- 1Translational Cancer Biology Research Unit, Cancer Research Malaysia, Subang Jaya, Malaysia
- 2Department of Oral and Maxillofacial Clinical Sciences, Faculty of Dentistry, University of Malaya, Kuala Lumpur, Malaysia
Immunotherapy, especially the immune checkpoint inhibitors (ICIs) such as the pembrolizumab and nivolumab have contributed to significant improvements in treatment outcomes and survival of head and neck cancer (HNC) patients. Still, only a subset of patients benefits from ICIs and hence the race is on to identify combination therapies that could improve response rates. Increasingly, genetic alterations that occur within cancer cells have been shown to modulate the tumor microenvironment resulting in immune evasion, and these have led to the emergence of trials that rationalize a combination of targeted therapy with immunotherapy. In this review, we aim to provide an overview of the biological rationale and current strategies of combining targeted therapy with the approved ICIs in HNC. We summarize the ongoing combinatorial clinical trials and discuss emerging immunomodulatory targets. We also discuss the challenges and gaps that have yet to be addressed, as well as future perspectives in combining these different drug classes.
Introduction
The immune checkpoint inhibitors (ICIs) have led to a paradigm shift in the treatment modalities for advanced-stage head and neck cancer (HNC) patients. In 2016, two ICIs targeting the programmed cell death -1 (PD-1), pembrolizumab and nivolumab were approved as second-line treatment for recurrent and metastatic (R/M) HNC (1, 2), and subsequently, pembrolizumab was approved as first-line treatment for advanced-stage HNC in 2019 (3). PD-1 is a membrane-bound receptor found on immune cells such as T cells, that upon binding to its ligand, programmed death ligand-1 (PD-L1) found on tumor cells, can prevent the attack by the cytolytic T cell, allowing cancer cells to escape from immune surveillance (4). Hence, ICIs that can block the PD1/PD-L1 interaction represents a promising therapeutic strategy (4).
Besides, being a relatively immune-inflamed cancer, the use of immunotherapy for HNC is rational since cytolytic T cells, and natural killer (NK) cells are present in high abundance in the tumor (5). However, HNC is also a relatively immunosuppressive tumor that have also developed other mechanisms to dampen or evade the immune system, suggesting that merely blocking these checkpoint molecules is not sufficient. These include tumor intrinsic genomic alterations that drive cancer development, presenting an opportunity to simultaneously suppress oncogenic signals and enhance immune activation to sensitize tumors to ICIs. Many molecular targeted agents in combination with ICIs are in clinical testing, and some have been approved as the standard of care, such as lenvatinib (6) and axitinib (7). In addition, actionable driver mutations such as in EGFR (8), BRAF (9), KRAS (10) could contribute to immune evasion, driving the emergence of new clinical trials to investigate the synergy between targeting these genetic events to improve ICIs response. Furthermore, common oncogenic mutations in HNC such as those leading to altered p53 or Wnt/beta-catenin signaling were found to modulate the immune microenvironment and potentially affect response towards ICIs (11–15). In particular, among metastatic HNC, p53 mutation was reported to be negative predictor of response towards ICIs (12).
The immunomodulatory mechanisms of the targeted agents might however be tissue- or context-specific (dependent on the genetic drivers). HNC comprises of tumors from the oral cavity, oropharynx, hypopharynx, and larynx. About 25% of HNC is estimated to be associated with human papilloma virus (HPV) infection, and this is especially prevalent and have prognostic implication in oropharyngeal cancer (16). Besides being molecularly and genetically distinct, HPV-positive HNC is also different from HPV-negative HNC in terms of its immune landscape (17), where HPV-positive HNC showed significantly higher immune cells infiltration and CD8 T cell activation (5). Although the KEYNOTE-012 trial has shown better response rates to pembrolizumab amongst HPV-positive HNC (18, 19), KEYNOTE-048 reported that clinical benefit is seen regardless of HPV status (20). More systematic review on the implications of HPV positivity in immunotherapy responses in HNC have been published recently in 17 and Wang et al., 2021 (21).
However, there remains a scarcity of information on the targeted agents that could synergize with ICIs in HNC. Hence, this review serves to provide an overview of the current strategies in combining targeted therapy with ICIs, as well as emerging immunomodulatory targets for HNC. Challenges and future perspectives will also be discussed.
Targeted Agents Tested in Combination With ICIs in HNC
Clinical trials in HNC reporting combination therapies involving ICIs, were identified from clinicaltrials.gov, with the search terms of “Head and Neck Cancer” for “Condition or disease” and “pembrolizumab”, “nivolumab”, “atezolimumab”, or “durvalumab” under “Other terms” [Curated on 1st Sep 2021]. A total of 49 trials involved the combination of molecular targeted agents with ICIs were summarized in Table 1 (Details in Supplementary Table 1 and Supplementary Figure 1). Whereas Figure 1 provides a schematic summary of current strategies and their corresponding mechanisms of action in immunomodulation. Whilst most of these trials are still in their early phases, we hope to provide an overview and discuss the rationale of these combinations as detailed below.
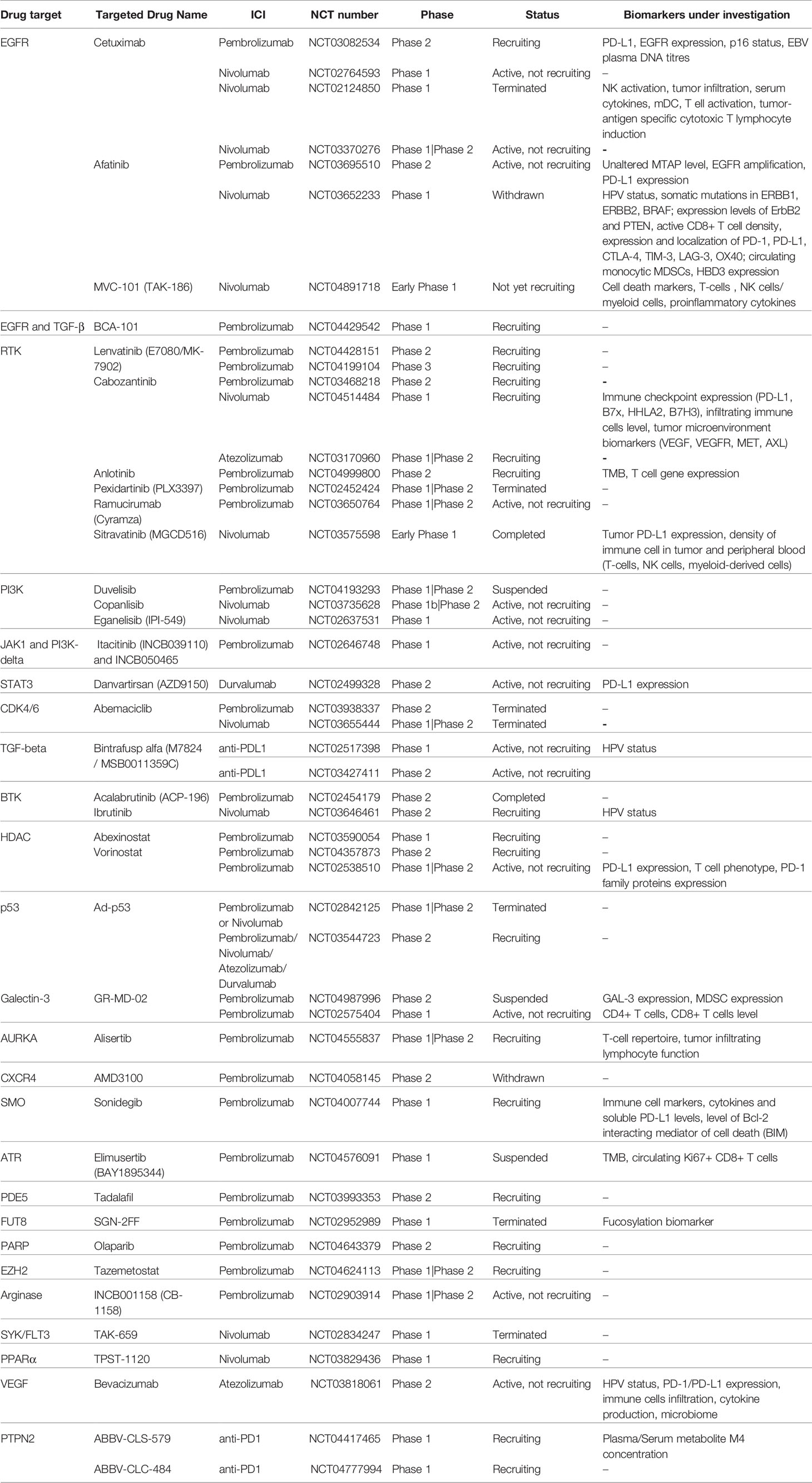
Table 1 Overview of clinical trials involving the combination of targeted agents with ICIs for HNC patients.
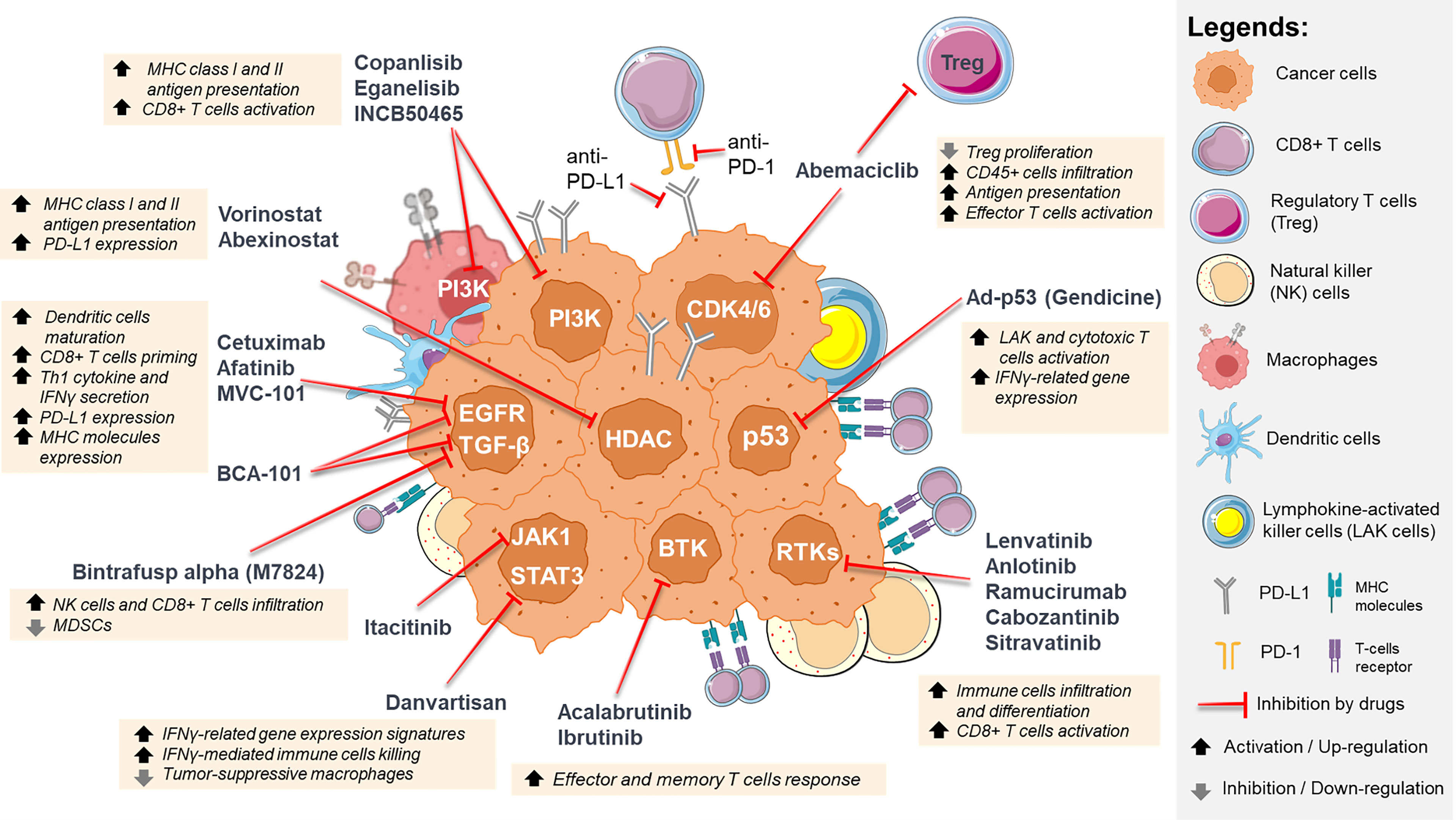
Figure 1 Summary of the immunomodulatory effects of inhibiting oncogenic signaling pathways in HNC. These targeted agents not only result in tumor-intrinsic killing, but also modulate the tumor microenvironment, such as increasing immune cell infiltration, activation and differentiation, increasing MHC class I and II antigen presentation, increasing PD-L1 expression, and inhibiting regulatory T cells proliferation. *For illustration purpose only, icons are not drawn in actual scale ratio. ** Some icons are taken from Servier Medical Art (smart.servier.com).
EGFR Inhibitors
Epidermal growth factor receptor (EGFR) is overexpressed in more than 80% of HNC (22) and in addition to its oncogenic role, activated EGFR signaling modulates the immune microenvironment, helping tumors to escape from the immune system. Therefore, EGFR inhibitors have important immune-related mechanisms of action. Cetuximab, an IgG1 monoclonal antibody (mAb) against EGFR is the only approved targeted therapy for HNC. Cetuximab can exert its anti-tumor activity through antibody-dependent cell-mediated cytotoxicity that is triggered by the binding of the IgG-Fc part of cetuximab to the CD16 molecule on NK cells (23). Cetuximab triggers the maturation of dendritic cells (DC) causing the initiation of adaptive immune responses through CD8+ T cells priming and Th1 cytokine release, resulting in antigen-specific immune responses in HNC patients (24). EGFR signaling downregulates the antigen presentation machinery, enabling HNC to evade the immune system. Inhibiting EGFR signaling restores the expression of major histocompatibility complex (MHC) molecules, and enhances secretion of interferon-gamma (IFNγ) which increases PD-L1 expression on tumor and immune cells within the tumor microenvironment (TME) (25). Two Phase 2 trials are investigating the combination of cetuximab with either pembrolizumab [NCT03082534] or nivolumab [NCT03370276] whilst the combination of cetuximab and nivolumab is being tested in a Phase 1 trial for p16-negative local-regionally advanced HNC [NCT02764593]. In NCT03082534, where patients received cetuximab and pembrolizumab, a promising overall response rate (ORR) of 45% among platinum-refractory patients with no prior anti-EGFR/immunotherapy, was reported (26). On the other hand, for the nivolumab and cetuximab combination in platinum-refractory R/M HNC, the 1-year progression free survival (PFS) and overall survival (OS) rates were 19% and 44%, respectively. Although patients with no prior ICI showed improved PFS and OS compared to those with prior ICI, the difference was not significant (27).
High-throughput screening of small molecule inhibitors also showed that EGFR inhibitors (including erlotinib, afatinib and gefitinib) could enhance T cell-mediated killing (28). Clinically, afatinib is being tested with pembrolizumab for platinum-refractory R/M HNC in a Phase 2 trial [NCT03695510 – ALPHA study] unveiling an impressive ORR of 41.4% (29), a drastic increase compared to 16% or 10-10.8% for pembrolizumab and afatinib monotherapy respectively (30, 31). Biomarkers predictive of response were also investigated in this study, whereby MTAP loss or mutation was found to be associated with lower response rates, while EGFR amplification was associated with 100% ORR. On the other hand, high PD-L1 (CPS score ≥20) was associated with higher ORR (63% vs 35% in CPS score <20), albeit not statistically significant (Fisher’s exact p-value = 0.23) (29).
A Phase 1 study was initiated to study the pharmacodynamics of MVC-101 and nivolumab within the TME [NCT04891718]. MVC-101, is a conditionally active T cell engager that contains two anti-EGFR antibodies, designed for tumor cell- selective targeting (32). Similarly, BCA-101, a chimeric EGFR/TGFβ-targeting antibody is being tested in a Phase 1 trial with pembrolizumab [NCT04429542], which could address not only the immunosuppressive mechanisms (33), but also overcome the resistance to EGFR inhibitors brought on by upregulation of signaling (34, 35).
RTK Inhibitors
Besides EGFR, other receptor tyrosine kinases (RTKs) implicated in HNC include the vascular endothelial growth factor receptor (VEGFR) and fibroblast growth factor receptor (FGFR). Clinical studies have demonstrated the synergistic effects of combining anti-angiogenic therapy with immunotherapy (36–38), as these could enhance immune cell differentiation and infiltration, and overcome the immune suppressive function of VEGF (38–40). In HNC, lenvatinib is being tested with pembrolizumab as first-line and second-line treatment [Phase 3, NCT04199104; Phase 2, NCT04428151]. Notably, lenvatinib has an immunomodulatory function by activating CD8+ T cells (41) and this combination treatment is approved for advanced RCC and endometrial cancer (42). Carbozantinib, an inhibitor of MET and VEGFR2 is also being tested with pembrolizumab [Phase 2, NCT03468218], nivolumab [Phase 1, NCT04514484] and atezolizumab [Phase 1/2, NCT03170960]. Further, anlotinib is being investigated with pembrolizumab as first-line therapy for R/M HNC with a combined positive score (CPS) ≥ 1 in a Phase 2 trial [NCT04999800]. Another VEGFR2 inhibitor ramucirumab, is undergoing Phase 2 trial with pembrolizumab [NCT03650764] whilst sitravatinib, a VEGFR inhibitor that also targets the TAM receptor family (TYRO3, AXL and MERTK) was combined with nivolumab in a window-of-opportunity (WOO) trial in neoadjuvant setting [NCT03575598] (42). Here, nine of ten patients showed clinical-to-pathological downstaging and immunophenotyping analyses confirmed the immunomodulatory effect of sitravatinib (42). Further investigation is needed to evaluate if this is contributed by the inhibition of the TAM receptors, which play a critical role in dampening NK cells mediated anti-tumor response (43, 44).
PI3K Inhibitors
Oncogenic mutations in PIK3CA and dysregulation of PI3K signaling have been actively investigated as a therapeutic target in HNC (45, 46). Whilst exerting tumor intrinsic effect on HNC growth, targeting PI3K signaling could also reverse its immune-suppressive effects. Activating mutations in PIK3CA or PTEN loss which are both frequent events in HNC, represses the induction of MHC class I and II expression by IFNγ (47). PI3K inhibitors, dactolisib and pictilisib can induce MHC molecules expression in HNC cell lines (47). PD-1 blockade has been shown to upregulate another checkpoint receptor, Tim-3 via AKT/S6 pathway which could allow the escape of anti-PD-1 blockade in the TME, providing further support for concomitant targeting AKT/S6 to improve the efficacy of ICIs (48). Recently, targeting of PI3Kγ/δ in the leucocytes using TG100-115 or IPI-145 has been shown to improve ICIs efficacy in pre-clinical HNC models (27–29). This is likely attributed by the abrogation of myeloid-derived suppressor cells (MDSC)-mediated immune suppression (49). However, at high dose, IPI-145 was shown to negatively impact the priming and effector function of antigen-specific T cells, reversing the tumor control achievable by low dose IPI-145 (49). Considering the narrow therapeutic window in the combination of PI3K inhibitors with ICI, careful trial design in scheduling and dosing is warranted.
Currently, three PI3K inhibitors are being tested with ICIs in HNC trials. Previous pharmacodynamic study of copanlisib [NCT02155582] showed suppression of factors associated with macrophages and regulatory T cells (Tregs), supporting the rationale for the combination with nivolumab [NCT03735628]. Another Phase 1 trial combined eganelisib (PI3Kγ inhibitor) with nivolumab, demonstrating positive response (ORR=20%) and disease control rates of 40% at interim analysis (50), among those who had one or two prior lines of chemotherapy. Following the encouraging outcome, a Phase 2 WOO study [NCT03795610] is ongoing for locally advanced HNC to evaluate the effect of eganelisib in modulating a PI3Kγ-mediated immune-suppressive signature. Interim biomarker analysis from another Phase 1 dose-finding trial [NCT02646748] showed that the PI3K-δ inhibitor (INCB050465) enhanced T cell activation, leading to the improved outcome when combined with pembrolizumab (51).
JAK/STAT Inhibitors
Activated JAK/STAT signaling increases tumor cell proliferation, treatment resistance and immune evasion in HNC (52, 53). A Phase 1 trial [NCT02646748] investigated itacitinib, a JAK inhibitor with pembrolizumab, but was inferior to the arm with PI3K-δ inhibitor plus pembrolizumab (51). The antagonistic effect of JAK inhibition with pembrolizumab, may be caused by the suppression of STAT1, which impairs IFNγ-mediated immune cell killing (54, 55). Indeed, trials in other solid tumors were terminated early due to poor efficacy, speculated to be caused by the impairment of the immune cell function with JAK inhibition (53, 56). While the inhibition of JAK has yielded contradicting results (53, 56), the selective inhibition of STAT3 is more promising as this targets both tumor intrinsic pathway signaling as well as STAT3 inhibition within the immune cells (54). Recently, the development of therapeutic antisense oligonucleotide such as danvartisan (AZD5190) has enabled the selective targeting of STAT3. On-treatment biopsies analysis from a Phase 1 study [NCT01563302] showed that danvatirsan upregulated IFNγ-related gene expression signatures (GES) (54). Furthermore, danvatirsan promoted pro-inflammatory cytokine expression and depleted immunosuppressive macrophages, enhancing T cell killing in syngeneic models (54). A Phase 2 trial investigating danvatirsan plus durvalumab (anti-PD-L1) as second-line treatment, is currently ongoing [NCT02499328]. Interim analysis showed an enhanced response (ORR of 26%) among second-line anti-PD-L1 naïve patients, compared with durvalumab with a CXCR2 inhibitor (AZD5069; ORR of 10%) with responses independent of HPV or PD-L1 status (57), and with on-treatment biopsies showing upregulation of IFNγ GES (57).
Cell Cycle Inhibitors
The cell cycle pathway is altered in almost all HNC (58), with CDK4 and CDK6 among the most studied drug targets (59). Abemaciclib, an inhibitor of CDK4/6 showed anti-tumor effect in HNC patient-derived xenograft harboring CCND1 amplification and/or CDKN2A mutation. The immune-modulatory functions of CDK4/6 inhibitors include increasing antigen representation, promoting infiltration of CD45+ cells into the tumor, activating effector T cells and inhibiting Treg proliferation (60, 61). A WOO trial [NCT04169074] is accessing the immune-modulation function of abemaciclib, among HPV-negative HNC patients in a neoadjuvant setting. Disappointingly, two trials in HNC [NCT03938337, NCT03655444] which tested the combination of abemaciclib with pembrolizumab and nivolumab respectively, were terminated early. The former was terminated due to high incidence of pulmonary toxicities, including death. Consistently, adverse events were also reported for non-small-cell lung cancer (62). Given the poor risk-benefit profile of the combination from several trials, the combination of CDK4/6 inhibitors with ICI would require dose adjustments and biomarkers that could identify the most suitable patients for this treatment.
TGF-β Inhibitors
Elevated expression of TGF-β is common in HNC and is associated with advanced disease and poor clinical outcome (63). Importantly, TGF-β signaling regulates both innate and adaptive immune signaling, impacting diverse types of immune cells (64). A first-in-class, bifunctional fusion protein, bintrafusp alfa (M7824) has been developed, composing of the extracellular domain of the human TGF-β receptor II, fused with an IgG1 avelumab-like anti-PDL1 antibody (65). M7824 increased infiltration of CD8+ T cells and NK cells into the tumor, while depleting MDSC in a murine model (66). Results from a Phase 1 study [NCT02517398] showed an ORR of 13%-16%, and a total clinical response rate of 22% (65). Encouragingly, early-phase trials of M7824 demonstrated efficacy seen in heavily pre-treated patients with “cold” tumors, whom tumor progressed/recurred after platinum therapy and are immunotherapy naive (66). Responses were seen regardless of PD-L1 status (ORR of 12% in PD-L1-positive vs 17% in PD-L1-negative), but HPV-positive HNC had significantly higher response rate (ORR of 33% in HPV-positive versus 5% in HPV-negative), perhaps due to the more enriched pre-existing immune response in these viral-driven cancers (65). As a result, a Phase 2 trial is now ongoing for HPV-associated oropharyngeal cancers [NCT03427411].
Others
Bruton tyrosine kinase (BTK) has recently shown ectopic expression in some solid tumors (67). Despite the low efficacy as a single agent, preclinical studies showed synergy in improving survival when BTK inhibitors were combined with immunotherapy (67), demonstrating enhanced effector and memory T cells responses (68). Ibrutinib with nivolumab are being tested in a Phase 2 trial for R/M HNC [NCT03646461], with patients stratified by HPV status and randomized in a 1:1 ratio to ibrutinib + cetuximab or ibrutinib + nivolumab arms. Another BTK inhibitor, acalabrutinib is being tested with pembrolizumab in advanced HNC patients, unfortunately all patients have discontinued treatment due to the lack of efficacy (ORR for combination = 16.7% vs pembrolizumab = 18.9%) and increase in adverse events [NCT02454179]. The limited clinical benefit and increased toxicity make it unfavorable to pursue BTK inhibitor in combination with ICI in HNC.
HNC shows hypoacetylated chromatin and inhibition of the histone deacetylases (HDAC) is seen as a promising strategy in targeting the cancer-stem-cell population of HNC (69). HDAC inhibitors can upregulate PD-L1 and MHC class I/II expression (70) and are being actively investigated for their role in potentiating response to ICIs (71). A recently completed Phase 2 trial [NCT02538510] showed favorable activity of vorinostat and pembrolizumab in HNC, with a higher ORR of 32% than pembrolizumab alone, albeit at the expense of higher toxicity (72). Another two trials combining either vorinostat [NCT04357873] or abexinostat [NCT03590054] with pembrolizumab are in Phase 1.
Gene therapy that restores functional tumor suppressor has also been explored. Gendicine, a gene therapy to deliver functional wildtype p53 into tumor cells was approved for HNC in 2003 in China (73). Experimental data showed that gendicine can activate lymphokine-activated killer cells (LAK) and cytotoxic T cells (73). A Phase 2 trial [NCT03544723] is ongoing to investigate the combination of gendicine with clinicians’ choice of ICIs. Preliminary gene expression analysis comparing pre- and post- gendicine-treated HNC tumor revealed upregulation of IFNγ-signaling genes, decreased TGF-β and β-catenin signaling (74) and increased CD8+ T cells signature, which is associated with increased clinical responses to ICIs (74).
New immunomodulatory targets have also been identified in vivo immuno-CRISPR/Cas9 screens and PTPN2 is among one of the top hits (75). CRISPR-mediated knockout of PTPN2 sensitized response towards anti-PD1 treatment, by enhancing IFNγ-mediated antigen presentation and increasing cytotoxic Tim-3+ CD8+ Tcells (76, 77). Two novel inhibitors targeting PTPN2 - BBV-CLS-579 [NCT04417465] and ABBV-CLS-484 [NCT04777994] are now being tested in combination with anti-PD1 in Phase 1 clinical trials, for LA and metastatic solid tumors, including HNC. Other targets from these screens shown to be able to enhance immune response through T cell suppression, M1 macrophage polarization and/or PD-L1 regulation include Asf1a (78) and eIF5B (79).
Other drugs targeting Galectin-3 (GR-MD-02), AURKA (alisertib), SMO (sonidegib), PDE5 (tadalafil), PARP (olaparib), EZH2 (tazemotostat), arginase (INCB001158) are in Phase 1 or 2 trials, in combination with pembrolizumab (Table 1). The PPARα antagonist, TPST-1120 in combination with nivolumab is in Phase 1 for advanced HNC [NCT03829436]. Whereas bevacizumab, a mAb targeting soluble VEGF is being tested with atezolizumab in a Phase 1 trial [NCT03818061]. Results from these trials will provide further insights into pathways that confer immunomodulatory effects in HNC.
Emerging Immunomodulatory Targets
Other emerging immunomodulatory targets in HNC preclinical studies or in clinical studies of other cancers are discussed below.
MAPK Pathway
The mitogen-activated protein kinases (MAPKs) are key regulators of cellular proliferation contributing to HNC progression (80). Apart from the established oncogenic roles, MAPK signaling pathway also promotes immunosuppression. In HNC cell lines, trametinib could enhance expressions of MHC class I and PD-L1 via STAT3 activation (81). The combination of trametinib with anti-PD-L1 significantly delayed tumor growth in HNC syngeneic models, possibly via increased infiltration of CD8+ T cells and enhanced antigen-presentation (81). Interestingly, HRAS mutations which are predominantly found in HNC are associated with elevated antitumor immune signatures (11). MAPK-mutated HNC wasa also found to be CD8+ T cell inflamed and inherently harbor immunoactive TME (82). Consistently, HNC with MAPK pathway mutations has been associated with better patient survival upon anti-PD1/PD-L1 treatment (82). Although no combinatorial trials for HNC are being tested currently, findings using syngenic models demonstrated that preceding MAPK inhibition with anti-PD-1/L1 could enhance CD8+ T cells clonal expansion and clinical investigation is warranted (83).
MTOR Pathway
Mammalian target of Rapamycin (mTOR) is one of the critical intracellular kinases within the oncogenic PI3K/AKT pathway. A combination of rapamycin and anti-PD-L1 mAb increased the durability of tumor responses and survival in highly immunogenic 4MOSC1 tumors (84). The addition of rapamycin preserved antigen-specific CD8+ tumor-infiltrating lymphocytes (TIL) and enhanced IFNγ secretion in both peripheral and infiltrating CD8+ T cells, leading to upregulation of MHC class I expression (84). Whilst it appears counterintuitive to use rapamycin which is clinically used as an immunosuppressant in transplant patients, emerging evidence has shown that inhibiting mTOR confers immune-activating function, particularly by promoting CD8+ T cells generation (85), suggesting therapeutic opportunities to boost the efficacy of immunotherapy.
Discussion
Challenges and Future Perspective
The scarcity of suitable mouse models for the immunological study of HNC is a major challenge. Only lately, the 4−nitroquinoline−1−oxide induced tumor in the immunocompetent C57BL/6 mice has led to the establishment of the JC1-2 and 4-MOSC syngeneic models (86, 87). These models are instrumental in identifying novel immunomodulatory targets and evaluating new immune-oncology drugs for HNC. The dynamic changes in the TME and immune landscape during treatment can be comprehensively studied using high-resolution technologies such as the single-cell sequencing and spatial-omics, affording the opportunity to identify immune-modulatory effects of specific drug and drug combinations. Intratumor heterogeneity and plasticity of the TME are two other challenges that could influence the outcome of combining targeted therapy with immunotherapy. Further, an appropriate dosage, the timing and sequence of the combination needs to be considered carefully for optimal benefit-risk profile.
Finally, the successful application of precision medicine is highly reliant on clinically-relevant predictive biomarkers, a major challenge that is still being overcome. In HNC, other than PD-L1 CPS for pembrolizumab, no other clinical biomarker has made its way to the clinic. The advent of genomic, transcriptomic, proteomic, and immunomic, which allow comprehensive integrative analysis, could lead to a better understanding of clinical response, driving the identification of specific biomarkers for each drug combination.
Conclusion
Combinations of ICIs with targeted agents have shown promising clinical efficacy, particularly those targeting EGFR (cetuximab, afatinib), RTK (lenvatinib, sitravatinib), STAT3 (danvartisan) and TGF-β (M7824). As most trials are still in early stages, an improved understanding of the genomics and immunology interplay and the lessons learned from trials in other solid tumors could guide rational and optimal trial designs in HNC. Furthermore, the increasing availability of HNC syngenic models and the application of advanced omic technologies could further fuel the development of combinatorial targeted therapy with ICIs further maximizing the clinical benefit of immunotherapy.
Author Contributions
Conceptualization - AC and SC. Data curation, figure preparation - AC. Writing, reviewing & editing - AC, PY, and SC. Supervision – SC. All authors contributed to the article and approved the submitted version.
Conflict of Interest
The authors declare that the research was conducted in the absence of any commercial or financial relationships that could be construed as a potential conflict of interest.
Publisher’s Note
All claims expressed in this article are solely those of the authors and do not necessarily represent those of their affiliated organizations, or those of the publisher, the editors and the reviewers. Any product that may be evaluated in this article, or claim that may be made by its manufacturer, is not guaranteed or endorsed by the publisher.
Acknowledgments
We thank Dr. Kue Peng Lim, the Head of Cancer Immunology and Immunotherapy Research Unit at Cancer Research Malaysia, for her critical review and constructive feedback on the manuscript. SC is supported by the Dr Siti Hasmah Mohd Ali Professional Chair Award. AC is supported by the Dr. Ranjeet Bhagwan Singh Research Grant 2021. AC and SC are supported by the Ong Hin Tiang & Ong Sek Pek Foundation, and other supporters of Cancer Research Malaysia.
Supplementary Material
The Supplementary Material for this article can be found online at: https://www.frontiersin.org/articles/10.3389/fonc.2022.837835/full#supplementary-material
References
1. FDA, U. S. Pembrolizumab (KEYTRUDA) (2016). Available at: https://www.fda.gov/drugs/resources-information-approved-drugs/pembrolizumab-keytruda.
2. FDA, U. S. Nivolumab for SCCHN (2016). Available at: https://www.fda.gov/drugs/resources-information-approved-drugs/nivolumab-scchn.
3. FDA, U. S. FDA Approves Pembrolizumab for First-Line Treatment of Head and Neck Squamous Cell Carcinoma (2019). Available at: https://www.fda.gov/drugs/resources-information-approved-drugs/fda-approves-pembrolizumab-first-line-treatment-head-and-neck-squamous-cell-carcinoma.
4. Gong J, Chehrazi-Raffle A, Reddi S, Salgia R. Development of PD-1 and PD-L1 Inhibitors as a Form of Cancer Immunotherapy: A Comprehensive Review of Registration Trials and Future Considerations. J Immunother Cancer (2018) 6:8–8. doi: 10.1186/s40425-018-0316-z
5. Mandal R, Senbabaoglu Y, Desrichard A, Havel JJ, Dalin MG, Riaz N, et al. The Head and Neck Cancer Immune Landscape and its Immunotherapeutic Implications. JCI Insight (2016) 1:e89829. doi: 10.1172/jci.insight.89829
6. FDA, U. S. FDA Approves Lenvatinib Plus Pembrolizumab for Advanced Renal Cell Carcinoma (2021). Available at: https://www.fda.gov/drugs/resources-information-approved-drugs/fda-approves-lenvatinib-plus-pembrolizumab-advanced-renal-cell-carcinoma.
7. FDA, U. S. FDA Approves Pembrolizumab Plus Axitinib for Advanced Renal Cell Carcinoma (2019). Available at: https://www.fda.gov/drugs/drug-approvals-and-databases/fda-approves-pembrolizumab-plus-axitinib-advanced-renal-cell-carcinoma.
8. Haratani K, Hayashi H, Tanaka T, Kaneda H, Sakai K, Hayashi K, et al. Tumor Immune Microenvironment and Nivolumab Efficacy in EGFR Mutation-Positive non-Small-Cell Lung Cancer Based on T790M Status After Disease Progression During EGFR-TKI Treatment. Ann Oncol Off J Eur Soc Med Oncol (2017) 28:1532–9. doi: 10.1093/annonc/mdx183
9. Ilieva KM, Correa I, Josephs DH, Karagiannis P, Egbuniwe IU, Cafferkey MJ, et al. Effects of BRAF Mutations and BRAF Inhibition on Immune Responses to Melanoma. Mol Cancer Ther (2014) 13:2769–83. doi: 10.1158/1535-7163.MCT-14-0290
10. Hamarsheh S, Groß O, Brummer T, Zeiser R. Immune Modulatory Effects of Oncogenic KRAS in Cancer. Nat Commun (2020) 11:5439. doi: 10.1038/s41467-020-19288-6
11. Lyu H, Li M, Jiang Z, Liu Z, Wang X. Correlate the TP53 Mutation and the HRAS Mutation With Immune Signatures in Head and Neck Squamous Cell Cancer. Comput Struct Biotechnol J (2019) 17:1020–30. doi: 10.1016/j.csbj.2019.07.009
12. Klinakis A, Rampias T. TP53 Mutational Landscape of Metastatic Head and Neck Cancer Reveals Patterns of Mutation Selection. EBioMedicine (2020) 58:102905. doi: 10.1016/j.ebiom.2020.102905
13. Xie J, Huang L, Lu YG, Zheng DL. Roles of the Wnt Signaling Pathway in Head and Neck Squamous Cell Carcinoma. Front Mol Biosci (2020) 7:590912. doi: 10.3389/fmolb.2020.590912
14. Lee SH, Koo BS, Kim JM, Huang SM, Rho YS, Bae WJ, et al. Wnt/β-Catenin Signalling Maintains Self-Renewal and Tumourigenicity of Head and Neck Squamous Cell Carcinoma Stem-Like Cells by Activating Oct4. J Pathol (2014) 234:99–107. doi: 10.1002/path.4383(2014
15. Wang B, Tian T, Kalland KH, Ke X, Qu Y. Targeting Wnt/beta-Catenin Signaling for Cancer Immunotherapy. Trends Pharmacol Sci (2018) 39:648–58. doi: 10.1016/j.tips.2018.03.008
16. Kreimer AR, Clifford GM, Boyle P, Franceschi S. Human Papillomavirus Types in Head and Neck Squamous Cell Carcinomas Worldwide: A Systematic Review. Cancer epidemiol Biomarkers Prev Publ Am Assoc Cancer Research cosponsored by Am Soc Prev Oncol (2005) 14:467–75. doi: 10.1158/1055-9965.EPI-04-0551
17. Canning M, Guo G, Yu M, Myint C, Groves MW, Byrd JK, et al. Heterogeneity of the Head and Neck Squamous Cell Carcinoma Immune Landscape and Its Impact on Immunotherapy. Front Cell Dev Biol (2019) 7:52. doi: 10.3389/fcell.2019.00052
18. Seiwert TY, Burtness B, Mehra R, Weiss J, Berger R, Eder JP, et al. Safety and Clinical Activity of Pembrolizumab for Treatment of Recurrent or Metastatic Squamous Cell Carcinoma of the Head and Neck (KEYNOTE-012): An Open-Label, Multicentre, Phase 1b Trial. Lancet Oncol (2016) 17:956–65. doi: 10.1016/S1470-2045(16)30066-3
19. Mehra R, Seiwert TY, Gupta S, Weiss J, Gluck I, Eder JP, et al. Efficacy and Safety of Pembrolizumab in Recurrent/Metastatic Head and Neck Squamous Cell Carcinoma: Pooled Analyses After Long-Term Follow-Up in KEYNOTE-012. Br J Cancer (2018) 119:153–9. doi: 10.1038/s41416-018-0131-9
20. Rischin D, Harrington KJ, Greil R, Soulieres D, Tahara M, de Castro G, et al. Protocol-Specified Final Analysis of the Phase 3 KEYNOTE-048 Trial of Pembrolizumab (Pembro) as First-Line Therapy for Recurrent/Metastatic Head and Neck Squamous Cell Carcinoma (R/M HNSCC). J Clin Oncol (2019) 37:6000–0. doi: 10.1200/JCO.2019.37.15_suppl.6000
21. Wang H, Zhao Q, Zhang Y, Zhang Q, Zheng Z, Liu S, et al. Immunotherapy Advances in Locally Advanced and Recurrent/Metastatic Head and Neck Squamous Cell Carcinoma and Its Relationship With Human Papillomavirus. Front Immunol (2021) 12:652054. doi: 10.3389/fimmu.2021.652054
22. Leemans CR, Braakhuis BJ, Brakenhoff RH. The Molecular Biology of Head and Neck Cancer. Nat Rev Cancer (2011) 11:9–22. doi: 10.1038/nrc2982
23. Lopez-Albaitero A, Lee SC, Morgan S, Grandis JR, Gooding WE, Ferrone S, et al. Role of Polymorphic Fc Gamma Receptor IIIa and EGFR Expression Level in Cetuximab Mediated, NK Cell Dependent In Vitro Cytotoxicity of Head and Neck Squamous Cell Carcinoma Cells. Cancer Immunol Immunother (2009) 58:1853–64. doi: 10.1007/s00262-009-0697-4
24. Srivastava RM, Lee SC, Andrade Filho PA, Lord CA, Jie HB, Davidson HC, et al. Cetuximab-Activated Natural Killer and Dendritic Cells Collaborate to Trigger Tumor Antigen-Specific T-Cell Immunity in Head and Neck Cancer Patients. Clin Cancer Res an Off J Am Assoc Cancer Res (2013) 19:1858–72. doi: 10.1158/1078-0432.CCR-12-2426
25. Concha-Benavente F, Kansy B, Moskovitz J, Moy J, Chandran U, Ferris RL. PD-L1 Mediates Dysfunction in Activated PD-1(+) NK Cells in Head and Neck Cancer Patients. Cancer Immunol Res (2018) 6:1548–60. doi: 10.1158/2326-6066.CIR-18-0062
26. Sacco AG, Chen R, Worden FP, Wong DJL, Adkins D, Swiecicki P, et al. Pembrolizumab Plus Cetuximab in Patients With Recurrent or Metastatic Head and Neck Squamous Cell Carcinoma: An Open-Label, Multi-Arm, non-Randomised, Multicentre, Phase 2 Trial. Lancet Oncol (2021) 22:883–92. doi: 10.1016/S1470-2045(21)00136-4
27. Chung CH, Bonomi M, Steuer CE, Li J, Bhateja P, Johnson M, et al. Concurrent Cetuximab and Nivolumab as a Second-Line or Beyond Treatment of Patients With Recurrent and/or Metastatic Head and Neck Squamous Cell Carcinoma: Results of Phase I/II Study. Cancers (2021) 13:1180. doi: 10.3390/cancers13051180
28. Lizotte PH, Hong RL, Luster TA, Cavanaugh ME, Taus LJ, Wang S, et al. A High-Throughput Immune-Oncology Screen Identifies EGFR Inhibitors as Potent Enhancers of Antigen-Specific Cytotoxic T-Lymphocyte Tumor Cell Killing. Cancer Immunol Res (2018) 6:1511–23. doi: 10.1158/2326-6066.CIR-18-0193
29. Kao HF, Liao BC, Huang YL, Huang HC, Chen CN, Chen TC, et al. Afatinib and Pembrolizumab for Recurrent or Metastatic Head and Neck Squamous Cell Carcinoma (ALPHA Study): A Phase II Study With Biomarker Analysis. Clin Cancer Res an Off J Am Assoc Cancer Res (2022). doi: 10.1158/1078-0432.CCR-21-3025
30. Bauml J, Seiwert TY, Pfister DG, Worden F, Liu SV, Gilbert J, et al. Pembrolizumab for Platinum- and Cetuximab-Refractory Head and Neck Cancer: Results From a Single-Arm, Phase II Study. J Clin Oncol Off J Am Soc Clin Oncol (2017) 35:1542–9. doi: 10.1200/JCO.2016.70.1524
31. Clement PM, Gauler T, Machiels JP, Haddad RI, Fayette J, Licitra LF, et al. Afatinib Versus Methotrexate in Older Patients With Second-Line Recurrent and/or Metastatic Head and Neck Squamous Cell Carcinoma: Subgroup Analysis of the LUX-Head & Neck 1 Trial. Ann Oncol Off J Eur Soc Med Oncol (2016) 27:1585–93. doi: 10.1093/annonc/mdw151
32. Panchal A, Seto P, Wall R, Hillier BJ, Zhu Y, Krakow J, et al. COBRA: A Highly Potent Conditionally Active T Cell Engager Engineered for the Treatment of Solid Tumors. MAbs (2020) 12:1792130. doi: 10.1080/19420862.2020.1792130
33. Thorsson V, Gibbs DL, Brown SD, Wolf D, Bortone DS, Ou Yang TH, et al. The Immune Landscape of Cancer. Immunity (2018) 48:812–830 e814. doi: 10.1016/j.immuni.2018.03.023
34. Janku F, Hanna GJ, Carvajal RD, Paik PK, Hernando-Calvo A, Gillison ML, et al. First-In-Human Phase I Study of the Bifunctional EGFR/Tgfβ Fusion Protein BCA101 in Patients With EGFR-Driven Advanced Solid Cancers. J Clin Oncol (2021) 39:3074–4. doi: 10.1200/JCO.2021.39.15_suppl.3074
35. Chai AWY, Tan AC, Cheong SC. Uncovering Drug Repurposing Candidates for Head and Neck Cancers: Insights From Systematic Pharmacogenomics Data Analysis. Sci Rep (2021) 11:23922. doi: 10.1038/s41598-021-03418-1
36. Motzer RJ, Robbins PB, Powles T, Albiges L, Haanen JB, Larkin J, et al. Avelumab Plus Axitinib Versus Sunitinib in Advanced Renal Cell Carcinoma: Biomarker Analysis of the Phase 3 JAVELIN Renal 101 Trial. Nat Med (2020) 26:1733–41. doi: 10.1038/s41591-020-1044-8
37. Reck M, Mok TSK, Nishio M, Jotte RM, Cappuzzo F, Orlandi F, et al. Atezolizumab Plus Bevacizumab and Chemotherapy in non-Small-Cell Lung Cancer (IMpower150): Key Subgroup Analyses of Patients With EGFR Mutations or Baseline Liver Metastases in a Randomised, Open-Label Phase 3 Trial. Lancet Respir Med (2019) 7:387–401. doi: 10.1016/S2213-2600(19)30084-0
38. Kwilas AR, Donahue RN, Tsang KY, Hodge JW. Immune Consequences of Tyrosine Kinase Inhibitors That Synergize With Cancer Immunotherapy. Cancer Cell Microenviron (2015) 2:e677. doi: 10.14800/ccm.677
39. Furukawa K, Nagano T, Tachihara M, Yamamoto M, Nishimura Y. Interaction Between Immunotherapy and Antiangiogenic Therapy for Cancer. Molecules (2020) 25:3900. doi: 10.3390/molecules25173900
40. Roland CL, Lynn KD, Toombs JE, Dineen SP, Udugamasooriya DG, Brekken RA. Cytokine Levels Correlate With Immune Cell Infiltration After Anti-VEGF Therapy in Preclinical Mouse Models of Breast Cancer. PloS One (2009) 4:e7669. doi: 10.1371/journal.pone.0007669
41. Kato Y, Tabata K, Kimura T, Yachie-Kinoshita A, Ozawa Y, Yamada K, et al. Lenvatinib Plus Anti-PD-1 Antibody Combination Treatment Activates CD8+ T Cells Through Reduction of Tumor-Associated Macrophage and Activation of the Interferon Pathway. PloS One (2019) 14:e0212513. doi: 10.1371/journal.pone.0212513
42. Oliva M, Chepeha D, Araujo DV, Diaz-Mejia JJ, Olson P, Prawira A, et al. Antitumor Immune Effects of Preoperative Sitravatinib and Nivolumab in Oral Cavity Cancer: SNOW Window-of-Opportunity Study. J Immunother Cancer (2021) 9. doi: 10.1136/jitc-2021-003476
43. Zhou L, Matsushima GK. Tyro3, Axl, Mertk Receptor-Mediated Efferocytosis and Immune Regulation in the Tumor Environment. Int Rev Cell Mol Biol (2021) 361:165–210. doi: 10.1016/bs.ircmb.2021.02.002
44. Paolino M, Penninger JM. The Role of TAM Family Receptors in Immune Cell Function: Implications for Cancer Therapy. Cancers (2016) 8:97. doi: 10.3390/cancers8100097
45. Cai Y, Dodhia S, Su GH. Dysregulations in the PI3K Pathway and Targeted Therapies for Head and Neck Squamous Cell Carcinoma. Oncotarget (2017) 8:22203–17. doi: 10.18632/oncotarget.14729
46. Marquard FE, Jucker M. PI3K/AKT/mTOR Signaling as a Molecular Target in Head and Neck Cancer. Biochem Pharmacol (2020) 172:113729. doi: 10.1016/j.bcp.2019.113729
47. Chandrasekaran S, Sasaki M, Scharer CD, Kissick HT, Patterson DG, Magliocca KR, et al. Phosphoinositide 3-Kinase Signaling Can Modulate MHC Class I and II Expression. Mol Cancer Res MCR (2019) 17:2395–409. doi: 10.1158/1541-7786.MCR-19-0545
48. Shayan G, Srivastava R, Li J, Schmitt N, Kane LP, Ferris RL. Adaptive Resistance to Anti-PD1 Therapy by Tim-3 Upregulation is Mediated by the PI3K-Akt Pathway in Head and Neck Cancer. Oncoimmunology (2017) 6:e1261779. doi: 10.1080/2162402X.2016.1261779
49. Davis RJ, Moore EC, Clavijo PE, Friedman J, Cash H, Chen Z, et al. Anti-PD-L1 Efficacy Can Be Enhanced by Inhibition of Myeloid-Derived Suppressor Cells With a Selective Inhibitor of PI3Kdelta/Gamma. Cancer Res (2017) 77:2607–19. doi: 10.1158/0008-5472.CAN-16-2534
50. Cohen E, Postow M, Sullivan R, Hong D, Yeckes-Rodin H, McCarter J, et al. 352 Updated Clinical Data From The Squamous Cell Carcinoma Of The Head And Neck (SCCHN) Expansion Cohort Of An Ongoing Ph1/1b Study Of Eganelisib (Formerly IPI-549) in Combination With NIVOLUMAB. J ImmunoTher Cancer (2020) 8:A214–5. doi: 10.1136/jitc-2020-SITC2020.0352
51. Kirkwood JM, Iannotti N, Cho D, O'Day S, Gibney G, Hodi FS, et al. Abstract CT176: Effect of JAK/STAT or PI3Kδ Plus PD-1 Inhibition on the Tumor Microenvironment: Biomarker Results From a Phase Ib Study in Patients With Advanced Solid Tumors. Cancer Res (2018) 78:CT176–6. doi: 10.1158/1538-7445.Am2018-ct176
52. Gillison ML, Akagi K, Xiao W, Jiang B, Pickard RKL, Li J, et al. Human Papillomavirus and the Landscape of Secondary Genetic Alterations in Oral Cancers. Genome Res (2019) 29:1–17. doi: 10.1101/gr.241141.118
53. Qureshy Z, Johnson DE, Grandis JR. Targeting the JAK/STAT Pathway in Solid Tumors. J Cancer Metastasis Treat (2020) 6:27. doi: 10.20517/2394-4722.2020.58
54. Proia TA, Singh M, Woessner R, Carnevalli L, Bommakanti G, Magiera L, et al. STAT3 Antisense Oligonucleotide Remodels the Suppressive Tumor Microenvironment to Enhance Immune Activation in Combination With Anti-PD-L1. Clin Cancer Res an Off J Am Assoc Cancer Res (2020) 26:6335–49. doi: 10.1158/1078-0432.CCR-20-1066
55. Verhoeven Y, Tilborghs S, Jacobs J, De Waele J, Quatannens D, Deben C, et al. The Potential and Controversy of Targeting STAT Family Members in Cancer. Semin Cancer Biol (2020) 60:41–56. doi: 10.1016/j.semcancer.2019.10.002 (2020
56. Heine A, Held SA, Daecke SN, Wallner S, Yajnanarayana SP, Kurts C, et al. The JAK-Inhibitor Ruxolitinib Impairs Dendritic Cell Function In Vitro and In Vivo. Blood (2013) 122:1192–202. doi: 10.1182/blood-2013-03-484642
57. Cohen EEW, Harrington KJ, Hong DS, Mesia R, Brana I, Perez Segura P, et al. A Phase Ib/II Study (SCORES) of Durvalumab (D) Plus Danvatirsen (DAN; AZD9150) or AZD5069 (CX2i) in Advanced Solid Malignancies and Recurrent/Metastatic Head and Neck Squamous Cell Carcinoma (RM-HNSCC): Updated Results. Ann Oncol (2018) 29:viii372. doi: 10.1093/annonc/mdy287
58. Cancer Genome Atlas, N. Comprehensive Genomic Characterization of Head and Neck Squamous Cell Carcinomas. Nature (2015) 517:576–82. doi: 10.1038/nature14129
59. van Caloen G, Machiels JP. Potential Role of Cyclin-Dependent Kinase 4/6 Inhibitors in the Treatment of Squamous Cell Carcinoma of the Head and Neck. Curr Opin Oncol (2019) 31:122–30. doi: 10.1097/CCO.0000000000000513
60. Petroni G, Formenti SC, Chen-Kiang S, Galluzzi L. Immunomodulation by Anticancer Cell Cycle Inhibitors. Nat Rev Immunol (2020) 20:669–79. doi: 10.1038/s41577-020-0300-y
61. Goel S, DeCristo MJ, Watt AC, BrinJones H, Sceneay J, Li BB, et al. CDK4/6 Inhibition Triggers Anti-Tumour Immunity. Nature (2017) 548:471–5. doi: 10.1038/nature23465
62. Pujol J-L, Vansteenkiste J, Paz-Ares Rodriguez L, Gregorc V, Mazieres J. Abemaciclib in Combination With Pembrolizumab for Stage IV KRAS-Mutant or Squamous NSCLC: A Phase 1b Study. JTO Clin Res Rep (2021) 2:100234. doi: 10.1016/j.jtocrr.2021.100234
63. White RA, Malkoski SP, Wang XJ. TGFbeta Signaling in Head and Neck Squamous Cell Carcinoma. Oncogene (2010) 29:5437–46. doi: 10.1038/onc.2010.306
64. Batlle E, Massague J. Transforming Growth Factor-Beta Signaling in Immunity and Cancer. Immunity (2019) 50:924–40. doi: 10.1016/j.immuni.2019.03.024
65. Cho BC, Daste A, Ravaud A, Salas S, Isambert N, McClay E, et al. Bintrafusp Alfa, a Bifunctional Fusion Protein Targeting TGF-Beta and PD-L1, in Advanced Squamous Cell Carcinoma of the Head and Neck: Results From a Phase I Cohort. J Immunother Cancer (2020) 8:e000664. doi: 10.1136/jitc-2020-000664
66. Strauss J, Heery CR, Schlom J, Madan RA, Cao L, Kang Z, et al. Phase I Trial of M7824 (MSB0011359C), a Bifunctional Fusion Protein Targeting PD-L1 and TGFbeta, in Advanced Solid Tumors. Clin Cancer Res an Off J Am Assoc Cancer Res (2018) 24:1287–95. doi: 10.1158/1078-0432.CCR-17-2653
67. Pal Singh S, Dammeijer F, Hendriks RW. Role of Bruton’s Tyrosine Kinase in B Cells and Malignancies. Mol Cancer (2018) 17:57. doi: 10.1186/s12943-018-0779-z
68. Sagiv-Barfi I, Kohrt HE, Czerwinski DK, Ng PP, Chang BY, Levy R. Therapeutic Antitumor Immunity by Checkpoint Blockade is Enhanced by Ibrutinib, an Inhibitor of Both BTK and ITK. Proc Natl Acad Sci United States America (2015) 112:E966–972. doi: 10.1073/pnas.1500712112
69. Giudice FS, Pinto DS Jr., Nor JE, Squarize CH, Castilho RM. Inhibition of Histone Deacetylase Impacts Cancer Stem Cells and Induces Epithelial-Mesenchyme Transition of Head and Neck Cancer. PloS One (2013) 8:e58672. doi: 10.1371/journal.pone.0058672
70. Woods DM, Sodre AL, Villagra A, Sarnaik A, Sotomayor EM, Weber J, et al. HDAC Inhibition Upregulates PD-1 Ligands in Melanoma and Augments Immunotherapy With PD-1 Blockade. Cancer Immunol Res (2015) 3:1375–85. doi: 10.1158/2326-6066.CIR-15-0077-T
71. Wang X, Waschke BC, Woolaver RA, Chen SMY, Chen Z, Wang JH. HDAC Inhibitors Overcome Immunotherapy Resistance in B-Cell Lymphoma. Protein Cell (2020) 11:472–82. doi: 10.1007/s13238-020-00694-x
72. Rodriguez CP, Wu QV, Voutsinas J, Fromm JR, Jiang X, Pillarisetty VG, et al. A Phase II Trial of Pembrolizumab and Vorinostat in Recurrent Metastatic Head and Neck Squamous Cell Carcinomas and Salivary Gland Cancer. Clin Cancer Res an Off J Am Assoc Cancer Res (2020) 26:837–45. doi: 10.1158/1078-0432.CCR-19-2214
73. Zhang WW, Li L, Li D, Liu J, Li X, Li W, et al. The First Approved Gene Therapy Product for Cancer Ad-P53 (Gendicine): 12 Years in the Clinic. Hum Gene Ther (2018) 29:160–79. doi: 10.1089/hum.2017.218
74. Sobol RE, Menander KB, Chada S, Wiederhold D, Sellman B, Talbott M, et al. Analysis of Adenoviral P53 Gene Therapy Clinical Trials in Recurrent Head and Neck Squamous Cell Carcinoma. Front Oncol (2021) 11:645745. doi: 10.3389/fonc.2021.645745
75. Ou X, Ma Q, Yin W, Ma X, He Z. CRISPR/Cas9 Gene-Editing in Cancer Immunotherapy: Promoting the Present Revolution in Cancer Therapy and Exploring More. Front Cell Dev Biol (2021) 9:674467. doi: 10.3389/fcell.2021.674467
76. Manguso RT, Pope HW, Zimmer MD, Brown FD, Yates KB, Miller BC, et al. In Vivo CRISPR Screening Identifies Ptpn2 as a Cancer Immunotherapy Target. Nature (2017) 547:413–8. doi: 10.1038/nature23270
77. LaFleur MW, Nguyen TH, Coxe MA, Miller BC, Yates KB, Gillis JE, et al. PTPN2 Regulates the Generation of Exhausted CD8(+) T Cell Subpopulations and Restrains Tumor Immunity. Nat Immunol (2019) 20:1335–47. doi: 10.1038/s41590-019-0480-4
78. Li F, Huang Q, Luster TA, Hu H, Zhang H, Ng W, et al. Epigenetic CRISPR Screen Identifies Asf1a as an Immunotherapeutic Target in Kras-Mutant Lung Adenocarcinoma. Cancer Discov (2020) 10:270–87. doi: 10.1158/2159-8290.Cd-19-0780
79. Suresh S, Chen B, Zhu J, Golden RJ, Lu C, Evers BM, et al. Eif5b Drives Integrated Stress Response-Dependent Translation of PD-L1 in Lung Cancer. Nat Cancer (2020) 1:533–45. doi: 10.1038/s43018-020-0056-0
80. Peng Q, Deng Z, Pan H, Gu L, Liu O, Tang Z. Mitogen-Activated Protein Kinase Signaling Pathway in Oral Cancer. Oncol Lett (2018) 15:1379–88. doi: 10.3892/ol.2017.7491
81. Kang SH, Keam B, Ahn YO, Park HR, Kim M, Kim TM, et al. Inhibition of MEK With Trametinib Enhances the Efficacy of Anti-PD-L1 Inhibitor by Regulating Anti-Tumor Immunity in Head and Neck Squamous Cell Carcinoma. Oncoimmunology (2019) 8:e1515057. doi: 10.1080/2162402X.2018.1515057
82. Ngan HL, Liu Y, Fong AY, Poon PHY, Yeung CK, Chan SSM, et al. MAPK Pathway Mutations in Head and Neck Cancer Affect Immune Microenvironments and ErbB3 Signaling. Life Sci Alliance (2020) 3. doi: 10.26508/lsa.201900545
83. Wang Y, Liu S, Yang Z, Algazi AP, Lomeli SH, Wang Y, et al. Anti-PD-1/L1 Lead-in Before MAPK Inhibitor Combination Maximizes Antitumor Immunity and Efficacy. Cancer Cell (2021) 39:1375–1387 e1376. doi: 10.1016/j.ccell.2021.07.023
84. Moore EC, Cash HA, Caruso AM, Uppaluri R, Hodge JW, Van Waes C, et al. Enhanced Tumor Control With Combination mTOR and PD-L1 Inhibition in Syngeneic Oral Cavity Cancers. Cancer Immunol Res (2016) 4:611–20. doi: 10.1158/2326-6066.CIR-15-0252
85. Pollizzi KN, Patel CH, Sun IH, Oh MH, Waickman AT, Wen J, et al. Mtorc1 and Mtorc2 Selectively Regulate CD8(+) T Cell Differentiation. J Clin Invest (2015) 125:2090–108. doi: 10.1172/JCI77746
86. Fu Y, Tian G, Li J, Zhang Z, Xu K. An HNSCC Syngeneic Mouse Model for Tumor Immunology Research and Preclinical Evaluation. Int J Mol Med (2020) 46:1501–13. doi: 10.3892/ijmm.2020.4680
Keywords: head and neck cancer, targeted therapy, immunotherapy, drug combinations, cancer genetics, immune checkpoint inhibitor (ICI)
Citation: Chai AWY, Yee PS and Cheong SC (2022) Rational Combinations of Targeted Therapy and Immune Checkpoint Inhibitors in Head and Neck Cancers. Front. Oncol. 12:837835. doi: 10.3389/fonc.2022.837835
Received: 17 December 2021; Accepted: 17 February 2022;
Published: 17 March 2022.
Edited by:
Jing Hong Wang, University of Pittsburgh Medical Center, United StatesReviewed by:
Yan Cui, Augusta University, United StatesDan Zandberg, University of Pittsburgh Medical Center, United States
Copyright © 2022 Chai, Yee and Cheong. This is an open-access article distributed under the terms of the Creative Commons Attribution License (CC BY). The use, distribution or reproduction in other forums is permitted, provided the original author(s) and the copyright owner(s) are credited and that the original publication in this journal is cited, in accordance with accepted academic practice. No use, distribution or reproduction is permitted which does not comply with these terms.
*Correspondence: Annie Wai Yeeng Chai, YW5uaWUuY2hhaUBjYW5jZXJyZXNlYXJjaC5teQ==