- 1Pathological Diagnosis and Research Center, The Affiliated Hospital of Guangdong Medical University, Zhanjiang, China
- 2Department of Urology, The University of Kansas Medical Center, Kansas City, KS, United States
Prostate cancer is a major health issue in western countries and is the second leading cause of cancer death in American men. Prostate cancer depends on the androgen receptor (AR), a transcriptional factor critical for prostate cancer growth and progression. Castration by surgery or medical treatment reduces androgen levels, resulting in prostatic atrophy and prostate cancer regression. Thus, metastatic prostate cancers are initially managed with androgen deprivation therapy. Unfortunately, prostate cancers rapidly relapse after castration therapy and progress to a disease stage called castration-resistant prostate cancer (CRPC). Currently, clinical treatment for CRPCs is focused on suppressing AR activity with antagonists like Enzalutamide or by reducing androgen production with Abiraterone. In clinical practice, these treatments fail to yield a curative benefit in CRPC patients in part due to AR gene mutations or splicing variations, resulting in AR reactivation. It is conceivable that eliminating the AR protein in prostate cancer cells is a promising solution to provide a potential curative outcome. Multiple strategies have emerged, and several potent agents that reduce AR protein levels were reported to eliminate xenograft tumor growth in preclinical models via distinct mechanisms, including proteasome-mediated degradation, heat-shock protein inhibition, AR splicing suppression, blockage of AR nuclear localization, AR N-terminal suppression. A few small chemical compounds are undergoing clinical trials combined with existing AR antagonists. AR protein elimination by enhanced protein or mRNA degradation is a realistic solution for avoiding AR reactivation during androgen deprivation therapy in prostate cancers.
Introduction
Prostate cancer is the second most common type of cancer diagnosed in men worldwide and the second leading cause of male cancer-related deaths in the U.S. (1). The American Cancer Society estimates about 268,490 new cases of prostate cancer and about 34,500 deaths from prostate cancer in the U.S. this year (1). According to the American Cancer Society data (cancer.org), patients with local or regional stage prostate cancer have nearly a 100% 5-year survival rate; however, the survival rate is only 30% for men diagnosed with distal metastasis.
Currently, localized prostate cancer is primarily treated with surgical removal of the gland or radiation therapy if a patient’s condition is not permissive for surgery. Distal metastasis occurs in high-risk patients, including locally advanced (positive surgical margin) or high-grade (Gleason sum score ≥ 8) tumors, which is the sole cause of death from prostate cancer (2). This short review work will discuss the current treatment options and recent development of anti-androgen receptor (AR) therapeutic approaches for metastatic prostate cancer (Table 1 and Figure 1).
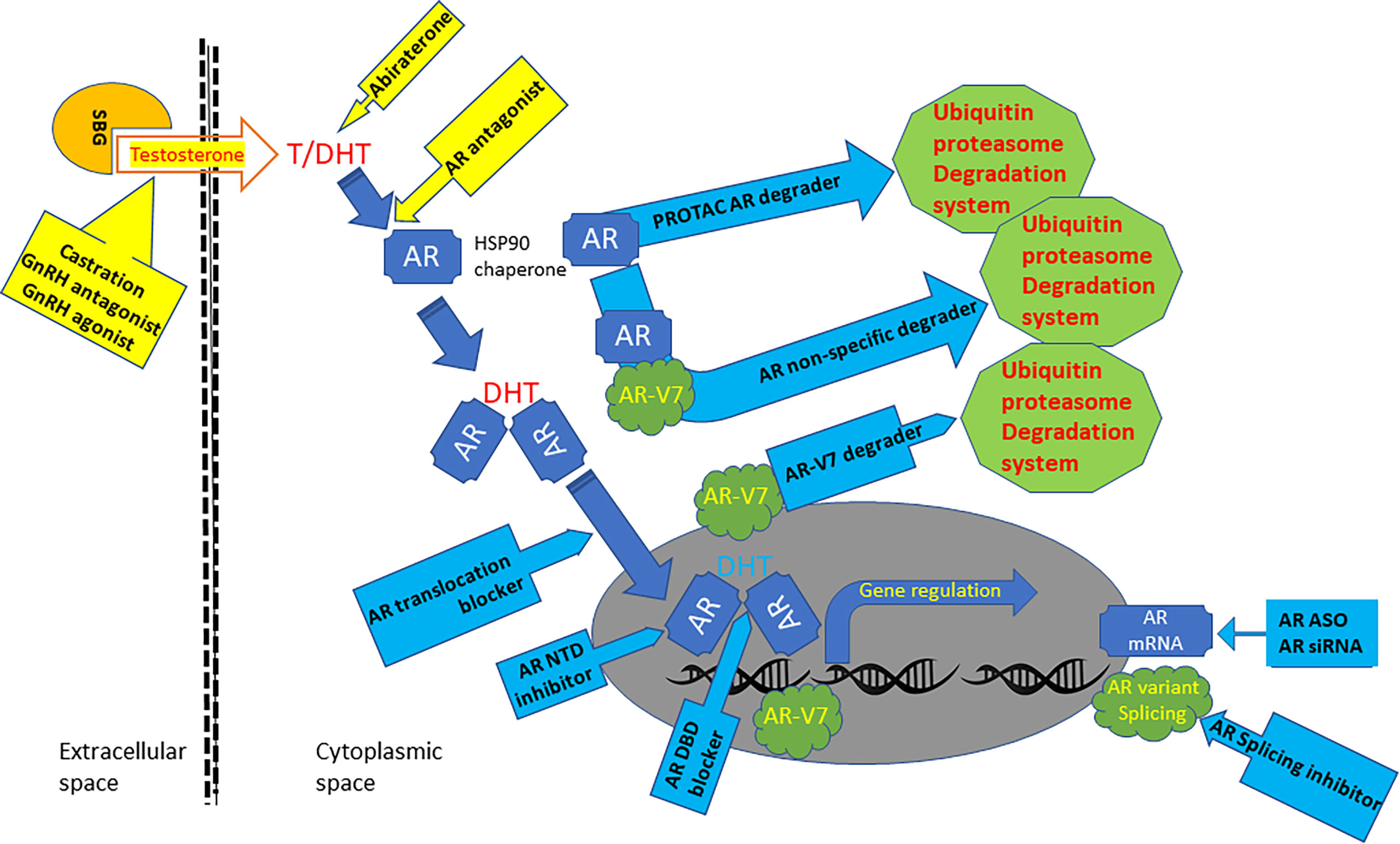
Figure 1 Graphic scheme of AR-targeted agents. Androgens are bonded with steroid-binding globulins (SBG) in the bloodstream for systemic circulation. Androgen testosterone (T) is converted to potent form dihydrotestosterone (DHT) in the cytoplasm by 5a-reductase. The AR protein bonds with HSP90 chaperones and resides in the cytoplasmic compartment before androgen binding. Androgen binding alters AR conformation and promotes its translocation into the nuclear compartment, where it interacts with chromatin DNA to regulate gene expression. AR gene mRNA is aberrantly spliced in advanced prostate cancers to generate variant proteins like AR-V7, which is constantly active without androgen binding. Current clinical therapies for metastatic prostate cancers (yellow background box) include castration, GnRH agonist and antagonist, Abiraterone, and AR antagonists. Several AR-targeted treatments under development (blue background box) include AR PROTAC and non-specific degraders, AR-V7 degraders, AR-NTD inhibitor, AR-DBD blocker, AR nuclear translocation blockers, AR splicing inhibitors.
Androgen Deprivation and Anti-Androgen Therapies in the Clinic
Metastatic prostate cancers are initially treated with androgen deprivation therapy (ADT) because prostate tissue (benign or malignant) expresses androgen receptor (AR) protein that is critical for prostate cancer development and progression (3, 4). Castration by surgery or medical treatment reduces androgen hormones, resulting in prostatic atrophy and cancer regression (5). This approach was developed eighty years ago in 1941 (3, 4). Since then, prostate cancer treatment has been mainly focused on reducing androgen levels and blocking androgen-induced AR activation (5). However, prostate cancers often relapse and progress to a stage termed as castration-resistant prostate cancers (CRPC) (67, 68), and the majority of these CRPCs still depend on the AR signaling for growth and progression (the AR addictiveness) (69, 70).
The mechanisms for CRPC progression include AR gene mutation, amplification, transcriptional splicing, and crosstalks with cellular signal pathways, plus de novo androgen synthesis by the malignant prostate cells (5). Therefore, clinical therapies use anti-androgens (Flutamide, Bicalutamide, Enzalutamide, Apalutamide, and Darolutamide) to competitively suppress androgen-induced AR activation or CYP17A1 inhibitor (Abiraterone) to reduce androgen production in prostate cancer tissues (5). So far in the clinic, these therapies provided certain clinical benefits of survival extension in CRPC patients (71). However, with the widespread use of Enzalutamide and Abiraterone, a subset of CRPC patients developed neuroendocrine progression, termed as anti-AR treatment-induced NEPC (t-NEPC) (72, 73), accounting for more than 25-30% mortality of CRPC fatality (74). There were multiple mechanisms involved in NEPC progression, including attenuated control of transcriptional factors, metabolic alterations, aberrant activation of cellular kinases, long noncoding RNAs, transcriptional splicing, and epigenetic modifications (75–87). It is postulated that extensive stress of AR inhibition under the long-term ADT condition forced an epigenetic reprogramming of CRPC cells into neuroendocrinal trans-differentiation (88–93). Treatment option for NEPC patients is limited in the clinic and the salvage platinum-based chemotherapy only provided very little survival benefit (75).
AR Protein Elimination Approaches in Preclinical Development Phase
The AR protein is a nuclear receptor expressed in benign and malignant prostate tissues, critical for prostate physiological functionality and prostate cancer progression (94, 95). As a transcriptional factor, the AR protein modulates gene expression after being activated by androgens via binding on its C-terminal ligand-binding domain (95). Given that hormone therapy, including ADT and anti-androgens for the last eighty years, has been failed to be a curable approach for metastatic prostate cancers, eliminating the AR protein in prostate cancer cells recently emerged as a realistic solution for a potentially curable result.
Antisense Oligonucleotide Technology
Antisense oligonucleotides (ASOs) are synthetic complementary single-stranded deoxyribonucleotides used to target messenger RNA (mRNA) of targeted genes, resulting in RNase H endonuclease-dependent mRNA cleavage or blockage of protein translation (6). Dr. Klocker’s group reported the first study using the ASO technology against the AR gene in 2000, which showed a suppressive effect on prostate cancer LNCaP cell growth (7). A follow-up study by the same group showed the in vivo effectiveness of suppressing LNCaP-derived xenograft tumors in nude mice (8). These initial results were supported by the studies from other groups (9, 10). Possibly due to the suppressive nature of ASOs on target gene expression, the AR protein was not eliminated from cancer cells. Also, the results only showed a moderate suppressive effect on tumor growth because of the difficulty in tissue delivery of the ASO molecules. However, these AR-targeted ASOs showed an enhanced effect when combined with other gene targets (EZH2 or Clusterin) for Enzalutamide-resistant CRPC models (11–14). A recent report achieved a successful in vivo delivery of AR-specific ASO using lipid-based nanotechnology. A profound suppressive effect was achieved in the prostate cancer xenograft model, together with a significant reduction of the AR protein levels in xenograft tumor tissues (96).
Small Interfering RNA Technology
Since the introduction of small interfering RNA (siRNA) technology in 2001 (97, 98), knocking down gene expression in living organisms became possible. To overcome the clinical obstacle of anti-AR treatment resistance, we hypothesized that eliminating AR protein from prostate cancer cells might completely shut down AR signaling, leading to cell death or growth arrest. Knocking down AR gene expression in prostate cancer cells resulted in profound apoptotic cell death in multiple prostate cancer cell lines, androgen-responsive or castration-resistant (15). Nanoparticle-based prostate cancer-specific delivery approach and adenoviral approach to systemically deliver the AR siRNA expression particles documented a rapid xenograft tumor regression and eradication owing to robust cell death in vivo (16, 17). These findings were overwhelmingly supported by reports from other groups using divergent approaches to knock down AR gene expression (18–23). These results confirmed that eliminating AR protein (full length or truncated) will overcome treatment resistance in advanced prostate cancers.
PROTAC Technology
PROTAC stands for proteolysis targeting chimera. It uses a small bifunctional molecule with two binding moieties connected by a linker to bring together a targeted protein and cellular proteolytic machinery, ubiquitin E3 ligase-mediated proteasome degradation system (99, 100). This technology selectively removes specific proteins like the AR protein for a therapeutic purpose (101, 102). Several descent review articles summarized the technique description and the usage of various E3 ligases (103–106). We will only discuss the PROTAC molecules designed for the AR protein.
The first AR-targeted PROTAC approach was reported in 2004, which used a synthetic peptide targeting the E3 ligase fused to either an artificial FKBP12 ligand or dihydrotestosterone (DHT) (24). After several optimizations, a potent AR-specific PROTAC molecule ARCC-4 was developed with a nanomole concentration efficiency (25). Its further modified version, ARV-110, is being tested in clinical trials in metastatic prostate cancer patients (26). The first trial is a phase-1b open-label clinical trial (NCT05177042) to assess the combination of ARV-110 and Abiraterone in patients with metastatic prostate cancer with PSA progression after Abiraterone treatment. It is estimated to finish at the end of April of 2023. The second one is a phase-1/2 open-label single-agent dose escalation and cohort expansion trial to assess the safety and tolerability of ARV-110 (NCT03888612). It will be finished at the end of February 2023.
The AR degrader (ARD) series of PROTAC molecules (ARD-61, -69, -266, -2128, -2585) were reported from Dr. Wang’s group at the University of Michigan (27–31). Their latest molecule, ARD-2585, is a potent (DC50 < 0.1 nM) oral agent and has at least 10-fold more potent than ARV-110 (27). These molecules differ in distinct E3 ligase binding domains, AR antagonists, and variable lengths of the linkers. Unfortunately, both ARV-110 and ARD-2585 molecules depend on binding with the AR LBD. Therefore, it is not effective on the AR splicing variants like AR-V7.
Other AR-targeted PROTAC molecules with animal testing data include TD-802 (DC50 = 12.5 nM) (32) and A031 (IC50 < 0.25 μM) (33) that promote degradation of the full-length AR protein. MTX-23 was shown to promote protein degradation of both the full-length and AR-V7 variant AR protein (DC50 = 0.37-2 μM) (34). In addition, three PROTAC molecules, A9/A16 (35, 36) and AR SNIPER-51 compounds (37), were only tested in cell culture models.
Other Unique Molecules for AR Degradation
UT-34 is a small molecule that exerts potent AR degradation activity in vitro (1-10 μM) and in vivo via ubiquitin-proteaseom pathway (38). It was optimized from its two previous versions, UT-69 and UT-155 (107). UT-34 binds with the AR N-terminal AF-1 domain and thus targets both the full-length and splicing variant proteins. UT-34 has a good pharmacological profile of oral bioavailability and suppressed xenograft tumor growth derived from Enzalutamide-resistant prostate cancer cells at a dose of 60 mg/kg/day (38).
Ailanthone was initially identified as an inhibitor of AR transactivation via a high throughput screening assay and was later found to induce protein degradation of both full-length and splicing variant AR proteins via targeting an HSP90 co-chaperon protein p23 (39). Ailanthone exhibited a strong anti-cancer effect in both in vitro cell culture models (0.2-0.4 μM) and in vivo xenograft models (2 mg/kg/day) of prostate cancer (39). It also showed excellent drug-like properties as tested in preclinical models (108, 109).
HG122 was identified as an inhibitor of AR activity via an MMTV-luciferase assay-based high throughput screening (40). HG122 suppressed AR-positive prostate cancer cell growth with an IC50 of 7-9 μM, compared to AR-negative cells at 20 μM. HG122 suppressed AR transcriptional activity and promoted AR degradation via the proteasome pathway. In animal experiments, HG122 suppressed 22RV1 cell-derived xenograft tumor growth by 82% at a dose of 10 mg/kg/day, compared to a 60% reduction by Enzalutamide at the exact dosing (40). However, it is unclear how HG122 promoted AR degradation by the proteasome machinery.
AR Splicing Variant V7-Specific Degraders and Inhibitors
The full-length AR protein has four distinct domains, N-terminal (NTD), DNA-binding (DBD), hinge region, and C-terminal ligand-binding (LBD). In prostate cancers, the transcriptional splicing variants of the AR gene have been linked to castration-resistance of prostate cancer after ADT and anti-AR therapy with Enzalutamide and Abiraterone (110–112). Because these AR variant proteins lack the AR C-terminal LBD region due to gene splicing truncated or deleted, they are not responding to current anti-AR drugs that target the LBD. Therefore, those PROTAC molecules using the LBD ligands are not working on these splicing variant AR proteins (113–115). These variant proteins represent a massive obstacle to clinical management in advanced prostate cancers.
Niclosamide is an FDA-approved oral anti-helminthic drug used to treat parasitic infections. In an AR-V7-driven luciferase-based high-throughput screening assay, Niclosamide was identified as an effective inhibitor of AR-V7 activity. A mechanistic study showed that it enhanced the AR-V7 protein degradation via the ubiquitin-proteasome pathway in prostate cancer cells at 0.5-1.0 μM without affecting the full-length AR protein (116). Combinational treatment with Enzalutamide and Niclosamide suppressed CRPC xenograft tumor growth in mice at a dose of 25 mg/kg/day (117). Although the first clinical trial (NCT02532114) with a single dose of Niclosamide was failed in reaching the effective serum concentration (118), a recent phase-Ib trial with reformulated Niclosamide plus Abiraterone achieved the proposed clinical benefit (119), representing a new hope for AR-V7 positive CRPC patients (NCT03123978/NCT02807805).
CUDC-101 is a small molecule of inhibitor for multiple targets, including histone deacetylase (HDAC), epidermal growth factor receptor (EGFR) and HER2/Neu. It was recently found to inhibit the transcriptional activities of the full-length AR and AR-v7 protein (0.3 μM for 24 h) via a HDAC-related mechanism in prostate cancer 22RV1 cells (41). It also suppressed 22RV1 cell-derived xenograft tumor growth in nude mice at a dose of 50 mg/kg/day for 14 days (41). However, severe side effects will be expected in a clinical test due to its action on multiple targets.
ASC-J9 is a curcumin analog (dimethyl-curcumin) with multiple protein targets (120–125), including the AR proteins (42–44). ASC-J9 induced protein degradation of the full-length AR and AR-V7 proteins via the ubiquitin-proteasome pathway in prostate cancer cells (44) and suppressed xenograft tumor growth derived from CRPC cells (42, 45). It overcame Enzalutamide resistance in preclinical CRPC xenograft models (46) and sensitized prostate cancers to radiation therapy in animal models (47). However, ASC-J9 was only tested in clinical trials for skin acne care (NCT01289574 and NCT00525499).
Thailanstatins are bacteria-derived natural products with potent inhibitory activity toward pre-mRNA splicing events (48). Since AR-V7 is mainly generated by pre-mRNA splicing (49), Thailanstatin D (TST-D) was tested in AR-V7 positive prostate cancer cells for cytotoxicity. TST-D was shown to reduce AR-V7 mRNA and protein levels (at 5 nM concentration) by disrupting the U2AF65/SAP155 splicing complex that is critical for the AR-V7 pre-mRNA expression and suppressed CRPC cell-derived xenograft tumor growth (50% inhibition at 0.3 mg/kg/day after four days) (50). It is postulated that combinational treatment of TST-D with Enzalutamide or Abiraterone might achieve a more profound anti-tumor effect in CRPC models.
Rutaecarpine is a cardiovascular protective alkaloid extracted from the Chinese medicine Evodia rutaecarpa (126). It was identified as a potent AR-V7 inhibitor in an AR-V7-driven luciferase screening assay (51). A mechanistic study revealed that Rutaecarpine promoted AR-V7 degradation by enhancing AR-V7 interaction with GPR78 and ubiquitin E3 ligase SIAH2. Its DC50 for AR-V7 degradation was about 20 μM and completely blocked 22RV1 cell-derived xenograft tumor growth in nude mice at 40 mg/kg/2day (51). Since it also did not affect the full-length AR protein, it is needed to test its synergistic effect with AR antagonists like Enzalutamide and Abiraterone in vivo.
Indisulam belongs to a new class of compound sulfonamide with potential antineoplastic activity (127) via selectively degrading oncogenic proteins like pre-mRNA splicing factor RBM39 (52). Because pre-mRNA splicing is critical for AR-V7 expression, Indisulam was shown to suppress AR-V7 expression via RBM39-dependent mechanism. Indisulam treatment blocked Enzalutamide-induced AR-V7 expression in VCaP cells (10 μM concentration) and suppressed VCaP cell-derived xenograft tumor growth in nude mice at a dose of 25 mk/kg/day (52).
Nobiletin is a plant flavonoid extracted from citrus peels and possesses broad anti-cancer activity (128, 129). A recent study showed that Nobiletin moderately reduced AR-V7 protein level in 22RV-1 cells at 20 μM concentration and synergistically suppressed (at 40 mg/kg/2day) 22RV1 cell-derived xenograft tumor growth with Enzalutamide (20 mg/kg/2day) (53). The mechanistic study revealed that Nobiletin disrupted AR-V7 interaction with two deubiquitinases, USP14 and USP22, leading to proteasome-based AR-V7 degradation (53).
AR N-Terminal Specific Inhibitors
In contrast to the CTD, the AR NTD has very few mutations without truncation (130). For example, the cBioportal database showed only 9 (0.145%) point-mutations identified from the NTD regions in 6334 prostate cancer specimens. There are two transactivation unit (TAU-1, aa100-370) and TAU-5 (aa360-485) motifs within the AR NTD (131). The TAU-1 motif is critical for the full-length AR activation after ligand binding, while the TAU-5 motif functions as a constitutive active motif for truncated AR protein (e.g., AR-V7) (132, 133). Especially, the TAU-1/TAU-5 motifs are rarely mutated or deleted in prostate cancer patients, making them a feasible target for prostate cancer therapy (130).
EPI series compounds are the first class of AR NTD inhibitors. The first compound EPI-001 was identified by screening a library of marine sponge extracts to inhibit AR NTD transactivation activity (134). EPI-001 binds to the TAU-5 motif and inhibits AR NTD activity at a relatively high dose (>25 μM in cell culture models) (135, 136). EPI compounds also suppressed tumor growth in VCaP and LNCaP95 cell-derived xenograft models at 100-200 mg/kg/day doses (135, 137). Although the older EPI compounds did not affect AR protein levels (the full length and AV variants), the new analog EPI-7170 suppressed AR-V7 expression in CRPC cells (138). EPI-002 (commercial name Ralaniten) is one of the four EPI-001 stereoisomers, and its pro-drug EPI-506 (Ralaniten acetate) was failed in a phase-I clinical trial due to excessive pill burden and poor oral bioavailability (139, 140). The newest analog, EPI-7386, showed 20-fold higher anti-androgenic potency than Ralaniten (141), and it is being tested in clinical trials in combination with Enzalutamide (NCT05075577/NCT04421222).
QW07 is a small synthetic molecule identified as an AR NTD-specific inhibitor via an AR-NTD-driven luciferase high-throughput screening (54). QW07 suppressed the activity of AR full-length and splicing variants at 5-8 μM in prostate cancer cells, which is more potent than EPI-001 (54). QW07 binds with the AR NTD directly and suppresses AR recruitment onto the target gene promoter. In animal xenograft experiments, QW07 inhibited tumor growth derived from prostate cancer 22RV1 and VCaP cells at a dose of 40 mg/kg/day, similar to EPI-001. However, QW07 did not affect AR protein expression (the full length or splicing variants).
AR Nuclear Translocation Blockers
As a transcription factor, the AR proteins translocate into the nuclear compartment after being activated by the androgens (5). In the nuclear, AR protein interacts with the androgen response elements in the gene promoter region to modulate gene expression. The AR protein has one nuclear localization sequence or signal (NLS) in each domain, the NTD region (aa294-556), the DBD-hinge region (aa617-633), and the LBD region (aa666-919) (142–144). In the absence of androgens, the AR protein is exported from the nuclear compartment via its nuclear export signal (NES, aa743-817) within the LBD region (145). In CRPC tissue or cells that androgen levels are deficient due to androgen deprivation therapy, the NLS in the NTD region is responsible for AR nuclear localization (143). Blocking AR nuclear translocation with a potent NLS inhibitor is feasible to suppress prostate cancer development and progression by shutting down AR-modulated gene expression.
EPPI and CPPI are small molecules identified as inhibitors of AR nuclear translocation in Dr. Z Wang’s lab using a 2GFP-AR fusing protein-based high-throughput screening approach (55). Both EPPI and CPPI at 25 μM inhibited AR nuclear localization in prostate cancer cells, which was reversed when the androgen level (R1881) was over 1.0 nM level, a physiological androgen concentration (56). Also, CPPI at a 50 mg/kg/day dose suppressed tumor growth in LNCaP but not PC-3 cell-derived xenograft models with or without castration, indicating an AR-specific effect (56). Further analysis revealed that CPPI blocked AR nuclear import and promoted AR degradation in the nuclear compartment through MDM2-dependent proteasome mechanism in CRPC cells (C4-2 and LNCaP95) and xenograft tumor models, leading to sharp retardation of tumor growth (57). No effect was observed for CPPI or EPPI on the AR variant proteins (57).
IMPPE (SID3712502) was another small molecule identified from the 2GFP-AR fusing protein screening assay with a robust inhibitory effect at 2.0 μM concentration on AR nuclear translocation and its downstream target PSA gene expression, plus downregulation of AR gene expression at a higher concentration of 10 μM (55). Further study found that IMPPE inhibited both full-length and LBD-lacking AR activity at a relatively high dose (>10 μM) and suppressed 22RV1 but not PC-3 cell-derived xenograft tumor growth at a dose of 25 mg/kg/day in castrated nude mice (58).
JJ-450 is an IMPPE scaffold analog with higher potency and better physicochemical properties (59). JJ-450 at 10 μM concentration inhibited both the transcriptional activities of the full-length and splicing variant AR proteins in CRPC cells by blocking AR binding to its target gene promoter without affecting AR protein levels (59). In CRPC xenograft models derived from 22RV1 and VCaP cells, JJ-450 at 10 mg/kg/day dose suppressed xenograft tumor growth by 60%, slightly better than Enzalutamide (59). Especially, JJ-450 was found to block the nuclear translocation and activity of the AR F876L mutant protein identified from Enzalutamide-resistant CRPC patients and LNCaP cells after long-term exposure to Enzalutamide (60–62).
AR DBDH Antagonists
The AR DBD-Hinge region has P-box and D-box motifs responsible for dimerization and DNA binding after androgen stimulation (146). Using a virtual in-silico drug design approach (63–65), a surface-exposed region (aa579-610) on the AR DBDH domain was discovered as a potential target site by small-molecule compounds, including VPC-14228 and VPC-14449 (66). These two compounds at 10 μM concentration selectively suppressed AR (full-length and splicing variant proteins) but not ER or GR activity by blocking AR interaction with the target gene promoters without affecting AR nuclear translocation and protein stability (66). In LNCaP cell-derived xenograft experiments, VPC-1449 at 100 mg/kg/day dose suppressed tumor growth at a similar extent as Enzalutamide (10 mg/kg/day) (66).
Conclusion and Perspectives
The AR protein is critical for prostate cancer progression by transcriptionally modulating gene expression after activation by androgens via binding on its LBD. Metastatic prostate cancers are initially treated with androgen deprivation or castration therapies (surgical or medical) based on the findings reported about 80-years ago. However, this androgen removal approach is not curative for prostate cancers, and the diseases often relapse and progress to the CRPC stage. Since most of these CRPCs are still AR addictive, current clinical therapies mainly focus on blocking androgen to bind with the AR LBD (AR antagonists) or reducing androgen production (CYP17a1 inhibitors) in non-testis tissues, including prostate cancer tissues. However, treatment resistance eventually develops in part due to AR gene mutation and mRNA splicing events (e.g., AR-V7) in virtually all CRPC patients. Furthermore, after long-term treatment with AR antagonists, up to 20% of CRPC patients will develop an even more aggressive subtype, neuroendocrinal prostate cancer (NEPC). Therefore, the androgen removal and blockage approach are non-curative and leads to a more aggressive disease.
To overcome this obstacle of treatment resistance, research has shifted from androgens to the AR protein in the last 20 years (Figure 1). The initial approach was the antisense oligonucleotides (ASO) targeting the AR mRNA to reduce AR protein production in prostate cancer cells. Due to the inhibitory nature of the ASO approach on protein production, tumor growth was only suppressed but not eradicated in xenograft models. In contrast, our group used the siRNA approach that efficiently eliminated the AR protein from prostate cancer cells. Nanoparticle-loaded AR siRNA resulted in xenograft tumor regression and eradication owing to robust cell death after AR protein removal in prostate cancer cells. Unfortunately, this AR siRNA project was stalled due to a failure in the patent application.
Targeting AR protein stability has emerged in recent years as the hotspot in developing new therapeutics for advanced prostate cancers, and several small molecules were reported to reduce AR protein stability. The curcumin analog ASC-J9, Ailanthone, HG122, and CUDC-101 induced AR protein degradation in prostate cancer cells. However, the AR or prostate cancer tissue specificity is not established with these small molecules. The PROTAC technique for AR-specific degradation showed a promising result. The AR PROTAC ARV-110 is tested as a combinational treatment with Abiraterone in a clinical trial. However, these AR CTD-targeting PROTACs utilized AR LBD ligands, and therefore, they are inactive on AR CTD splicing variants, a critical mechanism for treatment resistance in CRPC patients. Interestingly, some other agents specifically targeted the AR-V7 variant for degradation, including Niclosamide, CUDC-101, Thailanstatins, Rutaecarpine, Indisulam, and Nobiletin. Combining AR antagonists, PROTAC molecules, and AR-V7 inhibitors might provide synergistic effects in the clinic.
Targeting AR NTD is another approach to bypass AR CTD splicing defects. The first generation of AR NTD inhibitor EPI compounds was failed in clinical trials due to excessive bill burden. The second generation of EPI compound with 20-fold higher potency is being tested as a combinational treatment with Enzalutamide in a clinical trial. UT-34 targets the AR NTD and is also waiting for a clinical test.
AR nuclear translocation is an important event for its activity as a transcription factor. Two novel compounds, IMPPE and JJ-450, were recently developed to block AR nuclear translocation. These two compounds showed a very permissive result in animal models. In addition, an AR DBD blocking agent VPC-14449 was reported to suppress AR interaction with its target gene promoter in the nuclear compartment and was found to suppress tumor growth in mice. These compounds are all needed for clinical testing.
AR activity is only temporally suppressed during prostate cancer treatment by androgen deprivation and AR antagonists. Due to these treatment stresses, prostate cancer cells used other cellular signal pathways and/or splicing variants for AR reactivation, resulting in treatment resistance. Therefore, complete removal of the AR protein from prostate cancer cells will eliminate all events of AR reactivation after ADT and anti-AR therapy. Especially in the early phase of treatment, most prostate cancer cells are still AR-dependent. Simultaneously removal of the AR protein and androgens will result in robust cell death, leading to a possible curative result or long-term disease-free survival. In addition, early reduction of the AR protein in the androgen-responsive phase of prostate cancer will reduce the likelihood of transcriptional reprogramming (88, 93, 147). Also, tissue-specific delivery of the AR protein degradation agents will restrict potential side effects.
Author Contributions
All authors participated in drafting the manuscript. All authors contributed to the article and approved the submitted version.
Funding
This work was partially supported by a grant from KUMC Lied pilot program and DoD PCRP PC190026 to Benyi Li, MD/PhD.
Conflict of Interest
The authors declare that the research was conducted in the absence of any commercial or financial relationships that could be construed as a potential conflict of interest.
Publisher’s Note
All claims expressed in this article are solely those of the authors and do not necessarily represent those of their affiliated organizations, or those of the publisher, the editors and the reviewers. Any product that may be evaluated in this article, or claim that may be made by its manufacturer, is not guaranteed or endorsed by the publisher.
Acknowledgments
We are grateful for all the talented investors who developed those elegant AR-targeted agents. We are also sorry for not citing all the reports in the field due to the limited space in this mini-review article.
References
1. Siegel RL, Miller KD, Fuchs HE, Jemal A. Cancer Statistics, 2022. CA Cancer J Clin (2022) 72(1):7–33. doi: 10.3322/caac.21708
2. Pagliuca M, Buonerba C, Fizazi K, Di Lorenzo G. The Evolving Systemic Treatment Landscape for Patients With Advanced Prostate Cancer. Drugs (2019) 79(4):381–400. doi: 10.1007/s40265-019-1060-5
3. Huggins C. Prostatic Cancer Treated by Orchiectomy; the Five Year Results. J Am Med Assoc (1946) 131:576–81. doi: 10.1001/jama.1946.02870240008003
4. Van Poppel H, Abrahamsson PA. Considerations for the Use of Gonadotropin-Releasing Hormone Agonists and Antagonists in Patients With Prostate Cancer. Int J Urol (2020) 27(10):830–37. doi: 10.1111/iju.14303
5. Estebanez-Perpina E, Bevan CL, McEwan IJ. Eighty Years of Targeting Androgen Receptor Activity in Prostate Cancer: The Fight Goes on. Cancers (Basel) (2021) 13(3):509–27. doi: 10.3390/cancers13030509
6. Di Fusco D, Dinallo V, Marafini I, Figliuzzi MM, Romano B, Monteleone G. Antisense Oligonucleotide: Basic Concepts and Therapeutic Application in Inflammatory Bowel Disease. Front Pharmacol (2019) 10:305. doi: 10.3389/fphar.2019.00305
7. Eder IE, Culig Z, Ramoner R, Thurnher M, Putz T, Nessler-Menardi C, et al. Inhibition of LncaP Prostate Cancer Cells by Means of Androgen Receptor Antisense Oligonucleotides. Cancer Gene Ther (2000) 7(7):997–1007. doi: 10.1038/sj.cgt.7700202
8. Eder IE, Hoffmann J, Rogatsch H, Schafer G, Zopf D, Bartsch G, et al. Inhibition of LNCaP Prostate Tumor Growth In Vivo by an Antisense Oligonucleotide Directed Against the Human Androgen Receptor. Cancer Gene Ther (2002) 9(2):117–25. doi: 10.1038/sj.cgt.7700416
9. Ko YJ, Devi GR, London CA, Kayas A, Reddy MT, Iversen PL, et al. Androgen Receptor Down-Regulation in Prostate Cancer With Phosphorodiamidate Morpholino Antisense Oligomers. J Urol (2004) 172(3):1140–4. doi: 10.1097/01.ju.0000134698.87862.e6
10. Hamy F, Brondani V, Spoerri R, Rigo S, Stamm C, Klimkait T. Specific Block of Androgen Receptor Activity by Antisense Oligonucleotides. Prostate Cancer Prostat Dis (2003) 6(1):27–33. doi: 10.1038/sj.pcan.4500603
11. Yamamoto Y, Lin PJ, Beraldi E, Zhang F, Kawai Y, Leong J, et al. siRNA Lipid Nanoparticle Potently Silences Clusterin and Delays Progression When Combined With Androgen Receptor Cotargeting in Enzalutamide-Resistant Prostate Cancer. Clin Cancer Res (2015) 21(21):4845–55. doi: 10.1158/1078-0432.CCR-15-0866
12. Yamamoto Y, Loriot Y, Beraldi E, Zhang F, Wyatt AW, Al Nakouzi N, et al. Generation 2.5 Antisense Oligonucleotides Targeting the Androgen Receptor and Its Splice Variants Suppress Enzalutamide-Resistant Prostate Cancer Cell Growth. Clin Cancer Res (2015) 21(7):1675–87. doi: 10.1158/1078-0432.CCR-14-1108
13. Xiao L, Tien JC, Vo J, Tan M, Parolia A, Zhang Y, et al. Epigenetic Reprogramming With Antisense Oligonucleotides Enhances the Effectiveness of Androgen Receptor Inhibition in Castration-Resistant Prostate Cancer. Cancer Res (2018) 78(20):5731–40. doi: 10.1158/0008-5472.CAN-18-0941
14. De Velasco MA, Kura Y, Sakai K, Hatanaka Y, Davies BR, Campbell H, et al. Targeting Castration-Resistant Prostate Cancer With Androgen Receptor Antisense Oligonucleotide Therapy. JCI Insight (2019) 4(17):e122688–711. doi: 10.1172/jci.insight.122688
15. Liao X, Tang S, Thrasher JB, Griebling TL, Li B. Small-Interfering RNA-Induced Androgen Receptor Silencing Leads to Apoptotic Cell Death in Prostate Cancer. Mol Cancer Ther (2005) 4(4):505–15. doi: 10.1158/1535-7163.MCT-04-0313
16. Yang J, Xie SX, Huang Y, Ling M, Liu J, Ran Y, et al. Prostate-Targeted Biodegradable Nanoparticles Loaded With Androgen Receptor Silencing Constructs Eradicate Xenograft Tumors in Mice. Nanomed (Lond) (2012) 7(9):1297–309. doi: 10.2217/nnm.12.14
17. Sun A, Tang J, Terranova PF, Zhang X, Thrasher JB, Li B. Adeno-Associated Virus-Delivered Short Hairpin-Structured RNA for Androgen Receptor Gene Silencing Induces Tumor Eradication of Prostate Cancer Xenografts in Nude Mice: A Preclinical Study. Int J Cancer (2010) 126(3):764–74. doi: 10.1002/ijc.24778
18. Luna Velez MV, Verhaegh GW, Smit F, Sedelaar JPM, Schalken JA. Suppression of Prostate Tumor Cell Survival by Antisense Oligonucleotide-Mediated Inhibition of AR-V7 mRNA Synthesis. Oncogene (2019) 38(19):3696–709. doi: 10.1038/s41388-019-0696-7
19. Lee JB, Zhang K, Tam YY, Tam YK, Belliveau NM, Sung VY, et al. Lipid Nanoparticle siRNA Systems for Silencing the Androgen Receptor in Human Prostate Cancer In Vivo. Int J Cancer (2012) 131(5):E781–90. doi: 10.1002/ijc.27361
20. Snoek R, Cheng H, Margiotti K, Wafa LA, Wong CA, Wong EC, et al. In Vivo Knockdown of the Androgen Receptor Results in Growth Inhibition and Regression of Well-Established, Castration-Resistant Prostate Tumors. Clin Cancer Res (2009) 15(1):39–47. doi: 10.1158/1078-0432.CCR-08-1726
21. Cheng H, Snoek R, Ghaidi F, Cox ME, Rennie PS. Short Hairpin RNA Knockdown of the Androgen Receptor Attenuates Ligand-Independent Activation and Delays Tumor Progression. Cancer Res (2006) 66(21):10613–20. doi: 10.1158/0008-5472.CAN-06-0028
22. Haag P, Bektic J, Bartsch G, Klocker H, Eder IE. Androgen Receptor Down Regulation by Small Interference RNA Induces Cell Growth Inhibition in Androgen Sensitive as Well as in Androgen Independent Prostate Cancer Cells. J Steroid Biochem Mol Biol (2005) 96(3-4):251–8. doi: 10.1016/j.jsbmb.2005.04.029
23. Compagno D, Merle C, Morin A, Gilbert C, Mathieu JR, Bozec A, et al. SIRNA-Directed In Vivo Silencing of Androgen Receptor Inhibits the Growth of Castration-Resistant Prostate Carcinomas. PloS One (2007) 2(10):e1006. doi: 10.1371/journal.pone.0001006
24. Schneekloth JS Jr, Fonseca FN, Koldobskiy M, Mandal A, Deshaies R, Sakamoto K, et al. Chemical Genetic Control of Protein Levels: Selective In Vivo Targeted Degradation. J Am Chem Soc (2004) 126(12):3748–54. doi: 10.1021/ja039025z
25. Salami J, Alabi S, Willard RR, Vitale NJ, Wang J, Dong H, et al. Androgen Receptor Degradation by the Proteolysis-Targeting Chimera ARCC-4 Outperforms Enzalutamide in Cellular Models of Prostate Cancer Drug Resistance. Commun Biol (2018) 1:100. doi: 10.1038/s42003-018-0105-8
26. Snyder LB, Neklesa TK, Chen X, Dong H, Ferraro C, Gordon DA, et al. Discovery of ARV-110, a First in Class Androgen Receptor Degrading PROTAC for the Treatment of Men With Metastatic Castration Resistant Prostate Cancer. Cancer Res (2021) 81(13_Supplement):43. doi: 10.1158/1538-7445.AM2021-43
27. Xiang W, Zhao L, Han X, Qin C, Miao B, McEachern D, et al. Discovery of ARD-2585 as an Exceptionally Potent and Orally Active PROTAC Degrader of Androgen Receptor for the Treatment of Advanced Prostate Cancer. J Med Chem (2021) 64(18):13487–509. doi: 10.1021/acs.jmedchem.1c00900
28. Han X, Wang C, Qin C, Xiang W, Fernandez-Salas E, Yang CY, et al. Discovery of ARD-69 as a Highly Potent Proteolysis Targeting Chimera (PROTAC) Degrader of Androgen Receptor (AR) for the Treatment of Prostate Cancer. J Med Chem (2019) 62(2):941–64. doi: 10.1021/acs.jmedchem.8b01631
29. Han X, Zhao L, Xiang W, Qin C, Miao B, McEachern D, et al. Strategies Toward Discovery of Potent and Orally Bioavailable Proteolysis Targeting Chimera Degraders of Androgen Receptor for the Treatment of Prostate Cancer. J Med Chem (2021) 64(17):12831–54. doi: 10.1021/acs.jmedchem.1c00882
30. Zhao L, Han X, Lu J, McEachern D, Wang S. A Highly Potent PROTAC Androgen Receptor (AR) Degrader ARD-61 Effectively Inhibits AR-Positive Breast Cancer Cell Growth In Vitro and Tumor Growth In Vivo. Neoplasia (2020) 22(10):522–32. doi: 10.1016/j.neo.2020.07.002
31. Han X, Zhao L, Xiang W, Qin C, Miao B, Xu T, et al. Discovery of Highly Potent and Efficient PROTAC Degraders of Androgen Receptor (AR) by Employing Weak Binding Affinity VHL E3 Ligase Ligands. J Med Chem (2019) 62(24):11218–31. doi: 10.1021/acs.jmedchem.9b01393
32. Takwale AD, Jo SH, Jeon YU, Kim HS, Shin CH, Lee HK, et al. Design and Characterization of Cereblon-Mediated Androgen Receptor Proteolysis-Targeting Chimeras. Eur J Med Chem (2020) 208:112769. doi: 10.1016/j.ejmech.2020.112769
33. Chen L, Han L, Mao S, Xu P, Xu X, Zhao R, et al. Discovery of A031 as Effective Proteolysis Targeting Chimera (PROTAC) Androgen Receptor (AR) Degrader for the Treatment of Prostate Cancer. Eur J Med Chem (2021) 216:113307. doi: 10.1016/j.ejmech.2021.113307
34. Lee GT, Nagaya N, Desantis J, Madura K, Sabaawy HE, Kim WJ, et al. Effects of MTX-23, a Novel PROTAC of Androgen Receptor Splice Variant-7 and Androgen Receptor, on CRPC Resistant to Second-Line Antiandrogen Therapy. Mol Cancer Ther (2021) 20(3):490–9. doi: 10.1158/1535-7163.MCT-20-0417
35. Xie H, Liang JJ, Wang YL, Hu TX, Wang JY, Yang RH, et al. The Design, Synthesis and Anti-Tumor Mechanism Study of New Androgen Receptor Degrader. Eur J Med Chem (2020) 204:112512. doi: 10.1016/j.ejmech.2020.112512
36. Liang JJ, Xie H, Yang RH, Wang N, Zheng ZJ, Zhou C, et al. Designed, Synthesized and Biological Evaluation of Proteolysis Targeting Chimeras (PROTACs) as AR Degraders for Prostate Cancer Treatment. Bioorg Med Chem (2021) 45:116331. doi: 10.1016/j.bmc.2021.116331
37. Shibata N, Nagai K, Morita Y, Ujikawa O, Ohoka N, Hattori T, et al. Development of Protein Degradation Inducers of Androgen Receptor by Conjugation of Androgen Receptor Ligands and Inhibitor of Apoptosis Protein Ligands. J Med Chem (2018) 61(2):543–75. doi: 10.1021/acs.jmedchem.7b00168
38. Ponnusamy S, He Y, Hwang DJ, Thiyagarajan T, Houtman R, Bocharova V, et al. Orally Bioavailable Androgen Receptor Degrader, Potential Next-Generation Therapeutic for Enzalutamide-Resistant Prostate Cancer. Clin Cancer Res (2019) 25(22):6764–80. doi: 10.1158/1078-0432.CCR-19-1458
39. He Y, Peng S, Wang J, Chen H, Cong X, Chen A, et al. Ailanthone Targets P23 to Overcome MDV3100 Resistance in Castration-Resistant Prostate Cancer. Nat Commun (2016) 7:13122–35. doi: 10.1038/ncomms13122
40. Cong X, He Y, Wu H, Wang D, Liu Y, Shao T, et al. Regression of Castration-Resistant Prostate Cancer by a Novel Compound Hg122. Front Oncol (2021) 11:650919. doi: 10.3389/fonc.2021.650919
41. Sun H, Mediwala SN, Szafran AT, Mancini MA, Marcelli M. CUDC-101, a Novel Inhibitor of Full-Length Androgen Receptor (flAR) and Androgen Receptor Variant 7 (AR-V7) Activity: Mechanism of Action and In Vivo Efficacy. Horm Cancer (2016) 7(3):196–210. doi: 10.1007/s12672-016-0257-2
42. Yamashita S, Lai KP, Chuang KL, Xu D, Miyamoto H, Tochigi T, et al. ASC-J9 Suppresses Castration-Resistant Prostate Cancer Growth Through Degradation of Full-Length and Splice Variant Androgen Receptors. Neoplasia (2012) 14(1):74–83. doi: 10.1593/neo.111436
43. Wang R, Lin W, Lin C, Li L, Sun Y, Chang C. ASC-J9((R)) Suppresses Castration Resistant Prostate Cancer Progression via Degrading the Enzalutamide-Induced Androgen Receptor Mutant AR-F876L. Cancer Lett (2016) 379(1):154–60. doi: 10.1016/j.canlet.2016.05.018
44. Lai KP, Huang CK, Chang YJ, Chung CY, Yamashita S, Li L, et al. New Therapeutic Approach to Suppress Castration-Resistant Prostate Cancer Using ASC-J9 via Targeting Androgen Receptor in Selective Prostate Cells. Am J Pathol (2013) 182(2):460–73. doi: 10.1016/j.ajpath.2012.10.029
45. Cheng MA, Chou FJ, Wang K, Yang R, Ding J, Zhang Q, et al. Androgen Receptor (AR) Degradation Enhancer ASC-J9((R)) in an FDA-Approved Formulated Solution Suppresses Castration Resistant Prostate Cancer Cell Growth. Cancer Lett (2018) 417:182–91. doi: 10.1016/j.canlet.2017.11.038
46. Wang R, Sun Y, Li L, Niu Y, Lin W, Lin C, et al. Preclinical Study Using Malat1 Small Interfering RNA or Androgen Receptor Splicing Variant 7 Degradation Enhancer ASC-J9((R)) to Suppress Enzalutamide-Resistant Prostate Cancer Progression. Eur Urol (2017) 72(5):835–44. doi: 10.1016/j.eururo.2017.04.005
47. Chou FJ, Chen Y, Chen D, Niu Y, Li G, Keng P, et al. Preclinical Study Using Androgen Receptor (AR) Degradation Enhancer to Increase Radiotherapy Efficacy via Targeting Radiation-Increased AR to Better Suppress Prostate Cancer Progression. EBioMedicine (2019) 40:504–16. doi: 10.1016/j.ebiom.2018.12.050
48. Liu X, Biswas S, Berg MG, Antapli CM, Xie F, Wang Q, et al. Genomics-Guided Discovery of Thailanstatins A, B, and C As pre-mRNA Splicing Inhibitors and Antiproliferative Agents From Burkholderia Thailandensis Msmb43. J Nat Prod (2013) 76(4):685–93. doi: 10.1021/np300913h
49. Dehm SM, Tindall DJ. Alternatively Spliced Androgen Receptor Variants. Endocr Relat Cancer (2011) 18(5):R183–96. doi: 10.1530/ERC-11-0141
50. Wang B, Lo UG, Wu K, Kapur P, Liu X, Huang J, et al. Developing New Targeting Strategy for Androgen Receptor Variants in Castration Resistant Prostate Cancer. Int J Cancer (2017) 141(10):2121–30. doi: 10.1002/ijc.30893
51. Liao Y, Liu Y, Xia X, Shao Z, Huang C, He J, et al. Targeting GRP78-Dependent AR-V7 Protein Degradation Overcomes Castration-Resistance in Prostate Cancer Therapy. Theranostics (2020) 10(8):3366–81. doi: 10.7150/thno.41849
52. Melnyk JE, Steri V, Nguyen HG, Hann B, Feng FY, Shokat KM. The Splicing Modulator Sulfonamide Indisulam Reduces AR-V7 in Prostate Cancer Cells. Bioorg Med Chem (2020) 28(20):115712. doi: 10.1016/j.bmc.2020.115712
53. Liu Y, Yu C, Shao Z, Xia X, Hu T, Kong W, et al. Selective Degradation of AR-V7 to Overcome Castration Resistance of Prostate Cancer. Cell Death Dis (2021) 12(10):857. doi: 10.1038/s41419-021-04162-0
54. Peng S, Wang J, Chen H, Hu P, He XL, He Y, et al. Regression of Castration-Resistant Prostate Cancer by a Novel Compound QW07 Targeting Androgen Receptor N-Terminal Domain. Cell Biol Toxicol (2020) 36(5):399–416. doi: 10.1007/s10565-020-09511-x
55. Johnston PA, Nguyen MM, Dar JA, Ai J, Wang Y, Masoodi KZ, et al. Development and Implementation of a High-Throughput High-Content Screening Assay to Identify Inhibitors of Androgen Receptor Nuclear Localization in Castration-Resistant Prostate Cancer Cells. Assay Drug Dev Technol (2016) 14(4):226–39. doi: 10.1089/adt.2016.716
56. Masoodi KZ, Xu Y, Dar JA, Eisermann K, Pascal LE, Parrinello E, et al. Inhibition of Androgen Receptor Nuclear Localization and Castration-Resistant Prostate Tumor Growth by Pyrroloimidazole-Based Small Molecules. Mol Cancer Ther (2017) 16(10):2120–9. doi: 10.1158/1535-7163.MCT-17-0176
57. Lv S, Song Q, Chen G, Cheng E, Chen W, Cole R, et al. Regulation and Targeting of Androgen Receptor Nuclear Localization in Castration-Resistant Prostate Cancer. J Clin Invest (2021) 131(4):e141335–50. doi: 10.1172/JCI141335
58. Masoodi KZ, Eisermann K, Yang Z, Dar JA, Pascal LE, Nguyen M, et al. Inhibition of Androgen Receptor Function and Level in Castration-Resistant Prostate Cancer Cells by 2-[(Isoxazol-4-Ylmethyl)Thio]-1-(4-Phenylpiperazin-1-Yl)Ethanone. Endocrinology (2017) 158(10):3152–61. doi: 10.1210/en.2017-00408
59. Yang Z, Wang D, Johnson JK, Pascal LE, Takubo K, Avula R, et al. A Novel Small Molecule Targets Androgen Receptor and Its Splice Variants in Castration-Resistant Prostate Cancer. Mol Cancer Ther (2020) 19(1):75–88. doi: 10.1158/1535-7163.MCT-19-0489
60. Wu Z, Wang K, Yang Z, Pascal LE, Nelson JB, Takubo K, et al. A Novel Androgen Receptor Antagonist JJ-450 Inhibits Enzalutamide-Resistant Mutant AR(F876L) Nuclear Import and Function. Prostate (2020) 80(4):319–28. doi: 10.1002/pros.23945
61. Joseph JD, Lu N, Qian J, Sensintaffar J, Shao G, Brigham D, et al. A Clinically Relevant Androgen Receptor Mutation Confers Resistance to Second-Generation Antiandrogens Enzalutamide and ARN-509. Cancer Discov (2013) 3(9):1020–9. doi: 10.1158/2159-8290.CD-13-0226
62. Azad AA, Volik SV, Wyatt AW, Haegert A, Le Bihan S, Bell RH, et al. Androgen Receptor Gene Aberrations in Circulating Cell-Free DNA: Biomarkers of Therapeutic Resistance in Castration-Resistant Prostate Cancer. Clin Cancer Res (2015) 21(10):2315–24. doi: 10.1158/1078-0432.CCR-14-2666
63. Lack NA, Axerio-Cilies P, Tavassoli P, Han FQ, Chan KH, Feau C, et al. Targeting the Binding Function 3 (BF3) Site of the Human Androgen Receptor Through Virtual Screening. J Med Chem (2011) 54(24):8563–73. doi: 10.1021/jm201098n
64. Munuganti RS, Leblanc E, Axerio-Cilies P, Labriere C, Frewin K, Singh K, et al. Targeting the Binding Function 3 (BF3) Site of the Androgen Receptor Through Virtual Screening. 2. Development of 2-((2-Phenoxyethyl) Thio)-1h-Benzimidazole Derivatives. J Med Chem (2013) 56(3):1136–48. doi: 10.1021/jm3015712
65. Li H, Ren X, Leblanc E, Frewin K, Rennie PS, Cherkasov A. Identification of Novel Androgen Receptor Antagonists Using Structure- and Ligand-Based Methods. J Chem Inf Model (2013) 53(1):123–30. doi: 10.1021/ci300514v
66. Dalal K, Roshan-Moniri M, Sharma A, Li H, Ban F, Hassona MD, et al. Selectively Targeting the DNA-Binding Domain of the Androgen Receptor as a Prospective Therapy for Prostate Cancer. J Biol Chem (2017) 292(10):4359. doi: 10.1074/jbc.A117.553818
67. Xu J, Qiu Y. Current Opinion and Mechanistic Interpretation of Combination Therapy for Castration-Resistant Prostate Cancer. Asian J Androl (2019) 21(3):270–8. doi: 10.4103/aja.aja_10_19
68. Scher HI, Sawyers CL. Biology of Progressive, Castration-Resistant Prostate Cancer: Directed Therapies Targeting the Androgen-Receptor Signaling Axis. J Clin Oncol (2005) 23(32):8253–61. doi: 10.1200/JCO.2005.03.4777
69. Dai C, Heemers H, Sharifi N. Androgen Signaling in Prostate Cancer. Cold Spring Harb Perspect Med (2017) 7(9):a030452–5469. doi: 10.1101/cshperspect.a030452
70. Feng Q, He B. Androgen Receptor Signaling in the Development of Castration-Resistant Prostate Cancer. Front Oncol (2019) 9:858. doi: 10.3389/fonc.2019.00858
71. Crawford ED, Schellhammer PF, McLeod DG, Moul JW, Higano CS, Shore N, et al. Androgen Receptor Targeted Treatments of Prostate Cancer: 35 Years of Progress With Antiandrogens. J Urol (2018) 200(5):956–66. doi: 10.1016/j.juro.2018.04.083
72. Puca L, Vlachostergios PJ, Beltran H. Neuroendocrine Differentiation in Prostate Cancer: Emerging Biology, Models, and Therapies. Cold Spring Harb Perspect Med (2019) 9(2):a030593–612. doi: 10.1101/cshperspect.a030593
73. Niu Y, Guo C, Wen S, Tian J, Luo J, Wang K, et al. ADT With Antiandrogens in Prostate Cancer Induces Adverse Effect of Increasing Resistance, Neuroendocrine Differentiation and Tumor Metastasis. Cancer Lett (2018) 439:47–55. doi: 10.1016/j.canlet.2018.09.020
74. Huang YH, Zhang YQ, Huang JT. Neuroendocrine Cells of Prostate Cancer: Biologic Functions and Molecular Mechanisms. Asian J Androl (2019) 21(3):291–5. doi: 10.4103/aja.aja_128_18
75. Wang Y, Wang Y, Ci X, Choi SYC, Crea F, Lin D, et al. Molecular Events in Neuroendocrine Prostate Cancer Development. Nat Rev Urol (2021) 18(10):581–96. doi: 10.1038/s41585-021-00490-0
76. Zhang Y, Zheng D, Zhou T, Song H, Hulsurkar M, Su N, et al. Androgen Deprivation Promotes Neuroendocrine Differentiation and Angiogenesis Through CREB-EZH2-TSP1 Pathway in Prostate Cancers. Nat Commun (2018) 9(1):4080. doi: 10.1038/s41467-018-06177-2
77. Reina-Campos M, Linares JF, Duran A, Cordes T, L'Hermitte A, Badur MG, et al. Increased Serine and One-Carbon Pathway Metabolism by PKClambda/iota Deficiency Promotes Neuroendocrine Prostate Cancer. Cancer Cell (2019) 35(3):385–400.e9. doi: 10.1016/j.ccell.2019.01.018
78. Singh N, Ramnarine VR, Song JH, Pandey R, Padi SKR, Nouri M, et al. The Long Noncoding RNA H19 Regulates Tumor Plasticity in Neuroendocrine Prostate Cancer. Nat Commun (2021) 12(1):7349. doi: 10.1038/s41467-021-26901-9
79. Lee AR, Gan Y, Xie N, Ramnarine VR, Lovnicki JM, Dong X. Alternative RNA Splicing of the GIT1 Gene is Associated With Neuroendocrine Prostate Cancer. Cancer Sci (2019) 110(1):245–55. doi: 10.1111/cas.13869
80. VanDeusen HR, Ramroop JR, Morel KL, Bae SY, Sheahan AV, Sychev Z, et al. Targeting RET Kinase in Neuroendocrine Prostate Cancer. Mol Cancer Res (2020) 18(8):1176–88. doi: 10.1158/1541-7786.MCR-19-1245
81. Hu CY, Wu KY, Lin TY, Chen CC. The Crosstalk of Long Non-Coding RNA and MicroRNA in Castration-Resistant and Neuroendocrine Prostate Cancer: Their Interaction and Clinical Importance. Int J Mol Sci (2021) 23(1):392–408. doi: 10.3390/ijms23010392
82. Enriquez C, Cancila V, Ferri R, Sulsenti R, Fischetti I, Milani M, et al. Castration-Induced Downregulation of SPARC in Stromal Cells Drives Neuroendocrine Differentiation of Prostate Cancer. Cancer Res (2021) 81(16):4257–74. doi: 10.1158/0008-5472.CAN-21-0163
83. Baca SC, Takeda DY, Seo JH, Hwang J, Ku SY, Arafeh R, et al. Reprogramming of the FOXA1 Cistrome in Treatment-Emergent Neuroendocrine Prostate Cancer. Nat Commun (2021) 12(1):1979. doi: 10.1038/s41467-021-22139-7
84. Kim DH, Sun D, Storck WK, Welker Leng K, Jenkins C, Coleman DJ, et al. BET Bromodomain Inhibition Blocks an AR-Repressed, E2F1-Activated Treatment-Emergent Neuroendocrine Prostate Cancer Lineage Plasticity Program. Clin Cancer Res (2021) 27(17):4923–36. doi: 10.1158/1078-0432.CCR-20-4968
85. Li Y, Xie N, Chen R, Lee AR, Lovnicki J, Morrison EA, et al. RNA Splicing of the BHC80 Gene Contributes to Neuroendocrine Prostate Cancer Progression. Eur Urol (2019) 76(2):157–66. doi: 10.1016/j.eururo.2019.03.011
86. Guo H, Ci X, Ahmed M, Hua JT, Soares F, Lin D, et al. ONECUT2 is a Driver of Neuroendocrine Prostate Cancer. Nat Commun (2019) 10(1):278. doi: 10.1038/s41467-018-08133-6
87. Lee AR, Gan Y, Tang Y, Dong X. A Novel Mechanism of SRRM4 in Promoting Neuroendocrine Prostate Cancer Development via a Pluripotency Gene Network. EBioMedicine (2018) 35:167–77. doi: 10.1016/j.ebiom.2018.08.011
88. Gritsina G, Gao WQ, Yu J. Transcriptional Repression by Androgen Receptor: Roles in Castration-Resistant Prostate Cancer. Asian J Androl (2019) 21(3):215–23. doi: 10.4103/aja.aja_19_19
89. Cejas P, Xie Y, Font-Tello A, Lim K, Syamala S, Qiu X, et al. Subtype Heterogeneity and Epigenetic Convergence in Neuroendocrine Prostate Cancer. Nat Commun (2021) 12(1):5775. doi: 10.1038/s41467-021-26042-z
90. Liu Q, Pang J, Wang LA, Huang Z, Xu J, Yang X, et al. Histone Demethylase PHF8 Drives Neuroendocrine Prostate Cancer Progression by Epigenetically Upregulating Foxa2. J Pathol (2021) 253(1):106–18. doi: 10.1002/path.5557
91. Ge R, Wang Z, Montironi R, Jiang Z, Cheng M, Santoni M, et al. Epigenetic Modulations and Lineage Plasticity in Advanced Prostate Cancer. Ann Oncol (2020) 31(4):470–9. doi: 10.1016/j.annonc.2020.02.002
92. Davies A, Zoubeidi A, Selth LA. The Epigenetic and Transcriptional Landscape of Neuroendocrine Prostate Cancer. Endocr Relat Cancer (2020) 27(2):R35–50. doi: 10.1530/ERC-19-0420
93. Berger A, Brady NJ, Bareja R, Robinson B, Conteduca V, Augello MA, et al. N-Myc-Mediated Epigenetic Reprogramming Drives Lineage Plasticity in Advanced Prostate Cancer. J Clin Invest (2019) 129(9):3924–40. doi: 10.1172/JCI127961
94. Vickman RE, Franco OE, Moline DC, Vander Griend DJ, Thumbikat P, Hayward SW. The Role of the Androgen Receptor in Prostate Development and Benign Prostatic Hyperplasia: A Review. Asian J Urol (2020) 7(3):191–202. doi: 10.1016/j.ajur.2019.10.003
95. Liu S, Kumari S, Hu Q, Senapati D, Venkadakrishnan VB, Wang D, et al. A Comprehensive Analysis of Coregulator Recruitment, Androgen Receptor Function and Gene Expression in Prostate Cancer. Elife (2017) 6:e28482–513. doi: 10.7554/eLife.28482
96. Guan J, Guo H, Tang T, Wang Y, Wei Y, Seth P, et al. iRGD-Liposomes Enhance Tumor Delivery and Therapeutic Efficacy of Antisense Oligonucleotide Drugs Against Primary Prostate Cancer and Bone Metastasis. Adv Funct Mater (2021) 31(24):2100478–89. doi: 10.1002/adfm.202100478
97. Bernstein E, Caudy AA, Hammond SM, Hannon GJ. Role for a Bidentate Ribonuclease in the Initiation Step of RNA Interference. Nature (2001) 409(6818):363–6. doi: 10.1038/35053110
98. Elbashir SM, Harborth J, Lendeckel W, Yalcin A, Weber K, Tuschl T. Duplexes of 21-Nucleotide RNAs Mediate RNA Interference in Cultured Mammalian Cells. Nature (2001) 411(6836):494–8. doi: 10.1038/35078107
99. Sakamoto KM, Kim KB, Kumagai A, Mercurio F, Crews CM, Deshaies RJ. Protacs: Chimeric Molecules That Target Proteins to the Skp1-Cullin-F Box Complex for Ubiquitination and Degradation. Proc Natl Acad Sci USA (2001) 98(15):8554–9. doi: 10.1073/pnas.141230798
100. Burslem GM, Crews CM. Proteolysis-Targeting Chimeras as Therapeutics and Tools for Biological Discovery. Cell (2020) 181(1):102–14. doi: 10.1016/j.cell.2019.11.031
101. Bekes M, Langley DR, Crews CM. PROTAC Targeted Protein Degraders: The Past Is Prologue. Nat Rev Drug Discov (2022) 2022:1–20. doi: 10.1038/s41573-021-00371-6
102. Qi SM, Dong J, Xu ZY, Cheng XD, Zhang WD, Qin JJ. PROTAC: An Effective Targeted Protein Degradation Strategy for Cancer Therapy. Front Pharmacol (2021) 12:692574. doi: 10.3389/fphar.2021.692574
103. Chen X, Shen H, Shao Y, Ma Q, Niu Y, Shang Z. A Narrative Review of Proteolytic Targeting Chimeras (PROTACs): Future Perspective for Prostate Cancer Therapy. Transl Androl Urol (2021) 10(2):954–62. doi: 10.21037/tau-20-1357
104. Mohler ML, Sikdar A, Ponnusamy S, Hwang DJ, He Y, Miller DD, et al. An Overview of Next-Generation Androgen Receptor-Targeted Therapeutics in Development for the Treatment of Prostate Cancer. Int J Mol Sci (2021) 22(4):2124–43. doi: 10.3390/ijms22042124
105. Zeng S, Huang W, Zheng X, Liyan C, Zhang Z, Wang J, et al. Proteolysis Targeting Chimera (PROTAC) in Drug Discovery Paradigm: Recent Progress and Future Challenges. Eur J Med Chem (2021) 210:112981. doi: 10.1016/j.ejmech.2020.112981
106. Wang Y, Jiang X, Feng F, Liu W, Sun H. Degradation of Proteins by PROTACs and Other Strategies. Acta Pharm Sin B (2020) 10(2):207–38. doi: 10.1016/j.apsb.2019.08.001
107. Ponnusamy S, Coss CC, Thiyagarajan T, Watts K, Hwang DJ, He Y, et al. Novel Selective Agents for the Degradation of Androgen Receptor Variants to Treat Castration-Resistant Prostate Cancer. Cancer Res (2017) 77(22):6282–98. doi: 10.1158/0008-5472.CAN-17-0976
108. Hu P, Guo D, Xie J, Chen H, Hu S, Bian A, et al. Determining the Drug-Like Properties of Ailanthone, a Novel Chinese Medicine Monomer With Anti-CRPC Activity. Planta Med (2020) 86(7):482–8. doi: 10.1055/a-1125-0385
109. Tang S, Ma X, Lu J, Zhang Y, Liu M, Wang X. Preclinical Toxicology and Toxicokinetic Evaluation of Ailanthone, a Natural Product Against Castration-Resistant Prostate Cancer, in Mice. Fitoterapia (2019) 136:104161. doi: 10.1016/j.fitote.2019.04.016
110. Kanayama M, Lu C, Luo J, Antonarakis ES. AR Splicing Variants and Resistance to AR Targeting Agents. Cancers (Basel) (2021) 13(11):2563–78. doi: 10.3390/cancers13112563
111. Moll JM, Hofland J, Teubel WJ, de Ridder CMA, Taylor AE, Graeser R, et al. Abiraterone Switches Castration-Resistant Prostate Cancer Dependency From Adrenal Androgens Towards Androgen Receptor Variants and Glucocorticoid Receptor Signalling. Prostate (2022) 82(5):505–16. doi: 10.1002/pros.24297
112. Sun F, Chen HG, Li W, Yang X, Wang X, Jiang R, et al. Androgen Receptor Splice Variant AR3 Promotes Prostate Cancer via Modulating Expression of Autocrine/Paracrine Factors. J Biol Chem (2014) 289(3):1529–39. doi: 10.1074/jbc.M113.492140
113. Hu R, Dunn TA, Wei S, Isharwal S, Veltri RW, Humphreys E, et al. Ligand-Independent Androgen Receptor Variants Derived From Splicing of Cryptic Exons Signify Hormone-Refractory Prostate Cancer. Cancer Res (2009) 69(1):16–22. doi: 10.1158/0008-5472.CAN-08-2764
114. Kallio HML, Hieta R, Latonen L, Brofeldt A, Annala M, Kivinummi K, et al. Constitutively Active Androgen Receptor Splice Variants AR-V3, AR-V7 and AR-V9 are Co-Expressed in Castration-Resistant Prostate Cancer Metastases. Br J Cancer (2018) 119(3):347–56. doi: 10.1038/s41416-018-0172-0
115. Kohli M, Ho Y, Hillman DW, Van Etten JL, Henzler C, Yang R, et al. Androgen Receptor Variant AR-V9 Is Coexpressed With AR-V7 in Prostate Cancer Metastases and Predicts Abiraterone Resistance. Clin Cancer Res (2017) 23(16):4704–15. doi: 10.1158/1078-0432.CCR-17-0017
116. Liu C, Lou W, Zhu Y, Nadiminty N, Schwartz CT, Evans CP, et al. Niclosamide Inhibits Androgen Receptor Variants Expression and Overcomes Enzalutamide Resistance in Castration-Resistant Prostate Cancer. Clin Cancer Res (2014) 20(12):3198–210. doi: 10.1158/1078-0432.CCR-13-3296
117. Liu C, Armstrong CM, Lou W, Lombard AP, Cucchiara V, Gu X, et al. Niclosamide and Bicalutamide Combination Treatment Overcomes Enzalutamide- and Bicalutamide-Resistant Prostate Cancer. Mol Cancer Ther (2017) 16(8):1521–30. doi: 10.1158/1535-7163.MCT-16-0912
118. Schweizer MT, Haugk K, McKiernan JS, Gulati R, Cheng HH, Maes JL, et al. A Phase I Study of Niclosamide in Combination With Enzalutamide in Men With Castration-Resistant Prostate Cancer. PloS One (2018) 13(6):e0198389. doi: 10.1371/journal.pone.0198389
119. Parikh M, Liu C, Wu CY, Evans CP, Dall'Era M, Robles D, et al. Phase Ib Trial of Reformulated Niclosamide With Abiraterone/Prednisone in Men With Castration-Resistant Prostate Cancer. Sci Rep (2021) 11(1):6377. doi: 10.1038/s41598-021-85969-x
120. Hu H, Zhou H, Xu D. A Review of the Effects and Molecular Mechanisms of Dimethylcurcumin (ASC-J9) on Androgen Receptor-Related Diseases. Chem Biol Drug Des (2021) 97(4):821–35. doi: 10.1111/cbdd.13811
121. Tian H, Chou FJ, Tian J, Zhang Y, You B, Huang CP, et al. ASC-J9(R) Suppresses Prostate Cancer Cell Proliferation and Invasion via Altering the ATF3-PTK2 Signaling. J Exp Clin Cancer Res (2021) 40(1):3. doi: 10.1186/s13046-020-01760-2
122. Huang CP, Chen J, Chen CC, Liu G, Zhang Y, Messing E, et al. ASC-J9(R) Increases the Bladder Cancer Chemotherapy Efficacy via Altering the Androgen Receptor (AR) and NF-kappaB Survival Signals. J Exp Clin Cancer Res (2019) 38(1):275. doi: 10.1186/s13046-019-1258-0
123. Lin W, Luo J, Sun Y, Lin C, Li G, Niu Y, et al. ASC-J9((R)) Suppresses Prostate Cancer Cell Invasion via Altering the Sumoylation-Phosphorylation of STAT3. Cancer Lett (2018) 425:21–30. doi: 10.1016/j.canlet.2018.02.007
124. Wen S, Tian J, Niu Y, Li L, Yeh S, Chang C. ASC-J9((R)), and Not Casodex or Enzalutamide, Suppresses Prostate Cancer Stem/Progenitor Cell Invasion via Altering the EZH2-STAT3 Signals. Cancer Lett (2016) 376(2):377–86. doi: 10.1016/j.canlet.2016.01.057
125. Wen S, Niu Y, Lee SO, Yeh S, Shang Z, Gao H, et al. Targeting Fatty Acid Synthase With ASC-J9 Suppresses Proliferation and Invasion of Prostate Cancer Cells. Mol Carcinog (2016) 55(12):2278–90. doi: 10.1002/mc.22468
126. Tian KM, Li JJ, Xu SW. Rutaecarpine: A Promising Cardiovascular Protective Alkaloid From Evodia Rutaecarpa (Wu Zhu Yu). Pharmacol Res (2019) 141:541–50. doi: 10.1016/j.phrs.2018.12.019
127. Supuran CT. Indisulam: An Anticancer Sulfonamide in Clinical Development. Expert Opin Investig Drugs (2003) 12(2):283–7. doi: 10.1517/13543784.12.2.283
128. Goh JXH, Tan LT, Goh JK, Chan KG, Pusparajah P, Lee LH, et al. Nobiletin and Derivatives: Functional Compounds From Citrus Fruit Peel for Colon Cancer Chemoprevention. Cancers (Basel) (2019) 11(6):867–900. doi: 10.3390/cancers11060867
129. Ashrafizadeh M, Zarrabi A, Saberifar S, Hashemi F, Hushmandi K, Hashemi F, et al. Nobiletin in Cancer Therapy: How This Plant Derived-Natural Compound Targets Various Oncogene and Onco-Suppressor Pathways. Biomedicines (2020) 8(5):110–40. doi: 10.3390/biomedicines8050110
130. Sadar MD. Discovery of Drugs That Directly Target the Intrinsically Disordered Region of the Androgen Receptor. Expert Opin Drug Discov (2020) 15(5):551–60. doi: 10.1080/17460441.2020.1732920
131. Jenster G, van der Korput HA, Trapman J, Brinkmann AO. Identification of Two Transcription Activation Units in the N-Terminal Domain of the Human Androgen Receptor. J Biol Chem (1995) 270(13):7341–6. doi: 10.1074/jbc.270.13.7341
132. Berrevoets CA, Doesburg P, Steketee K, Trapman J, Brinkmann AO. Functional Interactions of the AF-2 Activation Domain Core Region of the Human Androgen Receptor With the Amino-Terminal Domain and With the Transcriptional Coactivator TIF2 (Transcriptional Intermediary Factor2). Mol Endocrinol (1998) 12(8):1172–83. doi: 10.1210/mend.12.8.0153
133. Metzger E, Muller JM, Ferrari S, Buettner R, Schule R. A Novel Inducible Transactivation Domain in the Androgen Receptor: Implications for PRK in Prostate Cancer. EMBO J (2003) 22(2):270–80. doi: 10.1093/emboj/cdg023
134. Andersen RJ, Mawji NR, Wang J, Wang G, Haile S, Myung JK, et al. Regression of Castrate-Recurrent Prostate Cancer by a Small-Molecule Inhibitor of the Amino-Terminus Domain of the Androgen Receptor. Cancer Cell (2010) 17(6):535–46. doi: 10.1016/j.ccr.2010.04.027
135. Myung JK, Banuelos CA, Fernandez JG, Mawji NR, Wang J, Tien AH, et al. An Androgen Receptor N-Terminal Domain Antagonist for Treating Prostate Cancer. J Clin Invest (2013) 123(7):2948–60. doi: 10.1172/JCI66398
136. De Mol E, Fenwick RB, Phang CT, Buzon V, Szulc E, de la Fuente A, et al. EPI-001, A Compound Active Against Castration-Resistant Prostate Cancer, Targets Transactivation Unit 5 of the Androgen Receptor. ACS Chem Biol (2016) 11(9):2499–505. doi: 10.1021/acschembio.6b00182
137. Yang YC, Banuelos CA, Mawji NR, Wang J, Kato M, Haile S, et al. Targeting Androgen Receptor Activation Function-1 With EPI to Overcome Resistance Mechanisms in Castration-Resistant Prostate Cancer. Clin Cancer Res (2016) 22(17):4466–77. doi: 10.1158/1078-0432.CCR-15-2901
138. Hirayama Y, Tam T, Jian K, Andersen RJ, Sadar MD. Combination Therapy With Androgen Receptor N-Terminal Domain Antagonist EPI-7170 and Enzalutamide Yields Synergistic Activity in AR-V7-Positive Prostate Cancer. Mol Oncol (2020) 14(10):2455–70. doi: 10.1002/1878-0261.12770
139. Maurice-Dror C, Le Moigne R, Vaishampayan U, Montgomery RB, Gordon MS, Hong NH, et al. A Phase 1 Study to Assess the Safety, Pharmacokinetics, and Anti-Tumor Activity of the Androgen Receptor N-Terminal Domain Inhibitor Epi-506 in Patients With Metastatic Castration-Resistant Prostate Cancer. Invest New Drugs (2021). doi: 10.1007/s10637-021-01202-6
140. Le Moigne R, Zhou HJ, Obst JK, Banuelos CA, Jian KZ, Williams D, et al. Lessons Learned From the Metastatic Castration Resistant Prostate Cancer Phase I Trial of EPI-506, a First-Generation Androgen Receptor N-Terminal Domain Inhibitor. J Clin Oncol (2019) 37(7_Supplement):257. doi: 10.1200/JCO.2019.37.7_suppl.257
141. Le Moigne R, Pearson P, Lauriault V, Hong NH, Virsik P, Zhou HJ, et al. Preclinical and Clinical Pharmacology of EPI-7386, an Androgen Receptor N-Terminal Domain Inhibitor for Castration-Resistant Prostate Cancer. J Clin Oncol (2021) 39(6_Supplement):119. doi: 10.1200/JCO.2021.39.6_suppl.119
142. Kaku N, Matsuda K, Tsujimura A, Kawata M. Characterization of Nuclear Import of the Domain-Specific Androgen Receptor in Association With the Importin Alpha/Beta and Ran-Guanosine 5'-Triphosphate Systems. Endocrinology (2008) 149(8):3960–9. doi: 10.1210/en.2008-0137
143. Dar JA, Masoodi KZ, Eisermann K, Isharwal S, Ai J, Pascal LE, et al. The N-Terminal Domain of the Androgen Receptor Drives its Nuclear Localization in Castration-Resistant Prostate Cancer Cells. J Steroid Biochem Mol Biol (2014) 143:473–80. doi: 10.1016/j.jsbmb.2014.03.004
144. Zhou ZX, Sar M, Simental JA, Lane MV, Wilson EM. A Ligand-Dependent Bipartite Nuclear Targeting Signal in the Human Androgen Receptor. Requirement for the DNA-Binding Domain and Modulation by NH2-Terminal and Carboxyl-Terminal Sequences. J Biol Chem (1994) 269(18):13115–23. doi: 10.1016/S0021-9258(17)36806-0
145. Saporita AJ, Zhang Q, Navai N, Dincer Z, Hahn J, Cai X, et al. Identification and Characterization of a Ligand-Regulated Nuclear Export Signal in Androgen Receptor. J Biol Chem (2003) 278(43):41998–2005. doi: 10.1074/jbc.M302460200
146. Shaffer PL, Jivan A, Dollins DE, Claessens F, Gewirth DT. Structural Basis of Androgen Receptor Binding to Selective Androgen Response Elements. Proc Natl Acad Sci USA (2004) 101(14):4758–63. doi: 10.1073/pnas.0401123101
Keywords: androgen receptor, prostate cancer, small interfering RNA, protein degradation, PROTAC
Citation: Huang J, Lin B and Li B (2022) Anti-Androgen Receptor Therapies in Prostate Cancer: A Brief Update and Perspective. Front. Oncol. 12:865350. doi: 10.3389/fonc.2022.865350
Received: 29 January 2022; Accepted: 17 February 2022;
Published: 10 March 2022.
Edited by:
Olivier Cuvillier, UPR8241 Laboratoire de Chimie de Coordination (LCC), FranceReviewed by:
Elahe A. Mostaghel, Fred Hutchinson Cancer Research Center, United StatesAntimo Migliaccio, University of Campania Luigi Vanvitelli, Italy
Copyright © 2022 Huang, Lin and Li. This is an open-access article distributed under the terms of the Creative Commons Attribution License (CC BY). The use, distribution or reproduction in other forums is permitted, provided the original author(s) and the copyright owner(s) are credited and that the original publication in this journal is cited, in accordance with accepted academic practice. No use, distribution or reproduction is permitted which does not comply with these terms.
*Correspondence: Benyi Li, YmxpQGt1bWMuZWR1