- 1Department of Neurohabilitation, Oslo University Hospital, Oslo, Norway
- 2Institute of Clinical Medicine, University of Oslo, Oslo, Norway
- 3Norwegian Defence Research Establishment (FFI), Kjeller, Norway
- 4Department of Pathology, Oslo University Hospital, Oslo, Norway
- 5Division of Cancer Medicine, Oslo University Hospital, Oslo, Norway
- 6Research Institute of Internal Medicine, Oslo University Hospital, Oslo, Norway
- 7Department of Neurosurgery, Oslo University Hospital, Oslo, Norway
Cystic glioblastomas are aggressive primary brain tumors that may both destroy and displace the surrounding brain tissue as they grow. The mechanisms underlying these tumors’ destructive effect could include exposure of brain tissue to tumor-derived cytokines, but quantitative cytokine data are lacking. Here, we provide quantitative data on leukocyte markers and cytokines in the cyst fluid from 21 cystic glioblastomas, which we compare to values in 13 brain abscess pus samples. The concentration of macrophage/microglia markers sCD163 and MCP-1 was higher in glioblastoma cyst fluid than in brain abscess pus; lymphocyte marker sCD25 was similar in cyst fluid and pus, whereas neutrophil marker myeloperoxidase was higher in pus. Median cytokine levels in glioblastoma cyst fluid were high (pg/mL): TNF-α: 32, IL-6: 1064, IL-8: 23585, tissue factor: 28, the chemokine CXCL1: 639. These values were not significantly different from values in pus, pointing to a highly pro-inflammatory glioblastoma environment. In contrast, levels of IFN-γ, IL-1β, IL-2, IL-4, IL-10, IL-12, and IL-13 were higher in pus than in glioblastoma cyst fluid. Based on the quantitative data, we show for the first time that the concentrations of cytokines in glioblastoma cyst fluid correlate with blood leukocyte levels, suggesting an important interaction between glioblastomas and the circulation. Preoperative MRI of the cystic glioblastomas confirmed both destruction and displacement of brain tissue, but none of the cytokine levels correlated with degree of brain tissue displacement or peri-tumoral edema, as could be assessed by MRI. We conclude that cystic glioblastomas are highly pro-inflammatory environments that interact with the circulation and that they both displace and destroy brain tissue. These observations point to the need for neuroprotective strategies in glioblastoma therapy, which could include an anti-inflammatory approach.
Introduction
Glial progenitor cells may give rise to malignant tumors ranging from the highly differentiated, low-grade astrocytomas to the undifferentiated, rapidly growing glioblastomas (1, 2); glioblastoma carries a median survival time of months only (3, 4). Because of the limitations to tumor expansion imposed by the rigid skull, it is often assumed that glioblastomas grow by destroying brain tissue (5–8) in addition to displacing it (4, 9). Indeed, several lines of evidence point to a destructive effect of glioblastomas on the surrounding brain tissue. Destruction of white matter tracts (in which glioblastomas tend to reside) has been shown with MRI-based diffusion tensor imaging (10), and loss of the neuronal marker N-acetyl-aspartate from white matter that has been infiltrated by glioblastoma has been shown with magnetic resonance spectroscopy (11). In agreement, glioblastoma patients have high circulating levels of neurofilament light chain, a marker of neuronal damage (12). Histologically, glioblastomas are seen to grow by invading the surrounding brain tissue (1, 9, 13), but the original brain tissue is hardly present within the tumor, in line with a destructive effect of glioblastomas on the brain tissue that has been invaded. A destructive or toxic effect of glioblastoma on neural cells has been replicated experimentally by several research groups (14–16). To our knowledge, no study has attempted to distinguish between destruction and displacement of brain tissue on pre-surgical MRIs of glioblastomas. This would be clinically valuable information, which may help predict functional outcome after surgery and thus guide patient information prior to surgery.
In principle, glioblastomas may cause destruction of normal brain tissue through a variety of processes that encompass an inflammatory response, physical strain due to the tissue distortion caused by the tumor, ischemia due to compression of vasculature or the inadequacy of the neovasculature established in the course of tumor growth, and exposure of the surrounding brain tissue to neuroactive or neurotoxic compounds. The last decades have seen the identification of several such glioblastoma-derived neuroactive compounds, including various cytokines (17, 18), glutamate (15, 19, 20), matrix metalloproteinases (21), hormones such as androgens, insulin, and erythropoietin (22–24), micro-RNAs in extracellular vesicles (25, 26), and extracellular nanotubes (27). Thus, glioblastomas may influence the surrounding brain tissue in a multi-modal fashion (25). However, the clinical importance of the various factors is not fully known, in part because of the practical difficulties of obtaining human material that allows not only the detection of such compounds, but also their quantification. One approach to solving this problem is offered by the fact that glioblastomas may have cystic compartments (28, 29). The cyst fluid is in close contact with both tumor cells and the surrounding brain tissue (Figure 1). Cyst fluid is aspirated during neurosurgery and lends itself to the quantitative study of the glioblastoma environment.
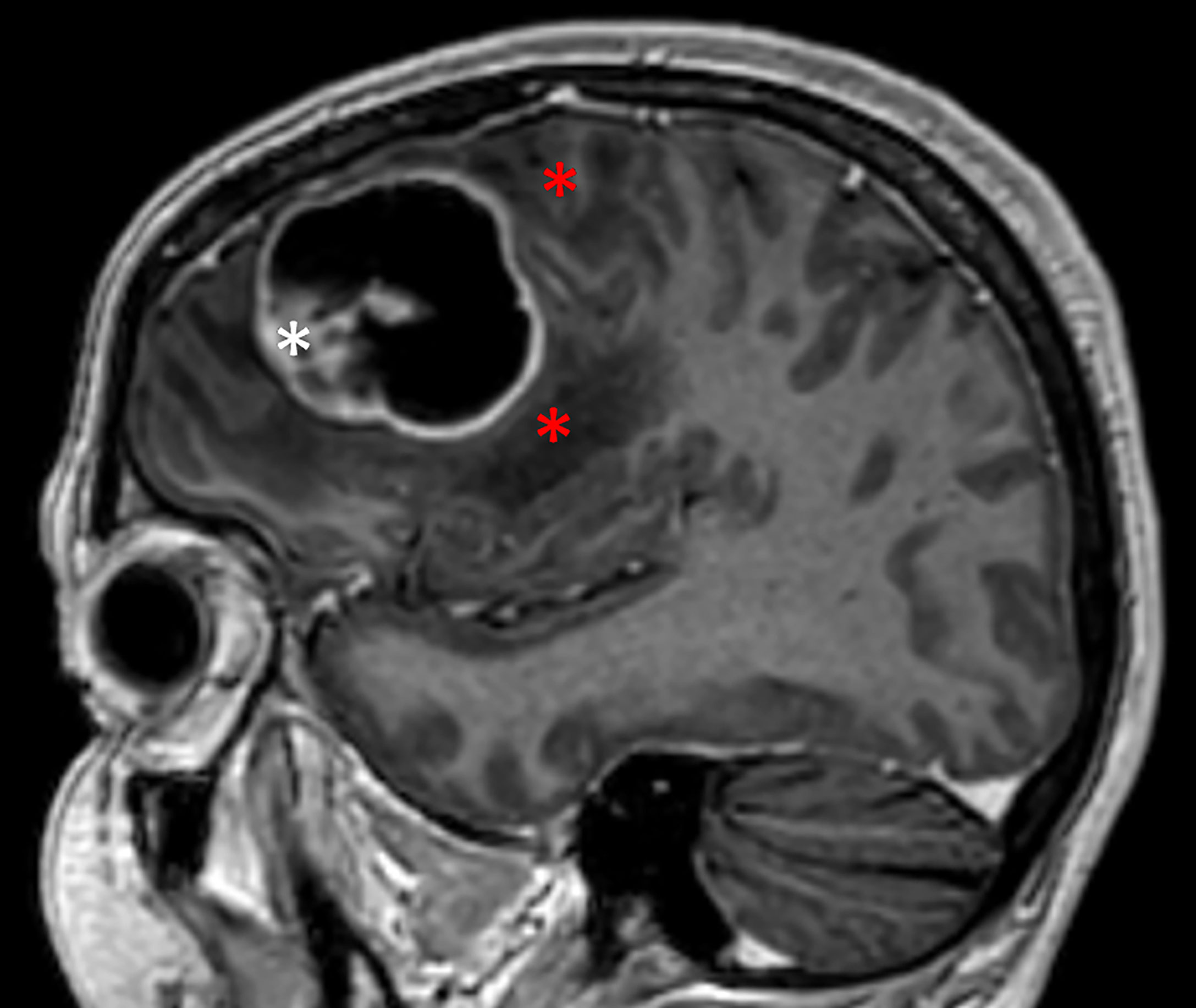
Figure 1 Cystic glioblastoma. Note how the cyst is in close contact with tumor tissue (white asterisk) and the surrounding brain tissue, both white matter and overlying neocortex. Red asterisks indicate the zone of peri-tumoral edema.
Some aspects of the presumed neurotoxicity of glioblastomas warrant mention. First, glioblastomas tend primarily to reside in, and spread along, the white matter tracts of the brain (Figure 2; 30). Glioblastomas, therefore, would be expected to impact axons or their myelinating oligodendroglia, the local fibrous astrocytes, or white matter neurons (31, 32) in addition to neuronal cell bodies in the cerebral cortex. In agreement, MRI-based studies confirm a major effect of glioblastomas on white matter integrity (10, 11). Second, analysis of the cyst fluid of cystic glioblastomas has shown that the concentration of the neurotoxic compound glutamate is highly variable between patients (33, 34). This finding suggests that the effect of glioblastomas on their surroundings, too, is variable. Third, glioblastomas harbor macrophages, microglia, and lymphocytes that secrete cytokines in addition to those secreted by the glioblastoma cells themselves (18, 25, 35–37). Thus, tumor-associated leukocytes probably contribute to the overall inflammatory effects of glioblastomas on the surrounding brain tissue.
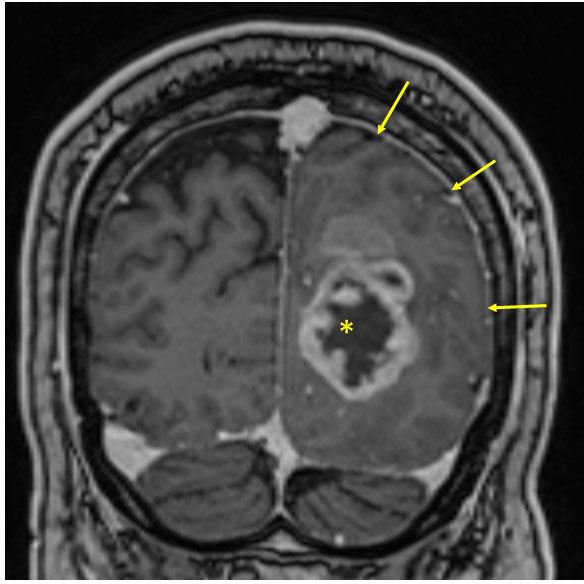
Figure 2 Solid glioblastoma in the left occipital lobe. Note how the tumor affects white matter (compare with contralateral side) and produces a mass effect with obliteration of the subarachnoidal spaces of the ipsilateral sulci (arrows). Centrally, this tumor has an area of necrosis (asterisk), which is not cystic.
The present study addresses two related issues: 1) whether glioblastomas entail inflammation that could mediate their destructive effect on the surrounding brain tissue, and 2) whether a destructive effect of glioblastomas on the surrounding brain tissue can be distinguished from displacement of brain tissue on pre-surgery MRI. With respect to the first issue, we analyzed cyst fluid from cystic glioblastomas and obtained quantitative data on cytokine concentrations, which we compared to cytokine concentrations in pus from bacterial brain abscesses, a highly pro-inflammatory environment. With respect to the second issue, we examined MRIs of the cystic and solid glioblastomas to evaluate whether displacement of brain tissue alone could account for the accommodation of the tumors within the restricted space of the skull, or whether destruction of brain tissue would be necessary to accommodate the tumor. We complemented these studies with an immunohistochemical analysis of cystic glioblastomas to look for evidence of a destructive effect of glioblastoma on brain tissue and to look for tumor-associated leukocytes that could shed light on the presence of cytokines in the cyst fluid of cystic glioblastomas.
Methods
Patients, neuroimaging, glioblastoma cyst fluid, and brain abscess pus
We prospectively enrolled patients with cystic glioblastoma and patients with bacterial brain abscess that underwent neurosurgery at The Department of Neurosurgery, The National Hospital, Oslo, Norway 2012-2021. All patients gave informed written consent to their participation. The study was approved by The Regional Committees for Medical and Health Research Ethics of Norway (concession# 2012/781 and 2012/617). There were no exclusion criteria. The glioblastoma cysts were identified as such intraoperatively from their highly fluid, non-solid, content. Cystic glioblastomas are rather infrequent, constituting approximately 8% of glioblastoma cases (28), hence the long period of enrolment.
To see if destruction of surrounding brain tissue could be a feature even of solid glioblastomas, we recruited 10 patients with solid glioblastoma retrospectively 2019-2021. Solid tumors, too, contained areas that could appear cystic or necrotic on MRI (compare Figures 1, 2), but at surgery these areas were identified as solid and necrotic.
During tumor surgery, tumor cyst fluid was aspirated into a polypropylene syringe. The cyst fluid was rapidly centrifuged at 3000 g and 4°C for 10 minutes, and the supernatant was frozen at -80°C until analysis. Brain abscess patients underwent pus evacuation through a minimally invasive procedure as described (38). Pus was rapidly centrifuged at 3000 g, and the supernatant was frozen at -80°C until analysis.
The diagnosis of glioblastoma was based on histological examination. Isocitrate dehydrogenase (IDH) mutation status was available for all patients. Pus from brain abscess patients underwent bacterial identification with polymerase chain reaction (PCR) or culture methods as per hospital routine (39, 40).
For glioblastoma patients with cystic glioblastomas, number of days of corticosteroid treatment to reduce brain edema (41) was recorded together with blood leukocyte count on the day of neurosurgery.
Cytokine measurements
The cytokines TNF-α, IFN-γ, IL-1β, IL-2, IL-4, IL-6, IL-8, IL-10, IL-12(p70), IL-13, MCP-1 (CCL2), the chemokine CXCL1 (Groα), and tissue factor were analyzed with the U-Plex Biomarker Group 1 (human) assay (Meso Scale Diagnostics, Rockville, MD, USA), which yields quantitative data on cytokine concentration in fluids. Simultaneously, some leukocyte markers were analyzed: soluble CD163 (sCD163), a marker of activated macrophages (42, 43), the soluble IL-2 receptor α-subunit, sCD25, a marker of lymphocytic activation (44–46), and the neutrophil marker myeloperoxidase (MPO; 47). IL-1β, IL-2, IL-12, TNF-α, and IFN-γ are considered typical Th1 cytokines, whereas IL-4, IL-10, and IL-13 are considered Th2 cytokines (48, 49). Tissue factor was analyzed as a pro-inflammatory and pro-coagulant factor (50) that is highly expressed in glioblastoma (51).
MRI-based evaluation of displacement of brain tissue surrounding the glioblastomas
All but one glioblastoma patient (who had cystic glioblastoma) underwent pre-operative MRI, including T1-weighted magnetization–prepared, rapid gradient echo (MPRAGE) images before and after intravenous infusion of gadolinium-based contrast agent (Clariscan 279.3 mg/mL, 0.2 mL/kg bodyweight, GE Healthcare, USA) and T2-weighted images. MRI was repeated days to weeks after surgery. Tumor and cyst volumes were calculated semi-automatically from post contrast T1-weighted images with the Smartbrush program (Brainlab, Feldkirchen, Germany). This method provides a minimum estimation of tumor volume (4, 52). The cystic glioblastomas that underwent MRI were the same as those that were analyzed with respect to cytokine content.
Degree of tissue displacement (mass effect) caused by the tumors and cysts was evaluated by an experienced neuroradiologist (see Acknowledgements) and classified as minimal, moderate or pronounced based on the degree of midline shift and compression of the cerebral ventricles and subarachnoid spaces caused by the tumors (Figure 2). For correlation assessments, the rating of mass effect (minimal, moderate, pronounced) was converted to the values 1, 2, and 3, respectively. T2-weighted fluid-attenuated inversion recovery (FLAIR) signal was used to evaluate peri-tumoral edema, which was also graded as minimal, moderate, or pronounced (converted to 1, 2, and 3, respectively, according to 53). Hence, the evaluation of whether tumors had caused tissue destruction was based on a relative lack of mass effect in spite of a substantial tumor volume. Post-operative MRIs were included to evaluate the impression of a destructive effect of the tumors on the surrounding tissue, keeping in mind that tumor resection implies the removal of some of the brain tissue surrounding the tumor and that post-surgery radiation therapy may cause some degree of gliosis and tissue retraction. However, because the last author (DD) performed the surgeries, we know that the resection of surrounding brain tissue was limited.
Histology
Tissue was removed from various parts of the glioblastomas during surgery, among them border zones between tumor and surrounding brain tissue, both white matter and neocortex. Tissue samples were fixed in 4% formaldehyde and embedded in paraffin. Paraffin sections were investigated with hematoxylin and eosin staining and with immunohistochemistry. For immunohistochemistry, we used antibodies against glial fibrillary acidic protein (GFAP), which stains glioblastoma cells (Glostrup, DK; product # M0761). Antibodies for neuronal markers were against neurofilament heavy chain (non-phosphorylated; Dako product # M0762), and the neuronal nuclear protein NeuN (Millipore-Chem; Merck, Darmstadt, Germany; product # MAB377, clone A60). For visualisation of macrophages and activated microglia we used antibodies against CD68 (DAKO product # M0814). For visualisation of T-lymphocytes we used antibodies against CD3 (Leica Biosystem, UK; product # CD3). For visualization of B-lymphocytes we used antibodies against CD20 (Dako product # M0755). Secondary antibodies were from rabbit (Dako). These antigens have been used previously as specific markers for glioblastoma-associated leukocytes (36).
Data presentation and statistics
Data on cytokine concentrations and volumes of tumors, cysts, and brain abscesses are given as absolute values. Data were analyzed with respect to normality with the Kolmogorov-Smirnov test. Differences between cytokine levels in glioblastoma cyst fluid and brain abscess pus were analyzed with the Mann-Whitney U test or the Student’s t-test, as appropriate. Correlations were analyzed with Pearson’s or Spearman’s test, as appropriate. P-values < 0.05 were considered statistically significant.
Results
Patients, glioblastomas, and brain abscesses
Twenty-one patients with cystic glioblastoma, four women and 17 men, aged 26-78 years (median age 65 years) had tumors in any of the cerebral lobes. Three out of the 21 patients with cystic glioblastoma had tumors with IDH mutations; according to the recent classification system (2) these are WHO grade IV astrocytomas, but are included in this series as they were considered glioblastomas at the time of diagnosis and were macroscopically indistinguishable from the rest of the group. Ten patients with solid glioblastoma, five women and five men, aged 41-78 years (median 59 years), had tumors in any of the cerebral lobes; none of these tumors had IDH mutations.
The cyst fluid that was aspirated from the glioblastoma cysts was highly fluid, transparent and of a color that varied from faint yellowish to colorless. Centrifugation of cyst fluid samples (2-5 mL) caused the precipitation of a few cells that could be seen by light microscopy; these cells, which constituted a minimal fraction of the cyst fluid, were not characterized further. All but one of the glioblastoma patients received corticosteroid treatment for 1-83 days (median 14 days) to reduce peri-tumoral edema. Doses were initially 16 mg methylprednisolone four times per day; these were gradually reduced to 4 mg four times per day, the rate of dose reduction depending on the severity of symptoms and the clinical response to treatment. After tumor resection, all patients received adjuvant temozolomide treatment and radiation therapy (3).
Thirteen patients, three women and ten men, aged 24-72 years (median age 53 years) had bacterial brain abscesses in any of the cerebral lobes. Abscess volumes were 2.7-42 cm3 (median 21 cm3). The aspirated pus was highly viscous, opaque and of a color that varied from yellowish to brown. Microscopy prior to centrifugation showed a high density of leukocytes, mostly neutrophils, which constituted >50% of the pus volume. PCR or bacterial culture identified Streptococcus intermedius in eight patients, Fusobacterium nucleatum in three, Aggregatibacter aphrophilus in two, and Parvimonas micra, Porphyromonas endodontalis, Propionebacterium acnes, and β-hemolytic streptococci group G in one patient each. (In two pus samples, three bacterial species were identified: F. nucleatum, P. micra, and P. acnes in one; S. intermedius, A. aphrophilus, and P. endodontalis in another). The bacterial identity did not correlate with abscess volumes or cell marker or cytokine levels.
Cell markers and cytokine levels in glioblastoma cyst fluid and brain abscess pus
In glioblastoma cyst fluid, the concentration of sCD163, a marker of activated macrophages and microglia (42, 43) was significantly higher than in brain abscess pus (Table 1). This was true also for MCP-1, a pro-inflammatory chemokine that is secreted mostly by monocytes, macrophages, and microglia (54). The level of sCD25, a lymphocyte marker (44–46), was not significantly different in glioblastoma cyst fluid and brain abscess pus. In contrast, the concentration of MPO, which is released from neutrophils (47), was much higher in brain abscess pus, in keeping with pus being dominated by neutrophils and in agreement with a previous study showing that MPO is a dominant protein in brain abscess pus (38). Similarly, the concentration of IL-1β, which may be released from both neutrophils and macrophages (55), was much higher in brain abscess pus than in glioblastoma cyst fluid. Histological analysis of tissue from cystic glioblastomas showed the presence of macrophages and lymphocytes in glioblastoma and their proximity to the cyst fluid (Figure 3; se images C, D, and E, specifically).
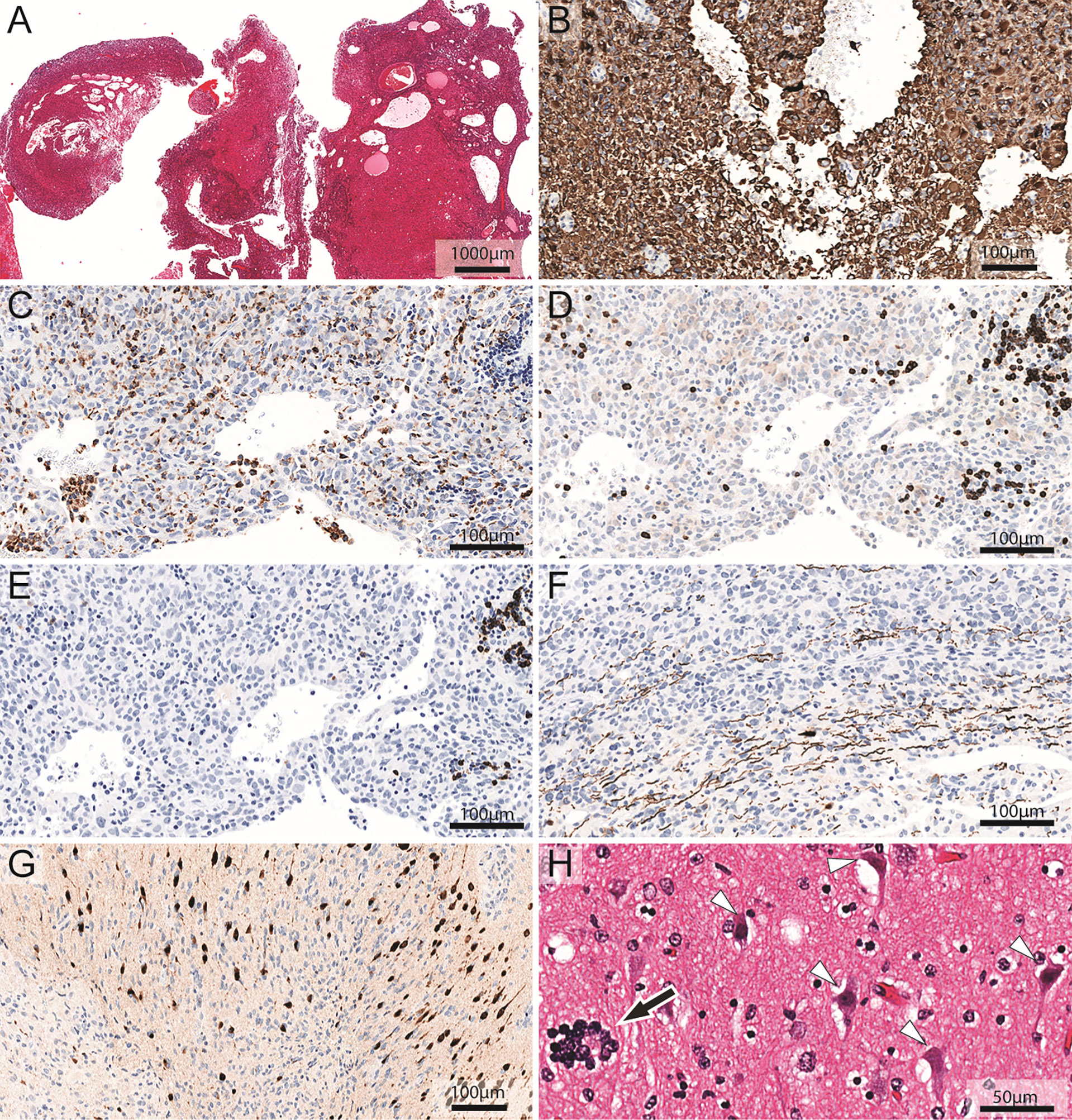
Figure 3 Neuropathological analysis of cystic glioblastoma. (A) Hematoxylin and eosin staining of a representative biopsy reveals intratumoral cysts. (B) Glioblastoma cells show strong immunoreactivity (brown color) for GFAP. (C) Anti-CD68 antibodies label macrophages and microglia cells (dark brown cells). The areas of the section without solid tissue represent cyst lumen. (D) Anti-CD3 antibodies label T-lymphocytes (dark brown cells). The areas of the section without solid tissue represent cyst lumen. (E) Anti-CD20 antibodies label immunoreactive B-lymphocytes (dark brown cells). The areas of the section without solid tissue represent cyst lumen. (F) The infiltrative growth pattern of the glioblastoma cells in white matter is evident among the axons that are stained dark brown for neurofilament heavy chain. (G) Glioblastoma cells infiltrate neocortical tissue where neurons are stained dark brown for NeuN. (H) Hematoxylin and eosin staining of neocortex shows damaged neurons (white arrowheads) with shrunken, triangular appearance and condensed chromatin. Prominent satellitosis (56) can be seen (black arrow). Please note the proximity of tumor-associated leukocytes to the cyst lumen (C–E). Note also how macrophages and lymphocytes may both be scattered throughout the tumor and appear in groups (C–E) and how neurons (brown nuclei) embedded among tumor cells (blue nuclei) appear pycnotic in the center of (G).
Cytokine levels were highly variable across the 21 glioblastoma cyst fluid samples (Table 1). For instance, levels of MCP-1, IL-6, IL-8, and the chemokine CXCL1 varied by >1000-fold. TNF-α levels varied similarly, from not being detectable in one patient to the maximum value being approximately 25 times higher than the median value. The glioblastoma cytokine levels correlated positively with one another (Table 2), reflecting that cytokine levels tended overall to be high, medium, or low.
In spite of the great variability in cytokine concentration, glioblastoma cyst fluid levels of TNF-α, IL-6, IL-8, and CXCL1 were not significantly different from those in brain abscess pus, and, as stated above, the level of MCP-1 was significantly higher in cyst fluid, all indicative of a highly pro-inflammatory glioblastoma environment (Table 1). The levels of IFN-γ, IL-1β, IL-2, IL-4, IL-10, IL-12, and IL-13, in contrast, were significantly higher in pus than in cyst fluid. The level of tissue factor, a non-cytokine pro-inflammatory and pro-coagulant compound, which may stimulate the release of cytokines such as IL-8 and the chemokine CXCL1 (50), was not significantly different in glioblastoma cyst fluid and brain abscess pus.
The three patients whose tumors harbored IDH mutations did not stand out in any way with respect to cyst fluid cytokine levels. For instance, their level of tissue factor, which reportedly is lower in tumors bearing IDH mutations (50), was 25, 46, and 89 pg/mL, respectively, which was at, or above, the median value for the group as a whole (Table 1).
The levels of several cytokines in glioblastoma cyst fluid correlated with blood leukocyte count (Table 3). The level of IL-10 in glioblastoma cyst fluid correlated with the number of days on steroid treatment (r=0.66; p=0.0015) in line with previous studies (57, 58); for the other cytokines, including the other Th2 type cytokines IL-4 and IL-13 (see Methods, section Cytokine measurements), there was no correlation with number of days of corticosteroid treatment (r values from -0.27 to 0.05; p values 0.23-0.80). Similarly, there was no correlation between number of days of corticosteroid treatment and blood leukocyte levels (r= -0.11; p=0.6). It should be kept in mind, however, that the dose of methylprednisolone was not the same throughout the treatment period (see Section Results, Patients, glioblastomas, and brain abscesses), which could obscure correlations with corticosteroid treatment.
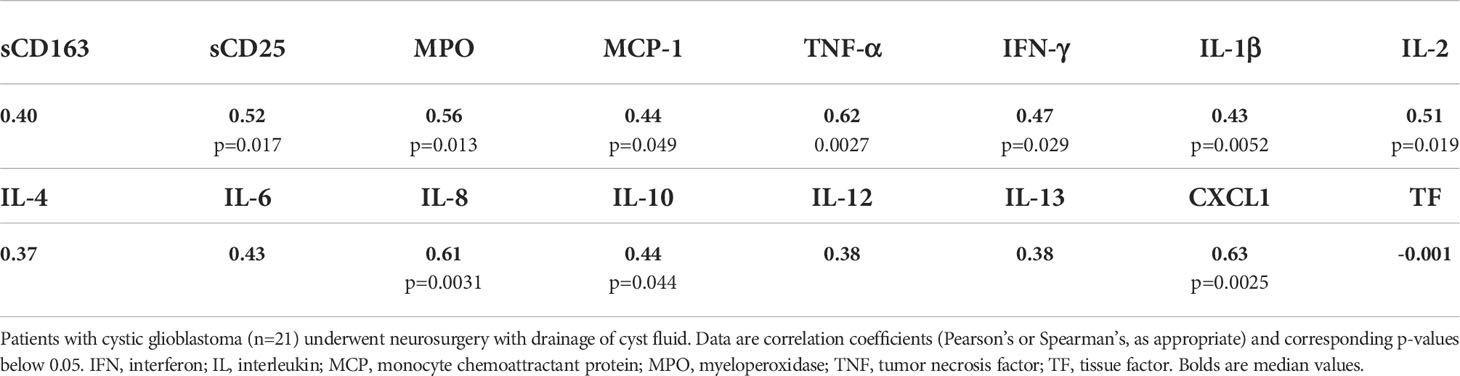
Table 3 Correlations between blood leukocyte count and levels of cell markers and cytokines in glioblastoma cyst fluid.
Median survival for the whole patient group was 18.5 months (range 2-100). Two patients, whose tumors carried IDH mutations survived for 81 and 100 months; one patient, whose glioblastoma did not carry an IDH mutation, survived for 85 months. When patients carrying IDH mutation were excluded, median survival was 17.5 months (range 2-85). There were no correlations between the level of any cytokine and survival, whether or not patients whose tumors carried IDH mutations were excluded (r= -0.22 – 0.28; p>0.2).
Glioblastoma volumes and assessment of displacement (mass effect) vs. destruction of surrounding brain tissue
Pre-operative MRIs were available for 20 out of the 21 patients with cystic glioblastomas that were analyzed with respect to cyst fluid cytokine content (the last patient underwent pre-operative CT only). Cyst volumes were 5-60 cm3 (median 28 cm3), whereas the volume of tumor tissue was 3.4-78 cm3 (median 19 cm3). The cyst volumes constituted 16-83% of the total tumor (solid tumor + cyst) with a median value of 48%.
In the patients with cystic glioblastomas, mass effect correlated with cyst volume (r=0.59; p=0.0079) and with tumor volume (r=0.47; p=0.045). In three of the 20 patients, mass effect was deemed minimal, in eight it was moderate, and in nine it was pronounced. In the patients with minimal and moderate mass effect, the relatively low degree of tissue displacement, in spite of substantial tumor and cyst volumes, was consistent with some brain tissue having been destroyed by tumor growth (Figure 4). In some patients, peri-tumoral edema contributed visibly to whatever mass effect there was (Figures 4C, E), but peri-tumoral edema and mass effect were not correlated in the group as a whole (r=0.15; p=0.55). Involvement of the neocortex could be seen in some patients with cystic glioblastomas (Figures 4C, E, G); this was also seen histologically (Figures 3G, H).
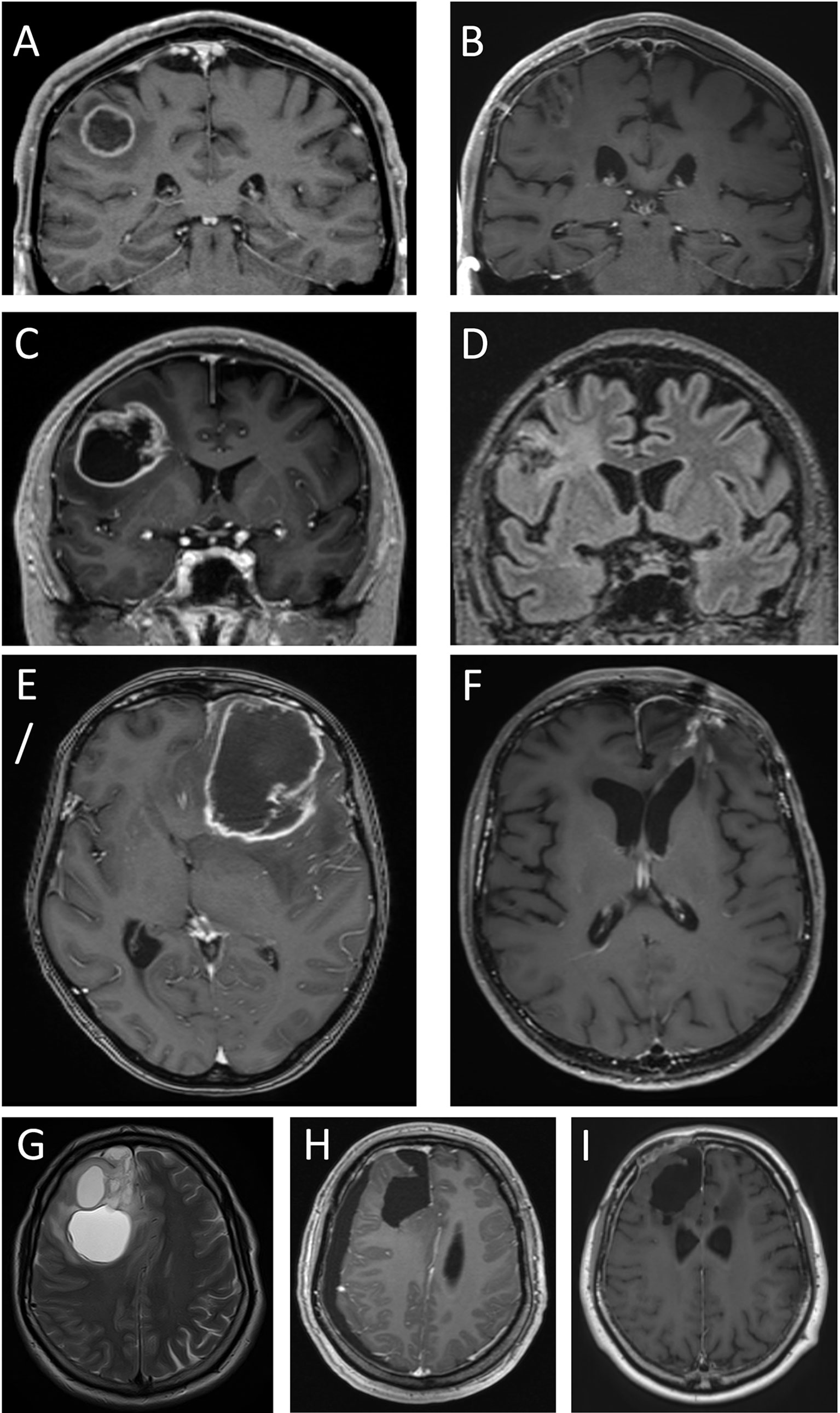
Figure 4 Four cystic glioblastomas before and after tumor surgery. T1 weighted MPRAGE MRIs after intravenous injection of gadolinium-based contrast medium (which gives the ring-formed bright signal in most images), except in D and G, which are T2-weighted and T2-weighted FLAIR, respectively. Images (A, C, E, G) show pre-surgical MRIs. Note the modest displacement of surrounding brain tissue (compare with contralateral side). Images (B, D, F, H, I) show MRIs after 240, 295, 330, 39 days, and 45 months, respectively. Tumors in images (A, C) were assessed as having minimal mass effect, tumors in images (E, G) had pronounced mass effect. Note the absence of brain tissue where the tumor and cyst resided; this comprises white matter and adjacent neocortex.
There were no significant correlations between mass effect on the one hand and cyst fluid levels of leukocyte markers or cytokines on the other hand (r values from -0.35 to -0.02; p values 0.15-0.95). Similarly, there were no significant correlations between degree of peri-tumoral edema as seen on T2-weighted FLAIR MRI on the one hand and levels of leukocyte markers or cytokines on the other (r values from -0.16 to 0.05; p values 0.52-0.98).
The contrast-enhancing component of solid glioblastomas (n=10) measured 1-58 cm3 (median volume 16 cm3). Displacement of brain tissue (mass effect) correlated with tumor volume (r=0.65; p=0.041). However, in five of the 10 patients, mass effect of the tumor was deemed minimal, in four it was moderate, and in one it was pronounced. In some patients with minimal mass effect, the relative absence of displacement of brain tissue in spite of a substantial tumor size appeared consistent with the notion that some brain tissue had been destroyed due to tumor growth (Figure 5). In some patients, the involvement of the neocortex, whether over the brain convexities (Figures 5A, G) or parasagittally (Figures 5C, E), was apparent on MRI.
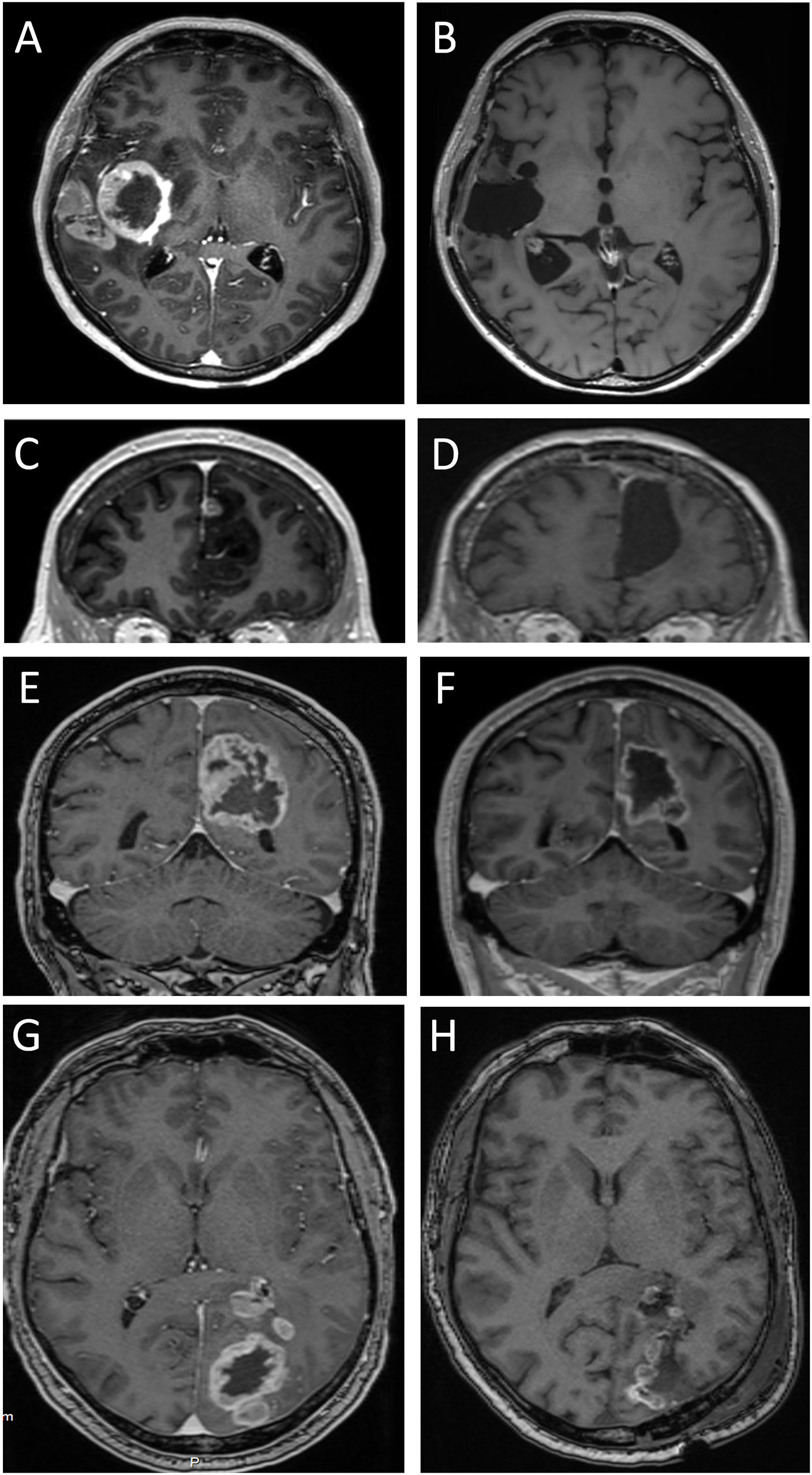
Figure 5 Four solid glioblastomas before and after tumor surgery. T1-weighted MPRAGE MRIs were obtained after intravenous injection of gadolinium-based contrast medium (which gives a bright, ring-formed signal in most images). Images (A, C, E, G) show pre-surgical MRIs. Tumors in images (A, C) were assessed as having minimal mass effect. Note the modest displacement of surrounding brain tissue (compare with contralateral side). Tumors in images (E, G) were assessed as having moderate and pronounced mass effect, respectively. Images (B, D, F, H) show MRIs after 400, 167, 26, and 4 days, respectively. Note the absence of brain tissue where the tumor resided. This includes both white matter and the overlying neocortex.
Histological analysis of cystic glioblastomas showed tumor cell infiltration of white matter and neocortex (Figures 3F, G). Neocortical neurons appeared damaged (Figure 3H). Although these histological images do not prove the neurotoxicity of glioblastoma cells in human brain, they illustrate the proximity of tumor cells to vulnerable neurons.
Assessment of post-surgery MRIs in glioblastoma patients
MRI performed days to weeks after surgical removal of the glioblastomas showed the presence of a resection cavity in patients with cystic or solid glioblastomas (Figures 4H, I; Figures 5B, D, F, H). In some patients, the loss of neocortical tissue was evident after tumor resection, whether over the brain convexities (Figures 4B, D, F, H, I; Figures 5B, H), or parasagittally (Figures 4H, I; Figures 5D, F). In some patients, loss of brain tissue was seen as tissue retraction leading to enlargement of the lateral ventricles (Figure 4F). These observations were a further suggestion that some brain tissue had been destroyed by the glioblastomas.
Discussion
High, but variable concentrations of pro-inflammatory cytokines in the glioblastoma environment. Possible impact on the surrounding brain tissue
We report here on concentrations of pro-inflammatory cytokines as they would appear in the microenvironment of cystic glioblastomas and the surrounding brain tissue. In general, cytokine levels were high. The median concentrations of TNF-α, IL-6, IL-8, MCP-1, and the chemokine CXCL1 in glioblastoma cyst fluid were far higher than previously published serum values for SARS covid-19 patients, patients with sepsis, and healthy controls (59–66). The values reported here are similar to CSF values after intracerebral and intraventricular hemorrhage, an acutely inflammatory condition (67). The conclusion that pro-inflammatory markers are high in the glioblastoma microenvironment was corroborated by the comparison with pus from bacterial brain abscesses, a highly pro-inflammatory environment: glioblastoma cyst fluid had levels of TNFα, IL-6, MCP-1, CXCL1, sCD163, sCD25, and tissue factor that were similar to or higher than those in pus, although the level of several other cytokines was higher in pus. The levels of MCP-1, IL6 and IL-8 in glioblastoma cyst fluid in the present study were similar to values reported previously in glioblastoma cyst fluid (Table 4); as can be seen from the table, some other inflammation-related proteins have also been detected in glioblastoma cyst fluid. However, in spite of the usefulness of analyzing glioblastoma cyst fluid for quantitative data on cytokines and related proteins, relatively few studies have been performed on this fluid for the last 30 years.
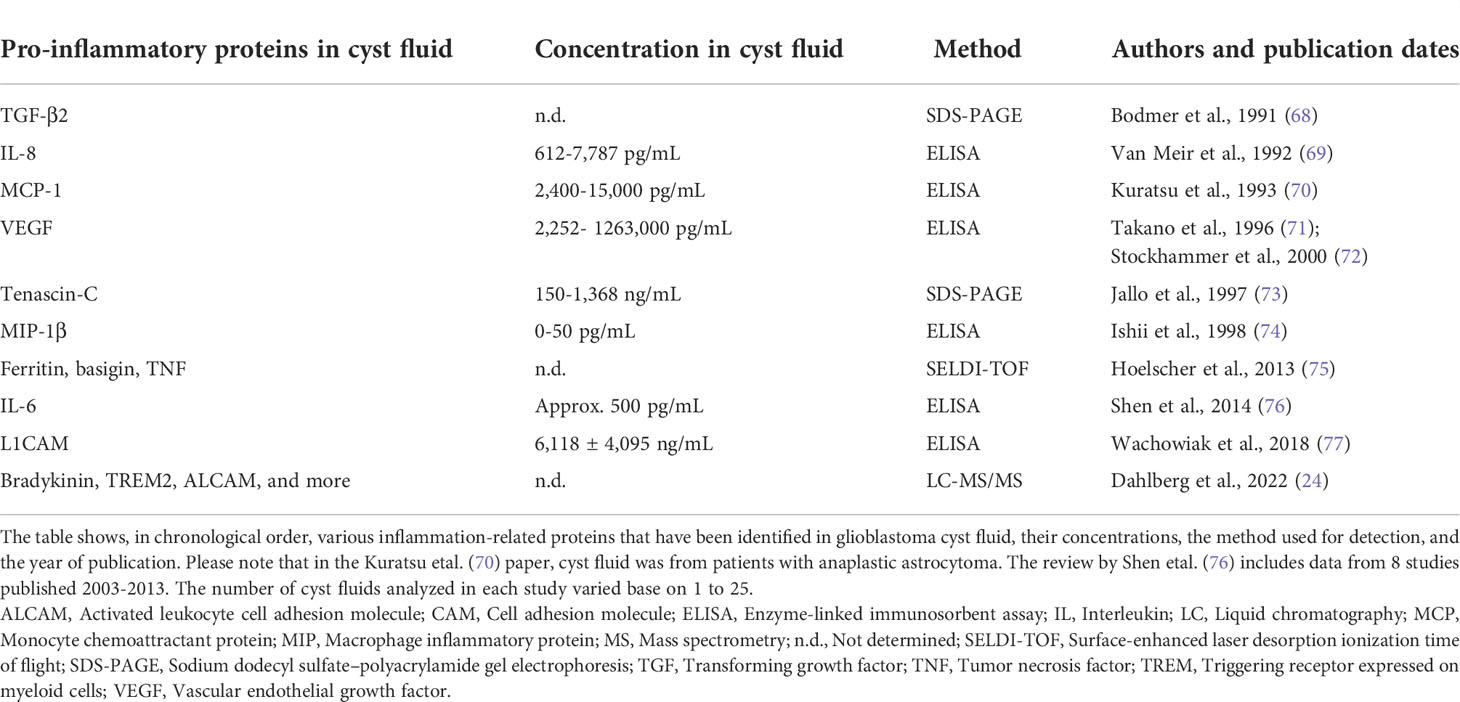
Table 4 Inflammation-related proteins identified in cyst fluid from cystic glioblastomas, as reported in the literature.
The high level of TNF-α in brain abscess pus was similar to that reported previously by Bajpai et al. (78). The importance of TNF-α, IL-6, and other cytokines for the acute host response against severe bacterial brain infection has been documented in experimental studies (79–81). The high concentration of these cytokines in glioblastoma cyst fluid is all the more remarkable.
We found that the levels of several cytokines in glioblastoma cyst fluid correlated with blood leukocyte counts. At present, we cannot say whether this correlation reflected systemic effects of the glioblastomas or, vice versa, that a high blood level of leukocytes leads to a high number of leukocytes entering the glioblastomas with ensuing high levels of cytokines. In either case, this observation may point to an important interaction between glioblastomas and the circulation.
A purpose of the present study was to investigate whether an inflammatory effect of glioblastomas could contribute to a destructive effect on the surrounding brain tissue. Indeed, the concentrations of inflammatory markers in glioblastoma cyst fluid pointed to a highly pro-inflammatory and potentially destructive environment. However, the concentrations of cytokines needed to affect brain tissue adversely is currently not known. Most cytokines have been reported to be involved in both neurodegenerative and regenerative processes. IL-8 receptors have been identified on axons in human brain (82), and the CXCL1 receptor, CXCR2, is expressed in CNS oligodendrocytes (83), which would allow for an inflammatory response in white matter to the high levels of IL-8 and CXCL1 in glioblastoma cyst fluid. However, CXCL1, which is formed by glioblastoma cells, leukocytes and astrocytes alike, and which could be important for tumor immunogenicity (84), may also have neuroprotective effects (85, 86). Overexpression of IL-6 in the brain causes neurodegeneration (87), but other studies have suggested a physiological role for IL-6 in the stabilisation of CNS axonal microtubules (88). Similarly, TNF-α may cause neuronal and oligodendroglial dysfunction and death, while at the same time acting as a trophic factor for brain cells (89), and the pro-inflammatory cytokines IL-6 and MCP-1 have roles in axonal regeneration after mechanical damage to the spinal cord (90–93). Therefore, we are not able to delineate the exact effects of the various cytokines, or their combined action, on the brain tissue surrounding glioblastomas. More research is needed on the effects of cytokines on the function, survival, and degeneration of the brain tissue surrounding a glioblastoma. There was, however, a striking variability in cytokine levels among patients. Thus, it may be assumed that the degree of inflammation probably varies between glioblastomas and that inflammation, to the extent that it affects normal brain tissue or tumor growth (25, 35, 37), does so differentially.
Some of the proteins detected in this study are considered to be fairly cell-specific: sCD163 and MCP-1 are markers of activated macrophages and microglia (42, 43, 54), sCD25 is a marker of activated lymphocytes (44–46), and MPO is primarily a marker of neutrophils (47). The high levels of these proteins suggested the presence of the corresponding leukocytes in glioblastomas or their cysts. Histologic analysis confirmed the presence of macrophages and/or microglia and lymphocytes in cystic glioblastomas, in agreement with previous studies in solid glioblastomas (18, 36).
The degree of edema surrounding a glioblastoma has been proposed as a predictor of poor survival (94, 95). The edema has been ascribed to the production of vascular endothelial growth factor (VEGF) by glioblastomas (96). In the present study, the high concentrations of various cytokines in the glioblastoma environment likely contributed to capillary leakage and edema formation. For instance, TNF-α, IL-1β, IL-6, and IL-8 have all been shown or suggested to mediate brain edema formation independently of one another (97–101).
The glioblastoma-mediated destruction of the surrounding brain tissue may be assessed qualitatively on pre-surgery MRI
MRI observations in the present study complement previous studies on the destructive effect of glioblastoma on the surrounding brain tissue. This destructive effect has been documented by magnetic resonance spectroscopy and diffusion tensor imaging of the brain (10, 11), by the high circulating levels of neurofilament in glioblastoma patients (12) and in experimental studies (14–16). In the present study, a destructive effect of glioblastoma was evident, because displacement alone (mass effect) could not account for the accommodation of the tumor within the brain tissue. This observations points to the need for neuroprotective strategies in glioblastoma therapy. Previously, glutamate receptor blocker perampanel has received interest as a drug that could reduce tumor growth and at the same time act as a neuroprotective agent, as has been shown in experimental animal studies (102), but the possibility of neuroprotection through antiepileptic drug treatment has been questioned (103). The finding in the present study of very high concentrations of cytokines in the cyst fluid of cystic glioblastomas points to immunomodulation as another possible neuroprotective approach.
Our assessment of the destruction caused by glioblastoma was qualitative. Future studies are needed to develop a quantitative method for MRI-based assessment of glioblastoma’s destructive effect in human brain.
The destructive process seemed to affect both white matter and the overlying neocortex. Several mechanisms could underlie a destructive process in the brain tissue surrounding the glioblastoma, but, presumably, these mechanisms are somewhat different for grey and white structures. A high inflammatory activity could be destructive in both types of brain tissue, whereas glutamate, which may be released by glioblastomas (15, 19, 20), would probably affect cortical neurons with glutamate receptors more than white matter. In contrast, physical strain, including stretching and distortion of brain tissue, could have a more pronounced effect on white matter axons. All destructive mechanisms are likely to produce secondary inflammatory responses that could be reflected in the cyst fluid levels of cytokines.
We did not see any correlation between cyst fluid levels of cytokines on the one hand and MRI-based assessment of displacement of brain tissue or peri-tumoral edema on the other. It remains to be investigated, with a method for quantitative assessment of brain tissue destruction, whether glioblastoma cytokine concentrations correlate with destruction of brain tissue.
Limitations
There are limitations to the comparison of glioblastoma cyst fluid to brain abscess pus with respect to cytokine levels. Even though brain abscess pus is a highly pro-inflammatory environment with a high content of neutrophils, myeloperoxidase, and various cytokines (38, 78), it is also a hostile environment with low concentration of glucose, low pH, and high ammonia levels (39, 104). These conditions may be unfavorable for cytokine formation. Further, differences in cytokine levels between glioblastoma cyst fluid and brain abscess pus may reflect differences in cell types and cell density in the two fluids (34, 104). However, in spite of these limitations, it appears clear that the level of pro-inflammatory factors is high in both fluids.
We did not characterize our tumor samples with respect to genetic alterations that are common in glioblastomas: TERT promoter mutation, EGFR amplification, or gain of chromosome 7 combined with loss of chromosome 10 (2), and so we do not know whether these genetic alterations would influence the inflammatory environment of glioblastoma.
Most glioblastomas are not cystic (28), and we cannot say whether the concentrations of cytokines in glioblastoma cyst fluid would be similar in solid tumors. Cytokines are assumed to contribute to the growth of solid glioblastomas (18, 25, 35, 37), but their concentration in the extracellular fluid of solid tumors remains a topic for further research.
The assessment of the destruction of brain tissue caused by glioblastoma was done in a qualitative manner by an experienced neuroradiologist. We have not presented a method for quantitative determination of destruction of the brain tissue surrounding a glioblastoma. In the absence of a quantitative method, we were unable to look for correlations between cyst fluid cytokine levels and degree of destruction of brain tissue. Such a method could be a valuable tool for prognostic evaluation of glioblastoma surgery, as displaced brain tissue would presumably regain some function after surgery, whereas destroyed tissue would not.
We did not follow our patients with MRIs to see if cytokine levels in glioblastoma cyst fluid correlated with formation of drop metastases or with spread of the tumor within the brain tissue. Cytokines probably have an important role in the proliferation and migration of glioblastomas (17, 18). The lack of a correlation between the levels of the measured cytokines on the one hand and patient survival on the other could suggest that any correlation between cytokine levels and glioblastoma migration and metastasis relies on cytokines other than the ones investigated in the present study.
We have not presented data on the expression of cytokine receptors in the brain tissue surrounding the glioblastomas. Expression of cytokine receptors would be a prerequisite for glioblastoma-derived cytokines to cause tissue damage. Investigation of cytokine receptor expression in the peri-tumoral brain tissue remains a task for future histopathological research.
Data availability statement
Restrictions apply to the datasets: The datasets presented in this article are not readily available due to issues of privacy, some raw data may not be made available upon request. Requests should be directed to the corresponding author(s).
Ethics statement
This study was reviewed and approved by The Regional Committees for Medical and Health Research Ethics of Norway (concession# 2012/781 and 2012/617). The patients/participants provided their written informed consent to participate in this study.
Author contributions
DD provided biological material and contributed to data analysis and drafting of the manuscript. PN provided histological analyses and contributed to data analysis and drafting of the manuscript. BeH provided cytokine analysis and contributed to data analysis and drafting of the manuscript. BjH contributed to data analysis and drafted the manuscript. All authors contributed to the article and approved the submitted version.
Acknowledgments
The authors wish to thank Ellen Lund Sagen for technical assistance with cytokine measurements and Dr. Anna Latysheva, PhD, for expert neuroradiological evaluation of MRIs.
Conflict of interest
The authors declare that the research was conducted in the absence of any commercial or financial relationships that could be construed as a potential conflict of interest.
Publisher’s note
All claims expressed in this article are solely those of the authors and do not necessarily represent those of their affiliated organizations, or those of the publisher, the editors and the reviewers. Any product that may be evaluated in this article, or claim that may be made by its manufacturer, is not guaranteed or endorsed by the publisher.
References
1. Louis DN, Perry A, Reifenberger G, von Deimling A, Figarella-Branger D, Cavenee WK, et al. The 2016 world health organization classification of tumors of the central nervous system: A summary. Acta Neuropathol (2016) 131(6):803–20. doi: 10.1007/s00401-016-1545-1
2. Louis DN, Perry A, Wesseling P, Brat DJ, Cree IA, Figarella-Branger D, et al. The 2021 WHO classification of tumors of the central nervous system: A summary. Neuro Oncol (2021) 23(8):1231–51. doi: 10.1093/neuonc/noab106
3. Stupp R, Mason WP, van den Bent MJ, Weller M, Fisher B, Taphoorn MJ, et al. Radiotherapy plus concomitant and adjuvant temozolomide for glioblastoma. N Engl J Med (2005) 352(10):987–96. doi: 10.1056/NEJMoa043330
4. Steed TC, Treiber JM, Brandel MG, Patel KS, Dale AM, Carter BS, et al. Quantification of glioblastoma mass effect by lateral ventricle displacement. Sci Rep (2018) 8(1):2827. doi: 10.1038/s41598-018-21147-w
5. Furnari FB, Fenton T, Bachoo RM, Mukasa A, Stommel JM, Stegh A, et al. Malignant astrocytic glioma: genetics, biology, and paths to treatment. Genes Dev (2007) 21(21):2683–710. doi: 10.1101/gad.1596707
6. Lukiw WJ, Cui JG, Li YY, Culicchia F. Up-regulation of micro-RNA-221 (miRNA-221; chr Xp11.3) and caspase-3 accompanies down-regulation of the survivin-1 homolog BIRC1 (NAIP) in glioblastoma multiforme (GBM). J Neurooncol (2009) 91(1):27–32. doi: 10.1007/s11060-008-9688-0
7. Liu XY, Zhang L, Wu J, Zhou L, Ren YJ, Yang WQ, et al. Inhibition of elongation factor-2 kinase augments the antitumor activity of temozolomide against glioma. PLoS One (2013) 8(11):e81345. doi: 10.1371/journal.pone.0081345
8. Gamble JT, Reed-Harris Y, Barton CL, La Du J, Tanguay R, Greenwood JA. Quantification of glioblastoma progression in zebrafish xenografts: Adhesion to laminin alpha 5 promotes glioblastoma microtumor formation and inhibits cell invasion. Biochem Biophys Res Commun (2018) 506(4):833–9. doi: 10.1016/j.bbrc.2018.10.076
9. Silbergeld DL, Rostomily RC, Alvord EC Jr. The cause of death in patients with glioblastoma is multifactorial: clinical factors and autopsy findings in 117 cases of supratentorial glioblastoma in adults. J Neurooncol (1991) 10(2):179–85. doi: 10.1007/BF00146880
10. Ormond DR, D'Souza S, Thompson JA. Global and targeted pathway impact of gliomas on white matter integrity based on lobar localization. Cureus (2017) 9(9):e1660. doi: 10.7759/cureus.1660
11. Stadlbauer A, Hammen T, Buchfelder M, Bachmair J, Dörfler A, Nimsky C, et al. Differences in metabolism of fiber tract alterations in gliomas: A combined fiber density mapping and magnetic resonance spectroscopic imaging study. Neurosurgery (2012) 71(2):454–63. doi: 10.1227/NEU.0b013e318258e332
12. Hepner A, Porter J, Hare F, Nasir SS, Zetterberg H, Blennow K, et al. Serum neurofilament light, glial fibrillary acidic protein and tau are possible serum biomarkers for activity of brain metastases and gliomas. World J Oncol (2019) 10(4-5):169–75. doi: 10.14740/wjon1228
13. Das KK, Kumar R. Pediatric glioblastoma. In: De Vleeschouwer S, editor. Glioblastoma. Brisbane (AU: Codon Publications (2017). Available at: https://www.ncbi.nlm.nih.gov/books/NBK469983/. doi: 10.15586/codon.glioblastoma.2017.ch15
14. Engebraaten O, Bjerkvig R, Lund-Johansen M, Wester K, Pedersen PH, Mørk S, et al. Interaction between human brain tumour biopsies and fetal rat brain tissue in vitro. Acta Neuropathol (1990) 81(2):130–40. doi: 10.1007/BF00334501
15. Ye ZC, Sontheimer H. Glioma cells release excitotoxic concentrations of glutamate. Cancer Res (1999) 59(17):4383–91.
16. Ogawa J, Pao GM, Shokhirev MN, Verma IM. Glioblastoma model using human cerebral organoids. Cell Rep (2018) 23(4):1220–9. doi: 10.1016/j.celrep.2018.03.105
17. Hambardzumyan D, Gutmann DH, Kettenmann H. The role of microglia and macrophages in glioma maintenance and progression. Nat Neurosci (2016) 19(1):20–7. doi: 10.1038/nn.4185
18. Basheer AS, Abas F, Othman I, Naidu R. Role of inflammatory mediators, macrophages, and neutrophils in glioma maintenance and progression: mechanistic understanding and potential therapeutic applications. Cancers (Basel) (2021) 13(16):4226. doi: 10.3390/cancers13164226
19. Buckingham SC, Campbell SL, Haas BR, Montana V, Robel S, Ogunrinu T, et al. Glutamate release by primary brain tumors induces epileptic activity. Nat Med (2011) 17(10):1269–74. doi: 10.1038/nm.2453
20. Lee SG, Kim K, Kegelman TP, Dash R, Das SK, Choi JK, et al. Oncogene AEG-1 promotes glioma-induced neurodegeneration by increasing glutamate excitotoxicity. Cancer Res (2011) 71(20):6514–23. doi: 10.1158/0008-5472.CAN-11-0782
21. Hagemann C, Anacker J, Ernestus RI, Vince GH. A complete compilation of matrix metalloproteinase expression in human malignant gliomas. World J Clin Oncol (2012) 3(5):67–79. doi: 10.5306/wjco.v3.i5.67
22. Pinacho-Garcia LM, Valdez RA, Navarrete A, Cabeza M, Segovia J, Romano MC. The effect of finasteride and dutasteride on the synthesis of neurosteroids by glioblastoma cells. Steroids (2020) 155:108556. doi: 10.1016/j.steroids.2019.108556
23. Jarabo P, de Pablo C, Herranz H, Martín FA, Casas-Tintó S. Insulin signaling mediates neurodegeneration in glioma. Life Sci Alliance (2021) 4(3):e202000693. doi: 10.26508/lsa.202000693
24. Dahlberg D, Rummel J, Distante S, De Souza GA, Stensland ME, Mariussen E, et al. Glioblastoma microenvironment contains multiple hormonal and non-hormonal growth-stimulating factors. Fluids Barriers CNS (2022) 19(1):45. doi: 10.1186/s12987-022-00333-z
25. Broekman ML, Maas SLN, Abels ER, Mempel TR, Krichevsky AM, Breakefield XO. Multidimensional communication in the microenvirons of glioblastoma. Nat Rev Neurol (2018) 14(8):482–95. doi: 10.1038/s41582-018-0025-8
26. de Mooij T, Peterson TE, Evans J, McCutcheon B, Parney IF. Short non-coding RNA sequencing of glioblastoma extracellular vesicles. J Neurooncol (2020) 146(2):253–63. doi: 10.1007/s11060-019-03384-9
27. Valdebenito S, Lou E, Baldoni J, Okafo G, Eugenin E. The novel roles of connexin channels and tunneling nanotubes in cancer pathogenesis. Int J Mol Sci (2018) 19(5):1270. doi: 10.3390/ijms19051270
28. Sarmiento JM, Nuño M, Ortega A, Mukherjee D, Fan X, Black KL, et al. Cystic glioblastoma: an evaluation of IDH1 status and prognosis. Neurosurgery (2014) 74(1):71–5. doi: 10.1227/NEU.0000000000000200
29. Curtin L, Whitmire P, Rickertsen CR, Mazza GL, Canoll P, Johnston SK, et al. Assessment of prognostic value of cystic features in glioblastoma relative to sex and treatment with standard-of-care. Front Oncol (2020) 10:580750. doi: 10.3389/fonc.2020.580750
30. Hong JH, Kang S, Sa JK, Park G, Oh YT, Kim TH, et al. Modulation of nogo receptor 1 expression orchestrates myelin-associated infiltration of glioblastoma. Brain (2021) 144(2):636–54. doi: 10.1093/brain/awaa408
31. Suárez-Solá ML, González-Delgado FJ, Pueyo-Morlans M, Medina-Bolívar OC, Hernández-Acosta NC, González-Gómez M, et al. Neurons in the white matter of the adult human neocortex. Front Neuroanat (2009) 3:7.2009. doi: 10.3389/neuro.05.007.2009
32. Köhler S, Winkler U, Hirrlinger J. Heterogeneity of astrocytes in grey and white matter. Neurochem Res (2021) 46(1):3–14. doi: 10.1007/s11064-019-02926-x
33. Wieser HG, Rudolph U, Blau N, Boison D, Imhof HG, Bernays R, et al. Amino acid composition of brain cysts: levels of excitatory amino acids in cyst fluid fail to predict seizures. Epilepsy Res (2003) 55(3):191–9. doi: 10.1016/s0920-1211(03)00106-2
34. Dahlberg D, Struys EA, Jansen EE, Mørkrid L, Midttun Ø, Hassel B. Cyst fluid from cystic, malignant brain tumors: A reservoir of nutrients, including growth factor-like nutrients, for tumor cells. Neurosurgery (2017) 80(6):917–24. doi: 10.1093/neuros/nyw101
35. Yeung YT, McDonald KL, Grewal T, Munoz L. Interleukins in glioblastoma pathophysiology: implications for therapy. Br J Pharmacol (2013) 168(3):591–606. doi: 10.1111/bph.12008
36. Orrego E, Castaneda CA, Castillo M, Bernabe LA, Casavilca S, Chakravarti A, et al. Distribution of tumor-infiltrating immune cells in glioblastoma. CNS Oncol (2018) 7(4):CNS21. doi: 10.2217/cns-2017-0037
37. Alghamri MS, McClellan BL, Hartlage MS, Haase S, Faisal SM, Thalla R, et al. Targeting neuroinflammation in brain cancer: uncovering mechanisms, pharmacological targets, and neuropharmaceutical developments. Front Pharmacol (2021) 12:680021. doi: 10.3389/fphar.2021.680021
38. Hassel B, De Souza GA, Stensland ME, Ivanovic J, Voie Ø, Dahlberg D. The proteome of pus from human brain abscesses: host-derived neurotoxic proteins and the cell-type diversity of CNS pus. J Neurosurg (2018) 129(3):829–37. doi: 10.3171/2017.4.JNS17284
39. Dahlberg D, Ivanovic J, Hassel B. High extracellular concentration of excitatory amino acids glutamate and aspartate in human brain abscess. Neurochem Int (2014) 69:41–7. doi: 10.1016/j.neuint.2014.03.001
40. Rogne AG, Müller EG, Udnaes E, Sigurdardottir S, Raudeberg R, Connelly JP, et al. β-amyloid may accumulate in the human brain after focal bacterial infection: An 18 f-flutemetamol positron emission tomography study. Eur J Neurol (2021) 28(3):877–83. doi: 10.1111/ene.14622
41. Zhou L, Shen Y, Huang T, Sun Y, Alolga RN, Zhang G, et al. The prognostic effect of dexamethasone on patients with glioblastoma: A systematic review and meta-analysis. Front Pharmacol (2021) 12:727707. doi: 10.3389/fphar.2021.727707
42. Lekva T, Gullestad L, Broch K, Aukrust P, Andreassen AK, Ueland T. Distinct patterns of soluble leukocyte activation markers are associated with etiology and outcomes in precapillary pulmonary hypertension. Sci Rep (2020) 10(1):18540. doi: 10.1038/s41598-020-75654-w
43. Ørntoft NW, Blé M, Baiges A, Ferrusquia J, Hernández-Gea V, Turon F, et al. Divergences in macrophage activation markers soluble CD163 and mannose receptor in patients with non-cirrhotic and cirrhotic portal hypertension. Front Physiol (2021) 12:649668. doi: 10.3389/fphys.2021.649668
44. Loughnan MS, Sanderson CJ, Nossal GJ. Soluble interleukin 2 receptors are released from the cell surface of normal murine b lymphocytes stimulated with interleukin 5. Proc Natl Acad Sci U S A (1988) 85(9):3115–9. doi: 10.1073/pnas.85.9.3115
45. Brusko TM, Wasserfall CH, Hulme MA, Cabrera R, Schatz D, Atkinson MA. Influence of membrane CD25 stability on T lymphocyte activity: implications for immunoregulation. PloS One (2009) 4(11):e7980. doi: 10.1371/journal.pone.0007980
46. Dendrou CA, Plagnol V, Fung E, Yang JH, Downes K, Cooper JD, et al. Cell-specific protein phenotypes for the autoimmune locus IL2RA using a genotype-selectable human bioresource. Nat Genet (2009) 41(9):1011–5. doi: 10.1038/ng.434
47. Aratani Y. Myeloperoxidase: Its role for host defense, inflammation, and neutrophil function. Arch Biochem Biophys (2018) 640:47–52. doi: 10.1016/j.abb.2018.01.004
48. Chiaramonte MG, Schopf LR, Neben TY, Cheever AW, Donaldson DD, Wynn TA. IL-13 is a key regulatory cytokine for Th2 cell-mediated pulmonary granuloma formation and IgE responses induced by schistosoma mansoni eggs. J Immunol (1999) 162(2):920–30.
49. Lee HL, Jang JW, Lee SW, Yoo SH, Kwon JH, Nam SW, et al. Inflammatory cytokines and change of Th1/Th2 balance as prognostic indicators for hepatocellular carcinoma in patients treated with transarterial chemoembolization. Sci Rep (2019) 9(1):3260. doi: 10.1038/s41598-019-40078-8
50. Mandoj C, Tomao L, Conti L. Coagulation in brain tumors: biological basis and clinical implications. Front Neurol (2019) 10:181. doi: 10.3389/fneur.2019.00181
51. Saidak Z, Soudet S, Lottin M, Salle V, Sevestre MA, Clatot F, et al. A pan-cancer analysis of the human tumor coagulome and its link to the tumor immune microenvironment. Cancer Immunol Immunother (2021) 70(4):923–33. doi: 10.1007/s00262-020-02739-w
52. Wen PY, Macdonald DR, Reardon DA, Cloughesy TF, Sorensen AG, Galanis E, et al. Updated response assessment criteria for high-grade gliomas: response assessment in neuro-oncology working group. J Clin Oncol (2010) 28(11):1963–72. doi: 10.1200/JCO.2009.26.3541
53. Pope WB, Sayre J, Perlina A, Villablanca JP, Mischel PS, Cloughesy TF. MR imaging correlates of survival in patients with high-grade gliomas. AJNR Am J Neuroradiol (2005) 26(10):2466–74.
54. Deshmane SL, Kremlev S, Amini S, Sawaya BE. Monocyte chemoattractant protein-1 (MCP-1): An overview. J Interferon Cytokine Res (2009) 29(6):313–26. doi: 10.1089/jir.2008.0027
55. Dinarello CA. Overview of the IL-1 family in innate inflammation and acquired immunity. Immunol Rev (2018) 281(1):8–27. doi: 10.1111/imr.12621
56. Civita P, Valerio O, Naccarato AG, Gumbleton M, Pilkington GJ. Satellitosis, a crosstalk between neurons, vascular structures and neoplastic cells in brain tumours; early manifestation of invasive behaviour. Cancers (Basel) (2020) 12(12):3720. doi: 10.3390/cancers12123720
57. Orci LA, Toso C, Mentha G, Morel P, Majno PE. Systematic review and meta-analysis of the effect of perioperative steroids on ischaemia-reperfusion injury and surgical stress response in patients undergoing liver resection. Br J Surg (2013) 100(5):600–9. doi: 10.1002/bjs.9035
58. Maneechotesuwan K, Kasetsinsombat K, Wongkajornsilp A, Barnes PJ. Role of autophagy in regulating interleukin-10 and the responses to corticosteroids and statins in asthma. Clin Exp Allergy (2021) 51(12):1553–65. doi: 10.1111/cea.13825
59. Sajadi SM, Khoramdelazad H, Hassanshahi G, Rafatpanah H, Hosseini J, Mahmoodi M, et al. Plasma levels of CXCL1 (GRO-alpha) and CXCL10 (IP-10) are elevated in type 2 diabetic patients: evidence for the involvement of inflammation and angiogenesis/angiostasis in this disease state. Clin Lab (2013) 59(1-2):133–7. doi: 10.7754/clin.lab.2012.120225
60. Downes K, Marcovecchio ML, Clarke P, Cooper JD, Ferreira RC, Howson JM, et al. Plasma concentrations of soluble IL-2 receptor α (CD25) are increased in type 1 diabetes and associated with reduced c-peptide levels in young patients. Diabetologia (2014) 57(2):366–72. doi: 10.1007/s00125-013-3113-8
61. Williams JC, Zhang X, Karki M, Chi YY, Wallet SM, Rudy BJ, et al. Soluble CD14, CD163, and CD27 biomarkers distinguish ART-suppressed youth living with HIV from healthy controls. J Leukoc Biol (2018) 103(4):671–80. doi: 10.1002/JLB.3A0717-294RR
62. Hadjadj J, Yatim N, Barnabei L, Corneau A, Boussier J, Smith N, et al. Impaired type I interferon activity and inflammatory responses in severe COVID-19 patients. Science (2020) 369(6504):718–24. doi: 10.1126/science.abc6027
63. Teixeira PC, Dorneles GP, Santana Filho PC, da Silva IM, Schipper LL, Postiga IAL, et al. Increased LPS levels coexist with systemic inflammation and result in monocyte activation in severe COVID-19 patients. Int Immunopharmacol (2021) 100:108125. doi: 10.1016/j.intimp.2021.108125
64. Ueland T, Heggelund L, Lind A, Holten AR, Tonby K, Michelsen AE, et al. Elevated plasma sTIM-3 levels in patients with severe COVID-19. J Allergy Clin Immunol (2021) 147(1):92–8. doi: 10.1016/j.jaci.2020.09.007
65. Prashant A, Vishwanath P, Kulkarni P, Sathya Narayana P, Gowdara V, Nataraj SM, et al. Comparative assessment of cytokines and other inflammatory markers for the early diagnosis of neonatal sepsis-a case control study. PloS One (2013) 8(7):e68426. doi: 10.1371/journal.pone.0068426
66. Pilarczyk K, Rath PM, Steinmann J, Thielmann M, Padosch SA, Dürbeck M, et al. Multiplex polymerase chain reaction to diagnose bloodstream infections in patients after cardiothoracic surgery. BMC Anesthesiol (2019) 19(1):59. doi: 10.1186/s12871-019-0727-5
67. Ziai WC, Parry-Jones AR, Thompson CB, Sansing LH, Mullen MT, Murthy SB, et al. Early inflammatory cytokine expression in cerebrospinal fluid of patients with spontaneous intraventricular hemorrhage. Biomolecules (2021) 11(8):1123. doi: 10.3390/biom11081123
68. Bodmer S, Huber D, Heid I, Fontana A. Human glioblastoma cell derived transforming growth factor-beta 2: evidence for secretion of both high and low molecular weight biologically active forms. J Neuroimmunol (1991) 34(1):33–42. doi: 10.1016/0165-5728(91)90096-p
69. Van Meir E, Ceska M, Effenberger F, Walz A, Grouzmann E, Desbaillets I, et al. Interleukin-8 is produced in neoplastic and infectious diseases of the human central nervous system. Cancer Res (1992) 52(16):4297–305.
70. Kuratsu J, Yoshizato K, Yoshimura T, Leonard EJ, Takeshima H, Ushio Y. Quantitative study of monocyte chemoattractant protein-1 (MCP-1) in cerebrospinal fluid and cyst fluid from patients with malignant glioma. J Natl Cancer Inst (1993) 85(22):1836–9. doi: 10.1093/jnci/85.22.1836
71. Takano S, Yoshii Y, Kondo S, Suzuki H, Maruno T, Shirai S, et al. Concentration of vascular endothelial growth factor in the serum and tumor tissue of brain tumor patients. Cancer Res (1996) 56(9):2185–90.
72. Stockhammer G, Obwegeser A, Kostron H, Schumacher P, Muigg A, Felber S, et al. Vascular endothelial growth factor (VEGF) is elevated in brain tumor cysts and correlates with tumor progression. Acta Neuropathol (2000) 100(1):101–5. doi: 10.1007/s004010051199
73. Jallo GI, Friedlander DR, Kelly PJ, Wisoff JH, Grumet M, Zagzag D. Tenascin-c expression in the cyst wall and fluid of human brain tumors correlates with angiogenesis. Neurosurgery (1997) 41(5):1052–9. doi: 10.1097/00006123-199711000-00007
74. Ishii N, Tada M, Sakuma S, Sawamura Y, Shinohe Y, Abe H. Human astrocytoma cells are capable of producing macrophage inflammatory protein-1beta. J Neurooncol (1998) 37(1):17–23. doi: 10.1023/a:1005959719927
75. Hoelscher M, Richter N, Melle C, von Eggeling F, Schaenzer A, Nestler U. SELDI-TOF analysis of glioblastoma cyst fluid is an approach for assessing cellular protein expression. Neurol Res (2013) 35(10):993–1001. doi: 10.1179/016164113X13756993777580
76. Shen F, Zhang Y, Yao Y, Hua W, Zhang HS, Wu JS, et al. Proteomic analysis of cerebrospinal fluid: toward the identification of biomarkers for gliomas. Neurosurg Rev (2014) 37(3):367–80. doi: 10.1007/s10143-014-0539-5
77. Wachowiak R, Krause M, Mayer S, Peukert N, Suttkus A, Müller WC, et al. Increased L1CAM (CD171) levels are associated with glioblastoma and metastatic brain tumors. Medicine (Baltimore) (2018) 97(38):e12396. doi: 10.1097/MD.0000000000012396
78. Bajpai A, Prasad KN, Mishra P, Singh AK, Gupta RK, Ojha BK. Distinct cytokine pattern in response to different bacterial pathogens in human brain abscess. J Neuroimmunol (2014) 273(1-2):96–102. doi: 10.1016/j.jneuroim.2014.05.009
79. Kielian T, Bearden ED, Baldwin AC, Esen N. IL-1 and TNF-alpha play a pivotal role in the host immune response in a mouse model of staphylococcus aureus-induced experimental brain abscess. J Neuropathol Exp Neurol (2004) 63(4):381–96. doi: 10.1093/jnen/63.4.381
80. Kielian T, Phulwani NK, Esen N, Syed MM, Haney AC, McCastlain K, et al. MyD88-dependent signals are essential for the host immune response in experimental brain abscess. J Immunol (2007) 178(7):4528–37. doi: 10.4049/jimmunol.178.7.4528
81. Stenzel W, Soltek S, Miletic H, Hermann MM, Körner H, Sedgwick JD, et al. An essential role for tumor necrosis factor in the formation of experimental murine staphylococcus aureus-induced brain abscess and clearance. J Neuropathol Exp Neurol (2005) 64(1):27–36. doi: 10.1093/jnen/64.1.27
82. Xia M, Qin S, McNamara M, Mackay C, Hyman BT. Interleukin-8 receptor b immunoreactivity in brain and neuritic plaques of alzheimer's disease. Am J Pathol (1997) 150(4):1267–74.
83. Tsai HH, Frost E, To V, Robinson S, Ffrench-Constant C, Geertman R, et al. The chemokine receptor CXCR2 controls positioning of oligodendrocyte precursors in developing spinal cord by arresting their migration. Cell (2002) 110(3):373–83. doi: 10.1016/s0092-8674(02)00838-3
84. Hu J, Zhao Q, Kong LY, Wang J, Yan J, Xia X, et al. Regulation of tumor immune suppression and cancer cell survival by CXCL1/2 elevation in glioblastoma multiforme. Sci Adv (2021) 7(5):eabc2511. doi: 10.1126/sciadv.abc2511
85. Semple BD, Kossmann T, Morganti-Kossmann MC. Role of chemokines in CNS health and pathology: A focus on the CCL2/CCR2 and CXCL8/CXCR2 networks. J Cereb Blood Flow Metab (2010) 30(3):459–73. doi: 10.1038/jcbfm.2009.240
86. Chang AL, Miska J, Wainwright DA, Dey M, Rivetta CV, Yu D, et al. CCL2 produced by the glioma microenvironment is essential for the recruitment of regulatory T cells and myeloid-derived suppressor cells. Cancer Res (2016) 76(19):5671–82. doi: 10.1158/0008-5472.CAN-16-0144
87. Campbell IL, Erta M, Lim SL, Frausto R, May U, Rose-John S, et al. Trans-signaling is a dominant mechanism for the pathogenic actions of interleukin-6 in the brain. J Neurosci (2014) 34(7):2503–13. doi: 10.1523/JNEUROSCI.2830-13.2014
88. Wareham LK, Echevarria FD, Sousa JL, Konlian DO, Dallas G, Formichella CR, et al. Interleukin-6 promotes microtubule stability in axons via Stat3 protein-protein interactions. iScience (2021) 24(10):103141. doi: 10.1016/j.isci.2021.103141
89. Probert L. TNF and its receptors in the CNS: The essential, the desirable and the deleterious effects. Neuroscience (2015) 302:2–22. doi: 10.1016/j.neuroscience.2015.06.038
90. Schwartz M, Solomon A, Lavie V, Ben-Bassat S, Belkin M, Cohen A. Tumor necrosis factor facilitates regeneration of injured central nervous system axons. Brain Res (1991) 545(1-2):334–8. doi: 10.1016/0006-8993(91)91309-o
91. Parish CL, Finkelstein DI, Tripanichkul W, Satoskar AR, Drago J, Horne MK. The role of interleukin-1, interleukin-6, and glia in inducing growth of neuronal terminal arbors in mice. J Neurosci (2002) 22(18):8034–41. doi: 10.1523/JNEUROSCI.22-18-08034.2002
92. Cafferty WB, Gardiner NJ, Das P, Qiu J, McMahon SB, Thompson SW. Conditioning injury-induced spinal axon regeneration fails in interleukin-6 knock-out mice. J Neurosci (2004) 24(18):4432–43. doi: 10.1523/JNEUROSCI.2245-02.2004
93. Kwon MJ, Shin HY, Cui Y, Kim H, Thi AH, Choi JY, et al. CCL2 mediates neuron-macrophage interactions to drive proregenerative macrophage activation following preconditioning injury. J Neurosci (2015) 35(48):15934–47. doi: 10.1523/JNEUROSCI.1924-15.2015
94. Schoenegger K, Oberndorfer S, Wuschitz B, Struhal W, Hainfellner J, Prayer D, et al. Peritumoral edema on MRI at initial diagnosis: An independent prognostic factor for glioblastoma? Eur J Neurol (2009) 16(7):874–8. doi: 10.1111/j.1468-1331.2009.02613.x
95. Liang HT, Chen WY, Lai SF, Su MY, You SL, Chen LH, et al. The extent of edema and tumor synchronous invasion into the subventricular zone and corpus callosum classify outcomes and radiotherapy strategies of glioblastomas. Radiother Oncol (2017) 125(2):248–57. doi: 10.1016/j.radonc.2017.09.024
96. Ramezani S, Vousooghi N, Joghataei MT, Chabok SY. The role of kinase signaling in resistance to bevacizumab therapy for glioblastoma multiforme. Cancer Biother Radiopharm (2019) 34(6):345–54. doi: 10.1089/cbr.2018.2651
97. Matsumoto T, Ikeda K, Mukaida N, Harada A, Matsumoto Y, Yamashita J, et al. Prevention of cerebral edema and infarct in cerebral reperfusion injury by an antibody to interleukin-8. Lab Invest (1997) 77(2):119–25.
98. Bémeur C, Qu H, Desjardins P, Butterworth RF. IL-1 or TNF receptor gene deletion delays onset of encephalopathy and attenuates brain edema in experimental acute liver failure. Neurochem Int (2010) 56(2):213–5. doi: 10.1016/j.neuint.2009.11.010
99. Park KJ, Kang SH, Chae YS, Yu MO, Cho TH, Suh JK, et al. Influence of interleukin-6 on the development of peritumoral brain edema in meningiomas. J Neurosurg (2010) 112(1):73–80. doi: 10.3171/2009.4.JNS09158
100. Clausen F, Hånell A, Israelsson C, Hedin J, Ebendal T, Mir AK, et al. Neutralization of interleukin-1β reduces cerebral edema and tissue loss and improves late cognitive outcome following traumatic brain injury in mice. Eur J Neurosci (2011) 34(1):110–23. doi: 10.1111/j.1460-9568.2011.07723.x
101. Herting CJ, Chen Z, Maximov V, Duffy A, Szulzewsky F, Shayakhmetov DM, et al. Tumour-associated macrophage-derived interleukin-1 mediates glioblastoma-associated cerebral oedema. Brain (2019) 142(12):3834–51. doi: 10.1093/brain/awz331
102. Lange F, Hartung J, Liebelt C, Boisserée J, Resch T, Porath K, et al. Perampanel add-on to standard radiochemotherapy in vivo promotes neuroprotection in a rodent F98 glioma model. Front Neurosci (2020) 14:598266. doi: 10.3389/fnins.2020.598266
103. Rigamonti A, Imbesi F, Silvani A, Gaviani P, Agostoni E, Porcu L, et al. Antiepileptic treatment and survival in newly diagnosed glioblastoma patients: Retrospective multicentre study in 285 Italian patients. J Neurol Sci (2018) 390:14–9. doi: 10.1016/j.jns.2018.04.004
Keywords: glioblastoma, macrophage, cytokine, tumor microenvironment, brain abscess, pus, inflammation, cyst fluid
Citation: Hassel B, Niehusmann P, Halvorsen B and Dahlberg D (2022) Pro-inflammatory cytokines in cystic glioblastoma: A quantitative study with a comparison with bacterial brain abscesses. With an MRI investigation of displacement and destruction of the brain tissue surrounding a glioblastoma. Front. Oncol. 12:846674. doi: 10.3389/fonc.2022.846674
Received: 07 January 2022; Accepted: 27 June 2022;
Published: 29 July 2022.
Edited by:
Andrea Salmaggi, ASST Lecco, ItalyReviewed by:
Roberto Würth, German Cancer Research Center (DKFZ), GermanySubhas K Konar, National Institute of Mental Health and Neurosciences (NIMHANS), India
Copyright © 2022 Hassel, Niehusmann, Halvorsen and Dahlberg. This is an open-access article distributed under the terms of the Creative Commons Attribution License (CC BY). The use, distribution or reproduction in other forums is permitted, provided the original author(s) and the copyright owner(s) are credited and that the original publication in this journal is cited, in accordance with accepted academic practice. No use, distribution or reproduction is permitted which does not comply with these terms.
*Correspondence: Bjørnar Hassel, Ympvcm5hci5oYXNzZWxAbWVkaXNpbi51aW8ubm8=