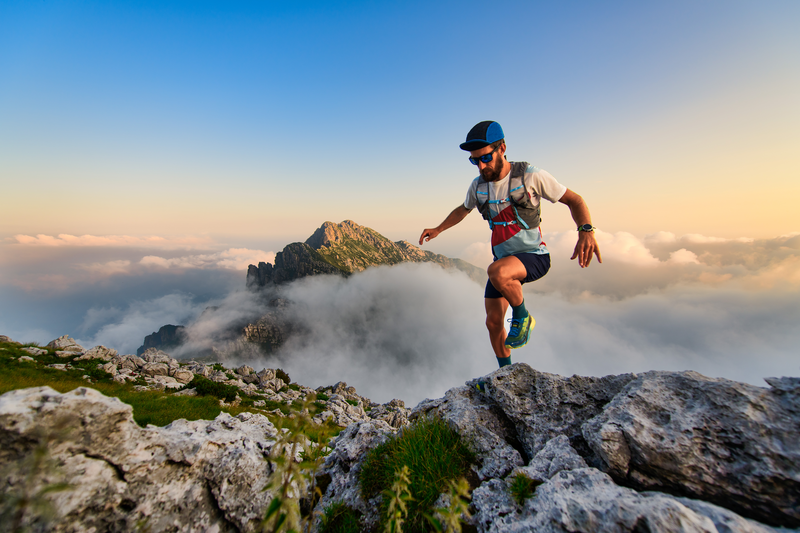
94% of researchers rate our articles as excellent or good
Learn more about the work of our research integrity team to safeguard the quality of each article we publish.
Find out more
REVIEW article
Front. Oncol. , 16 November 2022
Sec. Cancer Molecular Targets and Therapeutics
Volume 12 - 2022 | https://doi.org/10.3389/fonc.2022.1020675
Bladder cancer (BC) is a malignant disease with high rates of recurrence and mortality. It is mainly classified as non-muscle-invasive BC and muscle-invasive BC (MIBC). Often, MIBC is chemoresistant, which, according to cancer stem cells (CSCs) theory, is linked to the presence of bladder cancer stem cells (BCSCs). Sex-determining region Y- (SRY) Box transcription factor 2 (SOX2), which is a molecular marker of BCSCs, is aberrantly over-expressed in chemoresistant BC cell lines. It is one of the standalone prognostic factors for BC, and it has an inherently significant function in the emergence and progression of the disease. This review first summarizes the role of SRY-related high-mobility group protein Box (SOX) family genes in BC, focusing on the SOX2 and its significance in BC. Second, it discusses the mechanisms relevant to the regulation of SOX2. Finally, it summarizes the signaling pathways related to SOX2 in BC, suggests current issues to be addressed, and proposes potential directions for future research to provide new insights for the treatment of BC.
According to global data on new cases and deaths for all cancers combined in 2020, bladder cancer (BC) ranked 12th in the number of new cases of all cancers, with 573,278 new cases (1). Smoking and long-term exposure to chemical raw materials are two recognized pathogenic factors (2, 3). Bladder cancer is a highly heterogeneous malignant disease that is mainly classified as non-muscle-invasive BC (NMIBC; stages Ta, T1) and muscle-invasive BC (MIBC; stages ≥ T2) (4). Approximately 70% of BC cases diagnosed as NMIBC have a low risk of progression to MIBC, but NMIBC is prone to recurrence. Conversely, uroepithelial flat lesions and carcinoma in situ are very vulnerable to progression to MIBC (5). Although some advanced DNA methylation-based urine tests can diagnose BC early and give early treatment, the outcome of BC is still unsatisfactory (6, 7). The main treatments for BC include surgery, chemotherapy and immunotherapy (8). Transurethral resection of bladder tumor combined with bladder perfusion is the main treatment for NMIBC patients (9). Neoadjuvant chemotherapy combined with radical total cystectomy is the standard of treatment for patients with MIBC (10). However, cisplatin-based neoadjuvant chemotherapy (NAC) often causes drug resistance in MIBC patients (11). The use of immune checkpoint inhibitors approved by the US Food and Drug Administration has been unsatisfactory due to low response rates (12). Recent studies report key genes and regulatory pathways in lymphatic metastasis of BC that enhances the anti-tumor effect of cisplatin or immunotherapy (13, 14). Therefore, the development of targeted drugs may provide new clinical strategies to overcome BC chemoresistance.
There is a clear correlation between the aberrant expression of sex-determining region Y (SRY) associated high-mobility group (HMG) Box (SOX) transcription factors and carcinomas (15). Numerous studies have demonstrated that several members of the SOX family act as key regulators of tumor cells, which typically mediate the initiation of neoplasms and enhance tumorigenesis and proliferation (16). The SOX family transcription factors modulate tumor cell proliferation, metastasis, stemness, epithelial–mesenchymal transition (EMT), and drug resistance via multiple signaling pathways (17). Sex-determining region Y-Box transcription factor 2 (SOX2)—a member of the SOXB1 subgroup—has an encoded product comprising 317 amino peptides. The SOX2 gene, which is located on chromosome 3 at locus q26.3-q27, is a single exon and intronless gene with a core structure of a highly conserved HMG structural domain that intercalates with specific DNA sequences (18). In addition, SOX2 and its family members all possess C-terminal trans-activating structural domains, which act to recognize and bind to the promoter regions of target genes, thereby activating or repressing gene expression (19) (Figure 1).
Figure 1 Schematic illustration of structures of the Sox gene family involved in human bladder cancer. SOX2 belongs to SOX family group B1, SOX4 belongs to SOX family group C, SOX9 and SOX10 belong to SOX family group E. All family members have the DNA-binding HMG domain in common. (HMG, High-mobility group box domain; TAD, Transactivation domain; DD, Dimerization domain).
This article outlines the link between SOX family members and BC, highlights the SOX2 as a topic of discussion, and summarizes the mechanisms and signaling pathways that mediate SOX2 expression to provide new concepts for the management of BC.
The proteins of the SOX family are involved in embryonic development processes, such as cell differentiation and organ formation, and are linked to the maintenance of stem cells in adult tissues (20). In healthy organisms, gene expression is regulated precisely. However, in tumors, SOX genes are often dysregulated at the transcriptional, translational, and post-translational levels, and the aberrant activation of SOX genes is an important factor in tumorigenesis and progression (17). The SOX family is divided into 8 groups (A–H), with a total of more than 20 family members. In different tumor environments, SOX genes can function as oncogenes or tumor suppressor genes. For example, SOX10 is upregulated in BC and breast cancer and downregulated in colorectal cancer (21). In most tumors, SOX2, SOX4, SOX5, SOX8, and SOX9 act as oncogenes. In BC, the expression of the SOX2, SOX4, and SOX10 is upregulated, while the expression of the SOX9 is downregulated (22) (Figure 1).
The SOX4 gene is a member of the SOXC subgroup, and its expression is upregulated in BC (23). Studies have reported SOX4 upregulation to be significantly correlated with the grade, invasiveness, and poor outcome of BC (24). SOX4 is expressed in BC cell lines RT-114, J82, and 5637. Knocking down the expression of SOX4 can significantly inhibit the proliferation, metastasis, and stemness of BC cells (24, 25). The expression of the SOX10 gene—a member of the SOXE subgroup—is also upregulated in BC, and the aberrant overexpression of the SOX10 protein is an independent predictive factor for overall survival in BC. In the T24 and 5637 BC cell lines, the expression of SOX10 can promote the proliferation, migration, and invasion of BC cells (26). The expression of the SOX9 gene is upregulated in the majority of carcinomas, whereas in BC, SOX9 expression is inhibited. It has been reported that SOX9 promoter methylation is upregulated in BC and is significantly associated with BC grade and overall survival. The DNA methyltransferase inhibitor (DNMTi) 5-azacytidine (AZA) treated the BC cell line, and the J82 methylated cell line restored the expression of SOX9 at the transcriptional level compared with the RT4 unmethylated cell line (27). Finally, a single-cell sequencing analysis reported that SOX9 expression was higher in muscle-invasive tumors compared to non-muscle-invasive tumors (28). This section summarizes the essential roles of three members of the SOX family in the development and metastasis of BC by describing studies on SOX4, SOX10, and SOX9 in BC. Subsequently, we will focus our discussion on SOX2.
With the continued use of drugs, some resistant cell populations selectively survive in tumors, leading to the recurrence of carcinoma, and cancer stem cells (CSCs) are considered as significant contributors to this process (29). Cancer stem cells are a class of undifferentiated cell populations with stem cell characteristics that, in a hierarchical model, sit on the apex of tumor cells; furthermore, they can generate heterogeneous tumor cells and form tumors (30). The characteristics of BCSCs include slow growth and a tendency for dormancy. For slowly dividing cells, chemotherapy based on the DNA damage mechanism has little effect (31). Therefore, exploring key molecules representing the properties of BCSCs is particularly important.
In recent years, CSCs have been demonstrated in and isolated from various solid tumors, such as breast cancer (31). In 2008, She et al. (32) first identified side population (SP) cells (a subset of CSC-enriched subpopulations with the ability to divide asymmetrically, self-renew, and regulate tumor initiation in BC) using DyeCycle Violet reagent (DCV) staining. Subsequently, Yang et al. (33) separated a subset of surface markers CD44v6+/EMA− cells with enhanced capabilities of colony formation, self-renewal, and proliferation. In 2009, Chan et al. (34) isolated a CD44+/CK5+/CK20− cell subset in MIBC that was more tumorigenic in xenograft tumors compared to the CD44-/CK5-/CK20+ subset. In 2013, Peng et al. (35) identified BCSCs in the J82 cell line based on the CD133 cell surface marker. In contrast with the CD133− cell population, the CD133+ cell population was more invasive, tumorigenic, and radiotherapy/chemotherapy resistant. Furthermore, the expression of embryonic stem (ES) cell-related genes SOX2 and Octamer-binding transcription factor 4 (OCT4) was abnormally upregulated in the CD133+ population (35).
In poorly differentiated tumors, ES cell marker genes, such as SOX2, OCT4, and c-MYC, are preferentially aberrantly activated (36). Hepburn et al. (37) reported the characterization of ATP-binding cassette (ABC) superfamily G member 2 (ABCG2) in multiple BC cell lines and NMIBC samples along with the successful isolation of SP cells with high ABCG2 expression. Compared with non-SP cells with low ABCG2 expression, SOX2 gene enrichment was increased in the SP cells, and stronger colony-forming ability was exhibited. Although there may exist a regulatory relationship between the expression of ABCG2 and SOX2, it is not elaborated in the text. In 2017, Zhu et al. (38) obtained SOX2+ cell subsets via flow cytometry and found that these subsets also expressed BCSC markers Keratin-14 and CD44v6. They also found that a subset of SOX2+ cells maintained BC progression, and the ablation of this subset led to tumor regression; thus, SOX2 is also a marker for BCSCs (38). Most of the markers were identified based on the cell surface markers of SP, and it was confirmed that SP is enriched in BCSCs both in vivo and in vitro (39). It has been suggested that the aberrant activation of these pluripotent markers could be involved in therapeutic resistance mechanisms in carcinoma (40). Therefore, it is increasingly apparent that effective therapies are required to target the markers of BCSCs to address the issue of BC chemotherapy resistance. In this regard, SOX2 is a potential target, and it has been implicated in the promotion of BC progression and chemoresistance.
In normal human tissues, the role of transcription factor SOX2 is to maintain the self-renewal of embryonic stem cells and generate induced pluripotent stem cells (41). The upregulation of SOX2 has been detected in small-cell lung cancer and cancers of the prostate, colon, breast, and esophagus (42–46). In BC, the SOX2 gene is abnormally activated. According to different stages and grades, the expression frequency of SOX2 varies. The expression frequency of SOX2 in MIBC and high-grade NMIBC is higher than in low-grade NMIBC (47).
In prognosis, Ruan et al. (48) revealed that in an NMIBC cohort, SOX2 expression was significantly correlated with tumor size, number, and histological grade. Recurrence-free survival was higher in patients with low SOX2 expression compared to those with high SOX2 expression, and the difference was statistically significant. Multivariate cox regression analysis indicated that SOX2 is an independent prognostic factor for recurrence-free survival in patients with stage-T1 BC. Based on immunohistochemical results in the MIBC patient cohort, Matias et al. (49) took a different view; cytosolic staining for SOX2 was not associated with patient prognosis; differences in SOX2 cytoplasmic expression were not associated with patient histological grade; and weak staining in the cytoplasm predicted a poorer prognosis, but this difference was not statistically significant.
With regard to treatment outcome, high SOX2 expression was strongly associated with chemoresistance. A higher proportion of patients in the chemoresistant group had high SOX2 expression compared to those in the pre-NAC chemo-sensitive group, and a higher proportion of patients in the post-NAC chemoresistant group had high SOX2 expression compared to those in the pre-NAC chemoresistant group (49). However, the sample size of the MIBC cohort studied by Matias’ team was small and the differences were not statistically significant, so their results were not sufficiently convincing.
SOX2 is an essential transcription factor, and due to the lack of a suitable binding site, it is almost impossible to directly regulate by inhibitors (38, 50). In most current studies, the expression of SOX2 is indirectly regulated by modulating the expression of a certain protein or the conduction of a certain signaling pathway (28, 39, 51, 52). In this section, various mechanisms regulating SOX2 expression are discussed.
In eukaryotes, not all RNAs are translated into proteins, and non-coding RNAs (ncRNAs) are a category of RNAs that do not encode proteins. Such RNAs are classified as small ncRNAs and long ncRNAs; small ncRNAs include ribosomal RNA, transfer RNA, and microRNA (miRNA) (53). Evidence suggests that lncRNAs and miRNAs drive the occurrence and progression of human carcinomas by mediating the transcriptional and post-transcriptional levels of genes, i.e. mRNA degradation and protein translation failure (54).
MicroRNA is about 21–23 nucleotides in length and regulates the proliferation, differentiation, and apoptosis of BC cells (55). In 2015, Tomomi (56) reported that the overexpression of miR-145 can inhibit the expression of syndecan-1 and induce abnormally high SOX2 expression in BC cells, thereby inducing BC cell differentiation. Primarily, miRNAs modulate the post-transcriptional levels of downstream target genes that suppress protein translation by complementing the bases of the 3’ untranslated region (3’UTR) of the target gene’s mRNA (57). It was demonstrated that the expression of miR-200c, which was upregulated in BC, complemented the 3’UTR of SOX2 mRNA, thereby inhibiting SOX2 transcription (58). In 2019, Wang et al. (59) reported that the rs2910164 single-nucleotide polymorphism in the miR-146a precursor regulated the expression of miR-146a. The target gene of miR-146a is COX2 mRNA, and COX2 regulated the expression of SOX2. The overexpression of miR-146a resulted in the downregulation of COX2 transcription, which in turn, downregulated the transcription and translation of the SOX2 (Table 1).
The COX2/PGE2 signaling pathway induces the methylation of the promoter of the let-7 gene and downregulates its expression. The high-mobility group AT-hook 2 (HMGA2) is the downstream target gene of let-7; HMGA2 binds to the promoter region of SOX2 and upregulates its expression, while let-7 complements the HMGA2 mRNA 3’UTR to inhibit HMGA2 translation, which in turn, inhibits the transcription and translation of the SOX2 gene (60) (Table 1).
In BC, several lncRNAs capable of regulating SOX2 expression have been reported, and their modulation of such expression can be summarized in two approaches: 1) lncRNA directly regulate SOX2 expression, and 2) lncRNA as miRNA sponges to regulate SOX2 expression (Figure 2).
Figure 2 The functions of non-coding RNAs. (A) lncRNA directly regulates SOX2 expression. (B) lncRNA and circRNA act as miRNA sponges to regulate SOX2 expression. (lncRNA, long-non coding RNA; circRNA, circularRNA; miRNA, microRNA). By Figdraw.
Chen et al. (61) reported that an lncRNA called Low was expressed in BCSCs (lnc-LBCS); it could regulate the expression of SOX2, bind to the SOX2 promoter, and upregulate the level of H3K27me3. Furthermore, lnc-LBCS regulates the expression of heterogeneous nuclear ribonucleoprotein K (hnRNPK) and zeste homolog 2 (EZH2). Long non-coding LBCS mediates the recruitment of the hnRNPK-EZH2 complex to the SOX2 promoter, inducing H3K27me3 levels and thereby repressing SOX2 transcription and translation (61) (Figure 2A). A recent study confirmed this regulation, and the AK023096 lncRNA also promoted SOX2 expression by recruiting hnRNPK to mediate the methylation of the SOX2 promoter (62) (Figure 2A). It has been shown that lncRNA can function as miRNA sponge in cancer cells, attaching to miRNAs as a competing endogenous RNA (ceRNA), thus relieving the inhibitory effect of miRNAs on their target genes (63). In 2020, Zhan et al. (64) showed that SOX2-overlapping transcript (SOX2OT) is an lncRNA located in the intron region of SOX2, which is dominantly detected in the cytoplasm of BC cells. The expression of SOX2OT is aberrantly activated in BC and upregulates SOX2 expression. The knockdown of SOX2OT expression upregulates miR200-c expression, and silencing the expression of miR200-c reverses the downregulation of SOX2 (64) (Figure 2B). Consequently, SOX2OT upregulates SOX2 expression by sponging miR200-c in a ceRNA manner and inhibiting miR-200c complementation with the SOX2 mRNA 3’UTR (Table 1).
Circular RNA (circRNA) contain abundant miRNA binding sites and can act as miRNA sponges to regulate the post-transcriptional levels of their target genes (65) (Figure 2B). Lin et al. (66) demonstrated that the expression of circRNA, which is circularized from exons 9–15 of the filament protein A (FLNA) gene (circFLNA), is downregulated in BC. The overexpression of circFLNA resulted in the significant downregulation of SOX2 expression. The target gene of circFLNA was miR-216a-3p, and the overexpression of miR-216a-3p upregulated the expression of SOX2. Their studies also revealed that circFLNA served as miRNA sponge and competitively adsorbed miR-216a-3p, thereby upregulating B-cell translocation gene 2 (BTG2) expression (66) (Table 1). In their publication, SOX2 was positively regulated by miR-216a-3p, but the specific mechanism was not elucidated. BTG2 was a downstream target of miR-216a-3p, and whether the expression of SOX2 was regulated by BTG2 was not covered.
Investigations have revealed that EMT is involved in cancer invasion, metastasis, and drug resistance, and it is associated with poor prognosis in BC (67). Transforming growth factor β1 (TGF-β1), an EMT inducer, induced EMT in HTB-9 cells and upregulated the expression of SOX2 (68). Romaila et al. (69) reported that Escherichia coli caused EMT induction in T24 cells and upregulated SOX2 expression. Migita et al. demonstrated that TGF-β1 induced EMT in MIBC (i.e., UM-UC-3, T24) and NMIBC- (RT-4, JTC-30) derived cells and confirmed that in the latter, EMT significantly upregulated the expression of SOX2 (70). Pan et al. (52) reported that the dissociation of the OCT4–SOX2 compound in BC contributed to specific differentiation signals induced by EMT. In addition, the knockdown of SOX2 expression in 5637 cells inhibited EMT (71). In part, EMT promotes the expression of marker genes for CSCs, such as SOX2 in BC cells, and in turn, the dissociation of SOX2 promotes EMT in BC cells.
N6-methyladenosin (m6A) is the most prevalent modification in higher organism mRNAs. Modifications of m6A have an influential role in the induction of BC occurrence and invasiveness (72). It was suggested that methyltransferase-like 3 (METTL3) upregulates the m6A modification of AF4/FMR2 family member 4 (AFF4) mRNA and upregulates the expression of AFF4, which in turn binds directly to the SOX2 DNA promoter region and regulates the transcription of SOX2 (73). Both MYC and SOX2 are major regulators of self-renewal and tumorigenicity in CSCs, and METTL3 directly regulates m6A modifications on MYC mRNA and upregulates MYC transcription (74). Therefore, METTL3 may mediate SOX2 expression at both the transcriptional and post-transcriptional levels, potentially affecting BC genesis and invasion.
ChlA-F, a conformational derivative of Cheliensisin A, promotes the degradation of the SOX2 protein rather than inhibiting the transcription of SOX2. Further studies found that ChlA-F treatment upregulated both the transcription and translation of ubiquitin specific peptidase 8 (USP8) and that the knockdown of USP8 reversed the downregulation of SOX2 resulting from ChlA-F treatment. Additionally, ChlA-F induced the expression of HuR, which binds to USP8 mRNA and increases its stability. Therefore, ChlA-F promotes the ubiquitination and protein degradation of SOX2 by inducing HuR expression, whereby it upregulates the expression of USP8 and acts as an E3 ligase (58). ChlA-F regulates not only SOX2 transcription but also the stability of the SOX2 protein.
In recent years, although considerable improvements have been achieved in the treatment of BC, the mortality rate due to chemotherapy resistance has increased, again confirming that the development of novel targeted drugs is an effective treatment for BC. In this section, the role of SOX2 in BC treatment is summarized, and potential therapeutic strategies that target SOX2-related signaling pathways are discussed.
Among intravesical instillations after TURBt, Bacillus Calmette–Guérin (BCG) is the most successful method to treat and prevent recurrence or progression of NMIBC (2, 8). Peng et al. showed that the SOX2-expressing CD133+ cell population was more resistant to BCG treatment compared to the CD133- J82 population (35). However, the correlation of this resistance effect with SOX2 has not been reported. In addition, the complex comorbidities and side effects caused by BCG can severely affect patients’ quality of life and are not suitable for all patients (75). The approved application of immune checkpoint inhibitors alleviates this situation, with inhibition of programmed cell death protein-1/programmed cell death protein ligand 1 (PD-1/PD-L1) and cytotoxic T-cell antigen (CTLA4) being the main immune checkpoints (76). Recent study showed that SOX2 expression might be associated with PD-L1 expression. BC cells co-cultured with macrophages form tumor-hybrid cells (THC) with immunomodulatory ability (77). SOX2 expression was more abundant in THC compared to BC cells. Treatment of THC with phenylbutyrate, an immunomodulator and antitumor compound, downregulated SOX2 expression and upregulated PD-L1 expression simultaneously (78). However, the exact mechanism involved is unclear, and no relevant references have reported an association between SOX2 and response rates to immune checkpoint inhibitors.
Mitogen-activated protein kinase (MAPK) is widely expressed in multicellular organisms and has significant function in cell proliferation, differentiation, migration, and invasion (79). It can be classified into four subfamilies, namely, ERK, p38, JNK, and ERK5; each of these represents a pathway, with the ERK and JNK signaling pathways being the most deeply interconnected with BC (80). Hepburn et al. (37) reported that phosphorylated ERK (pERK) is aberrantly activated in SP cells and that MEK-specific inhibitors significantly suppress the colony-forming ability of such cells. Mitogen-activated protein kinase signaling pathways may be linked to the maintenance of marker genes in BCSCs. However, their study was limited by the mechanism. Hui et al.’s research (71) revealed the link between the MAPK/ERK signaling pathway and the expression of SOX2. RASAL2, a RAS GTPase-activating protein (RAS GAP), which is downregulated in BC tissues and cells and regulates the spheroid and colony-forming abilities of BC cells. It was reported that the knockdown of RASAL2 resulted in the upregulation of pERK expression, while the overexpression of RASAL2 resulted in its downregulation. Their studies also showed that MEK-specific inhibitors significantly downregulated SOX2 expression in BC cells and inhibited spheroid and colony-forming abilities (71) (Figure 3A). Therefore, RASAL2 inhibits SOX2 expression via the MAPK/ERK signaling pathway, which in turn, inhibits BC cell migration and stemness.
Figure 3 SOX2-related signaling pathway in bladder cancer. (A) MAPK/ERK signaling pathway. (B) MAPK/JNK signaling pathway. (C) SOX2-IGF2-AKT signaling pathway. (D) COX2/PGE2 Axis and YAP1-SOX2 signaling pathway. “P” stands for phosphorylation. By Figdraw.
ChlA-F selectively induces JNK and c-Jun phosphorylation and upregulates miR200-c transcription, which inhibits SOX2 protein translation and suppresses BC cell invasion (58) (Figure 3B). Therefore, targeting the ERK and JNK signaling pathways to inhibit the expression of SOX2 may be a potential target for BC therapy.
Tannic acid (TA) significantly inhibited BC cell viability and suppressed SOX2 expression (81). Further studies revealed that the treatment of cells with TA inhibited the AKT phosphorylation (pAKT) of Ser473 (81). Denise et al. (82) reported inducible nitric oxide synthase (iNOS) to be associated with the aggressiveness and recurrence of BC. The inhibition of iNOS expression significantly downregulated SOX2 expression and reduced matrix metallopeptidase 2 (MMP-2) activity in a mouse BC model (83). Evidence confirms that SOX2 induces both MMP-2 activity and the PI3K/AKT pathway involved in laryngeal cancer invasion (84). Thus, iNOS may regulate BC recurrence by upregulating SOX2 expression and inducing both MMP-2 activity and AKT phosphorylation.
Chiu et al. (85) clarified the underlying mechanism of SOX2 regulation of pAKT. In a low-serum culture, SOX2 maintained the viability of BC cells, and pAKT levels were upregulated in SOX2-expressing BC cells. IGF2 is a downstream gene of SOX2, which upregulates H3K4me3 in the IGF2 promoter. Thus, SOX2 upregulates pAKT of ser473 by inducing the expression of IGF2 and suppressing the expression of IGFBP1, thereby promoting the proliferation and invasion of BC cells (85) (Figure 3C). However, Wang et al. (86) reported that in esophageal cancer, pAKT upregulates SOX2 phosphorylation (pSOX2) at T116 and protects the SOX2 protein from ubiquitination degradation by Ubiquitin protein ligase E3 component n-recognin 5 (UBR5). It remains unknown if pAKT directly regulates the expression of SOX2 in BC, and the mechanism between AKT and SOX2 requires further investigation.
Arsenic is a widespread environmental contaminant and an essential factor in the pathogenesis of BC (87). In a long-term arsenic-induced (AS) in vitro model of the human normal urothelial cell line HUC1, the expression of SOX2 was upregulated in AS cells, and it was an integral and key factor for AS stem cell properties. Furthermore, the COX2 inhibitor was found to downregulate SOX2 expression, while PGE2 upregulated SOX2 expression. Therefore, SOX2 expression is regulated by COX2/PGE2 (88).
Due to the aberrant activation of the epidermal growth factor receptor (EGFR) pathway in BC, EGFR has been shown to be a potential target for the basal subtype of MIBC (89). The use of EGFR-targeted treatment resulted in the upregulation of both PGE2 and SOX2, which may be attributed to the enrichment of CSCs. These results indicate that the combined application of EGFR and COX2 inhibitors to AS cells resulted in the significant, but not complete, suppression of SOX2 expression compared with treatment with EGFR inhibitors alone (88). This is explained in a separate report by Ooki et al. (60), with Yes-associated protein 1 (YAP1) binding to the SOX2 enhancer region and upregulating SOX2 expression. The COX2 is the downstream target gene of YAP1, but YAP1 regulates SOX2 expression not exclusively via the COX2/PGE2 axis (88, 90). The YAP1–SOX2 and COX2/PGE2–SOX2 signaling pathways are modulated independently, although there is a negative feedback mechanism between the two (60) (Figure 3D). Additionally, EGFR upregulates YAP1 expression via the activation of the PI3K/AKT signaling pathway (91). Accordingly, due to the negative feedback regulation of COX2 inhibition, the combined inhibition of EGFR and COX2 is not accompanied by the upregulation of YAP1 expression (60). So, the strategy of co-inhibition boosted the short-term anti-tumor effect if the interaction between molecules and the potential for additional side effects is considered.
The primary roles of the Wnt/β-Catenin signaling pathway are the mediation of cell proliferation, metastasis, and differentiation (92). The Wnt/β-Catenin signaling pathway is aberrantly activated in BC and is associated with maintaining BC resistance to gemcitabine and paclitaxel (93). Guerrero et al. (94) reported SOX2 expression to be abnormally upregulated in the resistant HT1197 cell line compared with the 5637 cell line, which was not resistant to paclitaxel.
The aberrant activation of the hedgehog signaling pathway may be relevant to chemoresistance in BC (95). The expression of SOX2 was higher in T24 with cisplatin resistance than in WT T24 cells, inhibiting the expression of major hedgehog pathway markers; furthermore, SOX2 expression was also inhibited (96). Sonic hedgehog (Shh) is the main signaling protein of the hedgehog pathway, and BCSCs may originate from the basal urothelium expressing Shh (97). The Shh signaling pathway regulates BC cell EMT, which in turn, upregulates SOX2 expression (68). Although SOX2 is regulated by these classical pathways in BC cell lines with resistance, these reports do not explore the detailed mechanisms by which SOX2 governs BC chemoresistance. Accordingly, the study of genes downstream of SOX2 may be a future research direction.
Many studies have linked SOX2 with human carcinomas. The aberrantly high expression of SOX2 is associated with chemotherapy resistance in glioma, prostate cancer, rectal cancer, and other cancers. After the downregulation of SOX2 expression, tumors can recover their sensitivity to drugs (98). This review focused on the role of SOX2 in BC, with multiple reports describing the abnormally high expression of SOX2 in BC tissues, which is correlated with clinical grade and prognosis and is an independent prognostic factor for BC. Due to the special identity of SOX2 transcription factors, it is almost impossible to directly inhibit the expression of SOX2 via inhibitors. Numerous studies have reported that ncRNA, EMT, epigenetics, and signaling pathways mediate BC progression and chemoresistance by modulating the transcription or translation of SOX2, which has tremendous potential as a target for BC therapy. Notably, the effect mechanism of chemotherapeutic agents, such as cisplatin, is mainly via intracellular DNA structure, where it induces apoptosis. Under selective pressure, chemoresistance leads to an enrichment of CSCs in tumors, to some extent reinforcing drug resistance. As reported in the literature, the co-inhibition of multiple sites may be a new direction for the targeted modulation of SOX2, but it may cause additional side effects. While numerous studies on the regulation of SOX2 have been reported, only a few have examined the genes downstream of SOX2. Moreover, adult stem cells exist in various tissues of the human body and also express SOX2. Therefore, how to target and regulate the abnormally high expression of SOX2 in BC without affecting the homeostasis of normal tissue stem cells may also be a crucial point to be addressed.
In summary, in-depth research into the mechanisms of BC drug resistance and the identification of suitable targets and regulatory pathways to improve the responsiveness are essential future research directions for improving the current status of BC therapeutics. Despite reports of several mechanisms and signaling pathways that mediate SOX2, none have been used in clinical trials. Thus, there is still a long way to go in the field of BC targeted therapy. Complicated interactions between signaling pathways, protein-signaling pathways, and protein–protein interactions pose numerous obstacles. Exploring the critical pathways that regulate drug resistance in BC and developing precisely targeted drugs for clinical trials are needed for the further development of targeted therapies.
GC searched for literature and wrote the first draft of this article. YC and GC edited tables and figures. RX, XZ and GW reviewed the manuscript and polished the grammar. All authors contributed to the article and approved the submitted version.
This work was supported by the National Natural Science Foundation of China (No. 81860456); Key Project of Key Research and Development Plan of Jiangxi Province (Grant No. 20212BBG71013).
The authors declare that the research was conducted in the absence of any commercial or financial relationships that could be construed as a potential conflict of interest.
All claims expressed in this article are solely those of the authors and do not necessarily represent those of their affiliated organizations, or those of the publisher, the editors and the reviewers. Any product that may be evaluated in this article, or claim that may be made by its manufacturer, is not guaranteed or endorsed by the publisher.
1. Sung H, Ferlay J, Siegel RL, Laversanne M, Soerjomataram I, Jemal A, et al. Global cancer statistics 2020: Globocan estimates of incidence and mortality worldwide for 36 cancers in 185 countries. CA: Cancer J Clin (2021) 71(3):209–49. doi: 10.3322/caac.21660
2. Lenis AT, Lec PM, Chamie K. Bladder cancer. Jama (2020) 324(19):2006. doi: 10.1001/jama.2020.17601
3. van Osch FH, Jochems SH, van Schooten FJ, Bryan RT, Zeegers MP. Quantified relations between exposure to tobacco smoking and bladder cancer risk: A meta-analysis of 89 observational studies. Int J Epidemiol (2016) 45(3):857–70. doi: 10.1093/ije/dyw044
4. Abugomaa A, Elbadawy M, Yamawaki H, Usui T, Sasaki K. Emerging roles of cancer stem cells in bladder cancer progression, tumorigenesis, and resistance to chemotherapy: A potential therapeutic target for bladder cancer. Cells (2020) 9(1):235. doi: 10.3390/cells9010235
5. Seidl C. Targets for therapy of bladder cancer. Semin Nucl Med (2020) 50(2):162–70. doi: 10.1053/j.semnuclmed.2020.02.006
6. Chen X, Zhang J, Ruan W, Huang M, Wang C, Wang H, et al. Urine DNA methylation assay enables early detection and recurrence monitoring for bladder cancer. J Clin Invest (2020) 130(12):6278–89. doi: 10.1172/jci139597
7. Ruan W, Chen X, Huang M, Wang H, Chen J, Liang Z, et al. A urine-based DNA methylation assay to facilitate early detection and risk stratification of bladder cancer. Clin Epigenet (2021) 13(1):91. doi: 10.1186/s13148-021-01073-x
8. DeGeorge KC, Holt HR, Hodges SC. Bladder cancer: Diagnosis and treatment. Am Family physician (2017) 96(8):507–14. Available at: https://www.aafp.org/pubs/afp/issues/2017/1015/p507.html
9. Babjuk M, Burger M, Capoun O, Cohen D, Compérat EM, Dominguez Escrig JL, et al. European Association of urology guidelines on non-Muscle-Invasive bladder cancer (Ta, T1, and carcinoma in situ). Eur Urol (2022) 81(1):75–94. doi: 10.1016/j.eururo.2021.08.010
10. Patel VG, Oh WK, Galsky MD. Treatment of muscle-invasive and advanced bladder cancer in 2020. CA: Cancer J Clin (2020) 70(5):404–23. doi: 10.3322/caac.21631
11. Galluzzi L, Senovilla L, Vitale I, Michels J, Martins I, Kepp O, et al. Molecular mechanisms of cisplatin resistance. Oncogene (2012) 31(15):1869–83. doi: 10.1038/onc.2011.384
12. Baumann BC, Zaghloul MS, Sargos P, Murthy V. Adjuvant and neoadjuvant radiation therapy for locally advanced bladder cancer. Clin Oncol (Royal Coll Radiologists (Great Britain)) (2021) 33(6):391–9. doi: 10.1016/j.clon.2021.03.020
13. Huang M, Dong W, Xie R, Wu J, Su Q, Li W, et al. Hsf1 facilitates the multistep process of lymphatic metastasis in bladder cancer Via a novel Prmt5-Wdr5-Dependent transcriptional program. Cancer Commun (London England) (2022) 42(5):447–70. doi: 10.1002/cac2.12284
14. Zhang J, Zhou Q, Xie K, Cheng L, Peng S, Xie R, et al. Targeting wd repeat domain 5 enhances chemosensitivity and inhibits proliferation and programmed death-ligand 1 expression in bladder cancer. J Exp Clin Cancer Res CR (2021) 40(1):203. doi: 10.1186/s13046-021-01989-5
15. Abadi AJ, Zarrabi A, Hashemi F, Zabolian A, Najafi M, Entezari M, et al. The role of sox family transcription factors in gastric cancer. Int J Biol macromolecules (2021) 180:608–24. doi: 10.1016/j.ijbiomac.2021.02.202
16. Kumar P, Mistri TK. Transcription factors in sox family: Potent regulators for cancer initiation and development in the human body. Semin Cancer Biol (2020) 67(Pt 1):105–13. doi: 10.1016/j.semcancer.2019.06.016
17. Xu YR, Yang WX. Sox-mediated molecular crosstalk during the progression of tumorigenesis. Semin Cell Dev Biol (2017) 63:23–34. doi: 10.1016/j.semcdb.2016.07.028
18. Stevanovic M, Zuffardi O, Collignon J, Lovell-Badge R, Goodfellow P. The cdna sequence and chromosomal location of the human Sox2 gene. Mamm Genome Off J Int Mamm Genome Soc (1994) 5(10):640–2. doi: 10.1007/bf00411460
19. Novak D, Hüser L, Elton JJ, Umansky V, Altevogt P, Utikal J. Sox2 in development and cancer biology. Semin Cancer Biol (2020) 67(Pt 1):74–82. doi: 10.1016/j.semcancer.2019.08.007
20. She ZY, Yang WX. Sox family transcription factors involved in diverse cellular events during development. Eur J Cell Biol (2015) 94(12):547–63. doi: 10.1016/j.ejcb.2015.08.002
21. Castillo SD, Sanchez-Cespedes M. The sox family of genes in cancer development: Biological relevance and opportunities for therapy. Expert Opin Ther Targets (2012) 16(9):903–19. doi: 10.1517/14728222.2012.709239
22. Grimm D, Bauer J, Wise P, Krüger M, Simonsen U, Wehland M, et al. The role of sox family members in solid tumours and metastasis. Semin Cancer Biol (2020) 67(Pt 1):122–53. doi: 10.1016/j.semcancer.2019.03.004
23. Aaboe M, Birkenkamp-Demtroder K, Wiuf C, Sørensen FB, Thykjaer T, Sauter G, et al. Sox4 expression in bladder carcinoma: Clinical aspects and in vitro functional characterization. Cancer Res (2006) 66(7):3434–42. doi: 10.1158/0008-5472.Can-05-3456
24. Shen H, Blijlevens M, Yang N, Frangou C, Wilson KE, Xu B, et al. Sox4 expression confers bladder cancer stem cell properties and predicts for poor patient outcome. Int J Biol Sci (2015) 11(12):1363–75. doi: 10.7150/ijbs.13240
25. Liao C, Long Z, Zhang X, Cheng J, Qi F, Wu S, et al. Lncarsr sponges mir-129-5p to promote proliferation and metastasis of bladder cancer cells through increasing Sox4 expression. Int J Biol Sci (2020) 16(1):1–11. doi: 10.7150/ijbs.39461
26. Yin H, Qin C, Zhao Y, Du Y, Sheng Z, Wang Q, et al. Sox10 is over-expressed in bladder cancer and contributes to the malignant bladder cancer cell behaviors. Clin Trans Oncol Off Publ Fed Spanish Oncol Societies Natl Cancer Institute Mexico (2017) 19(8):1035–44. doi: 10.1007/s12094-017-1641-2
27. Aleman A, Adrien L, Lopez-Serra L, Cordon-Cardo C, Esteller M, Belbin TJ, et al. Identification of DNA hypermethylation of Sox9 in association with bladder cancer progression using cpg microarrays. Br J Cancer (2008) 98(2):466–73. doi: 10.1038/sj.bjc.6604143
28. Lai H, Cheng X, Liu Q, Luo W, Liu M, Zhang M, et al. Single-cell rna sequencing reveals the epithelial cell heterogeneity and invasive subpopulation in human bladder cancer. Int J Cancer (2021) 149(12):2099–115. doi: 10.1002/ijc.33794
29. Najafi M, Mortezaee K, Majidpoor J. Cancer stem cell (Csc) resistance drivers. Life Sci (2019) 234:116781. doi: 10.1016/j.lfs.2019.116781
30. Kreso A, Dick JE. Evolution of the cancer stem cell model. Cell Stem Cell (2014) 14(3):275–91. doi: 10.1016/j.stem.2014.02.006
31. Ohishi T, Koga F, Migita T. Bladder cancer stem-like cells: Their origin and therapeutic perspectives. Int J Mol Sci (2015) 17(1):43. doi: 10.3390/ijms17010043
32. She JJ, Zhang PG, Wang ZM, Gan WM, Che XM. Identification of side population cells from bladder cancer cells by dyecycle violet staining. Cancer Biol Ther (2008) 7(10):1663–8. doi: 10.4161/cbt.7.10.6637
33. Yang YM, Chang JW. Bladder cancer initiating cells (Bcics) are among ema-Cd44v6+ subset: Novel methods for isolating undetermined cancer stem (Initiating) cells. Cancer Invest (2008) 26(7):725–33. doi: 10.1080/07357900801941845
34. Chan KS, Espinosa I, Chao M, Wong D, Ailles L, Diehn M, et al. Identification, molecular characterization, clinical prognosis, and therapeutic targeting of human bladder tumor-initiating cells. Proc Natl Acad Sci United States America (2009) 106(33):14016–21. doi: 10.1073/pnas.0906549106
35. Huang P, Watanabe M, Kaku H, Ueki H, Noguchi H, Sugimoto M, et al. Cancer stem cell-like characteristics of a Cd133(+) subpopulation in the J82 human bladder cancer cell line. Mol Clin Oncol (2013) 1(1):180–4. doi: 10.3892/mco.2012.29
36. Ben-Porath I, Thomson MW, Carey VJ, Ge R, Bell GW, Regev A, et al. An embryonic stem cell-like gene expression signature in poorly differentiated aggressive human tumors. Nat Genet (2008) 40(5):499–507. doi: 10.1038/ng.127
37. Hepburn AC, Veeratterapillay R, Williamson SC, El-Sherif A, Sahay N, Thomas HD, et al. Side population in human non-muscle invasive bladder cancer enriches for cancer stem cells that are maintained by mapk signalling. PLos One (2012) 7(11):e50690. doi: 10.1371/journal.pone.0050690
38. Zhu F, Qian W, Zhang H, Liang Y, Wu M, Zhang Y, et al. Sox2 is a marker for stem-like tumor cells in bladder cancer. Stem Cell Rep (2017) 9(2):429–37. doi: 10.1016/j.stemcr.2017.07.004
39. Fang D, Kitamura H. Cancer stem cells and epithelial-mesenchymal transition in urothelial carcinoma: Possible pathways and potential therapeutic approaches. Int J Urol Off J Japanese Urological Assoc (2018) 25(1):7–17. doi: 10.1111/iju.13404
40. Hepburn AC, Steele RE, Veeratterapillay R, Wilson L, Kounatidou EE, Barnard A, et al. The induction of core pluripotency master regulators in cancers defines poor clinical outcomes and treatment resistance. Oncogene (2019) 38(22):4412–24. doi: 10.1038/s41388-019-0712-y
41. Weina K, Utikal J. Sox2 and cancer: Current research and its implications in the clinic. Clin Trans Med (2014) 3:19. doi: 10.1186/2001-1326-3-19
42. Paschalis A, Sheehan B, Riisnaes R, Rodrigues DN, Gurel B, Bertan C, et al. Prostate-specific membrane antigen heterogeneity and DNA repair defects in prostate cancer. Eur Urol (2019) 76(4):469–78. doi: 10.1016/j.eururo.2019.06.030
43. Lundberg IV, Edin S, Eklöf V, Öberg Å, Palmqvist R, Wikberg ML. Sox2 expression is associated with a cancer stem cell state and down-regulation of Cdx2 in colorectal cancer. BMC Cancer (2016) 16:471. doi: 10.1186/s12885-016-2509-5
44. Leis O, Eguiara A, Lopez-Arribillaga E, Alberdi MJ, Hernandez-Garcia S, Elorriaga K, et al. Sox2 expression in breast tumours and activation in breast cancer stem cells. Oncogene (2012) 31(11):1354–65. doi: 10.1038/onc.2011.338
45. Ten Kate FJC, van Olphen SH, Bruno MJ, Wijnhoven BPL, van Lanschot JJB, Looijenga LHJ, et al. Loss of sry-Box2 (Sox2) expression and its impact on survival of patients with oesophageal adenocarcinoma. Br J Surg (2017) 104(10):1327–37. doi: 10.1002/bjs.10553
46. Karachaliou N, Rosell R, Viteri S. The role of Sox2 in small cell lung cancer, lung adenocarcinoma and squamous cell carcinoma of the lung. Trans Lung Cancer Res (2013) 2(3):172–9. doi: 10.3978/j.issn.2218-6751.2013.01.01
47. Amini S, Fathi F, Mobalegi J, Sofimajidpour H, Ghadimi T. The expressions of stem cell markers: Oct4, nanog, Sox2, nucleostemin, bmi, zfx, Tcl1, Tbx3, Dppa4, and esrrb in bladder, colon, and prostate cancer, and certain cancer cell lines. Anat Cell Biol (2014) 47(1):1–11. doi: 10.5115/acb.2014.47.1.1
48. Ruan J, Wei B, Xu Z, Yang S, Zhou Y, Yu M, et al. Predictive value of Sox2 expression in transurethral resection specimens in patients with T1 bladder cancer. Med Oncol (Northwood London England) (2013) 30(1):445. doi: 10.1007/s12032-012-0445-z
49. Blomqvist M, Koskinen I, Löyttyniemi E, Mirtti T, Boström PJ, Taimen P. Prognostic and predictive value of Aldh1, Sox2 and ssea-4 in bladder cancer. Sci Rep (2021) 11(1):13684. doi: 10.1038/s41598-021-93245-1
50. Gayyed MF, Tawfiek ER. Utility of Sox2 and livin Co-expression in the prognosis of bladder cancer with bilharzial and non-bilharzial bladder status. World J Oncol (2015) 6(5):446–55. doi: 10.14740/wjon942w
51. Iskender B, Izgi K, Karaca H, Canatan H. Myrtucommulone-a treatment decreases pluripotency- and multipotency-associated marker expression in bladder cancer cell line htb-9. J Natural Medicines (2015) 69(4):543–54. doi: 10.1007/s11418-015-0923-7
52. Pan X, Cang X, Dan S, Li J, Cheng J, Kang B, et al. Site-specific disruption of the Oct4/Sox2 protein interaction reveals coordinated mesendodermal differentiation and the epithelial-mesenchymal transition. J Biol Chem (2016) 291(35):18353–69. doi: 10.1074/jbc.M116.745414
53. Esteller M. Non-coding rnas in human disease. Nat Rev Genet (2011) 12(12):861–74. doi: 10.1038/nrg3074
54. Matsui M, Corey DR. Non-coding rnas as drug targets. Nat Rev Drug Discovery (2017) 16(3):167–79. doi: 10.1038/nrd.2016.117
55. Tan W, Liu B, Qu S, Liang G, Luo W, Gong C. Micrornas and cancer: Key paradigms in molecular therapy. Oncol Lett (2018) 15(3):2735–42. doi: 10.3892/ol.2017.7638
56. Fujii T, Shimada K, Tatsumi Y, Hatakeyama K, Obayashi C, Fujimoto K, et al. Microrna-145 promotes differentiation in human urothelial carcinoma through down-regulation of syndecan-1. BMC Cancer (2015) 15:818. doi: 10.1186/s12885-015-1846-0
57. Berindan-Neagoe I, Monroig Pdel C, Pasculli B, Calin GA. Micrornaome genome: A treasure for cancer diagnosis and therapy. CA: Cancer J Clin (2014) 64(5):311–36. doi: 10.3322/caac.21244
58. Hua X, Huang M, Deng X, Xu J, Luo Y, Xie Q, et al. The inhibitory effect of compound chla-f on human bladder cancer cell invasion can be attributed to its blockage of Sox2 protein. Cell Death differentiation (2020) 27(2):632–45. doi: 10.1038/s41418-019-0377-7
59. Wang T, Yang Y, Wang Z, Zhang X, Li D, Wei J. A snp of mir-146a is involved in bladder cancer relapse by affecting the function of bladder cancer stem cells Via the mir-146a signallings. J Cell Mol Med (2020) 24(15):8545–56. doi: 10.1111/jcmm.15480
60. Ooki A, Del Carmen Rodriguez Pena M, Marchionni L, Dinalankara W, Begum A, Hahn NM, et al. Yap1 and Cox2 coordinately regulate urothelial cancer stem-like cells. Cancer Res (2018) 78(1):168–81. doi: 10.1158/0008-5472.Can-17-0836
61. Chen X, Xie R, Gu P, Huang M, Han J, Dong W, et al. Long noncoding rna lbcs inhibits self-renewal and chemoresistance of bladder cancer stem cells through epigenetic silencing of Sox2. Clin Cancer Res an Off J Am Assoc Cancer Res (2019) 25(4):1389–403. doi: 10.1158/1078-0432.Ccr-18-1656
62. Zhao F, Qin S, Huang L, Ding L, Shi X, Zhong G. Long noncoding rna Ak023096 interacts with hnrnp-K and contributes to the maintenance of self-renewal in bladder cancer stem-like cells. Exp Cell Res (2021) 409(2):112909. doi: 10.1016/j.yexcr.2021.112909
63. Qi X, Zhang DH, Wu N, Xiao JH, Wang X, Ma W. Cerna in cancer: Possible functions and clinical implications. J Med Genet (2015) 52(10):710–8. doi: 10.1136/jmedgenet-2015-103334
64. Zhan Y, Chen Z, He S, Gong Y, He A, Li Y, et al. Long non-coding rna Sox2ot promotes the stemness phenotype of bladder cancer cells by modulating Sox2. Mol Cancer (2020) 19(1):25. doi: 10.1186/s12943-020-1143-7
65. Chen L, Wang C, Sun H, Wang J, Liang Y, Wang Y, et al. The bioinformatics toolbox for circrna discovery and analysis. Briefings Bioinf (2021) 22(2):1706–28. doi: 10.1093/bib/bbaa001
66. Lin S, Wang L, Shi Z, Zhu A, Zhang G, Hong Z, et al. Circular rna circflna inhibits the development of bladder carcinoma through microrna mir-216a-3p/Btg2 axis. Bioengineered (2021) 12(2):11376–89. doi: 10.1080/21655979.2021.2008659
67. McConkey DJ, Choi W, Marquis L, Martin F, Williams MB, Shah J, et al. Role of epithelial-to-Mesenchymal transition (Emt) in drug sensitivity and metastasis in bladder cancer. Cancer metastasis Rev (2009) 28(3-4):335–44. doi: 10.1007/s10555-009-9194-7
68. Islam SS, Mokhtari RB, Noman AS, Uddin M, Rahman MZ, Azadi MA, et al. Sonic hedgehog (Shh) signaling promotes tumorigenicity and stemness Via activation of epithelial-to-Mesenchymal transition (Emt) in bladder cancer. Mol carcinogenesis (2016) 55(5):537–51. doi: 10.1002/mc.22300
69. Abd-El-Raouf R, Ouf SA, Gabr MM, Zakaria MM, El-Yasergy KF, Ali-El-Dein B. Escherichia coli foster bladder cancer cell line progression Via epithelial mesenchymal transition, stemness and metabolic reprogramming. Sci Rep (2020) 10(1):18024. doi: 10.1038/s41598-020-74390-5
70. Migita T, Ueda A, Ohishi T, Hatano M, Seimiya H, Horiguchi SI, et al. Epithelial-mesenchymal transition promotes Sox2 and nanog expression in bladder cancer. Lab investigation; J Tech Methods Pathol (2017). 97(5):567–76 doi: 10.1038/labinvest.2017.17
71. Hui K, Gao Y, Huang J, Xu S, Wang B, Zeng J, et al. Rasal2, a ras gtpase-activating protein, inhibits stemness and epithelial-mesenchymal transition Via Mapk/Sox2 pathway in bladder cancer. Cell Death Dis (2017) 8(2):e2600. doi: 10.1038/cddis.2017.9
72. Liu Q. Current advances in N6-methyladenosine methylation modification during bladder cancer. Front Genet (2021) 12:825109. doi: 10.3389/fgene.2021.825109
73. Gao Q, Zheng J, Ni Z, Sun P, Yang C, Cheng M, et al. The M(6)a methylation-regulated Aff4 promotes self-renewal of bladder cancer stem cells. Stem Cells Int (2020) 2020:8849218. doi: 10.1155/2020/8849218
74. Cheng M, Sheng L, Gao Q, Xiong Q, Zhang H, Wu M, et al. The M(6)a methyltransferase Mettl3 promotes bladder cancer progression Via Aff4/Nf-Κb/Myc signaling network. Oncogene (2019) 38(19):3667–80. doi: 10.1038/s41388-019-0683-z
75. Larsen ES, Joensen UN, Poulsen AM, Goletti D, Johansen IS. Bacillus calmette-guérin immunotherapy for bladder cancer: A review of immunological aspects, clinical effects and bcg infections. APMIS Acta pathologica microbiologica immunologica Scandinavica (2020) 128(2):92–103. doi: 10.1111/apm.13011
76. Massari F, Di Nunno V, Cubelli M, Santoni M, Fiorentino M, Montironi R, et al. Immune checkpoint inhibitors for metastatic bladder cancer. Cancer Treat Rev (2018) 64:11–20. doi: 10.1016/j.ctrv.2017.12.007
77. Aguirre LA, Montalbán-Hernández K, Avendaño-Ortiz J, Marín E, Lozano R, Toledano V, et al. Tumor stem cells fuse with monocytes to form highly invasive tumor-hybrid cells. Oncoimmunology (2020) 9(1):1773204. doi: 10.1080/2162402x.2020.1773204
78. Rubio C, Avendaño-Ortiz J, Ruiz-Palomares R, Karaivanova V, Alberquilla O, Sánchez-Domínguez R, et al. Toward tumor fight and tumor microenvironment remodeling: Pba induces cell cycle arrest and reduces tumor hybrid cells' pluripotency in bladder cancer. Cancers (2022) 14(2):287. doi: 10.3390/cancers14020287
79. Yang M, Huang CZ. Mitogen-activated protein kinase signaling pathway and invasion and metastasis of gastric cancer. World J Gastroenterol (2015) 21(41):11673–9. doi: 10.3748/wjg.v21.i41.11673
80. Schulz GB, Elezkurtaj S, Börding T, Schmidt EM, Elmasry M, Stief CG, et al. Therapeutic and prognostic implications of notch and mapk signaling in bladder cancer. Cancer Sci (2021) 112(5):1987–96. doi: 10.1111/cas.14878
81. Chen MC, Annseles Rajula S, Bharath Kumar V, Hsu CH, Day CH, Chen RJ, et al. Tannic acid attenuate akt phosphorylation to inhibit Umuc3 bladder cancer cell proliferation. Mol Cell Biochem (2022). doi: 10.1007/s11010-022-04454-9
82. Belgorosky D, Langle Y, Prack Mc Cormick B, Colombo L, Sandes E, Eiján AM. Inhibition of nitric oxide is a good therapeutic target for bladder tumors that express inos. Nitric Oxide Biol Chem (2014) 36:11–8. doi: 10.1016/j.niox.2013.10.010
83. Belgorosky D, Girouard J, Langle YV, Hamelin-Morrissete J, Marino L, Agüero EI, et al. Relevance of inos expression in tumor growth and maintenance of cancer stem cells in a bladder cancer model. J Mol Med (Berlin Germany) (2020) 98(11):1615–27. doi: 10.1007/s00109-020-01973-0
84. Yang N, Hui L, Wang Y, Yang H, Jiang X. Sox2 promotes the migration and invasion of laryngeal cancer cells by induction of mmp-2 Via the Pi3k/Akt/Mtor pathway. Oncol Rep (2014) 31(6):2651–9. doi: 10.3892/or.2014.3120
85. Chiu YF, Wu CC, Kuo MH, Miao CC, Zheng MY, Chen PY, et al. Critical role of Sox2-Igf2 signaling in aggressiveness of bladder cancer. Sci Rep (2020) 10(1):8261. doi: 10.1038/s41598-020-65006-z
86. Wang Z, Kang L, Zhang H, Huang Y, Fang L, Li M, et al. Akt drives Sox2 overexpression and cancer cell stemness in esophageal cancer by protecting Sox2 from Ubr5-mediated degradation. Oncogene (2019) 38(26):5250–64. doi: 10.1038/s41388-019-0790-x
87. Saint-Jacques N, Parker L, Brown P, Dummer TJ. Arsenic in drinking water and urinary tract cancers: A systematic review of 30 years of epidemiological evidence. Environ Health Global Access Sci Source (2014) 13:44. doi: 10.1186/1476-069x-13-44
88. Ooki A, Begum A, Marchionni L, VandenBussche CJ, Mao S, Kates M, et al. Arsenic promotes the Cox2/Pge2-Sox2 axis to increase the malignant stemness properties of urothelial cells. Int J Cancer (2018) 143(1):113–26. doi: 10.1002/ijc.31290
89. Rebouissou S, Bernard-Pierrot I, de Reyniès A, Lepage ML, Krucker C, Chapeaublanc E, et al. Egfr as a potential therapeutic target for a subset of muscle-invasive bladder cancers presenting a basal-like phenotype. Sci Trans Med (2014) 6(244):244ra91. doi: 10.1126/scitranslmed.3008970
90. Bora-Singhal N, Nguyen J, Schaal C, Perumal D, Singh S, Coppola D, et al. Yap1 regulates Oct4 activity and Sox2 expression to facilitate self-renewal and vascular mimicry of stem-like cells. Stem Cells (Dayton Ohio) (2015) 33(6):1705–18. doi: 10.1002/stem.1993
91. Fan R, Kim NG, Gumbiner BM. Regulation of hippo pathway by mitogenic growth factors Via phosphoinositide 3-kinase and phosphoinositide-dependent kinase-1. Proc Natl Acad Sci United States America (2013) 110(7):2569–74. doi: 10.1073/pnas.1216462110
92. Nusse R, Clevers H. Wnt/Β-catenin signaling, disease, and emerging therapeutic modalities. Cell (2017) 169(6):985–99. doi: 10.1016/j.cell.2017.05.016
93. Goto T, Kashiwagi E, Jiang G, Nagata Y, Teramoto Y, Baras AS, et al. Estrogen receptor-Β signaling induces cisplatin resistance in bladder cancer. Am J Cancer Res (2020) 10(8):2523–34. Available at: https://e-century.us/files/ajcr/10/8/ajcr0116855.pdf
94. Jiménez-Guerrero R, Belmonte-Fernández A, Flores ML, González-Moreno M, Pérez-Valderrama B, Romero F, et al. Wnt/Β-catenin signaling contributes to paclitaxel resistance in bladder cancer cells with cancer stem cell-like properties. Int J Mol Sci (2021) 23(1):450. doi: 10.3390/ijms23010450
95. Usui T, Sakurai M, Umata K, Elbadawy M, Ohama T, Yamawaki H, et al. Hedgehog signals mediate anti-cancer drug resistance in three-dimensional primary colorectal cancer organoid culture. Int J Mol Sci (2018) 19(4):1098. doi: 10.3390/ijms19041098
96. Chow PM, Chang YW, Kuo KL, Lin WC, Liu SH, Huang KH. Cdk7 inhibition by Thz1 suppresses cancer stemness in both chemonaïve and chemoresistant urothelial carcinoma Via the hedgehog signaling pathway. Cancer Lett (2021) 507:70–9. doi: 10.1016/j.canlet.2021.03.012
97. Syed IS, Pedram A, Farhat WA. Role of sonic hedgehog (Shh) signaling in bladder cancer stemness and tumorigenesis. Curr Urol Rep (2016) 17(2):11. doi: 10.1007/s11934-015-0568-9
Keywords: bladder cancer, SOX2, bladder cancer stem cells, chemoresistant, non-coding RNAs
Citation: Chen G, Chen Y, Xu R, Zhang G, Zou X and Wu G (2022) Impact of SOX2 function and regulation on therapy resistance in bladder cancer. Front. Oncol. 12:1020675. doi: 10.3389/fonc.2022.1020675
Received: 16 August 2022; Accepted: 01 November 2022;
Published: 16 November 2022.
Edited by:
Jack Schalken, Radboud University Medical Centre, NetherlandsCopyright © 2022 Chen, Chen, Xu, Zhang, Zou and Wu. This is an open-access article distributed under the terms of the Creative Commons Attribution License (CC BY). The use, distribution or reproduction in other forums is permitted, provided the original author(s) and the copyright owner(s) are credited and that the original publication in this journal is cited, in accordance with accepted academic practice. No use, distribution or reproduction is permitted which does not comply with these terms.
*Correspondence: Gengqing Wu, Z3lmeXVyb2xvZ3lAeWVhaC5uZXQ=; Xiaofeng Zou, Z3lmeXpvdXhmQDEyNi5jb20=
Disclaimer: All claims expressed in this article are solely those of the authors and do not necessarily represent those of their affiliated organizations, or those of the publisher, the editors and the reviewers. Any product that may be evaluated in this article or claim that may be made by its manufacturer is not guaranteed or endorsed by the publisher.
Research integrity at Frontiers
Learn more about the work of our research integrity team to safeguard the quality of each article we publish.