- 1Department of Neurosurgery, Xijing Hospital, Air Force Military Medical University, Xi’an, China
- 2Department of Neurology, Tangdu Hospital, Air Force Military Medical University, Xi’an, China
- 3Department of Anesthesiology, Xijing Hospital, Air Force Military Medical University, Xi’an, China
- 4State Key Laboratory of Cancer Biology, Department of Medical Genetics and Developmental Biology, Air Force Military Medical University, Xi’an, China
Glioblastoma (GBM) is a highly invasive neurological malignancy with poor prognosis. LncRNA-GAS5 (growth arrest-specific transcript 5) is a tumor suppressor involved in multiple cancers. In this study, we explored the clinical significance, biological function, and underlying mechanisms of GAS5 in GBM. We showed that lncRNA-GAS5 expression decreased in high-grade glioma tissues and cells, which might be associated with poor prognosis. GAS5 overexpression lowered cell viability, suppressed GBM cell migration and invasion, and impaired the stemness and proliferation of glioma stem cells (GSCs). We further discovered that GAS5 inhibited the viability of glioma cells through miR-let-7e and miR-125a by protecting SPACA6 from degradation. Moreover, GAS5 played an anti-oncogenic role in GBM through the combined involvement of let-7e and miR-125a in vivo and in vitro. Notably, these two miRNAs block the IL-6/STAT3 pathway in tumor tissues extracted from a xenograft model. Taken together, our study provides evidence for an important role of GAS5 in GBM by affecting the proliferation and migration of GSCs, thus providing a new potential prognostic biomarker and treatment strategy for GBM.
Introduction
Glioblastoma (GBM) is the most common malignant primary brain tumor in adults, with a median survival of barely 1.3 years upon detection (1, 2). The discouraging overall prognosis of gliomas has changed little over the decades despite medical advances in neurosurgery, chemotherapy, radiation, and many novel clinical trials (3). The proliferation and invasion capacities of GBMs make it extremely difficult for tumors to be resected completely. With the advent of molecular biology, new studies on gene regulation networks have provided directions for understanding the molecular mechanisms of intracranial tumors (4, 5). The classification of gliomas was reorganized by the 2016 revision of the World Health Organization Classification of Tumors of the Central Nervous System (CNS), which highlights molecular features in addition to histopathological appearance (6). Notably, the malignant features of glioma cells, including invasive growth, resistance to conventional chemotherapy or radiotherapy, and recurrence, are mainly attributed to glioma stem cells (GSCs). The GSCs display three prominent features of stem cells, i.e. self-renewal capacity, indefinite proliferation, and differentiation (7). Hence, GSCs are responsible for not only the infinite growth of GBM but also incomplete and residual tumor resection, which generally results in a poor prognosis after treatment. Therefore, a comprehensive understanding of the molecular pathogenesis of GSCs is urgently required.
Noncoding RNAs (ncRNAs) consist of microRNAs (miRNAs) and long ncRNAs (lncRNAs). LncRNAs are a type of RNAs of more than 200 nucleotides (nt) in length, and there is no solid evidence to prove that they encode peptides (8). Numerous studies have shown that lncRNAs play crucial roles in the malignant biology of tumors. Abnormal expression of lncRNAs in tumors indicates tumor progression as a specific marker for certain tumors and is likely to become a new therapeutic target (8, 9). It has been shown that lncRNAs are involved in chromosome remodeling or gene expression via post-transcriptional regulation (10–12), alternative splicing (13), and initiated X-chromosome inactivation (14). As suggested in recent studies, lncRNAs may influence cell proliferation, migration, and invasion in vitro and participate in the DNA damage response, tumorigenesis, and drug resistance (15–17). miRNAs are transcripts of approximately 21-23 nucleotides in size that are produced in the nucleus and cytoplasm of cells through a multi-step process (18). Traditionally, some abundant lncRNAs with complementary miRNA loci can act as competitive endogenous RNAs or miRNA sponges through direct base pairing to regulate gene expression, thereby inhibiting the function of miRNA-targeted mRNAs (19). Accumulating evidence has shown that lncRNAs are related to the stemness of GSCs through epigenetic inhibition of genes associated with multi-neuron differentiation or impact GSCs proliferation, migration, and invasion by binding to certain miRNAs and regulating downstream molecular pathways (20–22). Therefore, elucidation of the mechanisms and underlying relationship between lncRNAs and GSCs will help offer a new focus on the tumorigenesis and therapeutic response of GBM.
In recent years, LncRNA-GAS5 (growth arrest-specific transcript 5) has been reported as a tumor suppressor lncRNA in various human cancers. For instance, its downregulation has been observed in epithelial ovarian cancer, cervical cancer, lung cancer, and gliomas (23–27). GAS5 directly interacts with the WW structural domain of YAP to inhibit colorectal cancer progression in vitro and in vivo (28). Wang et al. found that GAS5 overexpression inhibits M2-like polarization of tumor-associated macrophages by enhancing the expression of PTEN, thereby inhibiting the proliferation and invasion of liver cancer cells (29). In addition, Ding et al. suggested that GAS5 attenuates glioma progression by eliminating microRNA-10b and Sirtuin1 (30). Therefore, new insights into the molecular mechanisms underlying GAS5 will provide important directions for future research. In this study, we identified a remarkable decrease in GAS5 expression in glioma tissues and cells, which was closely related to the viability and migration of GBM cells and GSCs. SPACA6 and its downstream miRNAs, let-7e, and miR-125a played a role in these phenomena, and the IL-6/IL-6R/STAT3 pathway is involved in the molecular mechanism of GAS5 as an anti-oncogene. These results indicate that GAS5 could attenuate GSCs activity and suppress glioma progression, which may provide novel strategies for glioma therapy.
Materials and methods
Ethics statement
The research strategy was approved by the Research Ethics Committee of the Xijing Hospital of the Airforce Medical University. All experiments were performed in accordance with relevant guidelines and regulations, and written informed consent was obtained from all the patients involved. Written consent for the use of all data was also obtained from the lead researcher (Principle Investigator, PI) of the neurosurgical glioma center of Xijing Hospital. The privacy rights of the human subjects were observed in our study.
Clinical specimens
Glioma tissues were obtained from 72 patients who underwent primary surgery and were histopathologically diagnosed with glioma at the Xijing Hospital. Samples were collected and stored in liquid nitrogen, and the remaining clinical data were obtained from the hospital case system and follow-up of patients.
Cell lines and cell culture
Four human GBM cell lines (U251, U87, A172, and T98) and a healthy glial cell line (HEB) were obtained from the American Type Culture Collection (ATCC), while other human GBM cell lines (SHG44) were purchased from the Wuhan Procell Life Technology Collection. The cells were cultured in DMEM (Gibco, USA) medium supplemented with 10% fetal bovine serum (FBS, Hyclone, USA) at 37 °C in a humidified environment with 5% CO2. The cells tested negative for mycoplasma contamination. Patient-derived glioblastoma stem-like cells (GSC-1 and GSC-2) were isolated from surgical samples. Briefly, tissues were mechanically minced and then digested with 0.1% trypsin (Invitrogen, USA) and 10 U mL−1 of DNase I (Promega, USA) at 37 °C for 45 min. ACK lysis buffer (Beyotime, China) was used to lyse red blood cells. The washed tissues were triturated by pipetting, passed through a 40 μm cell strainer, and grown in DMEM/F12 (Gibco, USA) supplemented with B27 (Gibco, USA), EGF (20 ng/mL) (Propotech, USA), bFGF (20 ng/mL) (Propotech, USA), and 1% penicillin/streptomycin (Life Technologies, China).
RNA extraction and quantitative real-time polymerase chain reaction (qRT-PCR)
Total RNA was extracted from GBM tissues and cancer cells using the TRIzol reagent (Invitrogen, USA). The concentration and purity of RNA were measured using an Eppendorf Biophotometer Plus. The PrimeScript™ RT Reagent Kit (Takara, Japan) was used to reverse-transcribe the lncRNAs and mRNA. Relative gene expression levels were determined using a SYBR Green PCR Kit (Takara, Japan). The relative quantity of gene expression was calculated using the standard 2−ΔΔCt method, with GAPDH as an internal control. Primer sequences are listed in Supplementary Table 1.
Cell counting kit-8 (CCK-8) assay
Cells were seeded in 96-well plates at a density of 2×103 cells/well, and cell viability was assessed using the CCK-8 Assay Kit (Dojindo Molecular Technologies, Japan), as instructed by the manufacturer. Samples were measured at OD 490 nm using the Bio-Rad Microplate Reader Model 680 (Bio-Rad, China).
Colony formation experiment
The colony formation experiment was performed 48 h after transfection. The cell lines were resuspended and plated in 10 cm culture plates at a densities of approximately 50/100/200 cells/well. The cells were cultured under standard conditions for three weeks, and the culture medium was changed every three days. Colonies formed by the clones were collected, washed twice with phosphate-buffered saline (PBS), fixed with formaldehyde solution, and stained with Giemsa stain (Xi ‘anYike Biotechnology Co., LTD, China). Colony formation was observed and photographed using an optical microscope and a digital camera.
Flow cytometry
CD133 expression and cell apoptosis were measured by flow cytometry. To detect the expression of CD133, a single-cell suspension was obtained from well-grown attached cells by trypsinization (without EDTA) under sterile conditions. Samples were stained with IgG1 Isotype Control Summary (R&D Systems, USA) for approximately 15 min at 4°Cin the dark. Next, cells were washed with cold PBS, centrifuged at 1000 rpm for 5 min at 4 °C, and subsequently stained with the human CD133-PE antibody (eBioscience, Thermo Scientific, USA) for approximately 30 min at 4°Cin the dark. A fluorescence-activated cell sorting (FACS) instrument, CantoII Flow Cytometer (BD Biosciences, USA), was used to examine the results.
Cells were plated in 6-well plates for the detection of apoptosis. After transfection, a single-cell suspension was obtained, and the cells were stained using the Annexin V-Fluorescein Isothiocyanate (FITC) Apoptosis Detection Kit (Beyotime, China) for 15 min at room temperature. Finally, the analysis was conducted using a FACS CantoII Flow Cytometer (BD Biosciences, USA) and FlowJo 10.0 software.
Scratch test
Cells were seeded in 6-well plates at a density of 5×105 cells/well. After transfection, the cells with complete confluence were allowed to draw five uniform straight lines along the central axis at a right angle to the bottom of the 6-well plate using a sterile 10-μL pipette tip. After rinsing three times with PBS, the cells were cultured in serum-free medium for 0.5 h. Subsequently, cell migration was observed and photographed at 0, 24, and 48 h under an inverted optical microscope.
Transwell assay
The transwell assay was performed using a transwell chamber (BD Biosciences, USA), and cells were placed in the upper chambers at a density of 5×103 cells/well. Subsequently, medium containing 10% FBS was added to the bottom of the chamber. After incubation for 24 h and fixation with 4% formaldehyde, the migrated cells on the lower surface were stained with 0.1% crystal violet. Finally, the cells were observed and counted using an inverted optical microscope.
Tumorsphere formation assay
The concentration of GSCs was adjusted to 5×103 cell/mL, and GSCs were cultured in 96-well plates at 100 uL/well with the medium changed every three days. Seven days after seeding, each well was examined, and the number and volume of spheres/cell aggregates were counted.
5’-Ethynyl-2’-deoxyuridine (EdU) assay
Cell proliferation was assessed using the EdU assay (Rainbow, Shanghai, China). GSCs were plated in 24-well plates at a density of 5×105 cells/mL. After seven days of cultured, the cells were incubated with 50 μM EdU working solution (5-ethynyl-2’-deoxyuridine preheated to 37°C) for 2 h. The cells were fixed with 4% paraformaldehyde and permeabilized with 0.5% Triton X-100. Afterward, 100 uL of click reaction solution was added to each slide, and nuclei were stained with Hoechst. Finally, the images were obtained using an inverted fluorescence microscope.
Protein preparation and western blotting
Total protein was prepared from GBM cells using pre-chilled RIPA buffer (Gibco, USA) with a proteinase and phosphatase inhibitor cocktail (Selleck, Shanghai, China). The PVDF membranes were incubated with primary antibodies overnight (Supplementary Table 2) at 4°C and then with an HRP-labeled secondary antibody (Zsbio Store-bio, Beijing, China) at room temperature for 1 h. The protein bands were visualized using a chemiluminescence reagent (ECL) kit (Boster, Wuhan, China).
RNA antisense purification assay
RNA antisense purification (RAP) assay was commissioned by Crystal Energy Biotechnology Co., Ltd. The experimental steps have been reported by Engreitz et al. (31), as follows: assay design, probe design, probe generation, cell harvesting and cross-linking, cell lysis, lysate preparation, hybridization, capture, washing, elution, RNA analysis, and DNA analysis.
Luciferase reporter assay
HEK293t cells were seeded into 24-well plates and cultured overnight, and let-7e and miR-125a wild-type cells with potential IL6/IL6R binding sites or mutants of each binding site (Supplementary Table 3) were generated and fused to the luciferase reporter pGL3-basic plasmid, alone or following pre-treatment with miR-7-5p mimics or inhibitor. According to the manufacturer’s protocol, the relative luciferase activity in each well was analyzed after 24 h using a dual-luciferase reporter assay system (Promega, USA).
Xenograft model in vivo
All animal experiments were approved by the Animal Experiment Administration Committee of the Air Force Military Medical University. All experiments were performed in accordance with the National Research Council’s Guide for the Care and Use of Laboratory Animals. Four-week-old athymic BALB/c nude mice were purchased from the Air Force Medical University Animal Center (Xi’an, China). U87 cells were transfected, and a total of 5 × 106 GBM cells per mouse were stereotactically injected into the skin or brain. The intracranial tumors were measured weekly using bioluminescence imaging. After a month, the subcutaneous tumors were measured. Subsequently, the mice were sacrificed and the tumor tissues were removed for experiments.
Statistical analysis
All statistical analyses were performed using SPSS (version 19.0; IBM Corporation, USA) and GraphPad Prism9.0 (GraphPad Software Inc., USA). Differences between groups were analyzed using the Student’s t-test or Cox proportional hazards model analysis. The in vitro experiments were repeated at least three times, and the data are presented as mean ± standard deviation (SD) from at least three independent experiments. Statistical significance is indicated by *P<0.05, **P<0.01, or ***P<0.001.
Results
LncRNA-GAS5 levels are decreased in high-grade glioma tissues and cells
Recently, some investigators have shown that lncRNA-GAS5 functions as a protein-binding RNA to directly regulate glioma progression (32, 33). To explore the role of GAS5 in glioma, we determined the expression of GAS5 in 72 glioma samples that were divided into high- and low-grades according to pathological classification. qRT-PCR analysis showed that GAS5 expression was lower in high-grade glioma tissues than in low-grade glioma tissues (Figure 1A). Moreover, with an increase in glioma grade, the expression of GAS5 declined drastically (Figure 1B), indicating a latent negative relationship between the expression level of GAS5 and glioma grade. To further investigate this correlation, we evaluated the clinical data of 72 patients and found that the pathological grading of glioma and the KPS score of patients significantly correlated with GAS5 expression (Table 1). In addition, the median survival of patients with high-grade glioma was significantly lower than that of those with low-grade gliomas (Figure 1C). Subsequently, patients were divided into different groups according to GAS5 expression, which revealed that the median survival of the high GAS5 expression group was significantly higher than that of the low expression group (Figure 1D). Moreover, we established a Cox proportional hazards model and found that the preoperative KPS score, tumor pathological grade, and lncRNA-GAS5 expression level significantly correlated with postoperative survival time, indicating that they were independent risk factors for patient prognosis (Table 2). Furthermore, five types of glioma cells (A172, SHG-44, T98, U251, U87) and one healthy glial cell line (HEB) were used as references. qRT-PCR showed that GAS5 expression considerably decreased in glioma cells (Figure 1E). Thus, all data indicated that GAS5 expression decreased in GBM tissues and cells, which might be associated with poor prognosis.
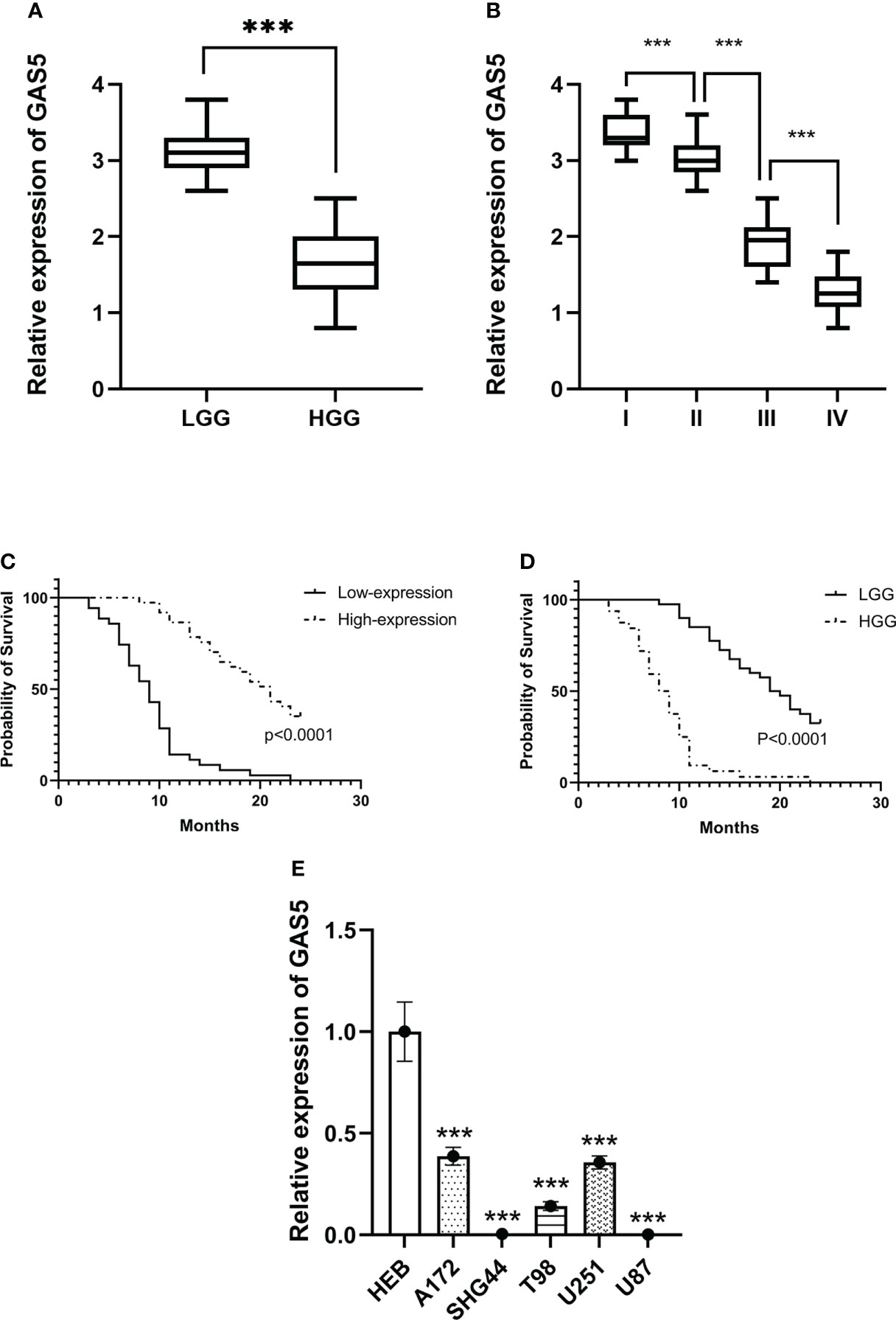
Figure 1 LncRNA-GAS5 is low-expressed in high-grade glioma tissues and cells. (A) GAS5 levels are measured in high grade glioma (HGG) tissues (n=36) and low-grade glioma (LGG) tissues (n=36) by qRT-PCR using GAPDH as a reference gene. (B) GAS5 levels are measured in WHO I (n=11), II (n=29), III (n=18) and IV (n=14) grade glioma tissues using GAPDH as a reference gene. (C) Kaplan-Meier survival curve of patients divided into HGG (n=36) and LGG (n=36) involved in our study is shown. (D) Kaplan-Meier survival curve of patients is divided into high-expression group and low-expression group according to the expression of GAS5 involved in our study is shown. (E) RNA is extracted from glial cell line (HEB) and five types of glioma cell lines (A172, SHG-44, T98, U251, U87) (n=3 in each cell lines), GAS5 expression level is measured by qRT-PCR using GAPDH as a reference gene. Unless otherwise noted, data are presented as the mean ± S.D. P value is determined by Student’s t-test (***P < 0.001).
LncRNA-GAS5 repressed the viability and migration of glioma cells
To explore the role of GAS5 in GBM progression, we enhanced or knocked down the expression of GAS5 in U251, U87, A172, T98, and SHG-44 cells by transfecting them with specific GAS5-overexpression or GAS5-shRNA lentiviruses. The results revealed that GAS5 expression remarkably increased in U87, A172, and SHG-44 cells and decreased in U87 cells after transfection (Supplementary Figure 1A). Hence, we used these cell lines for follow-up tests.
Cell viability was then assayed using the CCK8 assay at various time points (Figure 2A). The overexpression of GAS5 drastically diminished cellular survival, whereas knockdown of GAS5 enhanced cell survival. Colony formation assays indicated a significant decline in the rate of colony formation after GAS5 overexpression (Figure 2B), and knockdown of GAS5 increased the colony-forming ability of glioma cells, indicating that GAS5 impaired cell self-renewal. As indicated by flow cytometry assays, elevated GAS5 levels suppressed the apoptosis of glioma cells, but knockdown of GAS5 did not have an obvious influence on cell apoptosis (Figure 2C and Supplementary Figure 2C). In addition, the invasion and migration abilities of GBM cells were assessed. Transwell assays revealed that GAS5 overexpression suppressed the invasive ability of glioma cells (Figure 2D). Furthermore, the scratch test indicated that the overexpression of GAS5 inhibited the migration ability of cells (Figure 2E and Supplementary Figure 1B). Conversely, following GAS5 knockdown, the migratory ability of U87 cells was stronger than that of the control group (Figures 2D, E). Overall, the above experimental results revealed that GAS5 plays a negative role in promoting tumor progression and the malignancy of glioma cells.
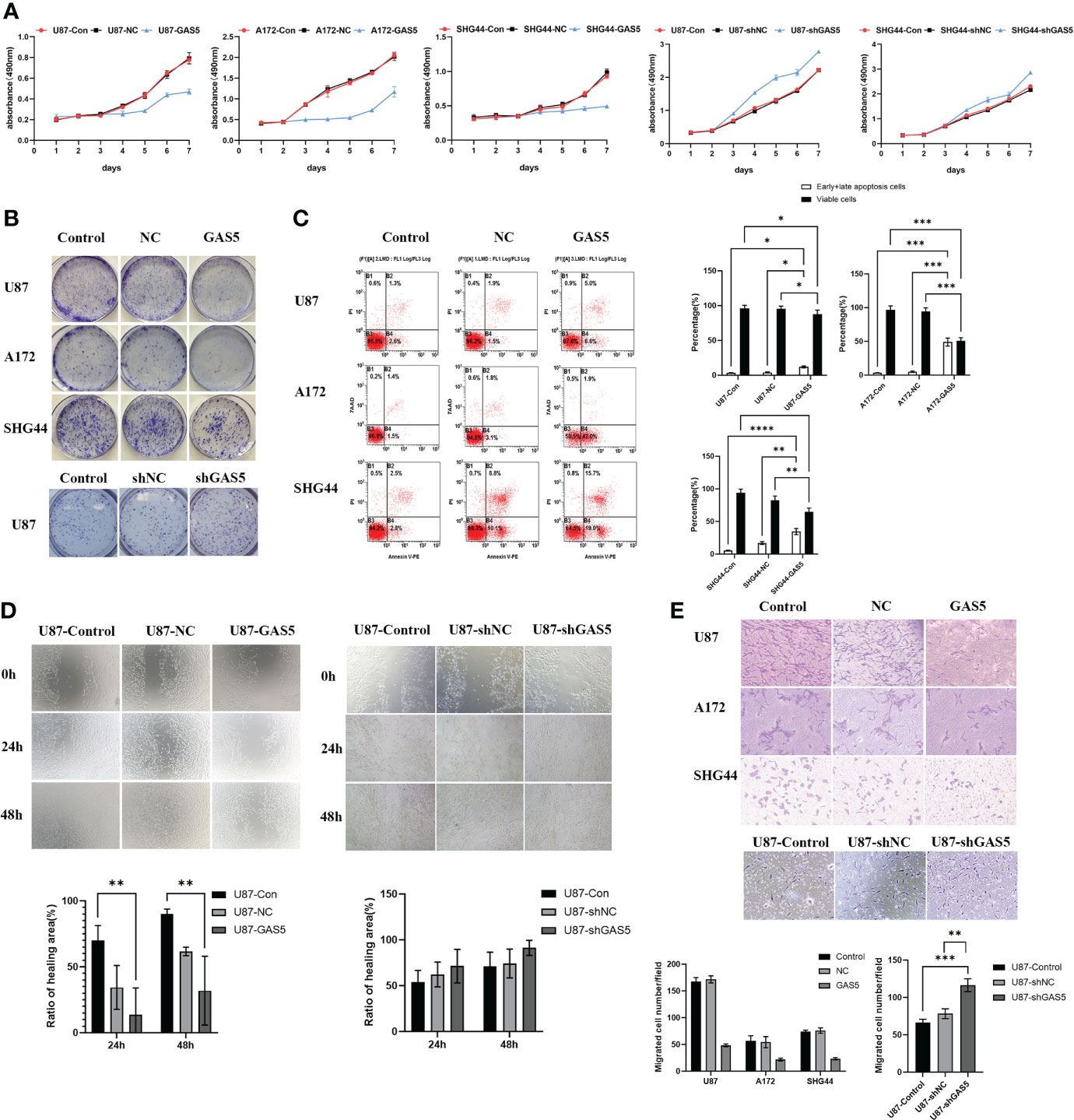
Figure 2 LncRNA-GAS5 overexpression diminished cell viability and suppressed the migration and invasion of GBM cells. (A) CCK-8 assay revealed the effect of GAS5 overexpression and knockdwon on the proliferation of parental U87, A172 and SHG44 cell lines with the cells are cultured for seven consecutive days and the absorbance of the cells are measured daily (n=6). (B) Colony formation assay of GAS5 overexpression and knockdwon and parental GBM cell lines (n=3). Representative images are shown. (C) Flow cytometric analysis of apoptosis in GAS5 overexpression and knockdwon and parental GBM cell lines (n=3). The statistical result of the flow cytometric analysis is shown. (D) Scratch test showed the healing ability of U87 cells (n=3). Representative images and the ratio of healing area are shown. (E) Transwell assay of the effect of GAS5 on the migration and invasion of GBM cells (n=3). Representative images and the migration cell number are shown. Data are presented as the mean ± S.D. P value is determined by Student’s t test (*P < 0.05, **P < 0.01, ***P < 0.001, ****P<0.0001).
LncRNA-GAS5 overexpression impaired the stemness and proliferation of GSCs
Although GAS5 has been shown to regulate glioma cells, its detailed mechanisms remain unclear. GSCs play a vital role in GBM progression and tumorigenesis. To clarify the function of GAS5 in GBM stem-like cells (GSCs), we cultured GSCs from GBM surgical specimens and named them GSC-1 and GSC-2 (Figure 3A). To further elucidate the stemness of GSC-1 and GSC-2, we analyzed the expression of the GSC marker CD133 and induced cell differentiation (Supplementary Figures 2A–C). As indicated by the results, GSC-1 and GSC-2 possessed the typical characteristics of stem cells and could be used in subsequent experiments. Further analysis indicated that overexpression of GAS5 in GSCs substantially diminished the expression of stem cell markers, including CD133 and Notch1, which are related to the stemness and malignant progression of gliomas (Figure 3B and Supplementary Figure 2D). Moreover, the results of tumorsphere formation, CCK-8, and EdU assays further verified the inhibitory role of GAS5 overexpression in GSC proliferation (Figures 3C–E). All these data revealed that GAS5 overexpression impaired the stemness and proliferation of GSCs.
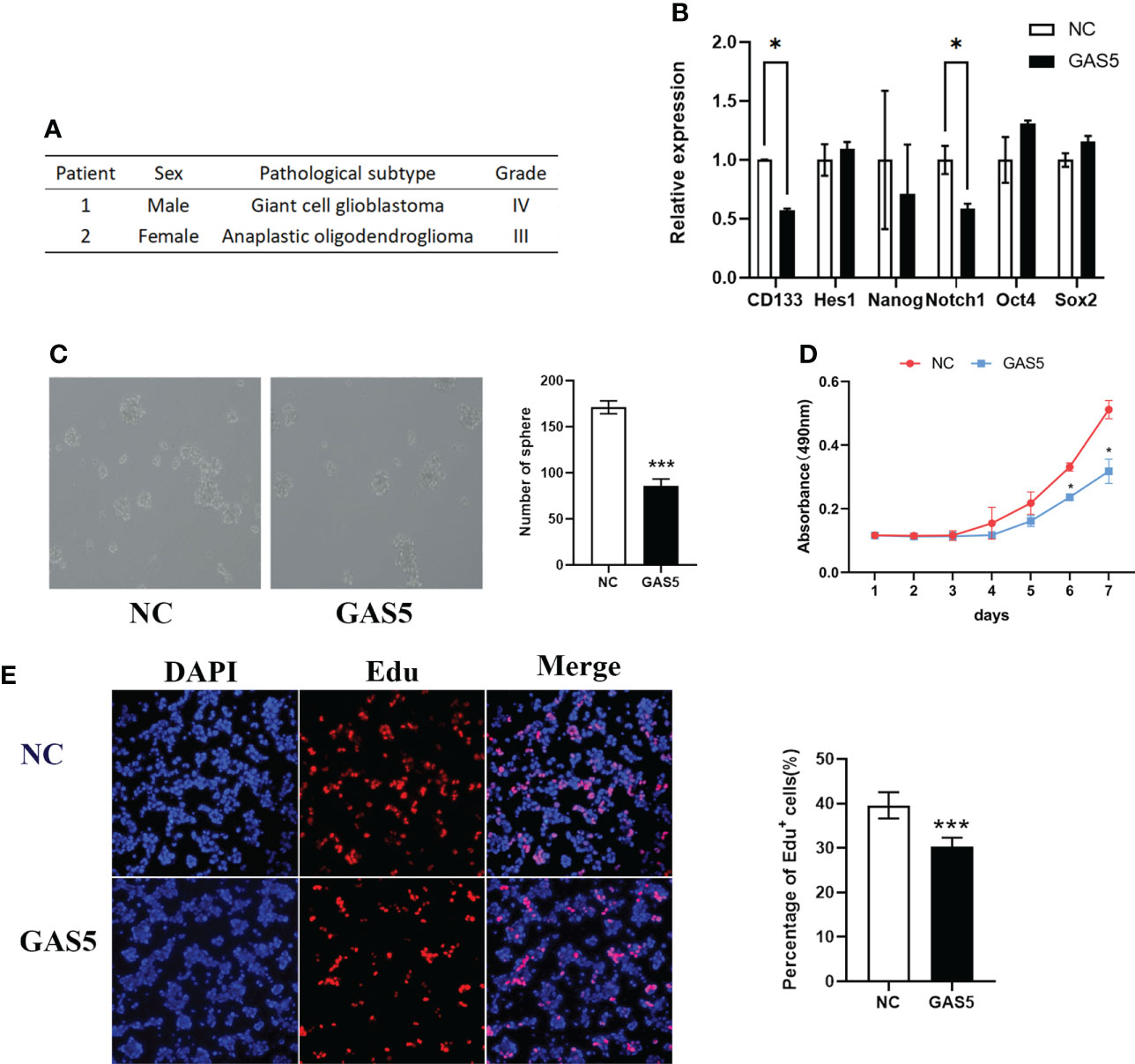
Figure 3 LncRNA-GAS5 over-expression impairs the stemness and proliferation of GSCs. (A) Information from the two clinical specimens we used to induce glioma stem cells. (B) qRT-PCR analysis of the expression of several stem cell marker molecules in GAS5 overexpression and parental GSCs (n=3). GAPDH is used as a reference gene. (C) Tumor spheres formation assay showed the upregulation of GAS5 affected the number and size of tumorspheres (n=3). (D) CCK-8 assay revealed the effect of GAS5 overexpression on the proliferation of GSCs with the cells are cultured for seven consecutive days and the absorbance of the cells are measured daily (n=6). (E) EdU assay showed the effect of GAS5 overexpression on DNA replication activity of GSC (n = 3). Data are presented as the mean ± S.D. P value is determined by Student’s t test (*P < 0.05, ***P < 0.001).
LncRNA-GAS5 restrained the proliferation and migration of GSCs through let-7e and miR-125a by modulating SPACA6
To clarify the molecular mechanism underlying the function of GAS5 in GSCs, we detected mRNAs that interacted with GAS5 in GSCs through an RNA antisense purification (RAP) assay and referred to the enrichment level and function of the gene (Supplementary Table 4). The results identified ALCAM, DDK6, GABRB3, MAPK3, MYSM1, RFWD, SPACA6, and STX6 as candidate genes that interact with GAS5, which were then subjected to validation by qRT-PCR via GAS5-overexpressing GCS. The results indicate that GAS5 substantially promoted SPACA6 expression (Figure 4A). Considering that SPACA6 is the host gene for let-7e, miR-125a, and miR-99b (https://www.ncbi.nlm.nih.gov/gene/406910), we quantified let-7e, miR-125a, and miR-99b in GAS5-overexpressing GSCs and found that let-7e and miR-125a were upregulated by GAS5 (Figure 4B). To further detect the correlation between the expression of SPACA6, let-7e, miR-125a, and GAS5, we evaluated these genes in healthy glial cells, GBM cells, and GSCs. The results indicated that the expression levels of SPACA6, let-7e, and miR-125a in GBM cells and GSCs were lower than that in normal glial cells, and the decrease in GSCs was more significant (Figure 4C). To clarify whether let-7e and miR-125a could act as anti-oncogenes, we transfected GSCs with mimics of these two miRNAs. The stemness properties of GSCs were verified by assessing their ability to form tumorspheres, and the results showed that let-7e and miR-125a mimics inhibited sphere formation (Figure 4D). Moreover, the CCK-8 assay results showed that let-7e and miR-125a repressed GSCs proliferation (Figure 4E). A transwell assay was also performed to evaluate the migration of glioma cells, which indicated that let-7e and miR-125a inhibited glioma cell invasion (Figure 4F). These results showed that let-7e and miR-125a inhibited the stemness, proliferation, and migration of GSCs, which was likely accomplished by the modulation of SPACA6.
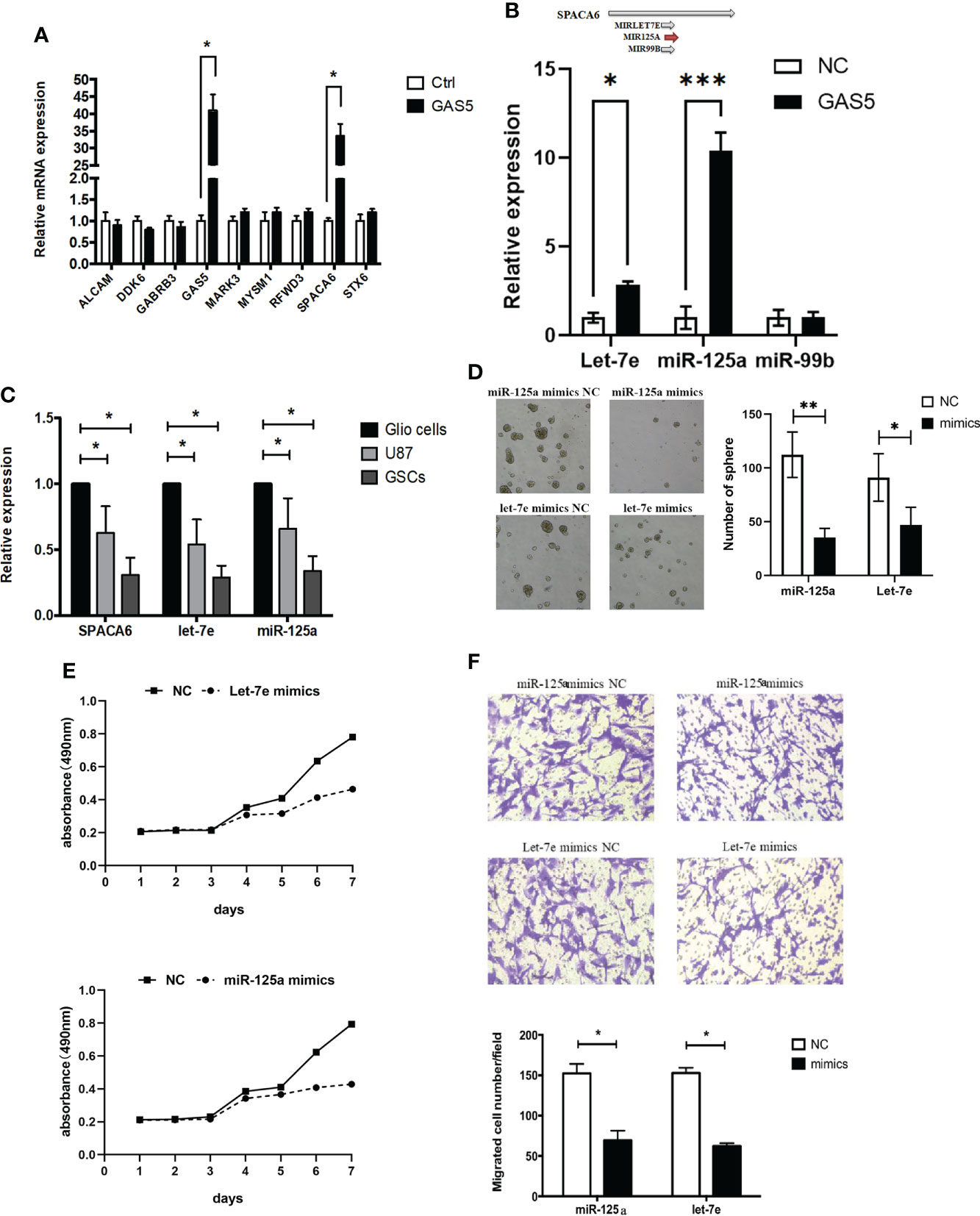
Figure 4 LncRNA-GAS5 restrains the proliferation and migration of GSCs through Let-7e and miR-125a by modulating SPACA6. (A) qRT-PCR analysis of the expression of several genes that are significant in the RAP assay in GAS5 overexpression and parental GSCs (n=3). GAPDH is used as a reference gene. (B) Upper: Three miRNAs with SPACA6 as host gene (data from https://www.ncbi.nlm.nih.gov/gene/406910). Lower: qRT-PCR analysis of the expression of let-7e, miR-125a and miR-99b in GAS5 overexpression and parental GSCs (n=3). GAPDH is used as a reference gene. (C) qRT-PCR analysis of the expression of SPACA6, let-7e and miR-125a respectively in glial cells, GBM cells and GSCs (n=3). GAPDH is used as a reference gene. (D) Tumor spheres formation assay showed miR-125a and let-7e mimics affected the number and size of tumorspheres (n=3). (E) CCK-8 assay revealed the effect of miR-125a and let-7e mimics on the proliferation of GSCs with the cells are cultured for seven consecutive days and the absorbance of the cells are measured daily (n=6). (F) Transwell assay of the effect of miR-125a and let-7e mimics on the migration and invasion of GSCs (n=3). Representative images and the migration cell number are shown. Data are presented as the mean ± S.D. P value is determined by Student’s t test (*P < 0.05, **P < 0.01, ***P < 0.001).
Let-7e and miR-125a suppressed the expression of the IL-6/IL-6R axis
Bioinformatics analysis using starBase v2.0 predicted that let-7e and miR-125a shared the same downstream target gene, IL-6R (Figure 5A). It has been reported that IL-6 signaling blocks GSC survival through activating STAT3 signaling (34–36). Therefore, we hypothesized that IL-6/IL-6R might play a role in regulating tumor suppressor genes in GBMs cells through a common downstream molecular mechanism. Therefore, we treated U87 cells with mimics of let-7e and miR-125a. The qRT-PCR and western blotting results showed that let-7e inhibited the expression of IL-6 and IL-6R simultaneously, while miR-125a inhibited the expression of IL-6R (Figures 5B, C). A luciferase reporter assay was performed to determine whether IL-6 and IL-6R are indeed targets of let-7e and miR-125a, through the 3’ UTR region. The results revealed that upregulation of let-7e resulted in deactivation of the 3’ UTR of IL6 and IL-6R, while the overexpression of miR-125a also decreased 3’ UTR activation of IL-6 (Figure 5D). These results indicate that let-7e exerts its biological effect by directly binding to the 3’ UTR of IL6, IL6R, and miR-125a.
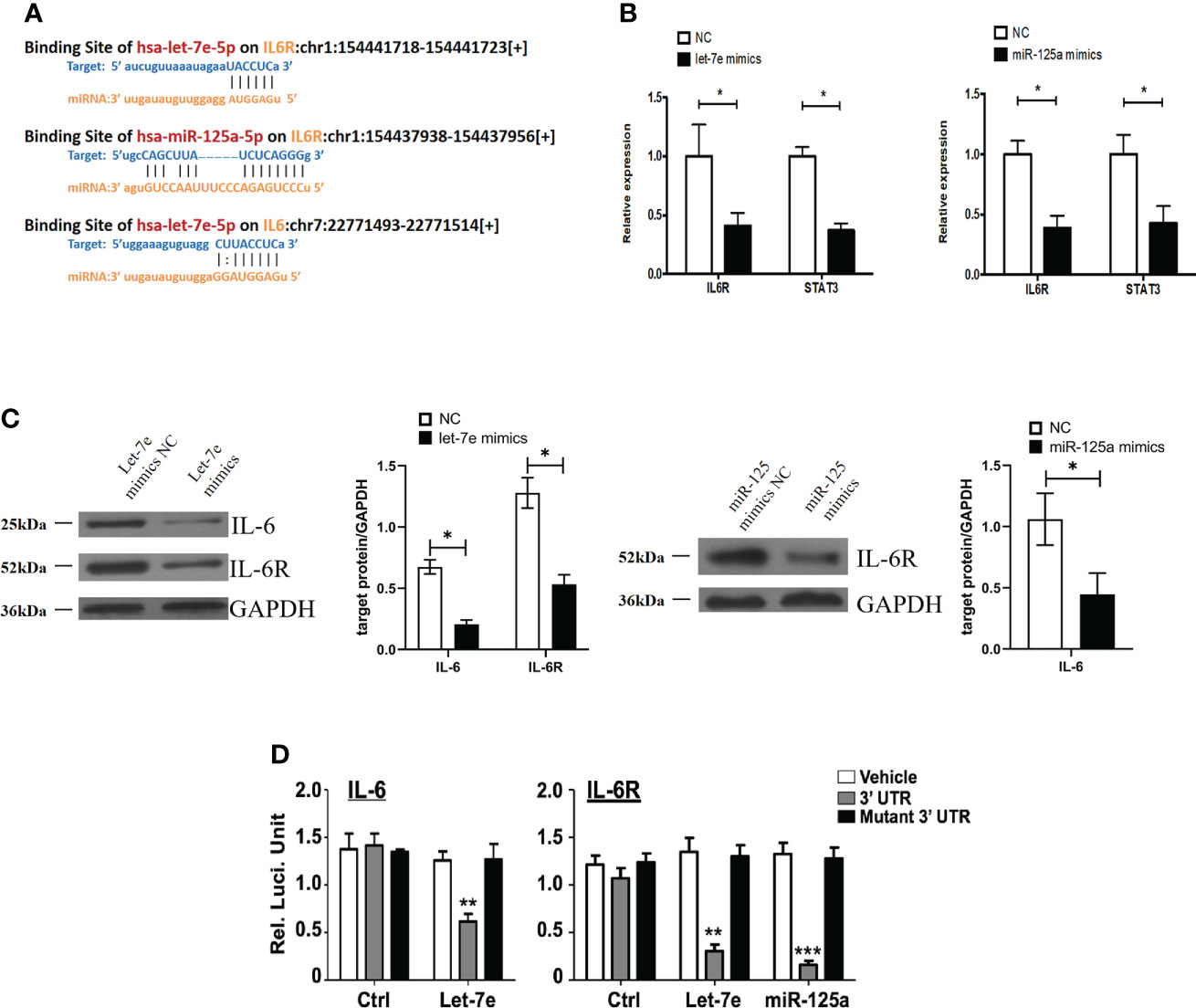
Figure 5 Let-7e inhibited the expression of IL-6 and IL-6R and miR-125a inhibited the expression of IL-6R. (A) Diagrams of binding side of let-7e and miR-125a matched the IL6 and IL6R gene. (B) Let-7e/miR-125a mimics are co-transfected into the U87 cells respectively, and then the expression of IL-6R is assessed by qRT-PCR analysis (n=3). GAPDH is used as a reference gene. (C) Western blot analysis of IL-6 and IL-6R proteins in U87 cells transfected with let-7e and miR-125a mimics (n=3). Quantitative results of western blot analysis are shown. (D) Let-7e and miR-125a mimics and reporter plasmids containing the wild type or mutant form of IL-6 3’UTR and IL-6R 3’UTR are co-transfected into the U87 cells, and then the luciferase activity is assessed after 48 h transfection. Expression of IL-6 and IL-6R are shown. Data are presented as the mean ± S.D. P value is determined by Student’s t test (*P < 0.05, **P < 0.01, ***P < 0.001).
LncRNA-GAS5 plays an antioncogenic role in GBM with the involvement of let-7e and miR-125a
To investigate the effect of let-7e and miR-125a on GAS5-overexpressing GSCs, a rescue experiment was performed. The results revealed that, after overexpression of GAS5, cellular viability remarkably diminished (Figure 6A). However, let-7e and miR-125a inhibitors markedly increased the proliferation of GSCs after GAS5 overexpression (Figure 6A). Additionally, when let-7e and miR-125a inhibitors were used simultaneously, the restorative effect on cell viability was stronger (Figure 6A). The effects of GAS5, let-7e, and miR-125 on tumor cell growth in vivo were verified in nude mice after injection of GAS5 overexpressing U87 cells subcutaneously or stereotactically into the brain. Compared to the control group, the tumorigenic ability of GAS5-overexpressing U87 cells in vivo was considerably reduced. Furthermore, the anti-tumor ability of GAS5-overexpression could be inhibited partly by let-7e and miR-125a inhibitors, individually or jointly, and the effect of the let-7e inhibitor was stronger than that of the miR-125a inhibitor (Figures 6B, C). To further explore the downstream molecular mechanisms of GAS5, we used tumor specimens from the aforementioned nude mice for validation. The western blotting results showed that the expression of IL6, IL6R, and p-STAT3 could be downregulated by GAS5-overexpression. Moreover, the expression of IL6R and p-STAT3 downregulated by GAS5-overexpression was elevated by let-7e and miR-125a inhibitors, and the expression of IL6 that was downregulated by GAS5 overexpression was elevated by a let-7e inhibitor (Figure 6D). These data indicate that lncRNA-GAS5 plays an anti-oncogenic role in GBM via the let-7e/miR-125a-IL-6/IL-6R/STAT3 axis.
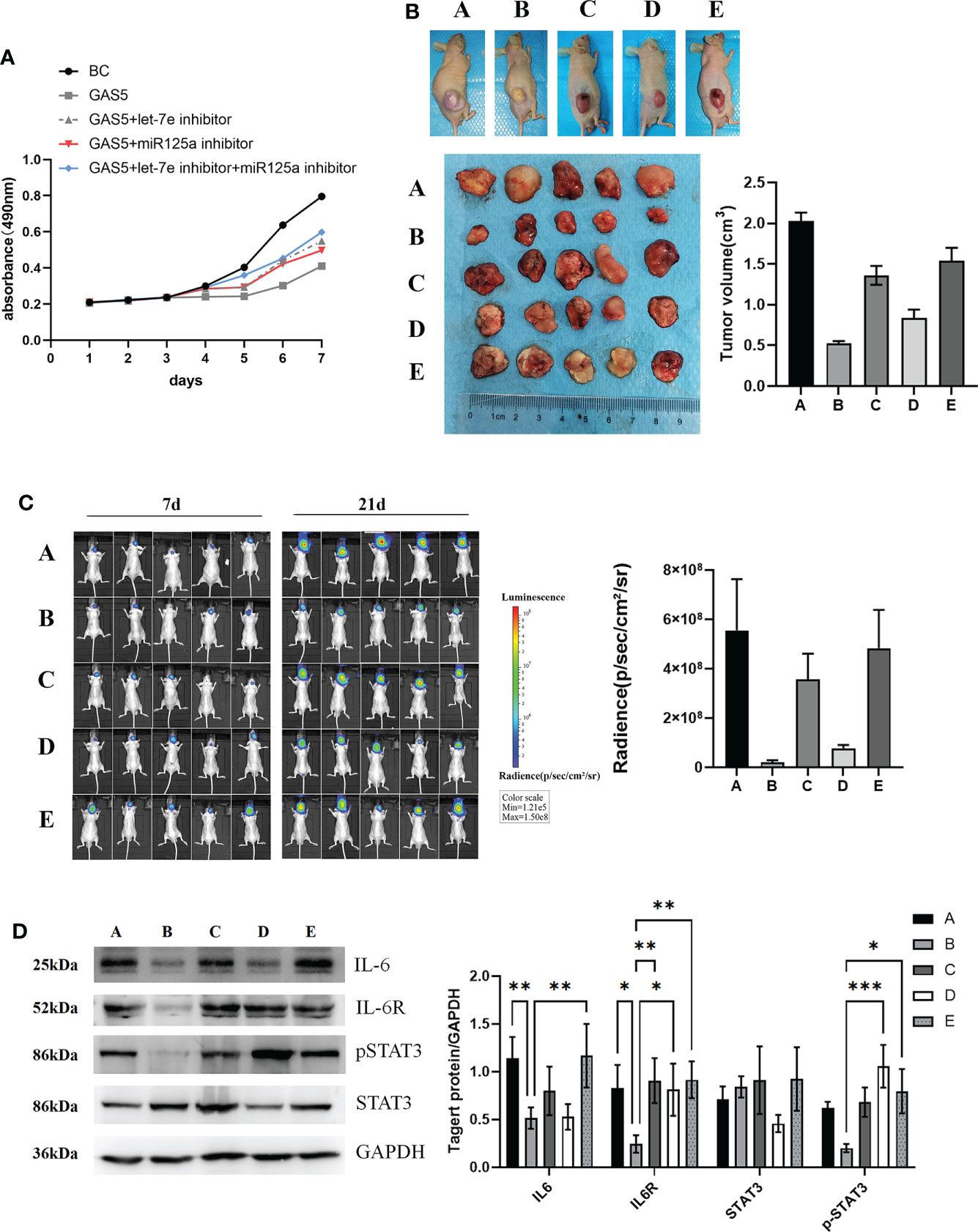
Figure 6 LncRNA-GAS5 plays the role of antioncogene in GBM with the involvement of let-7e and miR-125a. (A) Supernatant rescue assay showed the results of CCK-8 assay analysis of the effect of let-7e inhibitor/miR-125a inhibitor transfected on the GAS5 overexpression U87 cells (n=3). (B) U87 cells are transfected with GAS5 overexpression lentivirus, miR-125a inhibitor and let-7e inhibitor individually or jointly and they are injected subcutaneously (5×106 cells) into the nude mice (n=5/group). Five images of the subcutaneous tumor of nude mice and the tumor volume are shwon (tumor volume=0.5×l×l×w, l=tumor length, w=tumor width). (a: Control, b: GAS5 overexpression, c: GAS5 overexpression+let-7e inhibitor, d: GAS5 overexpression+miR125a inhibitor, e: GAS5 overexpression+let-7e inhibitor+miR125a inhibitor) (C) U87 cells are transfected with GAS5 overexpression lentivirus, miR-125a inhibitor and let-7e inhibitor individually or jointly and they are injected stereotactically into the brain (5×106 cells) into the nude mice (n=5/group). The bioluminescence imaging assay is used to detect the excitation fluorescence in the brain tumor of the nude mice and the relative photons flux is estimated. (a: Control, b: GAS5 overexpression, c: GAS5 overexpression+let-7e inhibitor, d: GAS5 overexpression+miR125a inhibitor, e: GAS5 overexpression+let-7e inhibitor+miR125a inhibitor) (D) Proteins are extracted from the nude mice tumors tissues that we formed subcutaneously before. IL-6, IL-6R, STAT3, pSTAT3 are determined by western blot analysis (n=3). Quantitative results of western blot analysis are shown. Data are presented as the mean ± S.D. P value is determined by Student’s t test (*P < 0.05, **P < 0.01, ***P < 0.001).
Discussion
The latest WHO classification of CNS tumors has updated molecular biomarkers based on tumor morphology to refine the CNS tumor classification (6). ncRNAs are also involved in several cancer-associated processes and are closely related to tumors prognosis (11, 37, 38). GSCs display striking cellular heterogeneity, which is a major contributor to the poor prognosis of patients with glioblastoma (39). Therefore, a better understanding of the molecular mechanisms of GSCs may provide new insights into GBM treatment. The current study revealed that GAS5 suppressed glioma cell growth, both in vitro and in vivo. We also found that GAS5 positively controls SPACA6, which targets two major microgenes, let-7e and miR-125, to regulate overlapping oncogenic properties in GSCs, of which the inhibition of the downstream IL-6/IL-6R/STAT3 pathway is involved in the molecular mechanism of GAS5 as an antioncogene.
In recent years, the role of GAS5 in tumorigenesis and progression has attracted attention in a wide variety of malignancies, and GAS5 has been discovered to affect various biological processes in tumors. For example, in pancreatic cancer, GAS5 functions as a competing endogenous RNA for miR-221 to suppress cell growth, metastasis, and gemcitabine resistance by mediating epithelial-mesenchymal transformation (EMT) and tumor stem cell self-renewal (40). Liu et al. proved that in glioma, exon 2 of GAS5 directly binds to the miR-18a-5p-binding site to negatively regulate miR-18a-5p, which facilitates the tumor-suppressor functions of GAS5 (41). In this study, we also found that downregulation of GAS5 in glioma could predict poor survival, and GAS5 overexpression could facilitate the migration and invasion of GBM cells and decrease cell viability and the antitumor effect of GAS5 in nude mice. LncRNAs can be secreted extracellularly in the form of exosomes, which makes lncRNAs in body fluids potentially useful as biomarkers to indicate tumor progression and malignancy and guides personalized treatment (25). Our study strongly corroborates the potential of GAS5 as a therapeutic target and clinical predictor of glioma.
Deregulation of ncRNAs has been linked to almost every cancer investigated to date and affects almost all major cancer hallmarks (42, 43). Elaborating the mechanism and crosstalk underlying ncRNAs will provide a deeper understanding of molecular therapeutic strategies and possible mechanisms of glioma pathogenesis. Compared to other types of RNA, lncRNAs have no major molecular prototypes and mainly interact with DNA or RNA in the form of modular domains by nucleic acid base pairing or with proteins via advanced RNA structures (44). One of the working mechanisms of lncRNAs that is widely acknowledged is that lncRNAs act as competitive endogenous RNA (competing for endogenous RNA, ceRNA) to competitively bind microRNAs, and the binding site of ceRNAs to mRNA partially relieves the negative regulatory effect of the corresponding miRNA on its target mRNA (45). Emerging evidence has indicated that GAS5 and other lncRNAs could sponge miRNAs to repress their expression and further suppress their downstream pathways, either in glioma or other cancers (32, 40, 41, 46–48). Nevertheless, we found that GAS5 interacts with SPACA6 mRNA and stabilizes its expression to improve the expression levels of let-7e and miR-125a (49). This conclusion could be used to supplement our understanding of how GAS5 acts as a ceRNA in gliomas. LncRNAs could perform their downstream biological functions by combining with mRNA transcripts, and the RAP assay results also suggested that GAS5 and SPACA6 could interact with each other. In addition, our investigation revealed that let-7e and miR-125 individually and synergistically function in tumor suppression in gliomas by blocking their downstream pathways, which adds new evidence to our speculation that GAS5 could protect SPACA6 from degradation by combining with it to act as an anti-oncogene. The finding that let-7e and miR-125a act as suppressors of the IL-6/IL-6R/STAT3 pathway also verified the repression function of the miRNAs in gene expression. In general, the regulatory mechanism proposed in our study enriched and verified the regulatory mechanism of ncRNAs and provided new strategies and targets for ncRNA-targeting glioma therapy.
Glioblastomas contain a specialized subgroup of tumor cells, called GSCs (21). Since the stemness of GSCs plays an essential role in the progression of GBM (7). Zhao et al. found that the GAS5/miR-196a-5p/FOXO1 axis inhibits GSC proliferation, migration, and invasion (48). Our study also revealed that GAS5 overexpression inhibited GSC proliferation, migration, and invasion, and promoted apoptosis by upregulating SPACA6 and its downstream miR-125a/let-7e pathways. Moreover, our study was the first to show that GAS5 can affect the stemness of glioma cells. The stemness of GSCs can be adjusted by epithelial-mesenchymal transition (EMT) (50), growth factor receptor variant III (EGFRvIII) (51), and certain miRNAs, such as miR-205, miR-103a-3p, and miR30 (52–54). It has also been reported that IL-6 signaling disturbs GSC survival through STAT3 activation (35). Cortez et al. suggested that miR-29b and miR-125a are the potential regulators of invasion in glioblastoma. Hence, we clarified that let-7e inhibited the expression of IL-6 and IL-6R simultaneously, and miR-125a inhibited the expression of IL-6R. LncRNA-GAS5 acts as an antioncogene in GSCs via the IL-6/IL-6R/STAT3 pathway. The let-7 gene is an essential developmental gene and let-7-family members many function as tumor suppressors in various cancers (55). Let-7e was previously identified to be downregulated by nuclear paraspeckle assembly transcript 1 (NEAT1) to promote oncogenesis in GSCs (56). In this study, however, we found that let-7e could inhibit GSC progression, which might relate different upstream and downstream miRNA regulatory crosstalk mechanisms. As a member of the lin-4 family of microRNAs, miR-125a plays a fundamental role in cell differentiation and development (57), and the reduction of the malignant progression of GSCs by downregulated miR-125a has not yet been reported. Our findings complement the mechanism by which ncRNAs act in the formation and progression of GSCs. However, to fully reveal the subcellular localization of GAS5 in glioma cells and further define the underlying mechanism by which GAS5 interacts directly with proteins, additional evaluations are warranted.
Conclusion
In summary, our study verified that the under-expression of GAS5 was related to a higher degree of malignancy and shorter survival time after surgery. GAS5 negatively regulates the malignant behavior of glioma cells and GSCs. Notably, GAS5 upregulated the expression of let-7e and miR-125a by reducing SPACA6 degradation and further repressing activation of the downstream IL6/IL-6R/STAT3 pathway. However, the complete elucidation of the underlying mechanism requires more evidence, and it will be important to determine whether GAS5 can directly bind to SPACA6 to prevent its degradation and affect downstream pathways.
Data Availability Statement
The original contributions presented in the study are included in the article/Supplementary Material. Further inquiries can be directed to the corresponding authors.
Ethics Statement
The studies involving human participants were reviewed and approved by Research Ethics Committee of Xijing Hospital of Airforce Medical University. The patients/participants provided their written informed consent to participate in this study. The animal study was reviewed and approved by Animal Experiment Administration Committee of the Air Force Military Medical University.
Author contributions
SZL, ZF and JLZ designed research and wrote the manuscript, SW, KXR, JZ, JL, BJ, XQW, YND, TTW and WHL performed experiments, XWF, YH and XH analyzed data, LW, YGW and ZF provided clinical samples, SZL and SW interpreted results of experiments and write the manuscript. All authors reviewed and approved the manuscript.
Funding
This study was supported by grants from National Natural Science Foundation of Shaanxi (2017JM8073).
Conflict of interest
The authors declare that the research was conducted in the absence of any commercial or financial relationships that could be construed as a potential conflict of interest.
Publisher’s note
All claims expressed in this article are solely those of the authors and do not necessarily represent those of their affiliated organizations, or those of the publisher, the editors and the reviewers. Any product that may be evaluated in this article, or claim that may be made by its manufacturer, is not guaranteed or endorsed by the publisher.
Supplementary material
The Supplementary Material for this article can be found online at: https://www.frontiersin.org/articles/10.3389/fonc.2022.803652/full#supplementary-material
References
1. Stupp R, Hegi ME, Mason WP, van den Bent MJ, Taphoorn MJB, Janzer RC, et al. Effects of radiotherapy with concomitant and adjuvant temozolomide versus radiotherapy alone on survival in glioblastoma in a randomised phase III study: 5-year analysis of the EORTC-NCIC trial. Lancet Oncol (2009) 10(5):459–66. doi: 10.1016/s1470-2045(09)70025-7
2. Lapointe S, Perry A, Butowski NA. Primary brain tumours in adults. Lancet (2018) 392(10145):432–46. doi: 10.1016/s0140-6736(18)30990-5
3. Van Meir EG, Hadjipanayis CG, Norden AD, Shu HK, Wen PY, Olson JJ. Exciting new advances in neuro-oncology: the avenue to a cure for malignant glioma. CA Cancer J Clin (2010) 60(3):166–93. doi: 10.3322/caac.20069
4. Maher EA, Furnari FB, Bachoo RM, Rowitch DH, Louis DN, Cavenee WK, et al. Malignant glioma: genetics and biology of a grave matter. Genes Dev (2001) 15(11):1311–33. doi: 10.1101/gad.891601
5. Louis DN, Perry A, Wesseling P, Brat DJ, Cree IA, Figarella-Branger D, et al. The 2021 WHO classification of tumors of the central nervous system: a summary. Neuro Oncol (2021) 23(8):1231–51. doi: 10.1093/neuonc/noab106
6. Louis DN, Perry A, Reifenberger G, von Deimling A, Figarella-Branger D, Cavenee WK, et al. The 2016 world health organization classification of tumors of the central nervous system: a summary. Acta Neuropathol (2016) 131(6):803–20. doi: 10.1007/s00401-016-1545-1
7. Prager BC, Bhargava S, Mahadev V, Hubert CG, Rich JN. Glioblastoma stem cells: Driving resilience through chaos. Trends Cancer (2020) 6(3):223–35. doi: 10.1016/j.trecan.2020.01.009
8. Matsui M, Corey DR. Non-coding RNAs as drug targets. Nat Rev Drug Discovery (2017) 16(3):167–79. doi: 10.1038/nrd.2016.117
9. Peng Z, Liu C, Wu M. New insights into long noncoding RNAs and their roles in glioma. Mol Cancer (2018) 17(1):61. doi: 10.1186/s12943-018-0812-2
10. Willingham AT, Orth AP, Batalov S, Peters EC, Wen BG, Aza-Blanc P, et al. A strategy for probing the function of noncoding RNAs finds a repressor of NFAT. Science (2005) 309(5740):1570–3. doi: 10.1126/science.1115901
11. Lee S, Kopp F, Chang TC, Sataluri A, Chen B, Sivakumar S, et al. Noncoding RNA NORAD regulates genomic stability by sequestering PUMILIO proteins. Cell (2016) 164(1-2):69–80. doi: 10.1016/j.cell.2015.12.017
12. Dinger ME, Pang KC, Mercer TR, Mattick JS. Differentiating protein-coding and noncoding RNA: challenges and ambiguities. PLoS Comput Biol (2008) 4(11):e1000176. doi: 10.1371/journal.pcbi.1000176
13. Tripathi V, Ellis JD, Shen Z, Song DY, Pan Q, Watt AT, et al. The nuclear-retained noncoding RNA MALAT1 regulates alternative splicing by modulating SR splicing factor phosphorylation. Mol Cell (2010) 39(6):925–38. doi: 10.1016/j.molcel.2010.08.011
14. Pacini G, Dunkel I, Mages N, Mutzel V, Timmermann B, Marsico A, et al. Integrated analysis of xist upregulation and X-chromosome inactivation with single-cell and single-allele resolution. Nat Commun (2021) 12(1):3638. doi: 10.1038/s41467-021-23643-6
15. Shahabi S, Kumaran V, Castillo J, Cong Z, Nandagopal G, Mullen DJ, et al. LINC00261 is an epigenetically regulated tumor suppressor essential for activation of the DNA damage response. Cancer Res (2019) 79(12):3050–62. doi: 10.1158/0008-5472.CAN-18-2034
16. Wang YQ, Jiang DM, Hu SS, Zhao L, Wang L, Yang MH, et al. SATB2-AS1 suppresses colorectal carcinoma aggressiveness by inhibiting SATB2-dependent snail transcription and epithelial-mesenchymal transition. Cancer Res (2019) 79(14):3542–56. doi: 10.1158/0008-5472.CAN-18-2900
17. Wu P, Cai J, Chen Q, Han B, Meng X, Li Y, et al. Lnc-TALC promotes O(6)-methylguanine-DNA methyltransferase expression via regulating the c-met pathway by competitively binding with miR-20b-3p. Nat Commun (2019) 10(1):2045. doi: 10.1038/s41467-019-10025-2
18. Rezaei O, Honarmand K, Nateghinia S, Taheri M, Ghafouri-Fard S. miRNA signature in glioblastoma: Potential biomarkers and therapeutic targets. Exp Mol Pathol (2020) 117:104550. doi: 10.1016/j.yexmp.2020.104550
19. Cesana M, Cacchiarelli D, Legnini I, Santini T, Sthandier O, Chinappi M, et al. A long noncoding RNA controls muscle differentiation by functioning as a competing endogenous RNA. Cell (2011) 147(2):358–69. doi: 10.1016/j.cell.2011.09.028
20. Katsushima K, Natsume A, Ohka F, Shinjo K, Hatanaka A, Ichimura N, et al. Targeting the notch-regulated non-coding RNA TUG1 for glioma treatment. Nat Commun (2016) 7:13616. doi: 10.1038/ncomms13616
21. Yao Y, Ma J, Xue Y, Wang P, Li Z, Liu J, et al. Knockdown of long non-coding RNA XIST exerts tumor-suppressive functions in human glioblastoma stem cells by up-regulating miR-152. Cancer Lett (2015) 359(1):75–86. doi: 10.1016/j.canlet.2014.12.051
22. Zhang S, Zhao BS, Zhou A, Lin K, Zheng S, Lu Z, et al. m(6)A demethylase ALKBH5 maintains tumorigenicity of glioblastoma stem-like cells by sustaining FOXM1 expression and cell proliferation program. Cancer Cell (2017) 31(4):591–606 e6. doi: 10.1016/j.ccell.2017.02.013
23. Long X, Song K, Hu H, Tian Q, Wang W, Dong Q, et al. Long non-coding RNA GAS5 inhibits DDP-resistance and tumor progression of epithelial ovarian cancer v ia GAS5-E2F4-PARP1-MAPK axis. J Exp Clin Cancer Res (2019) 38(1):345. doi: 10.1186/s13046-019-1329-2
24. Yang W, Xu X, Hong L, Wang Q, Huang J, Jiang L. Upregulation of lncRNA GAS5 inhibits the growth and metastasis of cervical cancer cells. J Cell Physiol (2019) 234(12):23571–80. doi: 10.1002/jcp.28926
25. Li C, Lv Y, Shao C, Chen C, Zhang T, Wei Y, et al. Tumor-derived exosomal lncRNA GAS5 as a biomarker for early-stage non-small-cell lung cancer diagnosis. J Cell Physiol (2019) 234(11):20721–7. doi: 10.1002/jcp.28678
26. Jin C, Zhao J, Zhang ZP, Wu M, Li J, Xiao GL, et al. Long non-coding RNA GAS5, by up-regulating PRC2 and targeting the promoter methylation of miR-424, suppresses multiple malignant phenotypes of glioma. J Neurooncol (2020) 148(3):529–43. doi: 10.1007/s11060-020-03544-2
27. Al Mourgi M, El Askary A, Gharib AF, Alzahrani R, Banjer HJ, Elsawy WH, et al. Circulating long non-coding RNA GAS5: A non-invasive molecular marker for prognosis, response to treatment and survival in non-small cell lung cancer. Cancer Invest (2021) 39(6-7):505–13. doi: 10.1080/07357907.2021.1928167
28. Ni W, Yao S, Zhou Y, Liu Y, Huang P, Zhou A, et al. Long noncoding RNA GAS5 inhibits progression of colorectal cancer by interacting with and triggering YAP phosphorylation and degradation and is negatively regulated by the m(6)A reader YTHDF3. Mol Cancer (2019) 18(1):143. doi: 10.1186/s12943-019-1079-y
29. Wang X, Li FY, Zhao W, Gao ZK, Shen B, Xu H, et al. Long non-coding RNA GAS5 overexpression inhibits M2-like polarization of tumour-associated macrophages in SMCC-7721 cells by promoting PTEN expression. Int J Exp Pathol (2020) 101(6):215–22. doi: 10.1111/iep.12374
30. Ding Y, Wang J, Zhang H, Li H. Long noncoding RNA-GAS5 attenuates progression of glioma by eliminating microRNA-10b and sirtuin 1 in U251 and A172 cells. Biofactors (2020) 46(3):487–96. doi: 10.1002/biof.1604
31. Engreitz J, Lander ES, Guttman M. RNA Antisense purification (RAP) for mapping RNA interactions with chromatin. Methods Mol Biol (2015) 1262:183–97. doi: 10.1007/978-1-4939-2253-6_11
32. Tan X, Jiang H, Fang Y, Han D, Guo Y, Wang X, et al. The essential role of long non-coding RNA GAS5 in glioma: interaction with microRNAs, chemosensitivity and potential as a biomarker. J Cancer (2021) 12(1):224–31. doi: 10.7150/jca.49203
33. Zhu XP, Pan SA, Chu Z, Zhou YX, Huang YK, Han DQ. LncRNA GAS5 regulates epithelial-mesenchymal transition and viability of glioma cells by targeting microRNA-106b and regulating PTEN expression. Neurosci Res (2021) 170:32–40. doi: 10.1016/j.neures.2020.08.009
34. Yao Y, Ye H, Qi Z, Mo L, Yue Q, Baral A, et al. B7-H4(B7x)-Mediated cross-talk between glioma-initiating cells and macrophages via the IL6/JAK/STAT3 pathway lead to poor prognosis in glioma patients. Clin Cancer Res (2016) 22(11):2778–90. doi: 10.1158/1078-0432.CCR-15-0858
35. Wang Q, Jia S, Wang D, Chen X, Kalvakolanu DV, Zheng H, et al. A combination of BRD4 and HDAC3 inhibitors synergistically suppresses glioma stem cell growth by blocking GLI1/IL6/STAT3 signaling axis. Mol Cancer Ther (2020) 19(12):2542–53. doi: 10.1158/1535-7163.MCT-20-0037
36. Zang J, Zheng MH, Cao XL, Zhang YZ, Zhang YF, Gao XY, et al. Adenovirus infection promotes the formation of glioma stem cells from glioblastoma cells through the TLR9/NEAT1/STAT3 pathway. Cell Commun Signal (2020) 18(1):135. doi: 10.1186/s12964-020-00598-7
37. Lan Y, Xiao X, He Z, Luo Y, Wu C, Li L, et al. Long noncoding RNA OCC-1 suppresses cell growth through destabilizing HuR protein in colorectal cancer. Nucleic Acids Res (2018) 46(11):5809–21. doi: 10.1093/nar/gky214
38. Ali T, Grote P. Beyond the RNA-dependent function of LncRNA genes. Elife (2020) 9:1025–36. doi: 10.7554/eLife.60583
39. Bhaduri A, Di Lullo E, Jung D, Muller S, Crouch EE, Espinosa CS, et al. Outer radial glia-like cancer stem cells contribute to heterogeneity of glioblastoma. Cell Stem Cell (2020) 26(1):48–63 e6. doi: 10.1016/j.stem.2019.11.015
40. Liu B, Wu S, Ma J, Yan S, Xiao Z, Wan L, et al. lncRNA GAS5 reverses EMT and tumor stem cell-mediated gemcitabine resistance and metastasis by target ing miR-221/SOCS3 in pancreatic cancer. Mol Ther Nucleic Acids (2018) 13:472–82. doi: 10.1016/j.omtn.2018.09.026
41. Li G, Cai Y, Wang C, Huang M, Chen J. LncRNA GAS5 regulates the proliferation, migration, invasion and apoptosis of brain glioma cells thro ugh targeting GSTM3 expression. the effect of LncRNA GAS5 on glioma cells. J Neurooncol (2019) 143(3):525–36. doi: 10.1007/s11060-019-03185-0
42. Calin GA, Croce CM. MicroRNA-cancer connection: the beginning of a new tale. Cancer Res (2006) 66(15):7390–4. doi: 10.1158/0008-5472.CAN-06-0800
43. Winkle M, El-Daly SM, Fabbri M, Calin GA. Noncoding RNA therapeutics - challenges and potential solutions. Nat Rev Drug Discovery (2021) 20(8):629–51. doi: 10.1038/s41573-021-00219-z
44. Guttman M, Rinn JL. Modular regulatory principles of large non-coding RNAs. Nature (2012) 482(7385):339–46. doi: 10.1038/nature10887
45. Salmena L, Poliseno L, Tay Y, Kats L, Pandolfi PP. A ceRNA hypothesis: the Rosetta stone of a hidden RNA language? Cell (2011) 146(3):353–8. doi: 10.1016/j.cell.2011.07.014
46. Gao ZQ, Wang JF, Chen DH, Ma XS, Yang W, Zhe T, et al. Long non-coding RNA GAS5 antagonizes the chemoresistance of pancreatic cancer cells through down-regulation of miR-181c-5p. BioMed Pharmacother (2018) 97:809–17. doi: 10.1016/j.biopha.2017.10.157
47. Zhao X, Wang P, Liu J, Zheng J, Liu Y, Chen J, et al. Gas5 exerts tumor-suppressive functions in human glioma cells by targeting miR-222. Mol Ther (2015) 23(12):1899–911. doi: 10.1038/mt.2015.170
48. Zhao X, Liu Y, Zheng J, Liu X, Chen J, Liu L, et al. GAS5 suppresses malignancy of human glioma stem cells via a miR-196a-5p/FOXO1 feedback loop. Biochim Biophys Acta Mol Cell Res (2017) 1864(10):1605–17. doi: 10.1016/j.bbamcr.2017.06.020
49. Kim A, Saikia P, Nagy LE. miRNAs involved in M1/M2 hyperpolarization are clustered and coordinately expressed in alcoholic hepatitis. Front Immunol (2019) 10:1295. doi: 10.3389/fimmu.2019.01295
50. Zhang J, Cai H, Sun L, Zhan P, Chen M, Zhang F, et al. LGR5, a novel functional glioma stem cell marker, promotes EMT by activating the wnt/beta-catenin pathway and predicts poor survival of glioma patients. J Exp Clin Cancer Res (2018) 37(1):225. doi: 10.1186/s13046-018-0864-6
51. Yin J, Park G, Kim TH, Hong JH, Kim YJ, Jin X, et al. Pigment epithelium-derived factor (PEDF) expression induced by EGFRvIII promotes self-renewal and tumor progression of glioma stem cells. PLoS Biol (2015) 13(5):e1002152. doi: 10.1371/journal.pbio.1002152
52. Huynh TT, Lin CM, Lee WH, Wu AT, Lin YK, Lin YF, et al. Pterostilbene suppressed irradiation-resistant glioma stem cells by modulating GRP78/miR-205 axis. J Nutr Biochem (2015) 26(5):466–75. doi: 10.1016/j.jnutbio.2014.11.015
53. Yu M, Xue Y, Zheng J, Liu X, Yu H, Liu L, et al. Linc00152 promotes malignant progression of glioma stem cells by regulating miR-103a-3p/FEZF1/CDC25A pathway. Mol Cancer (2017) 16(1):110. doi: 10.1186/s12943-017-0677-9
54. Che S, Sun T, Wang J, Jiao Y, Wang C, Meng Q, et al. miR-30 overexpression promotes glioma stem cells by regulating Jak/STAT3 signaling pathway. Tumour Biol (2015) 36(9):6805–11. doi: 10.1007/s13277-015-3400-8
55. Roush S, Slack FJ. The let-7 family of microRNAs. Trends Cell Biol (2008) 18(10):505–16. doi: 10.1016/j.tcb.2008.07.007
56. Gong W, Zheng J, Liu X, Ma J, Liu Y, Xue Y. Knockdown of NEAT1 restrained the malignant progression of glioma stem cells by activating microRNA let-7e. Oncotarget (2016) 7(38):62208–23. doi: 10.18632/oncotarget.11403
Keywords: Glioblastoma (GBM), growth arrest-specific transcript 5 (GAS5), stem cell, miR-125a, let-7e, IL6, STAT3.
Citation: Wu S, Ren K, Zhao J, Li J, Jia B, Wu X, Dou Y, Fei X, Huan Y, He X, Wang T, Lv W, Wang L, Wang Y, Zhao J, Fei Z and Li S (2022) LncRNA GAS5 represses stemness and malignancy of gliomas via elevating the SPACA6-miR-125a/let-7e Axis. Front. Oncol. 12:803652. doi: 10.3389/fonc.2022.803652
Received: 28 October 2021; Accepted: 03 August 2022;
Published: 29 August 2022.
Edited by:
Fred Lam, Massachusetts Institute of Technology, United StatesReviewed by:
Chenyu Lin, The Ohio State University, United StatesLi Maolan, Shanghai Jiao Tong University, China
Copyright © 2022 Wu, Ren, Zhao, Li, Jia, Wu, Dou, Fei, Huan, He, Wang, Lv, Wang, Wang, Zhao, Fei and Li. This is an open-access article distributed under the terms of the Creative Commons Attribution License (CC BY). The use, distribution or reproduction in other forums is permitted, provided the original author(s) and the copyright owner(s) are credited and that the original publication in this journal is cited, in accordance with accepted academic practice. No use, distribution or reproduction is permitted which does not comply with these terms.
*Correspondence: Sanzhong Li, c3VubnlfM2NAMTI2LmNvbQ==; Zhou Fei, ZmVpemhvdUBmbW11LmVkdS5jbg==; Junlong Zhao, YmlvX2p1bmxvbmd6aGFvQDE2My5jb20=
†These authors have contributed equally to this work and share first authorship