- 1Department of Medical Genetics, School of Medicine, Shahid Beheshti Universality of Medical Sciences, Tehran, Iran
- 2Department of Pharmacognosy, College of Pharmacy, Hawler Medical University, Erbil, Iraq
- 3School of Advancement, Centennial College, Toronto, ON, Canada
- 4The Ottawa Hospital Research Institute, University of Ottawa, Ottawa, ON, Canada
- 5Department of Anatomical Sciences, Faculty of Medicine, Birjand University of Medical Sciences, Birjand, Iran
- 6Institute of Human Genetics, Jena University Hospital, Jena, Germany
- 7Urology and Nephrology Research Center, Shahid Beheshti Universality of Medical Sciences, Tehran, Iran
- 8Skull Base Research Center, Loghman Hakim Hospital, Shahid Beheshti Universality of Medical Sciences, Tehran, Iran
Programmed cell death (PDCD) family of proteins includes at least 12 members, function of seven of them being more investigated. These members are PDCD1, PDCD2, PDCD4, PDCD5, PDCD6, PDCD7 and PDCD10. Consistent with the important roles of these proteins in the regulation of apoptosis, dysregulation of PDCDs is associated with diverse disorders ranging from intervertebral disc degeneration, amyotrophic lateral sclerosis, immune thrombocytopenia, type 1 diabetes, congenital hypothyroidism, Alzheimer’s disease to different types of cancers. More recently, the interaction between non-coding RNAs and different members of PDCD family is being discovered. In the current study, we described the functional interactions between PDCDs and two classes of non-coding RNAs, namely microRNAs (miRNAs) and long non-coding RNAs (lncRNAs). miR-21 and miR-183 are two miRNAs whose interactions with PDCDs have been assessed in different contexts. The lncRNAs interaction with PDCDs is mainly assessed in the context of neoplasia indicating the role of MALAT1, MEG3, SNHG14 and LINC00473 in this process.
1 Introduction
Programmed cell death (PDCD) has been observed as an important phenomenon during insect development about seven decades ago (1). Afterwards, several equivalent cell death phenomena have been recognized in vertebrates including human and this process has been retitled as apoptosis (2). This cellular process in contributes in eradication of foreign bodies and abnormal cells. Moreover, it has essential roles in the development of organisms, the homeostasis of the internal milieu and organ development. Abnormal regulation of apoptosis is strictly associated with immune disorders, developmental abnormalities, and cancers (3). Apoptosis is regulated by two types of proteins with one of them inhibiting cell death and the other group initiating this process. Genes regulating apoptosis are highly conserved between various species and genera (4). Different studies have shown wide expression of PDCD gene family members in normal adult tissues (4).
A comprehensive analysis of several PDCD gene family members in vertebrates and assessment of their sequences as well as alignment steps and 3D structure analyses has revealed no similarity in the structural domains between the PDCD family genes. In fact, PDCD4 family genes have no structural similarity but contain some conserved amino acid sequences (or so call motifs). Lamprey PDCD genes have been found to be highly homologous with the corresponding human PDCD genes. Figure 1 shows the conserved motifs between lamprey and human PDCD genes.
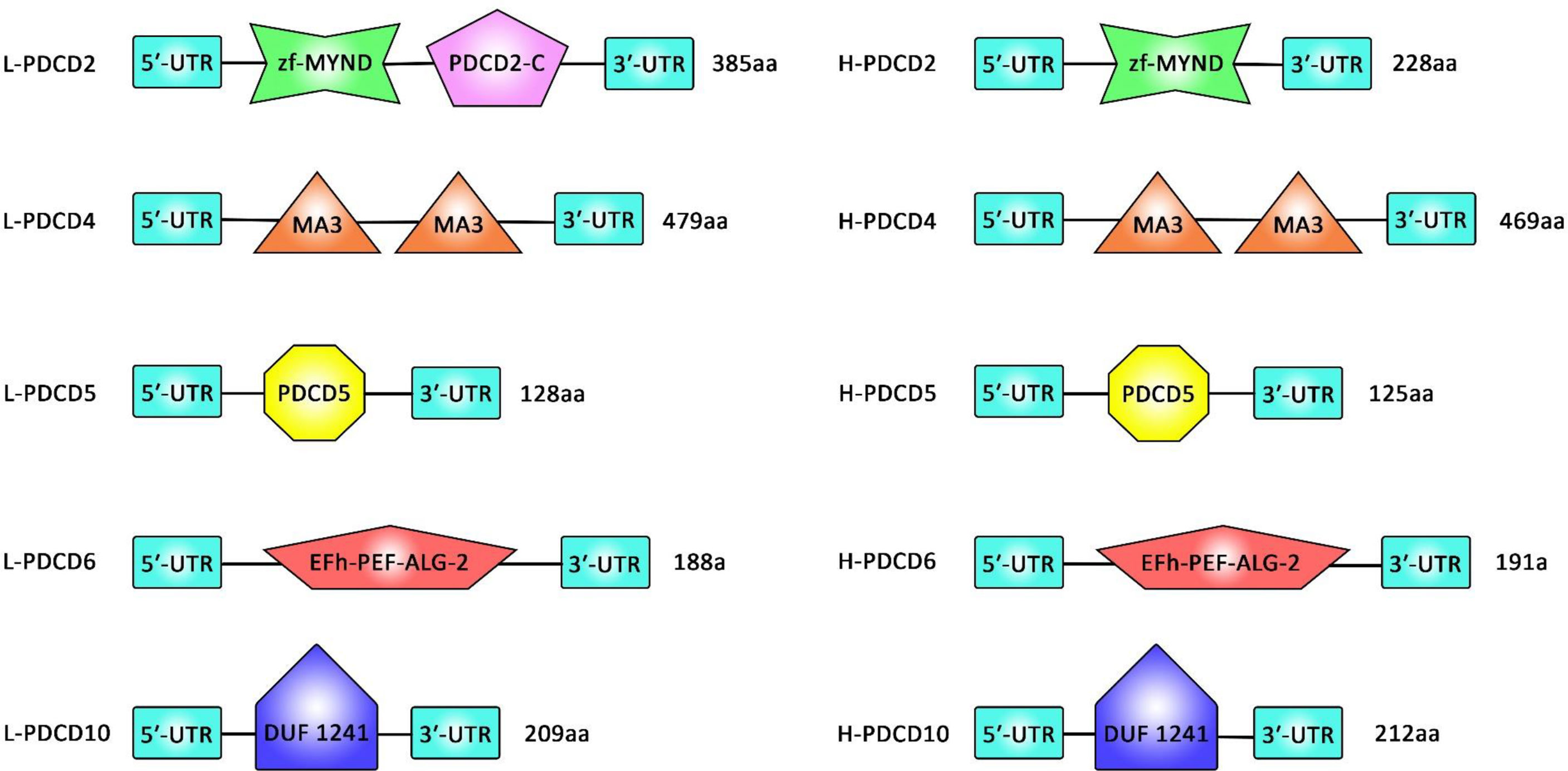
Figure 1 A comparison between the sequence of the domains of PDCD family genes (PDCD2, PDCD4, PDCD5, PDCD6, and PDCD10) in Lamprey and human.
Assessment of motifs in the PDCD proteins has resulted in identification of 16 distinct motifs in these proteins. All lamprey PDCD proteins have been shown to contain motif 13. PDCD2 proteins have been found to contain motifs 7, 9, 13, 15, and 16. Besides, motifs 4, 8, 10, 11, 12, 13, and 14 have been present in PDCD4 proteins. Finally, motifs 1, 2, and 13 have been detected in PDCD5 proteins. This bioinformatics analysis has indicated that individual motifs might contribute in the biological activity of PDCD proteins. Phylogenetic studies have also verified the conserved evolution of each PDCD gene in vertebrates (4).
PDCD1 has an important role in the regulation of the immune system responses and induction of self-tolerance through inhibition of activity of T cells (5). PDCD2 participates in the development of embryo and differentiation of stem cells (6, 7). PDCD4 participates in tumor evolution, cancer progression and metastatic processes (8, 9). In fact, PDCD4 participates in the regulation of transcription, translation, apoptototic pathways, and regulation of various signal transduction pathways (10). Expression of PDCD5 has been found to be increased in TF-1 cells undertaking apoptosis (11). PDCD6 has been shown to be involved in cell proliferation and death (12). PDCD7 participates in apoptosis induced by glucocorticoids and staphylococci (13). PDCD10 has diverse roles in proteins synthesis, apoptotic pathways, cell proliferation, and induction of tumors (14, 15).
Moreover, expression levels of PDCD genes have been demonstrated to be altered in tumor samples and cancer cell lines (16, 17). At present, there is no evidence demonstrating a conclusive link between members of PDCD gene family (4). Yet, assessment of transcriptomic data has shown remarkable alterations in expression of the PDCD gene family following treatment with certain drugs (18). Although 12 members of this family have been identified in the human genome, seven main genes, namely PDCD1 (alternatively named as PD-1), PDCD2, PDCD4, PDCD5, PDCD6, PDCD7 and PDCD10 are in the focus of mechanistical investigations (4). PD-1 has two ligands, namely PD-L1 and PD-L2 which are present on the surface of dendritic cells or macrophages (19).
More recently, the interaction between non-coding RNAs and different members of PDCD family is being discovered. In the current study, we described the functional interactions between PDCDs and two classes of non-coding RNAs, namely microRNAs (miRNAs) and long non-coding RNAs (lncRNAs). miRNAs are a group of small-sized non-coding RNAs that regulate gene expression at post-transcriptional level through base-pairing with mRNAs and inducing mRNA degradation or suppressing mRNA translation. LncRNAs can regulate gene expression at different levels through epigenetic mechanisms, modification of RNA stability and interaction with several types of biomolecules.
2 miRNAs and PDCD
miRNAs are transcripts with sizes about 22 nucleotides that regulate gene expression through binding with different regions of mRNAs, particularly their 3’ UTR (20). In addition to transcript degradation and translational suppression, miRNAs have been found to induce translation or modulate transcription (20). Most studies have shown that miRNAs regulate PDCD expression through binding with 3’ UTR of PDCD transcripts. This kind of interaction has been predicted by bioinformatics approaches and verified through luciferase assay. Binding of miRNAs with this region of PDCD transcript leads to down-regulation of expression of PDCDs. The degree of miRNA response elements complementarity defines whether the target mRNA is subjected to AGO2-dependent slicing or its translation is inhibited by miRNA-induced silencing complex and target mRNA decay (20). It has also been shown that in some situations, miRNAs may activate translation of target mRNAs or regulate transcription (20).
2.1 Interaction Between miRNAs and PDCD in Non-Neoplastic Disorders
2.1.1 Heart Diseases
Suppression of PDCD4 by miR-21 has been shown to contribute to the induction of fibroblastoid features during cardiac injury leading to cardiac fibrosis, since contributing in the pathogenesis of fibrogenic cardiac injury. In fact, pro-fibrogenic incitements, particularly TGF-β, can promote progression of epithelial-mesenchymal transition (EMT) in epicardial mesothelial cells, resulting in alterations in miRNAs signature, specifically expression of the pleiotropic miR-21. Ectopic expression of this miRNA has noticeably stimulated the fibroblast-like features causing by fibrogenic EMT, while miR-21 antagonism has suppressed this effect (21). Another study has shown up-regulation of miR-21 in macrophages in response to elevation of glucose levels. Elevation of glucose levels has been shown to stimulate apoptosis of macrophages. Concurrent inhibition of miR-21 and elevation of glucose concentrations results in enhancement of cell apoptosis (citation). Taken together, miR-21 levels have been changed by high glucose concentrations in macrophages, exerting a protective impact against glucose-induced macrophage apoptosis through suppression of PDCD4 expression (22). miR-208a-3p is another miRNA that could suppress expression of PDCD4, thus affecting autophagy in rat cardiomyoblasts (23). Meanwhile, the inhibitory effects of miR-499-5p on PDCD4 have been shown to decrease cardiomyocytes apoptosis and reduce myocardial infarct size (24). Finally, miR-613 through targeting PDCD10 could suppresses ischemia/reperfusion-induced cardiomyocyte apoptosis via regulating the PI3K/AKT signaling pathway (25). Table 1 shows the interaction between miRNAs and PDCD in heart diseases.
2.1.2 Polycystic Ovary Syndrome (PCOS)
Polycystic ovary syndrome (PCOS) is the utmost frequent female endocrine disease (26) being characterized by the presence of ovarian cysts, chronic anovulation, and clinical or biological signs of hyperandrogenism. People with PCOS may experience irregular menstrual periods, heavy periods, excess hair, acne, pelvic pain, difficulty getting pregnant, and patches of thick, darker, velvety skin (3) The primary characteristics of this syndrome include: hyperandrogenism, anovulation, insulin resistance, and neuroendocrine disruption Expression of miR-16 expression has been shown to be decreased in ovarian cortex tissues and serums of PCOS patients, parallel with up-regulation of PDCD4. Mechanistically, miR-16 enhances cell proliferation, facilitates cell cycle progression, and suppresses apoptosis in granulosa cells through inhibiting expression of PDCD4. Forced over-expression of PDCD4 has stopped the impact of miR-16 on growth and apoptosis of granulosa cells. Moreover, testosterone could reduce expression level of miR-16 and enhance PDCD4 levels, therefore hindering cell growth and enhancing apoptosis of granulosa cells (27). Another study in steroidogenic human ovarian granulose-like tumor cell line has shown that upregulation of miR-155 by targeting PDCD4 and regulating PI3K/AKT and JNK pathways could enhance proliferation, migration, and invasion of cells. Since miR-155 has been found to be up-regulated in PCOS samples, miR-155/PCDC4 axis might be involved in the pathogenesis of PCOS (28). Table 2 shows the interaction between miRNAs and PDCD in PCOS.
2.1.3 Other Non-Neoplastic Disorders
The impact of miRNAs on expression of PDCD is also involved in a variety of other non-neoplastic disorders. For instance, in the context of intervertebral disc degeneration, up-regulation of miR-21 could promote proliferation of human degenerated nucleus pulposus cells through regulating PDCD4 expression, enhancing phosphorylation of c-Jun protein, and activating AP-1-dependent transcription of MMP-2/9 (29). miR-183-5p, a highly expressed miRNA in neurons has been shown to inhibit expression of PDCD4. Neuronal expression of this miRNA is instantly increased in response to treatment with hydrogen peroxide, tunicamycin or TNF-α. Its up-regulation enhances survival of neurons under stress situations, while its silencing leads to death of neurons. In fact, miR-183-5p synchronizes apoptosis and necroptosis mechanisms through its direct interactions with PDCD4 and RIPK3. This function shields neurons against cell death under stress situations. Consistently, expression of miR-183-5p has been found to be reduced in amyotrophic lateral sclerosis patients and animal models enhances supporting the role of this miRNA in regulation of motor neuron survival (30). Another set of in vivo and in vitro experiments has shown that down-regulation of miR-21 could increase fibroblast apoptosis and prevent knee scar adhesion though influencing PDCD4 expression. In fact, miR-21 attenuates the impact of mitomycin on decreasing the number of fibroblasts through down-regulating PDCD4 levels (31). miR-16 is another miRNA that targets PDCD4. Through this route, miR-16 can inhibit activation of inflammatory macrophages during the atherosclerotic process through the MAPK and NF-κB pathways (32). In the context of steroid-induced avascular necrosis of femor, miR−206 by targeting PDCD4 could reduce cell viability and proliferation, and enhance apoptosis (33).
hsa-miR-424-5p has been found to bind with PD-1 stimulating immune responses through the mTORC signal transduction, thus participating in the pathoetiology of type I diabetes (34). Similarly, up-regulation of miR-28 by targeting PD-1 and regulating cytokine secretion could modulate exhaustive differentiation of T cells (35). Table 3 shows interaction between miRNAs and PDCD in diverse non-neoplastic disorders. Figure 2 demonstrates that aberrant expression of PDCD-interacting ncRNAs could play an effective role in causing several non-neoplastic disorders.
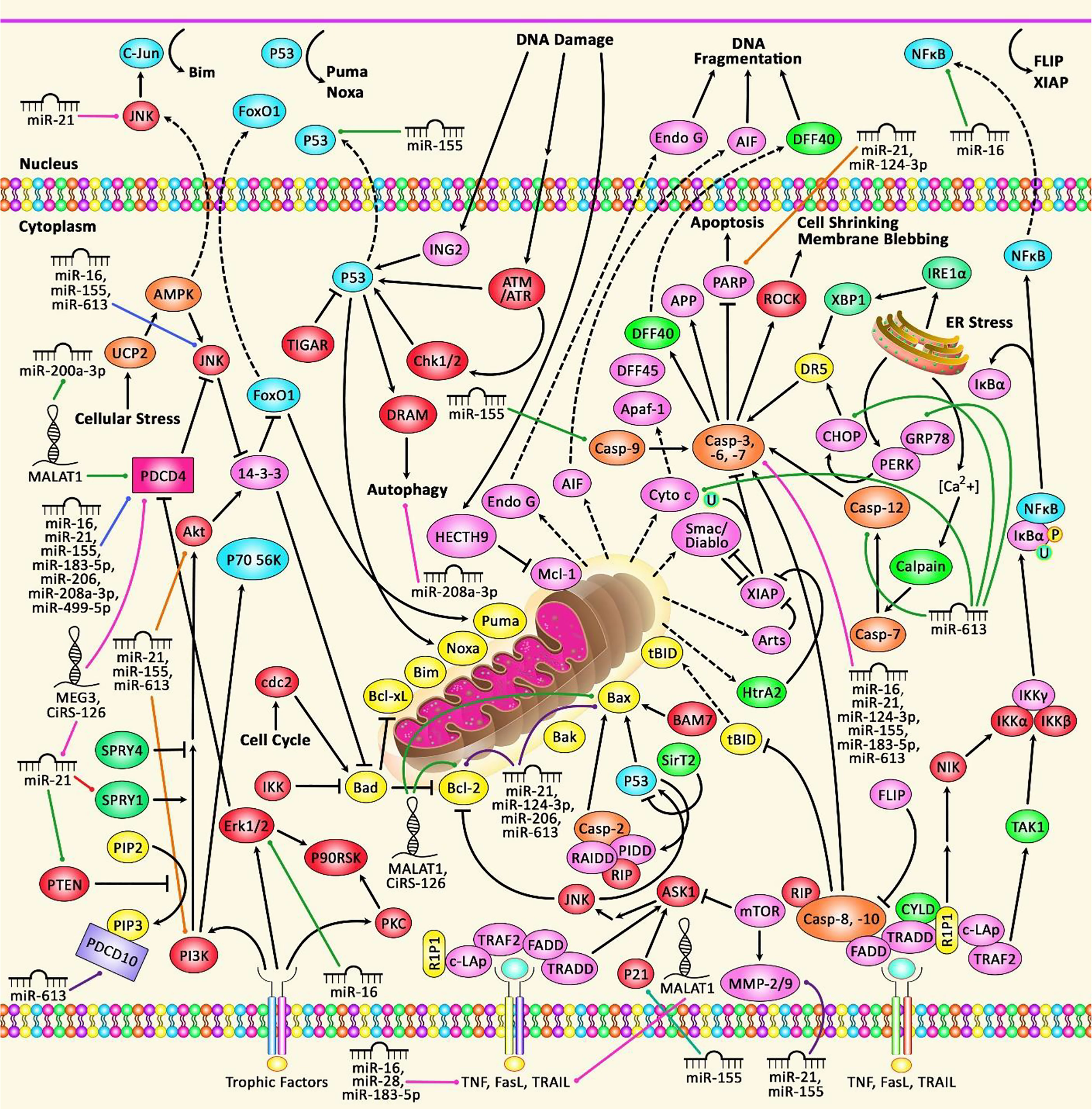
Figure 2 A schematic diagram of the functional interactions between PDCDs proteins and various ncRNAs in non-neoplastic disorders. Apoptosis, a gene-controlled process of programmed cell death (PCD), is a cascade which is induced in normal cells under physiological conditions and pathological stress. Dysregulation of apoptosis can result in uncontrolled cell proliferation that can play a significant role in causing various non-neoplastic disorders including heart diseases, polycystic ovary syndrome, and several immune disorders. The death receptors include Fas receptors, TNF receptors, and TRAIL receptors. As a surface receptor, TNF-R1 can interact with TNF to induce the recruitment of adaptor proteins FADD and TRADD, which can recruit a series of downstream factors, such as Caspase-8, that is a key modulator of the extrinsic cascade, eventually leading to cell apoptosis. In the intrinsic cascade, the functional outcome of the pro-apoptotic pathway is mitochondrial membrane perturbation and release of cytochrome c in the cytoplasm, where it can create a complex with APAF1 and the inactive form of caspase-9. In order to trigger the activation of caspase-9, this complex hydrolyzes adenosine triphosphate. Consequently, the initiator caspase-9 can cleave and upregulate the executioner caspases-3/6/7, leading to cell apoptosis (42, 43). Growing evidence suggests that dysregulation of PDCDs is associated with several non-neoplastic disorders and importantly their interaction with different ncRNAs (miRNAs and lncRNAs) is also detected.
2.2 Interaction Between miRNAs and PDCD in Neoplastic Disorders
2.2.1 Cervical, Ovarian and Breast Cancers
A number of dysregulated miRNAs in female cancers have been shown to exert regulatory roles on PDCD genes. For instance, the oncogenic miRNAs miR-150 (44) and miR-21 (45) have been shown to inhibit expression of PDCD4, thus enhancing cell proliferation and malignant behaviors of cervical cancer cells. miR-21 has similar role in ovarian (46) and breast cancers (47). miR-421/PDCD4, miR-27a-3p/PD-L1, miR-424/PD-L1, miR-26a-5p/PDCD10 and miR-26b-5p/PDCD10 are other routes of participation of miRNAs in the pathogenesis of breast cancer (Table 4).
2.2.2 Gastrointestinal Cancers
Two independent studies in esophageal squamous cell carcinoma have shown up-regulation of the PDCD4-interacting miRNA miR-183 in this type of cancer (54, 55). Up-regulation of this miRNA has resulted in down-regulation of PDCD4 expression, thus promoting proliferation and invasion of esophageal squamous cell carcinoma cells. Suppression of the PI3K/Akt signaling by LY294002 could increase PDCD4 expression and decrease miR-183 level in these cells (54). In this type of cancer, the inhibitory role of miR-21 on PDCD4 has been verified both in vitro and in vivo (56).
In gastric cancer, miR-21 has been found to affect carcinogenesis through interacting with PDCD4 (57) as well as PD-1/PD-L1 pathway (58). miR-940, miR-208a-3p, miR-23a/b, miR-129-1-3p, miR−46146, miR-503, miR-181b and miR-148a-3p are other miRNAs that participate in the pathogenesis of gastrointestinal cancers via regulation of expression of PDCD genes (Table 5).
2.2.3 Head and Neck Cancers
In head and neck squamous cell carcinoma, miR-21 has been reported to increase cell proliferation through decreasing expression of PDCD4 (74). Another study in this type of cancer has revealed that miR-375 inhibits IFN-γ-associated surface expression of PD-L1. Moreover, JAK2 has been identified as a valid target of miR-375. In fact, the suppressive effects of miR-375 on PD-L1 have been found to depend on the JAK2/STAT1 pathway. Taken together, through attenuation of PD-1/PD-L1 axis, miR-375 can enhance cellular immune response against tumor cells (75).
In oral squamous cell carcinoma cells, miR-21 could enhance tumor cell invasion through targeting PDCD4 (76). This miRNA has a similar effect in the carcinogenic process in salivary adenoid cystic carcinoma (77). In oropharyngeal cancer cells, the primary inhibition of PDCD4 is facilitated by miR-21 while continuous inhibition of its expression is facilitated by miR-499. Besides, the single miR-21 site could provoke the same degree of expression inhibition as the three miR-499 sites (32).
In tongue cancer, miR-155 has been found to target Pdcd4 transcript and inhibit its expression. Forced up-regulation of Pdcd4 or miR-155 silencing in these neoplastic cancer cells could reduce AP-1-associated transcription of the BIC promoter and reduces expression of miR-155. In fact, expression of miR-155 is regulated by a feedback circuit between Pdcd4, AP-1, and miR-155. Up-regulation of miR-155 results in progression of this type of cancer (78). Table 6 shows the interaction between miRNAs and PDCD in head and neck cancers.
2.2.4 Lung Cancer
The inhibitory role of miR-182 on PDCD4 is involved in the modulation of sensitivity of lung cancer cells to cisplatin (81). Moreover, resistance to this chemotherapeutic agent has been shown to be reversed through suppression of miR-141 (82). Similarly, miR-21 silencing could inhibit proliferation and migration of lung cancer cells via changing expression of PDCD4 (83). miR-182 silencing has inhibited growth and invasive properties of lung cancer cells through modulation of PDCD4 levels (55). On the other hand, miR-103 has been shown to exert its tumor suppressive roles in lung cancer through targeting PDCD10 (84). Table 7 shows the interaction between miRNAs and PDCD in lung cancer.
2.2.5 Nervous System Cancers
miR-21 has been shown to affect the carcinogenesis of malignant peripheral nerve sheath tumors (85), glioblastoma (86) and neuroblastoma (87) through modulation of PDCD4. Moreover, over-expression the PDCD4-targeting miR-96 has been shown to promote resistance of glioblastoma cells to radiotherapy (88). Table 8 shows the interaction between miRNAs and PDCD nervous system cancers.
2.2.6 Other Malignancies
The interaction between miRNAs and PDCD has been verified in other malignancies such as hematological malignancies, bladder and kidney cancers as well as melanoma. miR-21 has been the most assessed miRNA in this regard (Table 9). Figure 3 represents the role of several ncRNAs in several human cancers via interacting with PDCD genes.
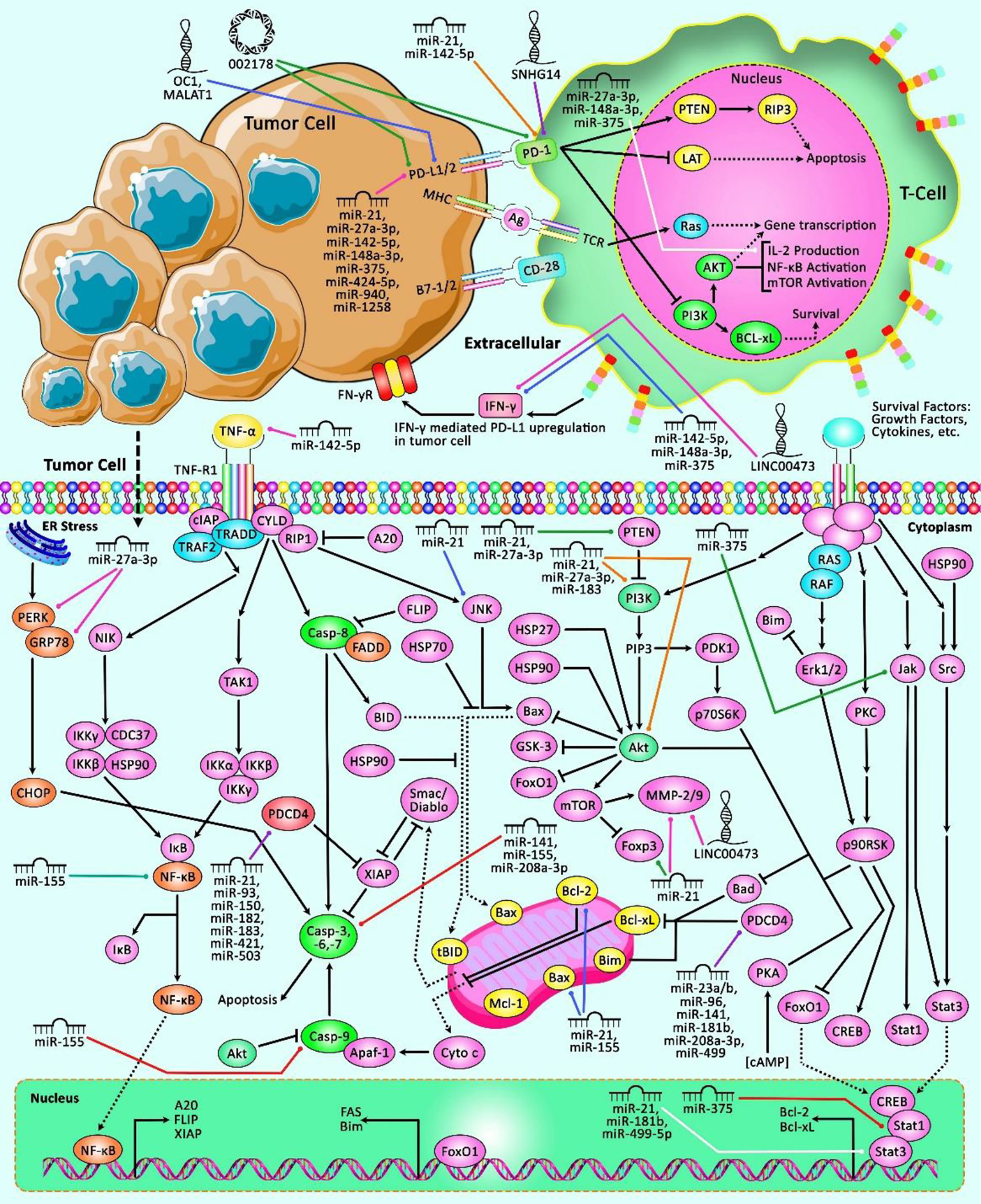
Figure 3 A schematic representation of the role of ncRNAs in modulating the PDCD genes in multiple human cancers. The loss of apoptotic control could cause tumor cells to survive longer and give more time for the accumulation of mutations that could, in turn, enhance invasiveness during tumor cell progression, stimulate angiogenesis, deregulate cell proliferation and interfere with differentiation (95). Apoptosis could be mediated via elevation or suppression of caspases. Besides, the PD-1/PD-L1 interaction could suppress T lymphocyte proliferation, survival and effector functions including cytotoxicity and cytokine release, and thereby could trigger apoptosis of tumor-specific T cells (96, 97). Previous studies have authenticated that several ncRNAs could have a crucial role in human cancers via interacting with various PDCD transcripts. In fact, aberrant expression of these ncRNAs could result in dysregulation of PDCDs, and therefore is associated with different kinds of cancer.
2.3 Association Between PDCD-Interacting miRNAs and Cancer Prognosis
Among PDCD-related miRNAs, overexpression of miR-183-5p, miR-21 and miR-93 has been correlated with shorter overall survival of patients with different types of neoplasms. However, miR-26a-5p, miR-103 and miR-183 have been revealed to have opposite effect (Table 10).
3 LncRNAs and PDCD
LncRNAs are transcripts with a wide range of length, i.e. 200 to thousands of nucleotides. They exert regulatory roles in different phases of gene expression through diverse action modalities (98). Limited numbers of lncRNAs have been found to affect expression of PDCDs. In fact, lncRNAs that participate in the regulation of PDCDs mostly affect expression of miRNAs and through this route they exert their function. For instance, MALAT1 has been shown to affect apoptosis of regulates cardiomyocytes through serving as a sponge for miR-200a-3p, a miRNA that inhibits PDCD4 expression. This function of MALAT1 has impacts in the cardiac injury produced by hypoxia/reperfusion in the course of myocardial infarction (98). Meanwhile, this lncRNA has been shown to affect progression of lung cancer through miR-200a-3p/PD-L1 axis (99). The known miR-21/PDCD4 axis has been shown to be controlled by two lncRNAs, namely MEG3 (90) and CiRS-126 (100), in the contexts of ischemic neuron death and PCOS, respectively.
The impact of Lnc-OC1 on PDCD-related pathways has been verified in endometrial cancer, where suppression of this lncRNA has been shown to stimulate apoptosis and decreased viability of neoplastic cells through influencing miR-34a/PD-L1 axis (69). Moreover, the lncRNA SNHG14 has been found to serve as a sponge for miR-5590-3p and enhance expression of ZEB1 to promote progression of certain type of B cell malignancy and facilitate evasion of malignant cell from immune responses through modulation of PD-1/PD-L1 checkpoint (101). Expression of this axis has also been shown to be regulated by a circular RNA, namely circRNA-002178 in lung cancer (69). Finally, LINC00473 can facilitate pathogenic course of pancreatic cancer through sponging miR-195-5p and enhancing expression of PD-L1 (102). In colorectal cancer cells, Linc00472 has been found to act as a sponge for miR-196a to release PDCD4. Besides, up-regulation of miR-196a or down-regulation of PDCD4 could reverse Linc00472-mediated suppression of cell proliferation and activation of apoptosis in these cells. Forced over-expression of Linc00472 could hinder tumor growth in animal models. Taken together, Linc00472 could suppress proliferation and induce apoptosis via enhancement of PDCD4 expression by decoying miR-196a (103). Table 11 shows the interaction between lncRNAs and PDCD in different conditions.
4 Discussion
As important regulators of apoptosis, PDCDs participate in normal development as well as several pathological conditions. Expression and function of PDCDs are under controls of several non-coding RNAs, especially miRNAs. In fact, miRNAs can affect pathoetiology of human disorders through suppression of expression of PDCDs. This type of interaction has been assessed in the context of cardiac disorders, PCOS, intervertebral disc degeneration, amyotrophic lateral sclerosis, immune thrombocytopenia, type 1 diabetes, congenital hypothyroidism, Alzheimer’s disease as well as different types of cancers. PCDC4 is the top member of this family in terms of interaction with miRNAs. In fact, miR-21, miR-208a-3p, miR-499-5p, miR-16, miR-155, miR-182, miR-141, miR-96, miR-182, miR-499, miR-503 and miR-183 have been verified as modulators of expression of PCDC4 in different tissues. Particularly, dysregulation of miR-21/PDCD4 axis has been identified as an important pathway in many diseases.
Although the roles of several PDCD-related miRNAs have been appraised in development of human cancers, data regarding their impact on patients’ survival is limited. In fact, miR-183-5p, miR-21, miR-93, miR-26a-5p, miR-103 and miR-183 are the only PDCD-related miRNAs whose association with cancer prognosis has been verified.
Since PDCDs affect an important hallmark of carcinogenesis, i.e. decreased response of cancer cells to pro-apoptotic stimuli, PDCD-related miRNAs represent an ideal group of therapeutic targets for cancers. Knock-down experiments in cell lines and animal models have revealed promising results, since down-regulation of PDCD-targeting miRNAs has substantially reduced viability of cancer cells and enhanced the response to apoptosis. These miRNAs have also been found to affect radio/chemoresistance of cancer cells, representing a novel avenue for enhancement of effectiveness of routine anti-cancer therapies.
The impact of lncRNAs on modulation of expression of PDCDs has been less studied. In fact, lncRNAs that affect expression of these proteins mainly act as molecular sponges for miRNAs. MALAT1/miR-200a-3p/PDCD4, ciRS-126/miR-21/PDCD4 and SNHG14/PD-1-miR-5590 are among lncRNA/miRNA/PDCD axes which contribute in the pathoetiology of human disorders. Thus, lncRNAs mainly affect expression of PDCD-interacting miRNAs and through this route they regulate expression of PDCDs.
5 Future Perspectives
Understanding the important roles of ncRNAs in regulation of function of PDCDs is expected to lead to design of specific treatments targeting these proteins via modulation of expression of ncRNAs. The role of PDCD5 and 7 in conjunction with ncRNAs is still unclear. These kinds of treatments can be theoretically used in a wide range of human disorders, including malignancies and disorders related with cell senescence or apoptosis. Considering the orchestrated effects of several miRNAs and lncRNAs on expressions of these proteins, targeting multiples points in this interaction network seems to be an efficient way for modulation of expressions of PDCDs.
Author Contributions
SG-F wrote the draft and revised it. MT designed and supervised the study. HS, BH, MM, and AB collected the data and designed the figures and tables. All the authors read the draft and approved the submission version.
Conflict of Interest
The authors declare that the research was conducted in the absence of any commercial or financial relationships that could be construed as a potential conflict of interest.
Publisher’s Note
All claims expressed in this article are solely those of the authors and do not necessarily represent those of their affiliated organizations, or those of the publisher, the editors and the reviewers. Any product that may be evaluated in this article, or claim that may be made by its manufacturer, is not guaranteed or endorsed by the publisher.
References
1. Lockshin RA, Williams CM. Programmed Cell Death–I. Cytology of Degeneration in the Intersegmental Muscles of the Pernyi Silkmoth. J Insect Physiol (1965) 11:123–33. doi: 10.1016/0022-1910(65)90099-5
2. Fadeel B, Orrenius S. Apoptosis: A Basic Biological Phenomenon With Wide-Ranging Implications in Human Disease. J Internal Med (2005) 258(6):479–517. doi: 10.1111/j.1365-2796.2005.01570.x
3. Cookson BT, Brennan MA. Pro-Inflammatory Programmed Cell Death. Trends Microbiol (2001) 9(3):113–4. doi: 10.1016/S0966-842X(00)01936-3
4. Guan X, Lu J, Sun F, Li Q, Pang Y. The Molecular Evolution and Functional Divergence of Lamprey Programmed Cell Death Genes. Front Immunol (2019) 10:1382. doi: 10.3389/fimmu.2019.01382
5. Syn NL, Teng MW, Mok TS, Soo RA. De-Novo and Acquired Resistance to Immune Checkpoint Targeting. Lancet Oncol (2017) 18(12):e731–41. doi: 10.1016/S1470-2045(17)30607-1
6. Kawakami T, Furukawa Y, Sudo K, Saito H, Takami S, Takahashi E, et al. Isolation and Mapping of a Human Gene (PDCD2) That Is Highly Homologous to Rp8, a Rat Gene Associated With Programmed Cell Death. Cytogenetic Genome Res (1995) 71(1):41–3. doi: 10.1159/000134058
7. Mu W, Munroe RJ, Barker AK, Schimenti JC. PDCD2 Is Essential for Inner Cell Mass Development and Embryonic Stem Cell Maintenance. Dev Biol (2010) 347(2):279–88. doi: 10.1016/j.ydbio.2010.08.026
8. Schmid T, Jansen AP, Baker AR, Hegamyer G, Hagan JP, Colburn NH. Translation Inhibitor Pdcd4 Is Targeted for Degradation During Tumor Promotion. Cancer Res (2008) 68(5):1254–60. doi: 10.1158/0008-5472.CAN-07-1719
9. Hayashi A, Aishima S, Miyasaka Y, Nakata K, Morimatsu K, Oda Y, et al. Pdcd4 Expression in Intraductal Papillary Mucinous Neoplasm of the Pancreas: Its Association With Tumor Progression and Proliferation. Hum Pathol (2010) 41(11):1507–15. doi: 10.1016/j.humpath.2010.02.019
10. Zhao M, Zhu N, Hao F, Song Y, Wang Z, Ni Y, et al. The Regulatory Role of Non-Coding RNAs on Programmed Cell Death Four in Inflammation and Cancer. Front Oncol (2019) 9:919. doi: 10.3389/fonc.2019.00919
11. Liu H, Wang Y, Zhang Y, Song Q, Di C, Chen G, et al. TFAR19, a Novel Apoptosis-Related Gene Cloned From Human Leukemia Cell Line TF-1, Could Enhance Apoptosis of Some Tumor Cells Induced by Growth Factor Withdrawal. Biochem Biophys Res Commun (1999) 254(1):203–10. doi: 10.1006/bbrc.1998.9893
12. Lo KW-H, Zhang Q, Li M, Zhang M. Apoptosis-Linked Gene Product ALG-2 Is a New Member of the Calpain Small Subunit Subfamily of Ca2+-Binding Proteins. Biochemistry (1999) 38(23):7498–508. doi: 10.1021/bi990034n
13. Peng S-Y, Chang K-W, Lin S-C, Tu H-F. miR-134 Targets Programmed Cell Death 7 (PDCD7) Gene to Modulate the Pathogenesis of Head and Neck Carcinoma. AACR (2015).
14. Dibble CF, Horst JA, Malone MH, Park K, Temple B, Cheeseman H, et al. Defining the Functional Domain of Programmed Cell Death 10 Through Its Interactions With Phosphatidylinositol-3, 4, 5-Trisphosphate. PLoS One (2010) 5(7):e11740. doi: 10.1371/journal.pone.0011740
15. Lu L, Ying K, Wei S, Liu Y, Lin H, Mao Y. Dermal Fibroblast-Associated Gene Induction by Asiaticoside Shown In Vitro by DNA Microarray Analysis. Br J Dermatol (2004) 151(3):571–8. doi: 10.1111/j.1365-2133.2004.06146.x
16. Madera S, Chervo MF, Chiauzzi VA, Pereyra MG, Venturutti L, Izzo F, et al. Nuclear PDCD4 Expression Defines a Subset of Luminal B-Like Breast Cancers With Good Prognosis. Horm Cancer (2020) 11(5-6):218–39. doi: 10.1007/s12672-020-00392-4
17. Wen C, Feng X, Yuan H, Gong Y, Wang G. Circ_0003266 Sponges miR-503-5p to Suppress Colorectal Cancer Progression via Regulating PDCD4 Expression. BMC Cancer (2021) 21(1):284. doi: 10.1186/s12885-021-07997-0
18. Kars MD, Işeri ÖD, Gündüz U. A Microarray Based Expression Profiling of Paclitaxel and Vincristine Resistant MCF-7 Cells. Eur J Pharmacol (2011) 657(1-3):4–9. doi: 10.1016/j.ejphar.2011.02.001
19. Ghiotto M, Gauthier L, Serriari N, Pastor S, Truneh A, Nunès JA, et al. PD-L1 and PD-L2 Differ in Their Molecular Mechanisms of Interaction With PD-1. Int Immunol (2010) 22(8):651–60. doi: 10.1093/intimm/dxq049
20. O'Brien J, Hayder H, Zayed Y, Peng C. Overview of microRNA Biogenesis, Mechanisms of Actions, and Circulation. Front Endocrinol (2018) 9:402. doi: 10.3389/fendo.2018.00402
21. Bronnum H, Andersen DC, Schneider M, Sandberg MB, Eskildsen T, Nielsen SB, et al. miR-21 Promotes Fibrogenic Epithelial-to-Mesenchymal Transition of Epicardial Mesothelial Cells Involving Programmed Cell Death 4 and Sprouty-1. PLoS One (2013) 8(2):e56280. doi: 10.1371/journal.pone.0056280
22. Shang YY, Fang NN, Wang F, Wang H, Wang ZH, Tang MX, et al. MicroRNA-21, Induced by High Glucose, Modulates Macrophage Apoptosis via Programmed Cell Death 4. Mol Med Rep (2015) 12(1):463–9. doi: 10.3892/mmr.2015.3398
23. Wang L, Ye N, Lian X, Peng F, Zhang H, Gong H. MiR-208a-3p Aggravates Autophagy Through the PDCD4-ATG5 Pathway in Ang II-Induced H9c2 Cardiomyoblasts. BioMed Pharmacother (2018) 98:1–8. doi: 10.1016/j.biopha.2017.12.019
24. Li Y, Lu J, Bao X, Wang X, Wu J, Li X, et al. MiR-499-5p Protects Cardiomyocytes Against Ischaemic Injury via Anti-Apoptosis by Targeting PDCD4. Oncotarget (2016) 7(24):35607–17. doi: 10.18632/oncotarget.9597
25. Wu Z, Qi Y, Guo Z, Li P, Zhou D. miR-613 Suppresses Ischemia-Reperfusion-Induced Cardiomyocyte Apoptosis by Targeting the Programmed Cell Death 10 Gene. Biosci Trends (2016) 10(4):251–7. doi: 10.5582/bst.2016.01122
26. Goodman NF, Cobin RH, Futterweit W, Glueck JS, Legro RS, Carmina E. American Association of Clinical Endocrinologists, American College of Endocrinology, and Androgen Excess and PCOS Society Disease State Clinical Review: Guide to the Best Practices in the Evaluation and Treatment of Polycystic Ovary Syndrome–Part 1. Endocrine Pract Off J Am Coll Endocrinol Am Assoc Clin Endocrinol (2015) 21(11):1291–300.
27. Fu X, He Y, Wang X, Peng D, Chen X, Li X, et al. MicroRNA-16 Promotes Ovarian Granulosa Cell Proliferation and Suppresses Apoptosis Through Targeting PDCD4 in Polycystic Ovarian Syndrome. Cell Physiol Biochem (2018) 48(2):670–82. doi: 10.1159/000491894
28. Xia H, Zhao Y. miR-155 Is High-Expressed in Polycystic Ovarian Syndrome and Promotes Cell Proliferation and Migration Through Targeting PDCD4 in KGN Cells. Artif Cells Nanomed Biotechnol (2020) 48(1):197–205. doi: 10.1080/21691401.2019.1699826
29. Chen B, Huang SG, Ju L, Li M, Nie FF, Zhang Y, et al. Effect of microRNA-21 on the Proliferation of Human Degenerated Nucleus Pulposus by Targeting Programmed Cell Death 4. Braz J Med Biol Res (2016) 49(6). doi: 10.1590/1414-431x20155020
30. Li C, Chen Y, Chen X, Wei Q, Ou R, Gu X, et al. MicroRNA-183-5p Is Stress-Inducible and Protects Neurons Against Cell Death in Amyotrophic Lateral Sclerosis. J Cell Mol Med (2020) 24(15):8614–22. doi: 10.1111/jcmm.15490
31. Chen H, Wang S, Sun Y, Wang J. Mitomycin C Induces Fibroblast Apoptosis and Reduces Intra-Articular Scar Adhesion by Regulating miR-21 and Its Target Programmed Cell Death 4. Fitoterapia (2020) 142:104392. doi: 10.1016/j.fitote.2019.104392
32. Liang X, Xu Z, Yuan M, Zhang Y, Zhao B, Wang J, et al. MicroRNA-16 Suppresses the Activation of Inflammatory Macrophages in Atherosclerosis by Targeting PDCD4. Int J Mol Med (2016) 37(4):967–75. doi: 10.3892/ijmm.2016.2497
33. Zhang Z, Jin A, Yan D. MicroRNA206 Contributes to the Progression of Steroidinduced Avascular Necrosis of the Femoral Head by Inducing Osteoblast Apoptosis by Suppressing Programmed Cell Death 4. Mol Med Rep (2018) 17(1):801–8. doi: 10.3892/mmr.2017.7963
34. Wang G, Yan Y, Zheng Z, Zhang T. The Mechanism of hsa-miR-424-5 Combining PD-1 Through mTORC Signaling Pathway to Stimulate Immune Effect and Participate in Type 1 Diabetes. Biosci Rep (2020) 40(3). doi: 10.1042/BSR20193800
35. Smith MC, Mader MM, Cook JA, Iversen P, Ajamie R, Perkins E, et al. Characterization of LY3023414, a Novel PI3K/mTOR Dual Inhibitor Eliciting Transient Target Modulation to Impede Tumor Growth. Mol Cancer Ther (2016) 15(10):2344–56. doi: 10.1158/1535-7163.MCT-15-0996
36. Zhang Y, Xiao Y, Ma Y, Liang N, Liang Y, Lu C, et al. ROS-Mediated miR-21-5p Regulates the Proliferation and Apoptosis of Cr(VI)-Exposed L02 Hepatocytes via Targeting PDCD4. Ecotoxicol Environ Saf (2020) 191:110160. doi: 10.1016/j.ecoenv.2019.110160
37. Chang Y, Chen X, Tian Y, Gao X, Liu Z, Dong X, et al. Downregulation of microRNA-155-5p Prevents Immune Thrombocytopenia by Promoting Macrophage M2 Polarization via the SOCS1-Dependent PD1/PDL1 Pathway. Life Sci (2020) 257:118057. doi: 10.1016/j.lfs.2020.118057
38. Hu X, Wang Y, Liang H, Fan Q, Zhu R, Cui J, et al. miR-23a/B Promote Tumor Growth and Suppress Apoptosis by Targeting PDCD4 in Gastric Cancer. Cell Death Dis (2017) 8(10):e3059. doi: 10.1038/cddis.2017.447
39. Li W, Song D, Sun Y, Lv Y, Lv J. microRNA-124-3p Inhibits the Progression of Congenital Hypothyroidism via Targeting Programmed Cell Death Protein 6. Exp Ther Med (2018) 15(6):5001–6. doi: 10.3892/etm.2018.6062
40. Feng MG, Liu CF, Chen L, Feng WB, Liu M, Hai H, et al. MiR-21 Attenuates Apoptosis-Triggered by Amyloid-Beta via Modulating PDCD4/ PI3K/AKT/GSK-3beta Pathway in SH-SY5Y Cells. BioMed Pharmacother (2018) 101:1003–7. doi: 10.1016/j.biopha.2018.02.043
41. Friedland DR, Eernisse R, Erbe C, Gupta N, Cioffi JA. Cholesteatoma Growth and Proliferation: Posttranscriptional Regulation by microRNA-21. Otol Neurotol (2009) 30(7):998–1005. doi: 10.1097/MAO.0b013e3181b4e91f
42. Loreto C, La Rocca G, Anzalone R, Caltabiano R, Vespasiani G, Castorina S, et al. The Role of Intrinsic Pathway in Apoptosis Activation and Progression in Peyronie’s Disease. BioMed Res Int (2014) 2014. 10.1155/2014/616149
43. Elmore S. Apoptosis: A Review of Programmed Cell Death. Toxicologic Pathol (2007) 35(4):495–516. doi: 10.1080/01926230701320337
44. Zhang Z, Wang J, Li J, Wang X, Song W. MicroRNA-150 Promotes Cell Proliferation, Migration, and Invasion of Cervical Cancer Through Targeting PDCD4. BioMed Pharmacother (2018) 97:511–7. doi: 10.1016/j.biopha.2017.09.143
45. Yao Q, Xu H, Zhang QQ, Zhou H, Qu LH. MicroRNA-21 Promotes Cell Proliferation and Down-Regulates the Expression of Programmed Cell Death 4 (PDCD4) in HeLa Cervical Carcinoma Cells. Biochem Biophys Res Commun (2009) 388(3):539–42. doi: 10.1016/j.bbrc.2009.08.044
46. Cappellesso R, Tinazzi A, Giurici T, Simonato F, Guzzardo V, Ventura L, et al. Programmed Cell Death 4 and microRNA 21 Inverse Expression Is Maintained in Cells and Exosomes From Ovarian Serous Carcinoma Effusions. Cancer Cytopathol (2014) 122(9):685–93. doi: 10.1002/cncy.21442
47. Abdulhussain MM, Hasan NA, Hussain AG. Interrelation of the Circulating and Tissue MicroRNA-21 With Tissue PDCD4 Expression and the Invasiveness of Iraqi Female Breast Tumors. Indian J Clin Biochem (2019) 34(1):26–38. doi: 10.1007/s12291-017-0710-1
48. Du G, Cao D, Meng L. miR-21 Inhibitor Suppresses Cell Proliferation and Colony Formation Through Regulating the PTEN/AKT Pathway and Improves Paclitaxel Sensitivity in Cervical Cancer Cells. Mol Med Rep (2017) 15(5):2713–9. doi: 10.3892/mmr.2017.6340
49. Wang YQ, Guo RD, Guo RM, Sheng W, Yin LR. MicroRNA-182 Promotes Cell Growth, Invasion, and Chemoresistance by Targeting Programmed Cell Death 4 (PDCD4) in Human Ovarian Carcinomas. J Cell Biochem (2013) 114(7):1464–73. doi: 10.1002/jcb.24488
50. Wang Y, Liu Z, Shen J. MicroRNA-421-Targeted PDCD4 Regulates Breast Cancer Cell Proliferation. Int J Mol Med (2019) 43(1):267–75. doi: 10.3892/ijmm.2018.3932
51. Yao X, Tu Y, Xu Y, Guo Y, Yao F, Zhang X. Endoplasmic Reticulum Stress-Induced Exosomal miR-27a-3p Promotes Immune Escape in Breast Cancer via Regulating PD-L1 Expression in Macrophages. J Cell Mol Med (2020) 24(17):9560–73. doi: 10.1111/jcmm.15367
52. Wu K, Mu XY, Jiang JT, Tan MY, Wang RJ, Zhou WJ, et al. Mirna26a5p and Mir26b5p Inhibit the Proliferation of Bladder Cancer Cells by Regulating PDCD10. Oncol Rep (2018) 40(6):3523–32. doi: 10.3892/or.2018.6734
53. Zhou Y, Yamamoto Y, Takeshita F, Yamamoto T, Xiao Z, Ochiya T. Delivery of miR-424-5p via Extracellular Vesicles Promotes the Apoptosis of MDA-MB-231 TNBC Cells in the Tumor Microenvironment. Int J Mol Sci (2021) 22(2). doi: 10.3390/ijms22020844
54. Ren LH, Chen WX, Li S, He XY, Zhang ZM, Li M, et al. MicroRNA-183 Promotes Proliferation and Invasion in Oesophageal Squamous Cell Carcinoma by Targeting Programmed Cell Death 4. Br J Cancer (2014) 111(10):2003–13. doi: 10.1038/bjc.2014.485
55. Yang M, Liu R, Li X, Liao J, Pu Y, Pan E, et al. miRNA-183 Suppresses Apoptosis and Promotes Proliferation in Esophageal Cancer by Targeting PDCD4. Molecules Cells (2014) 37(12):873–80. doi: 10.14348/molcells.2014.0147
56. Liu T, Liu Q, Zheng S, Gao X, Lu M, Yang C, et al. MicroRNA-21 Promotes Cell Growth and Migration by Targeting Programmed Cell Death 4 Gene in Kazakh's Esophageal Squamous Cell Carcinoma. Dis Markers (2014) 2014:232837. doi: 10.1155/2014/232837
57. Li L, Zhou L, Li Y, Lin S, Tomuleasa C. MicroRNA-21 Stimulates Gastric Cancer Growth and Invasion by Inhibiting the Tumor Suppressor Effects of Programmed Cell Death Protein 4 and Phosphatase and Tensin Homolog. J BUON (2014) 19(1):228–36.
58. Zheng X, Dong L, Wang K, Zou H, Zhao S, Wang Y, et al. MiR-21 Participates in the PD-1/PD-L1 Pathway-Mediated Imbalance of Th17/Treg Cells in Patients After Gastric Cancer Resection. Ann Surg Oncol (2019) 26(3):884–93. doi: 10.1245/s10434-018-07117-6
59. Liao J, Liu R, Shi YJ, Yin LH, Pu YP. Exosome-Shuttling microRNA-21 Promotes Cell Migration and Invasion-Targeting PDCD4 in Esophageal Cancer. Int J Oncol (2016) 48(6):2567–79. doi: 10.3892/ijo.2016.3453
60. Bhatti I, Lee A, James V, Hall RI, Lund JN, Tufarelli C, et al. Knockdown of microRNA-21 Inhibits Proliferation and Increases Cell Death by Targeting Programmed Cell Death 4 (PDCD4) in Pancreatic Ductal Adenocarcinoma. J Gastrointest Surg (2011) 15(1):199–208. doi: 10.1007/s11605-010-1381-x
61. Zhang R, Sui L, Hong X, Yang M, Li W. MiR-448 Promotes Vascular Smooth Muscle Cell Proliferation and Migration in Through Directly Targeting MEF2C. Environ Sci Pollution Res (2017) 24(28):22294–300. doi: 10.1007/s11356-017-9771-1
62. Duan X, Li W, Hu P, Jiang B, Yang J, Zhou L, et al. MicroRNA-183-5p Contributes to Malignant Progression Through Targeting PDCD4 in Human Hepatocellular Carcinoma. Biosci Rep (2020) 40(10). doi: 10.1042/BSR20201761
63. Zhu Q, Wang Z, Hu Y, Li J, Li X, Zhou L, et al. miR-21 Promotes Migration and Invasion by the miR-21-PDCD4-AP-1 Feedback Loop in Human Hepatocellular Carcinoma. Oncol Rep (2012) 27(5):1660–8. doi: 10.3892/or.2012.1682
64. Yang YL, Liu P, Li D, Yang Q, Li B, Jiang XJ. Stat-3 Signaling Promotes Cell Proliferation and Metastasis of Gastric Cancer Through PDCD4 Downregulation. Kaohsiung J Med Sci (2020) 36(4):244–9. doi: 10.1002/kjm2.12159
65. Motoyama K, Inoue H, Mimori K, Tanaka F, Kojima K, Uetake H, et al. Clinicopathological and Prognostic Significance of PDCD4 and microRNA-21 in Human Gastric Cancer. Int J Oncol (2010) 36(5):1089–95. doi: 10.3892/ijo_00000590
66. Fan Y, Che X, Hou K, Zhang M, Wen T, Qu X, et al. MiR-940 Promotes the Proliferation and Migration of Gastric Cancer Cells Through Up-Regulation of Programmed Death Ligand-1 Expression. Exp Cell Res (2018) 373(1-2):180–7. doi: 10.1016/j.yexcr.2018.10.011
67. Yin K, Liu M, Zhang M, Wang F, Fen M, Liu Z, et al. miR-208a-3p Suppresses Cell Apoptosis by Targeting PDCD4 in Gastric Cancer. Oncotarget (2016) 7(41):67321–32. doi: 10.18632/oncotarget.12006
68. Du Y, Wang D, Luo L, Guo J. miR-129-1-3p Promote BGC-823 Cell Proliferation by Targeting PDCD2. Anat Rec (Hoboken) (2014) 297(12):2273–9. doi: 10.1002/ar.23003
69. Wang J, Zhao X, Wang Y, Ren F, Sun D, Yan Y, et al. circRNA-002178 Act as a ceRNA to Promote PDL1/PD1 Expression in Lung Adenocarcinoma. Cell Death Dis (2020) 11(1):32. doi: 10.1038/s41419-020-2230-9
70. Liu Y, Uzair Ur R, Guo Y, Liang H, Cheng R, Yang F, et al. miR-181b Functions as an oncomiR in Colorectal Cancer by Targeting PDCD4. Protein Cell (2016) 7(10):722–34. doi: 10.1007/s13238-016-0313-2
71. Wu H, Xu L, Chen Y, Xu C. MiR-208a-3p Functions as an Oncogene in Colorectal Cancer by Targeting PDCD4. Biosci Rep (2019) 39(4). doi: 10.1042/BSR20181598
72. Nedaeinia R, Sharifi M, Avan A, Kazemi M, Nabinejad A, Ferns GA, et al. Inhibition of microRNA-21 via Locked Nucleic acid-anti-miR Suppressed Metastatic Features of Colorectal Cancer Cells Through Modulation of Programmed Cell Death 4. Tumour Biol (2017) 39(3):1010428317692261. doi: 10.1177/1010428317692261
73. Ashizawa M, Okayama H, Ishigame T, Thar Min AK, Saito K, Ujiie D, et al. miRNA-148a-3p Regulates Immunosuppression in DNA Mismatch Repair-Deficient Colorectal Cancer by Targeting PD-L1. Mol Cancer Res (2019) 17(6):1403–13. doi: 10.1158/1541-7786.MCR-18-0831
74. Sun Z, Li S, Kaufmann AM, Albers AE. miR-21 Increases the Programmed Cell Death 4 Gene-Regulated Cell Proliferation in Head and Neck Squamous Carcinoma Cell Lines. Oncol Rep (2014) 32(5):2283–9. doi: 10.3892/or.2014.3456
75. Wu Q, Zhao Y, Sun Y, Yan X, Wang P. miR-375 Inhibits IFN-Gamma-Induced Programmed Death 1 Ligand 1 Surface Expression in Head and Neck Squamous Cell Carcinoma Cells by Blocking JAK2/STAT1 Signaling. Oncol Rep (2018) 39(3):1461–8. doi: 10.3892/or.2018.6177
76. Reis PP, Tomenson M, Cervigne NK, Machado J, Jurisica I, Pintilie M, et al. Programmed Cell Death 4 Loss Increases Tumor Cell Invasion and Is Regulated by miR-21 in Oral Squamous Cell Carcinoma. Mol Cancer (2010) 9:238. doi: 10.1186/1476-4598-9-238
77. Jiang LH, Ge MH, Hou XX, Cao J, Hu SS, Lu XX, et al. miR-21 Regulates Tumor Progression Through the miR-21-PDCD4-Stat3 Pathway in Human Salivary Adenoid Cystic Carcinoma. Lab Invest (2015) 95(12):1398–408. doi: 10.1038/labinvest.2015.105
78. Zargar S, Tomar V, Shyamsundar V, Vijayalakshmi R, Somasundaram K, Karunagaran D. A Feedback Loop Between MicroRNA 155 (miR-155), Programmed Cell Death 4, and Activation Protein 1 Modulates the Expression of miR-155 and Tumorigenesis in Tongue Cancer. Mol Cell Biol (2019) 39(6). doi: 10.1128/MCB.00410-18
79. Shuang Y, Zhou X, Li C, Huang Y, Zhang L. MicroRNA503 Serves an Oncogenic Role in Laryngeal Squamous Cell Carcinoma via Targeting Programmed Cell Death Protein 4. Mol Med Rep (2017) 16(4):5249–56. doi: 10.3892/mmr.2017.7278
80. Wei C, Song H, Sun X, Li D, Song J, Hua K, et al. miR-183 Regulates Biological Behavior in Papillary Thyroid Carcinoma by Targeting the Programmed Cell Death 4. Oncol Rep (2015) 34(1):211–20. doi: 10.3892/or.2015.3971
81. Ning FL, Wang F, Li ML, Yu ZS, Hao YZ, Chen SS. MicroRNA-182 Modulates Chemosensitivity of Human Non-Small Cell Lung Cancer to Cisplatin by Targeting PDCD4. Diagn Pathol (2014) 9:143. doi: 10.1186/1746-1596-9-143
82. Fu WF, Chen WB, Dai L, Yang GP, Jiang ZY, Pan L, et al. Inhibition of miR-141 Reverses Cisplatin Resistance in Non-Small Cell Lung Cancer Cells via Upregulation of Programmed Cell Death Protein 4. Eur Rev Med Pharmacol Sci (2016) 20(12):2565–72.
83. Yang Y, Meng H, Peng Q, Yang X, Gan R, Zhao L, et al. Downregulation of microRNA-21 Expression Restrains Non-Small Cell Lung Cancer Cell Proliferation and Migration Through Upregulation of Programmed Cell Death 4. Cancer Gene Ther (2015) 22(1):23–9. doi: 10.1038/cgt.2014.66
84. Yang D, Wang JJ, Li JS, Xu QY. miR-103 Functions as a Tumor Suppressor by Directly Targeting Programmed Cell Death 10 in NSCLC. Oncol Res (2018) 26(4):519–28. doi: 10.3727/096504017X15000757094686
85. Itani S, Kunisada T, Morimoto Y, Yoshida A, Sasaki T, Ito S, et al. MicroRNA-21 Correlates With Tumorigenesis in Malignant Peripheral Nerve Sheath Tumor (MPNST) via Programmed Cell Death Protein 4 (PDCD4). J Cancer Res Clin Oncol (2012) 138(9):1501–9. doi: 10.1007/s00432-012-1223-1
86. Gaur AB, Holbeck SL, Colburn NH, Israel MA. Downregulation of Pdcd4 by Mir-21 Facilitates Glioblastoma Proliferation In Vivo. Neuro Oncol (2011) 13(6):580–90. doi: 10.1093/neuonc/nor033
87. Wang Z, Yao W, Li K, Zheng N, Zheng C, Zhao X, et al. Reduction of miR-21 Induces SK-N-SH Cell Apoptosis and Inhibits Proliferation via PTEN/Pdcd4. Oncol Lett (2017) 13(6):4727–33. doi: 10.3892/ol.2017.6052
88. Guo P, Yu Y, Tian Z, Lin Y, Qiu Y, Yao W, et al. Upregulation of miR-96 Promotes Radioresistance in Glioblastoma Cells via Targeting PDCD4. Int J Oncol (2018) 53(4):1591–600. doi: 10.3892/ijo.2018.4498
89. Xu X, Ge S, Jia R, Zhou Y, Song X, Zhang H, et al. Hypoxia-Induced miR-181b Enhances Angiogenesis of Retinoblastoma Cells by Targeting PDCD10 and GATA6. Oncol Rep (2015) 33(6):2789–96. doi: 10.3892/or.2015.3900
90. Wang X, Zuo D, Yuan Y, Yang X, Hong Z, Zhang R. MicroRNA-183 Promotes Cell Proliferation via Regulating Programmed Cell Death 6 in Pediatric Acute Myeloid Leukemia. J Cancer Res Clin Oncol (2017) 143(1):169–80. doi: 10.1007/s00432-016-2277-2
91. Wang LQ, Kumar S, Calin GA, Li Z, Chim CS. Frequent Methylation of the Tumour Suppressor miR-1258 Targeting PDL1: Implication in Multiple Myeloma-Specific Cytotoxicity and Prognostification. Br J Haematol (2020) 190(2):249–61. doi: 10.1111/bjh.16517
92. Fischer N, Goke F, Splittstosser V, Lankat-Buttgereit B, Muller SC, Ellinger J. Expression of Programmed Cell Death Protein 4 (PDCD4) and miR-21 in Urothelial Carcinoma. Biochem Biophys Res Commun (2012) 417(1):29–34. doi: 10.1016/j.bbrc.2011.11.035
93. Fan B, Jin Y, Zhang H, Zhao R, Sun M, Sun M, et al. MicroRNA21 Contributes to Renal Cell Carcinoma Cell Invasiveness and Angiogenesis via the PDCD4/cJun (AP1) Signalling Pathway. Int J Oncol (2020) 56(1):178–92. doi: 10.3892/ijo.2019.4928
94. Jiao J, Fan Y, Zhang Y. Expression and Clinicopathological Significance of microRNA-21 and Programmed Cell Death 4 in Malignant Melanoma. J Int Med Res (2015) 43(5):672–8. doi: 10.1177/0300060515583707
95. Hassan M, Watari H, AbuAlmaaty A, Ohba Y, Sakuragi N. Apoptosis and Molecular Targeting Therapy in Cancer. BioMed Res Int (2014) 2014. doi: 10.1155/2014/150845
96. Chowdhury I, Tharakan B, Bhat GK. Caspases—An Update. Comp Biochem Physiol Part B: Biochem Mol Biol (2008) 151(1):10–27. doi: 10.1016/j.cbpb.2008.05.010
97. Alsaab HO, Sau S, Alzhrani R, Tatiparti K, Bhise K, Kashaw SK, et al. PD-1 and PD-L1 Checkpoint Signaling Inhibition for Cancer Immunotherapy: Mechanism, Combinations, and Clinical Outcome. Front Pharmacol (2017) 8:561. doi: 10.3389/fphar.2017.00561
98. Sun R, Zhang L. Long Non-Coding RNA MALAT1 Regulates Cardiomyocytes Apoptosis After Hypoxia/Reperfusion Injury via Modulating miR-200a-3p/PDCD4 Axis. BioMed Pharmacother (2019) 111:1036–45. doi: 10.1016/j.biopha.2018.12.122
99. Wei S, Wang K, Huang X, Zhao Z, Zhao Z. LncRNA MALAT1 Contributes to Non-Small Cell Lung Cancer Progression via Modulating miR-200a-3p/Programmed Death-Ligand 1 Axis. Int J Immunopathol Pharmacol (2019) 33:2058738419859699. doi: 10.1177/2058738419859699
100. Lu J, Xue Y, Wang Y, Ding Y, Zou Q, Pan M, et al. CiRS-126 Inhibits Proliferation of Ovarian Granulosa Cells Through Targeting the miR-21-PDCD4-ROS Axis in a Polycystic Ovarian Syndrome Model. Cell Tissue Res (2020) 381(1):189–201. doi: 10.1007/s00441-020-03187-9
101. Zhao L, Liu Y, Zhang J, Liu Y, Qi Q. LncRNA SNHG14/miR-5590-3p/ZEB1 Positive Feedback Loop Promoted Diffuse Large B Cell Lymphoma Progression and Immune Evasion Through Regulating PD-1/PD-L1 Checkpoint. Cell Death Dis (2019) 10(10):731. doi: 10.1038/s41419-019-1886-5
102. Zhou WY, Zhang MM, Liu C, Kang Y, Wang JO, Yang XH. Long Noncoding RNA LINC00473 Drives the Progression of Pancreatic Cancer via Upregulating Programmed Death-Ligand 1 by Sponging microRNA-195-5p. J Cell Physiol (2019) 234(12):23176–89. doi: 10.1002/jcp.28884
Keywords: lncRNA, miRNA, programmed cell death protein, expression, biomarker
Citation: Ghafouri-Fard S, Hussen BM, Mohaqiq M, Shoorei H, Baniahmad A, Taheri M and Jamali E (2022) Interplay Between Non-Coding RNAs and Programmed Cell Death Proteins. Front. Oncol. 12:808475. doi: 10.3389/fonc.2022.808475
Received: 03 November 2021; Accepted: 25 February 2022;
Published: 23 March 2022.
Edited by:
Xiangbing Meng, The University of Iowa, United StatesReviewed by:
Hsin-Sheng Yang, University of Kentucky, United StatesHanuma Kumar Karnati, University of Nebraska Medical Center, United States
Jing Zhong, University of South China, China
Copyright © 2022 Ghafouri-Fard, Hussen, Mohaqiq, Shoorei, Baniahmad, Taheri and Jamali. This is an open-access article distributed under the terms of the Creative Commons Attribution License (CC BY). The use, distribution or reproduction in other forums is permitted, provided the original author(s) and the copyright owner(s) are credited and that the original publication in this journal is cited, in accordance with accepted academic practice. No use, distribution or reproduction is permitted which does not comply with these terms.
*Correspondence: Mohammad Taheri, bW9oYW1tYWQudGFoZXJpQHVuaS1qZW5hLmRl; Elena Jamali, ZWxlbmEuamFtYWxpQHlhaG9vLmNvbQ==