- 1Department of Clinical Cytology, Centre of Postgraduate Medical Education, Warsaw, Poland
- 2Department of Neurosurgery, Institute of Psychiatry and Neurology, Warsaw, Poland
- 3Department of Radiology, Institute of Psychiatry and Neurology, Warsaw, Poland
Gliomas are the most common primary malignant intracranial brain tumors. Their proliferative and invasive behavior is controlled by various epigenetic mechanisms. 5-hydroxymethylcytosine (5-hmC) is one of the epigenetic DNA modifications that employs ten-eleven translocation (TET) enzymes to its oxidation. Previous studies demonstrated altered expression of 5-hmC across gliomagenesis. However, its contribution to the initiation and progression of human gliomas still remains unknown. To characterize the expression profiles of 5-hmC and TET in human glioma samples we used the EpiJET 5-hmC and 5-mC Analysis Kit, quantitative real-time PCR, and Western blot analysis. A continuous decline of 5-hmC levels was observed in solid tissue across glioma grades. However, in glioblastoma (GBM), we documented uncommon heterogeneity in 5-hmC expression. Further analysis showed that the levels of TET proteins, but not their transcripts, may influence the 5-hmC abundance in GBM. Early tumor-related biomarkers may also be provided by the study of aberrant DNA hydroxymethylation in the blood of glioma patients. Therefore, we explored the patterns of TET transcripts in plasma samples and we found that their profiles were variously regulated, with significant value for TET2. The results of our study confirmed that DNA hydroxymethylation is an important mechanism involved in the pathogenesis of gliomas, with particular reference to glioblastoma. Heterogeneity of 5-hmC and TET proteins expression across GBM may provide novel insight into define subtype-specific patterns of hydroxymethylome, and thus help to interpret the heterogeneous outcomes of patients with the same disease.
Introduction
The vast majority (80%) of malignant brain tumors is represented by gliomas (1). They have been classified by the World Health Organization into four grades, with Grade IV glioblastoma (GBM) as the most aggressive form. From 2016 the WHO grading is based on histological and molecular characteristics that are observed amongst various stages of gliomas (2). Many studies focused on genomic or transcriptomic profiles of human gliomas demonstrated that although histologically similar, they constitute distinct subtypes (3–5) that are associated with different survival outcomes (6, 7). Therefore, systematic molecular analysis of human gliomas is essential for their comprehensive understanding. Aberrations in genes and molecular pathways, include IDH1/IDH2 and H3.1/H3.3 mutation or loss of TP53 tumor suppressor gene, can be used together with histopathological findings for gliomas classification (8, 9).
However, dysregulation of epigenomes seems to be the primary molecular mechanism involved in the pathogenesis of human gliomas. Epigenetic alterations, such as modifications of DNA (10, 11) and histones (12, 13), nucleosome remodeling (14, 15) and RNA-mediated silencing (16–18) are pointed out as a source of gliomas phenotypic heterogeneity. DNA methylation is the best-studied epigenetic change in this field (19–21), but some reports revealed the existence of other modifications in DNA methylome.
The ten-eleven translocation (TET) enzymes can alter DNA methylation status by converting 5-methylcytosine (5-mC) to 5-hydroxymethylcytosine (5-hmC), and later to 5-formylcytosine (5-fC) and 5-carboxylcytosine (5-caC) (22). 5-hmC may act as a transient intermediate in the process of 5-mC demethylation, as well as, may epigenetically regulate gene expression. Several reports found the relationship between 5-hmC level and glioma grades (10, 23–26). However, 5-hmC modification and its direct effect on gliomas biology need to be investigated.
Here, we picture the TET-dependent hydroxymethylation patterns in human gliomas. Using solid tumor tissues samples we demonstrate heterogeneity in 5-hmC expression across glioblastoma which can provide novel insight into define subtype-specific patterns of 5-hmC, and thus help to interpret the heterogeneous outcomes of patients with the same disease.
Materials and Methods
Clinical Samples
34 pairs of matched glioma tissues and blood samples were collected during standard neurosurgical tumor removals at the Department of Neurosurgery, Institute of Psychiatry and Neurology (Warsaw, Poland). Additionally, five independent random controls of blood samples were obtained from healthy volunteers. All solid tissues were submerged in the stayRNA solution (A&A Biotechnology) and stored at –80°C. Blood was collected into EDTA-treated tubes and centrifuged at 3500 rpm (MPW, Centrifuge MPW-350R, Rotor 1236B) for 10 min at 4°C. Then supernatant (plasma) was immediately transferred into the clean Eppendorf tubes and stored at –80°C.
5-hmC Quantification
The absolute level of 5-hmC in genomic DNA, previously extracted from 34 glioma tissues, was estimated with the EpiJET 5-hmC and 5-mC Analysis Kit (ThermoFisher Scientific). Briefly, 100 ng of DNA was glucosylated by T4 phage β-glucosyltransferase (T4 BGT), followed by subsequent digestion with Epi MspI and Epi HpaII enzymes. Then the percentage of cytosine modifications within CCGG sites was determined by quantitative real-time PCR (qRT-PCR) with a primer pair flanking recognition sequence. Primer sequences were as follows:
primer1_forward 5′-CTGTCATGGTGACAAAGGCATC-3′,
primer2_reverse 5′-CAGGATTTCTCTATTATGAAGACCTTG-3′.
The experiment was run in triplicate and the amount of 5-hmC was calculated as a percentage based on controls included in the kit.
Quantitative Real-Time PCR
Following Chomczynski`s protocol (27), total RNA of glioma tissues was extracted by TRIzol Reagent (Life Technologies), whereas Total RNA Mini Concentrator Kit (A&A Biotechnology) was used for the extraction of RNA from plasma samples. The concentration and purity of RNA samples were assessed by measuring the 260/280 ratio of absorbance values with the Synergy H4 spectrophotometer (BioTek). cDNA was synthesized from 500 ng of RNA using random hexamers and TaqMan Reverse Transcription Reagents (ThermoFisher Scientific) according to the manufacturer`s instructions. Transcript levels of TET family genes were determined by the quantitative real-time PCR using 5x HOT FirePolEvaGreen qPCR Mix (Solis Biodyne) and primer sets for TET1, TET2, TET3, and the housekeeping gene GAPDH. The primer sequences are listed in Supplementary Table S1. All samples were run in triplicate, and data were normalized to the expression of GAPDH (28), according to the ΔCt method. While the ΔΔCt method was applied for relative quantification in blood samples.
Western Blot
Thirty-four human glioma tissues were homogenized with TissueLyser LT homogenizer (Qiagen) in lysis buffer containing 2% SDS, pH 6.8 and protease inhibitors (Sigma). According to the protocol of Ericsson et al. (29), homogenates were incubated at 70°C and shaking at 1400 rpm (BioSan, Thermo-Shaker TS-100) for 10 min and then centrifuged at 12000 rpm (Eppendorf, Centrifuge 5415R, Rotor F45-24-11) for 5 min. The concentration of protein extracts was determined with the Bradford protein assay (Sigma). 15 µg of each protein sample was separated with 7% SDS-polyacrylamide gels and transferred onto nitrocellulose membranes using the Bio-Rad MINI Protean system. Immunoblotting with primary antibodies against TET1, TET2, TET3 (1:3000, ThermoFisher Scientific) and GAPDH (1:5000, Millipore) was performed overnight at 4°C, whereas secondary antibodies (a-mouse and a-rabbit, Vector) diluted 1:10000 were incubated with the membranes for one hour at room temperature. Blots were visualized with the WesternBright Quantum detection system (Advansta) on the UVITEC Cambridge scanner. Densitometry analysis was conducted using GelAnalyzer 2010 software.
Statistical Analysis
GraphPad Prism 7.02 software was used to analyze the data. Statistical significance of differences between groups was determined by One-way ANOVA or nonparametric Kruskal-Wallis tests followed by post hoc Tukey or Dunn analysis, as well as with Mann-Whitney U test (*p < 0.05, **p < 0.01, ***p < 0.001). The results are presented as the mean ± standard deviation of the mean (SD).
Results
Global Changes in 5-hmC Abundance in Gliomas
So far, decreased 5-hmC levels have been presented in a variety of tumors (30–32), suggesting that the loss of 5-hmC may be considered as an epigenetic hallmark of the disease. To evaluate the global changes in 5-hmC abundance in human brain tumors, we selected DNA of 34 glioma samples represented by different WHO grades and analyzed them with EpiJET 5-hmC and 5-mC Analysis Kit and quantitative real-time PCR. Clinical and epigenetic characteristics of glioma samples are shown in Table 1.
As can be seen in Figure 1 and Table 1, gliomagenesis has generated the changes of DNA hydroxymethylation. The 5-hmC expression correlated negatively with WHO grading, ranged from 8.2% in Grade II gliomas to 3.0% in Grade IV-low5-hmC GBM. Significant differences were observed between Grades II and III (p < 0.01), as well as Grades II and IV-low5-hmC (p < 0.01). While we observed a decreasing trend in 5-hmC expression with a higher tumor stage, we documented a strong elevation in 5-hmC level in around 30% of Grades IV GBM (Grade IV-high5-hmC). In this study group, the 5-hmC level was 20% and was significantly higher than in Grade IV-low5-hmC samples (3%, p < 0.001). To our knowledge, this is one of the few reports describing high variability in 5-hmC abundance at the tumor mass (10, 24). To clarify observed diversity, the GBMs samples were categorized according to their IDH1, and developmental status. The results had not revealed molecular differences between both categories in this scope. A great majority of samples (95%) were primary glioblastomas, while all of them demonstrated the absence of mutation in IDH1 gene.
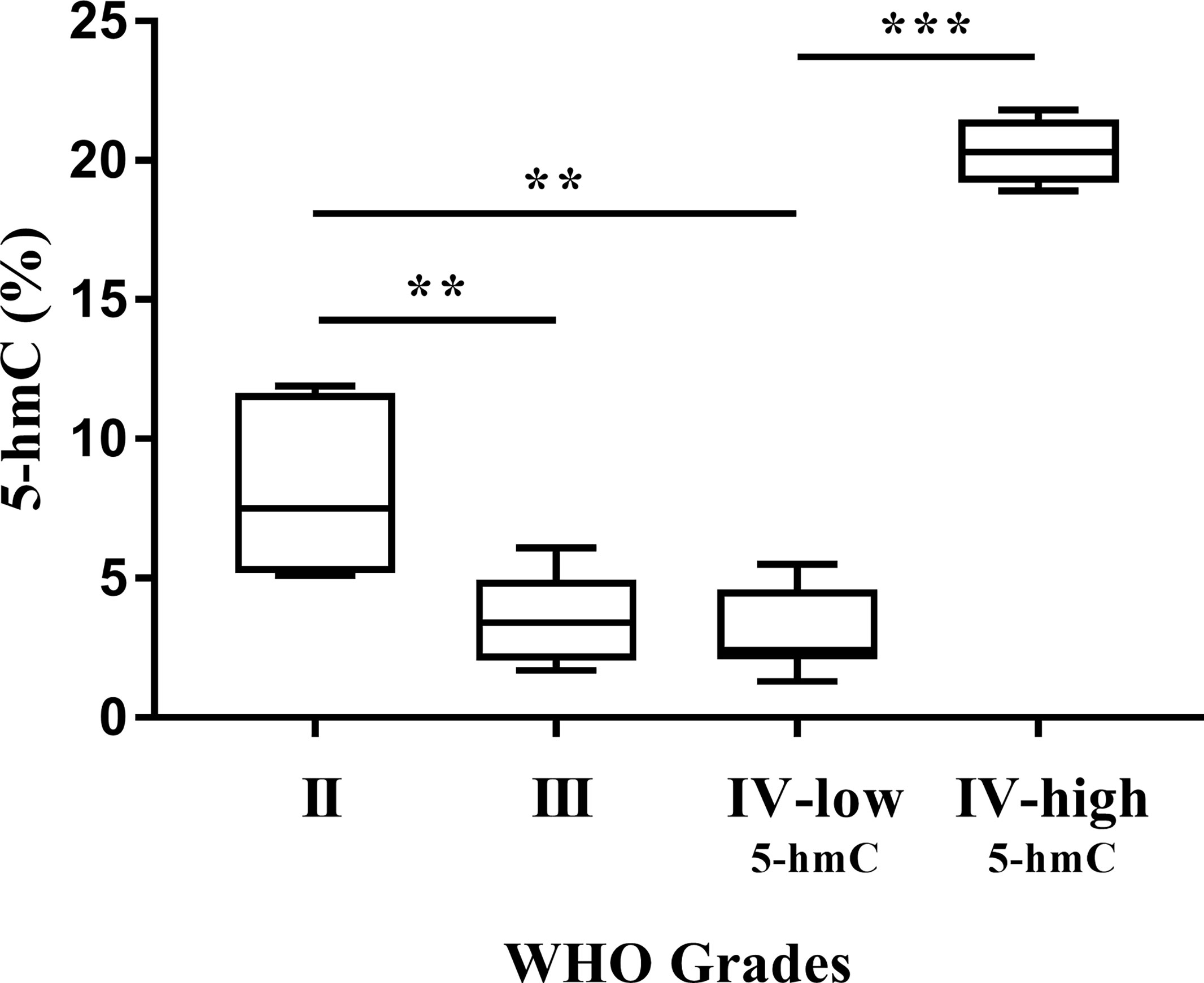
Figure 1 Global 5-hmC abundance (%) in gliomas. Increasing WHO grades of glioma are associated with a continuous decline in 5-hmC levels, except for glioblastoma (IV-low5-hmC and IV-high5-hmC). Differences among group means were evaluated by one-way ANOVA test (**p < 0.01, ***p < 0.001).
Recognized differences might be a consequence of alterations in the expression of TET enzymes that catalyze the conversion of 5-mC to 5-hmC.
TET Expression in Gliomas
To define the impact of TET enzymes on changes in DNA hydroxymethylation, we analyzed their expression at the gene and protein levels in solid tumor tissues. Quantitative real-time PCR was performed to examine the mRNA expression of TET family genes (TET1, TET2, and TET3) in 34 samples, including 6 Grade II gliomas, 7 Grade III gliomas and 21 Grade IV GBM. We found that the relative mRNA levels of all three TET genes were strongly reduced during glioma grades (Figures 2A, C, E). Their downregulation was significantly higher in Grade IV GBM in comparison with Grade II gliomas (TET1 and TET3 p < 0.001, TET2 p < 0.01) and Grade III gliomas (TET1 p < 0.001, TET2 and TET3 p < 0.01). As the decline in TET mRNA was associated with glioma grades and 5-hmC expression, we determined the levels of TET transcripts in two groups with high variability in total 5-hmC abundance across GBM (Grade IV-low5-hmC and Grade IV-high5-hmC, Figure 1). There were no significant differences in TET1 (p = 0.1322), TET2 (p = 0.2434) and TET3 (p = 0.8208) mRNA levels between glioblastoma Grade IV-low5-hmC and Grade IV-high5-hmC (Figures 2B, D, F). Next, we evaluated the levels of TET proteins in the same samples by Western blot. Expression levels of each TET proteins are shown in Supplementary Table S2. Immunoblotting revealed the absence of TET1 isoform at 235 kDa, which is mainly detected in embryonic stem cells. But, two additional bands at 162 kDa and 150 kDa were found in the majority of instances. TET2 and TET3 isoforms were discovered at 224 and 194 kDa, respectively (Figure 3A). As in the case of TET transcripts expression (Figures 2A, C, E), levels of TET proteins were negatively associated with advanced stages of gliomas. From Grade II gliomas to Grade IV GBM, we noticed 2-, 4- and 6-fold reduction in TET1162 and 150 kDa, TET2224 kDa and TET3194 kDa levels, respectively. Interestingly, in Grade IV samples, we observed high variability in TET isoforms expression pattern, which could affect 5-hmC abundance. Quantification of normalized values showed significant differences in TET1162 and 150 kDa (p < 0.01, p < 0.05), TET2224 kDa (p < 0.001) and TET3194 kDa (p < 0.05) protein levels between GBM Grade IV-low5-hmC and Grade IV-high5-hmC (Figures 3B–E). These findings suggested the potential role of TET proteins patterns in setting the 5-hmC level in Grade IV glioblastoma.
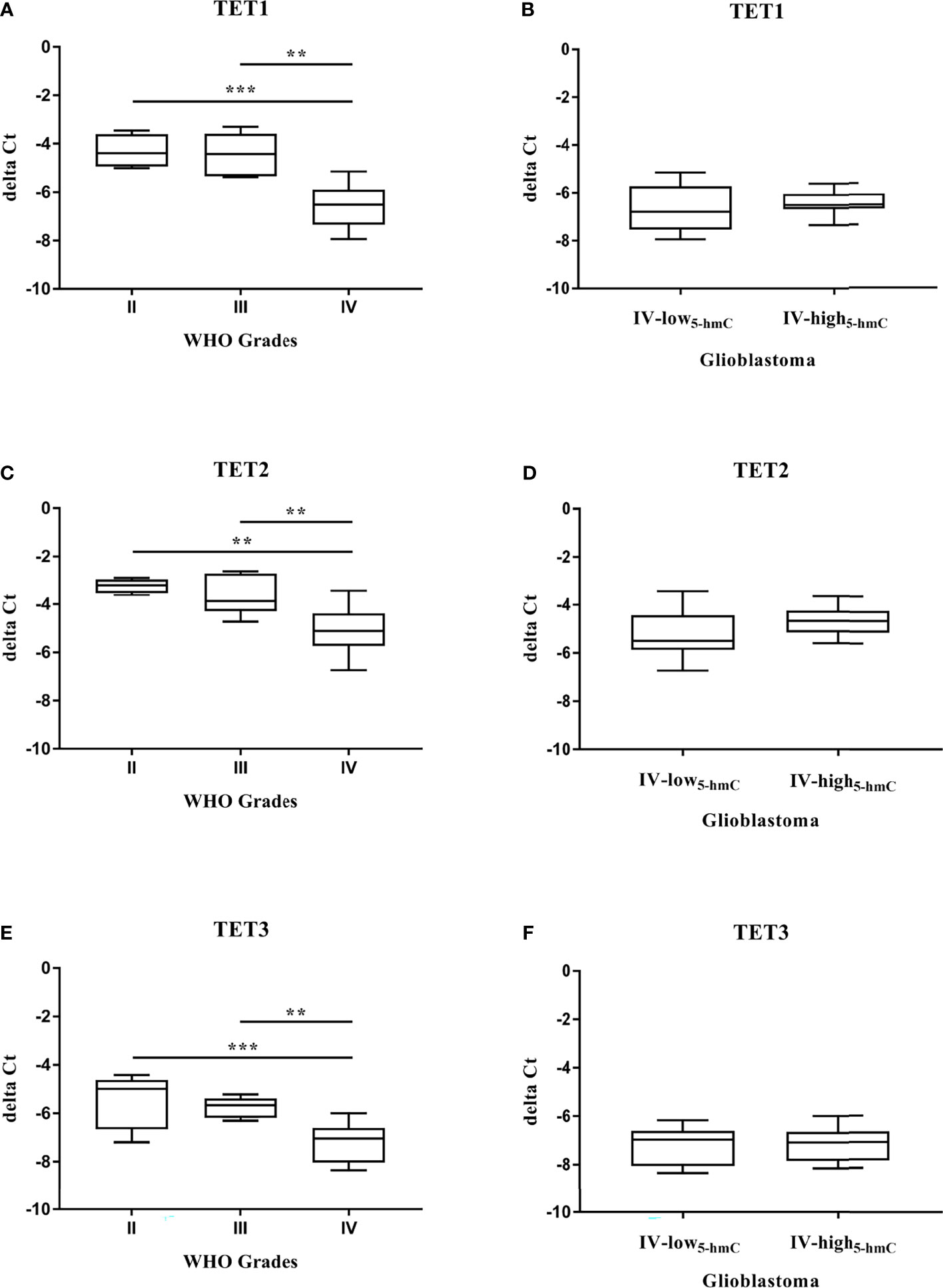
Figure 2 Quantitation of TET transcripts in gliomas. qRT–PCR analysis of TET mRNA (A–F). The expression of TET1 (A), TET2 (C), TET3 (E) mRNA significantly decreased at a higher WHO grade of glioma. Differences among group means were evaluated by one-way ANOVA test (**p < 0.01, ***p < 0.001). High variability in 5-hmC abundance across glioblastoma (Grade IV-low5-hmC and Grade IV-high5-hmC) was not linked with levels of TET1 (B), TET2 (D), TET3 (F) transcripts.
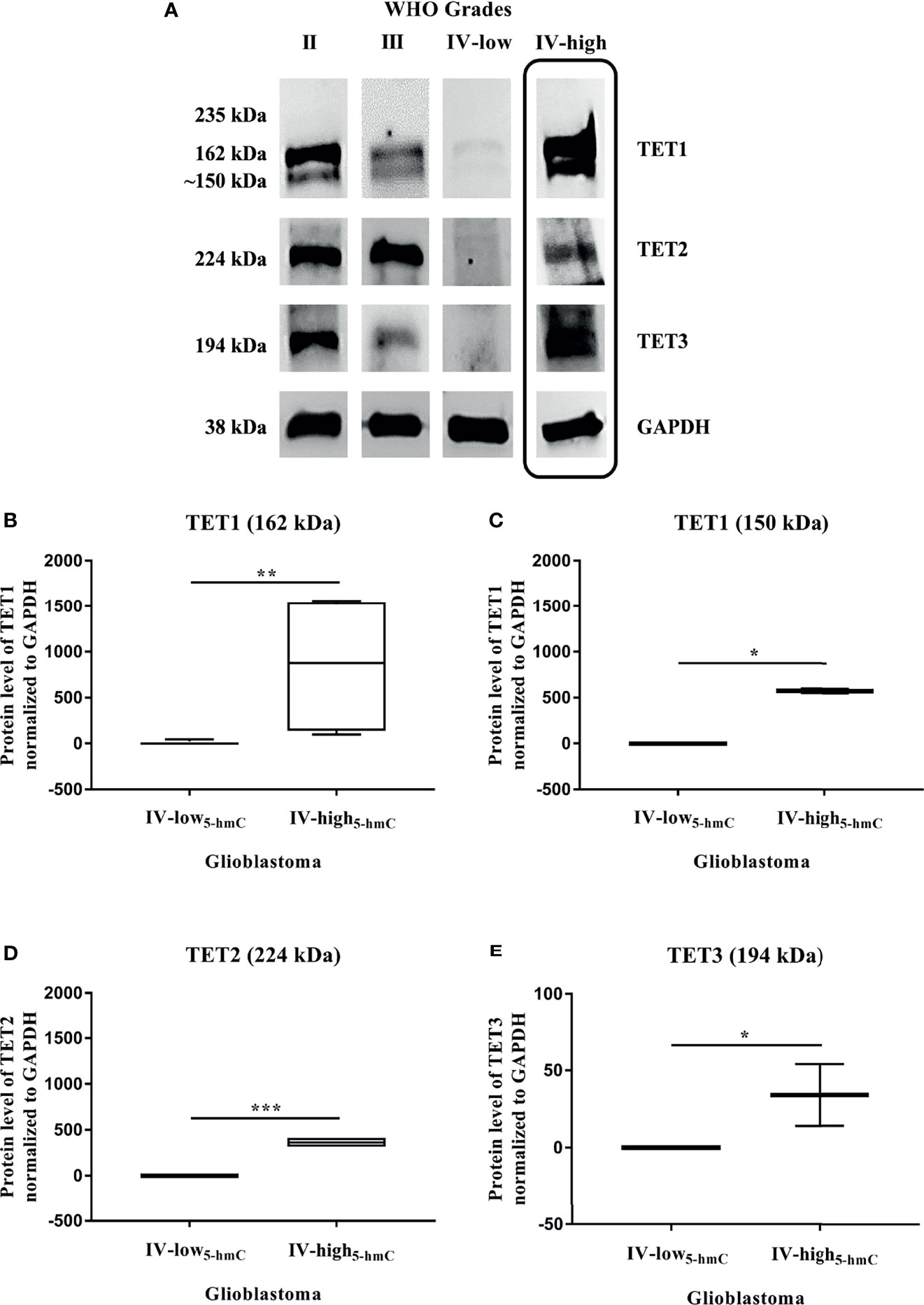
Figure 3 TET proteins expression in gliomas. Western blot analysis of TET proteins (A–E). Exemplary immunoblots of TET1, TET2, and TET3 proteins pictured loss of their expression across glioma stages, except some Grade IV samples (last column). Expression levels of these proteins were normalized with GAPDH (A). High variability in protein levels of TET1 (B, C), TET2 (D) and TET3 (E) correlated with 5-hmC abundance across glioblastoma (Grade IV-low5-hmC and Grade IV-high5-hmC). Differences between two groups were evaluated by Mann-Whitney U test (*p < 0.05, **p < 0.01, ***p < 0.001).
TET Transcripts Profiling in Plasma Samples
The prospect of distinguishing the aberrant DNA hydroxymethylation in the blood of glioma patients may indicate a powerful tool for early cancer detection or monitoring its progress. To explore the potential diagnostic features of TET transcripts profiling, we performed the quantitative real-time PCR on 34 plasma samples obtained from patients with Grade II or III or IV gliomas, and 5 healthy controls. Expression of TET genes was observed in the majority of examined samples, followed by specific numbers for TET1 (control: 80% vs. Grade: II-83%, III-57%, IV-70%), TET2 (control: 80% vs. Grade: II-83%, III-71%, IV-91%) and TET3 (control: 80% vs. Grade: II-50%, III-86%, IV-91%) transcript. Our results confirmed that the employed method was sensitive to low-input mRNA presented in plasma samples. The further analysis evaluated the relative mRNA levels of TET genes in plasma samples obtaining from patients with different WHO grades gliomas and compared them to healthy controls (Figures 4A–C). The results displayed various profiles for each transcript. While TET1 characterized slightly lower expression than control, TET3 was similar to control values. The expression of both transcripts was unaffected by WHO grading (Figures 4A, C). Whereas, the level of TET2 was significantly increased in plasma samples derived from patients with Grade II gliomas compared to controls (p < 0.01, Figure 4B). Validation of our preliminary results in a larger population of patients is needed to evaluate their use as potential biomarkers for early-stage gliomas diagnostics.
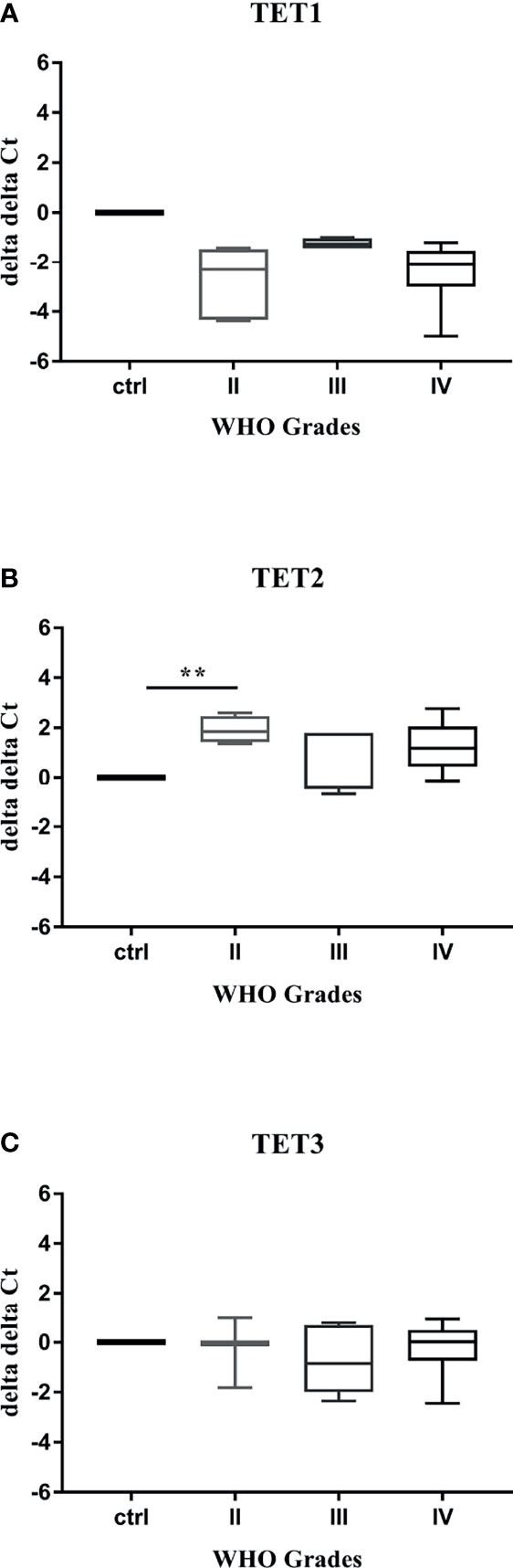
Figure 4 TET transcripts profiling in plasma samples. qRT–PCR analysis of TET mRNA (A–C). Levels of TET1 (A), TET2 (B), and TET3 (C) transcripts were variously regulated in plasma samples obtained from patients with gliomas. Statistical analysis performed using non-parametric Kruskal-Wallis test (**p < 0.01).
Discussion
Currently, the molecular landscape of brain tumors is described by epigenetic mechanisms include DNA methylation and hydroxymethylation (10, 11), histone modifications (12, 13), nucleosome remodeling (14, 15) and RNA-mediated silencing (16–18), that may clarify their etiologic evolution. Recently rediscovered, oxidized form of 5-methylcytosine (5-hmC) may act as a transient intermediate in the process of 5-mC demethylation or may epigenetically mark the cellular state itself with different biological roles.
In the present study, we characterize the epigenetic profile of DNA hydroxymethylation in human gliomas. Decreased level of 5-hmC was observed in glioma patients compared to healthy controls (33–35), but also it was related to glioma grades (10, 23–26). Our results confirm that the loss of 5-hmC is a hallmark of high-grade gliomas. However, we documented a significant increase of global 5-hmC abundance in almost a third of Grades IV GBM. This is one of the few findings that demonstrated heterogeneity in 5-hmC expression across the bulk of the glioblastoma (10, 24). According to recent research, the intra-tumoral diversity of 5-hmC levels among single GBM cells, that represent the proliferative, stem-like and tumorigenic states, could clarify unexpected results (36). But, dysregulation of TET enzymes function may be a possible biological explanation for the observed variability as well. The influence of TETs, including their genetic alterations and subcellular localization, on 5-hmC status in glioma cells, was described by several studies (23–25, 37, 38). Recently, epigenetic repression of histone marks (H4K16ac, H3K4me3, H3K9ac, H3K36me3, H3K4me1, H3K27ac) in TET3 gene has been postulated as a driver of glioblastoma development via genome-wide alteration of 5-hmC (39). Generally, it is believed that the decline in TET genes expression causes a widespread reduction of 5-hmC and poorer prognosis in glioma patients. We found that TET1, TET2, TET3 mRNA, and 5-hmC levels were decreased during glioma grades. However, similarly to Jin and Glowacka results (40, 41), there was no correlation between expression of TET transcripts and variability in 5-hmC abundance across the bulk of the glioblastoma. To define the complete impact of TETs on changes in DNA hydroxymethylation, we evaluated their protein levels in the same samples. Proteins produced by the TET2 and TET3 isoforms were expressed as expected bands at 224 kDa and 194 kDa. Instead of the 235 kDa canonical TET1 protein, we found two short isoforms (162 kDa and ~150 kDa). According to previous reports, they are exclusively activated from an alternate promoter in somatic cancer cells (42, 43). The levels of TET proteins, just as TET transcripts, were negatively associated with high-grade gliomas. However, Grade IV GBM samples were more variable in this field. Significant heterogeneity in the expression of TET2 protein in glioblastoma was also noted by Briand (44). We furthermore pointed out the relation between variable levels of TET proteins and 5-hmC abundance across glioblastoma. Our findings suggest that the regulation of TET transcription and translation can be made in a different way. For example, this observed imbalance may be a result of different actions of transcription factors, RNA binding proteins, miRNAs targeting mRNA or post-translational modifications (like phosphorylation, acetylation, glycosylation, etc.). Based on the patient-derived glioma stem cells (GSCs) model, the transcription factor (SOX2)-oncomiR (miR-10b-5p)-TET2 axis was identified, which plays an important role in promoting GBM oncogenesis (45). However, it is one of many potential mechanisms involved in glioblastoma growth.
In summary, we demonstrated that expression patterns of TET proteins and the 5-hmC abundance are changed in Grade IV GBM, but the molecular mechanism of this process still needs to be clarified.
Recently, many studies on gliomas indicated the presence of circulating cell-free coding and non-coding nucleic acids in blood or other biofluid samples (46–48). Therefore, liquid biopsy has become a new diagnostic tool that may establish and track the stage of cancer with particular biomarkers. Cai’s group, for example, has developed a noninvasive 5-hmC detection method in circulating cell-free DNA of glioma patients, which was able to distinguish the difference between GBM and lower-grade gliomas regardless of IDH1 mutation status (49). Here, we explore the potential diagnostic features of TET transcripts in plasma samples obtained from patients with gliomas. All three TET transcripts were detected in plasma, but their profiles differed from those in solid tissue. We showed that the plasma relative mRNA level for TET1 was decreased in every stage of glioma, while the TET3 level remained unchanged. The most promising results were provided by TET2 gene that was significantly increased in Grade II glioma. Validation of our preliminary results in a larger population of patients is needed to evaluate their use as potential biomarkers for glioma diagnostics.
To conclude, we found that global abundance of 5-hmC was negatively correlated with glioma WHO grades and variable across the bulk of the glioblastoma. It was followed by various TET proteins patterns in solid tumor tissues. In contrast, profiles of TET transcripts in plasma samples displayed its heterogeneity. However, significant overexpression of TET2 in Grade II gliomas might offer a new tool for effective diagnosis of lower-grade glioma patients. Our findings provide novel information about the potential role of TET epigenetic regulation in human gliomas, with particular reference to glioblastoma.
Data Availability Statement
All data generated during this study are included in the article. Further inquiries can be directed to the corresponding author.
Ethics Statement
The study was conformed to the standards set by the Declaration of Helsinki and approved by the ethics committee of the Centre of Postgraduate Medical Education (code: 34/PB/2017). Written informed consent was obtained from participants prior to specimen collection.
Author Contributions
MG designed all experiments, interpreted the results, and wrote the manuscript. AB-P, GL performed the experiments, analyzed the data. KŻ-B performed part of molecular analysis. AS-S, MC contributed to sample collection and registered clinical information. MS and MR managed sample collection; critically commented on the manuscript. All authors read and approved the final version.
Funding
This study was supported by a statutory grant 501-1-027-03-19 from the Centre of Postgraduate Medical Education in Warsaw.
Conflict of Interest
The authors declare that the research was conducted in the absence of any commercial or financial relationships that could be construed as a potential conflict of interest.
Publisher’s Note
All claims expressed in this article are solely those of the authors and do not necessarily represent those of their affiliated organizations, or those of the publisher, the editors and the reviewers. Any product that may be evaluated in this article, or claim that may be made by its manufacturer, is not guaranteed or endorsed by the publisher.
Acknowledgments
The authors thank Mrs. Monika Kubiak for help with the management of clinical information.
Supplementary Material
The Supplementary Material for this article can be found online at: https://www.frontiersin.org/articles/10.3389/fonc.2022.621460/full#supplementary-material
References
1. Ostrom QT, Cioffi G, Gittleman H, Patil N, Waite K, Kruchko C, et al. CBTRUS Statistical Report: Primary Brain and Other Central Nervous System Tumors Diagnosed in the United States in 2012-2016. Neuro Oncol (2019) 21(Suppl 5):v1–v100. doi: 10.1093/neuonc/noz150
2. Louis DN, Perry A, Reifenberger G, von Deimling A, Figarella-Branger D, Cavenee WK, et al. The 2016 World Health Organization Classification of Tumors of the Central Nervous System: A Summary. Acta Neuropathol (2016) 131:803–20. doi: 10.1007/s00401-016-1545-1
3. Sottoriva A, Spiteri I, Piccirillo SGM, Touloumis A, Collins VP, Marioni JC, et al. Intratumor Heterogeneity in Human Glioblastoma Reflects Cancer Evolutionary Dynamics. Proc Natl Acad Sci USA (2013) 110:4009–14. doi: 10.1073/pnas.1219747110
4. Nomura M, Mukasa A, Nagae G, Yamamoto S, Tatsuno K, Ueda H, et al. Distinct Molecular Profile of Diffuse Cerebellar Gliomas. Acta Neuropathol (2017) 134:941–56. doi: 10.1007/s00401-017-1771-1
5. Perrech M, Dreher L, Rohn G, Stavrinou P, Krischek B, Toliat M, et al. Qualitative and Quantitative Analysis of IDH1 Mutation in Progressive Gliomas by Allele-Specific qPCR and Western Blot Analysis. Technol Cancer Res Treat (2019) 18:1533033819828396. doi: 10.1177/1533033819828396
6. Marko NF, Toms SA, Barnett GH, Weil R. Genomic Expression Patterns Distinguish Long-Term From Short-Term Glioblastoma Survivors: A Preliminary Feasibility Study. Genomics (2008) 91:395–406. doi: 10.1016/j.ygeno.2008.01.002
7. Bernal Rubio YL, Gonzalez-Reymundez A, Wu KHH, Griguer CE, Steibel JP, de Los Campos G, et al. Whole-Genome Multi-Omic Study of Survival in Patients With Glioblastoma Multiforme. G3 (Bethesda) (2018) 8:3627–36. doi: 10.1534/g3.118.200391
8. Verhaak RGW, Hoadley KA, Purdom E, Wang V, Qi Y, Wilkerson MD, et al. Integrated Genomic Analysis Identifies Clinically Relevant Subtypes of Glioblastoma Characterized by Abnormalities in PDGFRA, IDH1, EGFR, and NF1. Cancer Cell (2010) 17:98–110. doi: 10.1016/j.ccr.2009.12.020
9. Aldape K, Zadeh G, Mansouri S, Reifenberger G, von Deimling A. Glioblastoma: Pathology, Molecular Mechanisms and Markers. Acta Neuropathol (2015) 129:829–48. doi: 10.1007/s00401-015-1432-1
10. Orr BA, Haffner MC, Nelson WG, Yegnasubramanian S, Eberhart CG. Decreased 5-Hydroxymethylcytosine is Associated With Neural Progenitor Phenotype in Normal Brain and Shorter Survival in Malignant Glioma. PloS One (2012) 7:e41036. doi: 10.1371/journal.pone.0041036
11. Ma J, Hou X, Li M, Ren H, Fang S, Wang X, et al. Genome-Wide Methylation Profiling Reveals New Biomarkers for Prognosis Prediction of Glioblastoma. J Cancer Res Ther (2015) 11(Suppl 2):C212–5. doi: 10.4103/0973-1482.168188
12. Vettermann FJ, Felsberg J, Reifenberger G, Hasselblatt M, Forbrig R, Berding G, et al. Characterization of Diffuse Gliomas With Histone H3-G34 Mutation by MRI and Dynamic 18F-FET PET. Clin Nucl Med (2018) 43:895–8. doi: 10.1097/RLU.0000000000002300
13. Castel D, Kergrohen T, Tauziede-Espariat A, Mackay A, Ghermaoui S, Lechapt E, et al. Histone H3 Wild-Type DIPG/DMG Overexpressing EZHIP Extend the Spectrum Diffuse Midline Gliomas With PRC2 Inhibition Beyond H3-K27M Mutation. Acta Neuropathol (2020) 139:1109–13. doi: 10.1007/s00401-020-02142-w
14. Diehl KL, Ge EJ, Weinberg DN, Jani KS, Allis CD, Muir TW. PRC2 Engages a Bivalent H3K27M-H3K27me3 Dinucleosome Inhibitor. Proc Natl Acad Sci USA (2019) 116:22152–7. doi: 10.1073/pnas.1911775116
15. Lu VM, Power EA, Zhang L, Daniels DJ. Unlocking the Translational Potential of Circulating Nucleosomes for Liquid Biopsy in Diffuse Intrinsic Pontine Glioma. biomark Med (2019) 13:597–600. doi: 10.2217/bmm-2019-0139
16. Cui H, Mu Y, Yu L, Xi YG, Matthiesen R, Su X, et al. Methylation of the miR-126 Gene Associated With Glioma Progression. Fam Cancer (2016) 15:317–24. doi: 10.1007/s10689-015-9846-4
17. Xue L, Wang Y, Yue S, Zhang J. The Expression of miRNA-221 and miRNA-222 in Gliomas Patients and Their Prognosis. Neurol Sci (2017) 38:67–73. doi: 10.1007/s10072-016-2710-y
18. Liu ZQ, Ren JJ, Zhao JL, Zang J, Long QF, Du JJ, et al. MicroRNA-144 Represses Gliomas Progression and Elevates Susceptibility to Temozolomide by Targeting CAV2 and FGF7. Sci Rep (2020) 10:4155. doi: 10.1038/s41598-020-60218-9
19. Hegi ME, Diserens AC, Gorlia T, Hamou MF, de Tribolet N, Weller M, et al. MGMT Gene Silencing and Benefit From Temozolomide in Glioblastoma. N Engl J Med (2005) 352:997–1003. doi: 10.1056/NEJMoa043331
20. Capper D, Jones DTW, Sill M, Hovestadt V, Schrimpf D, Sturm D, et al. DNA Methylation-Based Classification of Central Nervous System Tumours. Nature (2018) 555:469–74. doi: 10.1038/nature26000
21. Zhang M, Lv X, Jiang Y, Li G, Qiao Q. Identification of Aberrantly Methylated Differentially Expressed Genes in Glioblastoma Multiforme and Their Association With Patient Survival. Exp Ther Med (2019) 18:2140–52. doi: 10.3892/etm.2019.7807
22. Ito S, D`Alessio AC, Taranova OV, Hong K, Sowers LC, Zhang Y. Role of Tet Proteins in 5mc to 5hmc Conversion, ES-Cell Self-Renewal and Inner Cell Mass Specification. Nature (2010) 466:1129–33. doi: 10.1038/nature09303
23. Muller T, Gessi M, Waha A, Isselstein LJ, Luxen D, Freihoff D, et al. Nuclear Exclusion of TET1 Is Associated With Loss of 5-Hydroxymethylcytosine in IDH1 Wild-Type Gliomas. Am J Pathol (2012) 181:675–83. doi: 10.1016/j.ajpath.2012.04.017
24. Takai H, Masuda K, Sato T, Sakaguchi Y, Suzuki T, Suzuki T, et al. 5-Hydroxymethylcytosine Plays a Critical Role in Glioblastomagenesis by Recruiting the CHTOP-Methylosome Complex. Cell Rep (2014) 9:48–60. doi: 10.1016/j.celrep.2014.08.071
25. Kraus TFJ, Kolck G, Greiner A, Schierl K, Guibourt V, Kretzschmar HA. Loss of 5-Hydroxymethylcytosine and Intratumoral Heterogeneity as an Epigenomic Hallmark of Glioblastoma. Tumour Biol (2015) 36:8439–46. doi: 10.1007/s13277-015-3606-9
26. Zhang F, Liu Y, Zhang Z, Li J, Wan Y, Zhang L, et al. 5-Hydroxymethylcytosine Loss Is Associated With Poor Prognosis for Patients With WHO Grade II Diffuse Astrocytomas. Sci Rep (2016) 6:20882. doi: 10.1038/srep20882
27. Chomczynski P, Sacchi N. Single-Step Method of RNA Isolation by Acid Guanidinium Thiocyanate-Phenol-Chloroform Extraction. Anal Biochem (1987) 162:156–9. doi: 10.1006/abio.1987.9999
28. Grube S, Gottig T, Freitag D, Ewald C, Kalff R, Walter J. Selection of Suitable Reference Genes for Expression Analysis in Human Glioma Using RT-qPCR. J Neurooncol (2015) 123:35–42. doi: 10.1007/s11060-015-1772-7
29. Ericsson C, Peredo I, Nister M. Optimized Protein Extraction From Cryopreserved Brain Tissue Samples. Acta Oncol (2007) 46:10–20. doi: 10.1080/02841860600847061
30. Kudo Y, Tateishi K, Yamamoto K, Yamamoto S, Asaoka Y, Ijichi H, et al. Loss of 5-Hydroxymethylcytosine is Accompanied With Malignant Cellular Transformation. Cancer Sci (2012) 103:670–6. doi: 10.1111/j.1349-7006.2012.02213.x
31. Yang H, Liu Y, Bai F, Zhang JY, Ma SH, Liu J, et al. Tumor Development is Associated With Decrease of TET Gene Expression and 5-Methylcytosine Hydroxylation. Oncogene (2013) 32:663–9. doi: 10.1038/onc.2012.67
32. Chen Z, Shi X, Guo L, Li Y, Luo M, He J. Decreased 5-Hydroxymethylcytosine Levels Correlate With Cancer Progression and Poor Survival: A Systematic Review and Meta-Analysis. Oncotarget (2017) 8:1944–52. doi: 10.18632/oncotarget.13719
33. Johnson KC, Houseman EA, King JE, von Herrmann KM, Fadul CE, Christensen BC. 5-Hydroxymethylcytosine Localizes to Enhancer Elements and is Associated With Survival in Glioblastoma Patients. Nat Commun (2016) 7:13177. doi: 10.1038/ncomms13177
34. Fernandez AF, Bayon GF, Sierra MI, Urdinguio RG, Torano EG, Garcia MG, et al. Loss of 5hmc Identifies a New Type of Aberrant DNA Hypermethylation in Glioma. Hum Mol Genet (2018) 27:3046–59. doi: 10.1093/hmg/ddy214
35. Fu R, Ding Y, Luo J, Huang KM, Tang XJ, Li DS, et al. Ten-Eleven Translocation 1 Regulates Methylation of Autophagy-Related Genes in Human Glioma. Neuroreport (2018) 29:731–8. doi: 10.1097/WNR.0000000000001024
36. El-Habr EA, Dubois LG, Burel-Vandenbos F, Bogeas A, Lipecka J, Turchi L, et al. A Driver for GABA Metabolism in Controlling Stem and Proliferative Cell State Through GHB Production in Glioma. Acta Neuropathol (2017) 133:645–60. doi: 10.1007/s00401-016-1659-5
37. Putiri EL, Tiedemann RL, Thompson JJ, Liu C, Ho T, Choi JH, et al. Distinct and Overlapping Control of 5-Methylcytosine and 5-Hydroxymethylcytosine by the TET Proteins in Human Cancer Cells. Genome Biol (2014) 15:R81. doi: 10.1186/gb-2014-15-6-r81
38. Garcia MG, Carella A, Urdinguio RG, Bayon GF, Lopez V, Tejedor JR, et al. Epigenetic Dysregulation of TET2 in Human Glioblastoma. Oncotarget (2018) 9:25922–34. doi: 10.18632/oncotarget.25406
39. Carella A, Tejedor JR, Garcia MG, Urdinguio RG, Bayon GF, Sierra M, et al. Epigenetic Downregulation of TET3 Reduces Genome-Wide 5hmc Levels and Promotes Glioblastoma Tumorigenesis. Int J Cancer (2020) 146:373–87. doi: 10.1002/ijc.32520
40. Jin SG, Jiang Y, Qiu R, Rauch TA, Wang Y, Schackert G, et al. 5-Hydroxymethylcytosine Is Strongly Depleted in Human Cancers But its Levels Do Not Correlate With IDH1 Mutations. Cancer Res (2011) 71:7360–5. doi: 10.1158/0008-5472.CAN-11-2023
41. Glowacka WK, Jain H, Okura M, Maimaitiming A, Mamatjan Y, Nejad R, et al. 5-Hydroxymethylcytosine Preferentially Targets Genes Upregulated in Isocitrate Dehydrogenase 1 Mutant High-Grade Glioma. Acta Neuropathol (2018) 135:617–34. doi: 10.1007/s00401-018-1821-3
42. Zhang W, Xia W, Wang Q, Towers AJ, Chen J, Gao R, et al. Isoform Switch of TET1 Regulates DNA Demethylation and Mouse Development. Mol Cell (2016) 64:1062–73. doi: 10.1016/j.molcel.2016.10.030
43. Good CR, Madzo J, Patel B, Maegawa S, Engel N, Jelinek J, et al. A Novel Isoform of TET1 That Lacks a CXXC Domain Is Overexpressed in Cancer. Nucleic Acids Res (2017) 45:8269–81. doi: 10.1093/nar/gkx435
44. Briand J, Nadaradjane A, Vallette FM, Cartron P-F. The TET2 Expression Level Correlates With a Short Relapse Time in Glioblastoma Multiforme. J Clin Epigenet (2018) 4:12. doi: 10.21767/2472-1158.100097
45. Lopez-Bertoni H, Johnson A, Rui Y, Lal B, Sall S, Malloy M, et al. Sox2 Induces Glioblastoma Cell Stemness and Tumor Propagation by Repressing TET2 and Deregulating 5hmc and 5mc DNA Modifications. Signal Transduct Target Ther (2022) 7:37. doi: 10.1038/s41392-021-00857-0
46. Schwaederle M, Husain H, Fanta PT, Piccioni DE, Kesari S, Schwab RB, et al. Detection Rate of Actionable Mutations in Diverse Cancers Using a Biopsy-Free (Blood) Circulating Tumor Cell DNA Assay. Oncotarget (2016) 7:9707–17. doi: 10.18632/oncotarget.7110
47. Mouliere F, Mair R, Chandrananda D, Marass F, Smith CG, Su J, et al. Detection of Cell-Free DNA Fragmentation and Copy Number Alterations in Cerebrospinal Fluid From Glioma Patients. EMBO Mol Med (2018) 10:e9323. doi: 10.15252/emmm.201809323
48. Piccioni DE, Achrol AS, Kiedrowski LA, Banks KC, Boucher N, Barkhoudarian G, et al. Analysis of Cell-Free Circulating Tumor DNA in 419 Patients With Glioblastoma and Other Primary Brain Tumors. CNS Oncol (2019) 8:CNS34. doi: 10.2217/cns-2018-0015
Keywords: epigenetics, 5-hydroxymethylcytosine, ten-eleven translocation enzymes, brain tumors, glioblastoma
Citation: Brągiel-Pieczonka A, Lipka G, Stapińska-Syniec A, Czyżewski M, Żybura-Broda K, Sobstyl M, Rylski M and Grabiec M (2022) The Profiles of Tet-Mediated DNA Hydroxymethylation in Human Gliomas. Front. Oncol. 12:621460. doi: 10.3389/fonc.2022.621460
Received: 26 October 2020; Accepted: 17 March 2022;
Published: 14 April 2022.
Edited by:
Kamalakannan Palanichamy, The Ohio State University, United StatesReviewed by:
Joerg Robert Leheste, New York Institute of Technology, United StatesRasime Kalkan, Near East University, Cyprus
Copyright © 2022 Brągiel-Pieczonka, Lipka, Stapińska-Syniec, Czyżewski, Żybura-Broda, Sobstyl, Rylski and Grabiec. This is an open-access article distributed under the terms of the Creative Commons Attribution License (CC BY). The use, distribution or reproduction in other forums is permitted, provided the original author(s) and the copyright owner(s) are credited and that the original publication in this journal is cited, in accordance with accepted academic practice. No use, distribution or reproduction is permitted which does not comply with these terms.
*Correspondence: Marta Grabiec, bWFydGEuZ3JhYmllY0BjbWtwLmVkdS5wbA==
†These authors have contributed equally to this work