- 1Department of Thoracic Oncology, Cancer Center and State Key Laboratory of Biotherapy, West China Hospital, Sichuan University, Chengdu, China
- 2Laboratory of Clinical Cell Therapy, West China Hospital, Sichuan University, Chengdu, China
Cancer stem cells, a relatively small group of self-renewing cancer cells, were first isolated from acute myeloid leukemia. These cells can play a crucial role in tumor metastasis, relapse, and therapy resistance. The cancer stem cell theory may be applied to lung cancer and explain the inefficiency of traditional treatments and eventual recurrence. However, because of the unclear accuracy and illusive biological function of cancer stem cells, some researchers remain cautious about this theory. Despite the ongoing controversy, cancer stem cells are still being investigated, and their biomarkers are being discovered for application in cancer diagnosis, targeted therapy, and prognosis prediction. Potential lung cancer stem cell markers mainly include surface biomarkers such as CD44, CD133, epithelial cell adhesion molecule, and ATP-binding cassette subfamily G member 2, along with intracellular biomarkers such as aldehyde dehydrogenase, sex-determining region Y-box 2, NANOG, and octamer-binding transcription factor 4. These markers have different structures and functions but are closely associated with the stem potential and uncontrollable proliferation of tumor cells. The aberrant activation of major signaling pathways, such as Notch, Hedgehog, and Wnt, may be associated with the expression and regulation of certain lung cancer stem cell markers, thus leading to lung cancer stem cell maintenance, chemotherapy resistance, and cancer promotion. Treatments targeting lung cancer stem cell markers, including antibody drugs, nanoparticle drugs, chimeric antigen receptor T-cell therapy, and other natural or synthetic specific inhibitors, may provide new hope for patients who are resistant to conventional lung cancer therapies. This review provides comprehensive and updated data on lung cancer stem cell markers with regard to their structures, functions, signaling pathways, and promising therapeutic target approaches, aiming to elucidate potential new therapies for lung cancer.
1 Introduction
Considerable achievements have been made in improving patient survival by providing better treatments. However, several obstacles remain because of drug resistance, metastasis, and the lack of specific targeted drugs. The cancer stem cell (CSC) theory suggested that there is a relatively small group of self-renewing cancer cells that play a crucial role in tumor metastasis, relapse, and therapy resistance (1). However, because of the unclear accuracy and illusive biological function of CSCs, some researchers remain cautious and find this theory controversial (2, 3). In lung cancer, CSC theory may also explain the inefficiency of traditional treatments and eventual recurrence. Despite the ongoing controversy, CSCs are still being investigated and discovered (4).
CSCs are a subtle group of tumor cells with potential multidirectional differentiation capacity, high self-renewal, and tumorigenicity (1). They were first isolated from acute myeloid leukemia (5, 6) and may be derived from either regular tissue-specific stem cells or differentiated cells at tumor initiation to activate survival pathways and gain the ability to proliferate indefinitely (7). Because of their importance, CSC biomarkers can be used in cancer diagnosis, targeted therapy, and prognosis prediction. Potential markers of lung CSCs mainly include surface biomarkers, such as CD44, CD133, epithelial cell adhesion molecule (EpCAM), and ATP-binding cassette subfamily G member 2 (ABCG2), along with intracellular biomarkers, such as aldehyde dehydrogenase (ALDH), sex-determining region Y-box 2 (SOX2), NANOG, and octamer-binding transcription factor 4 (OCT4). This review focuses on the most frequently studied lung CSC markers with their structures, functions, potential mechanisms, and signaling pathways of therapy resistance and cancer relapse. The objective of this review was to provide updated data on lung CSC markers as promising therapeutic targets in patients with lung cancer, hoping to bring new hope on lung cancer treatment.
2 Structure and Function of Lung Cancer Stem Cell Markers
Various possible lung CSC markers have been identified to label heterogeneous lung CSC populations. A summary of the potential biomarkers for lung CSC, including surface and intracellular markers, is discussed below. Tables 1, 2 provide a comprehensive list of the most frequent stem cell markers in non-small cell lung cancer (NSCLC) and small cell lung cancer (SCLC), respectively, and describe their structures and main functions. Notably, one marker is usually not enough to distinguish lung CSCs accurately. Thus, using marker combinations is a wise approach to identify and isolate cells that may stimulate cancer formation, chemoresistance, and recurrence.
2.1 Potential Surface Markers for Lung Cancer Stem Cell
2.1.1 CD44
CD44 (P-glycoprotein 1), a type I transmembrane glycoprotein, belongs to the family of cell adhesion molecules, and its gene is located on human chromosome 11 (11p13). Human CD44 weighs 85–200 kDa and consists of 742 amino acids (8). Through selective shearing and post-translational modification, CD44 can form a variety of isoforms that can combine with a range of ligands on the cell surface and are involved in the physiological and pathological processes of cells. CD44 is a hyaluronic acid receptor, and the communication between CD44 and hyaluronic acid is speculated to induce cell shedding, metastasis, and invasion (9). CD44 is expressed in almost all types of tumors and is a potential marker of mesenchymal stem cells and CSCs (10). It was found that CD44high/CD24low cells lost epithelial cell markers and expressed more mesenchymal cell markers. These cells achieved the ability of self-renewal and heterogeneous differentiation, demonstrating that epithelial-to-mesenchymal transition (EMT) may help normal and tumor cells acquire stemness (11). In lung cancer, studies have shown that CD44 expression is higher in NSCLC than in SCLC, and the highest expression level was observed in lung squamous cell carcinoma (12). Cells derived from NSCLC cell lines were found to have chromosomal aberrations and 1p36 deletion, which significantly reduce the expression of the tumor suppressor gene miR-34a located at 1p36. However, overexpression of miR-34a attenuates the stem phenotype of CD44+ cells (13). CD44 regulates several signaling pathways to promote cancer progression, including Notch, Hedgehog (HH), Wnt, STAT3, Hippo, JNK, and RhoGTPase, and is a coreceptor that mediates signaling pathways by receptor tyrosine kinases (14, 15). Besides, CD44 is a key mediator of adhesion between endothelial cells, thus playing a major role in pathological angiogenesis (16). By binding to ezrin, radixin and moesin proteins, CD44 can bind to the cytoskeleton with the cell membrane, which facilitates cancer cell growth and metastasis. CD44 can also mediate tumor proliferation and immune evasion by promoting PD-L1 expression on the tumor cell surface (17). CD44 expression may play a significant role in epidermal growth factor receptor (EGFR)-mutant neoplasms, particularly in early NSCLC (18, 19), and CD44 is important in predicting EMT upon EGFR-TKI monotherapy in patients with lung cancer (20). CD44 and ALDH co-expressing cells, which are higher in lung squamous cell carcinoma, always show increased self-renewal capacity, enhanced migration ability, and tumorigenicity (21).
2.1.2 CD133
CD133 (prominin-1) is a cholesterol-binding five-fold transmembrane glycoprotein that was first isolated in 1997 from mouse neural and CD34+ human progenitor and hematopoietic stem cells (22). Subsequently, CD133 was identified as a potential CSC marker, and research on CD133 has been focused on lung, breast, colon, and liver cancers. The structure of CD133 contains one extracellular N-terminal, two intracellular loops rich in cysteine, two extracellular loops that possess nine N-linked glycosylation parts, and one cytoplasmic C-terminal. The human CD133 gene contains at least 37 exons, more than 150 kb in length, and is controlled by five promoters, encoding a 97–120 kDa glycoprotein composed of 865 amino acids (23).
In terms of its function in lung cancer, CD133 is not only a potential biomarker of lung CSC but also a potential therapeutic target and prognostic factor. CD133 correlates with metastasis, therapy resistance, and worse outcomes. CD133+ cells can expand, invade, and self-renew, whereas CD133- cells are terminally differentiated in lung cancer (24). Thus, targeting and destroying CD133+ lung CSCs may significantly inhibit tumor cell proliferation, migration, and invasion. However, the exact molecular mechanisms of CD133-related drug resistance remain elusive and require more attention. To date, evidence has shown that CD133 may regulate the growth and chemoresistance of CSCs by activating Wnt signaling, PI3K-AKT signaling, SRC-FAK signaling, interaction with EGFR, vascular endothelial growth factor autophagy, lipid metabolism, and low reactive oxygen species levels (25–27). In combination with other CSC biomarkers, such as CXCR4 (28) and BMI1 (29), CD133 is also positively correlated with EMT. And in hypoxic environment, up-regulation of OCT4 and SOX2 can induce CD133 expression in lung cancer cells (30).
2.1.3 EpCAM
EpCAM, also known as CD326, is a type I transmembrane polypeptide and weighs approximately 40 kDa. EpCAM is composed of 314 amino acids with a long extracellular N-terminal, a single-spanning transmembrane domain, and a short intracellular C-terminal. The human EpCAM gene is approximately 14 kb and is located on chromosome 2 (2p21) (31). EpCAM is a potential biomarker for neoplasms of epithelial origin and a homotypic calcium-independent cell adhesion molecule. In lung cancer, EpCAM has been found to be a downstream target of metastasis-associated protein 1 (MTA1), and MTA1 overexpression can increase EpCAM levels and enhance tumor metastasis, leading to poor prognosis (32). EpCAM expression is also related to CD44 and CD166, and the triple-positive (EpCAM+/CD44+/CD166+) markers in NSCLC indicate higher self-renewal ability, clonal heterogeneity, and stemness-associated gene expression (33).
2.1.4 ABCG2
ABCG2, also known as breast cancer resistance protein, is a 72 kDa xenobiotic half-transporter with 655 amino acids, and chromosome 4 (4q22) encodes the ABCG2 gene. ABCG2 consists of six transmembrane domains and only one ATP-binding domain, and it is detected in normal tissue cells and CSCs. Using cryo-electron microscopy, scholars have observed the high-resolution architecture of human ABCG2 and its interaction with inhibitory 5D3 antibody, suggesting its mechanism of multidrug recognition and transportation (34). ABCG2 is abundant in the side population phenotype in CSCs and effluxes Hoechst 33342 from cancer cells, associating ABCG2 with multidrug resistance (35). In lung CSCs, ABCG2 levels are elevated, and this may be related to the transcription factors Sp1 and Sp3, which can link with the promoter region of ABCG2 (36). Treatment of lung cancer cells with tobacco concentrates can lead to significant upregulation of ABCG2 and its transcription factors Sp1 and Nrf2, indicating that smoking may induce lung cancer partly through ABCG2 upregulation (37). Co-expression of ABCG2 and CD133 may lead to a higher risk of cisplatin resistance and tumor relapse (38).
2.2 Potential Intracellular Markers for Lung Cancer Stem Cell
2.2.1 ALDH
ALDH is crucial in acetyl-coenzyme oxidation and differentiation regulation of normal stem cells. In NSCLC, ALDH levels are significantly enhanced and are involved in therapy resistance, mainly due to the increased expression of ALDH1A1 (39). ALDH1A1 is a 50–55 kDa protein sharing 501 amino acids, and the human ALDH1A1 gene is located on chromosome 9 (9q21.13). ALDH exists and functions as tetramer or dimer, and its monomers comprise the coenzyme binding, catalytic, and “arm-like” oligomerization parts (40). ALDH1A1 may be a potential CSC marker in solid tumors, and its expression is positively correlated with an epithelial-like phenotype in NSCLC (41). Compared with ALDH- cells, ALDH+ cells have greater self-renewal and tumorigenic capacity, and the level of ALDH1A1 correlates with poor survival. ALDH1A1 also correlates with Notch3, CD44, and CD133, which are related to chemoresistance and poor prognosis (21, 42).
2.2.2 Transcription Factors
Transcription factors can drive cell migration and functional maturation, as well as control the multidirectional differentiation potency of embryonic stem cells and CSCs. They are the center of the cellular pluripotency regulatory network and control the transcription of pluripotency-related genes. In lung cancer, many studies have shown that intracellular transcription factors, such as SOX2, OCT4, and NANOG, are maladjusted and may therefore activate stemness genes and suppress differentiation genes.
SOX2 belongs to the sex-determining region Y-related high-mobility group box family and contributes to the maintenance of the pluripotency of embryonic stem cells and CSCs. SOX2 involves approximately 317 amino acids, and chromosome 3 (3q26.3) encodes the human SOX2 gene (43). SOX2 is a pleiotropic protooncogene related to stemness and EMT in lung cancer (44). It can regulate oncogenes, including c-MYC, Wnt1, Wnt2, and NOTCH1, and plays a major role in FGFR1-ERK1/2-SOX2 axis to stimulate metastasis (45). SOX2 can induce the expression of the tumor-related factors p63 and keratin 6 and suppress CDKN1A, which can rescue G1 cell cycle arrest in squamous cell carcinoma (46, 47), leading to cancer differentiation, migration, and invasion. In SCLC, SOX2 is crucial in the PIK3-AKT-SOX2 signaling pathway and may mediate chemoresistance (48). Genome-wide analysis has revealed that SOX2 amplification can drive the occurrence and development of SCLC.
OCT4, also known as POU5F1 or OCT3, is a member of the POU transcription factor family. Chromosome 6 (6p21.31) encodes the human OCT4 gene (POU5F1 gene) and has an average length of 16.4 kb. With multiple transcription initiation sites, it can transcribe different mRNA isoforms, thus translating into a variety of proteins (49). The translated protein contains an N-terminal domain that activates transcription, a conserved DNA-binding domain, a POU binding domain, and a C-terminal transactivation part. OCT4 plays a major role in maintaining and regaining pluripotency. In lung cancer, OCT4 expression is related to therapy resistance, cancer relapse, and worse outcomes (50). Studies have also shown that OCT4 promotes lung cancer progression by transcriptionally regulating the long non-coding RNAs NEAT1 and MALAT1 (51).
NANOG, a DNA-binding homeobox transcription factor, may promote cell proliferation, renewal, and stem properties (52). It has approximately 305 amino acids, and chromosome 12 (12p13.31) encodes the NANOG gene. NANOG protein is roughly divided into N-terminal, DNA-binding homeodomain, and C-terminal transcriptional activation part (53). In lung cancer, NANOG expression correlates with TNM stage, tumor differentiation, and survival (54). Thus, NANOG overexpression may be a promising target and predictive marker.
3 Major Signaling Pathways Related to Lung Cancer Stem Cell Markers
The ability of normal tissue stem cells to self-renew and differentiate is regulated by several signaling pathways, while the aberrant activation of these signals may be attributed to CSC maintenance, chemotherapy resistance, and cancer promotion. Certain biomarkers of lung CSCs are related and are partly regulated by these signals. The major signaling pathways currently found to be closely related to lung CSCs and their markers mainly include Notch, HH, and Wnt signaling (Figure 1 and Table 3).
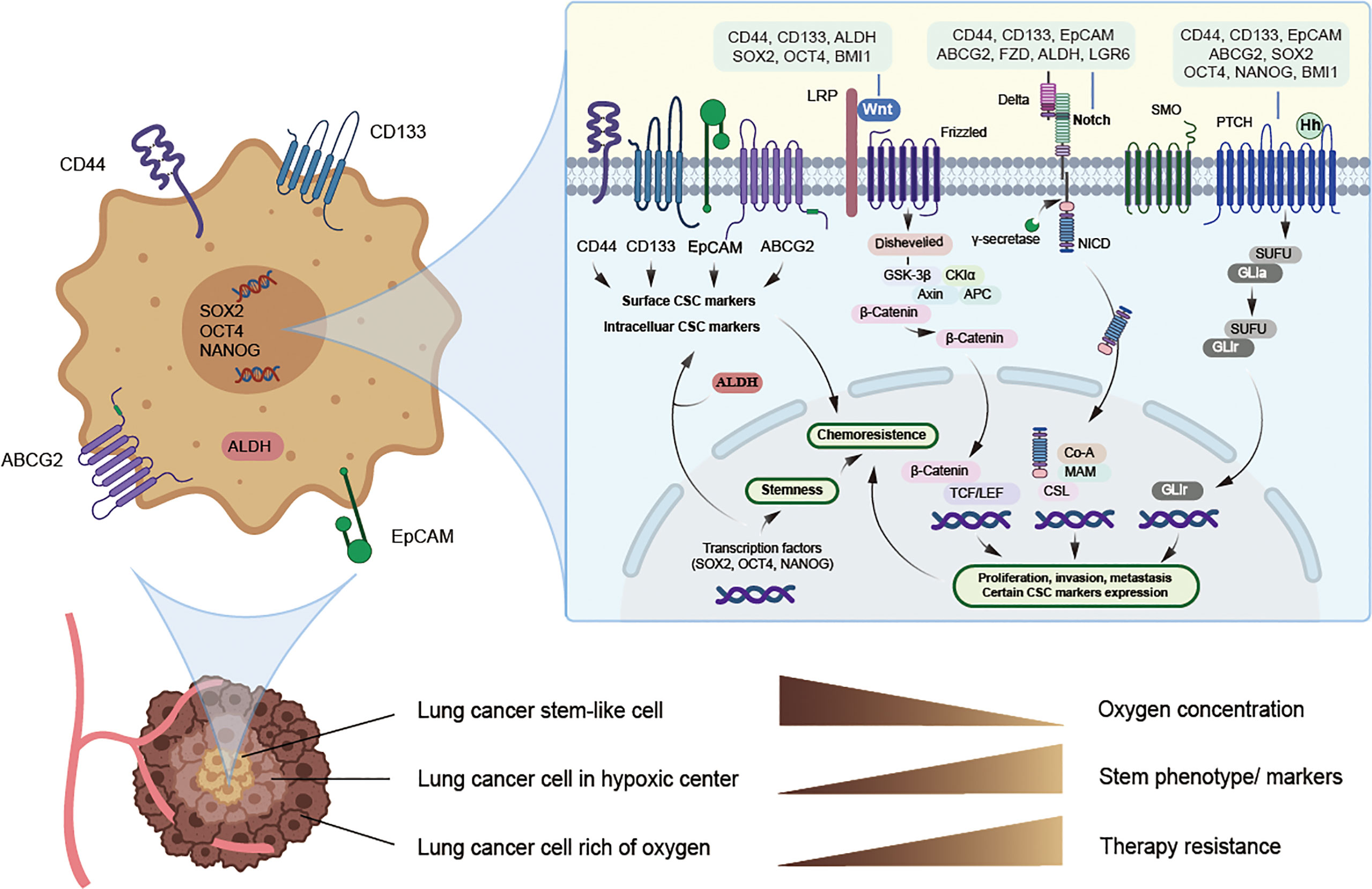
Figure 1 Potential lung cancer stem cell with its markers and major signaling pathways. CSCs can live deep in the hypoxic center of tumor and hypoxia may induce CSCs phenotype and the expression of CSC markers, thus promoting chemoresistance, while specific targeted therapy towards CSCs may solve this problem. Potential markers of lung cancer stem cells mainly include surface biomarkers, such as CD44, CD133, EpCAM and ABCG2, along with intracellular biomarkers, such as ALDH, SOX2, NANOG and OCT4, and they may act as promising therapeutic targets. Signaling pathways such as Notch, Hedgehog, and Wnt may jointly induce the expression of certain lung cancer stem cell markers and stimulate EMT, thus allowing tumor cells to obtain stemness and chemoresistance.
3.1 Notch Signaling Pathway
Notch signaling is widely distributed in different cell types and plays a major role in cell communication, proliferation, differentiation, apoptosis, adhesion, and EMT. It is also conducive to mediating the crosstalk between the different compartments of the tumor microenvironment. Notch signals pass through Delta-like ligand 1 (DLL1), DLL3, DLL4, Jagged1, or Jagged2 on a cell to the Notch receptor paralog (Notch1, Notch2, Notch3, or Notch 4) on an adjacent cell, which releases the Notch intracellular domain and converts Notch receptors into downstream signal transducers (55). Notch signaling is critical for stem characteristics, and its abnormal activation may stimulate the growth and metastasis of tumor cells through its downstream proteins. The main regulatory genes include the HES family genes, the proto-oncogenes MYC, cyclin-dependent kinase inhibitor 1A, cyclin D3 human epidermal growth factor receptor 2, and Notch regulated ankyrin repeat protein (56). The HES family genes are transcriptional repressors that control stem cell characteristics. Genetic mutations in Notch can sustain the survival of CSCs and extend to all levels of NOTCH signal members (57).
Notch signaling is crucial in various neoplasms including leukemia, lymphoma, breast cancer, lung cancer, glioblastoma, and colon cancer. It is related to certain lung CSC markers, such as CD44, CD133, ALDH, OCT4, and SOX2 (58–60). In NSCLC, Notch 1 and Notch 2 are highly expressed (61, 62) and gain self-renewal by the transcription factor HES1. Notch 1 also protected lung CSCs from cisplatin-induced cell death through a pathway independent of HES1 (63). In addition to promoting the proliferation of NSCLC cells, the Notch signaling pathway can also mediate the metastasis of NSCLC through the circulatory and lymphatic systems. However, the function of abnormal Notch activation in SCLC is debated. Studies have shown that Notch signaling in SCLC is both a tumor suppressor and a pro-oncogene (64). Notch gene function loss and Notch activation inhibition in SCLC suggest that Notch signaling inhibits SCLC growth and that the Notch pathway can prevent the differentiation of precursor cells into neuroendocrine differentiation during lung development (65–67). However, in preclinical models, Notch signaling blockade combined with chemotherapy can inhibit tumor growth and delay tumor recurrence, which suggests a pro-carcinogenic part of Notch in SCLC (64, 68). In addition, Notch signaling blockade with shRNA against NOTCH or γ-secretase inhibitors can significantly suppress the growth of CD44+, CD133+, or ALDH+ tumor cells, consistent with a decrease in lung cancer cell growth and chemoresistance (58, 59, 69). Studies have also shown that cisplatin resistance induces the enrichment of CD133+ cells, which is partly due to the abnormal activation of Notch signaling, suggesting that this pathway is a potential target in regulating lung CSCs (59).
3.2 HH Signaling Pathway
The HH signaling pathway is crucial for embryonic development, and its abnormal activation is also associated with stem cell regulation and tumor occurrence. The HH ligands have various isoforms, including sonic HH, Indian HH, and desert HH, which can link with the transmembrane receptors Patched and Smoothened. Only when the protein family members maintain full length can they function as transcription promoters, activating the expression of downstream genes. After Patched and Smoothened transcription, released signal cascades contribute to the activation of glioma-associated oncogene (GLI) transcription factors, thereby driving HH target gene transcription and thus leading to better stem property maintenance (70).
Abnormal activity of HH signals is common in various solid tumors, such as medulloblastoma, glioma, myeloma, pancreatic cancer, esophageal cancer, gastric cancer, breast cancer, and lung cancer. Sonic HH and GLI-1 are highly active in the repair of lung airway epithelial injury. Both in SCLC and NSCLC, the expression of GLI-1 and sonic HH proteins was evaluated and associated with chromosome instability and stemness (71–73). The HH and Wnt pathways can jointly induce CSC biomarkers, such as CD44, CD133, BMI1, and LGR5, and promote EMT, thereby promoting tumor cell infiltration and distant metastasis and allowing tumor cells to obtain stem cell characteristics and drug resistance capacity (74). GLI-1 is also a strong regulator of SOX2 and develops resistance to EGFR inhibitors in NSCLC (75). Studies have found that phosphorylated SOX2 can be recruited to the hedgehog acyltransferase (HHAT) promoter to enhance HHAT levels, which is conducive for maintaining stemness. Activation of the PKC1-SOX2-HHAT signal axis is conducive to maintaining the stem function of primary lung squamous cells (76). Activated HH signaling is also significantly related to drug transporters, such as ABCG2, thus promoting chemotherapeutic drug resistance. This pathway may regulate several aspects of the lung CSC state and increase cancer metastasis. It is, therefore, not surprising that the inhibitors of this pathway offer great clinical hope.
3.3 Wnt Signaling Pathway
As a complex protein network, Wnt signaling is mostly found in the development of the central nervous system and mammalian embryos (77). However, it is also associated with abnormal processes in various tumors, such as breast cancer, colorectal cancer, liver cancer, leukemia, and lung cancer. The three primary Wnt pathways are the canonical Wnt pathway, which is involved in gene expression, the noncanonical Wnt/planar cell polarity pathway, which coordinates the cytoskeleton, and the noncanonical Wnt/calcium pathway, which adjusts the concentration of intracellular calcium (78). All three Wnt signaling pathways are activated by Wnt protein ligands binding to Frizzled receptors and coreceptors, such as low-density lipoprotein receptor-related protein 5 and 6, which transmit the signal to various intracellular proteins. Wnt ligands are secreted proteins, and this pathway is often paracrine or autocrine. The Wnt signaling pathway can regulate cell differentiation and proliferation, and is important in carcinogenesis, invasion, progression, and CSC maintenance (79). Hypoxia can activate Wnt signaling pathway, promote the non-differentiation and self-renewal ability of CSCs (80, 81). What’s more, tumor-associated fibroblasts in the environment can activate Wnt and Notch signaling pathways, produce metalloproteinases such as MMP2, MMP3 and MMP9 to reshape the extracellular matrix and promote self-renewal of EMT and CSCs (82). And some inflammatory microenvironments, which promote NF-κB and Wnt signaling, can induce tumor non-stem cells to acquire tumor-initiation capabilities (83).
In human lung cancer, the main Wnt ligands are Wnt1, Wnt2, Wnt5a, and Wnt7a, and an increase in Wnt proteins, Wnt1 or Wnt5a alone, is significantly related to poor prognosis in NSCLC (84). Studies suggest that β-catenin promotes the number of lung CSCs mainly by decreasing differentiation, rather than promoting direct stem cell proliferation (85). The mutation of β-catenin in tumor cells can cause itself to be inactivated by phosphorylation and degraded by ubiquitination. β-catenin accumulates in large amounts in the cytoplasm and enters the nucleus to regulate cell division and stem cell-related genes, leading to uncontrolled cell proliferation and tumorigenesis (86). Wnt signaling can regulate various lung CSC markers such as NANOG and OCT4, and the different Wnt microenvironments in different types of NSCLC lead to imparities in ABC transporter expression, such as ABCB1 and ABCG2 (87–89). Through the non-canonical Wnt pathway, Wnt5a can increase the stem properties of ALDH+ lung CSC in cisplatin-resistant NSCLC (90). In addition, Wnt signaling activation partly results from EMT, which is crucial in CSC reorganization and maintenance. EMT has been reported to induce β-catenin/E-cadherin/SOX15 conversion into β-catenin/Twist1/TCF4, thus inducing the transcription of the lung CSC marker ABCG2 (91). Cell subpopulations with nuclear high β-catenin, Twist1, CD133 together with low E-cadherin, and SOX15 can be used as diagnostic markers in lung cancer (91, 92).
4 Lung Cancer Stem Cell Markers as Therapeutic Targets
Although great achievements have been made in improving survival in patients with lung cancer through conventional treatments and combinatorial therapies, concomitant congenital or acquired drug resistance still limits the therapeutic effect and leads to poor clinical outcomes. Targeting CSCs in the lung cancer microenvironment may be a promising therapy for patients who are currently resistant to traditional lung cancer treatments. From this perspective, lung CSC markers may not only help to identify and isolate these lung CSC subpopulations but may also be conducive to specific targeted therapies. Therapies targeting lung CSC markers mainly depend on the specific recognition and binding of antibodies to lung CSC surface markers, thus leading to a variety of antibody drugs and nanoparticle drugs. In addition, novel chimeric antigen receptor T (CAR-T) cell immunotherapy as well as inhibitors, such as natural compounds and siRNA knockdown, also play an increasingly important role in experiments and future clinical trials (Figure 2 and Table 4). Besides, there are some novel clinical trials regarding lung CSCs targeting like CSC-loaded DC vaccines without posted outcome (ClinicalTrials.gov: NCT02084823i) and targeting lung CSCs expressing TRAIL which is still recruiting (ClinicalTrials.gov: NCT03298763ii). In the succeeding sections, we will provide update on and discuss promising data of lung CSC markers as therapeutic targets, as well as emphasize topics for future investigations.
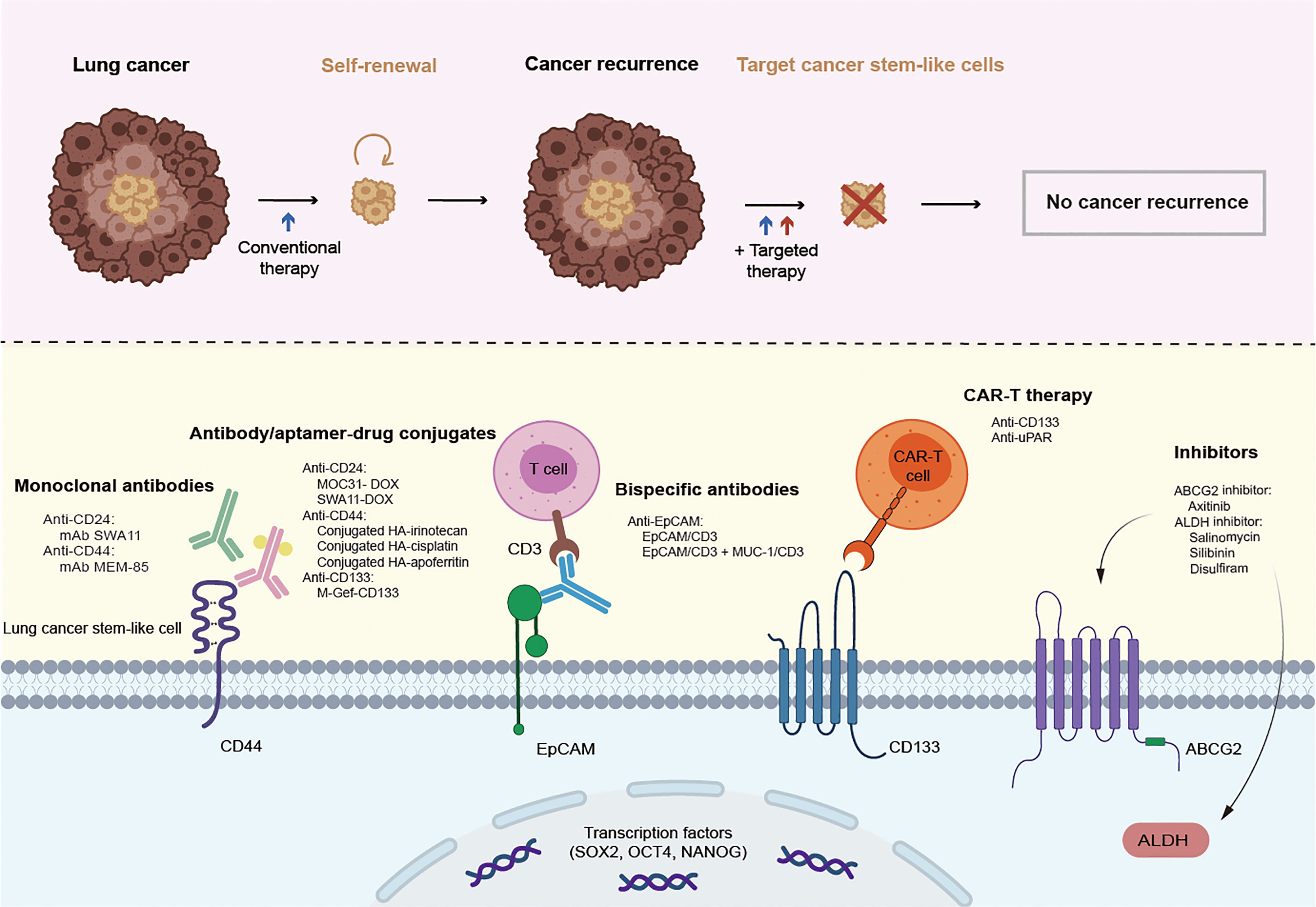
Figure 2 Promising therapies targeting lung cancer stem cell markers. CSCs are resistant to conventional therapy and lead to cancer recurrence, unless being specifically targeted. Therapies targeting lung cancer stem cell markers mainly rely on the specific recognition and binding of antibodies to lung cancer stem cell surface markers, thus leading to a variety of antibody drugs including monoclonal antibody, antibody-drug conjugate, bispecific antibody, and emerging nanoparticle drugs. In addition, novel CAR-T cell immunotherapy as well as inhibitors also play an increasingly important role in the experiments and future clinical trials.
4.1 Antibody Drugs Targeting Lung Cancer Stem Cell Markers
In recent years, antibody drugs have become the main new drugs developed because of their high specificity and low adverse reactions. They have been approved for a variety of clinical applications, as well as in the field of CSCs. Targeted therapies occur when antibodies specifically recognize and bind to certain lung CSC surface markers or signaling pathways. Antibodies targeting lung CSC markers mainly include monoclonal antibody (mAb), antibody/aptamer-drug conjugate (ADC), bispecific antibody (BsAb), and emerging nanoparticle drugs.
Therapeutic mAbs are a class of antibody drugs that have high homogeneity and pharmacological effects against a single antigen epitope. mAbs are characterized by strong targeting, low toxicity, and relatively easy development, ranking the mainstream of biotechnology drug research for a long time. At present, many mAbs targeting lung CSC markers have been used in basic experiments or clinical trials; for instance, mAb SWA11 targeting CD24 (93) and MEM-85 targeting CD44 (94). In addition, mAbs targeting lung CSC markers may strongly alter the tumor microenvironment and retard tumor growth (95).
ADCs are a class of drugs that conjugate mAbs to different numbers of small molecular cytotoxins through chemical conjugates. Chemotherapy and radiotherapy, as important complementary approaches of surgical treatment, play an active role in cancer treatment. However, chemotherapy drugs and radioactive substances can not only act on tumor cells, but also accidently injure normal tissue cells, thus causing greater adverse reactions. Fortunately, ADC drugs solve this issue by wisely conjugating cytotoxic drugs or radioactive substances to mAb, in which way ADCs combine the advantages of strong targeting and high tumor cytotoxicity and reduce the side effects to innocent normal cells. ADC drugs have also become a research hotspot for lung CSC targeting treatment in recent years. Some ADC drugs targeting CD44, such as conjugation of hyaluronan with irinotecan (96), cisplatin (97), and apoferritin (98), are effective in reducing or even eliminating stem cells in lung cancer. Numerous studies have shown that ADCs targeting CD133 (99–101), CD166 (102), EpCAM (103), and CXCR4 (104) can effectively inhibit the growth of lung CSCs. Because of the existence of the blood-brain tumor barrier in mAb treatment, systemic drugs have very limited efficacy in treating brain metastases. To address this problem, targeted drugs loaded with nanomaterials have been designed to effectively solve this problem through cross-cell and cell bypass pathways (105, 106).
BsAbs are novel antibodies that bind two different antigens simultaneously and do not exist naturally. The modified Fab fragment in the BsAb can bind to the epitope of cytotoxic drugs or the surface antigen of immune cells. Thus, BsAbs can not only target cancer cells and prevent cytotoxic effects on normal tissues, but also link with NK cells or CD8 T cells, which may eradicate cancer cells through a direct killing effect. In NSCLC, researchers have found that c-MET/CTLA-4 BsAb-targeting lung CSCs have potential therapeutic effects (107). Catumaxomab (108–110) or MuS110 (111, 112) can target EpCAM and CD3 and potentially inhibit local cancer growth. Scientists then combined EpCAM/CD3 BsAb with MUC-1/CD3 BsAb and surprisingly found greater specificity and better outcome (113), showing a possible immunotherapeutic approach.
Targeted therapy with antibody drugs brings new light to conquer tumors and CSCs. However, similar to other therapies, several concerns exist regarding safety risks, such as off-target effects, allergies, and cytokine storm. Compared with other tumors, antibody drugs for lung CSCs are few, probably because of the scarcity of suitable targets. Under these circumstances, basic and clinical research should be conducted to find more specific and better antigens toward lung CSCs and develop new carrier materials to promote the entry of antibody drugs into cancer tissue.
4.2 CAR-T Therapies Targeting Lung Cancer Stem Cell Markers
CAR-T therapy is used to isolate T cells from patients. It uses genetic engineering technology to transduce the CAR gene that specifically recognizes a tumor cell antigen into T cells, expands in vitro, and finally transplants it into patients to exert tumor-killing effect. It is MHC-free and therefore avoids tumor escape due to reduced MHC expression in tumor cells. CAR is composed of three parts: the intracellular region, which is the signaling molecule that mediates T cell activation, transmembrane region, and extracellular region, which is the variable region of mAb that can bind specific tumor antigens. As an innovative and promising therapeutic strategy, CAR-T therapy has remarkable efficacy and safety in the treatment of hematological tumors (114). It has also shed new light on solid cancer treatment, including lung cancer. However, CAR-T therapy is confronted with difficulties in treating solid tumors, mainly because of the scarcity of real tumor-specific antigens and the heterogeneity of tumor-associated antigens on the surface of solid tumors. Therefore, selecting specific antigens as targets for CAR-T cells to treat solid tumors is important, and the promising experimental and clinical use of CAR-T cells still needs more attention and effort. Given the potential to identify surface stem cell markers or antigens, CAR-T has become an appealing practice to target CSCs specifically. uPAR-specific CAR-T cells have also been reported to prolong survival and relieve liver fibrosis in mice with lung cancer (115). In SCLC, CAR-T cells targeting CD133 can migrate to cancer lesions, kill cancer cells, and improve survival, but cannot eliminate cancer cells. Combining CD133-specific CAR-T, CD73 blockage, and anti PD-1 therapy can particularly eradicate lung CSCs and may contribute to curable influence in a terminal disease (116). From this perspective, we should exert more effort to create stronger CAR-T structures and find better specific targets and therapy combination strategies.
5 Conclusions
Lung CSC is a subtle group of lung cancer cells with potential multidirectional differentiation capacity, high self-renewal, and tumorigenicity. Potential markers of lung CSCs mainly include surface biomarkers, such as CD44, CD133, EpCAM, and ABCG2, along with intracellular biomarkers, such as ALDH, SOX2, NANOG, and OCT4. These markers have different structures, but they are all related to the stem potential and uncontrollable proliferation of tumor cells. The aberrant activation of major signaling pathways, such as Notch, HH, and Wnt signaling pathways, may be related to the expression and regulation of certain lung CSC markers. For patients who are resistant to conventional lung cancer therapies, treatments targeting lung CSC markers may bring new hope. These treatments mainly include antibody drugs, nanoparticle drugs, CAR-T therapy, and other natural or synthetic specific inhibitors.
Although lung CSC theoretically provides many new ideas, the challenges brought about by it cannot be underestimated. First, the known lung CSC markers may not only exist in lung CSCs, and they merely have reference significance. lung CSCs and normal stem cells have similar functions and pathways, which makes them difficult to distinguish from each other during treatment; thus, it is difficult to show a satisfying targeting effect. More studies are needed to explore more specific markers and more targeted therapies against lung CSCs. Second, the specific molecular mechanisms and detailed signaling pathways that each lung CSC is used to control and regulate stem cell phenotypes are not well understood. Third, there is a lack of suitable animal models of lung CSCs for study. Establishing a new animal model, such as real human lung cancer, is important to clarify the mechanism of malignant transformation from lung stem cells to lung CSCs. Fourth, the tumor environmental factors and interactions between lung CSCs and other cells are poorly understood. Finally, more innovative and powerful approaches for the therapeutic targeting of lung CSCs should be explored. CAR-T therapy in solid cancer is promising but does not go smoothly, and lung CSC surface marker-specific CAR-T is rare. Identifying better CAR-T-specific targets and combination therapy against lung CSCs is important. Only continuous in-depth exploration can update the treatment and improve the prognosis of lung cancer in the future.
Author Contributions
The study idea was conceived by YL and YZ. YZ and LW were responsible for the collection and assembly of data. All authors were involved in the writing and final approval of the manuscript.
Funding
This work was supported by 1·3·5 project for disciplines of excellence, West China Hospital, Sichuan University [ZYJC21003] and the National Natural Science Foundation of China [grants 82073336].
Conflict of Interest
The authors declare that the research was conducted in the absence of any commercial or financial relationships that could be construed as a potential conflict of interest.
Publisher’s Note
All claims expressed in this article are solely those of the authors and do not necessarily represent those of their affiliated organizations, or those of the publisher, the editors and the reviewers. Any product that may be evaluated in this article, or claim that may be made by its manufacturer, is not guaranteed or endorsed by the publisher.
References
1. Clarke MF. Clinical and Therapeutic Implications of Cancer Stem Cells. N Engl J Med (2019) 380(23):2237–45. doi: 10.1056/NEJMra1804280
2. Luo YT, Cheng J, Feng X, He SJ, Wang YW, Huang Q. The Viable Circulating Tumor Cells With Cancer Stem Cells Feature, Where Is the Way Out? J Exp Clin Cancer Res (2018) 37(1):38. doi: 10.1186/s13046-018-0685-7
3. Visvader JE, Lindeman GJ. Cancer Stem Cells in Solid Tumours: Accumulating Evidence and Unresolved Questions. Nat Rev Cancer (2008) 8(10):755–68. doi: 10.1038/nrc2499
4. Hardavella G, George R, Sethi T. Lung Cancer Stem Cells-Characteristics, Phenotype. Transl Lung Cancer Res (2016) 5(3):272–9. doi: 10.21037/tlcr.2016.02.01
5. Lapidot T, Sirard C, Vormoor J, Murdoch B, Hoang T, Caceres-Cortes J, et al. A Cell Initiating Human Acute Myeloid Leukaemia After Transplantation Into Scid Mice. Nature (1994) 367(6464):645–8. doi: 10.1038/367645a0
6. Bonnet D, Dick JE. Human Acute Myeloid Leukemia Is Organized as a Hierarchy That Originates From a Primitive Hematopoietic Cell. Nat Med (1997) 3(7):730–7. doi: 10.1038/nm0797-730
7. Das PK, Pillai S, Rakib MA, Khanam JA, Gopalan V, Lam AKY, et al. Plasticity of Cancer Stem Cell: Origin and Role in Disease Progression and Therapy Resistance. Stem Cell Rev Rep (2020) 16(2):397–412. doi: 10.1007/s12015-019-09942-y
8. Goldstein LA, Zhou DF, Picker LJ, Minty CN, Bargatze RF, Ding JF, et al. A Human Lymphocyte Homing Receptor, the Hermes Antigen, Is Related to Cartilage Proteoglycan Core and Link Proteins. Cell (1989) 56(6):1063–72. doi: 10.1016/0092-8674(89)90639-9
9. Caon I, Bartolini B, Parnigoni A, Caravà E, Moretto P, Viola M, et al. Revisiting the Hallmarks of Cancer: The Role of Hyaluronan. Semin Cancer Biol (2020) 62:9–19. doi: 10.1016/j.semcancer.2019.07.007
10. Ouhtit A, Thouta R, Zayed H, Gaur RL, Fernando A, Rahman M, et al. Cd44 Mediates Stem Cell Mobilization to Damaged Lung Via Its Novel Transcriptional Targets, Cortactin and Survivin. Int J Med Sci (2020) 17(1):103–11. doi: 10.7150/ijms.33125
11. Mani SA, Guo W, Liao MJ, Eaton EN, Ayyanan A, Zhou AY, et al. The Epithelial-Mesenchymal Transition Generates Cells With Properties of Stem Cells. Cell (2008) 133(4):704–15. doi: 10.1016/j.cell.2008.03.027
12. Roudi R, Madjd Z, Korourian A, Mehrazma M, Molanae S, Sabet MN, et al. Clinical Significance of Putative Cancer Stem Cell Marker Cd44 in Different Histological Subtypes of Lung Cancer. Cancer Biomark (2014) 14(6):457–67. doi: 10.3233/cbm-140424
13. Sun D, Wu Y, Zhang S, Han Y, Shen J, Zheng W, et al. Distinct Roles of Mir-34 Family Members on Suppression of Lung Squamous Cell Carcinoma. BioMed Pharmacother (2021) 142:111967. doi: 10.1016/j.biopha.2021.111967
14. Bourguignon LY. Hyaluronan-Mediated Cd44 Activation of Rhogtpase Signaling and Cytoskeleton Function Promotes Tumor Progression. Semin Cancer Biol (2008) 18(4):251–9. doi: 10.1016/j.semcancer.2008.03.007
15. Orian-Rousseau V, Sleeman J. Cd44 Is a Multidomain Signaling Platform That Integrates Extracellular Matrix Cues With Growth Factor and Cytokine Signals. Adv Cancer Res (2014) 123:231–54. doi: 10.1016/b978-0-12-800092-2.00009-5
16. Ludwig N, Szczepanski MJ, Gluszko A, Szafarowski T, Azambuja JH, Dolg L, et al. Cd44(+) Tumor Cells Promote Early Angiogenesis in Head and Neck Squamous Cell Carcinoma. Cancer Lett (2019) 467:85–95. doi: 10.1016/j.canlet.2019.10.010
17. Kong T, Ahn R, Yang K, Zhu X, Fu Z, Morin G, et al. Cd44 Promotes Pd-L1 Expression and Its Tumor-Intrinsic Function in Breast and Lung Cancers. Cancer Res (2020) 80(3):444–57. doi: 10.1158/0008-5472.Can-19-1108
18. Akamine T, Tagawa T, Ijichi K, Toyokawa G, Takamori S, Hirai F, et al. The Significance of Cd44 Variant 9 in Resected Lung Adenocarcinoma: Correlation With Pathological Early-Stage and Egfr Mutation. Ann Surg Oncol (2019) 26(5):1544–51. doi: 10.1245/s10434-018-07137-2
19. Yin J, Zhang H, Wu X, Zhang Y, Li J, Shen J, et al. Cd44 Inhibition Attenuates Egfr Signaling and Enhances Cisplatin Sensitivity in Human Egfr Wild−Type Non−Small−Cell Lung Cancer Cells. Int J Mol Med (2020) 45(6):1783–92. doi: 10.3892/ijmm.2020.4562
20. Suda K, Murakami I, Yu H, Kim J, Tan AC, Mizuuchi H, et al. Cd44 Facilitates Epithelial-To-Mesenchymal Transition Phenotypic Change at Acquisition of Resistance to Egfr Kinase Inhibitors in Lung Cancer. Mol Cancer Ther (2018) 17(10):2257–65. doi: 10.1158/1535-7163.Mct-17-1279
21. Masciale V, Grisendi G, Banchelli F, D'Amico R, Maiorana A, Sighinolfi P, et al. Cd44+/Epcam+ Cells Detect a Subpopulation of Aldh(High) Cells in Human Non-Small Cell Lung Cancer: A Chance for Targeting Cancer Stem Cells? Oncotarget (2020) 11(17):1545–55. doi: 10.18632/oncotarget.27568
22. Weigmann A, Corbeil D, Hellwig A, Huttner WB. Prominin, a Novel Microvilli-Specific Polytopic Membrane Protein of the Apical Surface of Epithelial Cells, Is Targeted to Plasmalemmal Protrusions of Non-Epithelial Cells. Proc Natl Acad Sci USA (1997) 94(23):12425–30. doi: 10.1073/pnas.94.23.12425
23. Wen GM, Mou FF, Hou W, Wang D, Xia P. Integrative Analysis of Cd133 Mrna in Human Cancers Based on Data Mining. Stem Cell Rev Rep (2019) 15(1):23–34. doi: 10.1007/s12015-018-9865-2
24. Roy S, Lu K, Nayak MK, Bhuniya A, Ghosh T, Kundu S, et al. Activation of D2 Dopamine Receptors in Cd133+Ve Cancer Stem Cells in Non-Small Cell Lung Carcinoma Inhibits Proliferation, Clonogenic Ability, and Invasiveness of These Cells. J Biol Chem (2017) 292(2):435–45. doi: 10.1074/jbc.M116.748970
25. Jang JW, Song Y, Kim SH, Kim J, Seo HR. Potential Mechanisms of Cd133 in Cancer Stem Cells. Life Sci (2017) 184:25–9. doi: 10.1016/j.lfs.2017.07.008
26. Aghajani M, Mansoori B, Mohammadi A, Asadzadeh Z, Baradaran B. New Emerging Roles of Cd133 in Cancer Stem Cell: Signaling Pathway and Mirna Regulation. J Cell Physiol (2019) 234(12):21642–61. doi: 10.1002/jcp.28824
27. Adini A, Adini I, Ghosh K, Benny O, Pravda E, Hu R, et al. The Stem Cell Marker Prominin-1/Cd133 Interacts With Vascular Endothelial Growth Factor and Potentiates Its Action. Angiogenesis (2013) 16(2):405–16. doi: 10.1007/s10456-012-9323-8
28. Tu Z, Xie S, Xiong M, Liu Y, Yang X, Tembo KM, et al. Cxcr4 Is Involved in Cd133-Induced Emt in Non-Small Cell Lung Cancer. Int J Oncol (2017) 50(2):505–14. doi: 10.3892/ijo.2016.3812
29. Koren A, Rijavec M, Kern I, Sodja E, Korosec P, Cufer T. Bmi1, Aldh1a1, and Cd133 Transcripts Connect Epithelial-Mesenchymal Transition to Cancer Stem Cells in Lung Carcinoma. Stem Cells Int (2016) 2016:9714315. doi: 10.1155/2016/9714315
30. Iida H, Suzuki M, Goitsuka R, Ueno H. Hypoxia Induces Cd133 Expression in Human Lung Cancer Cells by Up-Regulation of Oct3/4 and Sox2. Int J Oncol (2012) 40(1):71–9. doi: 10.3892/ijo.2011.1207
31. Szala S, Kasai Y, Steplewski Z, Rodeck U, Koprowski H, Linnenbach AJ. Molecular Cloning of Cdna for the Human Tumor-Associated Antigen Co-029 and Identification of Related Transmembrane Antigens. Proc Natl Acad Sci USA (1990) 87(17):6833–7. doi: 10.1073/pnas.87.17.6833
32. Zhou N, Wang H, Liu H, Xue H, Lin F, Meng X, et al. Mta1-Upregulated Epcam Is Associated With Metastatic Behaviors and Poor Prognosis in Lung Cancer. J Exp Clin Cancer Res (2015) 34:157. doi: 10.1186/s13046-015-0263-1
33. Satar NA, Fakiruddin KS, Lim MN, Mok PL, Zakaria N, Fakharuzi NA, et al. Novel Triple−Positive Markers Identified in Human Non−Small Cell Lung Cancer Cell Line With Chemotherapy-Resistant and Putative Cancer Stem Cell Characteristics. Oncol Rep (2018) 40(2):669–81. doi: 10.3892/or.2018.6461
34. Taylor NMI, Manolaridis I, Jackson SM, Kowal J, Stahlberg H, Locher KP. Structure of the Human Multidrug Transporter Abcg2. Nature (2017) 546(7659):504–9. doi: 10.1038/nature22345
35. Kukal S, Guin D, Rawat C, Bora S, Mishra MK, Sharma P, et al. Multidrug Efflux Transporter Abcg2: Expression and Regulation. Cell Mol Life Sci (2021) 78(21-22):6887–939. doi: 10.1007/s00018-021-03901-y
36. Yang WJ, Song MJ, Park EY, Lee JJ, Park JH, Park K, et al. Transcription Factors Sp1 and Sp3 Regulate Expression of Human Abcg2 Gene and Chemoresistance Phenotype. Mol Cells (2013) 36(4):368–75. doi: 10.1007/s10059-013-0191-x
37. Zhang M, Mathur A, Zhang Y, Xi S, Atay S, Hong JA, et al. Mithramycin Represses Basal and Cigarette Smoke-Induced Expression of Abcg2 and Inhibits Stem Cell Signaling in Lung and Esophageal Cancer Cells. Cancer Res (2012) 72(16):4178–92. doi: 10.1158/0008-5472.Can-11-3983
38. Zhao W, Luo Y, Li B, Zhang T. Tumorigenic Lung Tumorospheres Exhibit Stem-Like Features With Significantly Increased Expression of Cd133 and Abcg2. Mol Med Rep (2016) 14(3):2598–606. doi: 10.3892/mmr.2016.5524
39. Lei HM, Zhang KR, Wang CH, Wang Y, Zhuang GL, Lu LM, et al. Aldehyde Dehydrogenase 1a1 Confers Erlotinib Resistance Via Facilitating the Reactive Oxygen Species-Reactive Carbonyl Species Metabolic Pathway in Lung Adenocarcinomas. Theranostics (2019) 9(24):7122–39. doi: 10.7150/thno.35729
40. Kim G, Azmi L, Jang S, Jung T, Hebert H, Roe AJ, et al. Aldehyde-Alcohol Dehydrogenase Forms a High-Order Spirosome Architecture Critical for Its Activity. Nat Commun (2019) 10(1):4527. doi: 10.1038/s41467-019-12427-8
41. de Aberasturi AL, Redrado M, Villalba M, Larzabal L, Pajares MJ, Garcia J, et al. Tmprss4 Induces Cancer Stem Cell-Like Properties in Lung Cancer Cells and Correlates With Aldh Expression in Nsclc Patients. Cancer Lett (2016) 370(2):165–76. doi: 10.1016/j.canlet.2015.10.012
42. Casagrande N, Borghese C, Agostini F, Durante C, Mazzucato M, Colombatti A, et al. In Ovarian Cancer Multicellular Spheroids, Platelet Releasate Promotes Growth, Expansion of Aldh+ and Cd133+ Cancer Stem Cells, and Protection Against the Cytotoxic Effects of Cisplatin, Carboplatin and Paclitaxel. Int J Mol Sci (2021) 22(6):3019. doi: 10.3390/ijms22063019
43. Wegner M. All Purpose Sox: The Many Roles of Sox Proteins in Gene Expression. Int J Biochem Cell Biol (2010) 42(3):381–90. doi: 10.1016/j.biocel.2009.07.006
44. Ferone G, Song JY, Sutherland KD, Bhaskaran R, Monkhorst K, Lambooij JP, et al. Sox2 Is the Determining Oncogenic Switch in Promoting Lung Squamous Cell Carcinoma From Different Cells of Origin. Cancer Cell (2016) 30(4):519–32. doi: 10.1016/j.ccell.2016.09.001
45. Wang K, Ji W, Yu Y, Li Z, Niu X, Xia W, et al. Fgfr1-Erk1/2-Sox2 Axis Promotes Cell Proliferation, Epithelial-Mesenchymal Transition, and Metastasis in Fgfr1-Amplified Lung Cancer. Oncogene (2018) 37(39):5340–54. doi: 10.1038/s41388-018-0311-3
46. Fukazawa T, Guo M, Ishida N, Yamatsuji T, Takaoka M, Yokota E, et al. Sox2 Suppresses Cdkn1a to Sustain Growth of Lung Squamous Cell Carcinoma. Sci Rep (2016) 6:20113. doi: 10.1038/srep20113
47. Drilon A, Rekhtman N, Ladanyi M, Paik P. Squamous-Cell Carcinomas of the Lung: Emerging Biology, Controversies, and the Promise of Targeted Therapy. Lancet Oncol (2012) 13(10):e418–26. doi: 10.1016/s1470-2045(12)70291-7
48. Tripathi SC, Fahrmann JF, Celiktas M, Aguilar M, Marini KD, Jolly MK, et al. Mcam Mediates Chemoresistance in Small-Cell Lung Cancer Via the Pi3k/Akt/Sox2 Signaling Pathway. Cancer Res (2017) 77(16):4414–25. doi: 10.1158/0008-5472.Can-16-2874
49. Mehravar M, Ghaemimanesh F, Poursani EM. An Overview on the Complexity of Oct4: At the Level of DNA, Rna and Protein. Stem Cell Rev Rep (2021) 17(4):1121–36. doi: 10.1007/s12015-020-10098-3
50. Mohiuddin IS, Wei SJ, Kang MH. Role of Oct4 in Cancer Stem-Like Cells and Chemotherapy Resistance. Biochim Biophys Acta Mol Basis Dis (2020) 1866(4):165432. doi: 10.1016/j.bbadis.2019.03.005
51. Jen J, Tang YA, Lu YH, Lin CC, Lai WW, Wang YC. Oct4 Transcriptionally Regulates the Expression of Long Non-Coding Rnas Neat1 and Malat1 to Promote Lung Cancer Progression. Mol Cancer (2017) 16(1):104. doi: 10.1186/s12943-017-0674-z
52. Liu S, Cheng K, Zhang H, Kong R, Wang S, Mao C, et al. Methylation Status of the Nanog Promoter Determines the Switch Between Cancer Cells and Cancer Stem Cells. Adv Sci (Weinh) (2020) 7(5):1903035. doi: 10.1002/advs.201903035
53. Hayashi Y, Caboni L, Das D, Yumoto F, Clayton T, Deller MC, et al. Structure-Based Discovery of Nanog Variant With Enhanced Properties to Promote Self-Renewal and Reprogramming of Pluripotent Stem Cells. Proc Natl Acad Sci USA (2015) 112(15):4666–71. doi: 10.1073/pnas.1502855112
54. Chang B, Park MJ, Choi SI, In KH, Kim CH, Lee SH. Nanog as an Adverse Predictive Marker in Advanced Non-Small Cell Lung Cancer Treated With Platinum-Based Chemotherapy. Onco Targets Ther (2017) 10:4625–33. doi: 10.2147/ott.S144895
55. Clara JA, Monge C, Yang Y, Takebe N. Targeting Signalling Pathways and the Immune Microenvironment of Cancer Stem Cells - A Clinical Update. Nat Rev Clin Oncol (2020) 17(4):204–32. doi: 10.1038/s41571-019-0293-2
56. Wang H, Zang C, Liu XS, Aster JC. The Role of Notch Receptors in Transcriptional Regulation. J Cell Physiol (2015) 230(5):982–8. doi: 10.1002/jcp.24872
57. Majumder S, Crabtree JS, Golde TE, Minter LM, Osborne BA, Miele L. Targeting Notch in Oncology: The Path Forward. Nat Rev Drug Discov (2021) 20(2):125–44. doi: 10.1038/s41573-020-00091-3
58. Sullivan JP, Spinola M, Dodge M, Raso MG, Behrens C, Gao B, et al. Aldehyde Dehydrogenase Activity Selects for Lung Adenocarcinoma Stem Cells Dependent on Notch Signaling. Cancer Res (2010) 70(23):9937–48. doi: 10.1158/0008-5472.Can-10-0881
59. Liu YP, Yang CJ, Huang MS, Yeh CT, Wu AT, Lee YC, et al. Cisplatin Selects for Multidrug-Resistant Cd133+ Cells in Lung Adenocarcinoma by Activating Notch Signaling. Cancer Res (2013) 73(1):406–16. doi: 10.1158/0008-5472.Can-12-1733
60. Katoh M, Katoh M. Precision Medicine for Human Cancers With Notch Signaling Dysregulation (Review). Int J Mol Med (2020) 45(2):279–97. doi: 10.3892/ijmm.2019.4418
61. Zhang K, Hong X, Song Z, Xu Y, Li C, Wang G, et al. Identification of Deleterious Notch Mutation as Novel Predictor to Efficacious Immunotherapy in Nsclc. Clin Cancer Res (2020) 26(14):3649–61. doi: 10.1158/1078-0432.Ccr-19-3976
62. Nguyen D, Rubinstein L, Takebe N, Miele L, Tomaszewski JE, Ivy P, et al. Notch1 Phenotype and Clinical Stage Progression in Non-Small Cell Lung Cancer. J Hematol Oncol (2015) 8:9. doi: 10.1186/s13045-014-0104-2
63. Zhang Y, Xu W, Guo H, Zhang Y, He Y, Lee SH, et al. Notch1 Signaling Regulates Self-Renewal and Platinum Chemoresistance of Cancer Stem-Like Cells in Human Non-Small Cell Lung Cancer. Cancer Res (2017) 77(11):3082–91. doi: 10.1158/0008-5472.Can-16-1633
64. Lim JS, Ibaseta A, Fischer MM, Cancilla B, O'Young G, Cristea S, et al. Intratumoural Heterogeneity Generated by Notch Signalling Promotes Small-Cell Lung Cancer. Nature (2017) 545(7654):360–4. doi: 10.1038/nature22323
65. Morimoto M, Nishinakamura R, Saga Y, Kopan R. Different Assemblies of Notch Receptors Coordinate the Distribution of the Major Bronchial Clara, Ciliated and Neuroendocrine Cells. Development (2012) 139(23):4365–73. doi: 10.1242/dev.083840
66. Tsao PN, Vasconcelos M, Izvolsky KI, Qian J, Lu J, Cardoso WV. Notch Signaling Controls the Balance of Ciliated and Secretory Cell Fates in Developing Airways. Development (2009) 136(13):2297–307. doi: 10.1242/dev.034884
67. George J, Lim JS, Jang SJ, Cun Y, Ozretić L, Kong G, et al. Comprehensive Genomic Profiles of Small Cell Lung Cancer. Nature (2015) 524(7563):47–53. doi: 10.1038/nature14664
68. Pietanza MC, Byers LA, Minna JD, Rudin CM. Small Cell Lung Cancer: Will Recent Progress Lead to Improved Outcomes? Clin Cancer Res (2015) 21(10):2244–55. doi: 10.1158/1078-0432.Ccr-14-2958
69. Liu J, Mao Z, Huang J, Xie S, Liu T, Mao Z. Blocking the Notch Pathway Can Inhibit the Growth of Cd133-Positive A549 Cells and Sensitize to Chemotherapy. Biochem Biophys Res Commun (2014) 444(4):670–5. doi: 10.1016/j.bbrc.2014.01.164
70. Chapouly C, Guimbal S, Hollier PL, Renault MA. Role of Hedgehog Signaling in Vasculature Development, Differentiation, and Maintenance. Int J Mol Sci (2019) 20(12):3076. doi: 10.3390/ijms20123076
71. Watkins DN, Berman DM, Burkholder SG, Wang B, Beachy PA, Baylin SB. Hedgehog Signalling Within Airway Epithelial Progenitors and in Small-Cell Lung Cancer. Nature (2003) 422(6929):313–7. doi: 10.1038/nature01493
72. Szczepny A, Rogers S, Jayasekara WSN, Park K, McCloy RA, Cochrane CR, et al. The Role of Canonical and Non-Canonical Hedgehog Signaling in Tumor Progression in a Mouse Model of Small Cell Lung Cancer. Oncogene (2017) 36(39):5544–50. doi: 10.1038/onc.2017.173
73. Yue D, Li H, Che J, Zhang Y, Tseng HH, Jin JQ, et al. Hedgehog/Gli Promotes Epithelial-Mesenchymal Transition in Lung Squamous Cell Carcinomas. J Exp Clin Cancer Res (2014) 33(1):34. doi: 10.1186/1756-9966-33-34
74. De Craene B, Berx G. Regulatory Networks Defining Emt During Cancer Initiation and Progression. Nat Rev Cancer (2013) 13(2):97–110. doi: 10.1038/nrc3447
75. Bora-Singhal N, Perumal D, Nguyen J, Chellappan S. Gli1-Mediated Regulation of Sox2 Facilitates Self-Renewal of Stem-Like Cells and Confers Resistance to Egfr Inhibitors in Non-Small Cell Lung Cancer. Neoplasia (2015) 17(7):538–51. doi: 10.1016/j.neo.2015.07.001
76. Justilien V, Walsh MP, Ali SA, Thompson EA, Murray NR, Fields AP. The Prkci and Sox2 Oncogenes Are Coamplified and Cooperate to Activate Hedgehog Signaling in Lung Squamous Cell Carcinoma. Cancer Cell (2014) 25(2):139–51. doi: 10.1016/j.ccr.2014.01.008
77. Nusse R, Clevers H. Wnt/β-Catenin Signaling, Disease, and Emerging Therapeutic Modalities. Cell (2017) 169(6):985–99. doi: 10.1016/j.cell.2017.05.016
78. Katoh M, Katoh M. Molecular Genetics and Targeted Therapy of Wnt-Related Human Diseases (Review). Int J Mol Med (2017) 40(3):587–606. doi: 10.3892/ijmm.2017.3071
79. Bugter JM, Fenderico N, Maurice MM. Mutations and Mechanisms of Wnt Pathway Tumour Suppressors in Cancer. Nat Rev Cancer (2021) 21(1):5–21. doi: 10.1038/s41568-020-00307-z
80. Dong HJ, Jang GB, Lee HY, Park SR, Kim JY, Nam JS, et al. The Wnt/β-Catenin Signaling/Id2 Cascade Mediates the Effects of Hypoxia on the Hierarchy of Colorectal-Cancer Stem Cells. Sci Rep (2016) 6:22966. doi: 10.1038/srep22966
81. Kumar B, Adebayo AK, Prasad M, Capitano ML, Wang R, Bhat-Nakshatri P, et al. Tumor Collection/Processing Under Physioxia Uncovers Highly Relevant Signaling Networks and Drug Sensitivity. Sci Adv (2022) 8(2):eabh3375. doi: 10.1126/sciadv.abh3375
82. Cabarcas SM, Mathews LA, Farrar WL. The Cancer Stem Cell Niche–There Goes the Neighborhood? Int J Cancer (2011) 129(10):2315–27. doi: 10.1002/ijc.26312
83. Marusyk A, Almendro V, Polyak K. Intra-Tumour Heterogeneity: A Looking Glass for Cancer? Nat Rev Cancer (2012) 12(5):323–34. doi: 10.1038/nrc3261
84. Jin J, Zhan P, Qian H, Wang X, Katoh M, Phan K, et al. Prognostic Value of Wingless-Type Proteins in Non-Small Cell Lung Cancer Patients: A Meta-Analysis. Transl Lung Cancer Res (2016) 5(4):436–42. doi: 10.21037/tlcr.2016.08.08
85. Reynolds SD, Zemke AC, Giangreco A, Brockway BL, Teisanu RM, Drake JA, et al. Conditional Stabilization of Beta-Catenin Expands the Pool of Lung Stem Cells. Stem Cells (2008) 26(5):1337–46. doi: 10.1634/stemcells.2008-0053
86. Yang J, Chen J, He J, Li J, Shi J, Cho WC, et al. Wnt Signaling as Potential Therapeutic Target in Lung Cancer. Expert Opin Ther Targets (2016) 20(8):999–1015. doi: 10.1517/14728222.2016.1154945
87. Zhang X, Lou Y, Wang H, Zheng X, Dong Q, Sun J, et al. Wnt Signaling Regulates the Stemness of Lung Cancer Stem Cells and Its Inhibitors Exert Anticancer Effect on Lung Cancer Spc-A1 Cells. Med Oncol (2015) 32(4):95. doi: 10.1007/s12032-014-0462-1
88. Vesel M, Rapp J, Feller D, Kiss E, Jaromi L, Meggyes M, et al. Abcb1 and Abcg2 Drug Transporters Are Differentially Expressed in Non-Small Cell Lung Cancers (Nsclc) and Expression Is Modified by Cisplatin Treatment Via Altered Wnt Signaling. Respir Res (2017) 18(1):52. doi: 10.1186/s12931-017-0537-6
89. Teng Y, Wang X, Wang Y, Ma D. Wnt/Beta-Catenin Signaling Regulates Cancer Stem Cells in Lung Cancer A549 Cells. Biochem Biophys Res Commun (2010) 392(3):373–9. doi: 10.1016/j.bbrc.2010.01.028
90. Yang J, Zhang K, Wu J, Shi J, Xue J, Li J, et al. Wnt5a Increases Properties of Lung Cancer Stem Cells and Resistance to Cisplatin Through Activation of Wnt5a/Pkc Signaling Pathway. Stem Cells Int (2016) 2016:1690896. doi: 10.1155/2016/1690896
91. Chang YW, Su YJ, Hsiao M, Wei KC, Lin WH, Liang CL, et al. Diverse Targets of β-Catenin During the Epithelial-Mesenchymal Transition Define Cancer Stem Cells and Predict Disease Relapse. Cancer Res (2015) 75(16):3398–410. doi: 10.1158/0008-5472.Can-14-3265
92. Su YJ, Chang YW, Lin WH, Liang CL, Lee JL. An Aberrant Nuclear Localization of E-Cadherin Is a Potent Inhibitor of Wnt/β-Catenin-Elicited Promotion of the Cancer Stem Cell Phenotype. Oncogenesis (2015) 4(6):e157. doi: 10.1038/oncsis.2015.17
93. Salnikov AV, Bretz NP, Perne C, Hazin J, Keller S, Fogel M, et al. Antibody Targeting of Cd24 Efficiently Retards Growth and Influences Cytokine Milieu in Experimental Carcinomas. Br J Cancer (2013) 108(7):1449–59. doi: 10.1038/bjc.2013.102
94. Škerlová J, Král V, Kachala M, Fábry M, Bumba L, Svergun DI, et al. Molecular Mechanism for the Action of the Anti-Cd44 Monoclonal Antibody Mem-85. J Struct Biol (2015) 191(2):214–23. doi: 10.1016/j.jsb.2015.06.005
95. Cao K, Pan Y, Yu L, Shu X, Yang J, Sun L, et al. Monoclonal Antibodies Targeting Non-Small Cell Lung Cancer Stem-Like Cells by Multipotent Cancer Stem Cell Monoclonal Antibody Library. Int J Oncol (2017) 50(2):587–96. doi: 10.3892/ijo.2016.3818
96. Alamgeer M, Neil Watkins D, Banakh I, Kumar B, Gough DJ, Markman B, et al. A Phase Iia Study of Ha-Irinotecan, Formulation of Hyaluronic Acid and Irinotecan Targeting Cd44 in Extensive-Stage Small Cell Lung Cancer. Invest New Drugs (2018) 36(2):288–98. doi: 10.1007/s10637-017-0555-8
97. Quan YH, Kim B, Park JH, Choi Y, Choi YH, Kim HK. Highly Sensitive and Selective Anticancer Effect by Conjugated Ha-Cisplatin in Non-Small Cell Lung Cancer Overexpressed With Cd44. Exp Lung Res (2014) 40(10):475–84. doi: 10.3109/01902148.2014.905656
98. Luo Y, Wang X, Du D, Lin Y. Hyaluronic Acid-Conjugated Apoferritin Nanocages for Lung Cancer Targeted Drug Delivery. Biomater Sci (2015) 3(10):1386–94. doi: 10.1039/c5bm00067j
99. Zhou J, Sun J, Chen H, Peng Q. Promoted Delivery of Salinomycin Sodium to Lung Cancer Cells by Dual Targeting Plga Hybrid Nanoparticles. Int J Oncol (2018) 53(3):1289–300. doi: 10.3892/ijo.2018.4474
100. Ma J, Zhuang H, Zhuang Z, Lu Y, Xia R, Gan L, et al. Development of Docetaxel Liposome Surface Modified With Cd133 Aptamers for Lung Cancer Targeting. Artif Cells Nanomed Biotechnol (2018) 46(8):1864–71. doi: 10.1080/21691401.2017.1394874
101. Huang X, Huang J, Leng D, Yang S, Yao Q, Sun J, et al. Gefitinib-Loaded Dspe-Peg2000 Nanomicelles With Cd133 Aptamers Target Lung Cancer Stem Cells. World J Surg Oncol (2017) 15(1):167. doi: 10.1186/s12957-017-1230-4
102. Chomet M, Schreurs M, Nguyen M, Howng B, Villanueva R, Krimm M, et al. The Tumor Targeting Performance of Anti-Cd166 Probody Drug Conjugate Cx-2009 and Its Parental Derivatives as Monitored by (89)Zr-Immuno-Pet in Xenograft Bearing Mice. Theranostics (2020) 10(13):5815–28. doi: 10.7150/thno.44334
103. Alibolandi M, Ramezani M, Abnous K, Sadeghi F, Atyabi F, Asouri M, et al. In Vitro and in Vivo Evaluation of Therapy Targeting Epithelial-Cell Adhesion-Molecule Aptamers for Non-Small Cell Lung Cancer. J Control Release (2015) 209:88–100. doi: 10.1016/j.jconrel.2015.04.026
104. Chittasupho C, Lirdprapamongkol K, Kewsuwan P, Sarisuta N. Targeted Delivery of Doxorubicin to A549 Lung Cancer Cells by Cxcr4 Antagonist Conjugated Plga Nanoparticles. Eur J Pharm Biopharm (2014) 88(2):529–38. doi: 10.1016/j.ejpb.2014.06.020
105. Jovčevska I, Muyldermans S. The Therapeutic Potential of Nanobodies. BioDrugs (2020) 34(1):11–26. doi: 10.1007/s40259-019-00392-z
106. Shah N, Mohammad AS, Saralkar P, Sprowls SA, Vickers SD, John D, et al. Investigational Chemotherapy and Novel Pharmacokinetic Mechanisms for the Treatment of Breast Cancer Brain Metastases. Pharmacol Res (2018) 132:47–68. doi: 10.1016/j.phrs.2018.03.021
107. Li JF, Niu YY, Xing YL, Liu F. A Novel Bispecific C-Met/Ctla-4 Antibody Targetting Lung Cancer Stem Cell-Like Cells With Therapeutic Potential in Human Non-Small-Cell Lung Cancer. Biosci Rep (2019) 39(5):BSR20171278. doi: 10.1042/bsr20171278
108. Amann M, Friedrich M, Lutterbuese P, Vieser E, Lorenczewski G, Petersen L, et al. Therapeutic Window of an Epcam/Cd3-Specific Bite Antibody in Mice Is Determined by a Subpopulation of Epcam-Expressing Lymphocytes That Is Absent in Humans. Cancer Immunol Immunother (2009) 58(1):95–109. doi: 10.1007/s00262-008-0529-y
109. Amann M, D'Argouges S, Lorenczewski G, Brischwein K, Kischel R, Lutterbuese R, et al. Antitumor Activity of an Epcam/Cd3-Bispecific Bite Antibody During Long-Term Treatment of Mice in the Absence of T-Cell Anergy and Sustained Cytokine Release. J Immunother (2009) 32(5):452–64. doi: 10.1097/CJI.0b013e3181a1c097
110. Amann M, Brischwein K, Lutterbuese P, Parr L, Petersen L, Lorenczewski G, et al. Therapeutic Window of Mus110, a Single-Chain Antibody Construct Bispecific for Murine Epcam and Murine Cd3. Cancer Res (2008) 68(1):143–51. doi: 10.1158/0008-5472.Can-07-2182
111. Sebastian M, Passlick B, Friccius-Quecke H, Jäger M, Lindhofer H, Kanniess F, et al. Treatment of Non-Small Cell Lung Cancer Patients With the Trifunctional Monoclonal Antibody Catumaxomab (Anti-Epcam X Anti-Cd3): A Phase I Study. Cancer Immunol Immunother (2007) 56(10):1637–44. doi: 10.1007/s00262-007-0310-7
112. Schlereth B, Kleindienst P, Fichtner I, Lorenczewski G, Brischwein K, Lippold S, et al. Potent Inhibition of Local and Disseminated Tumor Growth in Immunocompetent Mouse Models by a Bispecific Antibody Construct Specific for Murine Cd3. Cancer Immunol Immunother (2006) 55(7):785–96. doi: 10.1007/s00262-005-0082-x
113. Wang C, Chen S, Wu Y, Wu D, Wang J, Li F. The Combination Therapy With Epcam/Cd3 Bsab and Muc-1/Cd3 Bsab Elicited Antitumor Immunity by T-Cell Adoptive Immunotherapy in Lung Cancer. Int J Med Sci (2021) 18(15):3380–8. doi: 10.7150/ijms.61681
114. Elsallab M, Levine BL, Wayne AS, Abou-El-Enein M. Car T-Cell Product Performance in Haematological Malignancies Before and After Marketing Authorisation. Lancet Oncol (2020) 21(2):e104–e16. doi: 10.1016/s1470-2045(19)30729-6
115. Amor C, Feucht J, Leibold J, Ho YJ, Zhu C, Alonso-Curbelo D, et al. Senolytic Car T Cells Reverse Senescence-Associated Pathologies. Nature (2020) 583(7814):127–32. doi: 10.1038/s41586-020-2403-9
Keywords: cancer stem cell, lung cancer, marker, signaling pathway, targeted therapy
Citation: Zheng Y, Wang L, Yin L, Yao Z, Tong R, Xue J and Lu Y (2022) Lung Cancer Stem Cell Markers as Therapeutic Targets: An Update on Signaling Pathways and Therapies. Front. Oncol. 12:873994. doi: 10.3389/fonc.2022.873994
Received: 11 February 2022; Accepted: 25 April 2022;
Published: 26 May 2022.
Edited by:
Ira Ida Skvortsova, Innsbruck Medical University, AustriaReviewed by:
Maria Fernanda Rodrigues, Universidade Nove de Julho, BrazilSouzan Najafi, Tabriz University of Medical Sciences, Iran
Theodoros Karnavas, Alexander Fleming Biomedical Sciences Research Center, Greece
Copyright © 2022 Zheng, Wang, Yin, Yao, Tong, Xue and Lu. This is an open-access article distributed under the terms of the Creative Commons Attribution License (CC BY). The use, distribution or reproduction in other forums is permitted, provided the original author(s) and the copyright owner(s) are credited and that the original publication in this journal is cited, in accordance with accepted academic practice. No use, distribution or reproduction is permitted which does not comply with these terms.
*Correspondence: You Lu, cmFkeW91bHVAaG90bWFpbC5jb20=