- 1Henan Provincial Key Laboratory of Children’s Genetics and Metabolic Diseases, Children’s Hospital Affiliated to Zhengzhou University, Zhengzhou University, Zhengzhou, China
- 2Department of Pharmacy, Iqra University Islamabad Campus, Islamabad, Pakistan
- 3State Key Laboratory of Esophageal Cancer Prevention and Treatment, School of Pharmaceutical Sciences, Zhengzhou University, Zhengzhou, China
- 4Institute of Molecular Biology and Biotechnology, Bahauddin Zakariya University, Multan, Pakistan
- 5Department of Pharmaceutics, Riphah Institute of Pharmaceutical Sciences, Riphah International University, Islamabad, Pakistan
Lung cancer is one of the deadliest types of cancer responsible for thousands of cancer-related deaths. Its treatment has remained a challenge for researchers, but an increase in the knowledge of molecular pathways and biology of lung cancer has dramatically changed its management in recent decades. Immunotherapies and immunomodulation of lung cancer have previously failed for a long time but thanks to continuous research work and enthusiasm, now, this field is emerging as a novel effective therapy. Now, it is hope with potential benefits and promising results in the treatment of lung cancer. This review article focuses on immune checkpoints inhibitors: CTLA-4 inhibitors (ipilimumab and tremelimumab) and PDL-1 inhibitors (durvalumab and atezolizumab) that can be blocked to treat lung carcinoma. It is also focused on critically analyzing different studies and clinical trials to determine the potential benefits, risks, and adverse events associated with immunotherapeutic treatment.
Introduction
Lung cancer is considered one of the most lethal forms of solid cancer that have destroyed the lives of thousands of people internationally (1). According to WHO, lung cancer is the most common cause of tobacco-related deaths worldwide. Its survival rate is very low with just 5 years of survival in 18% of the patients diagnosed with this disease, which may be attributed to its late diagnosis in advanced stages (2). Generally, it is divided into two main types: small cell lung cancer (SCLC) or non-small cell lung cancer (NSCLC). NSCLC is the major subtype of lung carcinoma that accounts for 85% of the cases, and the available chemotherapies, radiotherapies, and surgical options are less effective against this type of carcinoma (3). In a survey in 2012, it was estimated that 1,800,000 new cases of lung carcinoma were reported, out of which, 1,600,000 died making the death rate of lung cancer 87% in 1 year. It is the second most common cause of cancer deaths in both men and women (4).
Thus, patients having lung cancer should be intervened in its early stage; otherwise, despite all of the treatment strategies, they are not able to survive stage IV of lung cancer (5). Standard chemotherapeutic drugs have been used over the years to treat NSCLC; however, now, advanced therapeutic options are required to overcome the limitations of cytotoxic drugs (6, 7). Even though all of the genetic alterations, especially anaplastic lymphoma kinase (ALK) fusion oncogene mutation and alteration in epidermal growth factor receptor (EGFR) in NSCLC, are well understood, certain limitations still need to be addressed (8–10). Recent research suggests that immune-mediated disruption of carcinogens is more beneficial than the standard chemotherapeutic approach, so immunotherapy is under consideration for lung cancer treatment (11).
Immunotherapy as a treatment option
The proliferation and malignancy of cancerous cells are linked with both the nature of the solid tumor and their association with the immune system of our body (12, 13). So, immunotherapy is the use of immunotherapeutic drugs that are designed to promote the immune-regulated destruction of cancerous cells. Generally, immunotherapy includes the use of monoclonal antibodies that targets checkpoint inhibitor signals on cancerous cells, immune system activators, vaccines, and cells of the same individual that triggers an immune response (autologous cells). Earlier studies show that immunotherapeutic drugs were not much effective against lung cancer, so the scientist thought that lung cancer is not dependent on our immune system (14). But recent research suggests that lung cancer can destroy our immune system in a variety of ways including the release of inhibitory cytokines, by reduction of chemicals that restrict the stimulation of T cells and by the disruption of major histocompatibility complex antigen expression (15). Nowadays, immunotherapeutic pathways are employed to treat and reduce harmful effects on already treated patients having lung cancer. These pathways include programmed death-1 (PD-1) pathway and the cytotoxic T-lymphocyte-associated antigen 4 (CTLA-4) pathway, T-cell immunoglobulin and mucin domain-containing protein 3 (TIM-3), T-cell immunoreceptor with lg and ITIM domains (TIGIT), and lymphocyte activation gene 3 (LAG-3) (16). Table 1 enlists the summary of immune checkpoint inhibitors.
CTLA-4 receptor
CTLA-4 is a membrane receptor expressed on T cells that regulates the activation of T cells. It is a CD28 homolog and binds with B7 but with more affinity than CD28. However, its binding with B7 does not produce stimulatory signals, rather it produces inhibitory signals (17). It has a negative impact on T-cell activation by following mechanisms (18, 19) (1). Following T-cell receptor (TCR) activation, CTLA-4 is upregulated and more avidly binds B7 than T lymphocyte receptor CD28, which inhibits T-cell proliferation and lowers cytokine release (20) (2). By generating inhibitory signals to diminish the immune response against the tumor in the early stages of carcinogenesis, CTLA-4 could reduce T-cell activation (3). CTLA-4 activates B7 to induce indoleamine-2, 3-dioxygenase (IDO), which catabolizes the amino acid tryptophan and consequently inhibits the proliferation of T cells (21, 22) (4). It has been suggested that CTLA-4 negatively regulates T-cell activation by suppressing the production of the zeta-associated protein (ZAP 70) or promoting the expression of Casitas-B-lineage lymphoma (Cbl) protein (23) (5). According to recent research, CTLA-4 also inhibits The PI3K/Akt pathways, cyclin D3, cyclin-dependent kinases (cdk4/cdk6), and nuclear transcription factors (NF-B) (24) (6) by increasing the movement of T cells, thus reducing the chance of T cells’ interaction with antigen-presenting cells (25) (Figures 1, 2).
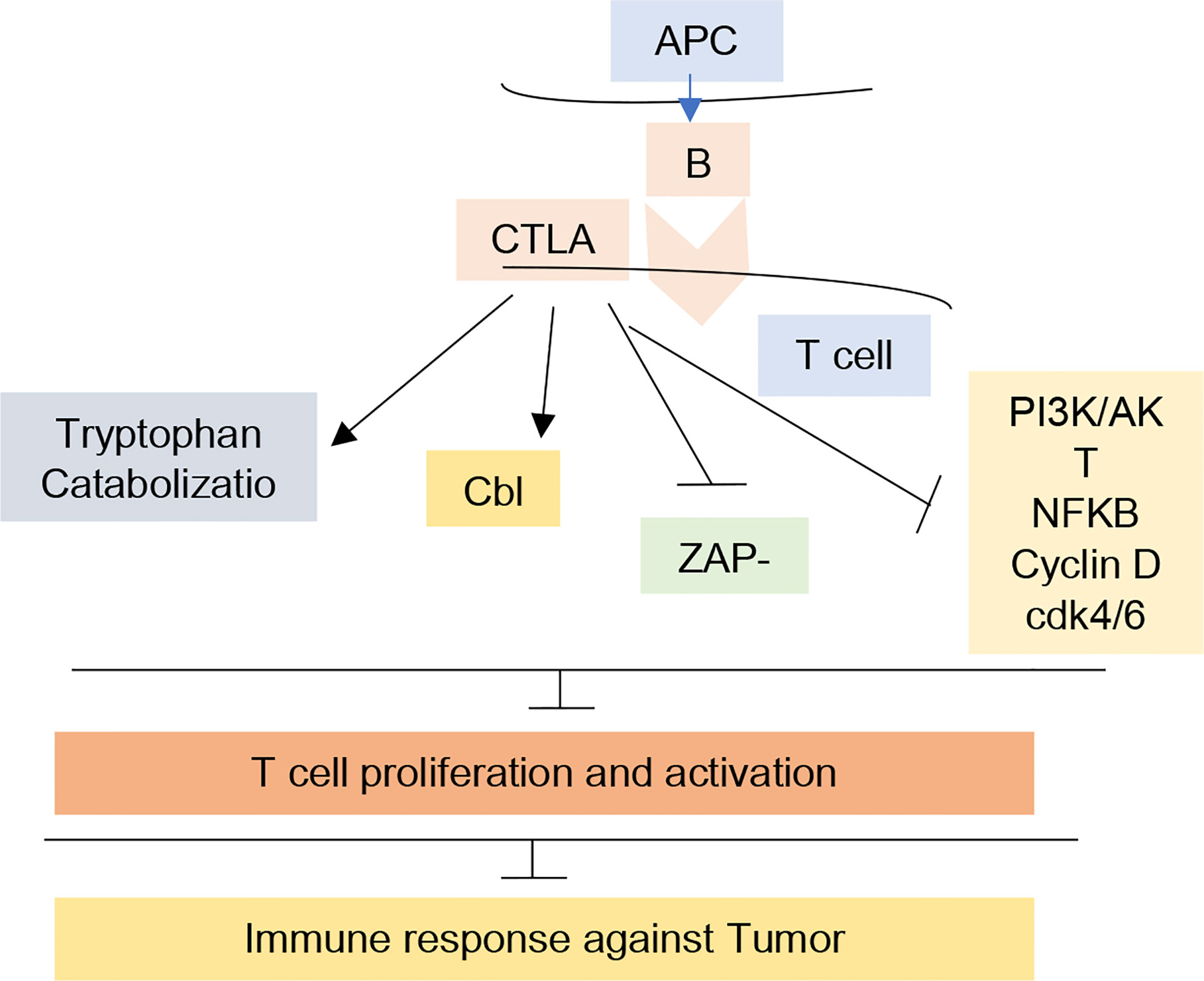
Figure 1 CTLA-4 mechanism for T-cell regulation. The figure illustrates the relation of CTLA-4 with Tryptophan catabolization, Cbl, ZAP-70, and PI3K pathways leading to inhibition of T-cell proliferation and activation and inhibited immune response against tumor. In cancer immunotherapy, CTLA-R receptor was the first receptor to be targeted clinically. Blockade of this receptor by antibodies prevent its interaction in immune system inhibition.
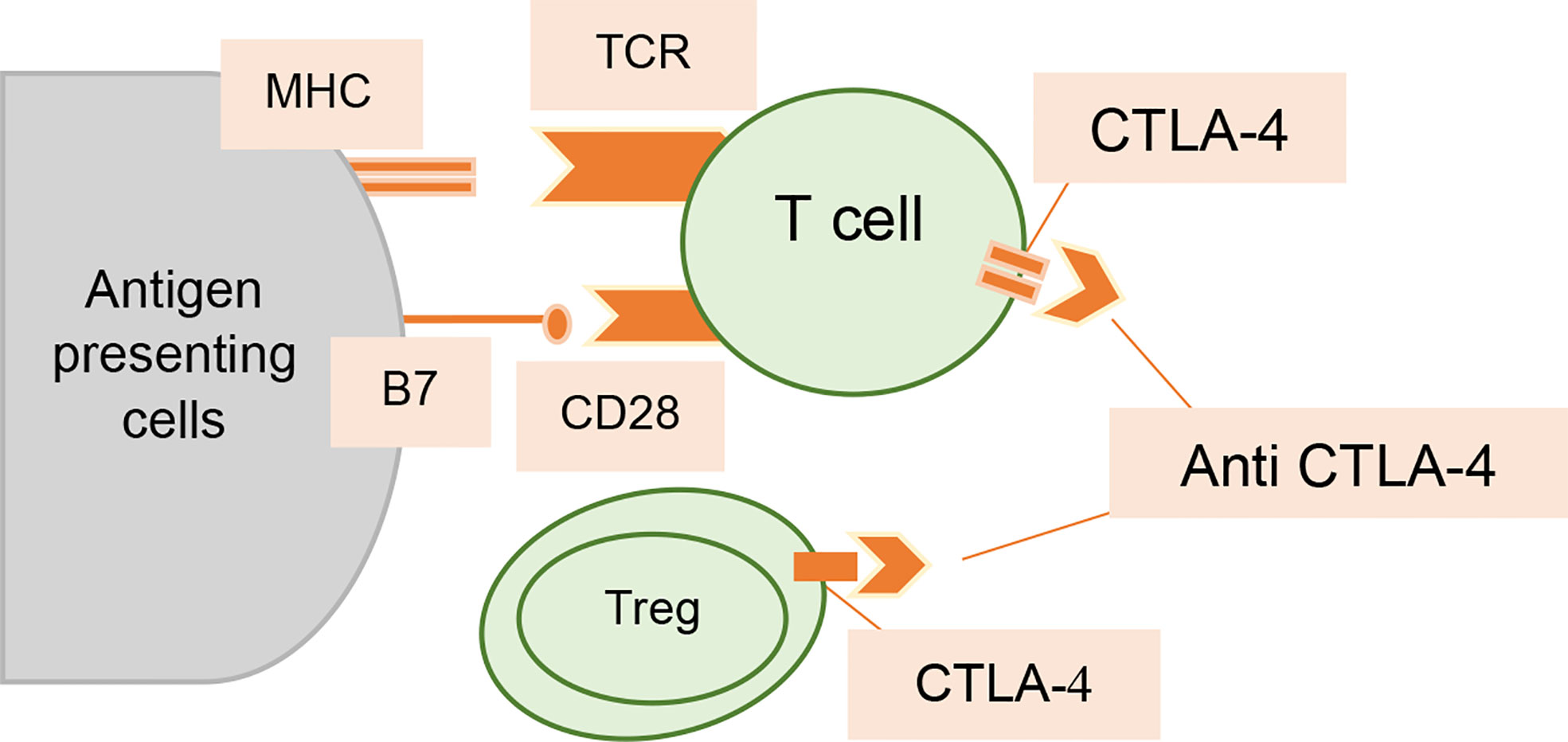
Figure 2 Interaction of anti-CTLA-4 antibodies with T cells. Increased T-cell activation and proliferation are made possible by CTLA-4 inhibition; it also lessens the immunosuppressive effects of Tregs.
CTLA-4 inhibitors
Ipilimumab
Ipilimumab is the most recognized inhibitor of CTLA-4. Ipilimumab is the first ever approved checkpoint inhibitor for the treatment of cancer. It is a fully humanized immunoglobulin monoclonal antibody that blocks the interaction of CTLA-4 with its ligand, thus blocking CTLA-4’s negative impact on T-cell regulation. Through this mechanism, ipilimumab allows the attack of immune system on tumor cells (26). Ipilimumab was first patented by University of California (27). However, ipilimumab monotherapy is not successful in treating non-small cell lung carcinoma. Thus, it is combined with other anticancer drugs I-e paclitaxel plus carboplatin with ipilimumab or ipilimumab with other immune checkpoint inhibitors (28). Ipilimumab shows promising results in prolonging the overall survival rates in the patients with advanced melanoma. Phases II and III clinical trials showed satisfactory survival rate of up to 3 years in almost 26% of the population when treated with ipilimumab (29, 30). However, this immune blockade is also associated with some adverse events including rashes, gastrointestinal tract abnormalities (inflammation of colon and diarrhea), pituitary gland inflammation (2% to 4%) (31), endocrine gland disorders (32), and effects on skin, kidney, liver, nervous system, and pancreas. Rash is considered as the characteristic adverse event of ipilimumab that does not occur while treating with any other anticancer agent (33).
Recent research reveals that anti–CTLA-4 antibodies may cause a severe and extensive form of inflammatory bowel illness, which recommends that patients receiving anti–CTLA-4 therapy should avoid using nonsteroidal anti-inflammatory medicines (NSAIDs).
These adverse effects may be associated with the expression of CTLA-4 receptors on pituitary gland leading to binding of ipilimumab with the receptor and induction of inflammation and adverse events and activation of the non-tumor specific T cells. Most of these effects are rare and manageable I-e effect of ipilimumab on liver, and endocrine system is less common (34). The risk of ipilimumab associated adverse events is dose dependent. A study conducted by Bertrand et al. showed that at 3mg/kg dose, 61% of the patients were having adverse events, whereas by increasing the dose to 10mg/kg, the rate of adverse events was increased up to 79% (35).
Tremelimumab
Tremelimumab is a fully humanized immunoglobulin monoclonal antibody (36). Tremelimumab prevents the interaction of CTLA-4 with its ligand, thus inhibiting its negative regulation that in turn reduces the regulatory T cell (Treg)–mediated suppressive response and activates the production and differentiation of T cells (37).
PD-1 receptor
PD-1 is a Type-1 integral polytopic protein that is regulated by the PDCD1 gene (38). It belongs to the family of CD28/CTLA-4 immunoglobulin antibodies and limits the expression of immune-mediated T cells. Based on the structure, it is divided into three main regions: an intracellular domain, an extracellular region containing IgV, and an aquaphobic cytoplasmic domain (39).
PD-1, a transmembrane protein has two ligands: PD-L1 and PD-L2. Both of these ligands work together to inhibit the immune system in response to external stimuli. PD-L1 is present on B cells, macrophages, resting T cells, vascular endothelial cells, and on islets of Langerhans in the pancreas, whereas PD-L2 is expressed only on dendritic cells (DCs) and on macrophages. As a result, it is less dominant in tumor cells as compared with PD-L1 ligand (40, 41).
Management, control, and expression of programmed death-1 (PD-1/PD-L1) pathway
In NSCLC, the expression of PD-L1 is regulated by a variety of inflammatory chemicals such as TNF-α, VEGF, cytokines IL-4, and IL-10 along with the most powerful chemical: IFN-γ (42, 43). The overexpression of PD-L1 in the surroundings of cancerous cells stimulates the inhibition of immune response and produces an “acquired immunity resistance” effect. In normal immune response, activated T cells produce interferon-gamma to kill the antigen, but an enhanced expression of PD-L1 in the micro-environment of cancerous cells promotes protection and proliferation of cancer cells (44).
In normal cells, the induction of PD-L1 is restricted by a gene named PTEN (phosphatase and tension homolog deleted on chromosome 10) gene. In lung cancer, the PTEN gene gets altered repeatedly. This mutated PTEN gene stimulates the S6K1 gene (45), enhances the PD-L1 mRNA translation, and causes overexpression of PD-L1 in the plasma membrane (46).
Generally, PD-1 restricts our immune system and induces self-resistance in response to infection (47). In cancerous cells, enhanced expression of PD-L1 and PD-L2 promotes their binding to activated T cells and restricts T-cell stimulation. This will facilitate the escape of the tumor from the T-cell invasion and promotes its proliferation and multiplication (48).
Immunotherapeutic drugs against programmed death-1 (PD-1) pathway
Immunoglobulins that target PD-1 transmembrane protein
Pembrolizumab
It is a type of immunoglobulin that is formed in the laboratory by the fusion of fragments of antibodies obtained from humans and rats and is monoclonal. It shows a high affinity toward PD-1 transmembrane protein and selectively binds with it. PD-1 protein has an alteration at C228P that is aimed at reducing Antibody-Dependent Cellular Cytotoxicity (ADCC) regulated by the Fc receptor on the surface of the antibody. The safety profile of Pembrolizumab and its activity against hard tumors is still under clinical research trials (49).
Nivolumab
It is an IgG4-humanized monoclonal immunoglobulin that is deprived of antibody-dependent cellular cytotoxicity (ADCC) and is sold under the brand Opvido. It binds with PD-1 transmembrane and restricts the activity of PD-1 on tumor-infiltrating lymphocytes (TIL) inside the cancerous cells of the lungs. This will prevent the escape of cancerous cells from our immune system through the production of T cells and the release of cytokines (50).
On 4 March 2015, U.S. Food and Drug Administration (FDA) has approved the safety and efficacy of nivolumab against NSCLC (49).
Atezolizumab
Atezolizumab is a fully humanized immunoglobulin monoclonal antibody that has a modified Fc domain that prevents the binding of Fc-receptor (51). Atezolizumab treatment has also demonstrated superior results in various cancer types with high levels of PD-L2 expression (52). In a phase 1b trial conducted to determine its safety, atezolizumab and chemotherapy were combined as the first line of treatment for NSCLC patients. Patients got atezolizumab in addition to 4–6 doses of chemotherapy that was platinum based, then atezolizumab as a maintenance treatment was given. Grades 3–4 toxicity was found in up to 13% of patients, with most of these cases being hematological and connected to chemotherapy. There was one recorded death from candidemia following an extended neutropenia (53). In a phase I clinical study with atezolizumab, the most common adverse effects were found to be dehydration, restlessness, shortness of breath, and pericardial effusion. No death was reported in this study. Regardless of PD-L1 expression, atezolizumab has recently been tested in combination with chemotherapy and bevacizumab in patients with metastatic non-squamous NSCLC that has not previously been treated.
Durvalumab
Durvalumab currently being evaluated for multiple malignancy treatment is an IgG1 monoclonal antibody that shows high affinity toward PD-L1 receptor. Durvalumab has a distinctive immunomodulatory activity and is potentially used as immune checkpoint inhibitor in cancer immunotherapy. In 2017, in United States, durvalumab was approved for advanced urothelial cnacer but was withdrawn in 2021 due to failure in improvement in survival. The indications were then expanded to include refractory NSCLC and SCLC. Some common side effects are associated with durvalumab including fatigue, headache, pain, diarrhea, weight loss, and so forth (54).
Immunoglobulins that block the PD-L1 pathway
An alternative treatment methodology that involves binding the therapeutic antibody to the PD-L1 receptor instead of PD-1 transmembrane protein is now introduced to restrict PD-1 activity. This targeted therapeutic method is more beneficial as compared with the inhibition of PD-1 protein, as it will reduce the immune response only in the surroundings of the cancerous cells. Hence, less damage to the normal cells occurs. Following antibodies are used, which selectively bind with the PD-L1 receptor.
MEDI4736
It is IgG1 immunoglobulin that is humanized in nature and binds specifically with the PD-L1 receptor. The Fc region of MEDI4736 is made in such a way that it will not produce
ADCC (antibody-dependent cell cytotoxicity) (55). Recent research suggests that its frequent use will help to decrease the size of the tumor even in the initial phase of treatment (56).
BMS-936559
It is highly specific IgG4 monoclonal immunoglobulin that is fully obtained from a human source and selectively binds with the PD-L1 receptor to restrict the attachment of PD-1 and PD-L1 to T cells of our immune system (57).
LAG-3
LAG-3 (lymphocyte-activation gene 3) having the lg-like domains 1–4 was discovered in 1990 and is also known as CD223. One of the domains of LAG-3, the domain 1 consists of additional ~30 sequence of amino acids, which is termed as extra loop. The cells expressing LAG-3 include the CD4+ and CD8+ T cells. The activated B cells, Tregs, plasmocytoid DCs, and NK T cells have also shown expressions of cell surface LAG-3. The potential ligands discovered for LAG-3 are the stable peptide-major histocompatibility complex class II (MHC-II), galectin-3, liver sinusoidal endothelial cell lectin (LSECtin), and fibrinogen-like protein 1. The tumor escape mechanism for lag-3 is similar to that of PD-1 acting as a crucial tumor therapeutic target after PD-1. Work is in progress to verify the effectiveness of LAG-3 immune checkpoint inhibitors either alone or in combination in clinical trials (58).
TIM-3
The TIM family of immunoregulatory proteins TIM-3 (T-cell immunoglobulin and mucin domain 3), a type I transmembrane protein, was first discovered in 2002 and have shown expression on T cells, NK cell, B cells, monocytes, and DCs (58). The ligands reported for TIM-3 and their mechanism have been summarized in Figure 3.
TGIT
TIGIT (T-cell immunoreceptor with immunoglobulin and ITIM domain) belongs to a poliovirus receptor-like protein family and is also called as WUCAM, Vstm3, and VSIG9. These proteins have an important role in restricting immune-related processes. TGIT was first discovered in 2009 and have an extracellular immunoglobulin variable domain. It belongs to the groups of checkpoints that are involved in the inhibition of NK and T-cell activation. The domains of TGIT are the transmembrane domain type 1, a cytoplasmic tail having two inhibitory motifs, one inhibitory motif of immunoreceptor tyrosine based, and one immunoglobulin tyrosine tail (ITT)–like motif. The expression of TGIT is found in activated CD4+ T and CD8+ T cells, follicular helper cells, NK cells, and Tregs (58) (Figure 4).
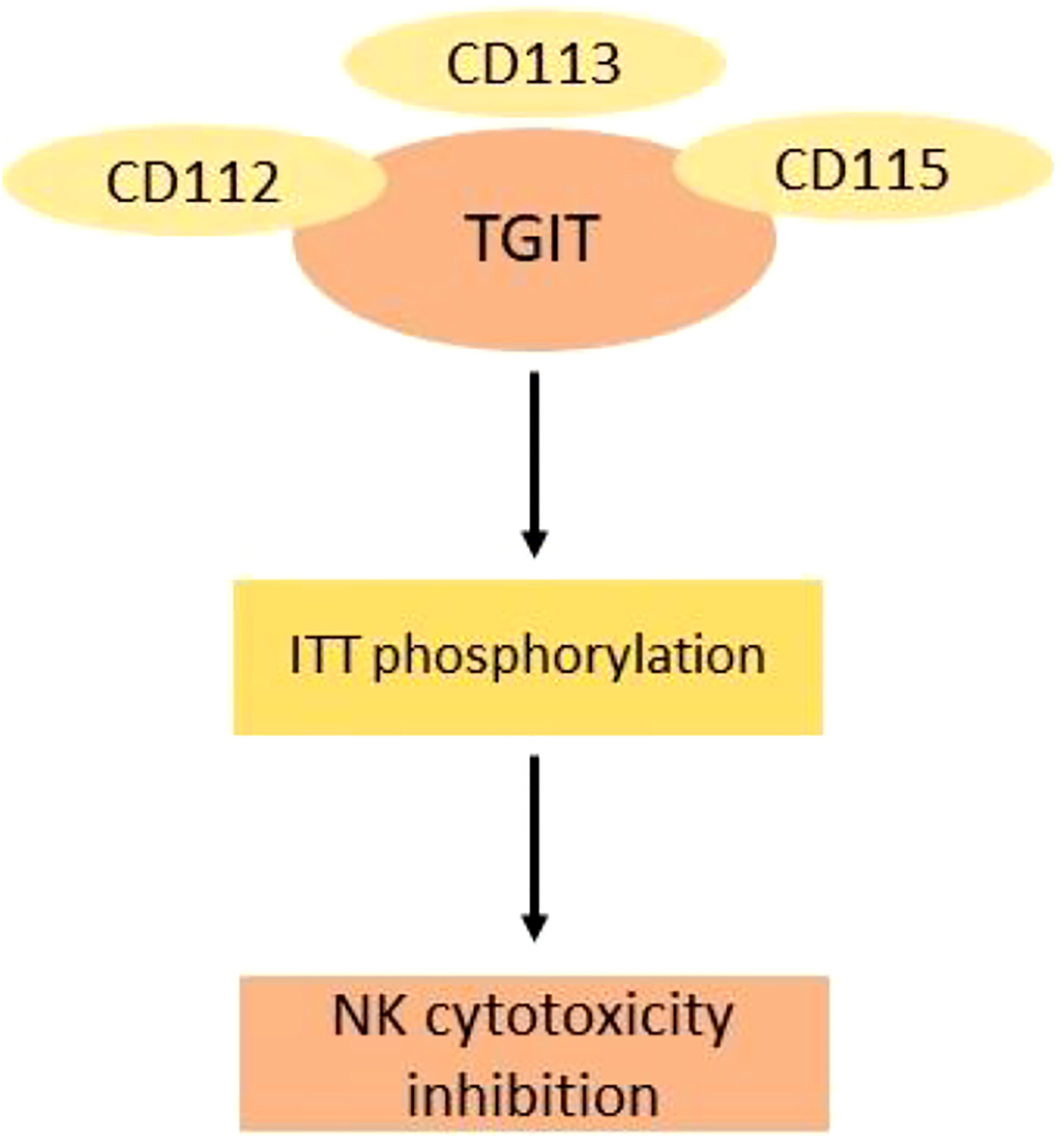
Figure 4 Mechanism of inhibition of cytotoxic NK cells by TGIT ligands. The binding of TGIT with its ligand in NK cells is inhibited by phosphorylation of ITT. CD115 has the highest affinity for TGIT.
Combination therapy for NSCLC
A novel approach of combination therapy has been employed nowadays to increase the clinical effectiveness of immunotherapeutic drugs and reducing their toxicity (59, 60). This involves the combination of PD-1/PD-L1 inhibitors with other chemotherapeutic drugs such as anti-angiogenic inhibitors, platinum-based cytotoxic drugs, immunomodulatory cytokines (IFN-y), and inhibitors of immune system checkpoints (61, 62). Research suggests that the combination of PD-1/PD-L1 inhibitors with immunomodulatory drugs will provide the most promising therapeutic results in NSCLC patients (63).
Therapies targeting the CTLA-4 and PD-1 receptors act on different receptors; thus, they have the potential to be used simultaneously for the synergistic action to treat advanced cancer. A study conducted by Das et al. showed that proliferative signal induced by CTLA-4 inhibition was found in transitional memory T cells, whereas PD-1 inhibition was connected to changes in genes thought to be involved in cytolysis and natural killer cell function. Thus, the dual blockade resulted in nonoverlapping changes in gene expression (64). Both anti–CTLA-4 and anti–PD-1 compounds have different impact on the level of cytokines. Preclinical studies have also showed the enhanced anticancer effect of dual therapy of anti PD-1 and anti CTLA-4 in comparison with single agent treatment (65).
Anti–PD-1 along with anti–CTLA-4 inhibitors offer superior toxicity profiles against lung cancer cells, longer overall survival rates, and higher objective response rates when compared with chemotherapy (66). Thus, it is the new standard of care for patients with newly diagnosed advanced NSCLC, provided they are in generally good health and do not have any contraindications to immunotherapeutic treatment (67).
IMpower 130 was evaluated in a study for the therapeutic efficacy of platinum-based chemotherapy in combination with atezolizumab for advanced NSCLC showing improved OS (overall survival) and PFS (progression free survival) in the combination group as compared with the treatment with chemotherapeutic agent alone (68). From this study, the U.S. FDA also approved the combination therapy of chemimmunotherapy with antiangiogenic agent for treating the nonsquamous NSCLC. The combination therapy including carboplatin-paclitexel-atezolizumab and bevacizumab also showed better OS and PFS (69). In another study, Keynote 189, promising therapeutic effects were observed for the chemimmunotherpay as compared with chemotherapy alone in NSCLC and were also the first clinical trial for chemimmunotherapy. The combination pembrolizumabd and platinum-based chemotherapy was used showing improved OS and PFS of NSCLC patients (70).
Discussion and conclusion
After decades of hard work and several researches, now, immunotherapy has become a reality, an effective treatment for treating lung carcinoma, and to eradicate cancer cells. Blockade of immune checkpoints has transformed lung cancer treatment. They have proven themselves more efficacious than the standard of care chemotherapeutic treatment. This treatment treats not only NSCLC but also SCLC in its extensive stage (either in the form of monotherapy or in combination). However, like other anti-cancer drugs, they have adverse effects (71). The adverse effects associated with immunotherapy are as a result of inflammation in response to immune hyperactivation. These adverse events are collectively termed as the immune-related adverse events (irAEs). Four probable mechanisms have been described for irAEs, but the exact pathophysiology involved in these events is unknown. The enhancement of preexisting antibody levels due to modulation of humoral immunity, the cross reactivity of T cells because of similar antigen, increase in the number of inflammatory cytokines, and the complement-mediated inflammation enhancement are the possible mechanisms leading toward the adverse events due to immunotherapy (72). For example, CTLA-4 inhibition can cause inflammation of colon, whereas PD-1 inhibition leads to pneumonitis that can be fatal. Because patients with lung cancer usually have low lung reserve due to current or past smoking history, pneumonitis is a particular concern in this population. Pneumonitis can thereby further worsen an already inadequate lung reserve and, in extreme situations, even result in death. Despite this worry, pneumonitis is an uncommon occurrence. In case of severe adverse effects, it is necessary to stop the immunotherapy or treatment with corticosteroid is essential (73). The meta-analysis on nivolumab by Zhao et al. proved a low risk of adverse effects when compared with the chemotherapy; however, some of them can be fatal and cannot be ignored (74).
Keeping in view their benefits in treating lung carcinoma, their future either in combination or as monotherapy seems to be bright in lung carcinoma. However, their true potential can be determined by additional studies with anti–PD-1 receptors. The future research can be focused on determining the answers to questions such as determination of the mechanism of immunotherapy resistance. Although the road to effective immunotherapy for lung cancer has not been easy, both animal and human researches have taught us important insights. Thanks to a greater understanding of lung cancer immunoescape and immunosubversion, together with cancer immunosurveillance, immunoediting, and methods to reawaken cancer immunity, we may now embark on a future full of possibilities in using immunotherapy as effective lung cancer treatment.
Author contributions
SM and UI: Study design. RA and SS: Conceptualization, Study design. YD: Project supervision, administration. All authors contributed to the article and approved the submitted version.
Conflict of interest
The authors declare that the research was conducted in the absence of any commercial or financial relationships that could be construed as a potential conflict of interest.
Publisher’s note
All claims expressed in this article are solely those of the authors and do not necessarily represent those of their affiliated organizations, or those of the publisher, the editors and the reviewers. Any product that may be evaluated in this article, or claim that may be made by its manufacturer, is not guaranteed or endorsed by the publisher.
References
1. Torre LA, Bray F, Siegel RL, Ferlay J, Lortet-Tieulent J, Jemal A. Global cancer statistics, 2012. CA: Cancer J Clin (2015) 65(2):87–108. doi: 10.3322/caac.21262
2. de Groot PM, Wu CC, Carter BW, Munden RF. The epidemiology of lung cancer. Trans Lung Cancer Res (2018) 7(3):220. doi: 10.21037/tlcr.2018.05.06
3. Zhou Y, Chen C, Zhang X, Fu S, Xue C, Ma Y, et al. Immune-checkpoint inhibitor plus chemotherapy versus conventional chemotherapy for first-line treatment in advanced non-small cell lung carcinoma: A systematic review and meta-analysis. J immunother. cancer (2018) 6(1):1–11. doi: 10.1186/s40425-018-0477-9
4. Globocan. Estimated cancer incidence, mortality and prevalence worldwide 2012 (2012). Available at: http://globocan.iarc.fr/Pages/fact_sheets_population.aspx.
5. Goldstraw P, Crowley J, Chansky K, Giroux DJ, Groome PA, Rami-Porta R, et al. The IASLC lung cancer staging project: proposals for the revision of the TNM stage groupings in the forthcoming (seventh) edition of the TNM classification of malignant tumours. J Thorac Oncol (2007) 2(8):706–14. doi: 10.1097/JTO.0b013e31812f3c1a
6. Abbas M, Kassim SA, Habib M, Li X, Shi M, Wang Z-C, et al. Clinical evaluation of serum tumor markers in patients with advanced-stage non-small cell lung cancer treated with palliative chemotherapy in China. Front Oncol (2020) 10:800. doi: 10.3389/fonc.2020.00800
7. Abbas M, Kassim SA, Wang ZC, Shi M, Hu Y, Zhu HL. Clinical evaluation of plasma coagulation parameters in patients with advanced-stage non-small cell lung cancer treated with palliative chemotherapy in China. Int J Clin Pract. (2020) 74(12):e13619. doi: 10.1111/ijcp.13619
8. Lee CK, Brown C, Gralla RJ, Hirsh V, Thongprasert S, Tsai C-M, et al. Impact of EGFR inhibitor in non–small cell lung cancer on progression-free and overall survival: A meta-analysis. J Natl Cancer Inst. (2013) 105(9):595–605. doi: 10.1093/jnci/djt072
9. Solomon BJ, Mok T, Kim D-W, Wu Y-L, Nakagawa K, Mekhail T, et al. First-line crizotinib versus chemotherapy in ALK-positive lung cancer. N Engl J Med (2014) 371:2167–77. doi: 10.1056/NEJMoa1408440
10. Shaw AT, Kim D-W, Mehra R, Tan DS, Felip E, Chow LQ, et al. Ceritinib in ALK-rearranged non–small-cell lung cancer. N Engl J Med (2014) 370:1189–97. doi: 10.1056/NEJMoa1311107
11. Abbas M, Baig MMFA, Zhang Y, Yang Y-S, Wu S, Hu Y, et al. A DNA-based nanocarrier for efficient cancer therapy. J Pharm Anal. (2021) 11(3):330–9. doi: 10.1016/j.jpha.2020.03.005
12. Matsushita H, Vesely MD, Koboldt DC, Rickert CG, Uppaluri R, Magrini VJ, et al. Cancer exome analysis reveals a T-cell-dependent mechanism of cancer immunoediting. Nature (2012) 482(7385):400–4. doi: 10.1038/nature10755
13. Hanahan D, Weinberg RA. Hallmarks of cancer: The next generation. cell (2011) 144(5):646–74. doi: 10.1016/j.cell.2011.02.013
14. Holt GE, Podack ER, Raez LE. Immunotherapy as a strategy for the treatment of non-small-cell lung cancer. Therapy (2011) 8(1):43. doi: 10.2217/thy.10.84
15. Aktar N, Yueting C, Abbas M, Zafar H, Paiva-Santos AC, Zhang Q, et al. Understanding of immune escape mechanisms and advances in cancer immunotherapy. J Oncol (2022) 2022:13. doi: 10.1155/2022/8901326
16. Vansteenkiste J, Zielinski M, Linder A, Dahabreh J, Gonzalez EE, Malinowski W, et al. Adjuvant MAGE-A3 immunotherapy in resected non–small-cell lung cancer: Phase II randomized study results. J Clin Oncol (2013) 31(19):2396–403. doi: 10.1200/JCO.2012.43.7103
17. Fallarino F, Fields PE, Gajewski TF. B7-1 engagement of cytotoxic T lymphocyte antigen 4 inhibits T cell activation in the absence of CD28. J Exp Med (1998) 188(1):205–10. doi: 10.1084/jem.188.1.205
18. Walunas TL, Lenschow DJ, Bakker CY, Linsley PS, Freeman GJ, Green JM, et al. CTLA-4 can function as a negative regulator of T cell activation. Immunity> (1994) 1(5):405–13. doi: 10.1016/1074-7613(94)90071-X
19. Krummel MF, Allison JP. CD28 and CTLA-4 have opposing effects on the response of T cells to stimulation. J Exp Med (1995) 182(2):459–65. doi: 10.1084/jem.182.2.459
20. Linsley PS, Greene J, Tan P, Bradshaw J, Ledbetter JA, Anasetti C, et al. Coexpression and functional cooperation of CTLA-4 and CD28 on activated T lymphocytes. J Exp Med (1992) 176(6):1595–604. doi: 10.1084/jem.176.6.1595
21. Boasso A, Herbeuval J-P, Hardy AW, Winkler C, Shearer GM. Regulation of indoleamine 2, 3-dioxygenase and tryptophanyl-tRNA-synthetase by CTLA-4-Fc in human CD4+ T cells. Blood (2005) 105(4):1574–81. doi: 10.1182/blood-2004-06-2089
22. Grohmann U, Orabona C, Fallarino F, Vacca C, Calcinaro F, Falorni A, et al. CTLA-4–ig regulates tryptophan catabolism in vivo. Nat Immunol (2002) 3(11):1097–101. doi: 10.1038/ni846
23. Li D, Gál I, Vermes C, Alegre M-L, Chong AS, Chen L, et al. Cutting edge: Cbl-b: one of the key molecules tuning CD28-and CTLA-4-mediated T cell costimulation. J Immunol (2004) 173(12):7135–9. doi: 10.4049/jimmunol.173.12.7135
24. Parry RV, Chemnitz JM, Frauwirth KA, Lanfranco AR, Braunstein I, Kobayashi SV, et al. CTLA-4 and PD-1 receptors inhibit T-cell activation by distinct mechanisms. Mol Cell Biol (2005) 25(21):9543–53. doi: 10.1128/MCB.25.21.9543-9553.2005
25. Masteller EL, Chuang E, Mullen AC, Reiner SL, Thompson CB. Structural analysis of CTLA-4 function in vivo. J Immunol (2000) 164(10):5319–27. doi: 10.4049/jimmunol.164.10.5319
26. Weber J. Anti–CTLA-4 antibody ipilimumab: case studies of clinical response and immune-related adverse events. oncol. (2007) 12(7):864–72. doi: 10.1634/theoncologist.12-7-864
27. Cameron F, Whiteside G, Perry C. Ipilimumab. Drugs (2011) 71(8):1093–104. doi: 10.2165/11594010-000000000-00000
28. Tomasini P, Khobta N, Greillier L, Barlesi F. Ipilimumab: Its potential in non-small cell lung cancer. Ther Adv Med Oncol (2012) 4(2):43–50. doi: 10.1177/1758834011431718
29. Hodi FS, O'day SJ, McDermott DF, Weber RW, Sosman JA, Haanen JB, et al. Improved survival with ipilimumab in patients with metastatic melanoma. New Engl J Med (2010) 363(8):711–23. doi: 10.1056/NEJMoa1003466
30. Robert C, Thomas L, Bondarenko I, O'Day S, Weber J, Garbe C, et al. Ipilimumab plus dacarbazine for previously untreated metastatic melanoma. New Engl J Med (2011) 364(26):2517–26. doi: 10.1056/NEJMoa1104621
31. Iwama S, De Remigis A, Callahan M, Slovin S, Wolchok J, Caturegli P. Pituitary expression of CTLA-4 mediates hypophysitis secondary to administration of CTLA-4 blocking antibody. Sci Transl Med (2014) 6:230ra45. doi: 10.1126/scitranslmed.3008002
32. Topalian SL, Sznol M, McDermott DF, Kluger HM, Carvajal RD, Sharfman WH, et al. Survival, durable tumor remission, and long-term safety in patients with advanced melanoma receiving nivolumab. J Clin Oncol (2014) 32(10):1020. doi: 10.1200/JCO.2013.53.0105
33. Lacouture ME, Wolchok JD, Yosipovitch G, Kähler KC, Busam KJ, Hauschild A. Ipilimumab in patients with cancer and the management of dermatologic adverse events. J Am Acad Dermatol (2014) 71(1):161–9. doi: 10.1016/j.jaad.2014.02.035
34. Tarhini A. Immune-mediated adverse events associated with ipilimumab ctla-4 blockade therapy: The underlying mechanisms and clinical management. Scientifica (2013) 2013:19. doi: 10.1155/2013/857519
35. Bertrand A, Kostine M, Barnetche T, Truchetet M-E, Schaeverbeke T. Immune related adverse events associated with anti-CTLA-4 antibodies: Systematic review and meta-analysis. BMC Med (2015) 13(1):1–14. doi: 10.1186/s12916-015-0455-8
36. Ribas A, Hanson DC, Noe DA, Millham R, Guyot DJ, Bernstein SH, et al. Tremelimumab (CP-675,206), a cytotoxic T lymphocyte–associated antigen 4 blocking monoclonal antibody in clinical development for patients with cancer. oncol. (2007) 12(7):873–83. doi: 10.1634/theoncologist.12-7-873
37. Fife BT, Bluestone JA. Control of peripheral T-cell tolerance and autoimmunity via the CTLA-4 and PD-1 pathways. Immunol Rev (2008) 224(1):166–82. doi: 10.1111/j.1600-065X.2008.00662.x
38. Ceeraz S, Nowak EC, Noelle RJ. B7 family checkpoint regulators in immune regulation and disease. Trends Immunol (2013) 34(11):556–63. doi: 10.1016/j.it.2013.07.003
39. Riley JL. PD-1 signaling in primary T cells. Immunol Rev (2009) 229(1):114–25. doi: 10.1111/j.1600-065X.2009.00767.x
40. Velcheti V, Schalper KA, Carvajal DE, Anagnostou VK, Syrigos KN, Sznol M, et al. Programmed death ligand-1 expression in non-small cell lung cancer. Lab Invest (2014) 94(1):107–16. doi: 10.1038/labinvest.2013.130
41. Boland JM, Kwon ED, Harrington SM, Wampfler JA, Tang H, Yang P, et al. Tumor B7-H1 and B7-H3 expression in squamous cell carcinoma of the lung. Clin Lung cancer (2013) 14(2):157–63. doi: 10.1016/j.cllc.2012.05.006
42. Sznol M, Chen L. Antagonist antibodies to PD-1 and B7-H1 (PD-L1) in the treatment of advanced human cancer. Clin Cancer Res (2013) 19(5):1021–34. doi: 10.1158/1078-0432.CCR-12-2063
43. Kondo A, Yamashita T, Tamura H, Zhao W, Tsuji T, Shimizu M, et al. Interferon-γ and tumor necrosis factor-α induce an immunoinhibitory molecule, B7-H1, via nuclear factor-κB activation in blasts in myelodysplastic syndromes. Blood J Am Soc Hematol. (2010) 114(22):2766.
44. Taube JM, Anders RA, Young GD, Xu H, Sharma R, McMiller TL, et al. Colocalization of inflammatory response with B7-h1 expression in human melanocytic lesions supports an adaptive resistance mechanism of immune escape. Sci Trans Med (2012) 4(127):127ra37–ra37. doi: 10.1126/scitranslmed.3003689
45. Crane C, Panner A, Murray J, Wilson S, Xu H, Chen L, et al. PI (3) kinase is associated with a mechanism of immunoresistance in breast and prostate cancer. Oncogene (2009) 28(2):306–12. doi: 10.1038/onc.2008.384
46. Parsa AT, Waldron JS, Panner A, Crane CA, Parney IF, Barry JJ, et al. Loss of tumor suppressor PTEN function increases B7-H1 expression and immunoresistance in glioma. Nat Med (2007) 13(1):84–8. doi: 10.1038/nm1517
47. Ramsay AG. Immune checkpoint blockade immunotherapy to activate anti-tumour T-cell immunity. Br J haematol. (2013) 162(3):313–25. doi: 10.1111/bjh.12380
48. Faulkner C, Palmer A, Williams H, Wragg C, Haynes HR, White P, et al. EGFR and EGFRvIII analysis in glioblastoma as therapeutic biomarkers. Br J neurosurg. (2015) 29(1):23–9. doi: 10.3109/02688697.2014.950631
49. Garon EB, Rizvi NA, Hui R, Leighl N, Balmanoukian AS, Eder JP, et al. Pembrolizumab for the treatment of non–small-cell lung cancer. New Engl J Med (2015) 372(21):2018–28. doi: 10.1056/NEJMoa1501824
50. Zhang Y, Huang S, Gong D, Qin Y, Shen Q. Programmed death-1 upregulation is correlated with dysfunction of tumor-infiltrating CD8+ T lymphocytes in human non-small cell lung cancer. Cell Mol Immunol (2010) 7(5):389–95. doi: 10.1038/cmi.2010.28
51. Reichert JM. Antibodies to watch in 2016. MAbs (2016). 8, 197–204. doi: 10.1080/19420862.2015.1125583
52. Schmid P, Hegde PS, Zou W, Kowanetz M, Mariathasan S, Molinero L, et al. Association of PD-L2 expression in human tumors with atezolizumab activity. Am Soc Clin Oncol (2016) 11506. doi: 10.1200/JCO.2016.34.15_suppl.11506
53. Bendell JC, Powderly JD, Lieu CH, Eckhardt SG, Hurwitz H, Hochster HS, et al. Safety and efficacy of MPDL3280A (anti-PDL1) in combination with bevacizumab (bev) and/or FOLFOX in patients (pts) with metastatic colorectal cancer (mCRC). Am Soc Clin Oncol (2015) 2015:704. doi: 10.1200/jco.2015.33.3_suppl.704
54. Faiena I, Cummings AL, Crosetti AM, Pantuck AJ, Chamie K, Drakaki A. Durvalumab: An investigational anti-PD-L1 monoclonal antibody for the treatment of urothelial carcinoma. Drug Design Dev Ther (2018) 12:209. doi: 10.2147/DDDT.S141491
55. Herbst RS, Gordon MS, Fine GD, Sosman JA, Soria J-C, Hamid O, et al. A study of MPDL3280A, an engineered PD-L1 antibody in patients with locally advanced or metastatic tumors. Am Soc Clin Oncol (2013) 3000. doi: 10.1200/jco.2013.31.15_suppl.3000
56. He J, Hu Y, Hu M, Li B. Development of PD-1/PD-L1 pathway in tumor immune microenvironment and treatment for non-small cell lung cancer. Sci Rep (2015) 5(1):1–9. doi: 10.1038/srep13110
57. Brahmer JR, Tykodi SS, Chow LQ, Hwu W-J, Topalian SL, Hwu P, et al. Safety and activity of anti–PD-L1 antibody in patients with advanced cancer. New Engl J Med (2012) 366(26):2455–65. doi: 10.1056/NEJMoa1200694
58. Ma L-R, Li J-X, Tang L, Li R-Z, Yang J-S, Sun A, et al. Immune checkpoints and immunotherapy in non−small cell lung cancer: Novel study progression, challenges and solutions. Oncol Letters. (2021) 22(5):1–11.
59. Li X, Abbas M, Li Y, Teng Y, Fang Y, Yu S, et al. Comparative effectiveness of pemetrexed-platinum doublet chemotherapy with or without bevacizumab as first-line therapy for treatment-naive patients with advanced nonsquamous non–small-cell lung cancer in China. Clin Ther (2019) 41(3):518–29. doi: 10.1016/j.clinthera.2019.02.004
60. Li X, Zhao X, Abbas M, Wang L, Li C, Liu S, et al. Comparative effectiveness study of single high-dose cisplatin with fractionated doses cisplatin in first-line therapy for treatment-naive Chinese patients with advanced non–small-cell lung cancer. Curr Problems Cancer (2019) 43(6):100466. doi: 10.1016/j.currproblcancer.2019.01.007
61. Deng L, Liang H, Burnette B, Beckett M, Darga T, Weichselbaum RR, et al. Irradiation and anti–PD-L1 treatment synergistically promote antitumor immunity in mice. J Clin Invest (2014) 124(2):687–95. doi: 10.1172/JCI67313
62. Vanneman M, Dranoff G. Combining immunotherapy and targeted therapies in cancer treatment. Nat Rev cancer (2012) 12(4):237–51. doi: 10.1038/nrc3237
63. Bald T, Landsberg J, Lopez-Ramos D, Renn M, Glodde N, Jansen P, et al. Immune cell–poor melanomas benefit from PD-1 blockade after targeted type I IFN ActivationCombination immunotherapy for immune cell–poor melanomas. Cancer discov. (2014) 4(6):674–87. doi: 10.1158/2159-8290.CD-13-0458
64. Das R, Verma R, Sznol M, Boddupalli CS, Gettinger SN, Kluger H, et al. Combination therapy with anti–CTLA-4 and anti–PD-1 leads to distinct immunologic changes in vivo. J Immunol (2015) 194(3):950–9. doi: 10.4049/jimmunol.1401686
65. Curran MA, Montalvo W, Yagita H, Allison JP. PD-1 and CTLA-4 combination blockade expands infiltrating T cells and reduces regulatory T and myeloid cells within B16 melanoma tumors. Proc Natl Acad Sci (2010) 107(9):4275–80. doi: 10.1073/pnas.0915174107
66. Rittmeyer A, Barlesi F, Waterkamp D, Park K, Ciardiello F, Von Pawel J, et al. Atezolizumab versus docetaxel in patients with previously treated non-small-cell lung cancer (OAK): a phase 3, open-label, multicentre randomised controlled trial. Lancet (2017) 389(10066):255–65. doi: 10.1016/S0140-6736(16)32517-X
67. Ackermann CJ, Reck M, Paz-Ares L, Barlesi F, Califano R. First-line immune checkpoint blockade for advanced non-small-cell lung cancer: Travelling at the speed of light. Lung Cancer (2019) 134:245–53. doi: 10.1016/j.lungcan.2019.06.007
68. West H, McCleod M, Hussein M, Morabito A, Rittmeyer A, Conter HJ, et al. Atezolizumab in combination with carboplatin plus nab-paclitaxel chemotherapy compared with chemotherapy alone as first-line treatment for metastatic non-squamous non-small-cell lung cancer (IMpower130): A multicentre, randomised, open-label, phase 3 trial. Lancet Oncol (2019) 20(7):924–37. doi: 10.1016/S1470-2045(19)30167-6
69. Socinski MA, Jotte RM, Cappuzzo F, Orlandi F, Stroyakovskiy D, Nogami N, et al. Atezolizumab for first-line treatment of metastatic nonsquamous NSCLC. New Engl J Med (2018) 378(24):2288–301. doi: 10.1056/NEJMoa1716948
70. Gandhi L, Rodríguez-Abreu D, Gadgeel S, Esteban E, Felip E, De Angelis F, et al. Pembrolizumab plus chemotherapy in metastatic non–small-cell lung cancer. New Engl J Med (2018) 378(22):2078–92. doi: 10.1056/NEJMoa1801005
71. Wang L, Abbas M, Wang F, Mikrani R, Li X, Fan L, et al. Real-life experience with nivolumab monotherapy in patients with advanced lung cancer: Efficacy and immune-related adverse events at jiangsu cancer hospital, nanjing, China. Curr Med Res Pract. (2020) 10(3):93–9. doi: 10.1016/j.cmrp.2020.05.004
72. Teixidor E, Bosch-Barrera J. The dark side of immunotherapy: Challenges facing the new hope in cancer treatment. Ann Trans Med (2019) 7(Suppl 6):S183. doi: 10.21037/atm.2019.07.69
73. Porcu M, De Silva P, Solinas C, Battaglia A, Schena M, Scartozzi M, et al. Immunotherapy associated pulmonary toxicity: Biology behind clinical and radiological features. Cancers (2019) 11(3):305. doi: 10.3390/cancers11030305
Keywords: lung cancer, immune checkpoint inhibitors, immunotherapy, non-small lung cancer, chemotherapy
Citation: Altaf R, Jadoon SS, Muhammad SA, Ilyas U and Duan Y (2022) Recent advances in immune checkpoint inhibitors for non-small lung cancer treatment. Front. Oncol. 12:1014156. doi: 10.3389/fonc.2022.1014156
Received: 17 August 2022; Accepted: 12 September 2022;
Published: 27 September 2022.
Edited by:
Liyun Shi, Nanjing University of Chinese Medicine, ChinaReviewed by:
Zhi-Gang Sun, Linyi Central Hospital, ChinaXiaoyou Li, Nanjing Medical University, China
Copyright © 2022 Altaf, Jadoon, Muhammad, Ilyas and Duan. This is an open-access article distributed under the terms of the Creative Commons Attribution License (CC BY). The use, distribution or reproduction in other forums is permitted, provided the original author(s) and the copyright owner(s) are credited and that the original publication in this journal is cited, in accordance with accepted academic practice. No use, distribution or reproduction is permitted which does not comply with these terms.
*Correspondence: Syed Aun Muhammad, YXVubXVoYW1tYWQ3OEB5YWhvby5jb20=; Umair Ilyas, dW1haXIuaWx5YXNAcmlwaGFoLmVkdS5waw==; Yongtao Duan, ZHVhbnlvbmd0YW84NjA0MDlAMTYzLmNvbQ==