- 1School of Basic Medical Science, Weifang Medical University, Weifang, China
- 2Department of Pathology, Weifang People’ s Hospital, Weifang, China
In recent years, the role of potassium channels in tumors has been intensively studied. Potassium channel proteins are widely involved in various physiological and pathological processes of cells. The expression and dysfunction of potassium channels are closely related to tumor progression. Potassium channel blockers or activators present antitumor effects by directly inhibiting tumor growth or enhancing the potency of classical antitumor agents in combination therapy. This article reviews the mechanisms by which potassium channels contribute to tumor development in various tumors in recent years, introduces the potential of potassium channels as diagnostic targets and therapeutic means for tumors, and provides further ideas for the proper individualized treatment of tumors.
Introduction
Potassium channels constitute the most prominent family, presenting 77 sequences encoding α pore-forming subunits among the ion channels (1). Potassium channels are the most widely distributed, subtype and complex class of protein molecules found so far, which are commonly involved in the physiological and pathological processes of cells, including cell proliferation and differentiation, pigmentation, migration, cell cycle progression, apoptosis, autophagy, metabolism, angiogenesis, stem cell dynamics and carcinogenesis. Potassium channels also play an essential role in maintaining the function of cells (2). In recent years, the role of potassium channels in cancer cell proliferation, invasion, migration and metastasis has gradually become well-known, suggesting that this channel may become a potential tumor diagnostic marker and therapeutic target. This paper reviews the mechanism of potassium channels in tumor development and introduces its research progress as an emerging candidate target and biological marker for potential anticancer therapy.
Classification of potassium channels
Potassium channels(K+ channels) are the most numerous and diverse ion channels expressed in excitable and non-excitable cells. According to its structure and function, it is mainly divided into four major categories: voltage-gated potassium channels(Kv), calcium-activated potassium channels(KCa), inwardly rectifying potassium channels(Kir), and Two-pore-domain potassium channels(K2P) (3).
Relationship between potassium channel and tumor
Potassium channels are critical regulators of cellular homeostasis and regulate important physiological processes. Intercellular ion redistribution from channel opening can influence cellular function, such as cell proliferation, migration and apoptosis. Abnormal channel expression and function can affect physiological processes and even carcinogenesis. For example, Kv1.3 expression is found to be altered in tissues such as breast cancer, pancreatic cancer, lung cancer, prostate cancer, B-cell lymphoma, and B-cell chronic lymphocytic leukemia(CLL)compared with normal tissues, and its increased activity can promote the development of cancer (1). Therefore, abnormal expression of K+ channels is a molecular target of oncogenic transformation, and it is also crucial to explore the relationship between potassium channels and tumors (4) (Figure 1).
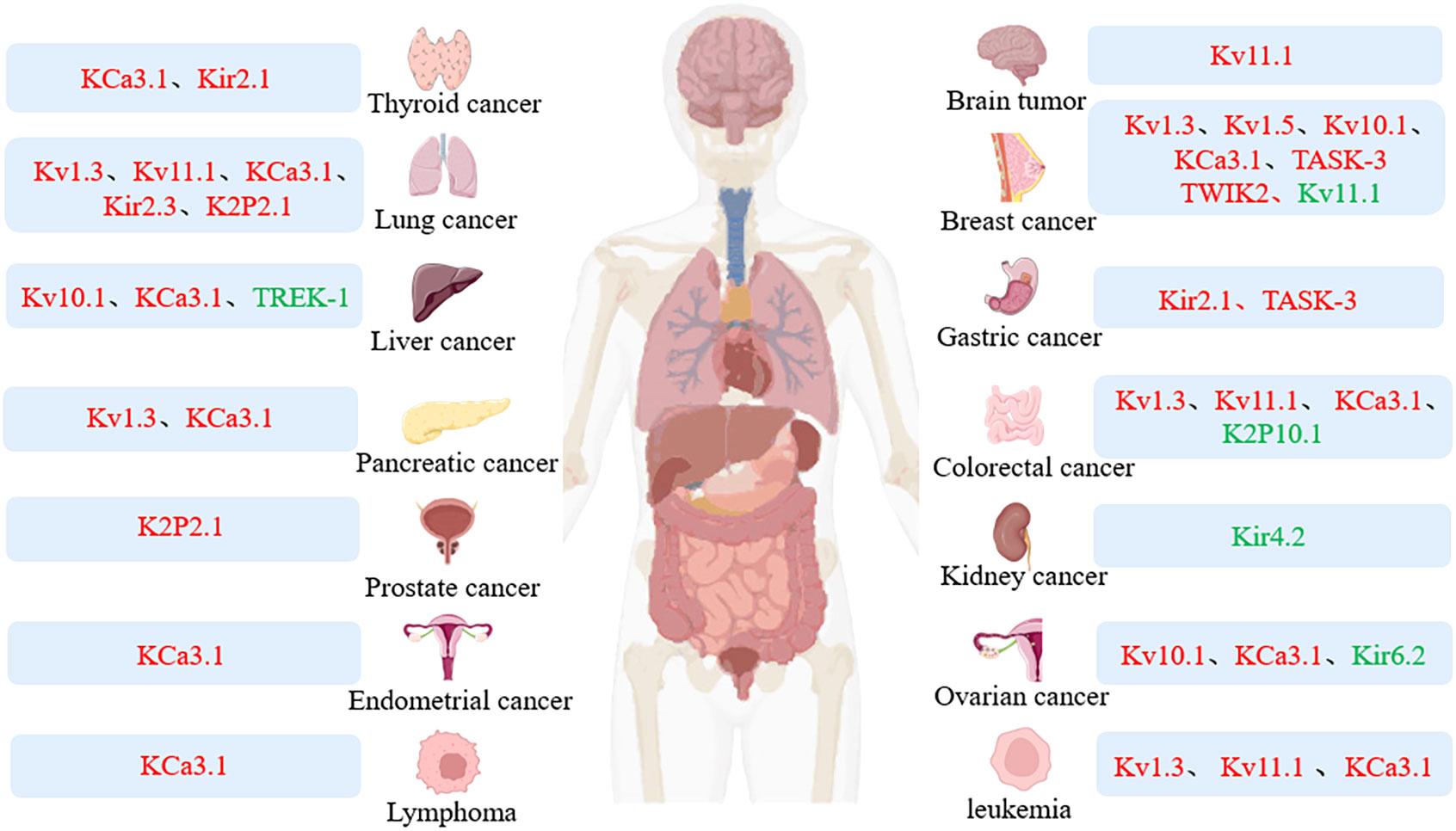
Figure 1 Expression of potassium channel in tumor. Colors represent different expression levels: red, high expression; green, low expression.
voltage-gated potassium channels
Among the different types of K+ channels encoded by 77 genes, Kv represents the largest and most complex family (5). There are currently 12 known isoforms(Kv1 – Kv12). They are essential regulators of cellular excitability and signaling and are expressed in most cells (6). Each Kv subunit consists of six transmembrane domains(S1 – S6). Kv is involved in a variety of physiological processes, such as smooth muscle contraction, cell volume control, cell cycle progression, cardiac repolarization and tumor cell proliferation (7). Kv1.3 (8, 9), Kv7.1 (10), Kv10.1 (11) and Kv11.1 (12) plays a vital role in cell cycle regulation and tumor development. Members of the Kv family play essential roles in both cell cycle regulation and apoptosis (13).
Kv1.3(KCNA3), a critical isoform in the Kv family, is selectively permeable to potassium ions and is activated upon changes in membrane potential (14). Overexpression of Kv1.3 channels has been observed in the breast, colon, smooth muscle, skeletal muscle, lymph node, and CLL. However, both Kv1.3 channel expression and Kv1.3 gene promoter methylation can be used as diagnostic and prognostic markers in breast, pancreatic and colorectal cancer(CRC) (9). In addition, the researchers found that Kv1.3 channel blockade was able to lead to phosphorylation of the transcription factor CREB in LUAD A549 cells and play a specific role in cell proliferation, apoptosis and differentiation, thereby affecting the development of lung cancer(LC) cells (15). Kv1.5 overexpression driving resting membrane potential(RMP) hyperpolarization may alter adhesion and morphology of triple-negative breast cancer(TNBC) cells and increase TNBC cell migration and invasion (16).
Kv7 consists of five different ion channels, Kv7.1-7.5, encoded by the KCNQ gene(KCNQ1-5), respectively. Kv7 ion channels, like other Kv channels, are tetramers and require assembly of four α-subunits to produce functional channels (6). KCNQ1 is a tumor suppressor gene in mice, and low Kv7.1 expression has been found to accelerate tumor progression and affect patient survival through analysis of tissue samples from CRC patients (10). There was a positive correlation between Kv7.1-KCNE3 expression levels and survival in CRC patients (17). As an antisense transcript of the KCNQ1 gene, KCNQ1OT1 is up-regulated in BC tissues and acts through the KCNQ1OT1miR-34aNotch3 axis to promote the progression of BC (18). However, the mechanism by which Kv7 channels play a role in tumors and strategies in clinical treatment need to be further investigated.
Kv10.1, also known as ethera-go-go-1(EAG1), is encoded by the KCNH1 gene and plays a critical role in tumor cell proliferation, angiogenesis, migration, and invasion (4). In vitro experiments have found that retinoblastoma protein(Rb) can regulate the expression of Kv10.1 and inhibit cancer cell proliferation and tumor development by decreasing the expression level of Kv10.1 (19). Kv10.1 is highly expressed in BC MCF-7 cells. The inhibition of Kv10.1 expression can up-regulate miR-126, thereby reducing the invasiveness of BC cells (20).
Kv11.1, also called ether-à-go-go(HERG), is encoded by the KCNH gene (12). In normal healthy tissues, its expression level is usually low, and it is high in tissues such as leukemia, ovarian cancer(OC), LC, and BC (21). Activation of Kv11.1 promotes Cav-1 dephosphorylation thereby reducing BC MDA-MB-231 cell migration and invasion (22). In glioblastoma(GBM) xenografts, high expression of HERG may be associated with the proliferation (23). In CRC cell lines, HERG1 presents high expression, and knockdown of HERG1 reduces the proliferation and tumorigenic ability of CRC cells (24). HERG1 is similarly overexpressed in hepatocellular carcinoma(HCC) but restricted to the early stages of tumor development (25).
calcium-activated potassium channels
Calcium-activated potassium channels are divided into large conductance calcium-activated potassium channels(KCa1.1), medium conductance calcium-activated potassium channels(KCa3.1), and small conductance calcium-activated potassium channels(KCa2.1-2.3). The large conductance potassium channel(BK) is a tetramer composed of six transmembrane domains(S1 – S6), and the central pore of each transmembrane domain is located in the S5 – S6 region. Small versus medium conductance(SK) is a tetramer composed of a and b subunits, in which the pore is formed by one subunit (26). KCa channels are expressed in almost all human cells and are essential determinants of hyperpolarization following action potentials and are widely involved in cellular activities such as cell cycle, proliferation, migration, and apoptosis.
KCa3.1 channels, also known as SK4 or IK, are encoded by the KCNN4 gene and are activated by elevated intracellular Ca2+ concentrations (27). KCa3.1 is a common oncogene. It has been found that KCa3.1 is involved in the development of OC, and blocking or down-regulating KCa3.1 expression in OC cells can reduce the migration of OC (28). KCa3.1 is highly expressed in TNBC and can promote migration and epithelial-to-mesenchymal transition(EMT) of TNBC cells (29). KCa3.1 is involved in cell proliferation by regulating G1/S transition and is a critical regulator of cell cycle progression and proliferation in BC (30). In endometrial cancer(ECa), the expression level of KCa3.1 is significantly upregulated in EC tissues compared with normal tissues, and lncRNA-14327.1 regulates migration and invasion of EC cells by stabilizing KCa3.1 protein and activating EMT (31). In HCC, KCa3.1 promotes invasion and metastasis in vitro by inducing MAPK/ERK and EMT pathways. Down-regulation of KCa3.1 can inhibit the proliferation ability of HCC cells (32). Another study similarly found that KCa3.1 promotes cell cycle progression and promotes cell migration and invasion in HCC by activating protein kinase 2(SKP2) and inducing EMT (33). KCa1.1 channel blockers encoded by KCNMA1 did not significantly alter the viability of HCC Huh7 cells under normoxic conditions but reduced cell proliferation capacity under hypoxic conditions. In vivo experiments revealed that blocking KCa1.1 channels inhibited hepatoma cell migration and invasion (34).
Inwardly rectifying potassium channels
The inward rectifier potassium channel family is a class of non-voltage-gated K+ channels, and current data suggest that there are seven subfamilies of Kir channels(Kir1.1 to Kir7.1), which can be usually divided into four categories according to function: classical Kir channels(Kir2.X), G-protein-activated Kir channels(Kir3.X), ATP-sensitive potassium channels(Kir6.X), and K + transport channels(Kir1.X, Kir4.X, Kir5.X, and Kir7.X) (35, 36). Kir is widely distributed in various tissues of the human body and has a variety of physiological functions, playing a pivotal role in maintaining resting potential, controlling cell excitability, and regulating cell volume, and its activity is controlled by a variety of mediators, such as binding proteins, phospholipids, and ions (37).
Kir2.1(KCNJ2) is associated with the development of papillary thyroid carcinoma(PTC), and interference with KCNJ2 inhibits cell proliferation, invasion, migration, and EMT processes (38). The researchers found that the lncRNA POU6F2-AS1 may act as a competitive endogenous RNA(ceRNA) for miR-34c-5p and promote the expression of Kir2.3(KCNJ4) and enhance the invasiveness of LC cells (39). Kir6.2(KCNJ11) and Sulfonylurea Receptor(ABCC9) gene expression is downregulated in OC compared to healthy tissues, and expression of Kir6.2 correlates with OC prognosis (40). In addition, Kir4.1(KCNJ10) expression is low in astrocytomas and oligodendrogliomas. MiR-5096, as a potential target of the KCNJ10 gene, can decrease Kir4.1 channel protein expression in GBM cells, and miR-5096 and Kir4.1 knockdown can increase glioma cell invasiveness (41). Kir4.2(KCNJ15) is lowly expressed in renal cell carcinoma(RCC). KCNJ15 overexpression inhibits RCC cell proliferation, migration, inhibits cell cycle and induces apoptosis by affecting EMT and matrix metalloproteinase-7 and p21 expression (42).
Two-pore-domain potassium channels
Two-pore domain potassium channels are a class of ion channels encoded by the KCNK gene family and have a unique membrane topology of four transmembrane helices and two pore domains. Based on their unique primary structure, physiological properties, and biological, the K2P channel family has 15 members divided into six subgroups(THIK, TASK, TRESK, TWIK, TALK, and TREK) functions. K2P channels are widely distributed and are involved in regulating cellular function and maintaining resting membrane potential (43, 44). Abnormal expression and dysfunction of K2P channels are closely related to the development of cancer (45).
TREK-1(KCNK2), a member of the K2P family, is by far the most studied K2P channel, and KCNK2 expression is decreased in HCC (46). Researchers have found that TASK-1(KCNK3) inhibits LUAD cell proliferation and glucose metabolism by regulating AMPK-TXNIP signaling pathway (47). In non-small cell lung cancer(NSCLC) cell line A549, TASK-1 presented high expression and was able to promote cell proliferation as well as inhibit apoptosis (48). Down-regulated lncRNA KCNK15-AS1 in pancreatic cancer(PC) tissues inhibits PC BxPC-3 cell invasion (49). TWIK-2(KCNK6) expression is significantly increased in BC, and its overexpression enhances the proliferation, invasion and migration of BC cells and may be involved in the malignant transformation of BC (50). TASK-3(KCNK9) promotes the proliferation and survival of cancer cells by enhancing their resistance to hypoxia and serum deprivation. Knockdown of TASK-3 in BC MDA-MB-231 cells reduced proliferation while inducing cellular senescence and cell cycle arrest (51). In gastric cancer(GC) cell lines, TASK-3 is a crucial protein involved in migration and cell survival, and low TASK-3 expression decreases cell proliferation and migration (52). In addition, TASK-3 was significantly upregulated in oral squamous cell carcinoma tissues in a rat model, and both TASK-1 and TRESK were downregulated in advanced poorly differentiated oral squamous cell carcinoma (53). KCNK15 is highly expressed in PC cells, and researchers have found that KCNK15-AS1 hinders the migration and proliferation of PC cells by regulating KCNK15 and the tumor suppressor gene PTEN (54).
Role of potassium channel in diagnosis and treatment of tumor
Potassium channels play a crucial role in cancer biology as one of the most widely distributed ion channels, the expression and dysfunction of potassium channels are closely related to tumor progression (Table 1). Potassium channels are aberrantly expressed in many tumor cells and play an essential role in cell proliferation and differentiation. Many studies have shown that pharmacological inhibition of specific isoforms is an effective method to inhibit proliferation, migration and invasion, as well as to increase apoptosis (65). Potassium channels can not only be used as markers for tumor diagnosis and prognosis but also provide new ideas for targeted cancer therapy.
Potassium channels: diagnostic markers and drug targets for tumors
Kv1.3 is located in the plasma membrane and intracellularly and plays a vital role in cell proliferation and apoptosis (66). The specific Kv1.3 channel inhibitor clofazimine, as a membrane-permeable small molecule organic compound, can inhibit cancer cell proliferation and induce cancer cell apoptosis through the mitochondrial pathway (9). The researchers found that the Kv1.3 inhibitor tetrahydropyran inhibited PC cell proliferation and induced apoptosis (58). In vitro experiments revealed that the mitochondrial Kv1.3 inhibitors PAPTP and PCARBTP were able to promote apoptosis in multiple myeloma cell lines L-363 and RPMI-8226 (67). Mitochondrial targeting inhibitors have been found to alter mitochondrial function by inhibiting Kv1.3 leading to reactive oxygen species mediated apoptosis of cancer cells in vivo in vitro and in vivo experiments in melanoma and pancreatic ductal adenocarcinoma (PDAC) (68). The Kv1.3 channel inhibitor memantine promoted acute lymphoblastic leukemia(ALL) cell death through AKT, ERK1/2, and MYC signaling pathways. Combined with AraC caused ALL cell proliferation arrest and cell death by increasing CytC release as well as promoting caspase-9 and caspase-3 activation (55). Kv1.3 channels are the only voltage-dependent potassium channels in the plasma membrane of human lymphocytes (69).Compared with healthy lymphocytes, Kv1.3 is highly expressed in the plasma membrane and mitochondria of human CLL cells. The mitogenic agent PAPTP, which specifically targets this channel, effectively kills pathological B cells in the spleen and peritoneal cavity. While inducing apoptosis in CLL B cells expressing high levels of Kv1.3, PAPTP does not alter the survival of healthy B and T cells (56). PAP-1, Psora-4, and clofazimine are inhibitors of Kv1.3 channels that induce apoptosis in human Jurkat leukemia T cells by inhibiting intracellular Kv1.3. They can also induce Bax/Bak-deficient human Jurkat leukemia T cell death. Studies have shown that Kv1.3 inhibitors are potent inducers of apoptosis in mitoKv1.3-expressing tumor cells and their mode of action is independent of Bax and Bak, which provides a new strategy for finding resistance mechanisms in tumor cells as well as identifying new targets for chemotherapy (57).
Kv10.1 is a standard tumor marker that is overexpressed in approximately 70% of human tumors and cancer cell lines, and channel inhibition reduces tumor growth (70). Chloroquine, as a Kv10.1 channel inhibitor, can inhibit outward potassium currents in BC cells, thereby reducing cell migration (71). Proanthocyanidin B1, a natural compound extracted from grape seeds, is a specific inhibitor of Kv10.1 channels, and inhibition of Kv10.1 currents can inhibit migration, proliferation, and xenograft tumor development in the hepatoma cell line Hh-7 cells as well as HepG2 cells (72). The researchers found that fusion of a single-domain antibody(nanobody) to Kv10.1 with tumor necrosis factor-related apoptosis-inducing ligand TRAIL showed strong apoptosis-inducing effects in different tumor models (73). The expression level of hEag1 is closely associated with shorter expected survival in acute myeloid leukemia(AML)patients. In AML, the authors found an interesting isoform-dependent phenomenon of hEag1 expression, with half of the cases of the most common subtypes M2 and M4 expressing hEag1, which is associated with increased age, higher relapse rates, and significantly shorter overall survival. However, hEag1 expression was not detected in CLL. The hEag1 blocker astemizole can increase the apoptotic response, inhibit cell migration in AML cells, and may serve as a potential target for treating AML (59).
Kv11.1 activator NS1643 exerts antitumor effects on TNBC in vivo and in vitro by causing DNA damage via a Ca2+ dependent mechanism (74). High Kv11.1 expression is associated with a favorable prognosis in estrogen receptor-negative breast cancer, and NS1643, a Kv11.1 activator, reduces the metastatic spread of breast tumors in vivo by inhibiting cell motility via β-catenin (75). Researchers have found that Kv11.1 is highly expressed in precancerous lesions of the gastrointestinal tract, and Kv11.1 can be used as an early diagnostic marker for gastrointestinal tumors (76). In addition, Kv11.1 is widely expressed in metastatic CRC, and combined treatment with Kv11.1 blockers and bevacizumab inhibits local tumor growth and metastatic spread (77). Another in vitro and in vivo experiment on the CRC model, clarithromycin, an antibiotic targeting Kv11.1, induced apoptosis and increased the cytotoxic effect of 5-fluorouracil (78). In addition, the researchers found that KCNQ1 can be used as a robust prognostic indicator of disease recurrence in patients with stage II and III colon cancer (79). Medulloblastoma(MB) is children’s most common malignant brain tumor, and EAG2 can promote brain tumor growth. Antipsychotic Thioridazine, an EAG2 channel blocker, reduces xenograft MB growth and metastasis. Analysis of case reports in patients receiving thioridazine showed a reduction in the extent of disease following thioridazine treatment. However, HERG channel blockers are at risk for cardiac arrhythmias, tardive dyskinesia, and ataxia, and it is essential to monitor closely for possible side effects (60). Dichloroacetate(DCA) is a metabolic modulator widely used in the treatment of inherited mitochondrial diseases. It has been found that DCA can activate Kv channels in all tumor cells, inhibit tumor growth, induce apoptosis by inhibiting mitochondrial pyruvate dehydrogenase kinase(PDK) and converting cell metabolism from glycolysis to aerobic oxidation of glucose, and this effect does not occur on normal cells (80). A large number of peptide toxins contained in scorpion venom act by regulating Kv activity and affect the activity of cancer cells, thus exerting anti-tumor effects (81). Thus, these studies suggest that effective strategies to use drugs that target ion channels for cancer treatment are beneficial.
KCa1.1 channel is a potential early marker of human cervical cancer(CC), and human cervical biopsies show differential KCNMA1 protein expression, and detection of this channel in CC screening programs may be helpful for early detection of CC (61). KCa2.2(KCNN2) is strongly associated with melanoma. Miconazole, a known cytochrome P-450 inhibitor, showed highly high antiproliferative activity in KCNN2-mediated melanoma cell lines (82). The KCNN3 gene encodes KCa2.3. Compared with normal, the expression levels of KCNN3 mRNA and protein were significantly lower in OC tissues, and low KCNN3 expression was associated with poor prognosis in OC (83). Alternatively, SigmaR1 acts as a stress-activated partner and requires increased calcium influx by triggering KCNN3. This drives BC and CRC cell migration and promotes tumor development. High SigmaR1 expression is associated with reduced overall survival in BC (84).
KCNN4 is highly expressed in PDAC. The combination of KCNN4 with TNM stage, lymph node metastasis and histological differentiation is an independent prognostic factor for overall survival in patients with PDAC (62). Increased KCa3.1 expression correlates with invasion of NSCLC cells. KCNN4 DNA hypomethylation and KCa3.1 overexpression have been found to correlate with poor prognosis in NSCLC and are also strong independent predictors of survival in patients with NSCLC (85). KCNN4 promotes the progression of PTC by inducing EMT and inhibiting apoptosis, and its expression correlates with disease-free survival, immune infiltration, and several other clinicopathological features. It can be used as a diagnostic and prognostic biomarker for PTC (86). In glioma cells, temozolomide(TMZ) exerts antitumor effects by inhibiting KCNN4 channel activity (87). The prototypical leukemic T-cell line Jurkat showed lower Kv1.3 and KCa current densities compared to healthy T-cells and other T-cell lines. In activated T cells upregulated by KCa3.1, Ca2+ influx is reduced after the application of KCa3.1-specific blockers, and selective KCa3.1 blockers may become a valuable view for anti-leukemia therapy (63). Another study found that ionizing radiation used clinically for fractionated radiotherapy doses was able to activate K+ channels, resulting in increased membrane conductance, inhibition of cell proliferation and migration, and induction of apoptosis in LUAD cells (88). Small molecule and channel-targeting antibodies can be used for imaging KCa3.1 channels in vitro and in vivo. Because KCa3.1 channel expression has predictive potential for prognosis and patient survival in different tumor entities, imaging probes targeting KCa3.1 channel expression can serve as necessary diagnostic tools (89).
ATP-sensitive potassium channel(KATP) is an inwardly rectifying potassium channel. Minoxidil acts as an activator of KATP channels and decreases TNBC cell invasion in combination with ranolazine (90). Kir2.1 is an important regulator of invasion of human GC. The oncogenic effect of this channel depends on its interaction with serine/threonine protein kinase 38(Stk38). Kir2.1 is highly expressed in GC and is positively correlated with depth of tumor invasion, metastatic status, and poor overall patient survival, and is a potential prognostic marker and therapeutic target (64). Kir2.3 overexpression is strongly associated with poor prognosis in patients with LUAD (39). In OC, minoxidil prevents tumor growth in OC xenograft models by stimulating Kir6.2/SUR2 channels to produce mitochondrial destruction and extensive DNA damage, altering the metabolic and oxidative status of cancer cells (40).
BL1249, a K2P2.1(TREK1) activator, was able to inhibit the proliferation and migration of the human PDAC cell line BxPC-3 cells (91). Several other subfamily members of K2P function to sense or switch mechanical stimuli and can serve as tumor diagnostic markers. K2P2.1 expression is elevated in LC but decreased in breast, gastrointestinal, and head and neck cancers. K2P2.1 is expressed in prostate cancer(PCa) but not found in normal prostate epithelial cells. K2P10.1 is down-regulated in CRC and renal clear cell carcinoma (92). In addition, in HCC, the expression of KCNK2, KCNK15, and KCNK17 is decreased, and KCNK9 expression is increased, which are all associated with a good prognosis in patients with liver cancer (46). In TNBC, high KCNK5, KCNK9, and KCNK2 expression showed poor prognosis (45). Alternatively, in thyroid cancer(TC) tissues, KCNK2, KCNK4, KCNK5, and KCNK15 play regulatory roles in the carcinogenesis and metastasis of TC and may serve as a potential therapeutic target in the future (93). Therefore, K+ channels can serve as a potential tumor-specific drug target, and targeting K+ channels alone or in combination with chemotherapy may become a promising new strategy for anticancer therapy (94).
Role of potassium channel in chemoresistance of tumor
In cancer therapy, multidrug resistance has been a major clinical barrier to cancer therapy, and 90% of chemotherapy failure cases are associated with tumor resistance (95). Kv10.1 is involved in the chemoresistance of OC cells. Decreased Kv10.1 expression is associated with good prognosis by tissue samples from patients treated with cisplatin chemotherapy and may serve as a potential indicator for predicting chemosensitivity (96). Cells endogenously overexpressing Kv10.1 were also more sensitive to inhibitors of mitochondrial metabolism than cells with low expression. Inhibition of Kv10.1 expression or function results in mitochondrial fragmentation, increased reactive oxygen species, and increased autophagy, providing novel strategies to overcome drug resistance in cancers with high Kv10.1 expression (97). In an antibody-based treatment strategy targeting Kv10.1, Kv10.1-specific single-chain Fv antibodies fused to soluble tumor necrosis factor-related apoptosis-inducing ligand(scFv62-TRAIL), combined with the chemotherapeutic agent doxorubicin can overcome cancer cell resistance to chemotherapy and selectively induce apoptosis (98). lncRNA potassium voltage-gated channel subfamily Q member 1 overlapping transcript 1(KCNQ1OT1) is highly expressed in adriamycin-resistant AML cells, and induction of Tspan3 expression by adsorption of miR-193a-3p promotes the progression of adriamycin-resistant and drug-resistant AML cells (99).
KCa is a novel target for tumor prognosis as well as overcoming chemoresistance. Gemcitabine is the first-line treatment for metastatic BC. Increased expression of KCNN4 leads to resistance of BC cells to gemcitabine, which leads to cell proliferation and resistance to apoptosis and antimetabolites. This phenomenon is reversed in vitro and in vivo when KCNN4 is blocked using the specific inhibitor TRAM-34 or when KCNN4 is knocked down directly (100). Another study found that KCa3.1 channel activation has a significant impact on oncogenic Ca2+ signaling, DNA damage response, and radioresistance in the MMTV-PyMT BC model and can be used as a new target for radiation therapy and maintenance therapy (101). KCNN4 also plays a role in chemoresistance in CRC, and the KCa3.1 activator SKA-31 and Kv11.1 inhibitor E4031 have synergistic effects with cisplatin in causing apoptosis and inhibiting proliferation, helping to improve the efficacy of cisplatin and overcome cisplatin resistance in CRC (102). KCa3.1 channel blockers can increase the sensitivity of NSCLC cells to erlotinib and overcome resistance (103). Increased expression of KCa3.1 channels by radiation can suppress the pro-invasive phenotype induced by counteracting radiation in tumor cells and inhibit tumor resistance after radiation therapy in GBM patients (104).
Kir2.1 promotes cell growth by reducing drug-induced apoptosis and cell cycle arrest, resulting in multidrug resistance. Kir2.1 is involved in cell growth and chemoresistance by regulating MRP1/ABCC1 expression in small cell LC cell lines H69 and H446 cells and can be used as a prognostic factor for small cell LC as well as a new target for chemoresistance (105). These studies suggest that potassium channels are critical modulators of tumor resistance, and targeting potassium channels may be a promising therapeutic strategy to overcome tumor multidrug resistance.
Role and mechanism of potassium channel in immunotherapy
Increasing evidence suggests that the immune microenvironment plays an essential role in tumor development and immunotherapy. In recent years, the study of potassium channels and cancer immunotherapy has gradually received attention (106). A study revealed a link between Kv1.3 channels and tumor infiltrating lymphocytes(TILs). A clinical study of head and neck cancer(HNC) found a 70% reduction in Kv1.3 channels in TILs, implying that loss of function of Kv1.3 channels in TILs may contribute to reduced immune surveillance in HNC (107). Kv1.3 is also a potential promoter of apoptosis. Intratumoral cell death increases extracellular K+ concentration, and high intracellular K+ inhibits T cell effector function (108). The expression level of the Kv11.1 channel is closely related to IL-8, IL-27 or VEGF, and the development of(CRC) also accompanies the up-regulation of the Kv11.1a expression level (109). Kv channel blocker 4-aminopyridine(4-AP) can inhibit the secretion of IL-6 and IL-1, thereby inhibiting glioma cell proliferation (110). Elevated extracellular potassium concentrations have been shown to inhibit T cell receptor(TCR) -driven Akt – mTOR phosphorylation and effector programs in vitro. Overexpression of the potassium channel Kv1.3 increases potassium efflux from tumor-specific T cells, thereby decreasing intracellular potassium ions, enhancing tumor clearance and survival in mice carrying melanoma, reducing tumor growth, and making T cells more effective in anticancer effects (111). The researchers found that KCa3.1 activators can enhance the killing effect on tumor cells by eliminating the inhibitory effect of adenosine on CD8+ T cell chemotaxis and promoting CD8+ T cell penetration into tumors (112). In CD8+ T cells, K+ efflux mediating KCNA3 activation increases antitumor function by promoting the expression of IFN-γ (111). KCa3.1 activators were able to significantly decrease the expression and secretion of the tumorigenic factors IL-8 and IL-10 in human monocytic leukemia derived M2 macrophages, and KCa3.1 activators inhibited IL-10 in tumor-associated macrophages(TAM) by ERK-CREB and JNK-c-Jun, thereby inhibiting IL-10-induced escape from tumor immune surveillance (113). Another study found that KCa3.1 activators have an inhibitory effect on IL-10 expression, and the Smad2/3 signaling pathway is involved in transcriptional inhibition of IL-10 in KCa3.1 activator-induced T-lymphoblastic leukemia HuT-78 cells, suggesting that KCa3.1 activators are a new therapeutic option that can inhibit IL-10 and evade the tumor-promoting activity of cancer immune surveillance (114). In addition, KCNN4 is significantly upregulated in many types of cancer tissues and plays an immunoregulatory role in the tumor microenvironment(TME), which is a powerful indicator of pan-cancer prognosis as well as immunotherapy (115). Extensive studies of KCa3.1 in immunotherapy have opened new avenues for treating tumors. Y4, as an antibody with the highest affinity binding, can induce channel internalization and inhibit the function of KCNK9, activate the anti-tumor immune response, increase cell death, and effectively inhibit the growth of human LC xenografts and mouse BC metastasis (116). Therefore, potassium channels play an important role in immunotherapy and may be an effective way to improve cancer immune surveillance and immunotherapy response (Figure 2).
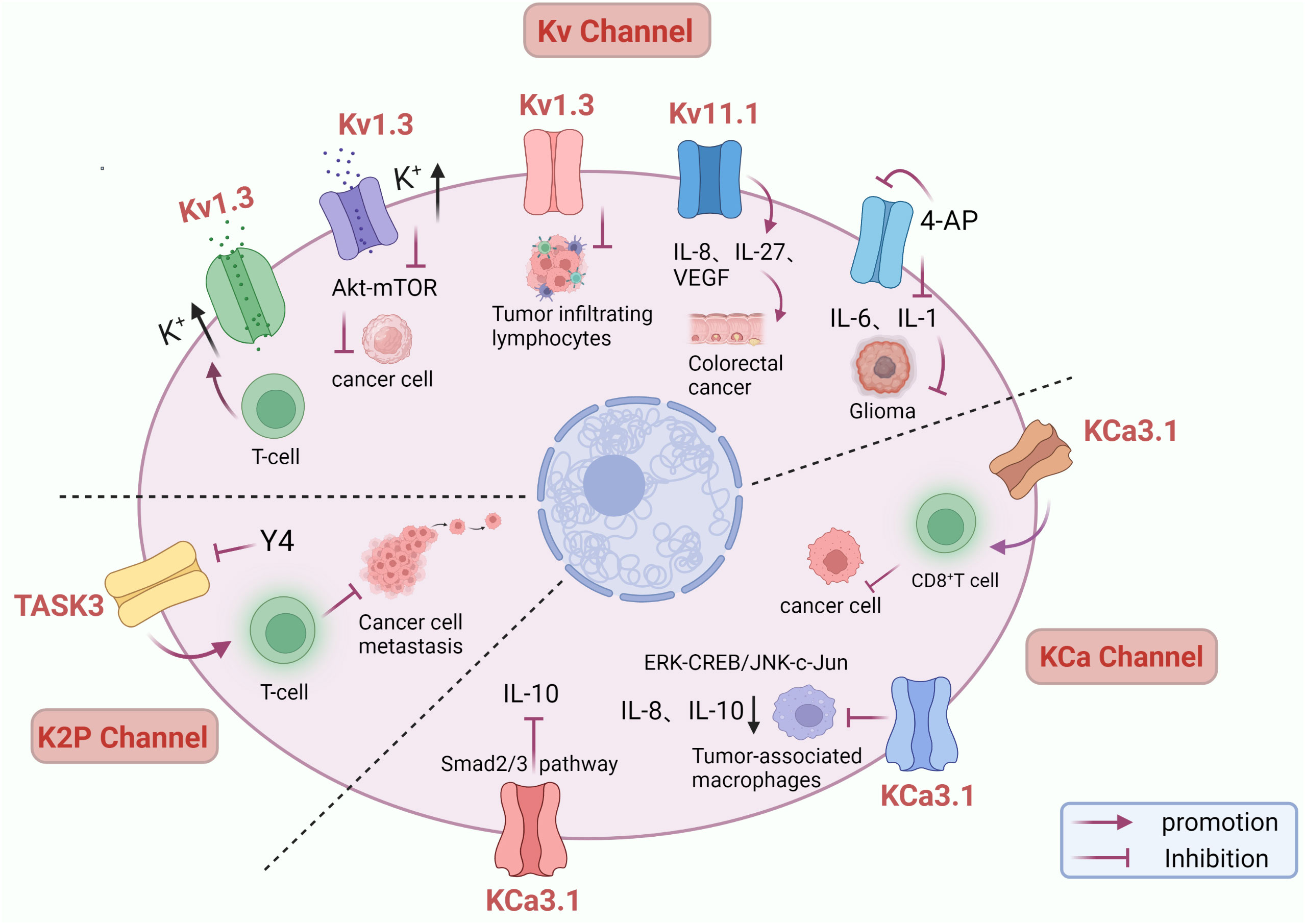
Figure 2 Role of potassium channels in immunotherapy. Inhibition of Kv1.3 channels leads to impaired tumor-infiltrating lymphocyte function and decreased ability to kill cancer cells. Kv1.3 overexpression decreases intracellular potassium and enhances antitumor function of T cells through activation of Akt – mTOR signaling. 4-AP blocked potassium channels and inhibited IL-6 and IL-1 secretion, inhibiting glioma cell proliferation. Up-regulation of Kv11.1 channel expression was accompanied by increased IL-8, IL-27, and VEGF, promoting colorectal cancer development. KCa3.1 activator suppress IL-10-induced tumor immune surveillance escape and IL-8-induced tumorigenicity and metastasis by inhibiting their production from TAMs through ERK-CREB and JNK-c-Jun cascades. KCa3.1 activator enhanced the killing effect of CD8 + T on tumor cells and induced IL-10 transcriptional inhibition in T lymphocytic leukemia through the Smad2/3 signaling pathway. Y4 mAb increases cell death by targeting inhibition of KCNK9 channels and activating anti-tumor immune responses.
Conclusions and outlook
Cancer is a significant public health problem worldwide and is currently the leading cause of human death in the world, and its morbidity and mortality are also increasing year by year (117). The main treatment methods include surgery, chemotherapy, radiotherapy, and biological therapy. Continued development of new therapeutic modalities and drug targets is essential in cancer studies. Among the genes affected during oncogene transformation, genes encoding ion channels inevitably exist and play an essential role in cell proliferation, apoptosis, and neovascularization (4). As novel biomarkers of many cancers, potassium channels play an essential role in diagnosing, prognosis, and treating tumors. Among them, Kv10.1 has been extensively studied, and its overexpression is associated with poor prognosis in various cancers and can be used as a potential indicator and treatment strategy to predict chemosensitivity (96). High expression of genes encoding Kv11.1 channels is associated with favorable prognosis in estrogen receptor-negative breast cancer (75). Extensive expression of Kv11.1 channels in gastrointestinal tumors may serve as an early diagnostic marker (76). Expression of KCNN4 is associated with disease-free survival, immune infiltration, and several other clinicopathological features and can serve as a diagnostic and prognostic biomarker in TC (86). Abnormal expression of the KCNK gene family in BC can be used as an ideal prognostic biomarker for BC patients (118). It is also a powerful indicator of pan-cancer prognosis (115). In addition, drugs related to potassium channels have gradually become the focus of current research. The specific Kv1.3 channel inhibitor clofazimine can inhibit cancer cell proliferation and induce cancer cell apoptosis through the mitochondrial pathway (9). Potassium channel blockers can inhibit cell migration of human endometrial carcinoma and inhibit proliferation and induce apoptosis of rat glioma cells (3, 119). Kv11.1 activator NS1643 exerts antitumor effects in TNBC by causing DNA damage via a Ca2+ -dependent mechanism (74). KCa3.1 activators SKA-31 and Kv11.1 inhibitors can overcome cisplatin resistance in CRC and contribute to improving cisplatin efficacy (102). Therefore, potassium channels play a crucial role in the clinical treatment of cancer. However, the interaction mechanism of the combination has not been widely studied and can be used as the focus of further in-depth study.
In addition, potassium ions also play an important role in immunotherapy, and potassium ions are the key to controlling the anticancer ability of T cells (120). Studies have shown a positive association between serum potassium and cancer risk. For every 1 SD increase in potassium, the risk of overall cancer increased by 16%. Higher levels of serum potassium may contribute to cancer development and growth through immune mechanisms (121). Kv channel blocker 4-AP can inhibit the secretion of IL-6 and IL-1, thereby inhibiting glioma cell proliferation (110). KCa3.1 activators can enhance the killing effect of CD8 + T on tumor cells by eliminating the inhibitory effect of adenosine on CD8+ T cell chemotaxis (112). KCa3.1 activators have an inhibitory effect on IL-10 expression and can address the tumor-promoting activity of cancer immune surveillance by inhibiting IL-10 (114). Therefore, potassium channels may be an effective way to improve cancer immune surveillance and immunotherapy response.
Potassium channels have been intensively studied in the context of cancer. It is not only a biological marker of cancer but also an effective prognostic tool and provides a direction for targeted therapy and immunotherapy of cancer. Although ion channels hold great promise in targeted cancer therapy, they still face significant challenges, of which personalized therapy, drug treatment sensitivity, and off-target toxicity of targeted potassium channel drugs are still poorly studied (2). Therefore, it is necessary to improve the mechanism of potassium channels as drug targets and play the role of potassium channels in precision medicine.
Author contributions
ML was responsible for collating and writing. PT, QZ and XM were responsible for collecting the data, and YZ was responsible for reviewing and proofreading. All authors contributed to the article and approved the submitted version.
Conflict of interest
The authors declare that the research was conducted in the absence of any commercial or financial relationships that could be construed as a potential conflict of interest.
Publisher’s note
All claims expressed in this article are solely those of the authors and do not necessarily represent those of their affiliated organizations, or those of the publisher, the editors and the reviewers. Any product that may be evaluated in this article, or claim that may be made by its manufacturer, is not guaranteed or endorsed by the publisher.
References
1. Pardo LA, Stühmer W. The roles of k(+) channels in cancer. Nat Rev Cancer (2014) 14(1):39–48. doi: 10.1038/nrc3635
2. Anderson KJ, Cormier RT, Scott PM. Role of ion channels in gastrointestinal cancer. World J Gastroenterol (2019) 25(38):5732–72. doi: 10.3748/wjg.v25.i38.5732
3. Liu J, Qu C, Han C, Chen MM, An LJ, Zou W. Potassium channels and their role in glioma: A mini review. Mol Membr Biol (2019) 35(1):76–85. doi: 10.1080/09687688.2020.1729428
4. Prevarskaya N, Skryma R, Shuba Y. Ion channels in cancer: Are cancer hallmarks oncochannelopathies? Physiol Rev (2018) 98(2):559–621. doi: 10.1152/physrev.00044.2016
5. Alexander S, Striessnig J, Kelly E, Marrion N, Peters J, Faccenda E, et al. The concise guide to pharmacology 2017/18: Voltage-gated ion channels. British journal of pharmacology (2017) 174:S160–S94. doi: 10.1111/bph.13884
6. Thompson E, Eldstrom J, Fedida D. Hormonal signaling actions on Kv7.1 (Kcnq1) channels. Annu Rev Pharmacol Toxicol (2021) 61:381–400. doi: 10.1146/annurev-pharmtox-010919-023645
7. Serrano-Novillo C, Capera J, Colomer-Molera M, Condom E, Ferreres JC, Felipe A. Implication of voltage-gated potassium channels in neoplastic cell proliferation. Cancers (2019) 11(3). doi: 10.3390/cancers11030287
8. Pérez-García MT, Cidad P, López-López JR. The secret life of ion channels: Kv1.3 potassium channels and proliferation. Am J Physiol Cell Physiol (2018) 314(1):C27–c42. doi: 10.1152/ajpcell.00136.2017
9. Teisseyre A, Palko-Labuz A, Sroda-Pomianek K, Michalak K. Voltage-gated potassium channel Kv1.3 as a target in therapy of cancer. Front Oncol (2019) 9:933. doi: 10.3389/fonc.2019.00933
10. Than BL, Goos JA, Sarver AL, O'Sullivan MG, Rod A, Starr TK, et al. The role of Kcnq1 in mouse and human gastrointestinal cancers. Oncogene (2014) 33(29):3861–8. doi: 10.1038/onc.2013.350
11. Urrego D, Sánchez A, Tomczak AP, Pardo LA. The electric fence to cell-cycle progression: Do local changes in membrane potential facilitate disassembly of the primary cilium?: timely and localized expression of a potassium channel may set the conditions that allow retraction of the primary cilium. Bioessays (2017) 39(6). doi: 10.1002/bies.201600190
12. He S, Moutaoufik MT, Islam S, Persad A, Wu A, Aly KA, et al. Herg channel and cancer: A mechanistic review of carcinogenic processes and therapeutic potential. Biochim Biophys Acta Rev Cancer (2020) 1873(2):188355. doi: 10.1016/j.bbcan.2020.188355
13. Szabò I, Zoratti M, Gulbins E. Contribution of voltage-gated potassium channels to the regulation of apoptosis. FEBS Lett (2010) 584(10):2049–56. doi: 10.1016/j.febslet.2010.01.038
14. Desales-Salazar E, Khusro A, Cipriano-Salazar M, Barbabosa-Pliego A, Rivas-Caceres RR. Scorpion venoms and associated toxins as anticancer agents: Update on their application and mechanism of action. J Appl Toxicol (2020) 40(10):1310–24. doi: 10.1002/jat.3976
15. Jang SH, Byun JK, Jeon WI, Choi SY, Park J, Lee BH, et al. Nuclear localization and functional characteristics of voltage-gated potassium channel Kv1.3. J Biol Chem (2015) 290(20):12547–57. doi: 10.1074/jbc.M114.561324
16. Payne SL, Ram P, Srinivasan DH, Le TT, Levin M, Oudin MJ. Potassium channel-driven bioelectric signalling regulates metastasis in triple-negative breast cancer. EBioMedicine (2022) 75:103767. doi: 10.1016/j.ebiom.2021.103767
17. Rapetti-Mauss R, Bustos V, Thomas W, McBryan J, Harvey H, Lajczak N, et al. Bidirectional Kcnq1:β-catenin interaction drives colorectal cancer cell differentiation. Proc Natl Acad Sci U.S.A. (2017) 114(16):4159–64. doi: 10.1073/pnas.1702913114
18. Ren Z, Xu Y, Wang X, Ren M. Kcnq1ot1 affects cell proliferation, invasion, and migration through a mir-34a / Notch3 axis in breast cancer. Environ Sci pollut Res Int (2022) 29(19):28480–94. doi: 10.1007/s11356-021-18434-x
19. Chávez-López MG, Zúñiga-García V, Castro-Magdonel BE, Vera E, Garrido E, Sánchez-Ramos J, et al. Eag1 gene and protein expression in human retinoblastoma tumors and its regulation by prb in hela cells. Genes (Basel) (2020) 11(2). doi: 10.3390/genes11020119
20. Turgut Cosan D, Soyocak A, Oner C. Inhibition of Voltage−Gated potassium channels affect expressions of mir-126 and mir-126* in breast cancer cell lines. Bratisl Lek Listy (2020) 121(2):111–6. doi: 10.4149/bll_2020_014
21. Rao VR, Perez-Neut M, Kaja S, Gentile S. Voltage-gated ion channels in cancer cell proliferation. Cancers (2015) 7(2):849–75. doi: 10.3390/cancers7020813
22. Jiang Y, Senyuk V, Ma K, Chen H, Qin X, Li S, et al. Pharmacological activation of potassium channel Kv11.1 with Ns1643 attenuates triple negative breast cancer cell migration by promoting the dephosphorylation of caveolin-1. Cells (2022) 11(15). doi: 10.3390/cells11152461
23. Pointer KB, Clark PA, Eliceiri KW, Salamat MS, Robertson GA, Kuo JS. Administration of non-torsadogenic human ether-à-Go-Go-Related gene inhibitors is associated with better survival for high herg-expressing glioblastoma patients. Clin Cancer Res (2017) 23(1):73–80. doi: 10.1158/1078-0432.Ccr-15-3169
24. Fortunato A. The role of Herg1 ion channels in epithelial-mesenchymal transition and the capacity of riluzole to reduce cisplatin resistance in colorectal cancer cells. Cell Oncol (Dordr) (2017) 40(4):367–78. doi: 10.1007/s13402-017-0328-6
25. de Guadalupe Chávez-López M, Pérez-Carreón JI, Zuñiga-García V, Díaz-Chávez J, Herrera LA, Caro-Sánchez CH, et al. Astemizole-based anticancer therapy for hepatocellular carcinoma (Hcc), and Eag1 channels as potential early-stage markers of hcc. Tumour Biol J Int Soc Oncodevelopmental Biol Med (2015) 36(8):6149–58. doi: 10.1007/s13277-015-3299-0
26. Maljevic S, Lerche H. Potassium channels: A review of broadening therapeutic possibilities for neurological diseases. J Neurol (2013) 260(9):2201–11. doi: 10.1007/s00415-012-6727-8
27. Mohr CJ, Steudel FA, Gross D, Ruth P, Lo WY, Hoppe R, et al. Cancer-associated intermediate conductance Ca(2+)-activated K+ channel K(Ca)3.1. Cancers (2019) 11(1). doi: 10.3390/cancers11010109
28. Robles-Martínez L, Garay E, Martel-Gallegos MG, Cisneros-Mejorado A, Pérez-Montiel D, Lara A, et al. K(Ca)3.1 activation Via P2y(2) purinergic receptors promotes human ovarian cancer cell (Skov-3) migration. Sci Rep (2017) 7(1):4340. doi: 10.1038/s41598-017-04292-6
29. Zhang P, Yang X, Yin Q, Yi J, Shen W, Zhao L, et al. Inhibition of Sk4 potassium channels suppresses cell proliferation, migration and the epithelial-mesenchymal transition in triple-negative breast cancer cells. PloS One (2016) 11(4):e0154471. doi: 10.1371/journal.pone.0154471
30. Faouzi M, Hague F, Geerts D, Ay AS, Potier-Cartereau M, Ahidouch A, et al. Functional cooperation between Kca3.1 and Trpc1 channels in human breast cancer: Role in cell proliferation and patient prognosis. Oncotarget (2016) 7(24):36419–35. doi: 10.18632/oncotarget.9261
31. Zhang Y, Zhang P, Chen L, Zhao L, Zhu J, Zhu T. The long non-coding rna-14327.1 promotes migration and invasion potential of endometrial carcinoma cells by stabilizing the potassium channel Kca3.1. Onco Targets Ther (2019) 12:10287–97. doi: 10.2147/ott.S226737
32. Li QT, Feng YM, Ke ZH, Qiu MJ, He XX, Wang MM, et al. Kcnn4 promotes invasion and metastasis through the Mapk/Erk pathway in hepatocellular carcinoma. J Investig Med (2020) 68(1):68–74. doi: 10.1136/jim-2019-001073
33. Du Y, Song W, Chen J, Chen H, Xuan Z, Zhao L, et al. The potassium channel Kca3.1 promotes cell proliferation by activating Skp2 and metastasis through the emt pathway in hepatocellular carcinoma. Int J Cancer (2019) 145(2):503–16. doi: 10.1002/ijc.32121
34. He Y, Lin Y, He F, Shao L, Ma W, He F. Role for calcium-activated potassium channels (Bk) in migration control of human hepatocellular carcinoma cells. J Cell Mol Med (2021) 25(20):9685–96. doi: 10.1111/jcmm.16918
35. Hibino H, Inanobe A, Furutani K, Murakami S, Findlay I, Kurachi Y. Inwardly rectifying potassium channels: Their structure, function, and physiological roles. Physiol Rev (2010) 90(1):291–366. doi: 10.1152/physrev.00021.2009
36. Abraham MR, Jahangir A, Alekseev AE, Terzic A. Channelopathies of inwardly rectifying potassium channels. FASEB J Off Publ Fed Am Societies Exp Biol (1999) 13(14):1901–10. doi: 10.1096/fasebj.13.14.1901
37. Zhang J, Han J, Li L, Zhang Q, Feng Y, Jiang Y, et al. Inwardly rectifying potassium channel 5.1: Structure, function, and possible roles in diseases. Genes Dis (2021) 8(3):272–8. doi: 10.1016/j.gendis.2020.03.006
38. Chen S, Huang M, Hu X. Interference with Kcnj2 inhibits proliferation, migration and emt progression of apillary thyroid carcinoma cells by upregulating Gng2 expression. Mol Med Rep (2021) 24(3):1–10. doi: 10.3892/mmr.2021.12261
39. Wu XY, Xie Y, Zhou LY, Zhao YY, Zhang J, Zhang XF, et al. Long noncoding rna Pou6f2-As1 regulates lung cancer aggressiveness through sponging mir-34c-5p to modulate Kcnj4 expression. Genet Mol Biol (2021) 44(2):e20200050. doi: 10.1590/1678-4685-gmb-2020-0050
40. Fukushiro-Lopes D, Hegel AD, Russo A, Senyuk V, Liotta M, Beeson GC, et al. Repurposing Kir6/Sur2 channel activator minoxidil to arrests growth of gynecologic cancers. Front Pharmacol (2020) 11:577. doi: 10.3389/fphar.2020.00577
41. Thuringer D, Chanteloup G, Boucher J, Pernet N, Boudesco C, Jego G, et al. Modulation of the inwardly rectifying potassium channel Kir4.1 by the pro-invasive mir-5096 in glioblastoma cells. Oncotarget (2017) 8(23):37681–93. doi: 10.18632/oncotarget.16949
42. Liu Y, Wang H, Ni B, Zhang J, Li S, Huang Y, et al. Loss of Kcnj15 expression promotes malignant phenotypes and correlates with poor prognosis in renal carcinoma. Cancer Manag Res (2019) 11:1211–20. doi: 10.2147/cmar.S184368
43. Schewe M, Nematian-Ardestani E, Sun H, Musinszki M, Cordeiro S, Bucci G, et al. A non-canonical voltage-sensing mechanism controls gating in K2p k(+) channels. Cell (2016) 164(5):937–49. doi: 10.1016/j.cell.2016.02.002
44. Piechotta PL, Rapedius M, Stansfeld PJ, Bollepalli MK, Ehrlich G, Andres-Enguix I, et al. The pore structure and gating mechanism of K2p channels. EMBO J (2011) 30(17):3607–19. doi: 10.1038/emboj.2011.268
45. Lee LM, Müntefering T, Budde T, Meuth SG, Ruck T. Pathophysiological role of K(2p) channels in human diseases. Cell Physiol Biochem Int J Exp Cell physiology biochemistry Pharmacol (2021) 55(S3):65–86. doi: 10.33594/000000338
46. Li WC, Xiong ZY, Huang PZ, Liao YJ, Li QX, Yao ZC, et al. Kcnk levels are prognostic and diagnostic markers for hepatocellular carcinoma. Aging (Albany NY) (2019) 11(19):8169–82. doi: 10.18632/aging.102311
47. Lin G, Lin L, Lin H, Chen W, Chen L, Chen X, et al. Kcnk3 inhibits proliferation and glucose metabolism of lung adenocarcinoma Via activation of ampk-txnip pathway. Cell Death Discovery (2022) 8(1):360. doi: 10.1038/s41420-022-01152-9
48. Leithner K, Hirschmugl B, Li Y, Tang B, Papp R, Nagaraj C, et al. Task-1 regulates apoptosis and proliferation in a subset of non-small cell lung cancers. PloS One (2016) 11(6):e0157453. doi: 10.1371/journal.pone.0157453
49. He Y, Hu H, Wang Y, Yuan H, Lu Z, Wu P, et al. Alkbh5 inhibits pancreatic cancer motility by decreasing long non-coding rna Kcnk15-As1 methylation. Cell Physiol Biochem Int J Exp Cell physiology biochemistry Pharmacol (2018) 48(2):838–46. doi: 10.1159/000491915
50. Hou X, Tang L, Li X, Xiong F, Mo Y, Jiang X, et al. Potassium channel protein Kcnk6 promotes breast cancer cell proliferation, invasion, and migration. Front Cell Dev Biol (2021) 9:616784. doi: 10.3389/fcell.2021.616784
51. Zúñiga R, Valenzuela C, Concha G, Brown N, Zúñiga L. Task-3 downregulation triggers cellular senescence and growth inhibition in breast cancer cell lines. Int J Mol Sci (2018) 19(4). doi: 10.3390/ijms19041033
52. Cikutović-Molina R, Herrada AA, González W, Brown N, Zúñiga L. Task-3 gene knockdown dampens invasion and migration and promotes apoptosis in kato iii and mkn-45 human gastric adenocarcinoma cell lines. Int J Mol Sci (2019) 20(23). doi: 10.3390/ijms20236077
53. Zavala WD, Foscolo MR, Kunda PE, Cavicchia JC, Acosta CG. Changes in the expression of the potassium channels Task1, Task3 and tresk in a rat model of oral squamous cell carcinoma and their relation to malignancy. Arch Oral Biol (2019) 100:75–85. doi: 10.1016/j.archoralbio.2019.02.007
54. He Y, Yue H, Cheng Y, Ding Z, Xu Z, Lv C, et al. Alkbh5-mediated M(6)a demethylation of Kcnk15-As1 inhibits pancreatic cancer progression Via regulating Kcnk15 and Pten/Akt signaling. Cell Death Dis (2021) 12(12):1121. doi: 10.1038/s41419-021-04401-4
55. Lowinus T, Heidel FH, Bose T, Nimmagadda SC, Schnöder T, Cammann C, et al. Memantine potentiates cytarabine-induced cell death of acute leukemia correlating with inhibition of K(V)1.3 potassium channels, akt and Erk1/2 signaling. Cell communication Signaling (2019) 17(1):5. doi: 10.1186/s12964-018-0317-z
56. Severin F, Urbani A, Varanita T, Bachmann M, Azzolini M, Martini V, et al. Pharmacological modulation of Kv1.3 potassium channel selectively triggers pathological b lymphocyte apoptosis in vivo in a genetic cll model. J Exp Clin Cancer Res (2022) 41(1):64. doi: 10.1186/s13046-022-02249-w
57. Leanza L, Henry B, Sassi N, Zoratti M, Chandy KG, Gulbins E, et al. Inhibitors of mitochondrial Kv1.3 channels induce Bax/Bak-independent death of cancer cells. EMBO Mol Med (2012) 4(7):577–93. doi: 10.1002/emmm.201200235
58. Gubič Š, Hendrickx LA, Shi X, Toplak Ž, Možina Š, Theemsche KMV, et al. Design of new potent and selective thiophene-based K(V)1.3 inhibitors and their potential for anticancer activity. Cancers (2022) 14(11). doi: 10.3390/cancers14112595
59. Agarwal JR, Griesinger F, Stühmer W, Pardo LA. The potassium channel ether à go-go is a novel prognostic factor with functional relevance in acute myeloid leukemia. Mol Cancer (2010) 9:18. doi: 10.1186/1476-4598-9-18
60. Huang X, He Y, Dubuc AM, Hashizume R, Zhang W, Reimand J, et al. Eag2 potassium channel with evolutionarily conserved function as a brain tumor target. Nat Neurosci (2015) 18(9):1236–46. doi: 10.1038/nn.4088
61. Ramírez A, Vera E, Gamboa-Domínguez A, Lambert P, Gariglio P, Camacho J. Calcium-activated potassium channels as potential early markers of human cervical cancer. Oncol Lett (2018) 15(5):7249–54. doi: 10.3892/ol.2018.8187
62. Jiang S, Zhu L, Yang J, Hu L, Gu J, Xing X, et al. Integrated expression profiling of potassium channels identifys Kcnn4 as a prognostic biomarker of pancreatic cancer. Biochem Biophys Res Commun (2017) 494(1-2):113–9. doi: 10.1016/j.bbrc.2017.10.072
63. Valle-Reyes S, Valencia-Cruz G, Liñan-Rico L, Pottosin I, Dobrovinskaya O. Differential activity of voltage- and Ca(2+)-dependent potassium channels in leukemic T cell lines: Jurkat cells represent an exceptional case. Front Physiol (2018) 9:499. doi: 10.3389/fphys.2018.00499
64. Ji CD, Wang YX, Xiang DF, Liu Q, Zhou ZH, Qian F, et al. Kir2.1 interaction with Stk38 promotes invasion and metastasis of human gastric cancer by enhancing Mekk2-Mek1/2-Erk1/2 signaling. Cancer Res (2018) 78(11):3041–53. doi: 10.1158/0008-5472.Can-17-3776
65. Capatina AL, Lagos D, Brackenbury WJ. Targeting ion channels for cancer treatment: Current progress and future challenges. Rev Physiol Biochem Pharmacol (2020) 183:1–43. doi: 10.1007/112_2020_46
66. Checchetto V, Prosdocimi E, Leanza L. Mitochondrial Kv1.3: A new target in cancer biology? Cell Physiol Biochem Int J Exp Cell physiology biochemistry Pharmacol (2019) 53(S1):52–62. doi: 10.33594/000000195
67. Kadow S, Schumacher F, Kramer M, Hessler G, Scholtysik R, Oubari S, et al. Mitochondrial Kv1.3 channels as target for treatment of multiple myeloma. Cancers (2022) 14(8). doi: 10.3390/cancers14081955
68. Leanza L, Romio M, Becker KA, Azzolini M, Trentin L, Managò A, et al. Direct pharmacological targeting of a mitochondrial ion channel selectively kills tumor cells. In vivo. Cancer Cell (2017) 31(4):516–31.e10. doi: 10.1016/j.ccell.2017.03.003
69. Valle-Reyes S, Dobrovinskaya O, Pottosin I. Kv1.3 current voltage dependence in lymphocytes is modulated by Co-culture with bone marrow-derived stromal cells: B and T cells respond differentially. Cell Physiol Biochem Int J Exp Cell physiology biochemistry Pharmacol (2020) 54(5):842–52. doi: 10.33594/000000273
70. Barriga-Montoya C, Huanosta-Gutiérrez A, Reyes-Vaca A, Hernández-Cruz A, Picones A, Gómez-Lagunas F. Inhibition of the k(+) conductance and Cole-Moore shift of the oncogenic Kv10.1 channel by amiodarone. Pflugers Archiv Eur J Physiol (2018) 470(3):491–503. doi: 10.1007/s00424-017-2092-x
71. Valdés-Abadía B, Morán-Zendejas R, Rangel-Flores JM, Rodríguez-Menchaca AA. Chloroquine inhibits tumor-related Kv10.1 channel and decreases migration of mda-Mb-231 breast cancer cells in vitro. Eur J Pharmacol (2019) 855:262–6. doi: 10.1016/j.ejphar.2019.05.017
72. Na W, Ma B, Shi S, Chen Y, Zhang H, Zhan Y, et al. Procyanidin B1, a novel and specific inhibitor of Kv10.1 channel, suppresses the evolution of hepatoma. Biochem Pharmacol (2020) 178:114089. doi: 10.1016/j.bcp.2020.114089
73. Hartung F, Krüwel T, Shi X, Pfizenmaier K, Kontermann R, Chames P, et al. A novel anti-Kv10.1 nanobody fused to single-chain trail enhances apoptosis induction in cancer cells. Front Pharmacol (2020) 11:686. doi: 10.3389/fphar.2020.00686
74. Fukushiro-Lopes DF, Hegel AD, Rao V, Wyatt D, Baker A, Breuer EK, et al. Preclinical study of a Kv11.1 potassium channel activator as antineoplastic approach for breast cancer. Oncotarget (2018) 9(3):3321–37. doi: 10.18632/oncotarget.22925
75. Breuer EK, Fukushiro-Lopes D, Dalheim A, Burnette M, Zartman J, Kaja S, et al. Potassium channel activity controls breast cancer metastasis by affecting β-catenin signaling. Cell Death Dis (2019) 10(3):180. doi: 10.1038/s41419-019-1429-0
76. Lastraioli E, Iorio J, Petrelli F, Tomezzoli A, Battista S, Ambrosio MR, et al. Herg1 potassium channel expression in colorectal adenomas: Comparison with other preneoplastic lesions of the gastrointestinal tract. Curr Issues Mol Biol (2022) 44(3):1326–31. doi: 10.3390/cimb44030089
77. Iorio J, Lastraioli E, Tofani L, Petroni G, Antonuzzo L, Messerini L, et al. Herg1 and hif-2α behave as biomarkers of positive response to bevacizumab in metastatic colorectal cancer patients. Transl Oncol (2020) 13(3):100740. doi: 10.1016/j.tranon.2020.01.001
78. Petroni G, Bagni G, Iorio J, Duranti C, Lottini T, Stefanini M, et al. Clarithromycin inhibits autophagy in colorectal cancer by regulating the Herg1 potassium channel interaction with Pi3k. Cell Death Dis (2020) 11(3):161. doi: 10.1038/s41419-020-2349-8
79. Uil SH, Coupé VMH, Bril H, Meijer GA, Fijneman RJA, Stockmann H. Kcnq1 and lymphovascular invasion are key features in a prognostic classifier for stage ii and iii colon cancer. BMC Cancer (2022) 22(1):372. doi: 10.1186/s12885-022-09473-9
80. Bonnet S, Archer SL, Allalunis-Turner J, Haromy A, Beaulieu C, Thompson R, et al. A mitochondria-k+ channel axis is suppressed in cancer and its normalization promotes apoptosis and inhibits cancer growth. Cancer Cell (2007) 11(1):37–51. doi: 10.1016/j.ccr.2006.10.020
81. Díaz-García A, Varela D. Voltage-gated K(+)/Na(+) channels and scorpion venom toxins in cancer. Front Pharmacol (2020) 11:913. doi: 10.3389/fphar.2020.00913
82. D'Arcangelo D, Scatozza F, Giampietri C, Marchetti P, Facchiano F, Facchiano A. Ion channel expression in human melanoma samples: In silico identification and experimental validation of molecular targets. Cancers (2019) 11(4). doi: 10.3390/cancers11040446
83. Liu X, Wei L, Zhao B, Cai X, Dong C, Yin F. Low expression of Kcnn3 may affect drug resistance in ovarian cancer. Mol Med Rep (2018) 18(2):1377–86. doi: 10.3892/mmr.2018.9107
84. Gueguinou M, Crottès D, Chantôme A, Rapetti-Mauss R, Potier-Cartereau M, Clarysse L, et al. The Sigmar1 chaperone drives breast and colorectal cancer cell migration by tuning Sk3-dependent Ca(2+) homeostasis. Oncogene (2017) 36(25):3640–7. doi: 10.1038/onc.2016.501
85. Bulk E, Ay AS, Hammadi M, Ouadid-Ahidouch H, Schelhaas S, Hascher A, et al. Epigenetic dysregulation of kca 3.1 channels induces poor prognosis in lung cancer. Int J Cancer (2015) 137(6):1306–17. doi: 10.1002/ijc.29490
86. Wen J, Lin B, Lin L, Chen Y, Wang O. Kcnn4 is a diagnostic and prognostic biomarker that promotes papillary thyroid cancer progression. Aging (Albany NY) (2020) 12(16):16437–56. doi: 10.18632/aging.103710
87. Yeh PS, Wu SJ, Hung TY, Huang YM, Hsu CW, Sze CI, et al. Evidence for the inhibition by temozolomide, an imidazotetrazine family alkylator, of intermediate-conductance Ca2+-activated k+ channels in glioma cells. Cell Physiol Biochem Int J Exp Cell physiology biochemistry Pharmacol (2016) 38(5):1727–42. doi: 10.1159/000443112
88. Roth B, Gibhardt CS, Becker P, Gebhardt M, Knoop J, Fournier C, et al. Low-dose photon irradiation alters cell differentiation Via activation of hik channels. Pflugers Archiv Eur J Physiol (2015) 467(8):1835–49. doi: 10.1007/s00424-014-1601-4
89. Todesca LM, Maskri S, Brömmel K, Thale I, Wünsch B, Koch O, et al. Targeting K(Ca)3.1 channels in cancer. Cell Physiol Biochem Int J Exp Cell physiology biochemistry Pharmacol (2021) 55(S3):131–44. doi: 10.33594/000000374
90. Qiu S, Fraser SP, Pires W, Djamgoz MBA. Anti-invasive effects of minoxidil on human breast cancer cells: Combination with ranolazine. Clin Exp metastasis (2022) 39(4):679–89. doi: 10.1007/s10585-022-10166-7
91. Sauter DR, Sørensen CE, Rapedius M, Brüggemann A, Novak I. Ph-sensitive k(+) channel trek-1 is a novel target in pancreatic cancer. Biochim Biophys Acta (2016) 1862(10):1994–2003. doi: 10.1016/j.bbadis.2016.07.009
92. Pethő Z, Najder K, Bulk E, Schwab A. Mechanosensitive ion channels push cancer progression. Cell Calcium (2019) 80:79–90. doi: 10.1016/j.ceca.2019.03.007
93. Lin X, Wu JF, Wang DM, Zhang J, Zhang WJ, Xue G. The correlation and role analysis of Kcnk2/4/5/15 in human papillary thyroid carcinoma microenvironment. J Cancer (2020) 11(17):5162–76. doi: 10.7150/jca.45604
94. Stegen B, Klumpp L, Misovic M, Edalat L, Eckert M, Klumpp D, et al. K(+) channel signaling in irradiated tumor cells. Eur Biophys J (2016) 45(7):585–98. doi: 10.1007/s00249-016-1136-z
95. Klopfleisch R, Kohn B, Gruber AD. Mechanisms of tumour resistance against chemotherapeutic agents in veterinary oncology. Veterinary J (London Engl 1997) (2016) 207:63–72. doi: 10.1016/j.tvjl.2015.06.015
96. Hui C, Lan Z, Yue-li L, Li-lin H, Li-lin H. Knockdown of Eag1 expression by rna interference increases chemosensitivity to cisplatin in ovarian cancer cells. Reprod Sci (2015) 22(12):1618–26. doi: 10.1177/1933719115590665
97. Hernández-Reséndiz I, Pacheu-Grau D, Sánchez A, Pardo LA. Inhibition of Kv10.1 channels sensitizes mitochondria of cancer cells to antimetabolic agents. Cancers (2020) 12(4). doi: 10.3390/cancers12040920
98. Hartung F, Pardo LA. Guiding trail to cancer cells through Kv10.1 potassium channel overcomes resistance to doxorubicin. Eur Biophys J (2016) 45(7):709–19. doi: 10.1007/s00249-016-1149-7
99. Sun H, Sun Y, Chen Q, Xu Z. Lncrna Kcnq1ot1 contributes to the progression and chemoresistance in acute myeloid leukemia by modulating Tspan3 through suppressing mir-193a-3p. Life Sci (2020) 241:117161. doi: 10.1016/j.lfs.2019.117161
100. Lin P, Li J, Ye F, Fu W, Hu X, Shao Z, et al. Kcnn4 induces multiple chemoresistance in breast cancer by regulating Bcl2a1. Am J Cancer Res (2020) 10(10):3302–15.
101. Mohr CJ, Gross D, Sezgin EC, Steudel FA, Ruth P, Huber SM, et al. K(Ca)3.1 channels confer radioresistance to breast cancer cells. Cancers (2019) 11(9). doi: 10.3390/cancers11091285
102. Pillozzi S, D'Amico M, Bartoli G, Gasparoli L, Petroni G, Crociani O, et al. The combined activation of K(Ca)3.1 and inhibition of K(V)11.1/Herg1 currents contribute to overcome cisplatin resistance in colorectal cancer cells. Br J Cancer (2018) 118(2):200–12. doi: 10.1038/bjc.2017.392
103. Glaser F, Hundehege P, Bulk E, Todesca LM, Schimmelpfennig S, Nass E, et al. K(Ca) channel blockers increase effectiveness of the egf receptor tk inhibitor erlotinib in non-small cell lung cancer cells (A549). Sci Rep (2021) 11(1):18330. doi: 10.1038/s41598-021-97406-0
104. D'Alessandro G, Monaco L, Catacuzzeno L, Antonangeli F, Santoro A, Esposito V, et al. Radiation increases functional Kca3.1 expression and invasiveness in glioblastoma. Cancers (2019) 11(3). doi: 10.3390/cancers11030279
105. Liu H, Huang J, Peng J, Wu X, Zhang Y, Zhu W, et al. Upregulation of the inwardly rectifying potassium channel Kir2.1 (Kcnj2) modulates multidrug resistance of small-cell lung cancer under the regulation of mir-7 and the Ras/Mapk pathway. Mol Cancer (2015) 14:59. doi: 10.1186/s12943-015-0298-0
106. Chandy KG, Norton RS. Immunology: Channelling potassium to fight cancer. Nature (2016) 537(7621):497–9. doi: 10.1038/nature19467
107. Chimote AA, Hajdu P, Sfyris AM, Gleich BN, Wise-Draper T, Casper KA, et al. Kv1.3 channels mark functionally competent Cd8+ tumor-infiltrating lymphocytes in head and neck cancer. Cancer Res (2017) 77(1):53–61. doi: 10.1158/0008-5472.Can-16-2372
108. Serrano-Albarrás A, Estadella I, Cirera-Rocosa S, Navarro-Pérez M, Felipe A. Kv1.3: A multifunctional channel with many pathological implications. Expert Opin Ther Targets (2018) 22(2):101–5. doi: 10.1080/14728222.2017.1420170
109. Abid MN, Qadir FA, Salihi A. Association between the serum concentrations and mutational status of il-8, il-27 and vegf and the expression levels of the herg potassium channel gene in patients with colorectal cancer. Oncol Lett (2021) 22(3):665. doi: 10.3892/ol.2021.12926
110. Saadat F, Zareighane Z, Safavifar F, Jalali SZ, Berahmeh A, Khorramizadeh MR. The repression of matrix metalloproteinases and cytokine secretion in glioblastoma by targeting k+ channel. Basic Clin Neurosci (2021) 12(6):737–44. doi: 10.32598/bcn.2021.1693.1
111. Eil R, Vodnala SK, Clever D, Klebanoff CA, Sukumar M, Pan JH, et al. Ionic immune suppression within the tumour microenvironment limits T cell effector function. Nature (2016) 537(7621):539–43. doi: 10.1038/nature19364
112. Chimote AA, Balajthy A, Arnold MJ, Newton HS, Hajdu P, Qualtieri J, et al. A defect in Kca3.1 channel activity limits the ability of Cd8(+) T cells from cancer patients to infiltrate an adenosine-rich microenvironment. Sci Signal (2018) 11(527). doi: 10.1126/scisignal.aaq1616
113. Ohya S, Matsui M, Kajikuri J, Kito H, Endo K. Downregulation of il-8 and il-10 by the activation of Ca(2+)-activated k(+) channel K(Ca)3.1 in thp-1-Derived M(2) macrophages. Int J Mol Sci (2022) 23(15). doi: 10.3390/ijms23158603
114. Matsui M, Kajikuri J, Kito H, Endo K, Hasegawa Y, Murate S, et al. Inhibition of interleukin 10 transcription through the Smad2/3 signaling pathway by Ca(2+)-activated k(+) channel K(Ca)3.1 activation in human T-cell lymphoma hut-78 cells. Mol Pharmacol (2019) 95(3):294–302. doi: 10.1124/mol.118.114405
115. Chen S, Su X, Mo Z. Kcnn4 is a potential biomarker for predicting cancer prognosis and an essential molecule that remodels various components in the tumor microenvironment: A pan-cancer study. Front Mol Biosci (2022) 9:812815. doi: 10.3389/fmolb.2022.812815
116. Sun H, Luo L, Lal B, Ma X, Chen L, Hann CL, et al. A monoclonal antibody against Kcnk9 k(+) channel extracellular domain inhibits tumour growth and metastasis. Nat Commun (2016) 7:10339. doi: 10.1038/ncomms10339
117. Siegel RL, Miller KD, Fuchs HE, Jemal A. Cancer statistics, 2022. CA: Cancer J Clin (2022) 72(1):7–33. doi: 10.3322/caac.21708
118. Zou Y, Xie J, Tian W, Wu L, Xie Y, Huang S, et al. Integrative analysis of kcnk genes and establishment of a specific prognostic signature for breast cancer. Front Cell Dev Biol (2022) 10:839986. doi: 10.3389/fcell.2022.839986
119. Erdem Kış E, Tiftik RN, Al Hennawi K, Ün İ. The role of potassium channels in the proliferation and migration of endometrial adenocarcinoma Hec1-a cells. Mol Biol Rep (2022) 49(8):7447–54. doi: 10.1007/s11033-022-07546-3
120. Vodnala S, Eil R, Kishton R, Sukumar M, Yamamoto T, Ha N, et al. T Cell stemness and dysfunction in tumors are triggered by a common mechanism. Science (New York, N. Y.) (2019) 363(6434):eaau0135. doi: 10.1126/science.aau0135
Keywords: potassium channel, tumor, drug target, chemoresistance, research progress
Citation: Li M, Tian P, Zhao Q, Ma X and Zhang Y (2023) Potassium channels: Novel targets for tumor diagnosis and chemoresistance. Front. Oncol. 12:1074469. doi: 10.3389/fonc.2022.1074469
Received: 19 October 2022; Accepted: 21 December 2022;
Published: 10 January 2023.
Edited by:
Tiago Rodrigues, Federal University of ABC, BrazilReviewed by:
Tao Zhou, Dali University, ChinaEdgar Paredes-Gamero, Federal University of Mato Grosso do Sul, Brazil
Copyright © 2023 Li, Tian, Zhao, Ma and Zhang. This is an open-access article distributed under the terms of the Creative Commons Attribution License (CC BY). The use, distribution or reproduction in other forums is permitted, provided the original author(s) and the copyright owner(s) are credited and that the original publication in this journal is cited, in accordance with accepted academic practice. No use, distribution or reproduction is permitted which does not comply with these terms.
*Correspondence: Yunxiang Zhang, emhhbmdiaW5nMTk5NTkyQDE2My5jb20=