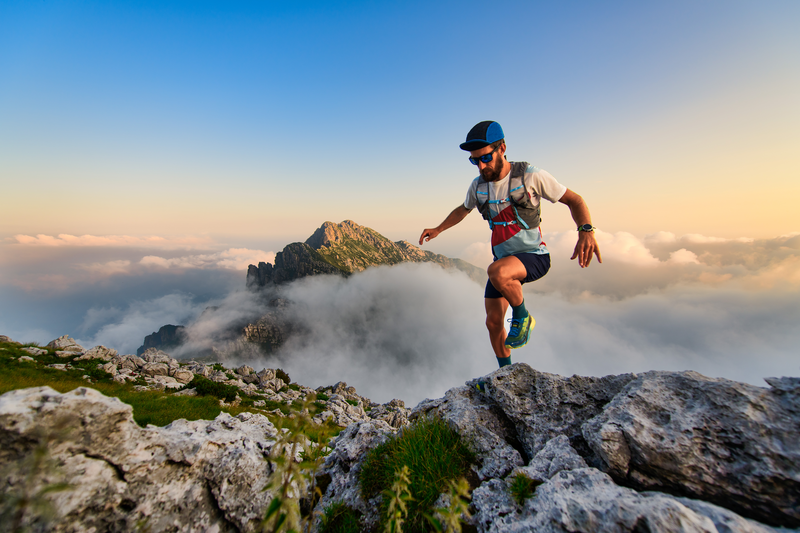
95% of researchers rate our articles as excellent or good
Learn more about the work of our research integrity team to safeguard the quality of each article we publish.
Find out more
REVIEW article
Front. Oncol. , 27 January 2022
Sec. Hematologic Malignancies
Volume 12 - 2022 | https://doi.org/10.3389/fonc.2022.789728
This article is part of the Research Topic Cellular and Molecular Mechanisms of Immune Checkpoint Blockers in Anti-Leukemia and Lymphoma Immune Therapy View all 6 articles
The development of immune checkpoint inhibitors, the monoclonal antibodies that modulate the interaction between immune checkpoint molecules or their ligands on the immune cells or tumor tissue has revolutionized cancer treatment. While there are various studies proving their efficacy in hematological malignancies, there is also a body of accumulating evidence indicating that immune checkpoint inhibitors’ clinical benefits are limited in such diseases. In addition, due to their regulatory nature that balances the immune responses, blockade of immune checkpoints may lead to toxic side effects and autoimmune responses, and even primary or acquired resistance mechanisms may restrict their success. Thus, the need for laboratory biomarkers to identify and monitor patient populations who are more likely respond to this type of therapy and the management of side effects seem critical. However, guidelines regarding the use of immune checkpoint inhibitors in hematological cancers and during follow-up are limited while there is no consensus on the laboratory parameters to be investigated for safety and efficacy of the treatment. This review aims to provide an insight into recent information on predictive and prognostic value of biomarkers and laboratory tests for the clinical follow up of hematological malignancies, with an emphasis on leukemia.
Therapeutic approaches targeting immunosuppressive features of the tumor microenvironment (TME) or cancer cells may reinforce antitumor immune responses in both solid and hematological malignancies. Up-regulation of inhibitory checkpoints on the surface of innate and acquired immune cells is considered as one of the important immunosuppressive features in most types of cancers (1). After the approval of cytotoxic T lymphocyte-associated molecule-4 (CTLA-4) inhibitor ipilimumab in 2011, immune checkpoint inhibitors (ICIs) have revolutionized cancer treatment (2). Under physiological conditions, immune checkpoint proteins (ICPs) that involve in various inhibitory and stimulatory pathways regulate the homeostasis between pro-inflammatory and anti-inflammatory signals while in cancer, the malignant cells promote an immunosuppressive tumor microenvironment to diminish anti-tumor immune response for immune evasion. Currently, clinically approved ICIs target either CTLA-4 or PD-1 signaling pathways while others targeting TIM3 and LAG-3 are currently under investigation (3, 4). CTLA-4 and PD-1 pathways differ from each other according to the periods of the immune response they involve (5). CTLA-4 expression is restricted to T cells, inhibiting cellular activation at the initial stages, mainly in lymph nodes while PD-1, expressed on T cells, B cells and myeloid cells regulates activated T cells in peripheral tissues. In summary, activation of both ICPs exerts similar effects on T-cell activity but their signaling mechanisms as well as the location where cellular interactions take place differ.
Immune checkpoint blockade (ICB) refers to usage of monoclonal antibodies which target immune checkpoint molecules or their ligands on the surface of immune and/or tumor cells which act by hampering the inhibitory mechanisms on T cells in addition to activating cells taking part in innate and adaptive immunity to exert an effective anti-tumor response (6, 7). This immunotherapeutic approach was first approved by FDA for the treatment of melanoma and non-small cell lung cancer (NSCLC), and its beneficial effects in treatment of hematological malignancies have also been proven (8–10). Historically, the promising effects of immunotherapy in the field of hematological malignancies have been initially recognized after success of stem cell transplantation (SCT) as well as various mAbs against tumor surface proteins. Accordingly, accumulating data have demonstrated that due to some genetic factors, the physiologic functions of inhibitory checkpoints are dysregulated in several hematologic diseases such as myelodysplastic syndrome (MDS), lymphoid neoplasms and acute myeloid leukemia (AML) (11). ICB therapies in hematological malignancies have primarily started with anti-CTLA-4 (Ipilimumab) and anti PD-1/PD-L1 antibodies (Pembrolizumab, Nivolumab, Atezolizumab, Avelumab, and Durvalumab) and there are numerous clinical trials evaluating their therapeutic benefits alone or in combination with other treatments (Table S1); however, they have shown clinical benefits mostly in certain types of hematological malignancies. Moreover, blockade of other inhibitory checkpoints such as LAG-3, TIM-3, and TIGIT or some innate checkpoints on NK cells and macrophage with therapeutic agents are under clinical evaluation as a potential approach to overcome acquired resistance (11–13). Some ongoing studies are investigating efficacy of ICB in association with other immunotherapies such as CAR-T cell therapy, with promising outcomes (14, 15).
Despite favorable clinical benefits of these immunotherapies in most types of hematologic malignancies, ICB is used only in the treatment of certain tumor types which have high infiltration of immune cells. Furthermore, due to some primary or acquired resistance mechanisms, checkpoint blockade therapies (CBTs) on their own are not able to achieve long-term disease control in leukemia, and thus, certain patients diagnosed with leukemia may not respond well to this treatment or benefit from it only during the initial stage. On the other hand, as inhibitory checkpoints play role in maintaining the balance of immune function, patients treated with these agents may suffer from a series of side effects called immune-related adverse events (irAEs) (16). Due to high cost of CBT, its use only in certain leukemia patients, and its potential toxicities or adverse effects, there is an unmet need for assessment of laboratory biomarkers to identify and monitor patient populations who are more likely respond to this type of therapy and management of side effects. Despite availability of several potential biomarkers and laboratory approaches, further studies are required since still there is no consensus. In solid tumors, checkpoints or their ligands’ expression levels in the tumor tissue as well as the serum levels of their soluble forms are usually evaluated, however in case of hematologic malignancies, it is more complex and identification of reliable predictive biomarkers is more difficult (17). Here, we aimed to review recent information on the predictive and prognostic value of laboratory methods and biomarkers for clinical follow-up of patients with leukemia or lymphoma who were treated with ICBs, particularly with anti-CTLA-4 and anti-PD-1/PD-L1 antibodies, the most widely used ICIs.
As mentioned above, regarding the physiologic role of ICIs in maintaining the immune system in balance, their blockade might result in several side effects and toxicities and/or provoke autoimmune reactions in both solid and hematologic malignancies. They include a wide spectrum of adverse effects including dermatologic, gastrointestinal, and hepatic disorders, iritis, hypophysitis, pneumonitis and endocrine events. These treatment-related complications which are usually less frequent in PD-1/PDL1 blocking compared to CTLA-4, are managed based on their grade. Even though it has been reported that some endocrine complications have resulted in permanent organ dysfunction necessitating hormone replacement therapy, other irAEs have typically been reversible and manageable (18, 19). Here, we describe some more common immune-related adverse events that may occur following CBT in hematological malignancies.
Previous studies have shown that blockade of PD-1/PD-L1 pathway is usually well-tolerated and associated with less adverse effects. Several studies have investigated adverse events with anti-PD-1 therapies during treatment of hematologic malignancies and have shown that most patients (approximately 78%) experience minor adverse events in the form of rash and thrombocytopenia, followed by fatigue, diarrhea, nausea, pruritus, and pyrexia. Approximately 22% of patients experienced Grade 3 or higher adverse events such as lymphopenia, stomatitis, increased lipase, MDS and pancreatitis. Such irAEs were reported in the treatment of diffuse large B-cell lymphoma (DLBCL) and Hodgkin lymphoma (HL) with pembrolizumab, where the most common side effect was neutropenia, followed by fatigue, diarrhea, and upper respiratory infections. Other studies indicated that anti-PD-1 therapy is associated with pancreatitis, lymph node pain, pneumonitis, and MDS (20–24). Furthermore, some of these studies have demonstrated Grade 3-4 neutropenia and thrombocytopenia (19% and 8%, respectively) following administration of pidilizumab (an anti-PD-1 antibody) after ASCT in patients with DLBCL, primary mediastinal B-cell lymphoma (PMBCL), and transformed indolent NHL, which are attributable to autoimmune etiology, but further studies are required to confirm this finding (20).
During treatment of hematological malignancies with anti-CTLA-4 antibodies (most commonly Ipilimumab), the most common Grade 3 adverse events were diarrhea and fatigue. Furthermore, AST elevation and thrombocytopenia have been also reported less frequently. Additionally, some other trials have evaluated efficacy and safety of ipilimumab in patients who had failed allo-SCT. Interestingly, administration of ipilimumab in hematopoietic malignancy after HSCT demonstrated a similar profile of toxicities (22). Davids et al. have investigated adverse events after use of ipilimumab in relapsed hematologic malignancies (including AML, lymphomas, myeloma, myelodysplastic syndrome, and myeloproliferative syndromes) following HSCT. In this study, patients received deferent doses of induction therapy with Ipilimumab (3 or 10 mg per kilogram of body weight every 3 weeks for a total of 4 doses). Of 28 evaluable patients, one case of acute GVHD of the gut and 3 cases of chronic GVHD of the liver were reported (25).
Leukemia develops in bone marrow and its surrounding microenvironment, which is a heterogenous environment with stromal cells, blood vessels, immune cells, and extracellular matrix (ECM) (26). It is easier to obtain a sample compared to solid tumors, however its heterogeneity, the need for expertise and the cost makes it challenging for clinical laboratories. Advanced technical expertise required for immunological assays that is centralized to advanced laboratories can also be considered as a drawback for hospital-based clinical laboratories.
There are three main mechanisms of resistance developed against ICI therapies; primary, adaptative and secondary or acquired resistance (26). Based on this classification, “primary” mechanism is referred to pre-existing resistance to immunotherapy and usually contains patients who do not respond at all to immunotherapeutic treatments, however, appearance of resistance as a Darwinian mechanism of adaptation is considered as “adaptive”. Finally, “acquired” (also called “secondary”) means appearance of resistance after immunotherapy instigation after a transient period of disease control. To understand and predict these mechanisms, there is a need for defining the “immune contexture” by analyzing the spatial localization, density, and functional orientation of immune cell populations in bone marrow and surrounding tissues (26). Routine immunophenotyping for leukemias and other hematological malignancies is an essential part of the diagnostic work-up. There are also some biomarkers that have been suggested for use in different types of resistance mechanisms such as myeloid-derived suppressor cells (MDSCs), tumor associated macrophages (TAMs) and tumor infiltrating cells (TILs) for solid tumors (26), yet there is not enough evidence to use these markers in clinical follow-up of leukemias.
The type of cells expressing immune checkpoint markers and their ligands are given in Figure 1. As will be observed, only drugs blocking PD-1/PD-L1, and CTLA-4/CD80-CD86 have been currently approved for clinical use. Other ICIs are on the line, and we need to explore the roles of these markers for clinical laboratories as well.
This review is focused on immunology- and hematology-related tests for ICIs since the biochemistry tests which are an important part of the follow-up are handled by other laboratory specialists, and they require extensive knowledge in these tests (Table 1).
Table 1 Suggested immunological tests for follow-up of patients administrating immune checkpoint inhibitors.
Leukemia subtypes are determined by using a combination of morphology, pathological findings based on morphology and immunohistochemistry, immunophenotyping, cytogenetics and molecular testing. All these tests require a high level of expertise throughout all stages of analysis, evaluation, and reporting. As we follow the response and relapse by morphology, immunohistochemistry, next generation cytometry and molecular testing in leukemias, adding checkpoint biomarkers to these routine assessments may contribute to ICIs therapy. There are FDA-approved ICIs tests such as PD-L1/PD-1 immunohistochemistry testing and Microsatellite Instability (27), and they all have specific limitations for leukemias that will be discussed in detail throughout this article.
PD-L1 expression on cells is considered a selection criterion for some solid tumors, but we do not yet have enough evidence on different subtypes of leukemia and their PD-L1 expression levels. Some researchers suggest that PD-L1 is a still imperfect biomarker since responses are observed in patients with low or negative expression tumors. While PD-L1 expression can be a predictive marker for one tumor subtype of the same tissue, it can also be a prognostic biomarker in leukemias as in lung cancers (28, 29). Currently, our knowledge regarding the involvement of PD-L1 in hematological malignancies are less investigated in comparison with solid tumors, and mostly related to the classical Hodgkin’s lymphoma (cHL), which has different characteristic features compared to AML (30–32). In terms of leukemia, Brodská et al. have reported the relationship between high PD-L1 expression and worse outcome in the presence of NPM1 and FLT3 mutations (30). Dong et al. indicated PD-L1 presence on AML cells induce T regulatory cells, whose frequency are known to increase in AML to exert their suppressive functions via promoting Foxp3 and PD-1 expression (33, 34). Rezayeen et al. underlined the role of PD-1/PD-L1 axis in drug resistance, suggesting its’ blockade may also promote chemotherapy sensitivity (35). Jimbu et al. mentioned the blockade of PD-1/PD-L1 axis in combination with hypomethylation agents have shown promising results while their administration as monotherapies failed, both in de novo and relapsed AML (32).
In contrast to PD-L1 expression which can be considered as a marker for certain solid tumors, the potential benefits of CTLA-4 targeted therapies’ cannot be predicted due to the (5) proteins’ low basal expression levels as well as the common presence of its’ ligand B7 (5). However, CTLA-4 involvement in chronic lymphocytic leukemia (CLL) (36–38) and ALL (39) have previously been reported. In AML, certain polymorphisms of the CTLA-4 gene have been associated with leukemic relapse as well as decreased survival (40).
Even for a simple antibody staining for PD-L1, there are technical difficulties such as clone of the antibodies, staining method, threshold levels for positive/negative, and standardization of assays. Choosing the most appropriate clone is a serious task for clinical laboratory staff, since a comparative study (41) on lung cancer samples implies that different clones have different performances and for different products, their specific clones of PD-L1 is required, which makes the process complicated. These differences in performance have been shown with epitope mapping experiments, and they may be attributed to different isoforms of PD-L1 (41). Further difficulties are expected in bone marrow and peripheral blood samples of leukemia patients due to heterogeneity of cell populations in samples. The FDA approved PD-L1 test is performed with a device (PD-L1 IHC 22C3 PharmDx assay) that uses two parameters; Tumor Proportion Score (TPS), which is the percentage of PD-L1 positive tumor cells in relation to viable tumor cells within the sample, and Combined Proportion Score (CPS), which identifies all PD-L1 positive cells in the sample including tumor cells, lymphocytes, and macrophages by membrane staining. There are cut-off values determined for solid tumors for these biomarkers such as TPS ≥1% and CPS ≥10% (27). At the moment, five different assays have FDA approval for these tests (42). Since most of available information is obtained through solid tissue research, clinical immunology and hematology laboratories may only implement these testing during their routine leukemia diagnostics after obtaining evidence from further research.
Higher tumor mutational burden is associated with a higher level of immunogenic neopeptides that would stimulate T cells in tumor microenvironment (43). A TMB value of ≥10 mutations/Mb is used as a predictive factor. Although FDA has approved this test as a companion diagnostic test (27), there are controversial results from different studies and manuscripts on predictive failure of this test in ICI treatments (44).
Microsatellite instability (MSI) is considered a marker for immunotherapy of solid tumors, but there are controversial views on the occurrence of MSI in hematological malignancies. In a study performed on 39 different cancer types, microsatellite instability was low in patients with chronic lymphocytic leukemia (CLL) (MSI-H=0.30), while patients with acute myeloid leukemia (AML) had zero MSI values (45).
Innate immune response and antigen presentation are crucial for anti-leukemic immune response, while effective protection may not be obtained and sustained without effector T cells (46). In this respect, detailed immunophenotyping of effector T cells in bone marrow samples (aspirates and biopsies) along with leukemia immunophenotyping during follow up will contribute to our understanding of immune response in immunotherapies. A flow cytometric panel, consisting of two tubes suitable for 13-color flow cytometry instruments for the evaluation of ICPs in leukemia samples is presented on Table 2 (47). In this panel, lymphocytes can be identified according to their forward scatter/side scatter localization, followed by the determination of common leukocyte antigen positivity by CD45 vs. side scatter gating. T cells and B cells can be differentiated by CD19 vs. CD3 plot and T cells can further be classified according to CD4+ (T helper) and CD8+ (T cytotoxic) expressions. Effector memory T cells can be differentiated between central memory cells (CD3+CCR7+) and effector memory cells (CD3+CCR7-) according to their CCR7 expression (48). CD28 positivity on CD3+CD8+ T cells identifies IFN-γ–producing, highly cytotoxic T cells (49) while CD95 on T cell gate identifies apoptotic cell population (50). Monocytes and NK cells can be discriminated according to CD14 vs. CD16 and CD14 vs. CD56 expressions, respectively. In the second tube, activation of CD3+CD4+ or CD3+CD8+ cells, identified from the lymphocyte gate can be analyzed by CD39 and CD69 expressions in addition to determination of tumor reactivity by the expression of CD137 (51–53). Similarly, expression levels of ICPs PD-1, PD-L1, CTLA-4, LAG3 and TIM3 on lymphocytes can be evaluated from the lymphocyte gate. The combination of CD45RO and CD45RA expression on lymphocyte gate can be used to identify naive T (CD45RA+) and primed/memory cells T (CD45RO) cells on either CD3+CD4 or CD3+CD8+ gates (54). In addition to use of the panel mentioned, a bone marrow aspirate is necessary to have a baseline status of ICPs at diagnosis and each time in order to measure outcome of the ICI therapies. Suggested parameters for this approach is presented in Table 3. Yet, due to the complex nature of flow cytometric analyses, standardized procedures are required for reproducibility (61).
Inflammation within the bone marrow is a risk for leukemias and is correlated with disease progression and prognosis (62, 63). Blood levels of neutrophils, lymphocytes, platelet counts and circulating proteins such as C-reactive Protein (CRP) and Interleukin-6 (IL-6) are used as markers of inflammation (64). HLA-DR expression by monocytes has been suggested as a predictive marker for inflammation especially for intensive care unit patients, but has not yet found its place in most of the clinical laboratories (65). Importance of identifying CD14+HLA-DRlow/neg monocytes in bone marrow of leukemia patients treated with ICIs should be recognized. This type of monocytes has been shown to negatively affect the responses to anti-CTLA-4 and anti-PD-1 treatments (66). Exploration of these differences for different type of leukemias, would add information to our understanding of immune response for ICIs in leukemias.
Humoral markers may be used as a surrogate marker of immunity in cancers as they have been used historically. CRP is an acute phase biomarker of inflammation and has been found to be high in diagnostic testing before ICIs therapies (67). It has been suggested that along with conventional biomarkers such as CRP and white blood cell counts; combinations of novel inflammatory parameters to specify organ damage should be developed and used (68). Studies have shown that serial cytokine measurements with serum cytokine signatures may be used as a predictive factor for ICIs therapies, but even though exposure to interferons is critical for PD-1/PD-L1 targeting cancer treatment, pro-inflammatory cytokines such as IL-2 or IFN-γ showed no significance in this respect (29, 67, 69). IFN-γ was reported to be produced by tumor infiltrating lymphocytes (TILs) in solid tumors and shown to upregulate PD-L1 expression on tumor cells (70). Measurement of soluble PD-L1 in patients with lung cancers has been associated with prognosis, and it requires further studies for use of immunotherapies in leukemia.
There are many publications about the effect of microbiome on the development of malignancies, infections, inflammatory and autoimmune diseases. A permissive microbiota is associated with development of hematologic malignancies (acute leukemia, myeloma and lymphoma) and also with other non-malignant hematological diseases (71). Therefore, manipulation of intestinal microbiota is expected to enhance the effectiveness of immune ICIs and reduce adverse effects of therapy (72). While there are many papers supporting this finding for different types of tumors, the number of studies on leukemia-microbiome interaction is limited. According to these studies, dysbiosis of microbiota may be induced by infections, chronic inflammations, epithelial barrier alterations, antigenic changes, and molecular mimicry mechanisms (71).
Development of anti-drug antibodies (ADAs) after using immunotherapies is an important concern both for clinicians and clinical laboratories, as they are considered as a part of resistance to therapy. ADAs may have an inactivating effect on the ICIs, and lead to diminished targeting, and an increase in the elimination of ADA-drug complexes and an immune response that will increase the risk for toxicity (73). Even though there are controversial studies on the subject, further studies on development and detection of these antibodies will provide more insight to patient-tailored therapies (74, 75).
Different types of ELISA methods, electrochemiluminescence and cytotoxicity tests can be used for detection of ADAs. Each laboratory will need to validate the procedures since none of these tests has not been approved for clinical use yet (74). More detailed information from clinical trials about ADAs and their effect on outcome of leukemia therapy will be helpful to consider ADA testing a routine application for clinical laboratories.
It has been shown that germline variants are predictive of tumor mutational burden and may be used as a predictive marker for ICIs (76). Inherited pathogenic germline variants in genes coding for CTLA-4 have been shown to react differently to ICIs compared to wild-type CTLA-4 (77). Germline variants in different immune cells also have the potential of modulating ICI response. More investigations on how germline variants influence the immune response to ICI treatments in different types of leukemia will contribute to our understanding of ICI therapies in hematological malignancies. All the tests listed above have their advantages and disadvantages for use in clinical laboratories, which are presented in Figure 2.
Figure 2 Advantages and disadvantages of different methods for measurement of checkpoint inhibitors and their interactions with other cells.
A comprehensive study published after analysis of >10,000 cancer samples proposed that there are six different immune subtypes across different cancer types, and wound healing, IFN-γ dominant, inflammatory, lymphocyte depleted, immunologically quiet and TGF-beta dominant subtypes differ by somatic aberrations, microenvironment and survival (78). In another study of two hundred (200) acute myeloid leukemia samples, the results for genetic and epigenetic properties of acute myeloid leukemias were published by The Cancer Genome Atlas Research Network in 2013 (79). Studies designed for understanding the immune landscape of hematological malignancies could contribute to use of ICIs considering these immune signatures. It is noteworthy to emphasize that acute myeloid leukemias have less somatic mutations than other cancer types (18 mutations vs. >50 mutations in other cancer types) (79).
Another challenge for clinical laboratories is bridging the genomic information with other clinical features of leukemias. Germline variants are not always detectable for all leukemias, and there are ongoing studies aiming to gain more precise information on the subject (80, 81). Clinical evaluation and use of these tests are challenging because of the lack of familiarity with this type of testing, the requirement for DNA samples that reasonably approximate the germline state and absence of standardization across the different brands of diagnostic platforms for genes that are sequenced as well as their capabilities for detection of full range of variants (82).
There is an unmet need for development of predictive markers for serious side effects such as myocarditis, encephalitis or acute hypophysitis, which could lead to death. Since the action mechanism of ICI treatments relies on the inhibition of immune response, treatment may end up with inflammation of tissues and organs (83). To be able to make predictions currently, available tests and novel tests for prediction of the inflammation should be included in routine testing. A guideline was developed for response criteria for use in clinical trials testing immunotherapies for solid tumors: iRECIST by Response Evaluation Criteria in Solid Tumors Working Group (RECIST) (84). Up to date, there is limited guideline information for use of ICIs in leukemias and for follow-up therapy in laboratories (85).
An experimental study has reported that the Teffs/Tregs ratio can be used as a predictive marker, and with a higher ratio, a better response is expected (86). Other studies on solid tumors also provide support to this finding (87). Exhausted T cells (CD8+PD-1+CTLA+ cells) are known to influence immune response to immunotherapy, and due to possibility of performing functional measurements such as cytokine measurements, it is possible to identify these exhausted T cells in leukemias (87–89). Only, there is a need for ready-to-use individual testing kits with reasonable prices that can be adapted to clinical laboratories from clinical trials. As mentioned by other authors, lack of “Conformite Europeenne” (CE) or “In Vitro Diagnostics” (IVD) labels for the reagents to be used in detection ICs and their counterparts requires more standardization and acceptance of the methods used for the standardization by clinical laboratories that are normally required to use the CE or IVD labeled products only (90). Extensive non-CE and non-IVD panels suggested by the same authors should be evaluated by more laboratories, as the clones chosen for this type of analysis may be different in laboratories, standardization suggestions are cumbersome and challenging with many variations in the measurement systems, reagents, preparation, and analysis methods.
In some solid tissue cancers, after ICI treatment a pseudo progression (an initial increase in tumor volume) may be observed, and pseudo progression should be distinguished from progressive disease for an accurate clinical decision (91). Detailed immunophenotyping of leukemias in a well-structured and standardized framework along with markers of ICP inhibitors will help to make decision on progression and pseudo progression. Employing a full panel of ICIs is suggested, since one marker disappears or is expressed low after treatment while other markers increase as a compensation mechanism.
A study performed for assessment of baseline serological autoantibody profile in prediction of responders to ICIs included lymphoma cases with other solid tumors and found that PD-1 and PD-L1 IgG2 autoantibodies were highly produced in approximately 20% of lymphoma responders. The authors suggest that using high-throughput protein microarrays for selected autoantibody markers can predict anti-PD-1 therapeutic response and guide the therapy (92). To implement this approach, there is a need for more evidence-based studies to confirm the prediction of response to ICIs in leukemias and other hematological malignancies. In a recently published study, measurement of CD8+LAG3+ cells in peripheral blood of solid tissue tumors were significantly correlated with response, survival and progression-free survival (73). Authors suggested that pretreatment measurement of these cells could be used for identifying actionable immune targets. Clinical trials using this panel and evaluating outcome of patients could provide more insight into use of LAG3+ cells as a marker for clinical decisions (88, 93).
Immunophenotyping of leukemias in pediatric and adult patients differ from several aspects. ACCELERATE and European Medicines Agency Pediatric Strategy Forum for medicinal product development of ICIs has published a statement paper for use of ICIs in pediatric patients and declared that; “The major challenge for developing ICIs for pediatric cancers is the lack of neoantigens (based on mutations) and corresponding antigen-specific T cells. Progress critically depends on understanding the immune macroenvironment and microenvironment and the ability of the adaptive immune system to recognize pediatric cancers in the absence of high neoantigen burden. Future clinical studies regarding administration of ICIs in children need to build upon strong biological hypotheses that take into account the distinctive immunobiology of childhood cancers in comparison to that of ICI responsive adult cancers” (94). Extensive profiling of patients in well-structured clinical trials will help understand immunobiology better and allow reaching to a consensus for follow-up.
In this article, we have focused on the possibilities and challenges ahead in clinical immunology and hematology laboratories. There are much more to be explored in imaging and other laboratories (clinical chemistry, clinical microbiology) along with the genetic laboratories in order to make the most accurate assessments for immunotherapies with ICIs. What we need to do in clinical laboratories is to develop standardized methods for all of the above-mentioned testing, work to offer cheaper and more accessible services to patients and develop new methods to be delivered in routine laboratories. We have come a long way since Rituximab was commercialized more than twenty years ago, and we have learned much about immunity during the COVID-19 pandemic period, but we still need to synchronize the tasks of clinical laboratories with the ever-expanding range of immunotherapies.
Drafting manuscript: BA, MS, CP, EG, MG-H, and GYD. Preparing the figures: CP and EG. Editing and revising the manuscript: GYD. All authors contributed to the article and approved the submitted version.
The authors declare that the research was conducted in the absence of any commercial or financial relationships that could be construed as a potential conflict of interest.
All claims expressed in this article are solely those of the authors and do not necessarily represent those of their affiliated organizations, or those of the publisher, the editors and the reviewers. Any product that may be evaluated in this article, or claim that may be made by its manufacturer, is not guaranteed or endorsed by the publisher.
We sincerely thank to Pervin Yanıkkaya Aydemir for carefully reading and editing the manuscript and Yeditepe University Rectorate for supporting us with the article processing fee.
The Supplementary Material for this article can be found online at: https://www.frontiersin.org/articles/10.3389/fonc.2022.789728/full#supplementary-material
1. Vinay DS, Ryan EP, Pawelec G, Talib WH, Stagg J, Elkord E, et al. Immune Evasion in Cancer: Mechanistic Basis and Therapeutic Strategies. Semin Cancer Biol (2015) 35:S185–98. doi: 10.1016/j.semcancer.2015.03.004
2. Marin-Acevedo JA, Kimbrough EO, Lou Y. Next Generation of Immune Checkpoint Inhibitors and Beyond. J Hematol Oncol (2021) 14(1):45. doi: 10.1186/s13045-021-01056-8
3. Qin S, Xu L, Yi M, Yu S, Wu K, Luo S. Novel Immune Checkpoint Targets: Moving Beyond PD-1 and CTLA-4. Mol Cancer (2019) 18(1):155. doi: 10.1186/s12943-019-1091-2
4. Acharya N, Sabatos-Peyton C, Anderson AC. Tim-3 Finds Its Place in the Cancer Immunotherapy Landscape. J Immunother Cancer (2020) 8(1). doi: 10.1136/jitc-2020-000911
5. Buchbinder EI, Desai A. CTLA-4 and PD-1 Pathways: Similarities, Differences, and Implications of Their Inhibition. Am J Clin Oncol (2016) 39(1):98–106. doi: 10.1097/COC.0000000000000239
6. Wei SC, Duffy CR, Allison JP. Fundamental Mechanisms of Immune Checkpoint Blockade Therapy. Cancer Discov (2018) 8(9):1069–86. doi: 10.1158/2159-8290.CD-18-0367
7. Bagchi S, Yuan R, Engleman EG. Immune Checkpoint Inhibitors for the Treatment of Cancer: Clinical Impact and Mechanisms of Response and Resistance. Annu Rev Pathol (2021) 16:223–49. doi: 10.1146/annurev-pathol-042020-042741
8. Hargadon KM, Johnson CE, Williams CJ. Immune Checkpoint Blockade Therapy for Cancer: An Overview of FDA-Approved Immune Checkpoint Inhibitors. Int Immunopharmacol (2018) 62:29–39. doi: 10.1016/j.intimp.2018.06.001
9. Garon EB, Rizvi NA, Hui R, Leighl N, Balmanoukian AS, Eder JP, et al. Pembrolizumab for the Treatment of Non–Small-Cell Lung Cancer. N Engl J Med (2015) 372(21):2018–28. doi: 10.1056/NEJMoa1501824
10. Hodi FS, O'Day SJ, McDermott DF, Weber RW, Sosman JA, Haanen JB, et al. Improved Survival With Ipilimumab in Patients With Metastatic Melanoma. N Engl J Med (2010) 363(8):711–23. doi: 10.1056/NEJMoa1003466
11. Ok CY, Young KH. Checkpoint Inhibitors in Hematological Malignancies. J Hematol Oncol (2017) 10(1):1–16. doi: 10.1186/s13045-017-0474-3
12. Pianko MJ, Liu Y, Bagchi S, Lesokhin AM. Immune Checkpoint Blockade for Hematologic Malignancies: A Review. Stem Cell Invest (2017) 4. doi: 10.21037/sci.2017.03.04
13. Salik B, Smyth MJ, Nakamura K. Targeting Immune Checkpoints in Hematological Malignancies. J Hematol Oncol (2020) 13(1):1–19. doi: 10.1186/s13045-020-00947-6
14. Cherkassky L, Morello A, Villena-Vargas J, Feng Y, Dimitrov DS, Jones DR, et al. Human CAR T Cells With Cell-Intrinsic PD-1 Checkpoint Blockade Resist Tumor-Mediated Inhibition. J Clin Invest (2016) 126(8):3130–44. doi: 10.1172/JCI83092
15. Neelapu SS, Tummala S, Kebriaei P, Wierda W, Gutierrez C, Locke FL, et al. Chimeric Antigen Receptor T-Cell Therapy—Assessment and Management of Toxicities. Nat Rev Clin Oncol (2018) 15(1):47–62. doi: 10.1038/nrclinonc.2017.148
16. Sosa A, Lopez Cadena E, Simon Olive C, Karachaliou N, Rosell R. Clinical Assessment of Immune-Related Adverse Events. Ther Adv Med Oncol (2018) 10:1758835918764628. doi: 10.1177/1758835918764628
17. Chen P-L, Roh W, Reuben A, Cooper ZA, Spencer CN, Prieto PA, et al. Analysis of Immune Signatures in Longitudinal Tumor Samples Yields Insight Into Biomarkers of Response and Mechanisms of Resistance to Immune Checkpoint Blockade. Cancer Discov (2016) 6(8):827–37. doi: 10.1158/2159-8290.CD-15-1545
18. De Velasco G, Je Y, Bossé D, Awad MM, Ott PA, Moreira RB, et al. Comprehensive Meta-Analysis of Key Immune-Related Adverse Events From CTLA-4 and PD-1/PD-L1 Inhibitors in Cancer Patients. Cancer Immunol Res (2017) 5(4):312–8. doi: 10.1158/2326-6066.CIR-16-0237
19. Naidoo J, Page D, Li BT, Connell LC, Schindler K, Lacouture ME, et al. Toxicities of the Anti-PD-1 and Anti-PD-L1 Immune Checkpoint Antibodies. Ann Oncol (2015) 26(12):2375–91. doi: 10.1093/annonc/mdv383
20. Armand P, Nagler A, Weller EA, Devine SM, Avigan DE, Chen Y-B, et al. Disabling Immune Tolerance by Programmed Death-1 Blockade With Pidilizumab After Autologous Hematopoietic Stem-Cell Transplantation for Diffuse Large B-Cell Lymphoma: Results of an International Phase II Trial. J Clin Oncol (2013) 31(33):4199. doi: 10.1200/JCO.2012.48.3685
21. Kong Y-CM, Flynn JC. Opportunistic Autoimmune Disorders Potentiated by Immune-Checkpoint Inhibitors Anti-CTLA-4 and Anti-PD-1. Front Immunol (2014) 5:206. doi: 10.3389/fimmu.2014.00206
22. Vick E, Mahadevan D. Programming the Immune Checkpoint to Treat Hematologic Malignancies. Expert Opin Invest Drugs (2016) 25(7):755–70. doi: 10.1080/13543784.2016.1175433
23. Chen R, Zinzani PL, Fanale MA, Armand P, Johnson NA, Brice P, et al. Phase II Study of the Efficacy and Safety of Pembrolizumab for Relapsed/Refractory Classic Hodgkin Lymphoma. J Clin Oncol (2017) 35(19):2125. doi: 10.1200/JCO.2016.72.1316
24. Zinzani PL, Ribrag V, Moskowitz CH, Michot J-M, Kuruvilla J, Balakumaran A, et al. Safety and Tolerability of Pembrolizumab in Patients With Relapsed/Refractory Primary Mediastinal Large B-Cell Lymphoma. Blood J Am Soc Hematol (2017) 130(3):267–70. doi: 10.1182/blood-2016-12-758383
25. Davids MS, Kim HT, Bachireddy P, Costello C, Liguori R, Savell A, et al. Ipilimumab for Patients With Relapse After Allogeneic Transplantation. N Engl J Med (2016) 375:143–53. doi: 10.1056/NEJMoa1601202
26. Pérez-Ruiz E, Melero I, Kopecka J, Sarmento-Ribeiro AB, García-Aranda M, De Las Rivas J. Cancer Immunotherapy Resistance Based on Immune Checkpoints Inhibitors: Targets, Biomarkers, and Remedies. Drug Resist Update (2020) 53:100718. doi: 10.1016/j.drup.2020.100718
27. Twomey JD, Zhang B. Cancer Immunotherapy Update: FDA-Approved Checkpoint Inhibitors and Companion Diagnostics. AAPS J (2021) 23(2):39. doi: 10.1208/s12248-021-00574-0
28. Horn L, Spigel DR, Vokes EE, Holgado E, Ready N, Steins M, et al. Nivolumab Versus Docetaxel in Previously Treated Patients With Advanced Non-Small-Cell Lung Cancer: Two-Year Outcomes From Two Randomized, Open-Label, Phase III Trials (CheckMate 017 and CheckMate 057). J Clin Oncol (2017) 35(35):3924–33. doi: 10.1200/JCO.2017.74.3062
29. Bellesoeur A, Torossian N, Amigorena S, Romano E. Advances in Theranostic Biomarkers for Tumor Immunotherapy. Curr Opin Chem Biol (2020) 56:79–90. doi: 10.1016/j.cbpa.2020.02.005
30. Brodská B, Otevřelová P, Šálek C, Fuchs O, Gašová Z, Kuželová K. High PD-L1 Expression Predicts for Worse Outcome of Leukemia Patients With Concomitant NPM1 and FLT3 Mutations. Int J Mol Sci (2019) 20(11). doi: 10.3390/ijms20112823
31. Bröckelmann PJ, Engert A. Checkpoint Inhibition in Hodgkin Lymphoma - A Review. Oncol Res Treat (2017) 40(11):654–60. doi: 10.1159/000481800
32. Jimbu L, Mesaros O, Popescu C, Neaga A, Berceanu I, Dima D, et al. Is There a Place for PD-1-PD-L Blockade in Acute Myeloid Leukemia? Pharm (Basel) (2021) 14(4). doi: 10.3390/ph14040288
33. Szczepanski MJ, Szajnik M, Czystowska M, Mandapathil M, Strauss L, Welsh A, et al. Increased Frequency and Suppression by Regulatory T Cells in Patients With Acute Myelogenous Leukemia. Clin Cancer Res (2009) 15(10):3325–32. doi: 10.1158/1078-0432.CCR-08-3010
34. Dong Y, Han Y, Huang Y, Jiang S, Huang Z, Chen R, et al. PD-L1 Is Expressed and Promotes the Expansion of Regulatory T Cells in Acute Myeloid Leukemia. Front Immunol (2020) 11:1710. doi: 10.3389/fimmu.2020.01710
35. Rezaeeyan H, Hassani SN, Barati M, Shahjahani M, Saki N. PD-1/PD-L1 as a Prognostic Factor in Leukemia. J Hematopathol (2017) 10(1):17–24. doi: 10.1007/s12308-017-0293-z
36. Mittal AK, Chaturvedi NK, Rohlfsen RA, Gupta P, Joshi AD, Hegde GV, et al. Role of CTLA4 in the Proliferation and Survival of Chronic Lymphocytic Leukemia. PloS One (2013) 8(8):e70352. doi: 10.1371/journal.pone.0070352
37. Karabon L, Partyka A, Ciszak L, Pawlak-Adamska E, Tomkiewicz A, Bojarska-Junak A, et al. Abnormal Expression of BTLA and CTLA-4 Immune Checkpoint Molecules in Chronic Lymphocytic Leukemia Patients. J Immunol Res (2020) 2020:6545921. doi: 10.1155/2020/6545921
38. Do P, Beckwith KA, Beaver L, Griffin BG, Mo X, Jones J, et al. Leukemic Cell Expressed CTLA-4 Suppresses T Cells Via Down-Modulation of CD80 By Trans-Endocytosis. Blood (2016) 128(22):3221. doi: 10.1182/blood.V128.22.3221.3221
39. Laurent S, Palmisano GL, Martelli AM, Kato T, Tazzari PL, Pierri I, et al. CTLA-4 Expressed by Chemoresistant, as Well as Untreated, Myeloid Leukaemia Cells can be Targeted With Ligands to Induce Apoptosis. Br J Haematol (2007) 136(4):597–608. doi: 10.1111/j.1365-2141.2006.06472.x
40. Pérez-García A, Brunet S, Berlanga JJ, Tormo M, Nomdedeu J, Guardia R, et al. CTLA-4 Genotype and Relapse Incidence in Patients With Acute Myeloid Leukemia in First Complete Remission After Induction Chemotherapy. Leukemia (2009) 23(3):486–91. doi: 10.1038/leu.2008.339
41. Hirsch FR, McElhinny A, Stanforth D, Ranger-Moore J, Jansson M, Kulangara K, et al. PD-L1 Immunohistochemistry Assays for Lung Cancer: Results From Phase 1 of the Blueprint PD-L1 IHC Assay Comparison Project. J Thorac Oncol (2017) 12(2):208–22. doi: 10.1016/j.jtho.2016.11.2228
42. FDA. List of Cleared or Approved Companion Diagnostic Devices (In Vitro and Imaging Tools) (2021). Available at: https://www.fda.gov/medical-devices/in-vitro-diagnostics/list-cleared-or-approved-companion-diagnostic-devices-in-vitro-and-imaging-tools (Accessed August, 29).
43. Marabelle A, Fakih M, Lopez J, Shah M, Shapira-Frommer R, Nakagawa K, et al. Association of Tumour Mutational Burden With Outcomes in Patients With Advanced Solid Tumours Treated With Pembrolizumab: Prospective Biomarker Analysis of the Multicohort, Open-Label, Phase 2 KEYNOTE-158 Study. Lancet Oncol (2020) 21(10):1353–65. doi: 10.1016/S1470-2045(20)30445-9
44. McGrail DJ, Pilie PG, Rashid NU, Voorwerk L, Slagter M, Kok M, et al. High Tumor Mutation Burden Fails to Predict Immune Checkpoint Blockade Response Across All Cancer Types. Ann Oncol (2021) 32(5):661–72. doi: 10.1016/j.annonc.2021.02.006
45. Bonneville R, Krook MA, Kautto EA, Miya J, Wing MR, Chen HZ, et al. Landscape of Microsatellite Instability Across 39 Cancer Types. JCO Precis Oncol (2017) 2017. doi: 10.1200/PO.17.00073
46. Pan C, Liu H, Robins E, Song W, Liu D, Li Z, et al. Next-Generation Immuno-Oncology Agents: Current Momentum Shifts in Cancer Immunotherapy. J Hematol Oncol (2020) 13(1):29. doi: 10.1186/s13045-020-00862-w
47. Holmberg-Thyden S, Grønbæk K, Gang AO, El Fassi D, Hadrup SR. A User's Guide to Multicolor Flow Cytometry Panels for Comprehensive Immune Profiling. Anal Biochem (2021) 627:114210. doi: 10.1016/j.ab.2021.114210
48. Unsoeld H, Krautwald S, Voehringer D, Kunzendorf U, Pircher H. Cutting Edge: CCR7+ and CCR7- Memory T Cells do Not Differ in Immediate Effector Cell Function. J Immunol (2002) 169(2):638–41. doi: 10.4049/jimmunol.169.2.638
49. Nicolet BP, Guislain A, van Alphen FPJ, Gomez-Eerland R, Schumacher TNM, van den Biggelaar M, et al. CD29 Identifies IFN-γ-Producing Human CD8(+) T Cells With an Increased Cytotoxic Potential. Proc Natl Acad Sci USA (2020) 117(12):6686–96. doi: 10.1073/pnas.1913940117
50. Paulsen M, Janssen O. Pro- and Anti-Apoptotic CD95 Signaling in T Cells. Cell Commun Signal (2011) 9:7. doi: 10.1186/1478-811X-9-7
51. Ziegler SF, Ramsdell F, Alderson MR. The Activation Antigen CD69. Stem Cells (1994) 12(5):456–65. doi: 10.1002/stem.5530120502
52. Raczkowski F, Rissiek A, Ricklefs I, Heiss K, Schumacher V, Wundenberg K, et al. CD39 Is Upregulated During Activation of Mouse and Human T Cells and Attenuates the Immune Response to Listeria Monocytogenes. PloS One (2018) 13(5):e0197151. doi: 10.1371/journal.pone.0197151
53. Ye Q, Song DG, Poussin M, Yamamoto T, Best A, Li C, et al. CD137 Accurately Identifies and Enriches for Naturally Occurring Tumor-Reactive T Cells in Tumor. Clin Cancer Res (2014) 20(1):44–55. doi: 10.1158/1078-0432.CCR-13-0945
54. Courville EL, Lawrence MG. Characteristic CD45RA/CD45RO Maturation Pattern by Flow Cytometry Associated With the CD45 C77G Polymorphism. Cytometry B Clin Cytom (2021) 100(5):602–5. doi: 10.1002/cyto.b.21993
55. Gu S, Zi J, Han Q, Song C, Ge Z. Elevated TNFRSF4 Gene Expression Is a Predictor of Poor Prognosis in Non-M3 Acute Myeloid Leukemia. Cancer Cell Int (2020) 20:146. doi: 10.1186/s12935-020-01213-y
56. Stoll A, Bruns H, Fuchs M, Volkl S, Nimmerjahn F, Kunz M, et al. CD137 (4-1BB) Stimulation Leads to Metabolic and Functional Reprogramming of Human Monocytes/Macrophages Enhancing Their Tumoricidal Activity. Leukemia (2021) 35(12):3482–96. doi: 10.1038/s41375-021-01287-1
57. Re F, Arpinati M, Testoni N, Ricci P, Terragna C, Preda P, et al. Expression of CD86 in Acute Myelogenous Leukemia Is a Marker of Dendritic/Monocytic Lineage. Exp Hematol (2002) 30(2):126–34. doi: 10.1016/S0301-472X(01)00768-8
58. Hobo W, Hutten TJA, Schaap NPM, Dolstra H. Immune Checkpoint Molecules in Acute Myeloid Leukaemia: Managing the Double-Edged Sword. Br J Haematol (2018) 181(1):38–53. doi: 10.1111/bjh.15078
59. Goltz D, Gevensleben H, Grunen S, Dietrich J, Kristiansen G, Landsberg J, et al. PD-L1 (CD274) Promoter Methylation Predicts Survival in Patients With Acute Myeloid Leukemia. Leukemia (2017) 31(3):738–43. doi: 10.1038/leu.2016.328
60. Zajac M, Zaleska J, Dolnik A, Bullinger L, Giannopoulos K. Expression of CD274 (PD-L1) Is Associated With Unfavourable Recurrent Mutations in AML. Br J Haematol (2018) 183(5):822–5. doi: 10.1111/bjh.15040
61. Lambert C, Yanikkaya Demirel G, Keller T, Preijers F, Psarra K, Schiemann M, et al. Flow Cytometric Analyses of Lymphocyte Markers in Immune Oncology: A Comprehensive Guidance for Validation Practice According to Laws and Standards. Front Immunol (2020) 11:2169. doi: 10.3389/fimmu.2020.02169
62. Zambetti NA, Ping Z, Chen S, Kenswil KJG, Mylona MA, Sanders MA, et al. Mesenchymal Inflammation Drives Genotoxic Stress in Hematopoietic Stem Cells and Predicts Disease Evolution in Human Pre-Leukemia. Cell Stem Cell (2016) 19(5):613–27. doi: 10.1016/j.stem.2016.08.021
63. Leimkühler NB, Schneider RK. Inflammatory Bone Marrow Microenvironment. Hematol Am Soc Hematol Educ Program (2019) 2019(1):294–302. doi: 10.1182/hematology.2019000045
64. Ravindranathan D, Master VA, Bilen MA. Inflammatory Markers in Cancer Immunotherapy. Biol (Basel) (2021) 10(4). doi: 10.3390/biology10040325
65. Monneret G, Lepape A, Voirin N, Bohé J, Venet F, Debard AL, et al. Persisting Low Monocyte Human Leukocyte Antigen-DR Expression Predicts Mortality in Septic Shock. Intensive Care Med (2006) 32(8):1175–83. doi: 10.1007/s00134-006-0204-8
66. Mengos AE, Gastineau DA, Gustafson MP. The CD14(+)HLA-DR(lo/neg) Monocyte: An Immunosuppressive Phenotype That Restrains Responses to Cancer Immunotherapy. Front Immunol (2019) 10:1147. doi: 10.3389/fimmu.2019.01147
67. Ozawa Y, Amano Y, Kanata K, Hasegwa H, Matsui T, Kakutani T, et al. Impact of Early Inflammatory Cytokine Elevation After Commencement of PD-1 Inhibitors to Predict Efficacy in Patients With Non-Small Cell Lung Cancer. Med Oncol (2019) 36(4):33. doi: 10.1007/s12032-019-1255-3
68. Bruserud O, Aarstad HH, Tvedt THA. Combined C-Reactive Protein and Novel Inflammatory Parameters as a Predictor in Cancer-What Can We Learn From the Hematological Experience? Cancers (Basel) (2020) 12(7). doi: 10.3390/cancers12071966
69. Garcia-Diaz A, Shin DS, Moreno BH, Saco J, Escuin-Ordinas H, Rodriguez GA, et al. Interferon Receptor Signaling Pathways Regulating PD-L1 and PD-L2 Expression. Cell Rep (2017) 19(6):1189–201. doi: 10.1016/j.celrep.2017.04.031
70. Mimura K, Teh JL, Okayama H, Shiraishi K, Kua LF, Koh V, et al. PD-L1 Expression Is Mainly Regulated by Interferon Gamma Associated With JAK-STAT Pathway in Gastric Cancer. Cancer Sci (2018) 109(1):43–53. doi: 10.1111/cas.13424
71. Fattizzo B, Cavallaro F, Folino F, Barcellini W. Recent Insights Into the Role of the Microbiome in Malignant and Benign Hematologic Diseases. Crit Rev Oncol Hematol (2021) 160:103289. doi: 10.1016/j.critrevonc.2021.103289
72. Dai Z, Zhang J, Wu Q, Fang H, Shi C, Li Z, et al. Intestinal Microbiota: A New Force in Cancer Immunotherapy. Cell Commun Signal (2020) 18(1):90. doi: 10.1186/s12964-020-00599-6
73. EEMA. Guideline on Immunogenicity Assessment of Therapeutic Proteins (2017). Available at: https://www.ema.europa.eu/en/documents/scientific-guideline/guideline-immunogenicity-assessment-therapeutic-proteins-revision-1_en.pdf (Accessed August, 29 2021).
74. van Brummelen EM, Ros W, Wolbink G, Beijnen JH, Schellens JH. Antidrug Antibody Formation in Oncology: Clinical Relevance and Challenges. Oncologist (2016) 21(10):1260–8. doi: 10.1634/theoncologist.2016-0061
75. Davda J, Declerck P, Hu-Lieskovan S, Hickling TP, Jacobs IA, Chou J, et al. Immunogenicity of Immunomodulatory, Antibody-Based, Oncology Therapeutics. J Immunother Cancer (2019) 7(1):105. doi: 10.1186/s40425-019-0586-0
76. Chatrath A, Ratan A, Dutta A. Germline Variants Predictive of Tumor Mutational Burden and Immune Checkpoint Inhibitor Efficacy. iScience (2021) 24(3):102248. doi: 10.1016/j.isci.2021.102248
77. Hamid O, Schmidt H, Nissan A, Ridolfi L, Aamdal S, Hansson J, et al. A Prospective Phase II Trial Exploring the Association Between Tumor Microenvironment Biomarkers and Clinical Activity of Ipilimumab in Advanced Melanoma. J Transl Med (2011) 9:204. doi: 10.1186/1479-5876-9-204
78. Thorsson V, Gibbs DL, Brown SD, Wolf D, Bortone DS, Ou Yang TH, et al. The Immune Landscape of Cancer. Immunity (2018) 48(4):812–30.e14. doi: 10.1016/j.immuni.2018.03.023
79. Ley TJ, Miller C, Ding L, Raphael BJ, Mungall AJ, Robertson A, et al. Genomic and Epigenomic Landscapes of Adult De Novo Acute Myeloid Leukemia. N Engl J Med (2013) 368(22):2059–74. doi: 10.1056/NEJMoa1301689
80. Li ST, Wang J, Wei R, Shi R, Adema V, Nagata Y, et al. Rare Germline Variant Contributions to Myeloid Malignancy Susceptibility. Leukemia (2020) 34(6):1675–8. doi: 10.1038/s41375-019-0701-8
81. Roloff GW, Drazer MW, Godley LA. Inherited Susceptibility to Hematopoietic Malignancies in the Era of Precision Oncology. JCO Precis Oncol (2021) 5):107–22. doi: 10.1200/PO.20.00387
82. Feurstein S, Drazer M, Godley LA. Germline Predisposition to Haematopoietic Malignancies. Hum Mol Genet (2021) 30(20):R225–35. doi: 10.1093/hmg/ddab141
83. Robert C. A Decade of Immune-Checkpoint Inhibitors in Cancer Therapy. Nat Commun (2020) 11(1):3801. doi: 10.1038/s41467-020-17670-y
84. Seymour L, Bogaerts J, Perrone A, Ford R, Schwartz LH, Mandrekar S, et al. iRECIST: Guidelines for Response Criteria for Use in Trials Testing Immunotherapeutics. Lancet Oncol (2017) 18(3):e143–52. doi: 10.1016/S1470-2045(17)30074-8
85. Brahmer JR, Abu-Sbeih H, Ascierto PA, Brufsky J, Cappelli LC, Cortazar FB, et al. Society for Immunotherapy of Cancer (SITC) Clinical Practice Guideline on Immune Checkpoint Inhibitor-Related Adverse Events. J Immunother Cancer (2021) 9(6). doi: 10.1136/jitc-2021-002435
86. Quezada SA, Peggs KS, Curran MA, Allison JP. CTLA4 Blockade and GM-CSF Combination Immunotherapy Alters the Intratumor Balance of Effector and Regulatory T Cells. J Clin Invest (2006) 116(7):1935–45. doi: 10.1172/JCI27745
87. Terranova-Barberio M, Pawlowska N, Dhawan M, Moasser M, Chien AJ, Melisko ME, et al. Exhausted T Cell Signature Predicts Immunotherapy Response in ER-Positive Breast Cancer. Nat Commun (2020) 11(1):3584. doi: 10.1038/s41467-020-17414-y
88. Li Z, Philip M, Ferrell PB. Alterations of T-Cell-Mediated Immunity in Acute Myeloid Leukemia. Oncogene (2020) 39(18):3611–9. doi: 10.1038/s41388-020-1239-y
89. Tang L, Wu J, Li CG, Jiang HW, Xu M, Du M, et al. Characterization of Immune Dysfunction and Identification of Prognostic Immune-Related Risk Factors in Acute Myeloid Leukemia. Clin Cancer Res (2020) 26(7):1763–72. doi: 10.1158/1078-0432.CCR-19-3003
90. Shibru B, Fey K, Fricke S, Blaudszun AR, Fürst F, Weise M, et al. Detection of Immune Checkpoint Receptors - A Current Challenge in Clinical Flow Cytometry. Front Immunol (2021) 12:694055. doi: 10.3389/fimmu.2021.694055
91. Kataoka Y, Hirano K. Which Criteria Should We Use to Evaluate the Efficacy of Immune-Checkpoint Inhibitors? Ann Transl Med (2018) 6(11):222. doi: 10.21037/atm.2018.04.17
92. Tan Q, Wang D, Yang J, Xing P, Yang S, Li Y, et al. Autoantibody Profiling Identifies Predictive Biomarkers of Response to Anti-PD1 Therapy in Cancer Patients. Theranostics (2020) 10(14):6399–410. doi: 10.7150/thno.45816
93. Shen R, Postow MA, Adamow M, Arora A, Hannum M, Maher C, et al. LAG-3 Expression on Peripheral Blood Cells Identifies Patients With Poorer Outcomes After Immune Checkpoint Blockade. Sci Transl Med (2021) 13(608). doi: 10.1126/scitranslmed.abf5107
94. Pearson ADJ, Rossig C, Lesa G, Diede SJ, Weiner S, Anderson J, et al. ACCELERATE and European Medicines Agency Paediatric Strategy Forum for Medicinal Product Development of Checkpoint Inhibitors for Use in Combination Therapy in Paediatric Patients. Eur J Cancer (2020) 127:52–66. doi: 10.1016/j.ejca.2019.12.029
Keywords: immune checkpoint blockade, immune checkpoint inhibitors (CPI), leukemia, clinical laboratory (cl), immune check point
Citation: Aru B, Soltani M, Pehlivanoglu C, Gürlü E, Ganjalikhani-Hakemi M and Yanikkaya Demirel G (2022) Comparison of Laboratory Methods for the Clinical Follow Up of Checkpoint Blockade Therapies in Leukemia: Current Status and Challenges Ahead. Front. Oncol. 12:789728. doi: 10.3389/fonc.2022.789728
Received: 05 October 2021; Accepted: 06 January 2022;
Published: 27 January 2022.
Edited by:
Swami P. Iyer, University of Texas MD Anderson Cancer Center, United StatesReviewed by:
Ling Xu, Jinan University, ChinaCopyright © 2022 Aru, Soltani, Pehlivanoglu, Gürlü, Ganjalikhani-Hakemi and Yanikkaya Demirel. This is an open-access article distributed under the terms of the Creative Commons Attribution License (CC BY). The use, distribution or reproduction in other forums is permitted, provided the original author(s) and the copyright owner(s) are credited and that the original publication in this journal is cited, in accordance with accepted academic practice. No use, distribution or reproduction is permitted which does not comply with these terms.
*Correspondence: Gülderen Yanikkaya Demirel, Z3VsZGVyZW4ueWRlbWlyZWxAeWVkaXRlcGUuZWR1LnRy
Disclaimer: All claims expressed in this article are solely those of the authors and do not necessarily represent those of their affiliated organizations, or those of the publisher, the editors and the reviewers. Any product that may be evaluated in this article or claim that may be made by its manufacturer is not guaranteed or endorsed by the publisher.
Research integrity at Frontiers
Learn more about the work of our research integrity team to safeguard the quality of each article we publish.