- 1Department of Neurosurgery, Carol Davila University of Medicine and Pharmacy, Bucharest, Romania
- 2Department of Neurosurgery, Bagdasar-Arseni Clinical Emergency Hospital, Bucharest, Romania
GFAPδ, the delta isoform of the glial fibrillary acidic protein, is mainly expressed in the subventricular zone of the brain, together with other neural stem cell markers like nestin. The authors of this paper were among the first that described in detail the expression of GFAPδ and its correlation with malignancy and invasiveness in cerebral astrocytoma. Later, several papers confirmed these findings, showing that the alternative splice variant GFAPδ is overexpressed in glioblastoma (CNS WHO grade 4) compared with lower grade gliomas. Other studies suggested that a high GFAPδ/α ratio is associated with a more malignant and invasive behavior of glioma cells. Moreover, the changing of GFAPδ/α ratio affects the expression of high-malignant genes. It is now suggested that discriminating between predominant GFAP isoforms, GFAPδ or GFAPα, is useful for assessing the malignancy state of astrocytoma, and may even contribute to the classification of gliomas. Therefore, the purpose of this paper is to review the literature with emphasize on the role of GFAPδ as a potential biomarker, and as a possible therapeutic target in glioblastoma.
Introduction
Glial fibrillar acid protein (GFAP) is a type III intermediate filament protein (IF) found in the cytoskeleton of central nervous system’s (CNS) glial cells (1). GFAP molecule contains 432 aminoacids and has head and tail domains flanking a central α-helical rod domain. Interestingly, during the evolution, more than 90% of the amino acid sequence is conserved among human, mouse, and rat (2). GFAP molecules, after posttranslational modification (mainly phosphorylation and citrullination), start assembling in a multistep process like other type III intermediate filament proteins (3). This process is initiated with monomers binding in a parallel fashion to form dimers, then tetramers are formed by antiparallel association of dimers, followed by lateral bindings that produce octamers, oligomers, and the final filament structures (4).
GFAP is largely expressed in the astrocytes of the central nervous system, but it can also be found in nonmyelinating Schwann cells and enteric glia. GFAP is expressed not only in normal brain tissue, but also in brain tumors like astrocytoma, where it is one of the most important markers for astrocyte lineage. GFAP is also expressed in other tumors like ependymoma (5), pleomorphic xanthoastrocytoma (6), and in other unexpected sites such as myoepithelial tissue and salivary gland tumors (7).
Since its first report by Eng et al. in 1969 (1, 8), 6 isoforms have been described, from human and rodent sources, with splice variants at both 5’ and 3’ ends (Table 1) (11–15, 22, 23).
The most abundant GFAP isoform in glial cells is GFAPα, which is the 432 amino acid protein homomerically assembled. The next GFAP isoforms discovered, GFAPβ and GFAPγ, are different from the main isoform by RNA start sites, with GFAPβ mRNA being upstream of that of GFAPα (11, 24) and GFAPγ beginning transcription at 130 nucleotides from the end of GFAPα intron 1 (12). GFAPβ and GFAPγ splice variants carry downstream of their transcription start sites the GFAPα exons. Among cytoskeleton intermediate filaments, alternative splicing is a well-described process in GFAP and synemin, producing additional isoforms (25). Other isoforms of interest produced by alternative splicing are GFAPδ and the latest described, GFAP kappa (Table 1) (15).
GFAPδ – Molecular Structure and Expression
In 1999, Condorelli et al. discovered a new transcript named GFAPδ, which was isolated from the rat hippocampus (Table 1) (13). GFAPδ transcript contained a previously undetected exon, exon 7a, which replaces the exons 8 and 9 from GFAPα. The result is a distinct C-terminal tail domain of GFAPδ compared to GFAPα sequence. Exon 7a, which is present in all mammals, including humans, is unique by its splice acceptor site and polyadenylation signal (26). From a functional viewpoint, the difference in the C-terminal tail domain is crucial. Therefore, GFAPδ by itself can aggregate and prevent normal filament assembly if its concentration (induced by transfection of astrocytic cell line) reaches a threshold concentration (10-30% of total GFAP) (27, 28).
The subventricular zone (SVZ) is a distinct region of the brain with specific features. One of the most important characteristics of this area is the presence of particular cell populations with stem-like properties. Numerous studies have identified a subpopulation of astrocytes as the multipotent neural stem cells (NSCs) of the adult mammalian brain (29–33). Interestingly, Roelofs and colleagues found that within all the areas that were tested from human postmortem brain specimens, the largest localization of GFAPδ immunopositive astrocytes was in the subependymal layer of each lateral ventricle (Figure 1A) (16). These astrocytes have a particular phenotype and form a ribbon of cells along the lateral ventricles (16). Even though the authors found that the population of GFAPδ -positive cells in the SVZ is considerably higher than the number of NSC in this area [approximated by Morshead et al. at 0.2-0.4% NSC (34)], they considered that a certain subgroup of GFAPδ -positive SVZ astrocytes represent the multipotent NSC (16).
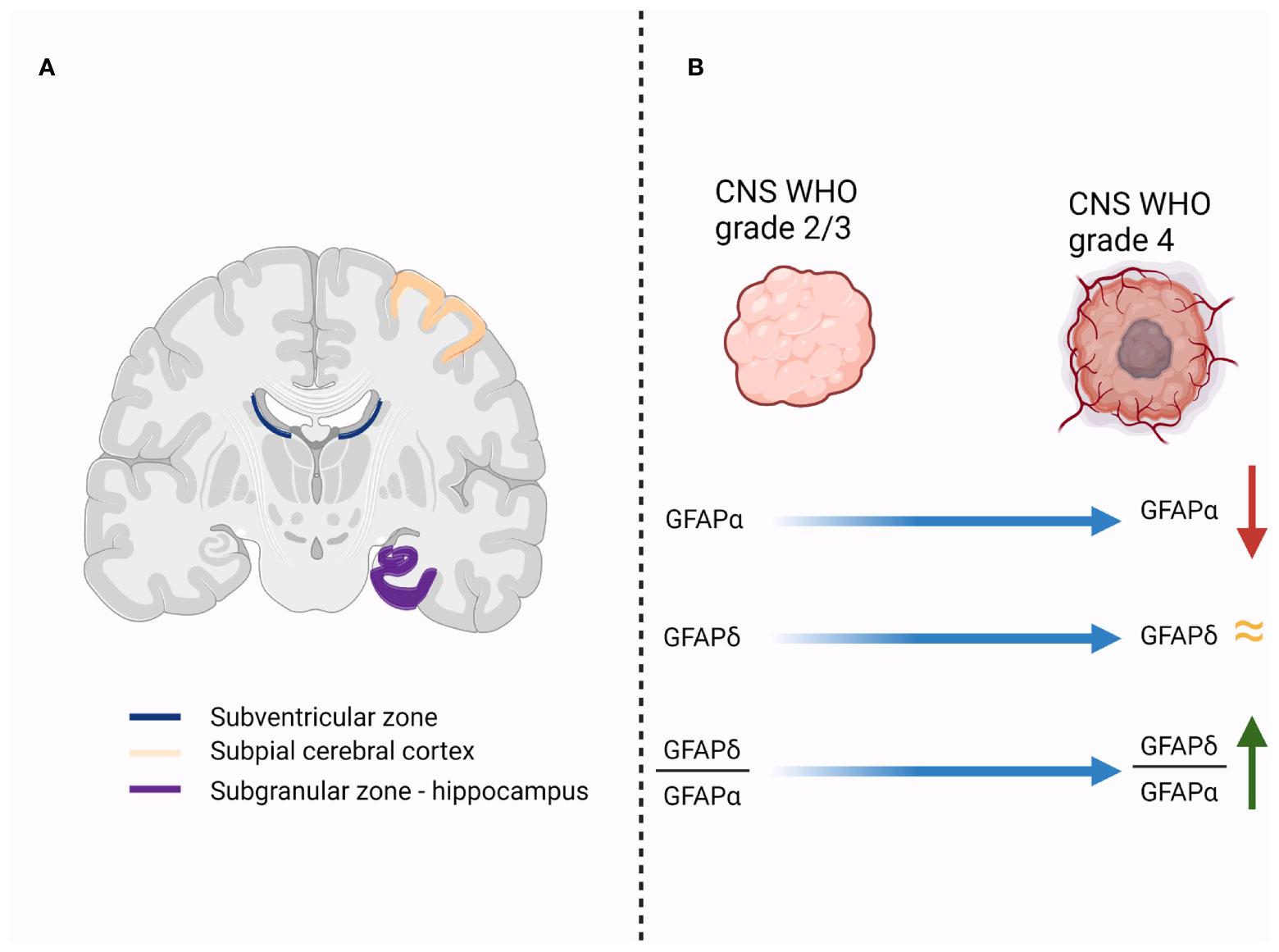
Figure 1 (A). Illustrates the regions in the human brain that have a high expression of GFAPδ-positive cells (subventricular zone, subpial cerebral cortex and subgranular zone of the hippocampus); (B). Initial studies showed that the expression of GFAPα decreases in higher grade gliomas, while the level of GFAPδ remains relatively the same. Therefore, the ratio between GFAPδ and GFAPα expression increases in higher-grade tumors, and it is associated with a more malignant profile.
Another study, published by van den Berge and colleagues, demonstrated that GFAPδ expressing cells were found not only in the SVZ but also in the rostral migratory stream (RMS) on the way to the olfactory bulb (35). Nestin, a marker for NSCs, proliferating cell nuclear antigen (PCNA) and Mcm2, which are cell proliferation markers are expressed simultaneously with GFAPδ in these cells (35). The authors support this hypothesis with evidence that GFAPδ expressing cells in the SVZ resemble immature astrocytes with neural stem cells behavior. Furthermore, vimentin, a marker for immature astrocytes and the general astrocyte marker GFAPα are co-expressed in these cells (8). Notably, GFAPδ-positive cells lacked expression of late markers of astrocyte development, such as glutamine synthetase (GS) and S100B. More important, to support their assertion is the evidence of co-expression, in GFAPδ-positive cells of the transcription factor Sox2, important for the maintenance of adult neurogenesis (36). Based on these results, GFAPδ acts as a marker of NSCs in the SVZ.
Sanai and colleagues raised the hypothesis that SVZ is probably the origin of cerebral gliomas (17). The transformation of these astrocytes with stem features, which occurs in the SVZ, followed by outward migration, could be the origin of astrocytomas (17). As these astrocytes (which reside in the SVZ) express GFAPδ, a reasonable assumption is that cerebral astrocytoma may retain the molecular signature and express GFAPδ. Our previous study confirmed this hypothesis and has demonstrated a statistically significant correlation between the grade of GFAPδ immunostaining and the grade of nestin immunostaining in cerebral astrocytoma (Table 1) (37). Moreover, a statistically significant correlation was found between the neuroimaging invasiveness of cerebral astrocytoma and GFAPδ immunostaining grade (18).
GFAPδ as a Marker of Invasiveness in Malignant Astrocytoma
Cerebral astrocytoma is the most common primary cerebral tumor, with an incidence slightly higher in the male population and is commonly encountered in adult age (38). There are four-grade astrocytomas, with CNS WHO grade 4 being the most malignant type with a median survival despite combined treatment (radical surgical resection followed by radiotherapy and chemotherapy) of approximately 15 months (39). The new WHO 2021 classification system includes several biomarkers to classify cerebral gliomas and to better predict the malignant behavior of these tumors (40). Adult type diffuse gliomas are therefore classified as isocitrate dehydrogenase (IDH)-mutant astrocytoma (graded CNS WHO 2/3/4), IDH mutant and 1p/19q codeleted oligodendroglioma (graded CNS WHO 2/3) and glioblastoma (GBM) IDH-wildtype (graded CNS WHO 4). Grading is based on natural history and invasiveness (40).
Invasion is one of the most important pathological features, which precludes total resection and favors an early tumor recurrence. Certain glioblastomas (CNS WHO grade 4 astrocytomas) have an unusual short clinical course to recurrence after radical resection followed by radiotherapy and chemotherapy and display a highly invasive re-growth pattern with tumor infiltration in the contralateral cerebral hemisphere or satellite tumors developed in distant locations from the original tumor site.
Several markers have been used to determine the prognostic of glioblastoma patients. The evaluation of the Ki-67 labelling index has been described for glioblastoma, but the existing data are controversial whether there is (41, 42) or not (43–45) a benefit on survival. The methylation test for MGMT (O6-methylguanine DNA methyltransferase) promoter is one of the most commonly used predictive markers (46) and while numerous studies have reported that the hypermethylation of MGMT promoter is associated with increased overall survival (OS) and progression-free survival (PFS) (47), other authors report no difference between the survival of patients with MGMT methylation and those without (48). Moreover, Poon et al. showed that the methylation status of the MGMT promoter influenced only the survival of patients that did not complete the temozolomide regimen, having a limited impact on the survival of patients that completed the regimen (49).
Regarding the role of GFAP as a biomarker, Ahmadipour et al. demonstrated by using immunohistochemical staining of paraffin-embedded glioblastoma samples that a GFAP value ≥75% is associated with worse survival, independent of the MGMT promoter methylation status or extent of resection (50). Another study by Sommerlath et al. assessed the differences between long and short-term survivors regarding the GFAP expression, MGMT status and Ki-67 index (51). A decreased Ki-67 index was observed in patients with increased survival, but the difference was significant only when compared with one of the two short-term survivor groups that were included in the study (51). MGMT promoter hypermethylation and GFAP-positive tumors were significantly associated with increased OS when compared to both short-term survivor groups and patients with GFAP-positive tumors had a longer survival independent of the MGMT promoter status (51). Considering these contradictory findings regarding the role of the overall expression of GFAP as a prognostic factor for glioblastoma patients, it is necessary to evaluate the expression of the GFAP isoforms.
Several studies highlighted the expression of high levels of GFAPδ in neurogenic stem cells (16, 35, 52) and in high-grade astrocytomas compared to lower-grade ones (37, 53, 54).
With the objective to accurately distinguish the differentiation state of astrocytomas, it will be necessary to assess the predominant GFAP isoform expression, either GFAPα or GFAPδ (55). Accordingly, the GFAPδ/α ratio is increased in grade IV astrocytoma (Figure 1B) (19).
Moeton et al. proved that increased GFAPδ expression changes the interaction of astrocytoma cells with the microenvironment, with significantly decreased motility by down-regulation of plectin, a protein involved in the filaments network and over-expression of the extracellular matrix component laminin (20).
Uceda-Castro et al., with the use of ex vivo brain slice invasion model and intravital imaging, showed different migratory dynamics of glioma cells depending on the GFAPδ and GFAPα expression levels. High-grade gliomas are associated with alternative splicing in GFAP expression, as GFAPα is downregulated while GFAPδ has an increased dominance in these tumors (Table 1) (21).
Also, GFAPδ showed to be a reliable marker for spinal cord astrocytoma diagnosis, with GFAPδ immunoreactivity being significantly correlated with spinal cord astrocytoma grade (56).
Kanski et al. demonstrated that inhibition of histone deacetylases (HDACs) reduces GFAP expression in astrocytoma cells and the ratio between GFAPδ and canonical isoform GFAPα increases in favor of GFAPδ (57). Histone alteration plays an essential role in glioblastoma genesis, progression and treatment resistance and depends on two types of enzymes, histone acetyltransferases (HATs) and HDACs. To maintain this balance, HDAC inhibitors (HDACis) are identified as novel agents for cancer therapy (58).
GFAPα/GFAPδ Ratio and the Malignant Profile of Cerebral Astrocytoma
Further studies focused on the expression of GFAP isoforms in cerebral astrocytomas showed that, while GFAPα expression is significantly lower in grade IV astrocytomas compared to grade II and grade III astrocytomas, the expression of the alternative splice variant GFAPδ tends to be maintained between astrocytoma grades (19). The result is an increase in GFAPδ expression compared to GFAPα, translated by higher GFAPδ/α ratio in grade IV astrocytoma compared to lower grade (Figure 1B) (19). More important, a higher GFAPδ/α ratio is not only an epiphenomenon associated with malignant profile of cerebral astrocytoma (19). Stassen and colleagues demonstrated that GFAPδ/α ratio regulates high-malignant genes and many of those genes are involved in the regulation of important biological process like the mitotic cell cycle, regulation of cell proliferation and regulation of phosphorylation (19). Therefore, the conclusion of the authors was that while searching for novel therapeutic targets for cerebral astrocytomas, modulating GFAP isoforms expression and selectively splicing should be considered (19).
Interestingly, a higher GFAPδ/α ratio induces not only changes in the genetic expression that regulates the biological process of the astrocytic cells, but also it activates genes involved in the interaction between glioma cells and the extracellular matrix (ECM) (59). One of the key molecules activated by an increased GFAPδ/α ratio in vitro is the dual-specificity phosphatase 4 (DUSP4), also called MAPK phosphatase 2 (19). In glioma patients, DUSP4 expression correlates with the GFAPδ/α ratio, and high expression is associated with worse prognosis (Table 1). This phosphatase plays a key role in MAPK-signaling pathway, which in turn regulates various tumor malignancy–related processes. In gliomas, mutations in the MAPK pathway and constitutive activation of the DUSP4 that target ERK and Janus kinase (JNK) are common (60–62). Moreover, DUSP4 activity influences key biological process dysregulated in gliomas like cell migration (63), invasion (64), proliferation (65), ECM degradation (66), and chemotherapy-induced cytotoxicity (67–69).
Another important gene, LAMA1, which encodes the laminin alpha1 chain of the ECM molecule laminin-111, was significantly increased in cells with a high GFAPδ/α ratio (19, 20). Other previous experiments demonstrated that GFAPδ/α ratio influenced the expression of a downstream effector of laminin-signaling activity, metalloproteinase 2 (19, 70). This is a well-studied metalloproteinase involved in cell invasion (71, 72) and is associated with glioma malignancy (73). Therefore, concerning cell–ECM interaction pathways changed by DUSP4 status, van Bodegraven and colleagues show that a high GFAPδ/α ratio enables glioma cells to have a greater invasiveness capability in the brain (Table 1) (55).
Future Directions and Conclusion
The poor prognosis of glioblastoma is primarily related to the local invasiveness and the tendency to relapse due to the radio- and chemotherapy resistance after surgical resection.
A recent meta-analysis also showed that GFAP levels measured from serum can be used to identify glioblastoma, but further studies are needed since currently the sensitivity of this method is still poor (74). Therefore, the assessment of GFAP in biofluids has a limited role.
However, when performing a biopsy procedure or a surgical resection of a glioblastoma, the high expression of GFAPδ, an alternative splice variant of GFAP, could predict the invasiveness and the increased risk for tumor recurrence. Therefore, it would be useful to regularly assess the immunohistochemical expression of GFAPδ (together with other glioblastoma markers) and patients with increased expression of GFAPδ in the glioblastoma samples from the initial surgery should be closely monitored after surgery. These patients should be stratified as high risk of early recurrence and should be closely followed-up by regular neuroimaging investigations.
Since a high GFAPδ/α ratio is associated with the expression of high-malignant genes and migratory dynamics of glioma cells, novel therapies should focus on balancing the ratio between GFAPα and GFAPδ to decrease the motility and invasiveness of malignant glioma cells. The regulation of histone acetylation has an essential role in glioblastoma and could be a promising target by reducing GFAP total expression. To date, new HDAC inhibitors are under study.
Author Contributions
FB conceptualized the paper. FB and GP made the figure. All authors performed the literature search, wrote the manuscript, and reviewed and approved the final form of the manuscript.
Conflict of Interest
The authors declare that the research was conducted in the absence of any commercial or financial relationships that could be construed as a potential conflict of interest.
Publisher’s Note
All claims expressed in this article are solely those of the authors and do not necessarily represent those of their affiliated organizations, or those of the publisher, the editors and the reviewers. Any product that may be evaluated in this article, or claim that may be made by its manufacturer, is not guaranteed or endorsed by the publisher.
Acknowledgments
The figure was created using BioRender.com.
References
1. Messing A, Brenner M. GFAP at 50. ASN Neuro (2020) 12:1759091420949680. doi: 10.1177/1759091420949680
2. Brenner M, Kisseberth WC, Su Y, Besnard F, Messing A. GFAP Promoter Directs Astrocyte-Specific Expression in Transgenic Mice. J Neurosci (1994) 14(3):1030. doi: 10.1523/JNEUROSCI.14-03-01030.1994
3. Snider NT, Omary MB. Post-Translational Modifications of Intermediate Filament Proteins: Mechanisms and Functions. Nat Rev Mol Cell Biol (2014) 15(3):163–77. doi: 10.1038/nrm3753
4. Herrmann H, Häner M, Brettel M, Ku N-O, Aebi U. Characterization of Distinct Early Assembly Units of Different Intermediate Filament Proteins11edited by W. Baumeister J Mol Biol (1999) 286(5):1403–20. doi: 10.1006/jmbi.1999.2528
5. Tena-Suck ML, Castillejos-López M, Díaz-Alba A, Sanchez-Garibay C. Expression of Glial Acidic Fibrillary Protein Delta and E-Cadherin in Ependymomas; Clinic-Pathological and Immunohistochemistry Correlation. J Histol Histopathol (2015) 2(1):17. doi: 10.7243/2055-091X-2-17
6. Ono T, Sasajima T, Shimizu H, Natsumeda M, Kanamori M, Asano K, et al. Molecular Features and Prognostic Factors of Pleomorphic Xanthoastrocytoma: A Collaborative Investigation of the Tohoku Brain Tumor Study Group. Neurologia Medico-Chirurgica (2020) 60(11):543–52. doi: 10.2176/nmc.oa.2020-0155
7. Huang J, Shrestha P, Takai Y, Mori M. Immunohistochemical Evaluation of GFAP in Salivary Gland Tumors. Acta Histochemica Cytochemica (1996) 29(2):85–92. doi: 10.1267/ahc.29.85
8. Eng LF, Ghirnikar RS, Lee YL. Glial Fibrillary Acidic Protein: Gfap-Thirty-One Years (1969–2000). Neurochemical Res (2000) 25(9):1439–51. doi: 10.1023/A:1007677003387
9. Eng LF, Vanderhaeghen JJ, Bignami A, Gerstl B. An Acidic Protein Isolated From Fibrous Astrocytes. Brain Res (1971) 28(2):351–4. doi: 10.1016/0006-8993(71)90668-8
10. Uyeda CT, Eng LF, Bignami A. Immunological Study of the Glial Fibrillary Acidic Protein. Brain Res (1972) 37(1):81–9. doi: 10.1016/0006-8993(72)90347-2
11. Feinstein DL, Weinmaster GA, Milner RJ. Isolation of Cdna Clones Encoding Rat Glial Fibrillary Acidic Protein: Expression in Astrocytes and in Schwann Cells. J Neurosci Res (1992) 32(1):1–14. doi: 10.1002/jnr.490320102
12. Zelenika D, Grima B, Brenner M, Pessac B. A Novel Glial Fibrillary Acidic Protein Mrna Lacking Exon 1. Mol Brain Res (1995) 30(2):251–8. doi: 10.1016/0169-328X(95)00010-P
13. Condorelli DF, Nicoletti VG, Barresi V, Conticello SG, Caruso A, Tendi EA, et al. Structural Features of the Rat GFAP Gene and Identification of a Novel Alternative Transcript. J Neurosci Res (1999) 56(3):219–28. doi: 10.1002/(SICI)1097-4547(19990501)56:3<219::AID-JNR1>3.0.CO;2-2
14. Nielsen AL, Holm IE, Johansen M, Bonven B, Jørgensen P, Jørgensen AL. A New Splice Variant of Glial Fibrillary Acidic Protein, GFAPƐ;, Interacts With the Presenilin Proteins *. J Biol Chem (2002) 277(33):29983–91. doi: 10.1074/jbc.M112121200
15. Blechingberg J, Holm IE, Nielsen KB, Jensen TH, Jørgensen AL, Nielsen AL. Identification and Characterization of Gfapκ, a Novel Glial Fibrillary Acidic Protein Isoform. Glia (2007) 55(5):497–507. doi: 10.1002/glia.20475
16. Roelofs RF, Fischer DF, Houtman SH, Sluijs JA, Van Haren W, Van Leeuwen FW, et al. Adult Human Subventricular, Subgranular, and Subpial Zones Contain Astrocytes With a Specialized Intermediate Filament Cytoskeleton. Glia (2005) 52(4):289–300. doi: 10.1002/glia.20243
17. Sanai N, Alvarez-Buylla A, Berger MS. Neural Stem Cells and the Origin of Gliomas. New Engl J Med (2005) 353(8):811–22. doi: 10.1056/NEJMra043666
18. Brehar FM, Arsene D, Brinduse LA, Gorgan MR. Immunohistochemical Analysis of GFAP-δ and Nestin in Cerebral Astrocytomas. Brain Tumor Pathol (2015) 32(2):90–8. doi: 10.1007/s10014-014-0199-8
19. Stassen OMJA, van Bodegraven EJ, Giuliani F, Moeton M, Kanski R, Sluijs JA, et al. Gfapδ/Gfapα Ratio Directs Astrocytoma Gene Expression Towards a More Malignant Profile. Oncotarget (2017) 8(50):88104–21. doi: 10.18632/oncotarget.21540
20. Moeton M, Kanski R, Stassen OMJA, Sluijs JA, Geerts D, van Tijn P, et al. Silencing GFAP Isoforms in Astrocytoma Cells Disturbs Laminin-Dependent Motility and Cell Adhesion. FASEB J (2014) 28(7):2942–54. doi: 10.1096/fj.13-245837
21. Uceda-Castro R, van Asperen JV, Vennin C, Sluijs JA, van Bodegraven EJ, Margarido AS, et al. GFAP Splice Variants Fine-Tune Glioma Cell Invasion and Tumour Dynamics by Modulating Migration Persistence. Sci Rep (2022) 12(1):424. doi: 10.1038/s41598-021-04127-5
22. Hol EM, Roelofs RF, Moraal E, Sonnemans MAF, Sluijs JA, Proper EA, et al. Neuronal Expression of GFAP in Patients With Alzheimer Pathology and Identification of Novel GFAP Splice Forms. Mol Psychiatry (2003) 8(9):786–96. doi: 10.1038/sj.mp.4001379
23. Galea E, Dupouey P, Feinstein DL. Glial Fibrillary Acidic Protein Mrna Isotypes: Expression In Vitro and In Vivo. J Neurosci Res (1995) 41(4):452–61. doi: 10.1002/jnr.490410404
24. Michelle Chin Chia L, Gunter M, Lang Z. Glial Fibrillary Acidic Protein Splice Variants in Hepatic Stellate Cells - Expression and Regulation. Mol Cells (2008) 25(3):376–84.
25. Hol EM, Pekny M. Glial Fibrillary Acidic Protein (GFAP) and the Astrocyte Intermediate Filament System in Diseases of the Central Nervous System. Curr Opin Cell Biol (2015) 32:121–30. doi: 10.1016/j.ceb.2015.02.004
26. Singh R, Nielsen AL, Johansen MG, Jørgensen AL. Genetic Polymorphism and Sequence Evolution of an Alternatively Spliced Exon of the Glial Fibrillary Acidic Protein Gene, GFAP☆. Genomics (2003) 82(2):185–93. doi: 10.1016/S0888-7543(03)00106-X
27. Nielsen AL, Jørgensen AL. Structural and Functional Characterization of the Zebrafish Gene for Glial Fibrillary Acidic Protein, GFAP. Gene (2003) 310:123–32. doi: 10.1016/S0378-1119(03)00526-2
28. Perng M-D, Wen S-F, Gibbon T, Middeldorp J, Sluijs J, Hol EM, et al. Glial Fibrillary Acidic Protein Filaments Can Tolerate the Incorporation of Assembly-Compromised GFAP-δ, But With Consequences for Filament Organization and αb-Crystallin Association. Mol Biol Cell (2008) 19(10):4521–33. doi: 10.1091/mbc.e08-03-0284
29. Doetsch F, García-Verdugo JM, Alvarez-Buylla A. Cellular Composition and Three-Dimensional Organization of the Subventricular Germinal Zone in the Adult Mammalian Brain. J Neurosci (1997) 17(13):5046–61. doi: 10.1523/JNEUROSCI.17-13-05046.1997
30. Doetsch F, Caillé I, Lim DA, García-Verdugo JM, Alvarez-Buylla A. Subventricular Zone Astrocytes Are Neural Stem Cells in the Adult Mammalian Brain. Cell (1999) 97(6):703–16. doi: 10.1016/S0092-8674(00)80783-7
31. Alvarez-Buylla A, García-Verdugo JM. Neurogenesis in Adult Subventricular Zone. J Neurosci (2002) 22(3):629–34. doi: 10.1523/JNEUROSCI.22-03-00629.2002
32. Steindler DA, Kadrie T, Fillmore H, Brannon Thomas L. The Subependymal Zone: “Brain Marrow”. In: Mize RR, Erzurumlu RS, editors. Progress in Brain Research, vol. 108 . Amsterdam: Elsevier (1996). p. 349–63.
33. Sanai N, Tramontin AD, Quiñones-Hinojosa A, Barbaro NM, Gupta N, Kunwar S, et al. Unique Astrocyte Ribbon in Adult Human Brain Contains Neural Stem Cells But Lacks Chain Migration. Nature (2004) 427(6976):740–4. doi: 10.1038/nature02301
34. Morshead CM, Craig CG, van der Kooy D. In Vivo Clonal Analyses Reveal the Properties of Endogenous Neural Stem Cell Proliferation in the Adult Mammalian Forebrain. Development (1998) 125(12):2251–61. doi: 10.1242/dev.125.12.2251
35. Van Den Berge SA, Middeldorp J, Zhang CE, Curtis MA, Leonard BW, Mastroeni D, et al. Longterm Quiescent Cells in the Aged Human Subventricular Neurogenic System Specifically Express GFAP-δ. Aging Cell (2010) 9(3):313–26. doi: 10.1111/j.1474-9726.2010.00556.x
36. Graham V, Khudyakov J, Ellis P, Pevny L. SOX2 Functions to Maintain Neural Progenitor Identity. Neuron (2003) 39(5):749–65. doi: 10.1016/S0896-6273(03)00497-5
37. Brehar FM, Gorgan MR. Gfap-δ and Nestin as Molecular Markers Related to the Cell Origins and Invasion in Human Gliomas. J Neurol Surg A Cent Eur Neurosurg (2014) 75(S 01):o009. doi: 10.1055/s-0034-1382170
38. Ostrom QT, Cioffi G, Waite K, Kruchko C, Barnholtz-Sloan JS. CBTRUS Statistical Report: Primary Brain and Other Central Nervous System Tumors Diagnosed in the United States in 2014–2018. Neuro-Oncology (2021) 23(Supplement_3):iii1–iii105. doi: 10.1093/neuonc/noab200
39. Koshy M, Villano JL, Dolecek TA, Howard A, Mahmood U, Chmura SJ, et al. Improved Survival Time Trends for Glioblastoma Using the SEER 17 Population-Based Registries. J Neuro-Oncol (2012) 107(1):207–12. doi: 10.1007/s11060-011-0738-7
40. Louis DN, Perry A, Wesseling P, Brat DJ, Cree IA, Figarella-Branger D, et al. The 2021 WHO Classification of Tumors of the Central Nervous System: A Summary. Neuro-Oncology (2021) 23(8):1231–51. doi: 10.1093/neuonc/noab106
41. Wong E, Nahar N, Hau E, Varikatt W, Gebski V, Ng T, et al. Cut-Point for Ki-67 Proliferation Index as a Prognostic Marker for Glioblastoma. Asia-Pacific J Clin Oncol (2019) 15(1):5–9. doi: 10.1111/ajco.12826
42. Yoshida Y, Nakada M, Harada T, Tanaka S, Furuta T, Hayashi Y, et al. The Expression Level of Sphingosine-1-Phosphate Receptor Type 1 Is Related to MIB-1 Labeling Index and Predicts Survival of Glioblastoma Patients. J Neuro-Oncol (2010) 98(1):41–7. doi: 10.1007/s11060-009-0064-5
43. Dahlrot RH, Bangsø JA, Petersen JK, Rosager AM, Sørensen MD, Reifenberger G, et al. Prognostic Role of Ki-67 in Glioblastomas Excluding Contribution From non-Neoplastic Cells. Sci Rep (2021) 11(1):17918. doi: 10.1038/s41598-021-95958-9
44. Moskowitz SI, Jin T, Prayson RA. Role of MIB1 in Predicting Survival in Patients With Glioblastomas. J Neuro-Oncol (2006) 76(2):193–200. doi: 10.1007/s11060-005-5262-1
45. Tsidulko AY, Kazanskaya GM, Kostromskaya DV, Aidagulova SV, Kiselev RS, Volkov AM, et al. Prognostic Relevance of NG2/CSPG4, CD44 and Ki-67 in Patients With Glioblastoma. Tumor Biol (2017) 39(9):1010428317724282. doi: 10.1177/1010428317724282
46. Holdhoff M, Ye X, Blakeley JO, Blair L, Burger PC, Grossman SA, et al. Use of Personalized Molecular Biomarkers in the Clinical Care of Adults With Glioblastomas. J Neuro-Oncol (2012) 110(2):279–85. doi: 10.1007/s11060-012-0968-3
47. Zhao H, Wang S, Song C, Zha Y, Li L. The Prognostic Value of MGMT Promoter Status by Pyrosequencing Assay for Glioblastoma Patients’ Survival: A Meta-Analysis. World J Surg Oncol (2016) 14(1):261. doi: 10.1186/s12957-016-1012-4
48. Egaña L, Auzmendi-Iriarte J, Andermatten J, Villanua J, Ruiz I, Elua-Pinin A, et al. Methylation of MGMT Promoter Does Not Predict Response to Temozolomide in Patients With Glioblastoma in Donostia Hospital. Sci Rep (2020) 10(1):18445. doi: 10.1038/s41598-020-75477-9
49. Poon MTC, Keni S, Vimalan V, Ip C, Smith C, Erridge S, et al. Extent of MGMT Promoter Methylation Modifies the Effect of Temozolomide on Overall Survival in Patients With Glioblastoma: A Regional Cohort Study. Neuro-Oncol Adv (2021) 3(1):1–11. doi: 10.1093/noajnl/vdab171
50. Ahmadipour Y, Gembruch O, Pierscianek D, Sure U, Jabbarli R. Does the Expression of Glial Fibrillary Acid Protein (GFAP) Stain in Glioblastoma Tissue Have a Prognostic Impact on Survival? Neurochirurgie (2020) 66(3):150–4. doi: 10.1016/j.neuchi.2019.12.012
51. Sommerlath VN, Buergy D, Etminan N, Brehmer S, Reuss D, Sarria GR, et al. Molecular Features of Glioblastomas in Long-Term Survivors Compared to Short-Term Survivors—A Matched-Pair Analysis. Radiat Oncol (2022) 17(1):15. doi: 10.1186/s13014-022-01984-w
52. Middeldorp J, Hol EM. GFAP in Health and Disease. Prog Neurobiol (2011) 93(3):421–43. doi: 10.1016/j.pneurobio.2011.01.005
53. Choi K-C, Kwak S-E, Kim J-E, Sheen SH, Kang T-C. Enhanced Glial Fibrillary Acidic Protein-δ Expression in Human Astrocytic Tumor. Neurosci Lett (2009) 463(3):182–7. doi: 10.1016/j.neulet.2009.07.076
54. Andreiuolo F, Junier M-P, Hol EM, Miquel C, Chimelli L, Leonard N, et al. Gfapδ Immunostaining Improves Visualization of Normal and Pathologic Astrocytic Heterogeneity. Neuropathology (2009) 29(1):31–9. doi: 10.1111/j.1440-1789.2008.00936.x
55. van Bodegraven EJ, van Asperen JV, Robe PAJ, Hol EM. Importance of GFAP Isoform-Specific Analyses in Astrocytoma. Glia (2019) 67(8):1417–33. doi: 10.1002/glia.23594
56. Heo DH, Kim SH, Yang K-M, Cho YJ, Kim KN, Yoon DH, et al. A Histopathological Diagnostic Marker for Human Spinal Astrocytoma: Expression of Glial Fibrillary Acidic Protein-δ. J Neuro-Oncol (2012) 108(1):45–52. doi: 10.1007/s11060-012-0801-z
57. Kanski R, Sneeboer MAM, van Bodegraven EJ, Sluijs JA, Kropff W, Vermunt MW, et al. Histone Acetylation in Astrocytes Suppresses GFAP and Stimulates a Reorganization of the Intermediate Filament Network. J Cell Sci (2014) 127(20):4368–80. doi: 10.1242/dev.118489
58. Chen R, Zhang M, Zhou Y, Guo W, Yi M, Zhang Z, et al. The Application of Histone Deacetylases Inhibitors in Glioblastoma. J Exp Clin Cancer Res (2020) 39(1):138. doi: 10.1186/s13046-020-01643-6
59. van Bodegraven EJ, van Asperen JV, Sluijs JA, van Deursen CBJ, van Strien ME, Stassen OMJA, et al. GFAP Alternative Splicing Regulates Glioma Cell–ECM Interaction in a DUSP4-Dependent Manner. FASEB J (2019) 33(11):12941–59. doi: 10.1096/fj.201900916R
60. Pandey V, Bhaskara VK, Babu PP. Implications of Mitogen-Activated Protein Kinase Signaling in Glioma. J Neurosci Res (2016) 94(2):114–27. doi: 10.1002/jnr.23687
61. Jeuken J, van den Broecke C, Gijsen S, Boots-Sprenger S, Wesseling P. RAS/RAF Pathway Activation in Gliomas: The Result of Copy Number Gains Rather Than Activating Mutations. Acta Neuropathologica (2007) 114(2):121–33. doi: 10.1007/s00401-007-0239-0
62. Ciriello G, Miller ML, Aksoy BA, Senbabaoglu Y, Schultz N, Sander C. Emerging Landscape of Oncogenic Signatures Across Human Cancers. Nat Genet (2013) 45(10):1127–33. doi: 10.1038/ng.2762
63. Lin H, Qiu S, Xie L, Liu C, Sun S. Nimbolide Suppresses Non-Small Cell Lung Cancer Cell Invasion and Migration via Manipulation of DUSP4 Expression and ERK1/2 Signaling. Biomedicine Pharmacother (2017) 92:340–6. doi: 10.1016/j.biopha.2017.05.072
64. Zhang R, Wang G, Zhang P-F, Zhang J, Huang Y-X, Lu Y-M, et al. Sanguinarine Inhibits Growth and Invasion of Gastric Cancer Cells via Regulation of the DUSP4/ERK Pathway. J Cell Mol Med (2017) 21(6):1117–27. doi: 10.1111/jcmm.13043
65. Lawan A, Al-Harthi S, Cadalbert L, McCluskey AG, Shweash M, Grassia G, et al. Deletion of the Dual Specific Phosphatase-4 (DUSP-4) Gene Reveals an Essential non-Redundant Role for MAP Kinase Phosphatase-2 (MKP-2) in Proliferation and Cell Survival *. J Biol Chem (2011) 286(15):12933–43. doi: 10.1074/jbc.M110.181370
66. Paumelle R, Tulasne D, Leroy C, Coll J, Vandenbunder B, Fafeur V. Sequential Activation of ERK and Repression of JNK by Scatter Factor/Hepatocyte Growth Factor in Madin-Darby Canine Kidney Epithelial Cells. Mol Biol Cell (2000) 11(11):3751–63. doi: 10.1091/mbc.11.11.3751
67. Cadalbert L, Sloss CM, Cameron P, Plevin R. Conditional Expression of MAP Kinase Phosphatase-2 Protects Against Genotoxic Stress-Induced Apoptosis by Binding and Selective Dephosphorylation of Nuclear Activated C-Jun N-Terminal Kinase. Cell Signalling (2005) 17(10):1254–64. doi: 10.1016/j.cellsig.2005.01.003
68. Al-Mutairi M, Al-Harthi S, Cadalbert L, Plevin R. Over-Expression of Mitogen-Activated Protein Kinase Phosphatase-2 Enhances Adhesion Molecule Expression and Protects Against Apoptosis in Human Endothelial Cells. Br J Pharmacol (2010) 161(4):782–98. doi: 10.1111/j.1476-5381.2010.00952.x
69. Schmid CA, Robinson MD, Scheifinger NA, Müller S, Cogliatti S, Tzankov A, et al. DUSP4 Deficiency Caused by Promoter Hypermethylation Drives JNK Signaling and Tumor Cell Survival in Diffuse Large B Cell Lymphoma. J Exp Med (2015) 212(5):775–92. doi: 10.1084/jem.20141957
70. Reich R, Blumenthal M, Liscovitch M. Role of Phospholipase D in Laminin-Induced Production of Gelatinase a (MMP-2) in Metastatic Cells. Clin Exp Metastasis (1995) 13(2):134–40. doi: 10.1007/BF00133618
71. Gialeli C, Theocharis AD, Karamanos NK. Roles of Matrix Metalloproteinases in Cancer Progression and Their Pharmacological Targeting. FEBS J (2011) 278(1):16–27. doi: 10.1111/j.1742-4658.2010.07919.x
72. Nakagawa T, Kubota T, Kabuto M, Fujimoto N, Okada Y. Secretion of Matrix Metalloproteinase-2 (72 Kd Gelatinase/Type IV Collagenase = Gelatinase a) by Malignant Human Glioma Cell Lines: Implications for the Growth and Cellular Invasion of the Extracellular Matrix. J Neuro-Oncol (1996) 28(1):13–24. doi: 10.1007/BF00300442
73. Ramachandran RK, Sørensen MD, Aaberg-Jessen C, Hermansen SK, Kristensen BW. Expression and Prognostic Impact of Matrix Metalloproteinase-2 (MMP-2) in Astrocytomas. PloS One (2017) 12(2):e0172234. doi: 10.1371/journal.pone.0172234
Keywords: GFAP, glioblastoma, GFAPδ/α ratio, invasiveness, subventricular zone
Citation: Radu R, Petrescu GED, Gorgan RM and Brehar FM (2022) GFAPδ: A Promising Biomarker and Therapeutic Target in Glioblastoma. Front. Oncol. 12:859247. doi: 10.3389/fonc.2022.859247
Received: 21 January 2022; Accepted: 24 February 2022;
Published: 18 March 2022.
Edited by:
Jose R. Pineda, University of the Basque Country, SpainReviewed by:
Gerardo Caruso, University Hospital of Policlinico G. Martino, ItalyCopyright © 2022 Radu, Petrescu, Gorgan and Brehar. This is an open-access article distributed under the terms of the Creative Commons Attribution License (CC BY). The use, distribution or reproduction in other forums is permitted, provided the original author(s) and the copyright owner(s) are credited and that the original publication in this journal is cited, in accordance with accepted academic practice. No use, distribution or reproduction is permitted which does not comply with these terms.
*Correspondence: George E. D. Petrescu, Z2VvcmdlLnBldHJlc2N1QHVtZmNkLnJv