- 1Department of Epidemiology and Cancer Control, St. Jude Children’s Research Hospital, Memphis, TN, United States
- 2Department of Cell and Molecular Biology, St. Jude Children’s Research Hospital, Memphis, TN, United States
- 3Department of Pathology, St. Jude Children’s Research Hospital, Memphis, TN, United States
- 4Department of Immunology, St. Jude Children’s Research Hospital, Memphis, TN, United States
- 5Department of Biostatistics, St. Jude Children’s Research Hospital, Memphis, TN, United States
- 6Department of Oncology, St. Jude Children’s Research Hospital, Memphis, TN, United States
Background: Over 50% of childhood cancer survivors are exercise intolerant, with maximal aerobic capacities comparable to individuals decades older, suggesting early physiologic ageing. In addition, 36% of survivors are obese. Optimal exercise capacity provides a foundation to support daily function and healthy body habitus and is associated with benefits to cognition, cardiovascular health, and longevity. Cellular senescence and inflammation are key mechanisms that drive age-related disease, quantifiable as biomarkers in peripheral blood.
Aims: This study aimed to evaluate associations between p16INKa, a biomarker of cellular senescence, and inflammation and exercise capacity among adult survivors of childhood cancer.
Materials and methods: Eligible survivors were recruited from the St. Jude Lifetime (SJLIFE) Cohort Study. Exercise capacity was assessed by maximal oxygen uptake (VO2, ml/kg/min) obtained via cardiopulmonary exercise testing using a modified Bruce protocol. Body fat (%) was determined from dual energy x-ray absorptiometry (DEXA). Peripheral blood samples were used to evaluate log2 p16INK4a mRNA expression, a biomarker of cellular senescence, and inflammation with high sensitivity C-reactive protein (hs-CRP) levels. Multivariable regression evaluated associations between p16INK4a, hs-CRP, body fat, and exercise capacity.
Results: Participants included 185 five-year childhood cancer survivors (mean age 36.6 [range 20.1 - 55.7] years, 44% male, 77% non-Hispanic white, 53% leukemia/lymphoma). Compared to males, females had lower peak VO2 (mean ± SD, 22.5 ± 8.2 vs. 28.8 ± 7.7 ml/kg/min, p<0.01), higher p16INK4a expression (9.6 ± 1.2 vs. 9.2 ± 1.2 fold, p=0.02), and hs-CRP concentration (5.9 ± 8.4 vs. 3.3 ± 3.9 mg/L, p=0.01). Among females (n=103), hs-CRP concentration (β -0.2, 95% CI -0.34 to -0.05, p=0.01) and p16INK4a expression (β-5.32, 95% CI 10.42 to -0.22, p=0.04) were inversely associated and statistically significant with peak exercise capacity, with a significant interaction between p16INK4a expression and body fat (β 0.15, 95% CI 0.02 to 0.28, p=0.03). Among males (n=82), p16INK4a expression (β -1.01, 95% CI -2.14 to 0.12, p=0.08), and body fat (β -0.54, 95% CI -0.70 to -0.38, p<0.01) were inversely associated with peak exercise capacity.
Conclusion: Inflammation and p16INK4a expression, a biomarker of cellular senescence, are associated with lower exercise capacity in childhood cancer survivors, suggesting potential targets or outcome measures for interventions designed to prevent or remediate accelerated physiologic ageing in this population.
Introduction
Significant advances in treatment of childhood cancers have contributed to five-year survival exceeding 85% (1, 2). However, childhood cancer survivors are at risk for adverse health outcomes associated with cancer treatment, including exercise intolerance. Exercise intolerance is the result of impairment of or poor integration of cardiovascular, autonomic, pulmonary, muscular, and neurosensory system function. Over 56% of survivors are exercise intolerant (VO2 peak <85% predicted) (3), with exercise capacities comparable to individuals’ decades older (4). Young adult survivors of childhood cancer with exercise intolerance have a 3.9-fold increased risk of mortality (3). Within this population, risk for exercise intolerance is highest among those exposed to cardiotoxic therapy such as anthracyclines and chest radiation (3). However, survivors not exposed are also at risk, suggesting that either the disease process or other systemic alteration such as inflammation or cellular damage also contribute to decline in exercise capacity.
Cellular senescence is the functional consequence of serious DNA damage (5), resulting in accumulation of cells unresponsive to growth stimuli. Although these cells appear to remain in a stable state of proliferation arrest, they are not benign. Senescent cells accumulate with age (6–10), secrete high levels of inflammatory cytokines, immune modulators, growth factors, and proteases, and are associated with an increased prevalence of age-related health conditions, including both subclinical inflammation and high fat and/or low lean body mass (11). Although senescent cells are largely undetectable in younger populations, p16INK4a expression (mRNA), a biomarker of biologic ageing and indicator of senescent cells in older adults, is elevated in skin biopsies of young survivors of childhood cancer exposed to radiation (12).
p16INK4a is an important tumor suppressor gene that prevents cells with damaged DNA from growing and dividing too rapidly (13). When expressed, p16INK4a binds to and inactivates cyclin-dependent kinases (CDK4, CDK6), preventing the phosphorylation of retinoblastoma protein (pRB), halting cell cycle progression and initiating cellular senescence (14–16). Expression of p16INK4a occurs in response to stress, such as DNA damaging radiation and chemotherapy, and is highly expressed in senescent cells (15). Thus, it is an excellent biomarker for cellular senescence (17). Given that children with cancer are exposed to cancer therapies capable of inducing DNA damage, and that accelerated physiologic ageing is evident in this population (18–20), it is possible that senescent cells, with their secretory properties, may contribute to the pathobiology of exercise intolerance.
Further, cellular senescence is also associated with abnormal body composition; senescent cells accumulate in white adipose tissue (21), increasing the release and circulation of senescence-associated secretory phenotype (SASP) (10, 22, 23). Unfortunately, children with cancer experience significant changes in body composition during treatment (24–28), with increased risk for both obesity and underweight that can persist into survivorship (28–30). Childhood cancer survivors also have poor dietary habits (31, 32), which may further influence adipose tissue senescence (33). Early accumulation of adipose tissue may be a reservoir for senescent cells and a source of inflammation (34), underlining the pathobiology of early onset of age-related chronic conditions in this population. Recent evidence from murine and human studies suggest that interventions resulting in clearance of cells expressing the p16INK4a gene are capable of delaying the onset of, and attenuate existing, metabolic abnormalities (33) and age-related conditions (11, 35), perhaps defining a potential targets for intervention among survivors.
In this study, we measured p16INK4a expression, a biomarker of biologic age and cellular senescence, and high sensitivity C-reactive protein (hs-CRP), a biomarker of inflammation, in young adult survivors of childhood cancer and evaluated the cross-sectional associations with body fat percent and exercise capacity. We hypothesized that p16INK4a expression and hs-CRP levels would be higher among survivors with high body fat and low exercise capacity.
Materials and methods
Study population
Participants for this study were St. Jude Lifetime Cohort (SJLIFE) members, a retrospective cohort with prospective follow-up designed to evaluate childhood cancer survivors as they age. The study design and characteristics of the study population have been previously described (36–38). Briefly, cohort members were diagnosed with childhood cancer between 1962 and 2012 and treated at St. Jude Children’s Research Hospital. For these analyses, participants were at least 18 years old, 10 years from their primary diagnosis, had no evidence of cancer recurrence, and had previous chemotherapy exposure. Potentially eligible participants returning for a second clinical visit to evaluate frail health (including measures of exercise intolerance and body composition) were randomly recruited to provide a blood sample until we reached a powered sample size of 196 participants. Pregnant women or those with a current cancer diagnosis were excluded. Medical records were abstracted by trained personnel to collect demographic information, including age at assessment, sex, height (m), and weight (kg).
Biomarkers
Cellular Senescence Expression of p16INK4a mRNA was determined from CD3 T-lymphocytes processed from peripheral blood samples. Cells were isolated and enriched to >90% purity using RosetteSep™ Human T Cell Enrichment Cocktail (STEMCELL Technologies, Cambridge, MA). Total RNA was isolated from T-lymphocytes (ZR-96 Quick-RNA kit, Zymo Research, Irvine, CA), and reverse transcribed into cDNA using ImProm-II reverse transcription system (Promega Corp., Madison, WI). cDNA was reversed transcribed using Taqman® quantitative reverse-transcription PCR (ThermoFisher Scientific, Waltham, MA) to determine p16INK4a mRNA expression levels. Expression of p16INK4a mRNA transcript was normalized to 18s ribosomal RNA (HS03003631, Applied Biosystems, ThermoFisher Scientific - US, Waltham, MA) as previously described (39, 40). Data were log transformed for analysis (log2).
Inflammation High sensitivity C-reactive protein (hs-CRP) concentration (mg/L) was determined from serum samples isolated from peripheral blood. Blood samples of 2ml were collected in serum preparation tubes and allowed to clot completely at room temperature. Samples were centrifuged at 1,000-2,000 x g for 10 minutes in a refrigerated centrifuge. Serum supernatant was separated from samples in 1ml aliquots into ARUP standard Transport Tubes and refrigerated until processed on a Quantitative Immunoturbidimetry assay (reference value ≤3.0 mg/L) (41).
Outcomes
Exercise capacity
Exercise capacity was determined via cardiopulmonary exercise testing (CPET) on a treadmill using a modified Bruce protocol (42). A leg (n=4) or arm (n=7) cycle ergometer was substituted using a ramp protocol if a participant was unable to walk on a treadmill (lower extremity paralysis, amputations without prostheses, or poor balance). Continuous breath by breath analysis, using a metabolic cart (Ultima CardioO2; MCG Diagnostics, St. Paul, MN), was used to estimate attainment of VO2 peak. Blood pressure was measured during each stage of the protocol, and a continuous 12-lead electrocardiogram (ECG) monitored cardiac symptoms (43). Cardiopulmonary exercise testing (CPET) was terminated for safety before maximal exertion for signs of ischemia (>2 mm ST depression), frequent arrhythmias (bigeminy and trigeminy), hypertensive blood pressure (BP) response (250/115 mm Hg), symptoms (e.g. angina, shortness of breath, wheezing), or failure of heart rate (HR) to increase with increased exercise intensity. Immediately at test termination, participants were asked for peak rating of perceived exertion (44).
Body fat
Body fat (percent [%]) was determined with dual x-ray absorptiometry (DEXA) using a total body scanning mode (QDR 4500, software version 13.3:3; Hologic, Bedford, MA) (45, 46).
Covariates
Smoking history
Participants self-reported their smoking history, and were classified as current, former, or never smokers.
Statistical analyses
Descriptive statistics characterized demographic and diagnosis related variables (Table 1). Given that exercise capacity and body composition are influenced by sex, we stratified analysis by sex. Comparisons between male and female participants were made using χ2 statistics or two sample t tests as appropriate. Associations between smoking history, hs-CRP concentration, and p16INK4a expression were evaluated using linear regression models. Smoking history was not associated with either hs-CRP concentration or p16INK4a expression, and was not retained in final multivariate models. Separate multivariable linear regression models were used to evaluate associations between p16INK4a expression or hs-CRP concentration, and exercise capacity (ml/kg/min). Smoking status was evaluated as a potential covariate. Models were stratified by sex and adjusted for body fat % and age at assessment. Two-way interactions between either p16INK4a expression or hs-CRP concentration and body fat % were evaluated in each model. All statistical analyses were performed using SAS 9.4 (SAS Institute, Inc., Cary, NC).
Results
Characteristics of study population
Among 2,823 potentially eligible survivors, 234 were contacted to provide a blood sample. Among these, 30 (12.8%) declined participation. Of 204 samples collected, 15 (7.4%) did not pass quality control (low RNA yield), and 4 (2.0%) samples were not shipped, resulting in 185 participants with complete phenotype and biomarker data (Figure 1). Demographics of study participants are displayed in Table 1. On average, survivors were 36.6 years old (range 20.1 to 55.7 years) and 28.7 (± 9.01) years from diagnosis. Forty-four percent were male, 77% were non-Hispanic white, and 44% had a primary diagnosis of Acute Lymphoblastic or Myeloid Leukemia. Females were older than males at time of assessment (p<0.01).
Exercise capacity and body fat
Female survivors had lower peak VO2 (mean (SD), 22.5 (8.2) vs. 28.8 (7.7) ml/kg/min, p<0.01) and higher body fat (39.2 (7.9) vs. 27.2 (8.2) %, p<0.01) compared to males (Supplemental Figure 1).
Association between inflammation and exercise capacity
Female survivors had higher hs-CRP concentrations (5.9 (8.4) vs. 3.3 (3.9) mg/L, p=0.01) than males (Figure 2A). The results of multivariable linear regression, stratified by sex, and adjusted for age (years), and body fat (%) are shown in Figure 3. Among the 103 female survivors, hs-CRP concentration (β -0.2, 95% CI -0.34 to -0.05, p=0.01), body fat (β -0.54, 95% CI -0.70 to -0.37, p<0.01), and age (β -0.25, 95% CI -0.41 to -0.10, p<0.01) were inversely associated with peak VO2 (ml/kg/min). Among the 82 male survivors, hs-CRP concentration (β -0.31, 95% CI -0.65 to 0.03, p=0.07), body fat (β -0.55, 95% CI -0.71 to -0.39, p<0.01), and age (β -0.32, 95% CI -0.39 to -0.07, p<0.01), were inversely associated with peak VO2 (ml/kg/min), though hs-CRP concentration did not achieve statistical significance.
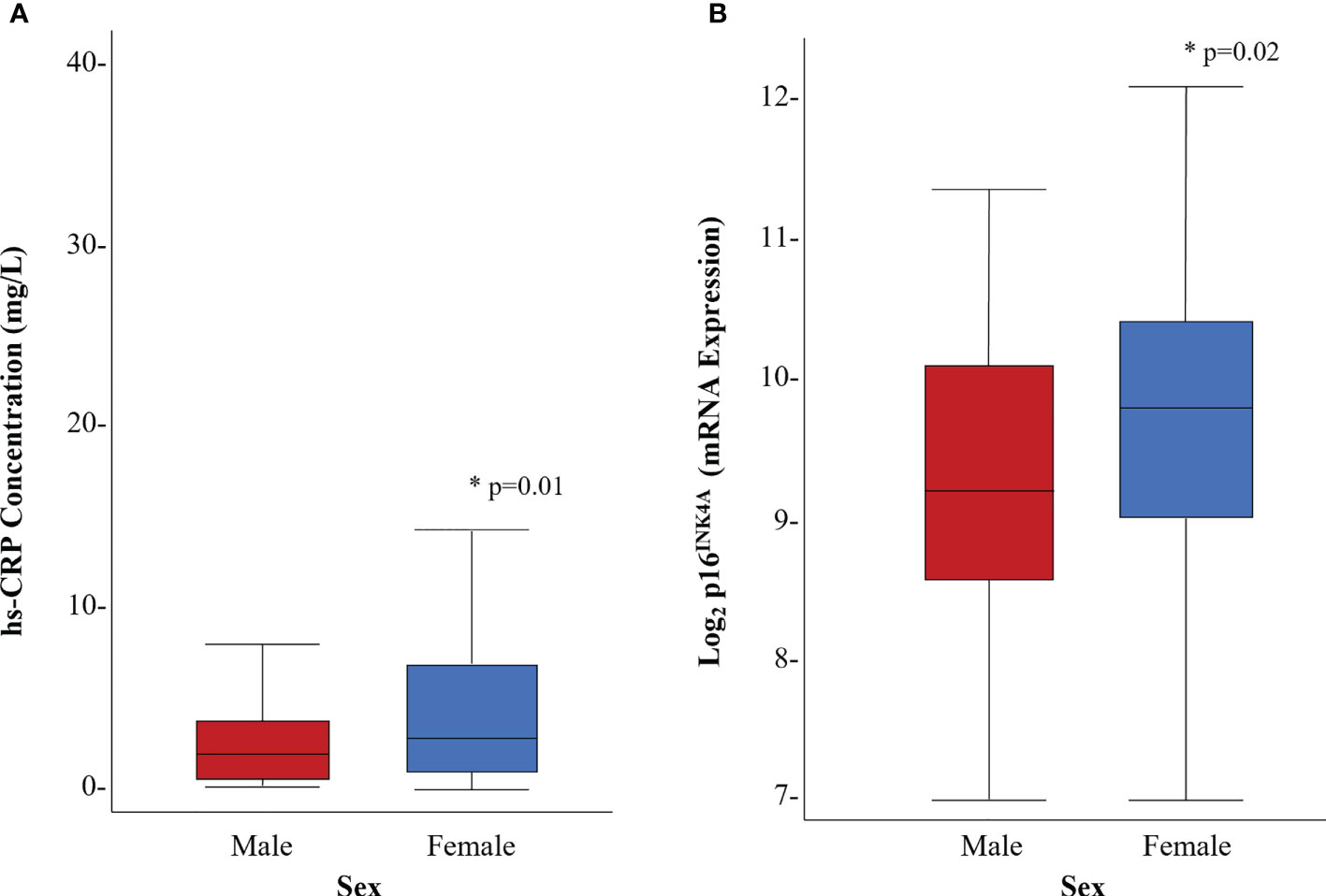
Figure 2 Distribution of biomarkers of inflammation and cellular senescence by sex. (A) hs-CRP concentration (mg/L) (B) p16 INK4A expression. * denotes statistical significance at p<0.05
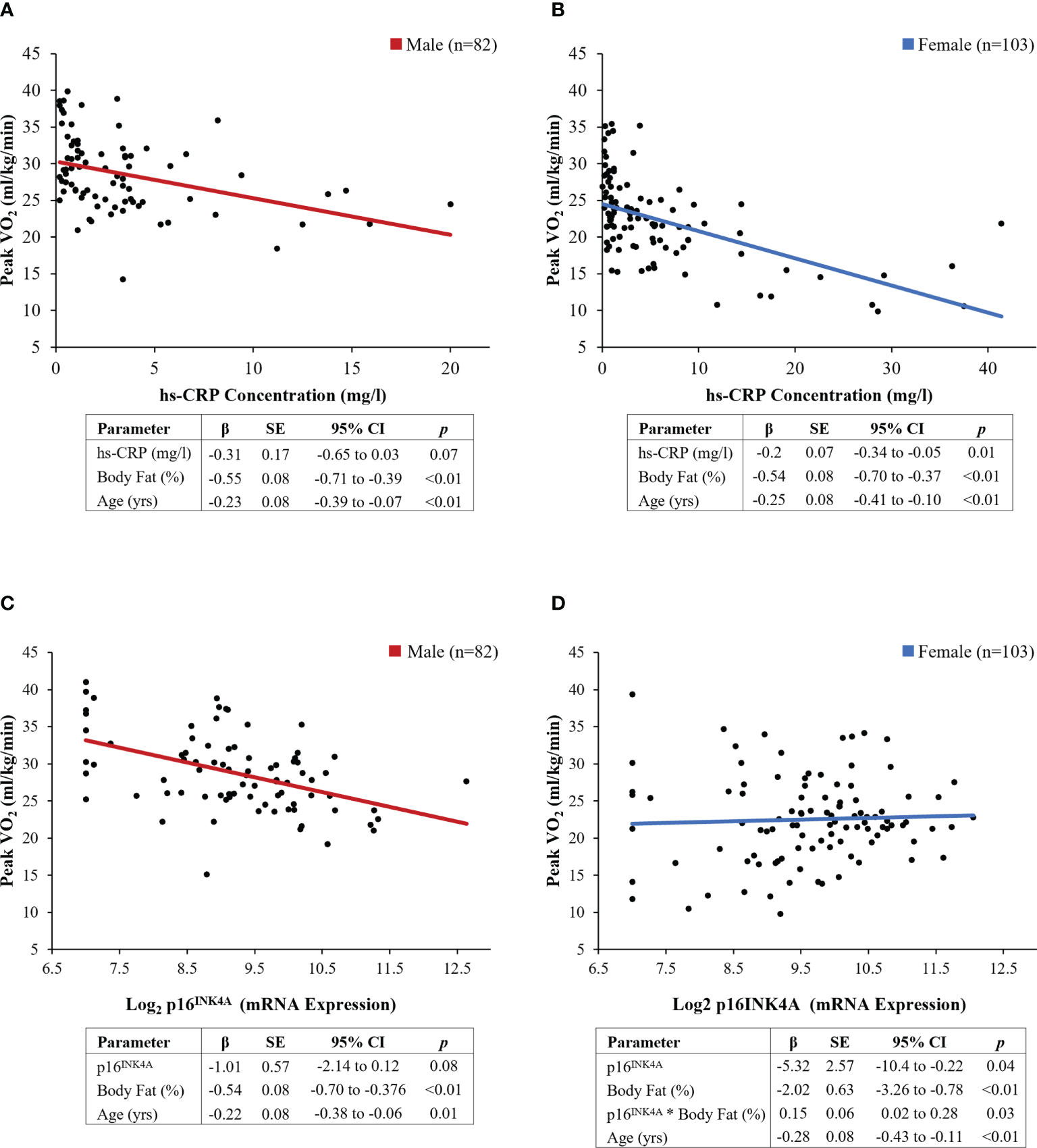
Figure 3 Results of sex-stratified multivariable models. (A, B) hs-CRP concentration (mg/L), (C, D) p16 INK4A expression. Regression lines represent the linear associations between individual biomarkers and exercise capacity, adjusted for covariates
Association between p16INK4a expression and exercise capacity
Female survivors had higher p16INK4a expression (9.6 (1.2) vs. 9.2 (1.2) fold, p=0.02) compared to male survivors (Figure 2B). Multivariable linear regression models, stratified by sex, were used to evaluate the association between p16INK4a expression and peak VO2 (ml/kg/min), adjusted for age (years) and body fat (%) (Figure 3). Among the 103 female survivors, p16INK4a expression (β -5.32, 95% CI -10.42 to -0.22, p=0.04), body fat (β -2.02, 95% CI -3.26 to -0.78, p<0.01), age (β -0.28, 95% CI -0.43 to -0.11, p<0.01), and the interaction between p16INK4a expression and body fat (β 0.15, 95% CI 0.02 to 0.28, p=0.03), were associated with peak VO2 (ml/kg/min). Among the 82 male survivors, p16INK4a expression (β -1.01, 95% CI -2.14 to 0.12, p=0.08), body fat (β -0.54, 95% CI -0.70 to -0.38, p<0.01), and age (β -0.22, 95% CI -0.38 to -0.06, p=0.01) were inversely associated with peak VO2 (ml/kg/min), though p16INK4a expression concentration did not achieve statistical significance.
To explore the interaction effect of p16INK4a expression and body fat on exercise capacity in females, data were sliced by progressing levels of body fat percent (Figure 4). Expression of p16INK4a was inversely associated with exercise capacity at body fat percentages less than 35%.
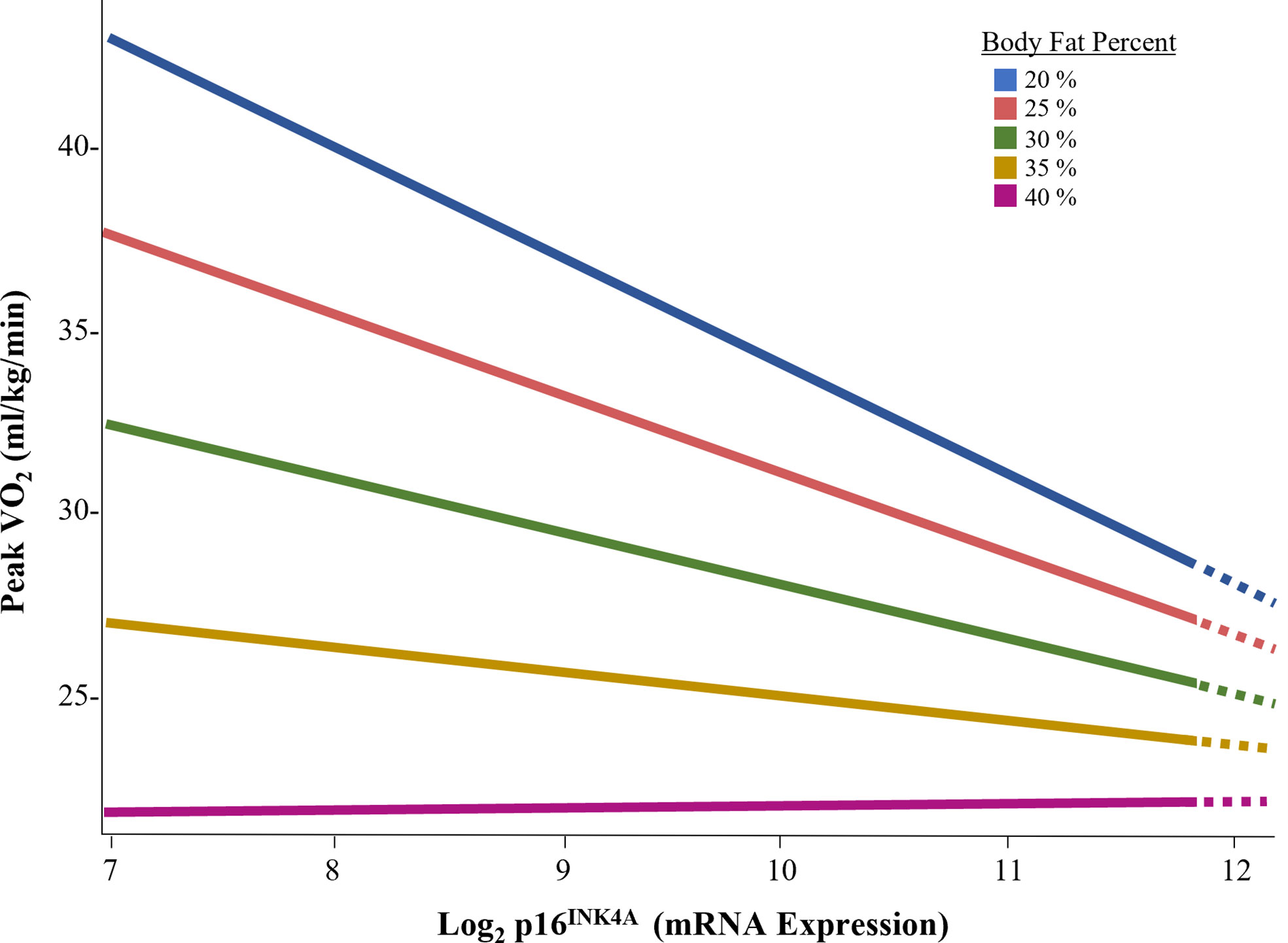
Figure 4 Association of p16INK4A expression and exercise capacity by body fat percent in female survivors.
Discussion
Adult survivors of childhood cancer are at risk for exercise intolerance, a predictor of all-cause mortality (3). In this study, we found increased p16INK4a and low-grade inflammation was associated with reduced exercise capacity. Among females, this association was only present among survivors with body fat percentages less than 35%. To our knowledge, this is the first study to demonstrate an association between a biomarker of cellular senescence, low-grade inflammation, and exercise capacity in childhood cancer survivors.
Expression of p16INK4a is generally undetectable in children and younger adults (39). However, it is detectable in peripheral blood T-lymphocytes among older adults and among young survivors of childhood cancer exposed to radiation (12). More recently, Smitherman et al (47) showed evidence of p16INK4a expression in young survivors of childhood, adolescent, and young adult cancers. They found elevated levels of p16INK4a to be associated with frailty, an age-associated phenotype indicating reduced physiological reserve. These data and our findings support the hypothesis that p16INK4a expression is present in other tissues (i.e. organs), and thus cellular senescence is potential mediator of physiologic deregulation in childhood cancer survivors.
Further, not only was elevated p16INK4a expression and hs-CRP concentration associated with lower peak VO2 and exercise intolerance, but the mean VO2 peak among our survivors was similar to values in persons several decades their senior (4). This is concerning as poor exercise capacity is associated with early mortality (48, 49), future cardiovascular events (50), and reduced cognitive reserve (51). Early impairments in exercise capacity concomitant with a hallmarks of ageing suggest that VO2 may be a new biomarker capable of identifying survivors at greatest risk of early onset of chronic conditions and mortality.
The detection of these ageing biomarkers is not surprising given recent work that identified other hallmarks of ageing, including reduced physiologic reserve (19, 52), telomere attrition (53), altered DNA methylation patterns (54), and mitochondrial dysfunction (55). Cellular senescence is an important biological mechanism, and is a part of normal ageing. Inherently designed to guard against proliferation of damaged cells, senescent cells lose the capacity to replicate. As a result of cell cycle arrest, senescent cells secrete proteins, including growth factors and proteases that alter tissue structure and function, and cytokines and chemokines with pro-inflammatory properties. The SASP promote a state of subclinical inflammation, which results in tissue fibrosis and deterioration (56). A similar mechanism may be responsible for the early onset of reduced exercise capacity seen among young adult survivors of childhood cancer. Early exposure to DNA damaging agents may trigger early accumulation of senescent cells that is not completely reversible.
Senescent cells also accumulate dysfunctional mitochondria, capable of influencing SASP production (57). Our recent work found association between decreased mitochondrial copy number (mtDNAcn) and increased odds for sarcopenia (55). Impaired skeletal muscle oxidative phosphorylation is implicated in exercise intolerance among induvial with mitochondrial myopathies (58). It is possible that mitochondrial dysfunction is the pathobiological mediator between elevated p16INK4a and hs-CRP levels in survivors with low exercise capacity.
Our data demonstrating elevated p16INK4a expression and hs-CRP concentration in survivors with impaired exercise capacity indicates a potential intervention target, given evidence that p16INK4a levels are modifiable, potentially with exercise (59, 60). In an animal model, Schafer et al (59) demonstrated an improvement in exercise capacity and a concomitant reduction in diet-induced p16INK4a mRNA expression in rodents who exercised. Resistance training, although primarily associated with muscle mass and strength gains, also has the potential to clear accumulated senescent cells. Yang et al. noted significant gains in muscle mass and an associated rapid clearance of senescent cells from skeletal muscle tissue in young men following a bout of resistance training (60). Given that childhood cancer survivors respond to both aerobic and resistance training, with improved exercise tolerance (61–63) and strength and mass gains (64), following exercise, it is possible that either aerobic training and/or resistance training may contribute senescent cells clearance. Additional research to determine if exercise, including type, frequency, intensity, and duration of activity, can clear senescent cells and either prevent or delay the cellular ageing in survivors. Further, cellular senescence is targetable through nutraceuticals (65). Agents such as Dasatinib and flavonoids (Quercetin; Fisetin, available as nutritional supplements) interfere with the senescent pathway, with evidence of safety, tolerability, and ability to alleviate physical dysfunction in adults with chronic disease (66). Currently, we have an open-label intervention trial (NCT04733534) which aims to establish preliminary evidence of efficacy, safety, and tolerability of two senolytic regimens to reduce markers of cellular senescence and improve frailty in adult survivors of childhood cancer. Survivors with reduced exercise capacity may also benefit from senolytic agents either alone or in combination with lifestyle modifications.
In general population, overweight and obese individual have higher proinflammatory plasma profiles, specifically higher hs-CRP, than non-overweight or obese individuals (67). In our study, female survivors had significantly higher levels of body fat, concomitant with higher p16INK4A expression, hs-CRP concentration, and lower peak VO2 (ml/kg/min) compared to males. Further, we noted a significant interaction between p16INK4a expression and body fat among female survivors, suggesting that the effect of cellular senescence on exercise capacity may be masked in females who have excess body fat. In our study, at body fat % values less than 35%, p16INK4a expression had a strong inverse association with exercise capacity. This association was not seen in females who had body fat % greater than 35% (68–70). Because adipose tissue is a harbor for senescent cells, is associated with reduced physical function, and is redistributed with ageing (71–73), it is possible that an evaluation of senescent cell expression in adipose tissue may have yielded different results. It is also possible that the burden of excess body fat is the primary driver of exercise capacity in females who are overweight or obese. Regardless, the influence of an interaction between body fat and biomarkers of ageing on exercise capacity are compelling and deserve further investigation.
The results of this analysis should be interpreted in the context of study limitations. Our population was small, and childhood cancer diagnoses were not evenly represented in the sample; almost half of the survivors had a history of childhood leukemia, followed by less than 20% with a history of lymphoma. While our study is limited by the use of a single biomarker of cellular senescence, our findings of detectable p16INK4a mRNA expression levels higher in young adult childhood cancer survivors than in persons of similar age in the general population (39), combined with the presence of low grade inflammation in our population, signifies that cellular senescence is a potential pathobiological mechanism for premature physiologic ageing in survivors of childhood cancer, contributing to exercise capacities comparable to adults decades older. Further, we did not compare p16INK4a and hs-CRP levels to individuals without a history of cancer. However, our mean hs-CRP value was higher than seen in a general population (41), suggesting a potential ongoing inflammatory milieu in this population. Liu et al (39) evaluated p16INK4a expression in peripheral blood T-cells in healthy adults, much older than our participants (age [years], median [range], 47 [18-76] vs. 36.3 [20.1-55.7]), who were also overweight (body mass index (BMI) [kg/m2], median [range], 26.5 [9.4-55.1] vs. 29.6 [16.5-57.4] kg/m2). However, our participants had higher expression of p16INK4a than healthy persons in the paper by Liu et al (39) (Figure 2B) (mean log2 p16INK4a mRNA expression 9.4 vs. 5.5). We suscept that individuals without a history of cancer would have lower levels of both biomarkers given their lack exposure to radiation and chemotherapeutic agents capable of inducing DNA damage. Additionally, our analysis was cross-sectional, and thus we are limited in the ability to determine the direction of causality between p16INK4a and exercise intolerance, and other participant characteristics, such as body composition. However, expression of p16INK4a was not independently associated with BMI (p=0.08) or body fat (p=0.07), which is consistent with findings by Liu et al. (39) Further, we previously showed that over 50% of childhood cancer survivors are exercise intolerant, at BMIs comparable to healthy community controls (3), thus challenging that high adiposity is the true etiology of senescence in our survivors. More likely, adiposity and the accumulation of senescent cells in adipose tissue is additive to the pathobiology of exercise intolerance in this population. However, adiposity is a potential source of senescence etiology and further investigations into its interplay with biologic ageing, cellular senescence, and inflammation is warranted in the survivor population.
Impact statement
Cellular senescence is implicated with advancing age and the onset of chronic condition and disease. Over 50% of young childhood cancer survivors are exercise intolerant, with maximal aerobic capacities comparable to individuals decades older, suggesting early physiologic ageing. In our study, biomarkers of cellular senescence and inflammation were associated with lower exercise capacity, which was further mediated by body fat in female survivors. To our knowledge, this is the first study to demonstrate an association between p16INK4a expression, low-grade inflammation, and exercise capacity in childhood cancer survivors. Our study contributes to growing body of evidence of accelerated ageing among childhood cancer survivors. Further, it highlights that interventions designed to improve exercise capacity and/or body composition have potential to remediate the accelerating ageing phenotype and early onset of chronic conditions seen among adult survivors of childhood cancer.
Data availability statement
The datasets analyzed for this study can be found in the St. Jude Cloud (https://www.stjude.cloud) (74).
Ethics statement
The studies involving human participants were reviewed and approved by St. Jude Children’s Research Hospital Institutional Review Board. The patients/participants provided their written informed consent to participate in this study.
Author contributions
CGG, MK, DKS, and KKN contributed to the conception and design of the research, analyzed, and interpreted the data. CGG, MDW, ML, and ZW performed the data acquisition and processing. CGG drafted the manuscript. All authors contributed to the article and approved the submitted version.
Funding
This work was supported by grants provided by the National Cancer Institute (CA195547, MMH and KKN; CA174851 KKN), the Cancer Center Support Grant (CA21765, CRR), and the American Lebanese Syrian Associated Charities (ALSAC).
Conflict of interest
The authors declare that the research was conducted in the absence of any commercial or financial relationships that could be construed as a potential conflict of interest.
Publisher’s note
All claims expressed in this article are solely those of the authors and do not necessarily represent those of their affiliated organizations, or those of the publisher, the editors and the reviewers. Any product that may be evaluated in this article, or claim that may be made by its manufacturer, is not guaranteed or endorsed by the publisher.
Supplementary material
The Supplementary Material for this article can be found online at: https://www.frontiersin.org/articles/10.3389/fonc.2022.1014661/full#supplementary-material
References
1. Siegel RL, Miller KD, Jemal A. Cancer statistics, 2019. CA Cancer J Clin (2019) 69:7–34. doi: 10.3322/caac.21551
2. Noone AM, Howlader N, Krapcho M, Miller D, Brest A, Yu M, et al. SEER cancer statistics review, 1975-2015, national cancer institute. (Bethesda, MD:National Cancer Institute, DCCPS, Surveillance Research Program) (2018). Available at: https://seer.cancer.gov/csr/1975_2015/. based on November 2017 SEER data submission, posted to the SEER web site.
3. Ness KK, Plana JC, Joshi VM, Luepker RV, Durand JB, Green DM, et al. Exercise intolerance, mortality, and organ system impairment in adult survivors of childhood cancer. J Clin Oncol (2020) 38:29–42. doi: 10.1200/JCO.19.01661
4. Shvartz E, Reibold RC. Aerobic fitness norms for males and females aged 6 to 75 years: a review. Aviat Space Environ Med (1990) 61:3–11.
5. Campisi J. Aging, cellular senescence, and cancer. Annu Rev Physiol (2013) 75:685–705. doi: 10.1146/annurev-physiol-030212-183653
6. Acosta JC, Banito A, Wuestefeld T, Georgilis A, Janich P, Morton JP, et al. A complex secretory program orchestrated by the inflammasome controls paracrine senescence. Nat Cell Biol (2013) 15:978–90. doi: 10.1038/ncb2784
7. De Cecco M, Jeyapalan J, Zhao X, Tamamori-Adachi M, Sedivy JM. Nuclear protein accumulation in cellular senescence and organismal aging revealed with a novel single-cell resolution fluorescence microscopy assay. Aging (Albany NY) (2011) 3:955–67. doi: 10.18632/aging.100372
8. Janzen V, Forkert R, Fleming HE, Saito Y, Waring MT, Dombkowski DM, et al. Stem-cell ageing modified by the cyclin-dependent kinase inhibitor p16INK4a. Nature (2006) 443:421–6. doi: 10.1038/nature05159
9. Krishnamurthy J, Ramsey MR, Ligon KL, Torrice C, Koh A, Bonner-Weir S, et al. p16INK4a induces an age-dependent decline in islet regenerative potential. Nature (2006) 443:453–7. doi: 10.1038/nature05092
10. Campisi J. Senescent cells, tumor suppression, and organismal aging: good citizens, bad neighbors. Cell (2005) 120:513–22. doi: 10.1016/j.cell.2005.02.003
11. Justice JN, Gregory H, Tchkonia T, LeBrasseur NK, Kirkland JL, Kritchevsky SB, et al. Cellular senescence biomarker p16INK4a+ cell burden in thigh adipose is associated with poor physical function in older women. J Gerontol A Biol Sci Med Sci (2018) 73:939–45. doi: 10.1093/gerona/glx134
12. Marcoux S, Le ON, Langlois-Pelletier C, Laverdiere C, Hatami A, Robaey P, et al. Expression of the senescence marker p16INK4a in skin biopsies of acute lymphoblastic leukemia survivors: A pilot study. Radiat Oncol (2013) 8:252. doi: 10.1186/1748-717X-8-252
13. LaPak KM, Burd CE. The molecular balancing act of p16(INK4a) in cancer and aging. Mol Cancer Res (2014) 12:167–83. doi: 10.1158/1541-7786.Mcr-13-0350
14. Vandenberk B, Brouwers B, Hatse S, Wildiers H. p16INK4a: A central player in cellular senescence and a promising aging biomarker in elderly cancer patients. J Geriatr Oncol (2011) 2:259–69. doi: 10.1016/j.jgo.2011.08.004
15. Ben-Porath I, Weinberg RA. The signals and pathways activating cellular senescence. Int J Biochem Cell Biol (2005) 37:961–76. doi: 10.1016/j.biocel.2004.10.013
16. Serrano M, Hannon GJ, Beach D. A new regulatory motif in cell-cycle control causing specific inhibition of cyclin D/CDK4. Nature (1993) 366:704–7. doi: 10.1038/366704a0
17. Kim WY, Sharpless NE. The regulation of INK4/ARF in cancer and aging. Cell (2006) 127:265–75. doi: 10.1016/j.cell.2006.10.003
18. Ness KK, Kirkland JL, Gramatges MM, Wang Z, Kundu M, McCastlain K, et al. Premature physiologic aging as a paradigm for understanding increased risk of adverse health across the lifespan of survivors of childhood cancer. J Clin Oncol (2018) 36:2206–15. doi: 10.1200/JCO.2017.76.7467
19. Ness KK, Krull KR, Jones KE, Mulrooney DA, Armstrong GT, Green DM, et al. Physiologic frailty as a sign of accelerated aging among adult survivors of childhood cancer: A report from the st. Jude lifetime cohort study. J Clin Oncol (2013) 31:4496–503. doi: 10.1200/JCO.2013.52.2268
20. Cupit-Link MC, Kirkland JL, Ness KK, Armstrong GT, Tchkonia T, LeBrasseur NK, et al. Biology of premature ageing in survivors of cancer. ESMO Open (2017) 2:e000250. doi: 10.1136/esmoopen-2017-000250
21. Smith U, Li Q, Ryden M, Spalding KL. Cellular senescence and its role in white adipose tissue. Int J Obes (Lond) (2021) 45:934–43. doi: 10.1038/s41366-021-00757-x
22. Coppé JP, Patil CK, Rodier F, Sun Y, Muñoz DP, Goldstein J, et al. Senescence-associated secretory phenotypes reveal cell-nonautonomous functions of oncogenic RAS and the p53 tumor suppressor. PLoS Biol (2008) 6:2853–68. doi: 10.1371/journal.pbio.0060301
23. Muñoz-Espín D, Serrano M. Cellular senescence: from physiology to pathology. Nat Rev Mol Cell Biol (2014) 15:482–96. doi: 10.1038/nrm3823
24. Browne EK, Zhou Y, Chemaitilly W, Panetta JC, Ness KK, Kaste SC, et al. Changes in body mass index, height, and weight in children during and after therapy for acute lymphoblastic leukemia. Cancer (2018) 124:4248–59. doi: 10.1002/cncr.31736
25. Warris LT, van den Akker ELT, Bierings MB, van den Bos C, Aarsen FK, Zwaan MC, et al. Eating behavior during dexamethasone treatment in children with acute lymphoblastic leukemia. Pediatr Blood Cancer (2017) 64:e26679. doi: 10.1002/pbc.26679
26. Zhang FF, Liu S, Chung M, Kelly MJ. Growth patterns during and after treatment in patients with pediatric ALL: A meta-analysis. Pediatr Blood Cancer (2015) 62:1452–60. doi: 10.1002/pbc.25519
27. Zhang FF, Kelly MJ, Saltzman E, Must A, Roberts SB, Parsons SK. Obesity in pediatric ALL survivors: a meta-analysis. Pediatrics (2014) 133:e704–15. doi: 10.1542/peds.2013-3332
28. Murphy AJ, White M, Elliott SA, Lockwood L, Hallahan A, Davies PS. Body composition of children with cancer during treatment and in survivorship. Am J Clin Nutr (2015) 102:891–6. doi: 10.3945/ajcn.114.099697
29. Ness KK, Baker KS, Dengel DR, Youngren N, Sibley S, Mertens AC, et al. Body composition, muscle strength deficits and mobility limitations in adult survivors of childhood acute lymphoblastic leukemia. Pediatr Blood Cancer (2007) 49:975–81. doi: 10.1002/pbc.21091
30. Ness KK, DeLany JP, Kaste SC, Mulrooney DA, Pui CH, Chemaitilly W, et al. Energy balance and fitness in adult survivors of childhood acute lymphoblastic leukemia. Blood (2015) 125:3411–9. doi: 10.1182/blood-2015-01-621680
31. Landy DC, Lipsitz SR, Kurtz JM, Hinkle AS, Constine LS, Adams MJ, et al. Dietary quality, caloric intake, and adiposity of childhood cancer survivors and their siblings: an analysis from the cardiac risk factors in childhood cancer survivors study. Nutr Cancer (2013) 65:547–55. doi: 10.1080/01635581.2013.770042
32. Zhang FF, Ojha RP, Krull KR, Gibson TM, Lu L, Lanctot J, et al. Adult survivors of childhood cancer have poor adherence to dietary guidelines. J Nutr (2016) 146:2497–505. doi: 10.3945/jn.116.238261
33. Pini M, Czibik G, Sawaki D, Mezdari Z, Braud L, Delmont T, et al. Adipose tissue senescence is mediated by increased ATP content after a short-term high-fat diet exposure. Aging Cell (2021) 20:e13421. doi: 10.1111/acel.13421
34. Tchkonia T, Morbeck DE, Von Zglinicki T, Van Deursen J, Lustgarten J, Scrable H, et al. Fat tissue, aging, and cellular senescence. Aging Cell (2010) 9:667–84. doi: 10.1111/j.1474-9726.2010.00608.x
35. Baker DJ, Wijshake T, Tchkonia T, LeBrasseur NK, Childs BG, van de Sluis B, et al. Clearance of p16Ink4a-positive senescent cells delays ageing-associated disorders. Nature (2011) 479:232–6. doi: 10.1038/nature10600
36. Howell CR, Bjornard KL, Ness KK, Alberts N, Armstrong GT, Bhakta N, et al. Cohort profile: The st. Jude lifetime cohort study (SJLIFE) for paediatric cancer survivors. Int J Epidemiol (2021) 50:39–49. doi: 10.1093/ije/dyaa203
37. Hudson MM, Ness KK, Nolan VG, Armstrong GT, Green DM, Morris EB, et al. Prospective medical assessment of adults surviving childhood cancer: study design, cohort characteristics, and feasibility of the st. Jude lifetime cohort study. Pediatr Blood Cancer (2011) 56:825–36. doi: 10.1002/pbc.22875
38. Ojha RP, Oancea SC, Ness KK, Lanctot JQ, Srivastava DK, Robison LL, et al. Assessment of potential bias from non-participation in a dynamic clinical cohort of long-term childhood cancer survivors: results from the st. Jude lifetime cohort study. Pediatr Blood Cancer (2013) 60:856–64. doi: 10.1002/pbc.24348
39. Liu Y, Sanoff HK, Cho H, Burd CE, Torrice C, Ibrahim JG, et al. Expression of p16(INK4a) in peripheral blood T-cells is a biomarker of human aging. Aging Cell (2009) 8:439–48. doi: 10.1111/j.1474-9726.2009.00489.x
40. Shields JM, Thomas NE, Cregger M, Berger AJ, Leslie M, Torrice C, et al. Lack of extracellular signal-regulated kinase mitogen-activated protein kinase signaling shows a new type of melanoma. Cancer Res (2007) 67:1502–12. doi: 10.1158/0008-5472.Can-06-3311
41. Pagana KD, Pagana TJ, Pagana TN. Mosby’s diagnostic and laboratory test reference. 14th ed. St. Loius, MO: Elsevier Health Sciences (2019).
42. Pescatello LS, American College of Sports Medicine, Riebe D, Thompson PD. ACSM’s guidelines for exercise testing and prescription. 9th ed. Philadelphia, PA: Wolters Kluwer (2013).
43. Wang CY, Haskell WL, Farrell SW, Lamonte MJ, Blair SN, Curtin LR, et al. Cardiorespiratory fitness levels among US adults 20-49 years of age: findings from the 1999-2004 national health and nutrition examination survey. Am J Epidemiol (2010) 171:426–35. doi: 10.1093/aje/kwp412
45. Thomas SR, Kalkwarf HJ, Buckley DD, Heubi JE. Effective dose of dual-energy X-ray absorptiometry scans in children as a function of age. J Clin Densitom (2005) 8:415–22. doi: 10.1385/jcd:8:4:415
46. Jensen MD, Kanaley JA, Roust LR, O’Brien PC, Braun JS, Dunn WL, et al. Assessment of body composition with use of dual-energy x-ray absorptiometry: Evaluation and comparison with other methods. Mayo Clin Proc (1993) 68:867–73. doi: 10.1016/s0025-6196(12)60695-8
47. Smitherman AB, Wood WA, Mitin N, Ayer Miller VL, Deal AM, Davis IJ, et al. Accelerated aging among childhood, adolescent, and young adult cancer survivors is evidenced by increased expression of p16(INK4a) and frailty. Cancer (2020) 126:4975–83. doi: 10.1002/cncr.33112
48. Ladenvall P, Persson CU, Mandalenakis Z, Wilhelmsen L, Grimby G, Svardsudd K, et al. Low aerobic capacity in middle-aged men associated with increased mortality rates during 45 years of follow-up. Eur J Prev Cardiol (2016) 23:1557–64. doi: 10.1177/2047487316655466
49. Lee DC, Sui X, Artero EG, Lee IM, Church TS, McAuley PA, et al. Long-term effects of changes in cardiorespiratory fitness and body mass index on all-cause and cardiovascular disease mortality in men: the aerobics center longitudinal study. Circulation (2011) 124:2483–90. doi: 10.1161/CIRCULATIONAHA.111.038422
50. Kodama S, Saito K, Tanaka S, Maki M, Yachi Y, Asumi M, et al. Cardiorespiratory fitness as a quantitative predictor of all-cause mortality and cardiovascular events in healthy men and women: A meta-analysis. JAMA (2009) 301:2024–35. doi: 10.1001/jama.2009.681
51. Hayes SM, Forman DE, Verfaellie M. Cardiorespiratory fitness is associated with cognitive performance in older but not younger adults. J Gerontol B Psychol Sci Soc Sci (2016) 71:474–82. doi: 10.1093/geronb/gbu167
52. Hayek S, Gibson TM, Leisenring WM, Guida JL, Gramatges MM, Lupo PJ, et al. Prevalence and predictors of frailty in childhood cancer survivors and siblings: A report from the childhood cancer survivor study. J Clin Oncol (2020) 38:232–47. doi: 10.1200/JCO.19.01226
53. Song N, Li Z, Qin N, Howell CR, Wilson CL, Easton J, et al. Shortened leukocyte telomere length associates with an increased prevalence of chronic health conditions among survivors of childhood cancer: A report from the st. Jude lifetime cohort. Clin Cancer Res (2020) 26:2362–71. doi: 10.1158/1078-0432.CCR-19-2503
54. Song N, Hsu CW, Pan H, Zheng Y, Hou L, Sim JA, et al. Persistent variations of blood DNA methylation associated with treatment exposures and risk for cardiometabolic outcomes in long-term survivors of childhood cancer in the st. Jude lifetime cohort. Genome Med (2021) 13:53. doi: 10.1186/s13073-021-00875-1
55. McCastlain K, Howell CR, Welsh CE, Wang Z, Wilson CL, Mulder HL, et al. The association of mitochondrial copy number with sarcopenia in adult survivors of childhood cancer. J Natl Cancer Inst (2021) 113:1570–80. doi: 10.1093/jnci/djab084
56. van Deursen JM. The role of senescent cells in ageing. Nature (2014) 509:439–46. doi: 10.1038/nature13193
57. Zlotorynski E. Defective mitochondria ignite the SASP. Nat Rev Mol Cell Biol (2020) 21:179. doi: 10.1038/s41580-020-0228-x
58. Taivassalo T, Jensen TD, Kennaway N, DiMauro S, Vissing J, Haller RG. The spectrum of exercise tolerance in mitochondrial myopathies: A study of 40 patients. Brain (2003) 126:413–23. doi: 10.1093/brain/awg028
59. Schafer MJ, White TA, Evans G, Tonne JM, Verzosa GC, Stout MB, et al. Exercise prevents diet-induced cellular senescence in adipose tissue. Diabetes (2016) 65:1606–15. doi: 10.2337/db15-0291
60. Yang C, Jiao Y, Wei B, Yang Z, Wu JF, Jensen J, et al. Aged cells in human skeletal muscle after resistance exercise. Aging (Albany NY) (2018) 10:1356–65. doi: 10.18632/aging.101472
61. Smith WA, Ness KK, Joshi V, Hudson MM, Robison LL, Green DM. Exercise training in childhood cancer survivors with subclinical cardiomyopathy who were treated with anthracyclines. Pediatr Blood Cancer (2013) 61(5):942–945. doi: 10.1002/pbc.24850
62. Jarvela LS, Kemppainen J, Niinikoski H, Hannukainen JC, Lahteenmaki PM, Kapanen J, et al. Effects of a home-based exercise program on metabolic risk factors and fitness in long-term survivors of childhood acute lymphoblastic leukemia. Pediatr Blood Cancer (2012) 59:155–60. doi: 10.1002/pbc.24049
63. Bourdon A, Grandy SA, Keats MR. Aerobic exercise and cardiopulmonary fitness in childhood cancer survivors treated with a cardiotoxic agent: a meta-analysis. Support Care Cancer (2018) 26:2113–23. doi: 10.1007/s00520-018-4208-z
64. Krull MR, Howell CR, Partin RE, Lanctot J, Phipps S, Klosky JL, et al. Protein supplementation and resistance training in childhood cancer survivors. Med Sci Sports Exerc (2020) 52:2069–77. doi: 10.1249/MSS.0000000000002345
65. Zhu Y, Tchkonia T, Pirtskhalava T, Gower AC, Ding H, Giorgadze N, et al. The achilles’ heel of senescent cells: From transcriptome to senolytic drugs. Aging Cell (2015) 14:644–58. doi: 10.1111/acel.12344
66. Justice JN, Nambiar AM, Tchkonia T, LeBrasseur NK, Pascual R, Hashmi SK, et al. Senolytics in idiopathic pulmonary fibrosis: Results from a first-in-human, open-label, pilot study. EBioMedicine (2019) 40:554–63. doi: 10.1016/j.ebiom.2018.12.052
67. Phillips CM, Tierney AC, Perez-Martinez P, Defoort C, Blaak EE, Gjelstad IM, et al. Obesity and body fat classification in the metabolic syndrome: impact on cardiometabolic risk metabotype. Obes (Silver Spring) (2013) 21:E154–61. doi: 10.1002/oby.20263
68. Okorodudu DO, Jumean MF, Montori VM, Romero-Corral A, Somers VK, Erwin PJ, et al. Diagnostic performance of body mass index to identify obesity as defined by body adiposity: a systematic review and meta-analysis. Int J Obes (Lond) (2010) 34:791–9. doi: 10.1038/ijo.2010.5
69. Deurenberg P, Andreoli A, Borg P, Kukkonen-Harjula K, de Lorenzo A, van Marken Lichtenbelt WD, et al. The validity of predicted body fat percentage from body mass index and from impedance in samples of five European populations. Eur J Clin Nutr (2001) 55:973–9. doi: 10.1038/sj.ejcn.1601254
70. Bosy-Westphal A, Geisler C, Onur S, Korth O, Selberg O, Schrezenmeir J, et al. Value of body fat mass vs anthropometric obesity indices in the assessment of metabolic risk factors. Int J Obes (Lond) (2006) 30:475–83. doi: 10.1038/sj.ijo.0803144
71. Hagg S, Jylhava J. Sex differences in biological aging with a focus on human studies. eLife (2021) 10:e63425. doi: 10.7554/eLife.63425
72. Bazzocchi A, Diano D, Ponti F, Andreone A, Sassi C, Albisinni U, et al. Health and ageing: A cross-sectional study of body composition. Clin Nutr (2013) 32:569–78. doi: 10.1016/j.clnu.2012.10.004
73. Bazzocchi A, Ponti F, Diano D, Moio A, Albisinni U, Pasquali R, et al. Abdominal adiposity by ultrasonography: A “pocket” database for reference standard in Italian people. Prim Care Diabetes (2014) 8:358–64. doi: 10.1016/j.pcd.2014.02.003
Keywords: cellular senescence, p16, inflammation, childhood cancer surivor, exercise capacity
Citation: Goodenough CG, Wogksch MD, Kundu M, Lear M, Thomas PG, Srivastava DK, Wang Z, Armstrong GT, Hudson MM, Robison LL and Ness KK (2022) Associations between exercise capacity, p16INK4a expression and inflammation among adult survivors of childhood cancer. Front. Oncol. 12:1014661. doi: 10.3389/fonc.2022.1014661
Received: 08 August 2022; Accepted: 13 October 2022;
Published: 08 November 2022.
Edited by:
Amanda Wurz, University of the Fraser Valley, CanadaReviewed by:
Andrew Smitherman, University of North Carolina at Chapel Hill, United StatesAlessandro Bitto, University of Washington, United States
Copyright © 2022 Goodenough, Wogksch, Kundu, Lear, Thomas, Srivastava, Wang, Armstrong, Hudson, Robison and Ness. This is an open-access article distributed under the terms of the Creative Commons Attribution License (CC BY). The use, distribution or reproduction in other forums is permitted, provided the original author(s) and the copyright owner(s) are credited and that the original publication in this journal is cited, in accordance with accepted academic practice. No use, distribution or reproduction is permitted which does not comply with these terms.
*Correspondence: Kirsten K. Ness, kiri.ness@stjude.org