- 1Department of General Surgery, Affiliated Hospital of Nantong University, Nantong, China
- 2Department of Gastroenterology, Affiliated Hospital of Nantong University, Nantong, China
- 3Department of Immunology, Medical College, Nantong University, Nantong, China
Background: Colony stimulating factor 1 receptor (CSF-1R) is a single channel III transmembrane receptor tyrosine kinase (RTK) and plays an important role in immune regulation and the development of various cancer types. The expression of CSF-1R in colon adenocarcinoma (COAD) and its prognostic value remain incompletely understood. Therefore, we aim to explore the prognostic value of CSF-1R in COAD and its relationship with tumor immunity.
Methods: CSF-1R expression in a COAD cohort containing 103 patients was examined using immunohistochemistry (IHC). The relationship between CSF-1R expression and clinicopathological parameters and prognosis was evaluated. Dual immunofluorescence staining was conducted to determine the localization of CSF-1R in COAD tissues. Univariate and multivariate Cox regression analysis were performed to evaluate independent prognostic factors. Transcriptomic profiles of CSF-1Rhigh and CSF-1Rlow tumor-associated macrophages (TAMs) were investigated. Gene enrichment analysis was used to explore the signal pathways related to CSF-1R. In addition, the relationship between CSF-1R in tumor microenvironment (TME) and tumor immunity was also studied.
Results: IHC analysis showed that CSF-1R was overexpressed in COAD, and higher expression was associated with shorter overall survival (OS). Immunofluorescence staining showed that CSF-1R was co-localized with macrophage marker CD68. Univariate and multivariate Cox regression analysis showed that CSF-1R was an independent prognostic factor for COAD. The results of gene enrichment analysis showed that CSF-1R was involved in tumor immune response and regulation of TME. In addition, CSF-1R was significantly correlated with TME, immune cell infiltration, TMB, MSI, Neoantigen, and immune checkpoint molecules.
Conclusion: CSF-1R can serve as an independent prognostic factor of COAD and promising immunotherapeutic target of COAD.
Introduction
According to the latest cancer statistics, Colorectal cancer (CRC) is the third most common cancer and the third leading cause of cancer-related mortality in the world (1). It is worth noting that the incidence and mortality rate of CRC have declined in recent decades (2), but the incidence rates for adolescents and young adults have been increasing steadily (3). The etiology of CRC is complicated, and attributed to assorted environmental and genetic factors, such as germline or sporadic genic mutations, obesity, sedentary lifestyle, unhealthy diets, alcohol drinking and smoking (4). Most of current therapies for CRC, including surgical resection and neoadjuvant chemotherapy, are ineffective to combat with advanced CRC (5, 6). Due to limited therapeutic options, the prognosis of advanced CRC remains poor, with a 5-year survival rate of 12% for stage IV CRC (7). Colon adenocarcinoma (COAD) is the most common type of CRC (8). Thus, there is unmet demand to develop new therapeutic agents against advanced COAD.
Immune evasion is a critical hallmark of solid cancer development (9). The establishment of immune suppressive tumor microenvironment (TME) is a prerequisite for the initiation and progression of a majority of solid tumors, including CRC (10, 11). The establishment of immunosuppressive environment involves a variety of cell types, such as tumor cells, immune cells, stromal cells. Stromal cells and immune cells are two key non-tumor cell populations in the TME. Stromal cells have been reported to drive CRC immune escape through the classical and non-classical secretory pathways (12, 13). Within tumor tissues, mesenchymal stromal cells (MSCs) are the key sources of assorted immunosuppressive cytokines, including TGF-β and IL-10, which modulates the functions of regulatory T cells (Treg) and cytotoxic T lymphocytes (CTLs) (14, 15). MSCs are heterogeneous populations and representative members of tumor-associated stromal cells include vascular endothelial cells (ECs) and cancer-associated fibroblasts (CAFs) (16). These cells may secret various cytokines, such as VEGF, CXCL1, CXCL2, IL-1 and IL-6 via hypoxia-inducible factor-1α (HIF-1α) and NF-κB pathways, leading to a tumor-promoting TME (17).
In addition to stromal cells, immune cells, such as tumor-associated macrophages (TAMs), natural killer T (NKT) cells, Treg and CTLs, are central components of immunosuppressive TME (18). TAMs are one of the most abundant cell populations within solid tumors and play key roles in the determination of tumor immune environment (19). TAMs may exert both anti-tumor and pro-tumor functions, depending on their activating pathways. While M1 classical activation mostly leads to an anti-tumor role of TAMs, alternatively activated TAMs typically promote immune evasion and tumor progression (20). Studies in recent years have characterized various TAM populations initiated by alternative activation, such as CD169+ TAMs and DC-SIGN+ TAMs and revealed their critical roles in tumor immune environment (21, 22). However, the role of different subgroups of TAMs in CRC development remains incompletely understood.
Colony-stimulating factor 1 receptor (CSF-1R) is a single channel III transmembrane receptor tyrosine kinase (RTK) and acts as a cell-surface receptor for colony-stimulating factor 1 (CSF-1) and interleukin 34 (IL34). CSF-1R signaling plays an indispensable role in the regulation of survival, proliferation and differentiation of macrophages and monocytes (23). Emerging data indicated that intratumor CSF-1/CSF-1R signaling can cause the recruitment of TAMs and the development of pro-tumor inflammatory environment, thereby leading to tumor growth and metastasis (24). Notably, CSF-1 has autocrine and paracrine manners in the TME, which adds a new layer of CSF-1R’s tumor-promoting role in malignant tumors (25). In addition to a role in regulating tumor immunity, CSF-1/CSF-1R axis also plays a key role in supporting tumor cell survival, proliferation and enhancing motility (26). Many studies have reported that the overexpression of CSF-1R is associated with the poor prognosis of many malignant tumors, including gastric cancer (27), breast cancer (28), renal cell carcinoma (29), etc. However, it remains controversial whether CSF-1/CSF-1R signaling mainly functions through regulating tumor immunity or tumor cell malignancy. Some studies indicated that CSF-1R is mainly expressed in tumor cells (29), while CSF-1R has also been reportedly expressed in TAMs and critically involved in tumor immune escape (30). Nevertheless, the expression and prognosis of CSF-1R and its relationship with tumor immunity in COAD are not clear.
In this study, we reported that CSF-1R expression was significantly more abundant in cancerous tissues than in adjacent cancerous tissues, and CSF-1R expression may serve as an independent prognostic predictor for worsened survival in patients with COAD. Immunofluorescence analysis revealed that CSF-1R was mainly expressed in TAMs of COAD. High levels of CSF-1Rhigh TAMs were associated with pro-tumor inflammatory environment. Our study provides a theoretical basis for targeting CSF-1R as an immunotherapeutic strategy against COAD.
Materials and Methods
Patients and Clinical Specimens
We conducted a retrospective study and recruited 103 COAD patients who underwent radical resection surgery of COAD in Nantong University Affiliated Hospital between 2010 and 2013. The clinicopathological and follow-up data of all patients were obtained and summarized in Table 1. All specimens of COAD were examined by two independent pathologists and according to the AJCC-stage version 7 system. The overall survival (OS) was defined as the time period between surgery and death or last follow-up. The research program was approved by the Ethics Committee of Nantong University Affiliated Hospital, and formal written consent was obtained from every patient.
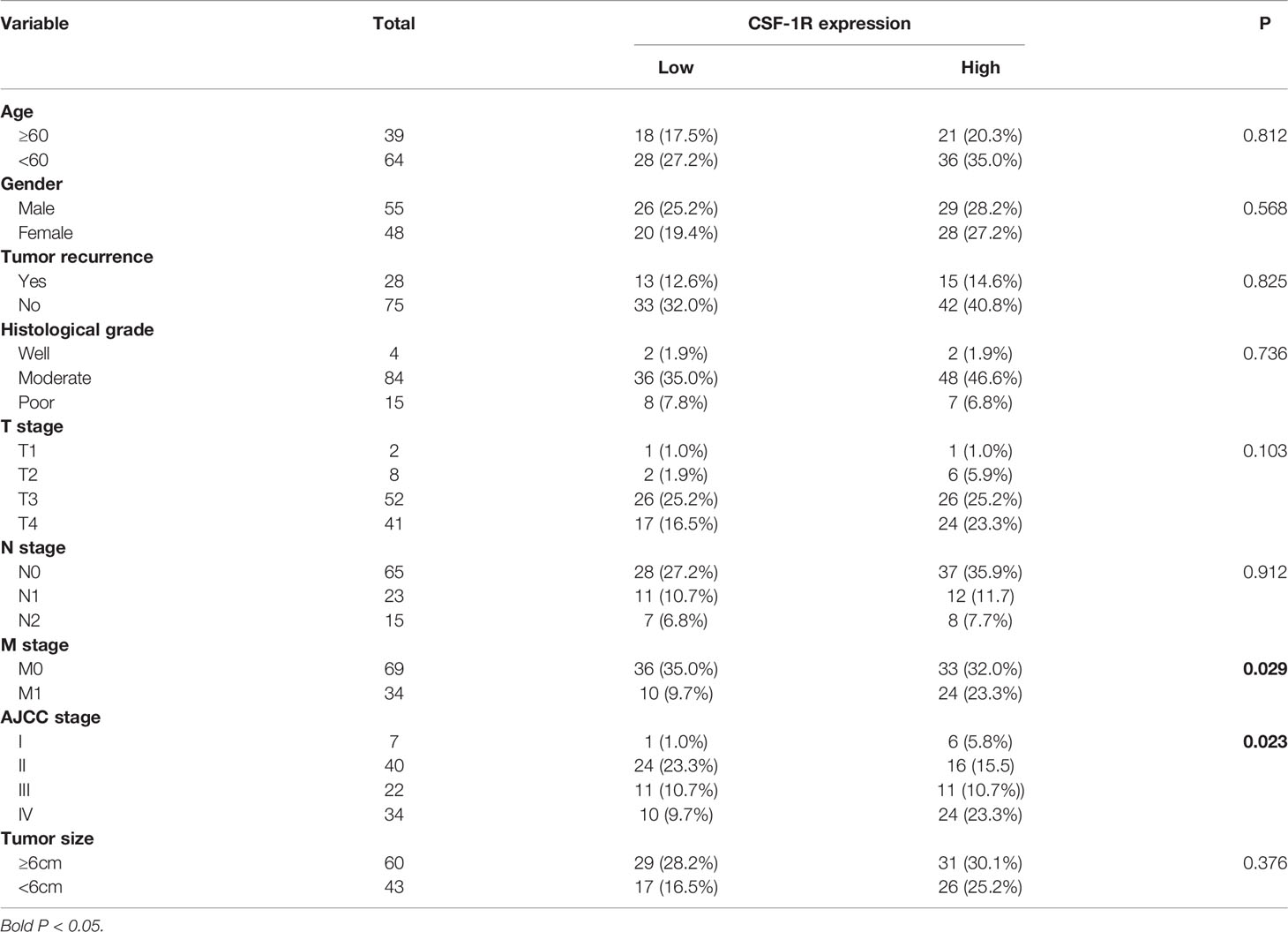
Table 1 Associations between CSF-1R expression and clinicopathological characteristics in 103 COAD patients.
Immunohistochemistry (IHC) and Immunofluorescence
Tissue microarrays (TMA) was prepared from COAD specimens by histological department of Nantong University Affiliated Hospital and subjected to immunohistochemistrical analysis. Briefly, TMAs were deparaffinized, hydrated, and heated to 121°C using an autoclave in sodium citrate buffer for 20 min to retrieve the antigen. Thereafter, the sections were blocked with 10% goat serum in 0.01M phosphate buffered saline (PBS) for 2h at room temperature, followed by incubation with an anti-CSF-1R rabbit polyclonal antibody (diluted 1:50, HPA012323 SIGMA) overnight at 4°C. The section was incubated with horseradish peroxidase (HRP)-conjugated sheep anti-rabbit IgG at 37°C for 30 min. The immunoreactivity was developed with DAB reagent (DAKO, Denmark). Finally, the slides were counterstained with hematoxylin. The immunostaining of each specimen was analyzed by two independent pathologists who were blinded to the patients’ clinic pathologic data. They evaluated the staining score of each specimen using the H score. The H score ranged from 0–300, multiplying the percentage of positive cells by the staining intensity (where 0, 1, 2, and 3 indicate negative, weak moderate, and strong staining, respectively). The best cutoff value for the staining score was selected with X-title Software. The patients were divided into two groups: CSF-1R-high (n = 57) and CSF-1R-low (n = 46).
For immunofluorescence staining, paraffin sections were deparaffinized, hydrated and antigen-retrieved, as mentioned above. Next, the sections were rinsed three times with PBS, and incubated with anti-CSF-1R antibody (1:50 dilution) and CD68 antibody (1:50 dilution, ab955 Abcam) overnight at 4°C. After washing using PBS for 3 times, and the slides were incubated with the following secondary antibodies for 1h at 37°C: Alexa Fluor488 goat anti-rabbit IgG (diluted 1:200, 111-545-144 Jackson); Alexa Fluor555 donkey anti-mouse IgG (diluted 1:300, A0453 Beyotime). Finally, the sections were mounted with Antifade Mounting solution containing 10μg/ml DAPI. Representative visual fields were acquired using a Leica DM5000 B microscope (Leica Microsystems, Wetzlar, Germany). Next, the fluorescence intensity of the CSF-1Rhigh TAMs in five different sections was examined and analyzed using the Image J software. Cells with immunostaining scores significantly higher (2.5-fold increase) than non TAMs were defined as CSF-1Rhigh TAMs.
Bioinformatics Analysis
The COAD expression data and corresponding clinical data were obtained from The Cancer Genome Atlas (TCGA) official website (http://gdc.cancer.gov). The TCGA data were all analyzed by the R software (version 4.1.0) and survival package. The expression data of CSF-1R in various CRC cells were obtained from the DepMap portal (https://depmap.org/portal/) database.
Preparation of Single Cell Suspension
We collected fresh specimens from five patients who underwent radical resection of COAD from the Nantong University Affiliated Hospital and obtained the informed consent of each patient. After the fresh tumor tissue clears the blood with normal saline, put into the tissue preservation solution (MACS Tissue Storage Solution, Miltenyi Biotec). Then put it on the ice and ship it to the laboratory. The tumor tissue was digested with human Tumor Dissociation Kit (Miltenyi biotec), and then the gentleMACS C test tube containing enzyme mixture and tissue was inverted and connected to gentleMACS Dissociator (31) (Miltenyi biotec) for mechanical dissociation. After dissociation, the sample was applied to a MACS SmartStrainer (30um) to remove any remaining larger particles from the single-cell suspension. Then wash cell MACS SmartStrainer with 20 mL of RPMI 1640. Finally, erythrocyte lysate was used to remove red blood cells. The prepared single cell suspension was used for flow cytometry analysis and cell sorting within 2 hours.
Flow Cytometry and RNA Sequencing
For fluorescence activated cell sorting (FACS), single cell suspension was prepared as described previously (22) from fresh COAD specimens and incubated with Human BD Fc Block (BD Bioscience). Then, the samples were stained with APCcy7 anti-CD45 (561863, BD bioscience), FITC anti-CD14 (555397, BD bioscience), and BV421 anti-CSF-1R (565347, BD bioscience) antibodies at 4°C for 30 min in dark. After washing cells 3 times with PBS, the cells were resuspended with staining buffer, and subjected to cell sorting using a BD FACS Aria3 flow cytometry. CSF-1Rlow TAMs (CD45+ CD14+ CSF-1Rlow) and CSF-1Rhigh TAMs (CD45+ CD14+ CSF-1Rhigh) were obtained from single-cell mixed suspension by flow cell sorting. Total RNA was isolated from the sorted samples using Qiagen RNeasy kit and subjected to cDNA library preparation using Smart-seq2 (32) scheme. The sequencing was performed using Illumina Novaseq6000 platform (33).
Sequence Analysis
We used R software (version 4.0.2) and Limma package (https://www.bioconductor.org/packages/release/bioc/html/limma.html) to analyze differentially expressed genes (DEGs) between CSF-1Rhigh TAMs and CSF-1Rlow TAMs and used ggplot2 and pheatmap packages to draw volcano map and heat map respectively. We selected | log2 (FC) | >1 and p <0.05 as the criteria for differential gene expression. Then, according to the changes of gene expression, DEGs were divided into two groups: up-regulated genes and down-regulated genes. The Gene Ontology (GO) and Kyoto Encyclopedia of Genes and Genomes (KEGG) enrichment analyses of DEGs were performed by DAVID (34) tool (https://david.ncifcrf.gov/tools.jsp).
Sanger Box Data Analysis Platform
We used Sanger Box data analysis platform (http://sangerbox.com/) and Pearson method to evaluate the correlation between CSF-1R expression and Tumor Mutation Burden (TMB), Microsatellite Instability (MSI) and Neoantigen. And Pearson’s correlation test was also used to evaluate the correlation between CSF-1R and immune checkpoint molecules.
Statistical Analysis
Statistical analyses were performed using spss20.0, GraphPad Prisim 8.0 and R 4.0.2. The relationships between CSF-1R expression and clinic pathological features were analyzed by Pearson’s chi-squared test. Survival analysis was performed using the Kaplan-Meier method and the logarithm rank tests. We used univariate and multivariate COX regression analysis to evaluate the correlation between different variables and OS. All the significance tests are bilateral, and bilateral p < 0.05 is considered statistically significant. We applied X-title (35) (Yale University version 3.6.1) to select the best cutoff point to evaluate biomarkers.
Result
CSF-1R Is Up-Regulated in COAD Tissues and Associated With Clinicopathological Parameters
We evaluated the expression of CSF-1R in 103 COAD specimens and 72 peritumoral specimens by IHC. The results showed that CSF-1R was largely absent in normal intestinal epithelial cells (Figure 1A). In contrast, abundant expression of CSF-1R was observed in COAD tissues. We employed H-score to evaluate the expression of CSF-1R in COAD tissues. The expression of CSF-1R was higher in tumor tissues than in peritumoral tissues (Figure 1B). The patients were divided into CSF-1R-high (n = 57) and CSF-1R-low (n = 46) using a H-score cutoff of 120. The correlations among CSF-1R expression and the clinicopathological parameters of COAD patients were evaluated (Table 1). CSF-1R expression was positively correlated with M stage (p=0.029) and AJCC stage (p=0.020). These data infer a role of CSF-1R in COAD progression.
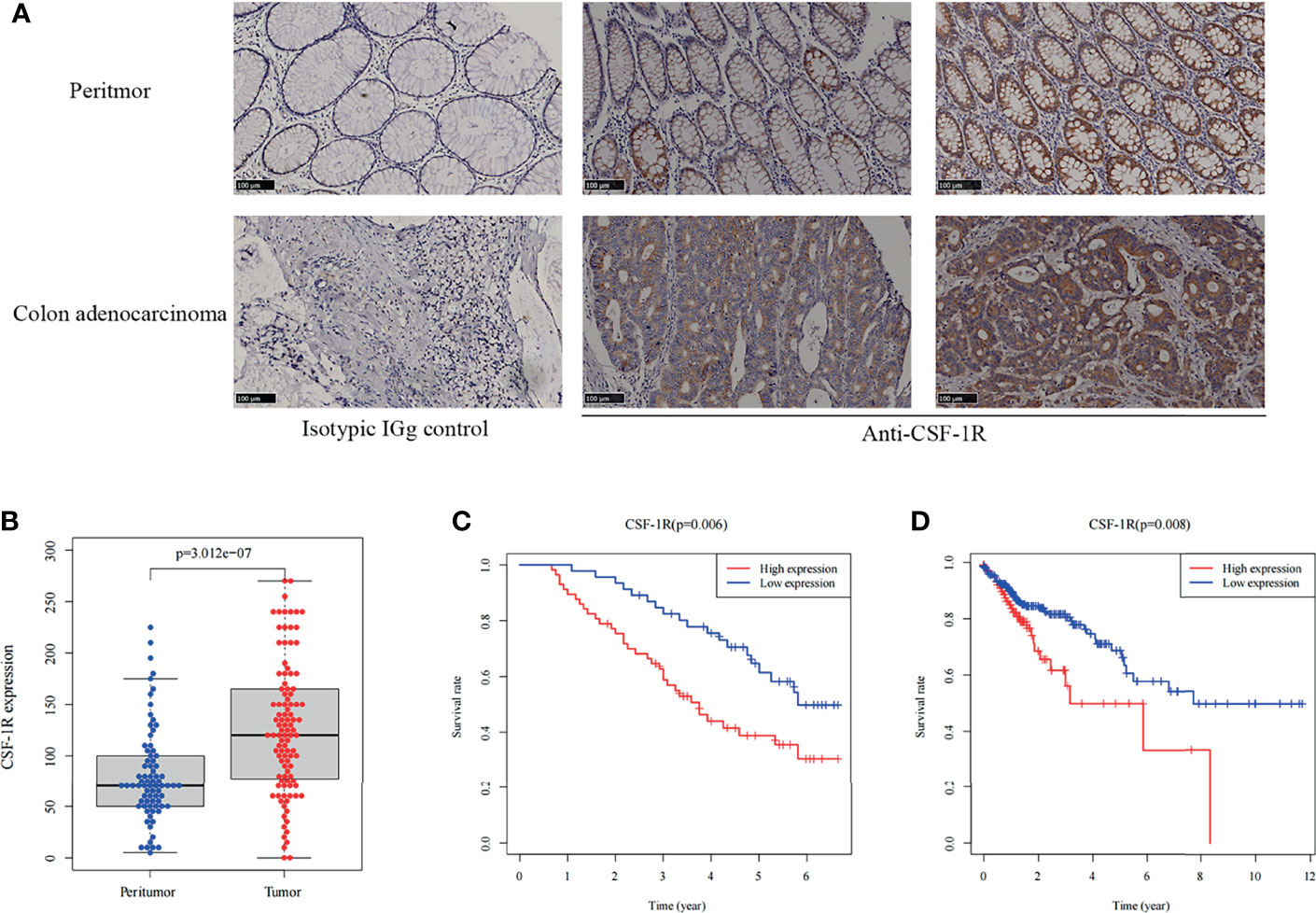
Figure 1 CSF-1R is highly expressed in COAD and associated with dismal prognosis. (A) Representative images of immunohistochemical in colon adenocarcinoma and peritumor tissues. (B) The boxplot of the CSF-1R expression between the tumor and peritumor tissues in 103 COAD patients. (C) Kaplan-Meier curves for low versus high CSF-1R expression in 103 COAD patients. (D) Kaplan-Meier curves for low versus high CSF-1R expression in TCGA COAD cohort.
CSF-1R Expression Predicts Poor Prognosis in COAD Patients From Two Independent Cohorts
In order to decipher the prognostic value of CSF-1R expression in COAD, we first investigated the survival difference between CSF-1R-low and CSF-1R-high patients using Kaplan-Meier survival analysis (Figure 1C). Compared with CSF-1R-low patients, patients with high expression of CSF-1R had significantly worsened prognosis. In addition to our own cohort, we also analyzed the prognostic value of CSF-1R in TCGA cohort. High expression of CSF-1R is also associated with unfavorable prognosis in COAD patients of TCGA cohort (Figure 1D). To determine whether CSF-1R expression and other clinicopathological variables can be used as independent prognostic indicators in patients with COAD, we also performed univariate and multivariate Cox regression analysis on 103 patients. As shown in Table 2, CSF-1R expression, along with tumor recurrence, AJCC-stage, N-stage, and M-stage could serve as an independent prognostic factor for patients with COAD.
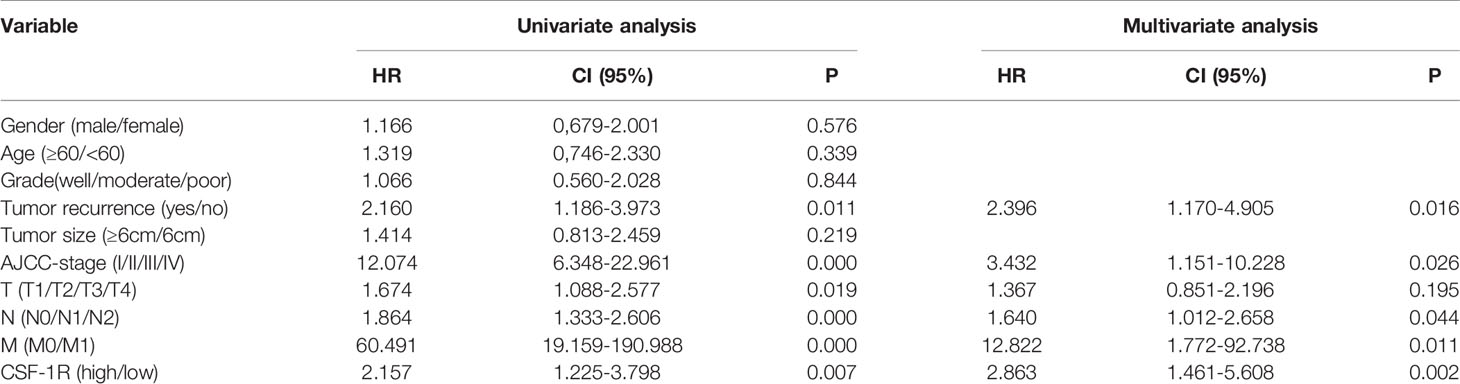
Table 2 Univariate and multivariate Cox regression analysis of clinicopathologic variables in 103 COAD patients.
Limited Expression and Perturbation Effects of CSF-1R in CRC Cells
Because the mechanisms by which CSF-1R promotes cancer development remain under debate, we aimed to clarify whether CSF-1R drives colorectal cancer progression via directly promoting tumor malignancy or facilitating pro-tumor inflammatory environment. To this end, we firstly analyzed whether CSF-1R is mainly expressed in CRC cells in COAD specimens. The expression profiles of CSF-1R in CRC lines and COAD tissues were obtained from Depmap portal and TCGA database, respectively. Notably, while TCGA data suggest abundant expression of CSF-1R in COAD tissues, substantially lower level of CSF-1R was identified in CRC lines, indicating that CSF-1R is not mainly expressed in tumor cells (Figure 2A). In agreement with these data, perturbation of CSF-1R does not cause strong growth retardation in CRC lines. CSF-1R may exhibit pro-proliferative or anti-proliferative effects in CRC cells, but these effects appear to be weak in all CRC lines (using depmap’s default perturbation effect cut-off of 0.5) (Figure 2B). These data suggest that CSF-1R facilitates COAD progression in a manner largely independent from its function within tumor cells.
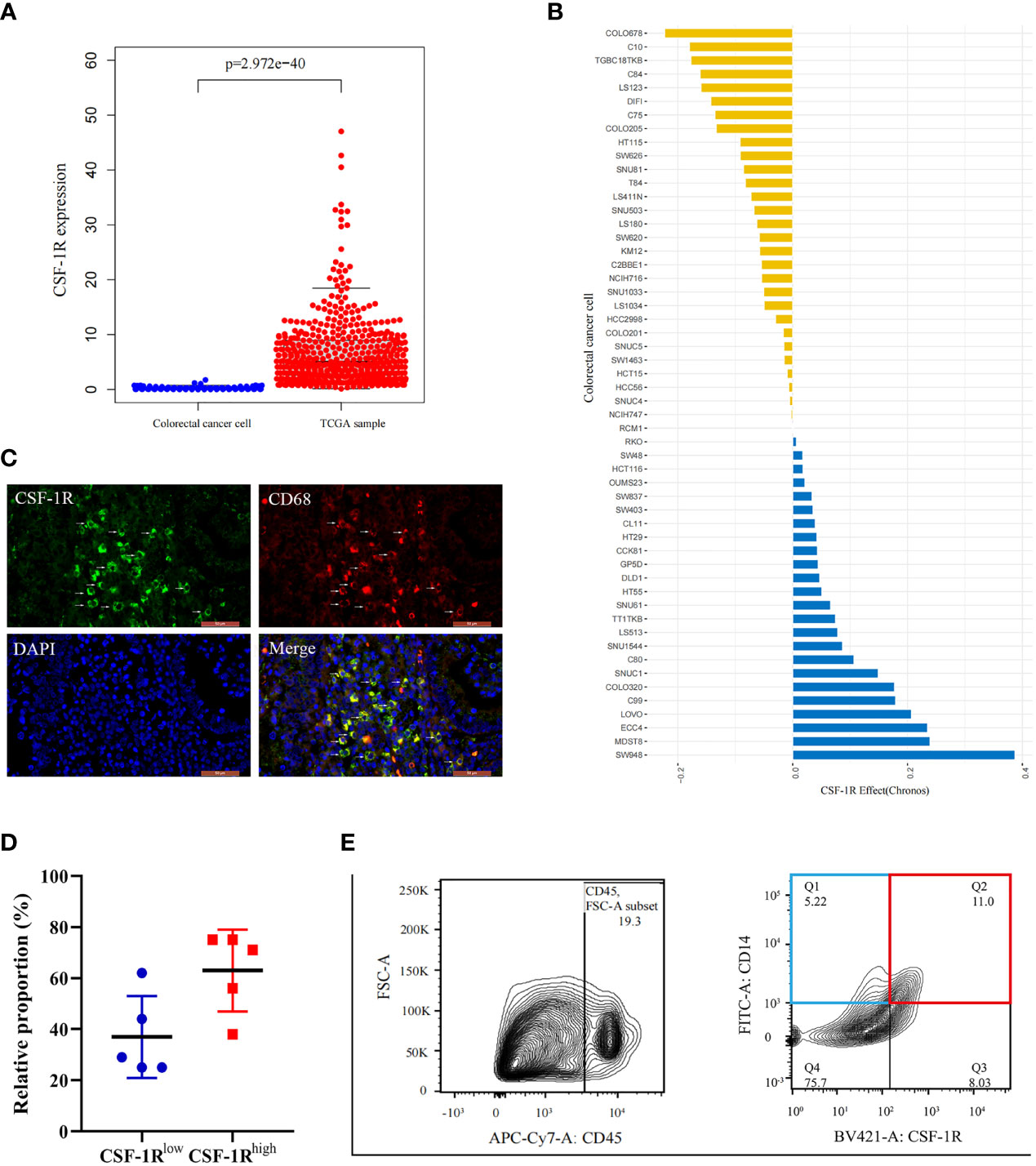
Figure 2 Characterization of CSF-1R distribution in COAD tissues and tis perturbation effects in CRC cells. (A).The boxplot shows the difference of CSF-1R expression between the TCGA samples and established colorectal cancer lines. (B) CSF-1R perturbation effects in CRC cells (A score of 0 is equivalent to a gene that is not essential whereas a score of -1 corresponds to the median of all common essential genes). (C) Immunofluorescence staining of CD68 and CSF-1R in COAD specimen. (D) The proportions of CSF-1Rlow TAMs and CSF-1Rhigh TAMs in COAD specimen. (E) Representative flow cytometric plot of the sorted CSF-1Rlow TAMs and CSF-1Rhigh TAMs.
CSF-1R Colocalizes With Macrophage Marker CD68
Mounting data suggest that CSF-1R is highly expressed in immune cells, particularly macrophages (30, 36). In order to verify whether CSF-1R is mainly expressed in macrophages in COAD, we performed immunofluorescence analysis to investigate the co-localization between CSF-1R and macrophage marker CD68. Immunofluorescence assay revealed a strong co-localization between CSF-1R and CD68 (Figure 2C). Therefore, we infer that CSF-1R is mainly expressed in TAMs in COAD specimens. In addition, the proportion of CSF-1Rhigh TAMs is typically higher than CSF-1Rlow TAMs in COAD specimens (Figure 2D). Based on these results, we speculate that CSF-1R may mainly regulate CRC immune environment through its expression in TAMs.
CSF-1Rhigh TAMs Is Involved in Multiple Tumor Immune Signaling Pathways
Next, we evaluated the role of CSF-1R in regulating TAM function and CRC immune environment. As shown in Figure 2E, we sorted CSF-1Rhigh TAMs and CSF-1Rlow TAMs using a BD FACS cell sorter and performed RNA sequencing (RNA-seq) to determine differentially expression genes (DEGs) between these two populations. According to the changes of gene expression, we identified 1298 up-regulated genes and 371 down-regulated genes. In order to explore the biological functions of these DEGs, we used DAVID tools to carry out GO and KEGG enrichment analysis. GO analysis showed that the up-regulated DEGs were mainly enriched in genes involved in inflammatory response, immune response and the regulation of TME and the down-regulated DEGs were strongly linked to respiratory chain and mitochondrial electron transport (Figures 3A, B). Likewise, KEGG analysis showed that pathways involved in immune response and tumor metabolism, such as Cytokine-cytokine receptor interaction, Toll-like receptor signaling pathway, PI3K-Akt signaling pathway and HIF-1 signaling pathway were enriched in upregulated DEGs (Figure 3C). However, down-regulation of DEGs mainly included pathways critical for some senile diseases and oxidative phosphorylation (Figure 3D). On the basis of the results of KEGG and GO, we speculate that CSF-1R may promote the development of COAD via modulating pro-tumor immune environments.
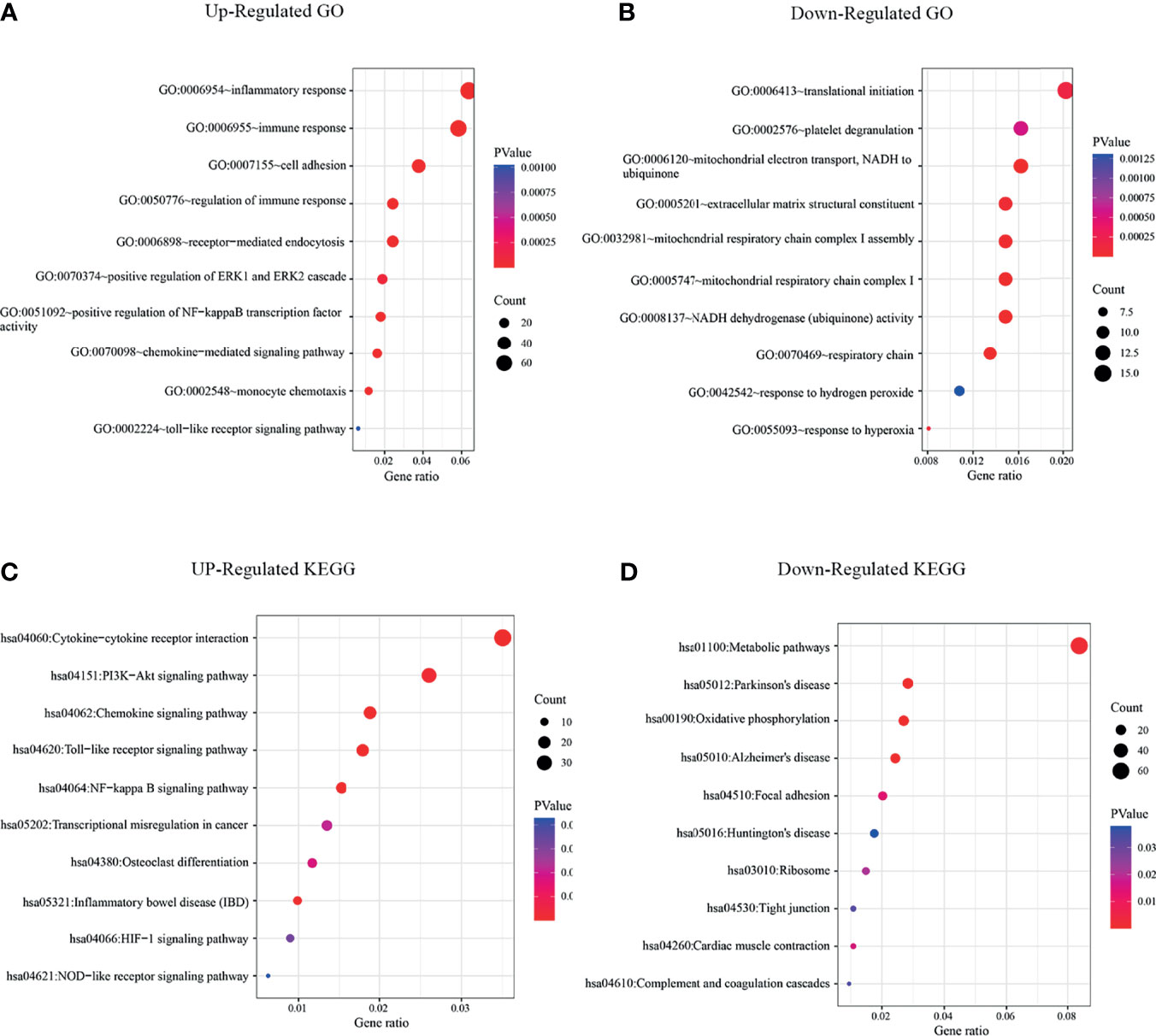
Figure 3 KEGG and GO enrichment analysis revealed that significant enrichments of immune signaling pathways in CSF-1Rhigh TAMs. (A) GO analysis of up-regulated signaling pathways in CSF-1Rhigh TAMs versus CSF-1Rlow TAMs. (B) GO analysis of down-regulated signaling pathways in CSF-1Rhigh TAMs versus CSF-1Rlow TAMs. (C) KEGG analysis of up-regulated signaling pathways in CSF-1Rhigh TAMs. (D) KEGG analysis of down-regulated signaling pathways in CSF-1Rhigh TAMs.
To validate this hypothesis, we explored the role of CSF-1R in tumor immunity in TCGA cohort by dividing patients into high CSF-1R expression and low CSF-1R expression. Using FDR<0.05 and NES>1.5 as cutoffs, we identified 10 signaling pathways that were significantly enriched in high CSF-1R expression, including Cytokine-cytokine receptor interaction, Chemokine signaling pathway, JAK-STAT signaling pathway, Toll-like receptor signaling pathway, B-cell receptor signaling pathway, T-cell receptor signaling pathway, Pathway in cancer, MAPK signaling pathway, VEGF signaling pathway, Colorectal cancer (Table 3 and Figure 4). These results suggest that high expression of CSF-1R is mainly involved in the regulation of immune microenvironment and tumor metabolism.
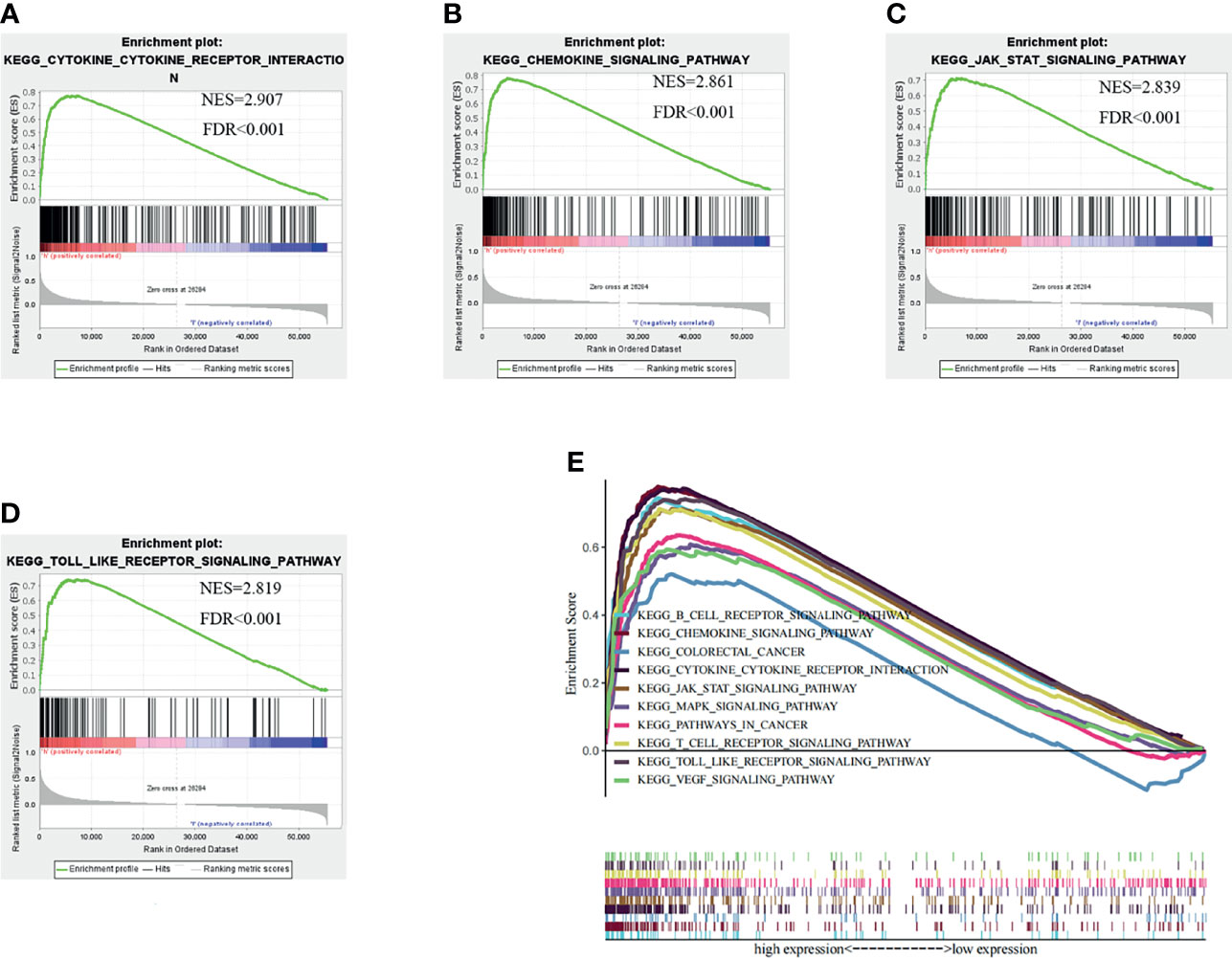
Figure 4 Gene set enrichment analysis (GSEA) results based on the CSF-1R expression in COAD from TCGA dataset. (A) Cytokine-Cytokine receptor interaction pathway. (B) Chemokine signaling pathway. (C) JAK-STAT signaling pathway. (D) Toll like receptor signaling pathway. (E) The ten significantly enriched signaling pathways based on their normalized enrichment score and the expression map.
CSF-1Rhigh TAMs Promotes COAD Progression by Modulating Tumor Immunity Environment
Immune cells and stromal cells are two main types of non-tumor components in TME and have been proposed to be valuable for tumor diagnosis and prognosis evaluation (37, 38). To further explore the relationship of CSF-1R and the TME, we used R software Estimate package to calculate the scores of immune cells, stromal cells and both of them, and then evaluated the associations among CSF-1R expression, immune cells, stromal cells and both of them. The results showed that CSF-1R expression was significantly associated with immune cell infiltration, stromal cell infiltration and both (Figure 5A). Therefore, we reasoned that CSF-1R mainly exerts pro-tumor functions in the TME. Then we analyzed the proportion of various immune cell types in the COAD by CIBERSORT rewinding calculation. We found that CSF-1R-high tumors contained more regulatory T cells (Tregs) and Macrophages M2 than CSF-1R-low tumors (Figure 5B). Of note, Tregs can inhibit the anti-tumor immune effects of DC cells, NK cells and effector T cells (Teff) through various mechanisms and are an important factor in the immunosuppressive TME (39). We reasoned that increased chemokines in CSF-1R tumors might drive enhanced Tregs recruitment. Therefore, we further analyzed the RNA sequencing results. Indeed, as shown in Figures 5C, D, a variety of Treg-recruiting cytokines, including CCL3, CCL4, CCL11, and CCL13 were upregulated in CSF-1Rhigh TAMs (40, 41). The above results suggest that CSF-1R + TAMs may inhibit anti-tumor immunity by recruiting Tregs to regulate COAD progression in the TME.
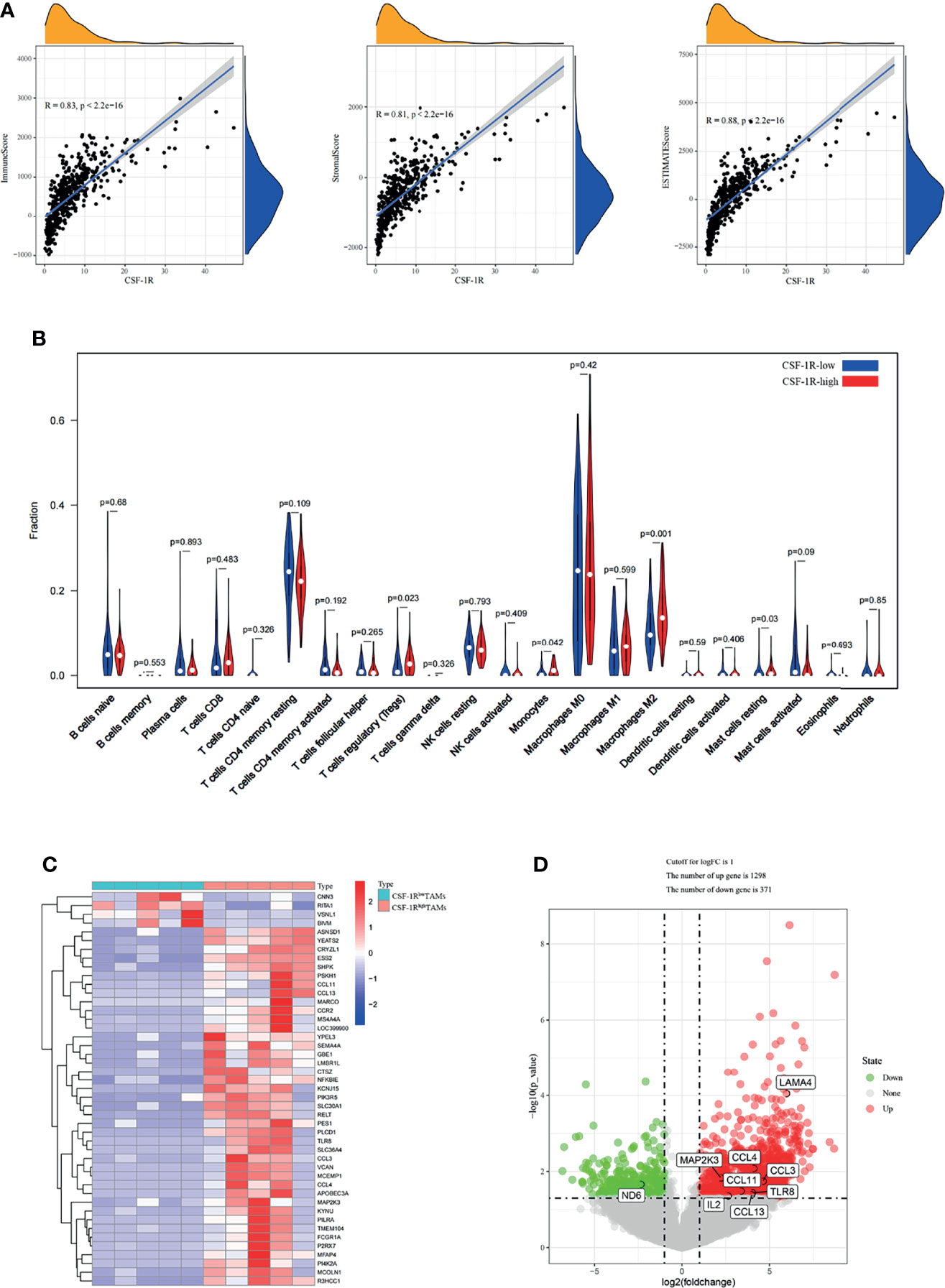
Figure 5 CSF-1Rhigh TAMs affect the immune landscape of colon adenocarcinoma. (A) Associations between CSF-1R expression and tumor microenvironment in COAD. (B) CIBERSORT analysis of the fractions of infiltrated immune cells in the COAD. (C) Heatmap of the partially differentially expressed genes between CSF-1Rlow TAMs and CSF-1Rhigh TAMs. (D) Volcano map of differentially expressed gene between CSF-1Rlow TAMs and CSF-1Rhigh TAMs. In the Volcano, p < 0.05 was set as the cut-off criterion of significant difference.
The Value of CSF-1R Expression in COAD Immunotherapy
To explore the potential associations between CSF-1R and other genes in COAD, We carried out PPI network analysis online through STRING website (https://string-db.org/). PPI network showed that 10 genes (CSF1, IL34, HRAS, GRB2, CBL, PIK3RI, TYROBP, STAP2, TNFSF11, and STAP2) were functionally related to CSF-1R (Figure 6A). So far, the value of targeting CSF-1R in COAD immunotherapy remains unclear. Previous studies have reported that TMB, MSI and Neoantigen can be used as biomarkers for the survival prognosis and immune checkpoint inhibitors’ efficacy (42–44). With the help of Sanger box website tool and Pearson method, we calculated the correlation between CSF-1R expression and TMB, MSI and Neoantigen. The results showed that the expression of CSF-1R in COAD was significantly correlated with TMB (p<0.001) (Figure 6B), MSI (p<0.001) (Figure 6C), and Neoantigen (p=0.004) (Figure 6D). In addition, we analyzed the relationship between CSF-1R and immune checkpoint molecules with the help of Sanger box website. We found that CSF-1R was significantly correlated with tumor-related immunosuppressive molecules including PDCD1, CTLA4, CD80, CD86, HAVCR2, etc. (Figure 7). These data indicates that CSF-1R may serve as a novel immunotherapeutic target for COAD.
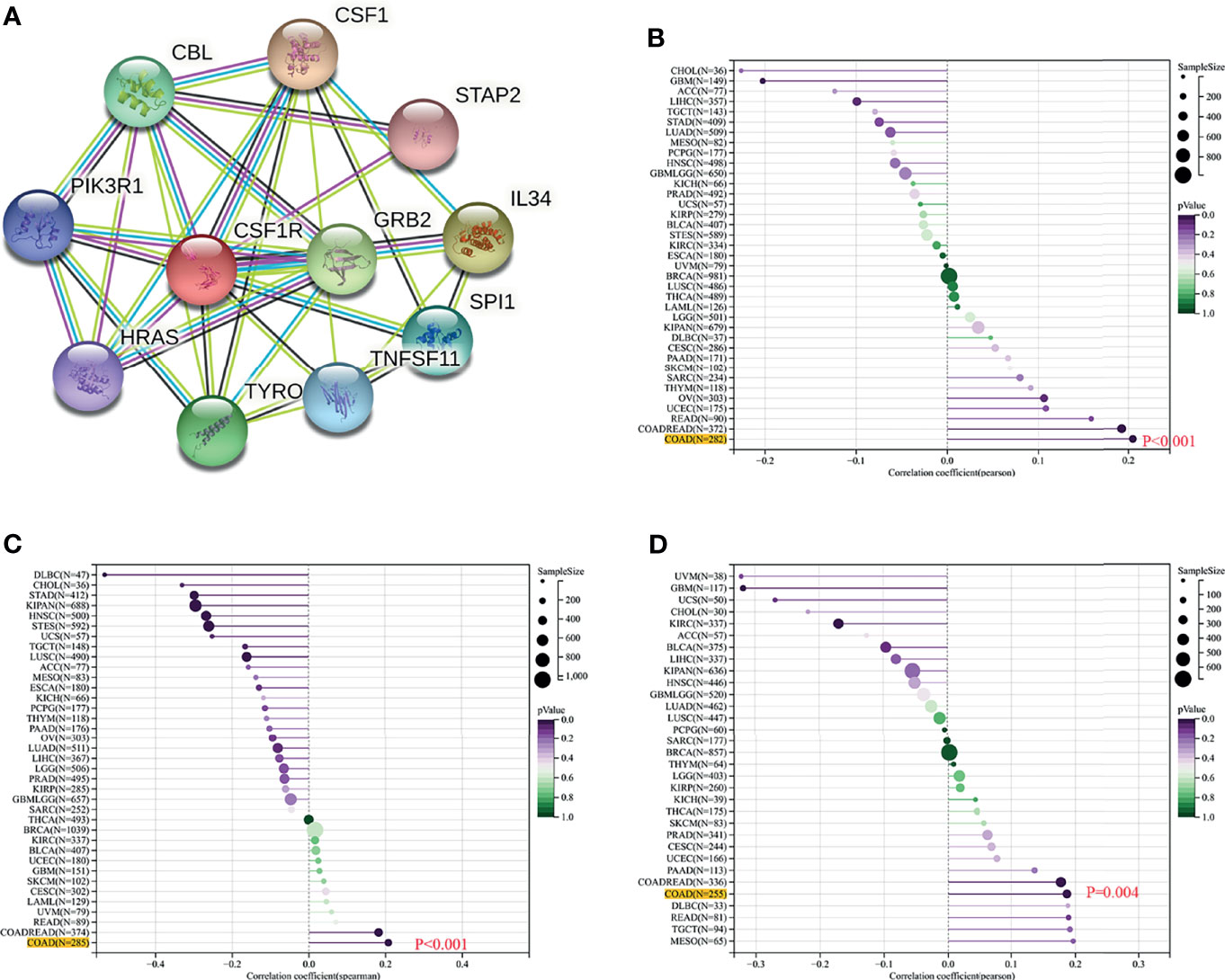
Figure 6 Value of CSF-1R in predicting the response to COAD immunotherapy. (A) PPI network based on CSF-1R expression. (B) Associations between CSF-1R expression and TMB. (C) Associations between CSF-1R expression and MSI. (D) Associations between CSF-1R expression and Neoantigen.
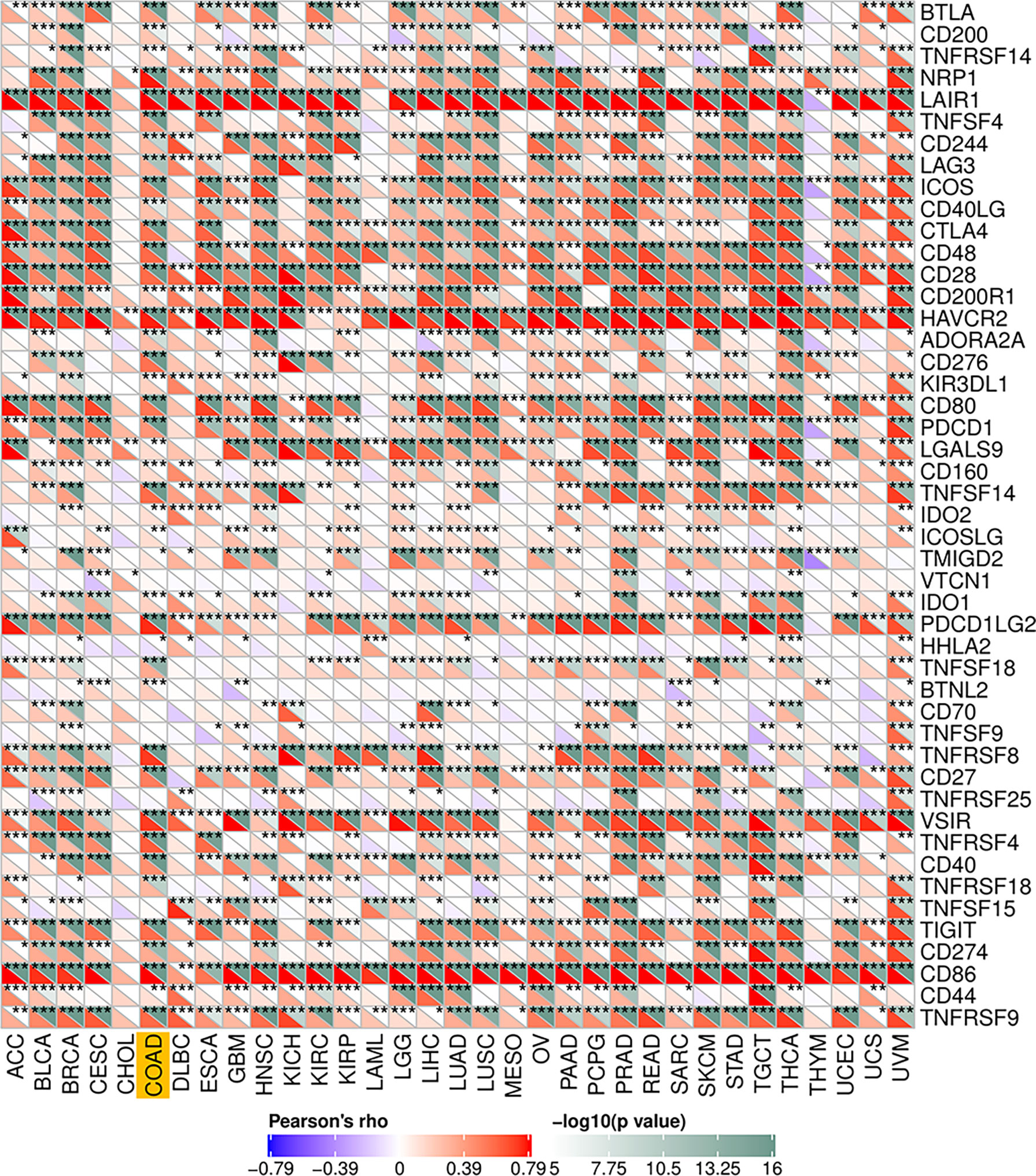
Figure 7 Associations between CSF-1R and immune checkpoint molecules. *P < 0.05, **P < 0.01, ***P < 0.001.
Discussion
Mounting studies have shown that CSF-1R overexpression leads to poor prognosis in various cancer types (29, 45). CSF-1R has been demonstrated to be an important player in the regulation of tumor immune responses. In this study, we firstly found that CSF-1R was largely up-regulated in COAD tissues, compared with para-carcinoma tissue. In addition, we observed that the high expression of CSF-1R was positively correlated with AJCC-stage and M-stage in COAD patients. Survival analysis showed that the OS of high-expression group was lower than that of low-expression group. Univariate and multivariate COX regression analysis confirmed that CSF-1R could serve as an independent risk index for poor prognosis in COAD patients. Moreover, we confirmed that CSF-1R mainly is expressed in COAD TAMs and contributes heavily to tumor immune environments. In summary, these results confirmed the important role of CSF-1R in the progression of COAD and emphasized the prognostic value of CSF-1R in COAD patients.
CSF-1R, as a member of the protein receptor tyrosine kinase (RTK) family, plays an important role in the development of solid tumors (46). While CSF-1R has been repeatedly used as a macrophage marker in normal tissues, recent studies suggested that blockade of CSF-1R does not abolish macrophage population in tumor tissues but alters macrophage polarization instead (47, 48). These data suggested that TAMs may not strictly rely on CSF-1R to survival. In addition, inhibition of CSF-1R may block M1 polarization and induces a M2 polarization, suggesting an involvement of CSF-1R signaling in TAM M1/M2 polarization (49). Data from other studies also revealed that CSF-1R expression varies greatly in macrophages (50). These studies indicated that CSF-1R may be preferentially expressed in a subpopulation of TAMs, and CSF-1R expression may influence the function of TAMs in TME. Previous studies indicated that CSF-1Rhigh TAMs can promote the occurrence of many tumors (24, 27). Our study findings indicated that CSF-1Rhigh TAMs were significantly correlated with Cytokine-cytokine receptor interaction, Chemokine signaling pathway, Toll-like receptor signaling pathway, PI3K-Akt signaling pathway, etc. Previous studies have shown that CXC chemokine family and its receptors can regulate tumor behavior by regulating angiogenesis, activating tumor specific immune response and stimulating tumor proliferation in an autocrine or paracrine manner (51). Moreover, it has been reported that increased levels of Toll-like receptors are related with the progression of colonic malignant tumors (52). What is more noteworthy is that CSF-1Rhigh TAMs may promote tumor immune escape by recruiting Tregs. Because tumor immune escape has been generally regarded as a key factor leading to COAD progress, we speculated that CSF-1Rhigh TAMs may contribute immune escape to promote COAD development.
To date, the value of targeting CSF-1R as an immunotherapeutic strategy in COAD remains unclear. It’s worth noting that CSF-1R expression was significantly correlated with TMB, MSI and Neoantigen in COAD. Furthermore, we found that CSF-1R was significantly correlated with tumor-related immunosuppressive molecules (including PDCD1, CTLA4, CD80, CD86, HAVCR2). PDCD1, also termed as PD1, is a member of the CD28-B7 family. The expression of PDCD1 on cancer cells is considered to be a key mechanism leading to tumor immune evasion (53). CTLA4, a key checkpoint for regulating autoimmune and antitumor responses, is an immune-suppressive receptor that plays an inhibitory role in T cell proliferation and activation (54). CTLA4 and PD1 play an important role in tumorigenesis and tumor immune tolerance and have been proved to be prognostic biomarkers for various cancer types (55). Studies have shown that PD1 and CTLA4 inhibitors have therapeutic potential in a variety of cancers, some of which have been approved for cancer treatments (56). For example, anti-CTLA4 therapy is the first immunotherapy approved by FDA (Food and Drug Administration), which has achieved significant results in metastatic melanoma (57). CD86 and CD80 are natural ligands of CTLA4, and CD80 has the potential to become the next generation of immunotherapeutic agents (58). HAVCR2 plays an inhibitory role in T cell-mediated immune response, and it is also widely regarded as a negative regulator of anti-tumor immunity, which expected to be an ideal target for the next generation of immunotherapy (59). The association of CSF-1R with these tumor immune checkpoint molecules indicates that CSF-1R may also serve as a valuable biomarker for predicting prognosis and an immunotherapeutic strategy against COAD.
Last, we admit that our research has several limitations. First, our studies were carried out using limited sample sizes, which may, to some extent, compromise the rigorousness of our conclusion. Secondly, the clinical information of TCGA database is limited, and the clinical information of individual samples is lost, which may affect our results. Finally, the molecular mechanisms underlying CSF-1R expression remain obscure. Indeed, our RNA-seq data indicated that CSF-1R may exhibit strong expression difference (fold change up to 100) between CSF-1Rhigh and CSF-1Rlow TAMs, underscoring complexity of the origination of the two TAM populations. Further studies are urgently needed to clarify the mechanism underpinning the regulation of CSF-1R expression in TAMs.
In summary, our study illustrates the prognostic value of CSF-1R in COAD, and CSF-1R can be regarded as an independent risk factor for the prognosis of COAD. Our study clarified that CSF-1R plays a critical role in COAD immune environment. CSF-1R is not mainly expressed in tumor cells and has very limited effects in directly regulating tumor malignancy in COAD. Instead, CSF-1R is strongly distributed in TAMs and CSF-1Rhigh TAMs represent a vital cell population in driving tumor immune tolerance. In addition, CSF-1Rhigh TAMs is involved in multiple immune response pathways and the recruitment of immune cells, such as Treg. These findings provide a theoretical basis for targeting CSF-1R as an immunotherapeutic strategy against COAD.
Data Availability Statement
The datasets presented in this study can be found in online repositories. The names of the repository/repositories and accession number(s) can be found below: https://www.ncbi.nlm.nih.gov/geo/, GSE193814.
Ethics Statement
The studies involving human participants were reviewed and approved by the Ethics Committee of Nantong University Affiliated Hospital. The patients/participants provided their written informed consent to participate in this study.
Author Contributions
XW and BH designed and performed the experiments. XW drafted the manuscript. FQ and JZ collected the clinical specimens and analyzed the data. FQ revised the manuscript. All authors agree to be accountable for the content of the work. All authors contributed to the article and approved the submitted version.
Funding
This study was supported by National Natural Science Foundation of China (81502057), and the Natural Science Foundation of the Jiangsu Higher Education (21KJB320016).
Conflict of Interest
The authors declare that the research was conducted in the absence of any commercial or financial relationships that could be construed as a potential conflict of interest.
Publisher’s Note
All claims expressed in this article are solely those of the authors and do not necessarily represent those of their affiliated organizations, or those of the publisher, the editors and the reviewers. Any product that may be evaluated in this article, or claim that may be made by its manufacturer, is not guaranteed or endorsed by the publisher.
Acknowledgments
We thank all the researchers for their participation and contributions.
References
1. Siegel RL, Miller KD, Fuchs HE, Jemal A. Cancer Statistics, 2021. CA: Cancer J Clin (2021) 71(1):7–33. doi: 10.3322/caac.21654
2. Miller KD, Siegel RL, Lin CC, Mariotto AB, Kramer JL, Rowland JH, et al. Cancer Treatment and Survivorship Statistics, 2016. CA: Cancer J Clin (2016) 66(4):271–89. doi: 10.3322/caac.21349
3. Connell LC, Mota JM, Braghiroli MI, Hoff PM. The Rising Incidence of Younger Patients With Colorectal Cancer: Questions About Screening, Biology, and Treatment. Curr Treat Options Oncol (2017) 18(4):23. doi: 10.1007/s11864-017-0463-3
4. Keum N, Giovannucci E. Global Burden of Colorectal Cancer: Emerging Trends, Risk Factors and Prevention Strategies. Nat Rev Gastroenterol Hepatol (2019) 16(12):713–32. doi: 10.1038/s41575-019-0189-8
5. Karpisheh V, Nikkhoo A, Hojjat-Farsangi M, Namdar A, Azizi G, Ghalamfarsa G, et al. Prostaglandin E2 as a Potent Therapeutic Target for Treatment of Colon Cancer. Prostaglandins Other Lipid Mediat (2019) 144:106338. doi: 10.1016/j.prostaglandins.2019.106338
6. Xu R, Zhou B, Fung PC, Li X. Recent Advances in the Treatment of Colon Cancer. Histol Histopathol (2006) 21(8):867–72. doi: 10.14670/hh-21.867
7. Miller KD, Nogueira L, Mariotto AB, Rowland JH, Yabroff KR, Alfano CM, et al. Cancer Treatment and Survivorship Statistics, 2019. CA: Cancer J Clin (2019) 69(5):363–85. doi: 10.3322/caac.21565
8. Chang K, Yuan C, Liu X. A New RBPs-Related Signature Predicts the Prognosis of Colon Adenocarcinoma Patients. Front Oncol (2021) 11:627504. doi: 10.3389/fonc.2021.627504
9. Mortezaee K. Immune Escape: A Critical Hallmark in Solid Tumors. Life Sci (2020) 258:118110. doi: 10.1016/j.lfs.2020.118110
10. Zhang L, Zhao Y, Dai Y, Cheng J-N, Gong Z, Feng Y, et al. Immune Landscape of Colorectal Cancer Tumor Microenvironment From Different Primary Tumor Location. Front Immunol (2018) 9:1578. doi: 10.3389/fimmu.2018.01578
11. Mei Y, Xiao W, Hu H, Lu G, Chen L, Sun Z, et al. Single-Cell Analyses Reveal Suppressive Tumor Microenvironment of Human Colorectal Cancer. Clin Transl Med (2021) 11(6):e422. doi: 10.1002/ctm2.422
12. O’Malley G, Heijltjes M, Houston AM, Rani S, Ritter T, Egan LJ, et al. Mesenchymal Stromal Cells (MSCs) and Colorectal Cancer: A Troublesome Twosome for the Anti-Tumour Immune Response? Oncotarget (2016) 7(37):60752–74. doi: 10.18632/oncotarget.11354
13. Endo E, Okayama H, Saito K, Nakajima S, Yamada L, Ujiie D, et al. A TGFβ-Dependent Stromal Subset Underlies Immune Checkpoint Inhibitor Efficacy in DNA Mismatch Repair-Deficient/Microsatellite Instability-High Colorectal Cancer. Mol Cancer Res (2020) 18(9):1402–13. doi: 10.1158/1541-7786.MCR-20-0308
14. García-Rocha R, Moreno-Lafont M, Mora-García ML, Weiss-Steider B, Montesinos JJ, Piña-Sánchez P, et al. Mesenchymal Stromal Cells Derived From Cervical Cancer Tumors Induce TGF-β1 Expression and IL-10 Expression and Secretion in the Cervical Cancer Cells, Resulting in Protection From Cytotoxic T Cell Activity. Cytokine (2015) 76(2):382–90. doi: 10.1016/j.cyto.2015.09.001
15. Yan Z, Zhuansun Y, Chen R, Li J, Ran P. Immunomodulation of Mesenchymal Stromal Cells on Regulatory T Cells and Its Possible Mechanism. Exp Cell Res (2014) 324(1):65–74. doi: 10.1016/j.yexcr.2014.03.013
16. Poggi A, Musso A, Dapino I, Zocchi MR. Mechanisms of Tumor Escape From Immune System: Role of Mesenchymal Stromal Cells. Immunol Lett (2014) 159(1-2):55–72. doi: 10.1016/j.imlet.2014.03.001
17. Erez N, Truitt M, Olson P, Arron ST, Hanahan D. Cancer-Associated Fibroblasts Are Activated in Incipient Neoplasia to Orchestrate Tumor-Promoting Inflammation in an NF-kappaB-Dependent Manner. Cancer Cell (2010) 17(2):135–47. doi: 10.1016/j.ccr.2009.12.041
18. Hui L, Chen Y. Tumor Microenvironment: Sanctuary of the Devil. Cancer Lett (2015) 368(1):7–13. doi: 10.1016/j.canlet.2015.07.039
19. Petty AJ, Yang Y. Tumor-Associated Macrophages: Implications in Cancer Immunotherapy. Immunotherapy (2017) 9(3):289–302. doi: 10.2217/imt-2016-0135
20. Chanmee T, Ontong P, Konno K, Itano N. Tumor-Associated Macrophages as Major Players in the Tumor Microenvironment. Cancers (2014) 6(3):1670–90. doi: 10.3390/cancers6031670
21. Hou X, Chen G, Zhao Y. Research Progress on CD169-Positive Macrophages in Tumors. Am J Transl Res (2021) 13(8):8589–97.
22. Hu B, Wang Z, Zeng H, Qi Y, Chen Y, Wang T, et al. Blockade of DC-SIGN Tumor-Associated Macrophages Reactivates Antitumor Immunity and Improves Immunotherapy in Muscle-Invasive Bladder Cancer. Cancer Res (2020) 80(8):1707–19. doi: 10.1158/0008-5472.CAN-19-2254
23. Wei S, Nandi S, Chitu V, Yeung YG, Yu W, Huang M, et al. Functional Overlap But Differential Expression of CSF-1 and IL-34 in Their CSF-1 Receptor-Mediated Regulation of Myeloid Cells. J Leukocyte Biol (2010) 88(3):495–505. doi: 10.1189/jlb.1209822
24. Koh YW, Park C, Yoon DH, Suh C, Huh J. CSF-1R Expression in Tumor-Associated Macrophages Is Associated With Worse Prognosis in Classical Hodgkin Lymphoma. Am J Clin Pathol (2014) 141(4):573–83. doi: 10.1309/ajcpr92tddfarisu
25. Patsialou A, Wyckoff J, Wang Y, Goswami S, Stanley ER, Condeelis JS. Invasion of Human Breast Cancer Cells In Vivo Requires Both Paracrine and Autocrine Loops Involving the Colony-Stimulating Factor-1 Receptor. Cancer Res (2009) 69(24):9498–506. doi: 10.1158/0008-5472.Can-09-1868
26. Achkova D, Maher J. Role of the Colony-Stimulating Factor (CSF)/CSF-1 Receptor Axis in Cancer. Biochem Soc Trans (2016) 44(2):333–41. doi: 10.1042/bst20150245
27. Chen D, Xiong L, Zhang L, Yu H, Xu Y, Wang M, et al. CSF1R is a Prognostic Biomarker and Correlated With Immune Cell Infiltration in the Gastric Cancer Microenvironment. Pharmacogenomics Pers Med (2021) 14:445–57. doi: 10.2147/pgpm.S301303
28. Richardsen E, Uglehus RD, Johnsen SH, Busund LT. Macrophage-Colony Stimulating Factor (CSF1) Predicts Breast Cancer Progression and Mortality. Anticancer Res (2015) 35(2):865–74.
29. Yang L, Liu Y, An H, Chang Y, Zhang W, Zhu Y, et al. High Expression of Colony-Stimulating Factor 1 Receptor Associates With Unfavorable Cancer-Specific Survival of Patients With Clear Cell Renal Cell Carcinoma. Ann Surg Oncol (2016) 23(3):1044–52. doi: 10.1245/s10434-015-4911-7
30. Inamura K, Shigematsu Y, Ninomiya H, Nakashima Y, Kobayashi M, Saito H, et al. CSF1R-Expressing Tumor-Associated Macrophages, Smoking and Survival in Lung Adenocarcinoma: Analyses Using Quantitative Phosphor-Integrated Dot Staining. Cancers (2018) 10(8):252. doi: 10.3390/cancers10080252
31. Jungblut M, Oeltze K, Zehnter I, Hasselmann D, Bosio A. Standardized Preparation of Single-Cell Suspensions From Mouse Lung Tissue Using the gentleMACS Dissociator. J Visual Exp: JoVE (2009) 29:1266. doi: 10.3791/1266
32. Picelli S, Björklund ÅK, Faridani OR, Sagasser S, Winberg G, Sandberg R. Smart-Seq2 for Sensitive Full-Length Transcriptome Profiling in Single Cells. Nat Methods (2013) 10(11):1096–8. doi: 10.1038/nmeth.2639
33. Modi A, Vai S, Caramelli D, Lari M. The Illumina Sequencing Protocol and the NovaSeq 6000 System. Methods Mol Biol (Clifton NJ) (2021) 2242:15–42. doi: 10.1007/978-1-0716-1099-2_2
34. Huang DW, Sherman BT, Tan Q, Kir J, Liu D, Bryant D, et al. DAVID Bioinformatics Resources: Expanded Annotation Database and Novel Algorithms to Better Extract Biology From Large Gene Lists. Nucleic Acids Res (2007) 35(Web Server issue):W169–75. doi: 10.1093/nar/gkm415
35. Camp RL, Dolled-Filhart M, Rimm DL. X-Tile: A New Bio-Informatics Tool for Biomarker Assessment and Outcome-Based Cut-Point Optimization. Clin Cancer Res (2004) 10(21):7252–9. doi: 10.1158/1078-0432.Ccr-04-0713
36. Ide H, Seligson DB, Memarzadeh S, Xin L, Horvath S, Dubey P, et al. Expression of Colony-Stimulating Factor 1 Receptor During Prostate Development and Prostate Cancer Progression. Proc Natl Acad Sci USA (2002) 99(22):14404–9. doi: 10.1073/pnas.222537099
37. Garcia-Gomez A, Rodríguez-Ubreva J, Ballestar E. Epigenetic Interplay Between Immune, Stromal and Cancer Cells in the Tumor Microenvironment. Clin Immunol (Orlando Fla) (2018) 196:64–71. doi: 10.1016/j.clim.2018.02.013
38. Dumauthioz N, Labiano S, Romero P. Tumor Resident Memory T Cells: New Players in Immune Surveillance and Therapy. Front Immunol (2018) 9:2076. doi: 10.3389/fimmu.2018.02076
39. Li C, Jiang P, Wei S, Xu X, Wang J. Regulatory T Cells in Tumor Microenvironment: New Mechanisms, Potential Therapeutic Strategies and Future Prospects. Mol Cancer (2020) 19(1):116. doi: 10.1186/s12943-020-01234-1
40. Mukaida N, Sasaki SI, Baba T. CCL4 Signaling in the Tumor Microenvironment. Adv Exp Med Biol (2020) 1231:23–32. doi: 10.1007/978-3-030-36667-4_3
41. Wang R, Huang K. CCL11 Increases the Proportion of CD4+CD25+Foxp3+ Treg Cells and the Production of IL−2 and TGF−β by CD4+ T Cells via the STAT5 Signaling Pathway. Mol Med Rep (2020) 21(6):2522–32. doi: 10.3892/mmr.2020.11049
42. Chan TA, Yarchoan M, Jaffee E, Swanton C, Quezada SA, Stenzinger A, et al. Development of Tumor Mutation Burden as an Immunotherapy Biomarker: Utility for the Oncology Clinic. Ann Oncol (2019) 30(1):44–56. doi: 10.1093/annonc/mdy495
43. Boland CR, Goel A. Microsatellite Instability in Colorectal Cancer. Gastroenterology (2010) 138(6):2073–87.e3. doi: 10.1053/j.gastro.2009.12.064
44. Desrichard A, Snyder A, Chan TA. Cancer Neoantigens and Applications for Immunotherapy. Clin Cancer Res (2016) 22(4):807–12. doi: 10.1158/1078-0432.Ccr-14-3175
45. Huang L, Xu X, Hao Y, Chen J, Li L, Cheng J, et al. Overexpression of CSF-1R in Nasopharyngeal Carcinoma. Romanian J Morphol Embryol = Rev Roumaine Morphol Embryol (2015) 56(4):1279–83.
46. Murga-Zamalloa C, Rolland DCM, Polk A, Wolfe A, Dewar H, Chowdhury P, et al. Colony-Stimulating Factor 1 Receptor (CSF1R) Activates AKT/mTOR Signaling and Promotes T-Cell Lymphoma Viability. Clin Cancer Res (2020) 26(3):690–703. doi: 10.1158/1078-0432.Ccr-19-1486
47. Ao J-Y, Zhu X-D, Chai Z-T, Cai H, Zhang Y-Y, Zhang K-Z, et al. Colony-Stimulating Factor 1 Receptor Blockade Inhibits Tumor Growth by Altering the Polarization of Tumor-Associated Macrophages in Hepatocellular Carcinoma. Mol Cancer Ther (2017) 16(8):1544–54. doi: 10.1158/1535-7163.MCT-16-0866
48. Pyonteck SM, Akkari L, Schuhmacher AJ, Bowman RL, Sevenich L, Quail DF, et al. CSF-1R Inhibition Alters Macrophage Polarization and Blocks Glioma Progression. Nat Med (2013) 19(10):1264–72. doi: 10.1038/nm.3337
49. Du X, Xu Y, Chen S, Fang M. Inhibited CSF1R Alleviates Ischemia Injury via Inhibition of Microglia M1 Polarization and NLRP3 Pathway. Neural Plast (2020) 2020:8825954. doi: 10.1155/2020/8825954
50. Rossetti S, Van Unen L, Touw IP, Hoogeveen AT, Sacchi N. Myeloid Maturation Block by AML1-MTG16 Is Associated With Csf1r Epigenetic Downregulation. Oncogene (2005) 24(34):5325–32. doi: 10.1038/sj.onc.1208651
51. Cabrero-de Las Heras S, Martínez-Balibrea E. CXC Family of Chemokines as Prognostic or Predictive Biomarkers and Possible Drug Targets in Colorectal Cancer. World J Gastroenterol (2018) 24(42):4738–49. doi: 10.3748/wjg.v24.i42.4738
52. Omrane I, Baroudi O, Kourda N, Bignon YJ, Uhrhammer N, Desrichard A, et al. Positive Link Between Variant Toll-Like Receptor 4 (Asp299Gly and Thr399Ile) and Colorectal Cancer Patients With Advanced Stage and Lymph Node Metastasis. Tumour Biol: J Int Soc Oncodevelopmental Biol Med (2014) 35(1):545–51. doi: 10.1007/s13277-013-1075-6
53. Fathi F, Zamani B, Piroozmand A, Mozafarpoor S, Seyedhashemi E, ArefNezhad R, et al. Programmed Cell Death 1 (PDCD1) Gene Haplotypes and Susceptibility of Patients to Basal Cell Carcinoma. Mol Biol Rep (2021) 48(3):2047–52. doi: 10.1007/s11033-020-06115-w
54. Khailaie S, Rowshanravan B, Robert PA, Waters E, Halliday N, Badillo Herrera JD, et al. Characterization of CTLA4 Trafficking and Implications for Its Function. Biophys J (2018) 115(7):1330–43. doi: 10.1016/j.bpj.2018.08.020
55. Liu JN, Kong XS, Huang T, Wang R, Li W, Chen QF. Clinical Implications of Aberrant PD-1 and CTLA4 Expression for Cancer Immunity and Prognosis: A Pan-Cancer Study. Front Immunol (2020) 11:2048. doi: 10.3389/fimmu.2020.02048
56. Darvin P, Toor SM, Sasidharan Nair V, Elkord E. Immune Checkpoint Inhibitors: Recent Progress and Potential Biomarkers. Exp Mol Med (2018) 50(12):1–11. doi: 10.1038/s12276-018-0191-1
57. Leven C, Padelli M, Carré JL, Bellissant E, Misery L. Immune Checkpoint Inhibitors in Melanoma: A Review of Pharmacokinetics and Exposure-Response Relationships. Clin Pharmacokinet (2019) 58(11):1393–405. doi: 10.1007/s40262-019-00789-7
58. de Vos L, Grünwald I, Bawden EG, Dietrich J, Scheckenbach K, Wiek C, et al. The Landscape of CD28, CD80, CD86, CTLA4, and ICOS DNA Methylation in Head and Neck Squamous Cell Carcinomas. Epigenetics (2020) 15(11):1195–212. doi: 10.1080/15592294.2020.1754675
Keywords: CSF-1R, colon adenocarcinoma, prognosis, pathway, tumor immunity
Citation: Wang X, Zhang J, Hu B and Qian F (2022) High Expression of CSF-1R Predicts Poor Prognosis and CSF-1Rhigh Tumor-Associated Macrophages Inhibit Anti-Tumor Immunity in Colon Adenocarcinoma. Front. Oncol. 12:850767. doi: 10.3389/fonc.2022.850767
Received: 08 January 2022; Accepted: 10 March 2022;
Published: 04 April 2022.
Edited by:
Kevin Dzobo, University of Cape Town, South AfricaReviewed by:
Salvatore J. Coniglio, Kean University, United StatesMelvin Anyasi Ambele, University of Pretoria, South Africa
Copyright © 2022 Wang, Zhang, Hu and Qian. This is an open-access article distributed under the terms of the Creative Commons Attribution License (CC BY). The use, distribution or reproduction in other forums is permitted, provided the original author(s) and the copyright owner(s) are credited and that the original publication in this journal is cited, in accordance with accepted academic practice. No use, distribution or reproduction is permitted which does not comply with these terms.
*Correspondence: Fei Qian, cWlhbmZlaW50QDE2My5jb20=; Baoying Hu, aHVieTg2QG50dS5lZHUuY24=
†These authors have contributed equally to this work and share first authorship