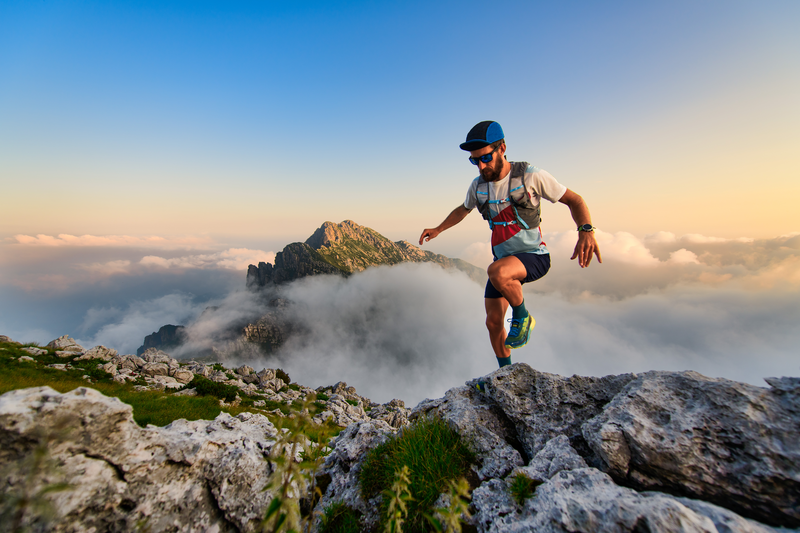
94% of researchers rate our articles as excellent or good
Learn more about the work of our research integrity team to safeguard the quality of each article we publish.
Find out more
CASE REPORT article
Front. Oncol. , 06 December 2022
Sec. Radiation Oncology
Volume 12 - 2022 | https://doi.org/10.3389/fonc.2022.1066191
This article is part of the Research Topic Case Reports in Radiation Oncology : 2022 View all 17 articles
Background: Pulsed low-dose-rate radiotherapy (pLDR) is a commonly used reirradiation technique for recurrent glioma, but its upfront use with temozolomide (TMZ) following primary resection of glioblastoma is currently under investigation. Because standard magnetic resonance imaging (MRI) has limitations in differentiating treatment effect from tumor progression in such applications, perfusion-weighted MRI (PWI) can be used to create fractional tumor burden (FTB) maps to spatially distinguish active tumor from treatment-related effect.
Methods: We performed PWI prior to re-resection in four patients with glioblastoma who had undergone upfront pLDR concurrent with TMZ who had radiographic suspicion for tumor progression at a median of 3 months (0-5 months or 0-143 days) post-pLDR. The pathologic diagnosis was compared to retrospectively-generated FTB maps.
Results: The median patient age was 55.5 years (50-60 years). All were male with IDH-wild type (n=4) and O6-methylguanine-DNA methyltransferase (MGMT) hypermethylated (n=1) molecular markers. Pathologic diagnosis revealed treatment effect (n=2), a mixture of viable tumor and treatment effect (n=1), or viable tumor (n=1). In 3 of 4 cases, FTB maps were indicative of lesion volumes being comprised predominantly of treatment effect with enhancing tumor volumes comprised of a median of 6.8% vascular tumor (6.4-16.4%).
Conclusion: This case series provides insight into the radiographic response to upfront pLDR and TMZ and the role for FTB mapping to distinguish tumor progression from treatment effect prior to redo-surgery and within 20 weeks post-radiation.
Glioblastoma is a primary central nervous system glioma designated as World Health Organization (WHO) grade 4 with wildtype isocitrate dehydrogenase (IDH-wt) (1). Patients with glioblastoma have a median overall survival of 12-15 months following diagnosis, with a five-year survival between 3 and 5.5% (2–5). The standard of care for glioblastoma includes surgical resection, chemoradiotherapy with temozolomide (TMZ) followed by adjuvant TMZ and tumor-treating fields (TTF) (5–9). Response to treatment is routinely assessed by magnetic resonance imaging (MRI). Pulsed low-dose-rate radiotherapy (pLDR) is a commonly used reirradiation technique for recurrent high-grade gliomas, but its upfront use with concurrent TMZ is currently under investigation (10–13). While other salvage therapies for recurrent high-grade glioma exist, including stereotactic radiosurgery, conformal external beam radiation, and brachytherapy, pLDR delivers radiation in subfractions at specific time intervals, taking advantage of the hyper-radiosensitivity of proliferating tumor cells to low doses of radiation, as well as the reduced toxicity to normal brain tissue (12, 14). Currently, there is limited data on the radiographic response to pLDR.
Distinguishing between treatment effect and tumor progression is challenging on standard imaging, with a definitive diagnosis only possible with pathologic confirmation. Radiotherapy may induce an inflammatory intraparenchymal response with subsequent necrosis and/or edema that is indistinguishable from, or intermingled with, tumor progression on MRI (15). Specifically, postcontrast MRI highlights blood-brain barrier disruption, which can be observed with both non-tumor and viable tumor tissue (15). Therefore, a new contrast-enhancing lesion arising within the radiation field of a treated glioblastoma can neither confirm nor refute progression of disease (16). A recent meta-analysis identified treatment effect in 36% of high-grade glioma cases (17). More recently, certain tumor and treatment factors have correlated with increased observation of treatment effect on MRI, including O6-methylguanine-DNA methyltransferase (MGMT) promoter methylation, radiation dose, dose per fraction, treatment duration, irradiated brain volume, and concurrent use of TMZ (18–22).
Fractional tumor burden (FTB) mapping is a novel radiographic biomarker that spatially distinguishes viable high-grade tumor from treatment effect within postcontrast T1-weighted enhancement on MRI (23). These maps are generated from dynamic susceptibility contrast (DSC) MR perfusion, which allows for the visualization of regional cerebral blood volume (RCBV) to identify neovascularization. FTB maps use tissue-validated standardized regional cerebral blood volume (sRCBV) thresholds (23, 24) and assign primary colors to different perfusion patterns: treatment effect (blue; sRCBV < 1.0), tumor/treatment effect admixture (yellow; 1.0 > sRCBV < 1.6), and viable tumor (red; sRCBV > 1.6). Standardized thresholds are advantageous in that they require minimal user input compared to thresholds normalized based on user-defined reference tissues (25). It was demonstrated that regions of high-grade vascular tumor (yellow + red) could be distinguished from regions of treatment effect (blue) with a sensitivity/specificity of 79.4%/90% and an accuracy of 85% (23). Hoxworth et al. prospectively validated FTB maps with tissue, observing an 85% accuracy of identifying voxels with at least 50% viable tumor (25). Of note, Iv et al. determined that either the red or blue regions were best at distinguishing tumor from treatment effect, using a different sRCBV threshold of 1.75 (26).
In this case series, we present four patients with glioblastoma treated with upfront pLDR with concurrent TMZ following maximal safe resection. Treatment response was assessed by 3 Tesla (3T) MRI with and without contrast, FTB maps, and radiographic findings were confirmed by pathology.
A 50-year-old male presented with a progressive cognitive decline, expressive aphasia, and personality changes. Brain MRI revealed a non-enhancing small region of increased T2-weighted signal in the right frontal lobe; a follow-up MRI 25 weeks later revealed an avidly enhancing mass exhibiting significant mass effect. He was eventually submitted for craniotomy and gross-total resection of the enhancing lesion. Integrated diagnosis was consistent with glioblastoma, WHO grade 4, IDH-wt, and MGMT hypermethylated. At four-weeks post-surgery, there was improvement in cognitive performance although personality changes persisted. He was treated with pLDR as part of a prospective phase II study (NCT04747145) to a total dose of 60 Gray (Gy) in 30 fractions with concurrent TMZ, which he tolerated with anticipated side effects. This was followed by adjuvant TMZ and TTF.
The patient completed a total of four cycles of TMZ and TTF before MRI of the brain, obtained at 20 weeks post-pLDR, which raised concerns for tumor progression. Increased nodular enhancement around the resection cavity (Figure 1A) was observed in the setting of the patient experiencing neurocognitive decline. FTB maps revealed predominantly treatment effect with 70.3% non-tumor, 22.9% tumor admixture, and 6.8% tumor tissue (Figures 1B, D).
Figure 1 Pre- and post-surgery MRI. (A) Postcontrast T1-weighted MRI obtained 20 weeks following the completion of pLDR and two weeks before redo-surgery. (B) Corresponding maps of FTB superimposed on the postcontrast T1-weighted MRI images. The FTB maps show areas of active tumor (red), treatment effect (blue), and transitional zone (yellow). (C) Immediate post-operative postcontrast T1-weighted MRI demonstrating extent of resection. (D) Distribution of T1-weighted enhancement volume based on vascularization as measured by FTB maps.
At 23 weeks post-pLDR, the patient was submitted for redo-craniotomy in the absence of FTB-guidance. Near-total resection of the enhancing lesion was later confirmed by postoperative MRI (Figure 1C). Microscopy of the five tissue specimens revealed a combination of necrosis, hyalinized and necrotic blood vessels, chronic inflammation, foamy macrophages, mineralization, and reactive gliosis, consistent with treatment effect. Therefore, the patient resumed adjuvant treatment with TMZ and TTF. Of note, compliance with TTF averaged 90% of “ON” time throughout the treatment period. At four weeks post-redo surgery, the patient remained clinically stable.
A 56-year-old male presented with a history of seizure and expressive aphasia. Brain MRI revealed a left parietal mass with surrounding vasogenic edema and mass effect. Craniotomy and gross-total resection were performed, and integrated diagnosis was consistent with glioblastoma WHO grade 4, IDH-wt, and MGMT unmethylated. The patient was treated with pLDR as a part of a prospective phase II study (NCT04747145) to a total dose of 60 Gy in 30 fractions with concurrent TMZ followed by adjuvant TMZ and TTF.
At one week post-pLDR, there was clinical decline with MRI demonstrating significant vasogenic edema for which a bevacizumab infusion was provided every two weeks at 10 mg/kg. It was discontinued after two infusions due to a poor response and continued clinical decline. At five weeks post-pLDR, MRI demonstrated an increase in the left parietal peripherally enhancing lesion size (Figure 2A). FTB maps revealed predominantly treatment effect within the contrast enhancement surrounding the resection cavity with 84.0% non-tumor, 9.6% tumor admixture, and 6.4% tumor (Figures 2B, D).
Figure 2 Pre- and post-surgery MRI. (A) Postcontrast T1-weighted MRI obtained five weeks post-pLDR and two weeks before redo-surgery. (B) Corresponding maps of FTB superimposed on the postcontrast T1-weighted MRI images. The FTB maps show areas of active tumor (red), treatment effect (blue), and transitional zone (yellow). (C) Post-surgery (four weeks) postcontrast T1-weighted MRI demonstrating extent of resection. (D) Distribution of T1-weighted enhancement volume based on vascularization as measured by FTB maps.
At eight weeks post-pLDR, redo-craniotomy was performed in the absence of FTB-guidance. Reduced vasogenic edema and regions of enhancement around the resection cavity were later confirmed by MRI performed four weeks post-surgery (Figure 2C). Three tissue specimens were evaluated, two of which showed necrosis and hyalinized vessels, consistent with treatment effect, while the last sample revealed hypercellular high-grade glioma. The patient continued adjuvant treatment with TMZ and TTF. At nine weeks post-redo surgery, the patient’s neurological symptoms included fatigue, right hemiparesis, and global aphasia. At 10 weeks post-redo surgery, he was restarted on bevacizumab at 10 mg/kg every 2 weeks, completing two infusions. Ultimately, he experienced a traumatic fall complicated by intraparenchymal hemorrhage and was transitioned to hospice.
A 55-year-old male presented with a seizure, visual impairment and personality changes. Brain MRI revealed an enhancing left occipital mass surrounded by diffuse FLAIR hyperintensity signal. Craniotomy and gross-total resection were performed and integrated diagnosis was consistent with glioblastoma WHO grade 4, IDH-wt, and MGMT unmethylated. Following surgery, his symptoms included right hemiparesis, global aphasia, and a right homonymous hemianopsia. The patient was enrolled in a prospective phase II study (NCT04747145) to be treated with pLDR to a total dose of 60 Gy in 30 fractions with concurrent TMZ followed by adjuvant TMZ and TTF.
Near completion of pLDR (fraction 24) and 8 weeks post-surgery, he was admitted for worsening symptoms of gait dysfunction, nausea and vomiting, and dysphagia. MRI revealed increased enhancement around the resection cavity with increased mass effect and left ventricular trapping (Figure 3A). FTB maps revealed predominantly treatment effect within the contrast enhancement surrounding the resection cavity with 64.6% non-tumor, 19.1% tumor admixture, and 16.4% tumor (Figures 3B, D). Radiation was withheld and redo-craniotomy was performed in the absence of FTB-guidance. Five tissue specimens revealed necrotic tissue and sparse reactive brain tissue, consistent with treatment effect. Neurologically, the patient improved in memory and speech with residual comprehensive aphasia and right visual field defect. Bevacizumab was subsequently started at 10 mg/kg every 2 weeks.
Figure 3 Pre- and post-surgery MRI. (A) Postcontrast T1-weighted MRI obtained four weeks post-pLDR treatment and one week before redo-surgery. (B) Corresponding maps of FTB superimposed on the postcontrast T1-weighted MRI images. The FTB maps show areas of active tumor (red), treatment effect (blue), and transitional zone (yellow). (C) Post-surgery (one week) postcontrast T1-weighted MRI demonstrating extent of resection. (D) Distribution of T1-weighted enhancement volume based on vascularization as measured by FTB maps.
The patient continued adjuvant treatment with bevacizumab, TMZ, and TTF. Of note, compliance with TTF averaged 75-90% of “ON” time throughout the treatment period. At one week post-surgery, brain MRI demonstrated retraction of the resection cavity and decreased peripheral enhancement along the surgical margins (Figure 3C). At 29 weeks post-redo surgery, the patient was transitioned to hospice, experienced respiratory failure caused by aspiration from worsening dysphagia and expired.
A 60-year-old male presented with progressive left hemiparesis and memory decline. Brain MRI revealed an enhancing mass within the right frontal lobe that was resected. Integrated diagnosis was consistent with glioblastoma WHO grade 4, IDH-wt, and MGMT unmethylated. There was significant postoperative improvement in symptoms. The patient was treated with pLDR as a part of a prospective phase II study (NCT04747145) to a total dose of 60 Gy in 30 fractions with concurrent TMZ followed by adjuvant TMZ. Adjuvant TTF were declined.
Follow-up brain MRI studies demonstrated progressively increasing enhancement around the resection cavity. At 13 weeks post-pLDR, FTB maps revealed 47.9% non-tumor, 19.2% tumor admixture, and 32.9% tumor within the contrast-enhancing tissue. Seven weeks later, brain MRI demonstrated increased enhancement, particularly at the superomedial resection cavity margin (Figure 4A). FTB maps were again obtained with increasing proportions of vascular tumor tissue suggesting progression: 42.9% non-tumor, 13.5% tumor admixture, and 43.6% tumor (Figures 4B, D).
Figure 4 Pre- and post-surgery MRI. (A) Postcontrast T1-weighted MRI obtained 20 weeks post-pLDR and 10 weeks before redo-surgery. (B) Corresponding maps of FTB superimposed on the postcontrast T1-weighted MRI images. The FTB maps show areas of active tumor (red), treatment effect (blue), and transitional zone (yellow). (C) Immediate post-operative postcontrast T1-weighted MRI with FLAIR demonstrating extent of resection, which encompasses regions of tumor identified in the FTB maps. (D) Distribution of T1-weighted enhancement volume based on vascularization as measured by FTB maps.
At 30 weeks post-pLDR, redo-craniotomy was performed in the absence of FTB-guidance. Significant debulking was later confirmed by MRI (Figure 4C). Two tissue specimens revealed moderately cellular atypical glial proliferation, supportive of active tumor. At three weeks post-redo surgery, the patient progressed with gait dysfunction, dysphagia, expressive aphasia, and left facial droop.
This case series describes examples of how FTB can improve radiologic accuracy when used as a biomarker to assess treated glioblastoma. In one study, participating physicians determined that they would change treatment in 93% of cases where the tumor fraction was predominant and not change treatment in 75% of cases where treatment effect was predominant, based on their interpretation of FTB maps (26). The authors suggested the potential use of FTB for providing guidance in deciding which patients need an operation (26). In all four of the cases presented here, there were concerns about tumor progression based on standard 3T MRI. Pathologic examination revealed treatment effect in two cases and viable tumor in the other two cases. FTB maps were indicative of lesion volumes being comprised of predominantly treatment effect in three cases and predominantly viable tumor in one case. From the three FTB maps in the former category, the median fraction of the enhancing tumor volume comprised of vascular tumor was 6.8% (range 6.4-16.4%).
Radiologic assessment of treated glioblastoma remains a challenge as an indistinguishable MRI pattern between treatment effect and viable tumor may develop in about 36% of these cases (17). Often, with no other options, biopsy or surgery is pursued for diagnostic confirmation, posing potentially unnecessary risks. This common scenario highlights the need for more accurate radiologic biomarkers that not only guide treatment but also prevent unnecessary surgical intervention. This is of particular importance with heterogenous tumors such as glioblastoma that are more prone to sampling error. FTB maps provide meaningful information regarding the spatial distribution of tumor and treatment effect within enhancing lesions and may be able to fill that gap.
There are several limitations to this study. FTB maps were generated retrospectively and therefore not used for surgical guidance or tissue confirmation in none of the four cases. It also does not provide information about the clinical course of the disease. Monitoring FTB over time may provide additional information to evaluate this, as demonstrated in Case 4. We also assume that the pathology reports are always accurate, when sampling bias may occur. For instance, it is unknown whether an active tumor is in the process of dying or may die in the near future, especially if it is sampled too soon following radiation. In parallel, bevacizumab as a treatment for symptomatic radiation necrosis in Cases 2 and 3 may have confounded their respective radiologic responses to pLDR, as it may reduce the area of enhancing necrosis by decreasing vascular permeability and inflammation (27). Finally, the small sample size from our single institution limits generalizability.
This case series provides insight into response to upfront pLDR concurrent with TMZ following resection of newly diagnosed glioblastoma. It also highlights the capacity of FTB maps to accurately distinguish tumor progression from treatment effect. Therefore, full validation of FTB mapping as a biomarker should be pursued as a prospective study with larger sample size.
The original contributions presented in the study are included in the article/supplementary material. Further inquiries can be directed to the corresponding author.
The studies involving human participants were reviewed and approved by Medical College of Wisconsin Institutional Review Board. The patients/participants provided their written informed consent to participate in this study. Written informed consent was obtained from the individual(s) for the publication of any potentially identifiable images or data included in this article.
FS-P, MS, WM, and MK treated the patients. DC and EC analyzed tissue samples and generated pathology reports for the patients. RA wrote the first draft of the manuscript and generated figures. MP and KS performed image processing and generated the FTB maps. MP, MS, FS, WM, MK, CK, DC, EC, JB, CS, and KS edited the paper. All authors contributed to the article and approved the submitted version.
Funding support was provided by NIH/NCI U01CA176110, NIH/NCI R01CA255123 and NIH/NCI R01CA264992.
Cathy Marszalkowski and Jaimy Pettit research coordinators, for obtaining consents from patients and managing the extensive brain tumor database.
KS has ownership interest in IQ-AI Ltd, and financial interest in Imaging Biometrics LLC. Processing software from Imaging Biometrics LLC was used for processing the MRI images. WM is a member of the medical advisory board for Prism Clinical Imaging Inc. KS also has ownership interest in Prism Clinical Imaging Inc.
The remaining authors declare that the research was conducted in the absence of any commercial or financial relationships that could be construed as a potential conflict of interest.
All claims expressed in this article are solely those of the authors and do not necessarily represent those of their affiliated organizations, or those of the publisher, the editors and the reviewers. Any product that may be evaluated in this article, or claim that may be made by its manufacturer, is not guaranteed or endorsed by the publisher.
1. Louis DN, Perry A, Wesseling P, Brat DJ, Cree IA, Figarella-Branger D, et al. The 2021 WHO classification of tumors of the central nervous system: A summary. Neuro Oncol (2021) 23:1231–51. doi: 10.1093/neuonc/noab106
2. Ostrom QT, Gittleman H, Xu J, Kromer C, Wolinsky Y, Kruchko C, et al. CBTRUS statistical report: Primary brain and other central nervous system tumors diagnosed in the united states in 2009-2013. Neuro Oncol (2016) 18(suppl_5):v1–v75. doi: 10.1093/neuonc/now207
3. Korja M, Raj R, Seppä K, Luostarinen T, Malila N, Seppälä M, et al. Glioblastoma survival is improving despite increasing incidence rates: A nationwide study between 2000 and 2013 in Finland. Neuro Oncol (2019) 21:370–9. doi: 10.1093/neuonc/noy164
4. Chien LN, Gittleman H, Ostrom QT, Hung KS, Sloan AE, Hsieh YC, et al. Comparative brain and central nervous system tumor incidence and survival between the united states and Taiwan based on population-based registry. Front Public Health (2016) 4:151. doi: 10.3389/fpubh.2016.00151
5. Stupp R, Mason WP, van den Bent MJ, Weller M, Fisher B, Taphoorn MJ, et al. Radiotherapy plus concomitant and adjuvant temozolomide for glioblastoma. N Engl J Med (2005) 352:987–96. doi: 10.1056/NEJMoa043330
6. Stupp R, Hegi ME, Mason WP, van den Bent MJ, Taphoorn MJ, Janzer RC, et al. Effects of radiotherapy with concomitant and adjuvant temozolomide versus radiotherapy alone on survival in glioblastoma in a randomised phase III study: 5-year analysis of the EORTC-NCIC trial. Lancet Oncol (2009) 10:459–66. doi: 10.1016/S1470-2045(09)70025-7
7. Herrlinger U, Tzaridis T, Mack F, Steinbach JP, Schlegel U, Sabel M, et al. Lomustine-temozolomide combination therapy versus standard temozolomide therapy in patients with newly diagnosed glioblastoma with methylated MGMT promoter (CeTeG/NOA-09): a randomised, open-label, phase 3 trial. Lancet (2019) 393:678–88. doi: 10.1016/S0140-6736(18)31791-4
8. Stupp R, Taillibert S, Kanner AA, Kesari S, Steinberg DM, Toms SA, et al. Maintenance therapy with tumor-treating fields plus temozolomide vs temozolomide alone for glioblastoma: A randomized clinical trial. JAMA (2015) 314:2535–43. doi: 10.1001/jama.2015.16669
9. Stupp R, Taillibert S, Kanner A, Read W, Steinberg D, Lhermitte B, et al. Effect of tumor-treating fields plus maintenance temozolomide vs maintenance temozolomide alone on survival in patients with glioblastoma: A randomized clinical trial. JAMA (2017) 318:2306–16. doi: 10.1001/jama.2017.18718
10. Cannon GM, Tomé WA, Robins HI, Howard SP. Pulsed reduced dose-rate radiotherapy: Case report: a novel re-treatment strategy in the management of recurrent glioblastoma multiforme. J Neurooncol (2007) 83:307–11. doi: 10.1007/s11060-007-9329-z
11. Murphy ES, Rogacki K, Godley A, Qi P, Reddy CA, Ahluwalia MS, et al. Intensity modulated radiation therapy with pulsed reduced dose rate as a reirradiation strategy for recurrent central nervous system tumors: An institutional series and literature review. Pract Radiat Oncol (2017) 7:e391–9. doi: 10.1016/j.prro.2017.04.003
12. Bovi JA, Prah MA, Retzlaff AA, Schmainda KM, Connelly JM, Rand SD, et al. Pulsed reduced dose rate radiotherapy in conjunction with bevacizumab or bevacizumab alone in recurrent high-grade glioma: Survival outcomes. Int J Radiat Oncol Biol Phys (2020) 108(4):979–86. doi: 10.1016/j.ijrobp.2020.06.020
13. Prabhu AV, Lee M, Galhardo E, Newkirk M, Rodriguez A, Xia F. Pulsed reduced dose-rate radiotherapy for previously irradiated tumors in the brain and spine. Surg Neurol Int (2021) 12:280. doi: 10.25259/SNI_589_2020
14. Ma CMC. Pulsed low dose-rate radiotherapy: Radiobiology and dosimetry. Phys Med Biol (2022) 67:03TR01. doi: 10.1088/1361-6560/ac4c2f
15. Nichelli L, Casagranda S. Current emerging MRI tools for radionecrosis and pseudoprogression diagnosis. Curr Opin Oncol (2021) 33:597–607. doi: 10.1097/CCO.0000000000000793
16. Raimbault A, Cazals X, Lauvin MA, Destrieux C, Chapet S, Cottier JP. Radionecrosis of malignant glioma and cerebral metastasis: A diagnostic challenge in MRI. Diagn Interv Imaging (2014) 95:985–1000. doi: 10.1016/j.diii.2014.06.013
17. Abbasi AW, Westerlaan HE, Holtman GA, Aden KM, van Laar PJ, van der Hoorn A. Incidence of tumour progression and pseudoprogression in high-grade gliomas: A systematic review and meta-analysis. Clin Neuroradiol (2018) 28:401–11. doi: 10.1007/s00062-017-0584-x
18. Brandes AA, Franceschi E, Tosoni A, Blatt V, Pession A, Tallini G, et al. MGMT promoter methylation status can predict the incidence and outcome of pseudoprogression after concomitant radiochemotherapy in newly diagnosed glioblastoma patients. J Clin Oncol (2008) 26:2192–7. doi: 10.1200/JCO.2007.14.8163
19. Verma N, Cowperthwaite MC, Burnett MG, Markey MK. Differentiating tumor recurrence from treatment necrosis: A review of neuro-oncologic imaging strategies. Neuro Oncol (2013) 15:515–34. doi: 10.1093/neuonc/nos307
20. Donovan EK, Parpia S, Greenspoon JN. Incidence of radionecrosis in single-fraction radiosurgery compared with fractionated radiotherapy in the treatment of brain metastasis. Curr Oncol (2019) 26:e328–33. doi: 10.3747/co.26.4749
21. Lee AW, Kwong DL, Leung SF, Tung SY, Sze WM, Sham JS, et al. Factors affecting risk of symptomatic temporal lobe necrosis: Significance of fractional dose and treatment time. Int J Radiat Oncol Biol Phys (2002) 53:75–85. doi: 10.1016/s0360-3016(02)02711-6
22. Brandsma D, Stalpers L, Taal W, Sminia P, van den Bent MJ. Clinical features, mechanisms, and management of pseudoprogression in malignant gliomas. Lancet Oncol (2008) 9:453–61. doi: 10.1016/S1470-2045(08)70125-6
23. Prah MA, Al-Gizawiy MM, Mueller WM, Cochran EJ, Hoffmann RG, Connelly JM, et al. Spatial discrimination of glioblastoma and treatment effect with histologically-validated perfusion and diffusion magnetic resonance imaging metrics. J Neurooncol (2018) 136:13–21. doi: 10.1007/s11060-017-2617-3
24. Hu LS, Eschbacher JM, Heiserman JE, Dueck AC, Shapiro WR, Liu S, et al. Reevaluating the imaging definition of tumor progression: Perfusion MRI quantifies recurrent glioblastoma tumor fraction, pseudoprogression, and radiation necrosis to predict survival. Neuro Oncol (2012) 14:919–30. doi: 10.1093/neuonc/nos112
25. Hoxworth JM, Eschbacher JM, Gonzales AC, Singleton KW, Leon GD, Smith KA, et al. Performance of standardized relative CBV for quantifying regional histologic tumor burden in recurrent high-grade glioma: Comparison against normalized relative CBV using image-localized stereotactic biopsies. AJNR Am J Neuroradiol (2020) 41:408–15. doi: 10.3174/ajnr.A6486
26. Iv M, Liu X, Lavezo J, Gentles AJ, Ghanem R, Lummus S, et al. Perfusion MRI-based fractional tumor burden differentiates between tumor and treatment effect in recurrent glioblastomas and informs clinical decision-making. AJNR Am J Neuroradiol (2019) 40:1649–57. doi: 10.3174/ajnr.A6211
Keywords: glioblastoma, pulsed low-dose-rate radiotherapy (pLDR), MRI, fractional tumor burden (FTB), relative cerebral blood volume (rCBV), pseudoprogression, treatment effect, tumor progression
Citation: Amidon RF, Santos-Pinheiro F, Straza M, Prah MA, Mueller WM, Krucoff MO, Connelly JM, Kleefisch CJ, Coss DJ, Cochran EJ, Bovi JA, Schultz CJ and Schmainda KM (2022) Case report: Fractional brain tumor burden magnetic resonance mapping to assess response to pulsed low-dose-rate radiotherapy in newly-diagnosed glioblastoma. Front. Oncol. 12:1066191. doi: 10.3389/fonc.2022.1066191
Received: 10 October 2022; Accepted: 21 November 2022;
Published: 06 December 2022.
Edited by:
Ianik Plante, KBRwyle, United StatesReviewed by:
Mattia Falchetto Osti, Sapienza University of Rome, ItalyCopyright © 2022 Amidon, Santos-Pinheiro, Straza, Prah, Mueller, Krucoff, Connelly, Kleefisch, Coss, Cochran, Bovi, Schultz and Schmainda. This is an open-access article distributed under the terms of the Creative Commons Attribution License (CC BY). The use, distribution or reproduction in other forums is permitted, provided the original author(s) and the copyright owner(s) are credited and that the original publication in this journal is cited, in accordance with accepted academic practice. No use, distribution or reproduction is permitted which does not comply with these terms.
*Correspondence: Kathleen M. Schmainda, a2F0aGxlZW5AbWN3LmVkdQ==
†These authors have contributed equally to this work
Disclaimer: All claims expressed in this article are solely those of the authors and do not necessarily represent those of their affiliated organizations, or those of the publisher, the editors and the reviewers. Any product that may be evaluated in this article or claim that may be made by its manufacturer is not guaranteed or endorsed by the publisher.
Research integrity at Frontiers
Learn more about the work of our research integrity team to safeguard the quality of each article we publish.