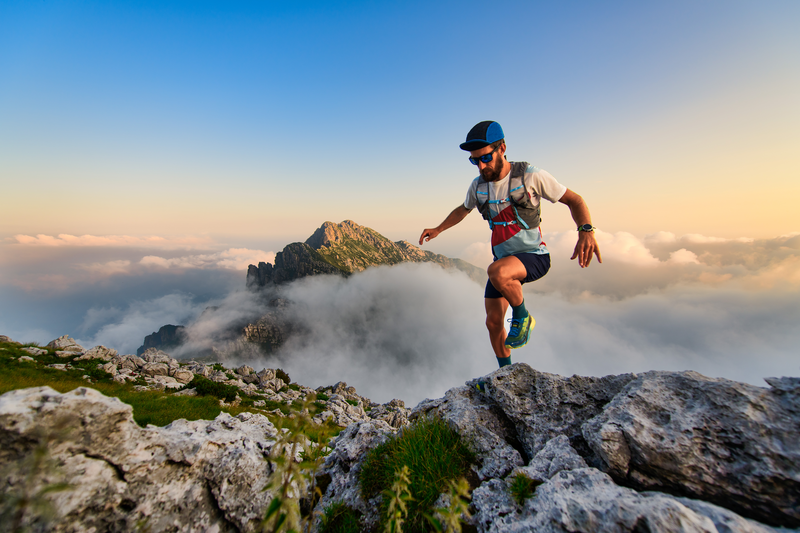
94% of researchers rate our articles as excellent or good
Learn more about the work of our research integrity team to safeguard the quality of each article we publish.
Find out more
REVIEW article
Front. Oncol. , 10 October 2022
Sec. Surgical Oncology
Volume 12 - 2022 | https://doi.org/10.3389/fonc.2022.1029830
This article is part of the Research Topic Surgery and COVID-19: Which strategies to apply in oncologic patients, Volume II View all 7 articles
COVID-19 infection caused by SARS-CoV-2 is considered catastrophic because it affects multiple organs, particularly those of the respiratory tract. Although the consequences of this infection are not fully clear, it causes damage to the lungs, the cardiovascular and nervous systems, and other organs, subsequently inducing organ failure. In particular, the effects of SARS-CoV-2-induced inflammation on cancer cells and the tumor microenvironment need to be investigated. COVID-19 may alter the tumor microenvironment, promoting cancer cell proliferation and dormant cancer cell (DCC) reawakening. DCCs reawakened upon infection with SARS-CoV-2 can populate the premetastatic niche in the lungs and other organs, leading to tumor dissemination. DCC reawakening and consequent neutrophil and monocyte/macrophage activation with an uncontrolled cascade of pro-inflammatory cytokines are the most severe clinical effects of COVID-19. Moreover, neutrophil extracellular traps have been demonstrated to activate the dissemination of premetastatic cells into the lungs. Further studies are warranted to better define the roles of COVID-19 in inflammation as well as in tumor development and tumor cell metastasis; the results of these studies will aid in the development of further targeted therapies, both for cancer prevention and the treatment of patients with COVID-19.
The development and spread of COVID-19 in the last 2 years have compelled scientists to focus on devising strategies for treating the infected patients as well as for managing the resultant health care emergency (1, 2). This reduced the scientists’ ability to focus on other important diseases, such as cancer (3, 3). Hospitals and universities have had to remodel themselves in the last few years, modifying their work and shifting their focus to studying the SARS-CoV-2- and COVID-related pathways and features (4, 5). Given that patients with COVID-19 required urgent medication and hospitalization, those with other morbidities were deprioritized, and their surgeries were rescheduled. This has impacted the collection of biological samples (fluids and tissues) for research, especially in the field of thoracic oncology (6, 7). Moreover, some studies have shown that the incidence of lung cancer was higher among patients who contracted COVID-19 than among those who did not and that the risk of developing severe illness and death was greater among patients with lung cancer than among those with other cancers (8, 9). This has steered the scientific community toward studying the possible connections between SARS-CoV-2 and lung cancer (10). The increased incidence of lung cancer among patients with COVID-19 is likely attributable to the severe immunosuppression caused by the virus, changes related to the inflammatory components and the cascade of immunogenic events activated by the virus, and, finally but not less importantly, the possibility that the virus is oncogenic (11, 12). A particularly interesting concept is that that an external factor, such as a viral infection that causes multiple changes to the microenvironment (e.g., extracellular matrix remodeling and cell-to-cell interactions especially with the immune system), awakens dormant cancer cells from quiescence (13, 14). This state of dormancy is characterized by tumor cells that no longer undergo cell division but are inactive in the G0–G1 state; however, an appropriate stimulus can trigger proliferation (13, 14). This theory is related to drug resistance and metastatic niche activation, which should be taken seriously in the case of lung cancer, especially, due to its capacity to disseminate to primary organs (13, 14).
Hence, understanding the biology of infection with SARS-CoV-2 among patients with lung cancer and their response to COVID-19 vaccines is of utmost importance (15–17). One of the most intriguing aspects of COVID-19 is that the expelled respiratory droplets containing the virus may settle on different surfaces or be suspended in the air, thereby facilitating further contamination (18). Moreover, the virus can survive on several surfaces and in different organs and tissues, contributing to the contagiousness of the disease (19). Apart from the bronchopulmonary tree, SARS-CoV-2 RNA has been detected in the head, digestive tract, nervous system, heart, and blood vessels, among others (20). Therefore, considering the easy transmissibility and virulence of SARS-CoV-2, it is imperative to observe strict hand-washing hygiene (21).
Furthermore, the virus can quickly modify its nature, and scientists are thus using modern techniques, such as next-generation sequencing, for routine viral DNA analysis (22–24). This approach is used for liquid biopsy samples and in case of autopsies with suspected infections, following the biosafety level 3 guidelines (22–25). The use of liquid biopsies allows new insights, and regulations have been adopted to protect pathologists and technicians in the last few years (25, 26). However, because SARS-CoV-2 has a high degree of virulence, the use of pneumatic air tube transport systems to deliver pathology samples from the hospitals to the laboratories is not allowed (25, 26). This problem has been discussed in numerous countries, including the United States, Spain, Italy, Germany, Portugal, Denmark, Scotland, the Netherlands, and Slovenia, although some centers are still using pneumatics to transfer pathology samples (26). The challenges associated with establishing new procedures and rules for managing COVID-19 have helped introduce new approaches on a global scale, through online webinars and conferences, and adaptations that encompass different approaches to be followed at hospitals and in laboratories (27, 28).
Despite the numerous vaccines that have been administrated in countries worldwide, new variants continue to spread globally; moreover, although fewer devastating outcomes are being reported, the infection rate is still increasing, and the effects of this virus on the human body, particularly on the lungs, have not been fully elucidated (29). The scientists are still trying to understand the roles of immunity in the progression and resolution of COVID-19 as well as tumor development (30, 31). Several studies have been conducted recently to comprehend the possible roles of the virus in cancer growth; however, at the moment, the scientific community scientists have no definitive findings to share (10–12).
One of the most considered problems during the first wave of COVID-19 was the overcrowding in intensive care units (ICUs), which the governments decided to close for all non-COVID-19 patients (32). The primary symptoms of COVID-19 include acute respiratory failure and acute respiratory distress syndrome (ARDS), which are the main reasons for admission to the ICUs (33). Patients with such severe symptoms required protective low-tidal volume and mechanical ventilation, which is considered the standard of care for moderate-to-severe ARDS (33, 34). Moreover, anesthesiologists expressed the need to establish additional respiratory support strategies to ensure the optimal use of these machines at low volumes (35). The government discussed this problem with other countries, and several invasive and noninvasive devices were shipped from China, North America, and other countries (36).
In normal lung functioning, in which breathing is a cyclic process driven by respiratory muscles, viscoelastic tissues get deformed during normal inspiration and are relieved when the pressure returns to the initial situation during expiration (37). Under nonphysiological conditions, when the pulmonary tissue deformation is greater than normal, the resultant stress, represented by the transpulmonary pressure in association with the global strain, may affect the lungs (37). In fact, under pathological conditions, strain and stress may cause ventilator-induced lung injury (38). In particular, high volumes of nonphysiological conditions may be harmful to the lung, increasing the risk of mortality among patients with ARDS (38). Throughout the pandemic, anesthesiologists in ICUs and pneumologists have uncovered knowledge that has led to the identification of different approaches for caring for patients with COVID-19 (39).
The most important feature to consider in an injured lung is the inhomogeneity of ventilation, which increases tissue damage, resulting in severe worsening of pulmonary gas exchange, thus promoting respiratory insufficiency in patients with ARDS (40). This vicious circle increases the stress on tissues, intensifying lung damage. Moreover, the inhomogeneous distribution of the opening pressure may overstretch the lungs and cause aerated areas to connect with poorly aerated lung regions (38, 41). This phenomenon associated with COVID-19 infection caused severe problems in ventilation, resulting in several deaths during the last waves of the pandemic (42).
A new four-dimensional tomographic approach can analyze the biomechanical setting of the lungs, defining the volume distribution, especially in the nonventilated lung regions (43, 44). A recent study conducted using healthy rats with spontaneous breathing reported heterogeneity in the volume, with consequent quantification of the deformation of lung areas and progression in time (35). The different ventilation in the various lung regions may be attributable to strain and stress, although other factors, such as alveolar–capillary barrier integrity, were considered (37). Upon follow-up with micro-computed tomography images, comparing animals with spontaneous breathing through controlled and uncontrolled mechanical ventilation, moderate-to-severe lung injury was observed in the nonaerated lung compartments (37); furthermore, the study findings demonstrated significant progression of the regional volumetric strain and heterogeneity after spontaneous breathing, with subsequent damage to the lung alveoli (37). Other studies were conducted to analyze lung heterogeneity in association with the severity of ARDS and subsequent mortality, particularly in relation to alveolar wall disruption, hemorrhage, hyperemia, and inflammation (45–48). Recently, researchers attempted to define a gene expression pathway related to lung homogeneity, although the gene expression is generally related to specific lung regions, in contrast with the damage, which is ubiquitous (49). This can perhaps be explained by the fact that biomarkers are mainly water soluble and diffusible in blood and in bronchial secretions (50–52).
Gattinoni et al. defined two phenotypes characterizing patients with COVID-19 infection in the lung: “non-ARDS” or “type 1” and “ARDS” or “type 2” (53, 54). However, although mechanical support is very important for patients with COVID-19 infection, it is not feasible to advocate a single guideline for this condition (55). Rather, the approach needs to be tailored to every individual patient to prevent serious complications (55, 56).
The pathophysiology of this virus is often characterized by respiratory failure; it is variable with moderate-to-severe hypoxemia (57). Accordingly, as initial respiratory support, the primary treatment choices include oxygen therapy, high-flow nasal cannula, and noninvasive ventilation. If these methods fail, mechanical ventilation becomes mandatory for improving lung ventilation, which is compromised upon infection with SARS-CoV-2 (58). One of the main strategies that has been adopted in the ICU to promote oxygenation is that the patients are maintained in the prone position, which also improves the functional residual capacity and ventilation/perfusion. This approach is also used in awake patients, although the associated principles have not been fully defined (58, 59).
In the last 2 years, the biological differentiation of SARS-CoV-2 has resulted in several DNA mutations, leading to different levels of viral virulence, with variable clinical consequences for patients (60–62). Studies have reported increasing evidence of genetic susceptibility to SARS and to several genomic differences in COVID-19 patients, which facilitate the entrance of the virus in patients with cancer (63). Moreover, the immunity of patients with cancer is low, and they are at a higher risk of exposure to infections, including COVID-19 (64). The lungs are the primary target of novel coronaviruses, and the scientific community is trying to define the roles of genetic mutations and immunity in patients contracting COVID-19 and related cancers (65–67). However, because the associated processes are extremely complicated, it is currently not possible to determine whether this virus plays a role in cancer development, although COVID-19 combined with a weakened immunity creates favorable conditions for cancer development (68, 69).
It has been recently reported that angiotensin-converting enzyme-2 (ACE2), and plasminogen-activator inhibitor type 1 (PAI-1), which have been proposed as receptors on mammalian host cells and seem to promote inflammation, angiogenesis, and coagulation, are the main molecular targets of the spike (S) protein of SARS-CoV-2 (70–72) (Figure 1). The serine protease transmembrane serine protease 2 (TMPRSS2), which seems to activate the S protein to enter into the cells, was also recently introduced as a molecular target (72–74). Moreover, the interaction between the virus and ACE2 receptor seems to be driven by the FURIN/PCSK3 cleavage of the S protein, facilitating viral invasion (75–77) (Figure 1). Basigin/CD147, a receptor glycoprotein of the immunoglobulin superfamily that acts as a mediator of the viral infection, was also reported recently (Figure 1) (78).
Figure 1 Graphical illustration of the expression of the key proteins in lung cancer and COVID-19. ACE2 and PAI-1 are expressed at high levels in lung cancer and cause inflammation and neo-angiogenesis in COVID-19. TMPRSS2 helps SARS-COV-2 to enter the host cells, whereas CD147 and FURIN are associated with genomic susceptibility to COVID‐19 in patients with lung cancer.
Besides studying these important protein receptors, which are considered to drive the entry of the virus into cells, researchers have also started to analyze the molecular profiles of the genes associated with the mutational patterns of the relevant targets (79, 80). These considerations were derived from the recent discovery of a possible correlation between the membrane proteins and lung adenocarcinoma (LUAD) and lung squamous carcinoma (LUSC) (81, 82). Specifically, these mutations have not only been detected but are also related to patient survival. In particular, a high percentage of genes targeting mutations have been found in the ACE2, TMPRSS2, CD147/BSG, and FURIN/PCSK3 genes (83).
For example, the TMPRSS2 gene expression was significantly reduced in patients with LUAD as compared with healthy patients, although the ACE2 expression was higher in the former group (84–86). Moreover, the TMPRSS2 gene expression was significantly reduced in patients with LUSC as compared with healthy patients, although the ACE2 expression was high in the patients with both LUAD and LUSC (87). No significant differences were noted in the expression of the CD147 and FURIN genes (88). Thus, defining the genomic susceptibility of patients with lung cancer to COVID‐19 remains challenging, and more experiments are warranted to assess the mechanisms of viral invasion, which may be the key to devising future treatments against the infections caused by SARS‐CoV‐2 (83, 89).
A significant amount of scientific literature has highlighted the possible involvement of ACE2 and TMPRSS2 gene mutations in cancer development (90). Specifically, in 2020, Stewart et al. (91), using different models of normal and malignant cells from the aerodigestive and respiratory tracts, respectively, found that ACE2 expression is highly correlated with the transcriptional, microRNA (miRNA), and metabolic signifiers of epithelial differentiation (91, 92). Moreover, the regulators of epithelial-to-mesenchymal transition (EMT) may play a role in the modulation of ACE2 expression. This biunivocal correlation may exert effects on infection caused by SARS-CoV-2 owing to the fact that it the virus reportedly increases EMT gene expression and metabolic alterations (93, 94). The association between COVID-19 and some gene variants directly affects the virus as well as lung involvement; moreover, the correlation between these proteins and the COVID-19-related EMT gene expression needs to be urgently explored by the scientific community to uncover the possible solutions and develop future targeted treatments (95).
One of the most important aspects related to COVID-19 is the cytokine storm, during which various inflammatory cytokines are developed, and the consequent cytokine release syndrome (CRS), which represents an acute attack of excessive cytokines from the immune system triggered by inflammatory responses and the immunity (96, 97). The CRS is characterized by high fever, erythema, edema, extreme fatigue, and nausea, which may also be associated with sepsis and multiple organ failure (98). The first approach by the cytokines and chemokines represents an important step against the viral infection; in particular, macrophages function as sentinels in the lungs, and SARS-CoV2 can infect these to induce this cascade as an early pathogenic mechanism (99, 100). The virus infects the dendritic cells, thereby inducing a cascade of cytokines and chemokines, including CCL3, CCL5, CCL2, and CXCL10 (23), which are the key components in the chemotactic approach induced by neutrophils, monocytes, and T cells, thereby calibrating a dysregulated response against infections caused by SARS-CoV-2 (101).
In particular, the immunodeficiency or the altered immune cell response induced by infection with this virus may lead to vivid outcomes among patients with cancer, owing to the complex relationship between the virus and cancer (102). Furthermore, patients with response have a higher risk of exposure and susceptibility to infections as compared with the normal population, making them more vulnerable to infection with SARS-CoV-2 (103). The main consequences of this factor include the overlapping of infections, an altered immune response, and faster progression of the virus and cancer; in addition to these aspects, a higher risk has been reported among patients with obesity, cardiovascular disease, hypertension, diabetes, and other comorbidities, which induce further complications during COVID-19 (104). Moreover, the scientific community has recently identified that the lung cancer population is at higher risk of contracting COVID-19 and that the aberrant expression of ACE2 in lung carcinoma renders the patients more susceptible to COVID-19 (105, 106). By definition, ACE2 is a regulator of the renin–angiotensin system and is located in a small subset of alveolar type II cells; its basic function is to convert angiotensin II into angiotensin (107, 108). Likewise, it has been reported that SARS-CoV-2 can attach to the ACE2 receptor through its S protein and thereby enter the cells (109, 110). Although ACE2 is also expressed in other organs such as the heart, kidneys, and intestine, the lungs are its target organ (111). Invasion by SARS-CoV-2 induces a lung immunoreaction against the virus, with the activation and amplification of host immunity, consequently inducing a cascade of cytokines, including interferon-γ, tumor necrosis factor, interleukin (IL)-1, IL-6, and IL-18, all of which play important roles in the toll-like receptor signaling pathway (Figure 2) (112, 113). This high level of cytokines induces ARDS and organ failure with consequent death of the patient; particularly, the presence of the virus increases lung cancer cell apoptosis, thereby downregulating the expression of ACE2 and causing altered vascular permeability, neutrophil infiltration, and lung edema (114). Interestingly, the maintenance of the ACE2 serum levels improves the survival of patients with lung cancer, increasing the immune system’s capacity to induct an inflammatory storm that can eliminate SARS-CoV-2 from the lungs (115). Finally, ACE2 may be considered a biomarker and future therapeutic target against COVID-19 infection, although several studies need to be conducted to confirm this aspect (116).
Figure 2 Invasion with SARS-CoV-2. The ACE2 receptor is the channel through which the virus attaches to the cells via its S protein and enters the cell. A massive immune response through the amplification of NK and T cells is thereby triggered, leading to the production of a wide range of cytokines, such as interferon-γ, tumor necrosis factor, interleukin (IL)-1, IL-6, and IL-18, which are responsible for the cytokine storm.
Apart from the importance of ACE2 in the pathogenesis of the novel coronavirus, a higher percentage of patients with lung cancer were found to have contracted COVID-19 (117). A recent study analyzed 2000 patients with COVID-19 and found that 1% of those had a history of lung cancer, indicating that patients with cancer may be susceptible to COVID-19 (118). A panel of genes, including SLC6A20, LZTFL1, CCR9, FYCO1, CXCR6, XCR1, ABO, RPL24, FOXP4, TMEM65, OAS1, KANSL1, TAC4, DPP9, RAVER1, PLEKHA4, and IFNAR2, were assessed for their mRNA expression to study a possible connection with COVID-19 and lung cancer (119). At the end of this analysis, immunohistochemistry combined with a comparison with the Human Protein Atlas helped validate only six genes (SLC6A20, FYCO1, FOXP4, TMEM65, XCR1, and OAS1) with significantly different protein expression levels (118). This finding suggests a potential genetic predisposition between COVID-19 and lung cancer, indicating that patients with lung cancer have a higher risk of contracting COVID-19 (120). Accordingly, a complex bioinformatics analysis assessing COVID-19 and different lung cancers was performed, also correlating with the severity of cancer, to apply knowledge and invest efforts to fight this pandemic infection, especially scouting out genetic association with it.
Because of this, an interesting aspect that needs to be considered is the use of anticancer drugs in patients with concomitant COVID-19 infection, contributing to the overall complexity of this scenario in the potential cross-interference between COVID-19 and lung cancer treatments (121). The scientific community is trying to define the main correlations among all of these aspects, although no definitive data are available currently (121, 122).
In this context, medical approaches, such as chemotherapy, immunotherapy, and radiotherapy, may play a dual role in affecting the targeted organs as well as the immunity of patients with cancer (123). In particular, immune checkpoint inhibitors (ICIs), which are considered promising against thoracic malignancies, may play important roles in inducing an immunomodulatory effect (124). The upregulation of T cells and the concomitant expression of PD-1, which can identify exhausted T cell subpopulations, both of which contribute to acute viral infection, have been reported in the early stage of COVID-19 infection. Accordingly, CTLA-4 or the PD-1/PD-ligand (L)-1 axis may enhance the expression of CD4 and CD8, reinforcing the exhausted T cells and better supporting the potential effect of T-lymphocytes against COVID-19 infection (121). However, this may result in boomerang effects given that immune system enhancement can result in a tremendously inflammatory stage–the cytokine storm–to fight against infection caused by SARS-CoV-2. Indeed, the most interesting aspect of ICIs is their ability to improve the “early phase of COVID-19,” thus avoiding possible dangerous immune responses to the viral infection (125).
Encouraging results have also been reported in patients with HIV, HBV, and/or HCV infections treated with ICIs during the COVID-19 pandemic; no adverse effects were noted in these patients in terms of viral infection and non-viral reactivation (126). The TERAVOLT study, an interesting investigation that is still in the preliminary stages, has reported no effects on the survival of patients with cancer treated with chemo-targeted therapies and ICIs and infected with COVID-19 (127). Besides the possible uses of the available medical treatments against cancer, in patients who are ineligible for targeted therapy, targeted ICIs alone or in combination with platinum are considered the gold standard, especially in terms of infection with SARS-CoV-2 (128). Moreover, the use of chemotherapy within one month preceding the diagnosis of COVID-19 is reportedly associated with the occurrence of severe infection and consequent complications (129).
Considering all the consequences of infection with SARS-CoV-2 on patients with cancer, it is very important to inform and train the COVID-19 frontline workers, including pulmonologists, infectious disease specialists, anesthesiologists, and radiologists, to select the best approaches and treatments, tailored to specific clinical cases. This approach may prove to be extremely beneficial for avoiding further complications related to drug treatment, which may induce immunomodulatory effects favoring cancer and viral infection. However, the data regarding the potential interference between patients with cancer and COVID-19 are not definitive. Consequently, the real dilemma for oncologists is to find a balance between protecting patients using the most effective therapies and reducing the risks of COVID-19 exposure and/or infection. In this direction, the international oncological societies worldwide have set several recommendations to guide clinicians to find a balance in terms of the safety and efficacy of cancer treatment and rescue therapy against viral infections (130). The most interesting approach related to these guidelines is based on the possibility of follow-up or evaluating patients using the telemedicine services to contain the number of patients in hospitals and, consequently, the risk of infection (131, 132). In these 2 years of the pandemic, we have witnessed new approaches and considerations for patients with severe infections as well as those with cancer, who need to be cured in real time despite the serious pandemic situation. This challenging scenario has opened up new avenues for the scientific community to bring innovation and progress further in the field of medicine.
The idea for the manuscript was conceived by BA and was further developed by VM, AVS. BA, VM, AVS, RT, and SC. EC, FS, and MD reviewed the manuscript All authors contributed to the article and approved the submitted version.
The Authors thank Prozetesis.org for the support.
The authors declare that the research was conducted in the absence of any commercial or financial relationships that could be construed as a potential conflict of interest.
All claims expressed in this article are solely those of the authors and do not necessarily represent those of their affiliated organizations, or those of the publisher, the editors and the reviewers. Any product that may be evaluated in this article, or claim that may be made by its manufacturer, is not guaranteed or endorsed by the publisher.
DCCs, dormant cancer cells; NETs, neutrophil extracellular traps; ARF, acute respiratory failure; ARDS, acute respiratory distress syndrome; HFNC, high flow nasal cannula; NIV, noninvasive ventilation; FCR, functional residual capacity; ACE 2, angiotensin-converting enzyme-2; LUAD, lung adenocarcinoma; LUSC, lung squamous carcinoma; TMPRSS2, Transmembrane Serine Protease 2; EMT, epithelial-to-mesenchymal transition; DCs, dendritic cells.
1. Cascella M, Rajnik M, Aleem A, Dulebohn SC, Di Napoli R. Features, evaluation, and treatment of coronavirus (COVID-19). In: StatPearls. Treasure Island (FL): StatPearls Publishing (2022). p. 32150360.
2. Cucinotta D, Vanelli M. WHO declares COVID-19 a pandemic. Acta Biomed (2020) 91(1):157–60. doi: 10.23750/abm.v91i1.9397
3. Maringe C, Spicer J, Morris M, Purushotham A, Nolte E, Sullivan R, et al. The impact of the COVID-19 pandemic on cancer deaths due to delays in diagnosis in England, UK: A national, population-based, modelling study. Lancet Oncol (2020) 21(8):1023–34. doi: 10.1016/S1470-2045(20)30388-0
4. Telenti A, Arvin A, Corey L, Corti D, Diamond MS, García-Sastre A, et al. After the pandemic: Perspectives on the future trajectory of COVID-19. Nature (2021) 596:495–504. doi: 10.1038/s41586-021-03792-w
5. Maison D, Jaworska D, Adamczyk D, Affeltowicz D. The challenges arising from the COVID-19 pandemic and the way people deal with them. A Qual longitudinal study. PloS One (2021) 16(10):e0258133. doi: 10.1371/journal.pone.0258133
6. Dingemans AC, Soo RA, Jazieh AR, Rice SJ, Kim YT, Teo LLS, et al. Treatment guidance for patients with lung cancer during the coronavirus 2019 pandemic. J Thorac Oncol (2020) 15(7):1119–36. doi: 10.1016/j.jtho.2020.05.001
7. De Simone B, Chouillard E, Sartelli M, Biffl WL, Di Saverio S, Moore EE, et al. The management of surgical patients in the emergency setting during COVID-19 pandemic: The WSES position paper. World J Emerg Surg (2021) 16:14. doi: 10.1186/s13017-021-00349-0
8. Lemos AEG, Silva GR, Gimba ERP, Matos ADR. Susceptibility of lung cancer patients to COVID-19: A review of the pandemic data from multiple nationalities. Thorac Cancer (2021) 12(20):2637–47. doi: 10.1111/1759-7714.14067
9. Venkatesulu BP, Chandrasekar VT, Girdhar P, Advani P, Sharma A, Elumalai T, et al. A systematic review and meta-analysis of cancer patients affected by a novel coronavirus. medRxiv [Preprint] (2020) 29:2020.05.27.20115303. doi: 10.1101/2020.05.27.20115303
10. Peravali M, Joshi I, Ahn J, Kim C. A systematic review and meta-analysis of clinical characteristics and outcomes in patients with lung cancer with coronavirus disease 2019. JTO Clin Res Rep (2021) 2(3):100141. doi: 10.1016/j.jtocrr.2020.100141
11. Han S, Zhuang Q, Chiang J, Tan SH, Chua GWY, Xie C, et al. Impact of cancer diagnoses on the outcomes of patients with COVID-19: A systematic review and meta-analysis. BMJ Open (2022) 12(2):e044661. doi: 10.1136/bmjopen-2020-044661
12. Belsky JA, Tullius BP, Lamb MG, Sayegh R, Stanek JR, Auletta JJ. COVID-19 in immunocompromised patients: A systematic review of cancer, hematopoietic cell and solid organ transplant patients. J Infect (2021) 82(3):329–38. doi: 10.1016/j.jinf.2021.01.022
13. Park SY, Nam JS. The force awakens: Metastatic dormant cancer cells. Exp Mol Med (2020) 52:569–81. doi: 10.1038/s12276-020-0423-z
14. Barkan D, El Touny LH, Michalowski AM, Smith JA, Chu I, Davis AS, et al. Metastatic growth from dormant cells induced by a col-i-enriched fibrotic environment. Cancer Res (2010) 70:5706–16. doi: 10.1158/0008-5472.CAN-09-2356
15. Rolfo C, Meshulami N, Russo A, Krammer F, García-Sastre A, Mack PC, et al. Lung cancer and severe acute respiratory syndrome coronavirus 2 infection: Identifying important knowledge gaps for investigation. J Thorac Oncol (2022) 17(2):214–27. doi: 10.1016/j.jtho.2021.11.001
16. Luo J, Rizvi H, Preeshagul IR, Egger JV, Hoyos D, Bandlamudi C, et al. COVID-19 in patients with lung cancer. Ann Oncol (2020) 31(10):1386–96. doi: 10.1016/j.annonc.2020.06.007
17. Bora VR, Patel BM. The deadly duo of COVID-19 and cancer! Front Mol Biosci (2021) 8:643004. doi: 10.3389/fmolb.2021.643004
18. Chia PY, Coleman KK, Tan YK, Ong SWX, Gum M, Lau SK, et al. Singapore 2019 novel coronavirus outbreak research team. Detection of air and surface contamination by SARS-CoV-2 in hospital rooms of infected patients. Nat Commun (2020) 11(1):2800. doi: 10.1038/s41467-020-16670-2
19. Hu B, Guo H, Zhou P, Shi ZL. Characteristics of SARS-CoV-2 and COVID-19. Nat Rev Microbiol (2021) 19:141–54. doi: 10.1038/s41579-020-00459-7
20. Trypsteen W, Van Cleemput J, Snippenberg WV, Gerlo S, Vandekerckhove L. On the whereabouts of SARS-CoV-2 in the human body: A systematic review. PloS Pathog (2020) 16(10):e1009037. doi: 10.1371/journal.ppat.1009037
21. Kumar A, Narayan RK, Prasoon P, Kumari C, Kaur G, Kumar S, et al. COVID-19 mechanisms in the human body-what we know so far. Front Immunol (2021) 12:693938. doi: 10.3389/fimmu.2021.693938
22. Datta S, Budhauliya R, Das B, Chatterjee S, Veer V. Next-generation sequencing in clinical virology: Discovery of new viruses. World J Virol (2015) 4(3):265–76. doi: 10.5501/wjv.v4.i3.265
23. Radford AD, Chapman D, Dixon L, Chantrey J, Darby AC, Hall N. Application of next-generation sequencing technologies in virology. J Gen Virol (2012) 93(Pt 9):1853–68. doi: 10.1099/vir.0.043182-0
24. Charre C, Ginevra C, Sabatier M, Regue H, Destras G, Brun S, et al. Evaluation of NGS-based approaches for SARS-CoV-2 whole genome characterisation. Virus Evol (2020) 6(2):veaa075. doi: 10.1093/ve/veaa075
25. Rakislova N, Marimon L, Ismail MR, Carrilho C, Fernandes F, Ferrando M, et al. Minimally invasive autopsy practice in COVID-19 cases: Biosafety and findings. Pathogens (2021) 10(4):412. doi: 10.3390/pathogens10040412
26. Hofman P. Challenges and issues surrounding the use for translational research of human samples obtained during the COVID-19 pandemic from lung cancer patients. Transl Lung Cancer Res (2020) 9(4):1543–53. doi: 10.21037/tlcr-20-594
27. García-Morales VJ, Garrido-Moreno A, Martín-Rojas R. The transformation of higher education after the COVID disruption: Emerging challenges in an online learning scenario. Front Psychol (2021) 12:616059. doi: 10.3389/fpsyg.2021.616059
28. Al-Balas M, Al-Balas HI, Jaber HM, Obeidat K, Al-Balas H, Aborajooh EA, et al. Distance learning in clinical medical education amid COVID-19 pandemic in Jordan: Current situation, challenges, and perspectives. BMC Med Educ (2020) 20(1):341. doi: 10.1186/s12909-020-02257-4
29. Aleem A, Akbar Samad AB, Slenker AK. Emerging variants of SARS-CoV-2 and novel therapeutics against coronavirus (COVID-19). In: StatPearls. Treasure Island (FL: StatPearls Publishing (2022).
30. Schultze JL, Aschenbrenner AC. COVID-19 and the human innate immune system. Cell (2021) 184(7):1671–92. doi: 10.1016/j.cell.2021.02.029
31. Sette A, Crotty S. Adaptive immunity to SARS-CoV-2 and COVID-19. Cell (2021) 184(4):861–80. doi: 10.1016/j.cell.2021.01.007
32. Riguzzi M, Gashi S. Lessons from the first wave of COVID-19: Work-related consequences, clinical knowledge, emotional distress, and safety-conscious behavior in healthcare workers in Switzerland. Front Psychol (2021) 12:628033. doi: 10.3389/fpsyg.2021.628033
33. Li X, Ma X. Acute respiratory failure in COVID-19: Is it "typical" ARDS? Crit Care (2020) 24(1):198. doi: 10.1186/s13054-020-02911-9
34. Aslan A, Aslan C, Zolbanin NM, Jafari R. Acute respiratory distress syndrome in COVID-19: Possible mechanisms and therapeutic management. Pneumonia (2021) 13:14. doi: 10.1186/s41479-021-00092-9
35. Silva PL, Rocco PRM. The basics of respiratory mechanics: Ventilator-derived parameters. Ann Transl Med (2018) 6(19):376. doi: 10.21037/atm.2018.06.06
36. Branson R, Dichter JR, Feldman H, Devereaux A, Dries D, Benditt J, et al. The US strategic national stockpile ventilators in coronavirus disease 2019: A comparison of functionality and analysis regarding the emergency purchase of 200,000 devices. Chest (2021) 159(2):634–52. doi: 10.1016/j.chest.2020.09.085
37. Cruces P, Retamal J, Hurtado DE, Erranz B, Iturrieta P, González C, et al. A physiological approach to understand the role of respiratory effort in the progression of lung injury in SARS-CoV-2 infection. Crit Care (2020) 24(1):494. doi: 10.1186/s13054-020-03197-7
38. Lutfi MF. The physiological basis and clinical significance of lung volume measurements. Multidiscip Respir Med (2017) 12:3. doi: 10.1186/s40248-017-0084-5
39. Guessoum SB, Marvaldi M, Thomas I, Lachal J, Carretier E, Moro MR, et al. The experience of anaesthesiology care providers in temporary intensive care units during the COVID-19 pandemic in France: A qualitative study. Anaesth Crit Care Pain Med (2022) 41(3):101061. doi: 10.1016/j.accpm.2022.101061
40. Basem JI, Roth AF, White RS, Tangel VE, Jiang SY, Choi JM, et al. Patient care in rapid-expansion intensive care units during the COVID-19 pandemic crisis. BMC Anesthesiol (2022) 22:209. doi: 10.1186/s12871-022-01752-z
41. Fardin L, Broche L, Lovric G, Mittone A, Stephanov O, Larsson A, et al. Imaging atelectrauma in ventilator-induced lung injury using 4D X-ray microscopy. Sci Rep (2021) 11(1):4236. doi: 10.1038/s41598-020-77300-x
42. Maes M, Higginson E, Pereira-Dias J, Curran MD, Parmar S, Khokhar F, et al. Ventilator-associated pneumonia in critically ill patients with COVID-19. Crit Care (2021) 25:25. doi: 10.1186/s13054-021-03460-5
43. Sharifi H, McDonald GC, Lee JK, Ajlouni MI, Chetty IJ, Zhong H. Four-dimensional computed tomography-based biomechanical measurements of pulmonary function and their correlation with clinical outcome for lung stereotactic body radiation therapy patients. Quant Imaging Med Surg (2019) 9(7):1278–87. doi: 10.21037/qims.2019.07.03
44. Fleming J, Conway J, Majoral C, Bennett M, Caillibotte G, Montesantos S, et al. Determination of regional lung air volume distribution at mid-tidal breathing from computed tomography: A retrospective study of normal variability and reproducibility. BMC Med Imaging (2014) 14:25. doi: 10.1186/1471-2342-14-25
45. Mariano CA, Sattari S, Maghsoudi-Ganjeh M, Tartibi M, Lo DD, Eskandari M. Novel mechanical strain characterization of ventilated ex vivo porcine and murine lung using digital image correlation. Front Physiol (2020) 11:600492. doi: 10.3389/fphys.2020.600492
46. Bates JHT, Smith BJ. Ventilator-induced lung injury and lung mechanics. Ann Transl Med (2018) 6(19):378. doi: 10.21037/atm.2018.06.29
47. Pellegrini M, Larina A, Mourtos E, Frithiof R, Lipcsey M, Hultström M, et al. A quantitative analysis of extension and distribution of lung injury in COVID-19: A prospective study based on chest computed tomography. Crit Care (2021) 25(1):276. doi: 10.1186/s13054-021-03685-4
48. Akgül AG, Şahin D, Temel U, Eliçora A, Dillioğlugil M, Maral Kır H, et al. Effect of nitric oxide synthase inhibitors in acute lung injury due to blunt lung trauma in rats. Turk Gogus Kalp Damar Cerrahisi Derg (2019) 27(1):63–72. doi: 10.5606/tgkdc.dergisi.2019.15936
49. Fassan M, Collesei A, Angerilli V, Sbaraglia M, Fortarezza F, Pezzuto F, et al. Multi-design differential expression profiling of COVID-19 lung autopsy specimens reveals significantly deregulated inflammatory pathways and SFTPC impaired transcription. Cells (2022) 11(6):1011. doi: 10.3390/cells11061011
50. Daamen AR, Bachali P, Owen KA, Kingsmore KM, Hubbard EL, Labonte AC, et al. Comprehensive transcriptomic analysis of COVID-19 blood, lung, and airway. Sci Rep (2021) 11(1):7052. doi: 10.1038/s41598-021-86002-x
51. Kermali M, Khalsa RK, Pillai K, Ismail Z, Harky A. The role of biomarkers in diagnosis of COVID-19 - a systematic review. Life Sci (2020) 254:117788. doi: 10.1016/j.lfs.2020.117788
52. Samprathi M, Jayashree M. Biomarkers in COVID-19: An up-To-Date review. Front Pediatr (2021) 8:607647. doi: 10.3389/fped.2020.607647
53. Gattinoni L, Chiumello D, Caironi P, Busana M, Romitti F, Brazzi L, et al. COVID-19 pneumonia: Different respiratory treatments for different phenotypes? Intensive Care Med (2020) 46(6):1099–102. doi: 10.1007/s00134-020-06033-2
54. Gattinoni L, Chiumello D, Rossi S. COVID-19 pneumonia: ARDS or not? Crit Care (2020) 24(1):154. doi: 10.1186/s13054-020-02880-z
55. Spruit MA, Holland AE, Singh SJ, Tonia T, Wilson KC, Troosters T. COVID-19: Interim guidance on rehabilitation in the hospital and post-hospital phase from a European respiratory society and American thoracic society-coordinated international task force. Eur Respir J (2020) 56(6):2002197. doi: 10.1183/13993003.02197-2020
56. Estenssoro E, Loudet CI, Dubin A, Kanoore Edul VS, Plotnikow G, Andrian M, et al. Clinical characteristics, respiratory management, and determinants of oxygenation in COVID-19 ARDS: A prospective cohort study. J Crit Care (2022) 71:154021. doi: 10.1016/j.jcrc.2022.154021
57. Swenson KE, Swenson ER. Pathophysiology of acute respiratory distress syndrome and COVID-19 lung injury. Crit Care Clin (2021) 37(4):749–76. doi: 10.1016/j.ccc.2021.05.003
58. Luján M, Sayas J, Mediano O, Egea C. Non-invasive respiratory support in COVID-19: A narrative review. Front Med (Lausanne) (2022) 8:788190. doi: 10.3389/fmed.2021.788190
59. Fossali T, Pavlovsky B, Ottolina D, Colombo R, Basile MC, Castelli A, et al. Effects of prone position on lung recruitment and ventilation-perfusion matching in patients with COVID-19 acute respiratory distress syndrome: A combined CT Scan/Electrical impedance tomography study. Crit Care Med (2022) 50(5):723–32. doi: 10.1097/CCM.0000000000005450
60. Harvey WT, Carabelli AM, Jackson B, Gupta RK, Thomson EC, Harrison EM, et al. SARS-CoV-2 variants, spike mutations and immune escape. Nat Rev Microbiol (2021) 19:409–24. doi: 10.1038/s41579-021-00573-0
61. Hoteit R, Yassine HM. Biological properties of SARS-CoV-2 variants: Epidemiological impact and clinical consequences. Vaccines (Basel) (2022) 10(6):919. doi: 10.3390/vaccines10060919
62. Akkiz H. Implications of the novel mutations in the SARS-CoV-2 genome for transmission, disease severity, and the vaccine development. Front Med (Lausanne) (2021) 8:636532. doi: 10.3389/fmed.2021.636532
63. SeyedAlinaghi S, Mehrtak M, MohsseniPour M, Mirzapour P, Barzegary A, Habibi P, et al. Genetic susceptibility of COVID-19: A systematic review of current evidence. Eur J Med Res (2021) 26(1):46. doi: 10.1186/s40001-021-00516-8
64. Jyotsana N, King MR. The impact of COVID-19 on cancer risk and treatment. Cell Mol Bioeng (2020) 13(4):285–91. doi: 10.1007/s12195-020-00630-3
65. Shah VK, Firmal P, Alam A, Ganguly D, Chattopadhyay S. Overview of immune response during SARS-CoV-2 infection: Lessons from the past. Front Immunol (2020) 11:1949. doi: 10.3389/fimmu.2020.01949
66. Alefishat E, Jelinek HF, Mousa M, Tay GK, Alsafar HS. Immune response to SARS-CoV-2 variants: A focus on severity, susceptibility, and preexisting immunity. J Infect Public Health (2022) 15(2):277–88. doi: 10.1016/j.jiph.2022.01.007
67. Diamond MS, Lambris JD, Ting JP, Tsang JS. Considering innate immune responses in SARS-CoV-2 infection and COVID-19. Nat Rev Immunol (2022) 22:465–70. doi: 10.1038/s41577-022-00744-x
68. Marelli G, Howells A, Lemoine NR, Wang Y. Oncolytic viral therapy and the immune system: A double-edged sword against cancer. Front Immunol (2018) 9:866. doi: 10.3389/fimmu.2018.00866
69. Waldman AD, Fritz JM, Lenardo MJ. A guide to cancer immunotherapy: From T cell basic science to clinical practice. Nat Rev Immunol (2020) 20(11):651–68. doi: 10.1038/s41577-020-0306-5
70. Bian J, Li Z. Angiotensin-converting enzyme 2 (ACE2): SARS-CoV-2 receptor and RAS modulator. Acta Pharm Sin B (2021) 11(1):1–12. doi: 10.1016/j.apsb.2020.10.006
71. Jackson CB, Farzan M, Chen B, Choe H. Mechanisms of SARS-CoV-2 entry into cells. Nat Rev Mol Cell Biol (2022) 23(1):3–20. doi: 10.1038/s41580-021-00418-x
72. Khan SS. The central role of PAI-1 in COVID-19: Thrombosis and beyond. Am J Respir Cell Mol Biol (2021) 65(3):238–40. doi: 10.1165/rcmb.2021-0208ED
73. Thunders M, Delahunt B. Gene of the month: TMPRSS2 (transmembrane serine protease 2). J Clin Pathol (2020) 73(12):773–6. doi: 10.1136/jclinpath-2020-206987
74. Schönfelder K, Breuckmann K, Elsner C, Dittmer U, Fistera D, Herbstreit F, et al. Transmembrane serine protease 2 polymorphisms and susceptibility to severe acute respiratory syndrome coronavirus type 2 infection: A German case-control study. Front Genet (2021) 12:667231. doi: 10.3389/fgene.2021.667231
75. Ming Y, Qiang L. Involvement of spike protein, furin, and ACE2 in SARS-CoV-2-Related cardiovascular complications. SN Compr Clin Med (2020) 2(8):1103–8. doi: 10.1007/s42399-020-00400-2
76. Vardhan S, Sahoo SK. Virtual screening by targeting proteolytic sites of furin and TMPRSS2 to propose potential compounds obstructing the entry of SARS-CoV-2 virus into human host cells. J Tradit Complement Med (2022) 12(1):6–15. doi: 10.1016/j.jtcme.2021.04.001
77. Hasan A, Paray BA, Hussain A, Qadir FA, Attar F, Aziz FM, et al. A review on the cleavage priming of the spike protein on coronavirus by angiotensin-converting enzyme-2 and furin. J Biomol Struct Dyn (2021) 39(8):3025–33. doi: 10.1080/07391102.2020.1754293
78. Behl T, Kaur I, Aleya L, Sehgal A, Singh S, Sharma N, et al. CD147-spike protein interaction in COVID-19: Get the ball rolling with a novel receptor and therapeutic target. Sci Total Environ (2022) 808:152072. doi: 10.1016/j.scitotenv.2021.152072
79. Naqvi AAT, Fatima K, Mohammad T, Fatima U, Singh IK, Singh A, et al. Insights into SARS-CoV-2 genome, structure, evolution, pathogenesis and therapies: Structural genomics approach. Biochim Biophys Acta Mol Basis Dis (2020) 1866(10):165878. doi: 10.1016/j.bbadis.2020.165878
80. Jungreis I, Sealfon R, Kellis M. SARS-CoV-2 gene content and COVID-19 mutation impact by comparing 44 sarbecovirus genomes. Nat Commun (2021) 12:2642. doi: 10.1038/s41467-021-22905-7
81. Xu R, Lu T, Zhao J, Li Q, Wang J, Peng B, et al. Identification of ubiquitinated substrate proteins and their gene expression patterns in lung adenocarcinoma. Ann Transl Med (2021) 9(22):1692. doi: 10.21037/atm-21-5645
82. Satpathy S, Krug K, Jean Beltran PM, Savage SR, Petralia F, Kumar-Sinha C, et al. Clinical proteomic tumor analysis consortium. A proteogenomic portrait Lung squamous Cell carcinoma. Cell (2021) 184(16):4348–71. doi: 10.1016/j.cell.2021.07.016
83. Ilikci Sagkan R, Akin-Bali DF. Structural variations and expression profiles of the SARS-CoV-2 host invasion genes in lung cancer. J Med Virol (2020) 92(11):2637–47. doi: 10.1002/jmv.26107
84. Liu X, Liu B, Shang Y, Cao P, Hou J, Chen F, et al. Decreased TMPRSS2 expression by SARS-CoV-2 predicts the poor prognosis of lung cancer patients through metabolic pathways and immune infiltration. Aging (Albany NY) (2022) 14(1):73–108. doi: 10.18632/aging.203823
85. Wang Q, Li L, Qu T, Li J, Wu L, Li K, et al. High expression of ACE2 and TMPRSS2 at the resection margin makes lung cancer survivors susceptible to SARS-CoV-2 with unfavorable prognosis. Front Oncol (2021) 11:644575. doi: 10.3389/fonc.2021.644575
86. Hoang T, Nguyen TQ, Tran TTA. Genetic susceptibility of ACE2 and TMPRSS2 in six common cancers and possible impacts on COVID-19. Cancer Res Treat (2021) 53(3):650–6. doi: 10.4143/crt.2020.950
87. Liu Z, Gu X, Li Z, Shan S, Wu F, Ren T. Heterogeneous expression of ACE2, TMPRSS2, and FURIN at single-cell resolution in advanced non-small cell lung cancer. J Cancer Res Clin Oncol (2022) 12:1–11. doi: 10.1007/s00432-022-04253-1
88. Sayed S. COVID-19 and malignancy: Exploration of the possible genetic and epigenetic interlinks and overview of the vaccination scenario. Cancer Treat Res Communx (2021) 28:100425. doi: 10.1016/j.ctarc.2021.100425
89. Niemi MEK, Daly MJ, Ganna A. The human genetic epidemiology of COVID-19. Nat Rev Genet (2022) 23:533–46. doi: 10.1038/s41576-022-00478-5
90. Ravaioli S, Tebaldi M, Fonzi E, Angeli D, Mazza M, Nicolini F, et al. ACE2 and TMPRSS2 potential involvement in genetic susceptibility to SARS-COV-2 in cancer patients. Cell Transplant (2020) 29:963689720968749. doi: 10.1177/0963689720968749
91. Stewart CA, Gay CM, Ramkumar K, Cargill KR, Cardnell RJ, Nilsson MB, et al. Lung cancer models reveal SARS-CoV-2-induced EMT contributes to COVID-19 pathophysiology. bioRxiv [ (2021) 16(11):1821–39. doi: 10.1101/2020.05.28.122291
92. Ferrero G, Carpi S, Polini B, Pardini B, Nieri P, Impeduglia A, et al. Intake of natural compounds and circulating microRNA expression levels: Their relationship investigated in healthy subjects with different dietary habits. Front Pharmacol (2021) 11:619200. doi: 10.3389/fphar.2020.619200
93. Zamorano Cuervo N, Grandvaux N. ACE2: Evidence of role as entry receptor for SARS-CoV-2 and implications in comorbidities. Elife (2020) 9:e61390. doi: 10.7554/eLife.61390
94. Lai YJ, Chao CH, Liao CC, Lee TA, Hsu JM, Chou WC, et al. Epithelial-mesenchymal transition induced by SARS-CoV-2 required transcriptional upregulation of snail. Am J Cancer Res (2021) 11(5):2278–90.
95. Wu S, Tian C, Liu P, Guo D, Zheng W, Huang X, et al. Effects of SARS-CoV-2 mutations on protein structures and intraviral protein-protein interactions. J Med Virol (2021) 93(4):2132–40. doi: 10.1002/jmv.26597
96. Stephenson E, Reynolds G, Botting RA, Calero-Nieto FJ, Morgan MD, Tuong ZK, et al. Cambridge Institute of therapeutic immunology and infectious disease-national institute of health research (CITIID-NIHR) COVID-19 BioResource collaboration, rostron AJ, Simpson AJ, Hambleton s, laurenti e, lyons PA, Meyer KB, nikolić MZ, Duncan CJA, smith KGC, teichmann SA, clatworthy MR, marioni JC, göttgens b, haniffa m. single-cell multi-omics analysis of the immune response in COVID-19. Nat Med (2021) 27(5):904–16. doi: 10.1038/s41591-021-01329-2
97. Li H, Huang F, Liao H, Li Z, Feng K, Huang T, et al. Identification of COVID-19-Specific immune markers using a machine learning method. Front Mol Biosci (2022) 9:952626. doi: 10.3389/fmolb.2022.952626
98. Tvedt THA, Vo AK, Bruserud Ø, Reikvam H. Cytokine release syndrome in the immunotherapy of hematological malignancies: The biology behind and possible clinical consequences. J Clin Med (2021) 10(21):5190. doi: 10.3390/jcm10215190
99. Costela-Ruiz VJ, Illescas-Montes R, Puerta-Puerta JM, Ruiz C, Melguizo-Rodríguez L. SARS-CoV-2 infection: The role of cytokines in COVID-19 disease. Cytokine Growth Factor Rev (2020) 54:62–75. doi: 10.1016/j.cytogfr.2020.06.001
100. Ricci D, Etna MP, Rizzo F, Sandini S, Severa M, Coccia EM. Innate immune response to SARS-CoV-2 infection: From cells to soluble mediators. Int J Mol Sci (2021) 22(13):7017. doi: 10.3390/ijms22137017
101. Zheng J, Wang Y, Li K, Meyerholz DK, Allamargot C, Perlman S. Severe acute respiratory syndrome coronavirus 2-induced immune activation and death of monocyte-derived human macrophages and dendritic cells. J Infect Dis (2021) 223(5):785–95. doi: 10.1093/infdis/jiaa753
102. Li YS, Ren HC, Cao JH. Correlation of SARS−CoV−2 to cancer: Carcinogenic or anticancer? (Review). Int J Oncol (2022) 60(4):42. doi: 10.3892/ijo.2022.5332
103. Seth G, Sethi S, Bhattarai S, Saini G, Singh CB, Aneja R. SARS-CoV-2 infection in cancer patients: Effects on disease outcomes and patient prognosis. Cancers (Basel) (2020) 12(11):3266. doi: 10.3390/cancers12113266
104. Huang X, Liang H, Zhang H, Tian L, Cong P, Wu T, et al. The potential mechanism of cancer patients appearing more vulnerable to SARS-CoV-2 and poor outcomes: A pan-cancer bioinformatics analysis. Front Immunol (2022) 12:804387. doi: 10.3389/fimmu.2021.804387
105. Samad A, Jafar T, Rafi JH. Identification of angiotensin-converting enzyme 2 (ACE2) protein as the potential biomarker in SARS-CoV-2 infection-related lung cancer using computational analyses. Genomics (2020) 112(6):4912–23. doi: 10.1016/j.ygeno.2020.09.002
106. Roberts J, Pritchard AL, Treweeke AT, Rossi AG, Brace N, Cahill P, et al. Why is COVID-19 more severe in patients with diabetes? The role of angiotensin-converting enzyme 2, endothelial dysfunction and the immunoinflammatory system. Front Cardiovasc Med (2021) 7:629933. doi: 10.3389/fcvm.2020.629933
107. Hu Y, Liu L, Lu X. Regulation of angiotensin-converting enzyme 2: A potential target to prevent COVID-19? Front Endocrinol (Lausanne) (2021) 12:725967. doi: 10.3389/fendo.2021.725967
108. Li Y, Zhou W, Yang L, You R. Physiological and pathological regulation of ACE2, the SARS-CoV-2 receptor. Pharmacol Res (2020) 157:104833. doi: 10.1016/j.phrs.2020.104833
109. Xia X. Domains and functions of spike protein in sars-Cov-2 in the context of vaccine design. Viruses (2021) 13(1):109. doi: 10.3390/v13010109
110. Duan L, Zheng Q, Zhang H, Niu Y, Lou Y, Wang H. The SARS-CoV-2 spike glycoprotein biosynthesis, structure, function, and antigenicity: Implications for the design of spike-based vaccine immunogens. Front Immunol (2020) 11:576622. doi: 10.3389/fimmu.2020.576622
111. Salamanna F, Maglio M, Landini MP, Fini M. Body localization of ACE-2: On the trail of the keyhole of SARS-CoV-2. Front Med (Lausanne) (2020) 7:594495. doi: 10.3389/fmed.2020.594495
112. Coperchini F, Chiovato L, Croce L, Magri F, Rotondi M. The cytokine storm in COVID-19: An overview of the involvement of the chemokine/chemokine-receptor system. Cytokine Growth Factor Rev (2020) 53:25–32. doi: 10.1016/j.cytogfr.2020.05.003
113. Chen R, Lan Z, Ye J, Pang L, Liu Y, Wu W, et al. Cytokine storm: The primary determinant for the pathophysiological evolution of COVID-19 deterioration. Front Immunol (2021) 12:589095. doi: 10.3389/fimmu.2021.589095
114. Montazersaheb S, Hosseiniyan Khatibi SM, Hejazi MS, Tarhriz V, Farjami A, et al. COVID-19 infection: An overview on cytokine storm and related interventions. Virol J (2022) 19:92. doi: 10.1186/s12985-022-01814-1
115. Perico L, Benigni A, Casiraghi F, Ng LFP, Renia L, Remuzzi G, et al. Immunity, endothelial injury and complement-induced coagulopathy in COVID-19. Nat Rev Nephrol (2021) 17:46–64. doi: 10.1038/s41581-020-00357-4
116. Maza MDC, Úbeda M, Delgado P, Horndler L, Llamas MA, van Santen HM, et al. ACE2 serum levels as predictor of infectability and outcome in COVID-19. Front Immunol (2022) 13:836516. doi: 10.3389/fimmu.2022.836516
117. Ni W, Yang X, Yang D, Bao J, Li R, Xiao Y, et al. Role of angiotensin-converting enzyme 2 (ACE2) in COVID-19. Crit Care (2020) 24(1):422. doi: 10.1186/s13054-020-03120-0
118. Pinato DJ, Tabernero J, Bower M, Scotti L, Patel M, Colomba E, et al. Prevalence and impact of COVID-19 sequelae on treatment and survival of patients with cancer who recovered from SARS-CoV-2 infection: Evidence from the OnCovid retrospective, multicentre registry study. Lancet Oncol (2021) 22(12):1669–80. doi: 10.1016/S1470-2045(21)00573-8
119. Qiu S, Hu Y. Are COVID-19 susceptibility genes related to lung cancer? J Infect (2021) 83(5):607–35. doi: 10.1016/j.jinf.2021.08.032
120. Zhang Y, Mao Q, Li Y, Cheng J, Xia Q, Chen G, et al. Cancer and COVID-19 susceptibility and severity: A two-sample mendelian randomization and bioinformatic analysis. Front Cell Dev Biol (2022) 9:759257. doi: 10.3389/fcell.2021.759257
121. Calabrò L, Rossi G, Covre A, Morra A, Maio M. COVID and lung cancer. Curr Oncol Rep (2021) 23(11):134. doi: 10.1007/s11912-021-01125-8
122. Casaluce F, Gridelli C. Narrative review of lung cancer treatment at the time of COVID-19 pandemia: Pitfall and issues. Transl Lung Cancer Res (2021) 10(1):475–82. doi: 10.21037/tlcr-20-640
123. Wang Y, Deng W, Li N, Neri S, Sharma A, Jiang W, et al. Combining immunotherapy and radiotherapy for cancer treatment: Current challenges and future directions. Front Pharmacol (2018) 9:185. doi: 10.3389/fphar.2018.00185
124. Naimi A, Mohammed RN, Raji A, Chupradit S, Yumashev AV, Suksatan W, et al. Tumor immunotherapies by immune checkpoint inhibitors (ICIs); the pros and cons. Cell Commun Signal (2022) 20:44. doi: 10.1186/s12964-022-00854-y
125. Rabaan AA, Al-Ahmed SH, Muhammad J, Khan A, Sule AA, Tirupathi R, et al. Role of inflammatory cytokines in COVID-19 patients: A review on molecular mechanisms, immune functions, immunopathology and immunomodulatory drugs to counter cytokine storm. Vaccines (Basel) (2021) 9(5):436. doi: 10.3390/vaccines9050436
126. Gambichler T, Reuther J, Scheel CH, Becker JC. On the use of immune checkpoint inhibitors in patients with viral infections including COVID-19. J Immunother Cancer (2020) 8(2):e001145. doi: 10.1136/jitc-2020-001145
127. Garassino MC, Whisenant JG, Huang LC, Trama A, Torri V, Agustoni F, et al. COVID-19 in patients with thoracic malignancies (TERAVOLT): First results of an international, registry-based, cohort study. Lancet Oncol (2020) 21(7):914–22. doi: 10.1016/S1470-2045(20)30314-4
128. Di Lorenzo G, Di Trolio R, Kozlakidis Z, Busto G, Ingenito C, Buonerba L, et al. COVID 19 therapies and anti-cancer drugs: A systematic review of recent literature. Crit Rev Oncol Hematol (2020) 152:102991. doi: 10.1016/j.critrevonc.2020.102991
129. Martín-Bravo C, Quirós R, Blancas I, Villatoro-Roldán R, Robles M, Alcaide J, et al. Incidence of COVID-19 in outpatients with cancer receiving active treatment in the context of a pandemic: An andalusian cohort study. Semin Oncol (2021) 48(2):145–51. doi: 10.1053/j.seminoncol.2021.01.002
130. Belkacemi Y, Grellier N, Ghith S, Debbi K, Coraggio G, Bounedjar A, et al. A review of the international early recommendations for departments organization and cancer management priorities during the global COVID-19 pandemic: Applicability in low- and middle-income countries. Eur J Cancer (2020) 135:130–46. doi: 10.1016/j.ejca.2020.05.015
131. Breton M, Sullivan EE, Deville-Stoetzel N, McKinstry D, DePuccio M, Sriharan A, et al. Telehealth challenges during COVID-19 as reported by primary healthcare physicians in Quebec and Massachusetts. BMC Fam Pract (2021) 22:192. doi: 10.1186/s12875-021-01543-4
Keywords: COVID-19, pandemic, virus, lung cancer, genes, mutations
Citation: Aramini B, Masciale V, Samarelli AV, Tonelli R, Cerri S, Clini E, Stella F and Dominici M (2022) Biological effects of COVID-19 on lung cancer: Can we drive our decisions. Front. Oncol. 12:1029830. doi: 10.3389/fonc.2022.1029830
Received: 27 August 2022; Accepted: 23 September 2022;
Published: 10 October 2022.
Edited by:
Alberto Testori, Humanitas University, ItalyReviewed by:
Emanuele Voulaz, Humanitas Research Hospital, ItalyCopyright © 2022 Aramini, Masciale, Samarelli, Tonelli, Cerri, Clini, Stella and Dominici. This is an open-access article distributed under the terms of the Creative Commons Attribution License (CC BY). The use, distribution or reproduction in other forums is permitted, provided the original author(s) and the copyright owner(s) are credited and that the original publication in this journal is cited, in accordance with accepted academic practice. No use, distribution or reproduction is permitted which does not comply with these terms.
*Correspondence: Beatrice Aramini, YmVhdHJpY2UuYXJhbWluaTJAdW5pYm8uaXQ=
†These authors share first authorship
‡These authors share last authorship
Disclaimer: All claims expressed in this article are solely those of the authors and do not necessarily represent those of their affiliated organizations, or those of the publisher, the editors and the reviewers. Any product that may be evaluated in this article or claim that may be made by its manufacturer is not guaranteed or endorsed by the publisher.
Research integrity at Frontiers
Learn more about the work of our research integrity team to safeguard the quality of each article we publish.