- 1Champalimaud Research, Champalimaud Foundation, Lisbon, Portugal
- 2Colorectal Surgery Department, Champalimaud Clinical Centre, Champalimaud Foundation, Lisbon, Portugal
- 3Radiation Oncology Department, Champalimaud Clinical Centre, Champalimaud Foundation, Lisbon, Portugal
- 4Gastroenterology Department, Champalimaud Clinical Centre, Champalimaud Foundation, Lisbon, Portugal
- 5Radiology Department, Champalimaud Clinical Centre, Champalimaud Foundation, Lisbon, Portugal
- 6Pathology Service, Champalimaud Clinical Centre, Champalimaud Foundation, Lisbon, Portugal
Neoadjuvant chemoradiation (nCRT) followed by surgery represents the standard of care in patients with locally advanced rectal cancer. Increasing radiotherapy (RT) doses and chemotherapy cycles with 5FU have been associated with increased rates of complete response, however these strategies imply significant toxicity. In the last years, epidemiologic findings have demonstrated that metformin is associated with significantly higher rates of pathological complete response to nCRT. Also, pre-clinical studies using cell lines provide evidence for the radiosensitive effect of metformin. However, no studies have been performed using rectal cancer patient samples to test this radiosensitive effect of metformin and compared it to the standard 5FU. Here, we designed an experimental study to compare both radiosensitizers in the zebrafish xenograft model (zAvatar), using rectal cancer surgical specimens and diagnostic biopsies. Patient zAvatars confirmed that metformin has indeed a powerful in vivo radiosensitizer effect, similar to 5FU. Our work confirms that metformin constitutes a promising less toxic alternative to the standard 5FU, which could be game changing in elderly/frail patients to optimize tumor regression.
Introduction
ESMO guidelines for neoadjuvant therapy in rectal cancer relies on two approaches: long course neoadjuvant chemoradiotherapy (LC-CRT), consisting of 25–28 fractions of 1.8–2Gy with concomitant administration of 5FU; and short-course radiotherapy (scRT), consisting of 5Gy over five consecutive days (5×5Gy), usually followed by 5FU infusion (1).
The benefit of irradiating the rectum before surgery has been associated with high rates of tumor shrinkage, resulting in better local control of the disease (2). In some cases, tumor response to radiotherapy (RT) leads to a complete disappearance of the tumor with excellent survival rates (3, 4). In addition, patients with endoscopic and radiological evidence of complete tumor response after nCRT may follow a non-operative management approach (Watch & Wait) avoiding all major consequences of surgery with similar oncological outcomes (5–7). In this scenario, two issues become most relevant: i) increase tumor response rates to radiotherapy to avoid surgery-related morbidity; ii) identify patients who will not respond to nCRT to avoid radiation-related morbidity and proceed immediately to surgery. Escalating radiation therapy doses and additional chemotherapy cycles have been associated with increased rates of complete response to nCRT (8–10). However, these strategies may increase toxicity and morbidity rates (11, 12).
Interestingly, some clinical studies associated the use of metformin (MET), a drug used for diabetes treatment, with higher rates of complete tumor response to nCRT in patients with rectal cancer (13, 14). In addition, experimental studies in vitro and in vivo using colorectal cancer (CRC) cell lines and mouse models, have demonstrated that association of MET with RT was as efficient as the classical association of RT with 5FU in impairing tumor growth, suggesting its use as an alternative radiosensitizing agent (15). However, to our knowledge no studies have yet been performed using rectal cancer patient samples in vivo.
In the last years a novel in vivo model has been developed, the zebrafish Patient Derived Xenograft -”zAvatar” model for personalized medicine (16–18). This assay relies on the injection of fluorescently labelled tumor cells into 2 days post fertilization (dpf) zebrafish embryos and accessing tumor behavior and response to anti-cancer therapy after 3-4 days. zAvatars offer speed, single-cell resolution, large numbers of transplants and evaluation of crucial cancer hallmarks, only possible due to the high genetic conservation between the human and the zebrafish genome. Recently, our group developed a protocol to assess in vivo radiotherapy response. We showed that is possible to distinguish radiosensitive from radioresistant tumors in zebrafish xenografts, even in polyclonal tumors, in just 4 days (19).
Here, we present a short report where we evaluated the radiosensitizing effect of metformin in vivo using zAvatars. We used not only CRC cell lines but also patient samples without in vitro expansion: rectal cancer surgical specimens and, importantly, rectal tumor diagnostic biopsies, which is technically challenging due to the small amount obtained by endoscopy. Our results provide further evidence of the radiosensitive effect of metformin in rectal cancer. Patient zAvatars tumors showed a diversity of responses but in general MET was beneficial in sensitizing the tumors to radiotherapy. This diversity of responses underlines the necessity for a personalized test prior to treatment, a clear unmet need in the oncology field.
Material and methods
Animal care and handling
In vivo experiments were performed using zebrafish (Danio rerio), which were maintained and handled in accordance with European Animal Welfare Legislation, Directive (2010/63/EU), and Champalimaud Fish Platform. The study and procedures were approved by the Ethical Committee and Portuguese institutional organizations: ORBEA (Animal Welfare and Ethics Body) and DGAV (Directorate General for Food and Veterinary).
Zebrafish lines
Experiments were performed using transparent Nacre, which has complete lack of melanocytes due to a mutation in the gene encoding the mitfa gene (20), and Tg(Fli1:eGFP), which allows the visualization of blood and lymphatic vessels, through the expression of eGFP linked to fli1 (endothelial marker) promoter (21).
Patient samples
The study was approved by the Ethics Committee of the Champalimaud Foundation. Rectal cancer patient samples were provided by Champalimaud Clinical Center’s (CCC) Digestive Unit, after signed informed consent. Patients’ inclusion criteria were: pathology confirmed adenocarcinoma of the rectum (tumor below 15 cm from the anal verge). Exclusion criteria: previous diagnosis of diabetes, under metformin treatment. Tissue from surgically resected rectal cancer samples and biopsies were collected in culture media containing a mixture of antibiotics and antifungals (Supplementary Table S1) and cryopreserved until injection. For microinjection, samples were thawed and minced in Mix1 (Supplementary Table S1) with subsequent mechanical tissue fragmentation and centrifugation (250xg, 4 min). The remaining tissue fragments were enzymatically digested in Mix2 (Supplementary Table S1), passed through a 70-um cell strainer and labeled at 37°C. Tumor cells were resuspended in Mix1 and checked for viability with Trypan Blue dye exclusion.
Human colorectal cancer cell lines
HCT116 (KRASG13D) and Hke3 (KRASWT) were kindly provided by Angela Relógio (Institute for Theoretical Biology, Berlin). Cell lines were tested for mycoplasma and authenticated through Short Tandem Repeat (STR) profiling.
Cell culture
Cell lines were expanded and maintained in Dulbecco’s Modified Eagle Medium (DMEM) High Glucose (Biowest) supplemented with 10% (v/v) Fetal Bovine Serum (FBS) (Sigma-Aldrich) and 1% (v/v) Penicillin-Streptomycin 10,000 U/mL (Hyclone). Cells were maintained with a humidified atmosphere at 5% CO2 and 37°C.
Cell staining
Cells were labeled with Vybrant CM-DiI (Thermo Fisher Scientific) at a concentration of 4μL/mL or with Deep Red (CellTracker, Thermo Fisher Scientific) at a concentration of 1μL/mL. Staining was performed according to manufacturer’s instructions. Cells were resuspended to a final concentration of 0,25 × 106 cells/μL.
Zebrafish xenograft microinjection
Labelled cells were microinjected using borosilicate glass capillaries under a fluorescence scope (Zeiss Axio Zoom.V16) with a mechanical pneumatic injector attached (Pneumatic Pico pump PV820, World Precision Instruments). Cells were injected into the perivitelline space (PVS) of anesthetized 2dpf zebrafish embryos. After injection, embryos were maintained at 34°C until the end of the experiments in E3 medium. At 1 day post-injection (dpi), zebrafish xenografts were screened regarding the presence or absence of a tumoral mass. Xenografts with severe edema, cells in the yolk sac, cell debris or non-injected zebrafish embryos were discarded (22). At 3 or 4dpi, xenografts were sacrificed, fixed with 4% formaldehyde (Thermo Scientific) at 4°C overnight and preserved at -20°C in 100% methanol (VWR Chemicals). Percentage of tumor implantation was calculated as follows:
Xenografts irradiation and drug administration
At 1dpi zebrafish xenografts were randomly distributed into different experimental conditions: control (non-treated and non-irradiated), radiation alone (25Gy), 5FU alone (5FU), metformin alone (MET), radiation+5FU (25Gy+5FU) or radiation+metformin (25Gy+MET). Radiation consisted of a single high dose of 25Gy at 1dpi, as previously described (19). Irradiation procedures and regimens were adapted for zebrafish xenografts by the Champalimaud Foundation Radiation Oncology Department. The 6MV X-rays beams with the corresponding prescription dose (25Gy) were calculated with the same algorithm used in clinical practice (ECLIPSE, Varian Medical System, CA) and was delivered via a linear accelerator (Truebeam, Varian Medical Systems, CA). Irradiation was targeted to the center of a defined area of 30x30cm where the 6-well plates with the anesthetized zebrafish were placed. The well plates were positioned with a source-to-surface distance of 100cm. No build up material was needed. Also, at 1dpi, 5FU (4.2 mM) or metformin (5 mM) were administered in E3 during two or three successive days, depending on the experiment. Both solutions were freshly prepared daily. Metformin concentration was calculated after performing a maximum tolerated concentration (MTC) experiment using 0.05mM, 0.5mM, and 5mM of metformin in non-injected zebrafish embryos (data not shown). We observed a phenotype with 0.05mM in Hke3 cells (induction of cell death and decrease in tumor size). However, since no toxic effects were observed in all concentrations, we decided to use the highest to be sure that a lack of response (resistance) is not due to a problem of drug availability.
Xenografts whole-mount immunofluorescence
Primary antibodies: anti-activated caspase3 (rabbit, Cell Signaling, 1:100, code#9661), anti-human mitochondria (mouse, Merck Millipore, 1:100, cat#MAB1273). Secondary antibodies: Alexa goat anti-rabbit 488 (Molecular Probes, 1:400), anti-mouse 488 (Molecular Probes, 1:400), and anti-mouse 647 (Molecular Probes 1:400) were applied simultaneously with DAPI. Xenografts were mounted with Mowiol.
Xenografts imaging and quantification
Xenografts were acquired using a Zeiss LSM 710 fluorescence confocal microscope, with a 5μM interval z-stack. Images were analyzed using ImageJ software, using the Cell Counter plugin (23). To assess tumor size, three representative slices of each tumor, from the top (Zfirst), middle (Zmiddle), and bottom (Zlast) were analyzed and a proxy of total cell number of the entire tumor (DAPI nuclei) was estimated as follows:
The number of mitotic figures and activated caspase3 were quantified manually, counting all cells in every slice (from Zfirst to Zlast) and the respective percentages were generated by dividing the values by the tumor size (n° of tumor cells).
Immunohistochemistry
Formalin-fixed paraffin embedded (FFPE) tissue sections from each patient were used to evaluate the P53 status by immunohistochemistry with the anti-P53 monoclonal antibody (P53-DO7-L-CE, Leica, cat#PA0057), using the Leica Bond Max automated system (Leica Biosystems). A mutant phenotype was considered when a diffuse and intense nuclear staining was observed in the tumor cells (overexpression), whereas a wild-type phenotype consisted in spare and mild nuclear expression.
KRAS analysis
Mutations in KRAS (NM_004985.4) were determined with the IdyllaTM real-time PCR automatized system (Biocartis).
Statistical analysis
Statistical analysis was performed using GraphPad Prism 8.0 software. All datasets were challenged by normality tests (D’Agostino and Pearson and the Shapiro–Wilk). A Gaussian distribution was only assumed for datasets that pass both normality tests and were analyzed by an unpaired t-test with Welch’s correction. Datasets without Gaussian distribution were analyzed by unpaired and nonparametric Man-Whitney test. For all the statistical analysis, p value is from a two-tailed test with a confidence interval of 95%. Statistical differences were considered significant whenever p< 0.05 and statistical output was represented by stars as follows: non-significant (ns) > 0.05, *p ≤ 0.05, **p ≤ 0.01, ***p ≤ 0.001 and ****p ≤ 0.0001. All graphs presented the results as average (AVG) ± standard error of the mean (SEM).
Results
Metformin has a similar radiosensitive effect to 5FU
In our previous work we developed a single dose radiotherapy protocol of 25Gy to assess radiosensitivity in vivo in just 4 days (19). Here, in order to evaluate whether the zebrafish xenograft model is able to reveal the radiosensitizing effect of metformin (MET), we chose two isogenic CRC that we previously characterized: radiosensitive HCT116 and radioresistant Hke3 cells (19). These two cells lines differ in their KRAS status: HCT116 harbor a KRASG13D mutation whereas the isogenic Hke3 cells are KRASWT (24).
CRC tumor cells were fluorescently labeled and injected into the periviteline space (PVS) of 2 days post fertilization (dpf) zebrafish embryos. To study in vivo the outcomes of radiation combined with metformin and its comparison to 5FU, 6 conditions were tested: control, 5FU, MET, 25Gy, 25Gy+5FU and 25Gy+MET (Figure 1). At 1 day post-injection (1dpi) injected embryos were submitted to 25Gy in a single radiation session. According to the condition, this procedure was immediately followed by the addition of 5FU or metformin in E3 medium for three consecutive days, replaced daily. Single 5FU and MET treatments were also delivered for three consecutive days starting at 1dpi. Control refers to non-irradiated and non-treated xenografts. At 4dpi xenografts were processed for immunofluorescence and confocal imaging. The impact of treatment was analyzed by quantifying proliferation (mitotic figures), induction of apoptosis (activated caspase3) and tumor size (number of tumor cells) (Figure 1).
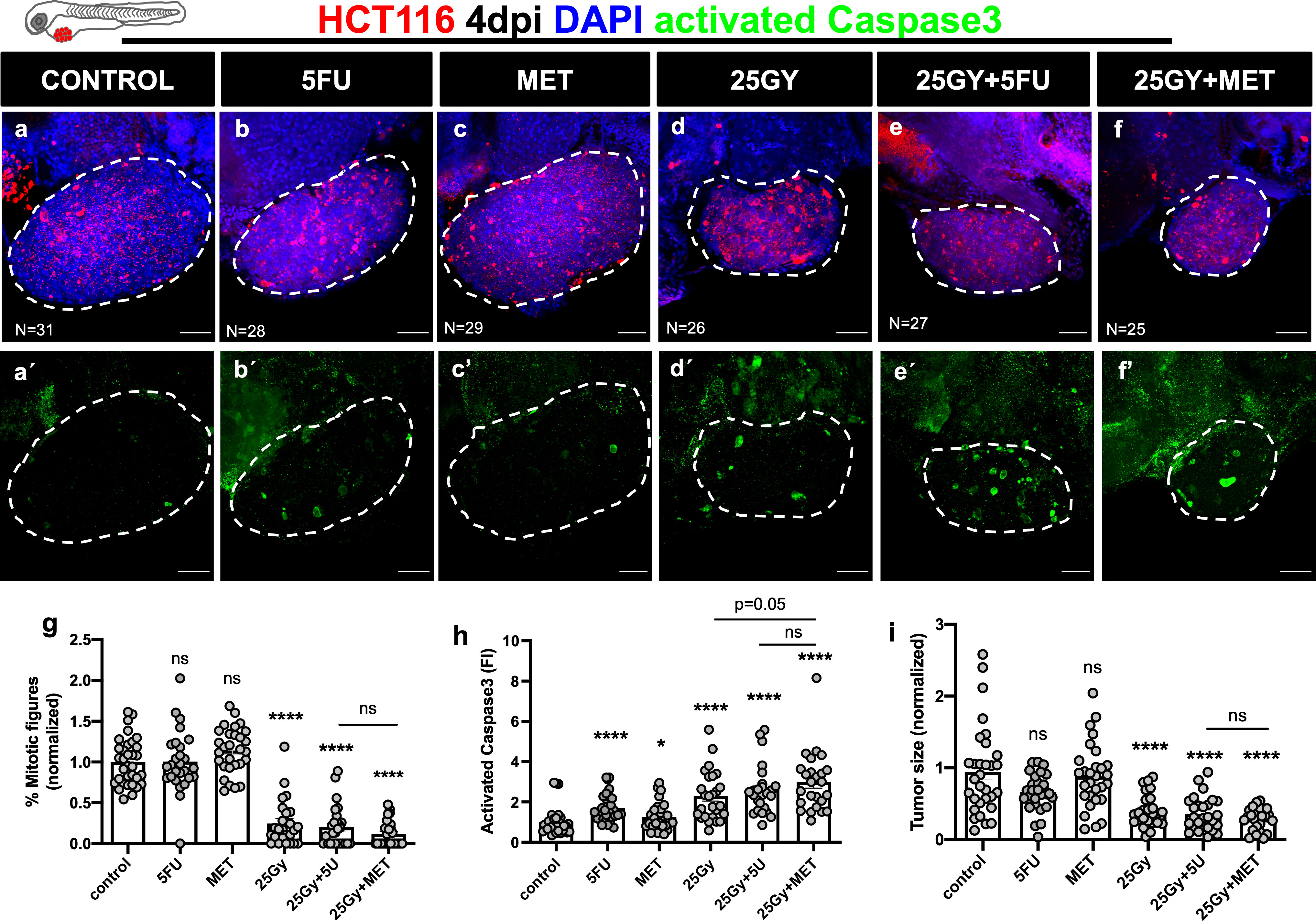
Figure 1 Human CRC cells HCT116 were injected into the PVS of 2dpf zebrafish embryos. At 1dpi xenografts were submitted to 5FU chemotherapy (B), metformin (C), single radiation dose of 25Gy (D) or combinations of 25Gy+5FU (E) or 25Gy+MET (F). HCT116 xenografts were treated for 3 consecutive days and compared to non-irradiated and non-treated controls (A). Maximum Z projections of activated Caspase3 are shown in green (A’–F’). At 4dpi, cell proliferation (% mitotic figures), apoptosis (% activated Caspase3, green), and tumor size (number of tumor cells, DAPI, blue) were analyzed and quantified (G–I respectively). Results are the average of 2 independent experiments and are expressed as mean ± SEM. Each dot represents a xenograft and the total number of xenografts analyzed is indicated in the images (A–F). Dashed white line is delimitating the tumor of each xenograft. Scale bars represent 50µm. Statistical results: (ns) > 0.05, *P≦0.05, ****P≦0.0001. Results were compared with control with exception of those that have a bar to indicate the compared groups.
In HCT116 xenografts we could not detect any significant difference in proliferation with 5FU treatment (p=0.9412), or MET alone (1.14 fold change, p=0.066) (Figure 1G). In contrast, RT alone or in combination with either 5FU or MET strongly impaired proliferation (75%, 80% and 88% reduction respectively, p<0.0001). However, no difference was observed between 25Gy+5FU vs 25Gy+MET (p=0.2295) (Figure 1G). In terms of cell death by apoptosis, we observed a clear effect of 25Gy+MET, with a ~3 fold increase in activated caspase3 (p<0.0001), similar to the effect of 5FU (fold increase ~2.60, p<0.0001). Again, we could not detect significant differences between 25Gy+5FU and 25Gy+MET (p=0.3255) conditions (Figure 1H).
Regarding tumor size, 5FU or MET single treatments did not led to a significant tumor shrinkage (fold decrease 0.66, p=0.1357 and 0.88, p=0.9298, respectively) (Figures 1B, C, I). In contrast, RT alone or in combination with 5FU or MET led to a strong and similar reduction in tumor size (59%, 64% and 72% shrinkage, respectively p<0.0001 for all) (Figures 1E, F, I). The difference between 25Gy+5FU and 25Gy+MET was not significant (p=0.4027) (Figure 1I).
In summary, our results show that MET has a similar radiosensitization effect as 5FU in HCT116 zebrafish xenografts.
Metformin is able to sensitize Hke3 xenografts to radiotherapy
Next, we generated radioresistant Hke3 xenografts to test whether MET could radiosensitize these refractory cells (25). Six conditions were tested as before: control, 5FU, MET, 25Gy, 25Gy+5FU and 25Gy+MET (Figures 2A-F). As expected, RT or its combination with 5FU had no significant effect in inducing apoptosis or tumor shrinkage (Figures 2D, E, G–I). Strikingly, combination of 25Gy+MET induced a significant induction of apoptosis (1.27 fold induction, p=0.0139) and reduction of tumor size (40% reduction of tumor size, p=0.030). Importantly, the difference between 25Gy and 25Gy+MET was significant, demonstrating its sensitizer effect over radiation (p=0.031) (Figure 2H). Regarding proliferation, combination of RT with MET had a clear synergistic effect and almost completely abolished the proliferation capacity of these cells (86% reduction, p<0.0001) (Figure 2G and Supplementary Figure S1).
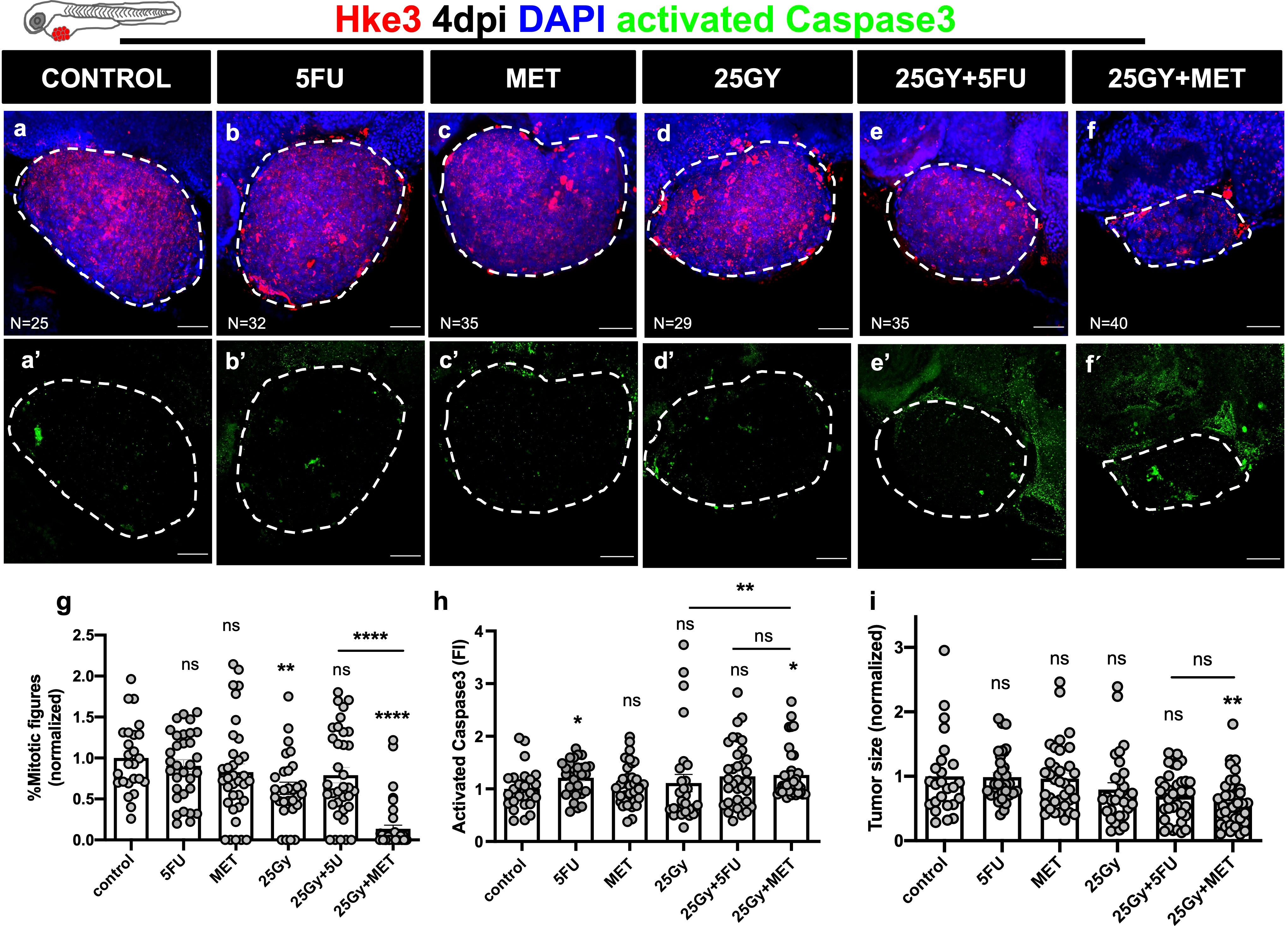
Figure 2 Human CRC cells Hke3 were injected into the PVS of 2dpf zebrafish embryos. At 1dpi xenografts were submitted to 5FU chemotherapy (B), metformin (C), single radiation dose of 25Gy (D) or combinations of 25Gy+5FU (E) or 25Gy+MET (F). HKe3 xenografts were treated for 3 consecutive days and compared to non-irradiated and non-treated controls (A). Maximum Z projections of activated Caspase3 are shown in green (A’–F’). At 4dpi, cell proliferation (% mitotic figures), apoptosis (% activated Caspase3, green), and tumor size (number of tumor cells, DAPI, blue) were analyzed and quantified (G–I respectively). Results are the average of 2 independent experiments and are expressed as mean ± SEM. Each dot represents a xenograft and the total number of xenografts analyzed is indicated in the images (A–F). Dashed white line is delimitating the tumor of each xenograft. Scale bars represent 50µm. Statistical results: (ns) > 0.05, *P≦0.05, ** P≦0.01, ****P≦0.0001. Results were compared with control with exception of those that have a bar to indicate the compared groups.
Although we observed a significant reduction in tumor size and proliferation with 25Gy+MET treatment, the impact on apoptosis induction was quite mild. This was puzzling since apoptosis represents one of the major types of cell death induced by ionizing radiation (26) and, therefore, is a very good surrogate for radiosensitivity. Also, in our previous work we showed that apoptosis is our main readout to define sensitivity/resistance to chemo and radiotherapy (18, 19). Therefore, we wondered whether the peak of apoptosis had occurred before. To investigate this, we repeated the same experiment but analyzed the xenografts at 2 timepoints: 3dpi (2 days of treatment) and 4dpi (3 days of treatment). Indeed, we were able to observe an earlier peak of apoptosis at 3dpi (from an AVG of 2.2% in controls to 4.7% in 25Gy+MET, corresponding to 2.13 fold induction of apoptosis, p<0.0001) (Supplementary Figure S2B). At this timepoint there is a remarkable sensitizer effect of 25Gy+MET over radiation alone in terms of cell death (p<0.0001) and a strong reduction of tumor size (p=0.016), when comparing these two conditions (Supplementary Figures S2B, C). Our results with Hke3 show that, indeed, MET can sensitize even radioresistant tumor cells, and in this case more efficiently than 5FU.
zAvatars show different responses to radiation combined with metformin
Next, we used surgical resected rectal cancer samples (Figure 3) and rectum diagnostic biopsies obtained through endoscopy before neoadjuvant treatment (Figure 4 and Supplementary Figures S5, 6). Both were used without in vitro expansion to generate zebrafish patient derived xenografts (zPDX or zAvatars) (Table 1). We first used surgical resected rectal cancer samples to optimize and test the feasibility of our protocol, and only after, we proceeded to the biopsy samples, which due to the small amount of tissue becomes a more challenging technique. Since we do not amplify the tumor samples to reduce caveats of in vitro selection and time, which is crucial for decision making, the restrict amount of patient tissue is the major limitation of our assay. This is why it was not possible to test 5FU and MET alone. Nevertheless, it is in this setting – diagnostic biopsies – that the neoadjuvant options of treatment need to be tested prior to treatment for a future personalized management of the disease.
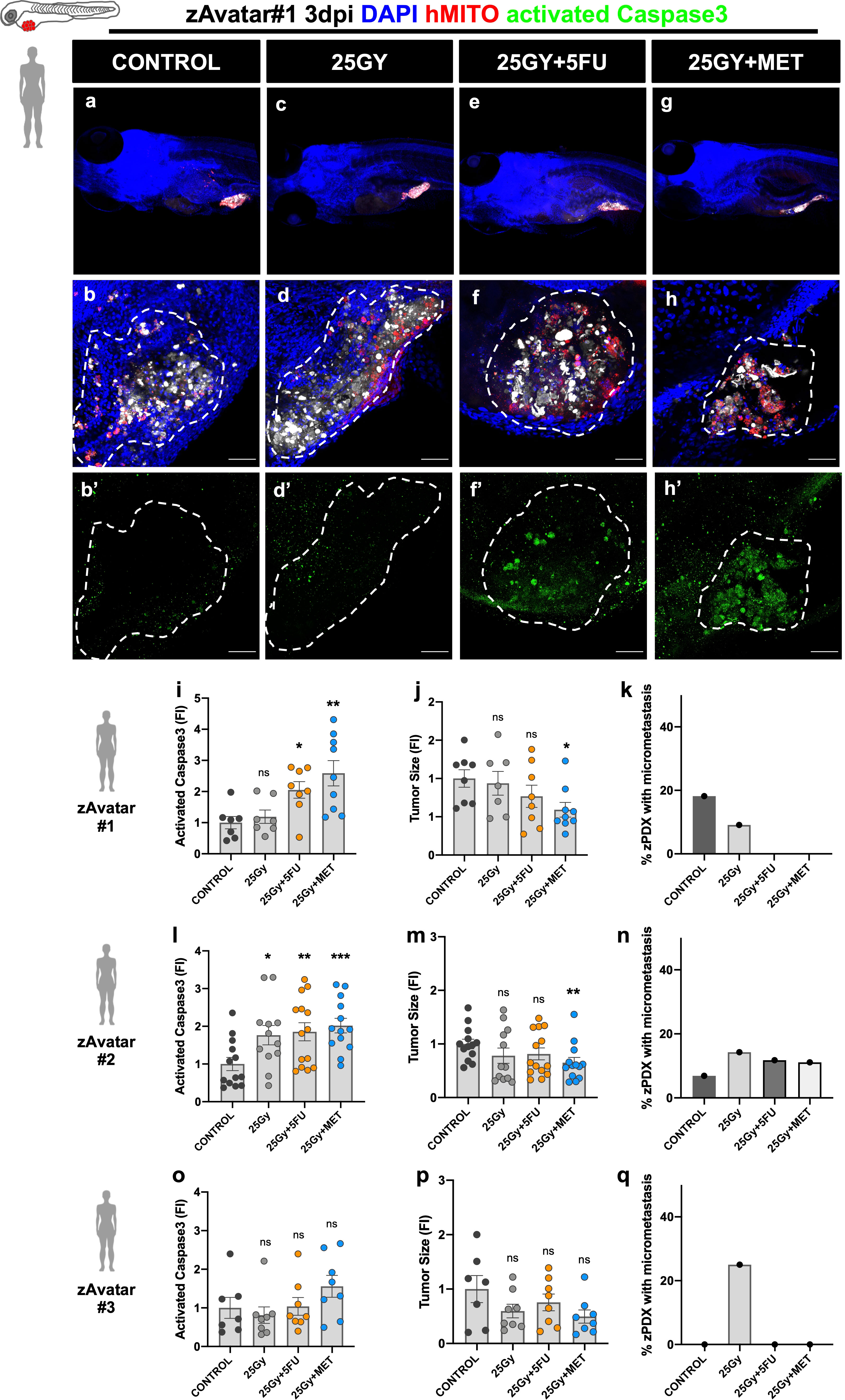
Figure 3 zAvatars were generated from rectum cancer surgical resected samples. One day after injection zAvatars were submitted to 25Gy radiation dose (C, D, D’), 25Gy+5FU (E, F, F’) or 25Gy+MET (G, H, H’) regimens, and compared with control (A, B, B’) At 3dpi, apoptosis (I, L, O), tumor size (J, M, P) and metastatic potential (K, N, Q) were analyzed. Tumor cells are labeled in white (cell tracker Deep Red), activated Caspase3 in green (B’–H’), DAPI in blue and human-mitochondria marker in red. Images correspond to zAvatar#1, and quantifications are shown for zAvatar#1, #2 and #3. Data is expressed as mean ± SEM and each dot represents a xenograft. Dashed white line is delimitating the tumor of each xenograft. Scale bars represent 50µm. Statistical results: (ns) > 0.05, *P≦0.05, **P≦0.01, ***P≦0.001. Results were compared with control with exception of those that have a bar to indicate the compared groups.
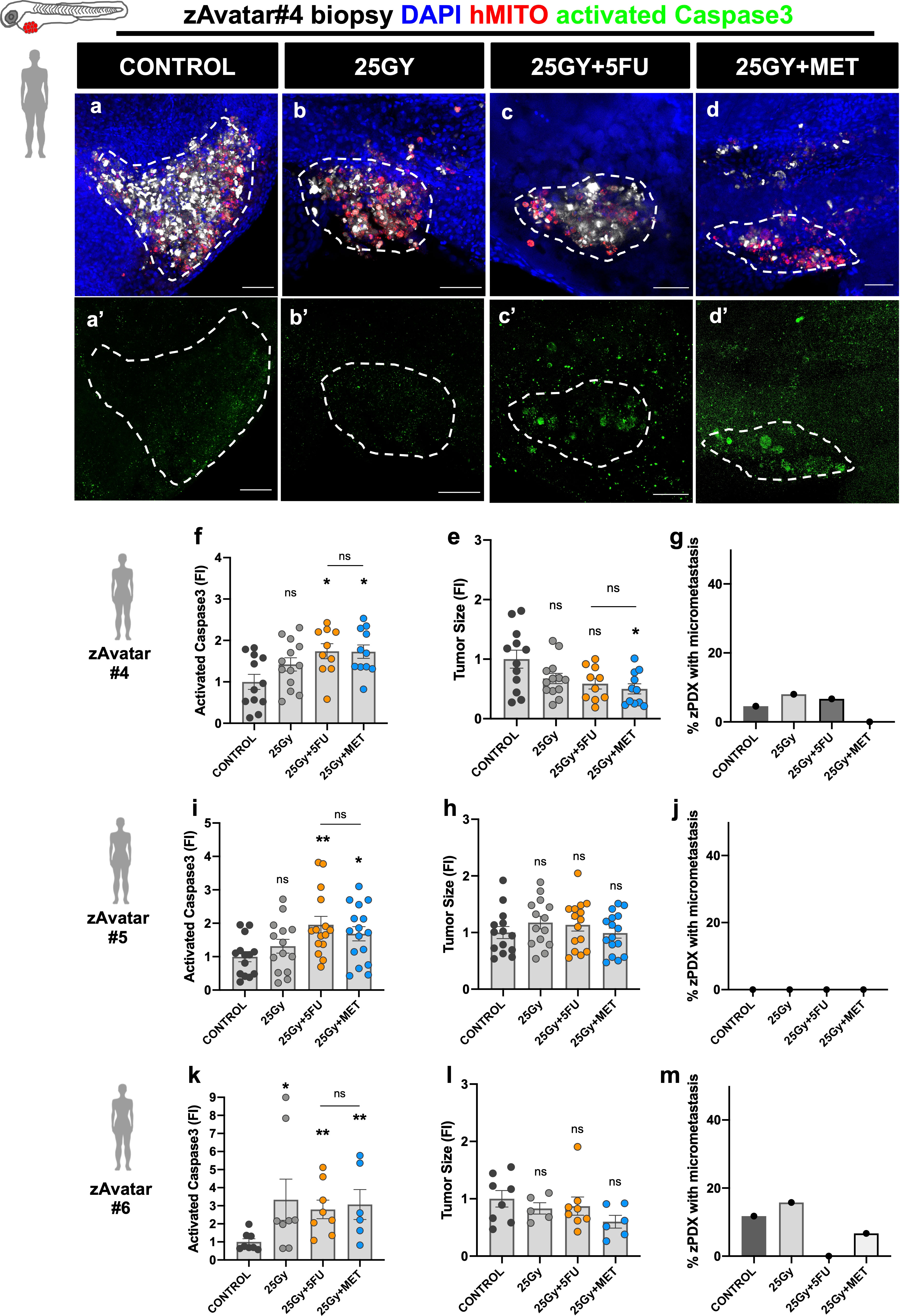
Figure 4 zAvatars were generated from naive rectal cancer biopsies. One day after injection zAvatars were submitted to 25Gy radiation dose (B, B’), 25Gy+5FU (C, C’) or 25Gy+MET (D, D’) regimens, and compared with control (A, A’) At 3dpi, apoptosis (F, I, K), tumor size (E, H, L) and metastatic potential (G, J, M) were analyzed. Tumor cells are labeled in white (Deep Red), activated Caspase3 in green (A’–D’), DAPI in blue and human-mitochondria marker in red. Images correspond to zAvatar#4, and quantifications are shown for zAvatar#4, #5 and #6. Data is expressed as mean ± SEM and each dot represents a xenograft. Dashed white line is delimitating the tumor of each xenograft. Scale bars represent 50µm. Statistical results: (ns) > 0.05, *P≦0.05, **P≦0.01. Results were compared with control with exception of those that have a bar to indicate the compared groups.
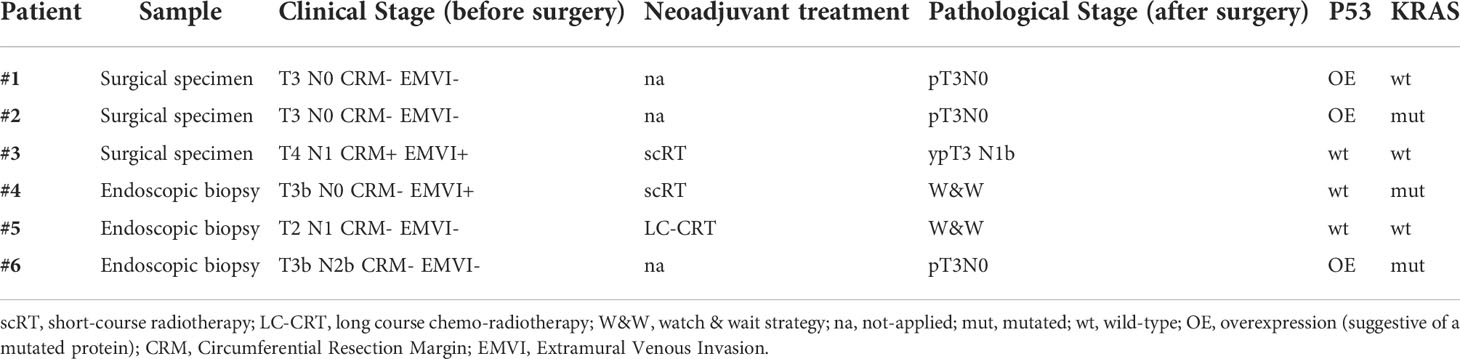
Table 1 Histopathological characterization of samples from rectal cancer patients included in the study.
Patient samples ranged from T2 to T4 tumor clinical stages. Analysis of the KRAS and P53 revealed a diversity of combinations of KRAS and P53 status (mutated/wild-type) (see Table 1 and Supplementary Figure S7). Samples were prepared for injection (see material and methods) and zAvatars were subjected to 4 different conditions at 1dpi, as previously described, and treated for 2 consecutive days. The percentages of implantation for all zAvatars are shown in Supplementary Figure S3.
Figure 3 shows results obtained with surgical resected rectal cancer samples from 3 patients (#1, #2, and #3). Two of the surgical samples were naïve (#1, #2) but one had been previously treated with scRT before surgery (#3). Representative images are shown for zAvatar#1 (Figures 3A–H), including zoom out images (Figures 3A–G), and representative images of zAvatar #2, and #3 are shown in Supplementary Figure S5. Figure 4 refers to zAvatars generated from rectal cancer biopsies obtained by endoscopy (#4, #5, and #6). All the endoscopic diagnostic biopsies were naïve. After clinical evaluation, two of the patients underwent radiotherapy treatments, scRT (#4) and LC-CRT (#5), whereas the third patient went directly to surgery (#6). Representative images are shown for zAvatar#4 (Figures 4A–D) and zAvatar#5 and zAvatar#6 are shown in Supplementary Figure S6. We analyzed induction of apoptosis and tumor shrinkage upon treatment, as well as the incidence of micrometastasis (percentage of zAvatars that presented micrometastasis in the tail, eye or gills; Figure 3 and Supplementary Figure S4).
Overall, taking into account the induction of apoptosis (Figures 3I, L, O, 4F, I, K), our results show that MET was able to sensitize tumor cells to RT to a similar extent as 5FU (25Gy+5FU and 25Gy+MET), with exception of one patient whose tumor cells appeared to be resistant (Patient#3). Of note, this patient presented a more advanced clinical stage and underwent radiotherapy before surgery, suggesting that we may have received radiation resistant clones, supporting our results that show resistance to radiotherapy (Figure 3O).
The impact of treatments on tumor size was not so evident (Figures 3J, M, P, 4E, H, L), but this is not surprising given the short time assay and our previous work showing that induction of apoptosis is the readout that best correlates with patient’s clinical response (18, 19).
Regarding the impact on the metastatic potential, we could not find a trend, but in some patients 25Gy+MET was able to impair the metastatic spread of the tumor cells (zAvatar#1, #4 and #6). Also, zAvatars#1 exhibited ~20% of micrometastasis in control, which may constitute a “red alert” for the respective patient, requiring a more thorough follow up (Figure 3Q). Interestingly, the P53 status seems to correlate with a higher basal metastatic potential in control conditions (zAvatar#1, #2 and #6, without treatment).
Altogether, our results with zAvatars show that responses to treatment are heterogeneous and highly dependent on each patient’s tumor cells, not correlating with either the P53 or the KRAS status or their combination, in our small sample. Importantly, radiation combined with metformin was never inferior to the classical combination of radiation with 5FU, confirming the promising power of this less toxic approach for patients with rectal cancer.
Discussion
Neoadjuvant chemoradiation is part of the standard of care for selected patients with rectal cancer, leading to significant tumor regression and sometimes achieving complete tumor responses. Increase of radiation therapy doses and/or the addition of more cycles of chemotherapy have been associated with higher tumor response rates. However, these strategies are associated with higher grades of toxicity (11, 12). Therefore, alternative regimens that improve tumor response rates without increasing treatment related morbidity are needed. In this context, the use of a well-tolerated radiosensitizing drug could be a perfect solution.
Metformin (MET) is a first line medication for the treatment of type II diabetes, presenting a well tolerable and a very low toxicity profile (27). Epidemiologic findings have demonstrated that metformin can reduce the risk of cancer in patients with type 2 diabetes (28–30). Moreover, a recent meta-analysis demonstrated the potential synergistic anti-tumor effects of metformin and radiotherapy on treatment of patients with cancer and type 2 diabetes (30). Specifically, in rectal cancer, a retrospective study showed an association of metformin use and improved tumor response to neoadjuvant chemoradiation (14). Furthermore, diabetic patients actively treated with metformin and affected with locally advanced rectal cancer (LARC) present higher rates of downstaging and pathological complete response (pCR) after CRT compared to those patients with LARC not taking metformin (31). In addition, it has been shown that metformin enhances tumor response of radiation therapy in several preclinical animal models using cell lines of lung, prostate and colon cancers (32–34). However, to our knowledge no studies have been performed with rectal patient samples.
Moreover, some other retrospective studies describe contradictory findings, where metformin were not protective or conferred any benefit in CRC (35–37). In most of these studies the dose and duration of metformin treatment were not known, probably contributing to the heterogeneity of the results (28, 30).
Therefore, pre-clinical studies using patient tumor samples and a randomized clinical trial where tumor stages, doses and treatment duration are controlled are essential to clarify the therapeutic effectiveness of metformin for the treatment of CRC (38).
In this work, we addressed the first issue – the use of patient tumor samples, without in vitro expansion. We tested whether we could detect the radiosensitizing effect of MET in the zebrafish xenograft model using patient samples xenografts (zPDX or zAvatars) and compare it with the standard 5FU. We first used two isogenic CRC cell lines (18, 24): HCT116 tumor cells harbor a KRASG13D mutation, rendering them highly proliferative and radiosensitive, and the isogenic KRASWT Hke3, that is less proliferative and is radioresistant (19). Our results show that MET has a similar radiosensitizer effect as 5FU in HCT116 xenografts. A recent publication showed similar effects in tumor growth impairment between RT/Metformin and RT/5FU, using a mouse model injected with SW480 CRC cell line (15). Interestingly, in Hke3 xenografts, MET can sensitize tumor cells to RT more efficiently than 5FU, constituting the only effective treatment for these radioresistant cells. Although the impact of KRAS mutations on radiosensitivity and MET is not clear, it has been shown that KRAS can influence and rewire the metabolic state of cancer cells and, therefore, could contribute to the different sensitivities to MET (39).
Next, we generated zAvatars from rectal tumor resected samples and diagnostic biopsies to test whether we could observe the radiosensitive effects of MET in patient samples. Our experiments with zAvatars demonstrate that response to the treatments tested are diverse and dependent of each patient’s tumor cells, but in general MET was able to sensitize tumor cells to RT to a similar extent as 5FU. Thus, the same radiosensitive effect could be achieved without chemotherapy-related toxicity. Nevertheless, there was one case where the tumor was resistant to all treatments, which corresponded to a surgical sample of a patient that was previously treated with scRT.
Patient samples used had different tumor stages (T2 to T4) and a variety of P53 and KRAS status. However, we could not detect any correlation between the P53 or the KRAS status or their combination with MET sensitivity, in our small sample. This suggests, like in most therapies, that there must be many more mutations and combinations (that we did not access) that contribute to the overall sensitivity. Genetic diversity influences multiple tumor phenotypes, like activation of signaling pathways, migration, metastization, senescence, metabolism, and ultimately impact on the sensitivity to treatment (16, 40). An extreme example shows that identical CRC cells, sharing the same genome, may have distinctive sensitivities to different therapies; indicating that besides the genetic heterogeneity, other factors like the environment/metabolism and epigenetics impact on therapy response (16, 41). This highlights the need for a functional test where tumor cells are directly challenged with therapies to access their sensitivity.
The exact mechanism of metformin anticancer action is not fully understood. There is substantial evidence indicating that metformin activates AMP-activated protein kinase (AMPK), inhibits mTOR-dependent translation initiation and affects cancer cell metabolism (42, 43). Also, when combined with radiation the mechanism of action has not been elucidated. Metformin cellular target is mitochondrial complex I, causing a severe depletion in ATP, increasing the production of reactive oxygen species and decreasing glutathione levels, exacerbating DNA damage (27, 30, 44). Other studies have suggested that MET can also reduce the expression levels of DNA repair enzymes and, consequently, impair DNA repair (45). In addition, MET is a potent modulator of glucose metabolism and potentially impacts on cancer progression (46). Although we did not address the mechanism of action of MET, the zebrafish xenograft model could be used in the future to test several hypotheses, for instance by adding high glucose concentrations (47) to the E3 medium or by treating xenografts with compound C, a AMPK inhibitor (48).
In conclusion, our work shows a powerful radiosensitive effect of MET in vivo using rectal cancer patient samples and, therefore, confirms that MET is a promising alternative to the standard 5FU protocol, which can be crucial for frail/elderly patients. Furthermore, zAvatars could be used to predict individual response prior to neoadjuvant therapy to tailor treatment in a more personalized manner, avoiding unnecessary toxicities.
Data availability statement
The raw data supporting the conclusions of this article will be made available by the authors, without undue reservation.
Ethics statement
The studies involving human participants were reviewed and approved by Ethics Committee of the Champalimaud Foundation. Rectal cancer patient samples were provided by Champalimaud Clinical Center’s (CCC) Digestive Unit. The patients/participants provided their written informed consent to participate in this study. The animal study was reviewed and approved by Ethical Committee of Champalimaud Foundation and Portuguese institutional organizations: ORBEA (Animal Welfare and Ethics Body) and DGAV (Directorate General for Food and Veterinary).
Author contributions
RF, BC and LF conceptualized the research. RF supervised the research. BC performed research. LF, OP and IS participated in selecting and analyzing patient data. RR-T, MC-M and AP provided patient tumor samples. BC, LF and RF wrote the paper. RF provided funding. All authors contributed with the critical reading of the manuscript. All authors have read and agreed to the published version of the manuscript.
Funding
We are grateful to Champalimaud Foundation, Congento (LISBOA-01-0145-FEDER-022170, co-financed by FCT/Lisboa2020) and FCT-PTDC/MEC-ONC/31627/2017 for funding.
Acknowledgments
We would like to thank the patients who participated in the study, the Champalimaud Digestive Unit (Ignacio Herrando, Pedro Vieira, José Azevedo, Hugo Domingos), the Champalimaud Radiation Unit (Carlo Greco, Sandra Vieira, Joep Stroom, Maria João Cardoso, Ruben Coutinho), the Champalimaud Foundation Biobank (CFB) and the Champalimaud Fish Platform.
Conflict of interest
The authors declare that the research was conducted in the absence of any commercial or financial relationships that could be construed as a potential conflict of interest.
Publisher’s note
All claims expressed in this article are solely those of the authors and do not necessarily represent those of their affiliated organizations, or those of the publisher, the editors and the reviewers. Any product that may be evaluated in this article, or claim that may be made by its manufacturer, is not guaranteed or endorsed by the publisher.
Supplementary material
The Supplementary Material for this article can be found online at: https://www.frontiersin.org/articles/10.3389/fonc.2022.862889/full#supplementary-material
Abbreviations
5FU, 5-Fluouracil; AMPK, AMP-activated protein kinase; CRC, Colorectal cancer; dpf, days post fertilization; LARC, Locally advanced rectal cancer; MET, Metformin; mTOR, Mammalian target of rapamycin; nCRT, Neoadjuvant chemoradiation; mut, mutated; pCR, Pathological complete response; PVS, Periviteline space; RT, Radiotherapy; TME, Total mesorectal excision; wt, wild-type; zAvatar, Zebrafish xenograft model.
References
1. Glynne-Jones R, Wyrwicz L, Tiret E, Brown G, Rödel C, Cervantes A, et al. Rectal cancer: ESMO clinical practice guidelines for diagnosis, treatment and follow-up. Ann Oncol Off J Eur Soc Med Oncol (2018) 29(Suppl 4):iv263. doi: 10.1093/annonc/mdy161
2. Sauer R, Becker H, Hohenberger W, Rödel C, Wittekind C, Fietkau R, et al. Preoperative versus postoperative chemoradiotherapy for rectal cancer. New Engl J Med (2004) 351(17):1731–40. doi: 10.1056/NEJMoa040694
3. Bosset J-F, Calais G, Mineur L, Maingon P, Radosevic-Jelic L, Daban A, et al. Enhanced tumorocidal effect of chemotherapy with preoperative radiotherapy for rectal cancer: Preliminary results–EORTC 22921. J Clin Oncol (2005) 23(24):5620–7. doi: 10.1200/JCO.2005.02.113
4. Maas M, Nelemans PJ, Valentini V, Das P, Rödel C, Kuo LJ, et al. Long-term outcome in patients with a pathological complete response after chemoradiation for rectal cancer: A pooled analysis of individual patient data. Lancet Oncol (2010) 11(9):835–44. doi: 10.1016/S1470-2045(10)70172-8
5. Dattani M, Heald RJ, Goussous G, Broadhurst J, São Julião GP, Habr-Gama A, et al. Oncological and survival outcomes in watch and wait patients with a clinical complete response after neoadjuvant chemoradiotherapy for rectal cancer: A systematic review and pooled analysis. Ann Surg (2018) 268(6):955–67. doi: 10.1097/SLA.0000000000002761
6. Habr-Gama A, Perez RO, Nadalin W, Sabbaga J, Ribeiro U Jr., Silva e Sousa AH Jr., et al. Operative versus nonoperative treatment for stage 0 distal rectal cancer following chemoradiation therapy: Long-term results. Ann surgery. (2004) 240(4):711–7. doi: 10.1097/01.sla.0000141194.27992.32
7. van der Valk MJM, Hilling DE, Bastiaannet E, Meershoek-Klein Kranenbarg E, Beets GL, Figueiredo NL, et al. Long-term outcomes of clinical complete responders after neoadjuvant treatment for rectal cancer in the international watch & wait database (IWWD): An international multicentre registry study. Lancet (London England) (2018) 391(10139):2537–45. doi: 10.1016/S0140-6736(18)31078-X
8. Appelt AL, Pløen J, Vogelius IR, Bentzen SM, Jakobsen A. Radiation dose-response model for locally advanced rectal cancer after preoperative chemoradiation therapy. Int J Radiat oncology biology Phys (2013) 85(1):74–80. doi: 10.1016/j.ijrobp.2012.05.017
9. Garcia-Aguilar J, Renfro LA, Chow OS, Shi Q, Carrero XW, Lynn PB, et al. Organ preservation for clinical T2N0 distal rectal cancer using neoadjuvant chemoradiotherapy and local excision (ACOSOG Z6041): Results of an open-label, single-arm, multi-institutional, phase 2 trial. Lancet Oncol (2015) 16(15):1537–46. doi: 10.1016/S1470-2045(15)00215-6
10. Habr-Gama A, São Julião GP, Vailati BB, Sabbaga J, Aguilar PB, Fernandez LM, et al. Organ preservation in cT2N0 rectal cancer after neoadjuvant chemoradiation therapy: The impact of radiation therapy dose-escalation and consolidation chemotherapy. Ann Surg (2019) 269(1):102–7. doi: 10.1097/SLA.0000000000002447
11. Ansari N, Solomon MJ, Fisher RJ, Mackay J, Burmeister B, Ackland S, et al. Acute adverse events and postoperative complications in a randomized trial of preoperative short-course radiotherapy versus long-course chemoradiotherapy for T3 adenocarcinoma of the rectum: Trans-Tasman radiation oncology group trial (TROG 01. 04). Ann Surg (2017) 265(5):882–8. doi: 10.1097/SLA.0000000000001987
12. Bujko K, Nowacki MP, Nasierowska-Guttmejer A, Michalski W, Bebenek M, Kryj M. Long-term results of a randomized trial comparing preoperative short-course radiotherapy with preoperative conventionally fractionated chemoradiation for rectal cancer. Br J Surg (2006) 93(10):1215–23. doi: 10.1002/bjs.5506
13. Kim JM, Park JW, Lee JH, Park YH, Park SJ, Cheon JH, et al. Survival benefit for metformin through better tumor response by neoadjuvant concurrent chemoradiotherapy in rectal cancer. Dis colon rectum (2020) 63(6):758–68. doi: 10.1097/DCR.0000000000001624
14. Skinner HD, Crane CH, Garrett CR, Eng C, Chang GJ, Skibber JM, et al. Metformin use and improved response to therapy in rectal cancer. Cancer Med (2013) 2(1):99–107. doi: 10.1002/cam4.54
15. Fernandes JM, Jandrey EHF, Koyama FC, Leite KRM, Camargo AA, Costa ÉT, et al. Metformin as an alternative radiosensitizing agent to 5-fluorouracil during neoadjuvant treatment for rectal cancer. Dis colon rectum (2020) 63(7):918–26. doi: 10.1097/DCR.0000000000001626
16. Costa B, Estrada MF, Mendes RV, Fior R. Zebrafish avatars towards personalized medicine–a comparative review between avatar models. Cells (2020) 9(2):293. doi: 10.3390/cells9020293
17. Fazio M, Ablain J, Chuan Y, Langenau DM, Zon LI. Zebrafish patient avatars in cancer biology and precision cancer therapy. Nat Rev Cancer (2020) 20(5):263–73. doi: 10.1038/s41568-020-0252-3
18. Fior R, Póvoa V, Mendes RV, Carvalho T, Gomes A, Figueiredo N, et al. Single-cell functional and chemosensitive profiling of combinatorial colorectal therapy in zebrafish xenografts. Proc Natl Acad Sci (2017) 114(39):E8234–E43. doi: 10.1073/pnas.1618389114
19. Costa B, Ferreira S, Póvoa V, Cardoso MJ, Vieira S, Stroom J, et al. Developments in zebrafish avatars as radiotherapy sensitivity reporters - towards personalized medicine. EBioMedicine (2020) 51:102578. doi: 10.1016/j.ebiom.2019.11.039
20. White RM, Sessa A, Burke C, Bowman T, LeBlanc J, Ceol C, et al. Transparent adult zebrafish as a tool for in vivo transplantation analysis. Cell Stem Cell (2008) 2(2):183–9. doi: 10.1016/j.stem.2007.11.002
21. Lawson ND, Weinstein BM. In vivo imaging of embryonic vascular development using transgenic zebrafish. Dev Biol (2002) 248(2):307–18. doi: 10.1006/dbio.2002.0711
22. Martinez-Lopez M, Póvoa V, Fior R. Generation of Zebrafish Larval Xenografts and Tumor Behavior Analysis. J. Vis. Exp. (2021) (172):e62373. doi: 10.3791/62373
23. Schindelin J, Arganda-Carreras I, Frise E, Kaynig V, Longair M, Pietzsch T, et al. Fiji: An open-source platform for biological-image analysis. Nat Methods (2012) 9(7):676–82. doi: 10.1038/nmeth.2019
24. Shirasawa S, Furuse M, Yokoyama N, Sasazuki T. Altered growth of human colon cancer cell lines disrupted at activated ki-ras. Sci (New York NY) (1993) 260(5104):85–8. doi: 10.1126/science.8465203
25. Charitou T, Srihari S, Lynn MA, Jarboui M-A, Fasterius E, Moldovan M, et al. Transcriptional and metabolic rewiring of colorectal cancer cells expressing the oncogenic KRASG13D mutation. Br J Cancer (2019) 121(1):37–50. doi: 10.1038/s41416-019-0477-7
26. Baskar R, Dai J, Wenlong N, Yeo R, Yeoh K-W. Biological response of cancer cells to radiation treatment. Front Mol Biosci (2014) 1(24). doi: 10.3389/fmolb.2014.00024
27. Li M, Li X, Zhang H, Lu Y. Molecular mechanisms of metformin for diabetes and cancer treatment. Front Physiol (2018) 9:1039. doi: 10.3389/fphys.2018.01039
28. Chevalier B, Pasquier D, Lartigau EF, Chargari C, Schernberg A, Jannin A, et al. Metformin: (future) best friend of the radiation oncologist? Radiotherapy Oncol J Eur Soc Ther Radiol Oncol (2020) 151:95–105. doi: 10.1016/j.radonc.2020.06.030
29. Gandini S, Puntoni M, Heckman-Stoddard BM, Dunn BK, Ford L, DeCensi A, et al. Metformin and cancer risk and mortality: A systematic review and meta-analysis taking into account biases and confounders. Cancer Prev Res (Philadelphia Pa) (2014) 7(9):867–85. doi: 10.1158/1940-6207.CAPR-13-0424
30. Rao M, Gao C, Guo M, Law BYK, Xu Y. Effects of metformin treatment on radiotherapy efficacy in patients with cancer and diabetes: A systematic review and meta-analysis. Cancer Manage Res (2018) 10:4881–90. doi: 10.2147/CMAR.S174535
31. Oh BY, Park YA, Huh JW, Cho YB, Yun SH, Lee WY, et al. Metformin enhances the response to radiotherapy in diabetic patients with rectal cancer. J Cancer Res Clin Oncol (2016) 142(6):1377–85. doi: 10.1007/s00432-016-2148-x
32. Storozhuk Y, Hopmans SN, Sanli T, Barron C, Tsiani E, Cutz JC, et al. Metformin inhibits growth and enhances radiation response of non-small cell lung cancer (NSCLC) through ATM and AMPK. Br J Cancer (2013) 108(10):2021–32. doi: 10.1038/bjc.2013.187
33. Zannella VE, Dal Pra A, Muaddi H, McKee TD, Stapleton S, Sykes J, et al. Reprogramming metabolism with metformin improves tumor oxygenation and radiotherapy response. Clin Cancer Res an Off J Am Assoc Cancer Res (2013) 19(24):6741–50. doi: 10.1158/1078-0432.CCR-13-1787
34. Zhang T, Zhang L, Zhang T, Fan J, Wu K, Guan Z, et al. Metformin sensitizes prostate cancer cells to radiation through EGFR/p-DNA-PKCS in vitro and in vivo. Radiat Res (2014) 181(6):641–9. doi: 10.1667/RR13561.1
35. Abdel-Rahman O. Impact of diabetes comorbidity on the efficacy and safety of FOLFOX first-line chemotherapy among patients with metastatic colorectal cancer: A pooled analysis of two phase-III studies. Clin Trans Oncol (2019) 21(4):512–8. doi: 10.1007/s12094-018-1939-8
36. Farmer RE, Ford D, Mathur R, Chaturvedi N, Kaplan R, Smeeth L, et al. Metformin use and risk of cancer in patients with type 2 diabetes: A cohort study of primary care records using inverse probability weighting of marginal structural models. Int J Epidemiol (2019) 48(2):527–37. doi: 10.1093/ije/dyz005
37. Vernieri C, Galli F, Ferrari L, Marchetti P, Lonardi S, Maiello E, et al. Impact of metformin use and diabetic status during adjuvant fluoropyrimidine-oxaliplatin chemotherapy on the outcome of patients with resected colon cancer: A TOSCA study subanalysis. Oncologist (2019) 24(3):385–93. doi: 10.1634/theoncologist.2018-0442
38. Kamarudin MNA, Sarker MMR, Zhou J-R, Parhar I. Metformin in colorectal cancer: Molecular mechanism, preclinical and clinical aspects. J Exp Clin Cancer Res (2019) 38(1):491. doi: 10.1186/s13046-019-1495-2
39. Bootsma S, van Neerven SM, Vermeulen L. Exploiting KRAS-mediated metabolic reprogramming as a therapeutic target. Nat Genet (2021) 53(1):9–10. doi: 10.1038/s41588-020-00758-y
40. Almendro V, Marusyk A, Polyak K. Cellular heterogeneity and molecular evolution in cancer. Annu Rev Pathol (2013) 8:277–302. doi: 10.1146/annurev-pathol-020712-163923
41. Kreso A, O'Brien CA, van Galen P, Gan OI, Notta F, Brown AM, et al. Variable clonal repopulation dynamics influence chemotherapy response in colorectal cancer. Sci (New York NY) (2013) 339(6119):543–8. doi: 10.1126/science.1227670
42. Buzzai M, Jones RG, Amaravadi RK, Lum JJ, DeBerardinis RJ, Zhao F, et al. Systemic treatment with the antidiabetic drug metformin selectively impairs p53-deficient tumor cell growth. Cancer Res (2007) 67(14):6745–52. doi: 10.1158/0008-5472.CAN-06-4447
43. Dowling RJ, Zakikhani M, Fantus IG, Pollak M, Sonenberg N. Metformin inhibits mammalian target of rapamycin-dependent translation initiation in breast cancer cells. Cancer Res (2007) 67(22):10804–12. doi: 10.1158/0008-5472.CAN-07-2310
44. Liu J, Hou M, Yuan T, Yi G, Zhang S, Shao X, et al. Enhanced cytotoxic effect of low doses of metformin combined with ionizing radiation on hepatoma cells via ATP deprivation and inhibition of DNA repair. Oncol Rep (2012) 28(4):1406–12. doi: 10.3892/or.2012.1932
45. Jeong YK, Kim M-S, Lee JY, Kim EH, Ha H. Metformin radiosensitizes p53-deficient colorectal cancer cells through induction of G2/M arrest and inhibition of DNA repair proteins. PLoS One (2015) 10(11):e0143596. doi: 10.1371/journal.pone.0143596
46. Salani B, Rio AD, Marini C, Sambuceti G, Cordera R, Maggi D. Metformin, cancer and glucose metabolism. Endocrine-Related Cancer (2014) 21(6):R461–R71. doi: 10.1530/ERC-14-0284
47. Varghese S, Samuel SM, Varghese E, Kubatka P, Büsselberg D. High glucose represses the anti-proliferative and pro-apoptotic effect of metformin in triple negative breast cancer cells. Biomolecules (2019) 9(1):16. doi: 10.3390/biom9010016
Keywords: rectal cancer, metformin, radiotherapy, neoadjuvant chemoradiation, zebrafish Avatars
Citation: Costa B, Fernandez LM, Parés O, Rio-Tinto R, Santiago I, Castillo-Martin M, Parvaiz A and Fior R (2022) Zebrafish Avatars of rectal cancer patients validate the radiosensitive effect of metformin. Front. Oncol. 12:862889. doi: 10.3389/fonc.2022.862889
Received: 26 January 2022; Accepted: 23 August 2022;
Published: 28 September 2022.
Edited by:
Kumar Bishnupuri, Washington University in St. Louis, United StatesReviewed by:
Fernanda Koyama, Oncoclinicas Group, BrazilMark De Ridder, Vrije University Brussel, Belgium
Copyright © 2022 Costa, Fernandez, Parés, Rio-Tinto, Santiago, Castillo-Martin, Parvaiz and Fior. This is an open-access article distributed under the terms of the Creative Commons Attribution License (CC BY). The use, distribution or reproduction in other forums is permitted, provided the original author(s) and the copyright owner(s) are credited and that the original publication in this journal is cited, in accordance with accepted academic practice. No use, distribution or reproduction is permitted which does not comply with these terms.
*Correspondence: Bruna Costa, YnJ1bmEuY29zdGFAcmVzZWFyY2guZmNoYW1wYWxpbWF1ZC5vcmc=; Laura M. Fernandez, bGF1cmEuZmVybmFuZGV6QGZ1bmRhY2FvY2hhbXBhbGltYXVkLnB0; Rita Fior, cml0YS5maW9yQHJlc2VhcmNoLmZjaGFtcGFsaW1hdWQub3Jn