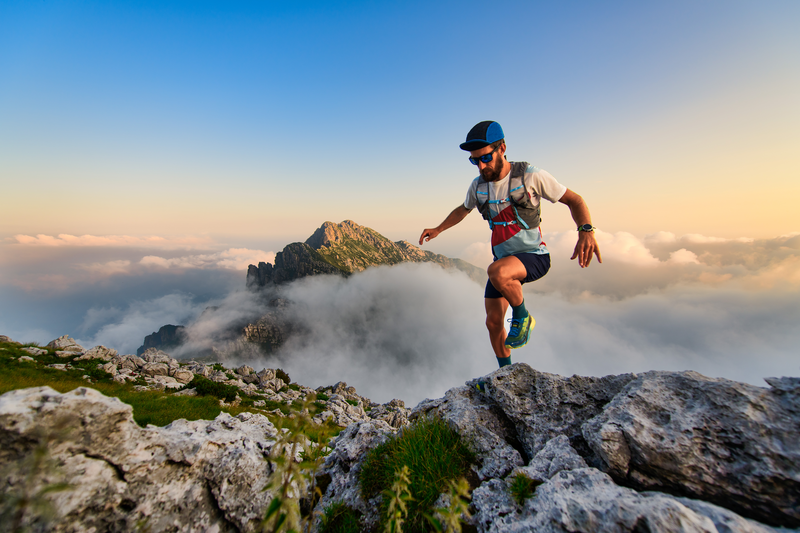
94% of researchers rate our articles as excellent or good
Learn more about the work of our research integrity team to safeguard the quality of each article we publish.
Find out more
MINI REVIEW article
Front. Oncol. , 20 December 2022
Sec. Pharmacology of Anti-Cancer Drugs
Volume 12 - 2022 | https://doi.org/10.3389/fonc.2022.1091782
This article is part of the Research Topic Overcoming Physiologic Barriers to Treatments for Hematologic Malignancies by Molecularly Targeting the Tumor Microenvironment View all 10 articles
Immune evasion through up-regulating checkpoint inhibitory receptors on T cells plays an essential role in tumor initiation and progression. Therefore, immunotherapy, including immune checkpoint inhibitor targeting programmed cell death protein 1 (PD-1) and chimeric antigen receptor T cell (CAR-T) therapy, has become a promising strategy for hematological malignancies. T cell immunoreceptor with immunoglobulin and ITIM domain (TIGIT) is a novel checkpoint inhibitory receptor expressed on immune cells, including cytotoxic T cells, regulatory T cells, and NK cells. TIGIT participates in immune regulation via binding to its ligand CD155. Blockage of TIGIT has provided evidence of considerable efficacy in solid tumors in preclinical research and clinical trials, especially when combined with PD-1 inhibition. However, the mechanism and function of TIGIT in hematological malignancies have not been comprehensively studied. In this review, we focus on the role of TIGIT in hematological malignancies and discuss therapeutic strategies targeting TIGIT, which may provide a promising immunotherapy target for hematological malignancies.
Hematological malignancy is a group of clonal malignant diseases of the hemopoietic system with highly invasive potential and heterogeneity. Despite those treatments, including chemotherapy and stem cell transplantation, improving survival, some patients still experience disease relapse without long-term survival, partly due to tumor evasion from immune recognition and killing by effector cells (1–3). In recent years, immune checkpoint blockade (ICB) therapy targeting cytotoxic T lymphocyte-associated protein 4 (CTLA-4) or programmed cell death protein 1 (PD-1), and chimeric antigen receptor T cell (CAR-T) therapy utilizing genetic engineering to alter T cells to produce transmembrane proteins on the cell surface with an extracellular antibody fragment domain that recognizes tumor antigen, brings a new direction for cancer immunotherapy (4–6). Although anti-PD-1 monoclonal antibodies (mAbs) and CAR-T therapies have been actively applied in relapsed and refractory lymphoma, multiple myeloma (MM), and leukemia, which also achieved remarkable success in some cases, a part of patients still have no response to these therapies (7–10). Therefore, in-depth research on immune checkpoint molecules’ interaction mechanisms and the discovery of novel target to overcome the barrier of immunotherapy are necessary. In addition, immune checkpoint inhibitor-related toxicity is another challenge. For example, Quagliariello reported that nivolumab and pembrolizumab would induce cardiotoxicity by increasing the inflammation of cardiomyocytes (11, 12). T cell immunoglobulin and ITIM domain (TIGIT), another inhibitory immune checkpoint molecule, has emerged as a potential target in cancer immunotherapy (13, 14). In this review, we focus on the immunomodulatory role and mechanism of TIGIT, discuss its potential as an immune target in hematological malignancies.
TIGIT, also named as V-set and immunoglobulin domain-containing protein 9 (VSIG9), V-set and transmembrane do-maincontaining protein 3 (VSTM3) and Washington University cell adhesion molecule (WUCAM), is a co-inhibitory molecule belonging to the immunoglobulin superfamily that was first discovered in 2009 (15–17). It consists of an extracellular immunoglobulin variable (IgV) domain, a type I transmembrane domain and an intracellular domain with an immunoreceptor tyrosine-based inhibitory motif (ITIM) and an immunoglobulin tail tyrosine (ITT)-like motif (18). TIGIT is exclusively expressed on natural killer (NK) cells and T cells, including CD8+ T cells, CD4+ T cells, and regulatory T cells (Tregs) (19, 20).
The relationship between TIGIT, its ligands, and competitive receptors is complex. On the one hand, the immunoglobulin variable domain of TIGIT shares sequence homology with members of the polio virus receptors (PVR) family, including CD155 (also named as Necl-5 or PVR), CD112 (also named as Nectin-2 or PVRL2), CD113 (also named as Nectin-3 or PVRL3), and Nectin-4 (PVRL4) (21, 22). CD155 is a member of the immunoglobulin superfamily, mainly expressed on dendritic cells (DCs), macrophages, and lymphocytes. CD112 belongs to single-pass type-I membrane glycoproteins, which is expressed on DCs and monocytes. Interestingly, CD155 and CD112 are over-expressed on different cancer cells as reported recently (23–26). In addition, CD155 has a higher affinity than CD112 to TIGIT, which became the primary ligand for TIGIT (27). By interacting with its ligands, TIGIT participates in the regulation of cellular immune function. On the other hand, TIGIT shares these ligands with other receptors, including CD226 (DNAM-1) and CD96 (TACTILE). As the costimulatory receptor, CD226 competes with TIGIT for binding to CD155 in spite of its lower affinity (27). Furthermore, CD226 also competes with TIGIT and CD112R (PVRIG) for binding to CD112 (28, 29). Therefore, CD226 also plays an essential role in immune regulation (Figure 1).
Figure 1 The interaction of TIGIT family receptors and ligands. TIGIT, CD226, CD96 and CD112R are expressed on T cells and NK cells. The ligands CD155, CD113, CD112 and PVRL4 are expressed on tumor cells or APCs. TIGIT delivers inhibitory signals by binding to CD155, CD113, CD112 and PVRL4, with highest affinity for CD155. CD226 and CD96 compete with TIGIT for binding to CD155, but with lower affinity than TIGIT. CD226 delivers activating signals. However, whether CD96 triggers inhibitory or activating signals remains to be determined. CD112R and CD226 also competitively binding to CD112, with higher affinity with CD112R. APCs, antigen presenting cells.
Through complex interaction with ligands, TIGIT family receptors transfer inhibitory signals to immune cells, contributing to innate and adaptive immunity regulation (30–32). On the one hand, TIGIT inhibits the activity of T cells intrinsically. Firstly, TIGIT binds to CD155 and transmits intracellular inhibitory signals, directly suppressing T cell receptor (TCR) expression and signaling. Engagement of TIGIT induces down-regulation of the TCRα chain and molecules that comprise the TCR complex, as well as reduction of TCR-induced p-ERK signaling and interferon-γ (IFNγ) production in CD8+ T cells (33, 34). Secondly, TIGIT possesses a higher affinity of CD155 when competing with its costimulatory counterpart CD226, which impaires T cell function by either directly disrupting homodimerization of CD226 or decreasing expression of T-bet and production of IFNγ (35, 36).
On the other hand, TIGIT can exogenously enhance the immunosuppressive functions of Treg cells. TIGIT is enriched in Treg cells, which is associated with the suppressive capacity of effector T cells. Conversely, CD226 inhibits the expansion of Treg cells and promotes the secretion of IFNγ and other effector cytokines (37, 38). TIGIT expression on Treg cells also suppresses the proliferation of effector T cells via increased production of interleukin-10 (IL-10) and fibrinogen-like protein 2 (Fgl2), as well as the response of pro-inflammatory T helper 1 (Th1) and Th17 cells, but not Th2 cells (39). Besides, TIGIT can suppress T cell activation through DCs and macrophage-mediated cytokines disturbance. TIGIT interacts with CD155 expressed on DCs, and induces phosphorylation of CD155 through extracellular signal-regulated kinase (ERK) signaling, consequently increasing the production of anti-inflammatory cytokine IL-10 and decreasing pro-inflammatory cytokine IL-12, which inhibits T cell function (40). TIGIT also enhances the secretion of IL-10 and reduces the secretion of IFNγ and tumor necrosis factor α (TNFα) via c-Maf nuclear translocation, which switches macrophages from M1 to anti-inflammatory M2 phenotype (41). In addition, TIGIT also directly induces exhaustion of tumor-infiltrating NK cells with lower expression of IFNγ and TNF or indirectly contributes to exhaustion of CD8+ T cells, impairing anti-tumor immune response (42) (Figure 2).
Figure 2 Mechanism of inhibitory role of TIGIT in immunoregulation. (1) Interaction of TIGIT with CD155 transmits intracellular inhibitory signals, which directly suppressed TCR signal and effector T cell function. (2) TIGIT inhibits CD226-induced T cell activation by disrupting CD226 homodimerization and decreasing IFNγ production. (3) TIGIT directly induces NK cell exhaustion, contributing to inactivation of CD8+ T cell. (4) TIGIT enhances Tregs mediated dysfunction of effector T cell by increased IL-10 and Fgl2, as well as inhibition of Th1 and Th17 cells. (5) TIGIT promotes macrophage switching from pro-inflammatory M1 to anti-inflammatory M2 phenotype through increased IL-10 and decreased IFNγ and TNFα. (6) TIGIT inhibits T cell function by DCs-mediated abnormal secretion of cytokines. IFNγ, interferon-γ; IL-10, interleukin-10; Fgl2, fibrinogen-like protein 2; Th1, T helper 1 cell; Th17, T helper 17 cell; TNFα, tumor necrosis factor α; DCs, dendritic cells; NK, natural killer.
Increasing evidence has demonstrated that TIGIT was highly expressed on tumor-infiltrating lymphocytes (TILs) in different hematological malignancies, resulting in tumor progression and poor outcomes. Josefsson reported that TIGHT expression was significantly higher in T cells of follicular lymphoma (FL) than in healthy controls. Besides, up-regulation of TIGIT was associated with the advanced disease stage (34, 43). Yang also reported that TIGIT expression was increased on TILs in FL, and TIGIT+ T cells predicted worse treatment response and inferior survival (44). Likewise, CD4+ TIGIT+ T cells were increased in chronic lymphocytic leukemia (CLL) patients, which was also correlated with unmutated immunoglobulin heavy chain variable region (IgHv) and advanced stage (45).
Furthermore, TIGIT was similarly up-regulated in classic Hodgkin lymphoma (cHL) and Sezary syndrome (46, 47). In acute myeloid leukemia (AML) patients, elevated TIGIT expression on CD8+ T cells was observed. High TIGIT expression was associated with primary refractory disease and relapse after allogeneic stem cell transplantation (allo-SCT) with poorer survival (48, 49). TIGIT was also reported to be increased on γδ T cells and NK cells, which became an independent risk factor for prognosis (50–52). In addition, increasing frequency of TIGIT on CD8+ T cells was reported in mice models of newly diagnosed and relapsed multiple myeloma, which positively correlated with tumor burden (53, 54). These studies indicated a negative role of TIGIT in anti-tumor immunity. Therefore, targeting TIGIT may be an effective approach for ICB therapy in hematological malignancies.
To date, immunotherapy targeting TIGIT has shown significant anti-tumor effects in several pieces of research. Catakovic reported that blockage of TIGIT by recombinant TIGIT-Fc would reduce CLL viability in vitro due to decreasing production of pro-survival cytokines IL-10 (45). In AML, TIGIT expression inhibited cytokine production and induced apoptosis of CD8+ T cells. Knockdown of TIGIT by siRNA could restore T cell function via decreasing susceptibility to apoptosis, simultaneously increasing production of TNFα and IFNγ. Besides, blockage of TIGIT significantly increased IFNγ production and NK cell degranulation, contributing to NK cells mediated anti-leukemia effects (52, 55).
Similarly, high TIGIT expression promoted T cells exhaustion, leading to myeloma progression. Conversely, the anti-TIGIT treatment prevented T cells exhaustion, decreased growth rate of tumor cells, and prolonged survival of MM mice (53). Guillerey also reported that either TIGIT deficiency or blockage by mAbs restored the immune function of anti-MM CD8+ T cells and improved survival in vivo (54).
In addition, dual blockade of TIGIT and PD-1 showed potential synergistic immune killing effects. On the one hand, Wang observed a higher frequency of TIGIT and PD-1 dual expression in AML patients, which was associated with a higher frequency of FLT3-ITD mutation and a lower remission rate (56). Studies showed that 68-84% of T cells had co-expression of TIGIT and PD-1 in hodgkin lymphoma (HL) (46, 57). A high frequency of TIGIT and PD-1 dual expression was also observed in CLL and FL (34, 58). On the other hand, Zhang reported that blockage of TIGIT alone only up-regulated TNFα in TIGIT+ CD4+ T cells and IFNγ, TNFα in TIGIT+ CD8+ T cells. However, combined inhibition of TIGIT, PD-1, and Tim-3 significantly up-regulated IL-2, IFNγ, and TNFα in both CD4+ and CD8+ T cells, which may enhance anti-leukemia immune responses (59). Based on the remarkable efficacy of anti-TIGIT mAbs in solid tumors and potential immune-killing effects mentioned above in preclinical research, human anti-TIGIT mAbs are being tested in phase 1/2 clinical trials either as a monotherapy or, in most studies, in combination with anti-PD-1/PD-L1 antibodies or chemotherapies for the treatment of malignant lymphoma and multiple myeloma (Table 1). In summary, these researches supported the progress of immunotherapy targeting the TIGIT axis in hematological malignancies.
Even though therapeutic strategy targeting TIGIT has provided evidence of encouraging efficacy in hematological malignancies, the immune-related adverse events (irAEs) mediated by over-activated T cells may result in multiple organ dysfunction and poor prognosis. Phase 1 study of the anti-TIGIT antibody vibostolimab reported that two patients suffered irAEs, including one adrenal insufficiency and one severe skin reaction (60). Another phase 1 study of anti-TIGIT antibody ociperlimab in combination with anti-PD-1 antibody tislelizumab in advanced solid tumors showed that 15 of 26 patients suffered irAEs, including three severe irAEs (grade≥3) (61). CITYSCAPE trial also reported that 69% of patients experienced irAEs after treated with anti-TIGIT antibody tiragolumab and anti-PD-L1 antibody atezolizumab, in which skin rash was the most common, followed by pancreatitis, hypothyroidism, colitis and diabetes mellitus (62). Therefore, clinicians should pay more attention to the immune toxicity of anti-TIGIT therapy.
The immune checkpoint molecule TIGIT plays an inhibitory role in anti-tumor immunity by inactivating immune effector cells. Up-regulation of TIGIT has been reported in various hematological malignancies, which predicts poor outcomes. Preclinical research has demonstrated that blocking TIGIT alone or combined with PD-1 improves anti-tumor immune responses. The clinical evidence of its efficacy in ongoing clinical trials, especially synergized with other immune checkpoint inhibitors, for example PD-1, lymphocyte activation gene 3 (LAG-3) and T-cell immunoglobulin-3 (TIM-3), is eagerly awaited. Furthermore, simultanously blockade of TIGIT and hypoxia-inducible factor 1-alpha (HIF-1α) may also become a potential treatment strategy (63). In the future, a comprehensive understanding of the intricate immunoregulatory network among TIGIT family members and other immune checkpoint molecules may provide more effective options for patients with hematological malignancies.
JZ contributed to the conception of this review, SJ and YZ were responsible for screening literatures and drafting the manuscript, FZ, XC, and JS edited tables and figures. All authors revised the manuscript and JZ gave the final approval of the manuscript.
This study was supported by Zhejiang Provincial Natural Science Foundation of China (LQ21H160036).
The authors declare that the research was conducted in the absence of any commercial or financial relationships that could be construed as a potential conflict of interest.
All claims expressed in this article are solely those of the authors and do not necessarily represent those of their affiliated organizations, or those of the publisher, the editors and the reviewers. Any product that may be evaluated in this article, or claim that may be made by its manufacturer, is not guaranteed or endorsed by the publisher.
ICB, immune checkpoint blockade; CTLA-4, cytotoxic T lymphocyte-associated protein 4; PD-1, programmed cell death protein 1; CAR-T, chimeric antigen receptor T cell; mAbs, monoclonal antibodies; MM, multiple myeloma; TIGIT, T cell immunoglobulin and ITIM domain; VSIG9, V-set and immunoglobulin domain-containing protein 9; VSTM3, V-set and transmembrane do-maincontaining protein 3; WUCAM, Washington University cell adhesion molecule; IgV, immunoglobulin variable; ITIM, immunoreceptor tyrosine-based inhibitory motif; ITT, immunoglobulin tail tyrosine; NK, natural killer; Tregs, regulatory T cells; PVR, polio virus receptors; DCs, dendritic cells; TCR, T cell receptor; IFNγ, interferon-γ; IL-10, interleukin-10; Fgl2, fibrinogen-like protein 2; Th1, T helper 1 cells; ERK, extracellular signal-regulated kinase; TNFα, tumor necrosis factor α; TILs, tumor infiltrating lymphocytes; FL, follicular lymphoma; CLL, chronic lymphocytic leukemia; IgHv, immunoglobulin heavy chain variable region; cHL, classic hodgkin lymphoma; AML, acute myeloid leukemia; allo-SCT, allogeneic stem cell transplantation; irAEs, immune-related adverse events; LAG-3, lymphocyte activation gene 3; TIM-3, T-cell immunoglobulin-3; HIF-1α, hypoxia-inducible factor 1-alpha.
1. Auberger P, Tamburini-Bonnefoy J, Puissant A. Drug resistance in hematological malignancies. Int J Mol Sci (2020) 21(17):6091. doi: 10.3390/ijms21176091
2. Shimada A. Hematological malignancies and molecular targeting therapy. Eur J Pharmacol (2019) 862:172641. doi: 10.1016/j.ejphar.2019.172641
3. Farnault L, Sanchez C, Baier C, Le Treut T, Costello RT. Hematological malignancies escape from NK cell innate immune surveillance: mechanisms and therapeutic implications. Clin Dev Immunol (2012) 2012:421702. doi: 10.1155/2012/421702
4. Salik B, Smyth MJ, Nakamura K. Targeting immune checkpoints in hematological malignancies. J Hematol Oncol (2020) 13(1):111. doi: 10.1186/s13045-020-00947-6
5. Haslauer T, Greil R, Zaborsky N, Geisberger R. CAR T-cell therapy in hematological malignancies. Int J Mol Sci (2021) 22(16):8996. doi: 10.3390/ijms22168996
6. Ok CY, Young KH. Checkpoint inhibitors in hematological malignancies. J Hematol Oncol (2017) 10(1):103. doi: 10.1186/s13045-017-0474-3
7. Annibali O, Crescenzi A, Tomarchio V, Pagano A, Bianchi A, Grifoni A, et al. PD-1 /PD-L1 checkpoint in hematological malignancies. Leuk Res (2018) 67:45–55. doi: 10.1016/j.leukres.2018.01.014
8. Song W, Zhang M. Use of CAR-T cell therapy, PD-1 blockade, and their combination for the treatment of hematological malignancies. Clin Immunol (2020) 214:108382. doi: 10.1016/j.clim.2020.108382
9. Hou JZ, Ye JC, Pu JJ, Liu H, Ding W, Zheng H, et al. Novel agents and regimens for hematological malignancies: recent updates from 2020 ASH annual meeting. J Hematol Oncol (2021) 14(1):66. doi: 10.1186/s13045-021-01077-3
10. Armand P, Lesokhin A, Borrello I, Timmerman J, Gutierrez M, Zhu L, et al. A phase 1b study of dual PD-1 and CTLA-4 or KIR blockade in patients with relapsed/refractory lymphoid malignancies. Leukemia. (2021) 35(3):777–86. doi: 10.1038/s41375-020-0939-1
11. Quagliariello V, Passariello M, Rea D, Barbieri A, Iovine M, Bonelli A, et al. Evidences of CTLA-4 and PD-1 blocking agents-induced cardiotoxicity in cellular and preclinical models. J Pers Med (2020) 10(4):179. doi: 10.3390/jpm10040179
12. Quagliariello V, Passariello M, Coppola C, Rea D, Barbieri A, Scherillo M, et al. Cardiotoxicity and pro-inflammatory effects of the immune checkpoint inhibitor pembrolizumab associated to trastuzumab. Int J Cardiol (2019) 292:171–9. doi: 10.1016/j.ijcard.2019.05.028
13. Chauvin JM, Zarour HM. TIGIT in cancer immunotherapy. J Immunother Cancer (2020) 8(2):e000957. doi: 10.1136/jitc-2020-000957
14. Liu L, You X, Han S, Sun Y, Zhang J, Zhang Y. CD155/TIGIT, a novel immune checkpoint in human cancers (Review). Oncol Rep (2021) 45(3):835–45. doi: 10.3892/or.2021.7943
15. Qiu D, Liu X, Wang W, Jiang X, Wu X, Zheng J, et al. TIGIT axis: novel immune checkpoints in anti−leukemia immunity. Clin Exp Med (2022). doi: s00262-018-2246-5/s10238-022-00817-0
16. Boles KS, Vermi W, Facchetti F, Fuchs A, Wilson TJ, Diacovo TG, et al. A novel molecular interaction for the adhesion of follicular CD4 T cells to follicular dendritic cells. Eur J Immunol (2009) 39(3):695–703. doi: 10.1002/eji.200839116
17. Levin SD, Taft DW, Brandt CS, Bucher C, Howard ED, Chadwick EM, et al. Vstm3 is a member of the CD28 family and an important modulator of T cell function. Eur J Immunol (2011) 41(4):902–15. doi: 10.1002/eji.201041136
18. Harjunpää H, Guillerey C. TIGIT as an emerging immune checkpoint. Clin Exp Immunol (2020) 200(2):108–19. doi: 10.1111/cei.13407
19. Ge Z, Peppelenbosch MP, Sprengers D, Kwekkeboom J. TIGIT, the next step towards successful combination immune checkpoint therapy in cancer. Front Immunol (2021) 12:699895. doi: 10.3389/fimmu.2021.699895
20. Stanietsky N, Simic H, Arapovic J, Toporik A, Levy O, Novik A, et al. The interaction of TIGIT with PVR and PVRL2 inhibits human NK cell cytotoxicity. Proc Natl Acad Sci U S A. (2009) 106(42):17858–63. doi: 10.1073/pnas.0903474106
21. Rotte A, Sahasranaman S, Budha N. Targeting TIGIT for immunotherapy of cancer: Update on clinical development. Biomedicines. (2021) 9(9):1277. doi: 10.3390/biomedicines9091277
22. Reches A, Ophir Y, Stein N, Kol I, Isaacson B, Charpak Amikam Y, et al. Nectin4 is a novel TIGIT ligand which combines checkpoint inhibition and tumor specificity. J Immunother Cancer (2020) 8(1):e000266. doi: 10.1136/jitc-2019-000266
23. Yeo J, Ko M, Lee DH, Park Y, Jin HS. TIGIT/CD226 axis regulates anti-tumor immunity. Pharm (Basel) (2021) 14(3):200. doi: 10.3390/ph14030200
24. Masson D, Jarry A, Baury B, Blanchardie P, Laboisse C, Lustenberger P, et al. Overexpression of the CD155 gene in human colorectal carcinoma. Gut. (2001) 49(2):236–40. doi: 10.1136/gut.49.2.236
25. Oshima T, Sato S, Kato J, Ito Y, Watanabe T, Tsuji I, et al. Nectin-2 is a potential target for antibody therapy of breast and ovarian cancers. Mol Cancer (2013) 12:60. doi: 10.1186/1476-4598-12-60
26. Lupo KB, Matosevic S. CD155 immunoregulation as a target for natural killer cell immunotherapy in glioblastoma. J Hematol Oncol (2020) 13(1):76. doi: 10.1186/s13045-020-00913-2
27. Solomon BL, Garrido-Laguna I. TIGIT: a novel immunotherapy target moving from bench to bedside. Cancer Immunol Immunother (2018) 67(11):1659–67. doi: s00262-018-2246-5/s00262-018-2246-5
28. Zhu Y, Paniccia A, Schulick AC, Chen W, Koenig MR, Byers JT, et al. Identification of CD112R as a novel checkpoint for human T cells. J Exp Med (2016) 213(2):167–76. doi: 10.1084/jem.20150785
29. Bottino C, Castriconi R, Pende D, Rivera P, Nanni M, Carnemolla B, et al. Identification of PVR (CD155) and nectin-2 (CD112) as cell surface ligands for the human DNAM-1 (CD226) activating molecule. J Exp Med (2003) 198(4):557–67. doi: 10.1084/jem.20030788
30. Jin HS, Park Y. Hitting the complexity of the TIGIT-CD96-CD112R-CD226 axis for next-generation cancer immunotherapy. BMB Rep (2021) 54(1):2–11. doi: 10.5483/BMBRep.2021.54.1.229
31. Blake SJ, Dougall WC, Miles JJ, Teng MW, Smyth MJ. Molecular pathways: Targeting CD96 and TIGIT for cancer immunotherapy. Clin Cancer Res (2016) 22(21):5183–8. doi: 10.1158/1078-0432.CCR-16-0933
32. Dougall WC, Kurtulus S, Smyth MJ, Anderson AC. TIGIT and CD96: new checkpoint receptor targets for cancer immunotherapy. Immunol Rev (2017) 276(1):112–20. doi: 10.1111/imr.12518
33. Joller N, Hafler JP, Brynedal B, Kassam N, Spoerl S, Levin SD, et al. Cutting edge: TIGIT has T cell-intrinsic inhibitory functions. J Immunol (2011) 3):1338–42. doi: 10.4049/jimmunol.1003081
34. Josefsson SE, Huse K, Kolstad A, Beiske K, Pende D, Steen CB, et al. T Cells expressing checkpoint receptor TIGIT are enriched in follicular lymphoma tumors and characterized by reversible suppression of T-cell receptor signaling. Clin Cancer Res (2018) 24(4):870–81. doi: 10.1158/1078-0432.CCR-17-2337
35. Johnston RJ, Comps-Agrar L, Hackney J, Yu X, Huseni M, Yang Y, et al. The immunoreceptor TIGIT regulates antitumor and antiviral CD8(+) T cell effector function. Cancer Cell (2014) 26(6):923–37. doi: 10.1016/j.ccell.2014.10.018
36. Lozano E, Dominguez-Villar M, Kuchroo V, Hafler DA. The TIGIT/CD226 axis regulates human T cell function. J Immunol (2012) 188(8):3869–75. doi: 10.4049/jimmunol.1103627
37. Fuhrman CA, Yeh WI, Seay HR, Saikumar Lakshmi P, Chopra G, Zhang L, et al. Divergent phenotypes of human regulatory T cells expressing the receptors TIGIT and CD226. J Immunol (2015) 195(1):145–55. doi: 10.4049/jimmunol.1402381
38. Fourcade J, Sun Z, Chauvin JM, Ka M, Davar D, Pagliano O, et al. CD226 opposes TIGIT to disrupt tregs in melanoma. JCI Insight (2018) 3(14):e121157. doi: 10.1172/jci.insight.121157
39. Joller N, Lozano E, Burkett PR, Patel B, Xiao S, Zhu C, et al. Treg cells expressing the coinhibitory molecule TIGIT selectively inhibit pro-inflammatory Th1 and Th17 cell responses. Immunity. (2014) 40(4):569–81. doi: 10.1016/j.immuni.2014.02.012
40. Yu X, Harden K, Gonzalez LC, Francesco M, Chiang E, Irving B, et al. The surface protein TIGIT suppresses T cell activation by promoting the generation of mature immunoregulatory dendritic cells. Nat Immunol (2009) 10(1):48–57. doi: 10.1038/ni.1674
41. Chen X, Lu PH, Liu L, Fang ZM, Duan W, Liu ZL, et al. TIGIT negatively regulates inflammation by altering macrophage phenotype. Immunobiology. (2016) 221(1):48–55. doi: 10.1016/j.imbio.2015.08.003
42. Zhang Q, Bi J, Zheng X, Chen Y, Wang H, Wu W, et al. Blockade of the checkpoint receptor TIGIT prevents NK cell exhaustion and elicits potent anti-tumor immunity. Nat Immunol (2018) 19(7):723–32. doi: 10.1038/s41590-018-0132-0
43. Josefsson SE, Beiske K, Blaker YN, Førsund MS, Holte H, Østenstad B, et al. TIGIT and PD-1 mark intratumoral T cells with reduced effector function in b-cell non-Hodgkin lymphoma. Cancer Immunol Res (2019) 7(3):355–62. doi: 10.1158/2326-6066.CIR-18-0351
44. Yang ZZ, Kim HJ, Wu H, Jalali S, Tang X, Krull JE, et al. TIGIT expression is associated with T-cell suppression and exhaustion and predicts clinical outcome and anti– PD-1 response in follicular lymphoma. Clin Cancer Res (2020) 26(19):5217–31. doi: 10.1158/1078-0432.CCR-20-0558
45. Catakovic K, Gassner FJ, Ratswohl C, Zaborsky N, Rebhandl S, Schubert M, et al. TIGIT expressing CD4+T cells represent a tumor-supportive T cell subset in chronic lymphocytic leukemia. Oncoimmunology. (2017) 7(1):e1371399. doi: 10.1080/2162402X.2017.1371399
46. Annibali O, Bianchi A, Grifoni A, Tomarchio V, Tafuri M, Verri M, et al. A novel scoring system for TIGIT expression in classic Hodgkin lymphoma. Sci Rep (2021) 11(1):7059. doi: 10.1038/s41598-021-86655-8
47. Anzengruber F, Ignatova D, Schlaepfer T, Chang YT, French LE, Pascolo S, et al. Divergent LAG-3 versus BTLA, TIGIT, and FCRL3 expression in sézary syndrome. Leuk Lymphoma (2019) 60(8):1899–907. doi: 10.1080/10428194.2018.1564827
48. Kong Y, Zhu L, Schell TD, Zhang J, Claxton DF, Ehmann WC, et al. T-Cell immunoglobulin and ITIM domain (TIGIT) associates with CD8+ T-cell exhaustion and poor clinical outcome in AML patients. Clin Cancer Res (2016) 22(12):3057–66. doi: 10.1158/1078-0432.CCR-15-2626
49. Hattori N, Kawaguchi Y, Sasaki Y, Shimada S, Murai S, Abe M, et al. Monitoring TIGIT/DNAM-1 and PVR/PVRL2 immune checkpoint expression levels in allogeneic stem cell transplantation for acute myeloid leukemia. Biol Blood Marrow Transplant (2019) 25(5):861–7. doi: 10.1016/j.bbmt.2019.01.013
50. Jin Z, Ye W, Lan T, Zhao Y, Liu X, Chen J, et al. Characteristic of TIGIT and DNAM-1 expression on Foxp3+ γδ T cells in AML patients. BioMed Res Int (2020) 2020:4612952. doi: 10.1155/2020/4612952
51. Jin Z, Lan T, Zhao Y, Du J, Chen J, Lai J, et al. Higher TIGIT+CD226- γδ T cells in patients with acute myeloid leukemia. Immunol Invest (2022) 51(1):40–50. doi: 10.1080/08820139.2020.1806868
52. Liu G, Zhang Q, Yang J, Li X, Xian L, Li W, et al. Increased TIGIT expressing NK cells with dysfunctional phenotype in AML patients correlated with poor prognosis. Cancer Immunol Immunother (2022) 71(2):277–87. doi: 10.1007/s00262-021-02978-5
53. Minnie SA, Kuns RD, Gartlan KH, Zhang P, Wilkinson AN, Samson L, et al. Myeloma escape after stem cell transplantation is a consequence of T-cell exhaustion and is prevented by TIGIT blockade. Blood. (2018) 132(16):1675–88. doi: 10.1182/blood-2018-01-825240
54. Guillerey C, Harjunpää H, Carrié N, Kassem S, Teo T, Miles K, et al. TIGIT immune checkpoint blockade restores CD8+ T cell immunity against multiple myeloma. Blood. (2018) 132(16):1689–94. doi: 10.1182/blood-2018-01-825265
55. Brauneck F, Seubert E, Wellbrock J, Schulze Zur Wiesch J, Duan Y, Magnus T. Combined blockade of TIGIT and CD39 or A2AR enhances NK-92 cell-mediated cytotoxicity in AML. Int J Mol Sci (2021) 22(23):12919. doi: 10.3390/ijms222312919
56. Wang M, Bu J, Zhou M, Sido J, Lin Y, Liu G, et al. CD8+T cells expressing both PD-1 and TIGIT but not CD226 are dysfunctional in acute myeloid leukemia (AML) patients. Clin Immunol (2018) 190:64–73. doi: 10.1016/j.clim.2017.08.021
57. Li W, Blessin NC, Simon R, Kluth M, Fischer K, Hube-Magg C, et al. Expression of the immune checkpoint receptor TIGIT in hodgkin’s lymphoma. BMC Cancer (2018) 18(1):1209. doi: 10.1186/s12885-018-5111-1
58. Hajiasghar-Sharbaf R, Asgarian-Omran H, Valadan R, Hossein-Nattaj H, Shekarriz R, Zaboli E, et al. CD8+ T-cells Co-expressing PD-1 and TIGIT are highly frequent in chronic lymphocytic leukemia. Iran J Allergy Asthma Immunol (2021) 20(6):751–63. doi: 10.18502/ijaai.v20i6.8027
59. Zhang X, Zhang H, Chen L, Feng Z, Gao L, Li Q. TIGIT expression is upregulated in T cells and causes T cell dysfunction independent of PD-1 and Tim-3 in adult b lineage acute lymphoblastic leukemia. Cell Immunol (2019) 344:103958. doi: 10.1016/j.cellimm.2019.103958
60. Niu J, Maurice-Dror C, Lee DH, Kim DW, Nagrial A, Voskoboynik M, et al. First-in-human phase 1 study of the anti-TIGIT antibody vibostolimab as monotherapy or with pembrolizumab for advanced solid tumors, including non-small-cell lung cancer. Ann Oncol (2022) 33(2):169–80. doi: 10.1016/j.annonc.2021.11.002
61. Frentzas S, Meniawy T, Kao S, Wang RH, Zheng H, Budha N, et al. ADVANTIG-105: Phase 1 dose-escalation study of anti-TIGIT monoclonal antibody ociperlimab (BGB-A1217) in combination with tislelizumab in patients with advanced solid tumors. J Clin Oncol (2021). doi: 10.1200/JCO.2021.39.15_suppl.2583
62. Rodriguez-Abreu D, Johnson ML, Hussein MA, Cobo M, Patel AJ, Secen NM, et al. Primary analysis of a randomized, double-blind, phase II study of the anti-TIGIT antibody tiragolumab (Tira) plus atezolizumab (Atezo) versus placebo plus atezo as first-line (1L) treatment in patients with PD-L1-Selected NSCLC (CITYSCAPE). J Clin Oncol (2020) 38(15_suppl):9503. doi: 10.1200/JCO.2020.38.15_suppl.9503
63. Fathi M, Bahmanpour S, Barshidi A, Rasouli H, Karoon Kiani F, Mahmoud Salehi Khesht A, et al. Simultaneous blockade of TIGIT and HIF-1α induces synergistic anti-tumor effect and decreases the growth and development of cancer cells. Int Immunopharmacol (2021) 101(Pt A):108288. doi: 10.1016/j.intimp.2021.108288
Keywords: TIGIT, immunotherapy, hematological malignancy, lymphoma, multiple myeloma, leukemia
Citation: Jin S, Zhang Y, Zhou F, Chen X, Sheng J and Zhang J (2022) TIGIT: A promising target to overcome the barrier of immunotherapy in hematological malignancies. Front. Oncol. 12:1091782. doi: 10.3389/fonc.2022.1091782
Received: 07 November 2022; Accepted: 06 December 2022;
Published: 20 December 2022.
Edited by:
Lili Feng, Harbin Engineering University, ChinaReviewed by:
Pooya Farhangnia, Iran University of Medical Sciences, IranCopyright © 2022 Jin, Zhang, Zhou, Chen, Sheng and Zhang. This is an open-access article distributed under the terms of the Creative Commons Attribution License (CC BY). The use, distribution or reproduction in other forums is permitted, provided the original author(s) and the copyright owner(s) are credited and that the original publication in this journal is cited, in accordance with accepted academic practice. No use, distribution or reproduction is permitted which does not comply with these terms.
*Correspondence: Jin Zhang, SmVhbnpoYW5nQHpqdS5lZHUuY24=
Disclaimer: All claims expressed in this article are solely those of the authors and do not necessarily represent those of their affiliated organizations, or those of the publisher, the editors and the reviewers. Any product that may be evaluated in this article or claim that may be made by its manufacturer is not guaranteed or endorsed by the publisher.
Research integrity at Frontiers
Learn more about the work of our research integrity team to safeguard the quality of each article we publish.