- 1Lecturer of Biomedical Sciences, Department of Biology, College of Natural and Computational Sciences, Arba Minch University, Arba Minch, Ethiopia
- 2Department of Microbial, Cellular and Molecular Biology, College of Natural and Computational Sciences, Addis Ababa University, Addis Ababa, Ethiopia
Cancer is one of the leading causes of death in the world, which is the second after heart diseases. Adenoviruses (Ads) have become the promise of new therapeutic strategy for cancer treatment. The objective of this review is to discuss current advances in the applications of adenoviral vectors in cancer therapy. Adenoviral vectors can be engineered in different ways so as to change the tumor microenvironment from cold tumor to hot tumor, including; 1. by modifying Ads to deliver transgenes that codes for tumor suppressor gene (p53) and other proteins whose expression result in cell cycle arrest 2. Ads can also be modified to express tumor specific antigens, cytokines, and other immune-modulatory molecules. The other strategy to use Ads in cancer therapy is to use oncolytic adenoviruses, which directly kills tumor cells. Gendicine and Advexin are replication-defective recombinant human p53 adenoviral vectors that have been shown to be effective against several types of cancer. Gendicine was approved for treatment of squamous cell carcinoma of the head and neck by the Chinese Food and Drug Administration (FDA) agency in 2003 as a first-ever gene therapy product. Oncorine and ONYX-015 are oncolytic adenoviral vectors that have been shown to be effective against some types of cancer. The Chiness FDA agency has also approved Oncorin for the treatment of head and neck cancer. Ads that were engineered to express immune-stimulatory cytokines and other immune-modulatory molecules such as TNF-α, IL-2, BiTE, CD40L, 4-1BBL, GM-CSF, and IFN have shown promising outcome in treatment of cancer. Ads can also improve therapeutic efficacy of immune checkpoint inhibitors and adoptive cell therapy (Chimeric Antigen Receptor T Cells). In addition, different replication-deficient adenoviral vectors (Ad5-CEA, Ad5-PSA, Ad-E6E7, ChAdOx1–MVA and Ad-transduced Dendritic cells) that were tested as anticancer vaccines have been demonstrated to induce strong antitumor immune response. However, the use of adenoviral vectors in gene therapy is limited by several factors such as pre-existing immunity to adenoviral vectors and high immunogenicity of the viruses. Thus, innovative strategies must be continually developed so as to overcome the obstacles of using adenoviral vectors in gene therapy.
1 Introduction
In the past few decades, gene therapy for diseases such as cancer using adenoviral vectors has been significantly advanced. Adenoviruses (Ads) can be used either as replication-competent Ads or replication-defective adenoviral vectors for gene therapy (1, 2). The following are the characteristics that made Ads one of the most favorable viruses for gene therapy:-1. Ads have unique ability to infect wide range of cell types (broad cell tropsim) and have the capacity to induce strong cell mediated immunity and humoral response (3); 2. The genetics of Ads has been well known and Ads have stable genome (4); 3. Ads have lytic replication cycle. This lytic replication cycle causes lysis of tumor cells (oncolysis) (5); 4. Adenoviral vectors have low pathogenicity and relatively safe and well-tolerated (4, 6); 5. Ads have large transgene carrying capacity about and can transduce both in dividing and non- dividing cells (4, 7, 8) and 6. Ads can infect professional antigen-presenting cells that are very effective in presenting antigens to T-cells (9). The objective of this review is to discuss current advances in applications of Ads in cancer therapy.
2 General Information On Adenoviruses
Ads are non-enveloped viruses with double stranded deoxy ribonucleic acid (DNA) genome. The genome of Ads ranges in size from 26 kb to 45 kb that is encompassed within icosahedral capsid (10, 11). The Ads virion size ranges from 90-100 nm in diameter. There are six kinds of proteins that constitute the adenoviral capsid: penton, fiber, hexon, IX, VIII, and IIIa. The IIIa involves in the assembly of the viral structure. The fiber and penton proteins involve in the attachment and entry of the Ads into host cells and the hexon constitutes most of the viral capsid (12, 13). The proteins IIIa, VIII, and IX make up the virion core, which are associated with the DNA genome. The VIII is important for the stability of the viral capsid (14–16).
The genes of Ads can be categorized into two classes: as early genes (five early genes) and late genes (five late genes). The Ads bind to host cell through its receptors, which includes scavenger receptors, CD46, integrin αvβ5 heparin sulfate proteoglycans, sialic acid etc) and gains entry into the cytoplasm (17–20).
After entry into the target host cell by micropinocytosis (21, 22), Ads first express the five early proteins that are coded by the five early genes (E1A, E1B, E2, E3 and E4 which are involved in protein synthesis and DNA replication. Structural proteins (L1-L5) are coded by the five late genes (15, 23, 24). Following replication, the adenoviral virion leaves the host cell by killing the host cell (lytic cycle). Newly produced Ads can infect wide range of host cells including antigen presenting cells and quiescent cells.
Ads belong to the family adenoviridae that is consisted of five genera: Mastadenoviruses, Avidadenoviruses, Siadenoviruses, Atadenoviruss and Ichtadenoviruse. The genus Mastadenovirus is consisted of Ads that infects humans (human adenoviruses) and non-human primates (25). The genus Aviadenovirus is consisted of Ads that are isolated from birds. Viruses that are isolated from birds, ovine, bovine, deer and possum belong to the genus Atadenoviruses. Ads that are isolated from fish belong to the genus Ichtadenoviruses. The genus Siadenovirus includes viruses that are isolated from invertebrates (25).
Human adenoviruses (HAds) are categorized into seven species (A-G) that are further divided into 57 serotypes (Ad1-Ad57) (1). Adenoviral serotyping is based on capsid proteins (VIII, hexon), phylogenetic distance (≥10%) in adenoviral genes that codes for protease, viral surface antigen neutralizing antibodies, and DNA polymerase (26–28).
HAds have worldwide distribution. Humans can be infected with more than one serotype or species of Ads that are usually acquired in early childhood, which leads to lifelong immunity (29). Ads account for 5% of common cold cases. Wild type Ads often cause mild illness in immunocomptetent individuals, which mainly affects the respiratory tract, eyes and digestive system (30). However, HAds causes severe illness in immunosuppressed individuals (31).
Ads induce diverse innate immune signaling pathways that result in the secretion of a number of proinflammatory cytokines. These proinflammatory cytokines result in the induction of robust adaptive humoral and cellular immune responses. The adaptive immune responses that develop against Ads include both T cells and neutralizing antibodies against the viral surface antigens such as hexon, penton, and fiber proteins (32–35).
Humans can be infected with different kinds of non-human Ads because of their broad tissue tropism and the structural similarity that they have with that of HAds. These characteristics subjected to use the non-human Ads as a vector for gene therapy and recombinant vaccine development so as to overcome the pre-existing antibodies that exists against human adenoviral vectors. There are numerous non-human adenoviral vectors that have used for recombinant vaccine development and gene therapy such as gorilla adenovirus vector (GC-46), chimpanzee adenoviral vectors (ChAdOx1 nCoV-19, ChAd1, ChAd2, ChAd3, ChAd5, ChAd6, ChAd7, and ChAd68); bovine adenoviral vectors; fowl adenoviral vectors; canine adenoviral vectors, ovine adenoviral vectors; porcine adenoviral vectors (36–40).
3 Construction Of Adenoviral Vectors
Adenoviruses have been engineered to make them efficient and safe vectors for human use as gene therapy and vaccine vectors by deleting certain genome sequences. So far three generations of adenoviral vectors have been developed to further improve the gene-carrying capacity and safety by deleting more genes. The three generations of adenoviral vectors are as follows:-
3.1 First-Generation Adenoviral Vectors
In the first generation, two genes are deleted (E1 and E3) so as to make the adenoviral vector replication defective, but keeping them to transduce host cells without killing them and liberating nearly 8 kb of space in the genome for the transgene and regulatory sequences (41, 42). These adenoviral vectors carry native tissue transduction capability and efficiently express the transgene in target host cells. The major challenge of the first-generation adenoviral vectors is immunogenicity and cellular toxicity.
3.2 Second Generation Adenoviral Vectors
In the second-generation adenoviral vectors, in addition to the E1/E3 genes, E2 and E4 regions are also deleted (43, 44). The second-generation adenoviral vectors provide additional space for larger cargo sequences (10.5 kb) and eliminated the possibility of generating replication-competent adenoviruses during amplification. Immunogenicity and cellular toxicity are still a major concern in the second-generation adenoviral vectors (45).
3.3 Third Generation Adenoviral Vectors
Third-generation adenoviral vectors are also called high capacity adenoviral vectors (HCAds) because they can accept cargo sequences up to 36 Kb (43, 46–48). The HCAds were generated by deleting all viral sequences except the ITRs and the packaging signal (49). For replication of third-generation adenovirus vectors in cell culture, instead of the complementation by the viral genes encoded by host cells, an additional adenoviral helper virus is provided. Therefore, the third-generation adenoviral vectors are also called helper dependent or gutless adenoviral vectors (50, 51).
Third-generation vectors have several benefits over first and second-generation adenoviral vectors, including less cellular toxicity and reduced immunogenicity and the HCAds can simultaneously encode multiple transgene cassettes (52, 53). The disadvantage of the HCAds is the fact that they are more complicated to first and second-generation adenoviral vectors and also have possibility of helper virus contamination (54).
4 Application Of Adenoviruses In Cancer Therapy
4.1 Cancer Immune Profiles: Immune Desert, Immune Excluded and Inflamed Tumor
Human cancers can be categorized into three immune profiles (immune phenotypes), depending on their immune status. The three types of cancer immune profiles include: immune deserts tumor, immune-excluded tumors and inflamed tumors. Immune deserts are tumors devoid of immune infiltration, antigen presentation (low major histocompatibility complex-I) and high tumor cells proliferation as seen in tumors such as Head and neck squamous cell carcinomas (HNSCC), glioblastomas, prostate cancer, pediatric malignancies, hormone receptor positive breast cancer (55–57). Immune excluded tumors are tumors with suppressed tumor microenvironment represented by T cells embedded in the tumor stromal microenvironment with high TGF-β signaling, myeloid inflammation, and angiogenesis (58–61). Inflamed tumors have an army of T-cells that are ready to destroy tumors inside the tumor micro-environment. Inflamed tumors are associated primarily with IFN-γ signaling, high tumor PD-L1, TILs, B cells, and intact antigen presentation (i.e., intact HLA and expression of MHC class I on the surface of tumor cells) (58, 62, 63).
The factors that derive suppression of anti-tumor immunity include; I. Increased frequencies of immune suppressive cells (such as T regulatory cells, and Myeloid-derived suppressor cells (MDSCs) (64–66); II. Impairing cytotoxic CD-8+ T-cells (CTLs) activation and infiltration (67–69); III. Up regulating immune checkpoint inhibitors receptors and their ligands (PD-1 and PD-L) (70); IV. Secretion of immunosuppressive cytokines (64, 65); and V. Escaping natural killer cells mediated killing of tumor (71).
Adenoviruses have become the promise of new therapeutic strategy for cancer treatment. Adenoviral vectors can be engineered in different ways so as to change the tumor microenvironment (TME) from cold tumor to hot tumor, including; 1. By modifying Ads to express cytokines, and other immune-modulatory molecules; 2. By modifying Ads to deliver tumor suppressor gene and code for tumor specific antigen. The other way to use Ads in cancer therapy is to use oncolytic adenoviruses, which directly kill tumor cells after replication (72–76)(Table 1).
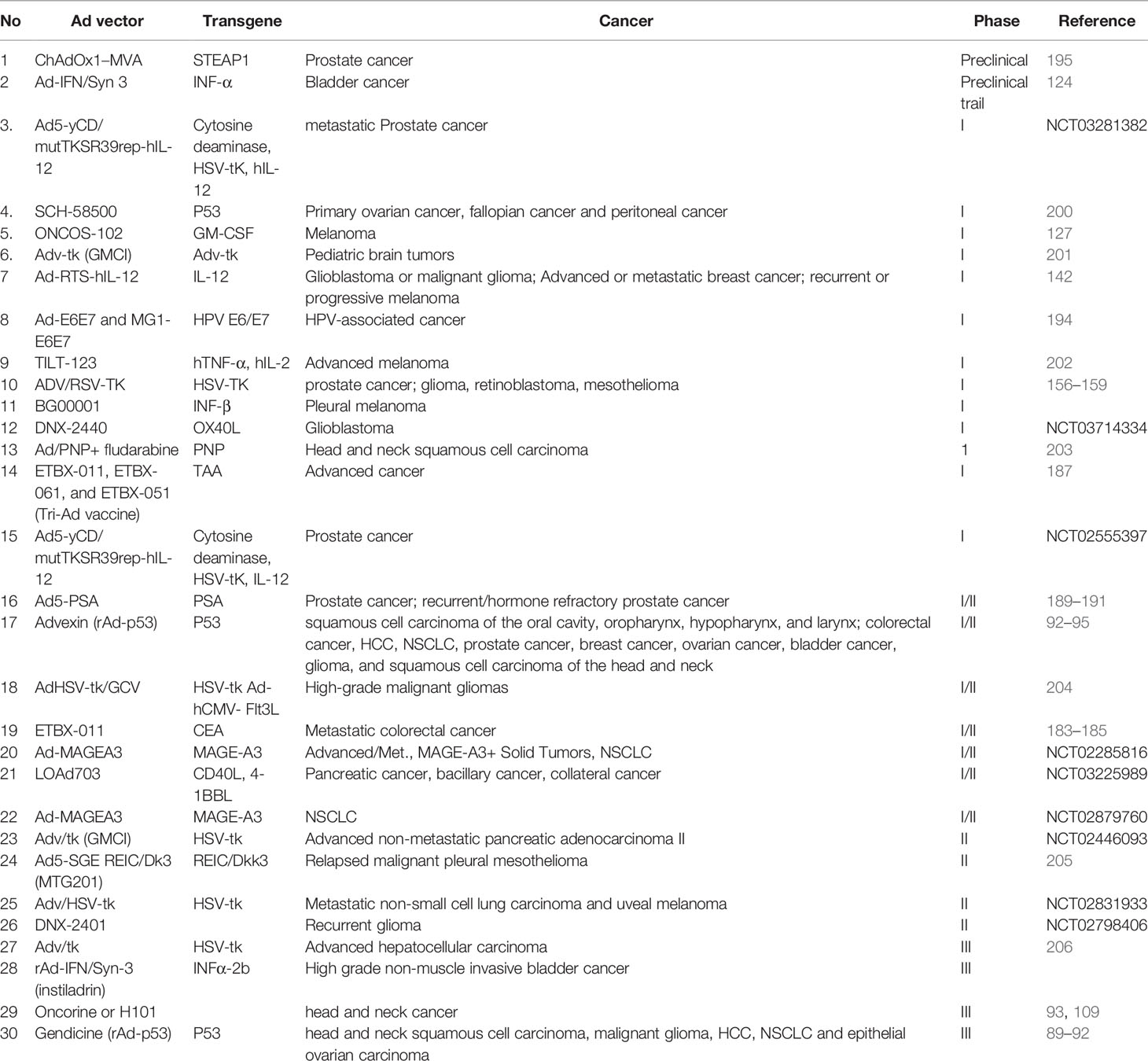
Table 1 Clinical trials using replicating and non-replicating adenoviral vectors for cancer therapy.
4.2 Adenoviral Vectors Coding for Tumor Suppressor Protein (p53)
One of the strategies that have been developed to use Ads in cancer therapy is to use replication-deficient adenoviral vectors to carry transgenes that codes for a tumor suppressor protein (p53) or proteins that induce apoptosis or cell cycle arrest (74, 76). Wild-type p53 prevents development of cancer by inhibiting the activation of oncogenes and inducing programmed cell death (apoptosis) when the cell’s DNA repair functions are insufficient to repair DNA damage (77). Suppression of p53 function is common in human cancers and 50% of cancers have mutations in the gene that codes for p53 protein (78, 79). For this reason, the p53 gene has become one of the target genes for transformation research of cancer gene therapy.
Gendicine is a replication-defective recombinant human p53 Ads vector (rAd-p53) expressing p53 proteins which inhibits the uncontrolled division of cancer cells and induces apoptosis of cancerous cells (84). Several studies that investigated the therapeutic efficacy of gendicine against HNSCC have showed good results. Gendicine combined with radiotherapy, chemotherapy, and other conventional treatment regimens demonstrated longer progression-free survival times than conventional treatments alone with no serious side effects except for transient fever or flu-like symptoms (80–87). For example, (80), conducted a clinical trial with 29 patients. Sixteen patients were treated with intratumoral injection of gendicine in combination with radiotherapy and 13 patients were treated with radiotherapy alone. The complete remission rate (also called complete response) in the patients treated with a combination of gendicine and radiotherapy was 5 times higher than the radiotherapy alone group (75% Vs 15%) (80).
Gendicine also showed promising results in treatment of cervical cancer patients. The 5-year overall survival rate of gendicine combined with radiotherapy group was 74.2% and the 5-year overall survival rate of the radiation alone group was 56.7%. Both the 5-year overall survival rate and disease free survival rate were significantly higher in the group treated with combination therapy (gendicine combined with radiotherapy) compared to the patients treated with radiation alone (88). In a clinical study of recurrent uterine sarcoma treated with gendicine combined with chemotherapy (89), the remission rate was 66.7%, and the disease control rate was 91.7%.
Gendicine was approved for treatment of squamous cell carcinoma of the head and neck by the Chinese Food and Drug Administration agency in 2003 as a first-ever gene therapy product to be used in combination with chemotherapy and have been in use for more than 15 years (90, 91). Gendicine has been also shown to be effective for treatment of different kinds of cancer in China including malignant glioma, epithelial ovarian carcinoma, Hepatocellular cancer (HCC), and Non-small cell lung cancer (NSCLC) (89–92).
Likewise, Advexin is a replication-defective recombinant human p53 adenoviral vector expressing p53 proteins. Advexin has a deletion on E3 and E1 genes that expresses a functional p53 protein from a Cytomegalovirus promoter (78, 79, 93). Advexin is similar to gendicine except that the p53 in gendicine is expressed from Rous Sarcoma Virus promoter (1). Advexin was proved efficacious against bladder cancer, ovarian cancer, prostate cancer, breast cancer, squamous cell carcinoma of the head and neck, hepatocellular carcinoma, colorectal cancer, squamous cell carcinoma of the oral cavity, oropharynx, hypopharynx, and larynx and non-small cell lung cancer (NSCLC) (92–95).
4.3 Oncolytic Adenoviruses as Anticancer Virotherapy
Oncolytic viruses are viruses that that specifically infect and replicate in a tumor cells and kill the cancer cells by their lytic replication (73, 75, 96). Oncolytic Ads, particularly oncolytic HAds are one of the leading candidate viruses for cancer virotherapy because of their good safety profile and high immunogenicity. Oncolytic Ads are genetically engineered Ads which acquired traits that enables them to infect and preferentially replicate in tumor cells (97). Oncolytic adenoviral vector technologies have been approved in some countries for treatment of cancer in humans (1, 75, 98). As compared with normal and quiescent cells, generally, tumor cells are more permissive to Ads (99), because of different reasons. The first reason is that the entire pattern of gene expression in cancer cells is conducive for Ad replication (100). The second reason is the fact that specific viral entry receptor is highly expressed in tumor cells. The other reason is the higher cell division and metabolic rate that take place in cancerous cells than that of normal and quiescent cells (101, 102). The advantage of an oncolytic Ads is not only to specifically replicate in and lyse tumor cells, but oncolytic adenoviruses can also stimulate potent anti-viral and anti-tumor immune responses for tumor-specific antigens that are released following lysis of Ads infected tumor cells (103–105).
Adenoviral vectors have been engineered to efficiently undergo oncolytic replication in cancer cells without replicating in healthy cells (106, 107). For example, ONYX-015 with a partial E1B gene deficiency is oncolytic adenoviral vector that infects and replicate in tumor cells that lacks p53 but unable to replicate in healthy cells expressing p53 (106). ONYX-015 has been demonstrated to be effective and well-tolerated oncolytic adenoviral vector that is reported to be more effective when given in combination with different cancer chemotherapies (107, 108). Oncorine (H101) is also a genetically modified oncolytic adenoviral vector expressing p53 gene. The Chinese food and drug administration agency has approved Oncorin for the treatment of head and neck cancer in combination with chemotherapy (93, 109).
4.4 Adenoviruses Expressing Immunomodulatory Molecules
Adenoviruses can be used in cancer therapy by modifying the viruses to stimulate antitumor immune response in different ways including by expressing cytokines, and other immune-modulatory molecules (72, 110–112).
4.4.1 Interferon Armed Adenoviruses
Interferons (IFNs) are the first group of cytokines that demonstrated efficacy in the treatment of malignancies. IFN signaling is mediated by binding of IFNs to their receptors and subsequent activation of Janus tyrosine kinase (JAK)-STAT signaling pathway (113). Interferon (IFN) has a strong antitumor effect and has been used in the treatment of pancreatic cancer. Some studies showed that IFN-α significantly prolongs survival rate (by 2 to 5 years) (114–116). However, there are limitations in using IFN-based therapies, including dose-limiting systemic toxicities and low intratumoral concentration of IFN because of its short half-life in the bloodstream (117, 118). In response to this, oncolytic adenoviruses have been engineered to express IFN, which showed positive outcomes in treatment of cancer.
Armstrong et al. (119, 120) has reported oncolytic adenoviruses expressing human IFN-α as a promising platform for selective, long-term expression of IFN in human pancreatic cancer tissues (119, 120). Armstrong et al. used the oncloytic adenovirus Ad5/Ad3-Cox2-ΔE3-ADP-IFN in their study, which was developed to selectively replicate within cancer cells expressing cyclooxygenase 2 (Cox2). In order to improve the infectivity and oncolysis of the oncolytic adenoviruses, they made genetic modification in the virus to include an Ad5/Ad3 chimeric fiber and overexpress the adenovirus death protein (ADP). The expression of adenovirus death protein (ADP) occurs during the late stage of infection a lytic infection. ADP promotes the release of progeny virus (virion) by accelerating the lysis and death of the host cell (121).
Similarly, other researchers have also reported as oncolytic adenoviruses expressing IFN-α have promising outcomes in treatment of cancer. The researchers reported that an oncolytic adenovirus (OAd-hamIFN) that was investigated in an immunocompetent Syrian hamster model of pancreatic ductal adenocarcinoma showed efficient viral replication in tumor, significant inhibition of tumor growth, and enhanced survival when used in combination with chemo therapies and radiation therapies (122, 123). Likewise, studies conducted by Tao etal. (124) have shown that significant tumor regression of bladder cancers occurred following administration of an adenovirus expressing human interferon α (Ad-IFNα) using a mouse superficial bladder cancer model in which human bladder tumors are growing (124).
4.4.2 GM-CSF Expressing Adenoviruses (ONCOS-102)
Granulocyte macrophage colony-stimulating factor (GM-CSF) promotes activation of T-cells and maturation of dendritic cells (125, 126). Therefore, an immunotherapy using adenoviruses that express GM-CSF transgene can be an effective anti-tumor therapy. ONCOS-102 is an oncolytic adenovirus that contains GM-CSF transgene (127). A clinical study (phase-I) in patients with advanced solid tumors including colon, lung, and ovarian cancers demonstrated a strong immune cell infiltrate into tumors without dose-limiting toxicities (127).
4.4.3 LOAd703 Expressing 4-1BBL and Trimerized CD40L
The other oncolytic adenovirus that expresses immunostimulatoy cytokine is LOAd703. LOAd703 is armed with 4-1BBL and trimerized CD40L that was shown to replicate and kill pancreatic cancer cells via oncolysis in both in vitro and in vivo assays (128).
4.4.4 TILT-123 (Expressing TNF- α)
TILT-123 is an oncolytic adenovirus that incorporates transgenes for human tumor necrosis factor alpha (TNF-α) and interleukin-2 (IL-2). TNF-α and IL-2 were shown to be promising T cell stimulating factors when used in combination with adoptive cell therapy (129, 130).
4.4.5 IL-12 and Other Cytokines Armed Adenoviruses
Interleukin-12 (IL-12) is a proinflammatory cytokine that initiates antitumor immune responses by promoting the generation of tumor-specific cytotoxic T lymphocytes (CTLs) and activating natural killer (NK) cells and CD4+ T cells (131, 132). Several studies demonstrated promising antitumor effects of IL-12 in mice having solid tumor and hematologic malignancies (133–137). However, findings from clinical trials indicated severe side effects of systemic administration of IL-12 that markedly dampened hopes of the successful use of this cytokine in cancer patients (138). But, the use of Ads containing IL-12 transgene seems critical for maximizing the density of IL-12 that reaches the tumors and alleviating the toxicity.
Ads that were engineered to express IL-12 have been shown to enhance immune stimulation and antitumor effect in a clinical trial (139) and pre-clinical studies (140). Likewise, a study by Wang et al. showed that oncolytic adenovirus (Ad-TD-nsIL-12) expressing IL-12 induces strong antitumor immune response against pancreatic cancer in Syrian hamster models without toxicity (141). Similarly, a replication-deficient adenoviral vector encoding human IL-12 p70 transgene (Ad-RTS-hIL-12) was also shown to have no toxic effect in phase one clinical trial (142). A recent trial found that intratumoral injection of Ad-RTS-hIL-12 was safe in patients with recurrent glioblastoma (142).
In addition to IL-12, there are also other cytokines, such as IL-24 and IL-13 that have been used to arm adenoviruses and have shown promising immune-activating properties in multiple preclinical cancer models (143–145). RANTES is another cytokine engineered in Ad that has been shown to enhance anticancer effect. In murine models of mammary adenocarcinoma and lymphoma, Ad-RANTES-E1A eradicated established tumors and inhibited metastases by recruiting DCs, macrophages, NK cells, and CD8+ T cells into the immunologically cold tumors (146).
4.4.6 Adenoviruses Armed With Bispecific T Cell Engager (BiTE)
Bispecific T cell engager (BiTE) is a kind of artificial antibody that represents an innovative immunotherapy approach which enhances patients’ immune response to tumors. BiTE has dual antigen specificity, allowing them to bind to two unique antigens at the same time, i.e. the BiTE bind simultaneously to both tumor associated antigen and T cell (usually CD3), ultimately stimulating T-cell activation, tumor killing and cytokine production (147). BiTE has been shown to be promising immunotherapy for the treatment of cancer in preclinical and clinical studies (148, 149). The therapeutic efficacy of BiTE can be improved by using BiTE in conjunction with adenoviruses.
Pomés et al. tested weather an OAd expressing FAP-targeting BiTE improve antitumor efficacy (150). FAP-BiTE (FBiTE) comprises two single chain variable fragments (ScFvs) joined by a flexible Gly-Ser linker. One scFv arm binds mouse and human Fibroblast Activation Protein (m/hFAP), while the other binds human CD3epsilon on the T cell receptor (TCR). The study showed that the FBiTEs activate and re-direct T-cells to FAP+ cells, which leads to enhance cytotoxicity in vitro and improve antitumor efficacy in vivo.
Likewise, Alemany group engineered an oncolytic adenovirus expressing an EGFR-targeting BiTE that showed improved T cell-mediated killing of cancer cells both in vivo and in vitro (151). They also demonstrated that anti-EGFR BiTE-armed OAd in combination with adoptive CAR-T cell therapy results in improved antitumor efficacy and prolonged survival of mice as a result of intratumoral T cell activation by BiTE (152).
Similarly, another research group (Fisher group) that developed BiTE armed oncolytic adenovirus (EnAd-SA-EpCAM) reported promising results in use of BiTE expressing adenoviruses against cancer (153). The BiTE of EnAd-SA-EpCAM binds to epithelial cell adhesion molecule (EpCAM) in cancer cells. The Fisher group reported that the EnAd-SA-EpCAM effectively activate endogenous T cells within the immune-suppressive microenvironment and exhibited killing of endogenous tumor cells without the addition of exogenous T cells (153).
Recently, the Fisher group engineered another BiTE armed oncolytic adenovirus (EnAd-FAP-BiTE), which is targeted fibroblast activation protein (FAP) in cancer-associated fibroblasts (CAFs). CAFs are the main cellular component of solid tumor TME. The EnAd-FAP-BiTE induced the activation of tumor-infiltrating T cells that target and kill CAFs (154). Likewise, another BiTE armed adenovirus (ICO15K-FBiTE) that was developed by the Fisher research group was shown to enhance overall antitumor efficacy without increasing the toxicity in mouse model (154).
4.4.7 ADV/HSV-TK
ADV/HSV-TK is an adenoviral vector expressing the herpes simplex virus (HSV) thymidine kinase (TK) gene. The HSV-TK protein has two principal functions, including 1. TK is a superantigen that stimulates a potent immune reaction and 2. A nucleotide analog product of prodrug phosphorylation lead to the death of dividing cancer cells (155). Herman et al. studied ADV/HSV-TK in combination with ganciclovir for the treatment of human prostate cancer (156). They reported that injection of ADV/HSV-TK into the prostate gland in the region with the greatest concentration of tumor cells resulted in significant reduction in tumor burden. Herman et al. also showed that ADV/HSV-TK was proven safe, with minimal toxicity (156). Likewise, other researchers also made similar observation on the efficacy and toxicology of ADV/HSV-TK against glioma, retinoblastoma, and mesothelioma (157–159).
4.5 Combination Therapy Using Adenoviruses and Chimeric Antigen Receptor (CAR) T Cells
One of the strategies that have been used for the immunotherapy of cancer is adoptive cell therapy, that includes chimeric antigen receptor (CAR) T cells, tumor-infiltrating lymphocytes (TIL), and T cell receptor modified (TCR) T cells (160). TCR-T cells are designed to encode receptors that specifically recognize cancer-specific antigens, and function through Major Histocompatibility Complex (MHC)-dependent mechanism, that limits their use (161). Whereas, CAR-T cell therapy functions through MHC-independent mechanism. CAR-T cell has been effective in the treatment of different types of cancer, which includes chronic lymphocytic leukemia and non-Hodgkin’s lymphoma (162, 163). However, the use of CAR-T cells as a monotherapy has not demonstrated much success in solid tumors because of immunosuppressive tumor microenvironment (TME) and poor tumor infiltration of CAR-T cells (164). Combination therapy with adenoviruses viruses provides one potential strategy for improvement of CAR-T therapy in solid tumors.
A study conducted by Watanabe and his colleagues demonstrated that the combination of oncolytic adenoviruses (expressing TNF-α and/or IL-2) and mesothelin-redirected CAR-T cells (meso-CAR-T) overcomes the immunosuppressive nature of the pancreatic cancer TME (165). Watanabe et al. demonstrated that tumors treated with the combination of the virus expressing TNF-α and IL-2 (Ad5/3-E2F-d24-TNF-α-IRES-IL-2 (OAd-TNFα-IL2) and meso-CAR-T cells were infiltrated with significantly more CD4+ and CD8+ T cells compared to monotherapy with meso-CAR-T cells, or a combination with meso-CAR-T cells and the parent adenovirus lacking cytokine expression (165). They also showed that meso-CAR-T cells in combination with OAd-TNFα-IL2 resulted in significantly higher accumulation of CAR-T cells at the tumor site when compared to meso-CAR-T monotherapy.
Likewise, a study that was done by a Suzuki group demonstrated better efficacy of CAR-T cell in treatment of prostate cancer when used in combination with oncolytic adenoviruses. In order to improve the efficacy of the CAR-T cell therapy, this research group (Suzuki group) used a combinatorial adenovirus vector (oncolytic adenovirus (Ad5Δ24) and helper-dependent adenovirus expressing a mini anti-PD-L1 antibody (HDAdPD-L1) collectively termed CAd-VECPDL1) in combination with human epidermal growth factor receptor 2 (HER2)-specific CAR-T cells (166). The findings showed that using this combination in an NSG mouse model was more effective in reducing tumor size and prolong survival of mice with prostate cancer (166).
The Suzuki group further modified the CAd-VECPDL1 vector by incorporating IL-12 (CAdVECIL12_PDL1) and tested it in a head and neck squamous cell carcinoma (HNSCC) model (167, 168). In a xenograft model of NSG mice (NOD scid gamma mouse), the combination of HER2-CAR-T cells and CAdVECIL12_PDL1 virus significantly prolonged survival of treated animals to more than 100 days as compared to 21–24 days in the control groups, and HER2-CAR-T cells were detected in the tumors of surviving mice over 100 days after initial therapy (168). The research group also used an orthotopic HNSCC model, establishing both primary tumors and lymphatic metastases, to test the aforementioned combination therapy. Mice that received both HER2-CAR-T cells and CAdVECIL12_PDL1 had improved tumor growth control at both primary and metastatic sites, maintained body weight, and had prolonged survival when compared to untreated and monotherapy groups (168). Taken together, combination therapy with oncolytic viruses provides one potential strategy for improvement of CAR-T therapy in solid tumors.
Tumor xenograft animal models provide us a research tool for preclinical drug response evaluation by determining anti-tumor efficacies; toxicity, tumorgenesis, pharmacokinetics and pharmacodynamics (169, 170). In addition, this research tool enables to have a better understanding on the involvement of certain oncogenes or tumor suppressors in tumor development (170). Mice are the most commonly used animals for tumor xenograft models. The advantageous of using mice as tumor xenograft models include: - I. The presence of comparable genome size with humans, II. Short reproductive cycle, III. Large litter size; IV. Low cost; V. Ease of manipulation (170).
4.6 Combination Therapy Using Adenoviruses and Antibodies Against Immune Checkpoint Proteins
4.6.1 Immune Checkpoint Inhibition
Immune checkpoints are regulators of the immune system. Immune checkpoints pathways prevent the immune system from attacking its own cells. Immune checkpoints works by means of immune check point proteins, including programmed cell death 1 protein (PD-1) and programmed cell death protein-ligand 1 (PD-L1), and cytotoxic T-lympotcyte antigen-4 (CTLA-4). One of the mechanisms by which tumor cells evade immunesurveillance is by activation of immune checkpoint pathways that suppress antitumor immune responses. PD-1 is the transmembrane programmed cell death 1 protein, which interacts with PD-L1 (PD-1 ligand 1, or CD274). The binding of PD-L1 on cancerous cell with PD-1 on T-cell surface results in inhibition of T-cell activity. Therefore, antibodies that bind with PD-L1 and or PD-1 can block the interaction of PD-L1 and PD-1, allowing T-cells to attack the tumor.
Immune checkpoint inhibitors therapy is a kind of immunotherapy that work by blocking the binding of checkpoint proteins (PD-1, PD-L and CTLA-4) with partner proteins so that T-cell became free and active to attack cancer cells. Ipilimumab is an anti-CTLA-4 antibody (that blocks CTLA-4 ligand and prevents inhibition T-cells) that was approved by the United States food and drug administrations (U.S. FDA) in 2010 for the treatment of advanced melanoma (171). However, the systemic administrations of immune checkpoint inhibitors have been shown to cause severe immune-related adverse events (172–174). One of the strategies that can be employed to overcome these obstacles of using immune checkpoint inhibitors (such as anti-PD-L1, anti-PD-1 and anti-CTLA-4) in cancer therapy is to utilize immune checkpoint inhibitors in combination with oncolytic adenoviruses.
4.6.1.1 Ad5/3-Δ24: Adenovirus Expressing Anti-CTLA-4 Antibodies
Ad5/3-Δ24 is an oncolytic adenovirus that was engineered to code for anti-CTLA4 antibody (175). Promising results have been achieved with the oncolytic adenovirus armed with anti-CTLA-4 antibodies (Ad5/3-Δ24) in mouse model. The local expression of anti-CTLA-4 antibody following administration of Ad5/3-Δ24-CTLA4 resulted in activation of T cells (175). In addition, a significantly higher concentration of antitumor antibody was produced, while plasma levels remained at safe concentrations. The anti-CTLA-4 antibodies also showed direct proapoptic effect both in vivo and in vitro (175).
4.6.1.2 Ad5-CMV-mIL2 and Ad5-CMV-mTNF- α in Combination With Anti-PD-1 Antibodies
Cervera-Carrascon and his colleagues that tested nonreplicating vectors expressing IL-2 (Ad5-CMV-mIL2) and TNF-α (Ad5-CMV-mTNF- α) in combination with programmed cell-death protein 1 (PD-1) blocking antibodies in a mouse model demonstrated complete regression of murine melanoma tumors, and prolonged survival of mice (176). Furthermore, they showed that the viral infection shifted the cytokine profile of the tumor microenvironment towards T-helper type 1, indicating that non-replicating adenoviral vectors significantly improve antitumor immunity (176).
4.6.1.3 DNX-2401 (Tasadenoturev) in Combination With Pembrolizumab
DNX-2401 is a replication-competent oncolytic adenovirus that selectively infects cancer cells lacking the normal retinoblastoma (Rb) protein signaling pathway (177). Aiken et al. tested DNX-2401 in combination with intravenous pembrolizumab (PD-1 immune checkpoint inhibitor) in patients with recurrent glioma and the findings showed that treatment of glioma with combination of DNX-2401 and pembrolizumab significantly improves the disease burden (178).
4.6.1.4 ONCOS102 in Combination With Pembrolizumab
Li et al. investigated the recombinant oncolytic adenovirus ONCOS-102 in combination with the antibody pembrolizumab (PD-1 immune checkpoint inhibitor) in patients with locally advanced or unresectable melanoma (161). They reported that combination therapy with ONCOS-102 and pembrolizumab resulted in regression of the disease and increases in circulating proinflammatory cytokines, and tumor specific T cells without dose limiting toxicities (161).
4.7 Adenoviral Vectors as Recombinant Anticancer Vaccines
Adenoviral vectors can also be used as a platform for anticancer vaccine development. This is based on the fact that adenoviral vectors can be engineered to stimulate antitumor immune response by expressing tumor-antigens. Replication-deficient adenoviral vectors are one of the viral vectors that have been extensively used as recombinant cancer vaccines as they cause potent cell mediated (cytotoxic CD8 T cells responses) and humoral immune response against transgenes expressed by the adenoviral vectors (1).
Cytotoxic CD8+ T cells are major constituent of anti-cancer immunity. The TCR of CD8+ T cells recognize tumor antigen presented by MHC-I and when bound, the cytotoxic CD8+ T cells triggers its cytotoxic activity. However, some tumor cells lower their MHC-I expression and avoid being detected by cytotoxic CD8+ T cells (179, 180). Another way that tumor cells use to escape cytotoxic CD8+ T cells by is to stop expressing molecules essential for co-stimulation of cytotoxic CD8+ T cells such as CD 86 or CD80 (181, 182).
4.7.1 ETBX-011(Ad5-CEA)
ETBX-011(also called Ad5-CEA) is an adenoviral vector-based cancer vaccine that is engineered to express a modified carcinoembryonic antigen (CEA) which contains the highly immunogenic epitope CAP1-6D. CEA is found in different kinds of cancer cells. ETBX-011 induces potent CEA-specific cell-mediated immune responses with antitumor activity (183). ETBX-011 is well-tolerated in metastatic colorectal cancer patients and has potential survival benefit (184, 185). This study showed evidence of a potential survival benefit: 25 patients treated at least twice with ETBX-011 exhibited a 12-month overall survival probability of 48% and a mean overall survival of 11 months (184, 185).
In order to overcome the challenge posed by tumor heterogeneity, such as the diversity of tumor associated antigens (TAA), a Tri-Ad vaccine (a combination of ETBX-011 with three different human TAA-expressing Ad vector vaccines (ETBX-011, ETBX-061, and ETBX-051) have been developed and tested in phase I clinical trial. The first, ETBX-061, is an Ad5-based adenovirus vector vaccine with the same backbone as the ETBX-011, but expressing a modified human mucin 1 (MUC1) gene. The modified MUC1 gene contains agonist epitopes designed to increase CTL antitumor immune responses. The other, ETBX-051 or Ad5-brachyury, encodes the entire brachyury gene with a deletion of 25 amino acids involved in DNA binding, and modified to express an enhancer T cell epitope (186). The Tri-Ad vaccine regimen induces antitumor cytotoxic T cell (CTL) responses and was proven safe and well tolerated in treatment of advanced cancer (187).
4.7.2 Ad5-PSA
Ad5-PSA is replication-deficient adenoviral vector that is engineered to express human prostate specific antigen (PSA). Ad5-PSA stimulates potent anti-PSA T cell responses and causes the destruction of PSA-secreting tumor cells both in preclinical (188) and clinical (189–191). Furthermore, Ad5-PSA prolongs survival and was demonstrated safe in patients with recurrent and hormone refractory prostate cancer (189–191).
4.7.3 Ad-E6E7 in Combination With and Ad-MAGEA3
Ad-E6E7 is another replication-deficient adenovirus-based anti-cancer vaccine, which expresses human papillomavirus (HPV) genes E6 and E7 (192, 193). MG1-E6E7 is an oncolytic maraba virus strain which also expresses the HPV genes E6 and E7. The combination of Ad-E6E7 and MG1-E6E7 induced potent tumor-specific responses in various mouse cancer models (194). In addition, the combination of Ad-E6E7 with MG1-E6E7 was proven to significantly prolong survival of mice with HPV-associated cancer (194).
4.7.4 ChAdOx1–MVA
Cappuccini et al. investigated the immunogenicity and efficacy of ChAdOx1–MVA against prostate cancer in mouse model (195). They reported that the ChAdOx1–MVA induced tumor specific cell mediated immune response. Furthermore, the ChAdOx1–MVA was proven to prolong the survival of the mice when used in combination with anti-PD-1 antibody (195).
4.7.5 Adenovirus-Transduced Dendritic Cells
The other approach to use adenoviral vectors for anticancer vaccine is the use Dendritic cells (DC)-based adenoviral vaccines. In this approach, DCs are transduced ex vivo with Ads encoding cancer specific antigens. The advantageous of transducing DC ex vivo instead of injecting Ads in vivo include: 1. Ex vivo transduction of DC overcomes pre-existing anti-viral immunity and induce effective anti-tumor responses (196, 197) and 2. Ex vivo transduced DC induces lower anti-viral antibody responses than that of Ads injected in vivo (196, 198).
Ad-transduced DCs have shown positive outcomes against both solid tumor and hematologic cancers (4, 66, 199). Butterfield et al. (199) tested an autologous DCs transduced ex vivo with Ads encoding the full-length melanoma antigen MART-1/Melan-A. They reported that the autologous DC-based adenoviral vaccine significantly induced cell mediated immune response (CD+8-T cells response) in metastatic melanoma patients (199). Similarly, in patients with advanced NSCLC, injections of autologous DCs resulted in induction of systemic tumor antigen-specific immune responses with enhanced CD8+T-cell infiltration (4).
4.7.6 Recombinant Gorilla Adenovirus HPV Vaccine (PRGN-2009)
Pellom et al. evaluated PRGN-2009, a therapeutic gorilla adenovirus HPV vaccine containing multiple cytotoxic T cell epitopes of the viral oncoproteins HPV 16/18 E6 and E7, including T cell enhancer agonist epitopes. The study revealed that PRGN-2009 treatment reduced tumor volume and increased CD8+ and CD4+ T cells in the tumor microenvironment of humanized mice bearing the human cervical tumor SiHa. The PRGN-2009 monotherapy in the syngeneic TC-1 model also reduced tumor volumes and weights, generated high levels of HPV16 E6–specific T cells, and increased multifunctional CD8+ and CD4+ T cells in the tumor microenvironment (40).
5 CHALLENGES AND SOLUTIONS OF USING ADENOVIRAL VECTORS IN CANCER THERAPY
The application of adenoviral vectors in cancer therapy and vaccine development is severely hampered by different factors including pre-existing immunity to the most common Ad vectors infecting the human population (207, 208); immunodominance of adenoviral antigens over the vaccine transgene antigen(s), and heterologous immunity with other pathogens (209, 210). The following are the details of the challenges and solutions of using adenoviral vectors in cancer therapy and vaccine development.
5.1 Pre-Existing Immunity in the Host
Pre-existing immunity of Ad vectors infecting the human population is the major factor that hampers the application of adenoviral vectors in cancer therapy and vaccine development (207, 208). The HAdV5 is the common serotype that infects humans, especially in developing countries (33, 211). HAd capsid proteins, particularly the hexon protein is very immunogenic (212). The host’s adaptive immunity arm detects the hexon protein and releases serotype-specific neutralizing antibodies (nAb) that block a post-entry step (213, 214). Therefore, during second contact with the same adenovirus serotype, the host nAb could rapidly neutralize it. In addition to the humoral immune response (antibodies), strong and sustained CD8+ T-cell responses follow adenoviral infections (3). Up to one-third of circulating T-cells against HAd have been reported to be CD4+ T-cells specific for a hexon epitope conserved between HAd serotypes. Thus, the host’s preexisting CD4+ T-lymphocytes might promptly respond to various subsequent adenovirus serotypes in either blood or gut (215). The following are the strategies that have been used to overcome the challenges of preexisting immunity in using adenoviral vectors.
5.1.1 Use of Non-Human Adenoviral Vectors
Non-human adenoviruses have been used to overcome the challenge of preexisting human adenoviral immunity. The commonly used nonhuman adenovirus vectors include gorilla adenovirus and chimpanzee-derived adenovirus vector (ChAd) (40). Several chimpanzee adenoviral vector-based vaccines, such as ChAd7 for Ebola virus, ChAd6 for rabies, and ChAd6, ChAd7, and ChAd9 for malaria, have shown high efficacy in animal models (216). Furthermore, ChAd63-based malaria and ChAd3-based hepatitis C virus vaccines have shown to be safe and highly immunogenic in phase I clinical trial (217, 218). Despite low seroprevalence of ChAd vectors in humans, pre-existing cross-reactive T cells against many conserved viral antigens are still a major concern. HAd-induced ChAd cross-reactive T cells have been reported against ChAd6, ChAd7, ChAd24, ChAd32, and ChAd68 (35, 38, 219, 220).
In addition to chimpanzee adenoviruses, several other adenoviruses derived from animals such as bovine, porcine, ovine, canine, and fowl are have been explored for vector development (221–224). The human population lack nAbs against these adenoviruses, and therefore, the vectors derived from these adenoviruses could be more efficacious in comparison to HAd and ChAd. The mouse models with experimentally induced pre-existing immunity by the administration of HAd vector demonstrated a lack of nAbs and CD4+ T cells against PAd3 and BAd3 (225). Furthermore, BAd3- or PAd3-based influenza virus vaccine demonstrated high efficacy even in the presence of pre-existing HAd5 immunity. There was also no effect of pre-existing HAd5 immunity on transgene expression, immunogenicity, and efficacy in animal models.
5.1.2 Heterologous Prime-Boost Strategy
Heterologous prime-boost strategy is another strategy to avoid pre-existing adenoviral vector immunity. Unlike homologous prime-boost immunizations, in this strategy, the priming and boosting are done by using different antigen delivery vehicle and/or vectors derived from either different serotypes of the same species or vectors from completely different host species. For instance priming with ChAd68 and boosting with ChAd1 or priming with DNA and boosting with HAd5 (226). Studies have shown that the heterologous prime-boost induces more robust immune responses compared to single vaccination or homologous prime-boost immunizations. The cellular immune responses induced by DNA prime and HAd boost were not affected by pre-existing HAd5 immunity. A preclinical study involving Plasmodium or SARS antigens encoded by Modified Vaccinia Ankara (MVA)/adenoviral vector as prime/boost showed induction of robust T cell and Ab responses of higher magnitude compared to Ad/DNA regimens (218, 227–229).
5.1.3 Routes of Immunization
Studies showed that different route of vaccinations can overcome the detrimental effect of pre-existing immunity. This result is partly due to evasion of tissue-resident Ad-specific T cells when using different routes of immunization. Tissue resident CD8 memory T cells are not systemic and do not prevent Ad vector infection in distant tissues (230–232). In preclinical study, HAd5-induced protective immune responses by intranasal/intratracheal immunization were not affected by pre-existing HAd5 immunity that had been induced by intramuscular administration of an unrelated HAd5 vector (233).
5.2 Immunodominance Over Transgene Immunity
Adenoviruses induce potent cell and antibody immune responses. A study has shown that adenoviral-derived epitopes can dominate over the transgene-derived epitopes and hinder the induction of transgene-specific immunity. This impairment of transgene-specific immune responses in naive vaccines is due to immune competition. Epitopes derived from an adenovirus vector were shown to inhibit the induction of HIV GagL85-93-specific CD8+ T cells (234). This study demonstrated that competition occurs at the level of responding CD8+ T cells, and co-immunization with an interleukin 2-encoding plasmid restored GagL85-93-specific CD8+ T cell responses in the presence of an adenoviral hexon486-494 epitope. The study suggests that adenoviral antigen-specific T-cell immunity is primed efficiently during adenoviral vector-based immunization, which can limit the immunogenicity of adenoviral vector-encoded transgenic antigens. This study suggest the need for modifications of adenoviral vector or the transgene used in immunization to dominate over the adenoviral vector-specific epitopes and induce more effective transgene specific immunity.
5.3 Heterologous Immunity Induced by Adenoviral Antigens
The other challenge of using adenoviral vectors in gene therapy is cross-reactive immune response induced by adenoviruses. For example, nAbs and T cells against HAd2 cross react with HAd35 and reduce the immunogenicity and efficacy of the HAd35 vectors (235, 236). Other studies showed that nAbs and T cells against HAd cross react with ChAd (ChAd6, ChAd7, ChAd24, ChAd32, and ChAd68) (35, 38, 219, 220). Adenoviruses can also induce robust cross-reactive immune responses against unrelated pathogen such as hepatitis C virus (HCV) antigens (209). Therefore, a careful evaluation of adenoviral-induced cross-reactive immune responses is needed before using adenoviruses in gene therapy and vaccine development.
6 Conclusions
Adenoviruses viruses have become the promise of new therapeutic strategy for cancer treatment. Adenoviral vectors can be engineered in different ways for cancer treatment including; 1. By modifying adenoviruses (Ads) to deliver transgenes that codes for tumor suppressor gene (p53) and other proteins whose expression result in cell cycle arrest; 2. By modifying Ads to express tumor specific antigens, cytokines, and other immune-modulatory molecules; 3. The other strategy is using oncolytic adenoviruses. However, the use of adenoviral vectors in cancer therapy is limited by several factors such as pre-existing immunity to adenoviral vectors; immunodominance of adenoviral antigens over the vaccine transgene antigen(s); and heterologous immunity with other pathogens. Thus, innovative strategies must be continually developed so as to overcome the obstacles of using adenoviral vectors in gene therapy.
Author Contributions
The author confirms being the sole contributor of this work and has approved it for publication.
Conflict of Interest
The authors declares that the research was conducted in the absence of any commercial or financial relationships that could be construed as a potential conflict of interest.
Publisher’s Note
All claims expressed in this article are solely those of the authors and do not necessarily represent those of their affiliated organizations, or those of the publisher, the editors and the reviewers. Any product that may be evaluated in this article, or claim that may be made by its manufacturer, is not guaranteed or endorsed by the publisher.
References
1. Wold WSM, Toth K. Adenovirus Vectors for Gene Therapy, Vaccination and Cancer Gene Therapy. Curr Gene Ther (2013) 13:421–33. doi: 10.2174/1566523213666131125095046
2. Sato-Dahlman M, Yamamoto M. The Development of Oncoltyic Adenovirus Therapy in the Past and Future—For the Case of Pancreatic Cancer. Curr Cancer Drug Targets (2018) 18:153–61. doi: 10.2174/1568009617666170222123925
3. Yang TC, Dayball K, Wan YH, Bramson J. Detailed Analysis of the CD8+ T-Cell Response Following Adenovirus Vaccination. J Virol (2003) 77:13407–11. doi: 10.1128/JVI.77.24.13407-13411.2003
4. Lee CS, Bishop ES, Zhang R, Yu X, Farina EM, Yan S, et al. Adenovirus-Mediated Gene Delivery: Potential Applications for Gene and Cell-Based Therapies in the New Era of Personalized Medicine. Genes Dis (2017) 4:43–63. doi: 10.1016/j.gendis.2017.04.001
5. Shenk T. Adenoviridae: The Viruses and Their Replication. In: Fields B, Knipe D, Howley P, editors. Virology, vol. Volume 2 . USA: Lipponcott-Raven: Philadelphia, PA (1996). p. 2111–48.
6. Thacker E, Timares L, Matthews Q. Strategies to Overcome Host Immunity to Adenovirus Vectors in Vaccine Development. Expert Rev Vaccines (2009) 8(6):761–77. doi: 10.1586/erv.09.29
7. Crystal RG. Adenovirus: The First Effective In Vivo Gene Delivery Vector. Hum Gene Ther (2014) 25:3–11. doi: 10.1089/hum.2013.2527
8. Gao J, Mese K, Bunz O, Ehrhardt A. State-Of-the-Art Human Adenovirus Vectorology for Therapeutic Approaches. FEBS Lett (2019) 593:3609–22. doi: 10.1002/1873-3468.13691
9. Robert-Guroff M. Replicating and non-Replicating Viral Vectors for Vaccine Development. Curr Opin Biotechnol (2007) 18:546–56. doi: 10.1016/j.copbio.2007.10.010
10. Breyer B, Jiang W, Cheng H, Zhou L, Paul R, Feng T, et al. Adenoviral Vector-Mediated Gene Transfer for Human Gene Therapy. Curr Gene Ther (2001) 2:149–62. doi: 10.2174/1566523013348689
11. Berk AJ. Adenoviridae. In: Knipe DM, Howley PM, editors. Fields Virology. Philadelphia, PA: Lippincott Williams & Wilkins (2013). p. 1704–31.
12. Loustalot F, Kremer EJ, Salinas S. The Intracellular Domain of the Coxsackievirus and Adenovirus Receptor Differentially Influences Adenovirus Entry. J Virol (2015) 89:9417–26. doi: 10.1128/JVI.01488-15
13. Baker AT, Greenshields-Watson A, Coughlan L, Davies JA, Uusi-Kerttula H, Cole DK, et al. Diversity Within the Adenovirus Fiber Knob Hypervariable Loops Influences Primary Receptor Interactions. Nat Commun (2019) 10:741. doi: 10.1038/s41467-019-08599-y
14. Wickham T, Carrion M, Kovesdi I. Targeting of Adenovirus Penton Base to New Receptors Through Replacement of its RGD Motif With Other Receptor-Specific Peptide Motifs. Gene Ther (1995) 2:750–6.
15. Crawford-Miksza L, Schnurr D. Analysis of 15 Adenovirus Hexon Proteins Reveals the Location and Structure of Seven Hypervariable Regions Containing Serotype-Specific Residues. J Virol (1996) 70(3):1836–44. doi: 10.1128/jvi.70.3.1836-1844.1996
16. CMM F, Rosa-Calatrava M, Conway J, Zubieta C, Cusack S, Ruigrok R, et al. A Quasi-Atomic Model of Human Adenovirus Type 5 Capsid. EMBO J (2005) 24:1645–54. doi: 10.1038/sj.emboj.7600653
17. Amberg N. Adenovirus Receptors: Implications for Targeting of Viral Vectors. Trends Pharmacol Sci (2012) 33:442–8. doi: 10.1016/j.tips.2012.04.005
18. Meier O, Gastaldelli M, Boucke K, Hemmi S, Greber UF. Early Steps of Clathrin-Mediated Endocytosis Involved in Phagosomal Escape of Fcγ Receptor-Targeted Adenovirus. J Virol (2005) 79:2604–13. doi: 10.1128/JVI.79.4.2604-2613.2005
19. Lyle C, McCormick F. Integrin αvβ5 is a Primary Receptor for Adenovirus in CAR- Negative Cells. Virol J (2010) 7:1–13. doi: 10.1186/1743-422X-7-148
20. Lenman A, Liaci MA, Liu Y, Årdahl C, Rajan A, Nilsson E, et al. Human Adenovirus 52 Uses Sialic Acid-Containing Glycoproteins and the Coxsackie and Adenovirus Receptor for Binding to Target Cells. PloS Pathog (2015) 11(2):e1004657. doi: 10.1371/journal.ppat.1004657
21. Gaggar A, Shayakhmetov DM, Lieber A. CD46 is a Cellular Receptor for Group B Adeno- Viruses. Nat Med (2003) 9:1408–12. doi: 10.1038/nm952
22. Amstutz B, Gastaldelli M, Kälin S, Imelli N, Boucke K, Wandeler E, et al. Subversion of CtBP1-Controlled Macropinocytosis by Human Adenovirus Serotype 3. EMBO J (2008) 27:956–69. doi: 10.1038/emboj.2008.38
23. McConnell M, Imperiale MJ. Biology of Adenovirus and its Use as a Vector for Gene Therapy. Hum Gene Ther (2004) 15:1022–33. doi: 10.1089/hum.2004.15.1022
24. Wold W, Horwitz M. Adenoviruses. In: Fields BN, Knipe DM, Howley PM, editors. Fields Virology, 5th ed. Philadelphia: Lippincott-Raven (2007). p. 2395–436.
25. Davison AJ, Benko M, Harrach B. Genetic Content and Evolution of Adenoviruses. J Gen Virol (2003) 84:2895–908. doi: 10.1099/vir.0.19497-0
26. Ginsberg HS. Identification and Classification of Adenoviruses. Virol (1962) 18:312–9. doi: 10.1016/0042-6822(62)90018-1
27. Wadell G. Adenoviridae: The Adenoviruses. In: Laboratory Diagnosis of Infectious Diseases Principles and Practice. New York, NY: Springer (1998).
28. Benkö M. Virus-Host H. Molecular Evolution of Adenoviruses. Curr Topics Micro- Biol Immunol (2003) 272:3–35. doi: 10.1007/978-3-662-05597-7_1
29. Yu B, Wang Z, Dong J, Wang C, Gu L, Sun C, et al. A Serological Survey of Human Adenovirus Serotype 2 and 5 Circulating Pediatric Populations in Changchun, China. Virol J (2012) 9:287. doi: 10.1186/1743-422X-9-287
30. Khanal S, Ghimire P, Dhamoon A. The Repertoire of Adenovirus in Human Disease: The Innocuous to the Deadly. Biomed (2018) 6:30. doi: 10.3390/biomedicines6010030
31. Ephros M, Friedman B, Elhasid R, Kra-Oz Z, Shaked-Mishan P, Sattinger J, et al. Incidence and Clinical Manifestations of Adenoviral Infection Among Children Undergoing Allogeneic Stem Cell Transplantation. IMAJ (2009) 11:744–8. doi: 10.1016/j.bbmt.2018.12.753
32. Sumida SM, Truittm D, Kishko M, Arthur J, Jackson S, Gorgone D, et al. Neutralizing Anti- Bodies and CD8+ T Lymphocytes Both Contribute to Immunity to Adenovirus Serotype 5 Vaccine Vectors. J Virol (2004) 78:2666–73. doi: 10.1128/JVI.78.6.2666-2673.2004
33. Sumida SM, Truitt DM, Lemckert AAC, Vogels R, Custers JHHV, Addo MM, et al. Neutralizing Antibodies to Adenovirus Serotype 5 Vaccine Vectors Are Directed Primarily Against the Adenovirus Hexon Protein. J Immunol (2005) 174:7179–85. doi: 10.4049/jimmunol.174.11.7179
34. Zhang S, Huang W, Zhou X, Zhao Q, Wang Q, Jia B. Seroprevalence of Neutralizing Anti- Bodies to Human Adenoviruses Type-5 and Type-26 and Chimpanzee Adenovirus Type- 68 in Healthy Chinese Adults. J Med Virol (2013) 85:1077–84. doi: 10.1002/jmv.23546
35. Fausther-Bovendo H, Kobinger G. Pre-Existing Immunity Against Ad Vectors: Humoral, Cellular, and Innate Response, What’s Important? Hum Vaccines Immunotherapeutics (2014) 10:2875–84. doi: 10.4161/hv.29594
36. Colloca S, Barnes E, Folgori A, Ammendola V, Capone S, Cirillo A, et al. Vaccine Vectors Derived From a Large Collection of Simian Adenoviruses Induce Potent Cellular Immunity Across Multiple Species. Sci Trans Med (2012) 4:115ra2–2. doi: 10.1126/scitranslmed.3002925
37. Ewer K, Sebastian S, Spencer A, Gilbert S, Hill A, Lambe T. Chimpanzee Adenoviral Vectors as Vaccines for Outbreak Pathogens. Hum Vaccines Immunotherapeutics (2017) 13:3020–32. doi: 10.1080/21645515.2017.1383575
38. Iampietro M, Larocca R, Provine N, Abbink P, Kang Z, Bricault C, et al. Immunogenicity and Cross-Reactivity of Rhesus Adenoviral Vectors. J Virol (2018) 92:e00159–18. doi: 10.1128/JVI.00159-18
39. Doremalen N, Lambe T, Spencer A, Belij-Rammerstorfer S, Purushotham J, Port J, et al. ChAdOx1 Ncov-19 Vaccination Prevents SARS-CoV-2 Pneumonia in Rhesus Macaques. bioRxiv preprint (2020). doi: 10.1101/2020.05.13.093195
40. Pellom ST, Rumfield CS, Morillon YM, Roller N, Poppe LK, Brough DE. Characterization of Recombinant Gorilla Adenovirus HPV Therapeutic Vaccine PRGN-2009. JCI Insight (2021) 8:1–13:6(7). doi: 10.1172/jci.insight.141912
41. Bett AJ, Haddara W, Prevec L, Graham FL. An Efficient and Flexible System for Construction of Adenovirus Vectors With Insertions or Deletions in Early Regions 1 and 3. Proc Natl Acad Sci USA (1994) 91:8802–6. doi: 10.1073/pnas.91.19.8802
42. Wold W, Doronin K, Toth K, Kuppuswamy M, Lichtenstein DL, Tollefson AE. Immune Responses to Adenoviruses: Viral Evasion Mechanisms and Their Implications for the Clinic. Curr Opin Immunol (1999) 11(4):380–6. doi: 10.1016/S0952-7915(99)80064-8
43. Lusky M, Christ M, Rittner K, Dieterle A, Dreyer D, Mourot B, et al. In Vitro and In Vivo Biology of Recombinant Adenovirus Vectors With E1, E1/E2A, or E1/E4 Deleted. J Virol (1998) 72:2022–32. doi: 10.1128/JVI.72.3.2022-2032.1998
44. Schaack J. Adenovirus Vectors Deleted for Genes Essential for Viral DNA Replication. Front Biosci: A J Virtual Library (2005) 1(10):1146–55. doi: 10.2741/1607
45. Wang Q, Finer M. Second-Generation Adenovirus Vectors. Nat Med (1996) 2:714–6. doi: 10.1038/nm0696-714
46. Kochanek S, Clemens P, Mitani K, Chen H, Chan S, Caskey C. A New Adenoviral Vector: Replacement of All Viral Coding Sequences With 28 Kb of DNA Independently Expressing Both Full-Length Dystrophin and Beta-Galactosidase. Proc Natl Acad Sci (1996) 93(12):5731–6. doi: 10.1073/pnas.93.12.5731
47. Puntel M, Muhammad A, Candolfi M, Salem A, Yagiz K, Farrokhi C, et al. A Novel Bicistronic High-Capacity Gutless Adenovirus Vector That Drives Constitutive Expression of Herpes Simplex Virus Type 1 Thymidine Kinase and Tet-Inducible Expression of Flt3L for Glioma Therapeutics. J Virol (2010) 84(12):6007–17. doi: 10.1128/JVI.00398-10
48. Ishizaki M, Kawano R, Uchida Y, Kimura E, Uchino M, Maeda Y. Gene Therapy for Duchenne Muscular Dystrophy by the Helper-Dependent Adenovirus Vector (HDAdv)—Mediated Full-Length Dystrophin Expression. Mol Ther (2018) 15(Supplement 1):S52. doi: 10.2174/156652312800840603
49. Parks R, Chen L, Anton M, Sankar U, Rudnicki M. A Helper-Dependent Adenovirus Vector System: Removal of Helper Virus by Cre-Mediated Excision of the Viral Packaging Signal. PNAS (1996) 93(24):13565–70. doi: 10.1073/pnas.93.24.13565
50. Fisher KJ, Choi H, Burda J, Chen S-J, Wilson J. Recombinant Adenovirus Deleted of All Viral Genes for Gene Therapy of Cystic Fibrosis. Virol (1996) 217(1):11–22. doi: 10.1006/viro.1996.0088
51. Hardy S, Kitamura M, Harris-Stansil T, Dai Y, Phipps M. Construction of Adenovirus Vectors Through Cre-Lox Recombination. J Virol (1997) 71(3):1842–9. doi: 10.1128/jvi.71.3.1842-1849.1997
52. Schiedner G, Morral N, Parks RJ, Wu Y, Koopmans SC, Langston C, et al. Genomic DNA Transfer With a High-Capacity Adenovirus Vector Results in Improved In Vivo Gene Expression and Decreased Toxicity. Nat Genet (1998) 18(2):180–3. doi: 10.1038/ng0298-180
53. Morral N, Parks R, Zhou H, Langston C, Schiedner G, Quinones J, et al. High Doses of a Helper-Dependent Adenoviral Vector Yield Supraphysiological Levels of Alpha1- Antitrypsin With Negligible Toxicity. Hum Gene Ther (1999) 81:2709–16. doi: 10.1089/hum.1998.9.18-2709
54. Palmer D, Ng P. Improved System for Helper-Dependent Adenoviral Vector Production. Molecular Therapy. J Am Soc Gene Ther (2003) 8(5):846–52. doi: 10.1016/j.ymthe.2003.08.014
55. Ferris RL. Immunology and Immunotherapy of Head and Neck Cancer. J Clin Oncol (2015) 33:3293–304. doi: 10.1200/JCO.2015.61.1509
56. Ferris RL, Blumenschein G Jr., Fayette J, Guigay J, Colevas AD, Licitra L, et al. Nivolumab for Recurrent Squamous-Cell Carcinoma of the Head and Neck. N Engl J Med (2016) 375:1856–67. doi: 10.1056/NEJMoa1602252
57. Cavalieri S, Rivoltini L, Bergamini C, Locati LD, Licitra L, Bossi P. Immuno-Oncology in Head and Neck Squamous Cell Cancers: News From Clinical Trials, Emerging Predictive Factors and Unmet Needs. Cancer Treat Rev (2018) 65:78–86. doi: 10.1016/j.ctrv.2018.03.003
58. Galon J, Bruni D. Approaches to Treat Immune Hot, Altered and Cold Tumours With Combination Immunotherapies. Nat Rev Drug Discovery (2019) 18:197–218. doi: 10.1038/s41573-018-0007-y
59. Hegde PS, Karanikas V, Evers S. The Where, the When, and the How of Immune Monitoring for Cancer Immunotherapies in the Era of Check-Point Inhibition. Clin Cancer Res (2016) 22:1865–74. doi: 10.1158/1078-0432.CCR-15-1507
60. Hugo W, Zaretsky JM, Sun L, Song C, Moreno BH, Hu-Lieskovan S, et al. Genomic and Transcriptomic Features of Response to Anti-PD-1 Therapy in Metastatic Melanoma. Cell (2016) 165:35–44. doi: 10.1016/j.cell.2016.02.065
61. Mariathasan S, Turley SJ, Nickles D, Castiglioni A, Yuen K, Wang Y, et al. TGFb Atten-Uates Tumour Response to PD-L1 Blockade by Contributing to Exclusion of T Cells. Nature (2018) 554:544–8. doi: 10.1038/nature25501
62. Herbst RS, Soria JC, Kowanetz M, Fine GD, Hamid O, Gordon MS, et al. Predictive Correlates of Response to the Anti-PD-L1 Antibody MPDL3280A in Cancer Patients. Nature (2014) 515:563–7. doi: 10.1038/nature14011
63. Ayers M, Lunceford J, Nebozhyn M, Murphy E, Loboda A, Kaufman DR, et al. IFN-G-Related mRNA Profile Predicts Clinical Response to PD-1 Blockade. J Clin Invest (2017) 127:2930–40. doi: 10.1172/JCI91190
64. Pratt G, Goodyear O, Moss P. Immunodeficiency and Immunotherapy in Multiple Myeloma. Br J Haematol (2007) 138:563–79. doi: 10.1111/j.1365-2141.2007.06705.x
65. Romano A, Conticello C, Cavalli M, Vetro C, La Fauci A, Parrinello NL, et al. Immunological Dysregulation in Multiple Myeloma Microenvironment. BioMed Res Int (2014) 2014:198539. doi: 10.1155/2014/198539
66. Wang D, Huang XF, Hong B, Song X-T, Hu L, Jiang M, et al. Efficacy of Intracellular Immune Checkpoint-Silenced DC Vaccine. JCI Insight (2018) 3:e98368. doi: 10.1172/jci.insight.98368
67. Nguyen N, Bellile E, Thomas D, McHugh J, Rozek L, Virani S, et al. Tumor Infiltrating Lymphocytes and Survival in Patients With Head and Neck Squamous Cell Carcinoma. Head Neck (2016) 38:1074–84. doi: 10.1002/hed.24406
68. Hoesli R, Birkeland AC, Rosko AJ, Issa M, Chow KL, Michmerhuizen NL, et al. Proportion of CD4 and CD8 Tumor Infiltrating Lymphocytes Predicts Survival in Persistent/Recurrent Laryngeal Squamous Cell Carcinoma. Oral Oncol (2018) 77:83–9. doi: 10.1016/j.oraloncology.2017.12.003
69. Spector ME, Bellile E, Amlani L, Zarins K, Smith J, Brenner JC, et al. Prognostic Value of Tumor-Infiltrating Lymphocytes in Head and Neck Squamous Cell Carcinoma. JAMA Otolaryngol Head Neck Surg (2019) 45(11):1012–19. doi: 10.1001/jamaoto.2019.2427
70. Quan H, Shan Z, Liu Z, Liu S, Yang L, Fang X, et al. The Repertoire of Tumor-Infiltrating Lymphocytes Within the Microenvironment of Oral Squamous Cell Carcinoma Reveals Immune Dysfunction. Cancer Immunol Immunother (2020) 69:465–76. doi: 10.1007/s00262-020-02479-x
71. Bisheshar SK, De Ruiter EJ, Devriese LA, Willems SM. The Prognostic Role of NK Cells and Their Ligands in Squamous Cell Carcinoma of the Head and Neck: A Systematic Review and Meta-Analysis. Oncoimmunol (2020) 9:1747345. doi: 10.1080/2162402X.2020.1747345
72. Seth P. Vector-Mediated Cancer Gene Therapy: An Overview. Cancer Biol Ther (2005) 4:512–7. doi: 10.4161/cbt.4.5.1705
73. Curiel D, Yamamoto M. Cancer Gene Therapy. Technol Cancer Res Treat (2005) 4:315–30. doi: 10.1177/153303460500400402
74. Zhou J, Huang Z, Wang Z, Liu S, Grandien A, Ernberg I, et al. Tumor Suppressor BLU Promotes TRAIL-Induced Apoptosis by Downregulating NF-κb Signaling in Nasopharyngeal Carcinoma. Oncotarget (2014) 8:43853–65. doi: 10.18632/oncotarget.14126
75. Shaw A, Suzuki M. Recent Advances in Oncolytic Adenovirus Therapies for Cancer. Curr Opin Virol (2016) 21:9–15. doi: 10.1016/j.coviro.2016.06.009
76. Saito H, Kitagawa K, Yoneda T, Fukui Y, Fujsawa M, Bautista D, et al. Combination of P53-DC Vaccine and rAd-P53 Gene Therapy Induced CTLs Cytotoxic Against P53-Deleted Human Prostate Cancer Cells In Vitro. Cancer Gene Ther (2017) 24:289–96. doi: 10.1038/cgt.2017.21
77. Brown CJ, Lain S, Verma CS, Fersht AR, Lane DP. Awakening Guardian Angels: Drugging the P53 Pathway. Nat Rev Cancer (2009) 9(12):862–73. doi: 10.1038/nrc2763
78. Quinlan DC, Davidson AG, Summers CL, Warden HE, Doshi HM. Accumulation of P53 Protein Correlates With a Poor Prognosis in Human Lung Cancer. Cancer Res (1992) 52:4828–31.
79. Isobe T, Hiyama K, Yoshida Y, Fujiwara Y, Yamakido M. Prognostic Significance of P53 and Ras Gene Abnormalities in Lung Adenocarcinoma Patients With Stage I Disease After Curative Resection. Jpn J Cancer Res (1994) 85:1240–6. doi: 10.1111/j.1349-7006.1994.tb02936.x
80. Chen CB, Pan JJ, Xu LY. Recombinant Adenovirus P53 Agent Injection Combined With Radiotherapy in Treatment of Nasopharyngeal Carcinoma: A Phase II Clinical Trial. Zhonghua Yi Xue Za Zhi (2003) 83(23):2033–5.
81. Zhang SW, Xiao SW, Liu CQ, Sun Y, Su X, Li D, et al. Treatment of Head and Neck Squamous Cell Carcinoma by Recombinant Adenovirus-P53 Combined With Radiotherapy: A Phase II Clinical Trial of 42 Cases. Zhonghua Yi Xue Za Zhi (2003) 83(23):2023–8.
82. Zhang SW, Xiao SW, Liu CQ, Sun Y, Su X, Li D, et al. Recombinant Adenovirus-P53 Gene Therapy Combined With Radiotherapy for Head and Neck Squamous-Cell Carcinoma. Zhonghua Zhong Liu Za Zhi (2005) 27(7):426–8.
83. Li L, Huang Y, Wang L, Li Y, Wen Y, Han B. A Combination Therapy of Selective Intraarterial Gendicine Infusion With Chemotherapy for Locally Advanced Head and Neck Carcinoma. Mol Ther (2006) 13:S20. doi: 10.1016/j.ymthe.2006.08.061
84. Ma WS, Ma JG, Xing LN. Efficacy and Safety of Recombinant Human Adenovirus P53 Combined With Chemoradiotherapy in the Treatment of Recurrent Nasopharyngeal Carcinoma. Anticancer Drugs (2012) 28(2):230–6. doi: 10.1097/CAD.0000000000000448
85. Si YF, He CC, Lan GP. Recombinant Adenovirus P53 Agent Injection Combined With Radiotherapy and Chemotherapy for Intermediate and Advanced Stage Nasopharyngeal Carcinoma. Chin J Clin Oncol (2009) 36:1031–9. doi: 10.1186/s40064-016-3574-6
86. Wang X, Wang J, Zhang J. Clinical Observations of Recombinant Adenovirus-P53 Combined With Radiotherapy on Nasopharyngeal Squamous Carcinoma. Mod J Int Tradit Chin West Med (2012) 21:924–34. doi: 10.1186/s40064-016-3574-6
87. Liu S, Chen P, Hu M, Tao Y, Chen L, Liu H, et al. Randomized, Controlled Phase II Study of Post-Surgery Radiotherapy Combined With Recombinant Adenoviral Human P53 Gene Therapy in Treatment of Oral Cancer. Cancer Gene Ther (2013) 20(6):375–8. doi: 10.1038/cgt.2013.30
88. Su X, Chen WJ, Xiao SW, Li X, Xu G, Pan J, et al. Effect and Safety of Recombinant Adenovirus-P53 Transfer Combined With Radiotherapy on Long-Term Survival of Locally Advanced Cervical Cancer. Hum Gene Ther (2016) 27(12):1008–14. doi: 10.1089/hum.2016.043
89. Xia Y, Du Z, Wang X, Li X. Treatment of Uterine Sarcoma With rAd-P53 (Gendicine) Followed by Chemotherapy: Clinical Study of TP53 Gene Therapy. Hum Gene Ther (2018) 29(2):242–50. doi: 10.1089/hum.2017.206
90. Chen G, Zhang S, He X, Liu S, Ma C, Zou X-P. Clinical Utility of Recombinant Ade- Noviral Human P53 Gene Therapy: Current Perspectives. Onco Targets Ther (2014) 7:1901–9. doi: 10.2147/OTT.S50483
91. Zhang W, Li L, Li D, Liu J, Li X, Li W, et al. The First Approved Gene Therapy Product for Cancer Ad-P53 (Gendicine): 12 Years in the Clinic. Hum Gene Ther (2018) 29(2):160–79. doi: 10.1089/hum.2017.218
92. Gabrilovich DI. INGN 201 (Advexin): Adenoviral P53 Gene Therapy for Cancer. Expert Opin Biol Ther (2006) 6:823–32. doi: 10.1517/14712598.6.8.823
93. Räty J, Pikkarainen J, Wirth T, Ylä-Herttuala S. Gene Therapy: The First Approved Gene-Based Medicines, Molecular Mechanisms, and Clinical Indications. Curr Mol Pharmacol (2008) 1:13–23. doi: 10.2174/1874467210801010013
94. Senzer N, Nemunaitis J. A Review of Contusugene Ladenovec (Advexin) P53 Therapy. Curr Opin Mol Ther (2009) 11:54–61.
95. Yoo GH, Moon J, LeBlanc M, Lonardo F, Urba S, Kim H, et al. A Phase 2 Trial of Surgery With Perioperative INGN 201 (Ad5CMV-P53) Gene Therapy Followed by Chemoradiotherapy for Advanced, Resectable Squamous Cell Carcinoma of the Oral Cavity, Oropharynx, Hypopharynx, and Larynx: Report of the Southwest Oncology Group. Arch Otolaryngol Neck Surg (2009) 135:869. doi: 10.1001/archoto.2009.122
96. Galluzzi L, Vacchelli E, Bravo-San Pedro JM. Classification of Current Anticancer Immunotherapies. Oncotarget (2014) 5:12472–508. doi: 10.18632/oncotarget.2998
97. Dong F, Wang L, John J, Davis JJ, Hu W, Zhang L, et al. Eliminating Established Tumor in Nu/Nu Nude Mice by a Tumor Necrosis Factor-A-Related Apoptosis-Inducing Ligand Armed Oncolytic Adenovirus. Clin Cancer Res (2006) 12:5224–30. doi: 10.1158/1078-0432.CCR-06-0244
98. Yu W, Fang H. Clinical Trials With Oncolytic Adenovirus in China. Curr Cancer Drug Targets (2007) 7:141–8. doi: 10.2174/156800907780058817
99. Vaillancourt M, Atencio I, Quijano E, Atencio I, Quijano E, Howe J, et al. Inefficient Killing of Quiescent Human Epithelial Cells by Replicating Adenoviruses: Potential Implications for Their Use as Oncolytic Agents. Cancer Gene Ther (2005) 12:691–8. doi: 10.1038/sj.cgt.7700840
100. Ferrari R, Pellegrini M, Horwitz GA, Xie W, Berk A, Kurdistan S. Epigenetic Reprogramming by Adenovirus E1a. Sci (2008) 321:1086–8. doi: 10.1126/science.1155546
101. Coffey MC, Strong JE, Forsyth PA, Lee PW. Reovirus Therapy of Tumors With Activated Ras Pathway. Sci (1998) 282:1332–4. doi: 10.1126/science.282.5392.1332
102. Aghi M, Visted T, Depinho RA, Chiocca EA. Oncolytic Herpes Virus With Defective ICP6 Specifically Replicates in Quiescent Cells With Homozygous Genetic Mutations in P16. Oncogene (2008) 27:4249–54. doi: 10.1038/onc.2008.53
103. Bartlett DL, Liu Z, Sathaiah M, Ravindranathan R, Guo Z, He Y, et al. Oncolytic Viruses as Therapeutic Cancer Vaccines. Mol Cancer (2013) 12:103. doi: 10.1186/1476-4598-12-103
104. Andtbacka RH, Kaufman HL, Collichio F, Amatruda T, Senzer N, Chesney J, et al. Talimogene Laherparepvec Improves Durable Response Rate in Patients With Advanced Melanoma. J Clin Oncol (2015) 33:2780–8. doi: 10.1200/JCO.2014.58.3377
105. Marelli G, Howells A, Lemoine NR, Wang Y. Oncolytic Viral Ther-Apy and the Immune System: A Double-Edged Sword Against Cancer. Front Immunol (2018) 9:866. doi: 10.3389/fimmu.2018.00866
106. Bischoff J, Kirn D, Williams A, Heise C, Horn S, Muna M, et al. An Adenovirus Mutant That Replicates Selectively in P53-Deficient Human Tumor Cells. Science (1996) 274:373–6. doi: 10.1126/science.274.5286.373
107. Heise C, Williams A, Xue S, Propst M, Kirn D. Intravenous Administration of ONYX- 015, a Selectively Replicating Adenovirus, Induces Antitumoral Efficacy. Cancer Res (1999) 59:2623–8.
108. Khuri FR, Nemunaitis J, Ganly I, Arseneau J, Tannock IF, Romel L, et al. A Controlled Trial of Intratumoral ONYX-015, a Selectively-Replicating Adenovirus, in Combination With Cisplatin and 5-Fluorouracil in Patients With Recurrent Head and Neck Cancer. Nat Med (2000) 6:nm0800_879.7. doi: 10.1038/78638
109. Guo W, Song H. Development of Gene Therapeutics for Head and Neck Cancer in China: From Bench to Bedside. Hum Gene Ther (2018) 29:180–7. doi: 10.1089/hum.2017.230
110. Cerullo V, Vähä-Koskela M, Hemminki A. Oncolytic Adenoviruses. Onco Immunol (2012) 1:979–81. doi: 10.4161/onci.20172
111. Yamamoto Y, Nagasato M, Yoshida T, Aoki K. Recent Advances in Genetic Modification of Adenovirus Vectors for Cancer Treatment. Cancer Sci (2017) 108:831–7. doi: 10.1111/cas.13228
112. Cerullo V, Capasso C, Vähä-Koskela M, Hemminki O, Hemminki A. Cancer-Targeted Oncolytic Adenoviruses for Modulation of the Immune System. Curr Cancer Drug Targets (2018) 8:124–38. doi: 10.2174/1568009617666170502152352
113. Lehtonen A, Matikainen S, Julkunen I. Interferons Up-Regulate STAT1, STAT2, and IRF Family Transcription Factor Gene Expression in Human Peripheral Blood Mononuclear Cells and Macrophages. J Immunol (1997) 159(2):794–803.
114. Nukui Y, Picozzi VJ, Traverso LW. Interferon-Based Adjuvant Chemoradiation Therapy Improves Survival After Pancreaticoduodenectomy for Pancreatic Adenocarcinoma. Am J Surg (2000) 179:367–71. doi: 10.1016/S0002-9610(00)00369-X
115. Picozzi VJ, Kozarek RA, Traverso LW. Interferon-Based Adjuvant Chemoradiation Therapy After Pancreaticoduodenectomy for Pancreatic Adenocarcinoma. Am J Surg (2003) 185:476–80. doi: 10.1016/S0002-9610(03)00051-5
116. Jensen EH, Armstrong L, Lee C, Tuttle TM, Vickers SM, Sielaff T, et al. Neoadjuvant Interferon-Based Chemoradiation for Borderline Resectable and Locally Advanced Pancreas Cancer: A Phase II Pilot Study. HPB (2014) 16:131–9. doi: 10.1111/hpb.12086
117. Tagliaferri P, Caraglia M, Budillon A, Marra M, Vitale G, Viscomi C. New Pharmacokinetic and Pharmacodynamic Tools for Interferon-Alpha (IFN-α) Treatment of Human Cancer. Cancer Immunol Immunother (2005) 54:1–10. doi: 10.1007/s00262-004-0549-1
118. Glue P, Fang JWS, Rouzier-Panis R, Raffanel C, Sabo R, Gupta SK. Pegylated Interferon-α2b: Pharmacokinetics, Pharmacodynamics, Safety, and Preliminary Efficacy Data. Clin Pharmacol Ther (2000) 68:556–67. doi: 10.1067/mcp.2000.110973
119. Armstrong L, Arrington A, Han J, Gavrikova T, Brown E, Yamamoto M, et al. Generation of a Novel, Cyclooxygenase-2-Targeted, Interferon-Expressing, Conditionally Replicative Adenovirus for Pancreatic Cancer Therapy. Am J Surg (2012a) 204:741–50. doi: 10.1016/j.amjsurg.2012.02.016
120. Armstrong L, Davydova J, Brown E, Han J, Yamamoto M, Vickers SM. Delivery of Interferon Alpha Using a Novel Cox2-Controlled Adenovirus for Pancreatic Cancer Therapy. Surg (2012b) 152:114–22. doi: 10.1016/j.surg.2012.02.017
121. Tollefson AE, Ryerse JS, Scaria A, Hermiston TW, Wold WS. The E3-11.6-kDa Adenovirus Death Protein (ADP) is Required for Efficient Cell Death:Characterization of Cells Infected With Adp Mutants. Virol (1996) 220:152–62. doi: 10.1006/viro.1996.0295
122. LaRocca CJ, Han J, Gavrikova T, Armstrong L, Oliveira AR, Shanley R, et al. Oncolytic Adenovirus Expressing Interferon Alpha in a Syngeneic Syrian Hamster Model for the Treatment of Pancreatic Cancer. Surg (2015) 157:888–98. doi: 10.1016/j.surg.2015.01.006
123. Salzwedel AO, LaRocca CJ, Han J, Shanley R, Yamamoto M, Davydova J. Combination of Interferon-Expressing Oncolytic Adenovirus With Chemotherapy and Radiation is Highly Synergistic in Hamster Model of Pancreatic Cancer. Oncotarget (2018) 9:18041–52. doi: 10.18632/oncotarget.24710
124. Tao Z, Connor R, Ashoori F, Dinney C, Munsell M, JA Philopena JA. Efficacy of a Single Intravesical Treatment With Ad-IFN/Syn 3 is Dependent on Dose and Urine IFN Concentration Obtained: Implications for Clinical Investigation. Cancer Gene Ther (2006) 13:125–30. doi: 10.1038/sj.cgt.7700865
125. Lee S, Margolin K. Cytokines in Cancer Immunotherapy. Cancers (Basel) (2011) 3:3856–93. doi: 10.3390/cancers3043856
126. Hamid O, Ismail R, Puzanov I. Intratumoral Immunotherapy—Update 2019. Oncologist (2020) 25(3):e423–38. doi: 10.1634/theoncologist.2019-0438
127. Ranki T, Pesonen S, Hemminki A, Partanen K, Kairemo K, Alanko T, et al. Phase I Study With ONCOS-102 for the Treatment of Solid Tumors - an Evaluation of Clinical Response and Exploratory Analyses of Immune Markers. J Immunother Cancer (2016) 4:17. doi: 10.1186/s40425-016-0121-5
128. Eriksson E, Milenova I, Wenthe J, Ståhle M, Leja-Jarblad J, Ullenhag G, et al. Shaping the Tumor Stroma and Sparking Immune Activation by CD40 and 4-1BB Signaling Induced by an Armed Oncolytic Virus. Clin Cancer Res (2017) 23:5846–57. doi: 10.1158/1078-0432.CCR-17-0285
129. Tähtinen S, Kaikkonen S, Merisalo-Soikkeli M, Grönberg-Vänberg-Vähä-Koskela S, Kanerva A, Parviainen S, et al. Favorable Alteration of Tumor Microenvironment by Immunomodulatory Cytokines for Efficient T-Cell Therapy in Solid Tumors. PloS One (2015) 10:e0131242. doi: 10.1371/journal.pone.0131242
130. Siurala M, Havunen R, Saha D, Lumen D, Airaksinen AJ, Tähtinen S, et al. Adenoviral Delivery of Tumor Necrosis Factor-α and Interleukin-2 Enables Successful Adoptive Cell Therapy of Immunosuppressive Melanoma. Mol Ther (2016) 24:1435–43. doi: 10.1038/mt.2016.137
131. Colombo MP, Trinchieri G. Interleukin-12 in Anti-Tumor Immunity and Immunotherapy. Cytokine Growth Factor Rev (2002) 13:155–68. doi: 10.1016/S1359-6101(01)00032-6
132. Del Vecchio M, Bajetta E, Canova S, Lotze MT, Wesa A, Parmiani G. Interleukin-12: Biological Properties and Clinical Application. Clin Cancer Res (2007) 13:4677–85. doi: 10.1158/1078-0432.CCR-07-0776
133. Brunda MJ, Luistro L, Warrier RR, Wright RB, Hubbard BR, Murphy M, et al. Antitumor and Antimetastatic Activity of Interleukin 12 Against Murine Tumors. J Exp Med (1993) 178:1223–30. doi: 10.1084/jem.178.4.1223
134. Teicher BA, Ara G, Buxton D, Leonard J, Schaub RG. Optimal Scheduling of Interleukin 12 and Chemotherapy in the Murine MB-49 Bladder Carcinoma and B16 Melanoma. Clin Cancer Res (1997) 3:1661–7.
135. Kozar K, Kaminski R, Switaj T, Ołdak T, Machaj E, Wysocki PJ, et al. Interleukin 12-Based Immunotherapy Improves the Antitumor Effectiveness of a Low-Dose 5-Aza-2′-Deoxycitidine Treatment in L1210 Leukemia and B16F10 Melanoma Models in Mice. Clin Cancer Res (2003) 9:3124–33.
136. Cao L, Zeng Q, Xu C, Shi S, Zhang Z, Sun X. Enhanced Antitumor Response Mediated by the Codelivery of Paclitaxel and Adenoviral Vector Expressing IL-12. Mol Pharm (2013) 10:1804–14. doi: 10.1021/mp300602j
137. Nguyen KG, Vrabel MR, Mantooth SM, Hopkins JJ, Wagner ES, Gabaldon TA, et al. Localized Interleukin-12 for Cancer Immunotherapy. Front Immunol (2020) 11:575–97. doi: 10.3389/fimmu.2020.575597
138. Lasek W, Zagozdzon R, Jakobisiak M. Interleukin 12: Still a Promising Candidate for Tumor Immunotherapy? Cancer Immunol Immunother (2014) 63:419–35. doi: 10.1007/s00262-014-1523-1
139. Sangro B, Mazzolini G, Ruiz J, Herraiz M, Quiroga J, Herrero I, et al. Phase I Trial of Intratumoral Injectio of an Adenovirus Encoding Interleukin-12 for Advanced Digestive Tumors. J Clin Oncol (2004) 22:1389–97. doi: 10.1200/JCO.2004.04.059
140. Bortolanza S, Bunuales M, Otano I, Gonzalez-Aseguinolaza G, Ortiz-de-Solorzano C, Perez D, et al. Treatment of Pancreatic Cancer With an Oncolytic Adenovirus Expressing Interleukin-12 in Syrian Hamsters. Mol Ther (2009) 17:614–22. doi: 10.1038/mt.2009.9
141. Wang P, Li X, Wang J, Gao D, Li Y, Li H, et al. Re-Designing Interleukin-12 to Enhance its Safety and Potential as an Anti-Tumor Immunotherapeutic Agent. Nat Commun (2017) 8:1395. doi: 10.1038/s41467-017-01385-8
142. Chiocca EA, Yu JS, Lukas RV, Solomon IH, Ligon KL, Nakashima H, et al. Regulatable Interleukin-12 Gene Therapy in Patients With Recurrent High-Grade Glioma: Results of a Phase 1 Trial. Sci Transl Med (2019) 11:5680. doi: 10.1126/scitranslmed.aaw5680
143. Santos JM, Cervera-Carrascon V, Havunen R, Zafar S, Siurala M, Sorsa S, et al. Adenovirus Coding for Interleukin-2 and Tumor Necrosis Factor Alpha Replaces Lymphodepleting Chemotherapy in Adoptive T Cell Therapy. Mol Ther (2018) 26:2243–54. doi: 10.1016/j.ymthe.2018.06.001
144. Liu L, Ma J, Qin L, Shi X, Si H, Wei Y. Interleukin-24 Enhancing Antitumor Activity of Chimeric Oncolytic Adenovirus for Treating Acute Promyelocytic Leukemia Cell. Med (Baltimore) (2019) 98:e15875. doi: 10.1097/MD.0000000000015875
145. Zhang KL, Li RP, Zhang BP, Gao ST, Li B, Huang CJ, et al. Efficacy of a New Oncolytic Adenovirus Armed With IL-13 Against Oral Carcinoma Models. Onco Targets Ther (2019) 12:6515–23. doi: 10.2147/OTT.S203638
146. Lapteva N, Aldrich M, Weksberg D, Rollins L, Goltsova T, Chen SY. Targeting the Intratumoral Dendritic Cells by the Oncolytic Adenoviral Vaccine Expressing RANTES Elicits Potent Antitumor Immunity. J Immunother (2009) 32:145–56. doi: 10.1097/CJI.0b013e318193d31e
147. Huehls A, Coupet T, Sentman C. Bispecific T Cell Engager for Cancer Immunotherapy. Immunol Cell Biol (2014) 93:290–6. doi: 10.1038/icb.2014.93
148. Brischwein K, Schlereth B, Guller B, Steiger C, Wolf A, Lutterbuese R, et al. MT110: A Novel Bispecfic Single Chain Antibody Construct With High Efficacy in Eradicating Established Tumors. Mol Immunol (2006) 43:1129–43. doi: 10.1016/j.molimm.2005.07.034
149. Bargou R, Leo E, Zugmaier G, Klinger M, Goebeler M, Knop S, et al. Tumor Regression in Cancer Patients by Very Low Doses of a T-Cell Engaging Antibody. Sci (2008) 321:974–7. doi: 10.1126/science.1158545
150. Pomés J, Fajardo CA, Sal MF, Arias-Badia M, Arias-Badia LA, Alemany R. Arming Oncolytic Adenovirus With FAP-Targeting Bispecific T-Cell Engager to Improve Antitumor Efficacy. Ann Oncol (2017) 28: supplement 11:XI18. doi: 10.1093/annonc/mdx711.042
151. Fajardo CA, Guedan S, Rojas LA, Moreno R, Arias-Badia M, de Sostoa J, et al. Oncolytic Adenoviral Delivery of an Egfr-Targeting T-Cell Engager Improves Antitumor Efficacy. Cancer Res (2017) 77:2052–63. doi: 10.1158/0008-5472.CAN-16-1708
152. Wing A, Fajardo CA, Posey AD, Shaw C, Da T, Young RM, et al. Improving CART-Cell Therapy of Solid Tumors With Oncolytic Virus-Driven Production of a Bispecific T-Cell Engager. Cancer Immunol Res (2018) 6:605–16. doi: 10.1158/2326-6066.CIR-17-0314
153. Freedman JD, Hagel J, Scott EM, Psallidas I, Gupta A, Spiers L, et al. Oncolytic Adenovirus Expressing Bispecific Antibody Targets T-Cell Cytotoxicity in Cancer Biopsies. EMBO Mol Med (2017) 9:1067–87. doi: 10.15252/emmm.201707567
154. Freedman JD, Duffy MR, Lei-Rossmann J, Muntzer A, Scott EM, Hagel J, et al. An Oncolytic Virus Expressing a T-Cell Engager Simultaneously Targets Cancer and Immunosuppressive Stromal Cells. Cancer Res (2018) 78:6852–65. doi: 10.1158/0008-5472.CAN-18-1750
155. Aguilar LK, Shirley LA, Chung VM, Marsh CL, Walker J, Coyle W, et al. Gene-Mediated Cytotoxic Immunotherapy as Adjuvant to Surgery or Chemoradiation for Pancreatic Adenocarcinoma. Cancer Immunol Immunother (2015) 64:727–36. doi: 10.1007/s00262-015-1679-3
156. Herman JR, Adler HL, Aguilar-Cordova E, Rojas-Martinez A, Woo S, Timme TL, et al. In Situ Gene Therapy for Adenocarcinoma of the Prostate: A Phase I Clinical Trial. Hum Gene Ther (1999) 10:1239–50. doi: 10.1089/10430349950018229
157. Chévez-Barrios P, Chintagumpala M, Mieler W, Paysse E, Boniuk M, Kozinetz C, et al. Response of Retinoblastoma With Vitreous Tumor Seeding to Adenovirus-Mediated Delivery of Thymidine Kinase Followed by Ganciclovir. J Clin Oncol (2005) 23:7927–35. doi: 10.1200/JCO.2004.00.1883
158. Sterman DH, Recio A, Vachani A, Sun J, Cheung L, DeLong P, et al. Long-Term Follow-Up of Patients With Malignant Pleural Mesothelioma Receiving High-Dose Adenovirus Herpes Simplex Thymidine Kinase/Ganciclovir Suicide Gene Therapy. Clin Cancer Res (2005) 11:7444–53. doi: 10.1158/1078-0432.CCR-05-0405
159. Maatta A-M, Samaranayake H, Pikkarainen J, Wirth T, Yla-Herttuala S. Adenovirus Mediated Herpes Simplex Virus-Thymidine Kinase/Ganciclovir Gene Therapy for Resectable Malignant Glioma. Curr Gene Ther (2009) 9:356–67. doi: 10.2174/156652309789753365
160. Guedan S, Ruella M, June CH. Emerging Cellular Therapies for Cancer. Annu Rev Immunol (2019) 37:145–71. doi: 10.1146/annurev-immunol-042718-041407
161. Li D, Li X, Zhou WL, Huang Y, Liang X, Jiang L, et al. Genetically Engineered T Cells for Cancer Immunotherapy. Signal Transduction Targeting Ther (2019) 4:35. doi: 10.1038/s41392-019-0070-9
162. Kochenderfer JN, Wilson WH, Janik JE, Dudley ME, Stetler-Stevenson M, Feldman SAJ, et al. Eradication of B-Lineage Cells and Regression of Lymphoma in a Patient Treated With Autologous T Cells Genetically Engineered to Recognize CD19. Blood (2010) 116:4099–102. doi: 10.1182/blood-2010-04-281931
163. Porter DL, Levine BL, Kalos M, Bagg A, June CH. Chimeric Antigen Receptor-Modified T Cells in Chronic Lymphoid Leukemia. N Engl J Med (2011) 365:725–33. doi: 10.1056/NEJMoa1103849
164. Newick K, Moon E, Albelda SM. Chimeric Antigen Receptor T-Cell Therapy for Solid Tumors. Mol Ther Oncolytics (2016) 3:16006. doi: 10.1038/mto.2016.6
165. Watanabe K, Luo Y, Da T, Guedan S, Ruella M, Scholler J, et al. Pancreatic Cancer Therapy With Combined Mesothelin-Redirected Chimeric Antigen Receptor T Cells and Cytokine-Armed Oncolytic Adenoviruses. JCI Insight (2018) 3:e99573. doi: 10.1172/jci.insight.99573
166. Tanoue K, Rosewell A, Watanabe N, Porter C, Rana B, Gottschalk S, et al. Armed Oncolytic Adenovirus-Expressing PD-L1 Mini-Body Enhances Antitumor Effects of Chimeric Antigen Receptor T Cells in Solid Tumors. Cancer Res (2017) 77:2040–51. doi: 10.1158/0008-5472.CAN-16-1577
167. Nishio N, Diaconu I, Liu H, Cerullo V, Caruana I, Hoyos V, et al. Armed Oncolytic Virus Enhances Immune Functions of Chimeric Antigen Receptor-Modified T Cells in Solid Tumors. Cancer Res (2014) 74:5195–205. doi: 10.1158/0008-5472.CAN-14-0697
168. Rosewell A, Porter CE, Watanabe N, Tanoue K, Sikora A, Gottschalk S, et al. Adenovirotherapy Delivering Cytokine and Checkpoint Inhibitor Augments CAR T Cells Against Metastatic Head and Neck Cancer. Mol Ther (2017) 25:2440–51. doi: 10.1016/j.ymthe.2017.09.010
169. Kelland LR. Of Mice and Men: Values and Liabilities of the Athymic Nude Mouse Model in Anticancer Drug Development. Eur J Cancer (2004) 40(6):827–36. doi: 10.1016/j.ejca.2003.11.028
170. Khaled WT, Liu P. Cancer Mouse Models: Past, Present and Future. Semin Cell Dev Biol (2014) 27:54–60. doi: 10.1016/j.semcdb.2014.04.003
171. Wolchok JD, Hodi FS, Weber JS, Allison JP, Urba WJ, Robert C, et al. Development of Ipilimumab: A Novel Immunotherapeutic Approach for the Treatment of Advanced Melanoma. Ann N Y Acad Sci (2013) 1291:1–13. doi: 10.1111/nyas.12180
172. Ribas A, Hanson DC, Noe DA, Millham R, Guyot DJ, Bernstein SH, et al. Tremelimumab (CP-675,206),a Cytotoxic T Lymphocyte–Associated Antigen 4 Blocking Monoclonal Antibody in Clinical Development for Patients With Cancer. Oncologist (2007) 12:873–83. doi: 10.1634/theoncologist.12-7-873
173. Hodi FS, O’Day SJ, McDermott DF, Weber RW, Sosman JA, Haanen JB, et al. Improved Survival With Ipilimumab in Patients With Metastatic Melanoma. N Engl J Med (2010) 363:711–23. doi: 10.1056/NEJMoa1003466
174. Kirkwood JM, Lorigan P, Hersey P, Hauschild A, Robert C, McDermott D, et al. Phase II Trial of Tremelimumab (CP-675,206) in Patients With Advanced Refractory or Relapsed Melanoma. Clin Cancer Res (2010) 16:1042–8. doi: 10.1158/1078-0432.CCR-09-2033
175. Dias JD, Hemminki O, Diaconu I, Hirvinen M, Bonetti A, Guse K, et al. Targeted Cancer Immunotherapy With Oncolytic Adenovirus Coding for a Fully Human Monoclonal Antibody Specific for CTLA-4. Gene Ther (2012) 19:988–98. doi: 10.1038/gt.2011.176
176. Cervera-Carrascon V, Siurala M, Santos JM, Havunen R, Tähtinen S, Karell P, et al. TNFa and IL-2 Armed Adenoviruses Enable Complete Responses by Anti-PD-1 Checkpoint Blockade. Oncoimmunology (2018) 7:e1412902. doi: 10.1080/2162402X.2017.1412902
177. Fueyo J, Gomez-Manzano C, Alemany R, Lee PS, McDonnell TJ, Mitlianga P, et al. A Mutant Oncolytic Adenovirus Targeting the Rb Pathway Produces Anti-Glioma Effect In Vivo. Oncogene (2000) 19:2–12. doi: 10.1038/sj.onc.1203251
178. Aiken R, Chen C, Cloughesy T, Colman H, Daras M, Groves M, et al. Atim-33. Interim Results of a Phase Ii Multi-Center Study of Oncolytic Adenovirus Dnx-2401 With Pembrolizumab for Recurrent Glioblastoma; Captive Study (Keynote-192). Neuro-Oncology (2019) 21:vi8–9. doi: 10.1093/neuonc/noz175.032
179. Daniyan AF, Brentjens RJ. Immunotherapy: Hiding in Plain Sight: Immune Escape in the Era of Targeted T-Cell-Based Immunotherapies. Nat Rev Clin Oncol (2017) 14(6):333–4. doi: 10.1038/nrclinonc.2017.49
180. Cai L, Michelakos T, Yamada T, Fan S, Wang X, Schwab JH, et al. Defective HLA Class I Antigen Processing Machinery in Cancer. Cancer Immunol Immunother (2018) 67(6):999–1009. doi: 10.1007/s00262-018-2131-2
181. Pettit SJ, Ali S, O'Flaherty E, Griffiths TR, Neal DE, Kirby JA. Bladder Cancer Immunogenicity: Expression of CD80 and CD86 is Insufficient to Allow Primary CD4+ T Cell Activation In Vitro. Clin Exp Immunol (1999) 116(1):48–56. doi: 10.1046/j.1365-2249.1999.00857.x
182. Tirapu I, Huarte E, Guiducci C, Arina A, Zaratiegui M, Murillo O, et al. Low Surface Expression of B7-1 (CD80) is an Immunoescape Mechanism of Colon Carcinoma. Cancer Res (2006) 66(4):2442–50. doi: 10.1158/0008-5472.CAN-05-1681
183. Gabitzsch ES, Morse MA, Lyerly HK, Balint J, Jones F. Immunotherapeutic Treatment of Metastatic Colorectal Cancer Using ETBX-011. J Clin Oncol (2014) 32:3093. doi: 10.1200/jco.2014.32.15_suppl.3093
184. Morse MA, Chaudhry A, Gabitzsch ES, Hobeika AC, Osada T, Clay TM, et al. Novel Adenoviral Vector Induces T-Cell Responses Despite Anti-Adenoviral Neutralizing Antibodies in Colorectal Cancer Patients. Cancer Immunol Immunother (2013) 62:1293–301. doi: 10.1007/s00262-013-1400-3
185. Balint JP, Gabitzsch ES, Rice A, Latchman Y, Xu Y, Messerschmidt GL, et al. Extended Evaluation of a Phase 1/2 Trial on Dosing, Safety, Immunogenicity, and Overall Survival After Immunizations With an Advanced-Generation Ad5 [E1-, E2b-]-CEA(6D) Vaccine in Late-Stage Colorectal Cancer. Cancer Immunol Immunother (2015) 64:977–87. doi: 10.1007/s00262-015-1706-4
186. Gabitzsch ES, Tsang KY, Palena C, David JM, Fantini M, Kwilas A, et al. The Generation and Analyses of a Novel Combination of Recombinant Adenovirus Vaccines Targeting Three Tumor Antigens as an Immunotherapeutic. Oncotarget (2015) 6:31344–59. doi: 10.18632/oncotarget.5181
187. Gatti-Mays ME, Redman JM, Donahue RN, Palena C, Madan RA, Karzai F, et al. A Phase I Trial Using a Multitargeted Recombinant Adenovirus 5 (CEA/MUC1/Brachyury)-Based Immunotherapy Vaccine Regimen in Patients With Advanced Cancer. Oncologist (2019) 25:1–6. doi: 10.1634/theoncologist.2019-0608
188. Elzey BD, Siemens DR, Ratliff TL, Lubaroff DM. Immunization With Type 5 Adenovirus Recombinant for a Tumor Antigen in Combination With Recombinant Canarypox Virus (Alvac) Cytokine Gene Delivery Induces Destruction of Established Prostate Tumors. Int J Cancer (2001) 94:842–9. doi: 10.1002/ijc.1556
189. Lubaroff DM, Konety B, Link BK, Ratliff TL, Madsen T, Shannon M, et al. Clinical Protocol: Phase I Study of an Adenovirus/Prostate-Specific Antigen Vaccine in Men With Metastatic Prostate Cancer. Hum Gene Ther (2006) 17:220–9. doi: 10.1089/hum.2006.17.220
190. Lubaroff DM, Williams RD, Vaena D, Joudi F, Brown J, Smith M, et al. An Ongoing Phase II Trial of an Adenovirus/PSA Vaccine for Prostate Cancer. Cancer Res (2012) 72:2692. doi: 10.3390/cancers12051295;191pub-id>
191. Melero I, Gaudernack G, Gerritsen W, Huber C, Parmiani G, Scholl S, et al. Therapeutic Vaccines for Cancer: An Overview of Clinical Trials. Nat. Rev. Clin Oncol (2014) 11:509–24. doi: 10.1038/nrclinonc.2014.111
192. Wieking BG, Vermeer DW, Spanos WC, Lee KM, Vermeer P, Lee WT, et al. A non-Oncogenic HPV 16 E6/E7 Vaccine Enhances Treatment of HPV Expressing Tumors. Cancer Gene Ther (2012) 19:667–74. doi: 10.1038/cgt.2012.55
193. Atherton MJ, Stephenson KB, Nikota JK, Hu QN, Nguyen A, Wan Y, et al. Preclinical Development of Peptide Vaccination Combined With Oncolytic MG1-E6E7 for HPV- Associated Cancer. Vaccine (2018) 36:2181–92. doi: 10.1016/j.vaccine.2018.02.070
194. Pol JG, Atherton MJ, Bridle BW, Stephenson KB, Le Boeuf F, Hummel JL, et al. Development and Applications of Oncolytic Maraba Virus Vaccines. Oncolytic Virother (2018) 7:117–28. doi: 10.2147/OV.S154494
195. Cappuccini F, Stribbling S, Pollock E, Hill AVS, Redchenko I. Immunogenicity and Efficacy of the Novel Cancer Vaccine Based on Simian Adenovirus and MVA Vectors Alone and in Combination With PD-1 MAb in a Mouse Model of Prostate Cancer. Cancer Immunol Immunother (2016) 65:701–13. doi: 10.1007/s00262-016-1831-8
196. Wan Y, Emtage P, Foley R, Carter R, Gauldie J. Murine Dendritic Cells Transduced With an Adenoviral Vector Expressing a Defined Tumor Antigen can Overcome Anti-Adenovirus Neutralizing Immunity and Induce Effective Tumor Regression. Int J Oncol (1999) 14:771–6. doi: 10.3892/ijo.14.4.771
197. Nikitina EY, Chada S, Muro-Cacho C, Fang B, Zhang R, Roth JA, et al. An Effective Immunization and Cancer Treatment With Activated Dendritic Cells Transduced With Full-Length Wild-Type P53. Gene Ther (2002) 9:345–52. doi: 10.1038/sj.gt.3301670
198. Brossart P, Goldrath AW, Butz EA. Virus-Mediated Delivery of Antigenic Epitopes Into Dendritic Cells as a Means to Induce CTL. J Immunol (1997) 158:3270–6.
199. Butterfield LH, Comin-Anduix B, Vujanovic L, Lee Y, Dissette VB, Yang J-Q, et al. Adenovirus MART-1–Engineered Autologous Dendritic Cell Vaccine for Metastatic Melanoma. J Immunother (2008) 31:294–309. doi: 10.1097/CJI.0b013e31816a8910
200. Buller RE, Runnebaum IB, Karlan BY, Horowitz JA, Shahin M, Buekers TM, et al. A Phase I/II Trial of RAd/P53 (SCH 58500) Gene Replacement in Recurrent Ovarian Cancer. Cancer Gene Ther (2002) 9:553–66. doi: 10.1038/sj.cgt.7700472
201. Kieran MW, Manley L, Chi SN, Marcus KJ, Manzanera AG, Polanco M, et al. Phase I Study of Gene-Mediated Cytotoxic Immunotherapy With AdV-Tk as Adjuvant to Surgery and Radiation for Pediatric Malignant Glioma and Recurrent Ependymoma. Neuro Oncol (2019) 21:537–46. doi: 10.1093/neuonc/noy202
202. Havunen R, Siurala M, Sorsa S, Grönberg-Vähä-Koskela S, Behr M, Tähtinen S, et al. Oncolytic Adenoviruses Armed With Tumor Necrosis Factor Alpha and Interleukin-2 Enable Successful Adoptive Cell Therapy. Mol Ther Oncolytics (2016) 4:77–86. doi: 10.1016/j.omto.2016.12.004
203. Behbahani TE, Rosenthal EL, Parker WB, Sorscher EJ. Intratumoral Generation of 2-Fluoroadenine to Treat Solid Malignancies of the Head and Neck. Head Neck (2019) 41:1979–83. doi: 10.1002/hed.25627
204. Lowenstein PR, Orringer DA, Sagher O, Heth J, Hervey-Jumper SL, Mammoser AG, et al. First-In-Human Phase I Trial of the Combination of Two Adenoviral Vectors Expressing HSV1-TK and FLT3L for the Treatment of Newly Diagnosed Resectable Malignant Glioma: Initial Results From the Therapeutic Reprogramming of the Brain Immune System. J Clin Oncol (2019) 37. doi: 10.1200/JCO.2019.37.15_suppl.2019
205. Goto Y, Ohe Y, Kuribayashi K, Nakano T, Okada M, Toyooka S, et al. P2.06-11 A Phase I/II Study of Intrapleural Ad-SGE-REIC Administration in Patients With Refractory Malignant Pleural Mesothelioma. J Thorac Oncol (2018) 13:S746. doi: 10.1016/j.jtho.2018.08.1266
206. Zhu R, Weng D, Lu S, Lin D, Wang M, Chen D, et al. Double-Dose Adenovirus-Mediated Adjuvant Gene Therapy Improves Liver Transplantation Outcomes in Patients With Advanced Hepatocellular Carcinoma. Hum Gene Ther (2018) 29:251–8. doi: 10.1089/hum.2017.114
207. Vogels R, Zuijdgeest D, van RR, Hartkoorn E, Damen I, de Bethune MP, et al. Replication-Deficient Human Adenovirus Type 35 Vectors for Gene Transfer and Vaccination: Efficient Human Cell Infection and Bypass of Preexisting Adenovirus Immunity. J Virol (2003) 77(15):8263–71. doi: 10.1128/JVI.77.15.8263-8271.2003
208. Nwanegbo E, Vardas E, Gao W, Whittle H, Sun H, Rowe D, et al. Prevalence of Neutralizing Antibodies to Adenoviral Serotypes 5 and 35 in the Adult Populations of The Gambia, South Africa, and the United States. Clin Diagn Lab Immunol (2004) 11(2):351–7. doi: 10.1128/CDLI.11.2.351-357.2004
209. Singh S, Vedi S, Samrat S, Li W, Kumar R, Agrawal B. Heterologous Immunity Between Adenoviruses and Hepatitis C Virus: A New Paradigm in HCV Immunity and Vaccines. PloS One (2016) 11(1):e0146404. doi: 10.1371/journal.pone.0146404
210. Agrawal B, Singh S, Gupta N, Li W, Vedi S, Kumar R. Unsolved Puzzles Surrounding HCV Immunity: Heterologous Immunity Adds Another Dimension. Int J Mol Sci (2017) 18(8):1626–39. doi: 10.3390/ijms18081626
211. Shiver JW, Emini EA. Recent Advances in the Development of HIV-1 Vaccines Using Replication-Incompetent Adenovirus Vectors. Annu. Rev Med (2004) 55:355–72. doi: 10.1146/annurev.med.55.091902.104344
212. Krause A, Joh JH, Hackett NR, Roelvink PW, Bruder JT, Wickham TJ, et al. Epitopes Expressed in Different Adenovirus Capsid Proteins Induce Different Levels of Epitope-Specific Immunity. J Virol (2006) 80:5523–30. doi: 10.1128/JVI.02667-05
213. Bradley RR, Maxfield LF, Lynch DM, Iampietro MJ, Borducchi EN, Barouch DH. Adenovirus Serotype 5-Specific Neutralizing Antibodies Target Multiple Hexon Hypervariable Regions. J Virol (2012) 86:1267–72. doi: 10.1128/JVI.06165-11
214. Smith JG, Cassany A, Gerace L, Ralston R, Nemerow GR. Neutralizing Antibody Blocks Adenovirus Infection by Arresting Microtubule-Dependent Cytoplasmic Transport. J Virol (2008) 82:6492–500. doi: 10.1128/JVI.00557-08
215. Olive M, Eisenlohr L, Flomenberg N, Hsu S, Flomenberg P. The Adenovirus Capsid Protein Hexon Contains a Highly Conserved Human CD4 + T-Cell Epitope. Hum Gene Ther (2002) 13:1167–78. doi: 10.1089/104303402320138952
216. Capone S, Meola A, Ercole B, Vitelli A, Pezzanera M, Ruggeri L, et al. A Novel Adenovirus Type 6 (Ad6)-Based Hepatitis C Virus Vector That Overcomes Preexisting Anti- Ad5 Immunity and Induces Potent and Broad Cellular Immune Responses in Rhesus Macaques. J Virol (2006) 80(4):1688–99. doi: 10.1128/JVI.80.4.1688-1699.2006
217. Biswas S, Choudhary P, Elias SC, Miura K, Milne KH, de Cassan SC, et al. Assessment of Humoral Immune Responses to Blood-Stage Malaria Antigens Following ChAd63- MVA Immunization, Controlled Human Malaria Infection and Natural Exposure. PloS One (2014) 9(9):e107903. doi: 10.1371/journal.pone.0107903
218. Swadling L, Capone S, Antrobus RD, Brown A, Richardson R, Newell EW, et al. A Human Vaccine Strategy Based on Chimpanzee Adenoviral and MVA Vectors That Primes, Boosts, and Sustains Functional HCV-Specific T Cell Memory. Sci Trans Med (2014) 6(261):261ra153. doi: 10.1126/scitranslmed.3009185
219. Leen A, Sili U, Vanin E, Jewell A, Xie W, Vignali D, et al. Conserved CTL Epitopes on the Adenovirus Hexon Protein Expand Subgroup Cross-Reactive and Subgroup-Specific CD8+ T Cells. Blood (2004) 104(8):2432–40. doi: 10.1182/blood-2004-02-0646
220. Veltrop-Duits L, Heemskerk B, Sombroek C, Vreeswijk T, Gubbels S, Ree T, et al. Human CD4+ T Cells Stimulated by Conserved Adenovirus 5 Hexon Peptides Recognize Cells Infected With Different Species of Human Adenovirus. Eur J Immunol (2006) 36(9):2410–23. doi: 10.1002/eji.200535786
221. Reddy PS, Idamakanti N, Zakhartchouk AN, Baxi MK, Lee JB, Pyne C, et al. Nucleotide Sequence, Genome Organization, and Transcription Map of Bovine Adenovirus Type 3. J Virol (1998) 72(2):1394–402. doi: 10.1128/JVI.72.2.1394-1402.1998
222. Hammond JM1, McCoy RJ, Jansen ES, Morrissy CJ, Hodgson AL, Johnson MA. Vaccination With a Single Dose of a Recombinant Porcine Adenovirus Expressing the Classical Swine Fever Virus Gp55 (E2) Gene Protects Pigs Against Classical Swine. Vaccine (2000) 18(11-12):1040–50. doi: 10.1016/S0264-410X(99)00347-3
223. Moffatt S, Hays J, HogenEsch H, Mittal S. Circumvention of Vector-Specific Neutralizing Antibody Response by Alternating Use of Human and non-Human Adenoviruses: Implications in Gene Therapy. Virol (2000) 272(1):159–67. doi: 10.1006/viro.2000.0350
224. Wüest T, Both GW, Prince AM, Hofmann C, Löser P. Recombinant Ovine Atadenovirus Induces a Strong and Sustained T Cell Response Against the Hepatitis C Virus NS3 Antigen in Mice. Vaccine (2004) 22:2717–21. doi: 10.1016/j.vaccine.2004.01.048
225. Guo J, Mondal M, Zhou D. Development of Novel Vaccine Vectors: Chimpanzee Adenoviral Vectors. Hum Vaccines Immunotherapeutics (2018) 14:1679–85. doi: 10.1080/21645515.2017.1419108
226. McCoy K, Tatsis N, Korioth-Schmitz B, Lasaro MO, Hensley SE, Lin S-W, et al. Effect of Preexisting Immunity to Adenovirus Human Serotype 5 Antigens on the Immune Responses of Nonhuman Primates to Vaccine Regimens Based on Human- or Chimpanzee- Derived Adenovirus Vectors. J Virol (2007) 81(12):6594–604. doi: 10.1128/JVI.02497-06
227. Hayton E-J, Rose A, Ibrahimsa U, Sorbo M, Capone S, Crook A, et al. Safety and Tolerability of Conserved Region Vaccines Vectored by Plasmid DNA, Simian Adenovirus and Modified Vaccinia Virus Ankara Administered to Human Immunodeficiency Virus Type 1-Uninfected Adults in a Randomized, Single-Blind Phase I Trial. PloS One (2014) 9(7):e101591. doi: 10.1371/journal.pone.0101591
228. Afolabi MO, Tiono AB, Adetifa UJ, Yaro J, Drammeh A, Nébié I, et al. Safety and Immunogenicity of ChAd63 and MVA ME-TRAP in West African Children and Infants. Mol Ther (2016) 24(8):1470–7. doi: 10.1038/mt.2016.83
229. Mensah VA, Gueye A, Ndiaye M, Edwards NJ, Wright D, Anagnostou NA, et al. Safety, Immunogenicity and Efficacy of Prime-Boost Vaccination With ChAd63 and MVA Encoding ME-TRAP Against Plasmodium Falciparum Infection in Adults in Senegal. PloS One (2016) 11(12):e0167951. doi: 10.1371/journal.pone.0167951
230. Xiang Z, Gao G, Reyes-Sandoval A, Li Y, Wilson J. Oral Vaccination of Mice With Adenoviral Vectors is Not Impaired by Preexisting Immunity to the Vaccine Carrier. J Virol (2003) 77(20):10780–9. doi: 10.1128/JVI.77.20.10780-10789.2003
231. Lin SW, Cun AS, Harris-McCoy K, Ertl HC. Intramuscular Rather Than Oral Administration of Replication-Defective Adenoviral Vaccine Vector Induces Specific CD8+ T Cell Responses in the Gut. Vaccine (2007) 25(12):2187–93. doi: 10.1016/j.vaccine.2006.11.044
232. Mackay LK, Stock AT, Ma JZ, Jones CM, Kent SJ, Mueller SN, et al. Long-Lived Epithelial Immunity by Tissue-Resident Memory T (TRM) Cells in the Absence of Persisting Local Antigen Presentation. Proc Natl Acad Sci United States America (2012) 109(18):7037–42. doi: 10.1073/pnas.1202288109
233. Richardson JS, Pillet S, Bello AJ. Airway Delivery of an Adenovirus-Based Ebola Virus Vaccine Bypasses Existing Immunity to Homologous Adenovirus in Nonhuman Primates. J Virol (2013) 87(7):3668–77. doi: 10.1128/JVI.02864-12
234. Kaulfub M, Wensing I, Windmann S, Hrycak C, Bayer W. Induction of Complex Immune Responses and Strong Protection Against Retrovirus Challenge by Adenovirus-Based Immunization Depends on the Order of Vaccine Delivery. RetroVirol (2017) 4(1):8. doi: 10.1186/s12977-017-0336-7
235. Barouch DH, Pau MG, Custers JH, Koudstaal W, Kostense S, Havenga MJ, et al. Immunogenicity of Recombinant Adenovirus Serotype 35 Vaccine in the Presence of Pre-Existing Anti-Ad5 Immunity. J Immunol (2004). doi: 10.4049/jimmunol.172.10.6290
236. Holterman L, Vogels R, van der Vlugt R, Sieuwerts M, Grimbergen J, Kaspers J, et al. Novel Replication-Incompetent Vector Derived From Adenovirus Type 11 (Ad11) for Vaccination and Gene Therapy: Low Seroprevalence and non-Cross-Reactivity With Ad5. J Virol (2004) 78(23):13207–15. doi: 10.1128/JVI.78.23.13207-13215.2004
Keywords: adenoviruses, cancer therapy, anticancer vaccine, gene therapy, adenoviruse, adenoviral vector
Citation: Tseha ST (2022) Role of Adenoviruses in Cancer Therapy. Front. Oncol. 12:772659. doi: 10.3389/fonc.2022.772659
Received: 15 December 2021; Accepted: 06 May 2022;
Published: 09 June 2022.
Edited by:
Kwong Tsang, Precision Biologics, Inc., United StatesReviewed by:
Pierpaolo Correale, Azienda ospedaliera ‘Bianchi-Melacrino-Morelli’, ItalyJustin David, Bristol Myers Squibb, United States
Copyright © 2022 Tseha. This is an open-access article distributed under the terms of the Creative Commons Attribution License (CC BY). The use, distribution or reproduction in other forums is permitted, provided the original author(s) and the copyright owner(s) are credited and that the original publication in this journal is cited, in accordance with accepted academic practice. No use, distribution or reproduction is permitted which does not comply with these terms.
*Correspondence: Sintayehu Tsegaye Tseha, sintayehu.tsegaye@amu.edu.et