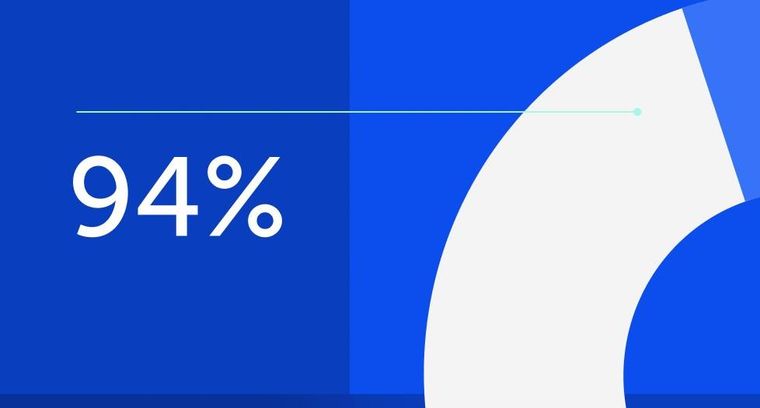
94% of researchers rate our articles as excellent or good
Learn more about the work of our research integrity team to safeguard the quality of each article we publish.
Find out more
REVIEW article
Front. Oncol., 02 February 2022
Sec. Gastrointestinal Cancers: Colorectal Cancer
Volume 12 - 2022 | https://doi.org/10.3389/fonc.2022.781233
This article is part of the Research TopicEmerging Therapeutic Targets, Potential Diagnostic or Prognostic markers for Colorectal CancerView all 28 articles
The role of the tumor microenvironment (TME) in the progression of colorectal cancer (CRC) and its acquisition of resistance to treatment become the research hotspots. As an important component of TME, the tumor-associated macrophages (TAMs) regulate multiple critical oncogenic processes, namely, occurrence, proliferation, metastasis, and drug resistance in CRC. In this review, we have discussed the functional and therapeutic significance of TAMs in CRC. M1 macrophages act as the tumor suppressor while M2 macrophages promote CRC. The polarization of TAMs is mainly regulated by the pathways such as NFKB1 pathways, STAT3 pathways, WNT5A pathways, and PI3K pathways in CRC. Furthermore, the M2 polarization of TAMs is not only controllable but also reversible. Finally, we provide insights into the TAMs-targeted therapeutic strategies.
CRC is the third common cancer in males and second in females, causing 600,000 deaths per year (1). Surgery, chemotherapy, radiotherapy, and targeted therapy are currently established as commonly used options for CRC patients (2–4). With advances in therapeutic strategies, the prognosis of CRC patients has been tremendously improved (5). Patients with stage IIIC colon cancer have a 5-year survival rate of 53%, while 58% for stage IIIC rectal cancer (6). However, drug resistance of CRC is common that prevents treatment from achieving the expected outcome (5, 7). The resistance mechanisms have been observed in CRC cells, namely, promoting degradation of drugs, inhibiting apoptosis, affecting drug delivery, interfering DNA damage and repair, regulating epigenetic factors, and inducing cell cycle arrest (4, 8–10). The treatment effect is not very satisfactory due to resistance. Patients with BRAF V600E-mutated metastatic colorectal cancer only have a median overall survival of 4 to 6 months due to multidrug resistance (11). Therefore, effective strategies are urgently needed to improve patient prognosis.
At present, TME plays an essential role in CRC and gradually gains prominence as a new therapeutic target for the reversal of resistance (12, 13). Advances in the understanding of the TME have contributed to the exploration of treatments for advanced CRC (14). To date, pembrolizumab and nivolumab, two anti-programmed death protein 1 (PDCD1) inhibitors, were approved by the FDA for treating mismatch-repair-deficient (dMMR) and microsatellite instability-high (MSI-H) metastatic colorectal cancer (mCRC) (15). Although clinical data have revealed that immunotherapy can double progression-free survival (PFS), the treatment can only be applied in patients with MSI-H-dMMR CRC, accounting for 15% of all CRC cases (16, 17). The limitation has prompted the search for novel therapeutic targets to improve clinical applications and the effects of immunotherapy.
TME is a complex network comprising cells (T cells, B cells, TAMs, myeloid-derived suppressor cells, cancer-associated fibroblasts, etc.) and non-cellular components (cytokines, proteins, oxygen, etc.) (18–22). As the main component of TME, TAMs can interact with tumor cells by secreting cytokines, participating in CRC processes (23–26). For example, TAMs secret CXCL8 triggering EGFR signaling of tumor cells which is the leading cause of drug resistance in refractory CRC with KRAS or BRAF V600E mutation (27). The expression of immune checkpoints such as PDCD1 in TAMs contributes to immunosuppressed microenvironment in CRC (25, 28–32). With high plasticity and heterogeneity, functions of TAMs in CRC are not limited to immunosuppression, and the M1 phenotype in TAM acts as an immunostimulator (33–35).The phenotypic plasticity in TAMs provides new insights for treatment. A lot of evidence has also revealed that phenotypes and infiltration of TAMs were related to the prognosis of CRC patients (36–39). Consequently, TAMs-targeted therapy is beneficial to improve therapy effectiveness and patient prognosis. The article aims to discuss the multiple roles of TAMs in CRC and summarize emerging treatments based on TAMs.
The development of novel anti-CRC treatment has been challenged by the complex tumor environment (40). Many studies also demonstrate that increased pro-tumor cells (such as T-reg cells) and decreased anti-tumor cells (such as CD8 T cells and NK cells) are responsible for tumor escaping immune surveillance (41, 42). Hence, understanding the tumor microenvironment is essential to develop efficient treatment strategies. The TME consist of cancer cells, tumor-associated neutrophils (TANs), myeloid-derived suppressor cells (MDSCs), cancer-associated fibroblasts (CAFs), tumor infiltrating lymphocytes (TILs), dendritic cells and all kinds of cytokines, and has been gradually confirmed to improve the malignant potential of tumors (43). TAMs, acting as an important component of TME, can not only directly act on tumor cells but also regulate other constituents of the TME, thus affecting tumor progression (44).
TANs have functional similarities to TAMs. Both cells above exert a dual effect on CRC development with several isoforms, but the interactions between TAMs and TANs in CRC need to be further investigated (45, 46). MDSCs possess immuno-suppressive properties (47). In CRC, TAMs could shape the inflammatory microenvironment by secreting cytokines to promote MDSC production and the recruitment of MDSCs contributes to the generation of TAMs (47, 48). This activation loop between TAMs and MDSCs help tumor expansion and immune evasion. Furthermore, TAMs induce matrix deposition and collagen fibrillogenesis by increasing the expression of collagens XIV and I in CAFs, forming tissue barriers for CRC invasion and metastasis (49). Similar to MDSC, CAFs also act on TAMs. Studies have shown that immunosuppressive factors secreted by CAFs induce polarization of M2-like TAMs (50). In terms of CRC prognosis, both TAMs and TILs have the potential to predict tumor recurrence and survival in CRC patients and the high density of TAMs and TILs indicates a longer survival (51). But the correlation between TAMs and TILs, just like that between TAMs and dendritic cells, still need further exploration (52). One of inflammation hallmarks of TAMs is the cytokine secretion (53). For example, the IL6 secreted by TAMs could promote CRC self-renewal and metastasis (54). TAMs can also expel proteins and enzymes including DOCK family through vesicle transport to mediate the activation of lymphocytes (55). The polarization procedure of TAMs requires the participation of cytokines including IL4, IL10 and so on (56, 57).
As we can see, TAMs interact with multiple constituents in TME to participate in the regulation of CRC. However, the current studies about the interaction between TAMs and immune-related factors are imperfect, which limits our ability to develop specific therapeutic treatments. It is undeniable that TAM plays an important role in CRC and further exploration of its specific role in the various processes of CRC should be conducted in order to find breakthrough points in interventions.
Initially, TAMs exist in the form of undifferentiated macrophages M0, and under some conditions, M0 could be polarized into M1 (generated by classical activation) or M2 phenotype (generated by alternative activation) (Figure 1) (58, 59). Stimulators of classical pathway, namely, bacterial components, interferon-γ (IFNG), lipopolysaccharide (LPS), and Toll-like receptor (TLR), polarize M0 into M1 phenotype, which exerts cancer inhibitory effect by releasing pro-inflammatory cytokines (such as IL1B and IL12) and cytotoxic substances (such as reactive oxygen species and TNF) (60–62). While M2 macrophages display tumor-promoting activity and can be further classified into four different subtypes, M2a (induced by IL4, IL13), M2b (induced by TLR), M2c (induced by glucocorticoid), and M2d (induced by IL6 and adenosines) (63). In vivo, with lower CCL22, CCL17, and IL12 expression, M2 cells secrete a series of molecules such as IL6 and CXCL8 to exert immunosuppressive functions (60, 64). Further insight into the functions of TAMs in CRC is the basis for effective therapeutic targeting.
Figure 1 The polarization of TAMs. TAMs have three subtypes, including undifferentiated macrophages (M0 phenotype), pro-inflammatory phenotype (M1 phenotype), and anti-inflammatory phenotype (M2 phenotype). When activated by bacterial components, interferon-γ (IFNG), lipopolysaccharide (LPS), and Toll-like receptor (TLR), M0 macrophages are polarized toward M1 through classical activation. With a high expression of IL6 and CXCL8, and lower levels of CCL22, CCL17, and IL12, M2 macrophages are generated through alternative activation of M0 macrophages. Depending on stimulators, they can be further classified into M2a, M2b, M2c, and M2d subtypes. M1 and M2 phenotypes secret cytokines to participate in tumor progression, and the role of both in CRC is antagonistic to each other. It is confirmed that M1 macrophages act as the tumor suppressor while M2 phenotype promotes CRC.
A prospective cohort study showed the amount of TAMs could change the effect of smoking on CRC occurrence (65). However the mechanisms of correlation between TAMs and CRC occurrence remain unclear. Cancer Stem Cell (CSC) is a kind of cancer cell phenotype having abilities of self-renewal, clonal tumor initiation, and rapid proliferation, taking part in tumorigenesis as the primary cell group (66). Therefore, in this part, we focus on the interaction between TAMs and CSC. In cancer cells, high expression of T-cell immunoglobulin domain and mucin domain 4 (TIMD4) and SIX1 protein indicates upregulated CSC-like properties. These cells would recruit TAMs and promote M2 polarization, leading to tumor interstitial remodeling (67, 68). In addition to proteins, RNAs regulate the functions of CSC. CSC could downregulate several microRNAs through a circRNA–microRNA–mRNA axis, thus assist the aggregation of downstream molecules, including SKIL, SMAD2, WNT5A, and so on (69).
Meanwhile, in clinical samples, it was found that overexpression of WNT5A could polarize TAMs into the M2 phenotype and regulate IL10 secretion (57). Nevertheless, the reason for the upregulation of WNT5A remains unclear. As in mammalian cells, microRNAs would be packed into exosomes to discharge and function extracellularly (70). There might be a possibility that CSC excretes microRNAs to connect with TAMs. Simultaneous, CSC is regulated by TAMs, and recent in vivo studies suggest the proportion of CD68+ TAMs at the tumor invasive front (TF) is correlated with the level of stem cell marker CD44v6 (71, 72). After infiltrating in the cancer area, M2 subtypes increase the ratio of CD44+/CD166+ tumor cells and the expression of acetaldehyde dehydrogenase 1 (ALDH1), which is the marker of CSC (57, 67, 73).
Many components of the immune microenvironment are implicated in proliferation, which is a primary hallmark of tumor (74). At present, the role of TAM in CRC proliferation has been widely reported. Through secreting transforming growth factor β1 (TGFB1), TAMs have abilities to upregulate vascular endothelial growth factor (VEGF) and interleukin-6 (IL6), and the latter binds with IL6 receptor on the tumor cell surface to promote CRC proliferation via activating STAT3 (75, 76). The extent of STAT3 activation is affected by diet, and dieting is considered a potential therapy that promotes anti-tumor immunity (77). Under fasting conditions, M2 polarization was inhibited, resulting in limited proliferation and increased apoptosis of CT26 colon cancer cells (78). A current study provided an important observation that serum starvation caused differentiation markers of T-reg, TGFB1, and FOXP3 rising (79). This evidence leaves us some questions that if TAMs could play a role in T-reg by modulation of TGFB1, fasting combined TGFB1 neutralizing antibody might show more potency against CRC proliferation. Based on the above research, eliminating M2 TAMs is regarded as an effective anti-cancer therapy. Yet, in eliminating macrophages of Ccr2−/− mice, TAMs can still proliferate and release cytokines to promote the growth of CRC (80). So, in addition to the activation of the M0 alternative pathway, there are other ways to generate M2 macrophages. Meanwhile, CRC could escape immune supervision through this bypass, and figuring out associated specific mechanisms would bring new understanding to clinical therapy.
During the malignant transformation, cancer cells undergo function acquisition and alteration due to genetic mutation (81). Among all the functions, the capacity of invasion is an essential criterion for judging malignant diseases (82). Many studies have observed that TAMs accelerate tumor invasion mainly by regulating the epithelial–mesenchymal transition (EMT) process during which tumor cells gradually discard epithelial characteristics and obtain mesenchymal phenotypes, generating circulating tumor cells and tumor stem cells (83, 84). After a positive correlation between TAMs and the EMT marker snail was observed, TAMs were confirmed to release transforming growth factor β (TGFB) to activate the TGFB/Smad2,3-4/Snail signaling pathway, and restraining the pathway with TGFB receptor inhibitor might reverse metastasis (84). Apart from TGFB, TAMs secret other cytokines, namely, IL4 and IL6, and the former indicates the formation of M2 phenotype, while the latter activates the STAT3 signaling pathway to trigger EMT in CRC (56, 75). EMT is an inter-regulating course, and there is an urgent need to lock the TAM/EMT axis. In recent years, intestinal microbiota has attracted much attention in immunomodulation (85, 86). The microbiota dysfunction can induce TAMs secreting IL6 and TNF to launch EMT of HT29 colorectal adenocarcinoma cells (87). Maybe intestinal microbiota is an excellent target to attenuate the M2 phenotype. In-depth analysis of M2 macrophage-derived exosomes (MDE) shows high expression of miR-155-5p, while the microRNA also participates in metabolism-related gene transcription of intestinal microflora (88, 89). The findings provide the basis of intestinal microflora regulation served as one target of inhibiting CRC metastasis.
The degree of macrophage infiltration can indirectly reflect the prognosis of patients (90). The risk of recurrence and cancer-associated mortality was doubled in patients with high M2 proportion in lesion compared with low M2 infiltration, and the high invasion of M1 macrophages in tumor matrix predicted a good prognosis and extended survival period (91). To further substantiate this, a subset of 168 patients with stage II/III colorectal cancer received follow-up interviews after operation. The results demonstrated that the patients with a high density of CD163+ TAMs have worse overall survival (OS) and progression-free survival (PFS) (92). For CRC patients, early detection and diagnosis are of great significance for a good outcome, and carcinoembryonic antigen (CEA), the most used biomarker for noninvasively detection, is less effective due to poor sensitivity (93). As same as CEA, TAMs are also distributed in the blood and barely influenced by surgery status (91, 92, 94). Prognostic information can be acquired by analyzing the proportion and polarization state of TAM, such as the ratio of CD206+/CD68+ TAM (95). A study consisting of 931 colorectal cancer patients showed the high M1/M2 ratio was suggestive of lower mortality in colorectal cancer (96). In a word, the infiltration density and polarization state of TAMs influence the prognosis of patients, yet the potential for reflecting prognosis needs further more clinical research (39).
Colorectal cancer is a highly heterogeneous disease due to the disorder of cell signal regulation mechanism (97). Abnormal activation of pathways is involved in the CRC cell-TAM regulatory loop, which contributes to tumor biology (98, 99). Consequently, the pathway is like a bridge to connect tumor and TAMs, and TAMs have been proved to be involved in many pathways important to CRC,namely, NFKB1 pathway, STAT3 pathway, WNT5A pathway, and PI3K pathway (Figure 2) (75, 98, 100, 101). While less evidence indicates tumor associated macrophages interact with Hedgehog pathway and Notch pathway, both of which are concerned with regeneration and renewal of the epithelium, this may be a pointcut for better understanding the role of TAMs in CRC (102, 103). This part will summarize the interaction between TAMs and four pathways frequently reactivating in colorectal cancer.
Figure 2 Pathways between TAMs and CRC. The interaction between CRC and TAMs relies on a complex molecular network. Tumor cells release molecules such as IL10 and Succinate, activating STAT3 pathway and PI3K pathway in macrophages. Consequently, pro-tumor cytokines, including IL6, CXCL8, and TGFB2, are secreted out of TAMs and targeted to their receptor on the surface of CRC cells. Specific inhibitors aiming at essential pathway points effectively reverse the pro-tumor process, typically miR-221-3P inhibiting STAT3 pathway by silencing JAK3. Besides, WNT5A and NFKB1 contribute to the network, and each pathway is not independent but interconnected. The secreted pro-tumor cytokines cause the response of JAK2, TNF, and NFKB1, serving CRC. A similar network can be seen both in TAMs and CRC, and suppressors inside it may have a dual function.
Src homology 2 domain-containing tyrosine phosphatase 2 (SHP2) has a tumor inhibitory effect in CRC. When SHP2 is deficient in TAMs, the phosphorylation of RELA protein is declined. It facilitates the polarization of TAMs to M2 phenotype, which can release IL6 and CXCL8 to increase the expression of TNF in CRC cells, leading to the activation of NFKB1 pathway and then promotes tumor angiogenesis and metastasis (26, 104). This procedure emphasizes the importance of epigenetics in TAMs. And enhancing the phosphorylation levels of RELA by knocking out N-myc downstream-regulated gene 2 (NDRG2) leads to IκBα upregulating. As a result, the monocyte polarization is induced toward M1-like macrophages, playing an inhibitory role in tumor (105). Additionally, M2 macrophages can secrete TGFB2 and activate NFKB1 pathway to regulate FERMT2, forming the TGFB2/NFKB1/FERMT2 axis to promote CRC cells invasion, and NFKB1 inhibitor Bay 11-7082 can reverse functions mentioned above (106, 107). Though there are no findings of associated clinical trials by far, all kinds of inhibitors have brought hopes for the treatment.
STAT3 is a component of the IL6 activated acute phase response factor (APRF) complex, which can be activated by various cytokines or growth factors (108). As we mentioned before, TAM can release cytokines, especially IL6, which could bind specific receptors on CRC cell surface, activating JAK2/STAT3 signaling pathway, downregulating tumor suppressor miR-506-3p, and relieve the inhibition of the latter on FOXQ1 that results in enhanced invasion and metastasis of CRC (25). Inhibition of this signaling pathway can reverse CRC metastasis caused by nicotinic acetylcholine receptor α7 (α7nAChR) knockout (107). Like IL6, IL10 secreted by CRC could induce TAM polarization to M2 macrophages through CaKMII/ERK/STAT3 pathway and promote cancer cell itself (57). On the contrary, with the effect of miR-221-3p for silencing JAK3/STAT3 activation, M2 TAMs begin exhibiting characteristics of M1 (109). The STAT3 pathway is not a one-way path, but a loop between CRC and TAMs, and the circle could be disrupted if the pathway was interrupted, which needs a lot more research exploring the association between TAMs and members in this loop.
WNT5A is a secreted glycoprotein belonging to the WNT family, and related signaling pathways mainly regulate cell proliferation, angiogenesis, and so on (110). As for CRC, WNT5A is expressed primarily in tumor matrix, especially in TAMs, but its specific biological function and related mechanism are not fully understood, which needs further exploration (57). WNT5A can form a cascade with CaKMII and ERK1. When the pathway is activated, TAM will be polarized to M2 phenotype and release IL10, aiming to promote the progression of tumor (57, 100). For the tight association of WNT5 with chemokine ligands, researchers subsequently focused on another molecule CCL2, and it was interesting that when WNT5A was used to treat undifferentiated macrophages, CCL2 mRNA was upregulated most significantly among all cytokines (111, 112). After silencing WNT5A expression, the level of ERK activation and expression of CCL2 declines, consequently inhibiting M2 polarization (111). As we all know, non-single but multiple pathways involve in CRC. For instance, the WNT5A pathway could link to the NFKB1 pathway under the action of ROR1, then sensitizing STAT3 (113). Therefore, the WNT5A signaling pathway plays an important role in CRC malignant biological behavior and macrophage infiltration, yet we still know little about how different pathways connect.
Phosphatidylinositol-3-kinase (PI3K) signaling pathway is intracellular, which can be activated by lots of cytokines, affecting TAM recruitment and polarization (114). Tumor cells can release succinate acting on macrophages and promote their transformation through the PI3K–hypoxia–inducible factor 1α (HIF1A) axis (22). MiR-934 is one of the activating factors released by exosomes, downregulating the expression of PTEN in TAM, consequently activating the PI3K/AKT1 signaling pathway to induce the M2 polarization (115). In addition to microRNA, CRC can also release type Iγ phosphatidylinositol phosphate kinase (PIPKIG) to trigger PI3K/AKT1/MTOR signaling pathway, promoting TAM recruitment in tumor area through reinforcement of CCL2 transcription, thus providing an appropriate environment for CRC development (116). M2 macrophages also release CXCL13 to trigger CXCL13/CXCR5/NFKB1 signaling to induce CRC liver metastasis (115).
Systematic chemotherapy is the standard treatment for patients with advanced CRC, which can effectively prolong the overall survival time. Whereas the survival rate of patients with stage IV CRC is only 10%, drug resistance was one of the causes of this phenomenon (117). With deepening research, TME immune components are shown to participate in resistance, which is demonstrated by great strides in immunotherapy (118, 119). Primarily programmed by TME, TAMs have a significant impact on the treatment efficiency of CRC (54, 120). It was found that the conditional medium from M1 could improve the oxaliplatin sensitivity in tumor-bearing mice and that from M2 reduces the sensitivity of CRC to 5-fluorouracil (5-FU), suppressing caspase-mediated apoptosis to protect tumor cells from chemotherapy (121, 122), whereas reducing TAM proportion or inducing M1 directional polarization can effectively increase the survival time of patients and improve the prognosis (123, 124). Though M1 and M2 macrophages antagonize each other, interference of TAM gene expression by exogenous application of drugs can reduce the proportion of M2 TAMs in tumor infiltration regions and promote the transformation from M2 to M1 phenotype exerting tumor inhibition (Figure 3) (58, 105, 125). Additionally, the drug tolerance initiated from TAMs is concerned with effluxion. Given the difficulties in finding novel therapeutic targets, efflux inhibitors specifically targeted M2 subtype might work miracle.
Figure 3 Treatments based on TAMs. M0 macrophages polarized towards M1 or M2 phenotypes to participate in growth, proliferation, metastasis, and drug resistance of CRC. Therefore many treatments are designed based on the polarization of TAMs. Odanacatib and Nitazoxanide, targeting CTSK and SIX1 respectively, both inhibit the alternative activation of undifferentiated macrophages, and MIC triggers the polarization of M1 macrophages. Meanwhile, nanoscale drug Ru @ ICG-BLZ NP can directly induce the conversion of M2 phenotype to M1 phenotype, showing a new method for clinical transformation. The role of cholesterol metabolism in CRC is gradually being valued, and a new anti-metabolism drug Trifluridine/Tipiracil was shown to weaken infiltration of M2 macrophages. Other therapy such as PDCD1 blockers and adoptive cell therapy could also act on TAMs and showing anti-tumor impact.
Immunotherapy, namely, immune checkpoint inhibitors, adoptive cell therapy, and tumor vaccines, has been popularized in CRC treatment (126). Programmed cell death protein 1 (PDCD1) is an immune checkpoint receptor. It has been reported that in CRC patients with high microsatellite instability, PDCD1 is highly expressed in M2 macrophages at the invasive region of CRC (127). The high PDCD1 expression of M1 phenotype, resulting in the degeneration of TAM phagocytosis, is positively correlated with disease state of CRC (128). PDCD1 blockers can enhance phagocytic ability of macrophages and prolong survival time of patients, confirming that PDCD1 therapy can directly aim at TAM. Besides, patients with more M2 macrophages infiltration in lesion areas have the potential to acquire better efficacy. As for adoptive cell therapy, the anti-tumor efficiency of the combination of tumor-directed anti-mesothelin CAR-T cells and M2 inhibitors has been verified, and TAM-associated adoptive cell therapy based on its specific markers, such as CD40, are being investigated (129). In cell models that stably express ovalbumin (OVA) peptide, the OVA vaccine could reduce the density of TAMs in CRC tissue, thus limiting tumor growth, and supplemental application of VEGFC/VEGFR3 neutralizing antibody could further inhibit the chemotaxis of M2 macrophages into CRC area, preventing CRC from escaping immune monitoring (130).
The long non-coding RNAs (lncRNAs) serve as both non-invasive biomarkers and targeted molecules in CRC (131). For instance, lncRNA RPPH1 secreted by CRC cells mediates M2 polarization, promoting tumor metastasis, but is lacking independent means of intervention (132). Intestinal microflora, an important regulator of intestinal microenvironment, has been shown to modulate lncRNA gene expression in various tissues (133). Additionally, microflora can also induce M0 to M2 phenotype polarization by secreting cathepsin K (CTSK), which binds to toll-like receptor 4 (TLR4), activating MTOR pathway (134). CTSK-specific inhibitor Odanacatib is administrated to curb the related pro-tumor effects, improving the prognosis of CRC patients (134). In addition to the regular medications, researchers nowadays exploited nanoparticles with inflammatory molecule releasing ability, Ru @ ICG-BLZ NP, which has high CRC specificity and low toxicity (125). It can release CSF-1R kinase inhibitor BLZ945 and repolarized TAM to M1 macrophages to show an anti-tumor effect which provides a new idea for clinical transformation of nanodrugs (125).
Homologous protein SIX1 is widely expressed in all kinds of cancers, and its overexpression results in upregulation of macrophage-specific colony stimulating factor, thus recruiting pro-tumor TAM in the CRC region (68). Its inhibitor, Nitazoxanide, is expected to silencing SIX1 by suppressing WNT/CTNNB1 pathway (135, 136). Metabolism disturbance could partly explain the mechanism for TAMs inducing drug resistance. Trifluridine/Tipiracil is a new anti-metabolism drug, and its combination with oxaliplatin can effectively exhaust M2 macrophages, thereby causing cytotoxic CD8+ T cell infiltration to compel tumor cell lysis (137). Through years of treatment, clinicians observed a strange phenomenon that patients with colitis have a specific resistance to CRC, but the mechanism is unknown. One of the benign disease characteristics is chronic inflammation that persists for a long time, and many inflammatory factors are involved in this process. Macrophage inhibitory cytokine (MIC) is one of them, which can recruit M0 macrophages and T cells to lesion sites and exert tumor immune regulation (138). Subsequently, more clinical trials are required to explore the anti-CRC effect of MIF biological agents. The combination between it and conventional therapy may provide more options for clinical CRC treatment.
A growing understanding of the functions and regulatory mechanisms of TAMs will enable us to explore their future clinical applications in CRC. In this review, we described different subtypes of TAMs and their associated mechanisms in colorectal cancer. Based on the above, TAMs-related treatment for CRC was summarized with potential for further research. Many factors are associated with the poor prognostic of CRC, especially the low early diagnostic rate and poor pharmacological reaction. Therefore, it is of great significance to explore the relevant mechanisms and early diagnostic methods beneficial to clinical prevention and treatment of CRC. TAM is an important component of the TME that mediates CRC proliferation, metastases, drug resistance, and so on. Once stimulated by tumor-associated factors, TAMs migrate to the tumor area at first, occurring polarization to M1 or M2 phenotype, and exerts tumor inhibition or promotion effects, respectively. Nevertheless, whether TAM already exists or transferred from the circulatory system in the CRC tumor area is still doubtful. Additionally, the function of TAM is mostly indirectly proved, and clinical research focusing on TAM is still lacking.
TAMs secrete cytokines (IL6, IL10, etc.) and exosomes (miR-21-5p, miR-155-5p, etc.) through NFKB1 signaling pathway, STAT3 signaling pathway, and other pathways to act on tumor cells and immune cells, regulating the process of CRC. Moreover, TAMs secrete many immunomodulatory proteins in the form of vesicles to consolidating the tumor-promoting roles of stromal cells. Apart from acting on tumor cells, TAMs could regulate other immune cells of TME, thus participating in the regulation of immune microenvironment. That phenomenon may be the cause of treatment failure. Drug resistance is one of the most troublesome problems during the treatment of CRC, and TAM polarization is closely related to the resistance. Especially, M2 phenotype can secrete various factors such as CCL2 to weaken the sensitivity of CRC to oxaliplatin and other drugs, which suggest that TAM is expected to become a tumor therapeutic target. The induction of directional differentiation in vitro is helpful to understand the capacity of different TAM subtypes further. However, the progress of TAM study is slow due to the difficulty of establishing associated models, and there is a long way to address this issue.
Both immune checkpoint inhibitor PDCD1 and tumor vaccine are capable of inhibiting M2 formation or promoting its depletion. PDCD1 takes TAM as a direct target to inhibit tumor progression. The development of nanoscale drug load particles, such as Ru @ ICG-BLZ NP, provides a new method for the clinical transformation of nanodrugs. Since TAMs could release cytokines, neutralizing antibodies against M2 macrophages associated factors also show high anti-tumor value. Limagne et al. (137) found that TAM could also guide direction of T cell infiltration and migration. Therefore, in addition to regulating macrophage polarization states, TAM-targeted therapeutics can improve efficacies of immune checkpoint inhibitors. All of these have shown bright therapeutic prospects and great research potential. However, there are still some limitations. The specific functional mechanism of immunotherapy such as PDCD1 on TAM is still ambiguous. Although TAM is currently considered to be a biomarker, more clinical experiments are required to confirm its efficiency. Furthermore, reactive oxygen species (ROS) can actuate macrophage activation and function (139). Does ROS related mechanism attend TAM tumor regulation process? Whether metabolomics and biological rhythm also affect TAM function, these questions are worthy of further studies. The main biomarkers of TAM, such as CD68, transmembrane receptors, and secretory proteins, are expected to be therapeutic targets (120). Based on this, CRC-specific immunotherapy has been developed, which shows great application prospects and clinical significance.
We follow standardized nomenclature for gene products recommended by experts (140).
YL drafted the manuscript. ZC designed the figures and revised the manuscript. JC has done critical revision, and approved this version of the article. All authors listed have made a substantial, direct, and intellectual contribution to the work and approved it for publication.
This work was supported by the National Natural Science and Technology Major Project of the thirteenth Five Year Plan (grant 2017ZX10203207) and the National Natural Science Foundation of China (grant 8200033791, 8207034966).
The authors declare that the research was conducted in the absence of any commercial or financial relationships that could be construed as a potential conflict of interest.
All claims expressed in this article are solely those of the authors and do not necessarily represent those of their affiliated organizations, or those of the publisher, the editors and the reviewers. Any product that may be evaluated in this article, or claim that may be made by its manufacturer, is not guaranteed or endorsed by the publisher.
The authors thank the Huashan Hospital for supporting information and also thank the Cancer Metastasis Institute for providing the study site.
1. Kolligs FT. Diagnostics and Epidemiology of Colorectal Cancer. Visc Med (2016) 32(3):158–64. doi: 10.1159/000446488
2. Guo L, Wang C, Qiu X, Pu X, Chang P. Colorectal Cancer Immune Infiltrates: Significance in Patient Prognosis and Immunotherapeutic Efficacy. Front Immunol (2020) 11:1052. doi: 10.3389/fimmu.2020.01052
3. Liu K, Lei S, Kuang Y, Jin Q, Long D, Liu C, et al. A Novel Mechanism of the C-Myc/NEAT1 Axis Mediating Colorectal Cancer Cell Response to Photodynamic Therapy Treatment. Front Oncol (2021) 11:652831. doi: 10.3389/fonc.2021.652831
4. Wang H. MicroRNAs and Apoptosis in Colorectal Cancer. Int J Mol Sci (2020) 21(15):5353. doi: 10.3390/ijms21155353
5. Diehl TM, Abbott DE. Molecular Determinants and Other Factors to Guide Selection of Patients for Hepatic Resection of Metastatic Colorectal Cancer. Curr Treat Options Oncol (2021) 22(9):82. doi: 10.1007/s11864-021-00878-5
6. Rawla P, Sunkara T, Barsouk A. Epidemiology of Colorectal Cancer: Incidence, Mortality, Survival, and Risk Factors. Prz Gastroenterol (2019) 14(2):89–103. doi: 10.5114/pg.2018.81072
7. Zhao F, Yang Z, Gu X, Feng L, Xu M, Zhang X. miR-92b-3p Regulates Cell Cycle and Apoptosis by Targeting CDKN1C, Thereby Affecting the Sensitivity of Colorectal Cancer Cells to Chemotherapeutic Drugs. Cancers (Basel) (2021) 13(13):3323. doi: 10.3390/cancers13133323
8. Luo D, Zhang Y, Yang S, Tian X, Lv Y, Guo Z, et al. Design, Synthesis and Biological Evaluation of Sphingosine-1-Phosphate Receptor 2 Antagonists as Potent 5-FU-Resistance Reversal Agents for the Treatment of Colorectal Cancer. Eur J Med Chem (2021) 225:113775. doi: 10.1016/j.ejmech.2021.113775
9. Wang H. MicroRNAs and Apoptosis in Colorectal Cancer. Int J Mol Sci (2020) 21(15). doi: 10.3390/ijms21155353
10. Chen N, Kong Y, Wu Y, Gao Q, Fu J, Sun X, et al. CAC1 Knockdown Reverses Drug Resistance Through the Downregulation of P-Gp and MRP-1 Expression in Colorectal Cancer. PLoS One (2019) 14(9):e0222035. doi: 10.1371/journal.pone.0222035
11. Kopetz S, Grothey A, Yaeger R, Van Cutsem E, Desai J, Yoshino T, et al. Encorafenib, Binimetinib, and Cetuximab in BRAF V600E-Mutated Colorectal Cancer. N Engl J Med (2019) 381(17):1632–43. doi: 10.1056/NEJMoa1908075
12. Nenkov M, Ma Y, Gaßler N, Chen Y. Metabolic Reprogramming of Colorectal Cancer Cells and the Microenvironment: Implication for Therapy. Int J Mol Sci (2021) 22(12):6262. doi: 10.3390/ijms22126262
13. Reidy E, Leonard NA, Treacy O, Ryan AE. A 3d View of Colorectal Cancer Models in Predicting Therapeutic Responses and Resistance. Cancers (Basel) (2021) 13(2):227. doi: 10.3390/cancers13020227
14. Giraldo NA, Sanchez-Salas R, Peske JD, Vano Y, Becht E, Petitprez F, et al. The Clinical Role of the TME in Solid Cancer. Br J Cancer (2019) 120(1):45–53. doi: 10.1038/s41416-018-0327-z
15. Ganesh K, Stadler ZK, Cercek A, Mendelsohn RB, Shia J, Segal NH, et al. Immunotherapy in Colorectal Cancer: Rationale, Challenges and Potential. Nat Rev Gastroenterol Hepatol (2019) 16(6):361–75. doi: 10.1038/s41575-019-0126-x
16. Biller LH, Schrag D. Diagnosis and Treatment of Metastatic Colorectal Cancer: A Review. JAMA (2021) 325(7):669–85. doi: 10.1001/jama.2021.0106
17. André T, Shiu KK, Kim TW, Jensen BV, Jensen LH, Punt C, et al. Pembrolizumab in Microsatellite-Instability-High Advanced Colorectal Cancer. N Engl J Med (2020) 383(23):2207–18. doi: 10.1056/NEJMoa2017699
18. Picoli CC, Gonçalves BÔP, Santos GSP, Rocha BGS, Costa AC, Resende RR, et al. Pericytes Cross-Talks Within the Tumor Microenvironment. Biochim Biophys Acta Rev Cancer (2021) 1876(2):188608. doi: 10.1016/j.bbcan.2021.188608
19. Yamaguchi R, Perkins G. Animal Models for Studying Tumor Microenvironment (TME) and Resistance to Lymphocytic Infiltration. Cancer Biol Ther (2018) 19(9):745–54. doi: 10.1080/15384047.2018.1470722
20. Liu Y, Zhou X, Wang X. Targeting the Tumor Microenvironment in B-Cell Lymphoma: Challenges and Opportunities. J Hematol Oncol (2021) 14(1):125. doi: 10.1186/s13045-021-01134-x
21. Ma S, Song W, Xu Y, Si X, Zhang D, Lv S, et al. Neutralizing Tumor-Promoting Inflammation With Polypeptide-Dexamethasone Conjugate for Microenvironment Modulation and Colorectal Cancer Therapy. Biomaterials (2020) 232:119676. doi: 10.1016/j.biomaterials.2019.119676
22. Wu JY, Huang TW, Hsieh YT, Wang YF, Yen CC, Lee GL, et al. Cancer-Derived Succinate Promotes Macrophage Polarization and Cancer Metastasis via Succinate Receptor. Mol Cell (2020) 77(2):213–27.e5. doi: 10.1016/j.molcel.2019.10.023
23. Zhang Y, Song J, Zhao Z, Yang M, Chen M, Liu C, et al. Single-Cell Transcriptome Analysis Reveals Tumor Immune Microenvironment Heterogenicity and Granulocytes Enrichment in Colorectal Cancer Liver Metastases. Cancer Lett (2020) 470:84–94. doi: 10.1016/j.canlet.2019.10.016
24. Shang P, Gao R, Zhu Y, Zhang X, Wang Y, Guo M, et al. VEGFR2-targeted antibody fused with IFN α mut regulates the tumor microenvironment of colorectal cancer and exhibits potent anti-tumor and anti-metastasis activity. Acta Pharm Sin B (2021) 11(2):420–33. doi: 10.1016/j.apsb.2020.09.008
25. Cheng Y, Zhu Y, Xu J, Yang M, Chen P, Xu W, et al. PKN2 in Colon Cancer Cells Inhibits M2 Phenotype Polarization of Tumor-Associated Macrophages via Regulating DUSP6-Erk1/2 Pathway. Mol Cancer (2018) 17(1):13. doi: 10.1186/s12943-017-0747-z
26. Wang S, Yao Y, Li H, Zheng G, Lu S, Chen W. Tumor-Associated Macrophages (TAMs) Depend on Shp2 for Their Anti-Tumor Roles in Colorectal Cancer. Am J Cancer Res (2019) 9(9):1957–69.
27. Ahmed S, Mohamed HT, El-Husseiny N, El Mahdy MM, Safwat G, Diab AA, et al. IL-8 Secreted by Tumor Associated Macrophages Contribute to Lapatinib Resistance in HER2-Positive Locally Advanced Breast Cancer via Activation of Src/STAT3/ERK1/2-Mediated EGFR Signaling. Biochim Biophys Acta Mol Cell Res (2021) 1868(6):118995. doi: 10.1016/j.bbamcr.2021.118995
28. Yahaya MAF, Lila MAM, Ismail S, Zainol M, Afizan N. Tumour-Associated Macrophages (TAMs) in Colon Cancer and How to Reeducate Them. J Immunol Res (2019) 2019:2368249. doi: 10.1155/2019/2368249
29. Zhang R, Qi F, Zhao F, Li G, Shao S, Zhang X, et al. Cancer-Associated Fibroblasts Enhance Tumor-Associated Macrophages Enrichment and Suppress NK Cells Function in Colorectal Cancer. Cell Death Dis (2019) 10(4):273. doi: 10.1038/s41419-019-1435-2
30. Fakih M, Ouyang C, Wang C, Tu TY, Gozo MC, Cho M, et al. Immune Overdrive Signature in Colorectal Tumor Subset Predicts Poor Clinical Outcome. J Clin Invest (2019) 129(10):4464–76. doi: 10.1172/jci127046
31. Oh N, Kim Y, Kweon HS, Oh WY, Park JH. Macrophage-Mediated Exocytosis of Elongated Nanoparticles Improves Hepatic Excretion and Cancer Phototherapy. ACS Appl Mater Interf (2018) 10(34):28450–7. doi: 10.1021/acsami.8b10302
32. Roumenina LT, Daugan MV, Noé R, Petitprez F, Vano YA, Sanchez-Salas R, et al. Tumor Cells Hijack Macrophage-Produced Complement C1q to Promote Tumor Growth. Cancer Immunol Res (2019) 7(7):1091–105. doi: 10.1158/2326-6066.Cir-18-0891
33. Wu J, Li H, Xie H, Wu X, Lan P. The Malignant Role of Exosomes in the Communication Among Colorectal Cancer Cell, Macrophage and Microbiome. Carcinogenesis (2019) 40(5):601–10. doi: 10.1093/carcin/bgy138
34. Ardighieri L, Missale F, Bugatti M, Gatta LB, Pezzali I, Monti M, et al. Infiltration by CXCL10 Secreting Macrophages Is Associated With Antitumor Immunity and Response to Therapy in Ovarian Cancer Subtypes. Front Immunol (2021) 12:690201. doi: 10.3389/fimmu.2021.690201
35. Chekaoui A, Ertl HCJ. PPARa Agonist Fenofibrate Enhances Cancer Vaccine Efficacy. Cancer Res (2021) 81(17):4431–40. doi: 10.1158/0008-5472.Can-21-0052
36. Yang Z, Zhang M, Peng R, Liu J, Wang F, Li Y, et al. The Prognostic and Clinicopathological Value of Tumor-Associated Macrophages in Patients With Colorectal Cancer: A Systematic Review and Meta-Analysis. Int J Colorectal Dis (2020) 35(9):1651–61. doi: 10.1007/s00384-020-03686-9
37. Ye L, Zhang T, Kang Z, Guo G, Sun Y, Lin K, et al. Tumor-Infiltrating Immune Cells Act as a Marker for Prognosis in Colorectal Cancer. Front Immunol (2019) 10:2368. doi: 10.3389/fimmu.2019.02368
38. Pan JH, Zhou H, Cooper L, Huang JL, Zhu SB, Zhao XX, et al. LAYN Is a Prognostic Biomarker and Correlated With Immune Infiltrates in Gastric and Colon Cancers. Front Immunol (2019) 10:6. doi: 10.3389/fimmu.2019.00006
39. Li J, Li L, Li Y, Long Y, Zhao Q, Ouyang Y, et al. Tumor-Associated Macrophage Infiltration and Prognosis in Colorectal Cancer: Systematic Review and Meta-Analysis. Int J Colorectal Dis (2020) 35(7):1203–10. doi: 10.1007/s00384-020-03593-z
40. Ruan H, Leibowitz BJ, Zhang L, Yu J. Immunogenic Cell Death in Colon Cancer Prevention and Therapy. Mol Carcinog (2020) 59(7):783–93. doi: 10.1002/mc.23183
41. Pancione M, Giordano G, Remo A, Febbraro A, Sabatino L, Manfrin E, et al. Immune Escape Mechanisms in Colorectal Cancer Pathogenesis and Liver Metastasis. J Immunol Res (2014) 2014:686879. doi: 10.1155/2014/686879
42. Westrich JA, Vermeer DW, Colbert PL, Spanos WC, Pyeon D. The Multifarious Roles of the Chemokine CXCL14 in Cancer Progression and Immune Responses. Mol Carcinog (2020) 59(7):794–806. doi: 10.1002/mc.23188
43. Roma-Rodrigues C, Mendes R, Baptista PV, Fernandes AR. Targeting Tumor Microenvironment for Cancer Therapy. Int J Mol Sci (2019) 20(4):840. doi: 10.3390/ijms20040840
44. Ngambenjawong C, Gustafson HH, Pun SH. Progress in Tumor-Associated Macrophage (TAM)-Targeted Therapeutics. Adv Drug Deliv Rev (2017) 114:206–21. doi: 10.1016/j.addr.2017.04.010
45. Galdiero MR, Bonavita E, Barajon I, Garlanda C, Mantovani A, Jaillon S. Tumor Associated Macrophages and Neutrophils in Cancer. Immunobiology (2013) 218(11):1402–10. doi: 10.1016/j.imbio.2013.06.003
46. Huang C, Ou R, Chen X, Zhang Y, Li J, Liang Y, et al. Tumor Cell-Derived SPON2 Promotes M2-Polarized Tumor-Associated Macrophage Infiltration and Cancer Progression by Activating PYK2 in CRC. J Exp Clin Cancer Res (2021) 40(1):304. doi: 10.1186/s13046-021-02108-0
47. Maisonneuve C, Tsang DKL, Foerster EG, Robert LM, Mukherjee T, Prescott D, et al. Nod1 Promotes Colorectal Carcinogenesis by Regulating the Immunosuppressive Functions of Tumor-Infiltrating Myeloid Cells. Cell Rep (2021) 34(4):108677. doi: 10.1016/j.celrep.2020.108677
48. Thiele Orberg E, Fan H, Tam AJ, Dejea CM, Destefano Shields CE, Wu S, et al. The Myeloid Immune Signature of Enterotoxigenic Bacteroides Fragilis-Induced Murine Colon Tumorigenesis. Mucosal Immunol (2017) 10(2):421–33. doi: 10.1038/mi.2016.53
49. Afik R, Zigmond E, Vugman M, Klepfish M, Shimshoni E, Pasmanik-Chor M, et al. Tumor Macrophages Are Pivotal Constructors of Tumor Collagenous Matrix. J Exp Med (2016) 213(11):2315–31. doi: 10.1084/jem.20151193
50. Garufi A, Traversi G, Cirone M, D'Orazi G. HIPK2 Role in the Tumor-Host Interaction: Impact on Fibroblasts Transdifferentiation CAF-Like. IUBMB Life (2019) 71(12):2055–61. doi: 10.1002/iub.2144
51. Kim SW, Kim YI, Mustafa B, Kim MJ, Jeong G, Ahn SM, et al. Distribution Pattern of Tumor Infiltrating Lymphocytes and Tumor Microenvironment Composition as Prognostic Indicators in Anorectal Malignant Melanoma. Mod Pathol (2021) 34(1):141–60. doi: 10.1038/s41379-020-0633-x
52. Geissler K, Fornara P, Lautenschläger C, Holzhausen HJ, Seliger B, Riemann D. Immune Signature of Tumor Infiltrating Immune Cells in Renal Cancer. Oncoimmunology (2015) 4(1):e985082. doi: 10.4161/2162402x.2014.985082
53. Yao X, Huang J, Zhong H, Shen N, Faggioni R, Fung M, et al. Targeting Interleukin-6 in Inflammatory Autoimmune Diseases and Cancers. Pharmacol Ther (2014) 141(2):125–39. doi: 10.1016/j.pharmthera.2013.09.004
54. Yin Y, Yao S, Hu Y, Feng Y, Li M, Bian Z, et al. The Immune-Microenvironment Confers Chemoresistance of Colorectal Cancer Through Macrophage-Derived Il6. Clin Cancer Res (2017) 23(23):7375–87. doi: 10.1158/1078-0432.Ccr-17-1283
55. Cianciaruso C, Beltraminelli T, Duval F, Nassiri S, Hamelin R, Mozes A, et al. Molecular Profiling and Functional Analysis of Macrophage-Derived Tumor Extracellular Vesicles. Cell Rep (2019) 27(10):3062–80.e11. doi: 10.1016/j.celrep.2019.05.008
56. Lin X, Wang S, Sun M, Zhang C, Wei C, Yang C, et al. miR-195-5p/NOTCH2-Mediated EMT Modulates IL-4 Secretion in Colorectal Cancer to Affect M2-Like TAM Polarization. J Hematol Oncol (2019) 12(1):20. doi: 10.1186/s13045-019-0708-7
57. Liu Q, Yang C, Wang S, Shi D, Wei C, Song J, et al. Wnt5a-Induced M2 Polarization of Tumor-Associated Macrophages via IL-10 Promotes Colorectal Cancer Progression. Cell Commun Signal (2020) 18(1):51. doi: 10.1186/s12964-020-00557-2
58. Popēna I, Ābols A, Saulīte L, Pleiko K, Zandberga E, Jēkabsons K, et al. Effect of Colorectal Cancer-Derived Extracellular Vesicles on the Immunophenotype and Cytokine Secretion Profile of Monocytes and Macrophages. Cell Commun Signal (2018) 16(1):17. doi: 10.1186/s12964-018-0229-y
59. Shinohara H, Kuranaga Y, Kumazaki M, Sugito N, Yoshikawa Y, Takai T, et al. Regulated Polarization of Tumor-Associated Macrophages by miR-145 via Colorectal Cancer-Derived Extracellular Vesicles. J Immunol (2017) 199(4):1505–15. doi: 10.4049/jimmunol.1700167
60. Tariq M, Zhang J, Liang G, Ding L, He Q, Yang B. Macrophage Polarization: Anti-Cancer Strategies to Target Tumor-Associated Macrophage in Breast Cancer. J Cell Biochem (2017) 118(9):2484–501. doi: 10.1002/jcb.25895
61. Wunderlich CM, Ackermann PJ, Ostermann AL, Adams-Quack P, Vogt MC, Tran ML, et al. Obesity Exacerbates Colitis-Associated Cancer via IL-6-Regulated Macrophage Polarisation and CCL-20/CCR-6-Mediated Lymphocyte Recruitment. Nat Commun (2018) 9(1):1646. doi: 10.1038/s41467-018-03773-0
62. Baig MS, Roy A, Rajpoot S, Liu D, Savai R, Banerjee S, et al. Tumor-Derived Exosomes in the Regulation of Macrophage Polarization. Inflamm Res (2020) 69(5):435–51. doi: 10.1007/s00011-020-01318-0
63. Akimoto M, Maruyama R, Takamaru H, Ochiya T, Takenaga K. Soluble IL-33 Receptor Sst2 Inhibits Colorectal Cancer Malignant Growth by Modifying the Tumour Microenvironment. Nat Commun (2016) 7:13589. doi: 10.1038/ncomms13589
64. Koh YC, Yang G, Lai CS, Weerawatanakorn M, Pan MH. Chemopreventive Effects of Phytochemicals and Medicines on M1/M2 Polarized Macrophage Role in Inflammation-Related Diseases. Int J Mol Sci (2018) 19(8):2208. doi: 10.3390/ijms19082208
65. Ugai T, Väyrynen JP, Haruki K, Akimoto N, Lau MC, Zhong R, et al. Smoking and Incidence of Colorectal Cancer Subclassified by Tumor-Associated Macrophage Infiltrates. J Natl Cancer Inst (2022) 114(1):68–77. doi: 10.1093/jnci/djab142
66. Li S, Xu F, Zhang J, Wang L, Zheng Y, Wu X, et al. Tumor-Associated Macrophages Remodeling EMT and Predicting Survival in Colorectal Carcinoma. Oncoimmunology (2018) 7(2):e1380765. doi: 10.1080/2162402x.2017.1380765
67. Tan X, Zhang Z, Yao H, Shen L. Tim-4 Promotes the Growth of Colorectal Cancer by Activating Angiogenesis and Recruiting Tumor-Associated Macrophages via the PI3K/AKT/mTOR Signaling Pathway. Cancer Lett (2018) 436:119–28. doi: 10.1016/j.canlet.2018.08.012
68. Xu H, Zhang Y, Peña MM, Pirisi L, Creek KE. Six1 Promotes Colorectal Cancer Growth and Metastasis by Stimulating Angiogenesis and Recruiting Tumor-Associated Macrophages. Carcinogenesis (2017) 38(3):281–92. doi: 10.1093/carcin/bgw121
69. Rengganaten V, Huang CJ, Tsai PH, Wang ML, Yang YP, Lan YT, et al. Mapping a Circular RNA-microRNA-mRNA-Signaling Regulatory Axis That Modulates Stemness Properties of Cancer Stem Cell Populations in Colorectal Cancer Spheroid Cells. Int J Mol Sci (2020) 21(21):7864. doi: 10.3390/ijms21217864
70. Ali Syeda Z, Langden SSS, Munkhzul C, Lee M, Song SJ. Regulatory Mechanism of MicroRNA Expression in Cancer. Int J Mol Sci (2020) 21(5):1723. doi: 10.3390/ijms21051723
71. Tam SY, Wu VWC, Law HKW. Hypoxia-Induced Epithelial-Mesenchymal Transition in Cancers: HIF-1α and Beyond. Front Oncol (2020) 10:486. doi: 10.3389/fonc.2020.00486
72. Rao G, Wang H, Li B, Huang L, Xue D, Wang X, et al. Reciprocal Interactions Between Tumor-Associated Macrophages and CD44-Positive Cancer Cells via Osteopontin/CD44 Promote Tumorigenicity in Colorectal Cancer. Clin Cancer Res (2013) 19(4):785–97. doi: 10.1158/1078-0432.Ccr-12-2788
73. Sharma M, Fonseca FP, Hunter KD, Radhakrishnan R. Loss of Oral Mucosal Stem Cell Markers in Oral Submucous Fibrosis and Their Reactivation in Malignant Transformation. Int J Oral Sci (2020) 12(1):23. doi: 10.1038/s41368-020-00090-5
74. Katoh M. Genomic Testing, Tumor Microenvironment and Targeted Therapy of Hedgehog-Related Human Cancers. Clin Sci (Lond) (2019) 133(8):953–70. doi: 10.1042/cs20180845
75. Zhong Q, Fang Y, Lai Q, Wang S, He C, Li A, et al. CPEB3 Inhibits Epithelial-Mesenchymal Transition by Disrupting the Crosstalk Between Colorectal Cancer Cells and Tumor-Associated Macrophages via IL-6r/STAT3 Signaling. J Exp Clin Cancer Res (2020) 39(1):132. doi: 10.1186/s13046-020-01637-4
76. Zhang D, Qiu X, Li J, Zheng S, Li L, Zhao H. TGF-β Secreted by Tumor-Associated Macrophages Promotes Proliferation and Invasion of Colorectal Cancer via miR-34a-VEGF Axis. Cell Cycle (2018) 17(24):2766–78. doi: 10.1080/15384101.2018.1556064
77. Liu C, Yao Z, Wang J, Zhang W, Yang Y, Zhang Y, et al. Macrophage-Derived CCL5 Facilitates Immune Escape of Colorectal Cancer Cells via the p65/STAT3-CSN5-PD-L1 Pathway. Cell Death Differ (2020) 27(6):1765–81. doi: 10.1038/s41418-019-0460-0
78. Sun P, Wang H, He Z, Chen X, Wu Q, Chen W, et al. Fasting Inhibits Colorectal Cancer Growth by Reducing M2 Polarization of Tumor-Associated Macrophages. Oncotarget (2017) 8(43):74649–60. doi: 10.18632/oncotarget.20301
79. Rahmani M, Mohammadnia-Afrouzi M, Nouri HR, Fattahi S, Akhavan-Niaki H, Mostafazadeh A. Human PBMCs Fight or Flight Response to Starvation Stress: Increased T-Reg, FOXP3, and TGF-β1 With Decreased miR-21 and Constant miR-181c Levels. BioMed Pharmacother (2018) 108:1404–11. doi: 10.1016/j.biopha.2018.09.163
80. Ye YC, Zhao JL, Lu YT, Gao CC, Yang Y, Liang SQ, et al. NOTCH Signaling via WNT Regulates the Proliferation of Alternative, CCR2-Independent Tumor-Associated Macrophages in Hepatocellular Carcinoma. Cancer Res (2019) 79(16):4160–72. doi: 10.1158/0008-5472.Can-18-1691
81. Li C, Xu J, Wang X, Zhang C, Yu Z, Liu J, et al. Whole Exome and Transcriptome Sequencing Reveal Clonal Evolution and Exhibit Immune-Related Features in Metastatic Colorectal Tumors. Cell Death Discov (2021) 7(1):222. doi: 10.1038/s41420-021-00607-9
82. Huang D, Sun W, Zhou Y, Li P, Chen F, Chen H, et al. Mutations of Key Driver Genes in Colorectal Cancer Progression and Metastasis. Cancer Metastasis Rev (2018) 37(1):173–87. doi: 10.1007/s10555-017-9726-5
83. Saitoh M. Involvement of Partial EMT in Cancer Progression. J Biochem (2018) 164(4):257–64. doi: 10.1093/jb/mvy047
84. Cai J, Xia L, Li J, Ni S, Song H, Wu X. Tumor-Associated Macrophages Derived TGF-β–Induced Epithelial to Mesenchymal Transition in Colorectal Cancer Cells Through Smad2,3-4/Snail Signaling Pathway. Cancer Res Treat (2019) 51(1):252–66. doi: 10.4143/crt.2017.613
85. Temraz S, Nassar F, Kreidieh F, Mukherji D, Shamseddine A, Nasr R. Hepatocellular Carcinoma Immunotherapy and the Potential Influence of Gut Microbiome. Int J Mol Sci (2021) 22(15):7800. doi: 10.3390/ijms22157800
86. Chen HL, Xing X, Zhang B, Huang HB, Shi CW, Yang GL, et al. Higher Mucosal Type II Immunity Is Associated With Increased Gut Microbiota Diversity in BALB/c Mice After Trichinella Spiralis Infection. Mol Immunol (2021) 138:87–98. doi: 10.1016/j.molimm.2021.07.014
87. Wan G, Xie M, Yu H, Chen H. Intestinal Dysbacteriosis Activates Tumor-Associated Macrophages to Promote Epithelial-Mesenchymal Transition of Colorectal Cancer. Innate Immun (2018) 24(8):480–9. doi: 10.1177/1753425918801496
88. Lan J, Sun L, Xu F, Liu L, Hu F, Song D, et al. M2 Macrophage-Derived Exosomes Promote Cell Migration and Invasion in Colon Cancer. Cancer Res (2019) 79(1):146–58. doi: 10.1158/0008-5472.Can-18-0014
89. Assmann TS, Cuevas-Sierra A, Riezu-Boj JI, Milagro FI, Martínez JA. Comprehensive Analysis Reveals Novel Interactions Between Circulating MicroRNAs and Gut Microbiota Composition in Human Obesity. Int J Mol Sci (2020) 21(24):9509. doi: 10.3390/ijms21249509
90. Pinto ML, Rios E, Durães C, Ribeiro R, Machado JC, Mantovani A, et al. The Two Faces of Tumor-Associated Macrophages and Their Clinical Significance in Colorectal Cancer. Front Immunol (2019) 10:1875. doi: 10.3389/fimmu.2019.01875
91. Waniczek D, Lorenc Z, Śnietura M, Wesecki M, Kopec A, Muc-Wierzgoń M. Tumor-Associated Macrophages and Regulatory T Cells Infiltration and the Clinical Outcome in Colorectal Cancer. Arch Immunol Ther Exp (Warsz) (2017) 65(5):445–54. doi: 10.1007/s00005-017-0463-9
92. Shibutani M, Maeda K, Nagahara H, Fukuoka T, Nakao S, Matsutani S, et al. The Peripheral Monocyte Count Is Associated With the Density of Tumor-Associated Macrophages in the Tumor Microenvironment of Colorectal Cancer: A Retrospective Study. BMC Cancer (2017) 17(1):404. doi: 10.1186/s12885-017-3395-1
93. Luo H, Zhao Q, Wei W, Zheng L, Yi S, Li G, et al. Circulating Tumor DNA Methylation Profiles Enable Early Diagnosis, Prognosis Prediction, and Screening for Colorectal Cancer. Sci Transl Med (2020) 12(524):eaax7533. doi: 10.1126/scitranslmed.aax7533
94. Zhao Y, Ge X, Xu X, Yu S, Wang J, Sun L. Prognostic Value and Clinicopathological Roles of Phenotypes of Tumour-Associated Macrophages in Colorectal Cancer. J Cancer Res Clin Oncol (2019) 145(12):3005–19. doi: 10.1007/s00432-019-03041-8
95. Feng Q, Chang W, Mao Y, He G, Zheng P, Tang W, et al. Tumor-Associated Macrophages as Prognostic and Predictive Biomarkers for Postoperative Adjuvant Chemotherapy in Patients With Stage II Colon Cancer. Clin Cancer Res (2019) 25(13):3896–907. doi: 10.1158/1078-0432.Ccr-18-2076
96. Väyrynen JP, Haruki K, Lau MC, Väyrynen SA, Zhong R, Dias Costa A, et al. The Prognostic Role of Macrophage Polarization in the Colorectal Cancer Microenvironment. Cancer Immunol Res (2021) 9(1):8–19. doi: 10.1158/2326-6066.Cir-20-0527
97. Invrea F, Punzi S, Petti C, Minelli R, Peoples MD, Bristow CA, et al. Synthetic Lethality Screening Highlights Colorectal Cancer Vulnerability to Concomitant Blockade of NEDD8 and EGFR Pathways. Cancers (Basel) (2021) 13(15):3805. doi: 10.3390/cancers13153805
98. Zhu Z, Hou Q, Guo H. NT5DC2 Knockdown Inhibits Colorectal Carcinoma Progression by Repressing Metastasis, Angiogenesis and Tumor-Associated Macrophage Recruitment: A Mechanism Involving VEGF Signaling. Exp Cell Res (2020) 397(1):112311. doi: 10.1016/j.yexcr.2020.112311
99. Liu H, Liang Z, Zhou C, Zeng Z, Wang F, Hu T, et al. Mutant KRAS Triggers Functional Reprogramming of Tumor-Associated Macrophages in Colorectal Cancer. Signal Transduct Target Ther (2021) 6(1):144. doi: 10.1038/s41392-021-00534-2
100. Feng Y, Liang Y, Zhu X, Wang M, Gui Y, Lu Q, et al. The Signaling Protein Wnt5a Promotes Tgfβ1-Mediated Macrophage Polarization and Kidney Fibrosis by Inducing the Transcriptional Regulators Yap/Taz. J Biol Chem (2018) 293(50):19290–302. doi: 10.1074/jbc.RA118.005457
101. Yang C, Dou R, Wei C, Liu K, Shi D, Zhang C, et al. Tumor-Derived Exosomal microRNA-106b-5p Activates EMT-Cancer Cell and M2-Subtype TAM Interaction to Facilitate CRC Metastasis. Mol Ther (2021) 29(6):2088–107. doi: 10.1016/j.ymthe.2021.02.006
102. Yan Z, Cheng M, Hu G, Wang Y, Zeng S, Huang A, et al. Positive Feedback of SuFu Negating Protein 1 on Hedgehog Signaling Promotes Colorectal Tumor Growth. Cell Death Dis (2021) 12(2):199. doi: 10.1038/s41419-021-03487-0
103. Heuberger J, Grinat J, Kosel F, Liu L, Kunz S, Vidal RO, et al. High Yap and Mll1 Promote a Persistent Regenerative Cell State Induced by Notch Signaling and Loss of P53. Proc Natl Acad Sci USA (2021) 118(22):e2019699118. doi: 10.1073/pnas.2019699118
104. Zhang T, Liu L, Lai W, Zeng Y, Xu H, Lan Q, et al. Interaction With Tumor−Associated Macrophages Promotes PRL−3−Induced Invasion of Colorectal Cancer Cells via MAPK Pathway−Induced EMT and NF−κb Signaling−Induced Angiogenesis. Oncol Rep (2019) 41(5):2790–802. doi: 10.3892/or.2019.7049
105. Li M, Lai X, Zhao Y, Zhang Y, Li M, Li D, et al. Loss of NDRG2 in Liver Microenvironment Inhibits Cancer Liver Metastasis by Regulating Tumor Associate Macrophages Polarization. Cell Death Dis (2018) 9(2):248. doi: 10.1038/s41419-018-0284-8
106. Wang Z, Yang Y, Cui Y, Wang C, Lai Z, Li Y, et al. Tumor-Associated Macrophages Regulate Gastric Cancer Cell Invasion and Metastasis Through Tgfβ2/NF-κb/Kindlin-2 Axis. Chin J Cancer Res (2020) 32(1):72–88. doi: 10.21147/j.issn.1000-9604.2020.01.09
107. Fei R, Zhang Y, Wang S, Xiang T, Chen W. α7 Nicotinic Acetylcholine Receptor in Tumor-Associated Macrophages Inhibits Colorectal Cancer Metastasis Through the JAK2/STAT3 Signaling Pathway. Oncol Rep (2017) 38(5):2619–28. doi: 10.3892/or.2017.5935
108. Hillmer EJ, Zhang H, Li HS, Watowich SS. STAT3 Signaling in Immunity. Cytokine Growth Factor Rev (2016) 31:1–15. doi: 10.1016/j.cytogfr.2016.05.001
109. Quero L, Tiaden AN, Hanser E, Roux J, Laski A, Hall J, et al. miR-221-3p Drives the Shift of M2-Macrophages to a Pro-Inflammatory Function by Suppressing JAK3/STAT3 Activation. Front Immunol (2019) 10:3087. doi: 10.3389/fimmu.2019.03087
110. Shi YN, Zhu N, Liu C, Wu HT, Gui Y, Liao DF, et al. Wnt5a and Its Signaling Pathway in Angiogenesis. Clin Chim Acta (2017) 471:263–9. doi: 10.1016/j.cca.2017.06.017
111. Liu Q, Song J, Pan Y, Shi D, Yang C, Wang S, et al. Wnt5a/CaMKII/ERK/CCL2 Axis Is Required for Tumor-Associated Macrophages to Promote Colorectal Cancer Progression. Int J Biol Sci (2020) 16(6):1023–34. doi: 10.7150/ijbs.40535
112. Lee GT, Kwon SJ, Kim J, Kwon YS, Lee N, Hong JH, et al. WNT5A Induces Castration-Resistant Prostate Cancer via CCL2 and Tumour-Infiltrating Macrophages. Br J Cancer (2018) 118(5):670–8. doi: 10.1038/bjc.2017.451
113. Chen Y, Chen L, Yu J, Ghia EM, Choi MY, Zhang L, et al. Cirmtuzumab Blocks Wnt5a/ROR1 Stimulation of NF-κb to Repress Autocrine STAT3 Activation in Chronic Lymphocytic Leukemia. Blood (2019) 134(13):1084–94. doi: 10.1182/blood.2019001366
114. Curigliano G, Shah RR. Safety and Tolerability of Phosphatidylinositol-3-Kinase (PI3K) Inhibitors in Oncology. Drug Saf (2019) 42(2):247–62. doi: 10.1007/s40264-018-0778-4
115. Zhao S, Mi Y, Guan B, Zheng B, Wei P, Gu Y, et al. Tumor-Derived Exosomal miR-934 Induces Macrophage M2 Polarization to Promote Liver Metastasis of Colorectal Cancer. J Hematol Oncol (2020) 13(1):156. doi: 10.1186/s13045-020-00991-2
116. Xue J, Ge X, Zhao W, Xue L, Dai C, Lin F, et al. Pipkiγ Regulates CCL2 Expression in Colorectal Cancer by Activating AKT-STAT3 Signaling. J Immunol Res (2019) 2019:3690561. doi: 10.1155/2019/3690561
117. Van der Jeught K, Xu HC, Li YJ, Lu XB, Ji G. Drug Resistance and New Therapies in Colorectal Cancer. World J Gastroenterol (2018) 24(34):3834–48. doi: 10.3748/wjg.v24.i34.3834
118. Cao D, Naiyila X, Li J, Huang Y, Chen Z, Chen B, et al. Potential Strategies to Improve the Effectiveness of Drug Therapy by Changing Factors Related to Tumor Microenvironment. Front Cell Dev Biol (2021) 9:705280. doi: 10.3389/fcell.2021.705280
119. DeNardo DG, Galkin A, Dupont J, Zhou L, Bendell J. GB1275, A First-in-Class CD11b Modulator: Rationale for Immunotherapeutic Combinations in Solid Tumors. J Immunother Cancer (2021) 9(8):e003005. doi: 10.1136/jitc-2021-003005
120. Larionova I, Tuguzbaeva G, Ponomaryova A, Stakheyeva M, Cherdyntseva N, Pavlov V, et al. Tumor-Associated Macrophages in Human Breast, Colorectal, Lung, Ovarian and Prostate Cancers. Front Oncol (2020) 10:566511. doi: 10.3389/fonc.2020.566511
121. Liu Z, Xie Y, Xiong Y, Liu S, Qiu C, Zhu Z, et al. TLR 7/8 Agonist Reverses Oxaliplatin Resistance in Colorectal Cancer via Directing the Myeloid-Derived Suppressor Cells to Tumoricidal M1-Macrophages. Cancer Lett (2020) 469:173–85. doi: 10.1016/j.canlet.2019.10.020
122. Wei C, Yang C, Wang S, Shi D, Zhang C, Lin X, et al. M2 Macrophages Confer Resistance to 5-Fluorouracil in Colorectal Cancer Through the Activation of CCL22/PI3K/AKT Signaling. Onco Targets Ther (2019) 12:3051–63. doi: 10.2147/ott.S198126
123. Dost Gunay FS, Kırmızı BA, Ensari A, İcli F, Akbulut H. Tumor-Associated Macrophages and Neuroendocrine Differentiation Decrease the Efficacy of Bevacizumab Plus Chemotherapy in Patients With Advanced Colorectal Cancer. Clin Colorectal Cancer (2019) 18(2):e244–50. doi: 10.1016/j.clcc.2018.12.004
124. Malesci A, Bianchi P, Celesti G, Basso G, Marchesi F, Grizzi F, et al. Tumor-Associated Macrophages and Response to 5-Fluorouracil Adjuvant Therapy in Stage III Colorectal Cancer. Oncoimmunology (2017) 6(12):e1342918. doi: 10.1080/2162402x.2017.1342918
125. Liu Y, Wen Y, Chen X, Zhu X, Yu Q, Gong Y, et al. Inflammation-Responsive Functional Ru Nanoparticles Combining a Tumor-Associated Macrophage Repolarization Strategy With Phototherapy for Colorectal Cancer Therapy. J Mater Chem B (2019) 7(40):6210–23. doi: 10.1039/c9tb01613a
126. Riley RS, June CH, Langer R, Mitchell MJ. Delivery Technologies for Cancer Immunotherapy. Nat Rev Drug Discov (2019) 18(3):175–96. doi: 10.1038/s41573-018-0006-z
127. Korehisa S, Oki E, Iimori M, Nakaji Y, Shimokawa M, Saeki H, et al. Clinical Significance of Programmed Cell Death-Ligand 1 Expression and the Immune Microenvironment at the Invasive Front of Colorectal Cancers With High Microsatellite Instability. Int J Cancer (2018) 142(4):822–32. doi: 10.1002/ijc.31107
128. Gordon SR, Maute RL, Dulken BW, Hutter G, George BM, McCracken MN, et al. PD-1 Expression by Tumour-Associated Macrophages Inhibits Phagocytosis and Tumour Immunity. Nature (2017) 545(7655):495–9. doi: 10.1038/nature22396
129. Rodriguez-Garcia A, Lynn RC, Poussin M, Eiva MA, Shaw LC, O'Connor RS, et al. CAR-T Cell-Mediated Depletion of Immunosuppressive Tumor-Associated Macrophages Promotes Endogenous Antitumor Immunity and Augments Adoptive Immunotherapy. Nat Commun (2021) 12(1):877. doi: 10.1038/s41467-021-20893-2
130. Tacconi C, Ungaro F, Correale C, Arena V, Massimino L, Detmar M, et al. Activation of the VEGFC/VEGFR3 Pathway Induces Tumor Immune Escape in Colorectal Cancer. Cancer Res (2019) 79(16):4196–210. doi: 10.1158/0008-5472.Can-18-3657
131. Xu W, Zhou G, Wang H, Liu Y, Chen B, Chen W, et al. Circulating lncRNA SNHG11 as a Novel Biomarker for Early Diagnosis and Prognosis of Colorectal Cancer. Int J Cancer (2020) 146(10):2901–12. doi: 10.1002/ijc.32747
132. Liang ZX, Liu HS, Wang FW, Xiong L, Zhou C, Hu T, et al. LncRNA RPPH1 Promotes Colorectal Cancer Metastasis by Interacting With TUBB3 and by Promoting Exosomes-Mediated Macrophage M2 Polarization. Cell Death Dis (2019) 10(11):829. doi: 10.1038/s41419-019-2077-0
133. Dempsey J, Zhang A, Cui JY. Coordinate Regulation of Long Non-Coding RNAs and Protein-Coding Genes in Germ-Free Mice. BMC Genomics (2018) 19(1):834. doi: 10.1186/s12864-018-5235-3
134. Li R, Zhou R, Wang H, Li W, Pan M, Yao X, et al. Gut Microbiota-Stimulated Cathepsin K Secretion Mediates TLR4-Dependent M2 Macrophage Polarization and Promotes Tumor Metastasis in Colorectal Cancer. Cell Death Differ (2019) 26(11):2447–63. doi: 10.1038/s41418-019-0312-y
135. Song W, Ma J, Lei B, Yuan X, Cheng B, Yang H, et al. Sine Oculis Homeobox 1 Promotes Proliferation and Migration of Human Colorectal Cancer Cells Through Activation of Wnt/β-Catenin Signaling. Cancer Sci (2019) 110(2):608–16. doi: 10.1111/cas.13905
136. Qu Y, Olsen JR, Yuan X, Cheng PF, Levesque MP, Brokstad KA, et al. Small Molecule Promotes β-Catenin Citrullination and Inhibits Wnt Signaling in Cancer. Nat Chem Biol (2018) 14(1):94–101. doi: 10.1038/nchembio.2510
137. Limagne E, Thibaudin M, Nuttin L, Spill A, Derangère V, Fumet JD, et al. Trifluridine/Tipiracil Plus Oxaliplatin Improves PD-1 Blockade in Colorectal Cancer by Inducing Immunogenic Cell Death and Depleting Macrophages. Cancer Immunol Res (2019) 7(12):1958–69. doi: 10.1158/2326-6066.Cir-19-0228
138. Pacheco-Fernández T, Juárez-Avelar I, Illescas O, Terrazas LI, Hernández-Pando R, Pérez-Plasencia C, et al. Macrophage Migration Inhibitory Factor Promotes the Interaction Between the Tumor, Macrophages, and T Cells to Regulate the Progression of Chemically Induced Colitis-Associated Colorectal Cancer. Mediators Inflamm (2019) 2019:2056085. doi: 10.1155/2019/2056085
139. Rendra E, Riabov V, Mossel DM, Sevastyanova T, Harmsen MC, Kzhyshkowska J. Reactive Oxygen Species (ROS) in Macrophage Activation and Function in Diabetes. Immunobiology (2019) 224(2):242–53. doi: 10.1016/j.imbio.2018.11.010
Keywords: colorectal cancer, tumor-associated macrophages, mechanism, tumor microenvironment, treatment
Citation: Li Y, Chen Z, Han J, Ma X, Zheng X and Chen J (2022) Functional and Therapeutic Significance of Tumor-Associated Macrophages in Colorectal Cancer. Front. Oncol. 12:781233. doi: 10.3389/fonc.2022.781233
Received: 22 September 2021; Accepted: 10 January 2022;
Published: 02 February 2022.
Edited by:
Zhanlong Shen, Peking University People’s Hospital, ChinaReviewed by:
Shuji Ogino, Brigham and Women’s Hospital and Harvard Medical School, United StatesCopyright © 2022 Li, Chen, Han, Ma, Zheng and Chen. This is an open-access article distributed under the terms of the Creative Commons Attribution License (CC BY). The use, distribution or reproduction in other forums is permitted, provided the original author(s) and the copyright owner(s) are credited and that the original publication in this journal is cited, in accordance with accepted academic practice. No use, distribution or reproduction is permitted which does not comply with these terms.
*Correspondence: Jinhong Chen, amluaG9uZ2NoQGhvdG1haWwuY29t
†These authors have contributed equally to this work and share first authorship
Disclaimer: All claims expressed in this article are solely those of the authors and do not necessarily represent those of their affiliated organizations, or those of the publisher, the editors and the reviewers. Any product that may be evaluated in this article or claim that may be made by its manufacturer is not guaranteed or endorsed by the publisher.
Research integrity at Frontiers
Learn more about the work of our research integrity team to safeguard the quality of each article we publish.