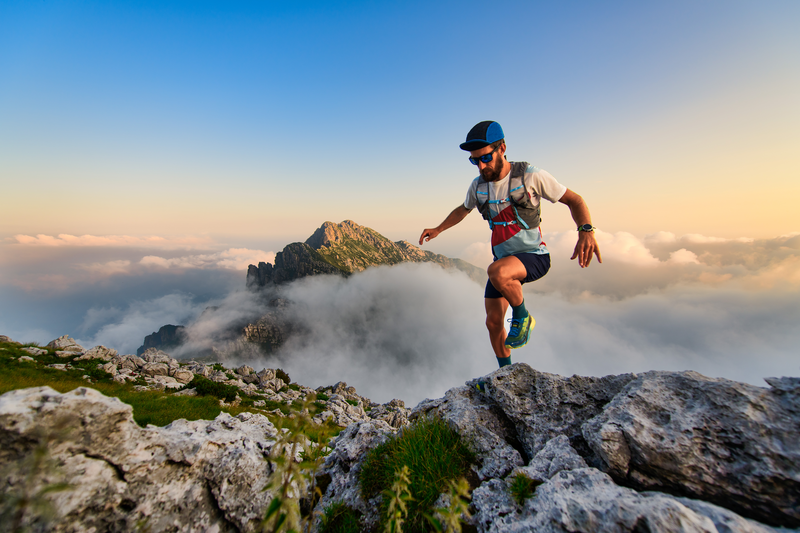
94% of researchers rate our articles as excellent or good
Learn more about the work of our research integrity team to safeguard the quality of each article we publish.
Find out more
REVIEW article
Front. Oncol. , 23 November 2022
Sec. Gastrointestinal Cancers: Colorectal Cancer
Volume 12 - 2022 | https://doi.org/10.3389/fonc.2022.1052672
This article is part of the Research Topic Reviews in Gastrointestinal Cancers View all 35 articles
Colorectal cancer (CRC) is a major global health problem and one of the major causes of cancer-related death worldwide. It is very important to understand the pathogenesis of CRC for early diagnosis, prevention strategies and identification of new therapeutic targets. Intercellular adhesion molecule-1 (ICAM-1, CD54) displays an important role in the the pathogenesis of CRC. It is a cell surface glycoprotein of the immunoglobulin (Ig) superfamily and plays an essential role in cell-cell, cell-extracellular matrix interaction, cell signaling and immune process. It is also expressed by tumor cells and modulates their functions, including apoptosis, cell motility, invasion and angiogenesis. The interaction between ICAM-1 and its ligand may facilitate adhesion of tumor cells to the vascular endothelium and subsequently in the promotion of metastasis. ICAM-1 expression determines malignant potential of cancer. In this review, we will discuss the expression, function, prognosis, tumorigenesis, polymorphisms and therapeutic implications of ICAM-1 in CRC.
Colorectal cancer (CRC) with a particularly high prevalence in China is a major health problem and one of the major causes of cancer-related death worldwide (1, 2). In recent years, both the morbidity and mortality of CRC have increased (1), thus it is very important to understand the pathogenesis of CRC for early diagnosis, prevention strategies and identification of new therapeutic targets. Some studies have focused on the identification of biomarkers in CRC, taking cell adhesion molecules (CAMs) as their subject.
CAMs refer to those cell surface structures that allow cells to adhere to each other and the extracellular matrix. They are critical regulators of cellular homeostasis and function, and play crucial roles in tumorigenesis, progression and metastasis (3, 4). Intercellular adhesion molecule-1(ICAM-1, CD54) is one of CAMs, which displays an important role in the the pathogenesis of CRC. In this review, we will discuss the expression, function, prognosis, tumorigenesis, polymorphisms and therapeutic implications of ICAM-1 in CRC.
ICAM-1, located on chromosome 19p13, is a cell surface glycoprotein of the immunoglobulin (Ig) superfamily of CAMs, and consists of 5 extracellular Ig-like domains, a transmembrane domain and a short cytoplasmic tail (5). The Ig-like domains mediate ICAM-1 interactions with its two major ligands, macrophage-1 antigen (Mac-1, CD11b/CD18) and lymphocyte function-associated antigen-1 (LFA-1, CD11a/CD18) (6).
ICAM-1 is expressed in various cell types (epithelial cells, keratinocytes, fibroblasts and immune cells) and plays an essential role in cell-cell, cell-extracellular matrix interaction, cell signaling and immune process (7, 8). It serves as a biosensor to transducer outside-in-signaling via association of its cytoplasmic domain with the actin cytoskeleton following ligand engagement of the extracellular domain. Upon ligation, ICAM-1 undergoes dimerization and clustering through homotypic binding between Ig domains (7, 8).
Epithelial cells (ECs) of normal human colon do not express ICAM-1, but it can be expressed subsequent to malignant transformation. It has also been shown that ICAM-1 is related to the mesothelial adhesion, malignant potential, occurrence and progression of CRC (9–12). The interaction between ICAM-1 and its ligand may facilitate adhesion of tumor cells to the vascular endothelium and promote metastasis subsequently. The patients with increased ICAM-1 expression have more advanced stage, as it promotes the tumor growth (11, 13). Its expression was also associated with the cell differentiation of CRC. Higher ICAM-1 expression was found in better differentiated CRC cells compared to lower ICAM-1 expression in poorly differentiated CRC cells, which demonstrated that ICAM-1 promoted CRC differentiation and retarded metastais (14). ICAM-1 may play an important role in the immune response. The increased ICAM-1 expression might reflect the elevated immunity against tumor cells and ICAM-1 renders tumor cells more sensitive to lymphocyte-mediated lysis (15).
The prognostic significance of ICAM-1 expression remains controversial in CRC. ICAM-1 plays a dual role in CRC, and its impact depends on whether this protein is expressed in a membrane-bound or a soluble form (16–20). In CRC, the increased expression of membrane-bound ICAM-1 was associated with the favorable prognosis (16, 21). Maeda K et al. reported a better prognosis for CRC patients with membrane-bound ICAM1-positive (16). Tachimori A et al. also reported that an increased membrane-bound ICAM-1 expression inhibited the tumour growth and was correlated with a favorable prognosis in CRC (22). Leqi Zhou et al. and Mlecnik B et al. reported that a high expression of ICAM-1 was relevant to a prolonged survival (21, 23).
The favorable prognosis may be attributed to two mechanisms: one is that ICAM-1 may play an important role in the immunosurveillance and enhances lymphocyte-mediated cytotoxicity (15, 24–26). T cells are important for killing tumor cells and the increased ICAM-1 expression on CD8+ T cells activates the antitumor function of CD8+ T cells. ICAM-1 expressed by tumor cells may lead to T cell-specific recognition and enhanced T cell adhesion (27). Tachimori et al. showed that more lymphocytes adhered to CRC cells when ICAM-1 expression was upregulated (22). The other potential mechanism is that ICAM-1 may play an important role in the tumor microenvironment (TME). Upregulation of ICAM-1 in CRC cells could increase cytotoxic lymphocytes (CTLs) infiltration and the expression of cytolytic immune effector molecules in the TME, which is associated with favorable prognosis in CRC (4, 28–30). Increased CTLs was observed in the TME of ICAM-1 positive CRC compared to that of ICAM-1 negative CRC (13). Fisher et al. showed that ICAM-1 blockade decreased CTLs infiltration in the TME (31). The increased ICAM-1 expression on other cells in the TME also enhances the tumor infiltration and function of CTLs (23, 32, 33).
However, in some reports, the ICAM-1 expression was correlated with a worse prognosis (34, 35). For instance, Ionescu C et al. reported overexpression of ICAM-1 was correlated with lower overall survial (OS) (34). There is no clear explanation for the apparently contrary roles of ICAM-1, suggesting that the function of ICAM-1 is context dependent and modulated by the action of other membrane receptors.
Several studies have focused on the factors regulating ICAM-1 expression, and the mechanisms seem to be multiple. Figure 1 demonstrates the potential mechanisms of ICAM-1 expression in CRC.
Figure 1 IL-6, Interleukin-6; IFN-γ, Interferon- γ; TNF-α, Tumor necrosis factor- α; SDF-1, Stromal cell derived factor-1; IL-β, Interleukin- β; MGP, Matrix gla protein; SphK1, Sphingosine kinase-1; JAK, Janus kinase; STAT3, Signal transducer and activator of transcription 3; STAT1, Signal transducer and activator of transcription 1; NFκB, NFκB pathway; JNK:c-Jun N-terminal kinase pathway; ERK, Extracellular signal-regulated kinase pathway; FAK, Focal adhesion kinase pathway; Rho/Rock, Rho/Rho-associated kinase pathway; ICAM-1, Intercellular adhesion molecule-1.
A variety of cytokines may regulate ICAM-1 expression, such as TNFα, IFNγ and IL-1β (36, 37). The regulatory mechanism is mainly involved in activating signal pathways through the binding on the ICAM-1 promoter.
TNF-α can regulate ICAM‐1 expression, which results in enhanced lymphocytic infiltration and tumor apoptosis (36). TNF-α response element is important in regulating ICAM-1 expression. This response element possesses an NF-κB binding site and NF-κB plays a significant role in TNF-α induced ICAM-1 expression (38).
IFNγ can also induce ICAM-1 expression and STAT-1 (signal transduction and activator of transcription) protein is upregulated during IFN-γ response (39). The IFN-γ response element (IRE) plays a important role in ICAM-1 expression (40). Upon IFN-γ stimulation, IRE forms a binding complex (IRE-BC) with nuclear proteins, which is required for induction of ICAM-1 expression.
IL-6 is a T-cell-derived cytokine that induces maturation of B cells. IL-6 plays a critical role in metastasis of cancer cells by modulating ICAM-1 expression (40). CRC patients exhibited high level of IL-6 and IL-6 induces ICAM-1 expression via IL-6 receptor. JAK-STAT3 pathway and the AP-1 binding site of ICAM-1 are involved in IL-6 mediated ICAM-1 expression (41).
IL-1β has been reported to induce ICAM-1 expression and is involved in multiple immune and inflammatory responses (42). IL-1β also activates NF-κB pathway, ERK pathway and JNK pathway for ICAM-1 expression.
Resistin is an adipose tissue-secreted form and could also be expressed in peripheral blood mononuclear cells, macrophages, and bone marrow cells (43). Resistin exerts its biological effects by binding to Toll-like receptor 4 (TLR4) and NF-κB can be activated by TLR4 which leads to ICAM-1 expression (44, 45).
The expression of ICAM-1 may be regulated by a few transcription factors, such as stromal cell derived factor-1 (SDF-1), Leptin, C/EBPβ (36).
Abnormal expression of SDF-1 has been detected in CRC and ICAM-1 expression was up-regulated by SDF-1 (46). MAPKs pathway may be involved in the SDF-1-mediated expression of ICAM-1 (47). By MAPKs pathways, SDF-1 activates NF-κB and C/EBPβ to bind to the promoter of ICAM-1, thus leading to ICAM-1 up-regulation in CRC cells (46).
Leptin can induce ICAM-1 expression and the Rho/ROCK (Rho-associated coiled-coil-forming protein kinase, ROCK) pathway may be involved in the leptin-mediated expression of ICAM-1 (48). Z Dong et al. revealed that leptin can induce ICAM-1 expression by the Rho/ROCK pathway (49).
SphK1 is an oncogene and is associated with angiogenesis, anti-apoptosis and survival of tumor cells (50). The SphK1 expression is enhanced in CRC and enhanced the ICAM-1expression by regulating the FAK pathway in CRC cells (51, 52). The ICAM-1 expression is upregulated with the overexpression of SphK1 and downregulated with the suppression of SphK1 in CRC cells.
MGP is a secreted, calcium-binding matrix protein. Overexpressed MGP could be found in CRC, and it may be associated with tumor progression and invasion. Li X et al. revealed that MGP expression increased in CRC and MGP promoted the phosphorylation of NF-κB by upregulating intracellular free calcium concentrations, activating the expression of ICAM-1 (53).
MicroRNAs (miRNAs) also play an important role in regulating the ICAM-1 expression in CRC. Recent studies indicate that ICAM-1 is a direct target of miRNAs, and these miRNAs bind to the untranslated region (UTR) of ICAM-1 and regulate ICAM-1 expression. Mir-221 binded to 3’UTR of ICAM-1 mRNA which resulted in transcription suppression of IFN-γ induced ICAM-1 expression (54). In addition, miR-222 and miR-339 have also been shown to bind 3’UTR of ICAM-1 promoter to suppress the ICAM-1 expression and promoted resistance of cancer cells to CTLs (55, 56). MiR-130 was induced by TNFα and lead to the increased ICAM-1 expression (57). MiR-141 binded to the 3’UTR of ICAM-1 directly and inhibited TNF-α induced ICAM-1 expression in ECs (58). Some miRNAs modulate ICAM-1 expression through down-regulating SphK1 expression in CRC cells, such as miR-613, miR-659-3p, miR-101. These miRNAs targeted SphK1 and downregulated ICAM-1 expression (59–62). SphK1 was an important target of miR-101, and miR-101 down-regulated SphK1 to inhibit ICAM-1 expression in CRC cells (62).
Genetic variations in the ICAM-1 gene can regulate the protein expression in various diseases. ICAM-1 rs5498 may affect the expression of ICAM-1 in CRC patients. Wang MY et al. reported that ICAM-1 rs5498 may affect the level of sICAM-1 (63). The level of sICAM-1 at ICAM-1 5498 allele locus in K individuals was higher than that at non-K allele. Wang QL et al. also reported patients with KK genotype showed an increased ICAM-1 expression in CRC and ICAM-1 expression was higher in patients with KK genotype than that with KE+EE genotypes (64). ICAM-1 5498 is a non-synonymous mutation, which leads to the increased expression of ICAM-1 and affects the function of ICAM-1.
ICAM-1 expression may decrease CRC metastasis. In CRC, the high expression of membrane-bound ICAM-1 was associated with a lower incidence of liver and lymph node metastases (14, 16, 17). The upregulation of ICAM-1 inhibited tumor metastasis in CRC cell lines (22, 23). Mlecnik B et al. reported upregulation of ICAM-1 in CRC cells lowered the frequency of distant metastasis (23). The transfection of ICAM-1 into CRC cells inhibited tumor metastasis (27). Tachimori A et al. also demonstrated that liver metastases decreased in CRC cells expressing ICAM-1 compared with CRC cells not expressing ICAM-1 (65).
Metastasis of CRC is a complex process that is influenced by a variety of factors. Among these factors, ICAM-1 plays a key role, but the mechanisms of how ICAM-1 decreases metastasis of CRC are not completely clear. One potential mechanism is that ICAM-1 can activate the immune system to prevent metastasis of CRC (13). ICAM-1 promotes recognition and destruction of tumor cells by the immune cells (4). ICAM-1 increased lymphocytes recruitment, promoted lymphocytes to attach to CRC cells and lymphocyte-mediated tumor lysis, which may improve the immunosurveillance and restrict tumor metastasis (20, 24). It can also sensitize metastatic tumor cells to CTL-mediated killing and prevent tumor metastasis (66). A second mechanism may be that ICAM-1, as a morphogen, enhances tumor cells attachment to the extracellular matrix by promoting motility in the context of remodeling. Taglia L et al. showed that ICAM-1 mediated tumor cells attachment to the extracellular matrix and prevented tumour cells from detaching from the primary tumor and thus retarded metastasis (16). Thirdly, ST6GAL1 could mediate tumor metastasis by regulating the stability of ICAM-1 (22). It might increase ICAM-1 stability through sialylation and consequently inhibit CRC metastasis (67, 68). Fourthly, the mechanism may be due to GRP’s activation of the immune surveillance system (2, 69). FAK phosphorylation mediates GRP’s activation of the immune system and ICAM-1 is the downstream proteins of FAK pathway (58–63, 66–72).
Apart from the membrane-bound ICAM-1 expressed on CRC cells, there exists a soluble form of ICAM-1 (sICAM-1) in serum. SICAM-1 was firstly identified in the serum of healthy volunteers by Seth et al, and its level is elevated in malignancies (73). Although the splice variant of sICAM-1 is truncated at the transmembrane domain, it retains all five extracellular Ig-like domains similarly to full-length ICAM-1 molecule, and its ability is conserved. In agreement with the small size of the transmembrane and cytoplasmic domains, sICAM-1 is only slightly smaller in size than its membrane-bound form.
The mechanism of sICAM-1 production is unclear, but it may be produced by proteolytic cleavage of membrane-bound ICAM-1, and released from the local cancer cell and enter the serum (73). Secondly, sICAM-1 is an inflammation-associated marker and is therefore increased in patients with an inflammatory TME. Thirdly, ICAM-1 rs5498 may have an effect on the levels of sICAM-1. Bielinski SJ et al reported the ICAM1 rs5498 G allele was associated with the level of sICAM-1 (74).
The level of sICAM-1 was elevated in CRC patients and can serve as a biomarker (Table 1) (15, 20, 75, 79, 80). The level of sICAM-1 was positively correlated with the tumor size, advanced stage and metastasis in CRC patients (9, 14, 15, 20, 75, 79–82). Basouglu et al. found that the serum sICAM-1 level was higher in CRC patients than that in the healthy controls and patients with advanced stage had higher sICAM-1 levels than those with a lower stage (81). Mantur et al. and Kang et al. also observed that CRC patients with higher sICAM-1 level were at a higher advanced stage (15, 75). The sICAM-1 level in patients with distant metastases increased compared with patients without metastases. High levels of sICAM-1 have been shown to be associated with liver metastasis in CRC (14, 15, 18). SICAM-1 levels decreased significantly after curative surgery for CRC (15).
Previous studies demonstrated that patients with higher sICAM-1 level revealed poor prognosis (14, 16, 23), while the patients with lower sICAM-1 level displayed an improved OS (83). Yamamoto Y et al. also reported high sICAM-1 level was associated with shorter OS in CRC patients treated with chemotherapy plus bevacizumab (84). Elevated sICAM-1 level is associated with a decreased OS and serves as independent prognostic biomarker, but the mechanisms are not completely clear. SICAM-1 can bind to circulating CTLs, inhibit the interaction between CTLs and tumour cells, and block immune recognition of tumor cells (85). It can also block NK cell-mediated toxicity and thus allow tumour cells to escape immune destruction (76). Moreover, it can promote angiogenesis and stimulate tumour cells growth (86). These findings are possible explanations for the poor prognosis.
SNPs are the most common type of DNA sequence and analysis of ICAM-1 SNPs is important for studying the genetic features of CRC. Previous studies have suggested that ICAM-1 SNPs are associated with the risk of CRC.
Several studies have assessed the relationship between CRC risk and ICAM-1 SNPs, but these results were controversial (Table 2). Some studies showed that ICAM-1 SNPs were associated with an increased CRC risk (5, 64, 87). George Theodoropoulos et al. firstly reported that ICAM-1 rs5498 was associated with an increasing CRC risk in CRC patients (87). Anbarasan C et al. and Wang QL et al. also reported ICAM-1 rs5498 increased the risk of CRC (5, 64). But in a meta-analysis, the ICAM-1 rs5498 decreased the risk of CRC in Caucasians (88). We found that ICAM-1 rs5498 was not correlated with the risk of CRC in Chinese CRC patients, but ICAM-1 rs5498 decreased the CRC risk in the subgroup of age≥61 (89). Ravindran Ankathil et al. found that ICAM-1 rs5498 did not show significant association for CRC risk in Malaysian CRC patients (90). In our previous study, ICAM-1 rs3093030 polymorphism did not influence CRC risk (89). For ICAM-1 rs179969 polymorphism, the frequencies of homozygous wild type was significantly higher in controls compared to CRC patients. The different findings may be due to different ethnicities, regions, ages or the limited sample sizes. In the future, an analysis of different SNPs may make it possible to describe the exact relations between polymorphisms and CRC risk.
ICAM-1 SNPs are correlated with differentiation of CRC. ICAM-1 rs5498 KK genotype in poorly differentiated patients was significantly higher than that in well- or moderately-differentiated patients, whereas ICAM-1 rs5498 KE+EE genotype in poorly-differentiated patients was lower than that in well- or moderately-differentiated patients. Wang QL also found that ICAM-1 rs5498 is significantly associated with well differentiation of CRC (64). Liu LB et al. reported ICAM-1 rs5498 was associated with the degree of tumor differentiation in the population of North China (91). The differentiation of CRC that correlates with ICAM-1 rs5498 may be of different ICAM-1 expression. ICAM-1 SNPs and multidrug resistance in CRC
MDR is one of the important factors leading to the failure of chemotherapy. Topo II and P-gp are MDR-associated protein and expression of them had a vital significance in chemotherapy for CRC. ICAM-1 rs5498 polymorphism was associated with MDR in CRC in a Chinese population (91). The high expression of Topo II and P-gp was observed in ICAM-1 rs5498 KK genotype, indicating that ICAM-1 rs5498 KK genotype might be associated with MDR in CRC (91).
Targeting ICAM-1 and its associated pathway might provide a new insight for treatments of CRC. However, ICAM-1 plays diverse roles in anti-tumor responses and immunity, therefore, the targeting treatments of ICAM-1 may be difficult. Blocking of ICAM-1 has been proven useful in rheumatoid arthritis (92), but targeting ICAM-1 in tumors have shown disappointing results.
Chimeric antigen receptor (CAR)-T cell therapy has shown remarkably effective in cancer treatment and ICAM-1 could be a promising target for CAR-T cells. Wei H et al. demonstrated ICAM1-specific CAR-T cells could recognize ICAM-1 expressing breast cancer cells and inhibit tumor growth in vitro and in vivo (93), which provided a reference for CAR-T cell therapy in CRC. Further, CpG-ODN (oligodeoxynucleotides, ODN) vaccination caused up-regulation of ICAM-1 on tumor-associated blood vessel endothelia leading to tumor-infiltration of T cells and tumor suppression in mouse model of pancreatic carcinoma (94). Administer cytokines is the straight way to increase inflammatory signals, but this could lead to severe adverse events, so delivery of cytokines directly to the tumor site could reduce adverse events (95). Angiogenic factors in the TME can decrease ICAM-1 expression, so targeting angiogenesis could also increase the ICAM-1 expression (96). NF-κB pathway plays a central role in ICAM-1 expression, so blocking NF-κB pathway can inhibit the ICAM-1 expression. The acai polyphenolic extract inhibited the ICAM-1 expression by targeting NF-κB pathway (97). Flubendazole, the benzimidazole derivative used in the treatment of parasitic disease, suppressed the growth of colon cells by down-regulation of NF-κB and ICAM-1 (98). Perhaps, with a better understanding of the various functions of ICAM-1 and how their expression and function are regulated, the clinical value of ICAM-1 could be revisited for the improvement of therapeutic strategies.
In this review, we provide the first comprehensive description of the knowledge regarding ICAM-1 in CRC. The pathogenesis of CRC involves various mechanisms, and ICAM-1 plays different roles. During cancer development, ICAM-1 mediates anti-tumor response including tumor antigen uptake, activation of tumor-specific T cells, leukocyte trafficking into the tumor site and tumor cell killing. ICAM-1 remains the focus of continued investigations and may serve as a promising prognostic biomarker, and a potential target for emerging therapies.
GT and YZ provided direction and guidance throughout the preparation of this manuscript. ZQ and YW wrote and edited the manuscript. WT and YX reviewed and made significant revisions to the manuscript. RQ, ZZ and YP collected and prepared the related papers. All authors contributed to the article and approved the submitted version.
This research was funded by “Liu Ge Yi Gong Cheng”of Jiangsu Province (Grant No. LGY2017022), Social Development Foundation of Zhenjiang (SH2019069) and Science Foundation of the Affiliated People’s Hospital of Jiangsu University (Y2019021-S)
The authors declare that the research was conducted in the absence of any commercial or financial relationships that could be construed as a potential conflict of interest.
All claims expressed in this article are solely those of the authors and do not necessarily represent those of their affiliated organizations, or those of the publisher, the editors and the reviewers. Any product that may be evaluated in this article, or claim that may be made by its manufacturer, is not guaranteed or endorsed by the publisher.
1. Bray F, Ferlay J, Soerjomataram I, Siegel RL, Torre LA, Jemal A. Global cancer statistics 2018: GLOBOCAN estimates of incidence and mortality worldwide for 36 cancers in 185 countries. CA Cancer J Clin (2018) 68:394–424. doi: 10.3322/caac.21492
2. Arnold M, Sierra MS, Laversanne M, Soerjomataram I, Jemal A, Bray F. Global patterns and trends in colorectal cancer incidence and mortality. Gut (2017) 66:683–91. doi: 10.1136/gutjnl-2015-310912
3. Ohene-Abuakwa Y, Pignatelli M. Adhesion molecules as diagnostic tools in tumor pathology. Int J Surg Pathol (2000) 8:191–200. doi: 10.1177/106689690000800306
4. Jung WC, Jang YJ, Kim JH, Park SS, Park SH, Kim SJ, et al. Expression of intercellular adhesion molecule-1 and e-selectin in gastric cancer and their clinical significance. J Gastric Cancer (2012) 12:140–8. doi: 10.5230/jgc.2012.12.3.140
5. Anbarasan C, Bavanilatha M, Latchumanadhas K, Ajit Mullasari S. ICAM-1 molecular mechanism and genome wide SNP’s association studies. Indian Heart J (2015) 67:282–7. doi: 10.1016/j.ihj.2015.03.005
6. Robledo O, Papaioannou A, Ochietti B, Beauchemin C, Legault D, Cantin A, et al. ICAM-1 isoforms: specific activity and sensitivity to cleavage by leukocyte elastase and cathepsin G. Eur J Immunol (2003) 33:1351–60. doi: 10.1002/eji.200323195
7. Reymond N, d’Água BB, Ridley AJ. Crossing the endothelial barrier during metastasis. Nat Rev Cancer (2013) 13:858–70. doi: 10.1038/nrc3628
8. Huang WC, Chan ST, Yang TL, Tzeng CC, Chen CC. Inhibition of ICAM-1 gene expression, monocyte adhesion and cancer cell invasion by targeting IKK complex: molecular and functional study of novel α-methylene-γ-butyrolactone derivatives. Carcinogenesis (2004) 25:1925–34. doi: 10.1093/carcin/bgh211
9. Dymicka-Piekarska V, Kemona H. Does colorectal cancer clinical advancement affect adhesion molecules (sP-selectin, sE-selectin and ICAM-1) concentration? Thromb Res (2009) 124:80–3. doi: 10.1016/j.thromres.2008.11.021
10. Lin YC, Shun CT, Wu MS, Chen CC. A novel anticancer effect of thalidomide: inhibition of intercellular adhesion molecule-1-mediated cell invasion and metastasis through suppression of nuclear factor-kappaB. Clin Cancer Res (2006) 12:7165–73. doi: 10.1158/1078-0432.CCR-06-1393
11. Roland CL, Harken AH, Sarr MG, Barnett CC Jr. ICAM-1 expression determines malignant potential of cancer. Surgery (2007) 141:705–7. doi: 10.1016/j.surg.2007.01.016
12. Hayes SH, Seigel GM. Immunoreactivity of ICAM-1 in human tumors, metastases and normal tissues. Int J Clin Exp Pathol (2009) 2:553–60.
13. Makrilia N, Kollias A, Manolopoulos L, Syrigos K. Cell adhesion molecules: role and clinical significance in cancer. Cancer Invest (2009) 27:1023–37. doi: 10.3109/07357900902769749
14. Taglia L, Matusiak D, Matkowskyj KA, Benya RV. Gastrin-releasing peptide mediates its morphogenic properties in human colon cancer by upregulating intracellular adhesion protein-1 (ICAM-1) via focal adhesion kinase. Am J Physiol Gastrointest Liver Physiol (2007) 292:G182–90. doi: 10.1152/ajpgi.00201.2006
15. Webb DS, Mostowski HS, Gerrard TL. Cytokine-induced enhancement of ICAM-1 expression results in increased vulnerability of tumor cells to monocyte-mediated lysis. J Immunol (1991) 146:3682–6.
16. Maeda K, Kang SM, Sawada T, Nishiguchi Y, Yashiro M, Ogawa Y, et al. Expression of intercellular adhesion molecule-1 and prognosis in colorectal cancer. Oncol Rep (2002) 9:511–4. doi: 10.3892/or.9.3.511
17. Mantur M, Snarska J, Koper O, Dzieciol J, Plonski A, Lemancewicz D. Serum sICAM, sVCAM and sE-selectin levels in colorectal cancer patients. Folia Histochem Cytobiol (2009) 47:621–5. doi: 10.2478/v10042-009-0077-0
18. Wimmenauer S, Keller H, Rückauer KD, Rahner S, Wolff-Vorbeck G, Kirste G, et al. Expression of CD44, ICAM-1 and n-CAM in colorectal cancer. correlation with the tumor stage and the phenotypical characteristics of tumor-infiltrating lymphocytes. Anticancer Res (1997) 17:2395–400.
19. Ksiazek K, Mikula-Pietrasik J, Catar R, Dworacki G, Winckiewicz M, Frydrychowicz M, et al. Oxidative stress-dependent increase in ICAM-1 expression promotes adhesion of colorectal and pancreatic cancers to the senescent peritoneal mesothelium. Int J Cancer (2010) 127:293–303. doi: 10.1002/ijc.25036
20. Arteta B, Lasuen N, Lopategi A, Sveinbjörnsson B, Smedsrød B, Vidal-Vanaclocha F. Colon carcinoma cell interaction with liver sinusoidal endothelium inhibits organ-specific antitumor immunity through interleukin-1-induced mannose receptor in mice. Hepatology (2010) 51:2172–82. doi: 10.1002/hep.23590
21. Zhou L, Zhang S, Zou X, Lu J, Yang X, Xu Z, et al. The β-galactoside α 2,6-sialyltranferase 1 (ST6GAL1) inhibits the colorectal cancer metastasis by stabilizing intercellular adhesion molecule-1 via sialylation. Cancer Manage Res (2019) 11:6185–99. doi: 10.2147/CMAR.S208631
22. Tachimori A, Yamada N, Sakate Y, Yashiro M, Maeda K, Ohira M, et al. Up regulation of ICAM-1 gene expression inhibits tumour growth and liver metastasis in colorectal carcinoma. Eur J Cancer (2005) 41:1802–10. doi: 10.1016/j.ejca.2005.04.036
23. Mlecnik B, Tosolini M, Charoentong P, Kirilovsky A, Bindea G, Berger A, et al. Biomolecular network reconstruction identifies T-cell homing factors associated with survival in colorectal cancer. Gastroenterology (2010) 138:1429–40. doi: 10.1053/j.gastro.2009.10.057
24. Ferrini S, Sforzini S, Cambiaggi A, Poggi A, Meazza R, Canevari S, et al. The LFA-1/ICAM cell adhesion pathway is involved in tumor-cell lysis mediated by bispecific monoclonal-antibody-targeted T lymphocytes. Int J Cancer (1994) 56:846–52. doi: 10.1002/ijc.2910560616
25. Kelly CP, O’Keane JC, Orellana J, Schroy PC, Yang S, LaMont JT, et al. Human colon cancer cells express ICAM-1 in vivo and support LFA-1-dependent lymphocyte adhesion in vitro. Am J Physiol (1992) 263:G864–70. doi: 10.1152/ajpgi.1992.263.6.G864
26. Dippold W, Wittig B, Schwaeble W, Mayet W, Meyer KH. Zum büschenfelde. expression of intercellular adhesion molecule 1 (ICAM-1, CD54) in colonic epithelial cells. Gut (1993) 34:1593–7. doi: 10.1136/gut.34.11.1593
27. Turner J, Rhee JG, Fabian DF, Lefor AT. Expression of ICAM-1 enhances in vivo lymphocyte adhesion in a murine fibrosarcoma. J Surg Oncol (1997) 66:39–44. doi: 10.1002/(SICI)1096-9098(199709)66:1<39::AID-JSO8>3.0.CO;2-O
28. Robbins PF, Kawakami Y. Human tumor antigens recognized by T cells. Curr Opin Immunol (1996) 8:628–36. doi: 10.1016/S0952-7915(96)80078-1
29. Harjunpää H, Llort Asens M, Guenther C, Fagerholm. Cell adhesion molecules SC. And their roles and regulation in the immune and tumor microenvironment. Front Immunol (2019) 10:1078. doi: 10.3389/fimmu.2019.01078
30. Zumwalde NA, Domae E, Mescher MF, Shimizu Y. ICAM-1-dependent homotypic aggregates regulate CD8 T cell effector function and differentiation during T cell activation. J Immunol (2013) 191:3681–93. doi: 10.4049/jimmunol.1201954
31. Scholer A, Hugues S, Boissonnas A, Fetler L, Amigorena S. Intercellular adhesion molecule-1-dependent stable interactions between T cells and dendritic cells determine CD8+ T cell memory. Immunity (2008) 28:258–70. doi: 10.1016/j.immuni.2007.12.016
32. Ino Y, Yamazaki-Itoh R, Shimada K, Iwasaki M, Kosuge T, Kanai Y, et al. Immune cell infiltration as an indicator of the immunemicroenvironment of pancreatic cancer. Br J Cancer (2013) 108:914–23. doi: 10.1038/bjc.2013.32
33. Fisher DT, Chen Q, Skitzki JJ, Muhitch JB, Zhou L, Appenheimer MM, et al. IL-6 trans-signaling licenses mouse and human tumor microvascular gateways for trafficking of cytotoxic T cells. J Clin Invest (2011) 121:3846–59. doi: 10.1172/JCI44952
34. Ionescu C, Braicu C, Chiorean R, Cojocneanu Petric R, Neagoe E, Pop L, et al. TIMP-1 expression in human colorectal cancer is associated with SMAD3 gene expression levels: a pilot study. J Gastrointestin Liver Dis (2014) 23:413–8. doi: 10.15403/jgld.2014.1121.234.smad
35. Schellerer VS, Langheinrich M, Hohenberger W, Croner RS, Merkeln S, Rau TT, et al. Tumor-associated fibroblasts isolated from colorectal cancer tissues exhibit increased ICAM-1 expression and affinity for monocytes. Oncol Rep (2014) 31:255–61. doi: 10.3892/or.2013.2860
36. Roebuck KA, Finnegan A. Regulation of intercellular adhesion molecule-1 (CD54) gene expression. J Leukoc Biol (1999) 66:876–88. doi: 10.1002/jlb.66.6.876
37. Look DC, Pelletier MR, Holtzman MJ. Selective interaction of a subset of interferon-gamma response element-binding proteins with the intercellular adhesion molecule-1 (ICAM-1) gene promoter controls the pattern of expression on epithelial cells. J Biol Chem (1994) 269:8952–8. doi: 10.1016/S0021-9258(17)37060-6
38. Chu LY, Hsueh YC, Cheng HL, Wu KK. Cytokine-induced autophagy promotes long-term VCAM-1 but not ICAM-1 expression by degrading late-phase IκBα. Sci Rep (2017) 7(1):12472. doi: 10.1038/s41598-017-12641-8
39. Tessitore A, Pastore L, Rispoli A, Cilenti L, Toniato E, Flati V, et al. Two γ-interferon-activation sites (GAS) on the promoter of the human intercellular adhesion molecule (ICAM-1) gene are required for induction of transcription by IFN-γ. Eur J Biochem (1998) 258(3):968–75. doi: 10.1046/j.1432-1327.1998.2580968.x
40. Lin YM, Chang ZL, Liao YY, Chou MC, Tang CH. IL-6 promotes ICAM-1 expression and cell motility in human osteosarcoma. Cancer Lett (2013) 328(1):135–43. doi: 10.1016/j.canlet.2012.08.029
41. Chuang JY, Huang YL, Yen WL, Chiang IP, Tsai MH, Tang CH. Syk/JNK/AP-1 signaling pathway mediates interleukin-6-promoted cell migration in oral squamous cell carcinoma. Int J Mol Sci (2014) 15(1):545–59. doi: 10.3390/ijms15010545
42. Yang C-M, Luo S-F, Hsieh H-L, Chi P-L, Lin C-C, Wu C-C, et al. Interleukin-1β induces ICAM-1 expression enhancing leukocyte adhesion in human rheumatoid arthritis synovial fibroblasts: involvement of ERK, JNK, AP-1, and NF-κB. J Cell Physiol (2010) 224(2):516–26. doi: 10.1002/jcp.22153
43. Ghaemmaghami S, Mohaddes SM, Hedayati M, Gorgian Mohammadi M, Dehbashi G. Resistin and visfatin expression in HCT-116 colorectal cancer cell line. Int J Mol Cell Med (2013) 2(3):143–50.
44. Benomar Y, Gertler A, de Lacy P, Crépin D, Ould Hamouda H, Riffault L, et al. Central resistin overexposure induces insulin resistance through toll-like receptor 4. Diabetes (2013) 62:102–14. doi: 10.2337/db12-0237
45. Huang WS, Yang JT, Lu CC, Chang SF, Chen CN, Su YP, et al. Fulvic acid attenuates resistin-induced adhesion of HCT-116 colorectal cancer cells to endothelial cells. Int J Mol Sci (2015) 16(12):29370–82. doi: 10.3390/ijms161226174
46. Kollmar O, Rupertus K, Scheuer C, Junker B, Tilton B, Schilling MK, et al. Stromal cell-derived factor-1 promotes cell migration and tumor growth of colorectal metastasis. Neoplasia (2007) 9:862–70. doi: 10.1593/neo.07559
47. Huang WS, Chin CC, Chen CN, Kuo YH, Chen TC, Yu HR, et al. Stromal cell-derived factor-1/CXC receptor 4 and β1 integrin interaction regulates urokinase-type plasminogen activator expression in human colorectal cancer cells. J Cell Physiol (2012) 227:1114–22. doi: 10.1002/jcp.22831
48. Jung CH, Lee WJ, Hwang JY, Seol SM, Kim YM, Lee YL, et al. The role of Rho/Rho-kinase pathway in the expression of ICAM-1 by linoleic acid in human aortic endothelial cells. Inflammation (2012) 35:1041–8. doi: 10.1007/s10753-011-9409-2
49. Dong Z, Fu S, Xu X, Yang Y, Du L, Li W, et al. Leptin-mediated regulation of ICAM-1 is Rho/ROCK dependent and enhances gastric cancer cell migration. Br J Cancer (2014) 110:1801–10. doi: 10.1038/bjc.2014.70
50. Maceyka M, Harikumar KB, Milstien S, Spiegel S. Sphingosine-1-phosphate signaling and its role in disease. Trends Cell Biol (2012) 22:50–60. doi: 10.1016/j.tcb.2011.09.003
51. Kawamori T, Kaneshiro T, Okumura M, Maalouf S, Uflacker A, Bielawski J, et al. Role for sphingosine kinase 1 in colon carcinogenesis. FASEB J (2009) 23:405–14. doi: 10.1096/fj.08-117572
52. Liu SQ, Su YJ, Qin MB, Mao YB, Huang JA, Tang GD. Sphingosine kinase 1 promotes tumor progression and confers malignancy phenotypes of colon cancer by regulating the focal adhesion kinase pathway and adhesion molecules. Int J Oncol (2013) 42(2):617–26. doi: 10.3892/ijo.2012.1733
53. Li X, Wei R, Wang M, Ma L, Zhang Z, Chen L, et al. MGP promotes colon cancer proliferation by activating the NF-κB pathway through upregulation of the calcium signaling pathway. Mol Ther Oncolytics (2020) 17:371–83. doi: 10.1016/j.omto.2020.04.005
54. Hu G, Gong AY, Liu J, Zhou R, Deng C, Chen XM. miR-221 suppresses ICAM-1 translation and regulates interferon-gamma-induced ICAM-1 expression in human cholangiocytes. Am J Physiol Gastrointest Liver Physiol (2010) 298:G542–50. doi: 10.1152/ajpgi.00490.2009
55. Duan M, Yao H, Hu G, Chen X, Lund AK, Buch S. HIV Tat induces expression of ICAM-1 in HUVECs: implications for miR-221/-222 in HIV-associated cardiomyopathy. PloS One (2013) 8:e60170. doi: 10.1371/journal.pone.0060170
56. Ueda R, Kohanbash G, Sasaki K, Fujita M, Zhu X, Kastenhuber ER, et al. Dicer-regulated microRNAs 222 and 339 promote resistance of cancer cells to cytotoxic T-lymphocytes by down-regulation of ICAM-1. Proc Natl Acad Sci USA (2009) 106(26):10746–51. doi: 10.1073/pnas.0811817106
57. Kim C, Lee H, Cho YM, Kwon OJ, Kim W, Lee EK. TNFalpha-induced miR-130 resulted in adipocyte dysfunction during obesity-related in flammation. FEBS Lett (2013) 587:3853–8. doi: 10.1016/j.febslet.2013.10.018
58. Liu RR, Li J, Gong JY, Kuang F, Liu JY, Zhang YS, et al. MicroRNA-141 regulates the expression level of ICAM-1 on endothelium to decrease myocardial ischemia-reperfusion injury. Am J Physiol Heart Circ Physiol (2015) 309:H1303–1313. doi: 10.1152/ajpheart.00290.2015
59. Yu HF, Duan P, Zhu HB, Rao DP. miR-613 inhibits bladder cancer proliferation and migration through targeting SphK1. Am J Transl Res (2017) 9(3):1213–21.
60. Wang L, Chen X, Sun X, Suo J. Long noncoding RNA LINC00460 facilitates colorectal cancer progression by negatively regulating miR-613. Onco Targets Ther (2020) 13:7555–69. doi: 10.2147/OTT.S254489
61. Li S, Fang Y, Qin H, Fu W, Zhang X. miR-659-3p is involved in the regulation of the chemotherapy response of colorectal cancer via modulating the expression of SPHK1. Am J Cancer Res (2016) 6(9):1976–85.
62. Chen MB, Yang L, Lu PH, Fu XL, Zhang Y, Zhu YQ, et al. MicroRNA-101 down-regulates sphingosine kinase 1 in colorectal cancer cells. Biochem Biophys Res Commun (2015) 463(4):954–60. doi: 10.1016/j.bbrc.2015.06.041
63. Wang MY, Bai DC, Zhu P, Fu Y, Bu DF, Zhang Y. Effects of ICAM-1 gene K469E, K56M polymorphisms on plasma sICAM-1 expression levels in Chinese yugur, Tibetan and han nationalities. Zhongguo Shi Yan Xue Ye Xue Za Zhi. (2012) 20:1205–11.
64. Wang QL, Li BH, Liu B, Liu YB, Liu YP, Miao SB, et al. Polymorphisms of the ICAM-1 exon 6 (E469K) are associated with differentiation of colorectal cancer. J Exp Clin Canc Res (2009) 28:139. doi: 10.1186/1756-9966-28-139
65. Alexiou D, Karayiannakis AJ, Syrigos KN, Zbar A, Kremmyda A, Bramis I, et al. Serum levels of e-selectin, ICAM-1 and VCAM-1 in colorectal cancer patients: correlations with clinicopathological features, patient survival and tumour surgery. Eur J Cancer (2001) 37(18):2392–7. doi: 10.1016/S0959-8049(01)00318-5
66. Yang M, Liu J, Piao C, Shao J, Du J. ICAM-1 suppresses tumor metastasis by inhibiting macrophage M2 polarization through blockade of efferocytosis. Cell Death Dis (2015) 6:e1780. doi: 10.1038/cddis.2015.144
67. Zhang S, Lu J, Xu Z, Zou X, Sun X, Xu Y, et al. Differential expression of ST6GAL1 in the tumor progression of colorectal cancer. Biochem Biophys Res Commun (2017) 486:1090–6. doi: 10.1016/j.bbrc.2017.03.167
68. Zhu Y, Srivatana U, Ullah A, Gagneja H, Berenson CS, Lance P. Suppression of a sialyltransferase by antisense DNA reduces invasiveness of human colon cancer cells in vitro. Biochim Biophys Acta (2001) 1536:148–60. doi: 10.1016/S0925-4439(01)00044-8
69. Glover S, Nathaniel R, Shakir L, Perrault C, Anderson R, Tran-Son-Tay R, et al. Transient upregulation of GRP and its receptor critically regulates colon cancer cell motility during remodeling. Am J Physiol Gastrointest Liver Physiol (2005) 288:G1274–82. doi: 10.1152/ajpgi.00108.2004
70. Patel O, Dumesny C, Giraud AS, Baldwin GS, Shulkes A. Stimulation of proliferation and migration of a colorectal cancer cell line by amidated and glycine-extended gastrin-releasing peptide via the same receptor. Biochem Pharmacol (2004) 68:2129–42. doi: 10.1016/j.bcp.2004.08.009
71. Glover S, Delaney M, Dematte C, Kornberg L, Frasco M, Tran-Son-Tay R, et al. Phosphorylation of focal adhesion kinase tyrosine 397 critically mediates gastrin-releasing peptide’s morphogenic properties. J Cell Physiol (2004) 199:77–88. doi: 10.1002/jcp.10456
72. Matkowskyj KA, Keller K, Glover S, Kornberg L, Tran-Son-Tay R, Benya RV. Expression of GRP and its receptor in well differentiated colon cancer cells correlates with the presence of focal adhesion kinase phosphorylated at tyrosines 397 and 407. J Histochem Cytochem (2003) 51:1041–8. doi: 10.1177/002215540305100807
73. Seth R, Raymond FD, Makgoba MW. Circulating ICAM-1 isoforms: diagnostic prospects for inflammatory and immune disorders. Lancet (1991) 338:83–4. doi: 10.1016/0140-6736(91)90077-3
74. Bielinski SJ, Pankow JS, Li N, Hsu FC, Adar SD, Jenny NS, et al. ICAM1 and VCAM1 polymorphisms, coronary artery calcium, and circulating levels of soluble ICAM-1: the multi-ethnic study of atherosclerosis (MESA). Atherosclerosis (2008) 201:339–44. doi: 10.1016/j.atherosclerosis.2008.02.031
75. Kang X, Wang F, Xie JD, Cao J, Xian PZ. Clinical evaluation of serum concentrations of intercellular adhesion molecule-1 in patients with colorectal cancer. World J Gastroenterol (2005) 11:4250–3. doi: 10.3748/wjg.v11.i27.4250
76. Becker JC, Dummer R, Hartmann AA, Burg G, Schmidt RE. Shedding of ICAM-1 from human melanoma cell lines induced by INF-gamma and TNF-alpha: functional consequences on cell mediated cytotoxicity. J Immunol (1991) 147:4398.
77. Toiyama Y, Miki C, Inoue Y, Okugawa Y, Koike Y, Yokoe T, et al. Soluble intercellular adhesion molecule-1 as a prognostic marker for stage II colorectal cancer patients. Ann Surg Oncol (2008) 15:1617–24. doi: 10.1245/s10434-008-9874-5
78. Dymicka-Piekarska V, Guzinska-Ustymowicz K, Kuklinski A, Kemona H. Prognostic significance of adhesion molecules (sICAM-1, sVCAM-1) and VEGF in colorectal cancer patients. Thromb Res (2012) 129:e47–50. doi: 10.1016/j.thromres.2011.12.004
79. Sanchez-Rovira P, Jimenez E, Carracedo J, Barneto IC, Ramirez R, Aranda E. Serum levels of intercellular adhesion molecule 1 (ICAM-1) in patients with colorectal cancer: inhibitory effect on cytotoxicity. Eur J Cancer (1998) 34:394–8. doi: 10.1016/S0959-8049(97)10033-8
80. Araki T, Miki C, Kusunoki M. Biological implications of circulating soluble intercellular adhesion molecule-1 in colorectal cancer patients. Scand J Gastroenterol (2001) 36:399–404. doi: 10.1080/003655201300051234
81. Basoglu M, Yildirgan MI, Taysi S, Yilmaz I, Kiziltunc A, Balik AA, et al. Levels of soluble intercellular adhesion molecule-1 and total sialic acid in serum of patients with colorectal cancer. J Surg Oncol (2003) 83(3):180–4. doi: 10.1002/jso.10257
82. Kitagawa T, Matsumoto K, Iriyama K. Serum cell adhesion molecules in patients with colorectal cancer. Surg Today (1998) 28:262–7. doi: 10.1007/s005950050118
83. Schellerer VS, Langheinrich MC, Zver V, Grützmann R, Stürzl M, Gefeller O, et al. Soluble intercellular adhesion molecule-1 is a prognostic marker in colorectal carcinoma. Int J Colorectal Disease (2019) 34:309–17. doi: 10.1007/s00384-018-3198-0
84. Yamamoto Y, Okamoto W, Makiyama A, Shitara K, Denda T, Ogura T, et al. Plasma ICAM-1 (pICAM-1) and plasma IL-8 (pIL-8) level as biomarker of metastatic colorectal cancer patients (mCRC) treated with mFOLFOX6/XELOX plus bevacizumab (BV) (WJOG7612GTR). J Clin Oncol (2018) 36:670. doi: 10.1200/JCO.2018.36.4_suppl.670
85. Becker JC, Termeer C, Schmidt RE, Brocher EB. Soluble intercellular adhesion molecule-1 inhibits MHC-restricted specific T cell/tumor interaction. J Immunol (1993) 151:7224.
86. Gho YS, Kim PN, Li HC, Elkin M, Kleinman HK. Stimulation of tumor growth by human soluble intercellular adhesion molecule-1. Cancer Res (2001) 61:4253–7.
87. Theodoropoulos G, Papaconstantinou I, Felekouras E, Nikiteas N, Karakitsos P, Panoussopoulos D, et al. Relation between common polymorphisms in genes related to inflammatory response and colorectal cancer. World J Gastroenterol (2006) 12:5037–43. doi: 10.3748/wjg.v12.i31.5037
88. Tang W, Wang Y, Chen Y, Gu H, Chen S, Kang M. Polymorphisms in the intercellular adhesion molecule 1 gene and cancer risk: a meta-analysis. Int J Clin Exp Med (2015) 8:11996–2008.
89. Zhiyuan Q, Xie Z, Qin R, Chen M, He H, Zhang Z, et al. Evaluation of ICAM-1 rs5498 and rs3093030 polymorphisms in chinese patients with colorectal cancer. DNA Cell Biol (2021) 40:384–92. doi: 10.1089/dna.2020.6089
90. Ankathil R, Mustapha MA, Aziz AAA, Shahpudin SNM, Zakaria AD, Hassan MRA, et al. Contribution of genetic polymorphisms of inflammation response genes on sporadic colorectal cancer predisposition risk in malaysian patients–a case control study. Asian Pac J Cancer Prev (2019) 20:1621–32. doi: 10.31557/APJCP.2019.20.6.1621
91. Liu LB, Liu T, Xin FZ. Correlations of ICAM-1 gene polymorphisms with susceptibility and multidrug resistance in colorectal cancer in a Chinese population. Med (Baltimore) (2017) 96:e7481. doi: 10.1097/MD.0000000000007481
92. Kavanaugh AF, Davis LS, Jain RI, Nichols LA, Norris SH, Lipsky PE. A phase I/II open label study of the safety and efficacy of an anti-ICAM-1 (intercellular adhesion molecule-1; CD54) monoclonal antibody in early rheumatoid arthritis. J Rheumatol (1996) 23(8):1338–44.
93. Wei H, Wang Z, Kuang Y, Wu Z, Zhao S, Zhang Z, et al. Intercellular adhesion molecule-1 as target for CAR-T-Cell therapy of triple-negative breast cancer. Front Immunol (2020) 11:573823. doi: 10.3389/fimmu.2020.573823
94. Garbi N, Arnold B, Gordon S, Hämmerling GJ, Ganss R. CpG motifs as proinflammatory factors render autochthonous tumors permissive for infiltration and destruction. J Immunol (2004) 172:5861–9. doi: 10.4049/jimmunol.172.10.5861
95. Peske JD, Woods AB, Engelhard VH. Control of CD8 T-cell infiltration into tumors by vasculature and microenvironment. Adv Cancer Res (2015) 128:263–307. doi: 10.1016/bs.acr.2015.05.001
96. Witkowska AM, Borawska MH. Soluble intercellular adhesion molecule-1 (sICAM-1): an overview. Eur Cytokine Netw (2004) 15:91–8.
97. Dias MM, Noratto G, Martino HS, Arbizu S, Peluzio Mdo C, Talcott S, et al. Pro-apoptotic activities of polyphenolics from açai (Euterpe oleracea martius) in human SW-480 colon cancer cells. Nutr Cancer (2014) 66(8):1394–405. doi: 10.1080/01635581.2014.956252
Keywords: ICAM-1, colorectal cancer, polymorphisms, prognosis, tumorigenesis
Citation: Qiu Z, Wang Y, Zhang Z, Qin R, Peng Y, Tang W, Xi Y, Tian G and Zhang Y (2022) Roles of intercellular cell adhesion molecule-1 (ICAM-1) in colorectal cancer: expression, functions, prognosis, tumorigenesis, polymorphisms and therapeutic implications. Front. Oncol. 12:1052672. doi: 10.3389/fonc.2022.1052672
Received: 05 October 2022; Accepted: 09 November 2022;
Published: 23 November 2022.
Edited by:
Kanjoormana Aryan Manu, Amala Cancer Research Centre, IndiaReviewed by:
Yeun-po Chiang, Downstate Health Sciences University, United StatesCopyright © 2022 Qiu, Wang, Zhang, Qin, Peng, Tang, Xi, Tian and Zhang. This is an open-access article distributed under the terms of the Creative Commons Attribution License (CC BY). The use, distribution or reproduction in other forums is permitted, provided the original author(s) and the copyright owner(s) are credited and that the original publication in this journal is cited, in accordance with accepted academic practice. No use, distribution or reproduction is permitted which does not comply with these terms.
*Correspondence: Guangyu Tian, OTgyOTg3MTMwQHFxLmNvbQ==; Yeqing Zhang, NTk1NDQ3NzM0QHFxLmNvbQ==
†These authors have contributed equally to this work
Disclaimer: All claims expressed in this article are solely those of the authors and do not necessarily represent those of their affiliated organizations, or those of the publisher, the editors and the reviewers. Any product that may be evaluated in this article or claim that may be made by its manufacturer is not guaranteed or endorsed by the publisher.
Research integrity at Frontiers
Learn more about the work of our research integrity team to safeguard the quality of each article we publish.