- Department of Medical Oncology, Vall d’Hebron University Hospital and Vall d’Hebron Institute of Oncology (VHIO), Barcelona, Spain
Gastrointestinal tumours are a heterogeneous group of neoplasms that arise in the gastrointestinal tract and hepatobiliary system. Their incidence is rising globally and they currently represent the leading cause of cancer-related mortality worldwide. Anti-angiogenic agents have been incorporated into the treatment armamentarium of most of these malignancies and have improved survival outcomes, most notably in colorectal cancer and hepatocellular carcinoma. New treatment combinations with immunotherapies and other agents have led to unprecedented benefits and are revolutionising patient care. In this review, we detail the mechanisms of action of anti-angiogenic agents and the preclinical rationale underlying their combinations with immunotherapies. We review the clinical evidence supporting their use across all gastrointestinal tumours, with a particular emphasis on colorectal cancer and hepatocellular carcinoma. We discuss available biomarkers of response to these therapies and their utility in routine clinical practice. Finally, we summarise ongoing clinical trials in distinct settings and highlight the preclinical rationale supporting novel combinations.
1 Introduction
The process of angiogenesis was identified in 1971 as one of the key steps in cancer progression, and has been considered a hallmark of cancer since 2000 (1, 2). Angiogenesis is a pathway that implies the growth of new capillary blood vessels to maintain oxygen and nutrient supplies during tumour expansion. Cancer cells develop this angiogenic capacity via an “angiogenic switch” triggered by the synthesis and delivery of different positive signals that encourage angiogenesis, such as vascular endothelial growth factor (VEGF) that binds to the VEGF receptor (VEGFR) located on endothelial cells. More molecules participate in this delicate equilibrium, which is a balance between proangiogenic and anti-angiogenic signals crucial for the angiogenic switch (3). Soon after the discovery of the angiogenic pathway, efforts were made to develop treatments to block this process. This included large monoclonal antibodies such as bevacizumab and small tyrosine kinase inhibitors (TKIs) including sorafenib, sunitinib, and regorafenib, that have been approved in different tumour types (4).
Gastrointestinal cancers are drivers of cancer mortality worldwide. Colorectal cancer (CRC) is the second cause of cancer-related deaths, liver cancer the third, and stomach cancer the fourth (5). Therefore, there is a global concern and a need to generate more efficient diagnostic and therapeutic approaches to increase patient survival, with anti-angiogenics representing an attractive target in this setting. VEGF expression is associated with poor prognosis in colorectal, gastric and pancreatic cancer (6–8). The value of testing anti-angiogenic therapy in different gastrointestinal cancers has been established, and today different treatments and combination regimens are available for these tumours (Figure 1), as summarised in this review. Nevertheless, there is an unmet need for a better understanding of the mechanism of resistance as well as of optimal selection of those patients more likely to benefit from VEGF-targeted therapy, thus novel therapeutic strategies are also reviewed.
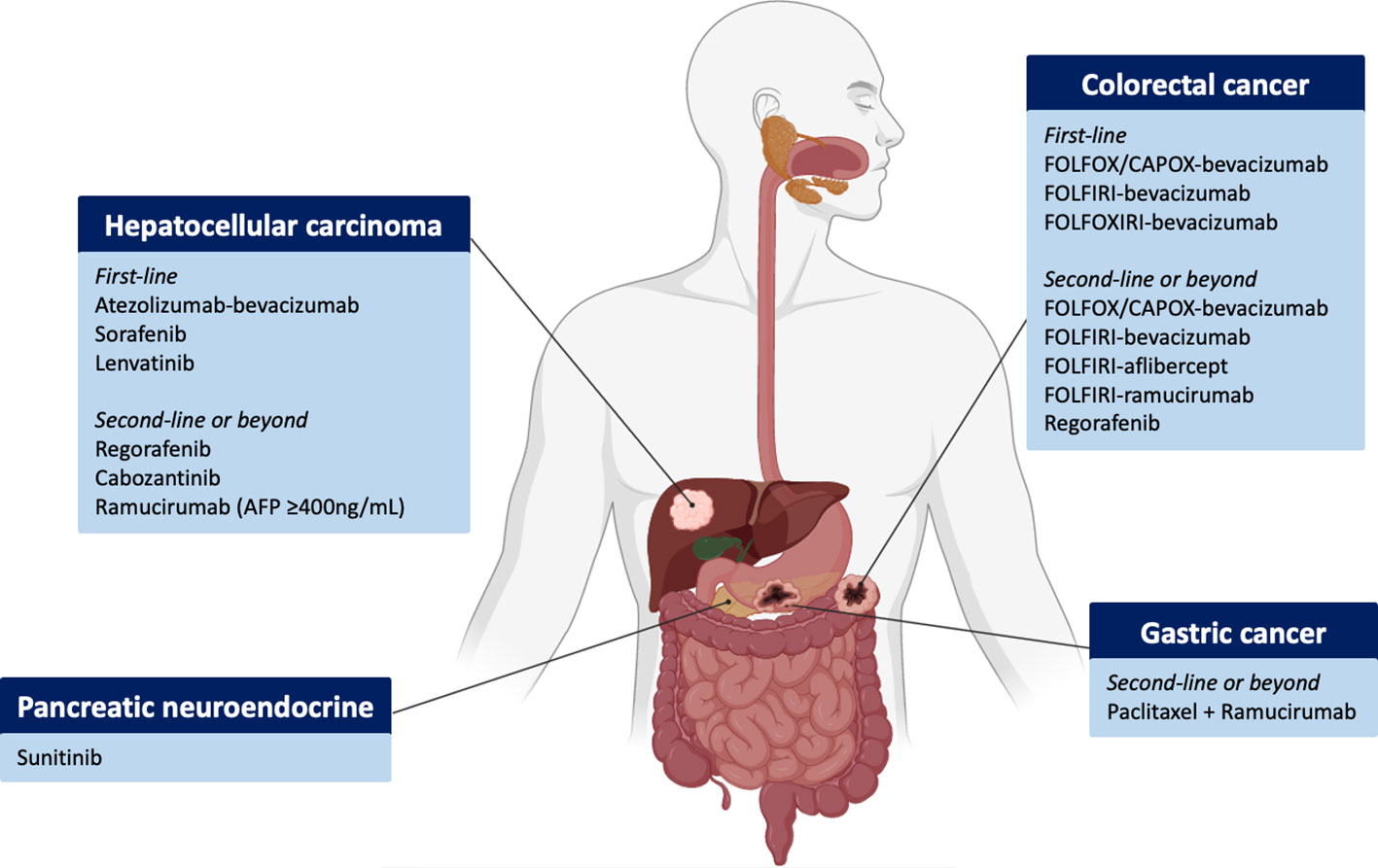
Figure 1 Overview of FDA-approved antiangiogenic agents across gastrointestinal and hepatobiliary tumours and disease settings. Figure generated with www.biorender.com.
2 Angiogenesis in gastrointestinal cancer
Angiogenesis is essential for tumour progression and develops following an “angiogenic switch” (9–11). The onset of this event is dictated by the balance of pro- and anti-angiogenic factors (10, 11), which eventually leads to chronic activation of proangiogenic factors favouring the formation of new, morphologically aberrant blood vessels that will sustain tumour development and foster metastatic spread (9). Despite angiogenesis being universal to all cancers, tumours exhibit diverse patterns of neo-vascularisation which may influence response to therapy. An example of this heterogeneity is seen with pancreatic ductal adenocarcinomas (PDAC) that are generally hypovascularised and hypoxic owing to the high desmoplastic microenvironment, which may limit drug delivery (12). Conversely, hepatocellular carcinomas (HCC) are hypervascular tumours with a characteristic radiological pattern and a highly abnormal vessel architecture resulting from the overexpression of VEGF (13, 14). Similarly, neuroendocrine tumours have a rich vascular supply and a dense microvascular network which together constitute one of the most useful diagnostic characteristics of these tumours (15). In addition to the observed between-tumour type heterogeneity, substantial differences exist amongst tumours belonging to the same anatomic location. In CRC, the Consensus Molecular Subtype (CMS) 4 (mesenchymal subtype), which constitutes ~25% of all CRC, is characterised by high stromal infiltration, increased angiogenesis and is associated with a significantly worse prognosis (16). Likewise, gastric cancers classified as genomically stable are enriched in angiogenic pathways (17). These traits may help to explain, at least in part, the different patterns of response to anti-angiogenic therapies in distinct tumour types and amongst patients.
2.1 Signalling pathways in angiogenesis
Different mechanisms may lead to the formation of new blood vessels, such as the proliferation of pre-existing endothelial cells (“sprouting angiogenesis”), recruitment of endothelial progenitor cells to the tumoural microenvironment (“vasculogenesis”), remodelling of pre-existing blood vessels (“intussusceptive angiogenesis”) or formation of new vessels by tumoural cells independently of endothelial cells (“vascular mimicry”) (18). In addition, tumours may develop close to pre-existing mature blood vessels thus ensuring an adequate blood supply without the need for developing new vessels (referred to as “vessel co-option”) (19). While all of these mechanisms are known to contribute to tumour vascularisation in gastrointestinal tumours, sprouting angiogenesis remains the dominant mechanism and is triggered by multiple proangiogenic pathways.
The most potent angiogenic pathway in cancer is the VEGF signalling pathway (20), which is composed of five ligands (VEGF-A, -B, -C, -D and placental growth factor [PlGF]) and three receptors (VEGFR-1, -2 and -3). In gastrointestinal cancers, hypoxia mainly upregulates VEGF-A in tumour cells. This in turn binds to VEGFR-2 that is expressed on endothelial cells, leading to proliferation, vascular permeability and endothelial cell migration (10, 20). The mammalian fibroblast growth factor (FGF) signals through the FGF receptors 1-4 (FGFR 1-4) to mediate multiple functions including angiogenesis, cellular proliferation, invasiveness and enhanced metastasis. In addition to VEGF and FGF, many other pathways have been shown to be highly relevant in regulating angiogenesis in these tumour types, including the platelet-derived growth factor (PDGF) family, and the angiopoeitin family which bind to the tyrosine kinases TIE-1 and TIE-2 (20).
2.2 Mechanism of action of anti-angiogenic drugs
Anti-angiogenic therapies commonly used in gastrointestinal malignancies can be broadly categorised into monoclonal antibodies and TKIs. The former includes bevacizumab, which binds to VEGF-A, ramucirumab (which inhibits VEGFR-2) and aflibercept, a decoy receptor that binds to all isoforms of VEGF-A. TKIs are small-molecule compounds that inhibit a broad range of protein kinases. They include sorafenib, lenvatinib, regorafenib, cabozantinib and sunitinib, amongst others (Figure 1). The main targets of sorafenib are VEGFR-1, -2, -3, PDGFR, RAF and KIT; regorafenib and sunitinib have a similar inhibitory profile, as does lenvatinib that additionally targets FGFR-1, -2, -3 and -4. Cabozantinib is a potent VEGFR-2 and MET inhibitor.
Anti-angiogenic therapies may mediate antitumour effects in at least 4 different mechanisms (Figure 2). Firstly, the development of these therapies stems from the hypothesis that starving tumours by depleting them of blood vessels will induce necrosis and slow tumour progression (21). However, fostering a hypoxic and nutrient-deprived microenvironment may also result in treatment resistance and insufficient efficacy (22). This has led to the concept of vascular normalisation (23), understood as the resulting effect on the tumour vasculature of a judicious use of anti-angiogenic drugs that may balance the excess of proangiogenic factors and lead to a remodelling and pruning of tumour blood vessels to normalize the tumour vasculature (24). This, in turn, will improve drug delivery and foster a less hostile microenvironment, thus increasing the efficacy of combination partners (22).
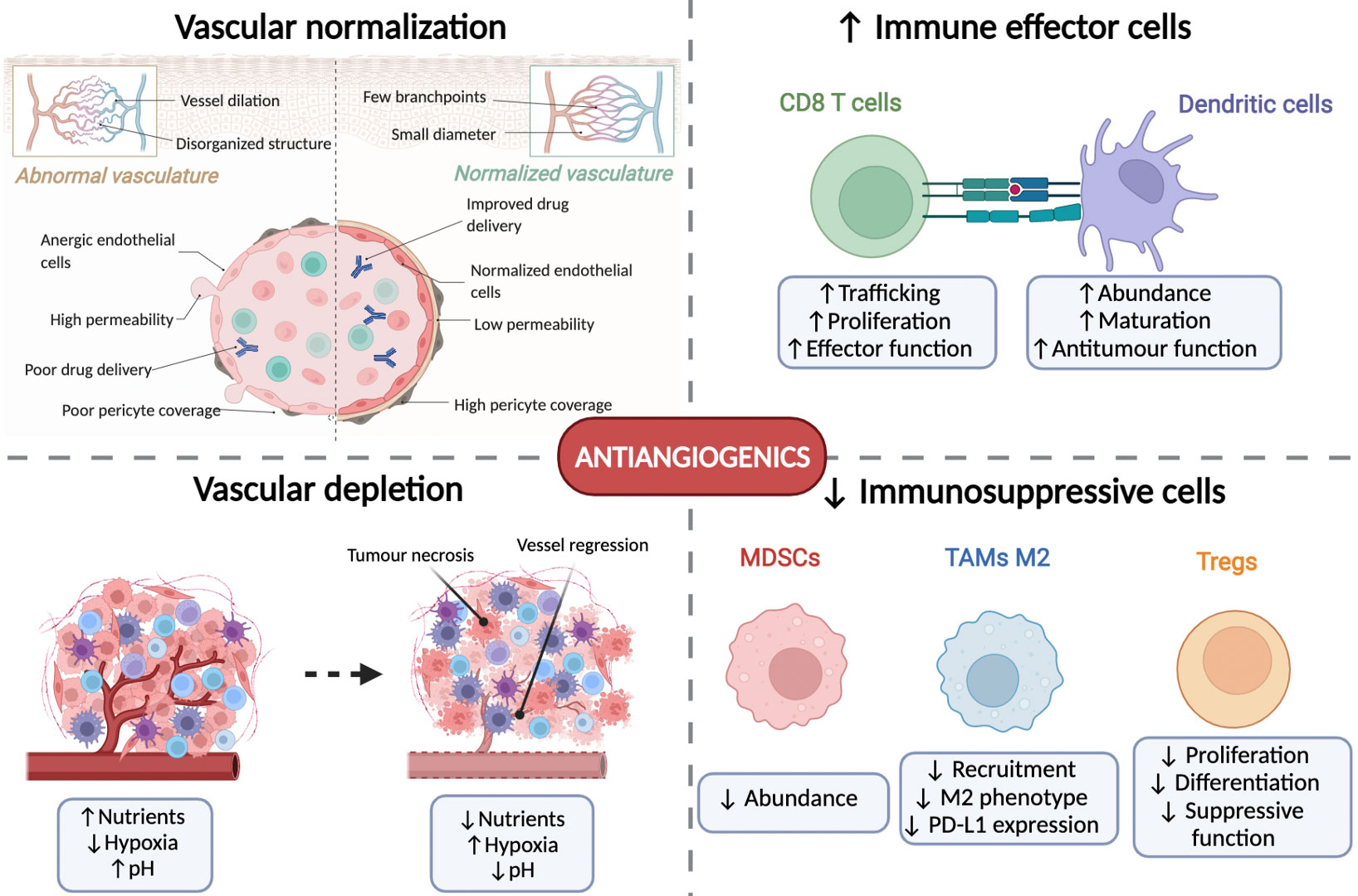
Figure 2 Mechanisms of action of antiangiogenic agents. Antiangiogenics exert their anti-tumoural effects via four axes. First, they induce normalisation of the tumour vasculature which improves drug delivery and oxygenation. Second, they induce vascular depletion which induces tumour starvation and necrosis. Thirdly, they favour an antitumour immune response by increasing the proliferation and activation of CD8+ T cells and dendritic cells. Finally, they decrease the presence and function of immunosuppressive cells including myeloid-derived suppressor cells (MDSCs), tumour-associated macrophages (TAMs) and T regulatory cells (Tregs). Figure generated with www.biorender.com.
Thirdly, increased attention has been placed on the immunomodulatory effects of anti-angiogenic therapies (Figure 2). Angiogenic modulating factors may alter the immune microenvironment through three established paths (25). First, VEGF can directly act on immune cells, leading to CD8+ T cell exhaustion, increased proliferation of T regulatory cells (Tregs), thereby fostering the expansion of myeloid-derived suppressor cells (MDSCs), and inhibiting the differentiation of monocytes to dendritic cells (DC) and decreasing DC maturation (26, 27). Secondly, the endothelium of tumour blood vessels creates a natural barrier for immune cells to infiltrate the microenvironment due to its lack of adhesion proteins (such as ICAM1 and VCAM1), as well as increased expression of proapoptotic molecules (FASL and galectin 1) and immunosuppressive molecules (PD-L1, PD-L2, TIM3 and IDO) (28). Finally, the hypoxic and acidotic tumour microenvironment also favours immunosuppressive changes, including the reprogramming of tumour-associated macrophages (TAMs) from an antitumour M1-like phenotype to a pro-tumoural M2-like phenotype, also decreasing the maturation and proliferation of DCs and increasing the proliferation of Tregs (26). All these factors will lead to a highly immunosuppressed microenvironment that could be potentially reversable with appropriate anti-angiogenic drugs.
These immunomodulatory effects have prompted the development of combinations of anti-angiogenic drugs with immune checkpoint inhibitors (ICIs). The rationale underlying these novel therapies is sound: ICIs increase the recruitment and/or activation of effector CD8+ T cells, DCs and natural killer cells and promote an antitumour M1 macrophage phenotype, while simultaneously decreasing the infiltration and activity of MDSCs, Treg cells and M2 macrophage polarisation (26, 29). Specific combinations in animal models further support this notion. Cabozantinib combined with anti-PD1 antibodies in syngeneic HCC mice models promoted the infiltration of neutrophils (30), and lenvatinib decreased the abundance of tumoural Tregs (31). Sorafenib specifically suppressed the activation of macrophages with an M2-like polarisation (32) and regorafenib favoured the infiltration of activated CXCR3+CD8+ T cells (33) and an M1-like macrophage polarisation (34). Similarly, sunitinib or antibodies blocking the VEGF/VEGF-R pathway in syngeneic colon mice models decreased the population of Tregs (35). Hence, combining therapies that target angiogenesis with immune stimulating agents represents a promising strategy that is being actively explored in clinical trials in many gastrointestinal, hepatobiliary and pancreatic tumours.
2.3 Mechanisms of resistance to anti-angiogenic drugs
Some tumours may be primarily resistant to anti-angiogenic drugs while others can develop mechanisms of resistance following drug exposure. This process of adaptation may undergo different sequential phases. In an early phase, tumours will upregulate alternative or redundant pro-angiogenic pathways that are not targeted by a specific drug, thus resulting in sustained angiogenesis despite optimal inhibition of the targeted pathway (36). Moreover, some tumours, such as PDAC, rely primarily on signalling pathways other than VEGF, leading to primary resistance to these inhibitors. In a later step, tumours adapt to hypoxia by promoting autophagy, which degrades cell components to promote survival in unfavourable conditions. In HCC, increased activation of mTOR or Akt pathways has been shown to trigger autophagy and cell survival when exposed to sorafenib, and can be overcome by combining sorafenib with autophagy inhibitors (37). The stress induced by antiangiogenic therapies stimulates inflammatory pathways and cytokines that lead to the recruitment of cells that favour angiogenesis, such as bone marrow-derived cells (BMDCs), myeloid-derived suppressor cells (MDSCs), endothelial progenitor cells, pericytes and cancer-associated fibroblasts (38, 39). In a late adaptation phase following exposure to antiangiogenic agents, tumours adopt different neovascularization modalities, including vessel co-option and vascular mimicry, which in turn may fuel metastatic spread and increase tumour aggressiveness (38).
An area of increasing interest and research is the significant heterogeneity of tumour endothelial cells which may contribute to resistance to anti-angiogenic drugs (40, 41). A single-cell analysis of endothelial cells following angiogenic inhibition has found that these cells can be broadly categorized into tip cells, transition and stalk-like cells. VEGF inhibition reduces all three subpopulations but has a particularly strong inhibitory effect on tip cells. In contrast, blockade of Dll4 promotes endothelial proliferation as well as tip cell markers (41). In liver tumours specifically, endothelial cancer cells show liver-specific gene expression signatures that are independent of the site of the original tumour, display distinct clusters that recapitulate tip-like and stalk-like characteristics and show stronger similarities to venous rather than endothelial cells (42). Furthermore, HCCs present endothelial cells that are reminiscent of fetal development, with a shared onco-fetal programme that is mediated in part by VEGF and NOTCH (43). In lung cancer, 13 different endothelial cell phenotypes have been described, including some subsets potentially involved in immune surveillance. This study further supports the notion that tip endothelial cells are particularly sensitive to antiangiogenic therapies (40).
3 Colorectal cancer
CRC is the third most frequently diagnosed cancer worldwide, and the second cause of cancer-related deaths (5). Unfortunately, approximately 20% of patients with CRC have metastatic spread at diagnosis (mCRC), and almost half of all patients will develop metastases during the course of the disease (44, 45). The incorporation of biological agents targeting two major pathways involved in mCRC such as the epidermal growth factor receptor (EGFR) targeted by panitumumab or cetuximab, and the VEGF signalling pathway suppressed by bevacizumab, aflibercept, ramucirumab and regorafenib have improved median overall survival (OS) to more than 30 months (46–48).
3.1 Clinical overview of anti-angiogenic drugs in mCRC
3.1.1 Advanced disease: First-line setting
To define the optimal strategy treatment for patients with mCRC, is crucial to take into account the Eastern Cooperative Oncology Group (ECOG) performance status of the patient, the sidedness of colon tumour, molecular status of all RAS and BRAF genes, microsatellite status and resectability of metastatic disease, in addition to the patient’s preferences and toxicity of the treatments. According to European Society for Medical Oncology (ESMO) guidelines, combination of biological agents with FOLFIRI or FOLFOX chemotherapy is standard treatment for the first and second line setting in mCRC (45, 49–55).
Bevacizumab is a recombinant humanised monoclonal antibody targeting the VEGF ligand A (VEGF-A) and is approved for use in mCRC patients in the first and second lines of therapy. Over the last 20 years, multiple clinical trials have investigated the combination of bevacizumab with chemotherapy in this setting. The first phase III trial published in 2004 explored the combination of bevacizumab with irinotecan, bolus fluorouracil and leucovorin (IFL) vs IFL alone. Median OS was 20.3 months in the group that received IFL plus bevacizumab, compared with 15.6 months in the group given IFL plus placebo, corresponding to an HR for death of 0.66 (P<0.001) (56). Since then, the benefit of adding this monoclonal antibody to cytotoxic chemotherapy backbone regimens that contain either oxaliplatin or irinotecan, or both, or a fluoropyrimidine as monotherapy has been explored and demonstrated. Table 1 summarizes the major clinical trials in this setting. In combination with first line oxaliplatin, the NO16966 trial demonstrated a benefit for the addition of bevacizumab to FOLFOX or CAPOX. There was a significant improvement in progression-free survival (PFS) with the addition of bevacizumab; however, the magnitude of benefit was smaller than expected, and neither median OS nor overall response rates (ORRs) were significantly higher in patients who received bevacizumab (53). The BECOME trial randomised patients with RAS mutant unresectable, liver-limited mCRC to receive bevacizumab plus mFOLFOX6 vs FOLFOX6 alone. This trial demonstrated higher ORR (55% vs 37%), median PFS (9.5 vs 5.6 months), median OS (25.7 vs 20.5 months), and complete (R0) resection rates (22.3% vs 5.8%) (57).
For patients who are not suitable for doublet chemotherapy, the combination of fluoropyrimidines plus bevacizumab has demonstrated superiority over fluoropyrimidine monotherapy. The phase III AVEX trial focused on elderly patients with mCRC (70 years-old or older), enrolling patients who were not candidates for a combination of oxaliplatin or irinotecan-based chemotherapy regimens. Patients were randomised to bevacizumab plus capecitabine vs capecitabine alone, and this study demonstrated that the combination regimen was well tolerated and significantly improved outcomes, with median PFS of 9.1 vs 5.1, respectively (HR 0.53; 95% CI, 0.41–0.69; P<0.0001) (58). Median OS was not significantly different between the two groups as the study was not sufficiently powered to detect such differences (20.7 months in the bevacizumab plus capecitabine group vs 16.8 months in the capecitabine alone group [HR 0.79, 95% CI 0.57–1.09; P=0.18]).
Unlike frail patients, there are also a group of fit patients with metastatic disease who will benefit from a high response rate, thus more intense chemotherapy backbones have been investigated. Phase II/III trials have explored the combination of FOLFOXIRI with or without bevacizumab vs doublet combinations with or without bevacizumab (59–63). A recent individual-patient data meta-analysis of these clinical trials was published, demonstrating that FOLFOXIRI plus bevacizumab significantly and meaningfully improves OS of patients with mCRC compared with bevacizumab-based doublets and offers advantages in PFS, ORR and R0 resection rate, albeit at the price of increased toxicity. In contrast to initial observations from the subgroup analysis of the TRIBE trial, no increased benefit was observed among patients with BRAFV600-mutant tumours in this meta-analysis (61). Thus, the use of FOLFOXIRI–bevacizumab should no longer be regarded as the first choice for patients with a BRAFV600E mutation, in whom the use of FOLFOX–bevacizumab is currently the upfront treatment option of choice.
The question of which biologic is preferable in first line treatment for all RAS wild-type (wt) mCRC was addressed in several phase III trials. In the phase III trial FIRE-3, patients with previously untreated KRAS wt mCRC (initially this trial recruited allcomers, however due to the emerging evidence for the negative predictive value of KRAS exon 2 mutations, a protocol amendment was submitted in October 2007 to limit the population) received FOLFIRI plus cetuximab or FOLFIRI plus bevacizumab (48). There was no significant difference in the primary endpoint of ORR (65.3% with cetuximab vs 58% with bevacizumab, HR 1.18, P=0.18). The median PFS was similar in both the groups (10 months in the cetuximab group and 10.3 months in the bevacizumab group [HR 1.06, 95% CI 0.88–1.26; P=0.55]). Surprisingly, when evaluating KRAS exon 2 wt patients, OS was significantly better in patients treated with cetuximab (OS 33.1 vs 25.6 months favouring cetuximab over bevacizumab, P=0.011).
CALGB 80405 was a large randomised phase III trial in which patients with previously untreated mCRC received either FOLFIRI or FOLFOX at enrolment and were then randomised to either bevacizumab, cetuximab, or both (46). Initially, as for the FIRE-3, this trial included patients unselected for RAS status, with an amendment restricting eligibility to patients with KRAS wt tumours. The findings demonstrated similar results across all four groups, suggesting that either chemotherapy backbone in combination with either an anti-EGFR or anti-VEGF therapy is an acceptable therapy option in patients with RAS wt tumours.
mCRC is a clinically and molecularly heterogeneous disease, which is partially explained by the anatomic location of the tumour, given that left and right-sided tumours are derive from different embryonic structures (64). Furthermore, the left and right colon also have physiologically distinct functions with different contacts and exposure to nutrients, and thus different microbiomes can be found from the proximal to the distal colon (65). Regarding these differences, studies have retrospectively investigated the correlation between laterality and response to treatment, concluding that sidedness of colon cancer is a predictive biomarker of response to biological agents. From a meta-analysis published in 2017 covering all first line studies, a significant predictive benefit was demonstrated for chemotherapy plus EGFR antibody therapy in patients with left-sided tumours (HR 0.75 [95% CI 0.67-0.84] and 0.78 [95% CI 0.70-0.87] for OS and PFS, respectively) (66–68). However, there was a trend, albeit no significant benefit for patients treated with chemotherapy with or without bevacizumab with right-sided tumours (HRs 1.12 [95% CI 0.87-1.45] and 1.12 [95% CI 0.87-1.44] for OS and PFS, respectively). Recent data presented at ASCO 2022 from the PARADIGM trial, that randomised patients with RAS wt mCRC to receive panitumumab plus mFOLFOX or bevacizumab plus mFOLFOX, demonstrated a clear benefit of anti-EGFR therapy for patients with left-sided colon cancer (OS 37.9 vs 34.3 months; HR 0.82, P=0.031) (69). Thus, for patients with left-sided RAS wt disease, a cytotoxic doublet plus an anti-EGFR antibody should be the treatment of choice. For patients with right-sided RAS wt disease or RAS mutant, cytotoxic combination with bevacizumab is the preferred option.
The combination of both VEGF and anti-EGFR treatments is not recommended for first-line therapy of mCRC in light of the results of the PACCE and CAIRO2 trials (70, 71).
Maintenance treatment is a therapeutic strategy that envisages a period of high-intensity chemotherapy, after which agents that are mainly responsible for cumulative toxicity are stopped, leaving patients with a more simple and non-toxic combination of treatments until progression disease. This approach differs from treatment interruption, in which drug withdrawal is permitted with treatment-free intervals. Maintenance is active and should be part of the mCRC treatment strategy, as active maintenance with fluoropyrimidines and bevacizumab has demonstrated improvement of PFS (but not OS) (72, 73).
3.1..2 Advanced disease: Second line setting
Several anti-angiogenic agents have demonstrated efficacy in mCRC in the second line setting.
Aflibercept is a fully humanised recombinant fusion protein composed of a modified immunoglobulin domain of VEGFR-1 joined to another immunoglobulin domain of human VEGFR-2 and fused to a fragment crystallizable portion of a human immunoglobulin, thus providing complete blockade of angiogenesis by targeting VEGF-A, VEFGF-B, and PIGF (74). In 2012, in the absence of evidence of improvement of OS in the second line in mCRC after progression on a first line oxaliplatin-containing regimen, the VELOUR trial was initiated (52). This randomised phase III double-blind study randomised 1226 patients into two groups, aflibercept or placebo every 2 weeks plus FOLFIRI. Data demonstrated advantages in OS, PFS and RR of aflibercept combined with FOLFIRI vs chemotherapy alone. Prior treatment with bevacizumab was permitted. The results showed an OS benefit favouring the aflibercept group, with an OS of 13.5 vs 12.1 months (HR 0.817; P=0.0032), PFS of 6.9 vs 4.67 months (HR 0.758; P<0.0001), and an ORR of 19.8% vs 11.1% (P=0.0001) with aflibercept plus FOLFIRI compared with placebo plus FOLFIRI, respectively. The effects of aflibercept exhibited a consistent trend of improved OS and PFS in pre-specified subgroup analyses based on previous treatment with bevacizumab. This higher efficacy of aflibercept was associated with an expected increase in adverse effects, with grade 3 and 4 adverse events (AEs) reported in 85.3% and 62.5% of patients, respectively.
The TML18147 trial was a randomised phase III trial that assessed the efficacy of bevacizumab beyond progression in patients with mCRC who had received first line treatment with bevacizumab (54). In this study, patients received bevacizumab with chemotherapy or chemotherapy alone, and demonstrated an improvement in OS for patients in the bevacizumab plus chemotherapy group (11.1 vs 9.8 months, respectively; P=0.0062).
Ramucirumab is a human monoclonal antibody that targets VEGFR-2. The phase III RAISE study evaluated the efficacy and safety of ramucirumab in combination with second line FOLFIRI compared with FOLFIRI plus placebo in mCRC patients who had progressed during or after first line therapy with bevacizumab and FOLFOX, independent of KRAS status (50). In this trial, a total of 1,072 patients were randomised to FOLFIRI with or without ramucirumab, showing a significant improvement in both OS and PFS (13.3 vs 11.7 months and 5.7 vs 4.5 months, respectively).
The results of these phase III trials support the benefit of continuing VEGF inhibition following prior exposure to bevacizumab. No direct comparison has been done, however the effects across all studies are of a similar magnitude, therefore the selection of bevacizumab, aflibercept or ramucirumab should be based on evaluating the toxicity profile, the interval free of bevacizumab, patient’s preference, reimbursement policy of each country and previous anti-EGFR in all patients with RAS wt mCRC.
3.1.3 Advanced disease: Refractory setting
Regorafenib is an oral inhibitor that blocks the activity of multiple protein kinases active in oncogenesis and the tumour microenvironment, with anti-angiogenic activity due to its dual-targeted VEGFR2 tyrosine kinase inhibition (75). The efficacy of regorafenib in the mCRC refractory setting was demonstrated in the CORRECT trial (76). This phase III trial explored the efficacy in terms of OS of regorafenib vs best supportive care in patients with mCRC who progressed on standard therapy. Previous anti-angiogenic treatment was permitted. Patients were randomised in a 2:1 ratio to receive either best supportive care plus oral regorafenib or placebo once daily. Median OS was 6.4 months in the regorafenib group vs 5.0 months in the placebo group (HR 0.77; P=0.0052).
TAS-102 is an oral combination of a thymidine-based nucleic acid analogue, trifluridine and a thymidine phosphorylase inhibitor, tipiracil hydrochloride. TAS-102 has demonstrated efficacy in terms of OS compared to best supportive care in patients with refractory mCRC (5.3 months with placebo vs 7.1 months with TAS-102; HR 0.68, 95% CI 0.58 to 0.81; P<0.001) (77). The combination of bevacizumab and TAS-102 was explored in a phase II trial which randomised patients to receive standard-dose TAS-102 with or without bevacizumab (78). Combination therapy was associated with a modest, although statistically significant, improvement in median PFS (4.6 vs 2.6 months, HR 0.45, 95% CI 0.29-0.72) and OS (9.4 vs 6.7 months, HR 0.55, 95% CI 0.32-0.94) and a higher ORR (67% vs 51%).
3.2 Predictive biomarkers of anti-angiogenic drugs in colorectal cancer
Despite the importance of anti-angiogenic treatment for targeting this critical pathway of the disease, not all patients with mCRC benefit from this treatment, and in addition, a large proportion of them present severe AEs. There is an unmet clinical need driving the search for biomarkers of response to anti-angiogenic therapy in mCRC. Nevertheless, established biomarkers to predict response to anti-angiogenic treatments in mCRC are yet to be identified, as the different biomarkers tested to date have failed to show clear clinical utility (79, 80).
Retrospective data suggest that hypertension could predict treatment efficacy of bevacizumab in patients with mCRC (81). Some studies are researching the role of imaging in the assessment of vascularity of mCRC by radiomics of MRI and CT scan, attempting to translate medical images into biological information about tumour angiogenic status (82). A post-hoc analysis of the VELOUR trial showed that patients with previous bevacizumab treatment showed higher levels of VEGF-A and PIGF, suggesting that it could be a mechanism of resistance, and a negative prognostic marker in these patients, without differences in OS or PFS regarding plasma levels of VEGF-A and PIGF (83). Other retrospective data support this prognostic role of plasma levels of VEGF-A, without implications in prediction of response to anti-angiogenic treatment (84–86). Furthermore, the pattern of histopathological growth may influence response to anti-angiogenic agents (87). Vessel co-option is implicated as a major mechanism of resistance to these therapies and could represent a simple yet valuable biomarker of response (88).
3.3 Novel therapeutic strategies targeting angiogenesis in colorectal cancer
As previous reviewed, bevacizumab, aflibercept, regorafenib and ramucirumab have significantly improved both PFS and OS of mCRC in different clinical settings, from first line to the refractory scenario. Novel antiangiogenic agents and innovate combinations have been developed in recent years.
Fruquintinib is a novel receptor TKI inhibiting VEGFR 1, 2 and 3. Safety of this novel molecule was evaluated in a phase Ib trial, enrolling Asian patients with refractory mCRC, showing a manageable toxicity profile with the dosage of 5 mg once daily for 3 weeks with a 4-week cycle, giving a disease control rate (DCR) of 83.3% and 16-week PFS of 65% (89). The phase III FRESCO trial was a multicentre Asian trial in which 416 patients were randomised using a 2:1 ratio to receive fruquintinib with best supportive care or placebo plus best supportive care (90). Patients who received previous VEGFR inhibitors were excluded. Significant improvements were seen in the active fruquintinib treatment arm compared with placebo for OS (9.3 vs 6.6 months; HR: 0.65; P<0.001), PFS (3.7 vs 1.8 months; P<0.001), ORR (4.7% vs 0.0%; P=0.01) and DCR (62.2% vs 12.3%; P<0.001). This benefit was independent of previous treatment with anti-angiogenic agents or molecular status. The global FRESCO-2 trial (NCT04322539) is ongoing to confirm the results of the phase III FRESCO trial conducted in China.
Microsatellite stable (MSS) mCRC patients do not respond to monotherapy immunotherapy as demonstrated in many clinical trials (91–94). This population represents 95% of all patients with mCRC. Different combinations of immunotherapy with cofactors are being tested to achieve a change of a cold immune microambient to a hot microambient. One promising combination explored is the association of anti-angiogenic treatment with immunotherapy, as the blockade of VEGF leads to vasculature normalisation, thus permitting tumour infiltration with effector immune cells and the maturation of DCs (95–97).
Lenvatinib is a multiple kinase inhibitor. It inhibits the three main VEGFRs, VEGFR1, 2 and 3, as well as FGFR1, 2, 3 and 4, PDGFR, c-Kit and the RET proto-oncogene. Combination of pembrolizumab and lenvatinib has demonstrated the activation of CD8+ T cells, reduction of TAMS, leading to tumour reduction in murine models (98). A phase II non-randomised trial explored the combination of pembrolizumab with lenvatinib in MSS mCRC, demonstrating an ORR of 22% and a median PFS of 2.3 months (99). An ongoing randomised phase III trial (NCT04776148) is comparing lenvatinib plus pembrolizumab to standard of care in refractory MSS mCRC patients.
4 Hepatocellular carcinoma
HCC is the third leading cause of cancer-related death worldwide and its incidence is increasing globally (5). Most patients will be diagnosed at or progress to advanced stages, where systemic therapies remain the only effective option (100). Anti-angiogenic therapies constitute the treatment backbone of advanced HCC and their combination with immunotherapies has provided unprecedented benefits to this population (100–102). However, this has not yet been translated into the intermediate and early disease settings, and is an area of active research (103).
4.1 Clinical overview of anti-angiogenic drugs in HCC
4.1.1 Advanced disease: First line setting
Sorafenib is the first TKI to demonstrate increased survival in HCC. It was approved in 2007 based on the results of the SHARP trial, an international, placebo-controlled phase III trial that randomised 602 patients with advanced HCC (BCLC-C or BCLC-B stage not amenable to transarterial chemo-embolisation [TACE]) with preserved liver function and performance status of 0-2, to sorafenib or placebo (Table 2). Sorafenib increased OS (median 10.7 vs 7.9 months, HR 0.69, 95% CI 0.55-0.87) and time to radiologic progression (median 5.5 vs 2.8 months, HR 0.58, 95% CI 0.45-0.74). The median duration of treatment was 5.3 months and the overall incidence of treatment-related AEs was 80% (104). These results were further supported by the Asia-Pacific trial, a randomised, confirmatory phase III trial with a similar design that was performed in China, South Korea and Taiwan, and randomised 271 patients to sorafenib or placebo (105). Sorafenib increased OS (median 6.5 vs 4.2 months, HR 0.68, 95% CI 0.5-0.93) although the median survival times were less than in the SHARP trial, owing to the inclusion of more advanced patients, with a higher proportion of BCLC-C patients (95% vs 82%), extrahepatic spread (69% vs 53%) and worse performance status (ECOG PS1 69% vs 38%).
Since the approval of sorafenib, it became the standard comparator arm in all phase III trials, most of which led to disappointing results (106–109). Lenvatinib is the first TKI to have demonstrated non-inferiority in terms of OS compared with sorafenib (110). The REFLECT trial was a phase III, international, sorafenib-controlled study that randomised 954 advanced HCC patients without main portal vein thrombosis, less than 50% of liver occupation and absence of invasion of the bile duct, to lenvatinib or sorafenib. Lenvatinib showed non-inferiority in terms of OS (median 13.6 vs 12.3 months, HR 0.92, 95% CI 0.79-1.06) but did not achieve superiority. However, lenvatinib did show superior PFS (median 7.4 vs 3.7 months, HR 0.66, 95% CI 0.57-0.77) and ORR (24.1% vs 9.2%, odds ratio 3.13, 95% CI 2.15-4.56) according to investigator assessment using mRECIST (110, 111). The open-label design of the study may have influenced the unexpected differences in treatment duration and time to progression between the sorafenib and lenvatinib arms (112), although subsequent real-world studies have confirmed the efficacy of lenvatinib (113).
The combination of atezolizumab-bevacizumab has become the new standard of care first line treatment in advanced HCC (114, 115). The IMbrave150 trial was a phase III international, sorafenib-controlled trial that enrolled 501 patients randomised in a 2:1 ratio to atezolizumab-bevacizumab or sorafenib. The trial met its primary endpoint of OS, showing an increase of 5.8 months at final analysis (median 19.2 vs 13.4 months, respectively, HR 0.66, 95% CI 0.52-0.85) (116). Additionally, the combination improved PFS (median 6.9 vs 4.3 months, HR 0.65, 95% CI 0.53-0.81) and ORR (30% vs 11%, P<0.001) (116). Treatment-related grade 3-4 AEs were observed in 43% of the patients in the atezolizumab-bevacizumab arm and 46% of the patients in the sorafenib arm. Importantly, five fatal upper gastrointestinal bleeding events were observed in the experimental arm, which were attributed to bevacizumab (116). Two previous phase II trials testing bevacizumab monotherapy had shown an increased risk of upper gastrointestinal bleeding in 7-11% of patients (117, 118). To mitigate this risk, a mandatory esophagogastroduodenoscopy had to be performed in the 6 months prior to enrolment and any varices had to be treated per local standard of care (114). A similarly designed phase III trial was reported in China and evaluated the combination of sintilimab, a programmed cell death protein 1 (PD-1) inhibitor, with IBI-305, a bevacizumab biosimilar. The trial randomised 571 patients to sintilimab-bevacizumab biosimilar or sorafenib in a 2:1 ratio and showed an improvement in OS (median not reached vs 10.4 months, HR 0.57, 95% CI 0.43-0.75) and PFS (median 4.6 vs 2.8 months, HR 0.56, 95% CI 0.46-0.7) (119). Both of these trials have demonstrated the efficacy of combining anti-VEGFA antibodies with ICIs.
The results of the COSMIC-312 trial, a phase III trial that tested the combination of cabozantinib and atezolizumab (120), enrolled 877 patients who were randomly assigned to the combination, cabozantinib or sorafenib in a 2:1:1 ratio. The dual primary endpoints are OS and PFS. The interim analysis demonstrated an improvement in PFS in the modified intention-to-treat population comprising the first 372 randomised patients (median 6.8 vs 4.2 months, HR 0.63, 95% CI 0.44-0.91), however, no improvement in OS was observed in the intention-to-treat population (median 15.4 vs 15.5 months, HR 0.9, 95% CI 0.69-1.18). The combination of TKIs with immunotherapies has been recently explored in two additional trials (121, 122). The LEAP-002 trial is an international, phase III, randomized, double blind study that enrolled 794 patients with advanced HCC and were randomly assigned to the combination of lenvatinib-pembrolizumab (N=395) or lenvatinib alone (N=399). The trial did not reach the prespecified threshold for none of the dual primary endpoints (OS and PFS). However, the median OS for the combination arm was the longest survival reported to date in the first-line setting (21.2 months). Importantly, this data further supported the role of lenvatinib monotherapy in this setting, with a median OS of 19 months (121). A second trial reported at ESMO 2022 was the combination of camrelizumab, an anti-PD1 monoclonal antibody, and rivoceranib, a VEGFR2-TKI, in the first-line setting. This was an international, phase III, open-label study that compared the combination to sorafenib (122). The dual primary endpoints were PFS and OS. The trial met it’s endpoints and showed a significant increase in OS (median 22.1 vs 15.2, HR 0.62 95% CI 0.49-0.8) and PFS (median 5.6 vs 3.7, HR 0.52 95% CI 0.41-0.65), as well as an increase in ORR (25.4 vs 5.9%). Despite these encouraging results, the combination will have to be tested in other populations as most of the included patients were Chinese. Furthermore, the open-label design of the study led to a high number of consent withdrawals in the control arm, which will have to be explored to understand its potential impact on the study results.
In China, the TKI donafenib has proved to be superior to sorafenib in the first line setting of advanced HCC (123).
4.1.2 Advanced disease: Second line setting
Three TKIs have demonstrated improved outcomes in the second line setting after progression on sorafenib, regorafenib (124), cabozantinib (125) and ramucirumab in patients with alpha-feto protein (AFP) levels ≥400 ng/mL (126). The RESORCE trial was an international, phase III, double-blind, placebo-controlled trial that randomised 573 patients to regorafenib or placebo and was stratified based on region, performance status, macrovascular invasion, extrahepatic spread and AFP levels (124). Importantly, only patients who had previously tolerated sorafenib, defined as patients who had received ≥400 mg/day for ≥20 of the last 28 days of treatment, were included. Regorafenib significantly improved OS compared to placebo (median 10.6 vs 7.8 months, HR 0.63, 95% CI 0.5-0.79) and PFS assessed by mRECIST (median 3.1 vs 1.5 months, HR 0.56, 95% CI 0.37-0.56) (124). The rate of grade 3-4 treatment-related AEs was 50% in the regorafenib arm compared with 17% in the placebo arm.
The CELESTIAL trial had a similar design to the RESORCE trial although prior tolerance to sorafenib was not mandatory and patients could have progressed on up to two lines of systemic treatment. The trial randomised 707 patients in a 2:1 ratio to cabozantinib or placebo, stratified by region, macrovascular invasion, extrahepatic spread and disease etiology (125). It met its primary endpoint of OS (median 10.2 vs 8 months, HR 0.76, 95% CI 0.63-0.92) and showed a significant prolongation of PFS (5.2 vs 1.9 months, HR 0.44, 95% CI 0.36-0.52). Therefore, cabozantinib is the only TKI with evidence of efficacy in HCC following two prior lines of systemic treatment.
Ramucirumab was initially tested in the phase III REACH trial in the second line setting of advanced HCC following progression to sorafenib (127). Despite the trial being negative, an exploratory subgroup analysis showed significant benefit in patients with a baseline AFP level of ≥400 ng/mL. Hence, the REACH-2 study was designed as an international, phase III, double-blind, placebo-controlled trial and randomised 292 patients with an AFP level ≥400 ng/mL to ramucirumab or placebo (126). Ramucirumab increased OS (median 8.5 vs 7.3 months, HR 0.71, 95% CI 0.53-0.95) and PFS (2.8 vs 1.6 months, HR 0.45, 95% CI 0.34-0.6). A pooled analysis of all patients with baseline AFP levels ≥400 ng/mL (N=542) in both REACH and REACH-2 trials confirmed the survival benefit (median 8.1 vs 5 months, HR 0.69, 95% CI 0.57-0.84) (126).
In China, the VEGFR-2 inhibitor apatinib has improved survival in the second or third line settings of advanced HCC following treatment with sorafenib or FOLFOX (128).
4.2 Early and intermediate setting
The use of anti-angiogenic therapies in earlier settings of HCC have so far provided disappointing results. In the early setting, the only phase III trial to have tested TKIs as adjuvant therapy is the STORM study, an international, phase III, double-blind, placebo-controlled trial that enrolled 1114 patients with HCC suitable for local treatment (either ablation or resection) and a high or intermediate risk of recurrence (defined as tumours >2 cm or vascular invasion or satellites) to either adjuvant sorafenib for 4 years or placebo. No difference was observed in the primary endpoint of recurrence-free survival (median 33.3 vs 33.7 months, HR 0.94, 95% CI 0.78-1.13) or in OS (median not reached, HR 0.995, 95% CI 0.76-1.3) (129).
In the intermediate setting, three TKIs have been tested in combination with TACE in four phase III trials (130), namely, sorafenib (131, 132), brivanib (133) and orantinib (134). Unfortunately, none of these trials demonstrated an OS benefit compared with TACE alone. Whilst all four trials had a similar design, the primary endpoints were different: BRISK-TA (133) and ORIENTAL (134) trials assessed OS, while the trials testing sorafenib used time to progression (131) or PFS (132). Despite these discouraging results, the TACTICS trial was recently published, testing the combination of sorafenib initiated 2-3 weeks before TACE compared with TACE alone (135). This was a phase II, open-label, multicentre trial that enrolled 156 patients with a co-primary endpoint of OS and PFS. However, the definition of progression in this trial was unconventional and included untreatable tumour progression, transient deterioration to Child-Pugh C or appearance of vascular invasion/extrahepatic spread. The trial demonstrated a significant improvement in PFS (median 25.2 vs 13.5 months, HR 0.59, 95% CI 0.41-0.87) (135) but did not show any improvement in OS at the final analysis (median 36.2 vs 30.8 months, HR 0.86, 95% CI 0.61-1.22), casting doubts on the true value of this unconventional definition of progression (136).
Therefore, to date, no anti-angiogenic therapy is recommended in earlier settings of HCC.
4.3 Predictive biomarkers of anti-angiogenic drugs in HCC
The field of biomarker discovery in HCC is daunting and has so far led to disappointing results. The only available FDA-approved biomarker to guide treatment decision is AFP before initiating ramucirumab, based on the results of the REACH and REACH-2 trials (126, 127). No other biomarker has proven capable of predicting response to other anti-angiogenic therapies.
An exploratory analysis of 10 plasma markers (Ang2, EGF, bFGF, VEGF, sVEGFR-2, sVEGFR-3, HGF, s-c-KIT, IGF-2 and Ras) of patients enrolled in the SHARP trial found that despite that Ang2 and VEGF independently predicted survival in the entire cohort, none of the biomarkers assessed could predict response to sorafenib (137). In the sorafenib arm, high s-c-KIT and low HGF showed a trend towards enhanced survival (P-values of interaction 0.081 and 0.073, respectively) (137). Given the inherent difficulties of acquiring tissue specimens from advanced HCC patients, the same authors performed a thorough transcriptomic assessment of patients enrolled in the STORM trial, who were surgically resected and received sorafenib in the adjuvant setting (138). Tumour specimens from 188 patients were analysed by gene expression profiling, targeted exome sequencing, immunohistochemistry and fluorescence in situ hybridisation for VEGFA. None of the tested biomarkers, gene signatures or mutations predicted survival. A 146-gene signature was generated that could predict improved recurrence-free survival with sorafenib, although this has not been translated into the clinical setting due to lack of validation (138). Additionally, genomic variations of the SCL15A2 gene, involved in drug transport, have been proposed as an additional biomarker of response to sorafenib (139). In line with this, a retrospective study found that the expression of OCT1 (another major player involved in sorafenib uptake) in the plasma membrane was associated with improved outcomes following sorafenib treatment (140). These studies highlight the importance of SLC transporters in sorafenib uptake and underline their possible impact on patient survival.
To identify potential biomarkers of response to regorafenib, an analysis of plasma from patients enrolled in the RESORCE trial was performed (141). The authors analysed 294 plasma proteins and 750 miRNAs. Additionally, next-generation sequencing of tumour tissue from 7 responders and 10 non-responders and expression of 770 genes involved in oncogenic and inflammatory pathways in 46 tumour tissues was performed (141). Decreased baseline plasma concentrations of five proteins (angiopoietin 1, cystatin B, the latency-associated peptide of transforming growth factor beta 1, oxidised low-density lipoprotein receptor 1 and C-C motif chemokine ligand 3) was associated with improved survival with regorafenib. Additionally, nine miRNAs were also associated with improved survival with regorafenib (MIR30A, MIR122, MIR125B, MIR200A, MIR374B, MIR15B, MIR107, MIR320, and MIR645) (141).
In a similar plasma analysis including VEGF, ANG2, FGF19, FGF21 and FGF23 of 407 patients included in the REFLECT study, a higher baseline level of FGF21 was predictive for longer OS with lenvatinib compared with sorafenib (P-value of interaction 0.0397) (142). Similarly, a plasma analysis of 674 patients included in the CELESTIAL trial did not identify any biomarkers predictive of response to cabozantinib (143). High levels of MET, HGF, GAS6, IL-8 and ANG2 and low levels of IGF-1 were associated with shorter survival in the placebo arm and this association was also observed for MET, IL-8, and ANG2 in the cabozantinib group (143).
More recently, an integrated molecular analysis was performed, comprising RNA sequencing, DNA sequencing and simple and multiplex immunohistochemistry of 358 patients included in the phase Ib GO30140 (144) and the phase III IMbrave150 trial (114, 116). This showed that pre-existing immunity, including the expression of a T effector transcriptomic signature and CD8+ T cell infiltration, predicted response to the combination of atezolizumab-bevacizumab, but not to sorafenib (145). Importantly, improved outcomes for the combination vs atezolizumab monotherapy was associated with high VEGFR-2 expression. Conversely, reduced benefit from the combination was associated with a low Treg/effector T cell ratio (145). These data highlight the synergistic effects of the combination of atezolizumab-bevacizumab and suggest several predictive biomarkers that will need validation in future trials.
4.4 Novel therapeutic strategies targeting angiogenesis in HCC
The breakthrough marked by the IMbrave150 trial with the atezolizumab-bevacizumab combination, has fuelled the development of multiple combinations that are being tested across all settings of this disease (102). Preclinical data strongly support combining anti-angiogenic drugs with immunotherapies and local treatments in the intermediate setting (101, 103). Local ablation or TACE increases the release of antigens, proinflammatory cytokines and proangiogenic factors (such as VEGF-A and HIF1) which promote an immune response that can be further sustained by increasing the activation of cytotoxic cells through immune checkpoint inhibition and decreasing the infiltration of immunosuppressive cells such as MDSCs and Tregs through the inhibition of angiogenesis (103). This constitutes the rationale for the design of trials combining TACE with durvalumab/bevacizumab (NCT03778957), atezolizumab/bevacizumab (NCT04712643) or pembrolizumab/lenvatinib (NCT04246177) (Table 3). More intriguingly, the outstanding survival outcomes observed with the atezolizumab and bevacizumab combination in the advanced setting, with a median OS of 19.2 months - which is similar to the expected survival of intermediate-stage HCC treated with TACE (100) - has sparked the development of two trials comparing standard TACE directly with systemic treatment (atezolizumab/bevacizumab [NCT04803994] or regorafenib/nivolumab [NCT04777851]) (Table 3).
Applying these combinations in earlier settings, when cure is still possible, is being eagerly pursued in phase III clinical trials. Three trials are currently exploring atezolizumab-bevacizumab (NCT04102098), camrelizumab/apatinib (NCT04639180) and sintilimab/bevacizumab (NCT04682210) in the postsurgical setting to decrease the risk of recurrence (Table 3). However, T-cell priming after the tumour was removed as this can be less efficient due to the close-to-non-existent tumour antigen burden (101). In addition, the high response rate achieved with new combinations could facilitate downstaging and improve tumour resectability when applied in the pre-surgical setting. Accordingly, a phase Ib study that enrolled 15 patients with unresectable HCC who received 8 weeks of neoadjuvant cabozantinib and nivolumab found that 13 patients ultimately underwent resection, 12 of whom had no residual tumour and 5 had major or complete pathological response. Importantly, none of the patients presented disease progression according to RECIST 1.1 (146).
Novel treatments and combinations are being explored in the advanced setting of HCC (Table 3). Lenvatinib-pembrolizumab constitutes one of the most promising combinations based on the phase Ib KEYNOTE-524 trial that enrolled 100 advanced HCC patients who had received no prior systemic treatment (147). The combination achieved an ORR of 46% according to mRECIST, with a disease control rate of 88%, a median OS of 22 months and median PFS of 9.3 months (147). This combination is currently being evaluated in a phase III trial (NCT03713593). To optimize the sequencing of TKIs and ICIs, the GOING trial (NCT04170556) is evaluating the priming effect of regorafenib monotherapy administered for 8 weeks prior to incorporating nivolumab into the regimen. Finally, the IMbrave251 trial (NCT04770896) is evaluating the combination of atezolizumab with sorafenib or lenvatinib in the second line following progression on atezolizumab-bevacizumab. These and other trials shown in Table 3 are likely to change the treatment landscape of HCC in the near future.
5 Gastroesophageal cancer
Gastroesophageal cancers are a group of aggressive and highly lethal neoplasms. Gastric cancer represents the fifth most common cancer and the fourth most common cause of cancer-related death, while oesophageal cancer ranks seventh in terms of incidence and sixth in terms of mortality (5). Despite substantial advances over the last decade, prognosis remains poor, with an overall 5-year OS rate of 29% and 20% for gastric and oesophageal cancer, respectively (5). The pre-malignant form of oesophageal adenocarcinoma, known as Barrett’s oesophagus, expresses high levels of VEGFR2 (148). In gastric cancer, VEGF expression in tumour tissue or blood are correlated with prognosis, stage and risk of metastasis (149). Human epidermal growth factor receptor 2 (HER2) therapy and anti-angiogenic agents are the only two biological targeted therapies that have improved OS in patients with gastric or gastroesophageal junction adenocarcinoma.
There are currently no anti-angiogenic therapies approved for the treatment of oesophageal cancer. Small anti-angiogenic molecules such as sunitinib (in combination with paclitaxel or FOLFIRI) or sorafenib (in combination with docetaxel and cisplatin), erlotinib (with bevacizumab and neoadjuvant chemoradiation), apatinib (as maintenance treatment after chemo-radiation in localised oesophageal squamous cell carcinoma) or anlotinib (as monotherapy in the refractory setting) have shown limited or no efficacy in small phase II trials (150–154). Combinations of TKIs with immunotherapy are being evaluated (155). Bevacizumab was evaluated in combination with chemotherapy in two phase II trials, and was safe but with limited benefits (156, 157).
In gastric cancer, bevacizumab was evaluated in the AVAGAST phase III trial, comparing standard chemotherapy with or without bevacizumab, failing to show improvement in OS (158, 159). The phase III REGARD trial randomised patients with advanced gastric cancer to receive ramucirumab or placebo as second line treatment, with an OS of 5.2 vs 3.8 months, respectively (HR 0.776; P=0.047) (160). Following these results, the FDA approved ramucirumab for advanced gastric and gastroesophageal junction adenocarcinomas in 2014. The RAINBOW phase III trial compared weekly paclitaxel in combination with ramucirumab or placebo in patients refractory to a fluoropyrimidine plus platinum combination. Median OS was 9.6 months in the ramucirumab plus paclitaxel arm vs 7.4 months in the placebo plus paclitaxel group (HR 0.807; P=0.017), and paclitaxel plus ramucirumab became a recommended standard second line treatment for gastric cancer (161). RAINFALL was a global phase III trial that compared cisplatin plus capecitabine or 5-FU in combination with ramucirumab or placebo in the first line setting of patients with gastric or gastroesophageal junction adenocarcinoma (162). This trial demonstrated an improvement in PFS (5.7 vs 5.4 months; HR 0.75, P=0.011) but not in OS (11.2 vs 10.7 months; HR 0.96). Multiple trials are testing the combination of immunotherapy with ramucirumab, with promising signals of efficacy (163–165).
Apatinib is a small TKI that was tested in a Chinese phase III trial that compared apatinib with placebo in patients with refractory advanced gastric cancer, showing a statistically significant difference in OS (6.5 vs 4.7 months; P=0.0156) that led to its approval by the Chinese regulatory agency (166). The ANGEL trial (NCT03042611) is ongoing to confirm these results in the global population.
Other molecules such as aflibercept (in combination with FOLFOX), sorafenib (in combination with capecitabine plus cisplatin in the first line setting), sunitinib (in combination with FOLFIRI in second or third line) pazopanib (in combination with 5-FU plus leucovorin plus oxaliplatin) or fruquintinib (in combination with paclitaxel in second line treatment in China) have been tested in phase II trials, showing only marginal benefit in PFS (151, 167–171).
6 Neuroendocrine cancer
Neuroendocrine tumours (NETs) are a heterogeneous family of neoplasms that can arise from almost everywhere throughout the body, as they originate from the diffuse neuroendocrine system. NETs are rare (less than 7 new cases/100,000 inhabitants/year), however their incidence has increased over the last few decades (172). Well-differentiated NETs are characterised by rich vascularisation, with this phenomenon known as the “neuroendocrine paradox”, as the vascularisation is inversely related to the grade of aggressiveness of the tumour. NETs show high expression of PDGFR and c-Kit, demonstrating the importance of the angiogenesis pathway of these tumours (173). Sunitinib and surufatinib have shown activity in phase III trials vs placebo.
Sunitinib was tested in a phase III placebo-controlled trial of patients with advanced, well-differentiated pancreatic NETs (pNETs), in which patients could have received prior treatment (174). The study was discontinued early as a difference between placebo and sunitinib arm was observed benefiting patients in the active control arm. Median PFS was 11.4 months in the sunitinib group compared with 5.5 months in the placebo group (HR: 0.42; P<0.001). Posterior actualised data showed an improvement of median OS (38.6 vs 29.1 months in sunitinib vs placebo, respectively) (175). Surufatinib has shown benefit in a phase III placebo-controlled trial in advanced pancreatic NETs in a Chinese population (median PFS 10.9 months for surufatinib vs 3.7 months for placebo; HR 0.49; P=0.0011) (176). This agent has also reported benefit over placebo for extra-pancreatic advanced NETs in a Chinese population (176). Different phase II trials have tested multikinase inhibitors such as pazopanib, lenvatinib and cabozantinib, showing clinical activity in patients with NETs (177–181). Two ongoing phase III trials are evaluating axitinib (NCT01744249) and cabozantinib (NCT03375320).
7 Pancreatic and biliary tract cancer
Pancreatic cancer is a highly lethal disease with a rising incidence of 0.5-1% per year and is expected to become the second leading cause of cancer death by 2030 in the United States (182). Multiagent chemotherapy is recommended across all stages of the disease, either perioperatively in resectable/borderline resectable disease or to improve survival outcomes in advanced stages (183, 184). To date, only gemcitabine combined with albumin-bound paclitaxel (185) and FOLFIRINOX (fluorouracil, irinotecan, oxaliplatin, leucovorin) (186) regimens have demonstrated superiority over gemcitabine monotherapy in the first line metastatic setting, while FOLFOX (fluorouracil, oxaliplatin, leucovorin) (187, 188) and fluorouracil combined with liposomal irinotecan (189) have improved outcomes in the second line setting following a gemcitabine-based regimen. Most trials testing anti-angiogenic agents were performed before these combinations were approved and were combined with gemcitabine monotherapy in the first line setting. Bevacizumab was tested in two independent trials (Table 4). First, the CALGB80303 phase III trial randomised 602 advanced pancreatic cancer patients to gemcitabine monotherapy or combined with bevacizumab and showed no improvement in OS (median 5.8 vs 5.9 months, HR 1.05, 95% CI 0.88-1.24) (190). A second study tested the combination of bevacizumab with gemcitabine and erlotinib compared with gemcitabine and erlotinib alone and found a significant improvement in PFS (median 4.6 vs 3.6 months, HR 0.73, 95% CI 0.61-0.86) but no difference in OS (median 7.1 vs 6 months, HR 0.89, 95% CI 0.74-1.07) (191). Aflibercept was explored in a similarly designed phase III trial in combination with gemcitabine but was stopped early due to futility (192) and in a phase III trial with elpamotide, a peptide targeting VEGFR-2, the primary endpoint of OS was not reached when combined with gemcitabine (193). Additionally, several randomised phase II and III investigations have explored the use of different TKIs combined with gemcitabine including axitinib (194), sorafenib (195) and sunitinib (196), also failing to show any significant survival improvement over gemcitabine alone. More recently, the HCRN GI14-198 phase II trial tested ramucirumab in combination with a multiagent chemotherapy and randomised 86 patients diagnosed with treatment-naïve advanced pancreatic cancer to modified FOLFIRINOX combined with ramucirumab or placebo (197). The trial failed to improve outcomes in terms of PFS, ORR and OS (197). The reasons behind the failure of these trials are largely unknown, although novel therapies modulating the desmoplastic microenvironment and tumour stroma may help to enhance the clinical activity of anti-angiogenic therapies in this disease.
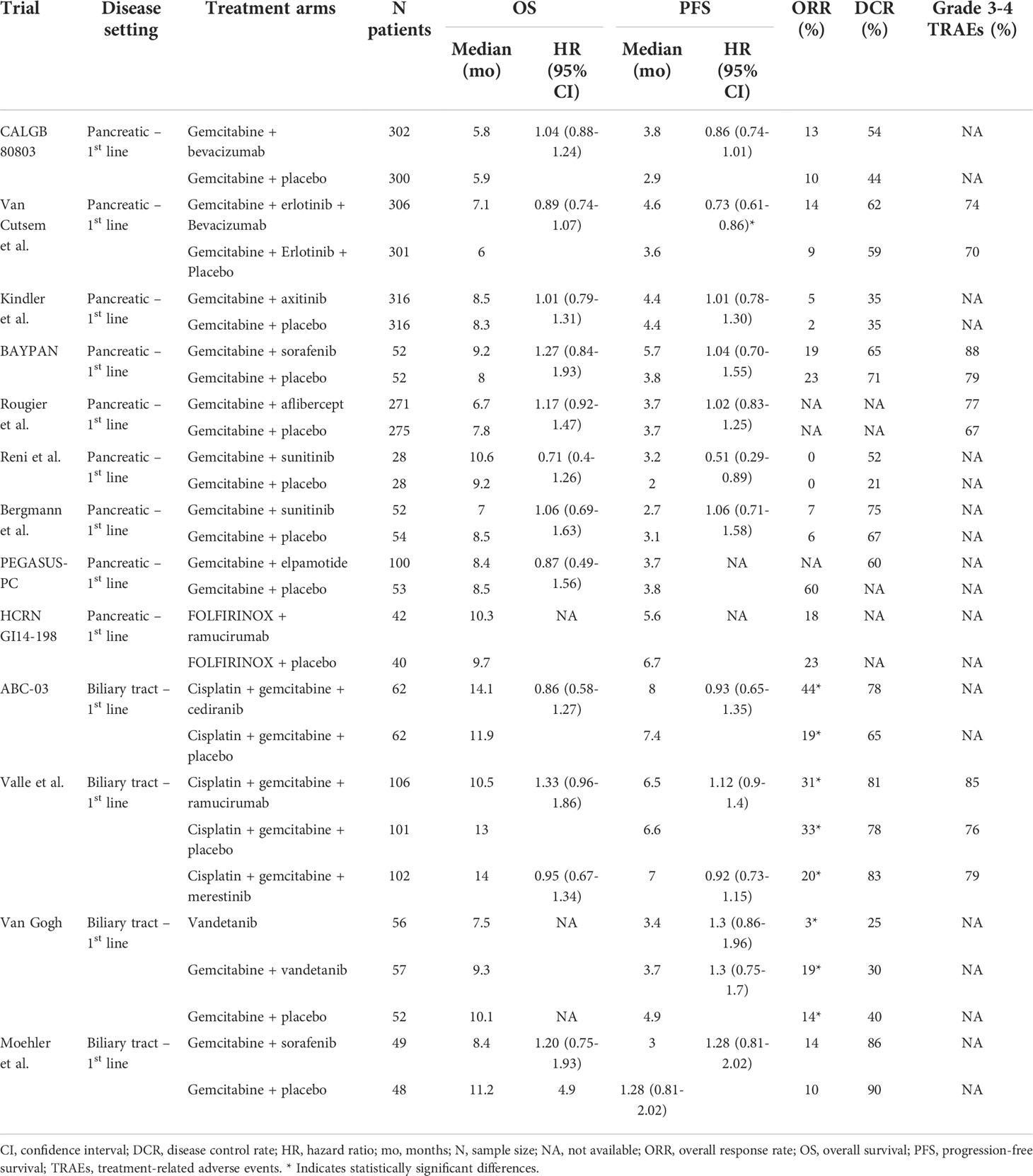
Table 4 Overview of selected phase II-III trials testing antiangiogenic agents in pancreatic and biliary tract cancer.
Biliary tract cancer refers to a spectrum of malignancies including cholangiocarcinoma and gallbladder adenocarcinoma (198). Their incidence is increasing globally, with a 5-year OS rate bordering 10%, and they represent ~2% of all cancer-related deaths worldwide annually (199). In advanced stages, the combination of cisplatin and gemcitabine has remained the established first line chemotherapy regimen for the past 12 years (200, 201), although this is likely to change given the improved survival observed with the addition of durvalumab in the TOPAZ-1 trial (202). The use of anti-angiogenic drugs has only been explored in phase II investigations and none of these combinations has reached later stages of development (Table 4). The largest of these trials was a randomised, phase II, three-arm trial exploring the combination of ramucirumab, merestinib or placebo with cisplatin-gemcitabine. The trial failed to meet its primary endpoint of PFS (median 6.5 vs 7 vs 6.6 months, ramucirumab vs placebo HR 1.12, 95% CI 0.9-1.4) (203). An exploratory analysis of mutations and gene expression signatures identified no predictive biomarkers (203). The ABC-03 trial was a randomised phase II trial that tested the combination of cediranib, an oral VEGFR-1, -2 and -3 inhibitor, with cisplatin and gemcitabine and showed no improvement in outcomes compared with placebo (204). In an exploratory biomarker analysis, circulating PDGFbb levels predicted benefit from cediranib (P-value of interaction 0.002) (204). Other trials that compared suboptimal chemotherapy regimens combined with sorafenib (205) or vandetanib (206) also failed to improve survival. New strategies, including the potential synergy of anti-angiogenic therapies when combined with immunotherapies and chemotherapy, are leading to novel combinations that could change the treatment landscape in the near future.
8 Discussion and future prospects
Anti-angiogenic therapies have been extensively evaluated across many gastrointestinal and hepatobiliary tumours. In some malignancies, such as HCC or CRC, these therapies provide unquestionable survival benefits either alone or combined with immunotherapy or chemotherapy, respectively. In others, such as oesophageal, biliary tract or pancreatic cancers, anti-angiogenics do not improve outcomes when combined with currently approved therapies. The development of novel anti-angiogenic strategies has stalled in recent years, partly due to the concurrent development of novel and highly effective drugs, especially immune-based therapies (207). However, whilst new anti-angiogenic drugs are not expected to enter the clinical setting in the near future, the use of approved anti-angiogenic therapies is likely to increase exponentially, owing to their highly synergistic effect with immunotherapies and other drug families (28). New combinations and applications in earlier disease settings is already being intensively explored in many diseases and will reshape the treatment scenario (101). Furthermore, recent studies have unveiled the heterogeneity of tumour endothelial cells and may support the use of patient-tailored antiangiogenic drug combinations to overcome this heterogeneity (40–42). Importantly, novel insights on resistance mechanisms support the combination of antiangiogenic drugs with distinct partners, such as inhibitors of the TGFß pathway, autophagy or CXCR4 (39). An intriguing area of research is the determination of the ideal dose of anti-angiogenic agents, especially when combined with other therapies. Until now, this has been based on the maximum tolerated dose in most cases, reflecting the belief that the higher dose of a drug will lead to a higher efficacy. Although this may be true for cytotoxic agents, this principle may not apply to anti-angiogenic drugs, where normalisation of blood vessels may be more important than vessel depletion to improve their synergistic effects (22).
Finally, the development of robust and validated biomarkers represents a clear unmet need in this field. It is unlikely that a unique biomarker common to distinct anti-angiogenic therapies will be identified owing to their diverse and heterogenous mechanisms of action, as well as inter and intra-tumoural heterogeneity. Furthermore, the utility of these potential biomarkers may be dependent on the combination partner, further emphasising the need to develop unique biomarkers for specific diseases and specific combinations. Despite these challenges, the importance of biomarker discovery in this field remains paramount. Radiomics, the study of vasculature in preclinical in vivo models and the analysis of new circulating angiogenic factors could shed some light on this unmet clinical need. Clinicians need additional tools to select the optimal therapy in each individual patient, given the increasingly complex treatment scenarios resulting from the continual approval of novel agents and combinations. Effective biomarkers would enable a better selection of patients and avoid unnecessary toxicities in those patients who are not expected to derive benefit from these agents. Therefore, adapting modern clinical trials to integrate biomarker-based objectives and pre-planned exploratory post-hoc analysis of baseline and on-treatment patient samples is fundamental and will become standard practice in the design of future trials.
Author contributions
NS and FC have contributed equally to this work and share first authorship. All authors contributed to the article and approved the submitted version.
Acknowledgments
The authors thank Sarah MacKenzie, PhD, for providing medical writing support.
Conflict of interest
Author EE declares personal financial interests for consulting/advisory role and/or honoraria, travel grants and research grants from Amgen, Bayer, Hoffman-La Roche, Merck Serono, Sanofi, Pierre Fabre, MSD, Organon, Novartis and Servier. EE also declares institutional financial interests in the form of financial support for clinical trials or contracted research for Amgen Inc, Array Biopharma Inc, AstraZeneca Pharmaceuticals LP, BeiGene, Boehringer Ingelheim, Bristol Myers Squibb, Celgene, Debiopharm International SA, F. Hoffmann-La Roche Ltd, Genentech Inc, HalioDX SAS, Hutchison MediPharma International, Janssen-Cilag SA, MedImmune, Menarini, Merck Health KGAA, Merck Sharp & Dohme, Merus NV, Mirati, Novartis Farmacéutica SA, Pfizer, Pharma Mar, Sanofi Aventis Recherche & Développement, Servier, Taiho Pharma USA Inc. Author JT reports personal financial interest in form of scientific consultancy role for Array Biopharma, AstraZeneca, Bayer, Boehringer Ingelheim, Chugai, Daiichi Sankyo, F. Hoffmann-La Roche Ltd, Genentech Inc, HalioDX SAS, Hutchison MediPharma International, Ikena Oncology, Inspirna Inc, IQVIA, Lilly, Menarini, Merck Serono, Merus, MSD, Mirati, Neophore, Novartis, Ona Therapeutics, Orion Biotechnology, Peptomyc, Pfizer, Pierre Fabre, Samsung Bioepis, Sanofi, Scorpion Therapeutics, Scandion Oncology, Seattle Genetics, Servier, Sotio Biotech, Taiho, Tessa Therapeutics and TheraMyc. And also educational collaboration with Imedex, Medscape Education, MJH Life Sciences, PeerView Institute for Medical Education and Physicians Education Resource (PER).
The remaining authors declare that the research was conducted in the absence of any commercial or financial relationships that could be construed as a potential conflict of interest.
Publisher’s note
All claims expressed in this article are solely those of the authors and do not necessarily represent those of their affiliated organizations, or those of the publisher, the editors and the reviewers. Any product that may be evaluated in this article, or claim that may be made by its manufacturer, is not guaranteed or endorsed by the publisher.
References
1. Hanahan D, Weinberg RA. The hallmarks of cancer. Cell (2000) 100:57–70. doi: 10.1016/S0092-8674(00)81683-9
2. Sherwood LM, Parris EE, Folkman J. Tumor angiogenesis: Therapeutic implications. New Engl J Med (1971) 285:1182–6. doi: 10.1056/NEJM197111182852108
3. Hanahan D, Folkman J. Patterns and emerging mechanisms of the angiogenic switch during tumorigenesis. Cell (1996) 86:353–64. doi: 10.1016/S0092-8674(00)80108-7
4. Lopes-Coelho F, Martins F, Pereira SA, Serpa J. Anti-angiogenic therapy: Current challenges and future perspectives. Int J Mol Sci (2021) 22. doi: 10.3390/IJMS22073765
5. Sung H, Ferlay J, Siegel RL, Laversanne M, Soerjomataram I, Jemal A, et al. Global cancer statistics 2020: GLOBOCAN estimates of incidence and mortality worldwide for 36 cancers in 185 countries. CA Cancer J Clin (2021) 71:209–49. doi: 10.3322/caac.21660
6. Takahashi Y, Tucker SL, Kitadai Y, Koura AN, Bucana CD, Cleary KR, et al. Vessel counts and expression of vascular endothelial growth factor as prognostic factors in node-negative colon cancer. Arch Surg (1997) 132:541–6. doi: 10.1001/ARCHSURG.1997.01430290087018
7. Liu L, Ma XL, Xiao ZL, Li M, Cheng SH, Wei YQ. Prognostic value of vascular endothelial growth factor expression in resected gastric cancer. Asian Pacific J Cancer Prev (2012) 13:3089–97. doi: 10.7314/APJCP.2012.13.7.3089
8. Karayiannakis AJ, Bolanaki H, Syrigos KN, Asimakopoulos B, Polychronidis A, Anagnostoulis S, et al. Serum vascular endothelial growth factor levels in pancreatic cancer patients correlate with advanced and metastatic disease and poor prognosis. Cancer Lett (2003) 194:119–24. doi: 10.1016/S0304-3835(03)00047-8
9. Hanahan D, Weinberg RA. Hallmarks of cancer: The next generation. Cell (2011) 144:646–74. doi: 10.1016/j.cell.2011.02.013
10. Bergers G, Benjamin LE. Tumorigenesis and the angiogenic switch. Nat Rev Cancer (2003) 3:401–10. doi: 10.1038/nrc1093
11. Baeriswyl V, Christofori G. The angiogenic switch in carcinogenesis. Semin Cancer Biol (2009) 19:329–37. doi: 10.1016/j.semcancer.2009.05.003
12. Olive KP, Jacobetz MA, Davidson CJ, Gopinathan A, McIntyre D, Honess D, et al. Inhibition of hedgehog signaling enhances delivery of chemotherapy in a mouse model of pancreatic cancer. Sci (1979) (2009) 324:1457–61. doi: 10.1126/science.1171362
13. Morse MA, Sun W, Kim R, He AR, Abada PB, Mynderse M, et al. The role of angiogenesis in hepatocellular carcinoma. Clin Cancer Res (2019) 25:912–20. doi: 10.1158/1078-0432.CCR-18-1254
14. Zhu AX, Duda DG, v. SD, Jain RK. HCC and angiogenesis: possible targets and future directions. Nat Rev Clin Oncol (2011) 8:292–301. doi: 10.1038/nrclinonc.2011.30
15. Scoazec J-Y. Angiogenesis in neuroendocrine tumors: Therapeutic applications. Neuroendocrinology (2013) 97:45–56. doi: 10.1159/000338371
16. Guinney J, Dienstmann R, Wang X, de Reyniès A, Schlicker A, Soneson C, et al. The consensus molecular subtypes of colorectal cancer. Nat Med (2015) 21:1350–6. doi: 10.1038/nm.3967
17. Cancer Genome Atlas Research NetworkComprehensive molecular characterization of gastric adenocarcinoma. Nature (2014) 513:202–9. doi: 10.1038/nature13480
18. Haas G, Fan S, Ghadimi M, de Oliveira T, Conradi L-C. Different forms of tumor vascularization and their clinical implications focusing on vessel Co-option in colorectal cancer liver metastases. Front Cell Dev Biol (2021) 9:612774. doi: 10.3389/fcell.2021.612774
19. Kuczynski EA, Vermeulen PB, Pezzella F, Kerbel RS, Reynolds AR. Vessel co-option in cancer. Nat Rev Clin Oncol (2019) 16:469–93. doi: 10.1038/s41571-019-0181-9
20. Lugano R, Ramachandran M, Dimberg A. Tumor angiogenesis: causes, consequences, challenges and opportunities. Cell Mol Life Sci (2020) 77:1745–70. doi: 10.1007/s00018-019-03351-7
21. Carmeliet P, Jain RK. Principles and mechanisms of vessel normalization for cancer and other angiogenic diseases. Nat Rev Drug Discovery (2011) 10:417–27. doi: 10.1038/nrd3455
22. Jain RK. Normalizing tumor microenvironment to treat cancer: Bench to bedside to biomarkers. J Clin Oncol (2013) 31:2205–18. doi: 10.1200/JCO.2012.46.3653
23. Jain RK. Normalizing tumor vasculature with anti-angiogenic therapy: A new paradigm for combination therapy. Nat Med (2001) 7:987–9. doi: 10.1038/nm0901-987
24. Jain RK. Normalization of tumor vasculature: An emerging concept in antiangiogenic therapy. Sci (1979) (2005) 307:58–62. doi: 10.1126/science.1104819
25. Khan KA, Kerbel RS. Improving immunotherapy outcomes with anti-angiogenic treatments and vice versa. Nat Rev Clin Oncol (2018) 15:310–24. doi: 10.1038/nrclinonc.2018.9
26. Fukumura D, Kloepper J, Amoozgar Z, Duda DG, Jain RK. Enhancing cancer immunotherapy using antiangiogenics: opportunities and challenges. Nat Rev Clin Oncol (2018) 15:325–40. doi: 10.1038/nrclinonc.2018.29
27. Rahma OE, Hodi FS. The intersection between tumor angiogenesis and immune suppression. Clin Cancer Res (2019) 25:5449–57. doi: 10.1158/1078-0432.CCR-18-1543
28. Huinen ZR, Huijbers EJM, van Beijnum JR, Nowak-Sliwinska P, Griffioen AW. Anti-angiogenic agents — overcoming tumour endothelial cell anergy and improving immunotherapy outcomes. Nat Rev Clin Oncol (2021) 18(8):527–40. doi: 10.1038/s41571-021-00496-y
29. Song Y, Fu Y, Xie Q, Zhu B, Wang J, Zhang B. Anti-angiogenic agents in combination with immune checkpoint inhibitors: A promising strategy for cancer treatment. Front Immunol (2020) 11:1956. doi: 10.3389/fimmu.2020.01956
30. Esteban-Fabró R, Willoughby CE, Piqué-Gili M, Montironi C, Abril-Fornaguera J, Peix J, et al. Cabozantinib enhances anti-PD1 activity and elicits a neutrophil-based immune response in hepatocellular carcinoma. Clin Cancer Res (2022), 28(11):2449–2460. doi: 10.1158/1078-0432.CCR-21-2517
31. Torrens L, Montironi C, Puigvehí M, Mesropian A, Leslie J, Haber PK, et al. Immunomodulatory effects of lenvatinib plus anti–programmed cell death protein 1 in mice and rationale for patient enrichment in hepatocellular carcinoma. Hepatology (2021) 74:2652–69. doi: 10.1002/hep.32023
32. Sprinzl MF, Puschnik A, Schlitter AM, Schad A, Ackermann K, Esposito I, et al. Sorafenib inhibits macrophage-induced growth of hepatoma cells by interference with insulin-like growth factor-1 secretion. J Hepatol (2015) 62:863–70. doi: 10.1016/j.jhep.2014.11.011
33. Shigeta K, Matsui A, Kikuchi H, Klein S, Mamessier E, Chen IX, et al. Regorafenib combined with PD1 blockade increases CD8 T-cell infiltration by inducing CXCL10 expression in hepatocellular carcinoma. J Immunother Cancer (2020) 8:e001435. doi: 10.1136/jitc-2020-001435
34. Ou D-L, Chen C-W, Hsu C-L, Chung C-H, Feng Z-R, Lee B-S, et al. Regorafenib enhances antitumor immunity via inhibition of p38 kinase/Creb1/Klf4 axis in tumor-associated macrophages. J Immunother Cancer (2021) 9:e001657. doi: 10.1136/jitc-2020-001657
35. Voron T, Colussi O, Marcheteau E, Pernot S, Nizard M, Pointet A-L, et al. VEGF-a modulates expression of inhibitory checkpoints on CD8+ T cells in tumors. J Exp Med (2015) 212:139–48. doi: 10.1084/jem.20140559
36. van Beijnum JR, Nowak-Sliwinska P, Huijbers EJM, Thijssen VL, Griffioen AW. The great escape; the hallmarks of resistance to antiangiogenic therapy. Pharmacol Rev (2015) 67:441–61. doi: 10.1124/pr.114.010215
37. Zhai B, Hu F, Jiang X, Xu J, Zhao D, Liu B, et al. Inhibition of akt reverses the acquired resistance to sorafenib by switching protective autophagy to autophagic cell death in hepatocellular carcinoma. Mol Cancer Ther (2014) 13:1589–98. doi: 10.1158/1535-7163.MCT-13-1043
38. Haibe Y, Kreidieh M, el Hajj H, Khalifeh I, Mukherji D, Temraz S, et al. Resistance mechanisms to anti-angiogenic therapies in cancer. Front Oncol (2020) 10:221. doi: 10.3389/fonc.2020.00221
39. Montemagno C, Pagès G. Resistance to anti-angiogenic therapies: A mechanism depending on the time of exposure to the drugs. Front Cell Dev Biol (2020) 8:584. doi: 10.3389/fcell.2020.00584
40. Goveia J, Rohlenova K, Taverna F, Treps L, Conradi L-C, Pircher A, et al. An integrated gene expression landscape profiling approach to identify lung tumor endothelial cell heterogeneity and angiogenic candidates. Cancer Cell (2020) 37:21–36.e13. doi: 10.1016/j.ccell.2019.12.001
41. Zhao Q, Eichten A, Parveen A, Adler C, Huang Y, Wang W, et al. Single-cell transcriptome analyses reveal endothelial cell heterogeneity in tumors and changes following antiangiogenic treatment. Cancer Res (2018) 78:2370–82. doi: 10.1158/0008-5472.CAN-17-2728
42. Zhao Q, Molina-Portela M del P, Parveen A, Adler A, Adler C, Hock E, et al. Heterogeneity and chimerism of endothelial cells revealed by single-cell transcriptome in orthotopic liver tumors. Angiogenesis (2020) 23:581–97. doi: 10.1007/s10456-020-09727-9
43. Sharma A, Seow JJW, Dutertre C-A, Pai R, Blériot C, Mishra A, et al. Onco-fetal reprogramming of endothelial cells drives immunosuppressive macrophages in hepatocellular carcinoma. Cell (2020) 183:377–394.e21. doi: 10.1016/j.cell.2020.08.040
44. National Cancer Institute, Bethesda M. Cancer stat facts: Colorectal cancer . Available at: https://seer.cancer.gov/statfacts/html/colorect.html.
45. van Cutsem E, Cervantes A, Nordlinger B, Arnold D. Metastatic colorectal cancer: ESMO clinical practice guidelines for diagnosis, treatment and follow-up. Ann Oncol (2014) 25:iii1–9. doi: 10.1093/annonc/mdu260
46. Venook AP, Niedzwiecki D, Lenz HJ, Innocenti F, Fruth B, Meyerhardt JA, et al. Effect of first-line chemotherapy combined with cetuximab or bevacizumab on overall survival in patients with KRAS wild-type advanced or metastatic colorectal cancer: A randomized clinical trial. JAMA (2017) 317:2392–401. doi: 10.1001/JAMA.2017.7105
47. Cremolini C, Loupakis F, Antoniotti C, Lupi C, Sensi E, Lonardi S, et al. FOLFOXIRI plus bevacizumab versus FOLFIRI plus bevacizumab as first-line treatment of patients with metastatic colorectal cancer: Updated overall survival and molecular subgroup analyses of the open-label, phase 3 TRIBE study. Lancet Oncol (2015) 16:1306–15. doi: 10.1016/S1470-2045(15)00122-9
48. Heinemann V, von Weikersthal LF, Decker T, Kiani A, Vehling-Kaiser U, Al-Batran S-E, et al. FOLFIRI plus cetuximab versus FOLFIRI plus bevacizumab as first-line treatment for patients with metastatic colorectal cancer (FIRE-3): a randomised, open-label, phase 3 trial. Lancet Oncol (2014) 15:1065–75. doi: 10.1016/S1470-2045(14)70330-4
49. Douillard J-Y, Oliner KS, Siena S, Tabernero J, Burkes R, Barugel M, et al. Panitumumab–FOLFOX4 treatment and RAS mutations in colorectal cancer. New Engl J Med (2013) 369:1023–34. doi: 10.1056/NEJMoa1305275
50. Tabernero J, Yoshino T, Cohn AL, Obermannova R, Bodoky G, Garcia-Carbonero R, et al. Ramucirumab versus placebo in combination with second-line FOLFIRI in patients with metastatic colorectal carcinoma that progressed during or after first-line therapy with bevacizumab, oxaliplatin, and a fluoropyrimidine (RAISE): a randomised, double-bli. Lancet Oncol (2015) 16:499–508. doi: 10.1016/S1470-2045(15)70127-0
51. van Cutsem E, Köhne C-H, Láng I, Folprecht G, Nowacki MP, Cascinu S, et al. Cetuximab plus irinotecan, fluorouracil, and leucovorin as first-line treatment for metastatic colorectal cancer: Updated analysis of overall survival according to tumor KRAS and BRAF mutation status. J Clin Oncol (2011) 29:2011–9. doi: 10.1200/JCO.2010.33.5091
52. van Cutsem E, Tabernero J, Lakomy R, Prenen H, Prausová J, Macarulla T, et al. Addition of aflibercept to fluorouracil, leucovorin, and irinotecan improves survival in a phase III randomized trial in patients with metastatic colorectal cancer previously treated with an oxaliplatin-based regimen. J Clin Oncol (2012) 30:3499–506. doi: 10.1200/JCO.2012.42.8201
53. Saltz LB, Clarke S, Díaz-Rubio E, Scheithauer W, Figer A, Wong R, et al. Bevacizumab in combination with oxaliplatin-based chemotherapy as first-line therapy in metastatic colorectal cancer: A randomized phase III study. J Clin Oncol (2008) 26:2013–9. doi: 10.1200/JCO.2007.14.9930
54. Bennouna J, Sastre J, Arnold D, Österlund P, Greil R, van Cutsem E, et al. Continuation of bevacizumab after first progression in metastatic colorectal cancer (ML18147): a randomised phase 3 trial. Lancet Oncol (2013) 14:29–37. doi: 10.1016/S1470-2045(12)70477-1
55. Peeters M, Price TJ, Cervantes A, Sobrero AF, Ducreux M, Hotko Y, et al. Final results from a randomized phase 3 study of FOLFIRI ± panitumumab for second-line treatment of metastatic colorectal cancer. Ann Oncol (2014) 25:107–16. doi: 10.1093/annonc/mdt523
56. Hurwitz H, Fehrenbacher L, Novotny W, Cartwright T, Hainsworth J, Heim W, et al. Bevacizumab plus irinotecan, fluorouracil, and leucovorin for metastatic colorectal cancer. New Engl J Med (2004) 350:2335–42. doi: 10.1056/NEJMoa032691
57. Tang W, Ren L, Liu T, Ye Q, Wei Y, He G, et al. Bevacizumab plus mFOLFOX6 versus mFOLFOX6 alone as first-line treatment for RAS mutant unresectable colorectal liver-limited metastases: The BECOME randomized controlled trial. J Clin Oncol (2020) 38:3175–84. doi: 10.1200/JCO.20.00174
58. Cunningham D, Lang I, Marcuello E, Lorusso V, Ocvirk J, Shin DB, et al. Bevacizumab plus capecitabine versus capecitabine alone in elderly patients with previously untreated metastatic colorectal cancer (AVEX): an open-label, randomised phase 3 trial. Lancet Oncol (2013) 14:1077–85. doi: 10.1016/S1470-2045(13)70154-2
59. Falcone A, Ricci S, Brunetti I, Pfanner E, Allegrini G, Barbara C, et al. Phase III trial of infusional fluorouracil, leucovorin, oxaliplatin, and irinotecan (FOLFOXIRI) compared with infusional fluorouracil, leucovorin, and irinotecan (FOLFIRI) as first-line treatment for metastatic colorectal cancer: The gruppo oncologico nord ovest. J Clin Oncol (2007) 25:1670–6. doi: 10.1200/JCO.2006.09.0928
60. Loupakis F, Cremolini C, Masi G, Lonardi S, Zagonel V, Salvatore L, et al. Initial therapy with FOLFOXIRI and bevacizumab for metastatic colorectal cancer. New Engl J Med (2014) 371:1609–18. doi: 10.1056/NEJMoa1403108
61. Cremolini C, Antoniotti C, Stein A, Bendell J, Gruenberger T, Rossini D, et al. Individual patient data meta-analysis of FOLFOXIRI plus bevacizumab versus doublets plus bevacizumab as initial therapy of unresectable metastatic colorectal cancer. J Clin Oncol (2020) 38:3314–24. doi: 10.1200/JCO.20.01225
62. Gruenberger T, Bridgewater J, Chau I, García Alfonso P, Rivoire M, Mudan S, et al. Bevacizumab plus mFOLFOX-6 or FOLFOXIRI in patients with initially unresectable liver metastases from colorectal cancer: the OLIVIA multinational randomised phase II trial. Ann Oncol (2015) 26:702–8. doi: 10.1093/annonc/mdu580
63. Cremolini C, Antoniotti C, Rossini D, Lonardi S, Loupakis F, Pietrantonio F, et al. Upfront FOLFOXIRI plus bevacizumab and reintroduction after progression versus mFOLFOX6 plus bevacizumab followed by FOLFIRI plus bevacizumab in the treatment of patients with metastatic colorectal cancer (TRIBE2): a multicentre, open-label, phase 3, randomised, controlled trial. Lancet Oncol (2020) 21:497–507. doi: 10.1016/S1470-2045(19)30862-9
64. Richman S, Adlard J. Left and right sided large bowel cancer : Have significant genetic differences in addition to well known clinical differences. BMJ : Br Med J (2002) 324:931. doi: 10.1136/BMJ.324.7343.931
65. Zhong M, Xiong Y, Ye Z, Zhao J, Zhong L, Liu Y, et al. Microbial community profiling distinguishes left-sided and right-sided colon cancer. Front Cell Infect Microbiol (2020) 10:498502. doi: 10.3389/fcimb.2020.498502
66. Domingo E, Ramamoorthy R, Oukrif D, Rosmarin D, Presz M, Wang H, et al. Use of multivariate analysis to suggest a new molecular classification of colorectal cancer. J Pathol (2013) 229:441–8. doi: 10.1002/PATH.4139
67. Arnold D, Lueza B, Douillard J-Y, Peeters M, Lenz H-J, Venook A, et al. Prognostic and predictive value of primary tumour side in patients with RAS wild-type metastatic colorectal cancer treated with chemotherapy and EGFR directed antibodies in six randomized trials. Ann Oncol (2017) 28:1713–29. doi: 10.1093/annonc/mdx175
68. Sanz-Pamplona R, Cordero D, Berenguer A, Lejbkowicz F, Rennert H, Salazar R, et al. Gene expression differences between colon and rectum tumors. Clin Cancer Res (2011) 17:7303–12. doi: 10.1158/1078-0432.CCR-11-1570
69. Yoshino T, Watanabe J, Shitara K, Yasui H, Ohori H, Shiozawa M, et al. Panitumumab (PAN) plus mFOLFOX6 versus bevacizumab (BEV) plus mFOLFOX6 as first-line treatment in patients with RAS wild-type (WT) metastatic colorectal cancer (mCRC): Results from the phase 3 PARADIGM trial. J Clin Oncol (2022) 40:LBA1–1. doi: 10.1200/JCO.2022.40.17_suppl.LBA1
70. Hecht JR, Mitchell E, Chidiac T, Scroggin C, Hagenstad C, Spigel D, et al. A randomized phase IIIB trial of chemotherapy, bevacizumab, and panitumumab compared with chemotherapy and bevacizumab alone for metastatic colorectal cancer. J Clin Oncol (2009) 27:672–80. doi: 10.1200/JCO.2008.19.8135
71. Tol J, Koopman M, Cats A, Rodenburg CJ, Creemers GJM, Schrama JG, et al. Chemotherapy, bevacizumab, and cetuximab in metastatic colorectal cancer. New Engl J Med (2009) 360:563–72. doi: 10.1056/NEJMoa0808268
72. Simkens LHJ, Van Tinteren H, May A, Ten Tije AJ, Creemers GJM, Loosveld OJL, et al. Maintenance treatment with capecitabine and bevacizumab in metastatic colorectal cancer (CAIRO3): a phase 3 randomised controlled trial of the Dutch colorectal cancer group. Lancet (2015) 385:1843–52. doi: 10.1016/S0140-6736(14)62004-3
73. Hegewisch-Becker S, Graeven U, Lerchenmüller CA, Killing B, Depenbusch R, Steffens C-C, et al. Maintenance strategies after first-line oxaliplatin plus fluoropyrimidine plus bevacizumab for patients with metastatic colorectal cancer (AIO 0207): a randomised, non-inferiority, open-label, phase 3 trial. Lancet Oncol (2015) 16:1355–69. doi: 10.1016/S1470-2045(15)00042-X
74. Holash J, Davis S, Papadopoulos N, Croll SD, Ho L, Russell M, et al. VEGF-trap: A VEGF blocker with potent antitumor effects. Proc Natl Acad Sci (2002) 99:11393–8. doi: 10.1073/pnas.172398299
75. Wilhelm SM, Dumas J, Adnane L, Lynch M, Carter CA, Schütz G, et al. Regorafenib (BAY 73-4506): A new oral multikinase inhibitor of angiogenic, stromal and oncogenic receptor tyrosine kinases with potent preclinical antitumor activity. Int J Cancer (2011) 129:245–55. doi: 10.1002/ijc.25864
76. Grothey A, Van Cutsem E, Sobrero A, Siena S, Falcone A, Ychou M, et al. Regorafenib monotherapy for previously treated metastatic colorectal cancer (CORRECT): an international, multicentre, randomised, placebo-controlled, phase 3 trial. Lancet (2013) 381:303–12. doi: 10.1016/S0140-6736(12)61900-X
77. Mayer RJ, van Cutsem E, Falcone A, Yoshino T, Garcia-Carbonero R, Mizunuma N, et al. Randomized trial of TAS-102 for refractory metastatic colorectal cancer. New Engl J Med (2015) 372:1909–19. doi: 10.1056/NEJMoa1414325
78. Pfeiffer P, Yilmaz M, Möller S, Zitnjak D, Krogh M, Petersen LN, et al. TAS-102 with or without bevacizumab in patients with chemorefractory metastatic colorectal cancer: an investigator-initiated, open-label, randomised, phase 2 trial. Lancet Oncol (2020) 21:412–20. doi: 10.1016/S1470-2045(19)30827-7
79. Cidon EU, Alonso P, Masters B. Markers of response to antiangiogenic therapies in colorectal cancer: Where are we now and what should be next? Clin Med Insights Oncol (2016) 10:41. doi: 10.4137/CMO.S34542
80. Rodriguez-Pascual J, Cubillo A. Dynamic biomarkers of response to antiangiogenic therapies in colorectal cancer: A review. Curr Pharmacogenomics Person Med (2017) 15:81–5. doi: 10.2174/1875692115666170815161754
81. Mir O, Coriat R, Cabanes L, Ropert S, Billemont B, Alexandre J, et al. An observational study of bevacizumab-induced hypertension as a clinical biomarker of antitumor activity. Oncologist (2011) 16:1325–32. doi: 10.1634/theoncologist.2010-0002
82. de la Pinta C, Castillo ME, Collado M, Galindo-Pumariño C, Peña C. Radiogenomics: Hunting down liver metastasis in colorectal cancer patients. Cancers (Basel) (2021) 13:5547. doi: 10.3390/cancers13215547
83. van Cutsem E, Paccard C, Chiron M, Tabernero J. Impact of prior bevacizumab treatment on VEGF-a and PlGF levels and outcome following second-line aflibercept treatment: Biomarker Post hoc analysis of the VELOUR trial. Clin Cancer Res (2020) 26:717–25. doi: 10.1158/1078-0432.CCR-19-1985
84. Parikh AR, Lee F-C, Yau L, Koh H, Knost J, Mitchell EP, et al. MAVERICC, a randomized, biomarker-stratified, phase II study of mFOLFOX6-bevacizumab versus FOLFIRI-bevacizumab as first-line chemotherapy in metastatic colorectal cancer. Clin Cancer Res (2019) 25:2988–95. doi: 10.1158/1078-0432.CCR-18-1221
85. Poon RT-P, Fan S-T, Wong J. Clinical implications of circulating angiogenic factors in cancer patients. J Clin Oncol (2001) 19:1207–25. doi: 10.1200/JCO.2001.19.4.1207
86. Jubb AM, Hurwitz HI, Bai W, Holmgren EB, Tobin P, Guerrero AS, et al. Impact of vascular endothelial growth factor-a expression, thrombospondin-2 expression, and microvessel density on the treatment effect of bevacizumab in metastatic colorectal cancer. J Clin Oncol (2006) 24:217–27. doi: 10.1200/JCO.2005.01.5388
87. Latacz E, Höppener D, Bohlok A, Leduc S, Tabariès S, Fernández Moro C, et al. Histopathological growth patterns of liver metastasis: updated consensus guidelines for pattern scoring, perspectives and recent mechanistic insights. Br J Cancer (2022) 127:988–1013. doi: 10.1038/s41416-022-01859-7
88. Kuczynski EA, Reynolds AR. Vessel co-option and resistance to anti-angiogenic therapy. Angiogenesis (2020) 23:55–74. doi: 10.1007/s10456-019-09698-6
89. Li J, Cao J, Xu R, Chen Z, Zhang J, Ji D, et al. A phase 1b study of VEGFR inhibitor fruquintinib in patients with pretreated advanced colorectal cancer. J Clin Oncol (2014) 32:3548–8. doi: 10.1200/jco.2014.32.15_suppl.3548
90. Li J, Qin S, Xu R-H, Shen L, Xu J, Bai Y, et al. Effect of fruquintinib vs placebo on overall survival in patients with previously treated metastatic colorectal cancer. JAMA (2018) 319:2486. doi: 10.1001/jama.2018.7855
91. O’Neil BH, Wallmark JM, Lorente D, Elez E, Raimbourg J, Gomez-Roca C, et al. Safety and antitumor activity of the anti–PD-1 antibody pembrolizumab in patients with advanced colorectal carcinoma. PloS One (2017) 12. doi: 10.1371/JOURNAL.PONE.0189848
92. Le DT, Kim TW, van Cutsem E, Geva R, Jäger D, Hara H, et al. Phase II open-label study of pembrolizumab in treatment-refractory, microsatellite instability–High/Mismatch repair–deficient metastatic colorectal cancer: KEYNOTE-164. J Clin Oncol (2020) 38:11–9. doi: 10.1200/JCO.19.02107
93. Marabelle A, Le DT, Ascierto PA, di Giacomo AM, de Jesus-Acosta A, Delord J-P, et al. Efficacy of pembrolizumab in patients with noncolorectal high microsatellite Instability/Mismatch repair–deficient cancer: Results from the phase II KEYNOTE-158 study. J Clin Oncol (2019) 38:1–10. doi: 10.1200/JCO.19.02105
94. Le DT, Uram JN, Wang H, Bartlett BR, Kemberling H, Eyring AD, et al. PD-1 blockade in tumors with mismatch-repair deficiency. New Engl J Med (2015) 372:2509–20. doi: 10.1056/NEJMoa1500596
95. Limagne E, Euvrard R, Thibaudin M, Rébé C, Derangère V, Chevriaux A, et al. Accumulation of MDSC and Th17 cells in patients with metastatic colorectal cancer predicts the efficacy of a FOLFOX-bevacizumab drug treatment regimen. Cancer Res (2016) 76:5241–52. doi: 10.1158/0008-5472.CAN-15-3164
96. Terme M, Pernot S, Marcheteau E, Sandoval F, Benhamouda N, Colussi O, et al. VEGFA-VEGFR pathway blockade inhibits tumor-induced regulatory T-cell proliferation in colorectal cancer. Cancer Res (2013) 73:539–49. doi: 10.1158/0008-5472.CAN-12-2325
97. Osada T, Chong G, Tansik R, Hong T, Spector N, Kumar R, et al. The effect of anti-VEGF therapy on immature myeloid cell and dendritic cells in cancer patients. Cancer Immunol. Immunother. (2008) 57:1115–24. doi: 10.1007/s00262-007-0441-x
98. Kato Y, Tabata K, Kimura T, Yachie-Kinoshita A, Ozawa Y, Yamada K, et al. Lenvatinib plus anti-PD-1 antibody combination treatment activates CD8+ T cells through reduction of tumor-associated macrophage and activation of the interferon pathway. PloS One (2019) 14:e0212513. doi: 10.1371/journal.pone.0212513
99. Gomez-Roca C, Yanez E, Im S-A, Castanon Alvarez E, Senellart H, Doherty M, et al. LEAP-005: A phase II multicohort study of lenvatinib plus pembrolizumab in patients with previously treated selected solid tumors–results from the colorectal cancer cohort. J Clin Oncol (2021) 39:94–4. doi: 10.1200/JCO.2021.39.3_suppl.94
100. Llovet JM, Kelley RK, Villanueva A, Singal AG, Pikarsky E, Roayaie S, et al. Hepatocellular carcinoma. Nat Rev Dis Primers (2021) 7:6. doi: 10.1038/s41572-020-00240-3
101. Llovet JM, Castet F, Heikenwalder M, Maini MK, Mazzaferro V, Pinato DJ, et al. Immunotherapies for hepatocellular carcinoma. Nat Rev Clin Oncol (2021) 19(3):151–72. doi: 10.1038/s41571-021-00573-2
102. Castet F, Willoughby CE, Haber PK, Llovet JM. Atezolizumab plus bevacizumab: A novel breakthrough in hepatocellular carcinoma. Clin Cancer Res (2021) 27:1827–9. doi: 10.1158/1078-0432.CCR-20-4706
103. Llovet JM, de Baere T, Kulik L, PhilippKK H, TF G, Meyer T, et al. Locoregional therapies in the era of molecular and immune treatments for hepatocellular carcinoma. Nat Rev Gastroenterol Hepatol (2021) 18:293–313. doi: 10.1038/s41575-020-00395-0
104. Llovet JM, Ricci S, Mazzaferro V, Hilgard P, Gane E, Blanc JF, et al. Sorafenib in advanced hepatocellular carcinoma. New Engl J Med (2008) 359:378–90. doi: 10.1056/NEJMoa0708857
105. Cheng AL, Kang YK, Chen Z, Tsao CJ, Qin S, Kim JS, et al. Efficacy and safety of sorafenib in patients in the Asia-pacific region with advanced hepatocellular carcinoma: a phase III randomised, double-blind, placebo-controlled trial. Lancet Oncol (2009) 10:25–34. doi: 10.1016/S1470-2045(08)70285-7
106. Cheng AL, Kang YK, Lin DY, Park JW, Kudo M, Qin S, et al. Sunitinib versus sorafenib in advanced hepatocellular cancer: Results of a randomized phase III trial. J Clin Oncol (2013) 31:4067–75. doi: 10.1200/JCO.2012.45.8372
107. Cainap C, Qin S, Huang WT, Chung IJ, Pan H, Cheng Y, et al. Linifanib versus sorafenib in patients with advanced hepatocellular carcinoma: Results of a randomized phase III trial. J Clin Oncol (2015) 33:172–9. doi: 10.1200/JCO.2013.54.3298
108. Zhu AX, Rosmorduc O, Evans TRJ, Ross PJ, Santoro A, Carrilho FJ, et al. Randomized, double-blind, placebo-controlled trial of sorafenib plus erlotinib in patients with advanced hepatocellular carcinoma. J Clin Oncol (2015) 33:559–66. doi: 10.1200/JCO.2013.53.7746
109. Johnson PJ, Qin S, Park JW, Poon RTP, Raoul JL, Philip PA, et al. Brivanib versus sorafenib as first-line therapy in patients with unresectable, advanced hepatocellular carcinoma: Results from the randomized phase III BRISK-FL study. J Clin Oncol (2013) 31:3517–24. doi: 10.1200/JCO.2012.48.4410
110. Kudo M, Finn RS, Qin S, Han KH, Ikeda K, Piscaglia F, et al. Lenvatinib versus sorafenib in first-line treatment of patients with unresectable hepatocellular carcinoma: a randomised phase 3 non-inferiority trial. Lancet (2018) 391:1163–73. doi: 10.1016/S0140-6736(18)30207-1
111. Llovet JM, Lencioni R. mRECIST for HCC: Performance and novel refinements. J Hepatol (2020) 72:288–306. doi: 10.1016/j.jhep.2019.09.026
112. Reig M, Bruix J. Lenvatinib: can a non-inferiority trial change clinical practice? Lancet (2018) 391:1123–4. doi: 10.1016/S0140-6736(18)30208-3
113. Singal AG, Nagar SP, Hitchens A, Davis KL, Iyer S. Real-world effectiveness of lenvatinib monotherapy among unresectable hepatocellular carcinoma patients in the USA. Future Oncol (2021) 17:2759–68. doi: 10.2217/fon-2021-0242
114. Finn RS, Qin S, Ikeda M, Galle PR, Ducreux M, Kim T-Y, et al. Atezolizumab plus bevacizumab in unresectable hepatocellular carcinoma. New Engl J Med (2020) 382:1894–905. doi: 10.1056/NEJMoa1915745
115. Casak SJ, Donoghue M, Fashoyin-Aje L, Jiang X, Rodriguez L, Shen Y-L, et al. FDA Approval summary: Atezolizumab plus bevacizumab for the treatment of patients with advanced unresectable or metastatic hepatocellular carcinoma. Clin Cancer Res (2021) 27(7):1836–41. doi: 10.1158/1078-0432.CCR-20-3407
116. Cheng A-L, Qin S, Ikeda M, Galle PR, Ducreux M, Kim T-Y, et al. Updated efficacy and safety data from IMbrave150: Atezolizumab plus bevacizumab vs Sorafenib for unresectable hepatocellular carcinoma. J Hepatol (2022) 76:862–73. doi: 10.1016/j.jhep.2021.11.030
117. Siegel AB, Cohen EI, Ocean A, Lehrer D, Goldenberg A, Knox JJ, et al. Phase II trial evaluating the clinical and biologic effects of bevacizumab in unresectable hepatocellular carcinoma. J Clin Oncol (2008) 26:2992–8. doi: 10.1200/JCO.2007.15.9947
118. Boige V, Malka D, Bourredjem A, Dromain C, Baey C, Jacques N, et al. Efficacy, safety, and biomarkers of single-agent bevacizumab therapy in patients with advanced hepatocellular carcinoma. Oncologist (2012) 17:1063–72. doi: 10.1634/theoncologist.2011-0465
119. Ren Z, Xu J, Bai Y, Xu A, Cang S, Du C, et al. Sintilimab plus a bevacizumab biosimilar (IBI305) versus sorafenib in unresectable hepatocellular carcinoma (ORIENT-32): a randomised, open-label, phase 2–3 study. Lancet Oncol (2021) 22:977–90. doi: 10.1016/S1470-2045(21)00252-7
120. Kelley RK, Rimassa L, Cheng A-L, Kaseb A, Qin S, Zhu AX, et al. Cabozantinib plus atezolizumab versus sorafenib for advanced hepatocellular carcinoma (COSMIC-312): a multicentre, open-label, randomised, phase 3 trial. Lancet Oncol (2022) 23:995–1008. doi: 10.1016/S1470-2045(22)00326-6
121. Finn R, Kudo M, Merle P, Meyer T, Qin S, Ikeda M, et al. LBA34 - primary results from the phase III LEAP-002 study: Lenvatinib plus pembrolizumab versus lenvatinib as first-line (1L) therapy for advanced hepatocellular carcinoma (aHCC). ESMO Congress (2022), 33(suppl_7):S808–69. doi: 10.1016/j.annonc.2022.08.031
122. Qin S, Chan L, Gu S, Bai Y, Ren Z, Lin X, et al. LBA35 - camrelizumab (C) plus rivoceranib (R) vs. sorafenib (S) as first-line therapy for unresectable hepatocellular carcinoma (uHCC): A randomized, phase III trial. ESMO Congress. (2022), 33 (suppl_7): S808–69.
123. Qin S, Bi F, Gu S, Bai Y, Chen Z, Wang Z, et al. Donafenib versus sorafenib in first-line treatment of unresectable or metastatic hepatocellular carcinoma: A randomized, open-label, parallel-controlled phase II-III trial. J Clin Oncol (2021) 39(27):3002–3011. doi: 10.1200/JCO.21.00163
124. Bruix J, Qin S, Merle P, Granito A, Huang YH, Bodoky G, et al. Regorafenib for patients with hepatocellular carcinoma who progressed on sorafenib treatment (RESORCE): a randomised, double-blind, placebo-controlled, phase 3 trial. Lancet (2017) 389:56–66. doi: 10.1016/S0140-6736(16)32453-9
125. Abou-Alfa GK, Meyer T, Cheng AL, El-Khoueiry AB, Rimassa L, Ryoo BY, et al. Cabozantinib in patients with advanced and progressing hepatocellular carcinoma. New Engl J Med (2018) 379:54–63. doi: 10.1056/NEJMoa1717002
126. Zhu AX, Kang YK, Yen CJ, Finn RS, Galle PR, Llovet JM, et al. Ramucirumab after sorafenib in patients with advanced hepatocellular carcinoma and increased α-fetoprotein concentrations (REACH-2): a randomised, double-blind, placebo-controlled, phase 3 trial. Lancet Oncol (2019) 20:282–96. doi: 10.1016/S1470-2045(18)30937-9
127. Zhu AX, Park JO, Ryoo BY, Yen CJ, Poon R, Pastorelli D, et al. Ramucirumab versus placebo as second-line treatment in patients with advanced hepatocellular carcinoma following first-line therapy with sorafenib (REACH): A randomised, double-blind, multicentre, phase 3 trial. Lancet Oncol (2015) 16:859–70. doi: 10.1016/S1470-2045(15)00050-9
128. Qin S, Li Q, Gu S, Chen X, Lin L, Wang Z, et al. Apatinib as second-line or later therapy in patients with advanced hepatocellular carcinoma (AHELP): a multicentre, double-blind, randomised, placebo-controlled, phase 3 trial. Lancet Gastroenterol Hepatol (2021) 6:559–68. doi: 10.1016/S2468-1253(21)00109-6
129. Bruix J, Takayama T, Mazzaferro V, Chau G-YY, Yang J, Kudo M, et al. Adjuvant sorafenib for hepatocellular carcinoma after resection or ablation (STORM): A phase 3, randomised, double-blind, placebo-controlled trial. Lancet Oncol (2015) 16:1344–54. doi: 10.1016/S1470-2045(15)00198-9
130. Haber PK, Puigvehí M, Castet F, Lourdusamy V, Montal R, Tabrizian P, et al. Evidence-based management of hepatocellular carcinoma: Systematic review and meta-analysis of randomized controlled trials (2002–2020). Gastroenterology (2021) 161:879–98. doi: 10.1053/j.gastro.2021.06.008
131. Kudo M, Imanaka K, Chida N, Nakachi K, Tak WY, Takayama T, et al. Phase III study of sorafenib after transarterial chemoembolisation in Japanese and Korean patients with unresectable hepatocellular carcinoma. Eur J Cancer (2011) 47:2117–27. doi: 10.1016/j.ejca.2011.05.007
132. Meyer T, Fox R, Ma YT, Ross PJ, James MW, Sturgess R, et al. Sorafenib in combination with transarterial chemoembolisation in patients with unresectable hepatocellular carcinoma (TACE 2): a randomised placebo-controlled, double-blind, phase 3 trial. Lancet Gastroenterol Hepatol (2017) 2:565–75. doi: 10.1016/S2468-1253(17)30156-5
133. Kudo M, Han G, Finn RS, Poon RTP, Blanc JF, Yan L, et al. Brivanib as adjuvant therapy to transarterial chemoembolization in patients with hepatocellular carcinoma: A randomized phase III trial. Hepatology (2014) 60:1697–707. doi: 10.1002/hep.27290
134. Kudo M, Cheng A-LL, Park J-W, Park J-H, Liang P-CC, Hidaka H, et al. Orantinib versus placebo combined with transcatheter arterial chemoembolisation in patients with unresectable hepatocellular carcinoma (ORIENTAL): a randomised, double-blind, placebo-controlled, multicentre, phase 3 study. Lancet Gastroenterol Hepatol (2018) 3:37–46. doi: 10.1016/S2468-1253(17)30290-X
135. Kudo M, Ueshima K, Ikeda M, Torimura T, Tanabe N, Aikata H, et al. Randomised, multicentre prospective trial of transarterial chemoembolisation (TACE) plus sorafenib as compared with TACE alone in patients with hepatocellular carcinoma: TACTICS trial. Gut (2020) 69:1492–501. doi: 10.1136/gutjnl-2019-318934
136. Kudo M, Ueshima K, Ikeda M, Torimura T, Aikata H, Izumi N, et al. TACTICS: Final overall survival (OS) data from a randomized, open label, multicenter, phase II trial of transcatheter arterial chemoembolization (TACE) therapy in combination with sorafenib as compared with TACE alone in patients (pts) with hepatocellular carcinoma (HCC). J Clin Oncol (2021) 39:270–0. doi: 10.1200/JCO.2021.39.3_suppl.270
137. Llovet JM, Peña CEA, Lathia CD, Shan M, Meinhardt G, Bruix J. Plasma biomarkers as predictors of outcome in patients with advanced hepatocellular carcinoma. Clin Cancer Res (2012) 18:2290–300. doi: 10.1158/1078-0432.CCR-11-2175
138. Pinyol R, Montal R, Bassaganyas L, Sia D, Takayama T, Chau G-YY, et al. Molecular predictors of prevention of recurrence in HCC with sorafenib as adjuvant treatment and prognostic factors in the phase 3 STORM trial. Gut (2019) 68:1065–75. doi: 10.1136/gutjnl-2018-316408
139. Lee Y-S, Kim BH, Kim BC, Shin A, Kim JS, Hong S-H, et al. SLC15A2 genomic variation is associated with the extraordinary response of sorafenib treatment: whole-genome analysis in patients with hepatocellular carcinoma. Oncotarget (2015) 6:16449–60. doi: 10.18632/oncotarget.3758
140. Geier A, Macias RIR, Bettinger D, Weiss J, Bantel H, Jahn D, et al. The lack of the organic cation transporter OCT1 at the plasma membrane of tumor cells precludes a positive response to sorafenib in patients with hepatocellular carcinoma. Oncotarget (2017) 8:15846–57. doi: 10.18632/oncotarget.15029
141. Teufel M, Seidel H, Köchert K, Meinhardt G, Finn RS, Llovet JM, et al. Biomarkers associated with response to regorafenib in patients with hepatocellular carcinoma. Gastroenterology (2019) 156:1731–41. doi: 10.1053/j.gastro.2019.01.261
142. Finn RS, Kudo M, Cheng A-L, Wyrwicz L, Ngan RKC, Blanc J-F, et al. Pharmacodynamic biomarkers predictive of survival benefit with lenvatinib in unresectable hepatocellular carcinoma: From the phase III REFLECT study. Clin Cancer Res (2021) 27:4848–58. doi: 10.1158/1078-0432.CCR-20-4219
143. Rimassa L, Kelley RK, Meyer T, Ryoo B-Y, Merle P, Park J-W, et al. Outcomes based on plasma biomarkers for the phase 3 CELESTIAL trial of cabozantinib versus placebo in advanced hepatocellular carcinoma. Liver Cancer (2022) 11:38–47. doi: 10.1159/000519867
144. Lee MS, Ryoo B-YB-Y, Hsu C-HC-H, Numata K, Stein S, Verret W, et al. Atezolizumab with or without bevacizumab in unresectable hepatocellular carcinoma (GO30140): an open-label, multicentre, phase 1b study. Lancet Oncol (2020) 21:808–20. doi: 10.1016/S1470-2045(20)30156-X
145. Zhu AX, Abbas AR, de Galarreta MR, Guan Y, Lu S, Koeppen H, et al. Molecular correlates of clinical response and resistance to atezolizumab in combination with bevacizumab in advanced hepatocellular carcinoma. Nat Med (2022) 28(8):1599–611. doi: 10.1038/s41591-022-01868-2
146. Ho WJ, Zhu Q, Durham J, Popovic A, Xavier S, Leatherman J, et al. Neoadjuvant cabozantinib and nivolumab convert locally advanced hepatocellular carcinoma into resectable disease with enhanced antitumor immunity. Nat Cancer (2021) 2(9):891–903. doi: 10.1038/s43018-021-00234-4
147. Finn RS, Ikeda M, Zhu AX, Sung MW, Baron AD, Kudo M, et al. Phase ib study of lenvatinib plus pembrolizumab in patients with unresectable hepatocellular carcinoma. J Clin Oncol (2020) 38:2960–70. doi: 10.1200/JCO.20.00808
148. Lord RVN, Park JM, Wickramasinghe K, DeMeester SR, Oberg S, Salonga D, et al. Vascular endothelial growth factor and basic fibroblast growth factor expression in esophageal adenocarcinoma and Barrett esophagus. J Thorac Cardiovasc Surg (2003) 125:246–53. doi: 10.1067/mtc.2003.203
149. Yoshikawa T, Tsuburaya A, Kobayashi O, Sairenji M, Motohashi H, Yanoma S, et al. Plasma concentrations of VEGF and bFGF in patients with gastric carcinoma. Cancer Lett (2000) 153:7–12. doi: 10.1016/S0304-3835(99)00426-7
150. Schmitt JM, Sommers SR, Fisher W, Ansari R, Robin E, Koneru K, et al. Sunitinib plus paclitaxel in patients with advanced esophageal cancer: A phase II study from the Hoosier oncology groupe. J Thorac Oncol (2012) 7:760–3. doi: 10.1097/JTO.0b013e31824abc7c
151. Moehler M, Gepfner-Tuma I, Maderer A, Thuss-Patience PC, Ruessel J, Hegewisch-Becker S, et al. Sunitinib added to FOLFIRI versus FOLFIRI in patients with chemorefractory advanced adenocarcinoma of the stomach or lower esophagus: a randomized, placebo-controlled phase II AIO trial with serum biomarker program. BMC Cancer (2016) 16:699. doi: 10.1186/s12885-016-2736-9
152. Sun W, Powell M, O’Dwyer PJ, Catalano P, Ansari RH, Benson AB. Phase II study of sorafenib in combination with docetaxel and cisplatin in the treatment of metastatic or advanced gastric and gastroesophageal junction adenocarcinoma: ECOG 5203. J Clin Oncol (2010) 28:2947–51. doi: 10.1200/JCO.2009.27.7988
153. Liu G, Wang Y, Wang C, He Y, E M. Clinical efficacy and safety of apatinib as maintenance treatment in patients with advanced esophageal squamous cell carcinoma. Expert Rev Clin Pharmacol (2020) 13:1423–30. doi: 10.1080/17512433.2020.1844004
154. Huang J, Xiao J, Fang W, Lu P, Fan Q, Shu Y, et al. Anlotinib for previously treated advanced or metastatic esophageal squamous cell carcinoma: A double-blind randomized phase 2 trial. Cancer Med (2021) 10:1681–9. doi: 10.1002/cam4.3771
155. Bang Y-J, Golan T, Dahan L, Fu S, Moreno V, Park K, et al. Ramucirumab and durvalumab for previously treated, advanced non–small-cell lung cancer, gastric/gastro-oesophageal junction adenocarcinoma, or hepatocellular carcinoma: An open-label, phase ia/b study (JVDJ). Eur J Cancer (2020) 137:272–84. doi: 10.1016/j.ejca.2020.06.007
156. Shah MA, Jhawer M, Ilson DH, Lefkowitz RA, Robinson E, Capanu M, et al. Phase II study of modified docetaxel, cisplatin, and fluorouracil with bevacizumab in patients with metastatic gastroesophageal adenocarcinoma. J Clin Oncol (2011) 29:868–74. doi: 10.1200/JCO.2010.32.0770
157. Uronis HE, Bendell JC, Altomare I, Blobe GC, Hsu SD, Morse MA, et al. A phase II study of capecitabine, oxaliplatin, and bevacizumab in the treatment of metastatic esophagogastric adenocarcinomas. Oncologist (2013) 18:271–2. doi: 10.1634/theoncologist.2012-0404
158. Ohtsu A, Shah MA, Van Cutsem E, Rha SY, Sawaki A, Park SR, et al. Bevacizumab in combination with chemotherapy as first-line therapy in advanced gastric cancer: a randomized, double-blind, placebo-controlled phase III study. J Clin Oncol (2011) 29:3968–76. doi: 10.1200/JCO.2011.36.2236
159. Ohtsu A, Shah MA, van Cutsem E, Rha SY, Sawaki A, Park SR, et al. Bevacizumab in combination with chemotherapy as first-line therapy in advanced gastric cancer: A randomized, double-blind, placebo-controlled phase III study. J Clin Oncol (2011) 29:3968–76. doi: 10.1200/JCO.2011.36.2236
160. Fuchs CS, Tomasek J, Yong CJ, Dumitru F, Passalacqua R, Goswami C, et al. Ramucirumab monotherapy for previously treated advanced gastric or gastro-oesophageal junction adenocarcinoma (REGARD): an international, randomised, multicentre, placebo-controlled, phase 3 trial. Lancet (2014) 383:31–9. doi: 10.1016/S0140-6736(13)61719-5
161. Wilke H, Muro K, van Cutsem E, Oh S-C, Bodoky G, Shimada Y, et al. Ramucirumab plus paclitaxel versus placebo plus paclitaxel in patients with previously treated advanced gastric or gastro-oesophageal junction adenocarcinoma (RAINBOW): a double-blind, randomised phase 3 trial. Lancet Oncol (2014) 15:1224–35. doi: 10.1016/S1470-2045(14)70420-6
162. Fuchs CS, Shitara K, di Bartolomeo M, Lonardi S, Al-Batran S-E, van Cutsem E, et al. Ramucirumab with cisplatin and fluoropyrimidine as first-line therapy in patients with metastatic gastric or junctional adenocarcinoma (RAINFALL): a double-blind, randomised, placebo-controlled, phase 3 trial. Lancet Oncol (2019) 20:420–35. doi: 10.1016/S1470-2045(18)30791-5
163. Chau I, Penel N, Arkenau H-T, Santana-Davila R, Calvo E, Soriano AO, et al. Safety and antitumor activity of ramucirumab plus pembrolizumab in treatment naïve advanced gastric or gastroesophageal junction (G/GEJ) adenocarcinoma: Preliminary results from a multi-disease phase I study (JVDF). J Clin Oncol (2018) 36:101–1. doi: 10.1200/JCO.2018.36.4_suppl.101
164. Bang Y-J, Golan T, Lin C-C, Kang Y-K, Wainberg ZA, Wasserstrom H, et al. Interim safety and clinical activity in patients (pts) with locally advanced and unresectable or metastatic gastric or gastroesophageal junction (G/GEJ) adenocarcinoma from a multicohort phase I study of ramucirumab (R) plus durvalumab (D). J Clin Oncol (2018) 36:92–2. doi: 10.1200/JCO.2018.36.4_suppl.92
165. Takahari D, Shoji H, Hara H, Esaki T, Machida N, Nagashima K, et al. Preliminary result of phase 1/2 study of ramucirumab plus nivolumab in patients with previously treated advanced gastric adenocarcinoma (NivoRam study). J Clin Oncol (2018) 36:4047–7. doi: 10.1200/JCO.2018.36.15_suppl.4047
166. Li J, Qin S, Xu J, Xiong J, Wu C, Bai Y, et al. Randomized, double-blind, placebo-controlled phase III trial of apatinib in patients with chemotherapy-refractory advanced or metastatic adenocarcinoma of the stomach or gastroesophageal junction. J Clin Oncol (2016) 34:1448–54. doi: 10.1200/JCO.2015.63.5995
167. Xu R, Zhang D, Shen L, Lin J, Huang J, Gong J, et al. A phase I/II trial of fruquintinib in combination with paclitaxel for second-line treatment in patients with advanced gastric cancer. J Clin Oncol (2017) 35:128–8. doi: 10.1200/JCO.2017.35.4_SUPPL.128
168. Enzinger PC, McCleary NJ, Zheng H, Abrams TA, Yurgelun MB, Azzoli CG, et al. Multicenter double-blind randomized phase II: FOLFOX + ziv-aflibercept/placebo for patients (pts) with chemo-naive metastatic esophagogastric adenocarcinoma (MEGA). J Clin Oncol (2016) 34:4–4. doi: 10.1200/jco.2016.34.4_suppl.4
169. Kang Y, Lee KH, Shen L, Yeh K, Hong YS, Park YI, et al. Randomized phase ii study of capecitabine and cisplatin with or without sorafenib in patients with metastatic gastric cancer: Stargate study. Ann Oncol (2014) 25:iv210. doi: 10.1093/annonc/mdu334.1
170. Thuss-Patience PC, Al-Batran S-E, Siveke JT, Homann N, Malfertheiner P, Glaeser D, et al. Pazopanib and 5-FU/oxaliplatin as first-line treatment in advanced gastric cancer: PaFLO, a randomized phase II study from the AIO (Arbeitsgemeinschaft internistische onkologie). J Clin Oncol (2015) 33:4033–3. doi: 10.1200/jco.2015.33.15_suppl.4033
171. Xu R, Zhang D, Shen L, Lin J, Huang J, Gong J, et al. A phase I/II trial of fruquintinib in combination with paclitaxel for second-line treatment in patients with advanced gastric cancer. J Clin Oncol (2017) 35:128–8. doi: 10.1200/JCO.2017.35.4_suppl.128
172. Dasari A, Shen C, Halperin D, Zhao B, Zhou S, Xu Y, et al. Trends in the incidence, prevalence, and survival outcomes in patients with neuroendocrine tumors in the united states. JAMA Oncol (2017) 3:1335. doi: 10.1001/jamaoncol.2017.0589
173. Gomez D, Malik HZ, Al-Mukthar A, Menon KV, Toogood GJ, Lodge JPA, et al. Hepatic resection for metastatic gastrointestinal and pancreatic neuroendocrine tumours: outcome and prognostic predictors. HPB (2007) 9:345–51. doi: 10.1080/13651820701504199
174. Raymond E, Dahan L, Raoul J-L, Bang Y-J, Borbath I, Lombard-Bohas C, et al. Sunitinib malate for the treatment of pancreatic neuroendocrine tumors. New Engl J Med (2011) 364:501–13. doi: 10.1056/NEJMoa1003825
175. Faivre S, Niccoli P, Castellano D, Valle JW, Hammel P, Raoul J-L, et al. Sunitinib in pancreatic neuroendocrine tumors: updated progression-free survival and final overall survival from a phase III randomized study. Ann Oncol (2017) 28:339–43. doi: 10.1093/annonc/mdw561
176. Xu J, Shen L, Bai C, Wang W, Li J, Yu X, et al. Surufatinib in advanced pancreatic neuroendocrine tumours (SANET-p): a randomised, double-blind, placebo-controlled, phase 3 study. Lancet Oncol (2020) 21:1489–99. doi: 10.1016/S1470-2045(20)30493-9
177. Chan JA, Faris JE, Murphy JE, Blaszkowsky LS, Kwak EL, McCleary NJ, et al. Phase II trial of cabozantinib in patients with carcinoid and pancreatic neuroendocrine tumors (pNET). J Clin Oncol (2017) 35:228–8. doi: 10.1200/JCO.2017.35.4_SUPPL.228
178. Phan AT, Halperin DM, Chan JA, Fogelman DR, Hess KR, Malinowski P, et al. Pazopanib and depot octreotide in advanced, well-differentiated neuroendocrine tumours: a multicentre, single-group, phase 2 study. Lancet Oncol (2015) 16:695–703. doi: 10.1016/S1470-2045(15)70136-1
179. Grande E, Capdevila J, Castellano D, Teulé A, Durán I, Fuster J, et al. Pazopanib in pretreated advanced neuroendocrine tumors: a phase II, open-label trial of the Spanish task force group for neuroendocrine tumors (GETNE). Ann Oncol (2015) 26:1987–93. doi: 10.1093/annonc/mdv252
180. Strosberg JR, Cives M, Hwang J, Weber T, Nickerson M, Atreya CE, et al. A phase II study of axitinib in advanced neuroendocrine tumors. Endocr Relat Cancer (2016) 23:411–8. doi: 10.1530/ERC-16-0008
181. Capdevila J, Fazio N, Lopez Lopez C, Teule A, Valle JW, Tafuto S, et al. Final results of the TALENT trial (GETNE1509): a prospective multicohort phase II study of lenvatinib in patients (pts) with G1/G2 advanced pancreatic (panNETs) and gastrointestinal (giNETs) neuroendocrine tumors (NETs). J Clin Oncol (2019) 37:4106–6. doi: 10.1200/JCO.2019.37.15_suppl.4106
182. Park W, Chawla A, O’Reilly EM. Pancreatic cancer. JAMA (2021) 326:851. doi: 10.1001/jama.2021.13027
183. Kleeff J, Korc M, Apte M, la Vecchia C, Johnson CD, Biankin A v., et al. Pancreatic cancer. Nat Rev Dis Primers (2016) 2:16022. doi: 10.1038/nrdp.2016.22
184. Mizrahi JD, Surana R, Valle JW, Shroff RT. Pancreatic cancer. Lancet (2020) 395:2008–20. doi: 10.1016/S0140-6736(20)30974-0
185. von Hoff DD, Ervin T, Arena FP, Chiorean EG, Infante J, Moore M, et al. Increased survival in pancreatic cancer with nab-paclitaxel plus gemcitabine. New Engl J Med (2013) 369:1691–703. doi: 10.1056/NEJMoa1304369
186. Conroy T, Desseigne F, Ychou M, Bouché O, Guimbaud R, Bécouarn Y, et al. FOLFIRINOX versus gemcitabine for metastatic pancreatic cancer. New Engl J Med (2011) 364:1817–25. doi: 10.1056/NEJMoa1011923
187. Pelzer U, Schwaner I, Stieler J, Adler M, Seraphin J, Dörken B, et al. Best supportive care (BSC) versus oxaliplatin, folinic acid and 5-fluorouracil (OFF) plus BSC in patients for second-line advanced pancreatic cancer: A phase III-study from the German CONKO-study group. Eur J Cancer (2011) 47:1676–81. doi: 10.1016/j.ejca.2011.04.011
188. Oettle H, Riess H, Stieler JM, Heil G, Schwaner I, Seraphin J, et al. Second-line oxaliplatin, folinic acid, and fluorouracil versus folinic acid and fluorouracil alone for gemcitabine-refractory pancreatic cancer: Outcomes from the CONKO-003 trial. J Clin Oncol (2014) 32:2423–9. doi: 10.1200/JCO.2013.53.6995
189. Wang-Gillam A, Li C-P, Bodoky G, Dean A, Shan Y-S, Jameson G, et al. Nanoliposomal irinotecan with fluorouracil and folinic acid in metastatic pancreatic cancer after previous gemcitabine-based therapy (NAPOLI-1): a global, randomised, open-label, phase 3 trial. Lancet (2016) 387:545–57. doi: 10.1016/S0140-6736(15)00986-1
190. Kindler HL, Niedzwiecki D, Hollis D, Sutherland S, Schrag D, Hurwitz H, et al. Gemcitabine plus bevacizumab compared with gemcitabine plus placebo in patients with advanced pancreatic cancer: Phase III trial of the cancer and leukemia group b (CALGB 80303). J Clin Oncol (2010) 28:3617–22. doi: 10.1200/JCO.2010.28.1386
191. van Cutsem E, Vervenne WL, Bennouna J, Humblet Y, Gill S, van Laethem J-L, et al. Phase III trial of bevacizumab in combination with gemcitabine and erlotinib in patients with metastatic pancreatic cancer. J Clin Oncol (2009) 27:2231–7. doi: 10.1200/JCO.2008.20.0238
192. Rougier P, Riess H, Manges R, Karasek P, Humblet Y, Barone C, et al. Randomised, placebo-controlled, double-blind, parallel-group phase III study evaluating aflibercept in patients receiving first-line treatment with gemcitabine for metastatic pancreatic cancer. Eur J Cancer (2013) 49:2633–42. doi: 10.1016/j.ejca.2013.04.002
193. Yamaue H, Tsunoda T, Tani M, Miyazawa M, Yamao K, Mizuno N, et al. Randomized phase II/III clinical trial of elpamotide for patients with advanced pancreatic cancer: PEGASUS-PC study. Cancer Sci (2015) 106:883–90. doi: 10.1111/cas.12674
194. Kindler HL, Ioka T, Richel DJ, Bennouna J, Létourneau R, Okusaka T, et al. Axitinib plus gemcitabine versus placebo plus gemcitabine in patients with advanced pancreatic adenocarcinoma: a double-blind randomised phase 3 study. Lancet Oncol (2011) 12:256–62. doi: 10.1016/S1470-2045(11)70004-3
195. Gonçalves A, Gilabert M, François E, Dahan L, Perrier H, Lamy R, et al. BAYPAN study: a double-blind phase III randomized trial comparing gemcitabine plus sorafenib and gemcitabine plus placebo in patients with advanced pancreatic cancer. Ann Oncol (2012) 23:2799–805. doi: 10.1093/annonc/mds135
196. Bergmann L, Maute L, Heil G, Rüssel J, Weidmann E, Köberle D, et al. A prospective randomised phase-II trial with gemcitabine versus gemcitabine plus sunitinib in advanced pancreatic cancer. Eur J Cancer (2015) 51:27–36. doi: 10.1016/j.ejca.2014.10.010
197. Shaib WL, Rupji M, Khair TA, Robin EL, El-Rayes BF, Huyck TK, et al. Phase II randomized, double-blind study of mFOLFIRINOX plus ramucirumab versus mFOLFIRINOX plus placebo in advanced pancreatic cancer patients (HCRN GI14-198). J Clin Oncol (2021) 39:413–3. doi: 10.1200/JCO.2021.39.3_suppl.413
198. Valle JW, Kelley RK, Nervi B, Oh D-Y, Zhu AX. Biliary tract cancer. Lancet (2021) 397:428–44. doi: 10.1016/S0140-6736(21)00153-7
199. Banales JM, Marin JJG, Lamarca A, Rodrigues PM, Khan SA, Roberts LR, et al. Cholangiocarcinoma 2020: the next horizon in mechanisms and management. Nat Rev Gastroenterol Hepatol (2020) 17:557–88. doi: 10.1038/s41575-020-0310-z
200. Okusaka T, Nakachi K, Fukutomi A, Mizuno N, Ohkawa S, Funakoshi A, et al. Gemcitabine alone or in combination with cisplatin in patients with biliary tract cancer: a comparative multicentre study in Japan. Br J Cancer (2010) 103:469–74. doi: 10.1038/sj.bjc.6605779
201. Valle J, Wasan H, Palmer DH, Cunningham D, Anthoney A, Maraveyas A, et al. Cisplatin plus gemcitabine versus gemcitabine for biliary tract cancer. New Engl J Med (2010) 362:1273–81. doi: 10.1056/NEJMoa0908721
202. Oh D-Y, Ruth He A, Qin S, Chen L-T, Okusaka T, Vogel A, et al. Durvalumab plus gemcitabine and cisplatin in advanced biliary tract cancer. NEJM Evid. (2022) 1(8). doi: 10.1056/EVIDoa2200015
203. Valle JW, Vogel A, Denlinger CS, He AR, Bai L-Y, Orlova R, et al. Addition of ramucirumab or merestinib to standard first-line chemotherapy for locally advanced or metastatic biliary tract cancer: a randomised, double-blind, multicentre, phase 2 study. Lancet Oncol (2021) 22:1468–82. doi: 10.1016/S1470-2045(21)00409-5
204. Valle JW, Wasan H, Lopes A, Backen AC, Palmer DH, Morris K, et al. Cediranib or placebo in combination with cisplatin and gemcitabine chemotherapy for patients with advanced biliary tract cancer (ABC-03): a randomised phase 2 trial. Lancet Oncol (2015) 16:967–78. doi: 10.1016/S1470-2045(15)00139-4
205. Moehler M, Maderer A, Schimanski C, Kanzler S, Denzer U, Kolligs FT, et al. Gemcitabine plus sorafenib versus gemcitabine alone in advanced biliary tract cancer: A double-blind placebo-controlled multicentre phase II AIO study with biomarker and serum programme. Eur J Cancer (2014) 50:3125–35. doi: 10.1016/j.ejca.2014.09.013
206. Santoro A, Gebbia V, Pressiani T, Testa A, Personeni N, Arrivas Bajardi E, et al. A randomized, multicenter, phase II study of vandetanib monotherapy versus vandetanib in combination with gemcitabine versus gemcitabine plus placebo in subjects with advanced biliary tract cancer: the VanGogh study. Ann Oncol (2015) 26:542–7. doi: 10.1093/annonc/mdu576
Keywords: anti-angiogenic, tyrosine kinase inhibitor, neoangiogenesis, gastrointestinal cancer, hepatobiliary tumour, hepatocellular carcinoma, colorectal cancer
Citation: Saoudi González N, Castet F, Élez E, Macarulla T and Tabernero J (2022) Current and emerging anti-angiogenic therapies in gastrointestinal and hepatobiliary cancers. Front. Oncol. 12:1021772. doi: 10.3389/fonc.2022.1021772
Received: 17 August 2022; Accepted: 26 September 2022;
Published: 10 October 2022.
Edited by:
Ana Sofia Fernandes, Universidade Lusófona Research Center for Biosciences & Health Technologies, PortugalReviewed by:
Elisa Lozano, University of Salamanca, SpainAndreas Pircher, Innsbruck Medical University, Austria
Copyright © 2022 Saoudi González, Castet, Élez, Macarulla and Tabernero. This is an open-access article distributed under the terms of the Creative Commons Attribution License (CC BY). The use, distribution or reproduction in other forums is permitted, provided the original author(s) and the copyright owner(s) are credited and that the original publication in this journal is cited, in accordance with accepted academic practice. No use, distribution or reproduction is permitted which does not comply with these terms.
*Correspondence: Teresa Macarulla, dG1hY2FydWxsYUB2aGlvLm5ldA==
†These authors have contributed equally to this work and share first authorship