- 1Division of Medical Oncology, Department of Medicine, University of Washington, Seattle, WA, United States
- 2Clinical Research Division, Fred Hutchinson Cancer Center, Seattle, WA, United States
Autologous chimeric antigen receptor T-cell (CAR-T) therapies targeting B-cell maturation antigen (BCMA) have revolutionized the field of multiple myeloma in the same way that the Ford Model T revolutionized the original CAR world a century ago. However, we are only beginning to understand how to improve the efficacy and usability of these cellular therapies. In this review, we explore three automotive analogies for innovation with BCMA CAR-T therapies: stronger engines, better mileage, and hassle-free delivery. Firstly, we can build stronger engines in terms of BCMA targeting: improved antigen binding, tools to modulate antigen density, and armoring to better reach the antigen itself. Secondly, we can improve “mileage” in terms of response durability through ex vivo CAR design and in vivo immune manipulation. Thirdly, we can implement hassle-free delivery through rapid manufacturing protocols and off-the-shelf products. Just as the Model T set a benchmark for car manufacturing over 100 years ago, idecabtagene vicleucel and ciltacabtagene autoleucel have now set the starting point for BCMA CAR-T therapy with their approvals. As with any emerging technology, whether automotive or cellular, the best in innovation and optimization is yet to come.
Introduction
Of the many car-related analogies that have entered the world of chimeric antigen receptor T-cell (CAR-T) therapy, the comparison between modern CAR-T therapies and the original Ford Model T is a particularly apt one. Although automobiles were available as early as 1893, the advent of the Model T in 1908 revolutionized the world through innovative and scalable manufacturing processes (1, 2). Similarly, first-generation CAR-T therapies were initially developed in the 1990s but were impractical due to poor in vivo activity (3, 4). Not until two decades later did the advent of modern CAR constructs with costimulatory domains revolutionize the treatment of multiple myeloma. Autologous CAR-T therapies targeting B-cell maturation antigen (BCMA) such as idecabtagene vicleucel (ide-cel) and ciltacabtagene autoleucel (cilta-cel) have offered response depths and durations among patients with highly refractory myeloma that would have been unimaginable even 5 years ago. In the CARTITUDE-1 trial of cilta-cel, for example, median progression-free-survival (PFS) has not yet been reached despite over 2 years of follow-up (5). Of the subset of CARTITUDE-1 participants evaluable for measurable residual disease (MRD), an astonishing 92% achieved MRD negativity at 10-5 despite having received 3+ prior lines of therapy for their cancer.
That being said, the need for automotive improvement in the year 1908 was just as pronounced as the need for BCMA CAR-T improvement today. Median PFS in the KarMMa trial of ide-cel was only 8.8 months, while Kaplan-Meier curves from CARTITUDE-1 continue to lack a plateau (5, 6). Slots for apheresis before BCMA CAR-T therapy remain in short supply, and the several-month interval required for CAR-T cell manufacturing (if successful at all) means that many patients do not receive CAR-T therapy despite undergoing T-cell collection (7, 8). Thankfully, many innovative strategies are under investigation to address these limitations. In this review, we discuss such approaches using the three broad automotive analogies shown in Figure 1 (1): stronger engines, referring to more potent BCMA targeting (2); better mileage, referring to longer duration of responses; and (3) hassle-free delivery, referring to reduced ‘brain-to-vein’ times between CAR-T consultation and infusion. While still investigational, these strategies collectively promise to dramatically improve the efficacy and practicality of BCMA CAR-T therapy in multiple myeloma.
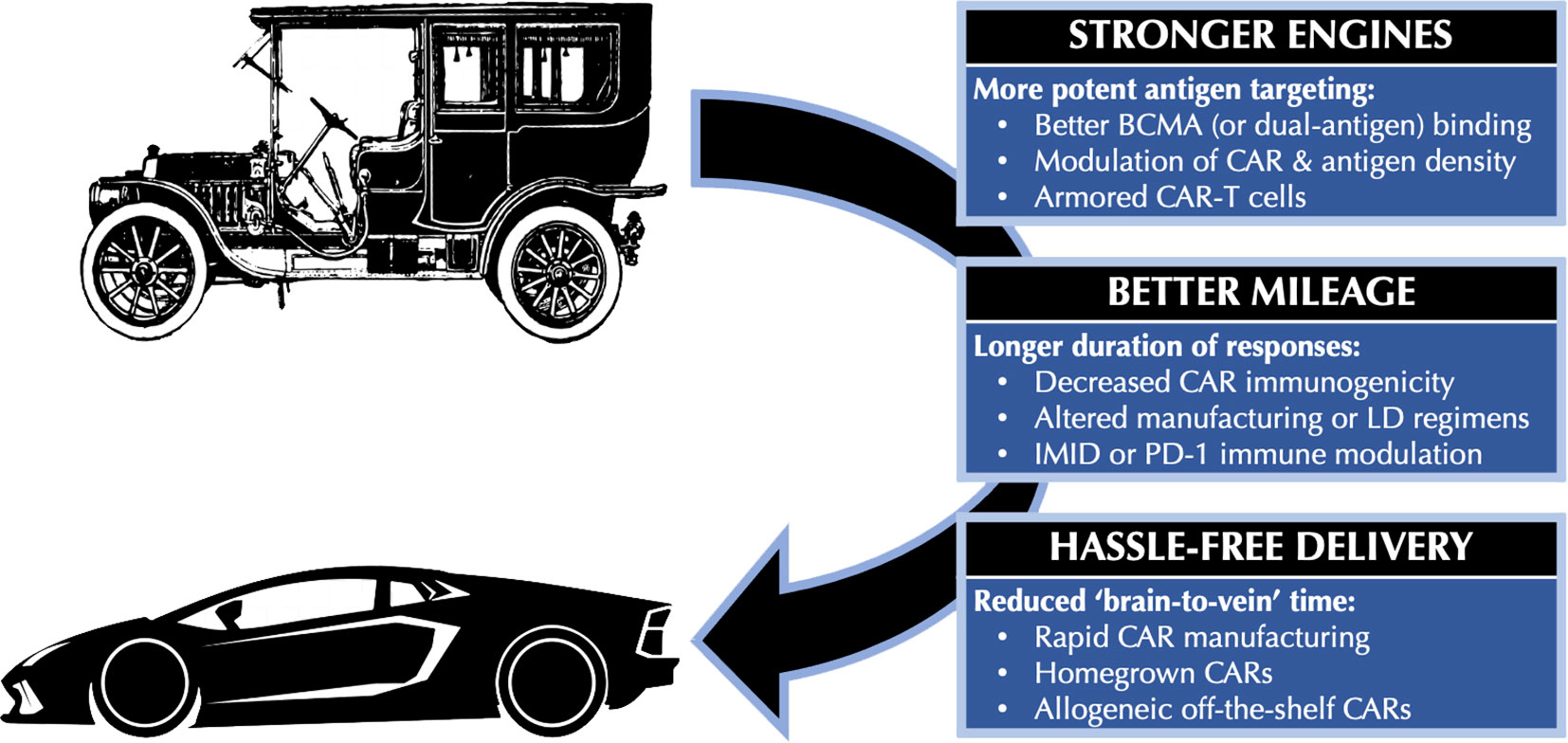
Figure 1 Automotive analogies for improving BCMA CAR-T therapy. BCMA, B-cell maturation antigen; CAR, chimeric antigen receptor; CAR-T, chimeric antigen receptor T-cell; IMID, immunomodulatory drug; LD, lymphodepletion; PD-1, Programmed Death 1.
Stronger engines: More potent antigen targeting
Improved BCMA target binding
The development of second-generation CARs with co-stimulatory domains to better mimic native T-cell receptor (TCR) function was a seminal step forward for the field of adoptive cellular therapy. Many strategies are under development to continue improving “under the hood” CAR signaling once the receptor binds its target. For example, third-generation CARs contain more than one co-stimulatory domain with the goal of more potently activating T cells (9, 10). Similarly, adding a chimeric CD38-binding costimulatory receptor to a BCMA-binding CAR may potentiate the activity and persistence of CAR-T cells (11). Most innovations to date, however, have focused on improving the odds of successful target binding on cancer cells through one of four approaches (1): alternative BCMA targeting domains (2), modulation of CAR or target antigen density (3), dual targeting of BCMA alongside other antigens, and (4) “armored” CARs to help CAR-T cells penetrate the tumor microenvironment.
Cilta-cel, formerly developed in China as LCAR-B38M and in the United States as JNJ-4528, is the most prominent example of alternative BCMA targeting. The second-generation CAR in cilta-cel contains two separate BCMA-binding fragments of heavy-chain-only antibodies (VHH) (12–14). The biepitopic targeting of BCMA with two separate llama-derived VHH domains is relatively unusual compared to most other CARs, which use a single-chain variable fragment (scFv, derived from both heavy and light chains) to bind a single epitope (15). Compared to other BCMA CAR-T therapies, the kinetics of toxicities such as cytokine release syndrome (CRS) and immune effector cell-associated neurotoxicity syndrome (ICANS) are slower with cilta-cel; additionally, the CARTITUDE-1 trial of cilta-cel has demonstrated impressive results with a median PFS exceeding 2 years (5, 13). However, differences in patient populations and disease burden make any cross-trial comparisons between cilta-cel and ide-cel extremely difficult. Optimal CAR-T doses may differ as well: for example, the KarMMa trial investigated flat dose levels between 150-450 million CAR-T cells per patient, while the CARTITUDE-1 trial used weight-based doses ranging between 0.5-1.0 million viable CAR-T cells per kilogram of body weight (6, 13). Further studies are needed to confirm the degree to which any of these characteristics – biepitopic BCMA binding, use of VHH rather than scFv domains, or weight-based dosing in this range – contribute to cilta-cel’s unique characteristics.
Modulation of CAR and target antigen density
Translational research suggests that CAR density and antigen binding affinity influence the functionality of BCMA CAR-T cells in unexpected ways. While one might suspect that more CARs per individual T cell would correspond to more cancer cell death, higher CAR expression may actually be associated with increased T-cell exhaustion and decreased CAR-T cell infiltration into the bone marrow (16). In the future, modifications to viral promoter genes or CRISPR-mediated precision regarding the location of CAR insertion into the host genome may allow for manipulation of expected levels of CAR expression per cell (17–19). Avoiding overly strong CAR affinity may lower T-cell exhaustion as well (20). Interestingly, lowering CAR affinity for target antigens may also lower the risk of trogocytosis, a complex process by which the CAR-T cell strips BCMA from the target cell before itself expressing the same molecule (and thus becoming a potential victim to fratricide by other CAR-T cells) (21, 22).
Modulation of target antigen density – namely, membrane-bound BCMA on myeloma cells – also warrants further investigation as well. Gamma secretase is used by plasma cells to shed BCMA from their surfaces, and pre-clinical work suggests that tumor exposure to gamma secretase inhibitors (GSI) can enhance the anti-tumor efficacy of CAR-T cells (23–26). In a subsequent Phase 1 study of 18 patients treated concurrently with an oral GSI and BCMA CAR-T therapy (including 7 who had received prior BCMA-targeted therapy), the overall response rate (ORR) was 89% and median PFS was 11 months (26, 27). In this study, numbers of BCMA molecules per cell increased by a median of 12-fold and a maximum of 157-fold after GSI exposure. Trials of GSIs combined with a number of BCMA-targeted non-CAR-T therapies, including bispecific antibodies and antibody-drug conjugates, are under way as well. As a caveat, a recent case report with whole-genome sequencing suggests that complete genetic knockout of the gamma secretase gene may be associated with worsened CRS due to extremely high BCMA expression and CAR-T cell activation (28).
Dual targeting of BCMA and other antigens
Because BCMA loss at a genetic level renders tumor cells resistant both to BCMA CAR-T therapy and to the effects of GSIs (29, 30), several groups have explored multi-antigen targeting to expand the versatility of CAR-T cells. An early such approach involved the APRIL protein (BCMA’s natural ligand), which also binds the related TACI protein on myeloma cells. Despite being able to target TACI on BCMA-negative myeloma cells, however, the APRIL-expressing AUTO2 CAR-T therapy is reportedly no longer under commercial development (31–33). Other distinct antigens in multiple myeloma include CD19 (the target of most CAR-T therapies in lymphoma), CD38 (the target of daratumumab and isatuximab), CS1/SLAMF7 (the target of elotuzumab), and GPRC5D (the target of the investigational bispecific antibody talquetamab). As shown in Table 1, the operationalization of dual-targeting CARs can vary widely from single-stalk tandem CARs to bicistronic CARs to “CAR-pools” (37, 38, 43, 44). To date, pre-clinical studies have shown mixed results in terms of which approach may be superior (37, 38).
As noted in Table 1, dual-targeting CARs have investigated a number of other antigens alongside BCMA including CD19 (34, 39–42), CD38 (35), CS1 (36, 37), and GPRC5D (38). CD19 is an interesting target given its absence on the vast majority of plasma cells; however, CD19 may be found on myeloma progenitor cells which contribute to relapse after traditional CAR-T therapies (45, 46). Indeed, the largest experience with dual-targeting CAR-T therapy in myeloma has come from a “CAR-pool” combination of CD19-targeting and BCMA-targeting CAR-T cells. Of 62 patients who received both CAR-T therapies on a Phase 2 trial, the ORR was 92% with a median PFS of 18.3 months (40). Peak expansion of both CAR-T populations occurred at similar timeframes, but most patients did not have detectable levels of either by 12 months. Randomized trials will be needed to assess how much this combinatorial approach adds beyond single BCMA-targeting in terms of efficacy. Additionally, given the infectious risks of simultaneously depleting both B cells and plasma cells, careful attention is needed to the rates of hypogammaglobulinemia and infections with dual-targeting approaches.
Armored CAR-T cells
Building on the idea of ‘generations’ of CAR design, so-called fourth-generation therapies incorporate secreted cytokines to increase the ability of CAR-T cells to infiltrate tumors, bind BCMA, and proliferate in response to antigen recognition (15, 43). This approach, called “armoring” or occasionally as “T-cells redirected for universal cytokine killing” (TRUCK), has shown some early promise in relapsed myeloma with both in vivo and in vitro studies (47–50). Given the known inhibitory effects of transforming growth factor beta (TGF-β) on T cells, one example of an armored BCMA CAR-T cell includes a dominant negative TGF-β receptor. This modified receptor cannot mediate negative signaling from TGF-β and thus protects CAR-T cells from suppression within the tumor microenvironment. In xenograft models, these armored BCMA CAR-T cells outperformed non-armored CAR-T cells with regard to tumor infiltration and expression of pro-inflammatory cytokines (49).
Another group has tested armored BCMA CAR-T cells harboring the BCL2L1 gene encoding BCL-XL, based on the observation that BCL-XL plays an important role in T cell survival after CD28 co-stimulation (50). Compared to non-armored BCMA CAR-T cells, BCL2-armored CAR-T cells demonstrated better disease control and survival in a murine model. Taken together, these translational efforts suggest a role for armored CAR-T cells in multiple myeloma. Other immunomodulatory strategies discussed later in this review, most notably the use of checkpoint inhibition through blockade of Programmed Death 1 (PD-1) or its ligand, may be the subject of future early-phase studies of armored CAR-T cells as well.
Better mileage: Longer duration of responses
Ex vivo changes to CAR design and manufacturing
A lower bound of the Model T’s fuel economy estimates that drivers in the early 20th century could expect to achieve about 13 miles per gallon (51). Similarly, the average patient with myeloma can expect an average of 1-3 years of remission after BCMA CAR-T therapy but not necessarily any longer. The majority of patients will thus unfortunately eventually experience disease relapse, even among patients who achieved MRD negativity initially. Strategies to improve the durability of responses to BCMA CAR-T therapy thus constitute a key area of innovation which can be classified into two groups (1): ex vivo approaches focused on CAR manufacturing, and (2) in vivo approaches focused on the modulation of the immune system.
In the ex vivo category, one well-studied strategy is to decrease the immunogenicity of CAR constructs to prevent native B cells from producing antidrug antibodies (ADA) against the CAR-T cells themselves. In the KarMMa trial of ide-cel, 65% of treated patients developed ADAs within 12 months of infusion. Of 28 patients who received a second therapeutic ide-cel infusion on this protocol, responses were observed in 50% of patients without ADAs versus 0% in the presence of ADAs (6). The original Phase 1 study of LCAR-B38M (the precursor to cilta-cel) also demonstrated higher rates of relapse among patients who developed ADAs (14). As a caveat, studies of CAR-T therapies across hematologic malignancies have yielded mixed results regarding whether ADAs are always clinically relevant in terms of CAR-T efficacy (52, 53).
Regardless, several BCMA CAR-T therapies have been developed with the goal of lowering ADA development. The most prominent approach relies on humanized CAR constructs that are ostensibly less immunogenic. In contrast, the CARs of ide-cel and cilta-cel are derived from murine and camelid antibody fragments respectively (54). Fully humanized CAR constructs targeting BCMA include orvacabtagene autoleucel (JCARH125, orva-cel, no longer under development), FHVH-BCMA-T, zevorcabtagene autoleucel (CT053), CT103A, and CC- 98633 (55–61). In two phase 1 trials of CT053 (n = 24) and CT103A (n = 71) respectively, ADAs have been reported in fewer than 5% of patients (59, 60). Another promising CAR-T therapy under early investigation is CART-ddBCMA, where the BCMA-binding domain has been chosen from a synthetic protein library followed by subsequent mutagenesis and testing to minimize rates of ex vivo immunogenicity (62). However, ADA incidence rates have not yet been fully reported from the ongoing Phase 1 trial of CART-ddBCMA (63, 64).
Beyond reducing immunogenicity, other tools to promote CAR-T cell persistence warrant brief mention as well. For example, changes to how T cells are cultured may promote memory-cell phenotypes and lower T cell exhaustion (20, 47). Exposure to phosphatidylinositol-3-kinase (PI3K) inhibitors during the CAR-T manufacturing process can improve T-cell fitness and reduce T-cell exhaustion, and bb21217 incorporated PI3K inhibition during the manufacturing of bb2121 (equivalent to ide-cel) with this goal in mind (65, 66). Although bb21217 is no longer under development, a more recent publication has suggested that the transduction of regulatory T cells (Treg) with CARs predicts shorter responses to CD19-directed CAR-T therapy (67). Because PI3K inhibitors are known to suppress Treg cells (68), future research may shed additional insights on the merits of Treg manipulation. Other promising strategies during the development of BCMA CAR-T cells include the addition of IL-15 and/or IL-7, which may promote persistence by limiting ex vivo CAR-T cell differentiation prior to infusion (69, 70). Similarly, knockout of the Prdm1 gene encoding the Blimp-1 transcription factor (involved in terminal differentiation of T cells) may improve BCMA CAR-T cell efficacy as well (71).
In vivo modulation of the immune system
In vivo strategies to promote CAR-T cell persistence are administered to patients either before or after their CAR-T infusions. For example, although most CAR-T therapies use weight-based combinations of fludarabine and cyclophosphamide for lymphodepletion (LD), suboptimal pharmacokinetic fludarabine concentrations may play an important role in relapse (72). Future LD improvements seek to improve CAR-T durability either by fostering “immunological space” for CAR-T cells to expand or alternatively by preventing ADA formation (73–75). For example, one cohort of the P-BCMA-101 trial (no longer under development) received rituximab as a component of LD with the goal of lowering ADAs (76). In the UNIVERSAL trial of ALLO-715 discussed later in this review, ALLO-547 (an anti-CD52 monoclonal antibody similar to alemtuzumab) depletes native T cells and can thus theoretically create more immunologic space for incoming CAR-T cells (77, 78). Importantly, of course, ALLO-715 CAR-T cells are engineered a priori to prevent their own inactivation by ALLO-647 (77).
Unlike in autologous stem cell transplantation where the role of maintenance therapy with the immunomodulatory drug (IMiD) lenalidomide is a well-established tool to prolong responses (79), it is unknown whether planned IMiD maintenance can improve outcomes after BCMA CAR-T therapy. Table 2 summarizes existing evidence as well some of the theoretical advantages and disadvantages of IMiD maintenance following BCMA CAR-T therapy (39, 80–88). The upcoming BMT-CTN 1902 trial of ide-cel and CARTITUDE-6 trial of cilta-cel will incorporate lenalidomide maintenance in some way after CAR-T therapy, albeit not in a randomized maintenance versus no-maintenance approach (89, 90). Newer successors to IMiD drugs, for example the cereblon E3 ligase modulator (CELMoD) iberdomide, may also play a role following BCMA CAR-T therapy in the future (91).
Checkpoint inhibition may be another way to modulate the immune system after BCMA CAR-T therapy. For example, pre-clinical data suggest that PD-1 knockout may improve the effector function of cytotoxic T cells against myeloma (92). Use of the PD-1 antagonist pembrolizumab has yielded encouraging responses in certain patients with post-CAR-T relapse in lymphoma as well (93, 94). As a word of caution, however, the KEYNOTE-185 trial of lenalidomide and dexamethasone with or without pembrolizumab in newly diagnosed patients with myeloma was closed prematurely because of a worsened mortality signal in patients who received pembrolizumab (95). Furthermore, in a case series of pembrolizumab and IMiD combinations following progressive disease after BCMA CAR-T therapy (n = 5), responses to checkpoint inhibition were limited in terms of depth and duration (96).
Given the complexity of reasons by which relapse occurs after BCMA CAR-T therapy, however, post-CAR-T checkpoint inhibition may still be beneficial for certain subsets of patients. The timing of PD-1 blockade after CAR-T therapy may also be important in terms of the degree to which T-cell exhaustion is reversed (20). As such, larger trials of PD-1 blockade after BCMA CAR-T therapy with pembrolizumab or nivolumab are ongoing (clinicaltrials.gov ID NCT04205409 and NCT05191472). In addition to evaluations of efficacy, these studies will be important to quantify the risk of immune-related adverse events attributable to PD-1 blockade in this setting – for example, autoimmune colitis or dermatitis – coupled with the theoretical risk of rebound CAR-T immune-related toxicities such as CRS and ICANS.
Hassle-free delivery: Minimization of brain-to-vein time
Rapid manufacturing protocols
In recent years, many electric car manufacturers have set up online car-purchasing portals and automatic vehicle delivery services to minimize the hassles inherent to visiting in-person dealerships and arranging payment financing (97). These steps can be thought of as minimizing “brain-to-key” time intervals between first contemplating a car purchase and having new car keys in hand. In contrast, prolonged “brain-to-vein” times between CAR-T consultation and cell infusion remain a stubbornly persistent barrier to the success of BCMA CAR-T therapy. Some of the difficulties early in brain-to-vein intervals include high patient demand, limited slot availability for apheresis, and scarce inpatient beds for post-infusion toxicity monitoring (98, 99). However, the bulk of brain-to-vein intervals is comprised by “vein-to-vein” times between T-cell apheresis and CAR-T infusion, which often span 2-3 months for patients currently receiving commercial BCMA CAR-T therapies. As summarized in Table 3, several novel production strategies are under early-phase investigation to improve this process.
One strategy is aimed at harnessing in vivo CAR-T expansion in patients post-infusion rather than depending on a sufficient number of cells to be cultured ex vivo pre-infusion. PHE885 is a BCMA CAR-T therapy made using a proprietary “T-Charge” platform that, in addition to reducing manufacturing time to 2 days or less, has been shown to preserve T-cell self-renewal and maturation capabilities (100, 106). Updated Phase 1 data with PHE885 show a 93% overall response rate and median peak CAR-T expansion at 21 days, with detectable product at 6 months after infusion (100, 101). “FasT CAR,” another proprietary platform reportedly allowing for ‘overnight manufacturing,’ has been used to produce the novel CAR-T therapy GC012F targeting BCMA and CD19 (34, 107). In a Phase 1 study of GC012F, the ORR exceeded 80% at all dose levels and 75% of patients achieved MRD negativity (34). Peak CAR-T expansion occurred at 10 days, with persistent product detectable as late as 2 years after infusion.
Additionally, two recently presented Phase 1 studies – one of CC-98633/BMS-986354 using a “NEX-T” platform and a separate one of IM21 using a “High Performance” platform – both have shown manufacturing times within 1 week with ORRs exceeding 90% (61, 103). Interestingly, the NEX-T platform reportedly uses machine learning and other insights to improve upon orvacabtagene autoleucel (orva-cel), the CAR-T therapy previously mentioned in terms of possessing a fully human CAR (61, 102). Unfortunately, the technical details of these investigational proprietary platforms have not yet been published. As noted in Table 3, another caveat is that manufacturing times do not necessarily equate to vein-to-vein intervals due to added time for shipping logistics and quality control testing (not to mention hospital staffing for the patient). Nevertheless, given the multi-month wait endured by patients currently waiting for BCMA CAR-T, we eagerly await more results from these and future studies.
Homegrown CARs
Another consideration for reducing vein-to-vein times is “homegrown” CAR-T therapies, whereby cell therapies are manufactured at the point of care by the same academic centers that will themselves administer the therapy to their patients. CAR-T therapies were pioneered at academic research centers, many of which retain Good Manufacturing Practice (GMP)-compliant production facilities for active homegrown CAR-T research protocols or have acquired automated CAR-T manufacturing devices with this goal in mind (99, 108). Moving the CAR “assembly line” back to academic centers offers the promise of reducing shipping times, increasing transparency around slot availability, simplifying chain-of-custody logistics, and lowering overall production costs (108). However, because CAR-T therapies are regulated by the FDA as cellular therapies rather than autologous blood products – more precisely, as advanced therapy medicinal products rather than “HCT/P” tissue-derived products – regulatory barriers may hinder the widespread adoption of homegrown CARs beyond academic centers with robust research infrastructure (109, 110).
For centers with this infrastructure in place, though, homegrown CAR-T therapies are quite promising. As a specific example in multiple myeloma, ARI0002h is a European second-generation lentiviral BCMA-directed CAR-T therapy. Recently presented data from a Phase 1 study of ARI0002h demonstrate a 100% manufacturing success rate, a median manufacturing time of 11 days, and a median PFS that has not yet been reached with 16 months of follow-up (104). Most patients also received a protocol-planned second ARI0002h infusion 4 months after their first one, after which 29% had a deepening of their responses. Interestingly, ARI0002h is administered with a fractionated dosing schedule (10% of target CAR-T dose on Day 0, then 30% on Day +3, then the remaining 60% on Day +7). This strategy has been shown to lower CRS severity in other malignancies and may facilitate outpatient CAR-T infusions in myeloma (111, 112). Fractionated CAR-T dosing is likely more practical with homegrown CAR-T therapies without the intermediary involvement of pharmaceutical companies. However, further studies of this approach are needed to characterize its efficacy.
Off-the-shelf allogeneic products
On first pass, allogeneic CAR-T cells derived from healthy donor T cells can solve many of the challenges described in this review. Large-scale production of allogeneic CAR-T cells would bypass the individualized requirements for slot availability, adequate lymphocyte counts for leukapheresis, and ex vivo manufacturing for each treated patient. These cells could undergo most quality control testing ahead of time and be stored at myeloma treatment centers themselves, hence the moniker that they could be taken “off the shelf” of a freezer and given directly to patients. T-cell apheresis products produced from healthy donors are free of disease and have not been exposed to multiple lines of chemotherapy. In contrast, both myeloma as well as its treatments can compromise the effector function of T-cells that serve as the source of autologous CAR-T products (113–115).
ALLO-715, the allogeneic BCMA-directed CAR-T described earlier in terms of enhanced lymphodepletion, is the allogeneic BCMA-directed CAR-T therapy with the largest evidence base to date. Using proprietary TALEN gene editing, the TCR alpha constant gene is disrupted in ALLO-715 to lower the risk of graft-versus-host disease (GVHD) while the CD52 gene is disrupted to allow for lymphodepletion of native T cells with an anti-CD52 antibody similar to alemtuzumab (116). In updated data from the Phase 1 UNIVERSAL trial of ALLO-715, the “brain-to-vein” time was less than 2 weeks with a median of only 5 days between enrollment and lymphodepletion initiation (77, 105). Of 21 patients treated at the highest dose level, the ORR was 71% with a median duration of response of 8.3 months. Serious infections, a particular complication of concern given the use of anti-CD52 antibodies in lymphodepletion, occurred in 29% of patients (77, 105).
Other investigational allogeneic BCMA CAR-T therapies include PBCAR269A, in which proprietary ARCUS gene editing is used to simultaneously knock out the native TCR and ‘knock in’ the anti-BCMA CAR (117). A Phase 1/2a trial (clinicaltrials.gov ID: NCT04171843) of PBCAR269A with or without the addition of nirogacestat, a GSI discussed earlier in this review in the context of BCMA targeting, is currently under way (24–26). CTX120 is another allogeneic BCMA CAR-T therapy where the TCR is replaced by a CAR; here, however, CRISPR/Cas9 editing is used and the Major Histocompatibility Complex (MHC) class 1 gene is also disrupted to lower the risk of GVHD (118). Finally, P-BCMA-ALLO101 uses a proprietary non-viral DNA delivery system alongside Cas-CLOVER gene editing to insert the CAR while removing the native TCR and MHC class 1 molecule (119, 120). First-in-human trials of these allogeneic CAR-T therapies are ongoing or upcoming, and important future considerations include infectious risks and long-term CAR-T cell persistence. That being said, just as the original Ford Model T favored factory-generated stock vehicles over custom point-of-care models, the potential benefits of “off-the-shelf” CAR-T therapies include greater consistency, lower manufacturing costs through scaling, and likely more equitable accessibility.
Discussion
One of the many quotes attributed to Henry Ford is a quip that he reportedly made during an interview about the Model T: “If I had asked people what they wanted, they would have said faster horses.” (121). Similarly, in the early decades of myeloma treatment, many felt that the pathway to achieve sustained remissions in myeloma would involve aggressive combinations of multi-drug chemotherapy regimens and tandem autologous transplantations (122, 123). The idea of immune system modulation certainly existed in the early days of myeloma therapy, most notably exemplified by the inclusion of interferon-based maintenance in the original “Total Therapy” protocol (122). But it was only with CAR-T therapy that immunotherapy rose to its position as the mainstay of treatment for patients with relapsed or refractory multiple myeloma.
As this review demonstrates, however, BCMA CAR-T therapy still has considerable room for improvement. Strategies to make these therapies more potent in terms of BCMA targeting, more consequential in terms of response durability, and more straightforward in terms of brain-to-vein times all warrant further investigation. Given that prolonged exposure to chemotherapy and the myeloma microenvironment may impair T-cell fitness, sequencing BCMA CAR-T therapy earlier in treatment paradigms may increase not only the size of the eligible patient population but also the efficacy of the T-cells being transduced (113, 124). And while outside the scope of this review, tools to improve the safety of BCMA CAR-T therapy will be as important in the long term as strategies to improve efficacy. These include the early use of immunosuppressive therapies to abrogate CRS and ICANS (125–127), enhanced bridging to lower the rare risk of Parkinsonian toxicities (128, 129), closer attention to infections and infectious prophylaxis (130–134), and CAR design modifications to abrogate life-threatening or long-term toxicities (76, 135, 136). Strategies to facilitate outpatient infusions of BCMA CAR-T therapy and shorten hospital lengths of stay are similarly important avenues for research in the field (137, 138).
With all these approaches under active investigation, is the perfect BCMA CAR-T therapy for multiple myeloma within reach? Not yet. As summarized in Table 4, the rubric for defining a theoretically perfect BCMA CAR-T therapy has four components: efficacy, safety, practicality, and accessibility. Even if the strategies outlined in this review make BCMA CAR-T therapy curative for the average patient with multiple myeloma, their efficaciousness in patients with ultra-high-risk features such as secondary plasma cell leukemia (PCL) will need to be elucidated. Even if the immunological interventions mentioned in the paragraph above lower the incidence of life-threatening CRS or ICANS, the impact of even low-grade CAR-T toxicities on frailer patients with myeloma will need to be mitigated. Even if CAR-T therapy becomes readily available in the outpatient setting, the possibility of supply-chain shortages with viral vectors and now with fludarabine for lymphodepletion are formidable barriers for all CAR-T therapies to overcome (139). Finally, giving the disproportionate increase in multiple myeloma cases in low- and middle-income countries (140), strategies to improve global access to BCMA CAR-T therapy will be imperative to ensure patient equity to this treatment modality some day.
Like any automobile, our review has two main blind spots in terms of the broader care of patients with MM. Firstly, given our focus on CAR T-cells, we have not discussed the applicability of these strategies to BCMA-targeted bispecific antibody (bsAb) therapies. The evidence base for bsAb therapies in multiple myeloma has been reviewed elsewhere, and the BCMA-targeting bsAb teclistamab has recently gained regulatory approval in Europe and the United States (141–146). While certain considerations like lengthy vein-to-vein times are unique to autologous CAR-T therapies, other strategies discussed herein such as the addition of GSIs or PD-1 blockade may be beneficial to BCMA-targeting bsAbs as well (147–149). Similarly, some of these approaches are also relevant to products relying on genetic manipulation of the native TCR itself (150–152). Finally, some of these BCMA-specific innovations may be applicable to other types of CAR-transduced lymphocytes as well: for example, Natural Killer cells, invariant NK T cells, and gamma-delta T cells (153–156).
Our second blind spot is our focus on BCMA, which is unlikely to remain the only target for CAR T therapy in myeloma. We have already discussed a number of other potential antigens in the context of concurrent dual antigen targeting alongside BCMA (34, 35, 37–42). However, several CAR-T therapies that bypass BCMA entirely by targeting other antigens instead have been studied in myeloma as well (10, 45, 46, 157–163). As more patients are exposed to BCMA-directed therapies and develop disease relapse thereafter, particularly in the setting of BCMA allelic loss, these types of CAR-T therapies targeting non-BCMA antigens will become increasingly critical. Alternatively, a time may come where these non-BCMA cellular therapies – for example, the bsAbs talquetamab and cevostamab – are even used before BCMA-directed therapies because of evidence that emerges to suggest better and more durable responses. Such a paradigm change, if it does occur some day, will of course require years’ if not decades’ worth of robust evidence from clinical trials of different approaches to therapy sequencing.
In conclusion, while BCMA CAR-T therapies have clearly revolutionized the treatment of multiple myeloma, they are far from perfect in their current iterations. The dozens of strategies discussed in this review to improve the efficacy of these therapies can be grouped into three categories: more potent BCMA targeting, longer durations of responses, and reduced brain-to-vein times. Not all such strategies will reach the finish line, of course. As mentioned in this review, once-promising BCMA CAR-T therapies that have been shelved by their manufacturers include (at least four) candidates with initially promising rationales: AUTO2, bb21217, orva-cel, and P-BCMA-101. That being said, we hope to see many more candidate therapies succeed in coming years to make clinical responses to BCMA CAR-T therapy deeper, more durable, and more practical. While the Ford Model T and subsequent automotive innovations have allowed us to traverse any road we please, these innovations in CAR-T design will allow us to focus on a singular road of paramount importance: namely, the road toward curing multiple myeloma.
Author contributions
All authors contributed to the article and approved the submitted version.
Conflict of interest
RB: Honoraria: Clinical Care Options; Honoraria: i3 Health; Honoraria: Curio Science; Consultancy: Sanofi Pasteur; Consultancy: Guidepoint Global; Consultancy; Janssen; Consultancy; Genentech/Roche. SL: Consultancy; Abbvie: Consultancy, Research Funding; Nektar: Research Funding; Cellectar: Consultancy; Bristol Myers Squibb: Research Funding; Janssen: Consultancy, Research Funding. AC: Harpoon: Research Funding; Secura Bio: Consultancy; Sanofi Aventis: Consultancy, Research Funding; GSK: Consultancy; Abbvie: Consultancy, Research Funding; Nektar: Research Funding; Cellectar: Consultancy; Bristol Myers Squibb: Research Funding; Janssen: Consultancy, Research Funding.
Publisher’s note
All claims expressed in this article are solely those of the authors and do not necessarily represent those of their affiliated organizations, or those of the publisher, the editors and the reviewers. Any product that may be evaluated in this article, or claim that may be made by its manufacturer, is not guaranteed or endorsed by the publisher.
References
2. Foellmi R, Wuergler T, Zweimüller J. The macroeconomics of model T. J Economic Theory (2014) 153:617–47. doi: 10.1016/j.jet.2014.03.002
3. Eshhar Z, Waks T, Gross G, Schindler DG. Specific activation and targeting of cytotoxic lymphocytes through chimeric single chains consisting of antibody-binding domains and the Γ or Ζ subunits of the immunoglobulin and T-cell receptors. Proc Natl Acad Sci (1993) 90:720–4. doi: 10.1073/pnas.90.2.720
4. Brocker T, Karjalainen K. Signals through T cell receptor-Ζ chain alone are insufficient to prime resting T lymphocytes. J Exp Med (1995) 181:1653–9. doi: 10.1084/jem.181.5.1653
5. Martin T, Usmani SZ, Berdeja JG, Agha M, Cohen AD, Hari P, et al. Ciltacabtagene autoleucel, an anti–B-Cell maturation antigen chimeric antigen receptor T-cell therapy, for Relapsed/Refractory multiple myeloma: Cartitude-1 2-year follow-up. J Clin Oncol Off J Am Soc Clin Oncol (2022) Online ahead of print. doi: 10.1200/JCO.22.00842
6. Munshi NC, Anderson LD Jr., Shah N, Madduri D, Berdeja J, Lonial S, et al. Idecabtagene vicleucel in relapsed and refractory multiple myeloma. N Engl J Med (2021) 384(8):705–16. doi: 10.1056/NEJMoa2024850
7. Kourelis T, Bansal R, Patel KK, Berdeja JG, Raje NS, Alsina M, et al. Ethical challenges with car T slot allocation with idecabtagene vicleucel manufacturing access. J Clin Oncol Off J Am Soc Clin Oncol (2022) 40(16_suppl):e20021. doi: 10.1200/JCO.2022.40.16_suppl.e20021
8. Mohyuddin GR, Atieh T, Ahmed N, Sborov D, McClune B, Abdallah AO, et al. Intention to treat versus modified intention-to-Treat analysis in b-cell maturation antigen and Cd19 chimeric antigen receptor trials: A systematic review and meta-analysis. Eur J Cancer (2021) 156:164–74. doi: 10.1016/j.ejca.2021.07.036
9. Green DJ, Pont M, Sather BD, Cowan AJ, Turtle CJ, Till BG, et al. Fully human bcma targeted chimeric antigen receptor T cells administered in a defined composition demonstrate potency at low doses in advanced stage high risk multiple myeloma. Blood (2018) 132(Supplement 1):1011. doi: 10.1182/blood-2018-99-117729
10. O'Neal J, Ritchey JK, Cooper ML, Niswonger J, Sofia Gonzalez L, Street E, et al. Cs1 car-T targeting the distal domain of Cs1 (Slamf7) shows efficacy in high tumor burden myeloma model despite fratricide of Cd8+Cs1 expressing car-T cells. Leukemia (2022) 36(6):1625–34. doi: 10.1038/s41375-022-01559-4
11. Katsarou A, Sjostrand M, Naik J, Mansilla-Soto J, Kefala D, Kladis G, et al. Combining a car and a chimeric costimulatory receptor enhances T cell sensitivity to low antigen density and promotes persistence. Sci Transl Med (2021) 13(623):eabh1962. doi: 10.1126/scitranslmed.abh1962
12. Zhao WH, Wang BY, Chen LJ, Fu WJ, Xu J, Liu J, et al. Four-year follow-up of lcar-B38m in relapsed or refractory multiple myeloma: A phase 1, single-arm, open-label, multicenter study in China (Legend-2). J Hematol Oncol (2022) 15(1):86. doi: 10.1186/s13045-022-01301-8
13. Berdeja JG, Madduri D, Usmani SZ, Jakubowiak A, Agha M, Cohen AD, et al. Ciltacabtagene autoleucel, a b-cell maturation antigen-directed chimeric antigen receptor T-cell therapy in patients with relapsed or refractory multiple myeloma (Cartitude-1): A phase 1b/2 open-label study. Lancet (2021) 398(10297):314–24. doi: 10.1016/s0140-6736(21)00933-8
14. Xu J, Chen LJ, Yang SS, Sun Y, Wu W, Liu YF, et al. Exploratory trial of a biepitopic car T-targeting b cell maturation antigen in Relapsed/Refractory multiple myeloma. Proc Natl Acad Sci U.S.A. (2019) 116(19):9543–51. doi: 10.1073/pnas.1819745116
15. Hanssens H, Meeus F, De Veirman K, Breckpot K, Devoogdt N. The antigen-binding moiety in the driver's seat of cars. Med Res Rev (2022) 42(1):306–42. doi: 10.1002/med.21818
16. Rodriguez-Marquez P, Calleja-Cervantes ME, Serrano G, Oliver-Caldes A, Palacios-Berraquero ML, Martin-Mallo A, et al. Car density influences antitumoral efficacy of bcma car T cells and correlates with clinical outcome. Sci Adv (2022) 8(39):eabo0514. doi: 10.1126/sciadv.abo0514
17. Rad SMA, Poudel A, Tan GMY, McLellan AD. Promoter choice: Who should drive the car in T cells? PLoS One (2020) 15(7):e0232915. doi: 10.1371/journal.pone.0232915
18. Ho JY, Wang L, Liu Y, Ba M, Yang J, Zhang X, et al. Promoter usage regulating the surface density of car molecules may modulate the kinetics of car-T cells in vivo. Mol Ther Methods Clin Dev (2021) 21:237–46. doi: 10.1016/j.omtm.2021.03.007
19. Eyquem J, Mansilla-Soto J, Giavridis T, van der Stegen SJ, Hamieh M, Cunanan KM, et al. Targeting a car to the trac locus with Crispr/Cas9 enhances tumour rejection. Nature (2017) 543(7643):113–7. doi: 10.1038/nature21405
20. Chow A, Perica K, Klebanoff CA, Wolchok JD. Clinical implications of T cell exhaustion for cancer immunotherapy. Nat Rev Clin Oncol (2022) Online ahead of print. doi: 10.1038/s41571-022-00689-z
21. Olson ML, Mause ERV, Radhakrishnan SV, Brody JD, Rapoport AP, Welm AL, et al. Low-affinity car T cells exhibit reduced trogocytosis, preventing rapid antigen loss, and increasing car T cell expansion. Leukemia (2022) 36(7):1943–6. doi: 10.1038/s41375-022-01585-2
22. Camviel N, Wolf B, Croce G, Gfeller D, Zoete V, Arber C. Both April and antibody-Fragment-Based car T cells for myeloma induce bcma downmodulation by trogocytosis and internalization. J Immunother Cancer (2022) 10(11):e005091. doi: 10.1136/jitc-2022-005091
23. Laurent SA, Hoffmann FS, Kuhn PH, Cheng Q, Chu Y, Schmidt-Supprian M, et al. Gamma-secretase directly sheds the survival receptor bcma from plasma cells. Nat Commun (2015) 6:7333. doi: 10.1038/ncomms8333
24. Green DJ, Pont M, Cowan AJ, Cole GO, Sather BD, Nagengast AM, et al. Response to bcma car-T cells correlates with pretreatment target antigen density and is improved by small molecule inhibition of gamma secretase. Blood (2019) 134(Supplement_1):1856. doi: 10.1182/blood-2019-129582
25. Pont MJ, Hill T, Cole GO, Abbott JJ, Kelliher J, Salter AI, et al. G-Secretase inhibition increases efficacy of bcma-specific chimeric antigen receptor T cells in multiple myeloma. Blood (2019) 134(19):1585–97. doi: 10.1182/blood.2019000050
26. Cowan AJ, Pont MJ, Sather BD, Turtle CJ, Till BG, Nagengast AM, et al. Efficacy and safety of fully human bcma car T cells in combination with a gamma secretase inhibitor to increase bcma surface expression in patients with relapsed or refractory multiple myeloma. Blood (2019) 134(Supplement_1):204. doi: 10.1182/blood-2019-129405
27. Cowan AJ, Pont M, Sather BD, Turtle CJ, Till BG, Libby E, et al. Safety and efficacy of fully human bcma car T cells in combination with a gamma secretase inhibitor to increase bcma surface expression in patients with relapsed or refractory multiple myeloma. Blood (2021) 138(Supplement 1):551. doi: 10.1182/blood-2021-154170
28. Li D, Que Y, Ding S, Hu G, Wang W, Mao X, et al. Anti-bcma car-T cells therapy for a patient with extremely high membrane bcma expression: A case report. J Immunother Cancer (2022) 10(9):e005403. doi: 10.1136/jitc-2022-005403
29. Samur MK, Fulciniti M, Aktas Samur A, Bazarbachi AH, Tai YT, Prabhala R, et al. Biallelic loss of bcma as a resistance mechanism to car T cell therapy in a patient with multiple myeloma. Nat Commun (2021) 12(1):868. doi: 10.1038/s41467-021-21177-5
30. Da Via MC, Dietrich O, Truger M, Arampatzi P, Duell J, Heidemeier A, et al. Homozygous bcma gene deletion in response to anti-bcma car T cells in a patient with multiple myeloma. Nat Med (2021) 27(4):616–9. doi: 10.1038/s41591-021-01245-5
31. Lee L, Draper B, Chaplin N, Philip B, Chin M, Galas-Filipowicz D, et al. An April-based chimeric antigen receptor for dual targeting of bcma and taci in multiple myeloma. Blood (2018) 131(7):746–58. doi: 10.1182/blood-2017-05-781351
32. Schmidts A, Ormhoj M, Choi BD, Taylor AO, Bouffard AA, Scarfo I, et al. Rational design of a trimeric April-based car-binding domain enables efficient targeting of multiple myeloma. Blood Adv (2019) 3(21):3248–60. doi: 10.1182/bloodadvances.2019000703
33. Popat R, Zweegman S, Cavet J, Yong K, Lee L, Faulkner J, et al. Phase 1 first-in-Human study of Auto2, the first chimeric antigen receptor (Car) T cell targeting April for patients with Relapsed/Refractory multiple myeloma (Rrmm). Blood (2019) 134(Supplement_1):3112. doi: 10.1182/blood-2019-126689
34. Du J, Juang H, Dong B, Gao L, Liu L, Ge J, et al. Updated results of a multicenter first-in-Human study of Bcma/Cd19 dual-targeting fast car-T Gc012f for patients with Relapsed/Refractory multiple myeloma (Rrmm). J Clin Oncol Off J Am Soc Clin Oncol (2022) 40(16_suppl):8005. doi: 10.1200/JCO.2022.40.16_suppl.8005
35. Mei H, Li C, Jiang H, Zhao X, Huang Z, Jin D, et al. A bispecific car-T cell therapy targeting bcma and Cd38 in relapsed or refractory multiple myeloma. J Hematol Oncol (2021) 14(1):161. doi: 10.1186/s13045-021-01170-7
36. Li C, Wang X, Wu Z, Luo W, Zhang Y, Kang Y, et al. Abstract 1998: Bispecific CS1-BCMA CAR-T cells are clinically active in relapsed or refractory multiple myeloma: An updated clinical study. Presented at: Annual Meeting, American Society of Hematology; New Orleans, LA, USA (2022).
37. Zah E, Nam E, Bhuvan V, Tran U, Ji BY, Gosliner SB, et al. Systematically optimized Bcma/Cs1 bispecific car-T cells robustly control heterogeneous multiple myeloma. Nat Commun (2020) 11(1):2283. doi: 10.1038/s41467-020-16160-5
38. de Larrea CF, Staehr M, Lopez AV, Ng KY, Chen Y, Godfrey WD, et al. Defining an optimal dual-targeted car T-cell therapy approach simultaneously targeting bcma and Gprc5d to prevent bcma escape-driven relapse in multiple myeloma. Blood Cancer Discovery (2020) 1(2):146–54. doi: 10.1158/2643-3230.bcd-20-0020
39. Shi X, Yan L, Shang J, Kang L, Yan Z, Jin S, et al. Anti-Cd19 and anti-bcma car T cell therapy followed by lenalidomide maintenance after autologous stem-cell transplantation for high-risk newly diagnosed multiple myeloma. Am J Hematol (2022) 97(5):537–47. doi: 10.1002/ajh.26486
40. Wang Y, Cao J, Gu W, Shi M, Lan J, Yan Z, et al. Long-term follow-up of combination of b-cell maturation antigen and Cd19 chimeric antigen receptor T cells in multiple myeloma. J Clin Oncol Off J Am Soc Clin Oncol (2022) 40:2246–56. doi: 10.1200/JCO.21
41. Yan L, Qu S, Shang J, Shi X, Kang L, Xu N, et al. Sequential Cd19 and bcma-specific car T-cell treatment elicits sustained remission of relapsed and/or refractory myeloma. Cancer Med (2020) 10(2):563–74. doi: 10.1002/cam4.3624
42. Yan Z, Cao J, Cheng H, Qiao J, Zhang H, Wang Y, et al. A combination of humanised anti-Cd19 and anti- bcma car T cells in patients with relapsed or refractory multiple myeloma: A single-arm, phase 2 trial. Lancet Haematol (2019) 6(10):e521–e9. doi: 10.1016/S2352-3026(19)30115-2
43. Bruno B, Wasch R, Engelhardt M, Gay F, Giaccone L, D'Agostino M, et al. European Myeloma network perspective on car T-cell therapies for multiple myeloma. Haematologica (2021) 106(8):2054–65. doi: 10.3324/haematol.2020.276402
44. van der Schans JJ, van de Donk N, Mutis T. Dual targeting to overcome current challenges in multiple myeloma car T-cell treatment. Front Oncol (2020) 10:1362. doi: 10.3389/fonc.2020.01362
45. Garfall AL, Maus MV, Hwang WT, Lacey SF, Mahnke YD, Melenhorst JJ, et al. Chimeric antigen receptor T cells against Cd19 for multiple myeloma. N Engl J Med (2015) 373(11):1040–7. doi: 10.1056/NEJMoa1504542
46. Garfall AL, Stadtmauer EA, Hwang WT, Lacey SF, Melenhorst JJ, Krevvata M, et al. Anti-Cd19 car T cells with high-dose melphalan and autologous stem cell transplantation for refractory multiple myeloma. JCI Insight (2018) 3(8):e120505. doi: 10.1172/jci.insight.120505
47. Lopez-Cantillo G, Uruena C, Camacho BA, Ramirez-Segura C. Car-T cell performance: How to improve their persistence? Front Immunol (2022) 13:878209. doi: 10.3389/fimmu.2022.878209
48. Yeku OO, Brentjens RJ. Armored car T-cells: Utilizing cytokines and pro-inflammatory ligands to enhance car T-cell anti-tumour efficacy. Biochem Soc Trans (2016) 44(2):412–8. doi: 10.1042/BST20150291
49. Alabanza LM, Xiong Y, Vu B, Webster B, Wu D, Hu P, et al. Armored bcma car T cells eliminate multiple myeloma and are resistant to the suppressive effects of tgf-beta. Front Immunol (2022) 13:832645. doi: 10.3389/fimmu.2022.832645
50. Maity R, Benaoudia S, Zemp F, Lee H, Barakat E, Leblay N, et al. A Bcl2l1 armoured bcma targeting car T cell to overcome exhaustion and enhance persistence in multiple myeloma. Blood (2021) 138(Supplement 1):327. doi: 10.1182/blood-2021-153871
51. National Public Radio, Montagne R. National public radio. model T: ‘Universal car’ sparked gasoline demand (2008). Available at: https://www.npr.org/2008/07/07/92216092/model-t-universal-car-sparked-gasoline-demand.
52. Khan AN, Chowdhury A, Karulkar A, Jaiswal AK, Banik A, Asija S, et al. Immunogenicity of car-T cell therapeutics: Evidence, mechanism and mitigation. Front Immunol (2022) 13:886546. doi: 10.3389/fimmu.2022.886546
53. Wagner DL, Fritsche E, Pulsipher MA, Ahmed N, Hamieh M, Hegde M, et al. Immunogenicity of car T cells in cancer therapy. Nat Rev Clin Oncol (2021) 18(6):379–93. doi: 10.1038/s41571-021-00476-2
54. Roex G, Timmers M, Wouters K, Campillo-Davo D, Flumens D, Schroyens W, et al. Safety and clinical efficacy of bcma car-T-Cell therapy in multiple myeloma. J Hematol Oncol (2020) 13(1):164. doi: 10.1186/s13045-020-01001-1
55. Mailankody S, Jakubowiak AJ, Htut M, Costa LJ, Lee K, Ganguly S, et al. Orvacabtagene autoleucel (Orva-cel), a b-cell maturation antigen (Bcma)-directed car T cell therapy for patients (Pts) with Relapsed/Refractory multiple myeloma (Rrmm): Update of the phase 1/2 evolve study (Nct03430011). J Clin Oncol (2020) 38(15_suppl):8504. doi: 10.1200/JCO.2020.38.15_suppl.8504
56. Mikkilineni L, Manasanch EE, Vanasse D, Brudno JN, Mann J, Sherry R, et al. Deep and durable remissions of relapsed multiple myeloma on a first-in-Humans clinical trial of T cells expressing an anti-B-Cell maturation antigen (Bcma) chimeric antigen receptor (Car) with a fully-human heavy-Chain-Only antigen recognition domain. Blood (2020) 136(Supplement 1):50–1. doi: 10.1182/blood-2020-138839
57. Wang D, Wang J, Hu G, Wang W, Xiao Y, Cai H, et al. A phase 1 study of a novel fully human bcma-targeting car (Ct103a) in patients with Relapsed/Refractory multiple myeloma. Blood (2021) 137(21):2890–901. doi: 10.1182/blood.2020008936
58. Chen W, Fu C, Cai Z, Li Z, Wang H, Yan L, et al. Sustainable efficacy and safety results from lummicar study 1: A phase 1/2 study of fully human b-cell maturation antigen-specific car T cells (Ct053) in Chinese subjects with relapsed and/or refractory multiple myeloma. Blood (2021) 138(Supplement 1):2821. doi: 10.1182/blood-2021-150124
59. Yang M, Zhang W, Yu K, Wang P, Jiang H, Chen L, et al. A novel bcma car-T-Cell therapy with optimized human scfv for treatment of Relapsed/Refractory multiple myeloma: Results from phase I clinical trials. Haematologica (2022) 107(8):1960–5. doi: 10.3324/haematol.2022.280629
60. Li C, Wang D, Song Y, Li J, Huang H, Chen B, et al. A phase 1/2 study of a novel fully human b-cell maturation antigen-specific car T cells (Ct103a) in patients with relapsed and/or refractory multiple myeloma. Blood (2021) 138(Supplement 1):547. doi: 10.1182/blood-2021-152576
61. Costa LJ, Kumar SK, Atrash S, Liedtke M, Kaur G, Derman BA, et al. Abstract 566: Results from the first phase 1 clinical study of the b-cell maturation antigen (BCMA) Nex T chimeric antigen receptor (CAR) T cell therapy CC-98633/BMS-986354 in patients (pts) with Relapsed/Refractory multiple myeloma (RRMM). Presented at: Annual Meeting, American Society of Hematology; New Orleans, LA, USA (2022).
62. Buonato JM, Edwards JP, Zaritskaya L, Witter AR, Gupta A, LaFleur DW, et al. Preclinical efficacy of bcma-directed car T cells incorporating a novel d domain antigen recognition domain. Mol Cancer Ther (2022) 21(7):1171–83. doi: 10.1158/1535-7163.MCT-21-0552
63. Frigault MJ, Bishop MM, Rosenblatt J, O’Donnell E, Raje N, Cook DJ, et al. Phase 1 study of cart-ddbcma for the treatment of subjects with relapsed and refractory multiple myeloma. Blood Adv (2022) Online ahead of print. doi: 10.1182/bloodadvances.2022007210
64. Frigault MJ, Bishop MR, O'Donnell EK, Raje NS, Mondillo A, Cook D, et al. Phase 1 study of cart-ddbcma, a car-T therapy utilizing a novel synthetic binding domain for the treatment of subjects with relapsed and refractory multiple myeloma. Blood (2020) 136(Supplement 1):2. doi: 10.1182/blood-2020-142931
65. Funk CR, Wang S, Chen KZ, Waller A, Sharma A, Edgar CL, et al. Pi3kdelta/Gamma inhibition promotes human cart cell epigenetic and metabolic reprogramming to enhance antitumor cytotoxicity. Blood (2022) 139(4):523–37. doi: 10.1182/blood.2021011597
66. Alsina M, Shah N, Raje NS, Jagannath S, Madduri D, Kaufman JL, et al. Updated results from the phase I crb-402 study of anti-bcma car-T cell therapy Bb21217 in patients with relapsed and refractory multiple myeloma: Correlation of expansion and duration of response with T cell phenotypes. Blood (2020) 136(Supplement 1):25–6. doi: 10.1182/blood-2020-140410
67. Good Z, Spiegel JY, Sahaf B, Malipatlolla MB, Ehlinger ZJ, Kurra S, et al. Post-infusion car treg cells identify patients resistant to Cd19-car therapy. Nat Med (2022) 28(9):1860–71. doi: 10.1038/s41591-022-01960-7
68. Chellappa S, Kushekhar K, Munthe LA, Tjonnfjord GE, Aandahl EM, Okkenhaug K, et al. The Pi3k P110delta isoform inhibitor idelalisib preferentially inhibits human regulatory T cell function. J Immunol (2019) 202(5):1397–405. doi: 10.4049/jimmunol.1701703
69. Battram AM, Bachiller M, Lopez V, Fernandez de Larrea C, Urbano-Ispizua A, Martin-Antonio B. Il-15 enhances the persistence and function of bcma-targeting CAR-T cells compared to IL-2 or IL-15/IL-7 by limiting CAR-T cell dysfunction and differentiation. Cancers (Basel) (2021) 13(14):3534. doi: 10.3390/cancers13143534
70. Joedicke JJ, Grosskinsky U, Gerlach K, Kunkele A, Hopken UE, Rehm A. Accelerating clinical-scale production of bcma car T cells with defined maturation stages. Mol Ther Methods Clin Dev (2022) 24:181–98. doi: 10.1016/j.omtm.2021.12.005
71. Battram AM, Oliver-Caldes A, Crespo MB, Cardus O, Moreno DF, Rodriguez-Lobato LG, et al. Abstract 976: Genetic disruption of Blimp-1 drastically augments the persistence and anti-tumour efficacy of BCMA-targeting CAR-T cells. Presented at: Annual Meeting, American Society of Hematology; New Orleans, LA, USA. (2022).
72. Fabrizio VA, Boelens JJ, Mauguen A, Baggott C, Prabhu S, Egeler E, et al. Optimal fludarabine lymphodepletion is associated with improved outcomes after car T-cell therapy. Blood Adv (2022) 6(7):1961–8. doi: 10.1182/bloodadvances.2021006418
73. Muranski P, Boni A, Wrzesinski C, Citrin DE, Rosenberg SA, Childs R, et al. Increased intensity lymphodepletion and adoptive immunotherapy–how far can we go? Nat Clin Pract Oncol (2006) 3(12):668–81. doi: 10.1038/ncponc0666
74. Gattinoni L, Finkelstein SE, Klebanoff CA, Antony PA, Palmer DC, Spiess PJ, et al. Removal of homeostatic cytokine sinks by lymphodepletion enhances the efficacy of adoptively transferred tumor-specific Cd8+ T cells. J Exp Med (2005) 202(7):907–12. doi: 10.1084/jem.20050732
75. Davda J, Declerck P, Hu-Lieskovan S, Hickling TP, Jacobs IA, Chou J, et al. Immunogenicity of immunomodulatory, antibody-based, oncology therapeutics. J Immunother Cancer (2019) 7(1):105. doi: 10.1186/s40425-019-0586-0
76. Costello CL, Cohen AD, Patel KK, Ali SS, Berdeja JG, Shah N, et al. Phase 1/2 study of the safety and response of p-Bcma-101 car-T cells in patients with Relapsed/Refractory (R/R) multiple myeloma (Mm) (Prime) with novel therapeutic strategies. Blood (2020) 136(Supplement 1):29–30. doi: 10.1182/blood-2020-142695
77. Mailankody S, Liedtke M, Sidana S, Matous JV, Chhabra S, Oluwole OO, et al. Universal updated phase 1 data validates the feasibility of allogeneic anti-bcma allo-715 therapy for Relapsed/Refractory multiple myeloma. Blood (2021) 138(Supplement 1):651. doi: 10.1182/blood-2021-145572
78. Tees MT, Neelapu SS, Hari P, Mailankody S, Miklos DB, Locke FL, et al. Safety and Pk/Pd of allo-647, an anti-Cd52 antibody, with fludarabine (Flu)/Cyclophosphamide (Cy) for lymphodepletion in the setting of allogeneic car-T cell therapy. J Clin Oncol (2021) 39(15_suppl):2527. doi: 10.1200/JCO.2021.39.15_suppl.2527
79. McCarthy PL, Holstein SA, Petrucci MT, Richardson PG, Hulin C, Tosi P, et al. Lenalidomide maintenance after autologous stem-cell transplantation in newly diagnosed multiple myeloma: A meta-analysis. J Clin Oncol Off J Am Soc Clin Oncol (2017) 35(29):3279–89. doi: 10.1200/JCO.10.1200/JCO.2017
80. Works M, Soni N, Hauskins C, Sierra C, Baturevych A, Jones JA. Lenalidomide enhances anti-bcma chimeric antigen receptor T cell function against multiple myeloma. Blood (2017) 130(Suppl_1):1794. doi: 10.1182/blood.V130.Suppl_1.1794.1794
81. Wang X, Walter M, Urak R, Weng L, Huynh C, Lim L, et al. Lenalidomide enhances the function of Cs1 chimeric antigen receptor-redirected T cells against multiple myeloma. Clin Cancer Res (2018) 24(1):106–19. doi: 10.1158/1078-0432.CCR-17-0344
82. Ping N, Qu C, Li M, Kang L, Kong D, Chen X, et al. Overall survival benefits provided by lenalidomide maintenance after chimeric antigen receptor T cell therapy in patients with Refractory/Relapsed diffuse Large b-cell lymphoma. Ann Transl Med (2022) 10(6):298. doi: 10.21037/atm-22-20
83. Reyes K, Liu Y, Huang C, Banerjee R, Martin T, Shah N. Salvage therapies and clinical outcomes after relapse following bcma car-T in patients with rrmm. Los Angeles, CA: International Myeloma Society (2022).
84. Hashmi H, Mirza A-S, Darwin A, Logothetis C, Garcia F, Kommalapati A, et al. Venous thromboembolism associated with Cd19-directed car T-cell therapy in Large b-cell lymphoma. Blood Adv (2020) 4(17):4086–90. doi: 10.1182/bloodadvances.2020002060
85. Jain T, Knezevic A, Pennisi M, Chen Y, Ruiz JD, Purdon TJ, et al. Hematopoietic recovery in patients receiving chimeric antigen receptor T-cell therapy for hematologic malignancies. Blood Adv (2020) 4(15):3776–87. doi: 10.1182/bloodadvances.2020002509
86. Gill S, Brudno JN. Car T-cell therapy in hematologic malignancies: Clinical role, toxicity, and unanswered questions. Am Soc Clin Oncol Educ Book (2021) 41:1–20. doi: 10.1200/EDBK_
87. Jones JR, Cairns DA, Gregory WM, Collett C, Pawlyn C, Sigsworth R, et al. Second malignancies in the context of lenalidomide treatment: An analysis of 2732 myeloma patients enrolled to the myeloma xi trial. Blood Cancer J (2016) 6(12):e506. doi: 10.1038/bcj.2016.114
88. Zhao G, Wei R, Feng L, Wu Y, He F, Xiao M, et al. Lenalidomide enhances the efficacy of anti-bcma car-T treatment in Relapsed/Refractory multiple myeloma: A case report and revies of the literature. Cancer Immunol Immunother (2022) 71(1):39–44. doi: 10.1007/s00262-021-02959-8
89. Blood & Marrow Transplant Clinical Trials Network. Bmt ctn 1902 / mm car-T to upgrade response - phase ii multicenter trial of anti-b cell maturation antigen chimeric antigen receptor T cell therapy for multiple myeloma patients with Sub-optimal response after autologous hematopoietic cell transplantation and maintenance lenalidomide . Available at: https://web.emmes.com/study/bmt2/protocol/1902_protocol/1902_protocol.html.
90. A study of daratumumab, bortezomib, lenalidomide and dexamethasone (Dvrd) followed by ciltacabtagene autoleucel versus daratumumab, bortezomib, lenalidomide and dexamethasone (Dvrd) followed by autologous stem cell transplant (Asct) in participants with newly diagnosed multiple myeloma (Cartitude-6) . Available at: https://www.clinicaltrials.gov/ct2/show/NCT05257083.
91. Soni N, Baturevych A, Works M, Qin J, Balakrishnan A, Ports M. Iberdomide Increases the Potency of the Anti-BCMA CAR T Cell Product Orvacabtagene Autoleucel (Orva-Cel). Presented at: Annual meeting, American Society of Gene & Cell Therapy (ASGCT); New York, NY, USA (2020).
92. Zhao Z, Shi L, Zhang W, Han J, Zhang S, Fu Z, et al. Crispr knock out of programmed cell death protein 1 enhances anti-tumor activity of cytotoxic T lymphocytes. Oncotarget (2018) 9(4):5208–15. doi: 10.18632/oncotarget.23730
93. Chong E, Alanio C, Svoboda J, Nasta SD, Landsburg DJ, Lacey SF, et al. Pembrolizumab for b-cell lymphomas relapsing after or refractory to Cd19-directed car T-cell therapy. Blood (2022) 139(7):1026–38. doi: 10.1182/blood.2021012634
94. Hill BT, Roberts ZJ, Xue A, Rossi JM, Smith MR. Rapid tumor regression from pd-1 inhibition after anti-Cd19 chimeric antigen receptor T-cell therapy in refractory diffuse Large b-cell lymphoma. Bone marrow Transplant (2020) 55(6):1184–7. doi: 10.1038/s41409-019-0657-3
95. Usmani SZ, Schjesvold F, Oriol A, Karlin L, Cavo M, Rifkin RM, et al. Pembrolizumab plus lenalidomide and dexamethasone for patients with treatment-naive multiple myeloma (Keynote-185): A randomised, open-label, phase 3 trial. Lancet Haematology (2019) 6(9):e448–e58. doi: 10.1016/s2352-3026(19)30109-7
96. Bernabei L, Garfall AL, Melenhorst JJ, Lacey SF, Stadtmauer EA, Vogl DT, et al. Pd-1 inhibitor combinations as salvage therapy for Relapsed/Refractory multiple myeloma (Mm) patients progressing after bcma-directed car T cells. Blood (2018) 132(Supplement 1):1973. doi: 10.1182/blood-2018-99-119514
97. Stenquist P. Why you might buy your next car online. Published 2022 Jun 21. New York, NY: New York Times. Available at: https://www.nytimes.com/2022/06/21/business/tesla-online-sales-dealerships.html.
98. Mikhael J, Fowler J, Shah N. Chimeric antigen receptor T-cell therapies: Barriers and solutions to access. JCO Oncol Pract (2022) Online ahead of print. doi: 10.1200/OP.22.00315.
99. Gajra A, Zalenski A, Sannareddy A, Jeune-Smith Y, Kapinos K, Kansagra A. Barriers to chimeric antigen receptor T-cell (Car-T) therapies in clinical practice. Pharmaceut Med (2022) 36(3):163–71. doi: 10.1007/s40290-022-00428-w
100. Sperling AS, Nikiforow S, Nadeem O, Mo CC, Laubach JP, Anderson KC, et al. Phase I study of Phe885, a fully human bcma-directed car-T cell therapy for Relapsed/Refractory multiple myeloma manufactured in <2 days using the T-charge Tm platform. Blood (2021) 138(Supplement 1):3864. doi: 10.1182/blood-2021-146646
101. Munshi N, Spencer A, Raab MS, Masood A, Martinez-Prieto M, Chu J, et al. Pb1983 trial-in-Progress: Phase ii study of Phe885, a b-cell maturation antigen-directed chimeric antigen receptor T-cell therapy, in adults with relapsed and/or refractory multiple myeloma. Hemasphere (2022) 6(EHA Supplement):S3. doi: 10.1097/01.HS9.0000850768.54900.41
102. Stanton D. Nex-T up: Bristol-Myers using machine learning to optimize future car-T (2020). Available at: https://bioprocessintl.com/bioprocess-insider/therapeutic-class/nex-t-up-bristol-myers-using-machine-learning-to-optimize-future-car-t/.
103. Liu H, Wang W, Yang Y, Feng R, Li J, Zhang C, et al. Abstract 4630: Phase I study of a BCMA-directed CAR-T cell therapy for Relapsed/Refractory multiple myeloma manufactured in <3 days using the high-performance platform. Presented at: Annual Meeting, American Society of Hematology; New Orleans, LA, USA (2022).
104. de Larrea CF, Gonzalez-Calle V, Oliver-Caldes A, Cabañas V, Rodriguez-Otero P, Espanol-Rego M, et al. S103 efficacy and safety of Ari0002h, an academic bcma-directed car-T cell therapy with fractionated initial therapy and booster dose in patients with Relapsed/Refractory multiple myeloma. Hemasphere (2022) 6(Suppl):S3. doi: 10.1097/01.HS9.0000843308.71619.a0
105. Mailankody S, Matous JV, Liedtke M, Sidana S, Oluwole O, Mohan M, et al. Abstract 2019: Universal updated phase 1 data highlights role of allogeneic anti-BCMA ALLO-715 therapy for Relapsed/Refractory multiple myeloma. Presented at: Annual Meeting, American Society of Hematology; New Orleans, LA, USA (2022).
106. Bu D, Bennett P, Barton N, Bradshaw L, Pinon-Ortiz M, Li X, et al. Identification and development of Phe885: A novel and highly potent fully human anti-bcma car-T manufactured with a novel T-charge Tm platform for the treatment of multiple myeloma. Blood (2021) 138(Supplement 1):2770. doi: 10.1182/blood-2021-148390
107. Zhang H, Gao L, Liu L, Wang J, Wang S, Gao L, et al. A bcma and Cd19 bispecific car-T for relapsed and refractory multiple myeloma. Blood (2019) 134(Supplement_1):3147. doi: 10.1182/blood-2019-131056
108. Saini KS, Svane IM, Juan M, Barlesi F, Andre F. Manufacture of adoptive cell therapies at academic cancer centers: Scientific, safety and regulatory challenges. Ann Oncol Off J Eur Soc Med Oncol / ESMO (2022) 33(1):6–12. doi: 10.1016/j.annonc.2021.09.020
109. Iglesias-Lopez C, Agusti A, Obach M, Vallano A. Regulatory framework for advanced therapy medicinal products in Europe and united states. Front Pharmacol (2019) 10:921. doi: 10.3389/fphar.2019.00921
110. Mitchell D, Kenderian SS, Rajkumar SV. Letting academic medical centers make car-T drugs would save billions . Available at: https://www.statnews.com/2019/11/20/car-t-drugs-academic-medical-centers-save-billions/.
111. Frey NV, Shaw PA, Hexner EO, Pequignot E, Gill S, Luger SM, et al. Optimizing chimeric antigen receptor T-cell therapy for adults with acute lymphoblastic leukemia. J Clin Oncol Off J Am Soc Clin Oncol (2019) 38:415–22. doi: 10.1200/JCO.19
112. Cohen AD, Garfall AL, Stadtmauer EA, Melenhorst JJ, Lacey SF, Lancaster E, et al. B cell maturation antigen-specific car T cells are clinically active in multiple myeloma. J Clin Invest (2019) 129(6):2210–21. doi: 10.1172/JCI126397
113. Garfall AL, Dancy EK, Cohen AD, Hwang WT, Fraietta JA, Davis MM, et al. T-Cell phenotypes associated with effective car T-cell therapy in postinduction vs relapsed multiple myeloma. Blood Adv (2019) 3(19):2812–5. doi: 10.1182/bloodadvances.2019000600
114. D'Souza C, Prince HM, Neeson PJ. Understanding the role of T-cells in the antimyeloma effect of immunomodulatory drugs. Front Immunol (2021) 12:632399. doi: 10.3389/fimmu.2021.632399
115. Cohen AD, Raje N, Fowler JA, Mezzi K, Scott EC, Dhodapkar MV. How to train your T cells: Overcoming immune dysfunction in multiple myeloma. Clin Cancer Res (2020) 26(7):1541–54. doi: 10.1158/1078-0432.CCR-19-2111
116. Sommer C, Boldajipour B, Kuo TC, Bentley T, Sutton J, Chen A, et al. Preclinical evaluation of allogeneic car T cells targeting bcma for the treatment of multiple myeloma. Mol Ther (2019) 27(6):1126–38. doi: 10.1016/j.ymthe.2019.04.001
117. Mohammed T, Mailankody S. "Off-the-Shelf" immunotherapies for multiple myeloma. Semin Oncol (2022) 49(1):60–8. doi: 10.1053/j.seminoncol.2022.01.001
118. Dar H, Henderson D, Padalia Z, Porras A, Mu D, Kyungah M, et al. Preclinical development of Ctx120, an allogeneic car-T cell targeting bcma. Blood (2018) 132(Supplement 1):1921. doi: 10.1182/blood-2018-99-116443
119. Tseng H, Zhang Y, Cranert SA, Richter M, Marquez K, Qiu J, et al. Memory phenotype in allogeneic anti-bcma car-T cell therapy (P-Bcma-Allo1) correlates with in vivo tumor control. Blood (2021) 138(Supplement 1):4802. doi: 10.1182/blood-2021-154034
120. Madison BB, Patil D, Richter M, Li X, Tong M, Cranert S, et al. Cas-clover is a novel high-fidelity nuclease for safe and robust generation of tscm-enriched allogeneic car-T cells. Mol Ther Nucleic Acids (2022) 29:979–95. doi: 10.1016/j.omtn.2022.06.003
121. Basiri A. How fast can our horses go? measuring the quality of positioning technologies. J Navigation (2021) 74(1):1–4. doi: 10.1017/s0373463320000673
122. Barlogie B, Jagannath S, Desikan KR, Mattox S, Vesole D, Siegel D, et al. Total therapy with tandem transplants for newly diagnosed multiple myeloma. Blood (1999) 93(1):55–65. doi: 10.1182/blood.V93.1.55
123. Alexanian R, Dimopoulos M. The treatment of multiple myeloma. N Engl J Med (1994) 330:484–9. doi: 10.1056/NEJM199402173300709
124. Mehta PH, Fiorenza S, Koldej RM, Jaworowski A, Ritchie DS, Quinn KM. T Cell fitness and autologous car T cell therapy in haematologic malignancy. Front Immunol (2021) 12:780442. doi: 10.3389/fimmu.2021.780442
125. Banerjee R, Marsal J, Huang C, Lo M, Kambhampati S, Kennedy VE, et al. Early time-to-Tocilizumab after bcma-directed car-T therapy in myeloma. Transpl Cell Ther (2021) 27(6):477.e1–.e7. doi: 10.1016/j.jtct.2021.03.004
126. Costa LJ, Mailankody S, Shaughnessy P, Hari P, Kaufman JL, Larson SM, et al. Anakinra (Akr) prophylaxis (Ppx) in patients (Pts) with Relapsed/Refractory multiple myeloma (Rrmm) receiving orvacabtagene autoleucel (Orva-cel). J Clin Oncol (2021) 39(15_suppl):2537. doi: 10.1200/JCO.2021.39.15_suppl.2537
127. Banerjee R, Fakhri B, Shah N. Toci or not toci: Innovations in the diagnosis, prevention, and early management of cytokine release syndrome. Leukemia lymphoma (2021) 62(11):2600–11. doi: 10.1080/10428194.2021.1924370
128. Van Oekelen O, Aleman A, Upadhyaya B, Schnakenberg S, Madduri D, Gavane S, et al. Neurocognitive and hypokinetic movement disorder with features of parkinsonism after bcma-targeting car-T cell therapy. Nat Med (2021) 27(12):2099–103. doi: 10.1038/s41591-021-01564-7
129. Cohen AD, Parekh S, Santomasso BD, Gallego Perez-Larraya J, van de Donk N, Arnulf B, et al. Incidence and management of car-T neurotoxicity in patients with multiple myeloma treated with ciltacabtagene autoleucel in cartitude studies. Blood Cancer J (2022) 12(2):32. doi: 10.1038/s41408-022-00629-1
130. Wang D, Mao X, Que Y, Xu M, Cheng Y, Huang L, et al. Viral Infection/Reactivation during long-term follow-up in multiple myeloma patients with anti-bcma car therapy. Blood Cancer J (2021) 11(10):168. doi: 10.1038/s41408-021-00563-8
131. Kambhampati S, Sheng Y, Huang CY, Bylsma S, Lo M, Kennedy V, et al. Infectious complications in patients with relapsed refractory multiple myeloma after bcma car T-cell therapy. Blood Adv (2022) 6(7):2045–54. doi: 10.1182/bloodadvances.2020004079
132. Mohan M, Nagavally S, Dhakal B, Radhakrishnan SV, Chhabra S, D'Souza A, et al. Risk of infections with b-cell maturation antigen-directed immunotherapy in multiple myeloma. Blood Adv (2022) 6(8):2466–70. doi: 10.1182/bloodadvances.2021006178
133. Josyula S, Pont MJ, Dasgupta S, Song X, Thomas S, Pepper G, et al. Pathogen-specific humoral immunity and infections in b cell maturation antigen-directed chimeric antigen receptor T cell therapy recipients with multiple myeloma. Transplant Cell Ther (2022) 28(6):304 e1– e9. doi: 10.1016/j.jtct.2022.03.005
134. Marzal-Alfaro MB, Escudero-Vilaplana V, Revuelta-Herrero JL, Collado-Borrell R, Herranz-Alonso A, Sanjurjo-Saez M. Chimeric antigen receptor T cell therapy management and safety: A practical tool from a multidisciplinary team perspective. Front Oncol (2021) 11:636068. doi: 10.3389/fonc.2021.636068
135. Lin L, Cho SF, Xing L, Wen K, Li Y, Yu T, et al. Preclinical evaluation of Cd8+ anti-bcma mrna car T cells for treatment of multiple myeloma. Leukemia (2021) 35(3):752–63. doi: 10.1038/s41375-020-0951-5
136. Fu W, Du J, Jiang H, Cheng Z, Wei R, Yu K, et al. Efficacy and safety of car-T therapy with safety switch targeting bcma for patients with Relapsed/Refractory multiple myeloma in a phase 1 clinical study. Blood (2019) 134(Supplement_1):3154. doi: 10.1182/blood-2019-127608
137. Banerjee R, Shah N, Dicker AP. Next-generation implementation of chimeric antigen receptor T-cell therapy using digital health. JCO Clin Cancer Inform (2021) 5:668–78. doi: 10.1200/CCI.21.00023
138. Alexander M, Culos K, Roddy J, Shaw JR, Bachmeier C, Shigle TL, et al. Chimeric antigen receptor T cell therapy: A comprehensive review of clinical efficacy, toxicity, and best practices for outpatient administration. Transplant Cell Ther (2021) 27(7):558–70. doi: 10.1016/j.jtct.2021.01.014
139. Maziarz RT, Diaz A, Miklos DB, Shah NN. Perspective: An international fludarabine shortage: Supply chain issues impacting transplantation and immune effector cell therapy delivery. Transplant Cell Ther (2022) 28(11):723–6. doi: 10.1016/j.jtct.2022.08.002
140. Cowan AJ, Allen C, Barac A, Basaleem H, Bensenor I, Curado MP, et al. Global burden of multiple myeloma: A systematic analysis for the global burden of disease study 2016. JAMA Oncol (2018) 4(9):1221–7. doi: 10.1001/jamaoncol.2018.2128
141. Dalla Palma B, Marchica V, Catarozzo MT, Giuliani N, Accardi F. Monoclonal and bispecific anti-bcma antibodies in multiple myeloma. J Clin Med (2020) 9(9):3022. doi: 10.3390/jcm9093022
142. Demel I, Bago JR, Hajek R, Jelinek T. Focus on monoclonal antibodies targeting b-cell maturation antigen (Bcma) in multiple myeloma: Update 2020. Br J haematology (2021) 193(4):705–22. doi: 10.1111/bjh.17235
143. Lancman G, Richter J, Chari A. Bispecifics, trispecifics, and other novel immune treatments in myeloma. Hematol Am Soc Hematol Educ Program (2020) 2020(1):264–71. doi: 10.1182/hematology.2020000110
144. Nathwani N, Bertamini L, Banerjee R, Gay F, Shah N, Krishnan A. When and how to treat relapsed multiple myeloma. am soc clin oncol educ book 41. (2021) 41:358–75. doi: 10.1200/EDBK_320129.
145. Shah UA, Mailankody S. Emerging immunotherapies in multiple myeloma. BMJ (2020) 370:m3176. doi: 10.1136/bmj.m3176
146. Zhou X, Einsele H, Danhof S. Bispecific antibodies: A new era of treatment for multiple myeloma. J Clin Med (2020) 9(7):2166. doi: 10.3390/jcm9072166
147. Chen H, Yu T, Lin L, Xing L, Cho SF, Wen K, et al. Gamma-secretase inhibitors augment efficacy of bcma-targeting bispecific antibodies against multiple myeloma cells without impairing T-cell activation and differentiation. Blood Cancer J (2022) 12(8):118. doi: 10.1038/s41408-022-00716-3
148. Frerichs KA, Broekmans MEC, Marin Soto JA, van Kessel B, Heymans MW, Holthof LC, et al. Preclinical activity of jnj-7957, a novel Bcmaxcd3 bispecific antibody for the treatment of multiple myeloma, is potentiated by daratumumab. Clin Cancer Res (2020) 26(9):2203–15. doi: 10.1158/1078-0432.CCR-19-2299
149. Vrohlings M, Müller J, Jungmichel S, Senn D, Howald AB, Schleier T, et al. Preclinical assessment of Cdr101 - a Bcmaxcd3xpd-L1 trispecific antibody with superior anti-tumor efficacy. Blood (2021) 138(Supplement 1):1583. doi: 10.1182/blood-2021-152160
150. Baeuerle PA, Ding J, Patel E, Thorausch N, Horton H, Gierut J, et al. Synthetic truc receptors engaging the complete T cell receptor for potent anti-tumor response. Nat Commun (2019) 10(1):2087. doi: 10.1038/s41467-019-10097-0
151. Rapoport AP, Stadtmauer EA, Binder-Scholl GK, Goloubeva O, Vogl DT, Lacey SF, et al. Ny-Eso-1-Specific tcr-engineered T cells mediate sustained antigen-specific antitumor effects in myeloma. Nat Med (2015) 21(8):914–21. doi: 10.1038/nm.3910
152. Stadtmauer EA, Fraietta JA, Davis MM, Cohen AD, Weber KL, Lancaster E, et al. Crispr-engineered T cells in patients with refractory cancer. Science (2020) 367(6481):eaba7365. doi: 10.1126/science.aba7365
153. Goodridge JP, Bjordahl R, Mahmood S, Reiser J, Gaidarova S, Blum R, et al. Ft576: Multi-specific off-the-Shelf car-nk cell therapy engineered for enhanced persistence, avoidance of self-fratricide and optimized mab combination therapy to prevent antigenic escape and elicit a deep and durable response in multiple myeloma. Blood (2020) 136(Supplement 1):4–5. doi: 10.1182/blood-2020-142750
154. Roex G, Campillo-Davo D, Flumens D, Shaw PAG, Krekelbergh L, De Reu H, et al. Two for one: Targeting bcma and Cd19 in b-cell malignancies with off-the-Shelf dual-car nk-92 cells. J Transl Med (2022) 20(1):124. doi: 10.1186/s12967-022-03326-6
155. O'Neal J, Ritchey J, Cooper M, Niswonger J, Gonzalez S, Lee BH, et al. Oab036: In vivo efficacy of bcma-Inkt-Car is enhanced by nt-I7, a long-acting il-7. Clin Lymphoma Myeloma Leukemia (2019) 19(10_suppl):e25. doi: 10.1016/j.clml.2019.09.037
156. Zhang X, Ng YY, Du Z, Li Z, Chen C, Xiao L, et al. Vgamma9vdelta2 T cells expressing a bcma-specific chimeric antigen receptor inhibit multiple myeloma xenograft growth. PLoS One (2022) 17(6):e0267475. doi: 10.1371/journal.pone.0267475
157. Drent E, Groen RW, Noort WA, Themeli M, Lammerts van Bueren JJ, Parren PW, et al. Pre-clinical evaluation of Cd38 chimeric antigen receptor engineered T cells for the treatment of multiple myeloma. Haematologica (2016) 101(5):616–25. doi: 10.3324/haematol.2015.137620
158. Ramos CA, Savoldo B, Torrano V, Ballard B, Zhang H, Dakhova O, et al. Clinical responses with T lymphocytes targeting malignancy-associated kappa light chains. J Clin Invest (2016) 126(7):2588–96. doi: 10.1172/JCI86000
159. Mathur R, Zhang Z, He J, Galetto R, Gouble A, Chion-Sotinel I, et al. Universal Slamf7-specific car T-cells as treatment for multiple myeloma. Blood (2017) 130(Suppl_1):502. doi: 10.1182/blood.V130.Suppl_1.502.502
160. Smith EL, Harrington K, Staehr M, Masakayan R, Jones J, Long TJ, et al. Gprc5d is a target for the immunotherapy of multiple myeloma with rationally designed car T cells. Sci Transl Med (2019) 11(485):eaau7746. doi: 10.1126/scitranslmed.aau7746
161. Baumeister SH, Murad J, Werner L, Daley H, Trebeden-Negre H, Gicobi JK, et al. Phase I trial of autologous car T cells targeting Nkg2d ligands in patients with Aml/Mds and multiple myeloma. Cancer Immunol Res (2019) 7(1):100–12. doi: 10.1158/2326-6066.CIR-18-0307
162. Rodriguez-Lobato LG, Ganzetti M, Fernandez de Larrea C, Hudecek M, Einsele H, Danhof S. Car T-cells in multiple myeloma: State of the art and future directions. Front Oncol (2020) 10:1243. doi: 10.3389/fonc.2020.01243
Keywords: myeloma, CAR-T, BCMA CAR-T therapy, gamma secretase inhibitor, ciltacabtagene autoleucel, idecabtagene vicleucel
Citation: Banerjee R, Lee SS and Cowan AJ (2022) Innovation in BCMA CAR-T therapy: Building beyond the Model T. Front. Oncol. 12:1070353. doi: 10.3389/fonc.2022.1070353
Received: 14 October 2022; Accepted: 08 November 2022;
Published: 24 November 2022.
Edited by:
Joshua Richter, Icahn School of Medicine at Mount Sinai, United StatesReviewed by:
Dina Schneider, Lentigen Technology, United StatesYaya Chu, New York Medical College, United States
Copyright © 2022 Banerjee, Lee and Cowan. This is an open-access article distributed under the terms of the Creative Commons Attribution License (CC BY). The use, distribution or reproduction in other forums is permitted, provided the original author(s) and the copyright owner(s) are credited and that the original publication in this journal is cited, in accordance with accepted academic practice. No use, distribution or reproduction is permitted which does not comply with these terms.
*Correspondence: Rahul Banerjee, rahul.banerjee.md@gmail.com
†ORCID: Rahul Banerjee, orcid.org/0000-0003-3781-5441