- 1Laboratory of translational cellular and molecular biomedicine, National Research Tomsk State University, Tomsk, Russia
- 2Cancer Research Institute, Tomsk National Research Medical Center, Russian Academy of Sciences, Tomsk, Russia
- 3Laboratory of Genetic Technologies, Siberian State Medical University, Tomsk, Russia
- 4Institute of Transfusion Medicine and Immunology, Mannheim Institute for Innate Immunoscience (MI3), Medical Faculty Mannheim, University of Heidelberg, Mannheim, Germany
- 5German Red Cross Blood Service Baden-Württemberg – Hessen, Mannheim, Germany
Tumor-associated macrophages (TAMs) are a heterogeneous population of myeloid cells that constitute up to 50% of the cell mass of human tumors. TAMs interact with the components of the tumor microenvironment (TME) by using scavenger receptors (SRs), a large superfamily of multifunctional receptors that recognize, internalize and transport to the endosomal/lysosomal pathway apoptotic cells, cytokines, matrix molecules, lipid modified lipoproteins and other unwanted-self ligands. In our review, we summarized state-of-the art for the role of macrophage scavenger receptors in tumor development and their significance as cancer biomarkers. In this review we focused on functional activity of TAM-expressing SRs in animal models and in patients, and summarized the data for different human cancer types about the prognostic significance of TAM-expressed SRs. We discussed the role of SRs in the regulation of cancer cell biology, cell-cell and cell-matrix interaction in TME, immune status in TME, angiogenesis, and intratumoral metabolism. Targeting of tumor-promoting SRs can be a promising therapeutic approach in anti-cancer therapy. In our review we provide evidence for both tumor supporting and tumor inhibiting functions of scavenger receptors expressed on TAMs. We focused on the key differences in the prognostic and functional roles of SRs that are specific for cancer types. We highlighted perspectives for inhibition of tumor-promoting SRs in anti-cancer therapy.
1. Introduction
Tumor-associated macrophages (TAMs) are key innate immune cells that control intratumoral inflammation, cancer cell proliferation, migration and metabolism, angiogenesis, and extracellular matrix composition (1–6). Major sources of TAMs are resident tissue macrophages as well as monocyte-derived macrophages, intensively recruited into the growing tumor by chemotactic factors, like CCL2 (4, 7, 8) The are two major vectors of macrophage polarization: M1-type, classically activated, pro-inflammatory, and M2-type, alternatively activated, generally considered as anti-inflammatory or tolerogenic macrophages (4–6). The classification based on the M1/M2 dichotomy is traditionally used as simplified schema to distinguish between two major directions of macrophage activity. M1 macrophages play an important role in the innate immune response, while M2 macrophages are involved in tissue repair, as well as in the progression of many types of cancer (9, 10).
Within tumor tissues, TAMs interact with cancer cells and with other cell types in tumor microenvironment (TME) not only by secreting different cytokines, chemokines and growth factors, but also by clearance of dying cells, soluble mediators and matrix components mediated by scavenger receptors (SRs). SRs can recognize and internalize high range of unwanted-self ligands including cytokines, growth factors, modified lipoproteins, apoptotic cells, as well as non-self ligands including bacteria, viruses and fungi (11–13).
SRs are large superfamily of transmembrane proteins with high structural diversity (13–15). SRs are categorized into classes A-L depending on their structure, cell-type specific expression and recognition of host-derived ligands (13, 16, 17). Functional diversity of SRs are crucial for numerous biological processes such as endocytosis, phagocytosis, cell adhesion, nutrient exchange and waste clearance, as well as immunity processes, e.g. inflammation regulation and antigen presentation (13, 14).
In tumors, SRs can be expressed by both tumor cells (TCs) and by components of the TME including macrophages, monocytes, endothelial cells and dendritic cells (15, 18, 19). Most commonly used SRs for the identification of TAM subpopulation in different types of tumors include CD68, CD163, CD204 and CD206 (8). Both tumoricidal M1 and tumor-promoting M2 macrophages express CD68, while M2 polarization can be identified by CD163, CD204 and CD206 biomarkers (8). However, this nomenclature does not fully reflect all phenotypic diversity of TAMs that can combine M1 and M2 features and functions (3).
In this review, we focus on functional activity of scavenger receptors expressed by TAMs in tumor. We summarize the latest knowledge on the functional activity of TAM-expressing scavenger receptors in the TME. We discuss how expression of scavenger receptors in TAMs can be used for the evaluation of prognostic value in numerous cancers.
2. Scavenger receptors expressed by TAMs
Several SRs play essential role in the regulation of TME where they can be expressed by TAMs, NK cells, dendritic cells, neutrophils, B cells, endothelial cells, epithelial cells and cancer cells (13). TAM-expressing scavenger receptors are structurally heterogeneous proteins that consist out of diverse structural domains including collagenouse domain, C-type lectin-like domain, fibronectin domain, EGF-like and others (Figure 1). SRs expressed by TAMs are involved in diverse signaling pathway and have a predictive value for tumor progression (Table 1) (23, 25–27, 29, 30, 48).
2.1. Class A scavenger receptors
Scavenger receptors of class A (SR-A) are transmembrane proteins containing a collagen-like domain with collagen-binding activity (15). SR-A family comprises five members: SR-A1 (CD204), SR-A3, SR-A4, SR-A5, and SR-A6 (MARCO), which recognize a variety of ligands such as LPS, LTA and integrins. SR-A are implicated in several pathologies including atherosclerosis, infectious diseases and cancer (13, 70, 71). In most of studies the question about tumor-specific ligand of CD204 was not addressed experimentally. The expression of SR-A was found on monocytes, macrophages, dendritic cells (DCs), mast cells and endothelial cells (71, 72). Out of five SR-A family members, only CD204 and MARCO have been found to be expressed by TAMs (21, 22, 25).
2.1.1. SR-A1/CD204
SR-A1 (also known as CD204, or MSR1) is a pattern recognition receptor expressed primarily on macrophages, and is involved in the inflammatory responses and tumorigenesis (21, 25, 73). CD204 has a dual role in cancer progression (21, 22, 26, 27, 74).
The mechanisms of CD204 anti-tumor activity in TME include inhibition of macrophage infiltration, inhibition of tumor cell migration and invasion, as well as suppression of tumor angiogenesis (21, 22) (Figure 2). In a mouse model of LLC, bone marrow-derived cells transplanted from CD204 KO (knock-out) mice into WT mice enhanced tumor growth and angiogenesis through elevated COX-2, SDF1, VEGF and MMP9 expression in tumor (21). CD204 deficiency activated recruitment of CD68+ and F4/80+ macrophages into tumor mass by upregulation of MCP-1 in the CD204 KO bone marrow transplantation model (22). Peritoneal macrophages isolated out of CD204 KO mice significantly enhanced the migration and invasion of lung cancer cells in vitro (22). Moreover, CD204 suppresses tumor development through the upregulation of serum amyloid A1 (SAA1) expression in TAMs via JNK/ERK/IκB/NFκB signaling pathways (22). CD204 deficiency promoted tumor growth, angiogenesis and TAM infiltration via skewing TAM phenotype toward M2 in murine glioma model (25). Tumor volume as well as the expression of angiogenic factors CD31, CD34, IB4 and VEGF were significantly elevated in CD204−/− mice in comparison with CD204+/+ mice in glioma model (25). In CD204−/− glioma, the number of VCAM1+ TAMs and CCR2+ TAM precursor cells was significantly elevated compared to CD204+/+ glioma (25). In vitro, CD204 deficiency resulted in the increased expression of M2-like markers (MMP2, TGFβ, MRC2, MGL1, FIZZ1), but no M1-like marker (TNFα) in the presence of GL261 glioma cells (25). Meta-analysis of patients with prostate cancer showed that increased expression of CD204 significantly correlated with better recurrence-free survival (RFS) (23). Immunohistochemical (IHC) analysis demonstrated that high expression of CD204 correlated with better survival rate and less recurrence than those with less CD204 expression in patients with glioma (25).
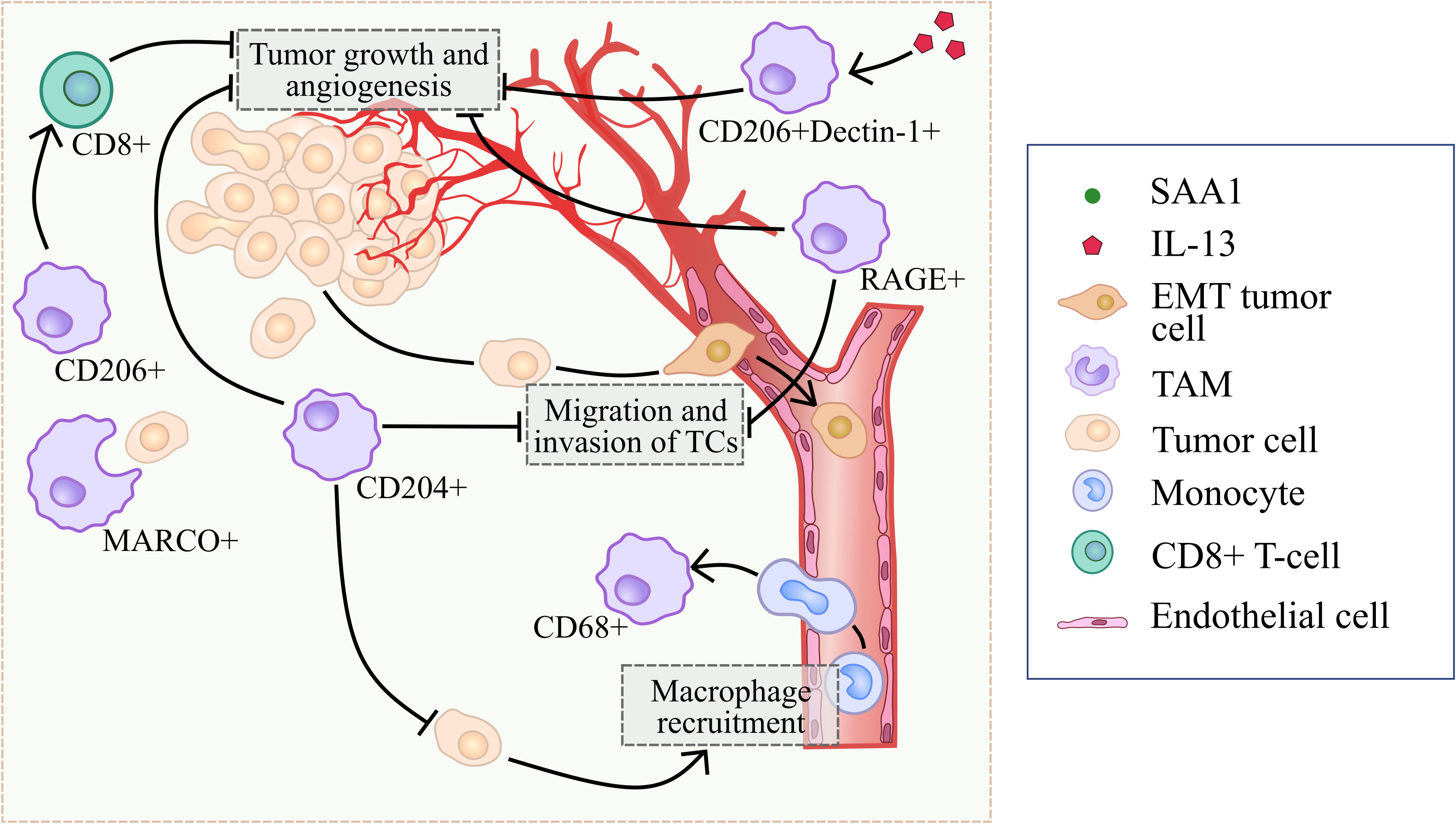
Figure 2 Summary of processes that are regulated by scavenger receptors in tumor-suppressing microenvironment.
However, controversial data also exist (Figure 3). Several studies demonstrated that CD204+TAMs promote tumor development and correlate with worse prognoses in prostate cancer, lung cancer, colorectal cancer, cervical cancer, breast cancers and oral squamous cell carcinoma patients (24, 26, 27, 74–76). Tumor-supporting function of CD204+ TAMs was demonstrated for lung cancer and glioma, however anti-tumor function was shown for breast cancer, ovarian cancer and pancreatic cancer (21, 24, 25, 27, 74–76). CIBERSORT analysis of CD204 mRNA expression obtained from TCGA database demonstrated that high CD204 expression correlated to high proportions of M2 macrophages and the expression of immunosuppressive molecules, including HIF1A, FAP, IL-10, and TGFB1 in breast cancer (27). CD204 KO macrophages reduced tumor cell invasion via TLR-dependent pathways upon co-culture with ID8 (ovarian cancer cell line) and Panc02 (pancreatic adenocarcinoma cell line) (26). Macrophage-specific loss of CD204 significantly reduced lung metastasis in a mouse model of pancreatic adenocarcinoma (26). In vitro CD204+ TAMs promoted proliferation, migration and invasion of MCF7, T47D, SKBR3 and MDA-MB-231 breast cancer cell lines (27). High CD204 expression in TAMs correlates with short overall survival (OS), disease-free survival (DFS) and RFS in colorectal cancer, cervical cancer, breast cancers and oral squamous cell carcinoma (OSCC) (24, 74–76). CD204 expression is associated with T stage, nodal involvement, lymphovascular invasion and tumor relapse after surgery in lung adenocarcinoma (25, 77). In prostate cancer, high CD204 protein expression in the main tumor area predicted a worse prognosis, while CD204 expression in seminal vesicle invasion area was positively associated with the biochemical recurrence (78).
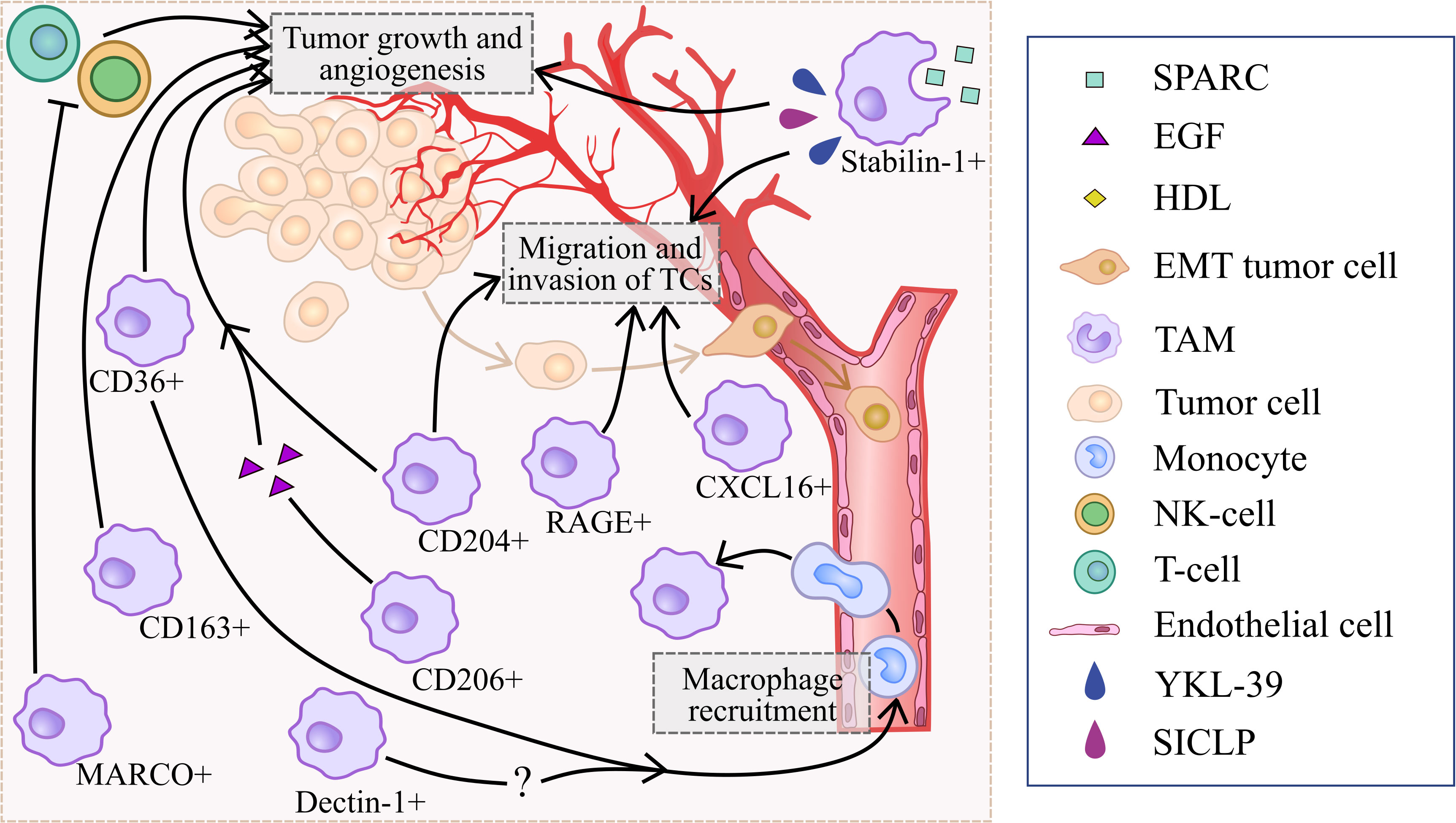
Figure 3 Summary of processes that are regulated by scavenger receptors in tumor-supporting microenvironment.
In summary, majority of reports show that CD204 correlates with good prognosis in prostate cancer and glioma, and with worse prognosis in colorectal cancer, cervical cancer, breast cancers, oral squamous cell carcinoma, lung cancer and prostate cancers. Murine experimental systems demonstrated both tumor-promoting and tumor-inhibiting role of CD204+ TAMs (Figures 2, 3).
2.1.2. SR-A1/CD204
SR-A6 (also known as macrophage receptor with collagenous structure, MARCO) is another member of the SR-A family that is also expressed by macrophages and is involved in clearance of cancer cells, in regulation of epithelial-mesenchymal transition (EMT), in the interferon-alpha response, and in antigen presentation (28, 79).
In vitro, TAMs suppress tumor development utilizing MARCO to phagocytose cancer cells (Figure 2) (28). MARCO overexpression in peritoneal murine macrophages led to the increased expression of SYK, PI3K and Rac-1, and facilitated macrophage-mediated phagocytosis of SL4 (colon carcinoma cell line) via binding to integrin β5 on cancer cells and activation of SYK-PI3K-Rac1 signaling pathway in TAMs in the co-culture system (28) (Figure 4).
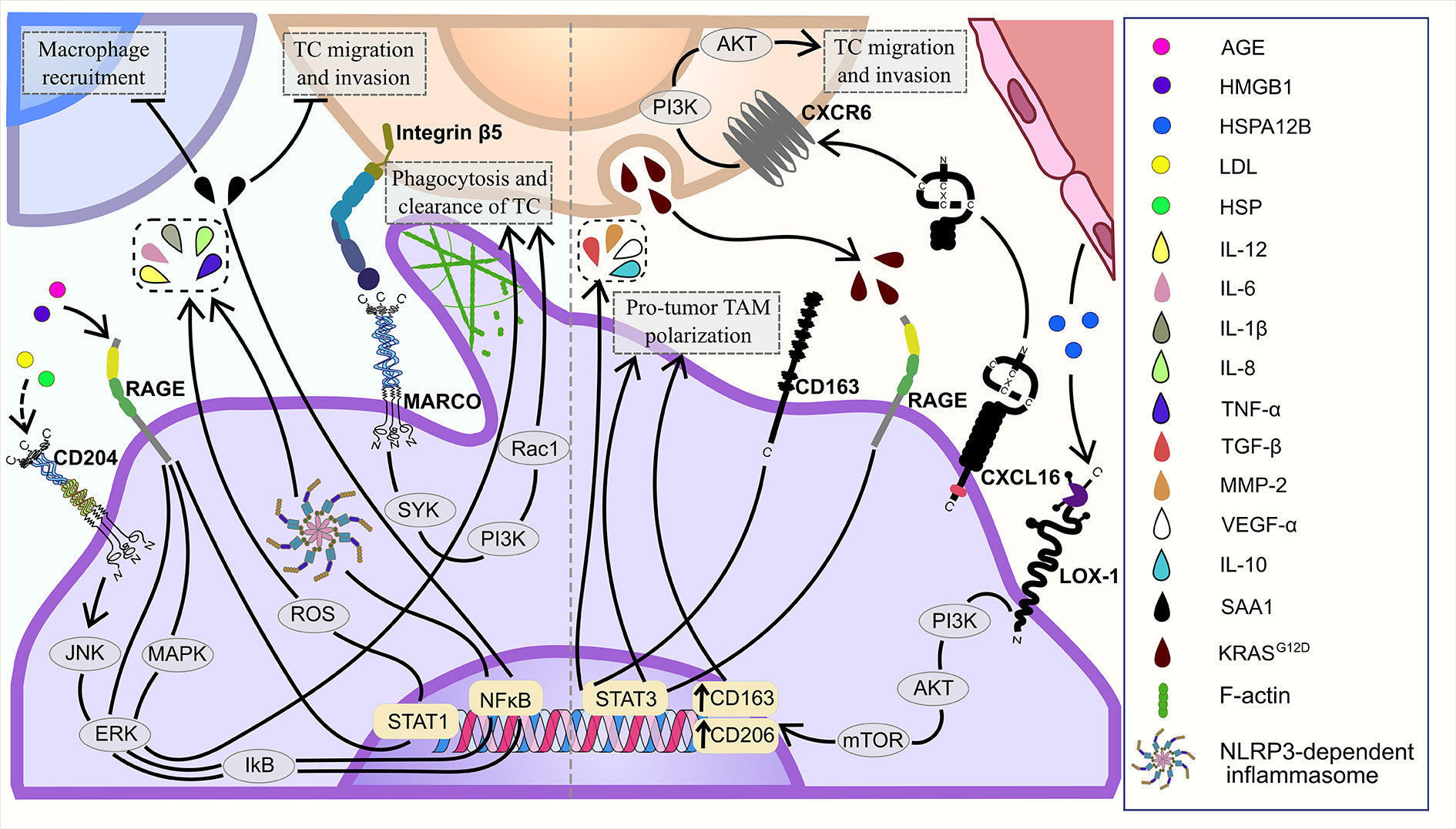
Figure 4 Major signaling pathways of TAM-expressing scavenger receptors in the TME: tumor-supporting and tumor-inhibiting.
Several studies on clinical material revealed tumor-supporting phenotype of MARCO-expressing TAMs (30, 32, 33). Single-cell transcriptomic analysis of glioblastoma demonstrated that a cluster of MARCO+ TAMs coincides with high expression of genes involved in epithelial-mesenchymal transition, angiogenesis, glycolysis, hypoxia and low expression of genes associated with interferon-alpha response, interferon-gamma response, allograft rejection, and TNFα signaling (32). MARCO+ TAMs support tumorigenesis by activating immunosuppression in the TME. Transcriptomic analysis of non-small cell lung cancer (NSCLC) samples showed that MARCO expression significantly correlated with gene expression of immunosuppressive TAM-related genes (CD163, MSR1, IL4R, CHIA, TGFB1, and IL10), genes involved in T-cell regulation (FOXP3, TGFB1, IL10, EBI3, PDCD1, and CTLA4) and genes encoding immune checkpoint molecules (PD-L1, PD-L1, VISTA, PD-1, and CTLA4) (30). High infiltration of MARCO+ TAMs in tumor was associated with worse OS and DFS in patients with squamous cell carcinoma (30) and pancreatic cancer (29).
MARCO-expressing TAMs suppressed tumoricidal activity of T cells and NK cells through skewing TAM phenotype toward anti-inflammatory one (Figure 3). In vitro MARCO-expressing TAMs suppressed activation, proliferation and IFNγ production in T cells, resulted in inhibition of T-cell killing activity towards NSCLC tumor cells (33). Moreover, human PBMC-derived MARCO+ TAMs inhibited migration, degranulation, proliferation and IFNγ production in NK cells (33). MARCO+ macrophages cultured with lung cancer cell lines displayed decreased expression of pro-inflammatory cytokines (TNFa, IL1B and IL12B) and increased expression of anti-inflammatory molecules (IL10, MRC1, COX2, TIMP1, and FIZZ1) (33). High expression of MARCO in TAMs from the tumor tissues was associated with increased OS in patients with hepatocellular carcinoma (HCC) (31).
Thus, role of SR-A family members depends on the tumor context. Despite strong tumor-supporting activity identified for several members of SR-A family expressed by TAMs (26, 27, 30, 32, 33), solid body of evidence is available for SR-A1 and SR-A6 demonstrating their anti-tumor action that depends primarily on the cancer types (20–22, 28) (Figure 3, Table 1).
2.2. B scavenger receptors
Class B scavenger receptors includes the following members: SR-B1, SR-B2 and SR-B3. Structurally Class B scavenger receptors are constructed out of two transmembrane domains flanking an extracellular loop, with both the N- and C-termini located within the cytoplasm (80). Class B scavenger receptors mediate transport of cholesterol and lipids, and are involved in tumor development (34, 35, 81). The role of TAMs expressing SR-B1 and SR-B3 was demonstrated in several types of cancers, including liposarcoma, nasopharyngeal carcinoma, breast cancer, colon cancer and prostate cancer (35, 36, 82).
2.2.1. SR-B1
Scavenger Receptor Class B Type 1 (SR-B1) is a transmembrane protein that act as a major high-density lipoprotein (HDL) receptor (81, 82). SR-B1 is expressed by endothelial cells, smooth muscle cells, keratinocytes, adipocytes, tumor cells and macrophages (19). In the TME, SR-B1 participates in HDL metabolism and promotes invasion, proliferation and metastasis of tumor cells (82). In macrophages, SR-B1 regulates cholesterol metabolism through selective uptake of HDL-cholesterol and cholesteryl esters (83). In a syngeneic mouse model of prostate cancer, knock out of SR-B1 inhibited HDL-mediated tumor growth and progression (84). In SR-B1−/− mice had lower levels of total cholesterol and HDL-cholesterol. SR-B1−/− mice developed smaller tumor compared to SR-B1+/+ mice, and SR-B1−/− mice showed also the decreased survival (84). Application of HDL-mimetic nanoparticles that interacted with SR-B1 reduced tumor growth in a mouse xenograft model for human nasopharyngeal carcinoma (82). Thus, the selective uptake of HDL-cholesterol by SR-B1 in macrophages is a promising pathway for pharmacological inhibition of pro-tumor TAM actions. SR-B1 activity in macrophages is mediated by Src/PI3K/Akt/Rac1 and PPARγ/LXRα signaling pathways (85, 86). The data about the role of TAM-expressing SR-B1 in cancer are limited, but SR-B1 expression was found in head and neck cancer, lung cancer, prostate cancer and breast cancer, where it positively correlates with the tumor aggressiveness and poor prognosis (82, 87, 88).
2.2.2. SR-B3/CD36
SR-B3 (also known as CD36) is expressed on monocytes, macrophages, platelets, endothelial cells, adipocytes (89, 90). CD36 mediates lipid uptake, ligand, clearance of apoptotic cells and cell-cell adhesion (90–92). CD36-expressing macrophages facilitate tumor progression, pro-tumor TAM polarization and mediate fatty acid uptake from TME (Figure 3) (35, 36, 93).
CD36 regulates polarization of TAMs towards pro-tumor phenotype and promotes tumor growth via regulation of fatty acid (FA) metabolism (94, 95). CD36 was demonstrated as a major SR on macrophages involved in the lipid uptake and accumulation, FA oxidation and oxidative phosphorylation (94, 95). In TME extracellular free fatty acids, including palmitic acid, oxLDL or oleic acid, are transported into cells via membrane-associated CD36 and promoted tumor growth and metastasis (94–97). Essential feature of CD36 is that its endocytic function is linked to the inflammatory pathways. In macrophages CD36 is involved in diverse signaling cascade including NF-κB pathway, TLR1/2 signaling, TLR4 signaling and NOD-, LRR-, and pyrin domain-containing protein inflammasome pathways (98).
In co-culture of human PBMCs and tumor cells CD36 regulates macrophage response by enhanced lipid uptake and increased expression of pro-tumor genes in modeled TAMs (Arg1, Ccl2) (34). Subcutaneously injection of CD36-KO TAMs in a mouse model of lymphoma decreased tumor volume, impaired TAMs infiltration into tumor site, increased expression of M1-signature genes and decreased expression of M2-signature genes (34). CD36 in TAMs mediates FA uptake through S100A4-PPAR-γ axis that promotes tumor growth in a mouse models of breast cancer and fibrosarcoma (35). In the mouse model of breast cancer CD36 regulates TME via clearance of tumor-derived miR-375, a prominent tumor suppressor (36, 93). In co-culture system of MCF-7 cells and human PBMCs, apoptotic tumor cell-derived miR-375 binds to LDL and is scavenged by TAMs via CD36 receptor resulting in increased macrophage migration and infiltration into tumor (36).
Association of CD36 with worse prognosis was demonstrated in several human cancers including bladder cancer, glioblastoma, oral carcinoma and gastric cancer (97, 99). However, currently prognostic significance of CD36 expressed specifically on TAMs is still unclear.
2.3. Class D scavenger receptors
Scavenger receptor SR-D1 (also known as CD68) is the only known class D scavenger receptor that is highly specifically expressed on macrophages and other mononuclear phagocytes but not on other cell types, even of myeloid origin. CD68 is the major biomarker for the quantification of total TAM amounts. CD68 is also a well-established pan-macrophage marker used as a cancer-associated diagnostic and prognostic marker (8, 13).
CD68+TAM infiltration and accumulation in tumor results in tumor progression and adverse prognosis in numerous cancers (8, 37, 38). In our recent review we have summarized data from large number of studies on patients with 5 types of cancer: breast, colorectal, lung, ovarian and prostate (9). Number of studies on patients representing diverse genetics, life style and geographical localizations indicate that amount of intramural CD68+TAMs positively correlates with negative prognosis, distant hematogenous and local lymphatic metastasis in breast, lung, ovarian and prostate cancers. However, amount of intramural CD68+TAMs showed negative correlation with the bad outcome in patients with colorectal cancer. Recent analysis of Genotype-Tissue Expression datasets (TCGA) and immunohistology have demonstrated that high expression of CD68 was correlated with worse prognosis in glioblastoma, renal clear cell carcinoma, lower-grade glioma, HCC, lung squamous cell carcinoma, thyroid carcinoma, and thymoma, but with favorable prognosis in chromophobe renal cell carcinoma (38). In laryngeal squamous cell carcinoma (LSCC), CD68+ cells were involved in angiogenesis and correlated with worse prognosis (37). High expression of CD68 was associated with CD34+ cells in tumor and low 5-year DFS in 45 patients with LSCC from China (37). High amounts of CD68+ TAMs in tumor nest correlated with recurrence in 184 cutaneous melanoma patients from Finland (39). Number of CD68+ TAMs in tumor stroma were positively correlated with tumor size in breast cancer both in 144 patients from Sweden and in 60 patients from Egypt (100, 101). Controversially, in melanoma, CD68+ TAMs characterized by M1 phenotype, however, not statistically significant correlations were found for the total amount of CD68+TAMs and clinical parameters of melanoma progression in patients (41). RNA-seq and IHC analysis of 57 human melanoma samples showed that CD68+ TAMs were associated with increased iNOS and arginase expression (41). In human osteosarcoma, elevated expression levels of macrophage and CD4 T-cell markers (defined as CD4/IFNGR2/CD68/CSF1R signature) was associated with favorable neoadjuvant chemotherapy responses (40).
In several independent studies amount of intratumoral CD68+ TAMs were indicative for reduced tumor growth and better prognosis (8). CD68+ macrophage infiltrates correlated with better RFS in 468 patients with ER-negative tumors from Scotland (102). In a Norway study of 553 primary NSCLCs CD68+ expression correlated with favorable NSCLC-specific survival (103). Correlation of high expression of CD68 with favorable prognosis was also demonstrated in colorectal cancer (104, 105). Thus, total amount of CD68+ TAMs is a potential prognostic biomarker that can predict negative scenario in progression for majority of human cancer types, however opposite correlations were identified for specific cancer types, in particular colorectal cancer, raising urgent question about intrinsic anti-tumor activates of TAMs that cannot be converted by growing tumor. However, functional role of CD68 in inflammation and carcinogenesis is not sufficiently understood despite its routine application as an immunochemical marker of TAMs.
2.4. Class E scavenger receptors
The class E of scavenger receptors comprises SR-E1, SR-E2, SR-E3 and SR-E4 members (13). The class E SRs belong to a subfamily of NK cell C-type lectin-like (CLEC) receptor family that plays role in diverse biological processes such as immune response, antigen presentation and phagocytosis (13). Several members of the Class E SR family are expressed in TAMs and involved in tumor progression (42, 43).
2.4.1. SR-E1/LOX-1
SR-E1 (also known as LOX-1) is mainly expressed by endothelial cells, but is also found on smooth muscle cells, cardiomiocytes, adipocytes, platelets and on TAMs (106). LOX-1 participates in multiple physiological and pathological processes, including lipid metabolism, cholesterol biosynthesis and tumorigenesis (107, 108). In tumors, LOX-1 regulates macrophage polarization (43). Correlation analysis of TCGA data, single-cell RNA-seq data and in vitro models showed, that TAMs increased the uptake of heat shock protein HSPA12B by LOX-1 that resulted in the activation of PI3K/Akt/mTOR signaling and enhanced M2-type marker expression (CD163 and CD206) in TAMs in head and neck squamous cell carcinoma (HNSC) (43) (Figure 4). At the moment, the detailed mechanism of LOX-1+ TAM activity in the TME is not well defined, but the prognostic value of LOX-1+ TAMs was found in colorectal cancer (42). IHC analysis demonstrated that low expression on TAMs was associated with poor OS in patients with colorectal cancer (42).
2.4.2. SR-E2/Dectin-1
SR-E2 (also known as Dectin-1) is a C-type lectin receptor that is involved in large number of biological processes such as phagocytosis, activation of signaling pathways, generation of reactive oxygen species (ROS) and production of cytokines (109). Dectin-1 is encoded by Clec7a gene and primary expressed on the surface of the myeloid-monocytic lineage cells including macrophages, but can be also found on neutrophils, dendritic cells, and on a minor subpopulation of splenic T cells (46, 110). Dectin-1 is an innate immune receptor playing role in anti-fungal immune response. In cancer, dectin-1 regulates immune microenvironment and has an ambiguous function in tumor progression (44, 46, 111).
IL-13-activated macrophages expressing both dectin-1 and mannose receptor (MR) inhibited T-cell lymphoma and ovarian adenocarcinoma progression via binding to tumoral sialic acid (44). Dectin-1 and MR interaction of with sialic acid enhanced antitumor effect of IL-13- activated macrophages in vitro (44). Depletion of dectin-1 and MR in IL-13-activated macrophages resulted in inhibition of TAM tumoricidal activity and decrease in death of Jurkat (human T-cell leukemia cell line) and EL4 (murine T-lymphoma cell line) tumor cells (44).
Dectin-1 promotes tumor progression via the regulation of immune microenvironment of human OSCC (111). Dectin-1 deficiency decreased the amount of IL-1β+ cells, Tregs, MDSC cells and PD-1 induction in CD8+ T cells resulted in slower dysplasia progression and lower number and size of tumors in mouse model of OSCC (111). Dectin-1 promotes pancreatic ductal adenocarcinoma (PDA) progression by enhanced TAM infiltration and by reprogramming TAMs towards M2 phenotype (46). In a mouse model of PDA, Clec7a deletion significantly reduced the infiltration of PDA with F4/80+, CD206+ and Arg1+ TAMs, as well as upregulated MHCII, TNF-α and iNOS expression in tumor (46). Moreover, depletion of Clec7a in macrophages in vivo elevated infiltration by CD4+ and CD8+ T cells selectively in wt hosts, but not in Clec7a−/− hosts, indicating that dectin-1-expressing macrophages drive T cell suppression in PDA (46). In renal cell carcinoma, high expression of tumor cell-derived but not TAM-derived dectin-1 was associated with shorter RFS and OS (45).
2.4.3. SR-E3/CD206
Scavenger receptor SR-E3 (also known as CD206) is a C-type lectin that mediates antigen presentation, endocytosis, phagocytosis and immune homeostasis (112, 113). It is commonly accepted that CD206 is a marker of tumor-supporting M2 phenotype of TAMs, but recent studies demonstrated controversial activity of CD206+ TAMs in tumor (47–49).
CD206+ TAMs produced EGF to promote OSCC progression in vitro (49). Proliferation and invasion of OSCC cells cultured with conditioned medium of CD206+ TAMs were strongly enhanced by EGF (49). CD206 mediated breast cancer post-chemotherapy progression (48). In mouse model of breast cancer high expression of CD206+F4/80+ TAMs was associated with tumor relapse and lymph node metastasis after cyclophosphamide treatment (48). Number of CD206+ TAMs positively correlated with worse clinical prognosis in OSCC, CRC, lung cancer (49, 114, 115).
In contrast, CD206+TAMs were shown to program T cells to attack melanoma tumor cells (47). Antigen cross-presentation in tumor remains to be a challenging issue for development of anti-cancer therapy. Primary human as well as mouse CD206+ macrophages were recently shown to be efficient in functional cross-presentation of soluble self-Ag and non-self-Ag, including tumor-associated Ag (TAA) (47). CD11b+CD206+ TAM were found to express a unique cell surface repertoire, promoting antigen cross-presentation and antigen-specific activation of CD8+ T cells. In murine tumor models, the levels of cross-presenting CD206+ TAMs correlated with reduced tumor burden (47). CD206+ TAMs also correlated with improved overall survival of cutaneous melanoma patients. It is an intriguing question to be addressed in future, which self-antigens can be presented to the adaptive immunity in different types of solid cancers by CD206 TAMs, and what is the impact of this process in overall role of CD206 in cancer (47).
2.5. Class G scavenger receptors
2.5.1. SR-G/CXCL16
CXCL16 (also known as SR-G1, or SR-PSOX) is a scavenger receptor mediating endocytosis of oxidized low-density lipoproteins (OxLDL). CXCL16 is primarily expressed on macrophages and dendritic cells. CXCL16 exists in both transmembrane and soluble forms. The soluble form acts as a chemokine specifically binding to CXCR6, and the transmembrane SR-G1 represents an adhesion molecule for CXCR6-expressing cells (116, 117).
CXCL16 has been shown to have a pro-tumoral function in papillary thyroid cancer (PTC) (53). In co-culture of PTC cells with primary monocytes or macrophage-like THP1 celles, high levels of CXCL16 were detected compared to separate PTC cell culture. Treatment of PTC cells with CXCL16 or co-culture with macrophages enhanced their migration potential. In turn, co-culture up-regulated the expression of M2-markers in macrophages, e.g., CD163, IL-10 and CD206, that was abrogated by an anti-CXCL16 antibody (53). An analysis of the TCGA PTC revealed an association of CXCL16 with M2 macrophage- and angiogenesis-related genes. High CXCL16 expression was associated with aggressive pathologic phenotypes, the higher TNM staging and lymph node metastasis in 77 patients with papillary thyroid cancers, in 25 patients with thyroid follicular adenomas, and 81 - with normal thyroid tissues from the SNUH cohort (51).
CXCL16 pro-tumoral activity was suggested for glioblastoma (GBM) patient’s (52). CXCL16 expression in GBM tissues was upregulated, compared to normal brain tissues. However, isolated tumor cells, even if cultured for 1-3 passages, had a substantial reduction in the CXCL16 expression levels. Treatment of mouse glioblastoma microglia with both recombinant and glioma-released CXCL16 increased the expression of anti-inflammatory genes ARG1, CHIL3, RETNLA and CD163 that was impaired by anti-CXCL16 antibodies. Microglia from glioma-bearing CXCR6-ko mice had lower expression levels of anti-inflammatory genes, compared to glioma-bearing wt mice that suggested CXCL16/CXCR6 axis involvement in the anti-inflammatory programming of microglia (Figure 4). Patient-derived GBM cells significantly increased cell chemotactic index, invasion and proliferation under CXCL16 exposure. Use of TCGA data with GBM patients revealed a significant increase in patient’s survival associated with CXCR6 deletion and a significant decrease in the survival associated with CXCR6 mRNA overexpression (52).
Human ovarian cancer tissue significantly increased expression of CXCL16 in comparison with both corresponding adjacent and para-cancerous tissues (54). The correlation analysis indicated a positive association of CXCL16 expression with an activation of macrophages in ovarian cancer (54). Macrophage-derived CXCL16 promoted migration and invasion of ovarian cancer SCOV3 cells by enhancing the activity of the PI3K/Akt pathway (54). Silencing of CXCR6 by shRNA in SCOV3 cells diminished above-mentioned effects of CXCL16 (54). In the co-culture of AIF1-overexpressed macrophages with hepatocellular carcinoma Hepa1-6 cells, CXCL16 secreted by macrophages enhanced proliferation and migration of cancer cells, and this effect was abrogated by a neutralizing antibody against CXCL16 (118).
We were able to find only one report describing CXCL16-mediating tumor-inhibiting function in colorectal cancer (CRC) (50). In co-culture of colorectal cancer SL4 cells with RAW 264.7 cells, CXCL16 induced tumor cell apoptosis mediated by TNFα-expressing macrophages. A susceptibility of CXCL16-overexpressing CRC cells to apoptosis was attenuated by neutralization of TNFα with a corresponding antibody (50).
In summary, CXCL16 tend to have predominantly a pro-tumoral role through promoting an anti-inflammatory phenotype of TAMs, and by activating proliferative and invasive potentials of cancer cells. Nevertheless, there is an evidence of CXCL16 anti-tumoral role too through sensitizing of CRC cells to apoptosis.
2.6. Class J scavenger receptors
2.6.1. SR-J1/RAGE
SR-J1 (AGER, or RAGE) is a cell surface receptor from the immunoglobulin superfamily that specifically binds advanced glycation end products (AGEs) (119). RAGE is the only member of class J scavenger receptors and capable of binding multiple ligands (72). Except for AGEs, SR-J1 recognizes HMGB1 (120), members of the S100 protein family (121), amyloid-beta peptide (122) and binds dsDNA and dsRNA directly (123). RAGE is expressed by diverse cell types, including macrophages, monocytes, endothelial cells, fibroblasts and smooth muscle cells (124).
Multiple evidences indicate a pro-inflammatory role of RAGE activation, in particular, the HMGB1-induced activation of RAGE in inflammation-related context (125–127). RAGE is involved in ROS production and M1 polarization of macrophages under AGE exposure (65, 128). In macrophages, AGEs significantly elevated the expression of IL-6, IL-12, TNFα and TLR4, as well as the phosphorylation levels of STAT1 in cytoplasm in RAGE/ROS dependent manner (Figure 4). TLR4 inhibition by siRNA diminished effect of the AGE-dependent RAGE activation, while both RAGE expression and ROS production remained unchanged. This evidence suggests TLR4 as a downstream regulator of RAGE activation and further ROS production (65).
RAGE was studied in human GBM treated with temozolomide (TMZ) (129). TMZ treatment caused HMGB1 release from GBM cells in tumor tissue of patients. Affinity examination showed that RAGE is the main receptor binding extracellular HMGB1. Immunofluorescent analysis of patients` GBM samples indicated co-localization of RAGE and HMGB1 on TAMs. In vitro stimulation of THP-1 macrophages with recombinant HMGB1 promoted release of pro-inflammatory cytokines through NLRP3-dependent inflammasomes that was diminished by RAGE inhibition (129). The mechanism of RAGE activation by HMGB1 was related to phosphorylation of ERK1/2 and IKB resulting in NFκB activation. In patients with GBM, HMGB1 expression is associated with improved OS. These results indicate that RAGE interaction with HMGB1 can be favorable factor in GBM treatment response (129). Irreversible electroporation caused the release of nucleus HMGB1 out of PDAC cells followed by binding of HMGB1 to RAGE in THP1-derived macrophages, that skewed macrophages toward pro-inflammatory phenotype via MAPK-ERK activation (130). Macrophages enhanced phagocytosis of dying electroporated PDAC cells. This effect was neutralized by RAGE inhibition. MAPK-ERK inhibition significantly decreased the RAGE expression and the release of autocrine HMGB1 by macrophages (130).
In vitro RAGE is equally expressed in both M1- and M2-polarized macrophages, but has distinct effects on the cancer cells that depends on a polarization state of macrophages (69). In contrast to M1 macrophages, HMGB1-dependent stimulation of RAGE facilitated pro-tumor activity in M2 macrophages. RAGE activation by HMGB1 enhanced invasion of gastric tumor cells (MKN45) in co-culture with M2-polarized THP1 macrophages and vice versa with M1 macrophages (66). RAGE induced VEGF production in M2 macrophages. The conditioned medium of M2 macrophages treated with HMGB1 stimulated the growth of endothelial cells in vitro; this effect was opposite for M1 macrophages. In contrast to M1 polarization, the RAGE activation in M2 macrophages did not lead to NFkB activation. Two negative regulators of the NFkB activation, SOCS1 and SHIP-1, were significantly upregulated under the HMGB1 exposure in M2 macrophages (66). HMGB1-mediated RAGE activation in THP1-derived M2 macrophages also stimulated lymphangiogenesis by increasing both proliferation and migration of lymphatic endothelial cells as well as VEGF-C production in M2, but not in M0 macrophages (67). RAGE inhibition significantly reduced M2-dependent lymphangiogenesis (67). HMGB1+CD163+ M2 macrophages were found as detrimental prognostic factors for OS in laryngeal squamous cell carcinoma patients (67). RAGE mediates chemotaxis of THP1-differentiated macrophages upon stimulation with a conditioned medium of S100A7-overexpressing breast cancer MDA-MB-231 cells. This effect was significantly abrogated by RAGE blockage (131).
In vivo RAGE-depleted mouse models of GBM indicated RAGE as a significant TAM-specific factor participating in inflammation and angiogenesis in the TME (69). Survival analysis of tumor-bearing mice revealed that RAGE ablation significantly prolonged survival of mice in comparison with wild type (wt) mice. RAGE-depleted tumor exhibited lower expression of pro-inflammatory cytokines, and RAGE-depleted TAMs expressed significantly lower levels of IL-6 and VEGF-A. RAGE expression in tumor microglia or bone marrow-derived macrophages stimulated angiogenesis in GBM. Patient’s GBM samples had abundance of CD163+ TAMs with high RAGE expression (69).
RAGE was shown to mediate uptake of an oncogenic mutant KRASG12D protein by peripheral blood mononuclear cell-derived macrophages during autophagy-dependent ferroptosis of PDAC cells (68). Under oxidative stress conditions, tumor cells released KRASG12D protein via exosomes secretion. Exosomes were engulfed by macrophages in a RAGE-dependent manner that was confirmed by the knockdown of RAGE by shRNAs in macrophages. KRASG12D promoted M2 polarization via STAT3-dependent fatty acid oxidation (FAO). Inhibition of FAO reduced mRNA expression of IL10, ARG1, and TGFB1 in the macrophages. The knockdown of RAGE and ablation of STAT3 by shRNA abrogated the FAO and the M2 polarization. In PDAC patients, high KRASG12D expression in CD68+ cells correlated with worse OS rates. The KRASG12D uptake by macrophages may significantly contribute to the human PDAC progression (68).
In summary, RAGE activation of TAMs has controversial impact on TME and tumor cells. Evidence indicates that pro-tumor and anti-tumor RAGE role through distinct TAMs activation depends on TME context and RAGE ligands. Is of great interest to identify in future the spectrum of tumor-specific sets of RAGE ligands, and to examine how cooperation of M1 or M2-specific receptors with RAGE can a decide about pro-and anti-tumor programming of TAMs.
2.7. Class H scavenger receptors
The class H scavenger receptors are transmembrane protein receptors containing in their extracellular part fasciclin, EGF-like and lamin-type domains. Class H scavenger receptors has two members: SR-H1 (also known as Stabilin-1, or Clever-1) and SR-H2 (known as stabilin-2, or HARE) (132, 133). Despite high similarity in domain organization and endocytic functions, stabilin-1, but not stabilin-2 is expressed on TAMs and plays an essential role in tumor development.
2.7.1. SR-H1/Stabilin-1
SR-H1, originally identified as stabilin-1 (134) and as CLEVER-1 (135) is multifunctional scavenger and intracellular sorting receptor with adhesive activities expressed by immunosuppressive monocytes and macrophages, sinusoidal endothelial cells and lymphatic endothelial cells (134, 136–138). Stabilin-1 performs endocytosis, phagocytosis, intracellular sorting of newly synthetized proteins and transcytosis of growth hormone family member placental lactogen (9, 139–144). Large body of evidence demonstrated that stabilin-1/CLEVER-1 can mediate cell-matrix and cell-cell interactions during primary tumor growth and in metastatic state (135, 138, 145–147).
Stabilin-1 is abundantly expressed on TAMs in number of solid cancers in patients and in murine models (8, 57, 132, 148). TAM-expressed stabilin-1 mediates clearance of tumor growth-inhibiting factor SPARC in a mouse model of breast cancer, and germinal knock-out of stabilin-1 results in the statistically significant reduction of primary tumor growth in this model (57). In orthotopic mouse models of lung cancer, breast cancer and lymphoma, genetic deficiency of macrophage stabilin-1 significantly reduced tumor growth (149). In stabilin-1 KO mice TME was shifted towards inflammatory program and was enriched in the activated endogenous CD8+ T cells. Immunotherapeutic blockade of stabilin-1 had similar consequences, and had synergistic effect with anti-PD-1 checkpoint inhibition (149).
Strong association of stabilin-1+ TAMs with worse prognosis was shown in several human cancers (55, 56, 150). Stabilin-1 expression in TAMs was associated with poor OS, RFS, tumor stage and histological grade in patients with urothelial carcinoma (56). High intratumoral expression of stabilin-1 on CD68+ TAMs was associated with poor DSS in stage I–IV rectal cancer (55). In contrast, high number of CD68+ stabilin-1+ TAMs correlated with longer DSS and predicted a favorable prognosis in early stage I colorectal cancer (CRC) patients (55).
Contribution of intracellular sorting function of stabilin-1 to tumor progression is linked to the ability of the extracellular domains of stabilin-1 to interact with at least two human chitinase-like proteins, SI-CLP and YKL-39, while the interaction with true chitinases CHIT1 and AMCase and with YKL-40 was not studied to date (6, 141, 151, 152).
We have demonstrated that stabilin-1 mediates intracellular delivery of newly synthetized SI-CLP, stabilin-1interacting chitinase-like protein, that interacted with a fasciclin domain of stabilin-1 in the yeast two-hybrid screening and in the affinity chromatography assay (141, 152). In a murine model for breast adenocarcinoma we demonstrated that SI-CLP being ectopically expressed in subcutaneously injected TS-A cells significantly reduced tumor growth and reduced infiltration of TAMs (153). Recently, we have also found that stabilin-1 is able to interact with YKL-39 (CHI3L2), that for a long-time was known as highly specific biomarker of rheumatoid arthritis, and later has been found to be overexpressed in glioblastoma affecting biology of transformed cells (6, 154). In patients with glioma high levels of CHI3L2 expressed in cancer cells and on microglia cells correlated with poor prognosis. Mechanistically the authors found that CHI3L2 induces the apoptosis of CD8+ T cells (155). We found that YKL-39 has two functions that can promote tumor growth: it stimulates monocyte migration, and it stimulates as well angiogenic activity of endothelial cells in vitro (6). In patients with breast cancer YKL-39 was exclusively expressed in TAMs in tumor mass, and elevated levels of YKL-39 in primary tumors significantly correlated with metastatic relapse after therapy onset (6). However, whether similar mechanisms can act in other types of cancer has to be studied, while application of purified SI-CLP and blocking agents for YKL-39 is a promising strategy to reprogram tumor-promoting microenvironment.
In summary, stabilin-1 has a highly complex function in cancer. Its deficiency is cancer-inhibiting, at least due to the reduction of SPARC clearance. Its ability to modulate concentrations of tumor-promoting YKL-39 and tumor-inhibiting SI-CLP can contribute to tumor growth and metastasis in a cancer-specific way, since not necessarily both proteins are present at the same time in TME. In particular, the role of stabilin-1 in CRC is of interest, while total amount of TAMs in this cancer type, in contrast to majority of other types, correlates with reduced tumor growth and good prognosis (8).
2.8. Class I scavenger receptors
SR-I (also known as CD163) is a hemoglobin-haptoglobin complex scavenger receptor that is mostly expressed in monocytes and macrophages (13, 156). In tumors, CD163 promotes tumor development and is associated with worth prognosis in breast cancer, head and neck cancer, lymphoma and melanoma (39, 59–62, 64, 100). CD163, mediates clearance of hemoglobin-haptoglobin complexes out of circulation is a silent way, however, in hyperglyceamic conditions this scavenging process leads to inflammatory macrophage responses (157).
CD163 is commonly defined as a marker for tumor-supporting TAM phenotype. In human breast cancer, CD163+ TAMs accumulation was inhibited by tumor suppressor TAp73 (58). Amount of CD163+ TAMs negatively correlated with Tap73 expression and positively correlated with tumor grade (58). High amount of CD68+ and CD163+ TAMs was associated with lymph node metastasis, high Ki67 expression and poor prognosis in 1579 breast cancer patients from Zhejiang Provincial People’s Hospital and Zhejiang Tiantai People’s Hospital (62, 100). Elevated levels of CD163+ TAMs in tumor stroma and tumor nest correlated with poor prognosis in 107 patients with triple negative breast cancer operated on at Dokkyo Medical University Hospital (59). CD163 was identified as a good predictor of pre-metastatic status of colorectal cancer (158). High levels of CD163+ cells were associated with tumor node metastasis stage, depth of infiltration, and lymphatic metastasis in 197 patients with colorectal cancer from China (158). Using multispectral immunofluorescence it was demonstrated that CD163+ cells have immunosuppressive phenotype in 17 patients with colorectal cancer who underwent resection of primary and liver metastases (159). High number of CD163+ cells was found in peritumoral region of tumor and in liver metastases (159).
Several studies confirmed that tumor-supporting effect of CD163+ TAMs is mediated by the activation of STAT3 signaling pathway (60, 63). Tumor-mediated activation of STAT3 in CD163+ TAMs resulted in pro-tumor TAM polarization (63). In vitro, conditioned medium from cholangiocarcinoma cell lines (HuCCT1, RBE and MEC) induced activation of STAT3 in modeled TAMs and enhanced production of IL-10, VEGF-α, TGF-β and MMP-2 in CD163+ TAMs (63) (Figure 4). CD163+ TAMs produced tumor-supporting cytokines (IL-6 and CXCL2) activated STAT3 in tumor cells and supported tumor progression. CD163-KO TAMs had decreased production of IL-6 and CXCL2 in comparison to WT TAMs in co-culture with MCA205 (mouse fibrosarcoma) cells (64). Conditioned medium of CD163-KO TAMs significantly impaired activation of STAT3 in MCA205 cells (64). CD163-expressing TAMs displayed elevated levels of pSTAT3 and correlated to poor prognosis in 77 patients with myeloma from STAT3 is over-activated within CD163pos bone marrow macrophages in both Multiple Myeloma and the benign pre-condition MGUS (60). Increased infiltration of both CD68+ and CD163+ TAMs in tumor mass correlated with decreased survival of 174 patients with gastroesophageal adenocarcinoma from Sweden (61). CD68 and CD163 overexpression was indicative for worse prognosis in 105 HCC patients from Japan (160). Increased levels of CD163+ TAMs correlated with decreased OS and higher histological grade in human sarcoma (64). Oppositely, in human primary melanoma low amount of CD163-expressing TAMs in tumor stroma was associated with recurrence and poor OS (39).
Overall, at least two molecular mechanism for tumor-supporting function of CD163+ TAMs were identified to date: inhibition of tumor suppressor TAp73 in breast cancer and activation of STAT3 signaling in TAMs and in r fibrosarcoma cells (58, 63, 64). Moreover, high expression of CD163+ TAMs was related to poor prognosis in breast cancer, gastroesophageal adenocarcinoma, HCC, human sarcoma, but not in melanoma patients (39, 59, 61, 62, 64).
3. Genetics of scavenger receptors
Deleterious germline mutations cause a broad range of distinct pathological conditions including cancer (161) There is limited information describing association of SR gene mutations with tumor progression, especially in non-malignant cells, e.g., macrophages. The only reliable evidence for such association are germline mutations in MSR1 coding scavenger receptor CD204 (162, 163). Genetic analysis of hereditary prostate cancer revealed significant co-segregation of prostate cancer with the nonsense mutation R293X in man of European descent and the missense mutation D174Y in man of African American descent (163). The truncating mutation R293X resulted in deletion of most of the collagen-like domain of MSR1 gene, including the ligand-binding region and the cysteine-rich domain. The missense mutation D174Y can affect proper polymerization of three MSR1 polypeptide chains. Both mutations disrupted MSR1 function that affected MSR1 ability to bind oxLDL involved in the oxidative stress. MSR1 is predominantly expressed by macrophages in both benign and cancerous prostate tissues, emphasizing the role of macrophage-derived mutated MSR1 in prostate cancer development (163).
MSR1 mutations are also involved in Barrett esophagus (BE) and esophageal adenocarcinoma (EAC) development (162). The nonsense R293X and missense L254V mutations contributed to BE/EAC risk, or were required for BE/EAC predisposition. The L254V mutation was found within the conserved coiled-coil domain of MSR1, so both R293X and L254V led to MSR1 function disruption. MSR1 mutation caused overexpression of key nuclear cell cycle molecule Cyclin D1 (CCND1) in BE and EAC tissue samples that was impaired by overexpression of wild-type MSR1 in HEK293 cells (162).
Association of MSR1 mutations with progression of prostate cancer and esophageal adenocarcinoma confirmed the involvement of this scavenger receptor to carcionogenesis.
4. Conclusions
Macrophage SRs have dual role in tumor development. Tumor-supporting activity mediated by macrophage SRs includes regulation of tumor invasion, proliferation and migration (for CD204, CD206, CXCL16, Stabilin-1, and RAGE), as well as M2-like TAM polarization (for CD36, LOX-1, CXCL16, CD 163, and RAGE) and tumor angiogenesis (for CD68, Dectin-1, RAGE). The anti-tumor functions of TAM-expressing SRs include suppression of tumor angiogenesis (for CD204), tumor invasion (for RAGE), inducing tumor cells clearance (for MARCO) and M1-like TAM polarization (for CD204 and RAGE). In cancer patients, number of TAM-expressed SRs (CD204, MARCO, CD68, LOX-1, Dectin-1, CD206, CXCL16, Stabilin-1, CD163, and RAGE) associates with negative and more sever prognosis. Targeting of tumor-promoting SRs can be a promising approach in cancer immunotherapy. Accumulating clinical data demonstrate that SRs can serve as potential prognostic biomarkers for patients with cancer (29, 84, 164). For example, in mouse model of triple negative breast cancer specific targeting of CD206+ TAMs inhibited tumorigenesis and metastatic dissemination of tumor cells (165). Application of antibodies against MARCO resulted in the reduction of tumor growth and inhibition of metastasis in murine models for melanoma and breast cancer (166). There are multiple studies describing effects of SR targeting in various cellular in vitro and pre-clinical in vivo models (145, 165, 167–169). Drug targeting of CD36 demonstrated promising results for patients with advanced soft tissue sarcoma in the initial clinical trials (167, 170). However, targeting CD36 failed in phase 2 clinical trials because of ineffective performance and severe adverse events. The complications can be explained by the expression of majority of various SRs on resident macrophages and on other cell types in healthy organs and tissues. Moreover, we still have very limited information about cancer-specific ligands of SRs, in particular for the ability of SRs to internalize and target for degradation cytokines and growth factors.
The investigation of the mechanisms of tumor development and progression mediated by SRs is a foreground goal for the developing immunotherapeutic approaches that can help to suppress tumor cell invasion, proliferation and migration, to inhibit macrophage recruitment and pro-tumor macrophage polarization as well as to enhance clearance of tumor cells by TAMs. Moreover, the ability of SRs to internalize both particles and molecular complexes still remains to be explored for the design of targeted drug delivery for macrophage re-programming in tumor microenvironment.
Author contributions
EK performed literature analysis and drafted the manuscript. EK designed Figures. PI and IL contributed to literature analysis and wrote manuscript chapters. JK developed the concept of the manuscript, designed tables, wrote manuscript chapters, edited final text. All authors contributed to manuscript revision, read and approved the submitted version.
Funding
This work was supported by grant 19-15-00151 of Russian Science Foundation.
Conflict of interest
The authors declare that the research was conducted in the absence of any commercial or financial relationships that could be construed as a potential conflict of interest.
Publisher’s note
All claims expressed in this article are solely those of the authors and do not necessarily represent those of their affiliated organizations, or those of the publisher, the editors and the reviewers. Any product that may be evaluated in this article, or claim that may be made by its manufacturer, is not guaranteed or endorsed by the publisher.
References
1. Zhang C, Yang M, Ericsson AC. Function of macrophages in disease: Current understanding on molecular mechanisms. Front Immunol (2021) 12:620510/BIBTEX. doi: 10.3389/FIMMU.2021.620510/BIBTEX
2. Varol C, Mildner A, Jung S. Macrophages: Development and tissue specialization. Annu Rev Immunol (2015) 33:643–75. doi: 10.1146/ANNUREV-IMMUNOL-032414-112220
3. Larionova I, Kazakova E, Patysheva M, Kzhyshkowska J. Transcriptional, epigenetic and metabolic programming of tumor-associated macrophages. Cancers (Basel) (2020) 12:1–40. doi: 10.3390/cancers12061411
4. Kzhyshkowska J, Grigoryeva E, Larionova I. Targeting the tumor-associated macrophages for ‘Normalizing’ cancer. Approaching Complex Dis (2020) 2:245–74. doi: 10.1007/978-3-030-32857-3_11
5. Patysheva M, Larionova I, Stakheyeva M, Grigoryeva E, Iamshchikov P, Tarabanovskaya N, et al. Effect of early-stage human breast carcinoma on monocyte programming. Front Oncol (2022) 11:800235/BIBTEX. doi: 10.3389/FONC.2021.800235/BIBTEX
6. Liu T, Larionova I, Litviakov N, Riabov V, Zavyalova M, Tsyganov M, et al. Tumor-associated macrophages in human breast cancer produce new monocyte attracting and pro-angiogenic factor YKL-39 indicative for increased metastasis after neoadjuvant chemotherapy. Oncoimmunology (2018) 7:e1436922. doi: 10.1080/2162402X.2018.1436922
7. Patysheva M, Frolova A, Larionova I, Afanas’ev S, Tarasova A, Cherdyntseva N, et al. Monocyte programming by cancer therapy. Front Immunol (2022) 13:994319. doi: 10.3389/FIMMU.2022.994319
8. Larionova I, Tuguzbaeva G, Ponomaryova A, Stakheyeva M, Cherdyntseva N, Pavlov V, et al. Tumor-associated macrophages in human breast, colorectal, lung, ovarian and prostate Cancers1. larionova, i. et al. tumor-associated macrophages in human breast, colorectal, lung, ovarian and prostate cancers. Front Oncol (2020) 10:566511. doi: 10.3389/fonc.2020.566511
9. Kzhyshkowska J, Workman G, Cardó-Vila M, Arap W, Pasqualini R, Gratchev A, et al. Novel function of alternatively activated macrophages: Stabilin-1-Mediated clearance of SPARC. J Immunol (2006) 176:5825–32. doi: 10.4049/jimmunol.176.10.5825
10. Riabov V, Gudima A, Wang N, Mickley A, Orekhov A, Kzhyshkowska J. Role of tumor associated macrophages in tumor angiogenesis and lymphangiogenesis. Front Physiol (2014) 5:75. doi: 10.3389/fphys.2014.00075
11. Lin Y, Xu J, Lan H. Tumor-associated macrophages in tumor metastasis: biological roles and clinical therapeutic applications. J Hematol Oncol (2019) 12:1–16. doi: 10.1186/S13045-019-0760-3
12. Patten DA, Wilkinson AL, O’Keeffe A, Shetty S. Scavenger receptors: Novel roles in the pathogenesis of liver inflammation and cancer. Semin Liver Dis (2021) 42(1):61–76. doi: 10.1055/S-0041-1733876
13. Taban Q, Mumtaz PT, Masoodi KZ, Haq E, Ahmad SM. Scavenger receptors in host defense: from functional aspects to mode of action. Cell Commun Signal (2022) 20:1–17. doi: 10.1186/S12964-021-00812-0/FIGURES/7
14. Ben J, Zhu X, Zhang H, Chen Q. Class A1 scavenger receptors in cardiovascular diseases. Br J Pharmacol (2015) 172:5523. doi: 10.1111/BPH.13105
15. Pombinho R, Sousa S, Cabanes D. Scavenger receptors: Promiscuous players during microbial pathogenesis. Crit. Reviews Microbio (2018) 44:685–700. doi: 10.1080/1040841X.2018.1493716
16. Yu X, Guo C, Fisher PB, Subjeck JR, Wang X-Y. Scavenger receptors: Emerging roles in cancer biology and immunology. Adv Cancer Res (2015) 128:309. doi: 10.1016/BS.ACR.2015.04.004
17. Patten DA, Shetty S. More than just a removal service: Scavenger receptors in leukocyte trafficking. Front Immunol (2018) 0:2904. doi: 10.3389/FIMMU.2018.02904
18. Dong H, Bullock TNJ. Metabolic influences that regulate dendritic cell function in tumors. Front Immunol (2014) 5:24/BIBTEX. doi: 10.3389/FIMMU.2014.00024/BIBTEX
19. Shen WJ, Azhar S, Kraemer FB. SR-B1: A unique multifunctional receptor for cholesterol influx and efflux. Annu Rev Physiol (2018) 80:95. doi: 10.1146/ANNUREV-PHYSIOL-021317-121550
20. Vasquez M, Simões I, Consuegra-Fernández M, Aranda F, Lozano F, Berraondo P. Exploiting scavenger receptors in cancer immunotherapy: Lessons from CD5 and SR-B1. Eur J Immunol (2017) 47:1108–18. doi: 10.1002/EJI.201646903
21. Ben J, Jin G, Zhang Y, Ma B, Bai H, Chen J, et al. Class a scavenger receptor deficiency exacerbates lung tumorigenesis by cultivating a procarcinogenic microenvironment in humans and mice. American J Res Crit Care Med (2012) 186:763–72. doi: 10.1164/RCCM.201204-0592OC
22. Zhang Y, Wei Y, Jiang B, Chen L, Bai H, Zhu X, et al. Scavenger receptor A1 prevents metastasis of non–small cell lung cancer via suppression of macrophage serum amyloid A1. Cancer Res (2017) 77:1586–98. doi: 10.1158/0008-5472.CAN-16-1569
23. Cao J, Liu J, Xu R, Zhu X, Zhao X, Qian BZ. Prognostic role of tumour-associated macrophages and macrophage scavenger receptor 1 in prostate cancer: a systematic review and meta-analysis. Oncotarget (2017) 8:83261. doi: 10.18632/ONCOTARGET.18743
24. Tada Y, Matsumi Y, Hara K, Miyauchi W, Sugesawa K, Uejima C, et al. Infiltration of CD204-overexpressing macrophages contributes to the progression of stage II and III colorectal cancer. Anticancer Res (2021) 41:4857–65. doi: 10.21873/ANTICANRES.15299
25. Zhang H, Zhang W, Sun X, Dang R, Zhou R, Bai H, et al. Class A1 scavenger receptor modulates glioma progression by regulating M2-like tumor-associated macrophage polarization. Oncotarget (2016) 7:50099–116. doi: 10.18632/ONCOTARGET.10318
26. Neyen C, Plüddemann A, Mukhopadhyay S, Maniati E, Bossard M, Gordon S, et al. Macrophage scavenger receptor a promotes tumour progression in murine models of ovarian and pancreatic cancer. J Immunol (2013) 190:3798. doi: 10.4049/JIMMUNOL.1203194
27. He Y, Zhou S, Deng F, Zhao S, Chen W, Wang D, et al. Clinical and transcriptional signatures of human CD204 reveal an applicable marker for the protumor phenotype of tumor-associated macrophages in breast cancer. Aging (2019) 11:10883. doi: 10.18632/AGING.102490
28. Xing Q, Feng Y, Sun H, Yang S, Sun T, Guo X, et al. Scavenger receptor MARCO contributes to macrophage phagocytosis and clearance of tumor cells. Exp Cell Res (2021) 408:112862. doi: 10.1016/J.YEXCR.2021.112862
29. Shi B, Chu J, Huang T, Wang X, Li Q, Gao Q, et al. The scavenger receptor MARCO expressed by tumor-associated macrophages are highly associated with poor pancreatic cancer prognosis. Front Oncol (2021) 11:771488. doi: 10.3389/FONC.2021.771488
30. La Fleur L, Boura VF, Alexeyenko A, Berglund A, Pontén V, Mattsson JSM, et al. Expression of scavenger receptor MARCO defines a targetable tumor-associated macrophage subset in non-small cell lung cancer. Int J Cancer (2018) 143:1741–52. doi: 10.1002/IJC.31545
31. Sun H, Song J, Weng C, Xu J, Huang M, Huang Q, et al. Association of decreased expression of the macrophage scavenger receptor MARCO with tumor progression and poor prognosis in human hepatocellular carcinoma. J Gastroenterol Hepatol (2017) 32:1107–14. doi: 10.1111/JGH.13633
32. Chen AX, Gartrell RD, Zhao J, Upadhyayula PS, Zhao W, Yuan J, et al. Single-cell characterization of macrophages in glioblastoma reveals MARCO as a mesenchymal pro-tumor marker. Genome Med (2021) 13:88. doi: 10.1186/S13073-021-00906-X
33. Fleur L, Botling J, He F, Pelicano C, Zhou C, He C, et al. Targeting MARCO and IL37R on immunosuppressive macrophages in lung cancer blocks regulatory T cells and supports cytotoxic lymphocyte function. Cancer Res (2021) 81:956–67. doi: 10.1158/0008-5472.CAN-20-1885/654261/AM/TARGETING-MARCO-AND-IL-37R-ON-IMMUNOSUPPRESSIVE
34. Su P, Wang Q, Bi E, Ma X, Liu L, Yang M, et al. Enhanced lipid accumulation and metabolism are required for the differentiation and activation of tumor-associated macrophages. Cancer Res (2020) 80:1438. doi: 10.1158/0008-5472.CAN-19-2994
35. Liu S, Zhang H, Li Y, Zhang Y, Bian Y, Zeng Y, et al. Original research: S100A4 enhances protumor macrophage polarization by control of PPAR-γ-dependent induction of fatty acid oxidation. J Immunother Cancer (2021) 9:e002548. doi: 10.1136/JITC-2021-002548
36. Frank AC, Ebersberger S, Fink AF, Lampe S, Weigert A, Schmid T, et al. Apoptotic tumor cell-derived microRNA-375 uses CD36 to alter the tumor-associated macrophage phenotype. Nat Commun (2019) 10:1135. doi: 10.1038/S41467-019-08989-2
37. Sun S, Pan X, Zhao L, Zhou J, Wang H, Sun Y. The expression and relationship of CD68-Tumor-Associated macrophages and microvascular density with the prognosis of patients with laryngeal squamous cell carcinoma. Clin Exp Otorhinolaryn (2016) 9:270. doi: 10.21053/CEO.2015.01305
38. Zhang J, Li S, Liu F, Yang K. Role of CD68 in tumor immunity and prognosis prediction in pan-cancer. Sci Rep (2022) 12:7844. doi: 10.1038/S41598-022-11503-2
39. Salmi S, Siiskonen H, Sironen R, Tyynelä-Korhonen K, Hirschovits-Gerz B, Valkonen M, et al. The number and localization of CD68+ and CD163+ macrophages in different stages of cutaneous melanoma. Melanoma Res (2019) 29:237–47. doi: 10.1097/CMR.0000000000000522
40. Song YJ, Xu Y, Zhu X, Fu J, Deng C, Chen H, et al. Immune landscape of the tumor microenvironment identifies prognostic gene signature CD4/CD68/CSF1R in osteosarcoma. Front Oncol (2020) 10:1198/FULL. doi: 10.3389/FONC.2020.01198/FULL
41. Tremble LF, McCabe M, Walker SP, McCarthy S, Tynan RF, Beecher S, et al. Differential association of CD68+ and CD163+ macrophages with macrophage enzymes, whole tumour gene expression and overall survival in advanced melanoma. Br J Cancer (2020) 123:1553–61. doi: 10.1038/s41416-020-01037-7
42. Katayama C, Yokobori T, Ozawa N, Suga K, Shiraishi T, Okada T, et al. Low level of stromal lectin-like oxidized LDL receptor 1 and CD8 + cytotoxic T-lymphocytes indicate poor prognosis of colorectal cancer. Cancer Rep (Hoboken NJ) (2021) 4:e1364. doi: 10.1002/CNR2.1364
43. Zhou J, Zhang A, Fan L. HSPA12B secreted by tumor-associated endothelial cells might induce M2 polarization of macrophages via activating PI3K/Akt/mTOR signaling. Onco Targets Ther (2020) 13:9103–11. doi: 10.2147/OTT.S254985
44. Alaeddine M, Prat M, Poinsot V, Gouazé-Andersson V, Authier H, Meunier E, et al. IL13-mediated dectin-1 and mannose receptor overexpression promotes macrophage antitumor activities through recognition of sialylated tumor cells. Cancer Immunol Res (2019) 7:321–34. doi: 10.1158/2326-6066.CIR-18-0213/470783/AM/IL13-MEDIATED-DECTIN-1-AND-MANNOSE-RECEPTOR
45. Xia Y, Liu L, Bai Q, Wang J, Xi W, Qu Y, et al. Dectin-1 predicts adverse postoperative prognosis of patients with clear cell renal cell carcinoma. Sci Rep (2016) 6:1–9. doi: 10.1038/srep32657
46. Daley D, Mani VR, Mohan N, Akkad N, Ochi A, Heindel DW, et al. Dectin 1 activation on macrophages by galectin 9 promotes pancreatic carcinoma and peritumoral immune tolerance. Nat Med (2017) 23:556–67. doi: 10.1038/nm.4314
47. Modak M, Mattes AK, Reiss D, Skronska-Wasek W, Langlois R, Sabarth N, et al. CD206+ tumor-associated macrophages cross-present tumor antigen and drive antitumor immunity. JCI Insight (2022) 7:e155022. doi: 10.1172/JCI.INSIGHT.155022
48. Zhang C, Yu X, Gao L, Zhao Y, Lai J, Lu D, et al. Noninvasive imaging of CD206-positive M2 macrophages as an early biomarker for post-chemotherapy tumor relapse and lymph node metastasis. Theranostics (2017) 7:4276–88. doi: 10.7150/THNO.20999
49. Haque ASMR, Moriyama M, Kubota K, Ishiguro N, Sakamoto M, Chinju A, et al. CD206 + tumor-associated macrophages promote proliferation and invasion in oral squamous cell carcinoma via EGF production. Sci Rep (2019) 9:14611. doi: 10.1038/S41598-019-51149-1
50. Kee JY, Ito A, Hojo S, Hashimoto I, Igarashi Y, Tsuneyama K, et al. CXCL16 suppresses liver metastasis of colorectal cancer by promoting TNF-α-induced apoptosis by tumor-associated macrophages. BMC Cancer (2014) 14:949. doi: 10.1186/1471-2407-14-949
51. Kim MJ, Sun HJ, Song YS, Yoo SK, Kim YA, Seo JS, et al. CXCL16 positively correlated with M2-macrophage infiltration, enhanced angiogenesis, and poor prognosis in thyroid cancer. Sci Rep (2019) 9:13288. doi: 10.1038/s41598-019-49613-z
52. Lepore F, D’Alessandro G, Antonangeli F, Santoro A, Esposito V, Limatola C, et al. CXCL16/CXCR6 axis drives Microglia/Macrophages phenotype in physiological conditions and plays a crucial role in glioma. Front Immunol (2018) 9:2750. doi: 10.3389/FIMMU.2018.02750
53. Cho SW, Kim YA, Sun HJ, Kim YA, Oh BC, Yi KH, et al. CXCL16 signaling mediated macrophage effects on tumor invasion of papillary thyroid carcinoma. Endocr Relat Cancer (2016) 23:113–24. doi: 10.1530/ERC-15-0196
54. Hong L, Wang S, Li W, Wu D, Chen W. Tumor-associated macrophages promote the metastasis of ovarian carcinoma cells by enhancing CXCL16/CXCR6 expression. Pathol Res Pract (2018) 214:1345–51. doi: 10.1016/J.PRP.2018.07.009
55. Ålgars A, Kemppinen L, Fair-Mäkelä R, Mustonen H, Haglund C, Jalkanen S. Stage I–IV colorectal cancer prognosis can be predicted by type and number of intratumoral macrophages and CLEVER-1+ vessel density. Cancers (2021) 13:5988. doi: 10.3390/CANCERS13235988
56. Wang B, Huang H, Yang M, Yang W, Liu Z, Hou W, et al. Microlocalization and clinical significance of stabilin-1+ macrophages in treatment-naïve patients with urothelial carcinoma of the bladder. World J Urol (2020) 38:709. doi: 10.1007/S00345-019-02853-0
57. Riabov V, Yin S, Song B, Avdic A, Schledzewski K, Ovsiy I, et al. Stabilin-1 is expressed in human breast cancer and supports tumor growth in mammary adenocarcinoma mouse model. Oncotarget (2016) 7:31097–110. doi: 10.18632/oncotarget.8857
58. Wolfsberger J, Sakil HAM, Zhou L, Van Bree N, Baldisseri E, De Souza Ferreira S, et al. TAp73 represses NF-κB–mediated recruitment of tumor-associated macrophages in breast cancer. Proc Natl Acad Sci U.S.A. (2021) 118:e2017089118. doi: 10.1073/PNAS.2017089118/-/DCSUPPLEMENTAL
59. Jamiyan T, Kuroda H, Yamaguchi R, Abe A, Hayashi M. CD68- and CD163-positive tumor-associated macrophages in triple negative cancer of the breast. Virchows Arch (2020) 477:767–75. doi: 10.1007/S00428-020-02855-Z/FIGURES/2
60. Andersen MN, Andersen NF, Lauridsen KL, Etzerodt A, Sorensen BS, Abildgaard N, et al. STAT3 is over-activated within CD163 pos bone marrow macrophages in both multiple myeloma and the benign pre-condition MGUS. Cancer Immunol Immunother (2022) 71:177–87. doi: 10.1007/S00262-021-02952-1
61. Jeremiasen M, Borg D, Hedner C, Svensson M, Nodin B, Leandersson K, et al. Tumor-associated CD68+, CD163+, and MARCO+ macrophages as prognostic biomarkers in patients with treatment-naïve gastroesophageal adenocarcinoma. Front Oncol (2020) 10:534761/BIBTEX. doi: 10.3389/FONC.2020.534761/BIBTEX
62. Ni C, Liu Y, Qiuran X, Hongjun Y, Wei W, Wenjie X, et al. CD68- and CD163-positive tumor infiltrating macrophages in non-metastatic breast cancer: a retrospective study and meta-analysis. J Cancer (2019) 10:4463–72. doi: 10.7150/JCA.33914
63. Hasita H, Komohara Y, Okabe H, Masuda T, Ohnishi K, Lei XF, et al. Significance of alternatively activated macrophages in patients with intrahepatic cholangiocarcinoma. Cancer Sci (2010) 101:1913–9. doi: 10.1111/j.1349-7006.2010.01614.x
64. Shiraishi D, Fujiwara Y, Horlad H, Saito Y, Iriki T, Tsuboki J, et al. CD163 is required for protumoral activation of macrophages in human and murine sarcoma. Cancer Res (2018) 78:3255–66. doi: 10.1158/0008-5472.CAN-17-2011
65. Liu Z, Ma Y, Cui Q, Xu J, Tang Z, Wang Y, et al. Toll-like receptor 4 plays a key role in advanced glycation end products-induced M1 macrophage polarization. Biochem Biophys Res Commun (2020) 531:602–8. doi: 10.1016/J.BBRC.2020.08.014
66. Rojas A, Delgado-López F, Perez-Castro R, Gonzalez I, Romero J, Rojas I, et al. HMGB1 enhances the protumoral activities of M2 macrophages by a RAGE-dependent mechanism. Tumour Biol (2016) 37:3321–9. doi: 10.1007/S13277-015-3940-Y
67. Su C, Jia S, Ma Z, Zhang H, Wei L, Liu H. HMGB1 promotes lymphangiogenesis through the activation of RAGE on M2 macrophages in laryngeal squamous cell carcinoma. Dis Markers (2022) 2022:18. doi: 10.1155/2022/4487435
68. Dai E, Han L, Liu J, Xie Y, Kroemer G, Klionsky DJ, et al. Autophagy-dependent ferroptosis drives tumor-associated macrophage polarization via release and uptake of oncogenic KRAS protein. Autophagy (2020) 16:2069–83. doi: 10.1080/15548627.2020.1714209
69. Chen X, Zhang L, Zhang IY, Liang J, Wang H, Ouyang M, et al. RAGE expression in tumor-associated macrophages promotes angiogenesis in glioma. Cancer Res (2014) 74:7285–97. doi: 10.1158/0008-5472.CAN-14-1240
70. Cheng C, Zheng E, Yu B, Zhang Z, Wang Y, Liu Y, et al. Recognition of lipoproteins by scavenger receptor class a members. J Biol Chem (2021) 297:100948. doi: 10.1016/J.JBC.2021.100948
71. Zani IA, Stephen SL, Mughal NA, Russell D, Homer-Vanniasinkam S, Wheatcroft SB, et al. Scavenger receptor structure and function in health and disease. Cells (2015) 4:178. doi: 10.3390/CELLS4020178
72. Alquraini A, El Khoury J. Scavenger receptors. Curr Biol (2020) 30:R790–5. doi: 10.1016/J.CUB.2020.05.051
73. Yuan Y, Zhao Q, Zhao S, Zhang P, Zhao H, Li Z, et al. Characterization of transcriptome profile and clinical features of a novel immunotherapy target CD204 in diffuse glioma. Cancer Med (2019) 8:3811. doi: 10.1002/CAM4.2312
74. Kubota K, Moriyama M, Furukawa S, Rafiul HASM, Maruse Y, Jinno T, et al. CD163+CD204+ tumor-associated macrophages contribute to T cell regulation via interleukin-10 and PD-L1 production in oral squamous cell carcinoma. Sci Rep (2017) 7:1–12. doi: 10.1038/s41598-017-01661-z
75. Kawachi A, Yoshida H, Kitano S, Ino Y, Kato T, Hiraoka N. Tumor-associated CD204+ M2 macrophages are unfavorable prognostic indicators in uterine cervical adenocarcinoma. Cancer Sci (2018) 109:863. doi: 10.1111/CAS.13476
76. Miyasato Y, Shiota T, Ohnishi K, Pan C, Yano H, Horlad H, et al. High density of CD204-positive macrophages predicts worse clinical prognosis in patients with breast cancer. Cancer Sci (2017) 108:1693. doi: 10.1111/CAS.13287
77. Sun Y, Xu S. Tumor-associated CD204-positive macrophage is a prognostic marker in clinical stage i lung adenocarcinoma. BioMed Res Int (2018) 2018:8459193. doi: 10.1155/2018/8459193
78. Yanai Y, Kosaka T, Mikami S, Hongo H, Yasumizu Y, Takeda T, et al. CD8-positive T cells and CD204-positive M2-like macrophages predict postoperative prognosis of very high-risk prostate cancer. Sci Rep (2021) 11:1–7. doi: 10.1038/s41598-021-01900-4
79. Getts DR, Martin AJ, Mccarthy DP, Terry RL, Hunter ZN, Yap WT, et al. Microparticles bearing encephalitogenic peptides induce T-cell tolerance and ameliorate experimental autoimmune encephalomyelitis. Nat Biotechnol (2012) 30:1217. doi: 10.1038/NBT.2434
80. Lenahan C, Huang L, Travis ZD, Zhang JH. Scavenger receptor class b type 1 (SR-B1) and the modifiable risk factors of stroke. Chin Neurosurg J (2019) 5:1–10. doi: 10.1186/S41016-019-0178-3
81. Xu G-H, Lou N, Shi H-C, Xu Y-C, Ruan H-L, Xiao W, et al. Up-regulation of SR-BI promotes progression and serves as a prognostic biomarker in clear cell renal cell carcinoma. BMC Cancer (2018) 18:1–12. doi: 10.1186/S12885-017-3761-Z/FIGURES/6
82. Zheng Y, Liu Y, Jin H, Pan S, Qian Y, Huang C, et al. Scavenger receptor BI is a potential biomarker of human nasopharyngeal carcinoma and its growth is inhibited by HDL-mimetic nanoparticles. Theranostics (2013) 3:477–86. doi: 10.7150/THNO.6617
83. Brundert M, Heeren J, Bahar-Bayansar M, Ewert A, Moore KJ, Rinninger F. Selective uptake of HDL cholesteryl esters and cholesterol efflux from mouse peritoneal macrophages independent of SR-BI. J Lipid Res (2006) 47:2408–21. doi: 10.1194/JLR.M600136-JLR200
84. Alicia Traughber C, Opoku E, Brubaker G, Major J, Lu H, Lorkowski SW, et al. Uptake of high-density lipoprotein by scavenger receptor class b type 1 is associated with prostate cancer proliferation and tumor progression in mice. J Biol Chem (2020) 295:8252–61. doi: 10.1074/JBC.RA120.013694
85. Ma X, Li SF, Qin ZS, Ye J, Zhao ZL, Fang HH, et al. Propofol up-regulates expression of ABCA1, ABCG1, and SR-B1 through the PPARγ/LXRα signaling pathway in THP-1 macrophage-derived foam cells. Cardiovasc Pathol (2015) 24:230–5. doi: 10.1016/J.CARPATH.2014.12.004
86. Tao H, Yancey PG, Babaev VR, Blakemore JL, Zhang Y, Ding L, et al. Macrophage SR-BI mediates efferocytosis via Src/PI3K/Rac1 signaling and reduces atherosclerotic lesion necrosis. J Lipid Res (2015) 56:1449–60. doi: 10.1194/JLR.M056689
87. Feng H, Wang M, Wu C, Yu J, Wang D, Ma J, et al. High scavenger receptor class b type I expression is related to tumor aggressiveness and poor prognosis in lung adenocarcinoma: A STROBE compliant article. Med (Baltimore) (2018) 97:e0203. doi: 10.1097/MD.0000000000010203
88. Li J, Wang J, Li M, Yin L, Li XA, Zhang TG. Up-regulated expression of scavenger receptor class b type 1 (SR-B1) is associated with malignant behaviors and poor prognosis of breast cancer. Pathol - Res Pract (2016) 212:555–9. doi: 10.1016/J.PRP.2016.03.011
89. Clemetson KJ, Clemetson JM. Platelet receptors. Platelets (2013) 9:169–94. doi: 10.1016/B978-0-12-387837-3.00009-2
90. Woo MS, Yang J, Beltran C, Cho S. Cell surface CD36 protein in Monocyte/Macrophage contributes to phagocytosis during the resolution phase of ischemic stroke in mice. J Biol Chem (2016) 291:23654. doi: 10.1074/JBC.M116.750018
91. Abumrad N, Storch J. Role of membrane and cytosolic fatty acid binding proteins in lipid processing by the small intestine. Physiol Gastroint Tract (2006) 2:1693–709. doi: 10.1016/B978-012088394-3/50069-6
92. Huh HY, Lo SK, Yesner LM, Silverstein RL. CD36 induction on human monocytes upon adhesion to tumor necrosis factor-activated endothelial cells. J Biol Chem (1995) 270:6267–71. doi: 10.1074/JBC.270.11.6267
93. Wei J, Lu Y, Wang R, Xu X, Liu Q, He S, et al. MicroRNA-375: potential cancer suppressor and therapeutic drug. Biosci Rep (2021) 41:BSR20211494. doi: 10.1042/BSR20211494
94. Luo X, Zheng E, Wei L, Zeng H, Qin H, Zhang X, et al. The fatty acid receptor CD36 promotes HCC progression through activating Src/PI3K/AKT axis-dependent aerobic glycolysis. Cell Death Dis (2021) 12:1–14. doi: 10.1038/s41419-021-03596-w
95. Bitorina AV, Oligschlaeger Y, Shiri-Sverdlov R, Theys J. Low profile high value target: The role of OxLDL in cancer. Biochim Biophys Acta - Mol Cell Biol Lipids (2019) 1864:158518. doi: 10.1016/J.BBALIP.2019.158518
96. Yang P, Su C, Luo X, Zeng H, Zhao L, Wei L, et al. Dietary oleic acid-induced CD36 promotes cervical cancer cell growth and metastasis via up-regulation Src/ERK pathway. Cancer Lett (2018) 438:76–85. doi: 10.1016/J.CANLET.2018.09.006
97. Pan J, Fan Z, Wang Z, Dai Q, Xiang Z, Yuan F, et al CD36 mediates palmitate acid-induced metastasis of gastric cancer via AKT/GSK-3β/β-catenin pathway. J Experim Clin Cancer Res (2019) 38:52. doi: 10.1186/s13046-019-1049-7
98. Chen Y, Zhang J, Cui W, Silverstein RL. CD36, a signaling receptor and fatty acid transporter that regulates immune cell metabolism and fate. J Exp Med (2022) 219:e20211314. doi: 10.1084/JEM.20211314/213166
99. Chen YJ, Liao WX, Huang SZ, Yu YF, Wen JY, Chen J, et al. Prognostic and immunological role of CD36: A pan-cancer analysis. J Cancer (2021) 12:4762. doi: 10.7150/JCA.50502
100. Medrek C, Pontén F, Jirström K, Leandersson K. The presence of tumor associated macrophages in tumor stroma as a prognostic marker for breast cancer patients. BMC Cancer (2012) 12:306. doi: 10.1186/1471-2407-12-306
101. Mwafy SE, El-Guindy DM. Pathologic assessment of tumor-associated macrophages and their histologic localization in invasive breast carcinoma. J Egypt Natl Canc Inst (2020) 32:1–11. doi: 10.1186/S43046-020-0018-8/TABLES/7
102. Mohammed ZMA, Going JJ, Edwards J, Elsberger B, Doughty JC, McMillan DC. The relationship between components of tumour inflammatory cell infiltrate and clinicopathological factors and survival in patients with primary operable invasive ductal breast cancer. Br J Cancer (2012) 107:864. doi: 10.1038/BJC.2012.347
103. Rakaee M, Busund LTR, Jamaly S, Paulsen EE, Richardsen E, Andersen S, et al. Prognostic value of macrophage phenotypes in resectable non–small cell lung cancer assessed by multiplex immunohistochemistry. Neoplasia (2019) 21:282. doi: 10.1016/J.NEO.2019.01.005
104. Nakayama Y, Nagashima N, Minagawa N, Inoue Y, Katsuki T, Onitsuka K, et al. Relationships between tumor-associated macrophages and clinicopathological factors in patients with colorectal cancer. Anticancer Res (2002) 22:4291–6.
105. Gulubova M, Ananiev J, Yovchev Y, Julianov A, Karashmalakov A, Vlaykova T. The density of macrophages in colorectal cancer is inversely correlated to TGF-β1 expression and patients’ survival. J Mol Histol (2013) 44:679–92. doi: 10.1007/S10735-013-9520-9
106. González-Chavarría I, Cerro RP, Parra NP, Sandoval FA, Zuñiga FA, Omazábal VA, et al. Lectin-like oxidized LDL receptor-1 is an enhancer of tumor angiogenesis in human prostate cancer cells. PloS One (2014) 9:e106219. doi: 10.1371/JOURNAL.PONE.0106219
107. Chen M, Masaki T, Sawamura T. LOX-1, the receptor for oxidized low-density lipoprotein identified from endothelial cells: Implications in endothelial dysfunction and atherosclerosis. Pharmacol Ther (2002) 95:89–100. doi: 10.1016/S0163-7258(02)00236-X
108. Barreto J, Karathanasis SK, Remaley A, Sposito AC. Role of LOX-1 (Lectin-like oxidized low-density lipoprotein receptor 1) as a cardiovascular risk predictor. Arterioscler Thromb Vasc Biol (2021) 41:153–66. doi: 10.1161/ATVBAHA.120.315421
109. Loures FV, Araújo EF, Feriotti C, Bazan SB, Costa TA, Brown GD, et al. Dectin-1 induces M1 macrophages and prominent expansion of CD8+IL-17+ cells in pulmonary paracoccidioidomycosis. J Infect Dis (2014) 210:762–73. doi: 10.1093/INFDIS/JIU136
110. Serezani CH, Kane S, Collins L, Morato-Marques M, Osterholzer JJ, Peters-Golden M. Macrophage dectin-1 expression is controlled by leukotriene B4 via a GM-CSF/PU.1 axis. J Immunol (2012) 189:906–15. doi: 10.4049/JIMMUNOL.1200257
111. Bhaskaran N, Jayaraman S, Quigley C, Mamileti P, Ghannoum M, Weinberg A, et al. The role of dectin-1 signaling in altering tumor immune microenvironment in the context of aging. Front Oncol (2021) 11:669066/BIBTEX. doi: 10.3389/FONC.2021.669066/BIBTEX
112. Martinez-Pomares L. The mannose receptor. J Leukoc Biol (2012) 92:1177–86. doi: 10.1189/JLB.0512231
113. Fan W, Yang X, Huang F, Tong X, Zhu L, Wang S. Identification of CD206 as a potential biomarker of cancer stem-like cells and therapeutic agent in liver cancer. Oncol Lett (2019) 18:3218. doi: 10.3892/OL.2019.10673
114. Guo Z, Song J, Hao J, Zhao H, Du X, Li E, et al. M2 macrophages promote NSCLC metastasis by upregulating CRYAB. Cell Death Dis (2019) 10:1–11. doi: 10.1038/s41419-019-1618-x
115. Feng Q, Chang W, Mao Y, He G, Zheng P, Tang W, et al. Tumor-associated macrophages as prognostic and predictive biomarkers for postoperative adjuvant chemotherapy in patients with stage II colon cancer. Clin Cancer Res (2019) 25:3896–907. doi: 10.1158/1078-0432.CCR-18-2076
116. Shimaoka T, Nakayama T, Fukumoto N, Kume N, Takahashi S, Yamaguchi J, et al. Cell surface-anchored SR-PSOX/CXC chemokine ligand 16 mediates firm adhesion of CXC chemokine receptor 6-expressing cells. J Leukoc Biol (2004) 75:267–74. doi: 10.1189/JLB.1003465
117. Shimaoka T, Kume N, Minami M, Hayashida K, Kataoka H, Kita T, et al. Molecular cloning of a novel scavenger receptor for oxidized low density lipoprotein, SR-PSOX, on macrophages. J Biol Chem (2000) 275:40663–6. doi: 10.1074/JBC.C000761200
118. Cai H, Zhu XD, Ao JY, Ye BG, Zhang YY, Chai ZT, et al. Colony-stimulating factor-1-induced AIF1 expression in tumor-associated macrophages enhances the progression of hepatocellular carcinoma. Oncoimmunology (2017) 6:e1333213. doi: 10.1080/2162402X.2017.1333213
119. Neeper M, Schmidt AM, Brett J, Yan SD, Wang F, Pan YCE, et al. Cloning and expression of a cell surface receptor for advanced glycosylation end products of proteins. J Biol Chem (1992) 267:14998–5004. doi: 10.1016/S0021-9258(18)42138-2
120. Sims GP, Rowe DC, Rietdijk ST, Herbst R, Coyle AJ. HMGB1 and RAGE in inflammation and cancer. Annu Rev Immunol (2010) 28:367–88. doi: 10.1146/ANNUREV.IMMUNOL.021908.132603
121. Leclerc E, Fritz G, Vetter SW, Heizmann CW. Binding of S100 proteins to RAGE: an update. Biochim Biophys Acta (2009) 1793:993–1007. doi: 10.1016/J.BBAMCR.2008.11.016
122. Yan S, Chen X, Fu J, Chen M, Zhu H, Roher A, et al. RAGE and amyloid-beta peptide neurotoxicity in alzheimer’s disease. Nature (1996) 382:685–91. doi: 10.1038/382685A0
123. Park HJ, Boyington JC. The 1.5 Å crystal structure of human receptor for advanced glycation endproducts (RAGE) ectodomains reveals unique features determining ligand binding. J Biol Chem (2010) 285:40762–70. doi: 10.1074/JBC.M110.169276
124. Azizian-Farsani F, Abedpoor N, Hasan Sheikhha M, Gure AO, Nasr-Esfahani MH, Ghaedi K. Receptor for advanced glycation end products acts as a fuel to colorectal cancer development. Front Oncol (2020) 10:552283. doi: 10.3389/FONC.2020.552283
125. Yang H, Wang H, Andersson U. Targeting inflammation driven by HMGB1. Front Immunol (2020) 11:484. doi: 10.3389/FIMMU.2020.00484
126. Yang H, Liu H, Zeng Q, Imperato GH, Addorisio ME, Li J, et al. Inhibition of HMGB1/RAGE-mediated endocytosis by HMGB1 antagonist box a, anti-HMGB1 antibodies, and cholinergic agonists suppresses inflammation. Mol Med (2019) 25:13. doi: 10.1186/S10020-019-0081-6
127. Fan H, Tang HB, Chen Z, Wang HQ, Zhang L, Jiang Y, et al. Inhibiting HMGB1-RAGE axis prevents pro-inflammatory macrophages/microglia polarization and affords neuroprotection after spinal cord injury. J Neuroinflamm (2020) 17:295. doi: 10.1186/S12974-020-01973-4
128. Rendra E, Riabov V, Mossel DM, Sevastyanova T, Harmsen MC, Kzhyshkowska J. Reactive oxygen species (ROS) in macrophage activation and function in diabetes. Immunobiology (2019) 224:242–53. doi: 10.1016/J.IMBIO.2018.11.010
129. Li Z, Fu WJ, Chen XQ, Wang S, Deng RS, Tang XP, et al. Autophagy-based unconventional secretion of HMGB1 in glioblastoma promotes chemosensitivity to temozolomide through macrophage M1-like polarization. J Exp Clin Cancer Res (2022) 41:74. doi: 10.1186/S13046-022-02291-8
130. He C, Sun S, Zhang Y, Xie F, Li S. The role of irreversible electroporation in promoting M1 macrophage polarization via regulating the HMGB1-RAGE-MAPK axis in pancreatic cancer. Oncoimmunology (2021) 10:1897295. doi: 10.1080/2162402X.2021.1897295
131. Nasser MW, Qamri Z, Deol YS, Ravi J, Powell CA, Trikha P, et al. S100A7 enhances mammary tumorigenesis through upregulation of inflammatory pathways. Cancer Res (2012) 72:604–15. doi: 10.1158/0008-5472.CAN-11-0669
132. Kzhyshkowska J. Multifunctional receptor stabilin-1 in homeostasis and disease. ScientificWorldJournal (2010). 10:2039–53. doi: 10.1100/tsw.2010.189
133. Harris EN, Baker E. Role of the hyaluronan receptor, stabilin-2/HARE, in health and disease. Int J Mol Sci 2020 Vol 21 Page 3504 (2020) 21:3504. doi: 10.3390/IJMS21103504
134. Politz O, Gratchev A, McCourt PPAG, Schledzewski K, Guillot P, Johansson SS, et al. Stabilin-1 and -2 constitute a novel family of fasciclin-like hyaluronan receptor homologues. Biochem J (2002) 362:155–64. doi: 10.1042/0264-6021:3620155
135. Palani S, Maksimow M, Miiluniemi M, Auvinen K, Jalkanen S, Salmi M. Stabilin-1/CLEVER-1, a type 2 macrophage marker, is an adhesion and scavenging molecule on human placental macrophages. Eur J Immunol (2011) 41:2052–63. doi: 10.1002/EJI.201041376
136. Irjala H, Johansson EL, Merinen M, Kontula K, Alanen K, Grenman R, et al. The same endothelial receptor controls lymphocyte traffic both in vascular and lymphatic vessels. Eur J Immunol (2003) 33:815–24. doi: 10.1002/EJI.200323859
137. Kzhyshkowska J, Gratchev A, Goerdt S. Stabilin-1, a homeostatic scavenger receptor with multiple functions. J Cell Mol Med (2006) 10:635. doi: 10.1111/J.1582-4934.2006.TB00425.X
138. Hollmén M, Figueiredo CR, Jalkanen S. New tools to prevent cancer growth and spread: A ‘Clever’ approach. Br J Cancer (2020) 123:501–9. doi: 10.1038/s41416-020-0953-0
139. Kzhyshkowska J, Gratchev A, Martens J-H, Pervushina O, Mamidi S, Johansson S, et al. Stabilin-1 localizes to endosomes and the trans-golgi network in human macrophages and interacts with GGA adaptors. J Leukoc Biol (2004) 76:1151–61. doi: 10.1189/JLB.0504300
140. Kzhyshkowska J, Gratchev A, Brundiers H, Mamidi S, Krusell L, Goerdt S. Phosphatidylinositide 3-kinase activity is required for stabilin-1-mediated endosomal transport of acLDL. Immunobiology (2005) 210:161–73. doi: 10.1016/j.imbio.2005.05.022
141. Kzhyshkowska J, Mamidi S, Gratchev A, Kremmer E, Schmuttermaier C, Krusell L, et al. Novel stabilin-1 interacting chitinase-like protein (SI-CLP) is up-regulated in alternatively activated macrophages and secreted via lysosomal pathway. Blood (2006) 107:3221–8. doi: 10.1182/blood-2005-07-2843
142. Kzhyshkowska J, Gratchev A, Schmuttermaier C, Brundiers H, Krusell L, Mamidi S, et al. Alternatively activated macrophages regulate extracellular levels of the hormone placental lactogen via receptor-mediated uptake and transcytosis. J Immunol (2008) 180:3028–37. doi: 10.4049/JIMMUNOL.180.5.3028
143. Park S, Jung M, Lee S, Kang K, Gratchev A, Riabov V, et al. Stabilin-1 mediates phosphatidylserine-dependent clearance of cell corpses in alternatively activated macrophages. J Cell Sci (2009) 122:3365–73. doi: 10.1242/JCS.049569
144. Silva-Bermudez LS, Sevastyanova TN, Schmuttermaier C, de la Torre C, Schumacher L, Klüter H, et al. Titanium nanoparticles enhance production and suppress stabilin-1-Mediated clearance of GDF-15 in human primary macrophages. Front Immunol (2021) 12:760577/XML/NLM. doi: 10.3389/FIMMU.2021.760577/XML/NLM
145. Karikoski M, Marttila-Ichihara F, Elima K, Rantakari P, Hollmén M, Kelkka T, et al. Clever-1/stabilin-1 controls cancer growth and metastasis. Clin Cancer Res (2014) 20:6452–64. doi: 10.1158/1078-0432.CCR-14-1236
146. Salmi M, Koskinen K, Henttinen T, Elima K, Jalkanen S. CLEVER-1 mediates lymphocyte transmigration through vascular and lymphatic endothelium. Blood (2004) 104:3849–57. doi: 10.1182/BLOOD-2004-01-0222
147. Virtakoivu R, Rannikko J, Viitala M, Vaura F, Takeda A, Lönnberg T, et al. Systemic blockade of clever-1 elicits lymphocyte activation alongside checkpoint molecule downregulation in patients with solid tumors. medRxiv (2020) 2020:11.11.20227777. doi: 10.1101/2020.11.11.20227777
148. Schledzewski K, Falkowski M, Moldenhauer G, Metharom P, Kzhyshkowska J, Ganss R, et al. Lymphatic endothelium-specific hyaluronan receptor LYVE-1 is expressed by stabilin-1+, F4/80+, CD11b+ macrophages in malignant tumours and wound healing tissue in vivo and in bone marrow cultures in vitro: implications for the assessment of lymphangiogenesis. J Pathol (2006) 209:67–77. doi: 10.1002/PATH.1942
149. Viitala M, Virtakoivu R, Tadayon S, Rannikko J, Jalkanen S, Hollmen M. Immunotherapeutic blockade of macrophage clever-1 reactivates the CD8 + T-cell response against immunosuppressive tumors. Clin Cancer Res (2019) 25:3289–303. doi: 10.1158/1078-0432.CCR-18-3016
150. Yin SP, Gao Y, Xie XS, Xu DD, Riabov V, Du WD. Accumulation of stabilin-1 positive macrophages in the early stage of gastric cancer is associated with short cumulative survival. Oncol Lett (2020) 19:2404–12. doi: 10.3892/OL.2020.11310
151. Zhang J, Gratchev A, Riabov V, Mamidi S, Schmuttermaier C, Krusell L, et al. A novel GGA-binding site is required for intracellular sorting mediated by stabilin-1. Mol Cell Biol (2009) 29:6097–105. doi: 10.1128/MCB.00505-09
152. Kzhyshkowska J, Yin S, Liu T, Riabov V, Mitrofanova I. Role of chitinase-like proteins in cancer. Biol Chem (2016) 397:231–47. doi: 10.1515/hsz-2015-0269
153. Yin S, Wang N, Riabov V, Mossel DM, Larionova I, Schledzewski K, et al. SI-CLP inhibits the growth of mouse mammary adenocarcinoma by preventing recruitment of tumor-associated macrophages. Int J Cancer (2020) 146:1396–408. doi: 10.1002/ijc.32685
154. Areshkov PO, Avdieiev SS, Balynska OV, LeRoith D, Kavsan VM. Two closely related human members of chitinase-like family, CHI3L1 and CHI3L2, activate ERK1/2 in 293 and U373 cells but have the different in-fluence on cell proliferation. Int J Biol Sci (2011) 8:39–48. doi: 10.7150/IJBS.8.39
155. Liu L, Yang Y, Duan H, He J, Sun L, Hu W, et al. CHI3L2 is a novel prognostic biomarker and correlated with immune infiltrates in gliomas. Front Oncol (2021) 11:611038/BIBTEX. doi: 10.3389/FONC.2021.611038/BIBTEX
156. PrabhuDas MR, Baldwin CL, Bollyky PL, Bowdish DME, Drickamer K, Febbraio M, et al. A consensus definitive classification of scavenger receptors and their roles in health and disease. J Immunol (2017) 198:3775–89. doi: 10.4049/JIMMUNOL.1700373
157. Matuschik L, Riabov V, Schmuttermaier C, Sevastyanova T, Weiss C, Klüter H, et al. Hyperglycemia induces inflammatory response of human macrophages to CD163-mediated scavenging of hemoglobin-haptoglobin complexes. Int J Mol Sci (2022) 23:1385. doi: 10.3390/IJMS23031385
158. Chen Y, Gao Y, Ma X, Wang Y, Liu J, Yang C, et al. A study on the correlation between M2 macrophages and regulatory T cells in the progression of colorectal cancer. Int J Biol Markers (2022) 37:412–20. doi: 10.1177/03936155221132572/ASSET/IMAGES/LARGE/10.1177_03936155221132572-FIG3.JPEG
159. He Y, Han Y, Hui FA, Li D, Wang B, Ji K, et al. Multi-perspective comparison of the immune microenvironment of primary colorectal cancer and liver metastases. J Transl Med (2022) 20:1–13. doi: 10.1186/S12967-022-03667-2/FIGURES/6
160. Minami K, Hiwatashi K, Ueno S, Sakoda M, Iino S, Okumura H, et al. Prognostic significance of CD68, CD163 and folate receptor-β positive macrophages in hepatocellular carcinoma. Exp Ther Med (2018) 15:4465–76. doi: 10.3892/ETM.2018.5959/HTML
161. Xu X, Zhou Y, Feng X, Li X, Asad M, Li D, et al. Germline genomic patterns are associated with cancer risk, oncogenic pathways, and clinical outcomes. Sci Adv (2020) 6:4905–32. doi: 10.1126/SCIADV.ABA4905/SUPPL_FILE/ABA4905_SM.PDF
162. Orloff M, Peterson C, He X, Ganapathi S, Heald B, Yang YR, et al. Germline mutations in MSR1, ASCC1, and CTHRC1 in patients with Barrett esophagus and esophageal adenocarcinoma. JAMA (2011) 306:410–9. doi: 10.1001/JAMA.2011.1029
163. Xu J, Lilly Zheng S, Komiya A, Mychaleckyj JC, Isaacs SD, Hu JJ, et al. Germline mutations and sequence variants of the macrophage scavenger receptor 1 gene are associated with prostate cancer risk. Nat Genet (2002) 32:321–5. doi: 10.1038/ng994
164. Feng H, Wang M, Wu C, Yu J, Wang D, Ma J, et al. High scavenger receptor class b type i expression is related to tumor aggressiveness and poor prognosis in lung adenocarcinoma. Med (United States) (2018) 97:e0203. doi: 10.1097/MD.0000000000010203
165. Lepland A, Malfanti A, Haljasorg U, Asciutto EK, Pickholz M, Bringas M, et al. Depletion of CD206+ tumour macrophages via a peptide-targeted star-shaped polyglutamate inhibits tumourigenesis and metastatic dissemination in mouse breast cancer models. bioRxiv (2021) 2021:12.29.474487. doi: 10.1101/2021.12.29.474487
166. Georgoudaki AM, Prokopec KE, Boura VF, Hellqvist E, Sohn S, Östling J, et al. Reprogramming tumor-associated macrophages by antibody targeting inhibits cancer progression and metastasis. Cell Rep (2016) 15:2000–11. doi: 10.1016/J.CELREP.2016.04.084
167. Eisinger S, Sarhan D, Boura VF, Ibarlucea-Benitez I, Tyystjärvi S, Oliynyk G, et al. Targeting a scavenger receptor on tumor-associated macrophages activates tumor cell killing by natural killer cells. Proc Natl Acad Sci U.S.A. (2020) 117:32005–16. doi: 10.1073/PNAS.2015343117/SUPPL_FILE/PNAS.2015343117.SM02.MP4
168. Pascual G, Avgustinova A, Mejetta S, Martín M, Castellanos A, Attolini CSO, et al. Targeting metastasis-initiating cells through the fatty acid receptor CD36. Nature (2017) 541:41–5. doi: 10.1038/nature20791
169. Probst P, Simmons R, Dinh H, Zuck M, Wall V, Bouchlaka M, et al. 271 development of OR2805, an anti-CD163 antibody derived from an elite responder to checkpoint inhibitor therapy that relieves immunosuppression caused by M2c macrophages. J Immunother Cancer (2021) 9:A294–4. doi: 10.1136/JITC-2021-SITC2021.271
Keywords: tumor-associated macrophage, scavenger receptor, angiogenesis, extracellular matrix, cancer, tumor microenvironment, endocytosis, phagocytosis
Citation: Kazakova E, Iamshchikov P, Larionova I and Kzhyshkowska J (2023) Macrophage scavenger receptors: Tumor support and tumor inhibition. Front. Oncol. 12:1096897. doi: 10.3389/fonc.2022.1096897
Received: 12 November 2022; Accepted: 13 December 2022;
Published: 06 January 2023.
Edited by:
Hans Raskov, Zealand University Hospital, DenmarkReviewed by:
George S. Karagiannis, Albert Einstein College of Medicine, United StatesAndras G. Lacko, University of North Texas Health Science Center, United States
Pil Soo Sung, The Catholic University of Korea, South Korea
Copyright © 2023 Kazakova, Iamshchikov, Larionova and Kzhyshkowska. This is an open-access article distributed under the terms of the Creative Commons Attribution License (CC BY). The use, distribution or reproduction in other forums is permitted, provided the original author(s) and the copyright owner(s) are credited and that the original publication in this journal is cited, in accordance with accepted academic practice. No use, distribution or reproduction is permitted which does not comply with these terms.
*Correspondence: Julia Kzhyshkowska, SnVsaWEuS3poeXNoa293c2thQG1lZG1hLnVuaS1oZWlkZWxiZXJnLmRl