- 1State Key Laboratory for Diagnosis and Treatment of Infectious Diseases, National Clinical Research Center for Infectious Diseases, Collaborative Innovation Center for Diagnosis and Treatment of Infectious Diseases, The First Affiliated Hospital, College of Medicine, Zhejiang University, Hangzhou, China
- 2Department for BioMedical Research, Hepatology, University of Bern, Bern, Switzerland
- 3Department of Biomedical Science, Burrell College of Osteopathic Medicine, Las Cruces, NM, United States
The ketogenic diet (KD) is a low-carbohydrate, high-fat diet regarded as a potential intervention for cancers owing to its effects on tumor metabolism and behavior. Hepatocellular carcinoma (HCC) is the most prevalent type of liver cancer, and its management is worth investigating because of the high fatality rate. Additionally, as the liver is the glucose and lipid metabolism center where ketone bodies are produced, the application of KD to combat HCC is promising. Prior studies have reported that KD could reduce the energy supply and affect the proliferation and differentiation of cancer cells by lowering the blood glucose and insulin levels. Furthermore, KD can increase the expression of hydroxymethylglutaryl-CoA synthase 2 (HMGCS2) in hepatocytes and regulate lipid metabolism to inhibit the progression of HCC. In addition, β-hydroxybutyrate can induce histone hyperacetylation and reduce the expression of inflammatory factors to alleviate damage to hepatocytes. However, there are few relevant studies at present, and the specific effects and safety of KD on HCC warrant further research. Optimizing the composition of KD and combining it with other therapies to enhance its anti-cancer effects warrant further exploration.
Introduction
Liver cancer deaths account for the third-highest number of cancer-related fatalities worldwide and rank sixth in the number of new cancer cases, thereby exerting a substantial impact on human health and the economy (1). According to World Health Organization estimates, over one million individuals will die from liver cancer in 2030 (2). Hepatocellular carcinoma (HCC) accounts for 75%–85% of all liver cancer cases, and the risk factors vary from one region to another. In Asia, hepatitis B virus (HBV) and aflatoxin exposure are the major causes, whereas the hepatitis C virus is the leading cause in Europe. In addition, because of the differences in diet and lifestyle, non-alcoholic fatty liver disease (NAFLD) accounts for 10%–20% of the HCC cases in the United States (3). In past decades, therapies for HCC have witnessed rapid progress, with the main options being surgical resection, orthotopic liver transplantation, radio-frequency ablation, chemotherapy, radiotherapy, and systemic drugs such as sorafenib (2). However, the prognosis of patients with HCC remains poor, and more efficient treatments with fewer side effects have yet to be proposed (4). Cancer cells have different metabolic characteristics when compared with normal cells. While the normal cells obtain energy through glycolysis and the citric acid cycle (TCA/Krebs cycle), cancer cells undergo increased glycolysis and use most of the pyruvate generated through this process for lactate production even when oxygen is available (5). The Warburg effect is a characteristic metabolism in tumor cells, in which cells shift the source of ATP production from oxidative phosphorylation to rapid anaerobic glycolysis. Therefore, Warburg effect promotes glucose uptake, reduces the amount of ATP produced, but increases the rate of ATP production and substances required for cell growth, and prevents apoptosis and immune escape (6). The Warburg effect has encouraged many scientists to determine whether tumor growth can interfere with metabolic pathways. Accordingly, the ketogenic diet (KD), a low-carbohydrate, high-fat diet proposed in 1920 to treat intractable epilepsy was found to be capable of reducing glucose metabolism and increasing lipid metabolism, thereby interfering with the Warburg effect and preventing tumor cell growth. This diet was later proven to have promising anti-cancer effects (7).
The anti-cancer effect of KD has been demonstrated in several clinical studies conducted in many types of tumors, such as glioblastoma, advanced malignant astrocytoma, invasive rectal cancer, breast cancer, ovarian cancer, and endometrial cancer. Additionally, KD application has led to improvements in metabolic indicators, such as total cholesterol, low-density lipoprotein (LDL), and insulin levels in patients with cancer (8). KD has alleviated cachexia in patients and improved their quality of life (9, 10). Notably, a clinical study has reported adverse effects of hyperuricemia, but this effect was associated with low patient compliance (11). Most clinical studies have not documented any serious adverse events linked to KD. However, the effect of KD on tumors is also influenced by the tumor type (12). For example, unlike the anti-cancer effects observed in prior studies, a high-fat ketogenic diet was found to promote tumor growth in a mouse model of melanoma (13). As the main metabolic organ in the body, the liver plays a crucial role in the metabolism of carbohydrates, lipids, and proteins. Furthermore, the liver acts as the site of ketone body (KB) production, and abnormalities in liver function in patients with HCC can also have an impact on metabolism (14). Hydroxymethylglutaryl-CoA synthase 2 (HMGCS2) is the key enzyme in the ketogenic process, but is not expressed in fetal liver. However, it is activated in adult liver. Studies have found that low HMGCS2 expression is associated with advanced clinical stage and poor prognosis in HCC (15). Therefore, it is especially necessary to understand the potential therapeutic role of KD in HCC treatment. A study by Healy et al. noted that KD reduced the tumor load in HCC mice (16). However, according to Byrne’s research, KD had no significant effect on the progression of HCC in mice (17). In conclusion, the specific effects of KD on HCC are unclear. Hence, in this review, we have summarized the metabolism of KBs, the types of ketogenic diets, the possible mechanisms of action of KD on HCC, and the data obtained from current pre-clinical and clinical studies, with a hope of providing a strong theoretical basis for subsequent studies.
Anabolic and Catabolic Metabolism of Ketone Bodies
Liver mitochondria are the site of KB production; KB mainly includes acetoacetate (AcAc), β-hydroxybutyrate (β-OHB), and acetone (Ac). Under normal conditions, the blood ketone levels range from 0.1–0.2 mM. KB production increases during starvation, long-term exercise, or diabetes, and blood ketones can reach a level as high as 1 mM after 24 hours of fasting (18). First, fatty acids (FAs) are catabolized into acetyl-CoA through β-oxidation, which then enter the citric acid cycle and release energy. Nonetheless, in the low-glucose state, acetyl-CoA is used for KB synthesis. Two molecules of acetyl-CoA are condensed to form acetoacetyl-CoA (AcAc-CoA) by acetoacetyl-CoA thiolase (ACAT1) and AcAc-CoA is used in the generation of β-hydroxy-β-methylglutaryl-CoA (HMG-CoA) by HMG-CoA synthase (HMGCS2), the rate-limiting enzyme in ketogenesis. Subsequently, HMG-CoA lyase degrades HMG-CoA into AcAc, which is further reduced to β-OHB under the action of β-hydroxybutyrate dehydrogenase (BDH). The above two KBs are the main forms used by the body. A fraction of the AcAc spontaneously undergoes decarboxylation to form acetone, which is excreted through urine or respiration, or is further metabolized into pyruvate, lactic acid, and acetic acid (19).
The liver can not oxidize KBs. Therefore, the KBs produced in the liver are transported through the bloodstream to be oxidized and utilized in extrahepatic tissues such as the heart, kidney, brain, and skeletal muscle. The β-OHB in the bloodstream is taken up by the mitochondria through the monocarboxylate transporter protein (MCT), and is then dehydrogenated to produce AcAc in the presence of BDH1. The key enzyme 3-oxoacid transferase 1 (OXCT1), also called succinyl CoA transferase (SCOT), converts AcAc and succinyl CoA to AcAc-CoA (20). AcAc-CoA is then converted to two molecules of acetyl CoA by the action of ACAT1. Finally, acetyl CoA is either oxidized and utilized via the TCA cycle or it is used for cholesterol synthesis (21) (Figure 1).
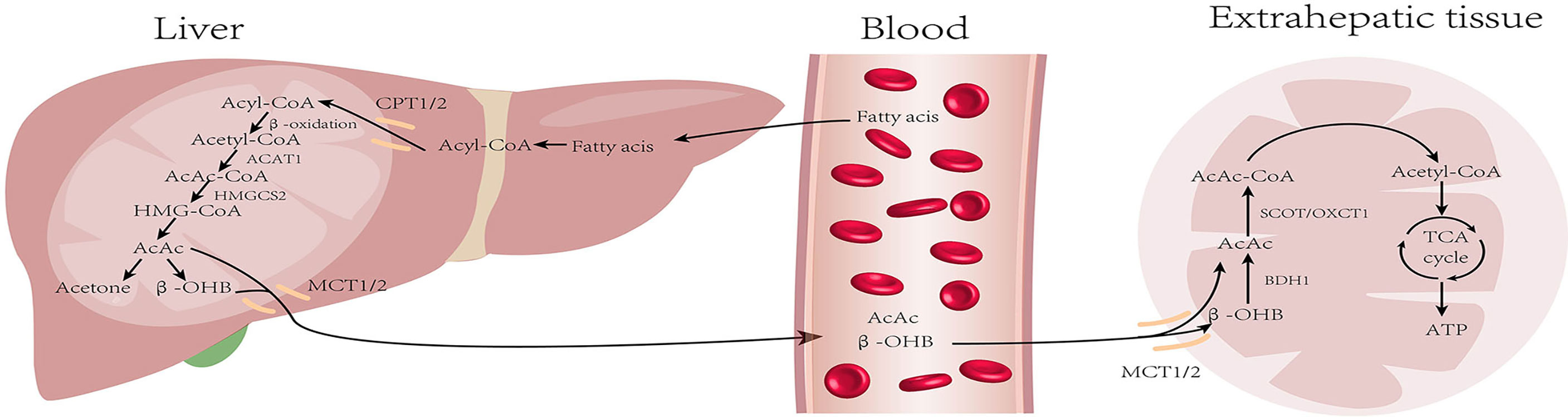
Figure 1 Metabolism of ketone bodies: During starvation and prolonged exercise, lipid hydrolysis is enhanced and fatty acid production increases. First, fatty acids (FAs) are activated into acyl-CoA and then decomposed into acetyl-CoA through β-oxidation. Acetyl-CoA is converted into ketone bodies in mitochondria within hepatocytes. Then, ketone bodies are transported by blood and utilized by extrahepatic tissues (heart, brain, kidney, and skeletal muscle) to regenerate acetyl-CoA. Finally, acetyl-CoA is oxidized through the tricarboxylic acid cycle for energy supply. (CPT, carnitin-palmitoyl transferase; MCT, monocarboxylate transporter).
Ketogenic Diet
The classical KD consists of a 4:1 or 3:1 ratio of fat content to carbohydrate plus protein, and requires all ingredients to be calculated and weighed precisely in grams (22). The fatty acids in the classical KD are long-chain triglycerides (LCT), which must be converted in the body before absorption. Later, Huttenlocher et al. proposed the medium-chain triglyceride ketogenic diet (MKD), which is more easily digested and absorbed than LCT, as medium-chain triglycerides (MCT) do not require emulsification, and can be directly degraded into glycerol and water-soluble medium-chain fatty acids (MCFA) (23). However, because the main component of the classical KD and MKD is fat, patients often experience some gastrointestinal disturbances, such as diarrhea and vomiting; hence, compliance is poor (24). Therefore, researchers have recommended a modified Atkins diet (MAD) and low glycemic index therapy (LGIT) ketogenic diet based on the classical KD (25–31) (Table 1).
Potential Effects of KD on Hepatocellular Carcinoma
Regulation of Glucose Metabolism
Tumor cells are characterized by rapid growth and high metabolism, and glucose transport protein (GLUT) aids in the entry of glucose into the cells for metabolism to enable cell growth. It has been found that GLUT1 expression is enhanced in HCC and exerts tumorigenic effects (32). Experiments on HCC mice revealed that the tumor incidence was 3–5 times higher in mice fed with a high-glucose diet when compared with those in the normal diet group (33). These findings indicate that glucose metabolism is significant for the development of HCC. In the absence of glucose availability, normal cells synthesize KBs in the mitochondria to supply energy for maintaining cell growth. However, tumor cells cannot perform ketone metabolism because of abnormalities in mitochondrial structure and function (34) (Figure 2). Moreover, the activities of SCOT and ACAT1, key enzymes in ketone metabolism, were low or undetectable in the tumor tissues, which confirmed the above idea (35). KD is a low-carbohydrate diet that reduces the energy supply to the cancer cells by lowering blood glucose levels in the body to exert anti-cancer effects. Clinical trials have demonstrated that insulin and insulin-like growth factor-1 (IGF-1) levels decreased with the consumption of KD for a period of time, and were negatively correlated with serum β-hydroxybutyric acid levels (36, 37). Indeed, insulin and IGF-1 play crucial regulatory roles in human growth and metabolism, and epidemiological evidence demonstrates that they can promote tumorigenesis and progression by augmenting tumor cell proliferation, migration, and angiogenesis (38). In addition, an HCC mouse trial showed a positive correlation between tumor load and post-prandial serum insulin levels (16). The phosphoinositide 3-kinase (PI3K) pathway is an intracellular signal transduction pathway that promotes cell proliferation and differentiation. Several inhibitors targeting this pathway are presently being investigated for the inhibition of tumor growth. However, clinical data allude that drugs targeting PI3K can cause adverse effects, such as hyperglycemia, thereby reactivating the PI3K pathway and diminishing the therapeutic effect (39). To address this issue, Hopkins et al. performed experiments on mouse tumor models. They found that the use of PI3K inhibitors in conjunction with a ketogenic diet inhibited the insulin feedback-induced activation of the mTORC1 pathway by lowering insulin levels, thereby enhancing the efficacy of PI3K inhibitors, resulting in an anti-tumor effect (40). The above-mentioned results suggest that KD can exert anti-cancer effects by regulating the process of glucose metabolism to reduce the energy supply of cells, thus inhibiting the growth of the cancer cells.
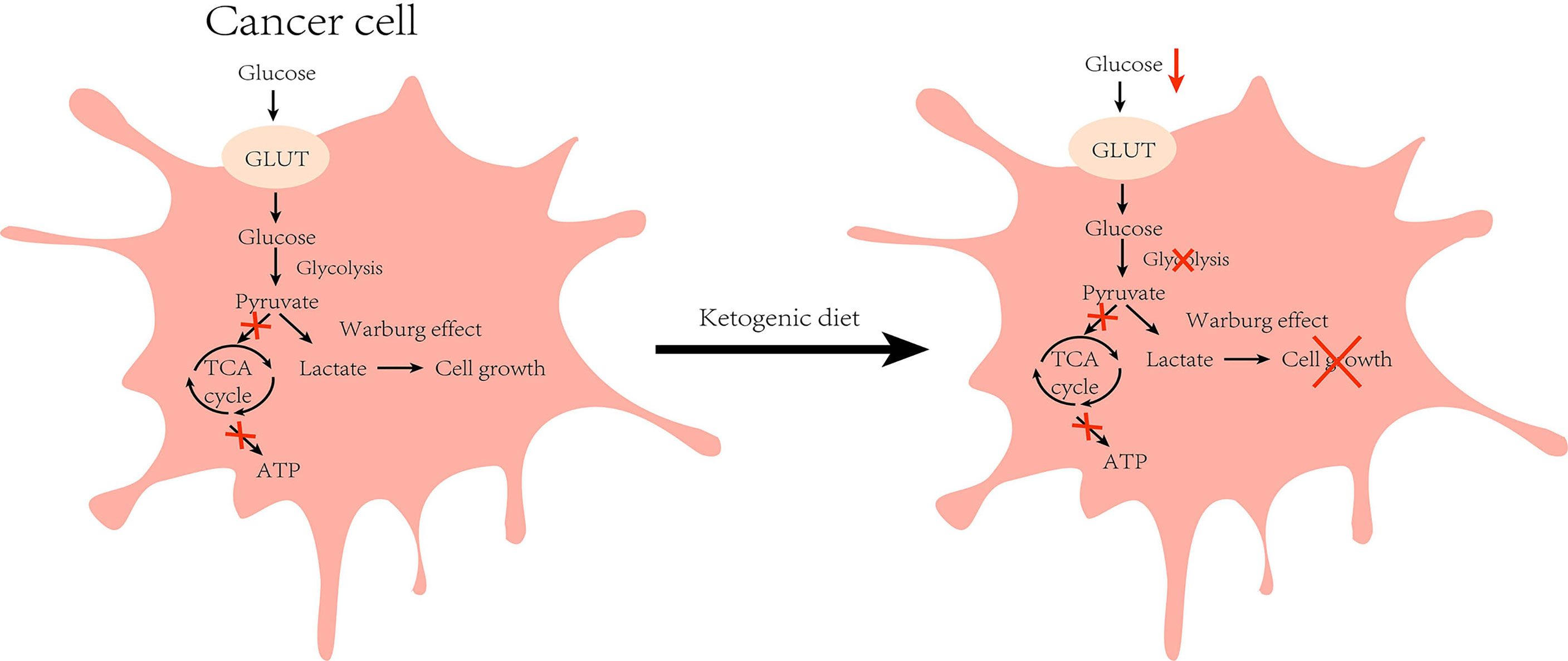
Figure 2 Effect of ketogenic diet on glucose metabolism in cancer cell: Cancer cells are characterized by higher glycolysis due to defective mitochondrial function, and the accumulated cytosolic pyruvate is used to produce lactate (Warburg effect) during normal diet. However, under ketogenic diet feeding, glucose intake is reduced and the growth of cancer cells is inhibited by reduced energy supply. (GLUT, glucose transporter).
Regulation of Lipid Metabolism
The liver is the site of lipid metabolism, and lipid metabolism tends to affect liver function. Abnormalities in lipid metabolism can cause NAFLD, which in turn can lead to the development of HCC. Under a normal diet, acetyl-CoA produced by the TCA cycle is converted into malonyl-CoA by ACC (the key enzyme in fatty acid synthesis), and then finally converted into fatty acids through a series of biosynthetic processes. Similarly, cholesterol biosynthesis also begins with acetyl-CoA, and the rate-limiting enzyme for its synthesis is HMGCS2 reductase (HMGCR). However under the KD, insufficient energy metabolism and low insulin inhibit the activity of ACC and HMGCR, leading to reduced lipid synthesis (41). Restriction of carbohydrate intake is adopted as a dietary intervention for patients with obesity, and studies have found multiple benefits with regard to NAFLD (42). Numerous clinical studies have established the positive effects of KD on patients with obesity and NAFLD. In a 6-day intervention involving 10 patients with obesity, Luukkonen et al. found that KD promoted hydrolysis of intrahepatic triglycerides (IHTG), improved insulin resistance, and decreased the serum insulin level in patients, resulting in decreased IHTG and body weight (43). In addition, Browning et al. performed a 2-week dietary intervention in patients with NAFLD and noted that the low-carbohydrate diet was more beneficial than the low-calorie diet in reducing hepatic triglyceride (TG) levels (44). Yancy et al. conducted a dietary intervention on 120 overweight, hyperlipidemic volunteers for 6 months, and observed that when compared with the low-fat diet, the low-carbohydrate diet was not only more effective in reducing the serum TG levels, but also in significantly lowering body weight and increasing the high-density lipoprotein cholesterol (HDL-C) levels (45). Foster et al. conducted long-term intervention in patients with obesity. While the results of their short-term trials matched the findings of Yancy et al., their results at 2 years found that only HDL-C levels were higher than those in the low-fat diet group, with no apparent variations in any other indicators (46). These results suggest that the effect of low carbohydrates on patients is influenced by the duration of the diet, and that prolonged intervention studies are needed to further illustrate the follow-up impact. Of note, not all low-carbohydrate diets are KD (47). The low-carbohydrate group in the above studies strictly controlled their carbohydrate intake, all of which were <20g/day. However, there were no strict restrictions regarding fat and protein contents, which met the requirements of MAD. It is worth noting that after feeding mice on a very low-carbohydrate ketogenic diet for 12 weeks, Garbow et al. found that the mice developed hepatic endoplasmic reticulum stress, steatosis, and cellular damage, causing a pattern of damage similar to the NAFLD phenotype, however, the occurrence of this phenomenon might have been the result of extreme KD (95.1% of calories from fat, 0.4% from carbohydrate, and 4.5% from protein) (48). In addition to this, some studies show that KD leads to systemic glucose intolerance and the development of NAFLD (49, 50). One case reported a woman who developed potential NAFLD after the KD with elevated liver enzymes (51). From these findings, it could be inferred that KD with various composition ratios can produce diverse or even opposing results. Watanabe et al. analyzed the current articles on KD and NAFLD, and found that a high-fat ketogenic diet (HFKD) significantly affects liver fat reduction. Nevertheless, this effect was evident in the short- and medium-term only, but diminished with time. Additionally, very low-calorie KD (VLCKD) can reduce body weight and improve hepatic steatosis (47). The above studies illustrate that the regulation of lipid metabolism by KD is influenced by several factors. At present, there are few articles about the KD as a risk factor for NAFLD. In general, KD exerts a positive effect on NAFLD. Since KD can regulate lipid metabolism and reduce body weight, it has shown a positive effect on cardiovascular function, and studies have also found that KD has a certain improvement in systolic and diastolic blood pressure (52, 53).
Moreover, many key enzyme inhibitors in lipid metabolism have demonstrated anti-cancer effects (54). HMGCS2 is an important enzyme in fatty acid oxidation (55). A pre-clinical study has demonstrated that HMGCS2 deficiency causes ketogenic disorders, leading to extensive hepatocellular damage and inflammation. Furthermore, the deficiency has been shown to result in hepatic TCA circulatory disturbances and promote the development of NAFLD (56). Through immunohistochemical staining, Wang et al. noted that HMGCS2 was highly expressed in healthy liver tissues. However, the expression was significantly decreased in liver cirrhosis and in HCC tissues. Also, the expression of HMGCS2 was negatively associated with the pathological grade and clinical stage of HCC. Knock-down of HMGCS2 promoted cell proliferation by enhancing the c-Myc/cyclinD1 pathway and inhibiting the caspase-dependent apoptosis pathway. The knockdown also up-regulated the epithelial–mesenchymal transition signal to enhance cell migration and promote tumorigenesis. Interestingly, supplementation of β-OHB to cells in which HMGCS2 was knocked down revealed a decrease in proliferation and migration of HCC cells. Mice fed with KD also had lower subcutaneous tumor growth rates than those on a standard diet (57). Furthermore, Wang et al. investigated the xenograft mouse mode (58). They were surprised to find that the tumors of KD-fed mice expressed higher levels of the HMGCS2 protein than those on a normal diet, and that the tumor size in the KD-fed mice group was inversely associated with HMGCS2 protein expression. These results demonstrate that KD can exert anti-HCC effects by enhancing the expression of HMGCS2 (Figure 3).
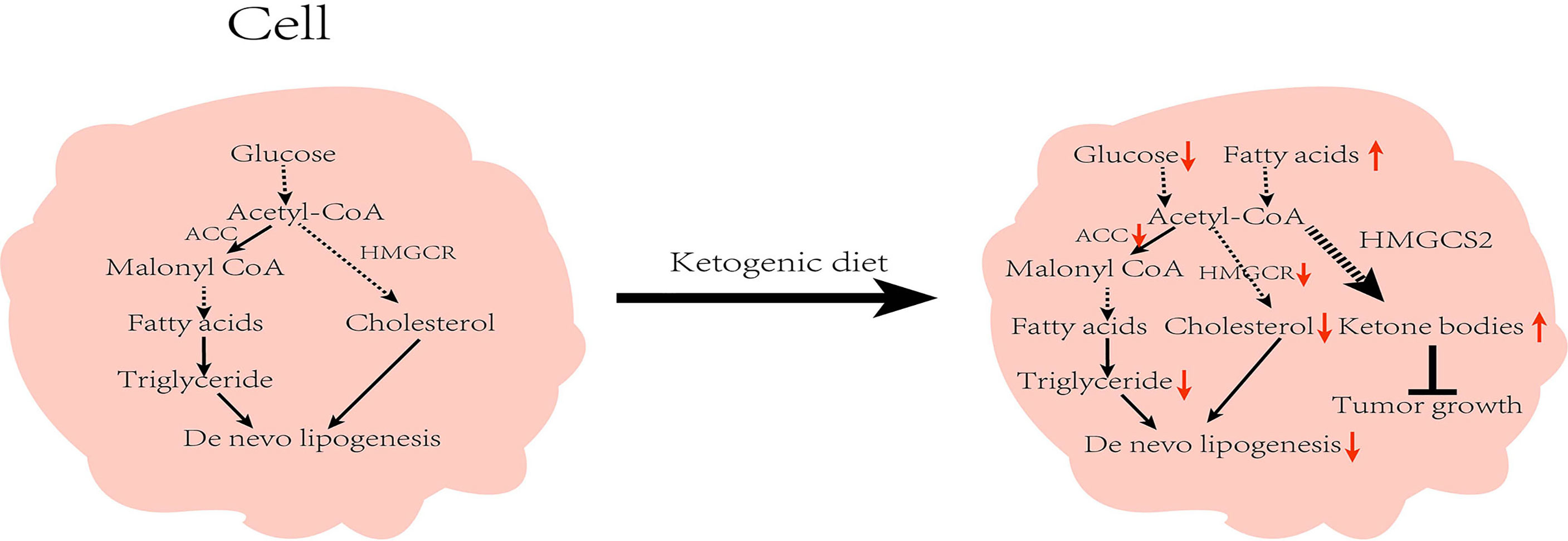
Figure 3 Effect of ketogenic diet on lipid metabolism in cells: While on the ketogenic diet, carbohydrates intake is reduced and the activity of acetyl-CoA carboxylase and β-hydroxy-β-methylglutaryl-CoA reductase are inhibited, which in turn reduces de novo lipid synthesis. Concurrently, increased fat mobilization and ketone body production inhibit tumor growth. ACC, Acetyl-CoA carboxylase; HMGCR, β-hydroxy-β-methylglutaryl-CoA reductase; HMGCS2; HMG-CoA synthase.
Regulation of Inflammatory Response
When compared with normal cells, more reactive oxygen species (ROS) are produced in tumor cells because of defective mitochondrial oxidative phosphorylation, which causes cellular oxidative damage (59). Studies have indicated that inflammation has a promotional effect on tumorigenesis and development, and many antagonists targeting inflammatory cytokines are being developed and investigated to prevent and treat cancer (60, 61). Viral hepatitis is a major cause of HCC, and it has been confirmed that inflammation can promote HCC development in multiple ways (62). Therefore, suppressing the inflammatory response of hepatocytes is an essential mechanism to combat HCC. β-OHB, the main component of KBs, has a butyrate-like structure, and is an endogenous inhibitor of histone deacetylase. The effect of β-OHB is dose-dependent. KD can augment serum β-OHB levels and exert anti-inflammatory and anti-tumor effects by inducing histone hyperacetylation (35). The anti-inflammatory effects of KBs have also been confirmed by analyzing mouse microglia. β-OHB may exert its anti-inflammatory effects by activating GPR109A and by inhibiting the NF-κB pathway, which decreases the expression of inflammatory factors, such as COX-2 (63). GPR109A is a protein receptor that inhibits tumor growth; β-OHB is a physiological agonist of GPR109A that exerts its tumor-suppressive effects by agonizing GPR109A (64). Moreover, a study found that the ketogenic diet can also produce anti-inflammatory effects by inhibiting the assembly of the NLRP3 inflammasome, a complex that can control the release of cytokines, such as tumor necrosis factor-alpha (TNF-α). Besides, TNF-α can interfere with insulin signaling, leading to insulin resistance (65). Many reports have shown that β-OHB exerts significant anti-inflammatory effects. By evaluating the effects of different β-OHB levels on cow hepatocytes, it was found that β-OHB induces activation of the NF-κB signaling pathway by up-regulating oxidative markers and down-regulating antioxidant markers, thereby causing inflammatory damage to the cells and increasing the oxidative status index with increasing β-OHB levels (66). Therefore, we speculate that the contradiction of the above results may be related to the level of β-OHB and cell type, as well as other factors. AcAc, another KB, was proven to promote oxidative stress in cellular assays, leading to cellular damage through the up-regulation of NADPH oxidase 4 (NOX4) expression and NADPH oxidase activity. The effect of ROS was stronger at 25 mM glucose than with KB alone (67). In response to some controversies about ROS production by KB, Meroni et al. separately examined cells with β-OHB and AcAc, and observed that KB activates the transcription factor, Nrf2, by inducing moderate oxidative stress, which further activates antioxidant defense systems to prevent damage (68). Collectively, the specific effect of KB on the inflammatory response remains unclear, but the types of KB and cell appear to be factors that influence its anti-inflammatory effect. More trials are necessary to pursue a greater in-depth understanding.
Pre-Clinical and Clinical Trials
Several pre-clinical trials have established KD as an effective anti-cancer strategy to delay tumor growth and prolong survival time, but the effect of KD on tumors is influenced by tumor type (69, 70). Little research has been conducted regarding KD and HCC. Huang et al. observed that serum glucose levels and body weight were significantly reduced in mice fed with KD. However, HCC cells under starvation can trigger the mTORC2-AKT-SP1 signaling, which promotes OXCT1 (a key ketolytic enzyme) expression and inhibits AMPK-mediated autophagy, thus protecting the cancer cells. The elevated KBs in vivo can promote HCC cell growth (71). OXCT1 is not routinely expressed in the adult liver (20). The above results may indicate a metabolic adaptation of HCC cells in the presence of nutritional deficiency. Most importantly, the above study reveals a positive relationship between OXCT1 expression and the clinical stage of HCC. Patients with high OXCT1 expression have a short survival time, which signifies that OXCT1 holds promise as a new biomarker for HCC. Moreover, studies have shown that the effect of KD on HCC mice is also affected by the intervention time. Diet interventions were started in the HCC mouse model in the 6th week, and the tumor burden and diversity of the mice in the KD-fed group were lower than those in the high-glucose diet group (16). However, when dietary intervention commenced at 40 weeks, KD did not have a significant effect on HCC (17), suggesting that early intervention with KD is beneficial in HCC. The above result is consistent with findings of previous studies. A meta-analysis showed that the timing of dietary intervention affects the therapeutic effect of KD, and that prophylactic KD intervention has a strong anti-cancer effect (72).
Clinical studies related to the impacts of KD on HCC are limited, and there are only two case reports on liver tumors (73). After applying KD monotherapy for 1 month, a patient’s blood glucose level decreased to normal, and ultrasound showed that the tumor had disappeared after 1 year of KD. The other patient also demonstrated a significant improvement in clinical performance. Furthermore, no serious side effects were reported during the therapeutic period. However, the specifics of this case report, such as the stage of the patient’s tumor and the specific composition of the KD, were not described, all of which can significantly impact treatment outcome. In addition, the small number of participants is a shortcoming of the report. Hence, the application of KD for treating HCC still lacks proper evidence to support it, and more clinical studies are needed to provide confirmation.
Adverse Effects of KD in Treating Cancer
Although most studies have proved the safety of KD in the treatment of cancer, some adverse effects related to KD have been reported in the literature, namely, gastrointestinal reactions, increased blood lipid levels, kidney stones, and fractures (12, 74). For example, Mansoor et al. found that a low-carbohydrate dietary intervention for more than 6 months in healthy people increased their LDL-C levels (75). Similarly, a 60-day KD intervention in rats resulted in several adverse effects, such as anemia, metabolic acidosis, and decreased plasma superoxide dismutase (SOD) levels (76). In addition, studies exploring carbon tetrachloride- and thioacetamide-induced liver fibrosis mouse models revealed that KD leads to the development of cirrhosis by increasing cholesterol content in the liver, which subsequently enhances the hepatic inflammatory response and decreases the antioxidant and detoxification capacity (77) (50).Most of the above-mentioned adverse events are important factors affecting HCC, which promote its occurrence through glucose metabolism, inflammatory response, and increased NAFLD mechanisms. Moreover, the ketogenic diet is different from the traditional diet in that the patient needs to prepare the meal correctly and cannot eat with friends and family normally, which can make the patient feel socially isolated. In addition, the cost of the patient’s diet due to the change in food composition will also change. These can contribute to psychosocial problems for the patient. Therefore, we should pay attention to these adverse reactions in future studies to ensure the safety and feasibility of KD.
Potential Future Research Directions
There are increasing reports of combination therapies against progression of various tumors, including HCC (78, 79). Similarly, the ketogenic diet could be combined with a secondary drug to enhance its therapeutic potential against HCC. For example, inhibitors of the mevalonate pathway could control the elevated cholesterol in the blood and liver after KD. It is well established that the rate-limiting step in cholesterol synthesis is the conversion of hydroxyl-methyl glutaryl-coenzyme A (HMG-CoA) into mevalonate. In fact, small molecule inhibitors of the mevalonate pathway increased the cell death of liver carcinoma cells in several in vitro studies (80–82). Statins are a classic inhibitor of mevalonate pathway, which are clinically used to reduce hypercholesterolemia. Several studies have found that statins can significantly reduce the risk of HCC (83). Furthermore, Thrift et al. found that statins also reduced mortality in HCC patients (84). Therefore, we suggest the use of KD combined with the inhibitors of mevalonate pathway such as statins for preclinical and clinical studies.
Recently, KD has been shown to be beneficial in treating many diseases, but different KD compositions tend to show varying effects. For example, in a 45-day randomized trial, significant improvements in weight, blood pressure, and some metabolic parameters were seen in three groups on a VLCKD containing whey, vegetable, or animal proteins. However, whey and vegetable proteins had a better safety profile than animal proteins, and whey protein demonstrated the strongest effect in regulating the gut microbiota and maintaining muscle performance (85). In addition, some large prospective cohort studies have confirmed the superiority of plant proteins, revealing that plant protein intake is negatively related to all-cause mortality. The replacement of animal proteins, especially processed and red meat proteins, with plant proteins reduces cancer and cardiovascular disease-related mortality (86, 87). These results could be attributed to the fact that plant proteins can improve insulin sensitivity and lower blood pressure and LDL levels. In the two pre-clinical trials on HCC discussed above, the proteins included in the KD were animal proteins. Therefore, the type of protein was not an influencing factor in the different effects (16, 17). However, one cannot help but wonder if KD containing plant proteins would yield more surprising results if it was used to intervene in HCC mice. In addition to the type of protein, the role played by the type of fat ingested can vary. Both the classical KD and MKD showed inhibitory effects on tumor growth when compared with the standard diet. However, the MKD containing omega-3 and medium-chain triglycerides had a more pronounced effect than the classical KD (88). The findings from these pre-clinical studies suggest that diet optimization should be performed in future studies to achieve the optimal anti-cancer effect of KD.
Owing to the abnormal mitochondrial function, the production of ROS is increased in tumor cells during mitochondrial respiration. Simultaneously, KD limits the regeneration of NADPH by converting sugar metabolism to lipid metabolism and by further increasing the oxidative stress level of the tumor cells (89). Because cancer cells have a higher oxidative stress response than the normal cells, as well as a higher sensitivity to radiotherapy and chemotherapy, KD can be used as an adjuvant therapy to kill the tumor cells selectively. It has been demonstrated that KD can enhance the effects of chemotherapy and radiotherapy in brain tumors (70). In addition, a prospective clinical trial involving nine patients supported the idea that KD is safe and feasible as an adjuvant in the standard treatment for glioblastoma (90). Similarly, women with breast cancer who received radiotherapy and KD had better metabolic indicators and a significant improvement in quality of life when compared with those on a standard diet (91). Radiotherapy is a critical treatment for HCC. Therefore, combining it with KD to improve treatment outcome holds promise.
Conclusion
Currently, treatment options for HCC are limited, and better therapeutic options for HCC are the need of the hour. KD is a therapy in which the dietary composition is altered, and is safer than drug therapy. Based on the available evidence, we speculate that KD may inhibit the growth of HCC by lowering blood glucose and insulin levels, regulating lipid metabolism, and alleviating the inflammatory response. Furthermore, KD can be considered as an adjuvant therapy for HCC. It has been found that KD intervention is effective in the early stages of the disease, but most patients are already in the middle to late stages when HCC is detected. Therefore, the use of KD prophylactically in the treatment of HCC is an issue that warrants further exploration. In addition to the timing of the intervention, the patient’s low compliance with KD is a major issue that should be addressed. Therefore, in future studies, the formulation of KD must be optimized to bridge the difference from the traditional diet, as well as to reduce the occurrence of adverse reactions. It is also necessary to increase patient education and support to improve compliance. Most importantly, there are few studies on the use of KD in HCC, and these do not fully reflect the therapeutic effects. Therefore, the long-term effects and safety of KD are remain unexplored. More studies with higher reliability should be designed to elucidate the mechanism of KD in the treatment of HCC and its application in clinical practice.
Author Contributions
Conceptualization, YL. Original draft preparation, YL, CJ, XY, and CL. Review and editing, YL, CJ, and PK. Supervision, JS. Funding acquisition, JS. All authors have read and agreed to the published version of the manuscript.
Funding
This research was funded by Chinese National Natural Science Foundation (Grant No. 81670567), and Scientific Research Fund of Zhejiang University (Grant No. XY2021030). Chaonan Jin was funded by China Scholarship Council.
Conflict of Interest
The authors declare that the research was conducted in the absence of any commercial or financial relationships that could be construed as a potential conflict of interest.
Publisher’s Note
All claims expressed in this article are solely those of the authors and do not necessarily represent those of their affiliated organizations, or those of the publisher, the editors and the reviewers. Any product that may be evaluated in this article, or claim that may be made by its manufacturer, is not guaranteed or endorsed by the publisher.
References
1. Sung H, Ferlay J, Siegel RL, Laversanne M, Soerjomataram I, Jemal A, et al. Global Cancer Statistics 2020: GLOBOCAN Estimates of Incidence and Mortality Worldwide for 36 Cancers in 185 Countries. CA: A Cancer J Clin (2021) 71(3):209–49. doi: 10.3322/caac.21660
2. Villanueva A. Hepatocellular Carcinoma. N Engl J Med (2019) 380(15):1450–62. doi: 10.1056/NEJMra1713263
3. Yang JD, Hainaut P, Gores GJ, Amadou A, Plymoth A, Roberts LR. A Global View of Hepatocellular Carcinoma: Trends, Risk, Prevention and Management. Nat Rev Gastroenterol Hepatol (2019) 16(10):589–604. doi: 10.1038/s41575-019-0186-y
4. Nishida N. Long-Term Prognosis and Management of Hepatocellular Carcinoma After Curative Treatment. Clin Mol Hepatology (2020) 26(4):480–3. doi: 10.3350/cmh.2020.0208
5. Liberti MV, Locasale JW. The Warburg Effect: How Does It Benefit Cancer Cells? Trends Biochem Sci (2016) 41(3):211–8. doi: 10.1016/j.tibs.2015.12.001
6. Icard P, Shulman S, Farhat D, Steyaert JM, Alifano M, Lincet H. How the Warburg Effect Supports Aggressiveness and Drug Resistance of Cancer Cells? Drug Resist Update (2018) 38:1–1. doi: 10.1016/j.drup.2018.03.001
7. Wheless JW. History of the Ketogenic Diet [Conference Paper]. Epilepsia (2008) 49(SUPPL. 8):3–5. doi: 10.1111/j.1528-1167.2008.01821.x
8. Weber DD, Aminzadeh-Gohari S, Tulipan J, Catalano L, Feichtinger RG, Kofler B. Ketogenic Diet in the Treatment of Cancer - Where Do We Stand? Mol Metab (2020) 33:102–21. doi: 10.1016/j.molmet.2019.06.026
9. Fearon KC, Borland W, Preston T, Tisdale MJ, Shenkin A, Calman KC. Cancer Cachexia: Influence of Systemic Ketosis on Substrate Levels and Nitrogen Metabolism. Am J Clin Nutr (1988) 47(1):42–8. doi: 10.1093/ajcn/47.1.42
10. Shukla SK, Gebregiworgis T, Purohit V, Chaika NV, Gunda V, Radhakrishnan P, et al. Metabolic Reprogramming Induced by Ketone Bodies Diminishes Pancreatic Cancer Cachexia. Cancer Metab (2014) 2:18–8. doi: 10.1186/2049-3002-2-18
11. Zahra A, Fath MA, Opat E, Mapuskar KA, Bhatia SK, Ma DC, et al. Consuming a Ketogenic Diet While Receiving Radiation and Chemotherapy for Locally Advanced Lung Cancer and Pancreatic Cancer: The University of Iowa Experience of Two Phase 1 Clinical Trials. Radiat Res (2017) 187(6):743–54. doi: 10.1667/rr14668.1
12. Klement RJ, Brehm N, Sweeney RA. Ketogenic Diets in Medical Oncology: A Systematic Review With Focus on Clinical Outcomes. Med Oncol (2020) 37(2):14. doi: 10.1007/s12032-020-1337-2
13. Xia S, Lin R, Jin L, Zhao L, Kang H-B, Pan Y, et al. Prevention of Dietary-Fat-Fueled Ketogenesis Attenuates BRAF V600E Tumor Growth. Cell Metab (2017) 25(2):358–73. doi: 10.1016/j.cmet.2016.12.010
14. Zarrinpar A. Metabolic Pathway Inhibition in Liver Cancer. SLAS Technol (2017) 22(3):237–44. doi: 10.1177/2472630317698683
15. Li J, Li M-H, Wang T-t, Liu X-n, Zhu X-t, Dai Y-z, et al. SLC38A4 Functions as a Tumour Suppressor in Hepatocellular Carcinoma Through Modulating Wnt/β-Catenin/MYC/HMGCS2 Axis. Br J Cancer (2021) 125(6):865–76. doi: 10.1038/s41416-021-01490-y
16. Healy ME, Chow JD, Byrne FL, Breen DS, Leitinger N, Li C, et al. Dietary Effects on Liver Tumor Burden in Mice Treated With the Hepatocellular Carcinogen Diethylnitrosamine. J Hepatol (2015) 62(3):599–606. doi: 10.1016/j.jhep.2014.10.024
17. Byrne FL, Hargett SR, Lahiri S, Roy RJ, Berr SS, Caldwell SH, et al. Serial MRI Imaging Reveals Minimal Impact of Ketogenic Diet on Established Liver Tumor Growth. Cancers (Basel) (2018) 10(9):312–8. doi: 10.3390/cancers10090312
18. Koronowski KB, Greco CM, Huang H, Kim J-K, Fribourgh JL, Crosby P, et al. Ketogenesis Impact on Liver Metabolism Revealed by Proteomics of Lysine β-Hydroxybutyrylation. Cell Rep (2021) 36(5):109487–7. doi: 10.1016/j.celrep.2021.109487
19. Glew RH. You Can Get There From Here: Acetone, Anionic Ketones and Even-Carbon Fatty Acids Can Provide Substrates for Gluconeogenesis. Niger J Physiol Sci (2010) 25(1):2–4.
20. Zhang S, Xie C. The Role of OXCT1 in the Pathogenesis of Cancer as a Rate-Limiting Enzyme of Ketone Body Metabolism. Life Sci (2017) 183:110–5. doi: 10.1016/j.lfs.2017.07.003
21. Puchalska P, Crawford PA. Multi-Dimensional Roles of Ketone Bodies in Fuel Metabolism, Signaling, and Therapeutics. Cell Metab (2017) 25(2):262–84. doi: 10.1016/j.cmet.2016.12.022
22. Roehl K, Sewak SL. Practice Paper of the Academy of Nutrition and Dietetics: Classic and Modified Ketogenic Diets for Treatment of Epilepsy. J Acad Nutr Dietetics (2017) 117(8):1279–92. doi: 10.1016/j.jand.2017.06.006
23. Huttenlocher PR, Wilbourn AJ, Signore JM. Medium-Chain Triglycerides as a Therapy for Intractable Childhood Epilepsy. Neurology (1971) 21(11):1097–103. doi: 10.1212/wnl.21.11.1097
24. Cervenka MC, Kossoff EH. Dietary Treatment of Intractable Epilepsy. Continuum (Minneap Minn) (2013) 19(3 Epilepsy):756–66. doi: 10.1212/01.CON.0000431396.23852.56
25. Kossoff EH, Zupec-Kania BA, Auvin S, Ballaban-Gil KR, Christina Bergqvist AG, Blackford R, et al. Practice Committee of the Child Neurology S. Optimal Clinical Management of Children Receiving Dietary Therapies for Epilepsy: Updated Recommendations of the International Ketogenic Diet Study Group. Epilepsia Open (2018) 3(2):175–92. doi: 10.1002/epi4.12225
26. Kossoff EH, Dorward JL. The Modified Atkins Diet [Conference Paper]. Epilepsia (2008) 49(SUPPL. 8):37–41. doi: 10.1111/j.1528-1167.2008.01831.x
27. Pfeifer HH, Thiele EA. Low-Glycemic-Index Treatment: A Liberalized Ketogenic Diet for Treatment of Intractable Epilepsy . Neurology (2005) 65(11):1810–2. doi: 10.1212/01.wnl.0000187071.24292.9e
28. Khodabakhshi A, Akbari ME, Mirzaei HR, Seyfried TN, Kalamian M, Davoodi SH. Effects of Ketogenic Metabolic Therapy on Patients With Breast Cancer: A Randomized Controlled Clinical Trial. Clin Nutr (2021) 40(3):751–8. doi: 10.1016/j.clnu.2020.06.028
29. Khodabakhshi A, Akbari ME, Mirzaei HR, Mehrad-Majd H, Kalamian M, Davoodi SH. Feasibility, Safety, and Beneficial Effects of MCT-Based Ketogenic Diet for Breast Cancer Treatment: A Randomized Controlled Trial Study. Nutr Cancer (2020) 72(4):627–34. doi: 10.1080/01635581.2019.1650942
30. Klement RJ, Schäfer G, Sweeney RA. A Ketogenic Diet Exerts Beneficial Effects on Body Composition of Cancer Patients During Radiotherapy: An Interim Analysis of the KETOCOMP Study. J Traditional Complement Med (2019) 10(3):180–7. doi: 10.1016/j.jtcme.2019.03.007
31. Woodhouse C, Ward T, Gaskill-Shipley M, Chaudhary R. Feasibility of a Modified Atkins Diet in Glioma Patients During Radiation and Its Effect on Radiation Sensitization. Curr Oncol (Toronto Ont) (2019) 26(4):e433–8. doi: 10.3747/co.26.4889
32. Amann T, Hellerbrand C. GLUT1 as a Therapeutic Target in Hepatocellular Carcinoma. Expert Opin Ther Targets (2009) 13(12):1411–27. doi: 10.1517/14728220903307509
33. Healy ME, Lahiri S, Hargett SR, Chow JDY, Byrne FL, Breen DS, et al. Dietary Sugar Intake Increases Liver Tumor Incidence in Female Mice. Sci Rep (2016) 6:22292–2. doi: 10.1038/srep22292
34. Seyfried TN, Kiebish MA, Marsh J, Shelton LM, Huysentruyt LC, Mukherjee P. Metabolic Management of Brain Cancer. Biochim Biophys Acta (2011) 1807(6):577–94. doi: 10.1016/j.bbabio.2010.08.009
35. Feng S, Wang H, Liu J, Aa J, Zhou F, Wang G. Multi-Dimensional Roles of Ketone Bodies in Cancer Biology: Opportunities for Cancer Therapy. Pharmacol Res (2019) 150:104500. doi: 10.1016/j.phrs.2019.104500
36. Cohen CW, Fontaine KR, Arend RC, Alvarez RD, Leath CA III, Huh WK, et al. A Ketogenic Diet Reduces Central Obesity and Serum Insulin in Women With Ovarian or Endometrial Cancer. J Nutr (2018) 148(8):1253–60. doi: 10.1093/jn/nxy119
37. Fine EJ, Segal-Isaacson CJ, Feinman RD, Herszkopf S, Romano MC, Tomuta N, et al. Targeting Insulin Inhibition as a Metabolic Therapy in Advanced Cancer: A Pilot Safety and Feasibility Dietary Trial in 10 Patients. Nutrition (2012) 28(10):1028–35. doi: 10.1016/j.nut.2012.05.001
38. Bowers LW, Rossi EL, O'Flanagan CH, deGraffenried LA, Hursting SD. The Role of the Insulin/IGF System in Cancer: Lessons Learned From Clinical Trials and the Energy Balance-Cancer Link. Front Endocrinol (2015) 6:77. doi: 10.3389/fendo.2015.00077
39. Janku F. Phosphoinositide 3-Kinase (PI3K) Pathway Inhibitors in Solid Tumors: From Laboratory to Patients. Cancer Treat Rev (2017) 59:93–101. doi: 10.1016/j.ctrv.2017.07.005
40. Hopkins BD, Pauli C, Du X, Wang DG, Li X, Wu D, et al. Suppression of Insulin Feedback Enhances the Efficacy of PI3K Inhibitors. Nature (2018) 560(7719):499–503. doi: 10.1038/s41586-018-0343-4
41. Bian X, Liu R, Meng Y, Xing D, Xu D, Lu Z. Lipid Metabolism and Cancer. J Exp Med (2021) 218(1):e20201606. doi: 10.1084/jem.20201606
42. Mardinoglu A, Wu H, Bjornson E, Zhang C, Hakkarainen A, Räsänen SM, et al. An Integrated Understanding of the Rapid Metabolic Benefits of a Carbohydrate-Restricted Diet on Hepatic Steatosis in Humans. Cell Metab (2018) 27(3):559–71.e5. doi: 10.1016/j.cmet.2018.01.005
43. Luukkonen PK, Dufour S, Lyu K, Zhang X-M, Hakkarainen A, Lehtimäki TE, et al. Effect of a Ketogenic Diet on Hepatic Steatosis and Hepatic Mitochondrial Metabolism in Nonalcoholic Fatty Liver Disease. Proc Natl Acad Sci USA (2020) 117(13):7347–54. doi: 10.1073/pnas.1922344117
44. Browning JD, Baker JA, Rogers T, Davis J, Satapati S, Burgess SC. Short-Term Weight Loss and Hepatic Triglyceride Reduction: Evidence of a Metabolic Advantage With Dietary Carbohydrate Restriction. Am J Clin Nutr (2011) 93(5):1048–52. doi: 10.3945/ajcn.110.007674
45. Yancy WS, Olsen MK, Guyton JR, Bakst RP, Westman EC. A Low-Carbohydrate, Ketogenic Diet Versus a Low-Fat Diet To Treat Obesity and Hyperlipidemia. Ann Internal Med (2004) 140(10):769–77. doi: 10.7326/0003-4819-140-10-200405180-00006
46. Foster GD, Wyatt HR, Hill JO, Makris AP, Rosenbaum DL, Brill C, et al. Weight and Metabolic Outcomes After 2 Years on a Low-Carbohydrate Versus Low-Fat Diet: A Randomized Trial. Ann Internal Med (2010) 153(3):147–57. doi: 10.7326/0003-4819-153-3-201008030-00005
47. Watanabe M, Tozzi R, Risi R, Tuccinardi D, Mariani S, Basciani S, et al. Beneficial Effects of the Ketogenic Diet on Nonalcoholic Fatty Liver Disease: A Comprehensive Review of the Literature. Obes Rev (2020) 21(8):e13024. doi: 10.1111/obr.13024
48. Garbow JR, Doherty JM, Schugar RC, Travers S, Weber ML, Wentz AE, et al. Hepatic Steatosis, Inflammation, and ER Stress in Mice Maintained Long Term on a Very Low-Carbohydrate Ketogenic Diet. Am J Physiol Gastrointest Liver Physiol (2011) 300(6):G956–67. doi: 10.1152/ajpgi.00539.2010
49. Jornayvaz FR, Jurczak MJ, Lee H-Y, Birkenfeld AL, Frederick DW, Zhang D, et al. A High-Fat, Ketogenic Diet Causes Hepatic Insulin Resistance in Mice, Despite Increasing Energy Expenditure and Preventing Weight Gain. Am J Physiol Endocrinol Metab (2010) 299(5):E808–15. doi: 10.1152/ajpendo.00361.2010
50. Schugar RC, Crawford PA. Low-Carbohydrate Ketogenic Diets, Glucose Homeostasis, and Nonalcoholic Fatty Liver Disease. Curr Opin Clin Nutr Metab Care (2012) 15(4):374–80. doi: 10.1097/MCO.0b013e3283547157
51. Anekwe CV, Chandrasekaran P, Stanford FC. Ketogenic Diet-Induced Elevated Cholesterol, Elevated Liver Enzymes and Potential Non-Alcoholic Fatty Liver Disease. Cureus (2020) 12(1):e6605–5. doi: 10.7759/cureus.6605
52. Nasser S, Vialichka V, Biesiekierska M, Balcerczyk A, Pirola L. Effects of Ketogenic Diet and Ketone Bodies on the Cardiovascular System: Concentration Matters. World J Diabetes (2020) 11(12):584–95. doi: 10.4239/wjd.v11.i12.584
53. Kosinski C, Jornayvaz FR. Effects of Ketogenic Diets on Cardiovascular Risk Factors: Evidence From Animal and Human Studies. Nutrients (2017) 9(5):517. doi: 10.3390/nu9050517
54. Pope ED 3rd, Kimbrough EO, Vemireddy LP, Surapaneni PK, Copland JA 3rd, Mody K. Aberrant Lipid Metabolism as a Therapeutic Target in Liver Cancer. Expert Opin Ther Targets (2019) 23(6):473–83. doi: 10.1080/14728222.2019.1615883
55. Vilà-Brau A, De Sousa-Coelho AL, Mayordomo C, Haro D, Marrero PF. Human HMGCS2 Regulates Mitochondrial Fatty Acid Oxidation and FGF21 Expression in HepG2 Cell Line. J Biol Chem (2011) 286(23):20423–30. doi: 10.1074/jbc.M111.235044
56. Cotter DG, Ercal B, Huang X, Leid JM, d'Avignon DA, Graham MJ, et al. Ketogenesis Prevents Diet-Induced Fatty Liver Injury and Hyperglycemia. J Clin Invest (2014) 124(12):5175–90. doi: 10.1172/JCI76388
57. Wang YH, Liu CL, Chiu WC, Twu YC, Liao YJ. HMGCS2 Mediates Ketone Production and Regulates the Proliferation and Metastasis of Hepatocellular Carcinoma. Cancers (Basel) (2019) 11(12):1876–94. doi: 10.3390/cancers11121876
58. Wang YH, Suk FM, Liao YJ. Loss of HMGCS2 Enhances Lipogenesis and Attenuates the Protective Effect of the Ketogenic Diet in Liver Cancer. Cancers (Basel) (2020) 12(7):1797–813. doi: 10.3390/cancers12071797
59. Galluzzi L, Morselli E, Kepp O, Vitale I, Rigoni A, Vacchelli E, et al. Mitochondrial Gateways to Cancer. Mol Aspects Med (2010) 31(1):1–20. doi: 10.1016/j.mam.2009.08.002
60. Crusz SM, Balkwill FR. Inflammation and Cancer: Advances and New Agents. Nat Rev Clin Oncol (2015) 12(10):584–96. doi: 10.1038/nrclinonc.2015.105
61. Balkwill F, Mantovani A. Inflammation and Cancer: Back to Virchow? Lancet (2001) 357(9255):539–45. doi: 10.1016/S0140-6736(00)04046-0
62. Yu L-X, Ling Y, Wang H-Y. Role of Nonresolving Inflammation in Hepatocellular Carcinoma Development and Progression. NPJ Precis Oncol (2018) 2(1):6–6. doi: 10.1038/s41698-018-0048-z
63. Fu S-P, Li S-N, Wang J-F, Li Y, Xie S-S, Xue W-J, et al. BHBA Suppresses LPS-Induced Inflammation in BV-2 Cells by Inhibiting NF-κb Activation. Mediators Inflamm (2014) 2014:983401–1. doi: 10.1155/2014/983401
64. Ristic B, Bhutia YD, Ganapathy V. Cell-Surface G-Protein-Coupled Receptors for Tumor-Associated Metabolites: A Direct Link to Mitochondrial Dysfunction in Cancer. Biochim Biophys Acta Rev Cancer (2017) 1868(1):246–57. doi: 10.1016/j.bbcan.2017.05.003
65. Mundi MS, Mohamed Elfadil O, Patel I, Patel J, Hurt RT. Ketogenic Diet and Cancer: Fad or Fabulous? J Parenteral Enteral Nutr (2021) 45(S2):S26–32. doi: 10.1002/jpen.2226
66. Shi X, Li X, Li D, Li Y, Song Y, Deng Q, et al. β-Hydroxybutyrate Activates the NF-κb Signaling Pathway to Promote the Expression of Pro-Inflammatory Factors in Calf Hepatocytes. Cell Physiol Biochem (2014) 33(4):920–32. doi: 10.1159/000358664
67. Kanikarla-Marie P, Jain SK. Hyperketonemia (Acetoacetate) Upregulates NADPH Oxidase 4 and Elevates Oxidative Stress, ICAM-1, and Monocyte Adhesivity in Endothelial Cells. Cell Physiol Biochem (2015) 35(1):364–73. doi: 10.1159/000369702
68. Meroni E, Papini N, Criscuoli F, Casiraghi MC, Massaccesi L, Basilico N, et al. Metabolic Responses in Endothelial Cells Following Exposure to Ketone Bodies. Nutrients (2018) 10(2):250. doi: 10.3390/nu10020250
69. Li J, Zhang H, Dai Z. Cancer Treatment With the Ketogenic Diet: A Systematic Review and Meta-Analysis of Animal Studies. Front Nutr (2021) 8:594408. doi: 10.3389/fnut.2021.594408
70. Woolf EC, Syed N, Scheck AC. Tumor Metabolism, the Ketogenic Diet and β-Hydroxybutyrate: Novel Approaches to Adjuvant Brain Tumor Therapy. Front Mol Neurosci (2016) 9:122. doi: 10.3389/fnmol.2016.00122
71. Huang D, Li T, Wang L, Zhang L, Yan R, Li K, et al. Hepatocellular Carcinoma Redirects to Ketolysis for Progression Under Nutrition Deprivation Stress. Cell Res (2016) 26(10):1112–30. doi: 10.1038/cr.2016.109
72. Klement RJ, Champ CE, Otto C, Kämmerer U. Anti-Tumor Effects of Ketogenic Diets in Mice: A Meta-Analysis. PloS One (2016) 11(5):e0155050–e0155050. doi: 10.1371/journal.pone.0155050
73. Motta J, Lobo L, Fabiola D. Ketogenic Diet Against Liver Tumor. Curr Developments Nutr (2020) 4(Supplement_2):338–8. doi: 10.1093/cdn/nzaa044_037
74. Longo R, Peri C, Cricri D, Coppi L, Caruso D, Mitro N, et al. Ketogenic Diet: A New Light Shining on Old But Gold Biochemistry. Nutrients (2019) 11(10):2497–519. doi: 10.3390/nu11102497
75. Mansoor N, Vinknes KJ, Veierød MB, Retterstøl K. Effects of Low-Carbohydrate Diets V. Low-Fat Diets on Body Weight and Cardiovascular Risk Factors: A Meta-Analysis of Randomised Controlled Trials. Br J Nutr (2016) 115(3):466–79. doi: 10.1017/S0007114515004699
76. Arsyad A, Idris I, Rasyid AA, Usman RA, Faradillah KR, Latif WOU, et al. Long-Term Ketogenic Diet Induces Metabolic Acidosis, Anemia, and Oxidative Stress in Healthy Wistar Rats. J Nutr Metab (2020) 2020:3642035–3642035. doi: 10.1155/2020/3642035
77. Liao Y-J, Wang Y-H, Wu C-Y, Hsu F-Y, Chien C-Y, Lee Y-C. Ketogenic Diet Enhances the Cholesterol Accumulation in Liver and Augments the Severity of CCl(4) and TAA-Induced Liver Fibrosis in Mice. Int J Mol Sci (2021) 22(6):2934. doi: 10.3390/ijms22062934
78. Finn RS, Qin S, Ikeda M, Galle PR, Ducreux M, Kim TY, et al. Atezolizumab Plus Bevacizumab in Unresectable Hepatocellular Carcinoma. N Engl J Med (2020) 382(20):1894–905. doi: 10.1056/NEJMoa1915745
79. Yau T, Park JW, Finn RS, Cheng AL, Mathurin P, Edeline J, et al. Nivolumab Versus Sorafenib in Advanced Hepatocellular Carcinoma (CheckMate 459): A Randomised, Multicentre, Open-Label, Phase 3 Trial. Lancet Oncol (2022) 23(1):77–90. doi: 10.1016/s1470-2045(21)00604-5
80. Kogure T, Ueno Y, Kimura O, Kondo Y, Inoue J, Fukushima K, et al. A Novel Third Generation Bisphosphonate, Minodronate (YM529), Prevented Proliferation and Migration of Hepatocellular Carcinoma Cells Through Inhibition of Mevalonate Pathway. Hepatol Res (2009) 39(5):479–89. doi: 10.1111/j.1872-034X.2008.00484.x
81. Takeda Y, Nakao K, Nakata K, Kawakami A, Ida H, Ichikawa T, et al. Geranylgeraniol, an Intermediate Product in Mevalonate Pathway, Induces Apoptotic Cell Death in Human Hepatoma Cells: Death Receptor-Independent Activation of Caspase-8 With Down-Regulation of Bcl-xL Expression. Jpn J Cancer Res (2001) 92(9):918–25. doi: 10.1111/j.1349-7006.2001.tb01181.x
82. Tabata Y, Omori M, Shidoji Y. Age-Dependent Decrease in Hepatic Geranylgeranoic Acid Content in C3H/HeN Mice and Its Oral Supplementation Prevents Spontaneous Hepatoma. Metabolites (2021) 11(9):634–48. doi: 10.3390/metabo11090634
83. Islam MM, Poly TN, Walther BA, Yang H-C, Jack Li Y-C. Statin Use and the Risk of Hepatocellular Carcinoma: A Meta-Analysis of Observational Studies. Cancers (Basel) (2020) 12(3):671. doi: 10.3390/cancers12030671
84. Thrift AP, Natarajan Y, Liu Y, El-Serag HB. Statin Use After Diagnosis of Hepatocellular Carcinoma Is Associated With Decreased Mortality. Clin Gastroenterol Hepatol (2019) 17(10):2117–25.e3. doi: 10.1016/j.cgh.2018.12.046
85. Basciani S, Camajani E, Contini S, Persichetti A, Risi R, Bertoldi L, et al. Very-Low-Calorie Ketogenic Diets With Whey, Vegetable, or Animal Protein in Patients With Obesity: A Randomized Pilot Study. J Clin Endocrinol Metab (2020) 105(9):2939–49. doi: 10.1210/clinem/dgaa336
86. Song M, Fung TT, Hu FB, Willett WC, Longo VD, Chan AT, et al. Association of Animal and Plant Protein Intake With All-Cause and Cause-Specific Mortality. JAMA Internal Med (2016) 176(10):1453–63. doi: 10.1001/jamainternmed.2016.4182
87. Budhathoki S, Sawada N, Iwasaki M, Yamaji T, Goto A, Kotemori A, et al. Association of Animal and Plant Protein Intake With All-Cause and Cause-Specific Mortality in a Japanese Cohort. JAMA Internal Med (2019) 179(11):1509–18. doi: 10.1001/jamainternmed.2019.2806
88. Hao GW, Chen YS, He DM, Wang HY, Wu GH, Zhang B. Growth of Human Colon Cancer Cells in Nude Mice is Delayed by Ketogenic Diet With or Without Omega-3 Fatty Acids and Medium-Chain Triglycerides. Asian Pac J Cancer Prev (2015) 16(5):2061–8. doi: 10.7314/apjcp.2015.16.5.2061
89. Allen BG, Bhatia SK, Anderson CM, Eichenberger-Gilmore JM, Sibenaller ZA, Mapuskar KA, et al. Ketogenic Diets as an Adjuvant Cancer Therapy: History and Potential Mechanism. Redox Biol (2014) 2:963–70. doi: 10.1016/j.redox.2014.08.002
90. van der Louw EJTM, Olieman JF, van den Bemt PMLA, Bromberg JEC, Oomen-de Hoop E, Neuteboom RF, et al. Ketogenic Diet Treatment as Adjuvant to Standard Treatment of Glioblastoma Multiforme: A Feasibility and Safety Study. Ther Adv Med Oncol (2019) 11:1758835919853958–1758835919853958. doi: 10.1177/1758835919853958
Keywords: ketogenic body, ketogenic diet, cancer cell metabolism, hepatocellular carcinoma, liver
Citation: Lan Y, Jin C, Kumar P, Yu X, Lenahan C and Sheng J (2022) Ketogenic Diets and Hepatocellular Carcinoma. Front. Oncol. 12:879205. doi: 10.3389/fonc.2022.879205
Received: 19 February 2022; Accepted: 11 April 2022;
Published: 04 May 2022.
Edited by:
Weihua Zhou, University of Michigan, United StatesReviewed by:
Sravya Palavalasa, University of Michigan, United StatesCopyright © 2022 Lan, Jin, Kumar, Yu, Lenahan and Sheng. This is an open-access article distributed under the terms of the Creative Commons Attribution License (CC BY). The use, distribution or reproduction in other forums is permitted, provided the original author(s) and the copyright owner(s) are credited and that the original publication in this journal is cited, in accordance with accepted academic practice. No use, distribution or reproduction is permitted which does not comply with these terms.
*Correspondence: Jifang Sheng, amlmYW5nX3NoZW5nQHpqdS5lZHUuY24=