- 1School of Stomatology, Weifang Medical University, Weifang, China
- 2Department of Oral Maxillofacio-Head and Neck Oncology, Shanghai Ninth People's Hospital, College of Stomatology, Shanghai Jiao Tong University School of Medicine, Shanghai, China
- 3Shanghai Key Laboratory of Stomatology, Shanghai Research Institute of Stomatology, National Center for Stomatology, National Clinical Research Center for Oral Diseases, Shanghai, China
Cells experience both endogenous and exogenous DNA damage daily. To maintain genome integrity and suppress tumorigenesis, individuals have evolutionarily acquired a series of repair functions, termed DNA damage response (DDR), to repair DNA damage and ensure the accurate transmission of genetic information. Defects in DNA damage repair pathways may lead to various diseases, including tumors. Accumulating evidence suggests that alterations in DDR-related genes, such as somatic or germline mutations, single nucleotide polymorphisms (SNPs), and promoter methylation, are closely related to the occurrence, development, and treatment of head and neck squamous cell carcinoma (HNSCC). Despite recent advances in surgery combined with radiotherapy, chemotherapy, or immunotherapy, there has been no substantial improvement in the survival rate of patients with HNSCC. Therefore, targeting DNA repair pathways may be a promising treatment for HNSCC. In this review, we summarized the sources of DNA damage and DNA damage repair pathways. Further, the role of DNA damage repair pathways in the development of HNSCC and the application of small molecule inhibitors targeting these pathways in the treatment of HNSCC were focused.
Introduction
Head and neck squamous cell carcinoma (HNSCC) is the sixth most common cancer worldwide, with approximately 650,000 new cases and 350,000 deaths each year (1). Oral squamous cell carcinoma (OSCC) is the most common malignant type of HNSCC (2). Major risk factors for HNSCC include tobacco use, excessive alcohol consumption, and human papillomavirus (HPV) infection (3–5). For patients with HPV-related HNSCC, the prognosis is favorable, with an overall survival (OS) rate of 95%-80% at 2-5 years (6). However, the prognosis of patients with non-HPV and smoking-related HNSCC remains poor, with a five-year survival rate of only approximately 50% (7). Current standard treatment options for HNSCC include surgery, chemotherapy, and radiation therapy, but recurrence rates are high, and about half of all HNSCC patients experience recurrence (8). Thus, the exploration of novel and effective therapies to improve the prognosis and survival of patients with HNSCC is warranted.
It is known that DNA damage and abnormal DNA damage response (DDR) may harm the integrity and stability of the whole genome and contribute to various diseases such as cancer (9). To maintain genome integrity and suppress tumorigenesis, individuals have acquired a series of repair functions, termed the DDR, during evolution to repair DNA damage and ensure the accurate transmission of genetic information. DDR is a complex kinase-based signaling pathway that senses, transduces, and responds appropriately to DNA damage to maintain genomic stability (10). Mutations in any component of DDR are considered to be related to the initiation and progression of as breast cancer (11), ovarian cancer (12), prostate cancer (13), colorectal cancer (14) and HNSCC (15), which have been previously reported. Therefore, targeting the DDR pathway may be a novel therapeutic approach for HNSCC treatment. This paper comprehensively reviewed the various reported sources of DNA damage and associated repair pathways in HNSCC and drugs targeting the abnormal DNA damage response pathway.
DNA damage repair pathway
It is estimated that cells experience thousands of DNA damage events daily, which can be divided into two broad categories based on their origin: endogenous and exogenous (16, 17). Most endogenous DNA damage arises from the hydrolysis and oxidation of chemically active DNA with water and naturally occurring intracellular reactive oxygen species (ROS) (18, 19). On the other hand, exogenous DNA damage is caused by environmental, physical, and chemical agents such as ultraviolet (UV) and ionizing radiation (IR), alkylating agents, and crosslinking agents (16). These agents can induce different types of DNA damage, such as abasic sites, mismatches, interstrand crosslinks, or single- and double-stranded breaks (19). The most harmful lesions and the most serious threat to cells are double-strand breaks (DSBs). If repaired ineffectively or incorrectly, DSBs can lead to carcinogenesis or cell death (20). Different types of DNA damage are identified and repaired by the corresponding DDR pathways, and these repair processes are key to maintaining the genetic stability of the cells.
DDR pathways include mismatch repair (MMR), base excision repair (BER), nucleotide excision repair (NER), homologous recombination (HR), and non-homologous terminal link (NHEJ) repair (21). A few specific lesions can also be removed using direct reversal repair (DR) pathways and interstrand crosslink (ICL) repair (16). These repair processes are key to maintaining the genetic stability of cells (22). We summarized the DNA damage and main DDR pathways and some associated proteins in Figure 1. The BER pathway repairs base lesions caused by oxidation, deamination, and alkylation (23). NER mainly removes bulky DNA adducts induced by UV radiation or chemotherapeutic drugs (24). The MMR system is responsible for correcting base-base mismatches and insertion or deletion mismatches generated during DNA replication (25). DSBs are the most severe type of DNA damage and can be repaired by HR, NHEJ, or both repair pathways (26). The HR pathway facilitates highly accurate DSB repair by utilizing homologous DNA sequences on the sister chromatid as a replication template during repair, while the NHEJ pathway does not require a homologous template and is highly efficient but intrinsically error-prone (18). ICL repair involves a complex interplay between multiple DNA repair pathways, including NER, HR, Fanconi anemia (FA), and translesion DNA synthesis (TLS) (27).
DDR involves the detection of DNA damage, activation of cell cycle checkpoints, induction and recruitment of repair factors to the damage sites, and the subsequent repair of the damage. Effective damage repair results in cell cycle resumption, whereas improper repair or damage beyond repair results in permanent cell cycle arrest (senescence) or programmed cell death (apoptosis). Failure to efficiently repair DNA damage can cause mutations and genome instability that drive cancer (19). Defective DDR pathways are a common characteristic of cancer. Alterations in DDR-related genes, such as somatic or germline mutations, single nucleotide polymorphisms(SNPs) and promoter methylation of key genes, and other epigenetic alterations, are closely related to the occurrence and development of cancer (17). Understanding the internal mechanism and correlations between DDR pathway alterations and cancer can improve the efficacy of anti-tumor therapy and play a role in prediction and prognosis.
In this review, we discuss recent progress in the research area of targeted DDR pathways for HNSCC.
DNA damage repair in HNSCC
It has been shown that germline and somatic alterations in DNA repair genes are associated with different susceptibility and prognosis in patients with HNSCC. In a retrospective study of 170 patients with HNSCC, BRCA2 and ARID1A were found to be the two DDR genes with the highest mutation rates, with 17.6% of patients having mutations in these two genes, followed by ATM and BRCA1 with 13.5% and 10% mutations, respectively (28). In addition, a recent circulating tumor (ctDNA) sequencing study of 75 patients with HNSCC found that the five most commonly altered genes were TP53, CDKN2A, TERT, BRCA2, and NOTCH1, and that 38.8% of patients had alterations in DNA repair genes (APC, ATM, BRCA1, or BRCA2) (29). For the first time, ctDNA alterations in TP53 and DNA repair genes were shown to be significantly associated with poor prognosis in HNSCC (29).
Another whole-exome sequencing study of 45 patients with oral and oropharyngeal cancer found that FANCG, CDKN2A, and TPP germline variants were strongly associated with HNSCC risk (30). At least one germline variation in DNA repair pathway genes was detected in 67% of patients (30). This study found that young adults with germline variants of the DNA repair gene had an increased risk of HNSCC susceptibility, while patients with germline variants of the DNA repair gene also had a higher five-year survival rate (30). Moreover, a high frequency of somatic alterations in TP53, CDKN2A, FAT1, and PIK3CA was confirmed in 521 patients with TCGA-HNSCC (30). TP53 has a central node in the interaction network between somatic and germline mutant genes (30). In addition, several significantly altered genomic features were found in OSCC patients with lymph node metastasis (LNM) compared with patients without LNM, including hotspot somatic mutations in TP53 and CASP8, rare nonsilent germline mutations in BRCA2 and FAT1, mutations in mitotic G2/M and NHEJ pathways, recurrent deletions of DNA repair genes by homologous recombination, and chromosomal instability (31). Furthermore, these characteristic changes were of high predictive value for LN metastasis, and LN+ patients with mutations in the NHEJ pathway had longer disease-free survival (31).
Abnormal expression of DDR-related genes is associated with the development, progression and treatment response of HNSCC (Table 1). In a study of 70 HNSCC patients and 46 healthy controls (HC), DDR-related parameters were disrupted in HNSCC patients. NER) (ERCC1, ERCC2/XPD, XPA, and XPC) and BER (APEX1, XRCC1) genes were downregulated in patients with HNSCC compared with the HC, whereas double-strand break repair (MRE11A, RAD50, RAD51, XRCC2) and mismatch repair (MLH1, MSH2, MSH3) genes were up-regulated (15). A study of NER gene expression levels in the peripheral blood lymphocytes of 483 subjects (251 HNSCC patients and 232 HC) found that XPA and XPB expression levels in HNSCC patients were significantly lower than those in the controls, and lower XPB gene expression levels were associated with an increased risk of HNSCC (35). In a study of 349 newly diagnosed HNSCC patients and 295 cancer-free controls, ERCC3 and XPA expression levels were significantly reduced in HNSCC patients compared with controls (36). Further analysis revealed a dose-dependent relationship between the increased risk of HNSCC and low ERCC3 and XPA expression levels (36). Further studies have shown that a lower NER capacity is associated with longer progression-free survival (15). A study of NER core proteins (ERCC1, XPF, and XPA) in 453 HNSCC patients found that high expression of ERCC1 and XPA was associated with poor OS in patients with OSCC, which may be related to chemotherapy resistance (32). However, in oropharyngeal SCC, high XPA expression was associated with a significantly better OS (32). Studies have showed that oropharyngeal SCCa patients with high ERCC1 expression may have better outcomes, independent of HPV status (49). However, it has also been reported that ERCC1 expression is not associated with the prognosis of oropharyngeal/oral SCCHN (50). In addition, polymorphisms of NER core genes (XPC, XPD and XPG) have been shown to be associated with the recurrence of SCCOP (51). The relationship between the expression of NER related genes and the prognosis of oropharyngeal carcinoma needs to be further studied. The differential expression of NER core protein in the head and neck subsites affects the OS of patients, which is of great significance for overcoming chemotherapy resistance in the HNSCC subtype (32). Moreover, studies have reported that high ERCC1 expression is significantly associated with enhanced chemotherapy response induced by 5-FU and cisplatin, suggesting that ERCC1 expression can be used as a biomarker to predict the efficacy of 5-FU and cisplatin chemotherapy for HNSCC (33). Conversely, another study showed that high ERCC1 expression was associated with cisplatin resistance and poor prognosis, while low ERCC1 expression was more sensitive to cisplatin in HNSCC (34). Meanwhile, previous studies have shown that the expression of NER protein (ERCC1, ERCC2) may be sensitive prognostic indicators of radiochemotherapy for locally advanced HNSCC (52–54). In addition, polymorphisms of ERCC1, ERCC2(XPD), XPA and XPC have been found to be associated with radiotherapy efficacy in a variety of tumors, including HNSCC, and may be important predictors of clinical outcomes in patients treated with radiotherapy (55–60). Studies have found that the expression of NER related genes and single nucleotide polymorphisms (SNPs) may also influence the severity of radiation-related side effects in patients (61–64). Thus, NER proteins is not only closely related to the occurrence of tumors, but it also is a predictor for the efficacy of radiotherapy and prognosis of tumors. In oral tongue squamous cell carcinoma (OTSCC), the expression of the proteins APE-1 and XRCC-1 (involved in BER) was found to have increased, and XRCC-1 expression was correlated with better clinical staging of OTSCC and negative lymph node metastasis (38). This suggests that this protein may play a protective role (38). In addition, previous studies have shown that APEX1 expression levels in HNC tumor tissues are significantly higher than those in normal tissues, and that high APEX1 expression is associated with an increased risk of HNC in the Pakistani population (37). The proteins MLH1 and MSH2 (involved in MMR) are overexpressed in oral leukoplakia with dysplasia and OSCC, especially in patients with comorbidities (39). In contrast, other studies have shown that the expression of MMR repair-associated genes MLH1, MSH2, MLH3, and PMS2 is decreased in OSCC (40). Low expression of these genes is associated with reduced DNA repair capacity and development of malignant lesions (40). Additionally, previous studies have reported that reduced expression of these genes is correlated with smoking-induced methylation in smokers at risk of cancer (41). DSBs are mainly repaired by the HR and NHEJ pathways. High expression of DSB repair-related genes (MRE11A, Rad50, RAD51, and XRCC2) in HNSCC patients may contribute to tumor acquisition and progression or induce drug-resistant phenotypes (15). A recent study also showed that high RAD51 expression was associated with the occurrence, development, and recurrence of OSCC, indicating a poor prognosis (43). Moreover, studies have shown that ATM and BRCA1 (both involved in HR) are lowly expressed in HNSCC and that low expression of both is associated with decreased OS and poor prognosis in HNSCC patients (44, 45). Mre11 (part of the MRN complex) is essential for DSB repair in normal cells. Studies have shown that the expression of Mre11 in oral cancer tissues is significantly higher than that in adjacent non-cancerous oral tissues and that oral cancers with high MRE11 expression have reduced OS and progression-free survival (42). In addition, the mediator of DNA damage checkpoint protein 1 (MDC1) is associated with the recruitment of DNA damage repair proteins to the site of DSBs (65). Clinical studies have shown that in OSCC (100 patients), nuclear and cytoplasmic MDC1 protein expression levels were 85% and 92%, respectively. Strong nuclear expression of MDC1 was significantly associated with lymph node metastasis and reduced relapse-free survival (RFS) in patients with OSCC. By contrast, patients with weak nuclear MDC1 expression benefited significantly from radiation therapy after surgery (66). Therefore, high expression of MDC1 correlates with the aggressiveness of OSCC and can serve as an important prognostic indicator for OSCC patients (66). The expression level of KU70/80, a protein involved in the NHEJ repair pathway, was higher in OSCC tissues than in normal tissues, and its high expression was associated with shorter OS of patients (46). In addition, Ku80 expression levels were associated with higher local recurrence rates after radiotherapy in patients, suggesting that Ku80 may be a potential prognostic biomarker for HNSCC patients (47). The expression of DNA-PKcs in HPV-positive oropharyngeal squamous cell carcinoma tissues was significantly lower than that in HPV-negative oropharyngeal squamous cell carcinoma tissues, and the expression of DNA-PKcs negatively correlated with the expression of HPV E6 and E7 (48). DNA-PKcs knockdown leads to an increased sensitivity of tumor cells to cisplatin and radiotherapy, and reduces cell migration and invasion (48).
SNPs in several DDR and DNA repair-related genes have been reported to be associated with an increased risk of HNSCC (Table 2), including ERCC1 (67), ERCC2/XPD (67–70), XPC (71, 72) and XPG (69) (involved in NER), APEX1 (73) and XRCC1 (74–76)(involved in BER), MLH1 (77), MSH2 (77, 78), MSH3 (77, 79) and EXO1 (77, 80) (involved in MMR), XRCC3 (70, 76, 81, 83, 84) and RAD51 (81–84, 90) (involved in HR), Ku70 (85)/80 (86, 87), XRCC4 (88, 89) and Lig4 (83)(involved in HR). Studies on these gene polymorphisms have potential benefits in identifying predictive biomarkers for the risk of HNSCC and provide useful evidence for the early detection of HNSCC. However, comprehensive functional investigations of DDR-related gene SNPs are still lacking. Therefore, further research on SNPs and risk alleles is required.
Additionally, epigenetic alterations have been reported to be closely related to the development and progression of cancer. A meta-analysis has suggested that hypermethylation of the MLH1 promoter is associated with HNSCC. Thus, methylated MLH1 could be a potential diagnostic biomarker for HNSCC (91). One study showed that epigenetic silencing of O6-methylguanine-DNA methyltransferase (MGMT, involved in the DR pathway) DNA repair enzyme through promoter hypermethylation (HmMGMT) may increase TP53 oncosuppressor gene mutations, thereby promoting HNSCC (92). In addition, patients with both hmMGMT and destructive TP53-mutations may have a poor prognosis (92). Hypermethylation of the DNA glycosylase NEi endonuclease VIII-like 1 (NEIL1) promoter has been demonstrated in HNSCC, with increased methylation levels in tumors compared to matched non-tumor cells. DNA methylation contributes to the downregulation of NEIL1, which is involved in the BER repair pathway, thereby increasing the sensitivity of HNSCC to chemotherapy or radiotherapy (93). Another study reported that methylation loss at the three-prime repair exonuclease 2 (TREX2) locus was observed in laryngeal cancer, and that low TREX2 DNA methylation was associated with elevated TREX2 expression and prolonged OS in laryngeal cancer (94).
In summary, these data show that DNA damage and aberrant DDR play an important role in the occurrence, development, treatment response, and prognosis of HNSCC (95).
New therapeutic approaches targeting the DDR pathway in HNSCC
PARP inhibitors
PARPs are a group of enzymes that utilize beta nicotinamide adenine dinucleotide (β-NAD+) to covalently add Poly(ADPribose) (PAR) chains onto target proteins, a process known as PARylation (96). The most studied and best understood is PARP1, which is the first member of the poly ADP-ribosyl polymerase (PARP) superfamily (97). PARP1 plays an important role in DNA damage repair and the maintenance of genome integrity (98). It binds to nuclear DNA single-strand break (SSB) and recruits a number of different DNA repair proteins, such as x-ray repair cross complimenting protein 1 (XRCC1), to repair the SSB (99). PARP1 is also involved in several other forms of DNA repair, including BER, NER, HR, NHEJ, and mismatch repair (99). Hence, inhibition of PARP1 and the related enzyme PARP2 could be a better therapeutic approach to target specific DNA repair pathways in cancer (100). Actually, PARP inhibitors have proven significant clinical benefits in a variety of solid tumors, including HNSCC (95, 101). Several PARP inhibitors, including olaparib, have been approved by the FDA for the treatment of cancers, including breast, ovarian, prostate and pancreatic cancers. Thus, we summarizes the application of DDR inhibitors in pre-clinical or reported clinical therapies combination with other anticancer therapies for HNSCC (Table 3). Moreover, the relevant registered clinical trials of DDR inhibitors, alone or combined with other therapies in HNSCC, are summarized in Table 4.
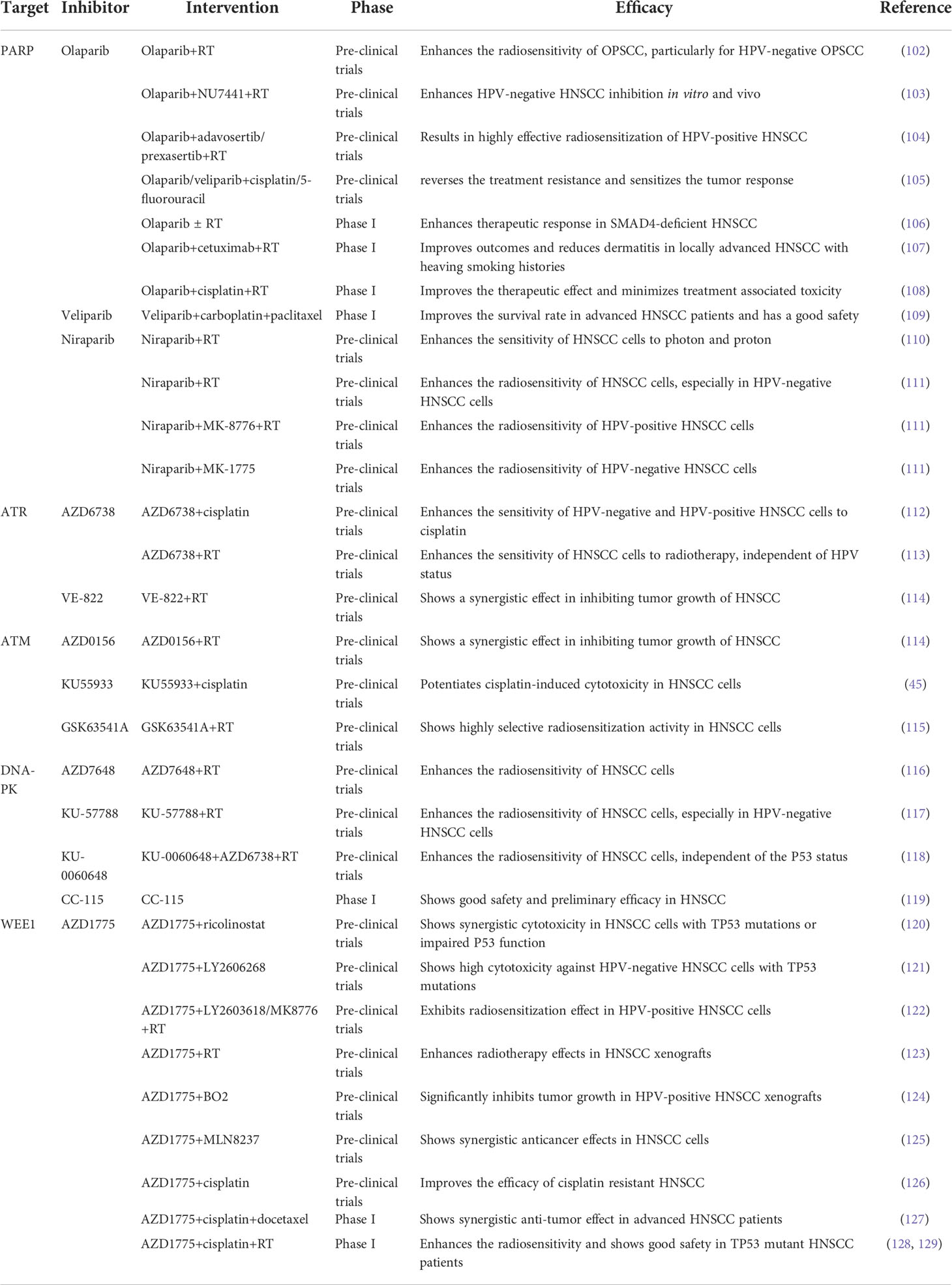
Table 3 DDR inhibitors in pre-clinical or reported clinical therapies combination with other anticancer therapies for HNSCC.
Olaparib
One study showed that the PARP inhibitor olaparib enhanced the apoptotic potential of curcumin by increasing DNA damage in oral cancer cells through inhibition of the BER cascade (100). The combination of the PARP inhibitor olaparib and radiotherapy enhances the radiosensitivity of OPSCC cells, particularly HPV-negative OPSCC cells (102). Similarly, studies have shown that the combination of the PARP inhibitor olaparib and DNA-PK inhibitor NU7441 with IR enhances HPV-negative HNSCC inhibition in both cell cultures and mice (103). In addition, the combination of the PARP inhibitor olaparib and Wee1/Chk1 inhibitor is a highly effective approach for radiosensitization of HPV-positive HNSCC cells (104). Olaparib alone or in combination with radiotherapy caused more DNA damage-associated cell death and reduced proliferation of SMAD4 deficient HNSCC cells (106). In addition, further phase I clinical trials have shown that olaparib in combination with radiotherapy enhances the therapeutic response of HNSCCs (106). A phase I trial demonstrated that olaparib combined with cetuximab and radiotherapy was well tolerated with reduced dermatitis within the radiation field in patients with locally advanced HNSCC with high-risk smoking-related tumors (107). Furthermore, phase 1 trials assessing the safety and tolerability of olaparib in combination with cisplatin and radiotherapy treatment regimens showed that the addition of olaparib improved the therapeutic effect and minimized treatment-associated toxicity to normal tissues (108). Inhibition of PARP, using olaparib or veliparib, partially reversed the resistance caused by cisplatin and 5-FU treatment, and the combination of PARP inhibitors and cisplatin and 5-FU significantly sensitized the tumor response (105).
Veliparib
Additional studies have shown that the PARP inhibitor veliparib (ABT-888) enhances the anti-angiogenic potential of curcumin through deregulation of NECTIN-4 in oral cancer (130). A recent phase I clinical trial demonstrated that veliparib combined with carboplatin and paclitaxel induction chemotherapy was well tolerated and improved the survival rate in patients with locoregionally advanced HNSCC (109). This indicates that combined induction therapy is safe and feasible.
Niraparib
A recent study reported that niraparib enhances the sensitivity of HNSCC cells to both photons and protons and increases the relative biological effectiveness of protons (110). This suggests that niraparib can improve the efficacy of photon and proton radiotherapy in patients with HNSCC patients (110). Niraparib effectively enhanced the radiosensitivity of HNSCCs, particularly HPV-negative HNSCC (111). The combination of niraparib and the Chk1 inhibitor MK-8776 further enhanced the radiosensitivity of HPV-positive HNSCC cells, whereas the combination of niraparib and the Wee1 inhibitor MK-1775 significantly increased the radiosensitivity of HPV-negative HNSCC cells (111).
Meanwhile, the combined application of PARP1 inhibitors and immunotherapy especially PD-1/PD-L1 inhibitors has also attracted much attention in recent years. It has been reported that defects in the DDR pathway may serve as predictive biomarkers of responsiveness to ICIs (131, 132). It has been found that patients with enrich DDR-related genes mutations exhibited higher response to ICIs therapy and was associated with prolonged progression-free survival (133, 134). Besides, a large number of clinical trials have demonstrated that the combination of DDR inhibitors and immune checkpoint inhibitors (ICIs) had synergistic benefit and was well tolerated, which had been observed in different types of solid tumors, including head and neck cancer (95), breast cancer (135), ovarian cancer (136), prostate cancer (137), small cell lung cancer (SCLC) (138), non-small cell lung cancer (NSCLC) (139) and colorectal cancer (140). Thus, we summarize the current clinical studies of PARP1 inhibitors in combination with PD-1/PD-L1 inhibitors as Supplementary Table 1.
ATR and ATM inhibitors
Ataxia telangiectasia and RAD3-related protein (ATR) and ataxia telangiectasia mutated protein (ATM), both members of the phosphatidylinositol 3-kinase-related kinase (PIKK) family, are key sensors of the DDR signaling pathway (141). ATM is activated in response to DSBs, whereas ATR is primarily triggered by replication stress and SSBs. Activation of ATM or ATR phosphorylates its downstream targets, Chk2 or Chk1 kinase, to induce cell cycle arrest and facilitate DNA repair (141). One study showed that the ATR inhibitor AZD6738 enhanced the sensitivity of HPV-negative and HPV-positive HNSCC cells and tumors to cisplatin (112). Recent studies have found that the ATR inhibitor AZD6738 enhances the sensitivity of HNSCC to radiotherapy independent of HPV status (113). The radiosensitizing effect of AZD6738 correlated with checkpoint kinase1 (CHK1)-mediated abrogation of G2/M-arrest (113). Another key mechanism by which ATM and ATR inhibitors selectively enhance tumor cell inactivation when combined with IR) may be through the inducing of senescence (142). A recent study showed that the ATM inhibitor KU55933 induces apoptotic signaling and potentiates cisplatin-induced cytotoxicity (45). GSK63541A, a novel ATM inhibitor, has been shown to have a highly selective radiosensitization activity that is superior to cisplatin and cetuximab (115). Notably, it exhibited virtually no cytotoxicity in normal cells (115). Consistent with this, a recent study reported that ATM and ATR inhibitors in combination with IR increased the death of HNSCC cells, while the combination therapy did not increase side effects (114).
DNA-PK inhibitors
While ATRi and ATMi have been shown to disturb cell cycle arrest and repair DSBs, cancer cells may resort to alternative repair mechanisms, such as DNA-PK-mediated NHEJ (D-NHEJ) (118). Therefore, inhibition of DNA-PK, another member of the PIKK family, may be a promising treatment method to block the DDR pathway in cancer cells after radiotherapy. A recent study suggested that DNA-PKcs inhibition in combination with radiation enhanced the radiosensitization of HNSCC cells to photons and protons and, in particular, inhibited the growth of relatively radioresistant HPV-negative HNSCC cells (117). In addition, studies have shown that the DNA-PK inhibitors KU57788 and IC87361 are more potent radiosensitizing agents than the PARP-1 inhibitors veliparib and olaparib at non-cytotoxic concentrations in HNSCC cell cultures, and that their activities can be enhanced by SLFN11 and hypoxia (143). Similarly, another study found that the DNA-PKi AZD7648 is a potent radiosensitizer in both human (UT-SCC-54C) and murine (SCCVII) HNSCC cells (116). Interestingly, the combination of ATR and DNA-PK inhibitors was more effective in increasing radiotherapy sensitivity, independent of the P53 status of HNSCC cells (118). A phase I trial demonstrated that CC-115, a dual inhibitor of mTOR kinase and DNA-PK, showed good safety and preliminary efficacy and could be a promising novel anticancer treatment agent (119).
WEE1 inhibitors
HPV-negative HNSCC has a poor prognosis. The most common mutational events in HPV-negative HNSCC are inactivation of the tumor suppressor genes TP53 (>85%) and CDKN2A (>57%), which significantly impair G1/S checkpoints, leading to dependence on other cell cycle checkpoints to repair ongoing replication damage (121). Wee1 is a serine/threonine kinase that regulates DNA damage-induced G2/M phase arrest by phosphorylation of cyclin-dependent protein kinase 1 (CDK1, also known as CDC2) at Tyr15 residues (144). Inhibition of Wee1 kinase activity abrogates the G2/M checkpoint and induces replication stress and DNA damage, leading to unrestrained or premature mitosis and subsequent cell death through a mitotic disaster (145).
Thus, WEE1 inhibitors may be a promising approach for treating HPV-negative HNSCC and other TP53 mutated cancers (121). One study showed that Wee1 inhibition (AZD1775) was also highly sensitive to head and neck precancerous cell lines, whereas normal keratinocytes were tolerant to the inhibitor. Therefore, Wee1 inhibition therapy could be used as an effective treatment for precancerous lesions to prevent cancer (146). In one study, a combination of the Wee1 inhibitor adavosertib (AZD1775) and ricolinostat, a selective inhibitor of histone deacetylase 6 (HDAC6), showed synergistic cytotoxicity in HNSCC with TP53 mutations or impaired p53 function caused by HPV infection, possibly by enhancing adavosertib-induced mitotic disaster (120). A recent study showed that adavosertib (AZD1775), in combination with the Chk1 inhibitor prexasertib (LY2606268), showed high cytotoxicity against HPV-negative HNSCC with a compromised G1/S checkpoint caused by TP53 mutations (121). In addition, the combined inhibition of Wee1 and Chk1 efficiently abrogated the G2-arrest induced by radiation and had a radiosensitizing effect on HPV-positive HNSCC cells (122). Moreover, Wee1 inhibitors (Wee1i), in combination with radiotherapy, enhanced the effects in HNSCC xenografts (123). Studies have shown that HPV-positive HNSCCs exhibit high FOXM1 activity, and Wee1i enhances sensitivity to HPV-positive HNSCC through the CDK1-FOXM1 pathway, which drives premature mitosis and DNA damage (147). Another study reported that the combination of adavosertib (AZD1775) and a Rad51 inhibitor (B02) significantly inhibited tumor growth in a xenograft mouse model carrying HPV-positive HNSCC, compared to HPV-negative HNSCC (124). The synergies between the two drugs are associated with excessive DNA damage and replication stress induced by the forced activation of CDK1 and reduction of Chk1 phosphorylation, ultimately leading to abnormal mitosis and apoptosis (124). In addition, studies have demonstrated that adavosertib (AZD1775) enhanced the anticancer effects of the AURKA inhibitor alisertib (MLN8237) in HNSCC, both in vitro and in vivo (125). The Wee1i AZD1775 combined with cisplatin can synergistically inhibit the proliferation and survival of cisplatin-resistant HNSCC cells by inducing DNA damage and apoptosis, suggesting that Wee1 inhibition can improve the efficacy of cisplatin-resistant HNSCC (126). The results of a phase I clinical trial showed that the triplet combination of adavosertib (AZD1775), cisplatin, and docetaxel was safe and well tolerated, showing promising anti-tumor efficacy in patients with advanced HNSCC (127). In addition, phase I studies showed that adavosertib and cisplatin combined with radiotherapy enhanced the efficacy and safety of patients with TP53-mutated HNSCC (128, 129).
Conclusions and perspectives
Several studies have demonstrated that the DDR system plays a crucial role in the occurrence, development, prognosis, and treatment of HNSCC. This review focuses on the possible DDR and repair pathway changes in HNSCC as well as the role of targeted DNA repair pathways in HNSCC therapy. Preclinical and clinical data suggest that the use of DDR inhibitors (PARP/ATM/ATR/DNA-PK/WEE1 inhibitors) alone or in combination with other therapies, such as radiotherapy or chemotherapy, is promising, which may provide a new direction for the treatment of HNSCC. However, the clinical application of targeted DNA repair therapy in HNSCC remains limited because of the lack of specific biomarkers. Thus, further investigations of the DDR mechanism in HNSCC are needed to identify possible early diagnostic biomarkers and therapeutic targets and to provide a theoretical basis for early diagnosis and targeted therapy of HNSCC.
Author contributions
HL, YJ, and AH conceived the article. HL and NH wrote the manuscript. MR and NH helped to improve the manuscript. All authors contributed to the article and approved the submitted version.
Funding
This study was supported by the National Natural Science Foundation of China (Grant Number: 81772870; 81472517; 82072983; 81777270). Shanghai Medical Garden Rising Star Talent Program [Hu [2021]. 99]. 2021 Youth Innovation Talent Introduction and Education Program of Shandong Province Universities (YJ).
Conflict of interest
The authors declare that the research was conducted in the absence of any commercial or financial relationships that could be construed as a potential conflict of interest.
Publisher’s note
All claims expressed in this article are solely those of the authors and do not necessarily represent those of their affiliated organizations, or those of the publisher, the editors and the reviewers. Any product that may be evaluated in this article, or claim that may be made by its manufacturer, is not guaranteed or endorsed by the publisher.
Supplementary material
The Supplementary Material for this article can be found online at: https://www.frontiersin.org/articles/10.3389/fonc.2022.1031944/full#supplementary-material
References
1. Bray F, Ferlay J, Soerjomataram I, Siegel RL, Torre LA, Jemal A. Global cancer statistics 2018: GLOBOCAN estimates of incidence and mortality worldwide for 36 cancers in 185 countries. CA Cancer J Clin (2018) 68(6):394–424. doi: 10.3322/caac.21492
2. Pons-Fuster López E, Gómez García F, López Jornet P. Combination of 5-florouracil and polyphenol EGCG exerts suppressive effects on oral cancer cells exposed to radiation. Arch Oral Biol (2019) 101:8–12. doi: 10.1016/j.archoralbio.2019.02.018
3. Chi AC, Day TA, Neville BW. Oral cavity and oropharyngeal squamous cell carcinoma–an update. CA Cancer J Clin (2015) 65(5):401–21. doi: 10.3322/caac.21293
4. Panarese I, Aquino G, Ronchi A, Longo F, Montella M, Cozzolino I, et al. Oral and oropharyngeal squamous cell carcinoma: prognostic and predictive parameters in the etiopathogenetic route. Expert Rev Anticancer Ther (2019) 19(2):105–19. doi: 10.1080/14737140.2019.1561288
5. Whiteside TL. Head and neck carcinoma immunotherapy: Facts and hopes. Clin Cancer Res an Off J Am Assoc For Cancer Res (2018) 24(1):6–13. doi: 10.1158/1078-0432.CCR-17-1261
6. O'Sullivan B, Huang SH, Su J, Garden AS, Sturgis EM, Dahlstrom K, et al. Development and validation of a staging system for HPV-related oropharyngeal cancer by the international collaboration on oropharyngeal cancer network for staging (ICON-s): A multicentre cohort study. Lancet Oncol (2016) 17(4):440–51. doi: 10.1016/S1470-2045(15)00560-4
7. Du E, Mazul AL, Farquhar D, Brennan P, Anantharaman D, Abedi-Ardekani B, et al. Long-term survival in head and neck cancer: Impact of site, stage, smoking, and human papillomavirus status. Laryngoscope (2019) 129(11):2506–13. doi: 10.1002/lary.27807
8. Zhao Z, Li D, Wu Z, Wang Q, Ma Z, Zhang C. Research progress and prospect of nanoplatforms for treatment of oral cancer. Front Pharmacol (2020) 11:616101. doi: 10.3389/fphar.2020.616101
9. Blackford AN, Jackson SP. ATM, ATR, and DNA-PK: The trinity at the heart of the DNA damage response. Mol Cell (2017) 66(6):801–17. doi: 10.1016/j.molcel.2017.05.015
10. Natale F, Rapp A, Yu W, Maiser A, Harz H, Scholl A, et al. Identification of the elementary structural units of the DNA damage response. Nat Commun (2017) 8:15760. doi: 10.1038/ncomms15760
11. He C, Kawaguchi K, Toi M. DNA Damage repair functions and targeted treatment in breast cancer. Breast Cancer (2020) 27(3):355–62. doi: 10.1007/s12282-019-01038-2
12. Mirza-Aghazadeh-Attari M, Ostadian C, Saei AA, Mihanfar A, Darband SG, Sadighparvar S, et al. DNA Damage response and repair in ovarian cancer: Potential targets for therapeutic strategies. DNA Repair (Amst) (2019) 80:59–84. doi: 10.1016/j.dnarep.2019.06.005
13. Burdak-Rothkamm S, Mansour WY, Rothkamm K. DNA Damage repair deficiency in prostate cancer. Trends Cancer (2020) 6(11):974–84. doi: 10.1016/j.trecan.2020.05.011
14. Arai H, Elliott A, Xiu J, Wang J, Battaglin F, Kawanishi N, et al. The landscape of alterations in DNA damage response pathways in colorectal cancer. Clin Cancer Res an Off J Am Assoc For Cancer Res (2021) 27(11):3234–42. doi: 10.1158/1078-0432.CCR-20-3635
15. Psyrri A, Gkotzamanidou M, Papaxoinis G, Krikoni L, Economopoulou P, Kotsantis I, et al. The DNA damage response network in the treatment of head and neck squamous cell carcinoma. ESMO Open (2021) 6(2):100075. doi: 10.1016/j.esmoop.2021.100075
16. Chatterjee N, Walker GC. Mechanisms of DNA damage, repair, and mutagenesis. Environ Mol Mutagen (2017) 58(5):235–63. doi: 10.1002/em.22087
17. Jiang M, Jia K, Wang L, Li W, Chen B, Liu Y, et al. Alterations of DNA damage repair in cancer: from mechanisms to applications. Ann Transl Med (2020) 8(24):1685. doi: 10.21037/atm-20-2920
18. Tubbs A, Nussenzweig A. Endogenous DNA damage as a source of genomic instability in cancer. Cell (2017) 168(4):644–56. doi: 10.1016/j.cell.2017.01.002
19. Carusillo A, Mussolino C. DNA Damage: From threat to treatment. Cells (2020) 9(7):1665. doi: 10.3390/cells9071665
20. Li J, Sun H, Huang Y, Wang Y, Liu Y, Chen X. Pathways and assays for DNA double-strand break repair by homologous recombination. Acta Biochim Biophys Sin (Shanghai) (2019) 51(9):879–89. doi: 10.1093/abbs/gmz076
21. Stewart RA, Pilié PG, Yap TA. Development of PARP and immune-checkpoint inhibitor combinations. Cancer Res (2018) 78(24):6717–25. doi: 10.1158/0008-5472.CAN-18-2652
22. Colombo CV, Gnugnoli M, Gobbini E, Longhese MP. How do cells sense DNA lesions? Biochem Soc Trans (2020) 48(2):677–91. doi: 10.1042/BST20191118
23. Obi I, Rentoft M, Singh V, Jamroskovic J, Chand K, Chorell E, et al. Stabilization of G-quadruplex DNA structures in schizosaccharomyces pombe causes single-strand DNA lesions and impedes DNA replication. Nucleic Acids Res (2020) 48(19):10998–1015. doi: 10.1093/nar/gkaa820
24. Mullenders LHF. Solar UV damage to cellular DNA: from mechanisms to biological effects. Photochem Photobiol Sci (2018) 17(12):1842–52. doi: 10.1039/c8pp00182k
25. Liu Q, Zhu X, Lindström M, Shi Y, Zheng J, Hao X, et al. Yeast mismatch repair components are required for stable inheritance of gene silencing. PloS Genet (2020) 16(5):e1008798. doi: 10.1371/journal.pgen.1008798
26. Rocha CRR, Silva MM, Quinet A, Cabral-Neto JB, Menck CFM. DNA Repair pathways and cisplatin resistance: an intimate relationship. Clinics (Sao Paulo) (2018) 73(suppl 1):e478s. doi: 10.6061/clinics/2018/e478s
27. Rogers CM, Simmons Iii RH, Fluhler Thornburg GE, Buehler NJ, Bochman ML. Fanconi anemia-independent DNA inter-strand crosslink repair in eukaryotes. Prog Biophys Mol Biol (2020) 158:33–46. doi: 10.1016/j.pbiomolbio.2020.08.005
28. Burcher KM, Faucheux AT, Lantz JW, Wilson HL, Abreu A, Salafian K, et al. Prevalence of DNA repair gene mutations in blood and tumor tissue and impact on prognosis and treatment in HNSCC. Cancers (Basel) (2021) 13(13):3118. doi: 10.3390/cancers13133118
29. Wilson HL, D'Agostino RB, Meegalla N, Petro R, Commander S, Topaloglu U, et al. The prognostic and therapeutic value of the mutational profile of blood and tumor tissue in head and neck squamous cell carcinoma. Oncologist (2021) 26(2):e279–89. doi: 10.1002/onco.13573
30. Cury SS, PMd M, FA M, LMd C, Chulam TC, Petersen AH, et al. Germline variants in DNA repair genes are associated with young-onset head and neck cancer. Oral Oncol (2021) 122:105545. doi: 10.1016/j.oraloncology.2021.105545
31. Biswas NK, Das C, Das S, Maitra A, Nair S, Gupta T, et al. Lymph node metastasis in oral cancer is strongly associated with chromosomal instability and DNA repair defects. Int J Cancer (2019) 145(9):2568–79. doi: 10.1002/ijc.32305
32. Prochnow S, Wilczak W, Bosch V, Clauditz TS, Muenscher A. ERCC1, XPF and XPA-locoregional differences and prognostic value of DNA repair protein expression in patients with head and neck squamous cell carcinoma. Clin Oral Investig (2019) 23(8):3319–29. doi: 10.1007/s00784-018-2751-0
33. Hasegawa Y, Goto M, Hanai N, Ozawa T, Hirakawa H. Predictive biomarkers for combined chemotherapy with 5-fluorouracil and cisplatin in oro- and hypopharyngeal cancers. Mol Clin Oncol (2018) 8(2):378–86. doi: 10.3892/mco.2017.1521
34. Kulkarni S, Solomon M, Pankaj D, Carnelio S, Chandrashekar C, Shetty N. Elucidating the role of excision repair cross-complement group 1 in oral epithelial dysplasia and early invasive squamous cell carcinoma: An immunohistochemical study. J Oral Maxillofac Pathol (2020) 24(1):20–5. doi: 10.4103/jomfp.JOMFP_60_19
35. Ren P, Niu X, Liu C, Liu J, Li H, Zhao Q, et al. Associations between expression levels of nine core nucleotide excision repair genes in lymphocytes and risk of head and neck squamous cell carcinomas in a Chinese population. Int J Clin Oncol (2020) 25(4):660–69. doi: 10.1007/s10147-019-01586-9
36. Han P, Liu H, Shi Q, Liu Z, Troy JD, Lee WT, et al. Associations between expression levels of nucleotide excision repair proteins in lymphoblastoid cells and risk of squamous cell carcinoma of the head and neck. Mol Carcinog (2018) 57(6):784–93. doi: 10.1002/mc.22801
37. Mahjabeen I, Baig RM, Sabir M, Kayani MA. Genetic and expressional variations of APEX1 are associated with increased risk of head and neck cancer. Mutagenesis (2013) 28(2):213–18. doi: 10.1093/mutage/ges074
38. Santana T, Sá MC, de Moura Santos E, Galvão HC, Coletta RD, RdA F. DNA Base excision repair proteins APE-1 and XRCC-1 are overexpressed in oral tongue squamous cell carcinoma. J Oral Pathol Med (2017) 46(7):496–503. doi: 10.1111/jop.12529
39. Donís SP, González AP, Alves MGO, do Carmo Carvalho BF, Ferreira CCP, Almeida JD, et al. MLH1, MSH2, MRE11, and XRCC1 in oral leukoplakia and oral squamous cell carcinoma. Appl Immunohistochem. Mol Morphol (2021) 29(8):613–18. doi: 10.1097/PAI.0000000000000929
40. Oliveira Alves MG, da Silva Miguel N, Ferreira CCP, Alvarenga ER, Tonon BM, de Barros PP, et al. Expression of DNA repair genes in oral squamous cell carcinoma using reverse transcription-quantitative polymerase chain reaction. Oral Surg Oral Med Oral Pathol Oral Radiol (2020) 130(3):298–305. doi: 10.1016/j.oooo.2020.06.003
41. Carta CFL, Oliveira Alves MG, de Barros PP, Campos MS, Scholz J, Jorge AOC, et al. Screening methylation of DNA repair genes in the oral mucosa of chronic smokers. Arch Oral Biol (2018) 92:83–7. doi: 10.1016/j.archoralbio.2018.04.017
42. Wang Y-Y, Chen Y-K, Lo S, Chi T-C, Chen Y-H, Hu SC-S, et al. MRE11 promotes oral cancer progression through RUNX2/CXCR4/AKT/FOXA2 signaling in a nuclease-independent manner. Oncogene (2021) 40(20):3510–32. doi: 10.1038/s41388-021-01698-5
43. Li Y, Li J, Sun J, Liu Y, Liu D, Du L, et al. Expression of RAD51 and its clinical impact in oral squamous cell carcinoma. Anal Cell Pathol (Amst) (2020) 2020:1827676. doi: 10.1155/2020/1827676
44. Essers PBM, van der Heijden M, Verhagen CVM, Ploeg EM, de Roest RH, Leemans CR, et al. Drug sensitivity prediction models reveal a link between DNA repair defects and poor prognosis in HNSCC. Cancer Res (2019) 79(21):5597–611. doi: 10.1158/0008-5472.CAN-18-3388
45. Wang Y-C, Lee K-W, Tsai Y-S, Lu H-H, Chen S-Y, Hsieh H-Y, et al. Downregulation of ATM and BRCA1 predicts poor outcome in head and neck cancer: Implications for ATM-targeted therapy. J Pers Med (2021) 11(5):389. doi: 10.3390/jpm11050389
46. Joshi JS, Vora HH, Ghosh NR, Tankshali RN, Jetly DH, Trivedi TI. Nonhomologous end joining repair pathway molecules as predictive biomarkers for patients with oral squamous cell carcinoma. J Cancer Res Ther (2021) 17(4):1031–38. doi: 10.4103/jcrt.JCRT_582_19
47. Nicolay NH, Wiedenmann N, Mix M, Weber WA, Werner M, Grosu AL, et al. Correlative analyses between tissue-based hypoxia biomarkers and hypoxia PET imaging in head and neck cancer patients during radiochemotherapy-results from a prospective trial. Eur J Nucl Med Mol Imaging (2020) 47(5):1046–55. doi: 10.1007/s00259-019-04598-9
48. Weaver AN, Cooper TS, Wei S, Carroll WR, Rosenthal EL, Yang ES. DNA-Pk Expression in oropharyngeal squamous cell carcinoma: Correlations with human papillomavirus status and recurrence after transoral robotic surgery. Head Neck (2017) 39(2):206–14. doi: 10.1002/hed.24562
49. Patel MR, Zhao N, Ang M-K, Stadler ME, Fritchie K, Weissler MC, et al. ERCC1 protein expression is associated with differential survival in oropharyngeal head and neck squamous cell carcinoma. Otolaryngol Head Neck Surg (2013) 149(4):587–95. doi: 10.1177/0194599813496522
50. An HJ, Jo H, Jung CK, Kang JH, Kim MS, Sun D-I, et al. Prognostic implication of ERCC1 protein expression in resected oropharynx and oral cavity cancer. Pathol Res Pract (2017) 213(8):949–55. doi: 10.1016/j.prp.2017.05.006
51. Song X, Sturgis EM, Jin L, Wang Z, Wei Q, Li G. Variants in nucleotide excision repair core genes and susceptibility to recurrence of squamous cell carcinoma of the oropharynx. Int J Cancer (2013) 133(3):695–704. doi: 10.1002/ijc.28051
52. Liang R, Lin Y, Liu ZH, Liao XL, Yuan CL, Liao SN, et al. Correlation between ERCC1 expression and concurrent chemotherapy and radiotherapy in patients with locally advanced nasopharyngeal cancer. Genet Mol Res (2015) 14(2):5804–11. doi: 10.4238/2015.May.29.12
53. Löck S, Linge A, Lohaus F, Ebert N, Gudziol V, Nowak A, et al. Biomarker signatures for primary radiochemotherapy of locally advanced HNSCC - hypothesis generation on a multicentre cohort of the DKTK-ROG. Radiother. Oncol J Eur Soc For Ther Radiol Oncol (2022) 169:8–14. doi: 10.1016/j.radonc.2022.02.009
54. Bauman JE, Austin MC, Schmidt R, Kurland BF, Vaezi A, Hayes DN, et al. ERCC1 is a prognostic biomarker in locally advanced head and neck cancer: results from a randomised, phase II trial. Br J Cancer (2013) 109(8):2096–105. doi: 10.1038/bjc.2013.576
55. Jin H, Xie X, Wang H, Hu J, Liu F, Liu Z, et al. ERCC1 Cys8092Ala and XRCC1 Arg399Gln polymorphisms predict progression-free survival after curative radiotherapy for nasopharyngeal carcinoma. PloS One (2014) 9(7):e101256. doi: 10.1371/journal.pone.0101256
56. Jiang C, Guo Y, Li Y, Kang J, Sun X, Wu H, et al. The association between the ERCC1/2 polymorphisms and radiotherapy efficacy in 87 patients with non-small cell lung cancer. J Thorac Dis (2021) 13(5):3126–36. doi: 10.21037/jtd-21-755
57. Carles J, Monzo M, Amat M, Jansa S, Artells R, Navarro A, et al. Single-nucleotide polymorphisms in base excision repair, nucleotide excision repair, and double strand break genes as markers for response to radiotherapy in patients with stage I to II head-and-neck cancer. Int J Radiat Oncol Biol Phys (2006) 66(4):1022–30. doi: 10.3389/fonc.2018.00290
58. Hu L-B, Chen Y, Meng X-D, Yu P, He X, Li J. Nucleotide excision repair factor XPC ameliorates prognosis by increasing the susceptibility of human colorectal cancer to chemotherapy and ionizing radiation. Front In Oncol (2018) 8:290. doi: 10.3389/fonc.2018.00290
59. Mahimkar MB, Samant TA, Kannan S, Tulsulkar J, Pai PS, Anantharaman D. Polymorphisms in GSTM1 and XPD genes predict clinical outcome in advanced oral cancer patients treated with postoperative radiotherapy. Mol Carcinog. (2012) 51 Suppl:1 E94–103. doi: 10.1002/mc.21868
60. Farnebo L, Tiefenböck K, Ansell A, Thunell LK, Garvin S, Roberg K. Strong expression of survivin is associated with positive response to radiotherapy and improved overall survival in head and neck squamous cell carcinoma patients. Int J Cancer (2013) 133(8):1994–2003. doi: 10.1002/ijc.28200
61. Wiebalk K, Schmezer P, Kropp S, Chang-Claude J, Celebi O, Debus J, et al. In vitro radiation-induced expression of XPC mRNA as a possible biomarker for developing adverse reactions during radiotherapy. Int J Cancer (2007) 121(10):2340–45. doi: 10.1158/1078-0432.CCR-04-2657
62. Chang-Claude J, Popanda O, Tan X-L, Kropp S, Helmbold I, von Fournier D, et al. Association between polymorphisms in the DNA repair genes, XRCC1, APE1, and XPD and acute side effects of radiotherapy in breast cancer patients. Clin Cancer Res an Off J Am Assoc For Cancer Res (2005) 11(13):4802–09. doi: 10.1016/j.oraloncology.2017.02.003
63. Borchiellini D, Etienne-Grimaldi MC, Bensadoun RJ, Benezery K, Dassonville O, Poissonnet G, et al. Candidate apoptotic and DNA repair gene approach confirms involvement of ERCC1, ERCC5, TP53 and MDM2 in radiation-induced toxicity in head and neck cancer. Oral Oncol (2017) 67:70–6. doi: 10.1016/j.oraloncology.2017.02.003
64. Zhang L, Yang M, Bi N, Ji W, Wu C, Tan W, et al. Association of TGF-β1 and XPD polymorphisms with severe acute radiation-induced esophageal toxicity in locally advanced lung cancer patients treated with radiotherapy. Radiother. Oncol J Eur Soc For Ther Radiol Oncol (2010) 97(1):19–25. doi: 10.1016/j.radonc.2010.08.015
65. Zhang X, Hu F, Liu L, Xu B. Effect of silencing of mediator of DNA damage checkpoint protein 1 on the growth of oral squamous cell carcinoma in vitro and in vivo. Eur J Oral Sci (2019) 127(6):494–99. doi: 10.1111/eos.12662
66. Dave JH, Vora HH, Ghosh NR, Trivedi TI. Mediator of DNA damage checkpoint protein 1 (MDC1) as a prognostic marker for patients with oral squamous cell carcinoma. J Oral Pathol Med (2017) 46(4):253–58. doi: 10.1111/jop.12558
67. Fernández-Mateos J, Seijas-Tamayo R, Adansa Klain JC, Pastor Borgoñón M, Pérez-Ruiz E, Mesía R, et al. Genetic susceptibility in head and neck squamous cell carcinoma in a Spanish population. Cancers (Basel) (2019) 11(4):493. doi: 10.3390/cancers11040493
68. Tejasvi MA, Maragathavalli G, Kumar PU, Ramakrishna M, Raghavan V, Ck AA. Impact of ERCC2 gene polymorphisms on OSCC susceptibility and clinical characteristics. Glob Med Genet (2020) 7(4):121–27. doi: 10.1055/s-0041-1722952
69. Nigam K, Yadav SK, Samadi FM, Bhatt ML, Gupta S, Sanyal S. Risk modulation of oral pre cancer and cancer with polymorphisms in XPD and XPG genes in north Indian population. Asian Pac J Cancer Prev (2019) 20(8):2397–403. doi: 10.31557/APJCP.2019.20.8.2397
70. dos Santos Pereira J, Fontes FL, de Medeiros SRB, de Almeida Freitas R, de Souza LB, da Costa Miguel MC. Association of the XPD and XRCC3 gene polymorphisms with oral squamous cell carcinoma in a northeastern Brazilian population: A pilot study. Arch Oral Biol (2016) 64:19–23. doi: 10.1016/j.archoralbio.2015.12.004
71. Wu C-N, Chang W-S, Shih L-C, Wang Y-C, Lee H-T, Yu C-C, et al. Interaction of DNA repair gene XPC with smoking and betel quid chewing behaviors of oral cancer. Cancer Genomics Proteomics (2021) 18(3 Suppl):441–49. doi: 10.21873/cgp.20270
72. Zhang Y, Li Z, Zhong Q, Zhou W, Chen X, Chen X, et al. Polymorphisms of the XPC gene may contribute to the risk of head and neck cancer: A meta-analysis. Tumour Biol (2014) 35(4):3917–31. doi: 10.1007/s13277-013-1520-6
73. Das S, Bhowmik A, Bhattacharjee A, Choudhury B, Naiding M, Laskar AK, et al. XPD, APE1, and MUTYH polymorphisms increase head and neck cancer risk: effect of gene-gene and gene-environment interactions. Tumour Biol (2015) 36(10):7569–79. doi: 10.1007/s13277-015-3472-5
74. Kabzinski J, Maczynska M, Kaczmarczyk D, Majsterek I. Influence of Arg399Gln, Arg280His and Arg194Trp XRCC1 gene polymorphisms of base excision repair pathway on the level of 8-oxo-guanine and risk of head and neck cancer in the polish population. Cancer Biomark (2021) 32(3):317–26. doi: 10.3233/CBM-203163
75. Xia S, Wu S, Wang M. The association between the XRCC1 Arg399Gln polymorphism and the risk of head and neck cancer: An updated meta-analysis including 14586 subjects. Technol Cancer Res Treat (2021) 20:15330338211033060. doi: 10.1177/15330338211033060
76. Avci H, Ergen A, Bireller ES, Ertugrul B, Cakmakoglu B. A strong relationship between oral squamous cell carcinoma and DNA repair genes. Biochem Genet (2017) 55(5-6):378–86. doi: 10.1007/s10528-017-9806-9
77. Nogueira GAS, Lourenço GJ, Oliveira CBM, Marson FAL, Lopes-Aguiar L, Costa EFD, et al. Association between genetic polymorphisms in DNA mismatch repair-related genes with risk and prognosis of head and neck squamous cell carcinoma. Int J Cancer (2015) 137(4):810–18. doi: 10.1002/ijc.29435
78. Demokan S, Suoglu Y, Ulusan M, Dalay N. Analysis of the hMSH2 gene variants in head and neck cancer. DNA Cell Biol (2010) 29(8):449–57. doi: 10.1089/dna.2009.1013
79. Mondal P, Datta S, Maiti GP, Baral A, Jha GN, Panda CK, et al. Comprehensive SNP scan of DNA repair and DNA damage response genes reveal multiple susceptibility loci conferring risk to tobacco associated leukoplakia and oral cancer. PloS One (2013) 8(2):e56952. doi: 10.1371/journal.pone.0056952
80. Tsai M-H, Tseng H-C, Liu C-S, Chang C-L, Tsai C-W, Tsou Y-A, et al. Interaction of Exo1 genotypes and smoking habit in oral cancer in Taiwan. Oral Oncol (2009) 45(9):e90–4. doi: 10.1016/j.oraloncology.2009.03.011
81. Sliwinski T, Walczak A, Przybylowska K, Rusin P, Pietruszewska W, Zielinska-Blizniewska H, et al. Polymorphisms of the XRCC3 C722T and the RAD51 G135C genes and the risk of head and neck cancer in a polish population. Exp Mol Pathol (2010) 89(3):358–66. doi: 10.1016/j.yexmp.2010.08.005
82. Kong F, Wu J, Hu L, Du Y, Pan Y. Association between RAD51 polymorphisms and susceptibility of head and neck cancer: A meta-analysis. . Int J Clin Exp Med (2015) 8(4):6412–19. doi: 10.1016/j.mrgentox.2008.07.013
83. Werbrouck J, De Ruyck K, Duprez F, Van Eijkeren M, Rietzschel E, Bekaert S, et al. Single-nucleotide polymorphisms in DNA double-strand break repair genes: association with head and neck cancer and interaction with tobacco use and alcohol consumption. Mutat Res (2008) 656(1-2):74–81. doi: 10.1016/j.mrgentox.2008.07.013
84. Gresner P, Gromadzinska J, Polanska K, Twardowska E, Jurewicz J. Wasowicz W. Genet variability Xrcc3 Rad51 modulates Risk Head Neck cancer Gene (2012) 504(2):166–74. doi: 10.1016/j.gene.2012.05.030
85. Bau D-T, Tseng H-C, Wang C-H, Chiu C-F, Hua C-H, Wu C-N, et al. Oral cancer and genetic polymorphism of DNA double strand break gene Ku70 in Taiwan. Oral Oncol (2008) 44(11):1047–51. doi: 10.1016/j.oraloncology.2008.02.008
86. Al-Hadyan KS, Al-Harbi NM, Al-Qahtani SS, Alsbeih GA. Involvement of single-nucleotide polymorphisms in predisposition to head and neck cancer in Saudi Arabia. Genet Test Mol Biomarkers (2012) 16(2):95–101. doi: 10.1089/gtmb.2011.0126
87. Hsu C-F, Tseng H-C, Chiu C-F, Liang S-Y, Tsai C-W, Tsai M-H, et al. Association between DNA double strand break gene Ku80 polymorphisms and oral cancer susceptibility. Oral Oncol (2009) 45(9):789–93. doi: 10.1016/j.oraloncology.2008.12.002
88. Chiu C-F, Tsai M-H, Tseng H-C, Wang C-L, Wang C-H, Wu C-N, et al. A novel single nucleotide polymorphism in XRCC4 gene is associated with oral cancer susceptibility in Taiwanese patients. Oral Oncol (2008) 44(9):898–902. doi: 10.1016/j.oraloncology.2007.11.007
89. Tseng H-C, Tsai M-H, Chiu C-F, Wang C-H, Chang N-W, Huang C-Y, et al. Association of XRCC4 codon 247 polymorphism with oral cancer susceptibility in Taiwan. Anticancer Res (2008) 28(3A):1687–91.
90. de Moura Santos E, de Pontes Santos HB, de Matos FR, Machado RA, Coletta RD, Galvão HC, et al. Clinicopathological significance of SNPs in RAD51 and XRCC3 in oral and oropharyngeal carcinomas. Oral Dis (2019) 25(1):54–63. doi: 10.1111/odi.12943
91. Li Q, Hong J, Shen Z, Deng H, Shen Y, Wu Z, et al. A systematic review and meta-analysis approach on diagnostic value of MLH1 promoter methylation for head and neck squamous cell carcinoma. Med (Baltimore) (2019) 98(43):e17651. doi: 10.1097/MD.0000000000017651
92. Matsuda S, Mafune A, Kohda N, Hama T, Urashima M. Associations among smoking, MGMT hypermethylation, TP53-mutations, and relapse in head and neck squamous cell carcinoma. PloS One (2020) 15(4):e0231932. doi: 10.1371/journal.pone.0231932
93. Chaisaingmongkol J, Popanda O, Warta R, Dyckhoff G, Herpel E, Geiselhart L, et al. Epigenetic screen of human DNA repair genes identifies aberrant promoter methylation of NEIL1 in head and neck squamous cell carcinoma. Oncogene (2012) 31(49):5108–16. doi: 10.1038/onc.2011.660
94. Weigel C, Chaisaingmongkol J, Assenov Y, Kuhmann C, Winkler V, Santi I, et al. DNA Methylation at an enhancer of the three prime repair exonuclease 2 gene (TREX2) is linked to gene expression and survival in laryngeal cancer. Clin Epigenet (2019) 11(1):67. doi: 10.1186/s13148-019-0666-5
95. Moutafi M, Economopoulou P, Rimm D, Psyrri A. PARP inhibitors in head and neck cancer: Molecular mechanisms, preclinical and clinical data. Oral Oncol (2021) 117:105292. doi: 10.1016/j.oraloncology.2021.105292
96. De Vos M, Schreiber V, Dantzer F. The diverse roles and clinical relevance of PARPs in DNA damage repair: current state of the art. Biochem Pharmacol (2012) 84(2):137–46. doi: 10.1016/j.bcp.2012.03.018
97. Curtin NJ, Szabo C. Poly(ADP-ribose) polymerase inhibition: past, present and future. Nat Rev Drug Discovery (2020) 19(10):711–36. doi: 10.1038/s41573-020-0076-6
98. Palazzo L, Ahel I. PARPs in genome stability and signal transduction: implications for cancer therapy. Biochem Soc Trans (2018) 46(6):1681–95. doi: 10.1042/BST20180418
99. Pascal JM. The comings and goings of PARP-1 in response to DNA damage. DNA Repair (Amst) (2018) 71:177–82. doi: 10.1016/j.dnarep.2018.08.022
100. Molla S, Hembram KC, Chatterjee S, Nayak D, Sethy C, Pradhan R, et al. PARP inhibitor olaparib enhances the apoptotic potentiality of curcumin by increasing the DNA damage in oral cancer cells through inhibition of BER cascade. Pathol Oncol Res (2020) 26(4):2091–103. doi: 10.1007/s12253-019-00768-0
101. Glorieux M, Dok R, Nuyts S. Novel DNA targeted therapies for head and neck cancers: clinical potential and biomarkers. Oncotarget (2017) 8(46):81662–78. doi: 10.18632/oncotarget.20953
102. Nickson CM, Moori P, Carter RJ, Rubbi CP, Parsons JL. Misregulation of DNA damage repair pathways in HPV-positive head and neck squamous cell carcinoma contributes to cellular radiosensitivity. Oncotarget (2017) 8(18):29963–75. doi: 10.18632/oncotarget.16265
103. Zeng L, Boggs DH, Xing C, Zhang Z, Anderson JC, Wajapeyee N, et al. Combining PARP and DNA-PK inhibitors with irradiation inhibits HPV-negative head and neck cancer squamous carcinoma growth. Front Genet (2020) 11:1036. doi: 10.3389/fgene.2020.01036
104. Hintelmann K, Berenz T, Kriegs M, Christiansen S, Gatzemeier F, Struve N, et al. Dual inhibition of PARP and the intra-S/G2 cell cycle checkpoints results in highly effective radiosensitization of HPV-positive HNSCC cells. Front Oncol (2021) 11:683688. doi: 10.3389/fonc.2021.683688
105. Wang F, Gouttia OG, Wang L, Peng A. PARP1 upregulation in recurrent oral cancer and treatment resistance. Front Cell Dev Biol (2021) 9:804962. doi: 10.3389/fcell.2021.804962
106. Hernandez AL, Young CD, Bian L, Weigel K, Nolan K, Frederick B, et al. PARP inhibition enhances radiotherapy of SMAD4-deficient human head and neck squamous cell carcinomas in experimental models. Clin Cancer Res (2020) 26(12):3058–70. doi: 10.1158/1078-0432.Ccr-19-0514
107. Karam SD, Reddy K, Blatchford PJ, Waxweiler T, DeLouize AM, Oweida A, et al. Final report of a phase I trial of olaparib with cetuximab and radiation for heavy smoker patients with locally advanced head and neck cancer. Clin Cancer Res an Off J Am Assoc Cancer Res (2018) 24(20):4949–59. doi: 10.1158/1078-0432.CCR-18-0467
108. de Haan R, van Werkhoven E, van den Heuvel MM, Peulen HMU, Sonke GS, Elkhuizen P, et al. Study protocols of three parallel phase 1 trials combining radical radiotherapy with the PARP inhibitor olaparib. BMC Cancer (2019) 19(1):901. doi: 10.1186/s12885-019-6121-3
109. Jelinek MJ, Foster NR, Zoroufy AJ, Schwartz GK, Munster PN, Seiwert TY, et al. A phase I trial adding poly(ADP-ribose) polymerase inhibitor veliparib to induction carboplatin-paclitaxel in patients with head and neck squamous cell carcinoma: Alliance A091101. Oral Oncol (2021) 114:105171. doi: 10.1016/j.oraloncology.2020.105171
110. Wang L, Cao J, Wang X, Lin E, Wang Z, Li Y, et al. Proton and photon radiosensitization effects of niraparib, a PARP-1/-2 inhibitor, on human head and neck cancer cells. Head Neck (2020) 42(9):2244–56. doi: 10.1002/hed.26155
111. Molkentine JM, Molkentine DP, Bridges KA, Xie T, Yang L, Sheth A, et al. Targeting DNA damage response in head and neck cancers through abrogation of cell cycle checkpoints. Int J Radiat Biol (2021) 97(8):1121–28. doi: 10.1080/09553002.2020.1730014
112. Leonard BC, Lee ED, Bhola NE, Li H, Sogaard KK, Bakkenist CJ, et al. ATR inhibition sensitizes HPV(-) and HPV(+) head and neck squamous cell carcinoma to cisplatin. Oral Oncol (2019) 95:35–42. doi: 10.1016/j.oraloncology.2019.05.028
113. Dok R, Glorieux M, Bamps M, Nuyts S. Effect of ATR inhibition in RT response of HPV-negative and HPV-positive head and neck cancers. Int J Mol Sci (2021) 22(4):1504. doi: 10.3390/ijms22041504
114. Faulhaber E-M, Jost T, Symank J, Scheper J, Bürkel F, Fietkau R, et al. Kinase inhibitors of DNA-PK, ATM and ATR in combination with ionizing radiation can increase tumor cell death in HNSCC cells while sparing normal tissue cells. Genes (Basel) (2021) 12(6):925. doi: 10.3390/genes12060925
115. Dohmen AJC, Qiao X, Duursma A, Wijdeven RH, Lieftink C, Hageman F, et al. Identification of a novel ATM inhibitor with cancer cell specific radiosensitization activity. Oncotarget (2017) 8(43):73925–37. doi: 10.18632/oncotarget.18034
116. Hong CR, Buckley CD, Wong WW, Anekal PV, Dickson BD, Bogle G, et al. Radiosensitisation of SCCVII tumours and normal tissues in mice by the DNA-dependent protein kinase inhibitor AZD7648. Radiother. Oncol J Eur Soc Ther Radiol Oncol (2022) 166:162–70. doi: 10.1016/j.radonc.2021.11.027
117. Vitti ET, Kacperek A, Parsons JL. Targeting DNA double-strand break repair enhances radiosensitivity of HPV-positive and HPV-negative head and neck squamous cell carcinoma to photons and protons. Cancers (Basel) (2020) 12(6):1490. doi: 10.3390/cancers12061490
118. Hafsi H, Dillon MT, Barker HE, Kyula JN, Schick U, Paget JT, et al. Combined ATR and DNA-PK inhibition radiosensitizes tumor cells independently of their p53 status. Front Oncol (2018) 8:245. doi: 10.3389/fonc.2018.00245
119. Munster P, Mita M, Mahipal A, Nemunaitis J, Massard C, Mikkelsen T, et al. First-In-Human phase I study of a dual mTOR kinase and DNA-PK inhibitor (CC-115) in advanced malignancy. Cancer Manag Res (2019) 11:10463–76. doi: 10.2147/cmar.S208720
120. Miyake K, Takano N, Kazama H, Kikuchi H, Hiramoto M, Tsukahara K, et al. Ricolinostat enhances adavosertib−induced mitotic catastrophe in TP53−mutated head and neck squamous cell carcinoma cells. Int J Oncol (2022) 60(5):54. doi: 10.3892/ijo.2022.5344
121. Deneka AY, Einarson MB, Bennett J, Nikonova AS, Elmekawy M, Zhou Y, et al. Synthetic lethal targeting of mitotic checkpoints in HPV-negative head and neck cancer. Cancers (Basel) (2020) 12(2):306. doi: 10.3390/cancers12020306
122. Busch C-J, Kröger MS, Jensen J, Kriegs M, Gatzemeier F, Petersen C, et al. G2-checkpoint targeting and radiosensitization of HPV/p16-positive HNSCC cells through the inhibition of Chk1 and Wee1. Radiother. Oncol J Eur Soc For Ther Radiol Oncol (2017) 122(2):260–66. doi: 10.1016/j.radonc.2016.11.017
123. Hu Z, Viswanathan R, Cheng H, Chen J, Yang X, Huynh A, et al. Inhibiting WEE1 and IKK-RELA crosstalk overcomes TNFα resistance in head and neck cancers. Mol Cancer Res (2022) 20(6):867–82. doi: 10.1158/1541-7786.MCR-21-0624
124. Lindemann A, Patel AA, Tang L, Tanaka N, Gleber-Netto FO, Bartels MD, et al. Combined inhibition of Rad51 and Wee1 enhances cell killing in HNSCC through induction of apoptosis associated with excessive DNA damage and replication stress. Mol Cancer Ther (2021) 20(7):1257–69. doi: 10.1158/1535-7163.MCT-20-0252
125. Lee JW, Parameswaran J, Sandoval-Schaefer T, Eoh KJ, Yang D-H, Zhu F, et al. (AURKA) and WEE1 inhibition demonstrates synergistic antitumor effect in squamous cell carcinoma of the head and neck. Clin Cancer Res an Off J Am Assoc For Cancer Res (2019) 25(11):3430–42. doi: 10.1158/1078-0432.CCR-18-0440
126. Yang Z, Liao J, Lapidus RG, Fan X, Mehra R, Cullen KJ, et al. Targeting Wee1 kinase to suppress proliferation and survival of cisplatin-resistant head and neck squamous cell carcinoma. Cancer Chemother Pharmacol (2022) 89(4):469–78. doi: 10.1007/s00280-022-04410-w
127. Méndez E, Rodriguez CP, Kao MC, Raju S, Diab A, Harbison RA, et al. A phase I clinical trial of AZD1775 in combination with neoadjuvant weekly docetaxel and cisplatin before definitive therapy in head and neck squamous cell carcinoma. Clin Cancer Res an Off J Am Assoc For Cancer Res (2018) 24(12):2740–48. doi: 10.1158/1078-0432.CCR-17-3796
128. Kong A, Good J, Kirkham A, Savage J, Mant R, Llewellyn L, et al. Phase I trial of WEE1 inhibition with chemotherapy and radiotherapy as adjuvant treatment, and a window of opportunity trial with cisplatin in patients with head and neck cancer: the trial protocol. BMJ Open (2020) 10(3):e033009. doi: 10.1136/bmjopen-2019-033009
129. Chera BS, Sheth SH, Patel SA, Goldin D, Douglas KE, Green RL, et al. Phase 1 trial of adavosertib (AZD1775) in combination with concurrent radiation and cisplatin for intermediate-risk and high-risk head and neck squamous cell carcinoma. Cancer (2021) 127(23):4447–54. doi: 10.1002/cncr.33789
130. Chatterjee S, Sinha S, Molla S, Hembram KC, Kundu CN. PARP inhibitor veliparib (ABT-888) enhances the anti-angiogenic potentiality of curcumin through deregulation of NECTIN-4 in oral cancer: Role of nitric oxide (NO). Cell Signal (2021) 80:109902. doi: 10.1016/j.cellsig.2020.109902
131. Jiang M, Jia K, Wang L, Li W, Chen B, Liu Y, et al. Alterations of DNA damage response pathway: Biomarker and therapeutic strategy for cancer immunotherapy. Acta Pharm Sin B (2021) 11(10):2983–94. doi: 10.1016/j.apsb.2021.01.003
132. Zhao P, Li L, Jiang X, ,Li Q. Mismatch repair deficiency/microsatellite instability-high as a predictor for anti-PD-1/PD-L1 immunotherapy efficacy. J Hematol Oncol (2019) 12(1):54. doi: 10.1186/s13045-019-0738-1
133. Strickland KC, Howitt BE, Shukla SA, Rodig S, Ritterhouse LL, Liu JF, et al. Association and prognostic significance of BRCA1/2-mutation status with neoantigen load, number of tumor-infiltrating lymphocytes and expression of PD-1/PD-L1 in high grade serous ovarian cancer. Oncotarget (2016) 7(12):13587–98. doi: 10.18632/oncotarget.7277
134. Le DT, Uram JN, Wang H, Bartlett BR, Kemberling H, Eyring AD, et al. PD-1 blockade in tumors with mismatch-repair deficiency. N Engl J Med (2015) 372(26):2509–20. doi: 10.1056/NEJMoa1500596
135. Domchek SM, Postel-Vinay S, Im S-A, Park YH, Delord J-P, Italiano A, et al. Olaparib and durvalumab in patients with germline BRCA-mutated metastatic breast cancer (MEDIOLA): An open-label, multicentre, phase 1/2, basket study. Lancet Oncol (2020) 21(9):1155–64. doi: 10.1016/S1470-2045(20)30324-7
136. Lee EK, Konstantinopoulos PA. Combined PARP and immune checkpoint inhibition in ovarian cancer. Trends Cancer (2019) 5(9):524–28. doi: 10.1016/j.trecan.2019.06.004
137. Karzai F, VanderWeele D, Madan RA, Owens H, Cordes LM, Hankin A, et al. Activity of durvalumab plus olaparib in metastatic castration-resistant prostate cancer in men with and without DNA damage repair mutations. J Immunother Cancer (2018) 6(1):141. doi: 10.1186/s40425-018-0463-2
138. Sen T, Rodriguez BL, Chen L, Corte CMD, Morikawa N, Fujimoto J, et al. Targeting DNA damage response promotes antitumor immunity through STING-mediated T-cell activation in small cell lung cancer. Cancer Discov (2019) 9(5):646–61. doi: 10.1158/2159-8290.CD-18-1020
139. Ramalingam SS, Thara E, Awad MM, Dowlati A, Haque B, Stinchcombe TE, et al. JASPER: Phase 2 trial of first-line niraparib plus pembrolizumab in patients with advanced non-small cell lung cancer. Cancer (2022) 128(1):65–74. doi: 10.1002/cncr.33885
140. Seyedin SN, Hasibuzzaman MM, Pham V, Petronek MS, Callaghan C, Kalen AL, et al. Combination therapy with radiation and PARP inhibition enhances responsiveness to anti-PD-1 therapy in colorectal tumor models. Int J Radiat Oncol Biol Phys (2020) 108(1):81–92. doi: 10.1016/j.ijrobp.2020.01.030
141. Karukonda P, Odhiambo D, Mowery YM. Pharmacologic inhibition of ataxia telangiectasia and Rad3-related (ATR) in the treatment of head and neck squamous cell carcinoma. Mol Carcinog (2022) 61(2):225–38. doi: 10.1002/mc.23384
142. Dobler C, Jost T, Hecht M, Fietkau R, Distel L. Senescence induction by combined ionizing radiation and DNA damage response inhibitors in head and neck squamous cell carcinoma cells. Cells (2020) 9(9):2012. doi: 10.3390/cells9092012
143. Lee TW, Wong WW, Dickson BD, Lipert B, Cheng GJ, Hunter FW, et al. Radiosensitization of head and neck squamous cell carcinoma lines by DNA-PK inhibitors is more effective than PARP-1 inhibition and is enhanced by SLFN11 and hypoxia. Int J Radiat Biol (2019) 95(12):1597–612. doi: 10.1080/09553002.2019.1664787
144. Portugal J, Mansilla S, Bataller M. Mechanisms of drug-induced mitotic catastrophe in cancer cells. Curr Pharm Des (2010) 16(1):69–78. doi: 10.2174/138161210789941801
145. Kao M, Green C, Sidorova J, Méndez E. Strategies for targeted therapy in head and neck squamous cell carcinoma using WEE1 inhibitor AZD1775. JAMA Otolaryngol. Head Neck Surg (2017) 143(6):631–33. doi: 10.1001/jamaoto.2016.4563
146. van Harten AM, de Boer DV, Martens-de Kemp SR, Buijze M, Ganzevles SH, Hunter KD, et al. Chemopreventive targeted treatment of head and neck precancer by Wee1 inhibition. Sci Rep (2020) 10(1):2330. doi: 10.1038/s41598-020-58509-2
Keywords: head and neck squamous cell carcinoma (HNSCC), DNA damage response (DDR), PARP1 inhibitor, ATR and ATM inhibitor, DNA-PK inhibitor, WEE1 inhibitor
Citation: Lei H, He A, Jiang Y, Ruan M and Han N (2022) Targeting DNA damage response as a potential therapeutic strategy for head and neck squamous cell carcinoma. Front. Oncol. 12:1031944. doi: 10.3389/fonc.2022.1031944
Received: 30 August 2022; Accepted: 05 October 2022;
Published: 21 October 2022.
Edited by:
Tomoya Yokota, Shizuoka Cancer Center, JapanReviewed by:
Ichiro Ota, Kindai University Nara Hospital, JapanMasahiro Nakayama, University of Tsukuba, Japan
Copyright © 2022 Lei, He, Jiang, Ruan and Han. This is an open-access article distributed under the terms of the Creative Commons Attribution License (CC BY). The use, distribution or reproduction in other forums is permitted, provided the original author(s) and the copyright owner(s) are credited and that the original publication in this journal is cited, in accordance with accepted academic practice. No use, distribution or reproduction is permitted which does not comply with these terms.
*Correspondence: Yingying Jiang, amlhbmd5eUB3Zm1jLmVkdS5jbg==; Min Ruan, ZG9jdG9ycnVhbm1pbkBzanR1LmVkdS5jbg==; Nannan Han, aGFubmFubmFuMTExQDEyNi5jb20=
†These authors have contributed equally to this work and share first authorship