- 1Department of Medicine, Duke University Medical Center, Durham, NC, United States
- 2Duke Cancer Institute Biostatistics, Duke University Medical Center, Durham, NC, United States
- 3Duke Center for Brain and Spine Metastasis, Duke Cancer Institute, Duke University Medical Center, Durham, NC, United States
- 4Department of Radiation Oncology, Duke University Medical Center, Durham, NC, United States
- 5Department of Biostatistics and Bioinformatics, Duke University Medical Center, Durham, NC, United States
- 6Department of Neurosurgery, Duke University Medical Center, Durham, NC, United States
Background: There is a concern that HER2-directed systemic therapies, when administered concurrently with stereotactic radiosurgery (SRS), may increase the risk of radiation necrosis (RN). This study explores the impact of timing and type of systemic therapies on the development of RN in patients treated with SRS for HER2+ breast cancer brain metastasis (BCBrM).
Methods: This was a single-institution, retrospective study including patients >18 years of age with HER2+ BCBrM who received SRS between 2013 and 2018 and with at least 12-month post-SRS follow-up. Presence of RN was determined via imaging at one-year post-SRS, with confirmation by biopsy in some patients. Demographics, radiotherapy parameters, and timing (“during” defined as four weeks pre- to four weeks post-SRS) and type of systemic therapy (e.g., chemotherapy, HER2-directed) were evaluated.
Results: Among 46 patients with HER2+ BCBrM who received SRS, 28 (60.9%) developed RN and 18 (39.1%) did not based on imaging criteria. Of the 11 patients who underwent biopsy, 10/10 (100%) who were diagnosed with RN on imaging were confirmed to be RN positive on biopsy and 1/1 (100%) who was not diagnosed with RN was confirmed to be RN negative on biopsy. Age (mean 53.3 vs 50.4 years, respectively), radiotherapy parameters (including total dose, fractionation, CTV and size target volume, all p>0.05), and receipt of any type of systemic therapy during SRS (60.7% vs 55.6%, p=0.97) did not differ between patients who did or did not develop RN. However, there was a trend for patients who developed RN to have received more than one agent of HER2-directed therapy independent of SRS timing compared to those who did not develop RN (75.0% vs 44.4%, p=0.08). Moreover, a significantly higher proportion of those who developed RN received more than one agent of HER2-directed therapy during SRS treatment compared to those who did not develop RN (35.7% vs 5.6%, p=0.047).
Conclusions: Patients with HER2 BCBrM who receive multiple HER2-directed therapies during SRS for BCBrM may be at higher risk of RN. Collectively, these data suggest that, in the eight-week window around SRS administration, if HER2-directed therapy is medically necessary, it is preferable that patients receive a single agent.
Introduction
Breast cancer is the most common malignancy diagnosed in women and the second leading cause of cancer-related mortality among women worldwide (1). Increased survival has been observed in breast cancer patients due to advances in early diagnosis/screening methods and improved treatments. However, long-term survival is complicated by increased prevalence of breast cancer brain metastasis (BCBrM), which is associated with poor prognosis and decreased quality of life (2). Specifically, breast cancer is the second most common primary origin of BrM, with 15-30% of patients estimated to develop BrM during the course of advanced disease (3, 4).
Human epidermal growth factor receptor 2-positive (HER2+) breast cancer is a subtype of breast cancer with a predilection for BrM (5). As many as 30% of patients with advanced, metastatic HER2+ breast cancer will develop BrM (6). Current standard of care options for HER2+ BCBrM include radiation therapy (stereotactic radiosurgery [SRS] or whole brain radiation therapy [WBRT]), brain permeable systemic therapies, and/or neurosurgical resection when appropriate (7). A multimodal approach combining these different treatment modalities has improved the overall survival and functional outcomes of patients with BCBrM. Specifically for radiation therapy, SRS is a highly effective form of radiation therapy that offers meaningful control of BrM (8). Because the vast majority of patients who present with BrM have both intracranial and extracranial disease, most of them will also receive systemic treatment.
Radiation-induced injury is one of the most significant complications of brain tumor irradiation (9). One of the important adverse effects associated with SRS is radiation necrosis (RN), which is a late complication of radiation injury and occurs in about 5-25% of treated patients (10, 11). RN often significantly impacts quality of life; for example, it often presents with neurological deficits such as headaches, nausea and seizures (12, 13). The mechanism of RN remains unclear, but the pathology involves inflammation and angiogenesis in a region of coagulative necrosis associated with breakdown of the blood-brain barrier. resulting in perilesional edema and heterogeneous contrast enhancement (14, 15). RN commonly occurs 3-12 months after radiotherapy (16, 17), though it can be observed as late as several years post radiosurgery, in our experience. Because the likelihood of RN depends on factors such as timing of radiation therapy, total dose, dose per fraction and volume irradiated (18–20), efforts to decrease the rate of RN have focused on controlling these radiotherapy parameters. However, recent studies have shown that rates of RN are higher in patients who received both SRS and immunotherapies or targeted therapies compared to those who received SRS alone (21, 22). This association is significant because most BCBrM patients receive concurrent systemic therapy as part of their treatment regimen. This study explores the impact of timing and type of systemic therapies on the development of RN in patients with HER2+ BCBrM treated with SRS.
Methods
This was a single-institution, retrospective study (approved by the Institutional Review Board) of patients >18 years of age with HER2+ BCBrM who received SRS between 2013 and 2018 with at least 12-month post-SRS follow-up. Demographics and baseline characteristics including age at the time of SRS, race, location of irradiated BrM, and number of BrM were collected. Relevant systemic and radiation treatment details were also recorded. Presence of RN was determined via magnetic resonance (MR) imaging one-year post-SRS (see details below). The rate of RN was also determined using biopsy reports of brain lesions if they were available at any time after SRS (i.e., not restricted to within one-year post-SRS). Patients with incomplete follow-up data and/or who were deceased within one-year post-SRS were excluded. Demographics and lesion characteristics considered included age at the time of SRS, race/ethnicity, time to SRS from date of brain metastasis, and location of brain metastasis.
Brain Metastases
Location of irradiated BrM was categorized as follows: frontal, parietal, temporal, occipital, cerebellar, midbrain/brainstem, and multiple. The number of BrM was considered as a binary variable (single or multiple).
Imaging and Pathological Criteria for Radiation Necrosis
MR imaging was used to diagnose RN within 12 months of receipt of SRS. For a given area, the diagnosis of RN was determined by: 1) the degree of hyperintensity on T2-weighted image and enhancement on contrast-enhanced T1-weighted image and 2) assessment and confirmation by neuroradiologists. For biopsy-confirmed cases with associated pathology reports, pure (tumor absent) and mixed (tumor present) RN were all considered as RN.
Radiotherapy Parameters
The total administered dose (in grays) and the number of fractions were collected. Other relevant radiotherapy parameters considered were clinical target volume (CTV), gross tumor volume (GTV), conformity index (CI), and volume receiving 12 gray (V12Gy). For V12Gy, only single-fraction SRS was considered.
Systemic Therapy
Types of systemic therapy included HER2-directed therapy (T-DM1, trastuzumab, pertuzumab, lapatinib), mitosis inhibitors (taxanes, vinca alkaloids, eribulin), DNA synthesis inhibitors (capecitabine, platinum, anthracycline, pemetrexed, cyclophosphamide, doxorubicin, gemcitabine), and other (all other systemic treatments). Timing of systemic therapy with regard to radiotherapy was defined as a binary variable as follows: 1) systemic therapy “during” radiotherapy meant the systemic therapy was administered within 4 weeks prior to Day 1 of SRS treatment through 4 weeks post-SRS Day 1; 2) “not during” radiotherapy meant systemic therapy was administered outside the 8-week window surrounding SRS. Number of systemic therapy agents overall, number of HER2-directed therapies, and use of T-DM1 were also considered.
Statistical Analysis
Categorical variables were summarized with frequencies and percentages and analyzed using Pearson’s Chi-squared test with Yates’ continuity correction. Continuous variables were summarized with means/standard deviations and medians/minimum and maximum and analyzed using the Wilcoxon rank sum test with continuity correction. Statistical significance was assessed at level alpha = 0.05. All statistical analyses were conducted using both SAS 9.4 (SAS Institute Inc., Cary, NC, USA) and R (RStudio, Inc; Boston, MA).
Results
An initial sample of 386 adult patients who were diagnosed with BCBrM were identified between 2013 and 2018. From this sample, 264 patients were excluded as they did not have HER2+ BCBrM. 68 patients were further excluded as they did not receive systemic therapy and/or SRS at our institution. Finally, seven patients who were deceased within one year following SRS were excluded. A final cohort of 46 patients remained after applying these exclusion criteria (Figure 1).
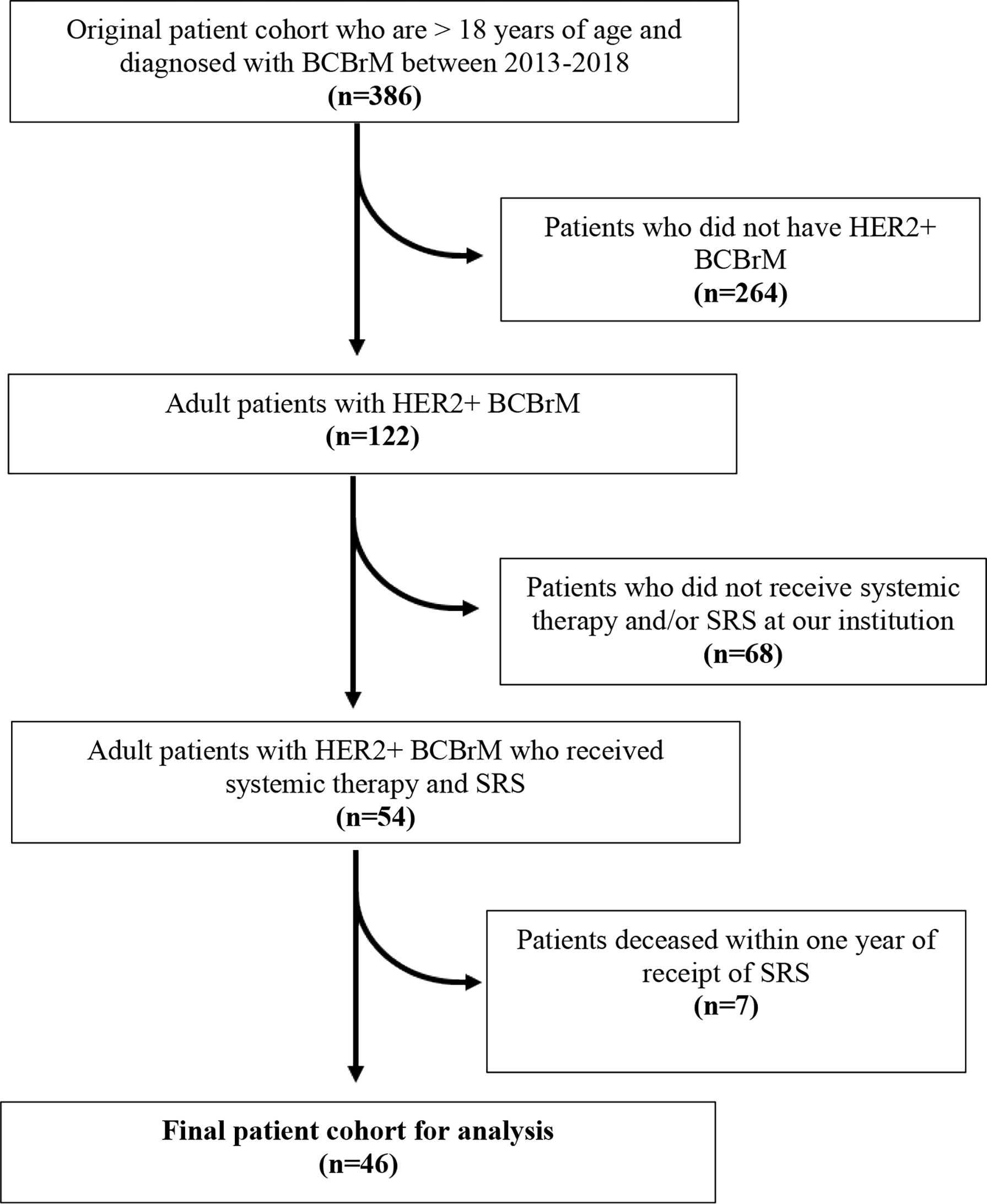
Figure 1 Consort diagram of selection of patient cohort. Inclusion and exclusion criteria applied for derivation of final sample cohort.
Patient Demographics and Lesion Characteristics
Among 46 patients with HER2+ BCBrM who received both SRS and systemic therapies, the mean age at time of SRS was 52.1 years and the cohort was predominantly white (76.1% vs 23.9% non-white). A majority of patients had a single treated BrM (63%) vs multiple (37%).
In this cohort of 46 patients, 28 (60.9%) developed RN and 18 (39.1%) did not based on imaging parameters. Of the 11 patients from whom tissue biopsy samples were obtained (average date of biopsy was 1.5 years after SRS), 10/10 (100%) patients who were diagnosed with RN on imaging were confirmed to have RN on biopsy (with 4 of those reported as pure RN and 6 as mixed RN/recurrence) and 1/1 (100%) patient who was determined to not have RN on imaging was confirmed to not have RN on biopsy. Age at time of SRS did not differ between those who developed RN and those who did not (mean 53.3 vs 50.4 years, respectively; p=0.54). There was a higher, though not statistically significant, percentage of African Americans in the RN group (28.6% vs 11.1%, p=0.24). Although there was no statistically significant difference between the anatomic location of BrM irradiation between the two cohorts, more patients who developed RN had a single BrM lesion (78.6%) as opposed to multiple BrM lesions (21.4%) (p=0.016). Conversely, more patients who did not develop RN had multiple BrM (61.1%) compared to those with a single lesion (38.9%) (p=0.016). The results are summarized in Table 1.
Radiation Treatment
Overall, the mean total dose of SRS administered was 21.9 ± 4.10 Gy. 60.9% of the patients underwent single-fraction SRS. The mean values for the measured radiotherapy parameters were as follows: CTV of 9.15 ± 13.0 cc, GTV of 5.39 ± 7.51 cc, CI of 1.32 ± 0.27, and V12Gy (for single-fraction only) of 7.14 ± 6.28 cc. When we compared the two groups, there were no significant differences in the total dose, fraction (1 vs 5), and all measured radiotherapy parameters (all p>0.05) as shown in Table 2.
Systemic Treatment
In the entire cohort, 58.7% of patients received any type of systemic therapy (i.e., HER2-directed therapy, mitosis inhibitors, DNA synthesis inhibitors, others) during SRS. Specifically, 43.5% received a HER2-directed therapy, 2.2% received a mitosis inhibitor therapy, 8.7% received both HER2-directed and mitosis inhibitor therapy, and 4.3% received other systemic therapy.
Receipt of any systemic therapy during SRS did not differ between patients who did or did not develop RN (60.7% vs 55.6%, p=0.97) (Table 3). However, patients who developed RN more commonly received more than one agent of HER2-directed therapy, independent of SRS timing, compared to those who did not develop RN (75.0% vs 44.4%, p=0.08). A significantly higher proportion of those who developed RN received more than one agent of HER2-directed therapy during SRS compared to those did not develop RN (35.7% vs 5.6%, p=0.047).
Discussion
In our cohort of 46 patients with HER2+ breast cancer and BrM who received SRS, 60.9% of them were determined to have RN on imaging. Of the 11 patients with RN who had a BrM biopsy specimen to evaluate, 10/10 who were diagnosed with RN on imaging were positive for RN on biopsy and 1/1 (100%) who was not diagnosed with RN on imaging was negative for RN. While the radiation treatment parameters did not differ significantly between those who did and did not develop RN, patients with RN were more often treated with more than one agent of HER2-directed therapy during SRS, as defined by 4 weeks prior to or after radiosurgery.
Challenges in Diagnosing Radiation Necrosis
There is no single best imaging modality used to diagnose RN. However, in current practice, MR imaging is the most common modality used to explore RN. Because it is often difficult to distinguish between RN and recurrence, surgical resection remains the best way to establish the histopathological diagnosis of RN along with providing relief from any symptomatic effects. Depending on the method of assessing RN, the rate of RN can vary widely from 7% (biopsy-proven) to 24% (imaging based) (20, 23). Hence, it is possible that our high rate of RN (60.9%) observed in our study could have been overestimated based on our primarily imaging-based diagnosis of RN. Biopsy was available for 10 of the 28 of those diagnosed with RN, of which all 10 were confirmed. If the rate of RN was calculated from biopsy-proven RN only, the rate would have been 21.7% which is still higher than those reported for biopsy-proven series (24, 25).
Although our ability to make a definitive diagnosis of RN versus recurrent tumor is currently based on histopathology, the pathology results can be limited by the variability of surgical site sampling which can give confounding results in the presence of both RN and tumor. To augment the current diagnostic capacity, attention has been focused on identifying the features and techniques that are important for making the distinction between RN and recurrence. For example, various imaging modalities using different sequences have been studied. Magnetic resonance spectroscopy, diffusion-weighted imaging, diffusion tensor imaging, and positron emission tomography (PET), among others, have surfaced as promising candidates for improving the current diagnostic rates (26, 27). Specifically for PET, fluciclovine is an amino acid radiotracer that has recently demonstrated good initial results for distinguishing RN from recurrent tumor among patients with brain metastases who were treated with SRS (28, 29). Furthermore, there also have been efforts to use radiomics and data mining to develop models that can effectively differentiate tumor from RN (30).
Significance of the Use of SRS and HER2-Directed Therapy on RN
BCBrM remains a significant challenge in the current era of improved extracranial disease control, owing to the lower efficacy of many systemic therapies in the brain (31). Given that HER2-driven cancers seem to preferentially metastasize to the brain, and the apparent brain-penetrance of some therapies (32, 33), we focused on the HER2-directed therapies. The effect of combination of HER2- directed therapies and SRS on overall survival/local control and RN is still unclear. For example, numerous studies have illustrated the benefit of concurrent use of lapatinib and SRS on overall survival and RN. Parsai et al. demonstrated that the use of lapatinib at any time of SRS administration was associated with improved overall survival (27.3 vs 19.5 months, p=0.03) with a lower risk of RN (1.3% vs 6.3% at 12 months, p<0.01) compared to those who received SRS without lapatinib in patients with HER2+ BCBrM (34). Miller et al. also showed that concurrent use of lapatinib/HER2-directed antibody treatment with SRS was associated with a lower 12-month cumulative incidence of RN (1.3% vs. 6.3%, p=0.001) compared to those who only received SRS. Furthermore, Kim et al. reported that the use of concurrent lapatinib with SRS did not increase the risk of RN compared to those who underwent SRS only (1.0% vs 3.5%, p=0.13) (35). On the other hand, the outcomes for T-DM1 are suboptimal. Carlson et al. showed in their case series that the overall rate of clinically significant RN among the patients in the treatment group who received SRS and T-DM1 was 57% (36). Geraud et al. also reported that RN was observed in 50% of patients who received T-DM1 concurrently with SRS compared to those who received T-DM1 sequentially to SRS (37). Thus, prior studies suggest that different agents (i.e. lapatinib as a tyrosine kinase inhibitor vs T-DM1 as an antibody drug conjugate) within the same class (HER2-directed) may have different risks on development of RN. While the current study was unable to assess differences between specific agents due to low numbers, this is a question warranting further exploration in future larger studies.
Although previous studies have looked into the effect of a specific therapy on the rate of RN for patients undergoing SRS, they have not considered the effect of different types or the number of systemic therapies (particularly HER2-directed therapies) on the development of RN during/following SRS. We found that a higher number (2 or more) of HER2-directed agents administered during SRS may increase the risk of development of RN. This result can be interpreted in two ways: 1) the toxicity of the HER2-directed therapies increases when used in combination and concurrently with SRS or 2) use of more HER2-directed therapies leads to longer survival and the observed higher rate of RN is a result of time bias. That is, there is an increased risk for RN in patients who live longer after their SRS treatment, allowing more time to observe the natural progression of RN (38). This is further supported by the observation that the diagnosis of RN was not made in the patients who deceased before their one-year follow-up. Nevertheless, our results suggest that timing and number of HER2-directed therapies matter when considering SRS for this patient population. These findings may be used to identify the group of patients with HER2+ BCBrMs with the highest risk for RN who would be candidates for preventative strategies in the future.
Limitations
Our study is limited by its retrospective design which is subject to selection and misclassification bias. Some patients with HER2+ BCBrM who received SRS and systemic therapy might not have been included in our cohort if they received their treatment outside of our institution and were screened out in the initial phase. Also, the seven patients who were diagnosed with RN via imaging only could have been misclassified because the difference between RN and local recurrence is difficult to discern with conventional imaging techniques. There could also be confounding factors that may be present that were not accounted for which could have affected the statistical significance of the results. Furthermore, our cohort is largely homogenous with regards to race which is predominantly Caucasian. Hence, the results may not be applicable to the greater population. Finally, the small sample size and diversity of treatment histories (e.g., the wide variety of systemic agents) contributed to the lack of power to detect statistical difference in the measured outcomes. The lack of adequate sample size also made it difficult to draw meaningful conclusions through logistic regression modeling.
Conclusion
Patients with HER2+ BCBrM who receive multiple agents of HER2-directed therapy during SRS for BCBrM may be at higher risk of RN. These data suggest during the eight-week window around SRS administration, if HER2-directed therapy is medically necessary, use of a single HER2-directed agent may lead to lower RN rates. Further investigation of next generation HER2-directed therapies, particularly comparing specific agents, in a larger cohort of patients will help refine best practices to minimize RN.
Data Availability Statement
The original contributions presented in the study are included in the article/supplementary material. Further inquiries can be directed to the corresponding author.
Ethics Statement
The studies involving human participants were reviewed and approved by Duke University Institutional Review Board (IRB). Written informed consent for participation was not required for this study in accordance with the national legislation and the institutional requirements.
Author Contributions
CP, AV, JK, SF, and CA were involved with the conception of the study. CP, EB, WG, and JH participated in analysis and interpretation of data. CP formulated the initial draft of the manuscript. All co-authors reviewed the final draft of the manuscript.
Funding
This study was funded by the Translating Duke Health Initiative as Carey K. Anders, MD is a Duke Health Scholar.
Conflict of Interest
JK reports research funding from Varian Medical Systems and the BioMimetix SBIR, consultant fees from Monteris and ownership of ClearSight RT, LLC. CA reports the following: Research funding: PUMA, Lilly, Merck, Seattle Genetics, Nektar, Tesaro, G1-Therapeutics, ZION, Novartis, Pfizer; Compensated consultant role: Genentech, Eisai, IPSEN, Seattle Genetics; Astra Zeneca, Novartis, Immunomedics, Elucida, Athenex; Royalties: UpToDate, Jones and Bartlet. SF reports the following: Salary support for unrelated research from: NIH R01 #NS1000866-04, American Cancer Society Research Scholar Grant #133394-RSG-19-030-01-DMC, NIH R38 #CA245204-02, and BioMimetix/NIH R44 #CA228694-02.
The remaining authors declare that the research was conducted in the absence of any commercial or financial relationships that could be construed as a potential conflict of interest.
Publisher’s Note
All claims expressed in this article are solely those of the authors and do not necessarily represent those of their affiliated organizations, or those of the publisher, the editors and the reviewers. Any product that may be evaluated in this article, or claim that may be made by its manufacturer, is not guaranteed or endorsed by the publisher.
References
1. Tong CWS, Wu M, Cho WCS, To KKW. Recent Advances in the Treatment of Breast Cancer. Front Oncol (2018) 8:227. doi: 10.3389/fonc.2018.00227
2. Quigley MR, Fukui O, Chew B, Bhatia S, Karlovits S. The Shifting Landscape of Metastatic Breast Cancer to the CNS. Neurosurg Rev (2013) 36(3):377–82. doi: 10.1007/s10143-012-0446-6
3. Tabouret E, Chinot O, Metellus P, Tallet A, Viens P, Goncalves A. Recent Trends in Epidemiology of Brain Metastases: An Overview. Anticancer Res (2012) 32(11):4655–62.
4. Kotecki N, Lefranc F, Devriendt D, Awada A. Therapy of Breast Cancer Brain Metastases: Challenges, Emerging Treatments and Perspectives. Ther Adv Med Oncol (2018) 10:1758835918780312. doi: 10.1177/1758835918780312
5. Lin NU, Gaspar LE, Soffietti R. Breast Cancer in the Central Nervous System: Multidisciplinary Considerations and Management. Am Soc Clin Oncol Educ Book (2017) 37:45–56. doi: 10.14694/EDBK_175338
6. Kennecke H, Yerushalmi R, Woods R, Speers CH, Nielsen TO, Gelmon K, et al. Metastatic Behavior of Breast Cancer Subtypes. J Clin Oncol Jul (2010) 28(20):3271–7. doi: 10.1200/JCO.2009.25.9820
7. Sammons S, Van Swearingen AED, Chung C, Anders CK. Advances in the Management of Breast Cancer Brain Metastases. Neurooncol Adv (2021) 3(Suppl 5):v63–74. doi: 10.1093/noajnl/vdab119
8. Nieder C, Grosu AL, Gaspar LE. Stereotactic Radiosurgery (SRS) for Brain Metastases: A Systematic Review. Radiat Oncol (2014) 9:155. doi: 10.1186/1748-717X-9-155
9. Sheline GE, Wara WM, Smith V. Therapeutic Irradiation and Brain Injury. Int J Radiat Oncol Biol Phys Sep (1980) 6(9):1215–28. doi: 10.1016/0360-3016(80)90175-3
10. Donovan EK, Parpia S, Greenspoon JN. Incidence of Radionecrosis in Single-Fraction Radiosurgery Compared With Fractionated Radiotherapy in the Treatment of Brain Metastasis. Curr Oncol (2019) 26(3):e328–33. doi: 10.3747/co.26.4749
11. Fokas E, Wacker U, Gross MW, Henzel M, Encheva E, Engenhart-Cabillic R. Hypofractionated Stereotactic Reirradiation of Recurrent Glioblastomas: A Beneficial Treatment Option After High-Dose Radiotherapy? Strahlenther Onkol (2009) 185(4):235–40. doi: 10.1007/s00066-009-1753-x
12. Sayan M, Mustafayev TZ, Balmuk A, Kefelioglu ESS, Gungot G, Chundury A, et al. Management of Symptomatic Radiation Necrosis After Stereotactic Radiosurgery and Clinical Factors for Treatment Response. Radiat Oncol J (2020) 38(3):176–80. doi: 10.3857/roj.2020.00171
13. Vellayappan B, Tan CL, Yong C, Khor LK, Koh WY, Yeo TT, et al. Diagnosis and Management of Radiation Necrosis in Patients With Brain Metastases. Front Oncol (2018) 8:395. doi: 10.3389/fonc.2018.00395
14. Nonoguchi N, Miyatake S, Fukumoto M, Kuroiwa T, Tsuji TK, Fukumoto TM, et al. The Distribution of Vascular Endothelial Growth Factor-Producing Cells in Clinical Radiation Necrosis of the Brain: Pathological Consideration of Their Potential Roles. J Neurooncol (2011) 105(2):423–31. doi: 10.1007/s11060-011-0610-9
15. Yoritsune E, Furuse M, Kuwabara H, Miyata T, Nonoguchi N, Kawabata S, et al. Inflammation as Well as Angiogenesis May Participate in the Pathophysiology of Brain Radiation Necrosis. J Radiat Res (2014) 55(4):803–11. doi: 10.1093/jrr/rru017
16. Giglio P, Gilbert MR. Cerebral Radiation Necrosis. Neurologist (2003) 9(4):180–8. doi: 10.1097/01.nrl.0000080951.78533.c4
17. Kargiotis O, Geka A, Rao JS, Kyritsis AP. Effects of Irradiation on Tumor Cell Survival, Invasion and Angiogenesis. J Neurooncol (2010) 100(3):323–38. doi: 10.1007/s11060-010-0199-4
18. Shah R, Vattoth S, Jacob R, Manzil FFP, O’Malley JP, Borghei P, et al. Radiation Necrosis in the Brain: Imaging Features and Differentiation From Tumor Recurrence. Radiographics (2012) 32(5):1343–59. doi: 10.1148/rg.325125002
19. Blonigen BJ, Steinmetz RD, Levin L, Lamba MA, Warnick RE, Breneman JC. Irradiated Volume as a Predictor of Brain Radionecrosis After Linear Accelerator Stereotactic Radiosurgery. Int J Radiat Oncol Biol Phys (2010) 77(4):996–1001. doi: 10.1016/j.ijrobp.2009.06.006
20. Minniti G, Clarke E, Lanzetta G, Osti MF, Trasimeni G, Bozzao A, et al. Stereotactic Radiosurgery for Brain Metastases: Analysis of Outcome and Risk of Brain Radionecrosis. Radiat Oncol (2011) 6(1):48. doi: 10.1186/1748-717X-6-48
21. Colaco RJ, Martin P, Kluger HM, Yu JB, Chiang VL. Does Immunotherapy Increase the Rate of Radiation Necrosis After Radiosurgical Treatment of Brain Metastases? J Neurosurg (2016) 125(1):17–23. doi: 10.3171/2015.6.JNS142763
22. Mudra S, Bhandari S, Tripathi P, Dunlap N, Kloecker G. Stereotactic Radiosurgery in the Era of Novel Systemic Therapy for Lung Cancer Brain Metastases. J Cancer Metastasis Treat (2019) 5(27):1–9. doi: 10.20517/2394-4722.2019.09
23. Chin LS, Ma L, DiBiase S. Radiation Necrosis Following Gamma Knife Surgery: A Case-Controlled Comparison of Treatment Parameters and Long-Term Clinical Follow Up. J Neurosurg (2001) 94(6):899–904. doi: 10.3171/jns.2001.94.6.0899
24. Shaw E, Scott C, Souhami L, Dinapoli R, Kline R, Loeffler J, et al. Single Dose Radiosurgical Treatment of Recurrent Previously Irradiated Primary Brain Tumors and Brain Metastases: Final Report of RTOG Protocol 90-05. Int J Radiat Oncol Biol Phys (2000) 47(2):291–8. doi: 10.1016/s0360-3016(99)00507-6
25. Sneed PK, Mendez J, Vemer-van den Hoek JGM, Seymour ZA, Ma L, Molinaro AM, et al. Adverse Radiation Effect After Stereotactic Radiosurgery for Brain Metastases: Incidence, Time Course, and Risk Factors. J Neurosurg JNS (2015) 123(2):373–86. doi: 10.3171/2014.10.Jns141610
26. Nael K, Bauer AH, Hormigo A, KLemole M, Germano IM, Puig J, et al. Multiparametric MRI for Differentiation of Radiation Necrosis From Recurrent Tumor in Patients With Treated Glioblastoma. Am J Roentgenol (2017) 210(1):18–23. doi: 10.2214/AJR.17.18003
27. Furuse M, Nonoguchi N, Yamada K, Shiga T, Combes JD, Ikeda N, et al. Radiological Diagnosis of Brain Radiation Necrosis After Cranial Irradiation for Brain Tumor: A Systematic Review. Radiat Oncol (2019) 14(1):28. doi: 10.1186/s13014-019-1228-x
28. Tom MC, DiFilippo F, Smile T, DiFilippo F, Jones S, Suh J, et al. 18f-Fluciclovine PET/CT to Distinguish Radiation Necrosis From Tumor Progression in Brain Metastases Treated With Stereotactic Radiosurgery: Results of a Prospective Pilot Study. Int J Radiat Oncol Biol Phys (2021) 111(Suppl 3):S27. doi: 10.1016/j.ijrobp.2021.07.089
29. Parent EE, Patel D, Nye JA, Li Z, Olson JJ, Schuster DM, et al. [(18)F]-Fluciclovine PET Discrimination of Recurrent Intracranial Metastatic Disease From Radiation Necrosis. EJNMMI Res (2020) 10(1):148–8. doi: 10.1186/s13550-020-00739-6
30. Park YW, Choi D, Park JE, Ahn SS, Kim H, Chang JH, et al. Differentiation of Recurrent Glioblastoma From Radiation Necrosis Using Diffusion Radiomics With Machine Learning Model Development and External Validation. Sci Rep (2021) 11(1):2913. doi: 10.1038/s41598-021-82467-y
31. Gori S, Rimondini S, De Angelis V, Colozza M, Bisagni G, Moretti G, et al. Central Nervous System Metastases in HER-2 Positive Metastatic Breast Cancer Patients Treated With Trastuzumab: Incidence, Survival, and Risk Factors. Oncologist (2007) 12(7):766–73. doi: 10.1634/theoncologist.12-7-766
32. Modi S, Saura C, Yamashita T, Park YH, Kim SB, Tamura K, et al. Trastuzumab Deruxtecan in Previously Treated HER2-Positive Breast Cancer. N Engl J Med (2019) 382(7):610–21. doi: 10.1056/NEJMoa1914510
33. Murthy RK, Loi S, Okines A, Paplomata E, Hamilton E, Hurvitz SA, et al. Tucatinib, Trastuzumab, and Capecitabine for HER2-Positive Metastatic Breast Cancer. N Engl J Med (2019) 382(7):597–609. doi: 10.1056/NEJMoa1914609
34. Parsai S, Miller JA, Juloori A, Chao ST, Kotecha R, Mohammadi AM, et al. Stereotactic Radiosurgery With Concurrent Lapatinib Is Associated With Improved Local Control for HER2-Positive Breast Cancer Brain Metastases. J Neurosurg JNS (2020) 132(2):503–11. doi: 10.3171/2018.10.Jns182340
35. Kim JM, Miller JA, Kotecha R, Chao ST, Ahluwalia MS, Peereboom DM, et al. Stereotactic Radiosurgery With Concurrent HER2-Directed Therapy Is Associated With Improved Objective Response for Breast Cancer Brain Metastasis. Neuro Oncol (2019) 21(5):659–68. doi: 10.1093/neuonc/noz006
36. Carlson JA, Nooruddin Z, Rusthoven C, Elias A, Borges VF, Diamond JR, et al. Trastuzumab Emtansine and Stereotactic Radiosurgery: An Unexpected Increase in Clinically Significant Brain Edema. Neuro Oncol (2014) 16(7):1006–9. doi: 10.1093/neuonc/not329
37. Geraud A, Xu HP, Beuzeboc P, Kirova YM. Preliminary Experience of the Concurrent Use of Radiosurgery and T-DM1 for Brain Metastases in HER2-Positive Metastatic Breast Cancer. J Neurooncol (2017) 131(1):69–72. doi: 10.1007/s11060-016-2265-z
Keywords: breast cancer, brain metastasis, stereotactic radiotherapy, systemic therapy, radiation necrosis
Citation: Park C, Buckley ED, Van Swearingen AED, Giles W, Herndon JE II , Kirkpatrick JP, Anders CK and Floyd SR (2022) Systemic Therapy Type and Timing Effects on Radiation Necrosis Risk in HER2+ Breast Cancer Brain Metastases Patients Treated With Stereotactic Radiosurgery. Front. Oncol. 12:854364. doi: 10.3389/fonc.2022.854364
Received: 13 January 2022; Accepted: 14 April 2022;
Published: 20 May 2022.
Edited by:
Ganesh Rao, University of Texas MD Anderson Cancer Center, United StatesReviewed by:
Alfredo Echeverria, Baylor College of Medicine, United StatesEric Lehrer, Icahn School of Medicine at Mount Sinai, United States
Copyright © 2022 Park, Buckley, Van Swearingen, Giles, Herndon, Kirkpatrick, Anders and Floyd. This is an open-access article distributed under the terms of the Creative Commons Attribution License (CC BY). The use, distribution or reproduction in other forums is permitted, provided the original author(s) and the copyright owner(s) are credited and that the original publication in this journal is cited, in accordance with accepted academic practice. No use, distribution or reproduction is permitted which does not comply with these terms.
*Correspondence: Scott R. Floyd, c2NvdHQuZmxveWRAZHVrZS5lZHU=
†These authors share senior authorship