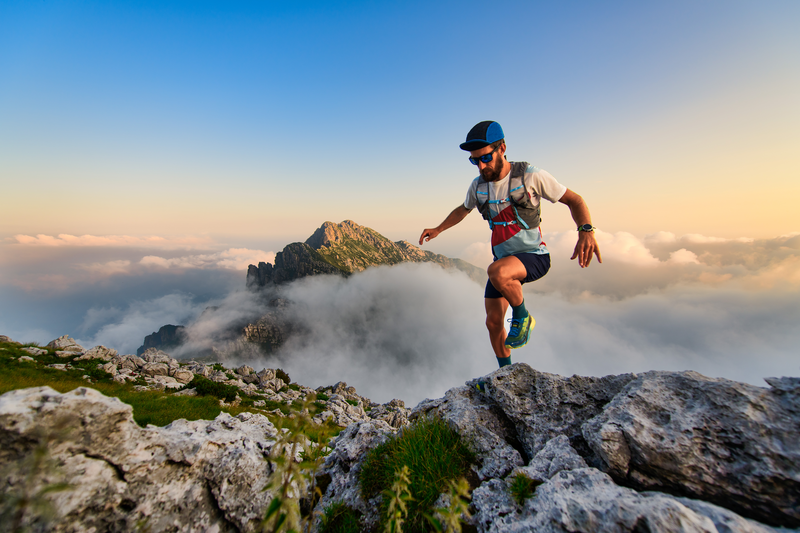
94% of researchers rate our articles as excellent or good
Learn more about the work of our research integrity team to safeguard the quality of each article we publish.
Find out more
REVIEW article
Front. Oncol. , 09 March 2022
Sec. Molecular and Cellular Oncology
Volume 12 - 2022 | https://doi.org/10.3389/fonc.2022.831366
This article is part of the Research Topic Role of Non-coding RNAs Mediating Wnt/β-catenin Signaling in Cancer View all 4 articles
Hepatocellular carcinoma (HCC) is considered the second most deadly cancer worldwide. Due to the absence of early diagnostic markers and effective therapeutic approaches, distant metastasis and increasing recurrence rates are major difficulties in the clinical treatment of HCC. Further understanding of its pathogenesis has become an urgent goal in HCC research. Recently, abnormal expression of long noncoding RNAs (lncRNAs) was identified as a vital regulator involved in the initiation and development of HCC. Activation of the Wnt/β-catenin pathway has been reported to obviously impact cell proliferation, invasion, and migration of HCC. This article reviews specific interactions, significant mechanisms and molecules related to HCC initiation and progression to provide promising strategies for treatment.
Liver cancer has become one of most prevalent malignant diseases worldwide, and hepatocellular carcinoma (HCC) accounts for most liver cancer cases (1–3). Owing to the increasing incidence and mortality, HCC is considered the second most deadly cancer worldwide (4). As clinical research is rapidly advancing, the short survival time and rising recurrence rate of HCC present difficulties in patient treatment (5–7). Thus, deeply exploring the pathogenesis mechanism could remarkably improve prognosis (8–10). HCC generally develops from chronic liver diseases commonly caused by infection with hepatitis B virus (HBV) and hepatitis C virus (HCV) (11–13), and other pathogenic factors also play important roles in the initiation of malignancy. Recently, emerging investigations have concentrated on the molecular mechanisms by which long noncoding RNAs (lncRNAs) regulate HCC tumorigenesis and progression by affecting Wnt/β-catenin signaling (14–16).
As diverse RNA detection methods are rapidly improved and widely applied, a large number of noncoding RNAs have been discovered and initially referred to in broad studies (17–19). LncRNAs (Long non-coding RNAs) are a special category of noncoding RNAs with no less than 200 nucleotides. Accumulating evidence has proven and emphasized the indispensable role of lncRNAs that participate in the pathogenesis of multiple cancers (20–22). For example, it was found that lncRNA HCG11 (human leukocyte antigen complex group 11) could enhance the expression of GFI1 (growth factor independence-1) to suppress the proliferation and invasion of cancer cells, functioning as a regulator of miR-942-5p via sponging in cervical cancer (23). Thus, lncRNA HCG11 is considered a vital suppressor of HCC. In addition, lncRNA DDX1-AS1 was confirmed to regulate HCC cell proliferation and has a strong correlation with a poor prognosis. It was reported that demethylation in HCC could increase the expression of DDX1-AS1 through the inhibition of P53 in the poly(ADP-ribose) polymerase 1 (PARP1)–p53 axis (24).
The Wnt/β-catenin pathway, an evolutionarily conserved signaling axis and a complicated protein network, plays a pivotal role in governing numerous physiological processes, including proliferation, differentiation, and tissue homeostasis (25–27). A previous study uncovered that dysregulation of the Wnt/β-catenin cascade has a major impact on the complicated developmental process of multiple cancers (28–30). For instance, overexpression of lncRNAs positively related to the Wnt/β-catenin signaling pathway plays an indispensable role in the progression of bladder cancer (31–33). Analogously, lncRNA AB073614 is highly expressed in glioma and indicates a shorter survival time; this lncRNA targets sex-determining region Y-box 7 (SOX7) to enhance the expression of Wnt/β-catenin and promote the proliferation and metastasis of glioma cells (34). In addition, the upregulation of lncRNA SNHG1 could promote the proliferation of Non-Small-Cell Lung Carcinoma (NSCLC) cells (35). SNHG1 overexpression is negatively correlated with miR-101-3p expression, and sex-determining region Y-box 9 (SOX9) serves as the downstream effector of miR-101-3p. SOX9 is considered a vital regulator of the activation of Wnt/β-catenin signaling (36–38).
Despite increased knowledge regarding associations between lncRNAs and the Wnt/β-catenin pathway in multiple cancers, the underlying mechanisms in the occurrence and development of HCC remain unclear. Therefore, exploration of the effect of interactions with lncRNAs and the Wnt/β-catenin pathway is still a rewarding direction. In this review, we summarized and highlighted the mechanisms of lncRNAs involved in the Wnt/β-catenin pathway in HCC. We expect that these discoveries could provide prospective and creative ideas for targeted treatment for HCC patients.
A previous report confirmed that the Wnt cascade is commonly divided into two pathways—the canonical and noncanonical signal pathways (39–41)—that mediate the biological process of cell proliferation and differentiation by affecting the transcriptional framework (42). Canonical signaling is known as the Wnt/β-catenin pathway, and the abnormal expression of β-catenin directly contributes to tumorigenesis (43–45). It was reported that destruction of β-catenin is mainly determined by the key degradation complex, which contains scaffold proteins (AXIN), the human tumor suppressor adenomatous polyposis coli (APC), glycogen synthase kinase 3β (GSK3β), and casein kinase 1 alpha 1 (CSNK1A1) (46–48). The degradation complex could lead to the phosphorylation of β-catenin at serine/threonine residues of the N-terminus; subsequently, beta-transducin repeat containing E3 ubiquitin protein (β-TRCP) can recognize and ubiquitinate phosphorylated β-catenin to facilitate its degradation in proteasome (46). Mutations of members from degradation complex presented remarkable correlation with hepatocarcinogenesis. Unphosphorylated CTNNB1 (β-catenin gene) accumulates in the cytoplasm and accesses the nucleus, interacting with T cell-specific factor (TCF)/lymphoid enhancer-binding factor (LEF) and activating a wide range of signaling cascades (30). When CTNNB1 dramatically accumulates in the nucleus and cytoplasm, the probability of HCC cell invasion, proliferation, and deterioration is significantly increased (49). Therefore, CTNNB1 knockdown could block the process of migration and invasion in HCC and improve patient prognosis (50).
Although mutation of CTNNB1 was shown to be associated with HBV-related cancer in previous research (51), other functional mechanisms of CTNNB1 in HBV-related HCC have also gradually been revealed (52). For instance, the HBV gene positively mediates the expression of von Willebrand factor C and EGF domains (VWCE/URG11) at high levels and binds with APC to boost the activation of CTNNB1. In humans, nineteen Wnt ligands and ten receptors from the frizzled class receptor (FZD) family have been verified (53). Several experiments confirmed that elevated expression of Wnt1 and Wnt3a is positively associated with the HCV core protein in HCC (54, 55). The mutual effects of Wnt3a and FZD7 (Frizzled class receptor 7) can trigger the Wnt signaling pathway. FZD7 upregulation might give rise to migration in the early stage of HCC (56, 57). The mechanisms of CTNNB1 involvement in HCV-related HCC have been explored in more detail than those of HBV-related tumors. HCV can increase miRNA-155 expression, facilitating the nuclear accumulation of CTNNB1 and triggering the expression of downstream targets (58). Additionally, NS5A (nonstructural protein 5A), the core protein of HCV, was found to accelerate GSK3β phosphorylation and actively regulate PI3K, contributing to increased CTNNB1 expression (54, 59, 60).
LncRNAs are no less than 200 nucleotides in length, and they are unable to encode proteins. According to the principle of correlative location for the closest coding genes, lncRNAs fall into the five following varieties: sense lncRNA, antisense lncRNA, intergenic lncRNA, intronic lncRNA, and bidirectional lncRNA (61–63). The distribution of lncRNAs in the nucleus or cytoplasm and the dysregulation of lncRNAs may bring about many diseases (64–66). Increasing experiments about lncRNA can offer a bright orientation of earlier diagnosis and effective treatment of HCC (67, 68).
Emerging investigations have revealed that lncRNAs mainly exert epigenetic, transcriptional or posttranscriptional regulation effects on downstream factors (Figure 1), leading to alterations in expression and stability (69). For example, lncRNA HOTAIR (HOX transcript antisense intergenic RNA) can increase the expression of DNMTs to catalyze epigenetic methylation of the promoter region of miR-122, filled with GC islands (70, 71), which leads to the inhibition of miR-122 expression. MiR-122 downregulation might induce the abnormal expression of cyclin G1 and promote cell proliferation in HCC (72). At the transcriptional level, lncRNAs have been reported to directly mediate and alter the expression of noncoding genes, such as miRNAs. In HCC, downregulated H19 was shown to be positively correlated with migration (73). H19 directly interacts with the hnRNP U/PCAF/RNA pol II complex, promoting the transcriptional expression of the miR-200 family through the acetylation of histone H3 in the promoter region. Moreover, the posttranscriptional regulation of lncRNAs is mainly regarded as the effect of alterations in alternative splicing and mRNA stability. The lncRNA termed metastasis-associated lung adenocarcinoma transcript 1 (MALAT1) is highly expressed in HCC and triggers the Wnt pathway, and cells with MALAT1 upregulation tend to exhibit increased splicing factor serine and arginine rich splicing factor 1 (SRSF1) expression. The mentioned effect can also induce apoptosis by alternatively splicing of S6 kinase 1 (S6 K1), further increasing the expression of the mTOR pathway to affect HCC progression (74). A number of lncRNAs also regulate downstream factors and affect important pathways involved in vital biological processes of HCC.
Figure 1 The regulation of lncRNAs in HCC at the epigenetic, transcriptional or posttranscriptional level. (A) LncRNA HOTAIR upregulates DNMTs to increase the methylation of the promoter of miR-122, decreasing the expression of miR-122 to stimulate HCC cell proliferation. (B) The interaction of lncRNA H19 and the hnRNP U/PCAF/RNA pol II complex upregulates the transcriptional expression of the miR-200 family. (C) LncRNA MALAT1 is highly expressed and induces the progression of HCC by triggering the Wnt pathway.
In emerging studies, a number of molecules were considered to serve as transcriptional regulators or directly mediate gene expression, participating in the regulation of the Wnt/β-catenin pathway by affecting the expression of several lncRNAs (75). It was confirmed that β-catenin-specific proteins were key regulators that triggered the Wnt/β-catenin cascade (76). Recently, the study of the involvement of the lncRNA/Wnt/β-catenin axis in the pathogenesis of malignant tumors has been successful (77, 78). We conclude that the primary lncRNA/Wnt/β-catenin axis plays a role in HCC (Table 1).
LncRNA colorectal neoplasia differentially expressed (CRNDE) has a tendency to be abnormally expressed in colorectal neoplasia samples (115, 116). Substantial experiments have gradually recognized the participation of CRNDE in cell proliferation, apoptosis, invasion, and migration in various malignant tumors (117–119). CRNDE expression is higher in HCC tissues than in adjacent tissues. In addition, overexpression of CRNDE could promote HCC cell proliferation, predicting a poorer prognosis in patients. Mechanistically, CRNDE is reported to upregulate the expression of cyclins and promote cell cycle transition from the G0/G1 phase to S phase (79). When CRNDE was silenced, inhibition of HCC cell proliferation, invasion, and metastasis was observed (80). Knockdown of CRNDE also reduced the expression of slug, twist, N‐cadherin, and vimentin, resulting in blockade of the oncogenic effect of epithelial to mesenchymal transition (EMT) induction. Numerous studies have demonstrated that EMT plays a vital role in invading and migrating in diverse cancers (120, 121). Activation of Wnt/β-catenin is considered an essential process in stimulating EMT progression (122). CRNDE downregulation might suppress the expression of Wnt2, Frizzled 4, and β‐catenin to affect the Wnt/β-catenin pathway. Wnt2 belongs to the WNT gene family and promotes the expression of β‐catenin to trigger the canonical Wnt pathway (123, 124). It was reported that the HCV core protein could increase the gene expression of Wnt2 in the SMMC7721 cell line (125). In addition, overexpression of Wnt2 mRNA was detected to exert an indispensable effect on disease processes, such as colorectal polyps, primary colorectal cancer, and the liver metastasis of colorectal cancer (126). In humans, nineteen Wnt ligands and ten receptors from the FZD family have been verified (51). Frizzled 4 downregulation represses the proliferation of cervical cancer cells by increasing the expression of miR-375 (127). These results indicated that high expression of CRNDE promotes the invasion of HCC cells by inducing upregulation of the Wnt/β‐catenin cascades. On the basis of the mentioned study, CRNDE is regarded as a potential therapeutic target for HCC.
In HCC, lncRNA ASB16 antisense RNA1 (ASB16-AS1) was found to aberrantly express in high level and have positively association with unsatisfied outcome (108). On the contrary, knockdown of ASB16-AS1 could exert the anti-tumor effect on biological function of cancer cell, containing proliferation, invasion, and metastasis. Simultaneously expression of miR-1827 presented increasing after knock of ASB16-AS1, which indicated the fact that activity of miR-1827 might be controlled by lncRNA ASB16-AS1. FZD4 (Frizzled class receptor 4) is regard as the significant molecule to active Wnt/β-catenin signaling pathway (128). Upregulated miR-1827 could negatively mediated expression of FZD4 to inhibit progression of HCC. When FZD4 was high-expressed, the effect from knockdown of ASB16-AS1 would be reversed. Briefly, lncRNA ASB16-AS1 might sponge miR-1827 to decrease its expression level, resulting in upregulating FZD and triggering Wnt/β-catenin pathway to involve in tumorigenesis of HCC. However, whether lncRNA ASB16-AS1 has upstream regulators to modulate lncRNA ASB16-AS1/miR-1827/FZD4/Wnt/β-catenin axis is still unclear, and more explorations and researches are required.
LncRNA LINC00346 was is highly expressed and plays tumor-promoting roles in several tumors (129). LINC00346 was commonly verified to be highly expressed in HCC tissues. LINC00346 functions as an oncogenic regulator, boosting the viability, migration, and developmental ability of HCC cells. Mechanistically, LINC00346 attenuates the suppressive effect of miR-542-3p on WDR18 (WD repeat domain 18)) expression by sponging miR-542-3p, contributing to increased Wnt/β-catenin expression in vitro and in vivo (130–132). MiR-542-3p presented diverse effects in several studies via the regulation of various signaling pathways. One study confirmed that miR-542-3p could trigger the TGF-β/Smad pathway to promote the migration of HCC cells. In contrast, miR-542-3p could downregulate FZD7/Wnt to block the proliferation of HCC cells in another study (97). WDR18 was observed to be expressed predominantly in HCC tissues and regarded as an oncogenic regulator of tumorigenesis in HCC. Activation of Wnt/β-catenin signaling cascades might partly depend on WDR18. Enhancing the expression of WDR18 could promote increased β-catenin expression, triggering the Wnt/β-catenin signaling pathway (133–135). Furthermore, accumulating evidence has proven that the final downstream target of the Wnt/β-catenin cascades is MYC in ALK-positive anaplastic large cell lymphoma and basal stem cells (81). However, more molecular mechanisms remain unreported, and further exploration of Wnt/β-catenin signaling in HCC is necessary.
LncRNA DUXAP10 was reported to be expressed at increasing levels in different malignant tumors (136), such as bladder cancer (137), prostate cancer (138), renal cell carcinoma (139), liver cancer (38) and colorectal cancer (140). Overexpression of DUXAP10 was observed in HCC tissues versus normal tissues (141, 142), which indicates that DUXAP10 is a risk predictor in patients with HCC (83). DUXAP10 overexpression is significantly correlated with the malignant behavior of HCC cells and could predict the survival time of patients with advanced-stage disease (143). Inhibition of DUXAP10 dramatically weakened the viability and proliferative ability of SMMC-7721 cells and HepG2 cells. In concordance with the result from ovarian cancer in DUXAP10. It was reported that silencing DUXAP10 caused damage to the development of lung cancer in vivo (144). In HCC, knockdown of DUXAP10 could mediate the promotion of apoptosis and suppression of cell proliferation. Additionally, downregulation of PI3K/Akt was affected by knockdown of DUXAP10 (140, 145). The PI3K/Akt signaling pathway was found to be involved in the process of invasion and migration as well as the Wnt/β-catenin signaling pathway. DUXAP10 is considered to regulate EMT to mediate the progression of HCC via PI3K/Akt and Wnt/β-catenin cascades. Thus, inhibition of DUXAP10 could strongly suppress EMT (82).
SOX9 is a member of the sex-determining region Y (146) box gene (SRY) superfamily and is a transcription factor (147) that plays an essential role in gene expression regulation (148, 149). A previous study confirmed the facilitative effect of SRY members in a variety of tumors. LncRNA SOX9 antisense RNA 1 (SOX9-AS1) is regarded to promote SOX9 expression. SOX9 has a positive association with the development of HCC and commonly contributes to the dismal outcome of patients (150). In a recent study, miRNA-138 is perceived as an inhibitor that affects HCC cell growth via the suppression of SOX9. Additionally, SOX9 might have an impact on mediating β-catenin expression in canonical signaling and may strongly affect the expression of downstream regulators, such as cyclin D1 and c-Myc (38), to promote the development of malignancies. Activation of the Wnt/β-catenin cascades exert an indispensable effect on cell proliferation, invasion, and metastasis in HCC (141, 142). Silencing of SOX9 or SOX9-AS1 reduces Wnt/β-catenin expression, leading to the inhibition of EMT. SOX9-AS1 is highly expressed in HCC tissue, but miRNA-5590-3p is expressed at low levels. SOX9-AS1 might modulate SOX9/Wnt/β-catenin by regulating miR-5590-3p. When mutations occur at the miRNA-5590-3p site on SOX9-AS1, the activating effect of SOX9 upregulation via the SOX9/β-catenin axis is attenuated. These results suggest that miRNAs might regulate SOX9-AS1 via SOX9/miR-5590-3p/Wnt/β-catenin axis (83).
Lnc RNA MiR143HG is downregulated in HCC cells and commonly indicates shorter survival time in patients. A recent study shed light on the function of miR-143HG in HCC and found that miR-143HG inhibited the activation of Wnt/β-catenin cascades to restrict the proliferation of HCC cells (84). In contrast, lncRNA-CR594175 was proven to be expressed at higher levels in primary HCC than in adjacent tumor tissues. LncRNA-CR594175 is considered an effector that reverses the downregulation of CTNNB1 from hsa-miR-142-3p, facilitating HCC progression by triggering the Wnt pathway (85). Similarly, patients with overexpression of miRNA194-2HG in HCC exhibit a poor prognosis. MiRNA194-2HG functions as a competing endogenous RNA (ceRNA) to sponge miR-1207-5p, contributing to enhanced cell invasion and migration in HCC by activating Wnt signaling (86). Another investigation found that lncRNA-TP53TG1 expression was low in HCC tissues. LncRNA-TP53TG1 expression is regarded as an independent prognostic factor in patients with HCC. Additionally, lncRNA-TP53TG1 acts as a suppressor in HCC to negatively influence several processes of cell proliferation, invasion and metastasis. The inhibition of proliferation via lncRNA-TP53TG1 is mediated by the alteration in the ubiquitination levels of PRDX4 (peroxiredoxin 4) protein and the activation of the Wnt pathway (151). Zhu et al. (87) revealed that lncRNA FEZF1-AS1 is upregulated in HCC and facilitates malignant behavior, such as early invasion and metastasis. The molecular mechanism showed that FEZF1-AS1 increases the development and progression of HCC through modulation of the miR-107/Wnt/β-catenin axis. A previous study indicated that miR-107 serves as a suppressor involved in a variety of cancers (152–154). In HCC, miR-107 downregulation was observed. Overexpression of miR-107 has been proven to downregulate β-catenin and wnt3a and increase the expression level of p-GSK-3β, resulting in the blockade of Wnt signaling activation. Thus, we can presume that the inhibitory lncRNA FEZF1-AS1 might interact with miRNA-107 to alter the expression of the Wnt pathway. In brief, these lncRNAs are reported to have a significant association with HCC prognosis in patients with HCC, and the mechanism by which lncRNAs regulate the Wnt/β-catenin pathway needs to be further explored. Fortunately, the mentioned study provides promising ideas for diagnostic or therapeutic approaches in HCC.
LncRNAs impact a variety of functions of HCC through the regulation of Wnt n cascades. Thus, we summarized the mechanisms related to the clinical features of HCC in Table 2, expecting to find effective approaches to prolong the survival time of patients.
CSCs were first verified in the xenotransplant process via the injection of acute myeloid leukemia (AML) cells into SCID mice, and their biological features resemble those of normal stem cells with self-renewal and differentiation abilities (155). The CSCs of HCC are termed liver cancer stem cells (LCSCs) or liver tumor-initiating cells (TICs), which have a crucial impact on tumor initiation, migration, and recurrence (156–158). Elevated expression of Linc00210 has been discovered in liver cancer and TICs. Emerging surface markers have been discovered to further identify TICs; these include CD13, CD133, CD24, and EPCAM (159–161). As a study reported, Wnt/β-catenin signaling is one of the pathways involved in liver cancer and is active in liver TICs (162). Linc00210 alleviates the suppressive role of CTNNBIP1, actively facilitating the binding of β-catenin and the TCF/LEF complex to trigger Wnt cascades. Furthermore, massive amplifications of linc00210 were shown to be vital for activating Wnt/β-catenin and stimulating TIC self-renewal (89). Likewise, LncTCF7 actively regulates TCF7 expression, primarily by recruiting the SWI/SNF complex. TCF7 upregulation remarkably increases the expression of Wnt signaling, inducing the initiation of self-renewal of TICs (89, 90). When TCF7 is expressed at low levels and the levels of Wnt downstream targets are reduced, the self-renewal capacity of TICs and tumorigenesis are rapidly attenuated in HCC. CSCs are similar to tissue-specific stem cells and control cell growth to significantly affect malignancy and tumor stage. TCF7 acts as an upstream activator of Wnt signaling to initiate the pathway (163, 164). Thus, overexpression of TCF7, which triggers the Wnt pathway, is required for the self-renewal of TICs and contributes to the promotion of HCC development. TCF7 was reported to participate in the tumorigenesis and progression of HCC (90) (Figure 2). On the basis of these findings that LncTCF7 impacts the biological processes of HCC via the TCF7/Wnt/β-catenin axis, it could be a promising therapeutic target to effectively improve the prognosis of HCC patients.
Figure 2 The specific mechanism of lncRNAs and the Wnt/β-catenin pathway in the development of HCC CSCs. Overexpression of linc00210 promotes the expression of β-catenin and the TCF/LEF complex to activate the Wnt/β-catenin pathway. lncTCF7 downregulation could impair the self-renewal ability of TICs in HCC.
In HCC, lncRNA PRR34-AS1 was found to present increased expression and is involved in the promotion of tumorigenesis. Inhibition of miR-296-5p could positively mediate cell proliferation in HCC. E2F2 and SOX12 are considered essential downstream targets of miR-296-5p. A previous study found that E2F2 plays a significant role in regulating PRR34-AS1 transcription, contributing to enhanced expression of PRR34-AS1 in HCC cells (91). PRR34-AS1 could drive the Wnt pathway via the upregulation of SOX12. PRR34-AS1 is highly expressed in HCC and promotes tumorigenesis, and this effect is closely related to the miR-296-5p/E2F2/SOX12 axis. Additionally, E2H2, an epigenetic regulator, participates in the process of tumorigenesis (165). In most HCC patients, E2H2 is highly expressed and acts as a vital oncogene (166, 167). The expression of H3K27me3 (histone H3 lysine 27 trimethylation) was elevated by silencing Wnt pathway suppressors from E2H2, presenting a positive association with development in HCC. These inhibiting factors of Wnt signaling mainly include Axin2, DKK1 and Prickle1 (168). LncRNA H19 exerts a pivotal effect on hepatocarcinogenesis by mediating the EZH2/Wnt/β-catenin signaling axis (169). A recent study confirmed that the combination of F2C and curcumin obviously blocks tumor initiation in HCC. Moreover, marked downregulation of E2H2, β-catenin, and H3K27me3 was observed in the F2C-treated group. However, Axin2, DKK1 and Prickle1 were upregulated in the F2C-treated group (92). Further exploration revealed that F2C could suppress the expression of EZH2-H19 and promote the upregulation of Wnt signaling inhibitors, resulting in the blockade of tumorigenicity in HCC.
The rapid proliferation and high aggressiveness of cancer cells might commonly indicate the tendency of most patients to exhibit dismal outcomes. Several experiments revealed that knockdown of lncRNA ANRIL significantly inhibited the proliferation, invasion, and metastasis of HCC cells but induced apoptosis (170). ANRIL silencing observably reduced the expression of miR-191 in HCC cells. When miR-191 was overexpressed, the effect of ANRIL knockdown was attenuated to some extent. In diverse studies, miRNA-191 is regarded as an oncogene related to the aggressiveness of HCC cells (146, 171). ANRIL silencing could modulate miR-191 expression to impede the upregulation of the NF-κB and Wnt/β-catenin pathways (93). The upregulation of down syndrome critical region 8 (DSCR8) was confirmed to have a vital impact on the promotion of cell proliferation and the cell cycle and the restriction of apoptosis. Knockdown of DSCR8 was found to reverse the corresponding effect (94). The specific mechanism of DSCR8 has been continually explored, and its role in sponging miR-485-5p in HCC cells has also emerged. Unlike DSCR8, miR-485-5p was downregulated in HCC tissues. It was reported that FZD7 functions as the essential receptor driving the Wnt/β-catenin cascade (172) and acts as an oncogene in HCC (57). MiR-485-5p was found to decrease FZD7 expression, increase β-catenin expression in the nucleus and cytoplasm, and increase c-Myc and cyclin D1 expression in HCC cells. Cyclin D1 and c-Myc are known to be downstream of Wnt/β-catenin signaling. Consequently, lncRNA DSCR8 might positively affect the activation of Wnt/β-catenin to accelerate the growth of HCC cells via the instrumental DSCR8/miR-485-5p/FZD7 axis (94).
FOXD2-AS1 overexpression was proven to predict a poor prognosis of patients with HCC. In contrast, knockdown of FOXD2-AS1 might exert a negative effect on proliferation, invasion, and EMT progression. EGR1 can promote FOXD2-AS1 expression at the transcriptional level by binding with an activator of FOXD2-AS1. The effect of FOXD2-AS1 on promoting proliferation, invasion, and EMT was proven to positively mediate the Wnt/β-catenin signaling pathway. The mechanism by which FOXD2-AS1 interacts with the Wnt/β-catenin pathway was further explored. FOXD2-AS1 might regulate targets by binding with EZH2, specifically resulting in the epigenetic silencing of downstream factors. EZH2 could lead to a reduction in the expression of DKK1. FOXD2-AS1 is considered a key regulator that enhances the expression of Wnt/β-catenin signaling by silencing DKK1 (95). Liang et al. (96) showed that lncRNA-NEF could induce the phosphorylation of β-catenin to suppress the Wnt/β-catenin signal cascade, subsequently driving FOXA2 expression to suppress HCC metastasis. Accumulating evidence has revealed that FOXA2 can suppress EMT to hinder the migration of liver cancer through the modulation of E-cadherin and MMP9 expression (173). However, recent research revealed that CASC2c tends to be expressed at low levels in HCC tissues and cells, while CASC2c expression strongly blocks proliferation and reduces aggressiveness but induces apoptosis in HCC. CASC2c is regarded as a critical regulator that mediates ERK1/2 and Wnt/β-catenin signaling, and high expression of CASC2c attenuates ERK1/2 and Wnt/β-catenin cascades (97). Thus, CASC2c upregulation inhibits the activation of ERK1/2 and the Wnt/β-catenin pathway to promote apoptosis and suppress invasion and proliferation. Despite the inhibitory role of CASC2c in HCC, many more experiments are needed to clarify the complex mechanism (Figure 3).
Figure 3 The specific mechanism of lncRNAs and the Wnt/β-catenin pathway in the proliferation, invasion and migration processes of HCC. LncRNA DSCR8 could sponge miR-485-5p to trigger Wnt/β-catenin signaling. Silencing of lncRNA ANRIL could block the progression of HCC by downregulating miRNA-191 to inhibit Wnt/β-catenin. Knockdown of lncRNA FOXD2-AS1 exerts a suppressive effect on the development of HCC via the inhibition of Wnt/β-catenin. Upregulation of CASC2c attenuates the activation of Wnt/β-catenin.
Overall, we elaborated the critical role of lncRNAs and the Wnt/β-catenin cascade in HCC to seek more hopeful and practical clinical applications to patients with HCC. Therefore, we further focused on diagnostic biomarkers, prognostic biomarkers and therapeutic targets of lncRNA and Wnt/β-catenin signaling.
Owing to the lack of characteristic symptoms in the early stages of HCC, most patients are diagnosed in advanced stages with short survival times. Thus, experts have explored more effective diagnostic biomarkers of HCC in recent years (174–176). It was reported that increasing expression of LINC00355:8 played the oncogenic role in proliferation, invasion and metastasis ability of HCC. Upregulated LINC00355:8 mediated miR-6777-3p expression by acting as the ceRNA, giving rising to activation of Wnt/β-catenin signaling (113). In addition, upregulation of lncRNA CASC15 was observed in HCC, which suggests its positive associations with larger tumor size, higher tumor stage, and early lymph node metastasis (99). Silencing of CASC15 suppressed HCC development. SOX4 is regarded as the downstream target of CASC15, and its increased expression has been proven to attenuate the effect of inhibiting CASC15. The upregulation of SOX4 by CASC15 was found to have a major impact on the Wnt/β-catenin cascade. LncRNA RUNX1-IT1 (the intronic transcript 1 of RUNX1) was reported to express lowly in HCC patients correlated with shorter survival time. Mechanistically, downregulated LncRNA RUNX1-IT1 could simultaneously mitigate MiR-632 and GSK-3β expression to cause restoration of β-catenin, remaining CSC property and promoting invasion and metastasis in HCC (162). With regard to the diagnostic roles of lncRNAs in HCC, more research is required.
Effective prognostic biomarkers have become an indispensable part of clinical estimates of recurrence probability in HCC. Kong et al. (100) found that overexpression of OTUD6B-AS1 in HCC tissues was generally related to poor outcomes for patients, which indicated its potential for prognosis prediction. When OTUD6B-AS1 was silenced in HCC, cell proliferation, invasion, and colony formation were rapidly restricted (100). LncRNA Small nucleolar RNA host gene 5 (SNHG5) includes six exons and two snoRNAs (177), competitively binding with miR-26a-5p to enhance activity of Wnt/β-catenin pathway, which advances hepatocellular carcinoma progression (106). Thus, high-expressed lncRNA SNHG5 have strong association with poor prognosis HCC patients. Although LINC01278 was downregulated in papillary thyroid carcinoma, it is expressed at high levels in HCC tissues. LINC01278 upregulation is frequently positively correlated with worse prognosis. When LINC01278 is expressed at low levels, the invasion and migration in HCC is weakened by alleviating the induction of β-catenin and TGF-β1 (101). These results provide numerous markers to precisely assess the recurrence probability of patients with HCC.
Targeted treatment has recently received broad attention from experts due to its potency and effectiveness in therapeutic methods of multiple cancers (178–180). LINC00662 is overexpressed in HCC and positively associated with tumor size, malignant behaviors and worse prognosis. It was reported that LINC00662 can interact with miR-15a, miR-16, and miR-107 to promote Wnt3a upregulation, triggering Wnt/β-catenin cascades in an autocrine manner. Therefore, the effect of advancing tumor growth and migration induced by LINC00662 might be related to activation of the Wnt pathway (102). Liu et al. (104) validated that lncRNA CCAL is overexpressed in HCC tissues and correlated with tumor size and migration. Decreased expression of CCAL was found to weaken the invasiveness of HCC. Mechanistically, downregulation of CCAL could reduce AP-2α expression and suppress Wnt/β-catenin signaling (104). Elevated expression of lncRNA DLGAP1-AS1 (discs, large homolog-associated protein 1 antisense RNA 1) could downregulate miR-26a-5p and miR-26b-5p to participate in tumorigenesis process of HCC (114). Inversely, silencing of DLGAP1-AS1 play the suppressive role in HCC. Inhibitors of miR-26a/b-5p might recover knockdown of DLGAP1-AS1 by stimulating activity of Wnt/β-catenin pathway. Therefore, lncRNA DLGAP1-AS1 might become an effective target for therapy of HCC patients and prolonging survival time. These findings offer hope for the development of clinical treatments in HCC.
The strikingly high incidence and mortality rates of HCC present threats to patient health and quality of life worldwide. The poor prognosis and rapid recurrence of HCC indicate the urgency of these studies and explorations on emerging methods for effective treatment. Fortunately, accumulating evidence has found that the interaction of lncRNAs and Wnt/β-catenin signaling provides a novel direction for understanding the pathogenesis of HCC that could improve clinical treatment and prolong survival time.
The Wnt/β-catenin pathway was proven to have a positive association with the EMT process. EMT is known as a regulator involved in the tumorigenesis and progression of several tumors. Thus, triggering the Wnt/β-catenin signaling pathway plays a vital role in the complicated mechanisms of various cancers. The degradation and accumulation of β-catenin have a great impact on the activation of the Wnt/β-catenin pathway. Recently, diverse lncRNAs have been demonstrated to participate in regulating β-catenin stability by affecting the formation of degradation complexes or regulating the transcriptional expression of β-catenin. Mechanistically, a wide range of lncRNAs are capable of functioning as ceRNAs to sponge targeted miRNAs, downstream effectors of lncRNAs, resulting in aberrant activation of the Wnt signaling pathway. Analogous mechanisms have also been identified in other malignant tumors and were found to be strongly related to the processes of cell proliferation, invasion, and metastasis.
The main focus of this review was to summarize most lncRNAs participating in the initiation and progression of hepatocellular carcinoma via the lncRNA/Wnt/β-catenin axis. These discoveries can be conducive to the identification of prospective molecular targets used for the effective treatment of HCC. However, several challenges have emerged in the application of clinical treatment. For instance, the upstream regulators of Wnt/β-catenin pathway activation vary, which suggests that other mechanisms and models affecting the pathogenesis of HCC remain unreported. Similarly, the structure and function of most lncRNAs are uncharacterized, which is an obstacle in research. Therefore, more research on lncRNAs related to Wnt/β-catenin and HCC is still needed.
All of the authors worked collaboratively on the work presented here. YH and WG designed the review. YX and XY wrote this manuscript. ZS searched the articles and made figures. All authors read and approved the final manuscript.
This work was supported by the National Natural Science Foundation of China (81902832), the Youth Talent Lifting Project of Henan Province (2021HYTP059), and Key Scientific Research Project of Henan Higher Education Institutions ofChina (21A320026). This work was also supported by Leading Talents of Zhongyuan Science and Technology Innovation (214200510027), Henan Provincial Medical Science and Technology Research Plan (SBGJ202102117 and SBGJ2018002), Henan Medical Science and Technology Joint Building Program (LHGJ20210324), Science and Technology Innovation Talents in Henan Universities (19HASTIT003), Outstanding Foreign Scientist Studio in Henan Province (GZS2020004), and the Gandan Xiangzhao Research Fund (GDXZ2022002).
The authors declare that the research was conducted in the absence of any commercial or financial relationships that could be construed as a potential conflict of interest.
All claims expressed in this article are solely those of the authors and do not necessarily represent those of their affiliated organizations, or those of the publisher, the editors and the reviewers. Any product that may be evaluated in this article, or claim that may be made by its manufacturer, is not guaranteed or endorsed by the publisher.
1. Bruix J, Gores GJ, Mazzaferro V. Hepatocellular Carcinoma: Clinical Frontiers and Perspectives. Gut (2014) 63(5):844–55. doi: 10.1136/gutjnl-2013-306627
2. Fornari F, Giovannini C, Piscaglia F, Gramantieri L. Elucidating the Molecular Basis of Sorafenib Resistance in HCC: Current Findings and Future Directions. J Hepatocell Carcinoma (2021) 8:741–57. doi: 10.2147/jhc.S285726
3. Xing M, Wang X, Kiken RA, He L, Zhang JY. Immunodiagnostic Biomarkers for Hepatocellular Carcinoma (HCC): The First Step in Detection and Treatment. Int J Mol Sci (2021) 22(11):6139. doi: 10.3390/ijms22116139
4. Forner A, Reig M, Bruix J. Hepatocellular Carcinoma. Lancet (London Engl) (2018) 391(10127):1301–14. doi: 10.1016/s0140-6736(18)30010-2
5. Lau W, Lai EJH INT. pdiH. Hepatocellular Carcinoma: Current Management and Recent Advances. Hepatobil Pancreat Dis Int (2008) 7(3):237–57.
6. Portolani N, Coniglio A, Ghidoni S, Giovanelli M, Benetti A, Tiberio GA, et al. Early and Late Recurrence After Liver Resection for Hepatocellular Carcinoma: Prognostic and Therapeutic Implications. Ann Surg (2006) 243(2):229–35. doi: 10.1097/01.sla.0000197706.21803.a1
7. Grandhi MS, Kim AK, Ronnekleiv-Kelly SM, Kamel IR, Ghasebeh MA, Pawlik TM. Hepatocellular Carcinoma: From Diagnosis to Treatment. Surg Oncol (2016) 25(2):74–85. doi: 10.1016/j.suronc.2016.03.002
8. Ogunwobi OO, Harricharran T, Huaman J, Galuza A, Odumuwagun O, Tan Y, et al. Mechanisms of Hepatocellular Carcinoma Progression. World J Gastroenterol (2019) 25(19):2279–93. doi: 10.3748/wjg.v25.i19.2279
9. Piñero F, Dirchwolf M, Pessôa MG. Biomarkers in Hepatocellular Carcinoma: Diagnosis, Prognosis and Treatment Response Assessment. Cells (2020) 9(6):1370. doi: 10.3390/cells9061370
10. El Jabbour T, Lagana SM, Lee H. Update on Hepatocellular Carcinoma: Pathologists' Review. World J Gastroenterol (2019) 25(14):1653–65. doi: 10.3748/wjg.v25.i14.1653
11. Fattovich G, Stroffolini T, Zagni I, Donato F. Hepatocellular Carcinoma in Cirrhosis: Incidence and Risk Factors. Gastroenterology (2004) 127(5 Suppl 1):S35–50. doi: 10.1053/j.gastro.2004.09.014
12. Kanda T, Goto T, Hirotsu Y, Moriyama M, Omata M. Molecular Mechanisms Driving Progression of Liver Cirrhosis Towards Hepatocellular Carcinoma in Chronic Hepatitis B and C Infections: A Review. Int J Mol Sci (2019) 20(6):1358. doi: 10.3390/ijms20061358
13. Rongrui L, Na H, Zongfang L, Fanpu J, Shiwen J. Epigenetic Mechanism Involved in the HBV/HCV-Related Hepatocellular Carcinoma Tumorigenesis. Curr Pharm Design (2014) 20(11):1715–25. doi: 10.2174/13816128113199990533
14. Liu F, Yuan JH, Huang JF, Yang F, Wang TT, Ma JZ, et al. Long Noncoding RNA FTX Inhibits Hepatocellular Carcinoma Proliferation and Metastasis by Binding MCM2 and miR-374a. Oncogene (2016) 35(41):5422–34. doi: 10.1038/onc.2016.80
15. Xu D, Yang F, Yuan JH, Zhang L, Bi HS, Zhou CC, et al. Long Noncoding RNAs Associated With Liver Regeneration 1 Accelerates Hepatocyte Proliferation During Liver Regeneration by Activating Wnt/β-Catenin Signaling. Hepatol (Baltimore Md) (2013) 58(2):739–51. doi: 10.1002/hep.26361
16. Zhu P, Wang Y, Huang G, Ye B, Liu B, Wu J, et al. Lnc-β-Catm Elicits EZH2-Dependent β-Catenin Stabilization and Sustains Liver CSC Self-Renewal. Nat Struct Mol Biol (2016) 23(7):631–9. doi: 10.1038/nsmb.3235
17. Klingenberg M, Matsuda A, Diederichs S, Patel T. Non-Coding RNA in Hepatocellular Carcinoma: Mechanisms, Biomarkers and Therapeutic Targets. J Hepatol (2017) 67(3):603–18. doi: 10.1016/j.jhep.2017.04.009
18. Ding B, Lou W, Xu L, Fan W. Non-Coding RNA in Drug Resistance of Hepatocellular Carcinoma. Biosci Rep (2018) 38(5):BSR20180915. doi: 10.1042/bsr20180915
19. Wang X, Sun W, Shen W, Xia M, Chen C, Xiang D, et al. Long Non-Coding RNA DILC Regulates Liver Cancer Stem Cells via IL-6/STAT3 Axis. J Hepatol (2016) 64(6):1283–94. doi: 10.1016/j.jhep.2016.01.019
20. Hu Q, Egranov SD, Lin C, Yang L. Long Noncoding RNA Loss in Immune Suppression in Cancer. Pharmacol Ther (2020) 213:107591. doi: 10.1016/j.pharmthera.2020.107591
21. Olivero CE, Martínez-Terroba E, Zimmer J, Liao C, Tesfaye E, Hooshdaran N, et al. P53 Activates the Long Noncoding RNA Pvt1b to Inhibit Myc and Suppress Tumorigenesis. Mol Cell (2020) 77(4):761–74.e8. doi: 10.1016/j.molcel.2019.12.014
22. Ta HQ, Whitworth H, Yin Y, Conaway M, Frierson HF Jr, Campbell MJ, et al. Discovery of a Novel Long Noncoding RNA Overlapping the LCK Gene That Regulates Prostate Cancer Cell Growth. Mol Cancer (2019) 18(1):113. doi: 10.1186/s12943-019-1039-6
23. Zhang Y, Zhang J, Mao L, Li X. Long Noncoding RNA HCG11 Inhibited Growth and Invasion in Cervical Cancer by Sponging miR-942-5p and Targeting GFI1. Cancer Med (2020) 9(19):7062–71. doi: 10.1002/cam4.3203
24. Xu M, Zhao X, Zhao S, Yang Z, Yuan W, Han H, et al. Landscape Analysis of lncRNAs Shows That DDX11-AS1 Promotes Cell-Cycle Progression in Liver Cancer Through the PARP1/p53 Axis. Cancer Lett (2021) 520:282–94. doi: 10.1016/j.canlet.2021.08.001
25. Salik B, Yi H, Hassan N, Santiappillai N, Vick B, Connerty P, et al. Targeting RSPO3-LGR4 Signaling for Leukemia Stem Cell Eradication in Acute Myeloid Leukemia. Cancer Cell (2020) 38(2):263–78.e6. doi: 10.1016/j.ccell.2020.05.014
26. Soleas JP, D'Arcangelo E, Huang L, Karoubi G, Nostro MC, McGuigan AP, et al. Assembly of Lung Progenitors Into Developmentally-Inspired Geometry Drives Differentiation via Cellular Tension. Biomaterials (2020) 254:120128. doi: 10.1016/j.biomaterials.2020.120128
27. Choi BR, Cave C, Na CH, Sockanathan S. GDE2-Dependent Activation of Canonical Wnt Signaling in Neurons Regulates Oligodendrocyte Maturation. Cell Rep (2020) 31(5):107540. doi: 10.1016/j.celrep.2020.107540
28. Clevers H, Nusse R. Wnt/β-Catenin Signaling and Disease. Cell (2012) 149(6):1192–205. doi: 10.1016/j.cell.2012.05.012
29. Le PN, Keysar SB, Miller B, Eagles JR, Chimed TS, Reisinger J, et al. Wnt Signaling Dynamics in Head and Neck Squamous Cell Cancer Tumor-Stroma Interactions. Mol Carcinog (2019) 58(3):398–410. doi: 10.1002/mc.22937
30. Zhang Y, Wang X. Targeting the Wnt/β-Catenin Signaling Pathway in Cancer. J Hematol Oncol (2020) 13(1):165. doi: 10.1186/s13045-020-00990-3
31. Zhang R, Wang J, Jia E, Zhang J, Liu N, Chi C. lncRNA BCAR4 Sponges Mir−370−3p to Promote Bladder Cancer Progression via Wnt Signaling. Int J Mol Med (2020) 45(2):578–88. doi: 10.3892/ijmm.2019.4444
32. Guo J, Chen Z, Jiang H, Yu Z, Peng J, Xie J, et al. The lncRNA DLX6-AS1 Promoted Cell Proliferation, Invasion, Migration and Epithelial-to-Mesenchymal Transition in Bladder Cancer via Modulating Wnt/β-Catenin Signaling Pathway. Cancer Cell Int (2019) 19:312. doi: 10.1186/s12935-019-1010-z
33. Chen M, Zhang R, Lu L, Du J, Chen C, Ding K, et al. LncRNA PVT1 Accelerates Malignant Phenotypes of Bladder Cancer Cells by Modulating miR-194-5p/BCLAF1 Axis as a ceRNA. Aging (2020) 12(21):22291–312. doi: 10.18632/aging.202203
34. Li Y, Zhu G, Zeng W, Wang J, Li Z, Wang B, et al. Long Noncoding RNA AB073614 Promotes the Malignance of Glioma by Activating Wnt/β-Catenin Signaling Through Downregulating SOX7. Oncotarget (2017) 8(39):65577–87. doi: 10.18632/oncotarget.19305
35. Cui Y, Zhang F, Zhu C, Geng L, Tian T, Liu H. Upregulated lncRNA SNHG1 Contributes to Progression of Non-Small Cell Lung Cancer Through Inhibition of miR-101-3p and Activation of Wnt/β-Catenin Signaling Pathway. Oncotarget (2017) 8(11):17785–94. doi: 10.18632/oncotarget.14854
36. Ma F, Ye H, He HH, Gerrin SJ, Chen S, Tanenbaum BA, et al. SOX9 Drives WNT Pathway Activation in Prostate Cancer. J Clin Invest (2016) 126(5):1745–58. doi: 10.1172/jci78815
37. Prévostel C, Rammah-Bouazza C, Trauchessec H, Canterel-Thouennon L, Busson M, Ychou M, et al. SOX9 Is an Atypical Intestinal Tumor Suppressor Controlling the Oncogenic Wnt/ß-Catenin Signaling. Oncotarget (2016) 7(50):82228–43. doi: 10.18632/oncotarget.10573
38. Santos JC, Carrasco-Garcia E, Garcia-Puga M, Aldaz P, Montes M, Fernandez-Reyes M, et al. SOX9 Elevation Acts With Canonical WNT Signaling to Drive Gastric Cancer Progression. Cancer Res (2016) 76(22):6735–46. doi: 10.1158/0008-5472.Can-16-1120
39. Bell IJ, Horn MS, Van Raay TJ. Bridging the Gap Between Non-Canonical and Canonical Wnt Signaling Through Vangl2. Semin Cell Dev Biol (2021) S1084-9521(21)00267-6. doi: 10.1016/j.semcdb.2021.10.004
40. Lai KKY, Nguyen C, Lee KS, Lee A, Lin DP, Teo JL, et al. Convergence of Canonical and Non-Canonical Wnt Signal: Differential Kat3 Coactivator Usage. Curr Mol Pharmacol (2019) 12(3):167–83. doi: 10.2174/1874467212666190304121131
41. Flores-Hernández E, Velázquez DM, Castañeda-Patlán MC, Fuentes-García G, Fonseca-Camarillo G, Yamamoto-Furusho JK, et al. Canonical and Non-Canonical Wnt Signaling Are Simultaneously Activated by Wnts in Colon Cancer Cells. Cell Signal (2020) 72:109636. doi: 10.1016/j.cellsig.2020.109636
42. van Amerongen R, Nusse R. Towards an Integrated View of Wnt Signaling in Development. Dev (Cambr Engl) (2009) 136(19):3205–14. doi: 10.1242/dev.033910
43. Zhang X, Wang L, Qu Y. Targeting the β-Catenin Signaling for Cancer Therapy. Pharmacol Res (2020) 160:104794. doi: 10.1016/j.phrs.2020.104794
44. Lim ZF, Ma PC. Emerging Insights of Tumor Heterogeneity and Drug Resistance Mechanisms in Lung Cancer Targeted Therapy. J Hematol Oncol (2019) 12(1):134. doi: 10.1186/s13045-019-0818-2
45. Wei CY, Zhu MX, Yang YW, Zhang PF, Yang X, Peng R, et al. Downregulation of RNF128 Activates Wnt/β-Catenin Signaling to Induce Cellular EMT and Stemness via CD44 and CTTN Ubiquitination in Melanoma. J Hematol Oncol (2019) 12(1):21. doi: 10.1186/s13045-019-0711-z
46. Stamos JL, Weis WI. The β-Catenin Destruction Complex. Cold Spring Harbor Perspect Biol (2013) 5(1):a007898. doi: 10.1101/cshperspect.a007898
47. Hart MJ, de los Santos R, Albert IN, Rubinfeld B, Polakis P. Downregulation of Beta-Catenin by Human Axin and Its Association With the APC Tumor Suppressor, Beta-Catenin and GSK3 Beta. Curr Biol CB (1998) 8(10):573–81. doi: 10.1016/s0960-9822(98)70226-x
48. Kimelman D, Xu W. Beta-Catenin Destruction Complex: Insights and Questions From a Structural Perspective. Oncogene (2006) 25(57):7482–91. doi: 10.1038/sj.onc.1210055
49. Khalaf AM, Fuentes D, Morshid AI, Burke MR, Kaseb AO, Hassan M, et al. Role of Wnt/β-Catenin Signaling in Hepatocellular Carcinoma, Pathogenesis, and Clinical Significance. J Hepatocell Carcinoma (2018) 5:61–73. doi: 10.2147/jhc.S156701
50. Lai TY, Su CC, Kuo WW, Yeh YL, Kuo WH, Tsai FJ, et al. β-Catenin Plays a Key Role in Metastasis of Human Hepatocellular Carcinoma. Oncol Rep (2011) 26(2):415–22. doi: 10.3892/or.2011.1323
51. Laurent-Puig P, Legoix P, Bluteau O, Belghiti J, Franco D, Binot F, et al. Genetic Alterations Associated With Hepatocellular Carcinomas Define Distinct Pathways of Hepatocarcinogenesis. Gastroenterology (2001) 120(7):1763–73. doi: 10.1053/gast.2001.24798
52. Lian Z, Liu J, Li L, Li X, Clayton M, Wu MC, et al. Enhanced Cell Survival of Hep3B Cells by the Hepatitis B X Antigen Effector, URG11, Is Associated With Upregulation of Beta-Catenin. Hepatol (Baltimore Md) (2006) 43(3):415–24. doi: 10.1002/hep.21053
53. Bhanot P, Brink M, Samos CH, Hsieh JC, Wang Y, Macke JP, et al. A New Member of the Frizzled Family From Drosophila Functions as a Wingless Receptor. Nature (1996) 382(6588):225–30. doi: 10.1038/382225a0
54. Liu J, Ding X, Tang J, Cao Y, Hu P, Zhou F, et al. Enhancement of Canonical Wnt/β-Catenin Signaling Activity by HCV Core Protein Promotes Cell Growth of Hepatocellular Carcinoma Cells. PloS One (2011) 6(11):e27496. doi: 10.1371/journal.pone.0027496
55. Fukutomi T, Zhou Y, Kawai S, Eguchi H, Wands JR, Li J. Hepatitis C Virus Core Protein Stimulates Hepatocyte Growth: Correlation With Upregulation of Wnt-1 Expression. Hepatol (Baltimore Md) (2005) 41(5):1096–105. doi: 10.1002/hep.20668
56. Kim M, Lee HC, Tsedensodnom O, Hartley R, Lim YS, Yu E, et al. Functional Interaction Between Wnt3 and Frizzled-7 Leads to Activation of the Wnt/beta-Catenin Signaling Pathway in Hepatocellular Carcinoma Cells. J Hepatol (2008) 48(5):780–91. doi: 10.1016/j.jhep.2007.12.020
57. Merle P, de la Monte S, Kim M, Herrmann M, Tanaka S, Von Dem Bussche A, et al. Functional Consequences of Frizzled-7 Receptor Overexpression in Human Hepatocellular Carcinoma. Gastroenterology (2004) 127(4):1110–22. doi: 10.1053/j.gastro.2004.07.009
58. Zhang Y, Wei W, Cheng N, Wang K, Li B, Jiang X, et al. Hepatitis C Virus-Induced Up-Regulation of microRNA-155 Promotes Hepatocarcinogenesis by Activating Wnt Signaling. Hepatol (Baltimore Md) (2012) 56(5):1631–40. doi: 10.1002/hep.25849
59. Park CY, Choi SH, Kang SM, Kang JI, Ahn BY, Kim H, et al. Nonstructural 5A Protein Activates Beta-Catenin Signaling Cascades: Implication of Hepatitis C Virus-Induced Liver Pathogenesis. J Hepatol (2009) 51(5):853–64. doi: 10.1016/j.jhep.2009.06.026
60. Street A, Macdonald A, McCormick C, Harris M. Hepatitis C Virus NS5A-Mediated Activation of Phosphoinositide 3-Kinase Results in Stabilization of Cellular Beta-Catenin and Stimulation of Beta-Catenin-Responsive Transcription. J Virol (2005) 79(8):5006–16. doi: 10.1128/jvi.79.8.5006-5016.2005
61. Huo X, Han S, Wu G, Latchoumanin O, Zhou G, Hebbard L, et al. Dysregulated Long Noncoding RNAs (lncRNAs) in Hepatocellular Carcinoma: Implications for Tumorigenesis, Disease Progression, and Liver Cancer Stem Cells. Mol Cancer (2017) 16(1):165. doi: 10.1186/s12943-017-0734-4
62. Ponting CP, Oliver PL, Reik W. Evolution and Functions of Long Noncoding RNAs. Cell (2009) 136(4):629–41. doi: 10.1016/j.cell.2009.02.006
63. Ransohoff JD, Wei Y, Khavari PA. The Functions and Unique Features of Long Intergenic Non-Coding RNA. Nat Rev Mol Cell Biol (2018) 19(3):143–57. doi: 10.1038/nrm.2017.104
64. Batista PJ, Chang HY. Long Noncoding RNAs: Cellular Address Codes in Development and Disease. Cell (2013) 152(6):1298–307. doi: 10.1016/j.cell.2013.02.012
65. Kopp F, Mendell JT. Functional Classification and Experimental Dissection of Long Noncoding RNAs. Cell (2018) 172(3):393–407. doi: 10.1016/j.cell.2018.01.011
66. Adams BD, Parsons C, Walker L, Zhang WC, Slack FJ. Targeting Noncoding RNAs in Disease. J Clin Invest (2017) 127(3):761–71. doi: 10.1172/jci84424
67. Wong CM, Tsang FH, Ng IO. Non-Coding RNAs in Hepatocellular Carcinoma: Molecular Functions and Pathological Implications. Nat Rev Gastroenterol Hepatol (2018) 15(3):137–51. doi: 10.1038/nrgastro.2017.169
68. Liu Z, Wang Y, Wang L, Yao B, Sun L, Liu R, et al. Long Non-Coding RNA AGAP2-AS1, Functioning as a Competitive Endogenous RNA, Upregulates ANXA11 Expression by Sponging miR-16-5p and Promotes Proliferation and Metastasis in Hepatocellular Carcinoma. J Exp Clin Cancer Res CR (2019) 38(1):194. doi: 10.1186/s13046-019-1188-x
69. Shi X, Sun M, Liu H, Yao Y, Song Y. Long Non-Coding RNAs: A New Frontier in the Study of Human Diseases. Cancer Lett (2013) 339(2):159–66. doi: 10.1016/j.canlet.2013.06.013
70. Lambert MP, Paliwal A, Vaissière T, Chemin I, Zoulim F, Tommasino M, et al. Aberrant DNA Methylation Distinguishes Hepatocellular Carcinoma Associated With HBV and HCV Infection and Alcohol Intake. J Hepatol (2011) 54(4):705–15. doi: 10.1016/j.jhep.2010.07.027
71. Murray-Stewart T, Sierra JC, Piazuelo MB, Mera RM, Chaturvedi R, Bravo LE, et al. Epigenetic Silencing of miR-124 Prevents Spermine Oxidase Regulation: Implications for Helicobacter Pylori-Induced Gastric Cancer. Oncogene (2016) 35(42):5480–8. doi: 10.1038/onc.2016.91
72. Cheng D, Deng J, Zhang B, He X, Meng Z, Li G, et al. LncRNA HOTAIR Epigenetically Suppresses miR-122 Expression in Hepatocellular Carcinoma via DNA Methylation. EBioMedicine (2018) 36:159–70. doi: 10.1016/j.ebiom.2018.08.055
73. Zhang L, Yang F, Yuan JH, Yuan SX, Zhou WP, Huo XS, et al. Epigenetic Activation of the MiR-200 Family Contributes to H19-Mediated Metastasis Suppression in Hepatocellular Carcinoma. Carcinogenesis (2013) 34(3):577–86. doi: 10.1093/carcin/bgs381
74. Malakar P, Shilo A, Mogilevsky A, Stein I, Pikarsky E, Nevo Y, et al. Long Noncoding RNA MALAT1 Promotes Hepatocellular Carcinoma Development by SRSF1 Upregulation and mTOR Activation. Cancer Res (2017) 77(5):1155–67. doi: 10.1158/0008-5472.Can-16-1508
75. Beroukhim R, Mermel CH, Porter D, Wei G, Raychaudhuri S, Donovan J, et al. The Landscape of Somatic Copy-Number Alteration Across Human Cancers. Nature (2010) 463(7283):899–905. doi: 10.1038/nature08822
76. Jiang R, Niu X, Huang Y, Wang X. β-Catenin Is Important for Cancer Stem Cell Generation and Tumorigenic Activity in Nasopharyngeal Carcinoma. Acta Biochim Biophys Sin (2016) 48(3):229–37. doi: 10.1093/abbs/gmv134
77. Ma Y, Yang Y, Wang F, Moyer MP, Wei Q, Zhang P, et al. Long Non-Coding RNA CCAL Regulates Colorectal Cancer Progression by Activating Wnt/β-Catenin Signalling Pathway via Suppression of Activator Protein 2α. Gut (2016) 65(9):1494–504. doi: 10.1136/gutjnl-2014-308392
78. Fan Y, Shen B, Tan M, Mu X, Qin Y, Zhang F, et al. Long Non-Coding RNA UCA1 Increases Chemoresistance of Bladder Cancer Cells by Regulating Wnt Signaling. FEBS J (2014) 281(7):1750–8. doi: 10.1111/febs.12737
79. Tang Q, Zheng X, Zhang J. Long Non-Coding RNA CRNDE Promotes Heptaocellular Carcinoma Cell Proliferation by Regulating PI3K/Akt /β-Catenin Signaling. Biomed Pharmacother = Biomed Pharmacother (2018) 103:1187–93. doi: 10.1016/j.biopha.2018.04.128
80. Zhu L, Yang N, Du G, Li C, Liu G, Liu S, et al. LncRNA CRNDE Promotes the Epithelial-Mesenchymal Transition of Hepatocellular Carcinoma Cells via Enhancing the Wnt/β-Catenin Signaling Pathway. J Cell Biochem (2018) 120(2):1156–64. doi: 10.1002/jcb.26762
81. Zhang N, Chen X. A Positive Feedback Loop Involving the LINC00346/β-Catenin/MYC Axis Promotes Hepatocellular Carcinoma Development. Cell Oncol (Dordrecht) (2020) 43(1):137–53. doi: 10.1007/s13402-019-00478-4
82. Han K, Li C, Zhang X, Shang L. DUXAP10 Inhibition Attenuates the Proliferation and Metastasis of Hepatocellular Carcinoma Cells by Regulation of the Wnt/β-Catenin and PI3K/Akt Signaling Pathways. Biosci Rep (2019) 39(5):BSR20181457. doi: 10.1042/bsr20181457
83. Zhang W, Wu Y, Hou B, Wang Y, Deng D, Fu Z, et al. A SOX9-AS1/miR-5590-3p/SOX9 Positive Feedback Loop Drives Tumor Growth and Metastasis in Hepatocellular Carcinoma Through the Wnt/β-Catenin Pathway. Mol Oncol (2019) 13(10):2194–210. doi: 10.1002/1878-0261.12560
84. Lin X, Xiaoqin H, Jiayu C, Li F, Yue L, Ximing X. Long Non-Coding RNA Mir143hg Predicts Good Prognosis and Inhibits Tumor Multiplication and Metastasis by Suppressing Mitogen-Activated Protein Kinase and Wnt Signaling Pathways in Hepatocellular Carcinoma. Hepatol Res Off J Jpn Soc Hepatol (2019) 49(8):902–18. doi: 10.1111/hepr.13344
85. Liu Q, Yu X, Yang M, Li X, Zhai X, Lian Y, et al. A Study of the Mechanism of lncRNA-CR594175 in Regulating Proliferation and Invasion of Hepatocellular Carcinoma Cells In Vivo and In Vitro. Infect Agents Cancer (2020) 15:55. doi: 10.1186/s13027-020-00321-8
86. Xu G, Zhu Y, Liu H, Liu Y, Zhang X. LncRNA MIR194-2hg Promotes Cell Proliferation and Metastasis via Regulation of miR-1207-5p/TCF19/Wnt/β-Catenin Signaling in Liver Cancer. OncoTargets Ther (2020) 13:9887–99. doi: 10.2147/ott.S264614
87. Yao J, Yang Z, Yang J, Wang ZG, Zhang ZY. Long Non-Coding RNA FEZF1-AS1 Promotes the Proliferation and Metastasis of Hepatocellular Carcinoma via Targeting miR-107/Wnt/β-Catenin Axis. Aging (2021) 13(10):13726–38. doi: 10.18632/aging.202960
88. Duan Y, Chen J, Yang Y, Qu Z, Lu Y, Sun D. LncRNA HOTAIR Contributes Taxol-Resistance of Hepatocellular Carcinoma Cells via Activating AKT Phosphorylation by Down-Regulating miR-34a. Biosci Rep (2020) 40(7):BSR20201627. doi: 10.1042/bsr20201627
89. Fu X, Zhu X, Qin F, Zhang Y, Lin J, Ding Y, et al. Linc00210 Drives Wnt/β-Catenin Signaling Activation and Liver Tumor Progression Through CTNNBIP1-Dependent Manner. Mol Cancer (2018) 17(1):73. doi: 10.1186/s12943-018-0783-3
90. Wang Y, He L, Du Y, Zhu P, Huang G, Luo J, et al. The Long Noncoding RNA Lnctcf7 Promotes Self-Renewal of Human Liver Cancer Stem Cells Through Activation of Wnt Signaling. Cell Stem Cell (2015) 16(4):413–25. doi: 10.1016/j.stem.2015.03.003
91. Qin M, Meng Y, Luo C, He S, Qin F, Yin Y, et al. lncRNA PRR34-AS1 Promotes HCC Development via Modulating Wnt/β-Catenin Pathway by Absorbing miR-296-5p and Upregulating E2F2 and SOX12. Mol Ther Nucleic Acids (2021) 25:37–52. doi: 10.1016/j.omtn.2021.04.016
92. Khan H, Ni Z, Feng H, Xing Y, Wu X, Huang D, et al. Combination of Curcumin With N-N-Butyl Haloperidol Iodide Inhibits Hepatocellular Carcinoma Malignant Proliferation by Downregulating Enhancer of Zeste Homolog 2 (EZH2) - lncRNA H19 to Silence Wnt/β-Catenin Signaling. Phytomed Int J Phytother Phytopharmacol (2021) 91:153706. doi: 10.1016/j.phymed.2021.153706
93. Huang D, Bi C, Zhao Q, Ding X, Bian C, Wang H, et al. Knockdown Long Non-Coding RNA ANRIL Inhibits Proliferation, Migration and Invasion of HepG2 Cells by Down-Regulation of miR-191. BMC Cancer (2018) 18(1):919. doi: 10.1186/s12885-018-4831-6
94. Wang Y, Sun L, Wang L, Liu Z, Li Q, Yao B, et al. Long Non-Coding RNA DSCR8 Acts as a Molecular Sponge for miR-485-5p to Activate Wnt/β-Catenin Signal Pathway in Hepatocellular Carcinoma. Cell Death Dis (2018) 9(9):851. doi: 10.1038/s41419-018-0937-7
95. Lei T, Zhu X, Zhu K, Jia F, Li S. EGR1-Induced Upregulation of lncRNA FOXD2-AS1 Promotes the Progression of Hepatocellular Carcinoma via Epigenetically Silencing DKK1 and Activating Wnt/β-Catenin Signaling Pathway. Cancer Biol Ther (2019) 20(7):1007–16. doi: 10.1080/15384047.2019.1595276
96. Liang WC, Ren JL, Wong CW, Chan SO, Waye MM, Fu WM, et al. LncRNA-NEF Antagonized Epithelial to Mesenchymal Transition and Cancer Metastasis via Cis-Regulating FOXA2 and Inactivating Wnt/β-Catenin Signaling. Oncogene (2018) 37(11):1445–56. doi: 10.1038/s41388-017-0041-y
97. Li QY, Yang K, Liu FG, Sun XG, Chen L, Xiu H, et al. Long Noncoding RNA CASC2c Inhibited Cell Proliferation in Hepatocellular Carcinoma by Inactivated ERK1/2 and Wnt/β-Catenin Signaling Pathway. Clin Trans Oncol Off Publ Fed Spanish Oncol Soc Natl Cancer Institute Mexico (2020) 22(3):302–10. doi: 10.1007/s12094-019-02223-7
98. Wu S, Chen S, Lin N, Yang J. Long Non-Coding RNA SUMO1P3 Promotes Hepatocellular Carcinoma Progression Through Activating Wnt/β-Catenin Signalling Pathway by Targeting miR-320a. J Cell Mol Med (2020) 24(5):3108–16. doi: 10.1111/jcmm.14977
99. Wang C, Zi H, Wang Y, Li B, Ge Z, Ren X. LncRNA CASC15 Promotes Tumour Progression Through SOX4/Wnt/β-Catenin Signalling Pathway in Hepatocellular Carcinoma. Artif Cells Nanomed Biotechnol (2020) 48(1):763–9. doi: 10.1080/21691401.2019.1576713
100. Kong S, Xue H, Li Y, Li P, Ma F, Liu M, et al. The Long Noncoding RNA OTUD6B-AS1 Enhances Cell Proliferation and the Invasion of Hepatocellular Carcinoma Cells Through Modulating GSKIP/Wnt/β-Catenin Signalling via the Sequestration of miR-664b-3p. Exp Cell Res (2020) 395(1):112180. doi: 10.1016/j.yexcr.2020.112180
101. Huang WJ, Tian XP, Bi SX, Zhang SR, He TS, Song LY, et al. The β-Catenin/TCF-4-LINC01278-miR-1258-Smad2/3 Axis Promotes Hepatocellular Carcinoma Metastasis. Oncogene (2020) 39(23):4538–50. doi: 10.1038/s41388-020-1307-3
102. Tian X, Wu Y, Yang Y, Wang J, Niu M, Gao S, et al. Long Noncoding RNA LINC00662 Promotes M2 Macrophage Polarization and Hepatocellular Carcinoma Progression via Activating Wnt/β-Catenin Signaling. Mol Oncol (2020) 14(2):462–83. doi: 10.1002/1878-0261.12606
103. Wang XL, Shi M, Xiang T, Bu YZ. Long Noncoding RNA DGCR5 Represses Hepatocellular Carcinoma Progression by Inactivating Wnt Signaling Pathway. J Cell Biochem (2019) 120(1):275–82. doi: 10.1002/jcb.27342
104. Liu Y, Yang Y, Wang T, Wang L, Wang X, Li T, et al. Long Non-Coding RNA CCAL Promotes Hepatocellular Carcinoma Progression by Regulating AP-2α and Wnt/β-Catenin Pathway. Int J Biol Macromol (2018) 109:424–34. doi: 10.1016/j.ijbiomac.2017.12.110
105. Chen W, You J, Zheng Q, Zhu YY. Downregulation of lncRNA OGFRP1 Inhibits Hepatocellular Carcinoma Progression by AKT/mTOR and Wnt/β-Catenin Signaling Pathways. Cancer Manage Res (2018) 10:1817–26. doi: 10.2147/cmar.S164911
106. Li Y, Guo D, Zhao Y, Ren M, Lu G, Wang Y, et al. Long Non-Coding RNA SNHG5 Promotes Human Hepatocellular Carcinoma Progression by Regulating miR-26a-5p/GSK3β Signal Pathway. Cell Death Dis (2018) 9(9):888. doi: 10.1038/s41419-018-0882-5
107. Fan X, Song J, Zhao Z, Chen M, Tu J, Lu C, et al. Piplartine Suppresses Proliferation and Invasion of Hepatocellular Carcinoma by LINC01391-Modulated Wnt/β-Catenin Pathway Inactivation Through ICAT. Cancer Lett (2019) 460:119–27. doi: 10.1016/j.canlet.2019.06.008
108. Yao X, You G, Zhou C, Zhang D. LncRNA ASB16-AS1 Promotes Growth And Invasion Of Hepatocellular Carcinoma Through Regulating miR-1827/FZD4 Axis And Activating Wnt/β-Catenin Pathway. Cancer Manage Res (2019) 11:9371–8. doi: 10.2147/cmar.S220434
109. Sun L, Wang L, Chen T, Shi Y, Yao B, Liu Z, et al. LncRNA RUNX1-IT1 Which Is Downregulated by Hypoxia-Driven Histone Deacetylase 3 Represses Proliferation and Cancer Stem-Like Properties in Hepatocellular Carcinoma Cells. Cell Death Dis (2020) 11(2):95. doi: 10.1038/s41419-020-2274-x
110. Shao J, Shi CJ, Li Y, Zhang FW, Pan FF, Fu WM, et al. LincROR Mediates the Suppressive Effects of Curcumin on Hepatocellular Carcinoma Through Inactivating Wnt/β-Catenin Signaling. Front Pharmacol (2020) 11:847. doi: 10.3389/fphar.2020.00847
111. Song XZ, Xu XJ, Ren XN, Ruan XX, Wang YL, Yao TT. LncRNA ANCR Suppresses the Progression of Hepatocellular Carcinoma Through the Inhibition of Wnt/β-Catenin Signaling Pathway. OncoTargets Ther (2020) 13:8907–17. doi: 10.2147/ott.S260556
112. Chen MH, Fu LS, Zhang F, Yang Y, Wu XZ. LncAY Controls BMI1 Expression and Activates BMI1/Wnt/β-Catenin Signaling Axis in Hepatocellular Carcinoma. Life Sci (2021) 280:119748. doi: 10.1016/j.lfs.2021.119748
113. Zhou F, Lei Y, Xu X, Zhou H, Liu H, Jiang J, et al. LINC00355:8 Promotes Cell Proliferation and Migration With Invasion via the MiR-6777-3p/Wnt10b Axis in Hepatocellular Carcinoma. J Cancer (2020) 11(19):5641–55. doi: 10.7150/jca.43831
114. Lin Y, Jian Z, Jin H, Wei X, Zou X, Guan R, et al. Long Non-Coding RNA DLGAP1-AS1 Facilitates Tumorigenesis and Epithelial-Mesenchymal Transition in Hepatocellular Carcinoma via the Feedback Loop of miR-26a/B-5p/IL-6/JAK2/STAT3 and Wnt/β-Catenin Pathway. Cell Death Dis (2020) 11(1):34. doi: 10.1038/s41419-019-2188-7
115. Krajewska M, Moss SF, Krajewski S, Song K, Holt PR, Reed JC. Elevated Expression of Bcl-X and Reduced Bak in Primary Colorectal Adenocarcinomas. Cancer Res (1996) 56(10):2422–7.
116. Ellis BC, Graham LD, Molloy PL. CRNDE, A Long Non-Coding RNA Responsive to Insulin/IGF Signaling, Regulates Genes Involved in Central Metabolism. Biochim Biophys Acta (2014) 1843(2):372–86. doi: 10.1016/j.bbamcr.2013.10.016
117. Hu CE, Du PZ, Zhang HD, Huang GJ. Long Noncoding RNA CRNDE Promotes Proliferation of Gastric Cancer Cells by Targeting miR-145. Cell Physiol Biochem Int J Exp Cell Physiol Biochem Pharmacol (2017) 42(1):13–21. doi: 10.1159/000477107
118. Meng YB, He X, Huang YF, Wu QN, Zhou YC, Hao DJ. Long Noncoding RNA CRNDE Promotes Multiple Myeloma Cell Growth by Suppressing miR-451. Oncol Res (2017) 25(7):1207–14. doi: 10.3727/096504017x14886679715637
119. Liu XX, Xiong HP, Huang JS, Qi K, Xu JJ. Highly Expressed Long Non-Coding RNA CRNDE Promotes Cell Proliferation Through PI3K/AKT Signalling in Non-Small Cell Lung Carcinoma. Clin Exp Pharmacol Physiol (2017) 44(8):895–902. doi: 10.1111/1440-1681.12780
120. Nieszporek A, Skrzypek K, Adamek G, Majka M. Molecular Mechanisms of Epithelial to Mesenchymal Transition in Tumor Metastasis. Acta Biochim Polonica (2019) 66(4):509–20. doi: 10.18388/abp.2019_2899
121. Wendt MK, Balanis N, Carlin CR, Schiemann WP. STAT3 and Epithelial-Mesenchymal Transitions in Carcinomas. Jak-Stat (2014) 3(1):e28975. doi: 10.4161/jkst.28975
122. De Craene B, Berx G. Regulatory Networks Defining EMT During Cancer Initiation and Progression. Nat Rev Cancer (2013) 13(2):97–110. doi: 10.1038/nrc3447
123. Cho SH, Cepko CL. Wnt2b/beta-Catenin-Mediated Canonical Wnt Signaling Determines the Peripheral Fates of the Chick Eye. Dev (Cambr Engl) (2006) 133(16):3167–77. doi: 10.1242/dev.02474
124. Goss AM, Tian Y, Tsukiyama T, Cohen ED, Zhou D, Lu MM, et al. Wnt2/2b and Beta-Catenin Signaling Are Necessary and Sufficient to Specify Lung Progenitors in the Foregut. Dev Cell (2009) 17(2):290–8. doi: 10.1016/j.devcel.2009.06.005
125. Liu J, Wang Z, Tang J, Tang R, Shan X, Zhang W, et al. Hepatitis C Virus Core Protein Activates Wnt/β-Catenin Signaling Through Multiple Regulation of Upstream Molecules in the SMMC-7721 Cell Line. Arch Virol (2011) 156(6):1013–23. doi: 10.1007/s00705-011-0943-x
127. Wang S, Xu L, Zhang Z, Wang P, Zhang R, He H, et al. Overexpressed miR-375-Loaded Restrains Development of Cervical Cancer Through Down-Regulation of Frizzled Class Receptor 4 (FZD4) With Liposome Nanoparticle as a Carrier. J Biomed Nanotechnol (2021) 17(9):1882–9. doi: 10.1166/jbn.2021.3145
128. Kaplan Z, Zielske SP, Ibrahim KG, Cackowski FC. Wnt and β-Catenin Signaling in the Bone Metastasis of Prostate Cancer. Life (Basel Switzerland) (2021) 11(10):1099. doi: 10.3390/life11101099
129. Baldinu P, Cossu A, Manca A, Satta MP, Sini MC, Rozzo C, et al. Identification of a Novel Candidate Gene, CASC2, in a Region of Common Allelic Loss at Chromosome 10q26 in Human Endometrial Cancer. Hum Mutat (2004) 23(4):318–26. doi: 10.1002/humu.20015
130. Baldinu P, Cossu A, Manca A, Satta MP, Sini MC, Palomba G, et al. CASC2a Gene Is Down-Regulated in Endometrial Cancer. Anticancer Res (2007) 27(1a):235–43.
131. Pei Z, Du X, Song Y, Fan L, Li F, Gao Y, et al. Down-Regulation of lncRNA CASC2 Promotes Cell Proliferation and Metastasis of Bladder Cancer by Activation of the Wnt/β-Catenin Signaling Pathway. Oncotarget (2017) 8(11):18145–53. doi: 10.18632/oncotarget.15210
132. Brosnan CA, Voinnet O. The Long and the Short of Noncoding RNAs. Curr Opin Cell Biol (2009) 21(3):416–25. doi: 10.1016/j.ceb.2009.04.001
133. Shi W, Zhang C, Ning Z, Hua Y, Li Y, Chen L, et al. Long Non-Coding RNA LINC00346 Promotes Pancreatic Cancer Growth and Gemcitabine Resistance by Sponging miR-188-3p to Derepress BRD4 Expression. J Exp Clin Cancer Res CR (2019) 38(1):60. doi: 10.1186/s13046-019-1055-9
134. Ye T, Ding W, Wang N, Huang H, Pan Y, Wei A. Long Noncoding RNA Linc00346 Promotes the Malignant Phenotypes of Bladder Cancer. Biochem Biophys Res Commun (2017) 491(1):79–84. doi: 10.1016/j.bbrc.2017.07.045
135. Liu H, Li J, Koirala P, Ding X, Chen B, Wang Y, et al. Long Non-Coding RNAs as Prognostic Markers in Human Breast Cancer. Oncotarget (2016) 7(15):20584–96. doi: 10.18632/oncotarget.7828
136. Yue C, Ren Y, Ge H, Yan L, Xu Y, Wang G, et al. Pseudogene DUXAP10 can be Used as a Diagnostic and Prognostic Biomarker in Human Cancers. J Cell Physiol (2019) 234(12):23685–94. doi: 10.1002/jcp.28937
137. Lv XY, Ma L, Chen JF, Yu R, Li Y, Yan ZJ, et al. Knockdown of DUXAP10 Inhibits Proliferation and Promotes Apoptosis in Bladder Cancer Cells via PI3K/Akt/mTOR Signaling Pathway. Int J Oncol (2018) 52(1):288–94. doi: 10.3892/ijo.2017.4195
138. Wang XF, Chen J, Gong YB, Qin YC, Wang L, Li NC. Long Non-Coding RNA DUXAP10 Promotes the Proliferation, Migration, and Inhibits Apoptosis of Prostate Cancer Cells. Eur Rev Med Pharmacol Sci (2019) 23(9):3699–708. doi: 10.26355/eurrev_201905_17793
139. Chen J, Wang XF, Qin YC, Gong YB, Wang L, Li NC. Downregulation of Long Non-Coding RNA DUXAP10 Inhibits Proliferation, Migration, and Invasion of Renal Cell Carcinoma. Eur Rev Med Pharmacol Sci (2020) 24(21):11041–51. doi: 10.26355/eurrev_202011_23589
140. Lian Y, Xu Y, Xiao C, Xia R, Gong H, Yang P, et al. The Pseudogene Derived From Long Non-Coding RNA DUXAP10 Promotes Colorectal Cancer Cell Growth Through Epigenetically Silencing of P21 and PTEN. Sci Rep (2017) 7(1):7312. doi: 10.1038/s41598-017-07954-7
141. Chao HM, Huang HX, Chang PH, Tseng KC, Miyajima A, Chern E. Y-Box Binding Protein-1 Promotes Hepatocellular Carcinoma-Initiating Cell Progression and Tumorigenesis via Wnt/β-Catenin Pathway. Oncotarget (2017) 8(2):2604–16. doi: 10.18632/oncotarget.13733
142. Hu Z, Wang P, Lin J, Zheng X, Yang F, Zhang G, et al. MicroRNA-197 Promotes Metastasis of Hepatocellular Carcinoma by Activating Wnt/β-Catenin Signaling. Cell Physiol Biochem Int J Exp Cell Physiol Biochem Pharmacol (2018) 51(1):470–86. doi: 10.1159/000495242
143. Ma HW, Xie M, Sun M, Chen TY, Jin RR, Ma TS, et al. The Pseudogene Derived Long Noncoding RNA DUXAP8 Promotes Gastric Cancer Cell Proliferation and Migration via Epigenetically Silencing PLEKHO1 Expression. Oncotarget (2017) 8(32):52211–24. doi: 10.18632/oncotarget.11075
144. Cidon EU. Systemic Treatment of Hepatocellular Carcinoma: Past, Present and Future. World J Hepatol (2017) 9(18):797–807. doi: 10.4254/wjh.v9.i18.797
145. Wei CC, Nie FQ, Jiang LL, Chen QN, Chen ZY, Chen X, et al. The Pseudogene DUXAP10 Promotes an Aggressive Phenotype Through Binding With LSD1 and Repressing LATS2 and RRAD in Non Small Cell Lung Cancer. Oncotarget (2017) 8(3):5233–46. doi: 10.18632/oncotarget.14125
146. Elyakim E, Sitbon E, Faerman A, Tabak S, Montia E, Belanis L, et al. hsa-miR-191 Is a Candidate Oncogene Target for Hepatocellular Carcinoma Therapy. Cancer Res (2010) 70(20):8077–87. doi: 10.1158/0008-5472.Can-10-1313
147. Foster JW, Dominguez-Steglich MA, Guioli S, Kwok C, Weller PA, Stevanović M, et al. Campomelic Dysplasia and Autosomal Sex Reversal Caused by Mutations in an SRY-Related Gene. Nature (1994) 372(6506):525–30. doi: 10.1038/372525a0
148. Afonja O, Raaka BM, Huang A, Das S, Zhao X, Helmer E, et al. RAR Agonists Stimulate SOX9 Gene Expression in Breast Cancer Cell Lines: Evidence for a Role in Retinoid-Mediated Growth Inhibition. Oncogene (2002) 21(51):7850–60. doi: 10.1038/sj.onc.1205985
149. Shi J, Guo J, Li X. Role of LASP-1, a Novel SOX9 Transcriptional Target, in the Progression of Lung Cancer. Int J Oncol (2018) 52(1):179–88. doi: 10.3892/ijo.2017.4201
150. Guo X, Xiong L, Sun T, Peng R, Zou L, Zhu H, et al. Expression Features of SOX9 Associate With Tumor Progression and Poor Prognosis of Hepatocellular Carcinoma. Diagn Pathol (2012) 7:44. doi: 10.1186/1746-1596-7-44
151. Chen B, Lan J, Xiao Y, Liu P, Guo D, Gu Y, et al. Long Noncoding RNA TP53TG1 Suppresses the Growth and Metastasis of Hepatocellular Carcinoma by Regulating the PRDX4/β-Catenin Pathway. Cancer Lett (2021) 513:75–89. doi: 10.1016/j.canlet.2021.04.022
152. Cheng F, Yang Z, Huang F, Yin L, Yan G, Gong G. microRNA-107 Inhibits Gastric Cancer Cell Proliferation and Metastasis by Targeting PI3K/AKT Pathway. Microb Pathog (2018) 121:110–4. doi: 10.1016/j.micpath.2018.04.060
153. Wang P, Liu X, Shao Y, Wang H, Liang C, Han B, et al. MicroRNA-107-5p Suppresses Non-Small Cell Lung Cancer by Directly Targeting Oncogene Epidermal Growth Factor Receptor. Oncotarget (2017) 8(34):57012–23. doi: 10.18632/oncotarget.18505
154. Su H, Tao T, Yang Z, Kang X, Zhang X, Kang D, et al. Circular RNA cTFRC Acts as the Sponge of MicroRNA-107 to Promote Bladder Carcinoma Progression. Mol Cancer (2019) 18(1):27. doi: 10.1186/s12943-019-0951-0
155. Lapidot T, Sirard C, Vormoor J, Murdoch B, Hoang T, Caceres-Cortes J, et al. A Cell Initiating Human Acute Myeloid Leukaemia After Transplantation Into SCID Mice. Nature (1994) 367(6464):645–8. doi: 10.1038/367645a0
156. Han J, Won M, Kim JH, Jung E, Min K, Jangili P, et al. Cancer Stem Cell-Targeted Bio-Imaging and Chemotherapeutic Perspective. Chem Soc Rev (2020) 49(22):7856–78. doi: 10.1039/d0cs00379d
157. Mandl MM, Zhang S, Ulrich M, Schmoeckel E, Mayr D, Vollmar AM, et al. Inhibition of Cdk5 Induces Cell Death of Tumor-Initiating Cells. Br J Cancer (2017) 116(7):912–22. doi: 10.1038/bjc.2017.39
158. Fusco P, Mattiuzzo E, Frasson C, Viola G, Cimetta E, Esposito MR, et al. Verteporfin Induces Apoptosis and Reduces the Stem Cell-Like Properties in Neuroblastoma Tumour-Initiating Cells Through Inhibition of the YAP/TAZ Pathway. Eur J Pharmacol (2021) 893:173829. doi: 10.1016/j.ejphar.2020.173829
159. Chen ZZ, Huang L, Wu YH, Zhai WJ, Zhu PP, Gao YF. LncSox4 Promotes the Self-Renewal of Liver Tumour-Initiating Cells Through Stat3-Mediated Sox4 Expression. Nat Commun (2016) 7:12598. doi: 10.1038/ncomms12598
160. Haraguchi N, Ishii H, Mimori K, Tanaka F, Ohkuma M, Kim HM, et al. CD13 Is a Therapeutic Target in Human Liver Cancer Stem Cells. J Clin Invest (2010) 120(9):3326–39. doi: 10.1172/jci42550
161. Yang ZF, Ho DW, Ng MN, Lau CK, Yu WC, Ngai P, et al. Significance of CD90+ Cancer Stem Cells in Human Liver Cancer. Cancer Cell (2008) 13(2):153–66. doi: 10.1016/j.ccr.2008.01.013
162. Takebe N, Miele L, Harris PJ, Jeong W, Bando H, Kahn M, et al. Targeting Notch, Hedgehog, and Wnt Pathways in Cancer Stem Cells: Clinical Update. Nat Rev Clin Oncol (2015) 12(8):445–64. doi: 10.1038/nrclinonc.2015.61
163. Cui L, Guan Y, Qu Z, Zhang J, Liao B, Ma B, et al. WNT Signaling Determines Tumorigenicity and Function of ESC-Derived Retinal Progenitors. J Clin Invest (2013) 123(4):1647–61. doi: 10.1172/jci65048
164. Weber BN, Chi AW, Chavez A, Yashiro-Ohtani Y, Yang Q, Shestova O, et al. A Critical Role for TCF-1 in T-Lineage Specification and Differentiation. Nature (2011) 476(7358):63–8. doi: 10.1038/nature10279
165. Marchesi I, Bagella L. Targeting Enhancer of Zeste Homolog 2 as a Promising Strategy for Cancer Treatment. World J Clin Oncol (2016) 7(2):135–48. doi: 10.5306/wjco.v7.i2.135
166. Cai MY, Tong ZT, Zheng F, Liao YJ, Wang Y, Rao HL, et al. EZH2 Protein: A Promising Immunomarker for the Detection of Hepatocellular Carcinomas in Liver Needle Biopsies. Gut (2011) 60(7):967–76. doi: 10.1136/gut.2010.231993
167. Song H, Yu Z, Sun X, Feng J, Yu Q, Khan H, et al. Androgen Receptor Drives Hepatocellular Carcinogenesis by Activating Enhancer of Zeste Homolog 2-Mediated Wnt/β-Catenin Signaling. EBioMedicine (2018) 35:155–66. doi: 10.1016/j.ebiom.2018.08.043
168. Cheng AS, Lau SS, Chen Y, Kondo Y, Li MS, Feng H, et al. EZH2-Mediated Concordant Repression of Wnt Antagonists Promotes β-Catenin-Dependent Hepatocarcinogenesis. Cancer Res (2011) 71(11):4028–39. doi: 10.1158/0008-5472.Can-10-3342
169. Zhang DM, Lin ZY, Yang ZH, Wang YY, Wan D, Zhong JL, et al. IncRNA H19 Promotes Tongue Squamous Cell Carcinoma Progression Through β-Catenin/GSK3β/EMT Signaling via Association With EZH2. Am J Trans Res (2017) 9(7):3474–86.
170. Ma J, Li T, Han X, Yuan H. Knockdown of LncRNA ANRIL Suppresses Cell Proliferation, Metastasis, and Invasion via Regulating miR-122-5p Expression in Hepatocellular Carcinoma. J Cancer Res Clin Oncol (2018) 144(2):205–14. doi: 10.1007/s00432-017-2543-y
171. He Y, Cui Y, Wang W, Gu J, Guo S, Ma K, et al. Hypomethylation of the hsa-miR-191 Locus Causes High Expression of Hsa-Mir-191 and Promotes the Epithelial-to-Mesenchymal Transition in Hepatocellular Carcinoma. Neoplasia (New York NY) (2011) 13(9):841–53. doi: 10.1593/neo.11698
172. Berger H, Wodarz A, Borchers A. PTK7 Faces the Wnt in Development and Disease. Front Cell Dev Biol (2017) 5:31. doi: 10.3389/fcell.2017.00031
173. Song Y, Washington MK, Crawford HC. Loss of FOXA1/2 Is Essential for the Epithelial-to-Mesenchymal Transition in Pancreatic Cancer. Cancer Res (2010) 70(5):2115–25. doi: 10.1158/0008-5472.Can-09-2979
174. Tsuchiya N, Sawada Y, Endo I, Saito K, Uemura Y, Nakatsura T. Biomarkers for the Early Diagnosis of Hepatocellular Carcinoma. World J Gastroenterol (2015) 21(37):10573–83. doi: 10.3748/wjg.v21.i37.10573
175. Luo P, Wu S, Yu Y, Ming X, Li S, Zuo X, et al. Current Status and Perspective Biomarkers in AFP Negative HCC: Towards Screening for and Diagnosing Hepatocellular Carcinoma at an Earlier Stage. Pathol Oncol Res POR (2020) 26(2):599–603. doi: 10.1007/s12253-019-00585-5
176. Trevisan França de Lima L, Broszczak D, Zhang X, Bridle K, Crawford D, Punyadeera C. The Use of Minimally Invasive Biomarkers for the Diagnosis and Prognosis of Hepatocellular Carcinoma. Biochim Biophys Acta Rev Cancer (2020) 1874(2):188451. doi: 10.1016/j.bbcan.2020.188451
177. Damas ND, Marcatti M, Côme C, Christensen LL, Nielsen MM, Baumgartner R, et al. SNHG5 Promotes Colorectal Cancer Cell Survival by Counteracting STAU1-Mediated mRNA Destabilization. Nat Commun (2016) 7:13875. doi: 10.1038/ncomms13875
178. Pérez-Herrero E, Fernández-Medarde A. Advanced Targeted Therapies in Cancer: Drug Nanocarriers, the Future of Chemotherapy. Eur J Pharm Biopharm Off J Arbeitsgemeinschaft fur Pharmazeutische Verfahrenstechnik Evol (2015) 93:52–79. doi: 10.1016/j.ejpb.2015.03.018
179. van den Bulk J, Verdegaal EM, de Miranda NF. Cancer Immunotherapy: Broadening the Scope of Targetable Tumours. Open Biol (2018) 8(6):180037. doi: 10.1098/rsob.180037
Keywords: hepatocellular carcinoma, long noncoding RNA, Wnt pathway, biomarker, prognosis
Citation: Xu Y, Yu X, Sun Z, He Y and Guo W (2022) Roles of lncRNAs Mediating Wnt/β-Catenin Signaling in HCC. Front. Oncol. 12:831366. doi: 10.3389/fonc.2022.831366
Received: 11 December 2021; Accepted: 14 February 2022;
Published: 09 March 2022.
Edited by:
Rongxin Zhang, Guangdong Pharmaceutical University, ChinaReviewed by:
Jaques Waisberg, Faculdade de Medicina do ABC, BrazilCopyright © 2022 Xu, Yu, Sun, He and Guo. This is an open-access article distributed under the terms of the Creative Commons Attribution License (CC BY). The use, distribution or reproduction in other forums is permitted, provided the original author(s) and the copyright owner(s) are credited and that the original publication in this journal is cited, in accordance with accepted academic practice. No use, distribution or reproduction is permitted which does not comply with these terms.
*Correspondence: Yuting He, ZmNjaGV5dDFAenp1LmVkdS5jbg==; Wenzhi Guo, ZmNjZ3Vvd3pAenp1LmVkdS5jbg==
Disclaimer: All claims expressed in this article are solely those of the authors and do not necessarily represent those of their affiliated organizations, or those of the publisher, the editors and the reviewers. Any product that may be evaluated in this article or claim that may be made by its manufacturer is not guaranteed or endorsed by the publisher.
Research integrity at Frontiers
Learn more about the work of our research integrity team to safeguard the quality of each article we publish.