estrogen-Related Receptor (ERR) Expression in Uterine Endometrial Cancers[J]. J Steroid Biochem Mol Biol (2009) 116(1–2):71–5. doi: 10.1016/j.jsbmb.2009.04.012
75. McGuirk S, Gravel S-P, Deblois G, Papadopoli DJ, Faubert B, Wegner A, et al. PGC-1α Supports Glutamine Metabolism in Breast Cancer[J]. Cancer Metab (2013) 1(1):22.
76. Deblois G, Smith HW, Tam IS, Gravel SP, Caron M, Savage P, et al. Errα Mediates Metabolic Adaptations Driving Lapatinib Resistance in Breast Cancer[J]. Nat Commun (2016) 7(1):12156.
77. Zou C, Yu S, Xu Z, Wu D, Ng CF, Yao X, et al. ERR α Augments HIF-1 Signalling by Directly Interacting With HIF-1 α in Normoxic and Hypoxic Prostate Cancer Cells: Errα and HIF-1α in Hypoxic Growth of Prostate Cancer[J]. J Pathol (2014) 233(1):61–73. doi: 10.1002/path.4329
78. Matsushima H, Mori T, Ito F, Yamamoto T, Akiyama M, Kokabu T, et al. Anti-Tumor Effect of Estrogen-Related Receptor Alpha Knockdown on Uterine Endometrial Cancer[J]. Oncotarget (2016) 7(23):34131–48. doi: 10.18632/oncotarget.9151
79. Park S, Chang C-Y, Safi R, Liu X, Baldi R, Jasper JS, et al. Errα-Regulated Lactate Metabolism Contributes to Resistance to Targeted Therapies in Breast Cancer[J]. Cell Rep (2016) 15(2):323–35. doi: 10.1016/j.celrep.2016.03.026
80. Audet-Walsh É, Papadopoli DJ, Gravel S-P, Yee T, Bridon G, Caron M, et al. The PGC-1α/Errα Axis Represses One-Carbon Metabolism and Promotes Sensitivity to Anti-Folate Therapy in Breast Cancer[J]. Cell Rep (2016) 14(4):920–31. doi: 10.1016/j.celrep.2015.12.086
81. Huang X, Wang X, Shang J, Zhang Z, Cui B, Lin Y, et al. Estrogen Related Receptor Alpha Triggers the Migration and Invasion of Endometrial Cancer Cells via Up Regulation of TGFB1[J]. Cell Adhesion Migration (2018) 12(6):538–47. doi: 10.1080/19336918.2018.1477901
82. Sun P, Mao X, Gao M, Huang M, Chen L, Ruan G, et al. Novel Endocrine Therapeutic Strategy in Endometrial Carcinoma Targeting Estrogen-Related Receptor & Alpha; by XCT790 and siRNA[J]. Cancer Manage Res (2018) 10:2521–35. doi: 10.2147/CMAR.S168043
83. Kokabu T, Mori T, Matsushima H, Yoriki K, Kataoka H, Tarumi Y, et al. Antitumor Effect of XCT790, an Errα Inverse Agonist, on Erα-Negative Endometrial Cancer Cells[J]. Cell Oncol (2019) 42(2):223–35. doi: 10.1007/s13402-019-00423-5
84. Mao X, Dong B, Gao M, Ruan G, Huang M, Braicu EI, et al. Dual Targeting of Estrogen Receptor α and Estrogen-Related Receptor α: A Novel Endocrine Therapy for Endometrial Cancer[J]. OncoTargets Ther (2019) 12:6757–67. doi: 10.2147/OTT.S216146
85. Yoriki K, Mori T, Kokabu T, Matsushima H, Umemura S, Tarumi Y, et al. Estrogen-Related Receptor Alpha Induces Epithelial-Mesenchymal Transition Through Cancer-Stromal Interactions in Endometrial Cancer[J]. Sci Rep (2019) 9(1):6697. doi: 10.1038/s41598-019-43261-z
86. Park S, Safi R, Liu X, Baldi R, Liu W, Liu J, et al. Inhibition of Errα Prevents Mitochondrial Pyruvate Uptake Exposing NADPH-Generating Pathways as Targetable Vulnerabilities in Breast Cancer[J]. Cell Rep (2019) 27(12):3587–3601.e4. doi: 10.1016/j.celrep.2019.05.066
87. Schoepke E, Billon C, Haynes KM, Avdagic A, Sitaula S, Sanders R, et al. A Selective Errα/γ Inverse Agonist, SLU-PP-1072, Inhibits the Warburg Effect and Induces Apoptosis in Prostate Cancer Cells[J]. ACS Chem Biol (2020) 15(9):2338–45. doi: 10.1021/acschembio.0c00670
88. Brindisi M, Fiorillo M, Frattaruolo L, Sotgia F, Lisanti MP, Cappello AR. Cholesterol and Mevalonate: Two Metabolites Involved in Breast Cancer Progression and Drug Resistance Through the Errα Pathway[J]. Cells (2020) 9(8):1819. doi: 10.3390/cells9081819
89. Ghanbari F, Mader S, Philip A. Cholesterol as an Endogenous Ligand of Errα Promotes Errα-Mediated Cellular Proliferation and Metabolic Target Gene Expression in Breast Cancer Cells[J]. Cells (2020) 9(8):1765.
90. Li X, Zhang K, Hu Y, Luo N. Errα Activates SHMT2 Transcription to Enhance the Resistance of Breast Cancer to Lapatinib via Modulating the Mitochondrial Metabolic Adaption[J]. Biosci Rep (2020) 40(1):BSR20192465.
91. Ghanbari F, Fortier A-M, Park M, Philip A. Cholesterol-Induced Metabolic Reprogramming in Breast Cancer Cells Is Mediated via the Errα Pathway[J]. Cancers (2021) 13(11):2605.
92. Ma L, Tao Y, Duran A, Llado V, Galvez A, Barger JF, et al. Control of Nutrient Stress-Induced Metabolic Reprogramming by Pkcζ in Tumorigenesis[J]. Cell (2013) 152(3):599–611. doi: 10.1016/j.cell.2012.12.028
93. Abdelazeem KNM, Singh Y, Lang F, Salker MS. Negative Effect of Ellagic Acid on Cytosolic pH Regulation and Glycolytic Flux in Human Endometrial Cancer Cells[J]. Cell Physiol Biochem (2017) 41(6):2374–82. doi: 10.1159/000475655
94. Jiang P, Sun W, Shen N, Huang X, Fu S. Identification of a Metabolism-Related Gene Expression Prognostic Model in Endometrial Carcinoma Patients[J]. BMC Cancer (2020) 20(1):864. doi: 10.1186/s12885-020-07345-8
95. Joseph JP, Harishankar MK, Pillai AA, Devi A. Hypoxia Induced EMT: A Review on the Mechanism of Tumor Progression and Metastasis in OSCC[J]. Oral Oncol (2018) 80:23–32. doi: 10.1016/j.oraloncology.2018.03.004
96. Sivridis E, Giatromanolaki A, Gatter KC, Harris AL, Koukourakis MI, Tumor and Angiogenesis Research Group. Association of Hypoxia-Inducible Factors 1? And 2? With Activated Angiogenic Pathways and Prognosis in Patients With Endometrial Carcinoma[J]. Cancer (2002) 95(5):1055–63. doi: 10.1002/cncr.10774
97. Caruana BT, Byrne FL. The NF-κb Signalling Pathway Regulates GLUT6 Expression in Endometrial Cancer[J]. Cell Signalling (2020) 73:109688. doi: 10.1016/j.cellsig.2020.109688
98. Villena JA. New Insights Into PGC-1 Coactivators: Redefining Their Role in the Regulation of Mitochondrial Function and Beyond[J]. FEBS J (2015) 282(4):647–72. doi: 10.1111/febs.13175
99. Sun P, Wei L, Denkert C, Lichtenegger W, Sehouli J. The Orphan Nuclear Receptors, Estrogen Receptor-Related Receptors: Their Role as New Biomarkers in Gynecological Cancer[J]. Anticancer Res (2006) 26(2C):1699–706.
100. Gao M, Sun P, Wang J, Zhao D, Wei L. Expression of Estrogen Receptor-Related Receptor Isoforms and Clinical Significance in Endometrial Adenocarcinoma[J]. Int J Gynecological Cancer (2006) 16(2):827–33. doi: 10.1111/j.1525-1438.2006.00527.x
101. Liu G, Sun P, Dong B, Sehouli J. Key Regulator of Cellular Metabolism, Estrogen-Related Receptor & Alpha; a New Therapeutic Target in Endocrine-Related Gynecological Tumor[J]. Cancer Manage Res (2018) 10:6887–95. doi: 10.2147/CMAR.S182466
102. Mao X, Lei H, Yi T, Su P, Tang S, Tong Y, et al. Lipid Reprogramming Induced by the TFEB-Errα Axis Enhanced Membrane Fluidity to Promote EC Progression[J]. J Exp Clin Cancer Res (2022) 41(1):28.
103. Sun P, Sehouli J, Denkert C, Mustea A, Könsgen D, Koch I, et al. Expression of Estrogen Receptor-Related Receptors, A Subfamily of Orphan Nuclear Receptors, as New Tumor Biomarkers in Ovarian Cancer Cells[J]. J Mol Med (2005) 83(6):457–67. doi: 10.1007/s00109-005-0639-3
104. Xia H, Dufour CR, Giguère V. Errα as a Bridge Between Transcription and Function: Role in Liver Metabolism and Disease[J]. Front Endocrinol (2019) 10:206. doi: 10.3389/fendo.2019.00206
105. Fradet A, Sorel H, Bouazza L, Goehrig D, Dépalle B, Bellahcène A, et al. Dual Function of Errα in Breast Cancer and Bone Metastasis Formation: Implication of VEGF and Osteoprotegerin[J]. Cancer Res (2011) 71(17):5728–38. doi: 10.1158/0008-5472.CAN-11-1431
106. Martí-Díaz R, Montenegro MF, Cabezas-Herrera J, Goding CR, Rodríguez-López JN, Sánchez-del-Campo L. Acriflavine, A Potent Inhibitor of HIF-1α, Disturbs Glucose Metabolism and Suppresses ATF4-Protective Pathways in Melanoma Under Non-Hypoxic Conditions[J]. Cancers (2020) 13(1):102.
107. Wei R, Li S, Yu G, Guan X, Liu H, Quan J, et al. Deciphering the Pyroptosis-Related Prognostic Signature and Immune Cell Infiltration Characteristics of Colon Cancer[J]. Front Genet (2021) 12:755384. doi: 10.3389/fgene.2021.755384
108. Hsu S-K, Li C-Y, Lin I-L, Syue WJ, Chen YF, Cheng KC, et al. Inflammation-Related Pyroptosis, A Novel Programmed Cell Death Pathway, and its Crosstalk With Immune Therapy in Cancer Treatment[J]. Theranostics (2021) 11(18):8813–35. doi: 10.7150/thno.62521
109. Howitt BE, Shukla SA, Sholl LM, Ritterhouse LL, Watkins JC, Rodig S, et al. Association of Polymerase E–Mutated and Microsatellite-Instable Endometrial Cancers With Neoantigen Load, Number of Tumor-Infiltrating Lymphocytes, and Expression of PD-1 and PD-L1[J]. JAMA Oncol (2015) 1(9):1319. doi: 10.1001/jamaoncol.2015.2151
110. Liang D, Hu M, Tang Q, Huang M, Tang L. Nine Pyroptosis-Related lncRNAs are Identified as Biomarkers for Predicting the Prognosis and Immunotherapy of Endometrial Carcinoma[J]. Int J Gen Med (2021) 14:8073–85. doi: 10.2147/IJGM.S338298
111. Zhang X, Yang Q. A Pyroptosis-Related Gene Panel in Prognosis Prediction and Immune Microenvironment of Human Endometrial Cancer[J]. Front Cell Dev Biol (2021) 9:705828. doi: 10.3389/fcell.2021.705828
112. Tang Z, Ji L, Han M, Xie J, Zhong F, Zhang X, et al. Pyroptosis is Involved in the Inhibitory Effect of FL118 on Growth and Metastasis in Colorectal Cancer[J]. Life Sci (2020) 257:118065. doi: 10.1016/j.lfs.2020.118065
113. Aghajanian C, Sill MW, Darcy KM, Greer B, McMeekin DS, Rose PG, et al. Phase II Trial of Bevacizumab in Recurrent or Persistent Endometrial Cancer: A Gynecologic Oncology Group Study[J]. J Clin Oncol (2011) 29(16):2259–65. doi: 10.1200/JCO.2010.32.6397
114. Mitamura T, Dong P, Ihira K, Kudo M, Watari H. Molecular-Targeted Therapies and Precision Medicine for Endometrial Cancer[J]. Japanese J Clin Oncol (2019) 49(2):108–20. doi: 10.1093/jjco/hyy159
115. Roncolato F, Lindemann K, Willson ML, Martyn J, Mileshkin L. PI3K/AKT/mTOR Inhibitors for Advanced or Recurrent Endometrial Cancer[J]. Cochrane Gynaecological, Neuro-Oncology and Orphan Cancer Group. Cochrane Database Systematic Rev (2019) 2019(10):CD012160. doi: 10.1002/14651858.CD012160.pub2
116. Ott PA, Bang Y-J, Berton-Rigaud D, Elez E, Pishvaian MJ, Rugo HS, et al. Safety and Antitumor Activity of Pembrolizumab in Advanced Programmed Death Ligand 1–Positive Endometrial Cancer: Results From the KEYNOTE-028 Study[J]. J OF Clin Oncol (2017) 35(22):2535–41.
117. Li Y, Bian Y, Wang K, Wan XP. POLE Mutations Improve the Prognosis of Endometrial Cancer via Regulating Cellular Metabolism Through AMF/AMFR Signal Transduction[J]. BMC Med Genet (2019) 20(1):202. doi: 10.1186/s12881-019-0936-2
118. Ge Y, Yoon S-H, Jang H, Jeong JH, Lee YM. Decursin Promotes HIF-1α Proteasomal Degradation and Immune Responses in Hypoxic Tumour Microenvironment[J]. Phytomedicine (2020) 78:153318. doi: 10.1016/j.phymed.2020.153318
Keywords: glucose metabolism, lipid metabolism, HIF-1α, ERRα, endometrial cancer
Citation: Su P, Yu L, Mao X and Sun P (2022) Role of HIF-1α/ERRα in Enhancing Cancer Cell Metabolism and Promoting Resistance of Endometrial Cancer Cells to Pyroptosis. Front. Oncol. 12:881252. doi: 10.3389/fonc.2022.881252
Received: 22 February 2022; Accepted: 25 May 2022;
Published: 21 June 2022.
Edited by:
Shaohua Xu, Tongji University, ChinaReviewed by:
Andraz Dovnik, Maribor University Medical Centre, SloveniaLuca Falzone, G. Pascale National Cancer Institute Foundation (IRCCS), Italy
Copyright © 2022 Su, Yu, Mao and Sun. This is an open-access article distributed under the terms of the Creative Commons Attribution License (CC BY). The use, distribution or reproduction in other forums is permitted, provided the original author(s) and the copyright owner(s) are credited and that the original publication in this journal is cited, in accordance with accepted academic practice. No use, distribution or reproduction is permitted which does not comply with these terms.
*Correspondence: Pengming Sun, sunfemy@hotmail.com