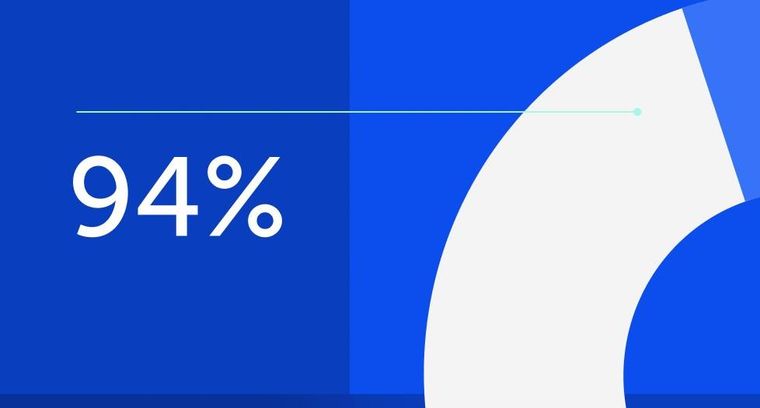
94% of researchers rate our articles as excellent or good
Learn more about the work of our research integrity team to safeguard the quality of each article we publish.
Find out more
BRIEF RESEARCH REPORT article
Front. Oncol., 21 March 2022
Sec. Hematologic Malignancies
Volume 12 - 2022 | https://doi.org/10.3389/fonc.2022.862991
FLT3-ITD mutations are common druggable alterations in patients with acute myeloid leukemia (AML) and associated with poor prognosis. Beside typical ITD mutations, point mutations and deletions in the juxtamembrane domain (JMD) have been observed. However, due to the low frequency of these alterations, there is only limited information on molecular and clinical associations. To evaluate the prognostic impact of non-ITD mutations in the FLT3 JMD region, we analyzed a large cohort of 1,539 adult AML patients treated in different protocols of the Study Alliance Leukemia, using next-generation sequencing. Non-ITD point mutations and deletions within the FLT3 JMD were identified with a prevalence of ~1.23% (n = 19). Both FLT3-ITD and non-ITD mutations were associated with a higher rate of NPM1 (42%–61%; p < 0.001) and DNMT3A mutations (37%–43%; p < 0.001), as well as an increased percentage of peripheral blood (54%–65%) and bone marrow blast cells (74%; p < 0.001), compared to FLT3-wild-type patients. Most significantly, AML patients with FLT3 non-ITD mutations had a higher rate of concomitant KMT2A-PTD mutations (37.5%; p < 0.001) as compared to FLT3-ITD (7%) or FLT3-wild-type cases (4.5%). In a multivariable analysis, FLT3 non-ITD mutations were not an independent prognostic factor. However, patients with dual FLT3 non-ITD and KMT2A-PTD mutations showed a trend for inferior outcome, which points at a functional interaction in this subset of AML.
The FMS-like tyrosine kinase 3 (FLT3) is a transmembrane receptor tyrosine kinase, which is expressed by hematopoietic stem cells and stimulates the development of myeloid and lymphoid progenitor cells (1). Mutations of the FLT3 gene are identified in ~30% of patients with acute myeloid leukemia (AML). About 20%–25% of AML patients show internal tandem duplications (FLT3-ITD), affecting the juxtamembrane domain (JMD) and/or the tyrosine kinase domain-1 (TKD1) of FLT3 (2). In addition, mutations in exon 20, coding for the TKD2 region (most frequently affecting codons D835 and I836), can be detected in 7%–10% of AML patients (FLT3-TKD) (1, 2).
Both FLT3-ITD and FLT3-TKD mutations constitutively activate the FLT3 kinase, inducing proliferation of leukemic populations, although differences in the signaling induced have been reported between these two mutations (1). While the clinical significance of FLT3-TKD mutations is uncertain, the presence of FLT3-ITD mutations in AML patients confers a poor prognosis with an increased risk of relapse and shorter overall survival (2, 3). Consequently, the FLT3-ITD mutational status is included in the current risk classification of the ELN-2017 recommendations and is recognized as target for specific TK inhibitors (2, 4, 5). However, several factors modify the prognostic impact of FLT3-ITD mutations, such as the allelic ratio and the presence of a concomitant NPM1 mutation (4).
In addition to typical ITD mutations, previous reports indicated the presence of rare non-ITD mutations (small deletions and point mutations) in the FLT3 JMD of AML patients (6–16). However, due to the low frequency of these alterations, there are so far only casuistic reports available, with limited information on molecular and clinical associations. To investigate the prevalence and prognostic impact of non-ITD mutations in the FLT3 JMD of adult patients with AML, we analyzed a large cohort of 1,539 patients with newly diagnosed AML to correlate these mutations with clinical characteristics, co-mutations, and outcome.
All patients (n = 1,539) investigated had newly diagnosed AML, were registered in clinical protocols of the Study Alliance Leukemia (SAL) (AML96, AML2003 or AML60+, SORAML), and had available biomaterial at diagnosis. Detailed descriptions of the treatment protocols have been published previously (17–20); all protocols included intensive induction chemotherapy and consolidation treatment according to cytogenetic risk groups. The study was in agreement with the Helsinki declaration and approved by the ethical board of the Technical University Dresden (EK98032010).
Molecular studies were performed on genomic DNA isolated from bone marrow (BM) aspirates or peripheral blood (PB) taken at diagnosis. DNA was extracted using the DNeasy Blood and Tissue Kit (Qiagen, Hilden, Germany) and quantified with the NanoDrop spectrophotometer. In addition to conventional fragment analysis, profiling of FLT3 JMD/TKD1 mutational status and associated co-mutations was performed by targeted resequencing using the TruSight Myeloid assay (Illumina, San Diego, CA, USA) (Supplementary Methods). Briefly, for FLT3 the panel covers the entire ITD region (AA 572–630). Libraries were sequenced paired-end (150 bp) on a NextSeq instrument (Illumina) and analyzed using the Sequence Pilot Software (JSI medical systems) with a 5% variant allele frequency (VAF) mutation calling cutoff. KMT2A-PTD (partial tandem duplication) mutations (formerly MLL-PTD) were analyzed on cDNA (reverse transcribed from 1 µg of total RNA; SuperScript VILO cDNA Synthesis Kit; Invitrogen, Carlsbad, CA, USA), using the Mentype AMLplex QS Kit (Biotype, Dresden, Germany) on a 3130xl Genetic Analyzer (Applied Biosystems, Foster, CA, USA).
Categorical variables between groups were compared using the chi-squared test or a 2-sided Fisher’s exact test. For continuous variables the non-parametric Mann–Whitney U test was applied. p values <0.05 were considered significant. To evaluate relapse-free survival (RFS) and overall survival (OS), the Kaplan–Meier method and the log-rank test were used. For multivariable analysis of prognostic factors, Cox-proportional hazard regression models were used for survival endpoints, and logistic regression models were used for CR. All statistical analyses were performed using the R environment for statistical computing version 4.0.3.
FLT3-ITD mutations were detected in 324 of 1,539 (21.1%) AML patients. Non-ITD mutations within the FLT3 JMD were found in 19 cases (1.23%) (Figure 1A). Patients with mutations in the TKD2 region (FLT3 Exon 20; D835 and I836; n = 104) were excluded from this analysis. Patients without JMD/TKD1 mutation were classified as FLT3-ITD wild-type (wt) (n = 1,196). Most non-ITD mutations were single-nucleotide missense variants (SNV; n = 15; Figure 1B). Deletions were found in 4 patients, exclusively comprising small in-frame deletions (1–4 amino acid residues). FLT3 non-ITD mutations were detected with a median VAF of 28% (range 7%–50%) at subclonal levels in the majority of patients (73.7%; Table S1). Recurrent point mutations affected residues V592 (n = 7), Y572 (n = 3), and L576 (n = 2) (Figure 1B). Compared to FLT3-wt patients, both ITD and non-ITD mutations had increased rates of concomitant mutations in NPM1 (60.7% and 42.1% vs. 23.4%; p < 0.001) and DNMT3A (43% and 37% vs. 24%; p < 0.001; Table 1 and Figure 1C). Vice versa, FLT3-wt patients were significantly more often affected by mutations in NRAS and KIT. No major difference between FLT3 mutant and -wt patients was observed for other frequently mutated genes in AML, such as IDH1 or TET2 (Table S2). Notably, non-ITD mutations (exclusively point mutations) were associated with a higher frequency of concomitant KMT2A-PTD mutations (37.5% vs. 7% and 4.5%; p < 0.001), compared to FLT3-ITD or FLT3-wt patients (Table 1 and Figure 1C). In addition to KMT2A-PTD, other 11q abnormalities were also significantly more frequent in patients with non-ITD variants (Table 1).
Figure 1 Clinical and molecular associations of FLT3 non-ITD mutations in AML. (A) Frequencies of molecular alterations detected in the FLT3 JMD/TKD1 region of 1,539 AML patients: ITD, (internal tandem duplication); PM, (point mutation); and Del, (deletion). (B) Schematic illustration showing the position of acquired FLT3 non-ITD mutations (triangles = point mutations, circles = deletions) and the domain structure of FLT3: lg-like, (ligand binding domain); TM, (transmembrane domain); JMD, (juxtamembrane domain); TKD1, (tyrosine kinase domain-1); KI, (kinase insert); TKD2, (tyrosine kinase domain-2). (C) Associated co-mutations in FLT3 non-ITD patients. KMT2A-PTD data are available for 16/19 patients with FLT3 non-ITD mutation. Genes with <10% mutation rate are not shown: EZH2, ASXL1, IKFZ1, NRAS, RUNX1, SRSF2, TP53. (D) Kaplan–Meier analysis showing the probability of overall survival (OS) for AML patients without molecular alterations in the FLT3-ITD region (wt; n = 1,196; black), ITD mutation (n = 324; red), and non-ITD mutation (n = 19; blue). (E) Results of the multivariable analysis of prognostic factors for overall survival, (OS); relapse-free survival, (RFS) and complete remission, (CR1).
For patients with FLT3 non-ITD mutations, the median follow-up was 108 months (IQR 80–127 months). The first complete remission (CR1) after initial treatment was achieved in 16/19 (84.2%) patients. The relapse rate after CR1 was 62.5% (10/16). Of these patients, 20% (2/10) achieved a second CR. The comparison between patients with FLT3 non-ITD and ITD variants revealed significant similarities (as compared to FLT3-wt patients) but also several differences of clinical parameters (Table 1). Patients with non-ITD mutations (median age 51; range 45–60 years) were diagnosed significantly more often with tAML (10.5% vs. 2.2%; p = 0.045) and had a higher rate of karyotype aberrations compared to FLT3-ITD patients (62.5% vs. 25.4%; p = 0.003). No differences were detected for the relative proportion of ELN-2017 adverse risk, the ECOG performance status, or the presence of a complex aberrant karyotype between both groups. Likewise, general similarities were observed for most laboratory parameters, such as WBC counts (median 39.6–46.09 Gpt l-1), PB (median 54–63 Gpt l-1), and BM blasts (median 74%). In contrast, FLT3-wt status was associated with a higher rate of ELN-2017 adverse risk (38.2%; p < 0.001) and complex aberrant karyotype (16.2%; p < 0.001), as well as lower WBC counts and lower rates of PB and BM blasts compared to FLT3 mutant patients. With respect to clinical outcome, FLT3 non-ITD mutations were not associated with CR rate (85%), relapse-free survival (median 18.5 months), or overall survival (median 31.7 months) in univariate analyses (Figure 1D and Table 1). Likewise, the multivariable analysis revealed that non-ITD mutations (as well as FLT3-ITD mutations) were not an independent prognostic factor for outcome (Figure 1E and Table S3). Interestingly, there was an insignificant trend toward inferior RFS (HR 6.64; CI 95% 0.691–63.9; p = 0.101; Figure 1E) for patients with the mutual presence of FLT3 non-ITD and KMT2A-PTD mutations (Figure S1 and Table S3). Regarding the effect of transplantation on outcome, only two (out of 19) patients with FLT3 non-ITD mutations underwent allogeneic hematopoietic stem cell transplantation (alloHSCT) without subsequent event (Figure S2). Thus, compared to FLT3-ITD and -wt patients, no significant differences were observed.
In the present study, we analyzed 1,539 adult AML patients for the prevalence and prognostic impact of non-ITD mutations in the JMD/TKD1 region of FLT3. We confirm that JMD point mutations and deletions are rare, but recurrent alterations in patients with AML at a frequency in the range of previous estimates (8, 9). Compared to AML, higher rates (~4-fold) of JMD non-ITD mutations were previously detected in patients with acute lymphoblastic leukemia (ALL) (8). Among non-ITD mutations in our cohort, the point mutations Y572C, V592G (9), L576Q (10), G583S (8), Y591H (11), and V592A (12, 13) as well as the deletion EY598_599del (14) were previously recognized as gain-of-function mutations that result in constitutive kinase activation and stimulate AML growth through aberrant STAT5 signaling (9, 12, 14). In addition, in one patient we identified a novel and likely damaging (PolyPhen score = 1) point mutation at Y599N (30% VAF), not previously reported in the literature. However, functional data indicate that any alteration of the JMD sequence interferes with the kinase auto-inhibition, similar to the effect of FLT3-ITD mutations (9, 11, 15). Accordingly, in vitro studies illustrated the response of AML cells with non-ITD JMD mutations to pharmacologic FLT3 inhibition (9, 10, 12, 14–16), which supports the idea to use any of the approved TKIs in patients with this type of mutation (5). In line with this, we demonstrate high similarities between patients with FLT3-ITD and non-ITD mutations, with respect to major laboratory parameters (i.e., an increased percentage of blast cells in PB and BM) and the rate of co-mutated driver variants, which is consistent with the mutational landscape and clinical phenotype typically observed for patients with FLT3-ITD mutations (1–3, 21). For example, a high rate of concomitant NPM1 and DNMT3A mutations but mutual exclusivity with downstream effectors such as NRAS in FLT3 mutant patients has been described in detail (21). Similar to our data, partial tandem duplications (PTD) of the histone methyltransferase KMT2A (MLL) occur in 3%–5% of AML cases and are typically enriched in patients with FLT3-ITD mutations (as compared to FLT3-wt) (21, 22). In addition, we show a not previously reported high association of KMT2A-PTD mutations with FLT3 JMD point mutations, which adds on initial observations on the genomic landscape of KMT2A-PTD-mutated AML (22). More importantly, although the presence of FLT3 non-ITD mutations alone was not an independent prognostic factor, patients with dual non-ITD and KMT2A-PTD mutations showed a trend for inferior outcome. While KMT2A-PTD mutations are typically associated with poor prognosis, isolated KMT2A-PTD mutations are not sufficient to establish leukemic transformation of hematopoietic cells, highlighting the importance of cooperating genetic lesions, such as FLT3 mutations in KMT2A-rearranged leukemias (22–24). Likewise, functional data indicate the transcriptional regulation of FLT3 by KMT2A via aberrant MEIS1 gene expression (25). More recently, the combined inhibition of menin-MLL (MLL1, KMT2A) and FLT3 demonstrated a synergistic therapeutic opportunity in these leukemia subtypes (26). However, mutations of genes associated with RAS signaling (i.e., FLT3) may be frequently lost or emerge during AML progression, with relevance for post-remission strategies (27). In this regard, future investigations are needed to evaluate the impact of FLT3 non-ITD mutations for relapse development. In conclusion, we show that FLT3 non-ITD mutations are rare but recurrent alterations in AML and associated with similar clinical features like FLT3-ITD variants. Interestingly, our data point at a functional interaction of FLT3 non-ITD mutations with KMT2A-PTD and 11q-abnormalities as cooperating genetic events, which might be indicative of a distinct pathway of genesis in this subset of AML.
The datasets presented in this article are not readily available because patients did not consent to have data uploaded to a public database. Requests to access the datasets should be directed to the corresponding author on reasonable request: Y2hyaXN0aWFuLnRoaWVkZUB1bmlrbGluaWt1bS1kcmVzZGVuLmRl.
The studies involving human participants were reviewed and approved by Technical University Dresden (EK98032010). The patients/participants provided their written informed consent to participate in this study.
Conception of the work: CT, SS. Sample/Data Collection: All authors. Aquisition/Analysis of Data: CT, SS. Bioinformatic Analysis: SS. Statistical Analysis: MK, SZ. Interpretation of Data: CT, SS. Drafted the manuscript: SS. Administrative support: GE, MB. All authors have read and approved the manuscript being submitted.
CT is CEO and co-owner of AgenDix GmbH, a company performing molecular diagnostics.
The authors declare that the research was conducted in the absence of any commercial or financial relationships that could be construed as a potential conflict of interest.
All claims expressed in this article are solely those of the authors and do not necessarily represent those of their affiliated organizations, or those of the publisher, the editors and the reviewers. Any product that may be evaluated in this article, or claim that may be made by its manufacturer, is not guaranteed or endorsed by the publisher.
For skillful technical assistance, M. Böhm and M. Hartwig are highly acknowledged.
The Supplementary Material for this article can be found online at: https://www.frontiersin.org/articles/10.3389/fonc.2022.862991/full#supplementary-material
1. Takahashi S. Downstream Molecular Pathways of FLT3 in the Pathogenesis of Acute Myeloid Leukemia: Biology and Therapeutic Implications. J Hematol Oncol (2011) 4:13. doi: 10.1186/1756-8722-4-13
2. Daver N, Schlenk RF, Russell NH, Levis MJ. Targeting FLT3 Mutations in AML: Review of Current Knowledge and Evidence. Leukemia (2019) 33(2):299–312. doi: 10.1038/s41375-018-0357-9
3. Port M, Böttcher M, Thol F, Ganser A, Schlenk R, Wasem J, et al. Prognostic Significance of FLT3 Internal Tandem Duplication, Nucleophosmin 1, and CEBPA Gene Mutations for Acute Myeloid Leukemia Patients With Normal Karyotype and Younger Than 60 Years: A Systematic Review and Meta-Analysis. Ann Hematol (2014) 93(8):1279–86. doi: 10.1007/s00277-014-2072-6
4. Döhner H, Estey E, Grimwade D, Amadori S, Appelbaum FR, Büchner T, et al. Diagnosis and Management of AML in Adults: 2017 ELN Recommendations From an International Expert Panel. Blood (2017) 129(4):424–47. doi: 10.1182/blood-2016-08-733196
5. Stone RM, Mandrekar SJ, Sanford BL, Laumann K, Geyer S, Bloomfield CD, et al. Midostaurin Plus Chemotherapy for Acute Myeloid Leukemia With a FLT3 Mutation. N Engl J Med (2017) 377(5):454–64. doi: 10.1056/NEJMoa1614359
6. Syampurnawati M, Tatsumi E, Furuta K, Hayashi Y. Four Novel Point Mutations in Exons 12, 13, and 14 of the FLT3 Gene. Leuk Res (2007) 31(6):877. doi: 10.1016/j.leukres.2006.07.020
7. Gianfelici V, Diverio D, Breccia M, Buffolino S, Derme V, Di Lascio A, et al. A Novel Point Mutation Within the Juxtamembrane Domain of the Flt3 Gene in Acute Myeloid Leukemia. Ann Hematol (2011) 90(7):845–6. doi: 10.1007/s00277-010-1092-0
8. Zhang Y, Zhang Y, Wang F, Wang M, Liu H, Chen X, et al. The Mutational Spectrum of FLT3 Gene in Acute Lymphoblastic Leukemia is Different From Acute Myeloid Leukemia. Cancer Gene Ther (2020) 27(1-2):81–8. doi: 10.1038/s41417-019-0120-z
9. Fröhling S, Scholl C, Levine RL, Loriaux M, Boggon TJ, Bernard OA, et al. Identification of Driver and Passenger Mutations of FLT3 by High-Throughput DNA Sequence Analysis and Functional Assessment of Candidate Alleles. Cancer Cell (2007) 12(6):501–13. doi: 10.1016/j.ccr.2007.11.005
10. Martínez-López J, Castro N, Rueda D, Canal A, Grande C, Ayala R. Use of Sorafenib as an Effective Treatment in an AML Patient Carrying a New Point Mutation Affecting the Juxtamembrane Domain of FLT3. Br J Haematol (2012) 158(4):555–8. doi: 10.1111/j.1365-2141.2012.09189.x
11. Rocnik JL, Okabe R, Yu JC, Lee BH, Giese N, Schenkein DP, et al. Roles of Tyrosine 589 and 591 in STAT5 Activation and Transformation Mediated by FLT3-ITD. Blood (2006) 108(4):1339–45. doi: 10.1182/blood-2005-11-011429
12. Reindl C, Bagrintseva K, Vempati S, Schnittger S, Ellwart JW, Wenig K, et al. Point Mutations in the Juxtamembrane Domain of FLT3 Define a New Class of Activating Mutations in AML. Blood (2006) 107(9):3700–7. doi: 10.1182/blood-2005-06-2596
13. Stirewalt DL, Meshinchi S, Kussick SJ, Sheets KM, Pogosova-Agadjanyan E, Willman CL, et al. Novel FLT3 Point Mutations Within Exon 14 Found in Patients With Acute Myeloid Leukaemia. Br J Haematol (2004) 124(4):481–4. doi: 10.1111/j.1365-2141.2004.04808.x
14. Chatain N, Perera RC, Rossetti G, Rossa J, Carloni P, Schemionek M, et al. Rare FLT3 Deletion Mutants may Provide Additional Treatment Options to Patients With AML: An Approach to Individualized Medicine. Leukemia (2015) 29(12):2434–8. doi: 10.1038/leu.2015.131
15. Young DJ, Nguyen B, Zhu R, Seo J, Li L, Levis MJ, et al. Deletions in FLT-3 Juxtamembrane Domain Define a New Class of Pathogenic Mutations: Case Report and Systematic Analysis. Blood Adv (2021) 5(9):2285–93. doi: 10.1182/bloodadvances.2020002876
16. Bruno S, Bandini L, Patuelli A, Robustelli V, Venturi C, Mancini M, et al. Case Report: A Novel Activating FLT3 Mutation in Acute Myeloid Leukemia. Front Oncol (2021) 11:728613. doi: 10.3389/fonc.2021.728613
17. Schaich M, Röllig C, Soucek S, Kramer M, Thiede C, Mohr B, et al. Cytarabine Dose of 36 G/M² Compared With 12 G/M² Within First Consolidation in Acute Myeloid Leukemia: Results of Patients Enrolled Onto the Prospective Randomized AML96 Study. J Clin Oncol (2011) 29(19):2696–702. doi: 10.1200/JCO.2010.33.7303
18. Röllig C, Kramer M, Gabrecht M, Hänel M, Herbst R, Kaiser U, et al. Intermediate-Dose Cytarabine Plus Mitoxantrone Versus Standard-Dose Cytarabine Plus Daunorubicin for Acute Myeloid Leukemia in Elderly Patients. Ann Oncol (2018) 29(4):973–8. doi: 10.1093/annonc/mdy030
19. Schetelig J, Schaich M, Schäfer-Eckart K, Hänel M, Aulitzky WE, Einsele H, et al. Hematopoietic Cell Transplantation in Patients With Intermediate and High-Risk AML: Results From the Randomized Study Alliance Leukemia (SAL) AML 2003 Trial. Leukemia (2015) 29(5):1060–8. doi: 10.1038/leu.2014.335
20. Röllig C, Serve H, Hüttmann A, Noppeney R, Müller-Tidow C, Krug U, et al. Addition of Sorafenib Versus Placebo to Standard Therapy in Patients Aged 60 Years or Younger With Newly Diagnosed Acute Myeloid Leukaemia (SORAML): A Multicentre, Phase 2, Randomised Controlled Trial. Lancet Oncol (2015) 16(16):1691–9. doi: 10.1016/S1470-2045(15)00362-9
21. Kiyoi H, Kawashima N, Ishikawa Y. FLT3 Mutations in Acute Myeloid Leukemia: Therapeutic Paradigm Beyond Inhibitor Development. Cancer Sci (2020) 111(2):312–22. doi: 10.1111/cas.14274
22. Sun QY, Ding LW, Tan KT, Chien W, Mayakonda A, Lin DC, et al. Ordering of Mutations in Acute Myeloid Leukemia With Partial Tandem Duplication of MLL (MLL-PTD). Leukemia (2017) 31(1):1–10. doi: 10.1038/leu.2016.160
23. Stam RW, den Boer ML, Schneider P, Meier M, Beverloo HB, Pieters R. D-HPLC Analysis of the Entire FLT3 Gene in MLL Rearranged and Hyperdiploid Acute Lymphoblastic Leukemia. Haematologica (2007) 92(11):1565–8. doi: 10.3324/haematol.11220
24. Stubbs MC, Kim YM, Krivtsov AV, Wright RD, Feng Z, Agarwal J, et al. MLL-AF9 and FLT3 Cooperation in Acute Myelogenous Leukemia: Development of a Model for Rapid Therapeutic Assessment. Leukemia (2008) 22(1):66–77. doi: 10.1038/sj.leu.2404951
25. Kühn MW, Song E, Feng Z, Sinha A, Chen CW, Deshpande AJ, et al. Targeting Chromatin Regulators Inhibits Leukemogenic Gene Expression in NPM1 Mutant Leukemia. Cancer Discov (2016) 6(10):1166–81. doi: 10.1158/2159-8290.CD-16-0237
26. Dzama MM, Steiner M, Rausch J, Sasca D, Schönfeld J, Kunz K, et al. Synergistic Targeting of FLT3 Mutations in AML via Combined Menin-MLL and FLT3 Inhibition. Blood (2020) 136(21):2442–56. doi: 10.1182/blood.2020005037
27. Onecha E, Rapado I, Luz Morales M, Carreño-Tarragona G, Martinez-Sanchez P, Gutierrez X, et al. Monitoring of Clonal Evolution of Acute Myeloid Leukemia Identifies the Leukemia Subtype, Clinical Outcome and Potential New Drug Targets for Post-Remission Strategies or Relapse. Haematologica (2021) 106(9):2325–33. doi: 10.3324/haematol.2020.254623
Keywords: FLT3-ITD, point mutations, acute myeloid leukemia (AML), clinical outcome, KMT2A-PTD
Citation: Stasik S, Kramer M, Zukunft S, Röllig C, Baldus CD, Platzbecker U, Serve H, Müller-Tidow C, Schäfer-Eckart K, Kaufmann M, Krause S, Sauer T, Hänel M, Neubauer A, Ehninger G, Bornhäuser M, Schetelig J, Middeke JM and Thiede C (2022) Point Mutations in the FLT3-ITD Region Are Rare but Recurrent Alterations in Adult AML and Associated With Concomitant KMT2A-PTD. Front. Oncol. 12:862991. doi: 10.3389/fonc.2022.862991
Received: 26 January 2022; Accepted: 23 February 2022;
Published: 21 March 2022.
Edited by:
Cyrus Khandanpour, University Hospital Münster, GermanyReviewed by:
Sheng-Li Xue, The First Affiliated Hospital of Soochow University, ChinaCopyright © 2022 Stasik, Kramer, Zukunft, Röllig, Baldus, Platzbecker, Serve, Müller-Tidow, Schäfer-Eckart, Kaufmann, Krause, Sauer, Hänel, Neubauer, Ehninger, Bornhäuser, Schetelig, Middeke and Thiede. This is an open-access article distributed under the terms of the Creative Commons Attribution License (CC BY). The use, distribution or reproduction in other forums is permitted, provided the original author(s) and the copyright owner(s) are credited and that the original publication in this journal is cited, in accordance with accepted academic practice. No use, distribution or reproduction is permitted which does not comply with these terms.
*Correspondence: Christian Thiede, Y2hyaXN0aWFuLnRoaWVkZUB1bmlrbGluaWt1bS1kcmVzZGVuLmRl
Disclaimer: All claims expressed in this article are solely those of the authors and do not necessarily represent those of their affiliated organizations, or those of the publisher, the editors and the reviewers. Any product that may be evaluated in this article or claim that may be made by its manufacturer is not guaranteed or endorsed by the publisher.
Research integrity at Frontiers
Learn more about the work of our research integrity team to safeguard the quality of each article we publish.