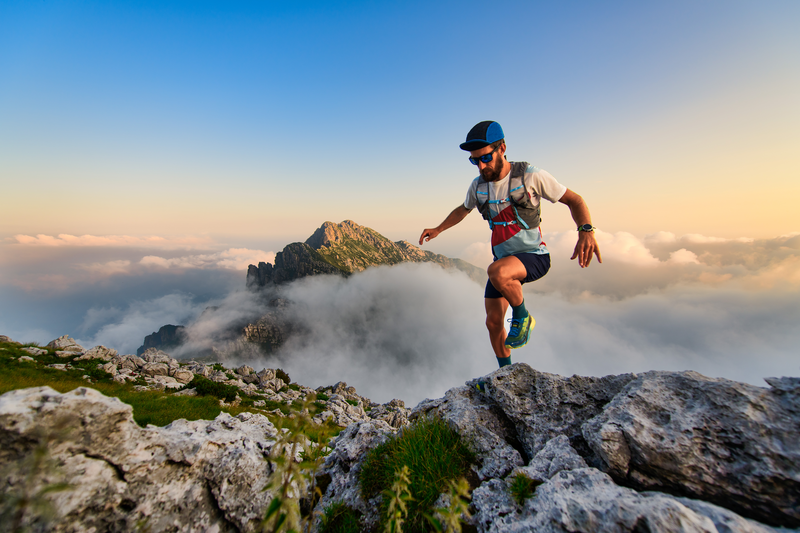
94% of researchers rate our articles as excellent or good
Learn more about the work of our research integrity team to safeguard the quality of each article we publish.
Find out more
REVIEW article
Front. Oncol. , 25 October 2022
Sec. Breast Cancer
Volume 12 - 2022 | https://doi.org/10.3389/fonc.2022.1043771
This article is part of the Research Topic The Tumor and Microenvironment Crosstalk in Breast Cancer: From Biology to Therapeutic Opportunity View all 12 articles
Breast cancer is the most common malignant disease in female patients worldwide and can spread to almost every place in the human body, most frequently metastasizing to lymph nodes, bones, lungs, liver and brain. The liver is a common metastatic location for solid cancers as a whole, and it is also the third most common metastatic site for breast cancer. Breast cancer liver metastasis (BCLM) is a complex process. Although the hepatic microenvironment and liver sinusoidal structure are crucial factors for the initial arrest of breast cancer and progression within the liver, the biological basis of BCLM remains to be elucidated. Importantly, further understanding of the interaction between breast cancer cells and hepatic microenvironment in the liver metastasis of breast cancer will suggest ways for the development of effective therapy and prevention strategies for BCLM. In this review, we provide an overview of the recent advances in the understanding of the molecular mechanisms of the hepatic microenvironment in BCLM formation and discuss current systemic therapies for treating patients with BCLM as well as potential therapeutic development based on the liver microenvironment-associated signaling proteins governing BCLM.
Breast cancer (BC) is the most common malignant disease in female patients worldwide (1, 2). Intrinsic BC subtypes by gene expression profiling include luminal A, luminal B, luminal/human epithelial growth factor receptor 2 (HER-2), HER-2 enriched, basal-like, and triple-negative (TN) non-basal (3). Currently, the 5-year survival rate for BC is over 90%. However, about 50% of patients diagnosed with BC will develop distant metastases (4), and the 5-year survival rate declines to less than 20% once distant metastases have developed (5, 6).
BC can spread to almost every place in human body, most frequently metastasizing to lymph nodes, bones, lungs, liver, and brain (7–9). The liver is one of the most common metastatic locations for solid malignant tumors, and it is also the third common metastatic organ for BC (10). Patients with breast cancer liver metastasis (BCLM) often suffer deterioration of liver function due to the aggravation of BC burden, which will threaten the lives of BC patients (11). The survival is only 4-8 months if BCLM is left untreated (12). Therefore, the treatment of BCLM is a significant issue globally. Thus far, no standard therapy has been established for BCLM (13). Currently, the treatments for BCLM include chemotherapy, immunotherapy (triple negative disease), targeted systemic therapies including endocrine therapy (luminal subtype), HER-2 target therapy (HER-2 enriched subtype), radiotherapy, and palliative therapy (11, 14). However, patients with BCLM frequently exhibit poor response to the current therapies and experience high mortality rates (15).
BCLM is a complex process. Its biological basis has not been well delineated. It has been found that the hepatic microenvironment plays a significant role in BCLM (16). An understanding of hepatic microenvironment in the liver colonization of metastatic BC cells is essential for developing novel and effective therapy for BCLM. In this review, we provide an overview of recent advances in molecular mechanisms of the hepatic microenvironment in BCLM formation and discuss current systemic therapies as well as potential therapeutic development based on the liver microenvironment-associated signaling proteins governing BCLM.
It is a long-standing observation that different subtypes of BC show distinct propensity of metastasizing to specific organs (3, 17, 18). Luminal breast cancer (LBC) preferentially metastasizes to the bones, while HER-2 and basal-like BC often develop visceral metastases including brain, liver and lung metastasis (19). Some studies report that the HER-2 enriched subtype found to exhibit a higher risk of developing liver metastasis (18, 20, 21). In contrast, other studies report that basal-like BC has a lower rate of liver metastasis (3). Although there are some discrepancies in reports about preferential organ sites of breast cancer metastasis, it is now accepted that particular metastatic sites are associated with different breast cancer subtypes (22).
It is well-established that preferred metastatic sites are mechanistically determined by molecular, cellular and microenvironment factors rather than random dissemination (23). Features of organ circulation may have a key role in determining the sites of metastatic disease as capillary networks in tissue arrest the circulating BC cells (10). Organs that receive similar amounts of blood and circulating tumor cells show differing abilities to accommodate disseminating BC cells and form metastases. This finding indicates that the “mechanical arrest” may not be the only explanation for organ tropism of BC metastasis (24, 25). Another explanation is the “seed and soil” hypothesis, which proposes the metastases form only when the disseminated BC cells are compatible to the distant organ microenvironment (26). The ability of BC cells to interact with tissue resident cells and the microenvironment factors may also determine the metastatic organs of BC (24). Therefore, the crosstalk between BC cells and liver tissue components provides key mechanisms that dictate BCLM (27–29). In this review, we will describe and summarize our current knowledge on the BCLM process.
The formation of BCLM involves a series of complex biological processes. The BC cells will undergo epithelial-to-mesenchymal transition (EMT), detach from the primary tumor, and intravasate through endothelial barriers into the blood circulation system (30, 31). Macrophages and mesenchymal stem cells (MSCs) contribute to EMT at primary BC. Cancer-associated fibroblasts (CAFs) and myeloid progenitor cells are recruited to invasive edge of primary BC and promote intravasation (31). Platelets are also involved in the survival of BC cells in circulation to extravasation sites (31). After circulating tumor cells extravasate into the parenchyma of the liver (32),they will enter a dormant state or form clinically detectable macrometastases (33, 34). BCLM is considered to comprise multi-steps: 1) The intravasation phase; 2) The premetastatic phase (35–37); 3) the tumor-infiltrating microvascular phase; 4) the pre-angiogenic micrometastatic phase; 5) the angiogenic micrometastatic phase; 6) the growth phase (38). The process of BCLM is summarized in Table 1 and Figure 1.
Figure 1 The process of liver metastasis formation in breast cancer. The whole process can be separated into six steps: 1) Intravasation phase: cancer cells intravasate into the circulation system under help of immune cells; 2) Premetastatic phase: HSCs, KCs, and immune cells in hepatic microenvironment form premetastatic niche; 3) Tumor-infiltrating microvascular phase: cancer cells extravasate into liver parenchyma through LSECs; 4) Pre-angiogenic micrometastatic phase: HSCs and immune cells are recruited into micrometastases and activate local stromal response; 5) Angiogenic micrometastatic phase: micrometastases become vascularized and interact with cells in the microenvironment; 6) Growth phase: metastases expansion under the stimulation of hepatocytes, HSCs, and immune cells. (HSC, Hepatic stellate cell; KC, Kupffer cell; LSEC, Liver sinusoidal endothelial cells).
Of the aforementioned steps, the “premetastatic phase” is essential for organ-specific metastasis formation and has recently gained much attention. Numerous studies have proposed that primary tumor-derived secreting factors are associated with the premetastatic niche formation in distant organs (36, 66). The vascular endothlial growth factor (VEGF) and transforming growth factor-β (TGF-β) show critical roles in premetastatic niche formation to promote BC metastasis (41–43). Moreover, chronic psychological stress can promote metastatic colonization of circulating BC cells by promoting a premetastatic niche through activating β-adrenergic signaling (36). BC secreted exosomes can fuse preferentially with organ-specific cells at their predicted destination to prepare the premetastatic niche and exosomal integrins can be used to predict organ-specific metastasis (44). In the liver, exosomes secreted from BC cells can reach liver and fuse with Kupffer cells to form premetastatic niche (44). Exosomal integrin αvβ5 uptake by Kupffer cells can induce Src phosphorylation and S100 gene expression to determine liver metastasis (44). Tumor-derived tissue inhibitor of metalloproteinases 1 (TIMP-1) was also found to induce liver metastasis via hepatic stromal cell derived factor 1 (SDF-1) and neutrophil recruitment (45). Despite these recent discoveries, the roles and mechanisms of premetastatic step in BCLM are currently not well understood.
The hepatic microenvironment into which disseminated BC cells invade and colonize is pivotal for the formation of BCLM. The hepatic microenvironment is highly regulated, relying heavily on the interaction between BC cells and resident cell populations (67). These interactions help nurture liver tissue to become fertile grounds for tumor cell seeding. The roles of different types of cells in the microenvironment of liver metastases are summarized in Table 2 and described below.
Cancer stem cells (CSCs) can interact with the hepatic microenvironment such as extracellular matrix, hypoxia or growth factors, all of which contribute to the metastasis (70). Knaack et al. cultured pancreatic cancer CSCs in vitro together with hepatic stellate cells and myofibroblasts to demonstrate the importance of these stromal cells in liver metastasis formation (71), indicating a connection between CSCs and liver microenvironment. Furthermore, Zhang et al. showed the CD44high/CD24- breast CSC population can activate TGF-β1 signaling and increase the invasive capacity and liver metastasis of BC (68). In line with this study, the cell surface adhesion molecule CD44 was found to enhance breast tumor invasion and metastasis to the liver (69).
When breast tumor cells enter the hepatic microcirculation, they first encounter liver sinusoidal endothelial cells (LSECs). LSECs are double-edged swords, as they can not only promote but also inhibit BCLM formation in the hepatic tissue microenvironment.
Regarding the tumoricidal activities of LSECs, many studies have shown that the tumor cells can obstruct the sinusoids to trigger an ischemia and inflammatory response. LSECs release cytotoxic cytokines, which have damaging effects in adjacent tumor cells (73–77). LSECs can also remove or degrade the enzymes that promote angiogenesis and metastasis (78). Whether the tumoricidal action of LSECs exert a prominent effect on BCLM remains to be determined.
On the other hand, tumor cells can activate Kupffer cells (KCs) to secrete proinflammatory cytokines, which induce LSECs to express adhesion molecules and help tumor cells extravasate into hepatic parenchyma (49–51, 72). Also, LSECs allow tumor cells directly adhere to the membrane proteins and promote metastasis (105). Although these findings came from studies on colorectal cancer or lung cancer, it is postulated that LSECs also possess tumor-promoting activities in BCLM.
Furthermore, some critical molecules from LSECs or their surrounding microenvironment are involved in liver metastasis (64). The expression of chemokine (C-X-C motif) receptor 4(CXCR4) in cancer cells is associated with increased expression of chemokine ligand CXCL12 in LSECs’ microenvironment and CXCR4-CXCL12 signaling drives metastasis (64). Intercellular adhesion molecule 1 (ICAM-1), signal transducer and activator of transcription (STAT)3, programmed cell death-ligand 1 (PD-L1), and microRNA-20a with its targeted proteins expressed by LSECs also play a pivotal role in the interaction between LSECs and cancer cells and thereby promote liver metastasis (64).
The main function of hepatocytes is helping metastatic BC cells in seeding and colonization of the liver. BC cells can directly interact with hepatocytes by forming tight-junction-like complexes with hepatocytes in the Disse space, the space between hepatocytes and sinusoids (83). Interestingly, metastatic BC cells exhibit a lower adherent ability with LSECs compared to the hepatocytes (52), suggesting that hepatocytes facilitate BC cell seeding in the liver.
Hepatocytes can release growth factors, such as insulin-like growth factor 1 (IGF-1) and hepatocyte growth factor (HGF) which promote liver metastasis (53). The overexpression of RON receptor has been reported in BC and RON can be activated by HGF-like protein secreted by hepatocytes. RON activation promote cancer cells growth, invasion and metastasis (53).
Liver macrophages can be divided into monocyte-derived recruited macrophages and liver resident KCs (84). The M1 to M2 repolarization of tumor-associated macrophages (TAMs) can prevent immunogenic, inflammatory responses while inducing neoangiogenesis and matrix remodeling, thus promoting breast cancer progression and metastasis (57, 58). It has been demonstrated that the EMT of BC cells is regulated by M2 macrophages in the liver metastatic microenvironment (39).
KCs, unlike monocyte-derived recruited macrophages, are permanent resident monocytes in the sinusoids. They can fuse with exosomes derived from BC cells and contribute to the premetastatic niche formation (44). On one hand, KCs can exhibit tumoricidal activity by releasing reactive oxygen species (ROS), cytotoxic cytokines, proteases, and recruitment of other inflammatory cells (85, 87, 88), particularly when the burden of tumor cells invading liver is excessive. The anti-tumor activity of KCs might base on the reruitment of natural killer (NK) cells by secreting inflammatory factors granulocyte macrophage colony stimulating factor (GM-CSF) and interferonγ(IFN-γ) (53). KCs can also decrease metastatic BC by increasing level of tumor necrosis factor (TNF)-α and interleukin (IL)-1β (53). On the other hand, KCs can also promote liver metastasis through secreting growth factors and cytokines including HGF, VEGF, IL-6, matrix metalloproteinase (MMP)9 and MMP14, as demonstrated in colorectal cancer studies (53, 59–61). However, whether KCs have these dichotomous effects in BCLM awaits to be examined.
The cancer-associated fibroblasts (CAFs) in the hepatic microenvironment are widely considered to be derived from hepatic stellate cells (HSCs) (106). It was found that HSCs can be induced to trans-differentiate into a proliferative and motile form called myofibroblasts by growth factors released from tumor cells or KCs during the development of micrometastases (107, 108). Functionally, activation of HSCs promotes liver metastasis by enhancing tumor cell adhesion, invasion, survival, and proliferation (56). Activated HSCs can also initiate angiogenesis by organizing endothelial cells (ECs) into a neovascular network and inducing LSECs and ECs to form vascular tubes within metastases (62, 63). Vascular endothlial growth factor receptor (VEGFR) are mainly distributed on the endothelial surface of tumor vessels, inhibit VEGFR can significantly suppress liver metastasis of BC. Hypoxia induced factor (HIF)-1 can activate TWIST and promote the binding of VEGF to VEGFR to contribute BCLM (92).
In addition, activated HSCs suppress antitumor immune response by inducing T cell apoptosis and releasing TGF-β (46, 93), consistent with the well-established notion that immune suppression by CAFs is mediated by CXCL12 or nicotinamide adenine dinucleotide phosphate oxidase 4 (NOX4), leading to exclusion of CD8+ T cells85,86. Notably, HSCs can modify the extracellular matrix (ECM), thereby facilitating or impairing BC cell migration and invasion (89, 90). Relaxin (RLN), an anti-fibrosis peptide in liver tissue preferentially target metastatic BC cells and activated HSCs. The increased expression of RLN can inhibit BCLM, where RLN gene might be a novel target for treating BCLM (92).
Neutrophils are innate immune cells. Clinical studies have demonstrated that increased neutrophil-to-lymphocyte ratio or immune-infl;ammation index is associated with poor survival in BC patients (109–111). Neutrophils can inhibit tumor growth through releasing cytolytic factors and recruiting CD8+ cytotoxic T cells or macrophages in the hepatic microenvironment (40, 54, 99). On the other hand, neutrophils have also been shown to promote cancer progression and metastasis via distinct mechanisms. For example, they can anchor circulating BC cells and enhance migration of BC cells. The tumor-interacting neutrophils may promote BCLM in a CD90-TIMP-1 juxtacrine-paracrine manner (55). Of note, a recent report showed that aged neutrophils can robustly enhance BCLM through releasing neutrophil extracellular traps, reactive oxygen species, VEGFs, and MMP-9 (96), which are involved in the well-known neutrophil response to infection and injury.
The myeloid-derived suppressor cells (MDSC) and regulatory T cells (Tregs) are known as immunosuppressive cells, and they induce an immune tolerance state that permits tumor growth by evading T-cell-mediated killing (47, 48, 65, 112). MDSCs can be recruited to the liver metastasis site by chemokines released by LSECs, KCs and HSCs (100). These MDSCs, especially S100A8/Gr1-positive MDSCs (101), can enhance the growth and aggressiveness of BC cells and consequently liver metastasis by producing arginase and IL-6 (102–104). Much effort has gone into the development of approaches to eliminate MDSCs, but these attempts have been met with little success (113). Notably, studies have shown that Tregs can exacerbate the development of liver metastasis in intra-abdominal malignancies (114). A direct relationship between Treg accumulation and BCLM has not been reported yet, and there remains an intriguing question as to the role of Tregs in BCLM.
Liver metastatic cancer cells spread through the systemic circulation and therefore liver metastases are rarely isolated (115). Only about 5-10% of patients with BCLM have isolated metastases confined to the liver with no evidence of metastatic disease at other sites (12, 116). The median survival time of untreated patients with BCLM is limited to a few months and is dependent on several prognostic factors (12, 117).
Survival of patients with metastatic BC is affected by many different clinical features, including age, race, marital status, performance status, tumor size, lymph node status, number of metastatic sites, history of treatments, and subtype (118). For BCLM patients, Eichbaum et al. found a prognostic benefit for BCLM patients who were hormone receptor (HR)+ and had an expression of Ki-67 <20% and p53 <50% (119). Similarly, in a review of 4,285 BCLM patients, Xie et al. found that those with the HR+/HER-2+ subtype had the longest median survival of 31.0 months. Patients who were HR-/HER-2+ had a median survival of 22.0 months, and those who had triple negative breast cancer (TNBC) had the shortest median survival of 8.0 months (120). It has been observed that patients with TNBC have the lowest survival after liver metastases in many other studies as well, given the lack of effective therapy (121, 122). In general, factors that may predict worse survival after liver metastasis include the triple negative phenotype, time from curative therapy to liver metastases, burden of tumor cells, and high histological grade of primary (115, 123, 124).
To date, there are few studies on prognostic molecular markers for patients with BCLM. Tian et al. showed that mutations in AKT1, ESR1, ERBB2, FGFR4, APOBEC cytidine deaminase, and defective DNA mismatch repair were significant genetic determinants for BCLM development and progression (125). The study by Yang et al. found that the PPFIA1 gene was markedly elevated in BCLM and associated with decreased disease-free survival (DFS) in HR+ BCLM patients (126). In HER-2+ BC patients, mutant CCND1 (P241P) and PIK3CA (E542K) led to significantly reduced DFS (127).
Systemic therapy remains the cornerstone of BCLM management. However, BCLM patients who are treated with systemic therapy have poor survival, particularly for triple negative or HR- subtypes (128). If treated with chemotherapy alone, the median survival of BC patients with solely liver metastasis or with limited disease elsewhere is between 19 months (with pre-taxane chemotherapy regimens) to 22-26 months (with taxane-containing regimens) (129). The five-year overall survival (OS) of patients with BCLM treated with systemic therapy is 8-12% (117, 130). It is highly likely that new systemic therapies recently approved for TNBC and HER-2+ BC may improve clinical outcomes in patients with BCLM.
For patients with non-TNBC subtypes, there are options for targeted systemic therapies. In the setting of metastatic HER-2+ tumors, trastuzumab in combination with systemic therapy is associated with longer OS and progression-free survival (PFS) compared to those treated with systemic therapy (14, 131, 132). Rossi et al. found that in patients with metastatic HER-2+ BC who were treated with trastuzumab and had liver-lung metastases (n=328), 4-year survival was 32.1% (133). Further, there has been significant innovation in HER-2-directed therapies in recent years. Currently, there are two Food and Drug Administration (FDA)-approved HER-2-directed antibody-drug conjugates (ADCs), trastuzumane-emtansine (T-DM1) and trastuzumab-deruxtecan (T-DXd), for HER-2+ metastatic BC (134). Interestingly, the DESTINY-Breast04 clinical trial showed that T-DXd also prolonged PFS and OS in HER-2-low metastatic BC patients than chemotherapy (135). Recently, the third HER-2-directed ADC, disitamab vedotin (RC48), received approval for treatment of metastatic gastric or gastroesophageal junction cancer in China in 2021. It may also soon become a treatment modality for HER-2+ metastatic BC (134). At present, there are a total of 11 ADCs that target HER family receptors in clinical trials.
In terms of HR+ disease, endocrine therapy in combination with cyclin-dependent kinase 4 & 6 inhibitors have shown to be effective in patients with both bone-only and visceral metastases (136). He et al. reviewed HR+ patients who were treated with fulvestrant. Fifty-one patients had liver-only metastases, and their PFS was 3.7 months (137). Recently, the SOLAR-1 trial revealed that the alpelisib plus fulvestrant treatment had a significantly benefit in median OS (37.2 months) compared to fulvestrant alone (22.8 months) for PIK3CA-mutant/HR+/HER-2- BC patients with lung and/or liver metastasis (138). Further, several new oral bioavailable selective estrogen receptor modulators/degraders (SERMs/SERDs), including lasfoxifene, bazedoxifene, LSZ102, and RAD1901, for ESR1 gene mutation are currently under clinical investigations to treat ESR1-mutant or endocrine therapy resistant metastatic BC (139). It remains to be determined whether SERMs/SERDs are effective treatment modalities for BCLM.
In contrast to other BC subtypes, there is no first-line targeted therapy for TNBC. The clinical impact of chemotherapy as the standard treatment of TNBC is limited (140). Sacituzumab govitecan (SG) is an antibody-drug conjugate composed of antibody targeting human trophoblast cell-surface antigen 2 (Trop-2), coupled to topoisomerase I inhibitor (SN-38) via a proprietary hydrolyzable linker. In ASCENT study, 42% of patients had liver metastasis. The median PFS (5.6 months) and OS (12.1 months) of metastatic TNBC patients in the SG group were significantly longer than in the chemotherapy group (141). Furthermore, immune checkpoint inhibitors (ICIs) in several clinical trials have shown positive results for treating metastatic BC recently (142). A phase III trial (KEYNOTE-355) utilized pembrolizumab plus chemotherapy to treat metastatic TNBC. For patients with PD-L1 expression (combined positive score [CPS] ≥10), median PFS was 9.7 months in pembrolizumab group vs. 5.6 months in placebo group (143). Another study used pembrolizumab and capecitabine for metastatic BC (144). However, metastatic BC only had moderate response to ICIs. PD-L1 positive, first-line therapy, high tumor-infiltrating lymphocytes, and high CD8+ T cell infiltrating are associated with better response to ICIs (142). BCLM reportedly had a lower response rate to ICI treatment when compared with the other metastatic locations (142). Therefore, exploring new targets and developing more effective therapy for BC with liver metastasis are urgently needed and would have tremendous clinical impact.
Given the critical roles of different cells in the hepatic microenvironment in BCLM formation, it is tempting to explore novel therapeutic approaches based on available interventional agents targeting the key signaling proteins in these cells. These potential treatment options for BCLM are summarized in Table 3 and described below.
Table 3 Hepatic microenvironment related therapeutic implications in breast cancer liver metastasis.
For BC stem cells in the hepatic microenvironment, the cell surface adhesion molecule CD44 has been shown to potentiate the invasion and metastasis of BC cells to the liver (68, 69). These studies suggest that CD44 may be a novel target for inhibiting BCLM. One phase I study utilized bivatuzumab mertansiene to treat metastatic BC patients with positive CD44v6, and estimated the pharmacokinetics and safety of the treatment (145). This study demonstrated that the bivatuzumab mertansine targeting CD44v6 could be a potential therapeutic option for metastatic BC patients that express CD44v6 (145).
Claudin-2 is a molecule that plays a key role in the formation of tight junctions. Previous studies have shown that liver metastatic BC cells express high levels of Claudin-2 and the protein is critical for the adhesion between BC cells and hepatocytes by acting as an adhesion molecule (52). Afadin, a Claudin-2-interacting partner, is also involved in BC cell metastasis to the liver (79). Furthermore, the Claudin-2 expressed by liver metastatic BC cells can increase the expression of integrin complexes in the surface of BC cells, leading to enhanced adhesion of BC cells to ECM components, such as type IV collagen and fibronectin (80). Although primary human BC samples express low levels of Claudin-2, most of the liver metastatic BC samples were found to display higher expression levels of Claudin-2 (80). Further supporting the significant role of Claudin-2 in BCLM formation, Tabaries et al. demonstrated that blocking tumor-hepatocyte interactions by inhibiting Claudin-2 expression using the Lyn-selective kinase inhibitor Bafetinib (INNO-406) can suppress BCLM growth (81). Currently, most studies on Bafetinib are in the preclinical stage, and there are a few phase I and phase II clinical trials of Befetinib in chronic leukemia, prostate cancer, and brain cancer.
The epithelial cell adhesion E-cadherin may also play an important role in BC cell interaction with hepatocytes. BC cells, which undergo EMT to escape from primary tumors, have been shown to re-express E-cadherin upon entering the liver microenvironment (82). This upregulation contributes to the adhesion with hepatocytes and promotes BC cell survival by activating extracellular regulated protein kinases (ERK) signaling (82, 146). Although the clinical relevance of breast tumor-hepatocyte or breast tumor-ECM interactions has not been well evaluated, disruption of interactions between BC cells and hepatocytes or ECM may serve as a potential strategy to inhibit BCLM. Furthermore, results of some preclinical studies suggest that ROS1 inhibitors, such as crizotinib, may be utilized to treat E-cadherin defective BC. The preclinical data provided theoretical basis to support the phase II clinical trials to evaluate the safety and efficacy of ROS1 inhibitors in E-cadherin defective BC patients (147). Currently, ROS1 inhibitors are widely used to treat lung cancer patients with ROS1 mutations, and there are also phase I and phase II clinical trials for ROS1 inhibitors to treat patients with other advanced or metastatic solid tumors.
For macrophages, targeting M2 macrophage polarization has been proposed as an anti-cancer treatment approach. The STAT6, a key effector and mediator of IL-4 and IL-13 function, is a potential therapeutic target in this regard (58). Notably, phospholipase D-2 (PLD-2) is an important player in BC progression and metastasis. In preclinical studies, PLD inhibitors [FIPI (dual PLD1/PLD2 inhibitor) or VU0155072-2 (PLD2 inhibitor)] were found to reduce the tumor-promoting macrophages and neutrophil infiltration in primary breast tumors and liver metastasis, thereby suppressing BCLM (86). In addition, Cao et al. showed that cabazitaxel could affect macrophages and improve the immunotherapy targeting CD47 in TNBC (148). The activation of macrophages by cabazitaxel combined with the CD47 blocking effect could drastically enhance the tumoricidal activity against TNBC cells, thus suppressing BCLM (148). Cabazitaxel is widely utilized to treat patients with metastatic castration resistant prostate cancer. Several phase I and phase II trial studies have shown the efficacy of cabazitaxel in metastatic BC patients so far (149). One recently study showed an increased NK cells in dormant heptic microenvironment. Interleukin-15 based immunotherapy could ensure a large number of NK cells to maintain dormancy and prevent BCLM through interferon-γ signalling (94). Actived HSCs secreted chemokine CXCL12 could induce NK cells quiescence through CXCR4 to promote BCLM (94). Normalizing NK cell pool might be a novel way to prevent BCLM.
For neutrophils, the immature low-density neutrophils (iLDNs) mobilized by cancer cell-derived granulocyte colony stimulating factor (G-CSF) can promote BCLM (150), and the liver metastatic growth may be facilitated by neutrophil-derived transferrin in BC (97). The neutrophil or the transferrin receptors depletion could inhibit transferrin production in the metastatic microenvironment and suppress BC metastasis (97). Furthermore, P53 may be a key regulator of pro-metastatic neutrophils. It was shown that blockade of Wnt secretion by LGK974, a Porcupine inhibitor blocking acylation of Wnt, or shRNA in p53-null BC cells reverse subsequent neutrophilic inflammation, resulting in reduced BCLM growth (98). To date, the WNT inhibitor LGK974 is under a phase I clinical study in patients with malignancies (151). Recently, Wang et al. found that the KIAA1199 could promote immunosuppressive neutrophils to infiltrate into the liver microenvironment, which indicated that KIAA1199 might be a potential therapeutic target to treat the liver metastasis (152). In addition, KIAA1199 carries out its function through the TGFβ-CXCL3/1-CXCR2 signaling pathway. Restoration of immune infiltration in the liver metastasis microenvironment can potentially be achieved by inhibiting KIAA1199 pharmacologically, and thereby allowing for suppression of liver metastasis and enhancement the response to ICI treatment (152). Further in vivo studies and clinical trials are needed to establish the utility of KIAA1199 inhibition in the treatment of BC with liver metastasis.
Although recent advances in the understanding of the molecular mechanisms of hepatic microenvironment in BCLM using various in vitro and in vivo models shed light on potential therapeutic targets, much work is needed to tie these findings to clinical relevance. Concomitantly, identification of biomarkers to predict BCLM risk, progression, treatment response, and patient survival will have a significant clinical impact. Further in-depth investigation of critical pathways and genetic changes underlying human BCLM would pave the way for developing new approaches for preventing and treating BCLM.
All authors listed have made a substantial, direct, and intellectual contribution to the work and approved it for publication.
XC is supported by National Institutes of Health (2R01CA151610), Department of Defense (W81XWH-18-1-0067) and Samuel Oschin Cancer Institute research development fund. AG is supported by the Fashion Footwear Charitable Foundation of New York, Inc., the Margie and Robert E. Petersen Foundation, and Linda and Jim Lippman Fund. This study is also supported by the National Natural Science Foundation of China (No. 81602189) and CSCO-Genecast Cancer Precision Therapy Research Fund (Y-2019Genecast-039). The funder was not involved in the study design, collection, analysis, interpretation of data, the writing of this article or the decision to submit it for publication.
Author RB has a consulting role for Navartis, Biotheranostics, AstraZeneca, Seattle Genetics and Gilead Inc. She is the honoraria of MJH Healthcare, WebMD, and AstraZeneca, and also a speaker for Eli Lilly. She is supported by the research fundings from Merck, Seattle Genetics, Takeda, Pfizer, and Eli Lilly.
The remaining authors declare that the research was conducted in the absence of any commercial or financial relationships that could be construed as a potential conflict of interest.
All claims expressed in this article are solely those of the authors and do not necessarily represent those of their affiliated organizations, or those of the publisher, the editors and the reviewers. Any product that may be evaluated in this article, or claim that may be made by its manufacturer, is not guaranteed or endorsed by the publisher.
1. Jemal A, Center MM, DeSantis C, Ward EM. Global patterns of cancer incidence and mortality rates and trends. Cancer Epidemiol Biomarkers Prev (2010) 19(8):1893–907. doi: 10.1158/1055-9965.EPI-10-0437
2. Diaby V, Tawk R, Sanogo V, Xiao H, Montero AJ. A review of systematic reviews of the cost-effectiveness of hormone therapy, chemotherapy, and targeted therapy for breast cancer. Breast Cancer Res Treat (2015) 151(1):27–40. doi: 10.1007/s10549-015-3383-6
3. Kennecke H, Yerushalmi R, Woods R, Cheang MCU, Voduc D, Speers CH, et al. Metastatic behavior of breast cancer subtypes. J Clin Oncol (2010) 28(20):3271–7. doi: 10.1200/JCO.2009.25.9820
4. Atalay G, Biganzoli L, Renard F, Paridaens R, Cufer T, Coleman R, et al. Clinical outcome of breast cancer patients with liver metastases alone in the anthracycline-taxane era: a retrospective analysis of two prospective, randomized metastatic breast cancer trials. Eur J Cancer. (2003) 39(17):2439–49. doi: 10.1016/S0959-8049(03)00601-4
5. Siegel R, Naishadham D, Jemal A. Cancer statistics, 2012. CA Cancer J Clin (2012) 62(1):10–29. doi: 10.3322/caac.20138
6. Gennari A, Conte P, Rosso R, Orlandini C, Bruzzi P. Survival of metastatic breast carcinoma patients over a 20-y period: a retrospective analysis based on individual patient data from six consecutive studies. Cancer (2005) 104(8):1742–50. doi: 10.1002/cncr.21359
7. Valastyan S, Weinberg RA. Tumor metastasis: molecular insights and evolving paradigms. Cell (2011) 147(2):275–92. doi: 10.1016/j.cell.2011.09.024
8. Eckhardt BL, Francis PA, Parker BS, Anderson RL. Strategies for the discovery and development of therapies for metastatic breast cancer. Nat Rev Drug Discovery (2012) 11(6):479–97. doi: 10.1038/nrd2372
9. Cao H, Zhang Z, Zhao S, He X, Yu H, Yin Q, et al. Hydrophobic interaction mediating self-assembled nanoparticles of succinobucol suppress lung metastasis of breast cancer by inhibition of VCAM-1 expression. J Control Release. (2015) 205:162–71. doi: 10.1016/j.jconrel.2015.01.015
10. Hess KR, Varadhachary GR, Taylor SH, Wei W, Raber MN, Lenzi R, et al. Metastatic patterns in adenocarcinoma. Cancer (2006) 106(7):1624–33. doi: 10.1002/cncr.21778
11. Diamond JR, Finlayson CA, Borges VF. Hepatic complications of breast cancer. Lancet Oncol (2009) 10(6):615–21. doi: 10.1016/S1470-2045(09)70029-4
12. Adam R, Aloia T, Krissat J, Bralet MP, Paule B, Giacchetti S, et al. Is liver resection justified for patients with hepatic metastases from breast cancer? Ann Surg (2006) 244(6):897–907. doi: 10.1097/01.sla.0000246847.02058.1b
13. Alcantara SB, Reed W, Willis K, Lee W, Brennan P, Lewis S. Radiologist participation in multi-disciplinary teams in breast cancer improves reflective practice, decision making and isolation. Eur J Cancer Care Engl (2014) 23(5):616–23. doi: 10.1111/ecc.12169
14. Hortobagyi GN. Trastuzumab in the treatment of breast cancer. N Engl J Med (2005) 353(16):1734–6. doi: 10.1056/NEJMe058196
15. Miller WR. Aromatase inhibitors: prediction of response and nature of resistance. Expert Opin Pharmacother. (2010) 11(11):1873–87. doi: 10.1517/14656566.2010.487863
16. Ma R, Feng Y, Chen J, Lin H, Liang X, Zheng H, et al. Mechanisms involved in breast cancer liver metastasis. J Transl Med (2015) 13:64. doi: 10.1186/s12967-015-0425-0
17. Lu X, Kang Y. Organotropism of breast cancer metastasis. J Mammary Gland Biol Neoplasia. (2007) 12(2-3):153–62. doi: 10.1007/s10911-007-9047-3
18. Bartmann C, Wischnewsky M, Stüber T, Stein R, Krockenberger M, Häusler S, et al. Pattern of metastatic spread and subcategories of breast cancer. Arch Gynecol Obstet. (2017) 295(1):211–23. doi: 10.1007/s00404-016-4225-4
19. Foulkes WD, Smith IE, Reis-Filho JS. Triple-negative breast cancer. N Engl J Med (2010) 363(20):1938–48. doi: 10.1056/NEJMra1001389
20. Smid M, Zhang Y, Sieuwerts AM, Yu J, Klijn JG, Foekens JA, et al. Subtypes of breast cancer show preferential site of relapse. Cancer Res (2008) 68(9):3108–14. doi: 10.1158/0008-5472.CAN-07-5644
21. Gerratana L, Fanotto V, Bonotto M, Bolzonello S, Minisini AM, Fasola G, et al. Pattern of metastasis and outcome in patients with breast cancer. Clin Exp Metastasis. (2015) 32(2):125–33. doi: 10.1007/s10585-015-9697-2
22. Jin L, Han B, Siegel E, Cui Y, Giuliano A, Cui X. Breast cancer lung metastasis: Molecular biology and therapeutic implications. Cancer Biol Ther (2018) 19(10):858–68. doi: 10.1080/15384047.2018.1456599
23. Yousefi M, Nosrati R, Salmaninejad A, Dehghani S, Shahryari A, Saberi A. Organ-specific metastasis of breast cancer: Molecular and cellular mechanisms underlying lung metastasis. Cell Oncol (Dordr) (2018) 41(2):123–40. doi: 10.1007/s13402-018-0376-6
24. Obenauf AC, Massague J. Surviving at a distance: organ specific metastasis. Trends Cancer. (2015) 1(1):76–91. doi: 10.1016/j.trecan.2015.07.009
25. Yousefi M, Bahrami T, Salmaninejad A, Nosrati R, Ghaffari P. S.H. ghaffari. lung cancer-associated brain metastasis: Molecular mechanisms and therapeutic options. Cell Oncol (Dordr). (2017) 40(5):419–41. doi: 10.1007/s13402-017-0345-5
26. Fidler IJ. The pathogenesis of cancer metastasis: the ‘seed and soil’ hypothesis revisited. Nat Rev Cancer. (2003) 3(6):453–8. doi: 10.1038/nrc1098
27. Park SY, Nam JS. The force awakens: metastatic dormant cancer cells. Exp Mol Med (2020) 52(4):569–81. doi: 10.1038/s12276-020-0423-z
28. Liu Y, Cao X. Characteristics and significance of the pre-metastatic niche. Cancer Cell (2016) 30(5):668–81. doi: 10.1016/j.ccell.2016.09.011
29. Mo Z, Cheong JYA, Xiang L, Le MTN, Grimson A, Zhang DX. Extracellular vesicle-associated organotropic metastasis. Cell Prolif. (2021) 54(1):e12948. doi: 10.1111/cpr.12948
30. Nieto MA. Epithelial plasticity: a common theme in embryonic and cancer cells. Science (2013) 342(6159):1234850. doi: 10.1126/science.1234850
31. Quail DF, Joyce JA. Microenvironmental regulation of tumor progression and metastasis. Nat Med (2013) 19(11):1423–37. doi: 10.1038/nm.3394
32. Wells A, Yates C, Shepard CR. E-cadherin as an indicator of mesenchymal to epithelial reverting transitions during the metastatic seeding of disseminated carcinomas. Clin Exp Metastasis. (2008) 25(6):621–8. doi: 10.1007/s10585-008-9167-1
33. Gunasinghe NP, Wells A, Thompson EW, Hugo HJ. Mesenchymal-epithelial transition (MET) as a mechanism for metastatic colonization in breast cancer. Cancer Metastasis Rev (2012) 31(3-4):469–78. doi: 10.1007/s10555-012-9377-5
34. Wells A, Grahovac J, Wheeler S, Ma B, Lauffenburger D. Targeting tumor cell motility as a strategy against invasion and metastasis. Trends Pharmacol Sci (2013) 34(5):283–9. doi: 10.1016/j.tips.2013.03.001
35. Brodt P. Role of the microenvironment in liver metastasis: From pre- to prometastatic niches. Clin Cancer Res (2016) 22(24):5971–82. doi: 10.1158/1078-0432.CCR-16-0460
36. Chen H, Liu D, Guo L, Cheng X, Guo N, Shi M. Chronic psychological stress promotes lung metastatic colonization of circulating breast cancer cells by decorating a pre-metastatic niche through activating β-adrenergic signaling. J Pathol (2018) 244(1):49–60. doi: 10.1002/path.4988
37. Peinado Héctor, Zhang H, Matei IR, Costa-Silva B, Hoshino A, Rodrigues G, et al. Pre-metastatic niches: Organ-specific homes for metastases. Nat Rev Cancer. (2017) 17(5):302–17. doi: 10.1038/nrc.2017.6
38. Vidal-Vanaclocha F. The prometastatic microenvironment of the liver. Cancer Microenviron. (2008) 1(1):113–29. doi: 10.1007/s12307-008-0011-6
39. Yang M, Ma Bo, Shao H, Clark AM, Wells A. Macrophage phenotypic subtypes diametrically regulate epithelial-mesenchymal plasticity in breast cancer cells. BMC Cancer. (2016) 16:419. doi: 10.1186/s12885-016-2411-1
40. Sionov RV, Fridlender ZG, Granot Z. The multifaceted roles neutrophils play in the tumor microenvironment. Cancer Microenviron. (2015) 8(3):125–58. doi: 10.1007/s12307-014-0147-5
41. Liu S, Jiang M, Zhao Q, Li S, Peng Y, Zhang P, et al. Vascular endothelial growth factor plays a critical role in the formation of the pre-metastatic niches via prostaglandin E2. Oncol Rep (2014) 32(6):2477–84. doi: 10.3892/or.2014.3516
42. Ye Y, Liu S, Wu C, Sun Z. TGFβ modulates inflammatory cytokines and growth factors to creat premetastatic microenvironment and stimulate lung metastasis. J Mol Histol. (2015) 46(4-5):365–75. doi: 10.1007/s10735-015-9633-4
43. Meng Du, Meng M, Luo A, Jing X, Wang G, Huang S, et al. Effects of VEGFR1+ hematopoietic progenitor cells on pre-metastatic niche formation and in vivo metastasis of breast cancer cells. J Cancer Res Clin Oncol (2019) 145(2):411–27. doi: 10.1007/s00432-018-2802-6
44. Hoshino A, Costa-Silva B, Shen TL, Rodrigues G, Hashimoto A, Tesic Mark M, et al. Tumour exosome integrins determine organotropic metastasis. NatureV (2015) 527(7578):329–35. doi: 10.1038/nature15756
45. Seubert B, Grunwald B, Kobuch J, Cui H, Schelter F, Schaten S, et al. Tissue inhibitor of metalloproteinases (TIMP)-1 creates a premetastatic niche in the liver through SDF-1/CXCR4-dependent neutrophil recruitment in mice. Hepatology (2015) 61(1):238–48. doi: 10.1002/hep.27378
46. Yingling JM, Blanchard KL, Sawyer JS. Development of TGF-beta signaling inhibitors for cancer therapy. Nat Rev Drug Discovery (2004) 3(12):1011–22. doi: 10.1038/nrd1580
47. Hadrup S, Donia M, Thor Straten P. Effector CD4 and CD8 T cells and their role in the tumor microenvironment. Cancer Microenviron. (2013) 6(2):123–33. doi: 10.1007/s12307-012-0127-6
48. Butt AQ, Mills KH. Immunosuppressive networks and checkpoints controlling antitumor immunity and their blockade in the development of cancer immunotherapeutics and vaccines. Oncogene (2014) 33(38):4623–31. doi: 10.1038/onc.2013.432
49. Auguste P, Fallavollita L, Wang N, Burnier J, Bikfalvi A, Brodt P. The host inflammatory response promotes liver metastasis by increasing tumor cell arrest and extravasation. Am J Pathol (2007) 170(5):1781–92. doi: 10.2353/ajpath.2007.060886
50. Khatib AM, Auguste P, Fallavollita L, Wang N, Samani A, Kontogiannea M, et al. Characterization of the host proinflammatory response to tumor cells during the initial stages of liver metastasis. Am J Pathol (2005) 167(3):749–59. doi: 10.1016/S0002-9440(10)62048-2
51. Laferriere J, Houle F, Taher MM, Valerie K, Huot J. Transendothelial migration of colon carcinoma cells requires expression of e-selectin by endothelial cells and activation of stress-activated protein kinase-2 (SAPK2/p38) in the tumor cells. J Biol Chem (2001) 276(36):33762–72. doi: 10.1074/jbc.M008564200
52. Tabaries S, Dupuy F, Dong Z, Monast A, Annis MG, Spicer J, et al. Claudin-2 promotes breast cancer liver metastasis by facilitating tumor cell interactions with hepatocytes. Mol Cell Biol (2012) 32(15):2979–91. doi: 10.1128/MCB.00299-12
53. Mielgo A, Schmid MC. Liver tropism in cancer: The hepatic metastatic niche. Cold Spring Harb Perspect Med (2020) 10(3):a037259. doi: 10.1101/cshperspect.a037259
54. Spicer JD, McDonald B, Cools-Lartigue JJ, Chow SC, Giannias B, Kubes P, et al. Neutrophils promote liver metastasis via mac-1-mediated interactions with circulating tumor cells. Cancer Res (2012) 72(16):3919–27. doi: 10.1158/0008-5472.CAN-11-2393
55. Wang Y, Chen J, Yang L, Li J, Wu W, Huang M, et al. Tumor-contacted neutrophils promote metastasis by a CD90-TIMP-1 juxtacrine-paracrine loop. Clin Cancer Res (2019) 25(6):1957–69. doi: 10.1158/1078-0432.CCR-18-2544
56. Kang N, Gores GJ, Shah VH. Hepatic stellate cells: partners in crime for liver metastases? Hepatology (2011) 54(2):707–13. doi: 10.1002/hep.24384
57. Xie L, Yang Y, Meng J, Wen T, Liu J, Xu H. Cationic polysaccharide spermine-pullulan drives tumor associated macrophage towards M1 phenotype to inhibit tumor progression. Int J Biol Macromol. (2019) 123:1012–9. doi: 10.1016/j.ijbiomac.2018.11.089
58. Binnemars-Postma K, Bansal R, Storm G, Prakash J. Targeting the Stat6 pathway in tumor-associated macrophages reduces tumor growth and metastatic niche formation in breast cancer. FASEB J (2018) 32(2):969–78. doi: 10.1096/fj.201700629R
59. Bayon LG, Izquierdo MA, Sirovich I, van Rooijen N, Beelen RH, Meijer S. Role of kupffer cells in arresting circulating tumor cells and controlling metastatic growth in the liver. Hepatology (1996) 23(5):1224–31. doi: 10.1002/hep.510230542
60. Wen SW, Ager EI, Christophi C. Bimodal role of kupffer cells during colorectal cancer liver metastasis. Cancer Biol Ther (2013) 14(7):606–13. doi: 10.4161/cbt.24593
61. Thomas P, Hayashi H, Zimmer R, Forse RA. Regulation of cytokine production in carcinoembryonic antigen stimulated kupffer cells by beta-2 adrenergic receptors: Implications for hepatic metastasis. Cancer Lett (2004) 209(2):251–7. doi: 10.1016/j.canlet.2003.12.027
62. Eveno C, Hainaud P, Rampanou A, Bonnin P, Bakhouche S, Dupuy E, et al. Proof of prometastatic niche induction by hepatic stellate cells. J Surg Res (2015) 194(2):496–504. doi: 10.1016/j.jss.2014.11.005
63. Wirz W, Antoine M, Tag CG, Gressner AM, Korff T, Hellerbrand C, et al. Hepatic stellate cells display a functional vascular smooth muscle cell phenotype in a three-dimensional co-culture model with endothelial cells. Differentiation (2008) 76(7):784–94. doi: 10.1111/j.1432-0436.2007.00260.x
64. Yang M, Zhang C. The role of liver sinusoidal endothelial cells in cancer liver metastasis. Am J Cancer Res (2021) 11(5):1845–60.
65. Facciabene A, Motz GT, Coukos G. T-Regulatory cells: key players in tumor immune escape and angiogenesis. Cancer Res (2012) 72(9):2162–71. doi: 10.1158/0008-5472.CAN-11-3687
66. Wortzel I, Dror S, Kenific CM, Lyden D. Exosome-mediated metastasis: Communication from a distance. Dev Cell (2019) 49(3):347–60. doi: 10.1016/j.devcel.2019.04.011
67. Ingber DE. Can cancer be reversed by engineering the tumor microenvironment? Semin Cancer Biol (2008) 18(5):356–64. doi: 10.1016/j.semcancer.2008.03.016
68. Zhang C, Gao H, Li C, Tu J, Chen Z, Su W, et al. TGFβ1 promotes breast cancer local invasion and liver metastasis by increasing the CD44high/CD24- subpopulation. Technol Cancer Res Treat (2018) 17:1533033818764497. doi: 10.1177/1533033818764497
69. Ouhtit A, Elmageed ZYA, Abdraboh ME, Lioe TF, Raj MHG. In vivo evidence for the role of CD44s in promoting breast cancer metastasis to the liver. Am J Pathol (2007) 171(6):2033–9. doi: 10.2353/ajpath.2007.070535
70. Lau EY, Ho NP, Lee TK. Cancer stem cells and their microenvironment: biology and therapeutic implications. Stem Cells Int (2017) 2017:3714190. doi: 10.1155/2017/3714190
71. Knaack H, Lenk L, Philipp LM, Miarka L, Rahn S, Viol F, et al. Liver metastasis of pancreatic cancer: the hepatic microenvironment impacts differentiation and self-renewal capacity of pancreatic ductal epithelial cells. Oncotarget (2018) 9(60):31771–86. doi: 10.18632/oncotarget.25884
72. Ou J, Peng Y, Deng J, Miao H, Zhou J, Zha L, et al. Endothelial cell-derived fibronectin extra domain a promotes colorectal cancer metastasis via inducing epithelial-mesenchymal transition. Carcinogenesis (2014) 35(7):1661–70. doi: 10.1093/carcin/bgu090
73. Balkwill F. Tumour necrosis factor and cancer. Nat Rev Cancer. (2009) 9(5):361–71. doi: 10.1038/nrc2628
74. Braet F, Nagatsuma K, Saito M, Soon L, Wisse E, Matsuura T. The hepatic sinusoidal endothelial lining and colorectal liver metastases. World J Gastroenterol (2007) 13(6):821–5. doi: 10.3748/wjg.v13.i6.821
75. Hehlgans T, Pfeffer K. The intriguing biology of the tumour necrosis factor/tumour necrosis factor receptor superfamily: players, rules and the games. Immunology (2005) 115(1):1–20. doi: 10.1111/j.1365-2567.2005.02143.x
76. Wang HH, McIntosh AR, Hasinoff BB, Rector ES, Ahmed N, Nance DM, et al. B16 melanoma cell arrest in themouse liver induces nitric oxide release and sinusoidal cytotoxicity: a natural hepatic defense against metastasis. Cancer Res (2000) 60(20):5862–9.
77. Yanagida H, Kaibori M, Yoshida H, Habara K, Yamada M, Kamiyama Y, et al. Hepatic ischemia/reperfusion upregulates the susceptibility of hepatocytes to confer the induction of inducible nitric oxide synthase gene expression. Shock (2006) 26(2):162–8. doi: 10.1097/01.shk.0000223130.87382.73
78. Jansen S, Andries M, Vekemans K, Vanbilloen H, Verbruggen A, Bollen M. Rapid clearance of the circulating metastatic factor autotaxin by the scavenger receptors of liver sinusoidal endothelial cells. Cancer Lett (2009) 284(2):216–21. doi: 10.1016/j.canlet.2009.04.029
79. Tabariès Sébastien, McNulty A, Ouellet Véronique, Annis MG, Dessureault M, Vinette M, et al. Afadin cooperates with claudin-2 to promote breast cancer metastasis. Genes Dev (2019) 33(3-4):180–93. doi: 10.1101/gad.319194.118
80. Tabariès S, Dong Z, Annis MG, Omeroglu A, Pepin F, Ouellet V, et al. Claudin-2 is selectively enriched in and promotes the formation of breast cancer liver metastases through engagement of integrin complexes. Oncogene (2011) 30(11):1318–28. doi: 10.1038/onc.2010.518
81. Tabaries S, Annis MG, Hsu BE, Tam CE, Savage P, Park M, et al. Lyn Modulates claudin-2 expression and is a therapeutic target for breast cancer liver metastasis. Oncotarget (2015) 6(11):9476–87. doi: 10.18632/oncotarget.3269
82. Kowalski PJ, Rubin MA, Kleer CG. E-cadherin expression in primary carcinomas of the breast and its distant metastases. Breast Cancer Res (2003) 5(6):R217–22. doi: 10.1186/bcr651
83. Roos E, Van de Pavert IV, Middelkoop OP. Infiltration of tumour cells into cultures of isolated hepatocytes. J Cell Sci (1981) 47:385–97. doi: 10.1242/jcs.47.1.385
84. Shapouri-Moghaddam A, Mohammadian S, Vazini H, Taghadosi M, Esmaeili SA, Mardani F, et al. Macrophage plasticity, polarization, and function in health and disease. J Cell Physiol (2018) 233(9):6425–40. doi: 10.1002/jcp.26429
85. Seki S, Habu Y, Kawamura T, Takeda K, Dobashi H, Ohkawa T, et al. The liver as a crucial organ in the first line of host defense: the roles of kupffer cells, natural killer (NK) cells and NK1.1 ag+ tcells in T helper 1 immune responses. Immunol Rev (2000) 174:35–46. doi: 10.1034/j.1600-0528.2002.017404.x
86. Henkels KM, Muppani NR, Gomez-Cambronero J. PLD-specific small-molecule inhibitors decrease tumor-associated macrophages and neutrophils infiltration in breast tumors and lung and liver metastases. PloS One (2016) 11(11):e0166553. doi: 10.1371/journal.pone.0166553
87. Timmers M, Vekemans K, Vermijlen D, Asosingh K, Kuppen P, Bouwens L, et al. Interactions between rat colon carcinoma cells and kupffer cells during the onset of hepatic metastasis. Int J Cancer. (2004) 112(5):793–802. doi: 10.1002/ijc.20481
88. Su W, Kitagawa T, Ito T, Oyama T, Lee CM, Kim YK, et al. Antitumor effect to IL-12 administration into the portal vein on murine liver metastasis. J Hepato Biliary Pancreatic Surg (2002) 9(4):503–10. doi: 10.1007/s005340200063
89. Fearon DT. The carcinoma-associated fibroblast expressing fibroblast activation protein and escape from immune surveillance. Cancer Immunol Res (2014) 2(3):187–93. doi: 10.1158/2326-6066.CIR-14-0002
90. Ford K, Hanley CJ, Mellone M, Szyndralewiez C, Heitz F, Wiesel P, et al. NOX4 inhibition potentiates immunotherapy by overcoming cancer-associated fibroblast-mediated CD8 T-cell exclusion from tumors. Cancer Res (2020) 80(9):1846–60. doi: 10.1158/0008-5472.CAN-19-3158
91. Eble JA, Niland S. The extracellular matrix in tumor progression and metastasis. Clin Exp Metastasis (2019) 36(3):171–98. doi: 10.1007/s10585-019-09966-1
92. Liu H, Li X, Li H, Feng L, Sun G, Sun G, et al. Potential molecular mechanisms and clinical progress in liver metastasis of breast cancer. BioMed Pharmacother. (2022) 149:112824. doi: 10.1016/j.biopha.2022.112824
93. Yu MC, Chen CH, Liang X, Wang L, Gandhi CR, Fung JJ, et al. Inhibition of T-cell responses by hepatic stellate cells via B7-H1- mediated T-cell apoptosis in mice. Hepatology (2004) 40(6):1312–21. doi: 10.1002/hep.20488
94. Correia AL, Guimaraes JC, Auf der Maur P, De Silva D, Trefny MP, Okamoto R, et al. Hepatic stellate cells suppress NK cell-sustained breast cancer dormancy. Nature (2021) 594(7864):566–71. doi: 10.1038/s41586-021-03614-z
95. Cortes E, Lachowski D, Rice A, Chronopoulos A, Robinson B, Thorpe S, et al. Retinoic acid receptor-β is downregulated in hepatocellular carcinoma and cirrhosis and its expression inhibits myosin-driven activation and durotaxis in hepatic stellate cells. Hepatology (2019) 69(2):785–802. doi: 10.1002/hep.30193
96. Peng Z, Liu C, Victor AR, Cao DY, Veiras LC, Bernstein EA, et al. Tumors exploit CXCR4hiCD62Llo aged neutrophils to facilitate metastatic spread. Oncoimmunology (2021) 10(1):1870811. doi: 10.1080/2162402X.2020.1870811
97. Liang W, Li Q, Ferrara N. Metastatic growth instructed by neutrophil-derived transferrin. Proc Natl Acad Sci U S A. (2018) 115(43):11060–5. doi: 10.1073/pnas.1811717115
98. Wellenstein MD, Coffelt SB, Duits DEM, Miltenburg MHv, Slagter M, Rink Ide, et al. Loss of p53 triggers WNT-dependent systemic inflammation to drive breast cancer metastasis. Nature (2019) 572(7770):538–42. doi: 10.1038/s41586-019-1450-6
99. McDonald B, Spicer J, Giannais B, Fallavollita L, Brodt P, Ferri LE. Systemic inflammation increases cancer cell adhesion to hepatic sinusoids by neutrophil mediated mechanisms. Int J Cancer. (2009) 125(6):1298–305. doi: 10.1002/ijc.24409
100. Zhao W, Zhang L, Xu Y, Zhang Z, Ren G, Tang K, et al. Hepatic stellate cells promote tumor progression by enhancement of immunosuppressive cells in an orthotopic liver tumor mouse model. Lab Invest. (2014) 94(2):182–91. doi: 10.1038/labinvest.2013.139
101. Tanriover G, Eyinc MB, Aliyev E, Dilmac S, Erin N. Presence of S100A8/Gr1-positive myeloid-derived suppressor cells in primary tumors and visceral organs invaded by breast carcinoma cells. Clin Breast Cancer (2018) 18(5):e1067–76. doi: 10.1016/j.clbc.2018.03.013
102. Keskinov AA, Shurin MR. Myeloid regulatory cells in tumor spreading and metastasis. Immunobiology (2015) 220(2):236–42. doi: 10.1016/j.imbio.2014.07.017
103. Kusmartsev S, Nefedova Y, Yoder D, Gabrilovich DI. Antigen-specific inhibition of CD8+ T cell response by immaturemyeloid cells in cancer is mediated by reactive oxygen species. J Immunol (2004) 172(2):989–99. doi: 10.4049/jimmunol.172.2.989
104. Oh K, Lee O-Y, Shon SY, Nam O, Ryu PoM, Seo MW, et al. A mutual activation loop between breast cancer cells and myeloid-derived suppressor cells facilitates spontaneous metastasis through IL-6 trans-signaling in a murine model. Breast Cancer Res (2013) 15(5):R79. doi: 10.1186/bcr3473
105. Enomoto K, Nishikawa Y, Omori Y, Tokairin T, Yoshida M, Ohi N, et al. Cell biology and pathology of liver sinusoidal endothelial cells. Med Electron Microsc. (2004) 37(4):208–15. doi: 10.1007/s00795-004-0261-4
106. Affo S, Yu L-X, Schwabe RF. The role of cancer-associated fibroblasts and fibrosis in liver cancer. Annu Rev Pathol (2017) 12:153–86. doi: 10.1146/annurev-pathol-052016-100322
107. Olaso E, Santisteban A, Bidaurrazaga J, Gressner AM, Rosenbaum J, Vidal-Vanaclocha F. Tumor-dependent activation of rodent hepatic stellate cells during experimental melanoma metastasis. Hepatology (1997) 26(3):634–42. doi: 10.1002/hep.510260315
108. Dou C, Liu Z, Tu K, Zhang H, Chen C, Yaqoob U, et al. P300 acetyltransferase mediates stiffness-induced activation of hepatic stellate cells into tumor-promoting myofibroblasts. Gastroenterology (2018) 154(8):2209–21. doi: 10.1053/j.gastro.2018.02.015
109. Qiu X, Song Y, Cui Y, Liu Y. Increased neutrophil–lymphocyte ratio independently predicts poor survival in non-metastatic triple-negative breast cancer patients. IUBMB Life (2018) 70(6):529–35. doi: 10.1002/iub.1745
110. Sun Yi, Li W, Li A-J, Su H, Yue J, Yu J. Increased systemic immune-infl;ammation index independently predicts poor survival for hormone receptor-negative, HER2-positive breast cancer patients. Cancer Manag Res (2019) 11:3153–62. doi: 10.2147/CMAR.S190335
111. Imamura M, Morimoto T, Egawa C, Fukui R, Bun A, Ozawa H, et al. Significance of baseline neutrophil-to-lymphocyte ratio for progression-free survival of patients with HER2-positive breast cancer treated with trastuzumab emtansine. Sci Rep (2019) 9(1):1811. doi: 10.1038/s41598-018-37633-0
112. Gabrilovich DI, Ostrand-Rosenberg S, Bronte V. Coordinated regulation of myeloid cells by tumours. Nat Rev Immunol (2012) 12(4):253–68. doi: 10.1038/nri3175
113. Ugel S, Delpozzo F, Desantis G, Papalini F, Simonato F, Sonda N, et al. Therapeutic targeting of myeloid-derived suppressor cells. Curr Opin Pharmacol (2009) 9(4):470–81. doi: 10.1016/j.coph.2009.06.014
114. Connolly MK, Clair JM-S, Bedrosian AS, Malhotra A, Vera V, Ibrahim J, et al. Distinct populations of metastases-enabling myeloid cells expand in the liver of mice harboring invasive and preinvasive intraabdominal tumor. J Leuk Biol (2010) 87(4):713–25. doi: 10.1189/jlb.0909607
115. Golse N, Adam R. Liver metastases from breast cancer: What role for surgery? indications and results. Clin Breast Cancer (2017) 17(4):256–65. doi: 10.1016/j.clbc.2016.12.012
116. He X, Zhang Q, Feng Y, Li Z, Pan Q, Xhao Y, et al. Resection of liver metastases from breast cancer: a multicentre analysis. Clin Transl Oncol (2020) 22(4):512–21. doi: 10.1007/s12094-019-02155-2
117. Yoo TG, Cranshaw I, Broom R, Pandanaboyana S, Bartlett A. Systematic review of early and long-term outcome of liver resection for metastatic breast cancer: Is there a survival benefit? Breast (2017) 32:162–72. doi: 10.1016/j.breast.2017.02.003
118. Wang R, Zhu Y, Liu X, Liao X, He J, Niu L. The clinicopathological features and survival outcomes of patients with different metastatic sites in stage IV breast cancer. BMC Cancer. (2019) 19(1):1091. doi: 10.1186/s12885-019-6311-z
119. Eichbaum MH, Kaltwasser M, Bruckner T, de Rossi TM, Schneeweiss A, Sohn C. Prognostic factors for patients with liver metastases from breast cancer. Breast Cancer Res Treat (2006) 96(1):53–62. doi: 10.1007/s10549-005-9039-1
120. Xie J, Xu Z. A population-based study on liver metastases in women with newly diagnosed breast cancer. Cancer Epidemiol Biomarkers Prev (2019) 28(2):283–92. doi: 10.1158/1055-9965.EPI-18-0591
121. Chen QF, Huang T, Shen L, Wu P, Huang ZL, Li W. Prognostic factors and survival according to tumor subtype in newly diagnosed breast cancer with liver metastases: A competing risk analysis. Mol Clin Oncol (2019) 11(3):259–69. doi: 10.3892/mco.2019.1890
122. Dittmar Y, Altendorf-Hofmann A, Schule S, Ardelt M, Dirsch O, Runnebaum IB, et al. Liver resection in selected patients with metastatic breast cancer: a single-centre analysis and review of literature. J Cancer Res Clin Oncol (2013) 139(8):1317–25. doi: 10.1007/s00432-013-1440-2
123. Duan XF, Dong NN, Zhang T, Li Q. The prognostic analysis of clinical breast cancer subtypes among patients with liver metastases from breast cancer. Int J Clin Oncol (2013) 18(1):26–32. doi: 10.1007/s10147-011-0336-x
124. Zegarac M, Nikolic S, Gavrilovic D, Kolarevic D, Jevric M, Nikolic-Tomasevic Z, et al. Prognostic importance of steroid receptor status for disease free and overall survival after surgical resection of isolated liver metastasis in breast cancer patients. J BUON. (2017) 22(1):192–9.
125. Tian C, Liu S, Wang Y, Song X. Prognosis and genomic landscape of liver metastasis in patients with breast cancer. Front Oncol (2021) 11:588136. doi: 10.3389/fonc.2021.588136
126. Yang J, Wu NN, Huang DJ, Luo YC, Huang JZ, He HY, et al. PPFIA1 is upregulated in liver metastasis of breast cancer and is a potential poor prognostic indicator of metastatic relapse. Tumour Biol (2017) 39(7):1010428317713492. doi: 10.1177/1010428317713492
127. Singla H, Kaur RP, Shafi G, Vashistha R, Banipal RPS, Kumar V, et al. Genomic alterations associated with HER2+ breast cancer risk and clinical outcome in response to trastuzumab. Mol Biol Rep (2019) 46(1):823–31. doi: 10.1007/s11033-018-4537-5
128. Paluch-Shimon S, Ben-Baruch N, Wolf I, Zach L, Kopolovic J, Kruglikova A, et al. Hormone receptor expression is associated with a unique pattern of metastatic spread and increased survival among HER2-overexpressing breast cancer patients. Am J Clin Oncol (2009) 32(5):504–8. doi: 10.1097/COC.0b013e3181967d72
129. Singletary SE, Walsh G, Vauthey JN, Curley S, Sawaya R, Weber KL, et al. A role for curative surgery in the treatment of selected patients with metastatic breast cancer. Oncologist (2003) 8(3):241–51. doi: 10.1634/theoncologist.8-3-241
130. Er O, Frye DK, Kau SW, Broglio K, Valero V, Hortobagyi GN, et al. Clinical course of breast cancer patients with metastases limited to the liver treated with chemotherapy. Cancer J (2008) 14(1):62–8. doi: 10.1097/PPO.0b013e3181629a7b
131. Del Mastro L, Lambertini M, Bighin C, Levaggi A, D’Alonzo A. Trastuzumab as first-line therapy in HER2-positive metastatic breast cancer patients. Expert Rev Anticancer Ther (2012) 12(11):1391–405. doi: 10.1586/era.12.107
132. Balduzzi S, Mantarro S, Guarneri V, Tagliabue L, Pistotti V, Moja L, et al. Trastuzumab-containing regimens for metastatic breast cancer. Cochrane Database Syst Rev (2014) 6):CD006242. doi: 10.1002/14651858.CD006242.pub2
133. Rossi M, Carioli G, Bonifazi M, Zambelli A, Franchi M, Moja L, et al. Trastuzumab for HER2+ metastatic breast cancer in clinical practice: Cardiotoxicity and overall survival. Eur J Cancer. (2016) 52:41–9. doi: 10.1016/j.ejca.2015.09.012
134. Yu J, Fang T, Yun C, Liu X, Cai X. Antibody-drug conjugates targeting the human epidermal growth factor receptor family in cancers. Front Mol Biosci (2022) 9:847835. doi: 10.3389/fmolb.2022.847835
135. Modi S, Jacot W, Yamashita T, Sohn J, Vidal M, Tokunaga E, et al. Trastuzumab deruxtecan in previously treated HER2-low advanced breast cancer. N Engl J Med (2022) 387(1):9–20. doi: 10.1056/NEJMoa2203690
136. Di Leo A, O’Shaughnessy J, Sledge GW, Martin M, Lin Y, Frenzel M, et al. Prognostic characteristics in hormone receptor-positive advanced breast cancer and characterization of abemaciclib efficacy. NPJ Breast Cancer. (2018) 4:41. doi: 10.1038/s41523-018-0094-2
137. He M, Li JJ, Zuo WJ, Ji L, Jiang YZ, Hu XC, et al. Metastatic breast cancer patients with lung or liver metastases should be distinguished before being treated with fulvestrant. Cancer Med (2019) 8(14):6212–20. doi: 10.1002/cam4.2453
138. André F, Ciruelos EM, Juric D, Loibl S, Campone M, Mayer IA, et al. Alpelisib plus fulvestrant for PIK3CA-mutated, hormone receptor-positive, human epidermal growth factor receptor-2-negative advanced breast cancer: final overall survival results from SOLAR-1. Ann Oncol (2021) 32(2):208–17. doi: 10.1016/j.annonc.2020.11.011
139. Hernando C, Ortega-Morillo B, Tapia M, Moragón S, Martínez MT, Eroles P, et al. Oral selective estrogen receptor degraders (SERDs) as a novel breast cancer therapy: Present and future from a clinical perspective. Int J Mol Sci (2021) 22(15):7812. doi: 10.3390/ijms22157812
140. Senkus E, Cardoso F, Pagani O. Time for more optimism in metastatic breast cancer? Cancer Treat Rev (2014) 40(2):220–8. doi: 10.1016/j.ctrv.2013.09.015
141. Bardia A, Hurvitz SA, Tolaney SM, Loirat D, Punie K, Oliveira M, et al. Sacituzumab govitecan in metastatic triple-negative breast cancer. N Engl J Med (2021) 384(16):1529–41. doi: 10.1056/NEJMoa2028485
142. Zou Y, Zou X, Zheng S, Tang H, Zhang L, Liu P, et al. Efficacy and predictive factors of immune checkpoint inhibitors in metastatic breast cancer: a systematic review and meta-analysis. Ther Adv Med Oncol (2020) 12:1758835920940928. doi: 10.1177/1758835920940928
143. Cortes J, Cescon DW, Rugo HS, Nowecki Z, Im SA, Yusof MM, et al. Pembrolizumab plus chemotherapy versus placebo plus chemotherapy for previously untreated locally recurrent inoperable or metastatic triple-negative breast cancer (KEYNOTE-355): a randomised, placebo-controlled, double-blind, phase 3 clinical trial. Lancet (2020) 396(10265):1817–28. doi: 10.1016/S0140-6736(20)32531-9
144. Shah AN, Flaum L, Helenowski I, Santa-Maria CA, Jain S, Rademaker A, et al. Phase II study of pembrolizumab and capecitabine for triple negative and hormone receptor-positive, HER2-negative endocrine-refractory metastatic breast cancer. J Immunother Cancer. (2020) 8(1):e000173. doi: 10.1136/jitc-2019-000173
145. Rupp U, Schoendorf-Holland E, Eichbaum M, Schuetz F, Lauschner I, Schmidt P, et al. Safety and pharmacokinetics of bivatuzumab mertansine in patients with CD44v6-positive metastatic breast cancer: final results of a phase I study. Anticancer Drugs (2007) 18(4):477–85. doi: 10.1097/CAD.0b013e32801403f4
146. Chao Y, Wu Q, Shepard C, Wells A. Hepatocyte induced re-expression of e-cadherin in breast and prostate cancer cells increases chemoresistance. Clin Exp Metastasis. (2012) 29(1):39–50. doi: 10.1007/s10585-011-9427-3
147. Bajrami I, Marlow R, van de Ven M, Brough R, Pemberton HN, Frankum J, et al. E-Cadherin/ROS1 inhibitor synthetic lethality in breast cancer. Cancer Discovery (2018) 8(4):498–515. doi: 10.1158/2159-8290.CD-17-0603
148. Cao X, Li B, Chen J, Dang J, Chen S, Gunes EG, et al. Effect of cabazitaxel on macrophages improves CD47-targeted immunotherapy for triple-negative breast cancer. J Immunother Cancer. (2021) 9(3):e002022. doi: 10.1136/jitc-2020-002022
149. Koutras A, Zagouri F, Koliou GA, Psoma E, Chryssogonidis I, Lazaridis G, et al. Phase 2 study of cabazitaxel as second-line treatment in patients with HER2-negative metastatic breast cancer previously treated with taxanes-a Hellenic cooperative oncology group (HeCOG) trial. Br J Cancer. (2020) 123(3):355–61. doi: 10.1038/s41416-020-0909-4
150. Hsu BE, Tabariès Sébastien, Johnson RM, Andrzejewski S, Senecal J, Lehuédé C, et al. Immature low-density neutrophils exhibit metabolic flexibility that facilitates breast cancer liver metastasis. Cell Rep (2019) 27(13):3902–3915.e6. doi: 10.1016/j.celrep.2019.05.091
151. Shah K, Panchal S, Patel B. Porcupine inhibitors: Novel and emerging anti-cancer therapeutics targeting the wnt signaling pathway. Pharmacol Res (2021) 167:105532. doi: 10.1016/j.phrs.2021.105532
Keywords: liver metastasis, pathogenesis, clinical implications, breast cancer, hepatic microenvironment
Citation: Liu C, Mohan SC, Wei J, Seki E, Liu M, Basho R, Giuliano AE, Zhao Y and Cui X (2022) Breast cancer liver metastasis: Pathogenesis and clinical implications. Front. Oncol. 12:1043771. doi: 10.3389/fonc.2022.1043771
Received: 14 September 2022; Accepted: 04 October 2022;
Published: 25 October 2022.
Edited by:
Ira Ida Skvortsova, Innsbruck Medical University, AustriaReviewed by:
Ozden Yalcin-Ozuysal, Izmir Institute of Technology, TurkeyCopyright © 2022 Liu, Mohan, Wei, Seki, Liu, Basho, Giuliano, Zhao and Cui. This is an open-access article distributed under the terms of the Creative Commons Attribution License (CC BY). The use, distribution or reproduction in other forums is permitted, provided the original author(s) and the copyright owner(s) are credited and that the original publication in this journal is cited, in accordance with accepted academic practice. No use, distribution or reproduction is permitted which does not comply with these terms.
*Correspondence: Yanxia Zhao, c29waGlhNzc4MUAxMjYuY29t; Xiaojiang Cui, WGlhb2ppYW5nLkN1aUBjc2hzLm9yZw==
Disclaimer: All claims expressed in this article are solely those of the authors and do not necessarily represent those of their affiliated organizations, or those of the publisher, the editors and the reviewers. Any product that may be evaluated in this article or claim that may be made by its manufacturer is not guaranteed or endorsed by the publisher.
Research integrity at Frontiers
Learn more about the work of our research integrity team to safeguard the quality of each article we publish.