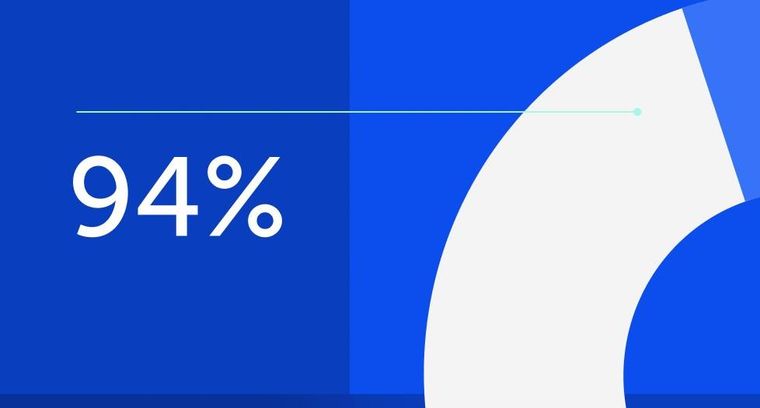
94% of researchers rate our articles as excellent or good
Learn more about the work of our research integrity team to safeguard the quality of each article we publish.
Find out more
REVIEW article
Front. Oncol., 16 January 2023
Sec. Molecular and Cellular Oncology
Volume 12 - 2022 | https://doi.org/10.3389/fonc.2022.1118101
This article is part of the Research TopicExtracellular Vesicles in Gastrointestinal CancersView all 5 articles
As phospholipid extracellular vesicles (EVs) secreted by various cells, exosomes contain non-coding RNA (ncRNA), mRNA, DNA fragments, lipids, and proteins, which are essential for intercellular communication. Several types of cells can secrete exosomes that contribute to cancer initiation and progression. Cancer cells and the immune microenvironment interact and restrict each other. Tumor-derived exosomes (TDEs) have become essential players in this balance because they carry information from the original cancer cells and express complexes of MHC class I/II epitopes and costimulatory molecules. In the present study, we aimed to identify potential targets for exosome therapy by examining the specific expression and mechanism of exosomes derived from cancer cells. We introduced TDEs and explored their role in different tumor immune microenvironment (TIME), with a particular emphasis on gastrointestinal cancers, before briefly describing the therapeutic strategies of exosomes in cancer immune-related therapy.
The tumorigenesis of cancer can be attributed to the interaction between cells and the tumor immune microenvironment. Normal cells undergo genetic changes upon chemical, biological, and physical stimulations, leading to cell growth and differentiation from an orderly and controllable state to uncontrolled monoclonal proliferation. Meanwhile, the immune system evades (1), stromal cell niche transformation (2), angiogenesis (3), and other changes in the tumor immune microenvironment (TIME), providing permissive conditions for the invasion and development of cancer cells and promoting the occurrence and development of cancers (4). At present, the mainstream regimens of cancer treatment include surgery, radiotherapy, and chemotherapy. However, the treatment effect remains poor due to the lack of specificity, and the side effects are significant, which has been confirmed in related studies on breast cancer (BC) (5, 6), colorectal cancer (CRC) (7–9), lung cancer (10), blood cancer (11, 12), and so on. With the in-depth study on the mechanism of cancerogenesis, targeted therapy provides a feasible scheme to overcome the limitations of traditional treatment approaches (13). Nucleic acids and proteins involved in cancer development both within the tumor cell and in the TIME can be potential targets for targeted therapy (14). At present, targeted therapies targeting human epidermal growth factor receptor 2 (HER-2), vascular endothelial growth factor (VEGF) (15), and tyrosine kinase are widely used in gastrointestinal cancers (16) and hematological cancers (17). However, targeting only these targets within cancer cells sometimes leads to the development of resistance and relapse (18–22). The TIME includes immune cells, fibroblasts, peripheral blood vessels, bone marrow (BM)-derived inflammatory cells, various signaling molecules, and extracellular matrix components. These components interact and communicate with each other to maintain the balance between procancer and anticancer mediators. However, cancers can shape the TIME into an immunosuppressive state conducive to tumor growth and disrupt this balance. Therefore, targeting the TIME, rebalancing the TIME, and restoring the immune self-inspection of the body itself can be considered a more comprehensive targeted therapy strategy with fewer side effects (23, 24).
As a therapeutic strategy, cancer immunotherapy aims to reshape the TIME, restore the cancer-killing function of immune cells, and enable cancer cells to be killed by the autoimmune system (25). Based on the understanding of cancer immune escape, several immunotherapies have been developed to inhibit cancer growth. Ipilimumab, an immune checkpoint cytotoxic T lymphocyte antigen 4 (CTLA-4) inhibitor, has successfully allowed 21% of patients to survive for more than 10 years (26). Programmed death 1 (PD-1) drugs help 30% of patients with the terminal disease survive for more than 5 years (27). An autologous CD19-targeting chimeric antigen receptor CAR T-cell product is approved for treating R/R B-cell acute lymphoblastic leukemia (ALL) and lymphoma (28). These immunotherapy methods provide exciting new options for clinical practice. However, cancer drug resistance and low efficacy suggest that we still have more content to explore for cancer immunotherapy.
As phospholipid extracellular vesicles (EVs) of approximately 40-100 nm in size, exosomes are secreted by almost all cells, which can contain microRNA (miRNA), mRNA, DNA fragments, lipids, and proteins and are essential substances for intercellular communication (29, 30) (Figure 1). These vesicles include some conserved proteins, such as tetraspanins (CD9, CD63, CD81, and CD82), cell membrane fusion and transport proteins (Rab2, Rab7, flotillin, and annexin), heat shock proteins (HSP70, HSP90 Hsp60, and Hsp20), cytoskeleton proteins (β-actin and tubulin), and synthesis proteins (Alix and Tsg101). Among them, CD63/TSG101 is the most commonly used exosome marker (31). Exosomes carry their contents throughout body fluids (blood, saliva, urine, and feces) and interact with target cells through direct fusion (32) or endocytosis (33, 34) to transfer the information to target cells and exert their effects. Studies have shown that tumor-derived exosomes (TDEs) can act on target cells to promote cancer angiogenesis (35, 36), facilitate the transformation of cancer stromal cells to a pro-cancer phenotype, and change the cancer niche (2, 37) to urge cancer initiation and progression. In clinical data statistics, the molecular content of exosomes has an excellent prognostic or predictive value in cancer progression and treatment response of cancer patients (38). Exosomes also include specific biomarkers of their cellular origin. Examples include costimulatory molecules derived from immune cells, such as B cells and dendritic cells (DCs) (39–41), and exosomes derived from cancer cells, which contain cancer-specific antigens (42), playing a vital role in cancer immune presentation and cancer-specific immune responses. Therefore, the research and application of exosomes in immunotherapy have attracted much attention (43). It was shown 20 years ago that vaccination with DCexos, derived from BM-derived DCs, has better anticancer efficacy than vaccination with DCs, supporting the development of cancer vaccines (44). Inhibition of exosomal PD-1 reduces resistance to PD-1 therapy (45). Here, we summarized the current knowledge regarding exosomes in cancers and the TIME, focusing on gastrointestinal cancers, and discussed their potential use in immunotherapy. Using this strategy, we can approach the enhancement of cancer immunotherapy from a more scientific perspective.
Figure 1 Exosomes are phospholipid extracellular vesicles that range in size from 40nm to 100nm and contain ncRNA, mRNA, DNA fragments, lipids, and proteins, including some conserved proteins and cell-specific biomarkers. It is essential for intercellular communication, transport, and flow in body fluids.
Various cells can secrete exosomes. Han et al. have found that exosomes containing miR-145 secreted by tumor-associated stroma in pancreatic ductal adenocarcinoma can act on adjacent pancreatic ductal adenocarcinoma cells to inhibit cancer cell growth (46). Cancer-associated fibroblasts (CAFs) are the main components of the TIME. Studies have found that exosomes secreted by CAFs transmit information, such as miR-181D-5P, miR-21, miR-143, and miR-378e, to BC cells, which promote stem cell characteristics, epithelial-mesenchymal transition (EMT) phenotype, and non-anchoring cell growth of BC cells to encourage the progression of cancer (47–49). CAFs can also secrete exosomes carrying SNHG17 to mediate osteosarcoma proliferation and metastasis (50). Adipocytes also secrete exosomes, leading to increased cancer cell migration and invasion (51). Mesenchymal stem cells (MSCs) also exist in the TIME and have immunomodulatory properties. Mao et al. have shown that BM-MSCs from p53-deficient mice (p53 mBM-MSCs) can regulate the Wnt/β-catenin pathway and deliver UBr2 to target cells, thereby promoting the growth and metastasis of gastric cancer (GC) (52). Ma et al. have found that exosomes derived from BM-MSCs transfected with miR-221 significantly promote the proliferation, migration, invasion, and adhesion of GC BGC-823 and SGC-7901 cells (53). Human BM-MSC exosomal miR-375 inhibits glioma cell progression through solute carrier family 31 member 1(SLC31A1)inhibition (54).
Immune cell-derived exosomes in the tumor microenvironment also play an important role in the occurrence and development of cancers. As early as 2012, Luana et al. have proposed an important role of natural killer (NK) cell-derived exosomes in immune surveillance and homeostasis (55). Aladin and colleagues showed that NK cell-derived exosomes eliminated leukemia cells isolated from patients with leukemia (56). Zitvogel’s earlier study showed that DC cell-derived exosomes were as effective as DC cells in inducing tumor immune responses, which also provided theoretical support for cancer vaccine research (44). Romagnoli et al. indicated that breast cancer cells treated with DC cell-derived exosomes were more effective in stimulating T cell responses than breast cancer cells without exosome treatment (57). Cai and colleagues showed that CD8+ T cell-derived Exos can promote tumor cell invasion and lung metastasis through the Fas pathway (58).Xie et al. have demonstrated that Natural CD8+25+ regulatory T cell-secreted exosomes can inhibit CD8+ T cell responses and antitumor immunity (59). Wang et al. have shown that exosomes derived from Tumor-associated macrophages up-regulate miR-192-5p to promote endometrial cancer cells apoptosis and effectively suppress the progression of endometrial cancer (60). Exosomes secreted by polymorphonuclear (PMN) myeloid-derived suppressor cells (MDSCs), which are immunomodulatory cells present in the TIME, significantly inhibit NK cell-mediated anticancer activity, suggesting that the detection of PMN-MDSC content may have prognostic value (61). miR-326 in M1 macrophage exosomes promotes apoptosis of liver cancer cells and inhibits cancer proliferation and migration by downregulating the expression of NF-κB in liver cancer (62).
Cancers interact with various cells in their TIME. In the above review, we briefly discuss the role of non-tumor derived exosomes in tumors, next, we focused on the role of TDEs.
TDEs function in both autocrine and paracrine ways in the TIME. They can act on the cancer cells themselves or on the surrounding cells and tissues to affect cancer development, which has been confirmed in various cancers (Table 1). Furthermore, TDEs can affect other non-cancer cells and participate in the transfer of metastatic capacity. For example, exosomes released by malignant BC cells are taken up by less malignant cancer cells located within the same cancer, thereby promoting the migration and metastatic potential of low-grade malignant cancer cells (100). In addition, TDEs can act on immune cells in the microenvironment to regulate cancer immunity and affect the balance between cancer promotion and cancer suppression in the TIME.
Gastrointestinal cancer is the fourth most deadly cancer in the world (120). Despite great progress in surgery combined with radiotherapy and chemotherapy, its early diagnosis and late prognosis are still noteworthy (121). Tumor-derived exosomes are early participants in the tumor’s participation in the immunosuppressive microenvironment, which in turn affects the survival and treatment response of gastric cancer patients (122). Exploring the mechanism of gastrointestinal cancer-derived exosomes on tumor immune microenvironment will provide more targets for diagnosis and treatment.
Gastrointestinal TDEs play a vital role in gastrointestinal cancerogenesis. TDEs can act on cancer cells to promote cancer proliferation, metastasis, and invasion by transferring information, such as proteins, circular RNAs (circRNA), miRNA, lncRNA (Figure 2). TEDs delivery proteins affect GC cells.Zhao et al. have reported that lysine-specific demethylase 1 (LSD1) in gastric TDEs can act on receptor GC cells to promote cancer stemness (63). Sun and colleagues have revealed that ADAM17 in TDEs can cleave the E-cadherin junction to promote cancer cell metastasis (64). TEDs delivery circRNA affect GC cells. Overall, circRNAs usually affect the expressions of downstream genes by acting as miRNA sponges. Li et al. have suggested that CircRNA NIMA-related kinase 9 (circNEK9; hsa_circ_0032683) in gastric TDEs promotes the proliferation, migration, and invasion of GC cells through the miR-409-3p/MAP7 axis (65). Lu et al. have shown that the expression of circ-RanGAP1(hsa_circ_0063526) is high in GC exosomes and promotes the progression of GC through the miR-877-3p/VEGFA axis (66).Gao and coworkers have reported that circCOG2(hsa_circ_0016866) in the exosomes of colon cancer cells promotes CRC progression by sponging miR-1305 to promote the proliferation, migration, and invasion of CRC (67). TEDs delivery miRNA affect GC cells. Liu et al. have indicated that miR-501 in exosomes derived from GC promotes the proliferation, migration, and invasion of cancer cells through the subsequent inactivation of caspase-9/-3 and phosphorylation of Akt (68). Li and colleagues have revealed that miR-675-3p in exosomes binds to the 3ʹ untranslated region of CXXC4, downregulates the expression of CXXC4, and upregulates MAPK/PD-L1, thereby mediating immunosuppression and increasing immune escape (69). Liang et al. have demonstrated that in a coculture system of EVs and T cells, miR-1290 can upregulate programmed death ligand 1(PD-L1) through the Grhl2/ZEB1 pathway and promote the inhibitory effect of GC cells on T-cell activation (70). Liu et al. have shown that miR-106b-3p in exosomes acts on DLC-1 to promote EMT, cancer cell migration, and invasion (71). Sun et al. have pointed out that exosomes can deliver miR-335-5p to metastatic CRC cells, resulting in the EMT of cancer cells and promoting invasion and metastasis of CRC cells (72). TEDs delivery lncRNA affect GC cells.In a study by Zhang and collaborators, gastric TDEs promote the proliferation and migration of GC cells by transporting FOXM1 related long noncoding RNA (FRLnc1) (73). Xian et al. have shown that lncRNA KCNQ1OT1 contained in exosomes secreted by CRC can increase the expression of PD-L1 protein in cancer cells through the miR-30a-5p/USP22 pathway and mediate immune escape (74).
Figure 2 Exosomes’ role in gastrointestinal tumors. Cancer-derived exosomes can promote tumor proliferation, metastasis, and invasion by transferring information such as proteins, circRNA, miRNA, and lncRNA to tumor cells. It can also affect immune cells, causing an immunosuppressive environment and promoting tumor immune escape. Exosomes also affect other cells in the immune microenvironment and other tissue cells, directing them in a more tumor-friendly direction. And encourage tumor cells to spread to distant locations.
Gastrointestinal TDEs can also act on immune cells to induce an immunosuppressive environment and promote cancer proliferation and metastasis. As early as 2005, Huber et al. have shown that human CRC cells induce T-cell death by releasing proapoptotic microbubbles (123), suggesting that the role of exosomes in the TIME should not be underestimated. Liu et al. have suggested that GC cell exosomes change the gene expression and cytokine secretion level of CD8+ T cells to promote the formation of an immunosuppressive microenvironment (75). Li and colleagues have found that miR-135B-5p in GC exosomes reduces the survival rate of V-γ-9 V-δ-2T cells and induces cell apoptosis through the miR-135B-5p/SP1( specificity protein 1) axis. Reduced production of the cytotoxic cytokines interferon γ (IFN-γ) and tumor necrosis factor α (TNF-α) favors cancer progression (76). In addition, Zhang et al. have indicated that TDEs induce neutrophil autophagy through the HMGB1/TLR4/NF-κB signaling pathway (77). Shi et al. have shown that exosomal transport of HMGB1 activates STAT3, which upregulates PD-L1 expression on neutrophils at the genetic level and suppresses T-cell immunity (78). Hinata et al. have shown that exosomes from Epstein‐Barr virus-associated GC inhibit DC maturation (79). Takano et al. have reported that CRC serum exosomal miR-203 promotes CRC liver metastasis by enhancing M2 polarization (80). Ren and coworkers have reported that exosomes secreted by GC can carry miR-107 to the host MDSCs and induce their expansion and activation by targeting DICER1 and PTEN genes to generate an immunosuppressive microenvironment and promote cancer progression (81). Tang has demonstrated that high expression of exosomal TGF-β1 induces an increase in Tregs in GC-draining LNs, which is involved in cancer lymph node metastasis (82).
Gastrointestinal TDEs also affect other cells in the TIME, making them more suitable for cancer development. Zhang et al. have suggested that HSPC111 promotes the expression and secretion of CXCL5 by altering lipid metabolism in CAFs, inducing EMT, and promoting CRC cell migration through the CXCL5-CXCR2 axis (83). Huang and colleagues have found that miR-1246 is highly expressed in exosomes secreted by colon cancer cells, promotes fibroblast proliferation and M2 polarization through the Akt/mTOR/STAT3 axis, and reduces cancer size in vivo (84). Naito and colleagues have revealed that exosomes secreted by highly metastatic GC cells can differentiate fibroblast lines in the microenvironment into chemokine-producing fibroblasts, acting directly on the TIME to make it suitable for cancer growth (85). Qiu et al. have suggested that miR-519a-3p in gastric TDEs activates the MAPK/ERK pathway by targeting DUSP2, leading to M2-like polarization, indirectly inducing angiogenesis, and promoting cancer metastasis (86). Wang et al. have reported that gastric TDEs can destroy the vascular endothelial barrier and promote cancer metastasis (87). Sun and coworkers have reported that high expression of IRF-2 in exosomes can lead to lymphatic vessel endothelium and lymphatic grid formation in sentinel lymph nodes, forming a premetastatic niche (88). Zhang et al. and Liu et al. have shown that one circRNA (hsa_circ_0010522) and miR-155 in exosomes derived from GC cells can act on MSCs to induce their differentiation into brown fat, promote the formation of cancer cachexia, and accelerate the death of patients (89, 90).
Exosomes can also affect other tissue cells and promote the metastasis of cancer cells. Mesenchymal transformation and matrix degeneration are prerequisites for disrupting the mesothelial barrier. Zhu et al. have suggested that miR-106a in gastric TDEs can be transferred to peritoneal mesothelial cells. It also activates TGF-β signaling by targeting Smad7 and TIMP2 to induce mesothelial mesenchymal transition and extracellular matrix degeneration, thereby promoting metastasis and dissemination of GC (91). Lin et al. have reported that downregulation of miR-486-5p in gastric TDEs promotes peritoneal EMT, thereby accelerating peritoneal metastasis of GC (92).
Exosomes are involved in the occurrence and progression of hematological cancers. Exosomal miR-181a secreted by pediatric acute lymphoblastic leukemia (PALL) cells can promote cancer development by upregulating proliferation genes and downregulating apoptosis genes, and silencing this gene can inhibit cancer cell proliferation (30).Silencing TGF-β1 in leukemia-derived exosomes can promote CD4+ T cell proliferation and Th1 cytokine secretion, thereby stimulating specific cytotoxic lymphocytes and NK cytotoxicity more effectively and enhancing antileukemic immunity (93, 94). Ling et al. have demonstrated that diffuse large B-cell lymphoma (DLBCL)-derived exosomes can promote the transformation of macrophages into the M2 type (95). Lou and colleagues have found that downregulation of exosomal miR-7e-5p induces apoptosis in M1 macrophages, leading to immune surveillance and transformation of follicular lymphoma (FL) (96). Ferguson Bennit et al. and Ling et al. have shown that treatment with exosomes does not significantly alter NK-cell functionality, while extracellular survivin decreases NK group 2D receptor (NKG2D) levels and the intracellular protein levels of perforin, granzyme B, TNF-α, and IFN-γ (97). In a study by Umezu and collaborators, exosomes derived from the leukemic cell line K562 highly express miR-92a, which can promote endothelial cell migration and tube formation (98). Chen et al. have shown that exosomes produced by acute myeloid leukemia (AML) KG1A cells can promote the production of IL-8 by bone marrow stromal cells (BMSCs) to promote leukemia drug resistance (99).
Exosomes are involved in the occurrence and progression of BC. BC cells with high metastatic capacity can secrete exosomes carrying miR-200 for transfer to nonmetastatic cells, promoting less metastatic cells to distant metastasis (100). Dong et al. have demonstrated that BC cell-derived exosome PTPRO inhibits BC invasion and migration by promoting macrophage M1 polarization (101). Ham et al. have suggested that Gp130 is a receptor for IL-6, which is present in BC cell-derived exosomes, and it promotes macrophage polarization to M2 and the formation of a cancer immunosuppressive microenvironment, thereby promoting cancer development (102).Zhou and colleagues have revealed that in the early stage of BC, the content of miR-105 in cancer and circulation is closely related to the expression of the targeted tight junction protein ZO-1 and the metastatic progression of cancer, which is mainly achieved by downregulating the tight junction and destroying the barrier function of the endothelial cell monolayer (36). A previous study by Guo et al. has shown that BC cell-derived exosomes transport miR-20a-5p to promote osteoclast formation and thereby participate in bone metastasis of BC cells (103). Even in triple-negative breast cancer, Li et al. have shown that exosomes loaded with PD-L1 secreted by BC cells can inhibit the TIME and promote the progression of triple-negative breast cancer (104).
Exosomes are involved in the occurrence and progression of prostate cancer. Wang and colleagues have revealed that the coculture of exosomes secreted by low-grade prostate cancer cells with highly malignant prostate cancer cells inhibits the proliferation, migration, and invasion of highly malignant cells (105). Singh et al. have demonstrated that exosomes derived from metastatic prostate cancer transfer αvβ3 integrin, distorting the function of non-cancer cells and promoting their migratory phenotype (106). Li and coworkers have reported that exosomes derived from prostate cancer can increase the expression of CXCR4 in MDSCs by activating the TLR2/NF-κB pathway, thereby promoting the migration of MDSCs to the TIME and enhancing the formation of a cancer immunosuppressive microenvironment (107). Zou et al. have reported that prostate cancer-derived exosomal miR-1275 promotes osteoblast activity and may be involved in cancer bone metastasis (108).
Exosomes are involved in the occurrence and progression of glioma. Glioma is cancer originating from glial cells. Experiments have shown that exosomes secreted by glioma-associated stem cells increase the invasiveness of GSSCs and GBM cell lines in vitro, which is related to Semaphorin7A (SEMA7A) in glioma-associated stem cells (109). Li et al. have demonstrated that circGLIS3 (hsa_circ_0002874, originating from exon 2 of GLIS3) in exosomes promotes high-grade glioma invasion by regulating Ezrin phosphorylation (110).Wang Xu and colleagues have revealed that TDEs of glioblastoma (GBM) can fuse to the surface of T cells, increase the concentration of adenosine around T cells, activate adenosine receptor 2A (A2AR), and inhibit aerobic glycolysis in T cells, thereby suppressing T-cell clonal proliferation by reducing energy production. It promotes the formation of a cancer immunosuppressive microenvironment (111). Acquired drug resistance restricts the conventional treatment of gliomas. Zhang and colleagues have found that lncRNA SBF2 antisense RNA 1 (lncRNA SBF2-AS1)-enriched exosomes are involved in temozolomide resistance of thermoresponsive GBM (112).
Exosomes are involved in the occurrence and progression of lung cancer. Lung cancer is one of the malignant cancers with the fastest increasing morbidity and mortality. Mao et al. have reported that miR-2682-5p in non-small cell lung cancer (NSCLC) exosomes can inhibit the viability and migration of NSCLC cells and promote cell apoptosis through the HDAC1/ADH1A axis (113). Chen et al. have recently demonstrated that circular ubiquitin-specific protease-7 (circUSP7) in NSCLC TDEs inhibits the secretion of IFN-γ, TNF-α, granzyme-b, and perforin by CD8+T cells and promotes the formation of a cancer immunosuppressive microenvironment (114). Liu et al. have pointed out that lung TDEs can promote the formation of an immunosuppressive microenvironment by enhancing M2 polarization, leading to cancer progression (115).
Hou and coworkers have reported that salivary gland adenoid cystic carcinoma (SACC)-derived exosomes can act on human umbilical vein endothelial cells to promote cancer invasion (116). Ambrosini et al. have reported that uveal melanoma exosomes promote cancer progression by inducing a cancer immunosuppressive microenvironment through macrophage migration inhibitory factor (MIF) (117). Chen et al. have demonstrated that Nasopharyngeal carcinoma-derived exosomes transfer the macrophage MIF, inhibit macrophage ferroptosis, and promote M2 polarization (118). Xia et al. have indicated that exosomes derived from clear cell renal cell carcinoma evade innate immune surveillance by regulating the TGF-β/SMAD pathway, thereby inducing NK-cell dysfunction (119).
Exosomes provide a therapeutic strategy for cancer immunotherapy (Figure 3). Exosomes are involved in the formation of cancer immunosuppressive microenvironment. Therefore, targeting the production process of tumor exosomes may become an effective treatment modality.As an inert biocompatible substance, exosomes can exert their superior drug delivery advantages to treat cancers. In addition, exosomes also play an essential role in the high-profile immune checkpoint inhibitor PD-1/PD-L1 treatment. Finally, TDEs carry information from primary cells and express complexes of MHC class I/II epitopes and costimulatory molecules, which provide novel strategies for cancer vaccines.
Figure 3 (A) Tumor-derived exosomes can act directly on tumors or be transported to the tumor site through the blood to cause tumor development. Targeting the targets produced by exosomes is a strategy for tumor treatment. (B) Exosomes have become good carriers for drug delivery due to their good biocompatibility. Exosomes can be derived from milk, mesenchymal stem cells, HEK293 cells, dendritic cells, red blood cells, cancer cells, etc. Anticancer drugs can be transferred into exosomes by co-incubation, electroporation, and chemical transfection. (C) Exosomes competitively consume PD-L1 antibody and participate in the resistance of PD-L1 antibody treatment. (D) Therapeutic strategies for exosome vaccines. Monocytes were induced to differentiate into immature DC cells in vitro and then treated with the corresponding tumor-associated antigens (TAAs), Toll-Like Receptors (TLRs) and chemokines such as IFN-γ make DC cells differentiate and mature and secrete exosomes carrying specific tumor antigens, which can directly activate the body’s immunity.
As mentioned above, exosomes contain various small molecular substances that mediate the occurrence and development of cancers. Therefore, targeting the production of exosomes is a strategy to increase or decrease certain small molecular substances to affect the development of cancers. Guan et al. have indicated that high-dose Proton-pump inhibitors (PPIs) suppress cancer size in cancer-bearing BALB/c nude mice, most likely mediated by inhibiting exosome release through suppressing ATP production (124). Huang and colleagues have found that the anticancer effect of lecithin is mediated by the reduction of exosome production and thus the downregulation of miR-1246 carried by them (125). Wang and colleagues have revealed that antigen-presenting cell (APC)-activated lncRNA (lncrNA-APC1) can directly regulate the stability of Rab5b mRNA to inhibit the production of exosomes in CRC cells, thereby suppressing angiogenesis and limiting cancer growth and proliferation (126). Similarly, promoting the secretion of exosomes can also achieve some anticancer effects. Xu et al. have indicated that hyperthermia can promote the secretion of exosomes through Rab7b, upregulate the transfer of FOS and CREB5 anticancer genes into drug-resistant cancer cells, and increase the sensitivity of BC cells to doxorubicin (Dox) (127). All these studies provide potential therapeutic targets. The RAB27A-targeting inhibitor tipifarnib (128), sphingomyelinase inhibitor GW4869 (129), manumycin A (130), and other drugs have been developed, and the clinical safety and efficacy of these drugs need to be further studied.
Exosomes can be loaded with both small and large molecules, supporting their use as therapeutic tools for treating various diseases, including cancer (131). The construction of engineered exosomes loaded with drugs provides a more efficient, less toxic and more targeted drug delivery strategy (132, 133). It has attracted attention due to its good biocompatibility (134, 135). Exosomes can be derived from milk, MSCs, HEK293 cells, DCs, red blood cells, and cancer cells (136). Agrawal et al. have suggested that milk-derived exosomes used for oral delivery of paclitaxel exhibit more marked inhibitory effects in vivo (137). Kanchanapally and colleagues have found that macrophage-derived adriamycin (DOX)-loaded exosomes are more cytotoxic than TDEs (132). This finding provides a basis for selecting raw materials for engineered exosomes. In addition, exosomes carry different peptides, tetraspanins and integrins to target specific cells, which provides strategies for the construction of more specific drug delivery (138–141). Cui et al. used a bone-targeting peptide to modify exosomes to deliver siShn3, which achieved the anti-osteoporosis effect of targeting osteoblasts (142). Compared with liposomes, exosomes have a stronger targeting effect, mainly because CD47 has the ability to evade the phagocytosis of mononuclear macrophages, which was confirmed in the study of Sushrut Kamerkar (143). Therefore, it is not difficult to think that artificial modification of exosomes can better enhance their targeting ability. Zhang et al. integrated hybrid membrane proteins from red blood cells and MCF-7 cancer cells into exosomes and designed an artificial chimeric exosome. Membrane proteins from red blood cells contain high CD47 levels and can inhibit phagocytosis, while membrane proteins from MCF-7 cancer cells contain specific adhesion proteins that can adhere to homologous cancer cells. This exosome obtained significant anti-cancer ability in mouse experiments (144). In addition, Qi et al. shown that superparamagnetic nanoparticle modified exosomes enhanced cancer targeting under external magnetic field and suppressed liver cancer in mice (145). Therefore, exosomes are highly valuable drug tools.
The exosome carrier strategy can also be combined with sonodynamic therapy and nanomedicine to give full play to the advantages of both sides. Wang et al. have developed ExoCe6+R848 by coincubating ultrasound-sensitive Chlorin e6 (Ce6) and R848 into exosomal membranes. Under ultrasound, EXOCe6+R848 stimulates robust maturation of cancer-infiltrating DCs, promotes M1 macrophage polarization and proinflammatory cytokine production, and decreases anti-inflammatory cytokines. This process further inhibits Tregs in the TIME and activates effector T cells for anticancer purposes (146). Although much attention has been paid to drugs, such as nanomedicines (147, 148), the exosome carrier strategy has attracted much attention due to controversial toxicity, immune rejection, and stability (149). Wu and colleagues have used catalase (CAT) and the sonosensitive agent indocyanine green-loaded silica nanoparticles (CAT@SiO2), which is known as a biodegradable nanoplatform (termed as CSI), to coat macrophage exosomes with CSI to form CSI@Ex-A. In addition, exosomes overcome the blood-brain barrier obstruction of the original CSI, making it more effective in clinical practice (150). Various methods exist to transfer anticancer drugs into exosomes, including coincubation, electroporation, and chemical transfection (151, 152) However, the transfer efficiency of multiple processes is different, and exploring more effective construction strategies requires more in-depth research.
Dual-targeting exosome immunotherapy based on exosome delivery to activate the immune system and inhibit immune checkpoints is a more effective emerging strategy. Fan et al. have suggested that by using a dual-targeting exosome-loaded drug, cGAMP (cGAMP@dual-anti-Exos), whose surface is modified with two antibodies (anti-PD-L1 and anti-CD40), two-step activation of DCs and blocking of cancer cell PD-L1, the survival rate of mice inoculated with the drug is up to 60%. In contrast, all other mice die after 40 days, indicating that the drug significantly improves cancer suppression (153). Wang et al. have used the homing ability of cancer cells and macrophages to develop a chimera that secretes outside the body. The cancer cell nucleus embedded within macrophages causes the secretion of macrophage cancer signals to carry body secretion. It relies on the homing ability and lymph nodes to achieve double targets, activates the body’s immune system, removes cancer immunosuppression, and increases anticancer ability. When combined with the immune checkpoint inhibitor PD-1 treatment, chimeric exosomes significantly prolong the survival of a mouse model of cancer recurrence (154).
PD-1/PD-L1 is a crucial target of immune checkpoint inhibitor therapy. PD-L1 in TDEs can induce PD-L1 expression on immune cells, such as neutrophils, inhibit T-cell activation, and promote cancer growth (78, 155). In immunotherapy, exosomes are involved in resistance to immune checkpoint inhibitor therapy targeting PD-L1. One possible mechanism is that exosomes deplete PD-L1 antibodies and increase resistance to PD-L1 therapy (156). In a study by Mauro and collaborators, anti-PD-L1 antibodies synergize with exosomal PD-L1 blockers to inhibit cancer growth. This finding suggests that exosomal PD-L1 is a novel target for relieving cancer drug resistance (45). Various strategies have been developed based on the above mechanisms to target exosomal PD-L1. Blocking any step in exosome production can reduce exosomal PD-L1 expression (157). Therefore, drugs that block exosome production can reduce exosome PD-L1. Shen et al. have shown that blockade of the LSD1 target specifically reduces exosomal PD-L1 expression without reducing intracellular PD-L1 expression and activates T-cell killing, providing a more precise choice of targets for inhibiting exosomal PD-L1 expression (158). In addition, increasing the elimination of exosomes can be therapeutic, such as in vitro ultrafiltration (159), while this approach also removes exosomes from normal cells, and its safety needs to be studied. Wang Min et al. have demonstrated that lactadherin promotes the clearance of circulating cancer cell-derived EVs, which also provides an exosome clearance mode (87). Direct inhibition of PD-L1 production is also a strategy. Shin et al. have reported that sulfamethoxazole effectively reduces exosomal PD-L1 content, thus allowing the anti-PD-L1 antibody to exert a potent anticancer effect. In addition, because inhibition of exosomal PD-L1 enhances T-cell immunity, it also increases the response rate to anti-PD-1 antibodies (160).
Exosomes have the potential to be used as cancer vaccines. As carriers of information transmission, exosomes carry information about the original cell and express complexes of MHC class I/II epitopes and costimulatory molecules. Therefore, they can also activate CD8 T cells. Moreover, exosomes have good biological stability and bioavailability, providing a basis for their application in cancer vaccines (44, 161–163). As early as 2004, Altieri and coworkers have reported that plasmacytoma cells release exosomes in vitro, and a single dose (5 µg) of exosomal protein combined with an isogenic vaccine can protect 80% of mice against wild-type cancer challenge, making exosome vaccines possible (164). There are two significant obstacles to the success of DC vaccines: cancer-mediated immunosuppression and the functional limitations of commonly used monocyte-derived DCs, which are effectively avoided by using cancer cell exosomes (165). It has been found that vaccination with DCexos (derived from BMDCs) has better anticancer efficacy than vaccination with DCs (44, 166).
Exosomes can be designed as vaccine adjuvants. Yildirim and colleagues have developed BC cell-derived exosomes carrying two immune adjuvants, the TLR9 ligand, K-type CpG ODN and a TLR3 ligand, p(I:C), to achieve more vigorous cellular and humoral anticancer immunity. In addition, it can inhibit cancer growth in mice (167). Experiments have shown that transfer of CCL22 siRNA into immunologically dead TDEs can inhibit Treg expansion and enhance anticancer effects. Better anticancer efficacy has been achieved when combined with chemotherapy drugs (168). Exosomes derived from M1-polarized macrophages can induce the release of a Th1 cytokine repertoire and antigen-specific cytotoxic T-cell responses, acting as immunopotentiating agents for cancer vaccines (169), most likely through downregulation of Wnt signaling (170). Kavitha et al. have demonstrated that exosomes from mouse embryonic stem cells engineered to produce GM-CSF activate a higher CD8+ T effector, Th1-cell response, and significantly block cancer growth (171). Huang et al. have developed an exosome loaded with Hiltonol (TLR3 agonist) and ICD (immunogenic cell death) inducer human neutrophil elastase (ELANE). In addition, the engineered Hiltonol-elane-α-la exosomes (HELA-Exos) are modified with α-lactalbumin on the exosome surface, which induces strong anticancer immunity (172). Phung and colleagues combined immune checkpoint blockade strategies with cancer vaccines, and modified anti-CTLA-4 antibodies on the surface of exosomes by lipid anchoring, which effectively promoted T cell targeting and increased tumor-specific T cell responses, leading to significant tumor growth inhibition (173). Modifying exosomes with MART-1 peptides to induce a stronger immune response (168). These strategies show the potential of exosomes as cancer vaccines to specifically activate anti-tumor immunity.
This article reviews the research progress of exosomes, mainly taking gastrointestinal cancers as an example, and introduces the research progress of exosomes in cancers and cancer immunotherapy. Clearly, understanding the mechanism of action of cancer exosomes in cancers provides new options for therapeutic targets, as well as promising biomarkers for the delivery of exosome-loaded small molecules that can be engineered for less invasive cancer diagnosis (174). However, the discovery of more efficient exosome isolation methods is a challenge (112). Chen et al. have explored a microfluidic device for on-chip isolation for immunomagnetic separation and in situ detection of blood exosomes. The device also has a high prediction accuracy for cancer extracellular markers, with a sensitivity of 90% and a specificity of 95% (175). Furthermore, Thakur and colleagues have developed an in-house novel exosome detection/separation strategy and a localized surface plasmon resonance biosensor with self-assembled gold nanoislands (SAM-AuNIs) (176). This finding provides a method for us to make better use of exosomes.
There is still a long way to go for exosome-related research. Previous studies have shown that cancer cell chromosomal DNA fragments can be found in TDEs, while the specific sorting mechanism has not been determined (177). Moreover, some of the studies reviewed in this paper are limited to in vitro experiments, and the data and conclusions in vivo are likely to be affected by environmental interactions. Therefore, its guidance for clinical practice is limited, and more studies are needed to support it. In addition, most studies at this stage are limited to small nucleic acids and proteins, and the role of lipid components has also attracted much attention in recent years (178). In cancer treatment, exosomes can be designed as carriers or targets to develop therapeutic strategies to relieve therapeutic drug resistance. As potential vectors for dual-targeted therapy, ARG1 (179), TIM3and EBAG9 (180) have been confirmed to exist in exosomes, while there are few studies, and their more precise regulatory mechanisms need to be further studied. In conclusion, although significant progress has been made in the analysis of exosomes, many issues still need to be studied further. These include better strategies for sorting exosomes carrying genetic materials, improved extraction and detection methods, and more stable and accurate strategies for modifying drugs delivered via exosomes. Finally, exosomes show great promise as cancer immunotherapy. As knowledge grows, therapeutic approaches based on exosomes will become increasingly used in clinical settings.
YL and WG designed and conceptualized the review. YC and YL wrote the manuscript. WG and YL revised the manuscript. All authors contributed to the article and approved the submitted version.
This study was supported by the Youth Science Fund Project of the National Natural Science Foundation of China (grant no. 81800100) and the Project of Changzhou Health Leading Talent Project during the 14th Five Year Plan Period (grant no. KY20221336).
Thanks to Zhang Yan and Ji Ling for their patient guidance and help.
The authors declare that the research was conducted in the absence of any commercial or financial relationships that could be construed as a potential conflict of interest.
All claims expressed in this article are solely those of the authors and do not necessarily represent those of their affiliated organizations, or those of the publisher, the editors and the reviewers. Any product that may be evaluated in this article, or claim that may be made by its manufacturer, is not guaranteed or endorsed by the publisher.
1. Huber V, Camisaschi C, Berzi A, Ferro S, Lugini L, Triulzi T, et al. Cancer acidity: An ultimate frontier of tumor immune escape and a novel target of immunomodulation. Semin Cancer Biol (2017) 43:74–89. doi: 10.1016/j.semcancer.2017.03.001
2. Dumontet E, Pangault C, Roulois D, Desoteux M, Léonard S, Marchand T, et al. Extracellular vesicles shed by follicular lymphoma b cells promote polarization of the bone marrow stromal cell niche. Blood (2021) 138(1):57–70. doi: 10.1182/blood.2020008791
3. Hida K, Maishi N, Torii C, Hida Y. Tumor angiogenesis–characteristics of tumor endothelial cells. Int J Clin Oncol (2016) 21(2):206–12. doi: 10.1007/s10147-016-0957-1
4. Roma-Rodrigues C, Mendes R, Baptista PV, Fernandes AR. Targeting tumor microenvironment for cancer therapy. Int J Mol Sci (2019) 20(4):1–31. doi: 10.3390/ijms20040840
5. DiSipio T, Rye S, Newman B, Hayes S. Incidence of unilateral arm lymphoedema after breast cancer: A systematic review and meta-analysis. Lancet Oncol (2013) 14(6):500–15. doi: 10.1016/s1470-2045(13)70076-7
6. Okwuosa TM, Anzevino S, Rao R. Cardiovascular disease in cancer survivors. Postgraduate Med J (2017) 93(1096):82–90. doi: 10.1136/postgradmedj-2016-134417
7. Kim MJ, Kim YS, Park SC, Sohn DK, Kim DY, Chang HJ, et al. Risk factors for permanent stoma after rectal cancer surgery with temporary ileostomy. Surgery (2016) 159(3):721–7. doi: 10.1016/j.surg.2015.09.011
8. Dault R, Rousseau MP, Beaudoin A, Frenette MA, Lemay F, Beauchesne MF. Impact of oxaliplatin-induced neuropathy in patients with colorectal cancer: A prospective evaluation at a single institution. Curr Oncol (Toronto Ont) (2016) 23(1):e65–9. doi: 10.3747/co.23.2780
9. Cruzado JA, López-Santiago S, Martínez-Marín V, José-Moreno G, Custodio AB, Feliu J. Longitudinal study of cognitive dysfunctions induced by adjuvant chemotherapy in colon cancer patients. Supportive Care cancer: Off J Multinational Assoc Supportive Care Cancer (2014) 22(7):1815–23. doi: 10.1007/s00520-014-2147-x
10. Poghosyan H, Sheldon LK, Leveille SG, Cooley ME. Health-related quality of life after surgical treatment in patients with non-small cell lung cancer: A systematic review. Lung Cancer (Amsterdam Netherlands) (2013) 81(1):11–26. doi: 10.1016/j.lungcan.2013.03.013
11. Allegra A, Innao V, Basile G, Pugliese M, Allegra AG, Pulvirenti N, et al. Post-chemotherapy cognitive impairment in hematological patients: Current understanding of chemobrain in hematology. Expert Rev Hematol (2020) 13(4):393–404. doi: 10.1080/17474086.2020.1738213
12. Zhang B, Zhang X, Li M, Kong L, Deng X, Yu J. How breast cancer chemotherapy increases the risk of leukemia: Thoughts about a case of diffuse Large b-cell lymphoma and leukemia after breast cancer chemotherapy. Cancer Biol Ther (2016) 17(2):125–8. doi: 10.1080/15384047.2016.1139233
13. Lee YT, Tan YJ, Oon CE. Molecular targeted therapy: Treating cancer with specificity. Eur J Pharmacol (2018) 834:188–96. doi: 10.1016/j.ejphar.2018.07.034
14. Robertson DS. The development of tumor cell characteristics. J Cell Physiol (2014) 229(6):705–10. doi: 10.1002/jcp.24498
15. Aoyagi K, Kouhuji K, Kizaki J, Isobe T, Hashimoto K, Shirouzu K. Molecular targeting to treat gastric cancer. World J Gastroenterol (2014) 20(38):13741–55. doi: 10.3748/wjg.v20.i38.13741
16. Li X, Xu J, Xie J, Yang W. Research progress in targeted therapy and immunotherapy for gastric cancer. Chin Med J (2022) 135(11):1299–313. doi: 10.1097/cm9.0000000000002185
17. Kobayashi Y. Molecular target therapy in hematological malignancy: Front-runners and prototypes of small molecule and antibody therapy. Japanese J Clin Oncol (2011) 41(2):157–64. doi: 10.1093/jjco/hyq189
18. Chen Z, Li Y, Tan B, Zhao Q, Fan L, Li F, et al. Progress and current status of molecule-targeted therapy and drug resistance in gastric cancer. Drugs Today (Barcelona Spain: 1998) (2020) 56(7):469–82. doi: 10.1358/dot.2020.56.7.3112071
19. Sanford DS, Kantarjian H, O’Brien S, Jabbour E, Cortes J, Ravandi F. The role of ponatinib in Philadelphia chromosome-positive acute lymphoblastic leukemia. Expert Rev Anticancer Ther (2015) 15(4):365–73. doi: 10.1586/14737140.2015.1025256
20. Browne BC, Crown J, Venkatesan N, Duffy MJ, Clynes M, Slamon D, et al. Inhibition of Igf1r activity enhances response to trastuzumab in her-2-Positive breast cancer cells. Ann oncology: Off J Eur Soc Med Oncol (2011) 22(1):68–73. doi: 10.1093/annonc/mdq349
21. Dalton HJ, Pradeep S, McGuire M, Hailemichael Y, Ma S, Lyons Y, et al. Macrophages facilitate resistance to anti-vegf therapy by altered vegfr expression. Clin Cancer research: An Off J Am Assoc Cancer Res (2017) 23(22):7034–46. doi: 10.1158/1078-0432.Ccr-17-0647
22. Hou Z, Meng C, Yang F, Deng Y, Han X, Liu H. Mapping tyrosine kinases based on a tk activity-representing peptide library reveals a role for src in H1975 drug resistance. J Proteome Res (2022) 21(4):1105–13. doi: 10.1021/acs.jproteome.1c00980
23. Lamplugh Z, Fan Y. Vascular microenvironment, tumor immunity and immunotherapy. Front Immunol (2021) 12:811485. doi: 10.3389/fimmu.2021.811485
24. Wang W, Green M, Choi JE, Gijón M, Kennedy PD, Johnson JK, et al. Cd8(+) T cells regulate tumour ferroptosis during cancer immunotherapy. Nature (2019) 569(7755):270–4. doi: 10.1038/s41586-019-1170-y
25. Lv B, Wang Y, Ma D, Cheng W, Liu J, Yong T, et al. Immunotherapy: Reshape the tumor immune microenvironment. Front Immunol (2022) 13:844142. doi: 10.3389/fimmu.2022.844142
26. Schadendorf D, Hodi FS, Robert C, Weber JS, Margolin K, Hamid O, et al. Pooled analysis of long-term survival data from phase ii and phase iii trials of ipilimumab in unresectable or metastatic melanoma. J Clin oncology: Off J Am Soc Clin Oncol (2015) 33(17):1889–94. doi: 10.1200/jco.2014.56.2736
27. Hamid O, Robert C, Daud A, Hodi FS, Hwu WJ, Kefford R, et al. Five-year survival outcomes for patients with advanced melanoma treated with pembrolizumab in keynote-001. Ann oncology: Off J Eur Soc Med Oncol (2019) 30(4):582–8. doi: 10.1093/annonc/mdz011
28. Zhao J, Song Y, Liu D. Clinical trials of dual-target car T cells, donor-derived car T cells, and universal car T cells for acute lymphoid leukemia. J Hematol Oncol (2019) 12(1):17. doi: 10.1186/s13045-019-0705-x
29. Kahlert C, Kalluri R. Exosomes in tumor microenvironment influence cancer progression and metastasis. J Mol Med (Berlin Germany) (2013) 91(4):431–7. doi: 10.1007/s00109-013-1020-6
30. Haque S, Vaiselbuh SR. Silencing of exosomal mir-181a reverses pediatric acute lymphocytic leukemia cell proliferation. Pharm (Basel Switzerland) (2020) 13(9):1–16. doi: 10.3390/ph13090241
31. Samanta S, Rajasingh S, Drosos N, Zhou Z, Dawn B, Rajasingh J. Exosomes: New molecular targets of diseases. Acta pharmacologica Sin (2018) 39(4):501–13. doi: 10.1038/aps.2017.162
32. Raposo G, Stoorvogel W. Extracellular vesicles: Exosomes, microvesicles, and friends. J Cell Biol (2013) 200(4):373–83. doi: 10.1083/jcb.201211138
33. Escrevente C, Keller S, Altevogt P, Costa J. Interaction and uptake of exosomes by ovarian cancer cells. BMC Cancer (2011) 11:108. doi: 10.1186/1471-2407-11-108
34. Feng D, Zhao WL, Ye YY, Bai XC, Liu RQ, Chang LF, et al. Cellular internalization of exosomes occurs through phagocytosis. Traffic (Copenhagen Denmark) (2010) 11(5):675–87. doi: 10.1111/j.1600-0854.2010.01041.x
35. Png KJ, Halberg N, Yoshida M, Tavazoie SF. A microrna regulon that mediates endothelial recruitment and metastasis by cancer cells. Nature (2011) 481(7380):190–4. doi: 10.1038/nature10661
36. Zhou W, Fong MY, Min Y, Somlo G, Liu L, Palomares MR, et al. Cancer-secreted mir-105 destroys vascular endothelial barriers to promote metastasis. Cancer Cell (2014) 25(4):501–15. doi: 10.1016/j.ccr.2014.03.007
37. Guo Y, Ji X, Liu J, Fan D, Zhou Q, Chen C, et al. Effects of exosomes on pre-metastatic niche formation in tumors. Mol Cancer (2019) 18(1):39. doi: 10.1186/s12943-019-0995-1
38. Guney Eskiler G, Kazan N, Haciefendi A, Deveci Ozkan A, Ozdemir K, Ozen M, et al. The prognostic and predictive values of differential expression of exosomal receptor tyrosine kinases and associated with the Pi3k/Akt/Mtor signaling in breast cancer patients undergoing neoadjuvant chemotherapy. Clin Trans oncology: Off Publ Fed Spanish Oncol Societies Natl Cancer Institute Mexico (2022) 18. doi: 10.1007/s12094-022-02959-9
39. Admyre C, Johansson SM, Paulie S, Gabrielsson S. Direct exosome stimulation of peripheral human T cells detected by elispot. Eur J Immunol (2006) 36(7):1772–81. doi: 10.1002/eji.200535615
40. Utsugi-Kobukai S, Fujimaki H, Hotta C, Nakazawa M, Minami M. Mhc class I-mediated exogenous antigen presentation by exosomes secreted from immature and mature bone marrow derived dendritic cells. Immunol Lett (2003) 89(2-3):125–31. doi: 10.1016/s0165-2478(03)00128-7
41. Raposo G, Nijman HW, Stoorvogel W, Liejendekker R, Harding CV, Melief CJ, et al. B lymphocytes secrete antigen-presenting vesicles. J Exp Med (1996) 183(3):1161–72. doi: 10.1084/jem.183.3.1161
42. Wolfers J, Lozier A, Raposo G, Regnault A, Théry C, Masurier C, et al. Tumor-derived exosomes are a source of shared tumor rejection antigens for ctl cross-priming. Nat Med (2001) 7(3):297–303. doi: 10.1038/85438
43. van der Mude A. A proposed information-based modality for the treatment of cancer. Bio Syst (2022) 211:104587. doi: 10.1016/j.biosystems.2021.104587
44. Zitvogel L, Regnault A, Lozier A, Wolfers J, Flament C, Tenza D, et al. Eradication of established murine tumors using a novel cell-free vaccine: Dendritic cell-derived exosomes. Nat Med (1998) 4(5):594–600. doi: 10.1038/nm0598-594
45. Poggio M, Hu T, Pai CC, Chu B, Belair CD, Chang A, et al. Suppression of exosomal pd-L1 induces systemic anti-tumor immunity and memory. Cell (2019) 177(2):414–27.e13. doi: 10.1016/j.cell.2019.02.016
46. Han S, Gonzalo DH, Feely M, Rinaldi C, Belsare S, Zhai H, et al. Stroma-derived extracellular vesicles deliver tumor-suppressive mirnas to pancreatic cancer cells. Oncotarget (2018) 9(5):5764–77. doi: 10.18632/oncotarget.23532
47. Donnarumma E, Fiore D, Nappa M, Roscigno G, Adamo A, Iaboni M, et al. Cancer-associated fibroblasts release exosomal micrornas that dictate an aggressive phenotype in breast cancer. Oncotarget (2017) 8(12):19592–608. doi: 10.18632/oncotarget.14752
48. Wang H, Wei H, Wang J, Li L, Chen A, Li Z. Microrna-181d-5p-Containing exosomes derived from cafs promote emt by regulating Cdx2/Hoxa5 in breast cancer. Mol Ther Nucleic Acids (2020) 19:654–67. doi: 10.1016/j.omtn.2019.11.024
49. Li H, Lin X, Yang D, Chen Z, Wang X, Re F, et al. Cancer-associated fibroblasts support bone tropic metastasis by acting as coordinators between the tumor microenvironment and bone matrix in breast cancer. Neoplasma (2021) 68(1):10–22. doi: 10.4149/neo_2020_200905N951
50. Zhao A, Zhao Z, Liu W, Cui X, Wang N, Wang Y, et al. Carcinoma-associated fibroblasts promote the proliferation and metastasis of osteosarcoma by transferring exosomal lncrna Snhg17. Am J Trans Res (2021) 13(9):10094–111.
51. Lazar I, Clement E, Dauvillier S, Milhas D, Ducoux-Petit M, LeGonidec S, et al. Adipocyte exosomes promote melanoma aggressiveness through fatty acid oxidation: A novel mechanism linking obesity and cancer. Cancer Res (2016) 76(14):4051–7. doi: 10.1158/0008-5472.Can-16-0651
52. Mao J, Liang Z, Zhang B, Yang H, Li X, Fu H, et al. Ubr2 enriched in P53 deficient mouse bone marrow mesenchymal stem cell-exosome promoted gastric cancer progression Via Wnt/B-catenin pathway. Stem Cells (Dayton Ohio) (2017) 35(11):2267–79. doi: 10.1002/stem.2702
53. Ma M, Chen S, Liu Z, Xie H, Deng H, Shang S, et al. Mirna-221 of exosomes originating from bone marrow mesenchymal stem cells promotes oncogenic activity in gastric cancer. OncoTargets Ther (2017) 10:4161–71. doi: 10.2147/ott.S143315
54. Deng SZ, Lai MF, Li YP, Xu CH, Zhang HR, Kuang JG. Human marrow stromal cells secrete microrna-375-Containing exosomes to regulate glioma progression. Cancer Gene Ther (2020) 27(3-4):203–15. doi: 10.1038/s41417-019-0079-9
55. Lugini L, Cecchetti S, Huber V, Luciani F, Macchia G, Spadaro F, et al. Immune surveillance properties of human nk cell-derived exosomes. J Immunol (Baltimore Md: 1950) (2012) 189(6):2833–42. doi: 10.4049/jimmunol.1101988
56. Samara A, Anbar M, Shapira S, Zemlyansky A, Zozovsky A, Raanani P, et al. Using natural killer cell-derived exosomes as a cell-free therapy for leukemia. Hematological Oncol (2022) 40:1–12. doi: 10.1002/hon.3111
57. Romagnoli GG, Zelante BB, Toniolo PA, Migliori IK, Barbuto JA. Dendritic cell-derived exosomes may be a tool for cancer immunotherapy by converting tumor cells into immunogenic targets. Front Immunol (2014) 5:692. doi: 10.3389/fimmu.2014.00692
58. Cai Z, Yang F, Yu L, Yu Z, Jiang L, Wang Q, et al. Activated T cell exosomes promote tumor invasion Via fas signaling pathway. J Immunol (Baltimore Md: 1950) (2012) 188(12):5954–61. doi: 10.4049/jimmunol.1103466
59. Xie Y, Zhang X, Zhao T, Li W, Xiang J. Natural Cd8⁺25⁺ regulatory T cell-secreted exosomes capable of suppressing cytotoxic T lymphocyte-mediated immunity against B16 melanoma. Biochem Biophys Res Commun (2013) 438(1):152–5. doi: 10.1016/j.bbrc.2013.07.044
60. Wang Y, Ma H, Li Y, Su R. Mir-192-5p-Modified tumor-associated macrophages-derived exosome suppressed endometrial cancer progression through targeting Irak1/Nf-Kb signaling. Reprod Sci (Thousand Oaks Calif) (2022) 29(2):436–47. doi: 10.1007/s43032-021-00789-8
61. Tumino N, Besi F, Martini S, Di Pace AL, Munari E, Quatrini L, et al. Polymorphonuclear myeloid-derived suppressor cells are abundant in peripheral blood of cancer patients and suppress natural killer cell anti-tumor activity. Front Immunol (2021) 12:803014. doi: 10.3389/fimmu.2021.803014
62. Bai ZZ, Li HY, Li CH, Sheng CL, Zhao XN. M1 macrophage-derived exosomal microrna-326 suppresses hepatocellular carcinoma cell progression Via mediating nf-Kb signaling pathway. Nanoscale Res Lett (2020) 15(1):221. doi: 10.1186/s11671-020-03432-8
63. Zhao LJ, Li YY, Zhang YT, Fan QQ, Ren HM, Zhang C, et al. Lysine demethylase Lsd1 delivered Via small extracellular vesicles promotes gastric cancer cell stemness. EMBO Rep (2021) 22(8):e50922. doi: 10.15252/embr.202050922
64. Sun J, Lu Z, Fu W, Lu K, Gu X, Xu F, et al. Exosome-derived Adam17 promotes liver metastasis in colorectal cancer. Front Pharmacol (2021) 12:734351. doi: 10.3389/fphar.2021.734351
65. Yu L, Xie J, Liu X, Yu Y, Wang S. Plasma exosomal Circnek9 accelerates the progression of gastric cancer Via mir-409-3p/Map7 axis. Digestive Dis Sci (2021) 66(12):4274–89. doi: 10.1007/s10620-020-06816-z
66. Lu J, Wang YH, Yoon C, Huang XY, Xu Y, Xie JW, et al. Circular rna circ-Rangap1 regulates vegfa expression by targeting mir-877-3p to facilitate gastric cancer invasion and metastasis. Cancer Lett (2020) 471:38–48. doi: 10.1016/j.canlet.2019.11.038
67. Gao L, Tang X, He Q, Sun G, Wang C, Qu H. Exosome-transmitted Circcog2 promotes colorectal cancer progression Via mir-1305/Tgf-B2/Smad3 pathway. Cell Death Discovery (2021) 7(1):281. doi: 10.1038/s41420-021-00680-0
68. Liu X, Lu Y, Xu Y, Hou S, Huang J, Wang B, et al. Exosomal transfer of mir-501 confers doxorubicin resistance and tumorigenesis Via targeting of blid in gastric cancer. Cancer Lett (2019) 459:122–34. doi: 10.1016/j.canlet.2019.05.035
69. Li P, Luo X, Xie Y, Li P, Hu F, Chu J, et al. Gc-derived evs enriched with microrna-675-3p contribute to the Mapk/Pd-L1-Mediated tumor immune escape by targeting Cxxc4. Mol Ther Nucleic Acids (2020) 22:615–26. doi: 10.1016/j.omtn.2020.08.020
70. Liang Y, Liu Y, Zhang Q, Zhang H, Du J. Tumor-derived extracellular vesicles containing microrna-1290 promote immune escape of cancer cells through the Grhl2/Zeb1/Pd-L1 axis in gastric cancer. Trans research: J Lab Clin Med (2021) 231:102–12. doi: 10.1016/j.trsl.2020.12.003
71. Liu H, Liu Y, Sun P, Leng K, Xu Y, Mei L, et al. Colorectal cancer-derived exosomal mir-106b-3p promotes metastasis by down-regulating dlc-1 expression. Clin Sci (London England: 1979) (2020) 134(4):419–34. doi: 10.1042/cs20191087
72. Sun X, Lin F, Sun W, Zhu W, Fang D, Luo L, et al. Exosome-transmitted mirna-335-5p promotes colorectal cancer invasion and metastasis by facilitating emt Via targeting Rasa1. Mol Ther Nucleic Acids (2021) 24:164–74. doi: 10.1016/j.omtn.2021.02.022
73. Zhang Y, Chen L, Ye X, Wu Z, Zhang Z, Sun B, et al. Expression and mechanism of exosome-mediated a Foxm1 related long noncoding rna in gastric cancer. J nanobiotechnology (2021) 19(1):133. doi: 10.1186/s12951-021-00873-w
74. Xian D, Niu L, Zeng J, Wang L. Lncrna Kcnq1ot1 secreted by tumor cell-derived exosomes mediates immune escape in colorectal cancer by regulating pd-L1 ubiquitination Via mir-30a-5p/Usp22. Front Cell Dev Biol (2021) 9:653808. doi: 10.3389/fcell.2021.653808
75. Liu J, Wu S, Zheng X, Zheng P, Fu Y, Wu C, et al. Immune suppressed tumor microenvironment by exosomes derived from gastric cancer cells Via modulating immune functions. Sci Rep (2020) 10(1):14749. doi: 10.1038/s41598-020-71573-y
76. Li J, Sun L, Chen Y, Zhu J, Shen J, Wang J, et al. Gastric cancer-derived exosomal mir-135b-5p impairs the function of Vγ9vδ2 T cells by targeting specificity protein 1. Cancer immunology immunotherapy: CII (2022) 71(2):311–25. doi: 10.1007/s00262-021-02991-8
77. Zhang X, Shi H, Yuan X, Jiang P, Qian H, Xu W. Tumor-derived exosomes induce N2 polarization of neutrophils to promote gastric cancer cell migration. Mol Cancer (2018) 17(1):146. doi: 10.1186/s12943-018-0898-6
78. Shi Y, Zhang J, Mao Z, Jiang H, Liu W, Shi H, et al. Extracellular vesicles from gastric cancer cells induce pd-L1 expression on neutrophils to suppress T-cell immunity. Front Oncol (2020) 10:629. doi: 10.3389/fonc.2020.00629
79. Hinata M, Kunita A, Abe H, Morishita Y, Sakuma K, Yamashita H, et al. Exosomes of Epstein-Barr virus-associated gastric carcinoma suppress dendritic cell maturation. Microorganisms (2020) 8(11):1–15. doi: 10.3390/microorganisms8111776
80. Takano Y, Masuda T, Iinuma H, Yamaguchi R, Sato K, Tobo T, et al. Circulating exosomal microrna-203 is associated with metastasis possibly Via inducing tumor-associated macrophages in colorectal cancer. Oncotarget (2017) 8(45):78598–613. doi: 10.18632/oncotarget.20009
81. Ren W, Zhang X, Li W, Feng Q, Feng H, Tong Y, et al. Exosomal mirna-107 induces myeloid-derived suppressor cell expansion in gastric cancer. Cancer Manage Res (2019) 11:4023–40. doi: 10.2147/cmar.S198886
82. Tang D, Liu S, Shen H, Deng G, Zeng S. Extracellular vesicles promote the formation of pre-metastasis niche in gastric cancer. Front Immunol (2022) 13:813015. doi: 10.3389/fimmu.2022.813015
83. Zhang C, Wang XY, Zhang P, He TC, Han JH, Zhang R, et al. Cancer-derived exosomal Hspc111 promotes colorectal cancer liver metastasis by reprogramming lipid metabolism in cancer-associated fibroblasts. Cell Death Dis (2022) 13(1):57. doi: 10.1038/s41419-022-04506-4
84. Huang YJ, Huang TH, Yadav VK, Sumitra MR, Tzeng DT, Wei PL, et al. Preclinical investigation of ovatodiolide as a potential inhibitor of colon cancer stem cells Via downregulating sphere-derived exosomal B-Catenin/Stat3/Mir-1246 cargoes. Am J Cancer Res (2020) 10(8):2337–54.
85. Naito Y, Yamamoto Y, Sakamoto N, Shimomura I, Kogure A, Kumazaki M, et al. Cancer extracellular vesicles contribute to stromal heterogeneity by inducing chemokines in cancer-associated fibroblasts. Oncogene (2019) 38(28):5566–79. doi: 10.1038/s41388-019-0832-4
86. Qiu S, Xie L, Lu C, Gu C, Xia Y, Lv J, et al. Gastric cancer-derived exosomal mir-519a-3p promotes liver metastasis by inducing intrahepatic M2-like macrophage-mediated angiogenesis. J Exp Clin Cancer research: CR (2022) 41(1):296. doi: 10.1186/s13046-022-02499-8
87. Wang M, Cai W, Yang AJ, Wang CY, Zhang CL, Liu W, et al. Gastric cancer cell-derived extracellular vesicles disrupt endothelial integrity and promote metastasis. Cancer Lett (2022) 545:215827. doi: 10.1016/j.canlet.2022.215827
88. Sun B, Zhou Y, Fang Y, Li Z, Gu X, Xiang J. Colorectal cancer exosomes induce lymphatic network remodeling in lymph nodes. Int J Cancer (2019) 145(6):1648–59. doi: 10.1002/ijc.32196
89. Zhang H, Zhu L, Bai M, Liu Y, Zhan Y, Deng T, et al. Exosomal circrna derived from gastric tumor promotes white adipose browning by targeting the mir-133/Prdm16 pathway. Int J Cancer (2019) 144(10):2501–15. doi: 10.1002/ijc.31977
90. Liu Y, Wang M, Deng T, Liu R, Ning T, Bai M, et al. Exosomal mir-155 from gastric cancer induces cancer-associated cachexia by suppressing adipogenesis and promoting brown adipose differentiation Via C/Epbβ. Cancer Biol Med (2022) 19(9):1301–14. doi: 10.20892/j.issn.2095-3941.2021.0220
91. Zhu M, Zhang N, Ma J, He S. Integration of exosomal mir-106a and mesothelial cells facilitates gastric cancer peritoneal dissemination. Cell signalling (2022) 91:110230. doi: 10.1016/j.cellsig.2021.110230
92. Lin XM, Wang ZJ, Lin YX, Chen H. Decreased exosome-delivered mir-486-5p is responsible for the peritoneal metastasis of gastric cancer cells by promoting emt progress. World J Surg Oncol (2021) 19(1):312. doi: 10.1186/s12957-021-02381-5
93. Huang F, Wan J, Hao S, Deng X, Chen L, Ma L. Tgf-B1-Silenced leukemia cell-derived exosomes target dendritic cells to induce potent anti-leukemic immunity in a mouse model. Cancer immunology immunotherapy: CII (2017) 66(10):1321–31. doi: 10.1007/s00262-017-2028-5
94. Huang F, Wan J, Hu W, Hao S. Enhancement of anti-leukemia immunity by leukemia-derived exosomes Via downregulation of tgf-B1 expression. Cell Physiol biochemistry: Int J Exp Cell physiology biochemistry Pharmacol (2017) 44(1):240–54. doi: 10.1159/000484677
95. Ling HY, Yang Z, Wang PJ, Sun Y, Ju SG, Li J, et al. Diffuse Large b-cell lymphoma-derived exosomes push macrophage polarization toward M2 phenotype Via Gp130/Stat3 signaling pathway. Chemico-biological Interact (2022) 352:109779. doi: 10.1016/j.cbi.2021.109779
96. Lou X, Fu J, Zhao X, Zhuansun X, Rong C, Sun M, et al. Mir-7e-5p downregulation promotes transformation of low-grade follicular lymphoma to aggressive lymphoma by modulating an immunosuppressive stroma through the upregulation of fasl in M1 macrophages. J Exp Clin Cancer research: CR (2020) 39(1):237. doi: 10.1186/s13046-020-01747-z
97. Ferguson Bennit HR, Gonda A, Kabagwira J, Oppegard L, Chi D, Licero Campbell J, et al. Natural killer cell phenotype and functionality affected by exposure to extracellular survivin and lymphoma-derived exosomes. Int J Mol Sci (2021) 22(3):1–18. doi: 10.3390/ijms22031255
98. Umezu T, Ohyashiki K, Kuroda M, Ohyashiki JH. Leukemia cell to endothelial cell communication Via exosomal mirnas. Oncogene (2013) 32(22):2747–55. doi: 10.1038/onc.2012.295
99. Chen T, Zhang G, Kong L, Xu S, Wang Y, Dong M. Leukemia-derived exosomes induced il-8 production in bone marrow stromal cells to protect the leukemia cells against chemotherapy. Life Sci (2019) 221:187–95. doi: 10.1016/j.lfs.2019.02.003
100. Le MT, Hamar P, Guo C, Basar E, Perdigão-Henriques R, Balaj L, et al. Mir-200-Containing extracellular vesicles promote breast cancer cell metastasis. J Clin Invest (2014) 124(12):5109–28. doi: 10.1172/jci75695
101. Dong H, Xie C, Jiang Y, Li K, Lin Y, Pang X, et al. Tumor-derived exosomal protein tyrosine phosphatase receptor type O polarizes macrophage to suppress breast tumor cell invasion and migration. Front Cell Dev Biol (2021) 9:703537. doi: 10.3389/fcell.2021.703537
102. Ham S, Lima LG, Chai EPZ, Muller A, Lobb RJ, Krumeich S, et al. Breast cancer-derived exosomes alter macrophage polarization Via Gp130/Stat3 signaling. Front Immunol (2018) 9:871. doi: 10.3389/fimmu.2018.00871
103. Guo L, Zhu Y, Li L, Zhou S, Yin G, Yu G, et al. Breast cancer cell-derived exosomal mir-20a-5p promotes the proliferation and differentiation of osteoclasts by targeting Srcin1. Cancer Med (2019) 8(12):5687–701. doi: 10.1002/cam4.2454
104. Li C, Qiu S, Jin K, Zheng X, Zhou X, Jin D, et al. Tumor-derived microparticles promote the progression of triple-negative breast cancer Via pd-L1-Associated immune suppression. Cancer Lett (2021) 523:43–56. doi: 10.1016/j.canlet.2021.09.039
105. Wang X, Wang X, Zhu Z, Li W, Yu G, Jia Z, et al. Prostate carcinoma cell-derived exosomal microrna-26a modulates the metastasis and tumor growth of prostate carcinoma. Biomedicine pharmacotherapy = Biomedecine pharmacotherapie (2019) 117:109109. doi: 10.1016/j.biopha.2019.109109
106. Singh A, Fedele C, Lu H, Nevalainen MT, Keen JH, Languino LR. Exosome-mediated transfer of Avβ3 integrin from tumorigenic to nontumorigenic cells promotes a migratory phenotype. Mol Cancer research: MCR (2016) 14(11):1136–46. doi: 10.1158/1541-7786.Mcr-16-0058
107. Li N, Wang Y, Xu H, Wang H, Gao Y, Zhang Y. Exosomes derived from rm-1 cells promote the recruitment of mdscs into tumor microenvironment by upregulating Cxcr4 Via Tlr2/Nf-Kb pathway. J Oncol (2021) 2021:5584406. doi: 10.1155/2021/5584406
108. Zou Z, Dai R, Deng N, Su W, Liu P. Exosomal mir-1275 secreted by prostate cancer cells modulates osteoblast proliferation and activity by targeting the Sirt2/Runx2 cascade. Cell Transplant (2021) 30:9636897211052977. doi: 10.1177/09636897211052977
109. Manini I, Ruaro ME, Sgarra R, Bartolini A, Caponnetto F, Ius T, et al. Semaphorin-7a on exosomes: A promigratory signal in the glioma microenvironment. Cancers (2019) 11(6):1–20. doi: 10.3390/cancers11060758
110. Li Y, Chen J, Chen Z, Xu X, Weng J, Zhang Y, et al. Circglis3 promotes high-grade glioma invasion Via modulating ezrin phosphorylation. Front Cell Dev Biol (2021) 9:663207. doi: 10.3389/fcell.2021.663207
111. Wang M, Jia J, Cui Y, Peng Y, Jiang Y. Cd73-positive extracellular vesicles promote glioblastoma immunosuppression by inhibiting T-cell clonal expansion. Cell Death Dis (2021) 12(11):1065. doi: 10.1038/s41419-021-04359-3
112. Zhang Z, Yin J, Lu C, Wei Y, Zeng A, You Y. Exosomal transfer of long non-coding rna Sbf2-As1 enhances chemoresistance to temozolomide in glioblastoma. J Exp Clin Cancer research: CR (2019) 38(1):166. doi: 10.1186/s13046-019-1139-6
113. Mao G, Mu Z, Wu D. Exosome-derived mir-2682-5p suppresses cell viability and migration by Hdac1-Silence-Mediated upregulation of Adh1a in non-small cell lung cancer. Hum Exp Toxicol (2021) 40(12_suppl):S318–s30. doi: 10.1177/09603271211041997
114. Chen SW, Zhu SQ, Pei X, Qiu BQ, Xiong D, Long X, et al. Cancer cell-derived exosomal Circusp7 induces Cd8(+) T cell dysfunction and anti-Pd1 resistance by regulating the mir-934/Shp2 axis in nsclc. Mol Cancer (2021) 20(1):144. doi: 10.1186/s12943-021-01448-x
115. Liu Y, Li L, Song X. Exosomal Circpvt1 derived from lung cancer promotes the progression of lung cancer by targeting mir-124-3p/Ezh2 axis and regulating macrophage polarization. Cell Cycle (Georgetown Tex) (2022) 21(5):514–30. doi: 10.1080/15384101.2021.2024997
116. Hou J, Wang F, Liu X, Song M, Yin X. Tumor-derived exosomes enhance invasion and metastasis of salivary adenoid cystic carcinoma cells. J Oral Pathol medicine: Off Publ Int Assoc Oral Pathologists Am Acad Oral Pathol (2018) 47(2):144–51. doi: 10.1111/jop.12654
117. Ambrosini G, Rai AJ, Carvajal RD, Schwartz GK. Uveal melanoma exosomes induce a prometastatic microenvironment through macrophage migration inhibitory factor. Mol Cancer research: MCR (2022) 20(4):661–9. doi: 10.1158/1541-7786.Mcr-21-0526
118. Chen W, Zuo F, Zhang K, Xia T, Lei W, Zhang Z, et al. Exosomal mif derived from nasopharyngeal carcinoma promotes metastasis by repressing ferroptosis of macrophages. Front Cell Dev Biol (2021) 9:791187. doi: 10.3389/fcell.2021.791187
119. Xia Y, Zhang Q, Zhen Q, Zhao Y, Liu N, Li T, et al. Negative regulation of tumor-infiltrating nk cell in clear cell renal cell carcinoma patients through the exosomal pathway. Oncotarget (2017) 8(23):37783–95. doi: 10.18632/oncotarget.16354
120. Dekker E, Tanis PJ, Vleugels JLA, Kasi PM, Wallace MB. Colorectal cancer. Lancet (London England) (2019) 394(10207):1467–80. doi: 10.1016/s0140-6736(19)32319-0
121. Fu M, Gu J, Jiang P, Qian H, Xu W, Zhang X. Exosomes in gastric cancer: Roles, mechanisms, and applications. Mol Cancer (2019) 18(1):41. doi: 10.1186/s12943-019-1001-7
122. Quail DF, Joyce JA. Microenvironmental regulation of tumor progression and metastasis. Nat Med (2013) 19(11):1423–37. doi: 10.1038/nm.3394
123. Huber V, Fais S, Iero M, Lugini L, Canese P, Squarcina P, et al. Human colorectal cancer cells induce T-cell death through release of proapoptotic microvesicles: Role in immune escape. Gastroenterology (2005) 128(7):1796–804. doi: 10.1053/j.gastro.2005.03.045
124. Guan XW, Zhao F, Wang JY, Wang HY, Ge SH, Wang X, et al. Tumor microenvironment interruption: A novel anti-cancer mechanism of proton-pump inhibitor in gastric cancer by suppressing the release of microrna-carrying exosomes. Am J Cancer Res (2017) 7(9):1913–25.
125. Huang YJ, Huang TH, Yadav VK, Sumitra MR, Tzeng DT, Wei PL, et al. Erratum: Preclinical investigation of ovatodiolide as a potential inhibitor of colon cancer stem cells Via downregulating sphere-derived exosomal B-Catenin/Stat3/Mir-1246 cargoes. Am J Cancer Res (2020) 10(12):4640–2.
126. Wang FW, Cao CH, Han K, Zhao YX, Cai MY, Xiang ZC, et al. Apc-activated long noncoding rna inhibits colorectal carcinoma pathogenesis through reduction of exosome production. J Clin Invest (2019) 129(2):727–43. doi: 10.1172/jci122478
127. Xu D, Tang WJ, Zhu YZ, Liu Z, Yang K, Liang MX, et al. Hyperthermia promotes exosome secretion by regulating Rab7b while increasing drug sensitivity in adriamycin-resistant breast cancer. Int J hyperthermia: Off J Eur Soc Hyperthermic Oncology North Am Hyperthermia Group (2022) 39(1):246–57. doi: 10.1080/02656736.2022.2029585
128. Datta A, Kim H, McGee L, Johnson AE, Talwar S, Marugan J, et al. High-throughput screening identified selective inhibitors of exosome biogenesis and secretion: A drug repurposing strategy for advanced cancer. Sci Rep (2018) 8(1):8161. doi: 10.1038/s41598-018-26411-7
129. Lallemand T, Rouahi M, Swiader A, Grazide MH, Geoffre N, Alayrac P, et al. Nsmase2 (Type 2-neutral sphingomyelinase) deficiency or inhibition by Gw4869 reduces inflammation and atherosclerosis in apoe(-/-) mice. Arteriosclerosis thrombosis Vasc Biol (2018) 38(7):1479–92. doi: 10.1161/atvbaha.118.311208
130. Datta A, Kim H, Lal M, McGee L, Johnson A, Moustafa AA, et al. Manumycin a suppresses exosome biogenesis and secretion Via targeted inhibition of Ras/Raf/Erk1/2 signaling and hnrnp H1 in castration-resistant prostate cancer cells. Cancer Lett (2017) 408:73–81. doi: 10.1016/j.canlet.2017.08.020
131. Aqil F, Gupta RC. Exosomes in cancer therapy. Cancers (2022) 14(3):1–4. doi: 10.3390/cancers14030500
132. Kanchanapally R, Deshmukh SK, Chavva SR, Tyagi N, Srivastava SK, Patel GK, et al. Drug-loaded exosomal preparations from different cell types exhibit distinctive loading capability, yield, and antitumor efficacies: A comparative analysis. Int J nanomedicine (2019) 14:531–41. doi: 10.2147/ijn.S191313
133. Jiang Y, Wang F, Wang K, Zhong Y, Wei X, Wang Q, et al. Engineered exosomes: A promising drug delivery strategy for brain diseases. Curr medicinal Chem (2022) 29(17):3111–24. doi: 10.2174/0929867328666210902142015
134. Pullan JE, Confeld MI, Osborn JK, Kim J, Sarkar K, Mallik S. Exosomes as drug carriers for cancer therapy. Mol pharmaceutics (2019) 16(5):1789–98. doi: 10.1021/acs.molpharmaceut.9b00104
135. Liao W, Du Y, Zhang C, Pan F, Yao Y, Zhang T, et al. Exosomes: The next generation of endogenous nanomaterials for advanced drug delivery and therapy. Acta biomaterialia (2019) 86:1–14. doi: 10.1016/j.actbio.2018.12.045
136. Chen L, Wang L, Zhu L, Xu Z, Liu Y, Li Z, et al. Exosomes as drug carriers in anti-cancer therapy. Front Cell Dev Biol (2022) 10:728616. doi: 10.3389/fcell.2022.728616
137. Agrawal AK, Aqil F, Jeyabalan J, Spencer WA, Beck J, Gachuki BW, et al. Milk-derived exosomes for oral delivery of paclitaxel. Nanomedicine: Nanotechnology biology Med (2017) 13(5):1627–36. doi: 10.1016/j.nano.2017.03.001
138. Nolte-’t Hoen EN, Buschow SI, Anderton SM, Stoorvogel W, Wauben MH. Activated T cells recruit exosomes secreted by dendritic cells Via lfa-1. Blood (2009) 113(9):1977–81. doi: 10.1182/blood-2008-08-174094
139. Rana S, Yue S, Stadel D, Zöller M. Toward tailored exosomes: The exosomal tetraspanin web contributes to target cell selection. Int J Biochem Cell Biol (2012) 44(9):1574–84. doi: 10.1016/j.biocel.2012.06.018
140. Kim H, Yun N, Mun D, Kang JY, Lee SH, Park H, et al. Cardiac-specific delivery by cardiac tissue-targeting peptide-expressing exosomes. Biochem Biophys Res Commun (2018) 499(4):803–8. doi: 10.1016/j.bbrc.2018.03.227
141. Hoshino A, Costa-Silva B, Shen TL, Rodrigues G, Hashimoto A, Tesic Mark M, et al. Tumour exosome integrins determine organotropic metastasis. Nature (2015) 527(7578):329–35. doi: 10.1038/nature15756
142. Cui Y, Guo Y, Kong L, Shi J, Liu P, Li R, et al. A bone-targeted engineered exosome platform delivering sirna to treat osteoporosis. Bioactive materials (2022) 10:207–21. doi: 10.1016/j.bioactmat.2021.09.015
143. Kamerkar S, LeBleu VS, Sugimoto H, Yang S, Ruivo CF, Melo SA, et al. Exosomes facilitate therapeutic targeting of oncogenic kras in pancreatic cancer. Nature (2017) 546(7659):498–503. doi: 10.1038/nature22341
144. Zhang KL, Wang YJ, Sun J, Zhou J, Xing C, Huang G, et al. Artificial chimeric exosomes for anti-phagocytosis and targeted cancer therapy. Chem Sci (2019) 10(5):1555–61. doi: 10.1039/c8sc03224f
145. Qi H, Liu C, Long L, Ren Y, Zhang S, Chang X, et al. Blood exosomes endowed with magnetic and targeting properties for cancer therapy. ACS nano (2016) 10(3):3323–33. doi: 10.1021/acsnano.5b06939
146. Wang D, Wan Z, Yang Q, Chen J, Liu Y, Lu F, et al. Sonodynamical reversion of immunosuppressive microenvironment in prostate cancer Via engineered exosomes. Drug delivery (2022) 29(1):702–13. doi: 10.1080/10717544.2022.2044937
147. Hu Q, Sun W, Wang C, Gu Z. Recent advances of cocktail chemotherapy by combination drug delivery systems. Advanced Drug delivery Rev (2016) 98:19–34. doi: 10.1016/j.addr.2015.10.022
148. Sanjay ST, Zhou W, Dou M, Tavakoli H, Ma L, Xu F, et al. Recent advances of controlled drug delivery using microfluidic platforms. Advanced Drug delivery Rev (2018) 128:3–28. doi: 10.1016/j.addr.2017.09.013
149. Abbina S, Takeuchi LE, Anilkumar P, Yu K, Rogalski JC, Shenoi RA, et al. Blood circulation of soft nanomaterials is governed by dynamic remodeling of protein opsonins at nano-biointerface. Nat Commun (2020) 11(1):3048. doi: 10.1038/s41467-020-16772-x
150. Wu T, Liu Y, Cao Y, Liu Z. Engineering macrophage exosome disguised biodegradable nanoplatform for enhanced sonodynamic therapy of glioblastoma. Advanced materials (Deerfield Beach Fla) (2022) 34(15):e2110364. doi: 10.1002/adma.202110364
151. Aqil F, Munagala R, Jeyabalan J, Agrawal AK, Kyakulaga AH, Wilcher SA, et al. Milk exosomes - natural nanoparticles for sirna delivery. Cancer Lett (2019) 449:186–95. doi: 10.1016/j.canlet.2019.02.011
152. Kim MS, Haney MJ, Zhao Y, Mahajan V, Deygen I, Klyachko NL, et al. Development of exosome-encapsulated paclitaxel to overcome mdr in cancer cells. Nanomedicine: Nanotechnology biology Med (2016) 12(3):655–64. doi: 10.1016/j.nano.2015.10.012
153. Fan Y, Zhou Y, Lu M, Si H, Li L, Tang B. Responsive dual-targeting exosome as a drug carrier for combination cancer immunotherapy. Res (Washington DC) (2021) 2021:9862876. doi: 10.34133/2021/9862876
154. Wang S, Li F, Ye T, Wang J, Lyu C, Qing S, et al. Macrophage-tumor chimeric exosomes accumulate in lymph node and tumor to activate the immune response and the tumor microenvironment. Sci Trans Med (2021) 13(615):eabb6981. doi: 10.1126/scitranslmed.abb6981
155. Yang Y, Li CW, Chan LC, Wei Y, Hsu JM, Xia W, et al. Exosomal pd-L1 harbors active defense function to suppress T cell killing of breast cancer cells and promote tumor growth. Cell Res (2018) 28(8):862–4. doi: 10.1038/s41422-018-0060-4
156. Vautrot V, Bentayeb H, Causse S, Garrido C, Gobbo J. Tumor-derived exosomes: Hidden players in pd-1/Pd-L1 resistance. Cancers (2021) 13(18):1–21. doi: 10.3390/cancers13184537
157. Ostrowski M, Carmo NB, Krumeich S, Fanget I, Raposo G, Savina A, et al. Rab27a and Rab27b control different steps of the exosome secretion pathway. Nat Cell Biol (2010) 12(1):19–30. doi: 10.1038/ncb2000
158. Shen DD, Pang JR, Bi YP, Zhao LF, Li YR, Zhao LJ, et al. Lsd1 deletion decreases exosomal pd-L1 and restores T-cell response in gastric cancer. Mol Cancer (2022) 21(1):75. doi: 10.1186/s12943-022-01557-1
159. Marleau AM, Chen CS, Joyce JA, Tullis RH. Exosome removal as a therapeutic adjuvant in cancer. J Trans Med (2012) 10:134. doi: 10.1186/1479-5876-10-134
160. Shin JM, Lee CH, Son S, Kim CH, Lee JA, Ko H, et al. Sulfisoxazole elicits robust antitumour immune response along with immune checkpoint therapy by inhibiting exosomal pd-L1. Advanced Sci (Weinheim Baden-Wurttemberg Germany) (2022) 9(5):e2103245. doi: 10.1002/advs.202103245
161. André F, Chaput N, Schartz NE, Flament C, Aubert N, Bernard J, et al. Exosomes as potent cell-free peptide-based vaccine. i. dendritic cell-derived exosomes transfer functional mhc class I/Peptide complexes to dendritic cells. J Immunol (Baltimore Md: 1950) (2004) 172(4):2126–36. doi: 10.4049/jimmunol.172.4.2126
162. Pitt JM, André F, Amigorena S, Soria JC, Eggermont A, Kroemer G, et al. Dendritic cell-derived exosomes for cancer therapy. J Clin Invest (2016) 126(4):1224–32. doi: 10.1172/jci81137
163. Yao Y, Fu C, Zhou L, Mi QS, Jiang A. Dc-derived exosomes for cancer immunotherapy. Cancers (2021) 13(15):1–19. doi: 10.3390/cancers13153667
164. Altieri SL, Khan AN, Tomasi TB. Exosomes from plasmacytoma cells as a tumor vaccine. J immunotherapy (Hagerstown Md: 1997) (2004) 27(4):282–8. doi: 10.1097/00002371-200407000-00004
165. Fu C, Zhou L, Mi QS, Jiang A. Dc-based vaccines for cancer immunotherapy. Vaccines (2020) 8(4):1–16. doi: 10.3390/vaccines8040706
166. Fu C, Zhou L, Mi QS, Jiang A. Plasmacytoid dendritic cells and cancer immunotherapy. Cells (2022) 11(2):1–15. doi: 10.3390/cells11020222
167. Yildirim M, Yildirim TC, Turay N, Bildik T, Ibibik B, Evcili I, et al. Tlr ligand loaded exosome mediated immunotherapy of established mammary tumor in mice. Immunol Lett (2021) 239:32–41. doi: 10.1016/j.imlet.2021.08.004
168. Zhou W, Chen X, Zhou Y, Shi S, Liang C, Yu X, et al. Exosomes derived from immunogenically dying tumor cells as a versatile tool for vaccination against pancreatic cancer. Biomaterials (2022) 280:121306. doi: 10.1016/j.biomaterials.2021.121306
169. Cheng L, Wang Y, Huang L. Exosomes from M1-polarized macrophages potentiate the cancer vaccine by creating a pro-inflammatory microenvironment in the lymph node. Mol therapy: J Am Soc Gene Ther (2017) 25(7):1665–75. doi: 10.1016/j.ymthe.2017.02.007
170. Lv F, Liu H, Zhao G, Zhao E, Yan H, Che R, et al. Therapeutic exosomal vaccine for enhanced cancer immunotherapy by mediating tumor microenvironment. iScience (2022) 25(1):103639. doi: 10.1016/j.isci.2021.103639
171. Yaddanapudi K, Meng S, Whitt AG, Al Rayyan N, Richie J, Tu A, et al. Exosomes from gm-csf expressing embryonic stem cells are an effective prophylactic vaccine for cancer prevention. Oncoimmunology (2019) 8(3):1561119. doi: 10.1080/2162402x.2018.1561119
172. Huang L, Rong Y, Tang X, Yi K, Qi P, Hou J, et al. Engineered exosomes as an in situ dc-primed vaccine to boost antitumor immunity in breast cancer. Mol Cancer (2022) 21(1):45. doi: 10.1186/s12943-022-01515-x
173. Phung CD, Pham TT, Nguyen HT, Nguyen TT, Ou W, Jeong JH, et al. Anti-Ctla-4 antibody-functionalized dendritic cell-derived exosomes targeting tumor-draining lymph nodes for effective induction of antitumor T-cell responses. Acta biomaterialia (2020) 115:371–82. doi: 10.1016/j.actbio.2020.08.008
174. Alipoor SD, Mortaz E, Varahram M, Movassaghi M, Kraneveld AD, Garssen J, et al. The potential biomarkers and immunological effects of tumor-derived exosomes in lung cancer. Front Immunol (2018) 9:819. doi: 10.3389/fimmu.2018.00819
175. Chen W, Li H, Su W, Qin J. Microfluidic device for on-chip isolation and detection of circulating exosomes in blood of breast cancer patients. Biomicrofluidics (2019) 13(5):054113. doi: 10.1063/1.5110973
176. Thakur A, Qiu G, Ng SP, Guan J, Yue J, Lee Y, et al. Direct detection of two different tumor-derived extracellular vesicles by Sam-aunis lspr biosensor. Biosensors bioelectronics (2017) 94:400–7. doi: 10.1016/j.bios.2017.03.036
177. Kahlert C, Melo SA, Protopopov A, Tang J, Seth S, Koch M, et al. Identification of double-stranded genomic DNA spanning all chromosomes with mutated kras and P53 DNA in the serum exosomes of patients with pancreatic cancer. J Biol Chem (2014) 289(7):3869–75. doi: 10.1074/jbc.C113.532267
178. Machala M, Slavík J, Kováč O, Procházková J, Pěnčíková K, Pařenicová M, et al. Changes in sphingolipid profile of Benzo[a]Pyrene-transformed human bronchial epithelial cells are reflected in the altered composition of sphingolipids in their exosomes. Int J Mol Sci (2021) 22(17):1–17. doi: 10.3390/ijms22179195
179. Czystowska-Kuzmicz M, Sosnowska A, Nowis D, Ramji K, Szajnik M, Chlebowska-Tuz J, et al. Small extracellular vesicles containing arginase-1 suppress T-cell responses and promote tumor growth in ovarian carcinoma. Nat Commun (2019) 10(1):3000. doi: 10.1038/s41467-019-10979-3
Keywords: extracellular vesicle, exosome, tumor immune microenvironment, gastrointestinal cancer, immunotherapy
Citation: Cao Y, Xu P, Shen Y, Wu W, Chen M, Wang F, Zhu Y, Yan F, Gu W and Lin Y (2023) Exosomes and cancer immunotherapy: A review of recent cancer research. Front. Oncol. 12:1118101. doi: 10.3389/fonc.2022.1118101
Received: 07 December 2022; Accepted: 29 December 2022;
Published: 16 January 2023.
Edited by:
Li Liang, National Center for Liver Cancer, ChinaReviewed by:
Yanping Wei, Eastern Hepatobiliary Surgery Hospital, ChinaCopyright © 2023 Cao, Xu, Shen, Wu, Chen, Wang, Zhu, Yan, Gu and Lin. This is an open-access article distributed under the terms of the Creative Commons Attribution License (CC BY). The use, distribution or reproduction in other forums is permitted, provided the original author(s) and the copyright owner(s) are credited and that the original publication in this journal is cited, in accordance with accepted academic practice. No use, distribution or reproduction is permitted which does not comply with these terms.
*Correspondence: Yan Lin, dGhlbG1hLWxpbnlhbkAxNjMuY29t; Weiying Gu, Z3V3ZWl5aW5nMjAxN0AxMjYuY29t
†These authors have contributed equally to this work and share first authorship
‡These authors have contributed equally to this work
Disclaimer: All claims expressed in this article are solely those of the authors and do not necessarily represent those of their affiliated organizations, or those of the publisher, the editors and the reviewers. Any product that may be evaluated in this article or claim that may be made by its manufacturer is not guaranteed or endorsed by the publisher.
Research integrity at Frontiers
Learn more about the work of our research integrity team to safeguard the quality of each article we publish.