- 1Division of Radiotherapy and Imaging, The Institute of Cancer Research, London, United Kingdom
- 2Department of Imaging, The Royal Marsden National Health Service (NHS) Foundation Trust, London, United Kingdom
- 3Radiation Oncology, The Christie National Health Service (NHS) Foundation Trust Manchester, Manchester, United Kingdom
- 4The Division of Cancer Sciences, University of Manchester, Manchester, United Kingdom
- 5Centre for Evolution and Cancer, The Institute of Cancer Research, London, United Kingdom
- 6Radiation Oncology, Mount Vernon Cancer Centre, Northwood, United Kingdom
Introduction
Tissues become hypoxic when their oxygen consumption exceeds their supply. In tumors, neoangiogenesis results in disordered vascular morphology and this leads to inadequate oxygen supply to rapidly growing cells (1). However, because vessels have variations in their vascular tone, hypoxic status is a dynamic rather than a static event; it may be transient and is often cyclical (2). Cyclical hypoxia with a median periodicity of 15 mins has been described in xenografts and head and neck cancers in patients (3). Hypoxia is important because it is well accepted as a poor prognostic factor in for patients with a range of cancer types (4–7) where it has been associated with disease that is progressive, resistant and metastatic (8).
Under hypoxic conditions, oxygen-sensitive transcription factors (hypoxia inducible factors [HIFs]) are upregulated. In the endometrium, HIF1α expression increases as the tissue undergoes changes from normality to being premalignant and then to become adenocarcinoma. This is paralleled by increased angiogenesis in the endometrium, suggesting that HIF1α and thus tissue hypoxia might be a key regulator in endometrial carcinogenesis (9). A poorer prognosis in patients with EC expressing HIF-1α has been demonstrated in a metaanalysis with a hazard ratio of 2.29 (10), and its link to tumor aggressiveness at other cancer sites is documented (11). Hypoxic status also has been associated with mutations of multiple genes in endometrial cancer (EC) (12). In other cancer types, driver mutations in p53, MYC and PTEN are enriched in hypoxic tumors (13) with an effect of hypoxia on mutational load (14). Therefore, as hypoxia is likely to determine the evolutionary trajectories and as a result the management and outcomes of cancer, imaging tumor hypoxic status in EC may offer prognostic value and facilitate personalisation of treatment strategies (15).
Endometrial cancer: Diagnosis, staging and the changing molecular landscape
EC, (9,300 new cases per annum in the UK (16), 65,620 new cases in 2020 in USA (17) usually presents with post-menopausal bleeding and is detected at an early stage. Diagnostic confirmation on pipelle sampling of the endometrium or at hysteroscopy is followed by pelvic magnetic resonance imaging (MRI) for disease staging (18). Endometrioid and mucinous carcinomas are classified as type I and serous and clear cell carcinomas as type II. The former are usually low grade and low stage at presentation and the latter high grade and advanced stage. Disease outcome depends on tumor grade, stage, subtype, depth of myometrial invasion, lymphovascular space invasion and lymph node involvement (19). In fact, in type I endometrial adenocarcinoma, high expression of HIF-1 α showed a significant correlation with higher grade of the tumor, depth of myometrial invasion, adnexal invasion and clinical stage (20), which strengthens the argument for hypoxia driving tumor progression by favouring selection of adverse genetic clones.
Molecular classification is now used to define risk groups in EC, namely deoxyribose nucleic acid (DNA) polymerase ɛ ultramutated (POLEmut), mismatch repair-deficient (MMRd), p53 mutant (p53abn) and those EC lacking any of these alterations, referred to as NSMP (non-specific molecular profile) (21). Prognosis is extremely good in POLE and poor in p53 mutant cancers (22) with poor clinical outcomes in the latter group being independent of histology grade or stage (23–25); the other two categories fall between these two extremes. TP53 mutations are highly prevalent in the serous (Type II) subtype (88% of 42 serous ECs (26), and are also present in a subset of endometroid (Type I) carcinomas (15% of 186 endometroid ECs (26). Recent data show that a subset of p53mut EC is homologous recombination‐deficient (HRD), and some of these EC can arise in the context of germline breast cancer (BRCA)1/2 mutations (27–29). The exact prevalence of HRD in p53mut EC is currently unknown; in a small and selected set of cases it was 46% (28).
The biological and genetic mechanisms that causally link hypoxia with progression of disease are being unravelled. Hypoxia and associated acidosis activate the TP53 dependent stress response and apoptosis (30). This then provides selective evolutionary pressure for the emergence of mutants in the TP53 response (13). Such genetic variants then preferentially expand as a population. Their fitness benefit is however more than simple survival. They are intrinsically more resistant to many of the therapeutic modalities that operate via the TP53 apoptosis pathway. Their failure to undergo TP53 driven cell cycle arrest and DNA repair also leads to genetic instability (31). Additionally, as hypoxia mimics the mesenchymal stem cell niche (32), surviving TP53 mutants undergo Epithelial-Mesenchymal transition (EMT) to migratory, stem cell phenotypes. Hence the hypoxic microenvironment encourages the selection of cancer cell populations that have an expanded pool of stem cells (the critical units of selection in cancer progression) that are likely to be genetically unstable. These biological features fuel both disease progression and the likelihood of treatment resistance (33).
Methodology and challenges of imaging hypoxia
The prognostic relevance of hypoxia in EC has been determined largely by using the expression of HIF-1α (12) and the presence of tumor necrosis (34). Although the correlation of HIF-1α with imaging estimates of hypoxia is variable (35), an association has been demonstrated in EC (15). On imaging, hypoxia may be measured indirectly or directly. Traditionally, tumor vasculature has been imaged using ultrasound and computerized tomography (CT). Doppler ultrasound, based on the frequency shift of moving echo-generating components in flowing blood, has been used to classify endometrial pathologies (36). With contrast-enhanced CT, extracted metrics relate to blood flow, blood volume and vascular permeability (37). Although increased vascularity in tumors is highly disorganised and leaky, often indicating an increased hypoxic status (38), it is not a direct measurement.
Positron Emission Tomography (PET) uses hypoxia-specific tracers such as 18F-labelled nitroimidazoles and copper (Cu)-labelled diacetyl-bis(N4-methylthiosemicarbazone) analogues (39). Under hypoxic conditions, free nitro radicals are retained within the cell. Though commonly used 18F-fluoroimidazole (18F-FMISO) (40) has relatively low uptake, slow kinetics and is influenced by non-hypoxic metabolism. 18F-FAZA [1-(5-fluoro-5-deoxy-α-D-arabinofuranosyl)-2-nitroimidazole)] offers better resolution and signal-to-noise ratio (41). Cu complex agents with diacetyl-bis(N4-methylthiosemicarbazone) (ATSM) ligand under hypoxic conditions cannot be reversibly oxidised by the cell also making Cu-ATSM a possible means for evaluating hypoxia in the clinic (42–44). However, its specificity is debatable and validation with pimonidazole stained tissues has been variable and tumor type specific (45, 46)
A shift to non-invasive hypoxia imaging with MRI is advantageous (47). In blood oxygen level dependent (BOLD) MRI, also known as intrinsic susceptibility-weighted MRI, paramagnetic deoxyhaemoglobin within red blood cells (in contrast to non-paramagnetic oxyhaemoglobin) increases the MR transverse relaxation rate (R2*, the inverse of the transverse relaxation time T2*), of water in blood and surrounding tissues. Variations in perfusion mean that the relationship between R2* and tissue pO2 is non-linear and perfusion dependent. Nevertheless, BOLD-MRI is sensitive to changes in pO2 within vessels and in tissues adjacent to perfused vessels (48, 49). R2* has been shown to correlate positively with tissue hypoxia score (HP5) and oxygen pressure (50) and with HIF-2α expression in colorectal cancer with different tumor stages (51, 52). Advantages of the BOLD-MRI technique for measurement of hypoxia are lack of need for externally administered contrast media, easy repeatability, near real-time visualisation of time-dependent changes and a measure independent of blood flow. Nevertheless, the variability of the measurement (53), means that measuring a change in R2* following an oxygen challenge may be preferable particularly as they have been shown to correlate strongly with pimonidazole staining in tumor models (54).
Oxygen in solution and deoxy Hb also affect the longitudinal relaxation rate of tissues (55) and are exploited in the technique of oxygen-enhanced (OE)- MRI, also known as tumor oxygen level dependent contrast (TOLD). Their effect on the rate of longitudinal proton relaxation (R1) can be enhanced by the inhalation of 100% O2 which results in an increase in the relaxation rate in normoxic tissues, primarily due to an increase in dissolved oxygen (56). A measurable signal change of up to 20% is achievable in normoxic tissues with 100% O2 inhalation on clinical scanners (57) that can distinguish them from hypoxic tissue (58). OE-MRI has been validated in pre-clinical studies (59, 60) and had initial clinical translation (61, 62) with promising results. MRI measures of hypoxia can be implemented as an extension of the imaging staging examination but require standardisation of image acquisition and analysis methodology prior to clinical use.
Endometrial cancer– opportunities for adjusting management strategies to hypoxic status
Management of EC is primarily surgical as patients with uterus-confined low-risk disease are often cured by surgery. Prognostic factors that describe groups by their risk of recurrence (histological type and grade, age, tumor size, and lymphovascular space involvement (63) are used to determine need for adjuvant therapies. Several trials have compared external beam radiotherapy (EBRT) after surgery versus observation after surgery in intermediate and high-risk disease: the PORTEC 1 (64), ASTEC/EN5 (65) and Gynecologic Oncology Group (GOG) (66) trials all showed a reduced risk of vaginal and pelvic relapse though overall survival did not differ. PORTEC 2 then showed that equivalent locoregional control could be achieved with vaginal brachytherapy without the toxicity of EBRT, so that adjuvant brachytherapy is the standard-of-care in patients with intermediate-risk disease following surgery (67). More recently, the PORTEC-3 trial, concluded that molecular classification has strong prognostic value in high-risk EC, with significantly improved recurrence-free survival with adjuvant chemoradiotherapy compared to radiotherapy alone for p53abnormal tumors, regardless of histologic type (68).
Hypoxia imaging and its link to TP53 status offers potential to refine management strategies by selecting patients through prognostic stratification. Pre-operative hypoxia imaging could identify the women who would most benefit from adjuvant radiotherapy and select women who might benefit from EBRT rather than adjuvant brachytherapy alone. Also, in hypoxic tumors post-surgery, where there is residual disease, it may be possible to dose-escalate with either brachytherapy, external beam radiotherapy or the use of a radiosensitiser, or omit or dose de-escalation when tumor hypoxia is not demonstrated. In locally advanced endometrial cancer (stage III) treated primarily with chemoradiotherapy, hypoxia imaging may also indicate those who would benefit from hypoxia modification with a radiosensitiser. Drugs like carbogen and nicotinamide can be combined with radiation without increasing late toxicity but may improve survival outcomes as in muscle-invasive bladder cancer (69). The effect of hypoxia on the immune tumor microenvironment is complex, but it is likely to promote resistance to immune modulatory approaches (70).
Neoadjuvant chemotherapy (NAC) has primarily been trialled in patients with Stage 4 or metastatic disease at presentation with uterine papillary serous carcinomas (71) to facilitate optimal surgical cytoreduction. More recently this has been extended to endometrioid adenocarcinoma (72, 73) where the use of NAC to enable cytoreductive surgery resulted in an increased progression-free and overall survival (74). It may be possible to refine the use of NAC further if tumor hypoxic status along with tumor stage and volume were considered to select patients likely to respond. Finally, the association of hypoxia with genetic instability and DNA damage repair efficacy (supported by the prevalence of HRD in p53 mutated EC (28)), indicates that hypoxia imaging could be an important predictive selector for women who benefit from agents such as poly adenosine diphosphate ribose polymerase (PARP) inhibitors although their use in EC remains to be established.
As with EC in the primary setting, locally recurrent EC that shows a high degree of hypoxia may benefit from dose-escalation or use of a radiosensitiser. Development of prognostic models based on hypoxic status and molecular profiling (75) may change approaches to maintenance therapies and surveillance. Poor prognostic tumors at risk of progressive disease thus identified may benefit from a more aggressive surveillance strategy adapted to their risk. It may also enable implementation of future maintenance therapy approaches in suitable patient cohorts.
Discussion and concluding remarks
The technical validation and demonstration of target monitoring with hypoxia imaging remains a major challenge. Continuous measurements indicate variable O2 saturation, there is heterogeneity within each tumor and between tumor sites in the same patient (76) and thresholds for differentiating normoxic from hypoxic tissues in imaging studies are lacking. The impact of hypoxia on chemoresistance has been long established (77), but the range of hypoxia particularly across the different molecular subtypes needs to be understood. Recording hypoxia within tumors requires obsessive attention to imaging technique, adherence to imaging protocols such as those mandated by the Quantitative Imaging Biomarkers Alliance, and as with all biomarker studies, an establishment and understanding of the reproducibility of the measurement (78, 79). Despite these restrictions, when implemented according to protocol, hypoxia imaging with MRI can be a simple add-on to the staging examination of endometrial cancer. The additional imaging time of 10 mins for a BOLD evaluation and 10 mins for a TOLD evaluation is clinically achievable and can even be implemented in conjunction to increase the robustness of hypoxia evaluation. Methods such as MR fingerprinting may in future also allow simultaneous acquisition of both the T2* and T1 information (80) before, during and after the oxygen challenge, markedly reducing image acquisition time. The derived information is a useful adjunct to that already available and has the potential to substantially alter and enhance the management options offered to patients (Figure 1).
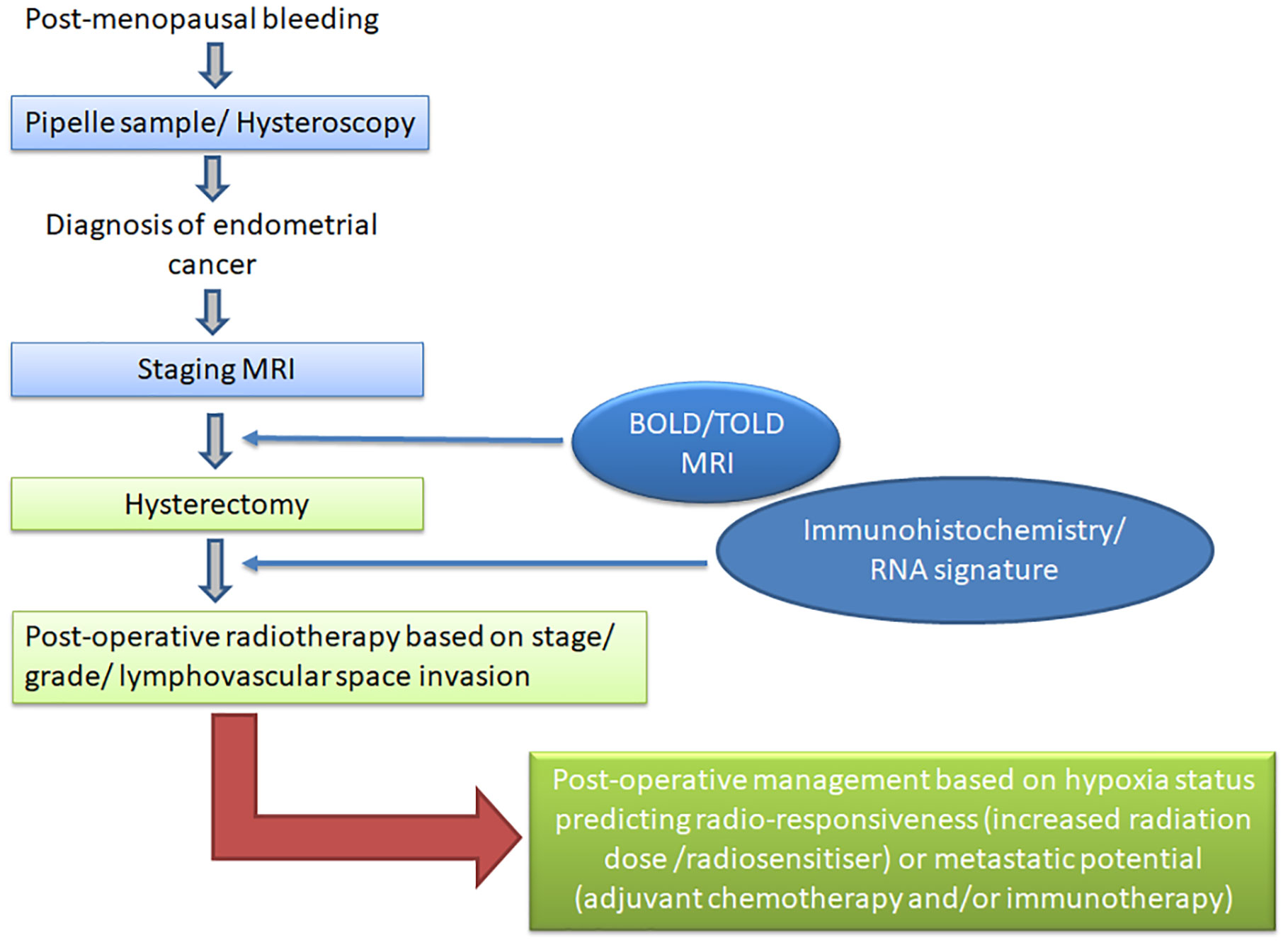
Figure 1 Diagnostic and management pathway for patients with endometrial cancer. Current diagnostic tests are shown in light blue and proposed hypoxia imaging in dark blue; current management options are shown in light green and alternative strategies based on hypoxia imaging and molecular stratification in dark green.
Author contributions
All authors contributed to the design, drafting, writing and editing of this opinion piece.
Funding
AC, JPBO'C and PJH are supported by the NIHR Manchester Biomedical Research Centre.
Conflict of interest
The authors declare that the research was conducted in the absence of any commercial or financial relationships that could be construed as a potential conflict of interest.
Publisher’s note
All claims expressed in this article are solely those of the authors and do not necessarily represent those of their affiliated organizations, or those of the publisher, the editors and the reviewers. Any product that may be evaluated in this article, or claim that may be made by its manufacturer, is not guaranteed or endorsed by the publisher.
References
1. Jain RK. Normalization of tumor vasculature: an emerging concept in antiangiogenic therapy. Science (2005) 307:58–62. doi: 10.1126/science.1104819
2. Singleton DC, Macann A, Wilson WR. Therapeutic targeting of the hypoxic tumour microenvironment. Nat Rev Clin Oncol (2021) 18:751–72. doi: 10.1038/s41571-021-00539-4
3. Panek R, Welsh L, Baker LCJ, Schmidt MA, Wong KH, Riddell AM, et al. Noninvasive imaging of cycling hypoxia in head and neck cancer using intrinsic susceptibility MRI. Clin Cancer Res (2017) 23:4233–41. doi: 10.1158/1078-0432.CCR-16-1209
4. Muz B, de la Puente P, Azab F, Azab AK. The role of hypoxia in cancer progression, angiogenesis, metastasis, and resistance to therapy. Hypoxia (Auckl) (2015) 3:83–92. doi: 10.2147/HP.S93413
5. Horsman MR, Vaupel P. Pathophysiological basis for the formation of the tumor microenvironment. Front Oncol (2016) 6:66. doi: 10.3389/fonc.2016.00066
6. Popper HH. Progression and metastasis of lung cancer. Cancer Metastasis Rev (2016) 35:75–91. doi: 10.1007/s10555-016-9618-0
7. Tafani M, Sansone L, Limana F, Arcangeli T, De Santis E, Polese M, et al. The interplay of reactive oxygen species, hypoxia, inflammation, and sirtuins in cancer initiation and progression. Oxid Med Cell Longev (2016) 2016:3907147. doi: 10.1155/2016/3907147
8. Unwith S, Zhao H, Hennah L, Ma D. The potential role of HIF on tumour progression and dissemination. Int J Cancer (2015) 136:2491–503. doi: 10.1002/ijc.28889
9. Horree N, van Diest PJ, van der Groep P, Sie-Go DM, Heintz AP. Hypoxia and angiogenesis in endometrioid endometrial carcinogenesis. Cell Oncol (2007) 29:219–27. doi: 10.1155/2007/434731
10. Zhu P, Shen L, Ren Q, Zeng Q, He X. Prognostic and clinicopathological significance of hypoxia-inducible factor-1alpha in endometrial cancer: A meta-analysis. Front Oncol (2020) 10:587420. doi: 10.3389/fonc.2020.587420
11. Daimiel I. Insights into hypoxia: Non-invasive assessment through imaging modalities and its application in breast cancer. J Breast Cancer (2019) 22:155–71. doi: 10.4048/jbc.2019.22.e26
12. Cai Y, Wang B, Xu W, Liu K, Gao Y, Guo C, et al. Endometrial cancer: Genetic, metabolic characteristics, therapeutic strategies and nanomedicine. Curr Med Chem (2021) 28:8755–81. doi: 10.2174/0929867328666210705144456
13. Bhandari V, Li CH, Bristow RG, Boutros PC, Consortium P. Divergent mutational processes distinguish hypoxic and normoxic tumours. Nat Commun (2020) 11:737. doi: 10.1038/s41467-019-14052-x
14. Hassan Venkatesh G, Bravo P, Shaaban Moustafa Elsayed W, Amirtharaj F, Wojtas B, Abou Khouzam R, et al. Hypoxia increases mutational load of breast cancer cells through frameshift mutations. Oncoimmunology (2020) 9:1750750. doi: 10.1080/2162402X.2020.1750750
15. Berg A, Fasmer KE, Mauland KK, Ytre-Hauge S, Hoivik EA, Husby JA, et al. Tissue and imaging biomarkers for hypoxia predict poor outcome in endometrial cancer. Oncotarget (2016) 7:69844–56. doi: 10.18632/oncotarget.12004
16. (2022). Available at: https://www.cancerresearchuk.org/health-professional/cancer-statistics/statistics-by-cancer-type/uterine-cancer/ (Accessed 8th August 2022).
17. Siegel RL, Miller KD, Jemal A. Cancer statistics, 2020. CA Cancer J Clin (2020) 70:7–30. doi: 10.3322/caac.21590
18. Koskas M, Amant F, Mirza MR, Creutzberg CL. Cancer of the corpus uteri: 2021 update. Int J Gynaecol Obstet (2021) 155 Suppl 1:45–60. doi: 10.1002/ijgo.13866
19. Prat J. Prognostic parameters of endometrial carcinoma. Hum Pathol (2004) 35:649–62. doi: 10.1016/j.humpath.2004.02.007
20. Pansare V, Munkarah AR, Schimp V, Haitham Arabi M, Saed GM, Morris RT, et al. Increased expression of hypoxia-inducible factor 1alpha in type I and type II endometrial carcinomas. Mod Pathol (2007) 20:35–43. doi: 10.1038/modpathol.3800718
21. Jamieson A, Thompson EF, Huvila J, Gilks CB, McAlpine JN. p53abn endometrial cancer: understanding the most aggressive endometrial cancers in the era of molecular classification. Int J Gynecol Cancer (2021) 31:907–13. doi: 10.1136/ijgc-2020-002256
22. Allo G, Bernardini MQ, Wu RC, Shih Ie M, Kalloger S, Pollett A, et al. ARID1A loss correlates with mismatch repair deficiency and intact p53 expression in high-grade endometrial carcinomas. Mod Pathol (2014) 27:255–61. doi: 10.1038/modpathol.2013.144
23. Stelloo E, Nout RA, Osse EM, Jurgenliemk-Schulz IJ, Jobsen JJ, Lutgens LC, et al. Improved risk assessment by integrating molecular and clinicopathological factors in early-stage endometrial cancer-combined analysis of the PORTEC cohorts. Clin Cancer Res (2016) 22:4215–24. doi: 10.1158/1078-0432.CCR-15-2878
24. Kommoss S, McConechy MK, Kommoss F, Leung S, Bunz A, Magrill J, et al. Final validation of the ProMisE molecular classifier for endometrial carcinoma in a large population-based case series. Ann Oncol (2018) 29:1180–8. doi: 10.1093/annonc/mdy058
25. Yano M, Ito K, Yabuno A, Ogane N, Katoh T, Miyazawa M, et al. Impact of TP53 immunohistochemistry on the histological grading system for endometrial endometrioid carcinoma. Mod Pathol (2019) 32:1023–31. doi: 10.1038/s41379-019-0220-1
26. Schultheis AM, Martelotto LG, De Filippo MR, Piscuglio S, Ng CK, Hussein YR, et al. TP53 mutational spectrum in endometrioid and serous endometrial cancers. Int J Gynecol Pathol (2016) 35:289–300. doi: 10.1097/PGP.0000000000000243
27. de Jonge MM, Ritterhouse LL, de Kroon CD, Vreeswijk MPG, Segal JP, Puranik R, et al. Germline BRCA-associated endometrial carcinoma is a distinct clinicopathologic entity. Clin Cancer Res (2019) 25:7517–26. doi: 10.1158/1078-0432.CCR-19-0848
28. de Jonge MM, Mooyaart AL, Vreeswijk MP, de Kroon CD, van Wezel T, van Asperen CJ, et al. Linking uterine serous carcinoma to BRCA1/2-associated cancer syndrome: A meta-analysis and case report. Eur J Cancer (2017) 72:215–25. doi: 10.1016/j.ejca.2016.11.028
29. de Jonge MM, Auguste A, van Wijk LM, Schouten PC, Meijers M, Ter Haar NT, et al. Frequent homologous recombination deficiency in high-grade endometrial carcinomas. Clin Cancer Res (2019) 25:1087–97. doi: 10.1158/1078-0432.CCR-18-1443
30. Pan Y, Oprysko PR, Asham AM, Koch CJ, Simon MC. p53 cannot be induced by hypoxia alone but responds to the hypoxic microenvironment. Oncogene (2004) 23:4975–83. doi: 10.1038/sj.onc.1207657
31. Luoto KR, Kumareswaran R, Bristow RG. Tumor hypoxia as a driving force in genetic instability. Genome Integr (2013) 4:5. doi: 10.1186/2041-9414-4-5
32. Mohyeldin A, Garzon-Muvdi T, Quinones-Hinojosa A. Oxygen in stem cell biology: a critical component of the stem cell niche. Cell Stem Cell (2010) 7:150–61. doi: 10.1016/j.stem.2010.07.007
33. Greaves M. Evolutionary determinants of cancer. Cancer Discov (2015) 5:806–20. doi: 10.1158/2159-8290.CD-15-0439
34. Bredholt G, Mannelqvist M, Stefansson IM, Birkeland E, Bo TH, Oyan AM, et al. Tumor necrosis is an important hallmark of aggressive endometrial cancer and associates with hypoxia, angiogenesis and inflammation responses. Oncotarget (2015) 6:39676–91. doi: 10.18632/oncotarget.5344
35. Thureau S, Piton N, Gouel P, Modzelewski R, Dujon A, Baste JM, et al. First comparison between [18f]-FMISO and [18f]-faza for preoperative pet imaging of hypoxia in lung cancer. Cancers (Basel) (2021) 13:1–12. doi: 10.3390/cancers13164101
36. Van Den Bosch T, Verbakel JY, Valentin L, Wynants L, De Cock B, Pascual MA, et al. Typical ultrasound features of various endometrial pathologies described using international endometrial tumor analysis (IETA) terminology in women with abnormal uterine bleeding. Ultrasound Obstet Gynecol (2021) 57:164–72. doi: 10.1002/uog.22109
37. Lin M, Zhang Q, Song Y, Yu X, Ouyang H, Xie L, et al. Differentiation of endometrial adenocarcinoma from adenocarcinoma of cervix using kinetic parameters derived from DCE-MRI. Eur J Radiol (2020) 130:109190. doi: 10.1016/j.ejrad.2020.109190
38. Ozbudak IH, Karaveli S, Simsek T, Erdogan G, Pestereli E. Neoangiogenesis and expression of hypoxia-inducible factor 1alpha, vascular endothelial growth factor, and glucose transporter-1 in endometrioid type endometrium adenocarcinomas. Gynecol Oncol (2008) 108:603–8. doi: 10.1016/j.ygyno.2007.11.028
39. Chia K, Fleming IN, Blower PJ. Hypoxia imaging with PET: which tracers and why? Nucl Med Commun (2012) 33:217–22. doi: 10.1097/MNM.0b013e32834eacb7
40. Krohn KA, Link JM, Mason RP. Molecular imaging of hypoxia. J Nucl Med (2008) 49 Suppl 2:129S–48S. doi: 10.2967/jnumed.107.045914
41. Postema EJ, McEwan AJ, Riauka TA, Kumar P, Richmond DA, Abrams DN, et al. Initial results of hypoxia imaging using 1-alpha-D: -(5-deoxy-5-[18F]-fluoroarabinofuranosyl)-2-nitroimidazole ( 18F-FAZA). Eur J Nucl Med Mol Imaging (2009) 36:1565–73. doi: 10.1007/s00259-009-1154-5
42. Dehdashti F, Grigsby PW, Lewis JS, Laforest R, Siegel BA, Welch MJ. Assessing tumor hypoxia in cervical cancer by PET with 60Cu-labeled diacetyl-bis(N4-methylthiosemicarbazone). J Nucl Med (2008) 49:201–5. doi: 10.2967/jnumed.107.048520
43. Piccardo A, Paparo F, Puntoni M, Righi S, Bottoni G, Bacigalupo L, et al. (64)CuCl2 PET/CT in prostate cancer relapse. J Nucl Med (2018) 59:444–51. doi: 10.2967/jnumed.117.195628
44. Minagawa Y, Shizukuishi K, Koike I, Horiuchi C, Watanuki K, Hata M, et al. Assessment of tumor hypoxia by 62Cu-ATSM PET/CT as a predictor of response in head and neck cancer: a pilot study. Ann Nucl Med (2011) 25:339–45. doi: 10.1007/s12149-011-0471-5
45. McCall KC, Humm JL, Bartlett R, Reese M, Carlin S. Copper-64-diacetyl-bis(N(4)-methylthiosemicarbazone) pharmacokinetics in FaDu xenograft tumors and correlation with microscopic markers of hypoxia. Int J Radiat Oncol Biol Phys (2012) 84:e393–399. doi: 10.1016/j.ijrobp.2012.05.005
46. Hansen AE, Kristensen AT, Jorgensen JT, McEvoy FJ, Busk M, van der Kogel AJ, et al. (64)Cu-ATSM and (18)FDG PET uptake and (64)Cu-ATSM autoradiography in spontaneous canine tumors: comparison with pimonidazole hypoxia immunohistochemistry. Radiat Oncol (2012) 7:89. doi: 10.1186/1748-717X-7-89
47. Dewhirst MW, Birer SR. Oxygen-enhanced MRI is a major advance in tumor hypoxia imaging. Cancer Res (2016) 76:769–72. doi: 10.1158/0008-5472.CAN-15-2818
48. Tatum JL, Kelloff GJ, Gillies RJ, Arbeit JM, Brown JM, Chao KS, et al. Hypoxia: importance in tumor biology, noninvasive measurement by imaging, and value of its measurement in the management of cancer therapy. Int J Radiat Biol (2006) 82:699–757. doi: 10.1080/09553000601002324
49. Howe FA, Robinson SP, McIntyre DJ, Stubbs M, Griffiths JR. Issues in flow and oxygenation dependent contrast (FLOOD) imaging of tumours. NMR BioMed (2001) 14:497–506. doi: 10.1002/nbm.716
50. Chopra S, Foltz WD, Milosevic MF, Toi A, Bristow RG, Menard C, et al. Comparing oxygen-sensitive MRI (BOLD R2*) with oxygen electrode measurements: a pilot study in men with prostate cancer. Int J Radiat Biol (2009) 85:805–13. doi: 10.1080/09553000903043059
51. Fan B, Wang XY, Yang XD, Zhong H, Wu CX, Jiang XX. Blood oxygen level-dependent MRI for the monitoring of neoadjuvant chemotherapy in breast carcinoma: initial experience. Magn Reson Imaging (2011) 29:153–9. doi: 10.1016/j.mri.2010.08.014
52. Ring J, Persigehl T, Remmele S, Heindel W, Dahnke H, Bremer C. Monitoring of bevacizumab-induced antiangiogenic treatment effects by "steady state" ultrasmall superparamagnetic iron oxide particles magnetic resonance imaging using robust multiecho DeltaR2* relaxometry. Invest Radiol (2011) 46:326–30. doi: 10.1097/RLI.0b013e3182045457
53. Leutritz T, Seif M, Helms G, Samson RS, Curt A, Freund P, et al. Multiparameter mapping of relaxation (R1, R2*), proton density and magnetization transfer saturation at 3 T: A multicenter dual-vendor reproducibility and repeatability study. Hum Brain Mapp (2020) 41:4232–47. doi: 10.1002/hbm.25122
54. Virani N, Kwon J, Zhou H, Mason R, Berbeco R, Protti A. In vivo hypoxia characterization using blood oxygen level dependent magnetic resonance imaging in a preclinical glioblastoma mouse model. Magn Reson Imaging (2021) 76:52–60. doi: 10.1016/j.mri.2020.11.003
55. Young IR, Clarke GJ, Bailes DR, Pennock JM, Doyle FH, Bydder GM. Enhancement of relaxation rate with paramagnetic contrast agents in NMR imaging. J Comput Tomogr (1981) 5:543–7. doi: 10.1016/0149-936X(81)90089-8
56. O'Connor JPB, Robinson SP, Waterton JC. Imaging tumour hypoxia with oxygen-enhanced MRI and BOLD MRI. Br J Radiol (2019) 92:20180642. doi: 10.1259/bjr.20180642
57. O'Connor JP, Jackson A, Buonaccorsi GA, Buckley DL, Roberts C, Watson Y, et al. Organ-specific effects of oxygen and carbogen gas inhalation on tissue longitudinal relaxation times. Magn Reson Med (2007) 58:490–6. doi: 10.1002/mrm.21357
58. Hammond EM, Asselin MC, Forster D, O'Connor JP, Senra JM, Williams KJ. The meaning, measurement and modification of hypoxia in the laboratory and the clinic. Clin Oncol (R Coll Radiol) (2014) 26:277–88. doi: 10.1016/j.clon.2014.02.002
59. O'Connor JP, Boult JK, Jamin Y, Babur M, Finegan KG, Williams KJ, et al. Oxygen-enhanced MRI accurately identifies, quantifies, and maps tumor hypoxia in preclinical cancer models. Cancer Res (2016) 76:787–95. doi: 10.1158/0008-5472.CAN-15-2062
60. Cao-Pham TT, Joudiou N, Van Hul M, Bouzin C, Cani PD, Gallez B, et al. Combined endogenous MR biomarkers to predict basal tumor oxygenation and response to hyperoxic challenge. NMR BioMed (2017) 30:1–12. doi: 10.1002/nbm.3836
61. Linnik IV, Scott ML, Holliday KF, Woodhouse N, Waterton JC, O'Connor JP, et al. Noninvasive tumor hypoxia measurement using magnetic resonance imaging in murine U87 glioma xenografts and in patients with glioblastoma. Magn Reson Med (2014) 71:1854–62. doi: 10.1002/mrm.24826
62. Salem A, Little RA, Latif A, Featherstone AK, Babur M, Peset I, et al. Oxygen-enhanced MRI is feasible, repeatable, and detects radiotherapy-induced change in hypoxia in xenograft models and in patients with non-small cell lung cancer. Clin Cancer Res (2019) 25:3818–29. doi: 10.1158/1078-0432.CCR-18-3932
63. Murali R, Soslow RA, Weigelt B. Classification of endometrial carcinoma: more than two types. Lancet Oncol (2014) 15:e268–278. doi: 10.1016/S1470-2045(13)70591-6
64. Creutzberg CL, van Putten WL, Koper PC, Lybeert ML, Jobsen JJ, Warlam-Rodenhuis CC, et al. Surgery and postoperative radiotherapy versus surgery alone for patients with stage-1 endometrial carcinoma: multicentre randomised trial. PORTEC Study Group Post Operative Radiat Ther Endometrial Carcinoma. Lancet (2000) 355:1404–11. doi: 10.1016/S0140-6736(00)02139-5
65. Group AES, Blake P, Swart AM, Orton J, Kitchener H, Whelan T, et al. Adjuvant external beam radiotherapy in the treatment of endometrial cancer (MRC ASTEC and NCIC CTG EN.5 randomised trials): pooled trial results, systematic review, and meta-analysis. Lancet (2009) 373:137–46. doi: 10.1016/S0140-6736(08)61767-5
66. Keys HM, Roberts JA, Brunetto VL, Zaino RJ, Spirtos NM, Bloss JD, et al. A phase III trial of surgery with or without adjunctive external pelvic radiation therapy in intermediate risk endometrial adenocarcinoma: a gynecologic oncology group study. Gynecol Oncol (2004) 92:744–51. doi: 10.1016/j.ygyno.2003.11.048
67. Nout RA, Smit VT, Putter H, Jurgenliemk-Schulz IM, Jobsen JJ, Lutgens LC, et al. Vaginal brachytherapy versus pelvic external beam radiotherapy for patients with endometrial cancer of high-intermediate risk (PORTEC-2): an open-label, non-inferiority, randomised trial. Lancet (2010) 375:816–23. doi: 10.1016/S0140-6736(09)62163-2
68. Leon-Castillo A, de Boer SM, Powell ME, Mileshkin LR, Mackay HJ, Leary A, et al. Molecular classification of the PORTEC-3 trial for high-risk endometrial cancer: Impact on prognosis and benefit from adjuvant therapy. J Clin Oncol (2020) 38:3388–97. doi: 10.1200/JCO.20.00549
69. Song YP, Mistry H, Irlam J, Valentine H, Yang L, Lane B, et al. Long-term outcomes of radical radiation therapy with hypoxia modification with biomarker discovery for stratification: 10-year update of (Bladder carbogen nicotinamide) phase 3 randomized trial (ISRCTN45938399). Int J Radiat Oncol Biol Phys (2021) 110:1407–15. doi: 10.1016/j.ijrobp.2021.03.001
70. Noman MZ, Hasmim M, Messai Y, Terry S, Kieda C, Janji B, et al. Hypoxia: a key player in antitumor immune response. a review in the theme: Cellular responses to hypoxia. Am J Physiol Cell Physiol (2015) 309:C569–579. doi: 10.1152/ajpcell.00207.2015
71. Wilkinson-Ryan I, Frolova AI, Liu J, Stewart Massad L, Thaker PH, Powell MA, et al. Neoadjuvant chemotherapy versus primary cytoreductive surgery for stage IV uterine serous carcinoma. Int J Gynecol Cancer (2015) 25:63–8. doi: 10.1097/IGC.0000000000000321
72. de Lange NM, Ezendam NPM, Kwon JS, Vandenput I, Mirchandani D, Amant F, et al. Neoadjuvant chemotherapy followed by surgery for advanced-stage endometrial cancer. Curr Oncol (2019) 26:e226–32. doi: 10.3747/co.26.4655
73. Khouri OR, Frey MK, Musa F, Muggia F, Lee J, Boyd L, et al. Neoadjuvant chemotherapy in patients with advanced endometrial cancer. Cancer Chemother Pharmacol (2019) 84:281–5. doi: 10.1007/s00280-019-03838-x
74. Philp L, Kanbergs A, Laurent JS, Growdon WB, Feltmate C, Goodman A. The use of neoadjuvant chemotherapy in advanced endometrial cancer. Gynecol Oncol Rep (2021) 36:100725. doi: 10.1016/j.gore.2021.100725
75. Fjeldbo CS, Julin CH, Lando M, Forsberg MF, Aarnes EK, Alsner J, et al. Integrative analysis of DCE-MRI and gene expression profiles in construction of a gene classifier for assessment of hypoxia-related risk of chemoradiotherapy failure in cervical cancer. Clin Cancer Res (2016) 22:4067–76. doi: 10.1158/1078-0432.CCR-15-2322
76. Fleming IN, Manavaki R, Blower PJ, West C, Williams KJ, Harris AL, et al. Imaging tumour hypoxia with positron emission tomography. Br J Cancer (2015) 112:238–50. doi: 10.1038/bjc.2014.610
77. Vaupel P, Thews O, Hoeckel M. Treatment resistance of solid tumors: role of hypoxia and anemia. Med Oncol (2001) 18:243–59. doi: 10.1385/MO:18:4:243
78. Shukla-Dave A, Obuchowski NA, Chenevert TL, Jambawalikar S, Schwartz LH, Malyarenko D, et al. Quantitative imaging biomarkers alliance (QIBA) recommendations for improved precision of DWI and DCE-MRI derived biomarkers in multicenter oncology trials. J Magn Reson Imaging (2019) 49:e101–21. doi: 10.1002/jmri.26518
79. O'Connor JP, Aboagye EO, Adams JE, Aerts HJ, Barrington SF, Beer AJ, et al. Imaging biomarker roadmap for cancer studies. Nat Rev Clin Oncol (2017) 14:169–86. doi: 10.1038/nrclinonc.2016.162
Keywords: hypoxia, endometrial cancer, magnetic resonance imaging (MRI), oxygen-enhanced MRI, molecular subtypes
Citation: deSouza NM, Choudhury A, Greaves M, O’Connor JPB and Hoskin PJ (2022) Imaging hypoxia in endometrial cancer: How and why should it be done? Front. Oncol. 12:1020907. doi: 10.3389/fonc.2022.1020907
Received: 16 August 2022; Accepted: 21 October 2022;
Published: 09 November 2022.
Edited by:
Alessio G. Morganti, University of Bologna, ItalyReviewed by:
Milly Buwenge, University of Bologna, ItalyCopyright © 2022 deSouza, Choudhury, Greaves, O’Connor and Hoskin. This is an open-access article distributed under the terms of the Creative Commons Attribution License (CC BY). The use, distribution or reproduction in other forums is permitted, provided the original author(s) and the copyright owner(s) are credited and that the original publication in this journal is cited, in accordance with accepted academic practice. No use, distribution or reproduction is permitted which does not comply with these terms.
*Correspondence: Nandita M. deSouza, nandita.desouza@icr.ac.uk