- 1Department of Postgraduates, Bengbu Medical College, Bengbu, China
- 2Department of Hepatobiliary and Pancreatic Surgery and Minimally Invasive Surgery, Zhejiang Provincial People’s Hospital, Affiliated People’s Hospital, Hangzhou Medical College, Hangzhou, China
- 3Cancer Center, General Surgery, Zhejiang Provincial People’s Hospital, Affiliated People’s Hospital, Hangzhou Medical College, Hangzhou, China
- 4Obstetrics and Gynecology, Zhejiang Provincial People’s Hospital, Affiliated People’s Hospital, Hangzhou Medical College, Hangzhou, China
- 5Key Laboratory of Gastroenterology of Zhejiang Province, Zhejiang Provincial People’s Hospital, Affiliated People’s Hospital, Hangzhou Medical College, Hangzhou, China
In most cancers, forkhead box N3 (FOXN3) acts as a transcriptional inhibitor to suppress tumor proliferation, but in pancreatic cancer, the opposite effect is observed. To confirm and investigate this phenomenon, FOXN3 expression in various carcinomas was determined using GEPIA2 and was found to be highly expressed in pancreatic cancer. Kaplan-Meier plotter was then used for survival analysis, revealing that high FOXN3 expression in pancreatic cancer might be associated with a poor prognosis. Similarly, clinical samples collected for immunohistochemical staining and survival analysis showed consistent results. The RNA-seq data of pancreatic cancer patients from the TCGA were then downloaded, and the differential expression gene set was obtained using R for gene set enrichment analysis (GSEA). The intersection of the above gene sets and FOXN3-related genes was defined as related differentially expressed gene sets (DEGs), and enrichment analysis was performed using Gene Ontology (GO) and the Kyoto Encyclopedia of Genes and Genomes (KEGG). Finally, we analyzed the relationship between FOXN3 and immune infiltration in pancreatic cancer. Collectively, our findings reveal that FOXN3 is involved in the occurrence and progression of pancreatic cancer and may be useful as a prognostic tool in pancreatic cancer immunotherapy.
Introduction
Pancreatic cancer (PC) is one of the most deadly malignant tumors, with a five years relative survival rate of only 9% due to the unsatisfactory surgical outcome, difficulties of early diagnosis and high metastasis rate (1). PC rank the third leading cause of cancer-related death, and its incidence has rised over the past years (2). Pancreatic ductal adenocarcinoma (PDAC) accounts for about 85% of all PCs (3). It is, therefore, the most common histological pancreatic cancer subtype representative of PC (4). As a result, understanding the FOXN3-related molecular mechanisms of PDAC is critical for early diagnosis and targeted treatment strategies.
The proteins encoded by the Forkhead box (FOX) gene family are involved in the expression of important genes that regulate the development, proliferation and aging of various organs (5). For instance, the FOXO subfamily is involved in regulating metabolism, antioxidant stress, and cell cycle arrest (6, 7). In contrast, the FOXP subfamily is primarily involved in immune response, with FOXP3 being a key marker of Treg cells and is critical for immune homeostasis and self-tolerance (8). Meanwhile, FOXA has been shown to promote the binding of nuclear hormone receptors (9). As a result, the FOX family has received a lot of attention and is considered a potential therapeutic target in many diseases.
Pati et al. first isolated FOXN3 from yeast cells and identified multiple checkpoint mutations (10). Since FOXN3 has the ability to suppress many DNA damage-activated checkpoint mutations, it is also known as checkpoint suppressor 1 (CHSE1). FOXN3 is a member of the forkhead box family, which has a similar DNA-binding domain and is involved in organ differentiation, development, cell growth, and cancer (11). FOXN is divided into six subclasses (FOXN1–FOXN6), with FOXN3 being the only member lacking transcriptional activation domains (12). Previous studies revealed that FOXN3 could regulate other genes as a transcription factor in a variety of cancers. Moreover, the FOXN3-NEAT1-SIN3A complex was found to promote breast cancer cell diffusion and metastasis in vivo (13). FOXN3 possesses a unique forkhead box region that can interact with the promoter of the E2F5 transcription factor, inhibiting hepatocellular cancer cell proliferation by downregulating E2F5 mRNA and protein expression (14). The downregulation of FOXN3 could inactive the Wnt/β-catenin pathway to promote the growth and invasion of papillary thyroid cancer and colon cancer (15, 16). Nevertheless, the clinical significance of FOXN3 expression in PC remains indistinct. The present study aims to assess the clinicopathological value and prognostic significance of FOXN3 expression in pancreatic cancer. The study workflow is shown in Figure 1.
Materials and methods
GEPIA2 database analysis
GEPIA2, a web server for analyzing the RNA sequencing expression data from The Cancer Genome Atlas (TCGA) and the Genotype-Tissue Expression project (GTEx) (http://gepia2.cancer-pku.cn), was used to analyze the expression and prognosis of FOXN3 in PDAC (17).
Screening of differentially expressed genes (DEGs)
The RNA-seq data of TCGA_PDAC (source: https://xenabrowser.net/datapages/ n=177) was used to identify DEGs between FOXN3 high and low expression subgroups; defined by the median expression level of FOXN3. Using the “DESeq2” R package screened the DEGs, and the heatmap was constructed using the “pheatmap” R package. P <0.05, log FC > 1.5 was used to define significantly up-regulated DEGs, while P<0.05, log FC <-1.5 was used to identify significantly down-regulated DEGs.
Kaplan–Meier plotter
Kaplan-Meier plotter was utilized to estimate the effect of genes on survival in different cancer types (http://kmplot.com) (18). The PDAC samples from TCGA database were divided into two groups according to the cutoff points determined for FOXN3 expression level. Overall survival (OS) was defined as the time from initial diagnosis of PDAC to the time to death or the last follow-up, while recurrence-free survival (RFS) was defined as the time from the first diagnosis to disease relapse. The hazard ratio (HR) and p-value had been labeled.
Enrichment analysis
Gene ontology (GO) analysis consists of three parts, biological processes (BP), cellular component (CC), and molecular function (MF), that explore the biological function of the gene of interest from different aspects. In contrast, Kyoto encyclopedia of genes and genomes (KEGG) pathway analysis is used to further identify enriched biological pathways. Here, we used R to perform GO and KEGG pathway analysis based on DEGs with low expression vs. high expression of FOXN3 and FOXN3-related genes.
TISIDB
The Tumor and Immune System Interaction Data Base (TISIDB) (http://cis.hku.hk/TISIDB/index.php) is an online platform that can be used to explore the relationship between the tumor and the immune system (19). The interaction between tumor cells and the immune system plays an important role in cancer initiation, progression, and treatment, so elucidating the interaction between tumor and immune system will help predict immunotherapy response and develop new immunotherapy targets. In this study, the TISIDB was used to investigate the association of FOXN3 with 28 immune cells.
Patients and specimens
A total of 36 PDAC patients were enrolled from the Zhejiang Province People’s Hospital, China, for tumor and adjacent nontumor tissue collection. All patients were treated for the first time without any antitumor treatment such as chemotherapy and radiotherapy, and were pathologically diagnosed as PDAC after surgery. This research was approved by the Ethics Committee of Zhejiang Province People’s Hospital, and each patient agreed to a written informed consent form (QT2022276). All specimens were paraffin-embedded and fixed.
Immunohistochemical determination
Immunohistochemical (IHC) staining of FOXN3 was conducted on paired tumors and adjacent normal tissues for 36 PDAC cases. IHC analysis was performed to investigate altered protein expression using FOXN3 antibodies (Abcam, 1:100) in 36 paraffin-embedded and archived pancreatic cancers. Two blinded independent pathologists reviewed and graded the immunostaining degree of formalin-fixed and paraffin-embedded sections. The fraction was determined by combining the proportion of positive staining tumor cells with the staining intensity. The scores from the two independent pathologists were combined into an average score for further comparative evaluation. The tumor cell proportion score was as follows: 0, no positive tumor cells; 1, <10% positive tumor cells; 2. 10%~35% positive tumor cells; 3. 35-75% positive tumor cells; 4, >75% positive tumor cells. Dyeing intensity was graded according to the following standards: 1. No dyeing; 2. Weak dyeing (light yellow); 3. Moderate staining (tan); 4. Strong staining (brown). The staining index (SI) was calculated as the product of the staining intensity score and the proportion of positive tumor cells. Using this assessment method, we assessed protein expression in malignant lesions by determining SI, with possible scores of 0, 2, 3, 4, 6, 8, 9, 12, and 16. Then the median of SI of 6 was selected as the cutoff value, and the samples with SI >6 were determined to be highly expressed, while SI ≤ 6 samples were determined to be of low expression.
Quantitative RT-PCR
The expression of FOXN3 in pancreatic cancer and its adjacent tissues was detected by RT-PCR. Briefly, Total RNA(1 ug) was extracted(TRIzol reagent; Invitrogen, Carlsbad, CA, USA) for reverse transcription into cDNA according to the manufacturer’s instructions. Then, to get the expression level of FOXN3 mRNA, we used real-time PCR by the SYBR Green quantitative PCR kit (Invitrogen) and an Applied Biosystems 7300 real-time PCR system (Carlsbad, CA, USA) according to the manufacturer’s instructions. FOXN3 forward primer, 5′-TCTGACATGCCCTACGATGC-3′, reverse primer, 5′-CTATGCACCACAACGACCCT-3′. All data were normalized to the GAPDH (forward primer, 5’-GACAACTTTGGCATCGT-3’; reverse primer, 5’-ATGCAGGGATGATGTTC TGG-3’). Expression level of FOXN3 were calculated by a comparative Ct method.
Results
Distribution of FOXN3 expression in cancer tissues
The TCGA and the GTEx database were used to analyze FOXN3 expression in generalized carcinoma on the GEPIA2 online platform, revealing that FOXN3 was down-regulated in Bladder Urothelial Carcinoma (BLCA), Breast invasive carcinoma (BRCA), Cervical squamous cell carcinoma and endocervical adenocarcinoma (CESC), Colon adenocarcinoma (COAD), etc. In contrast, FOXN3 was up-regulated in Kidney Chromophobe (KICH), Pancreatic adenocarcinoma (PDAC) and Thymoma (YHYM) (Figures 2A, B). Consistently, our IHC results also confirmed that FOXN3 was highly expressed in pancreatic cancer tissue samples (Figure 3A). The results of IHC staining showed that nucleus staining was dominant(Figure 3B). And we used qRT-PCR to understand the mRNA expression level of FOXN3, and the results showed that FOXN3 expression was significantly increased in cancer tissues compared with normal pancreatic tissues (Figure S1).
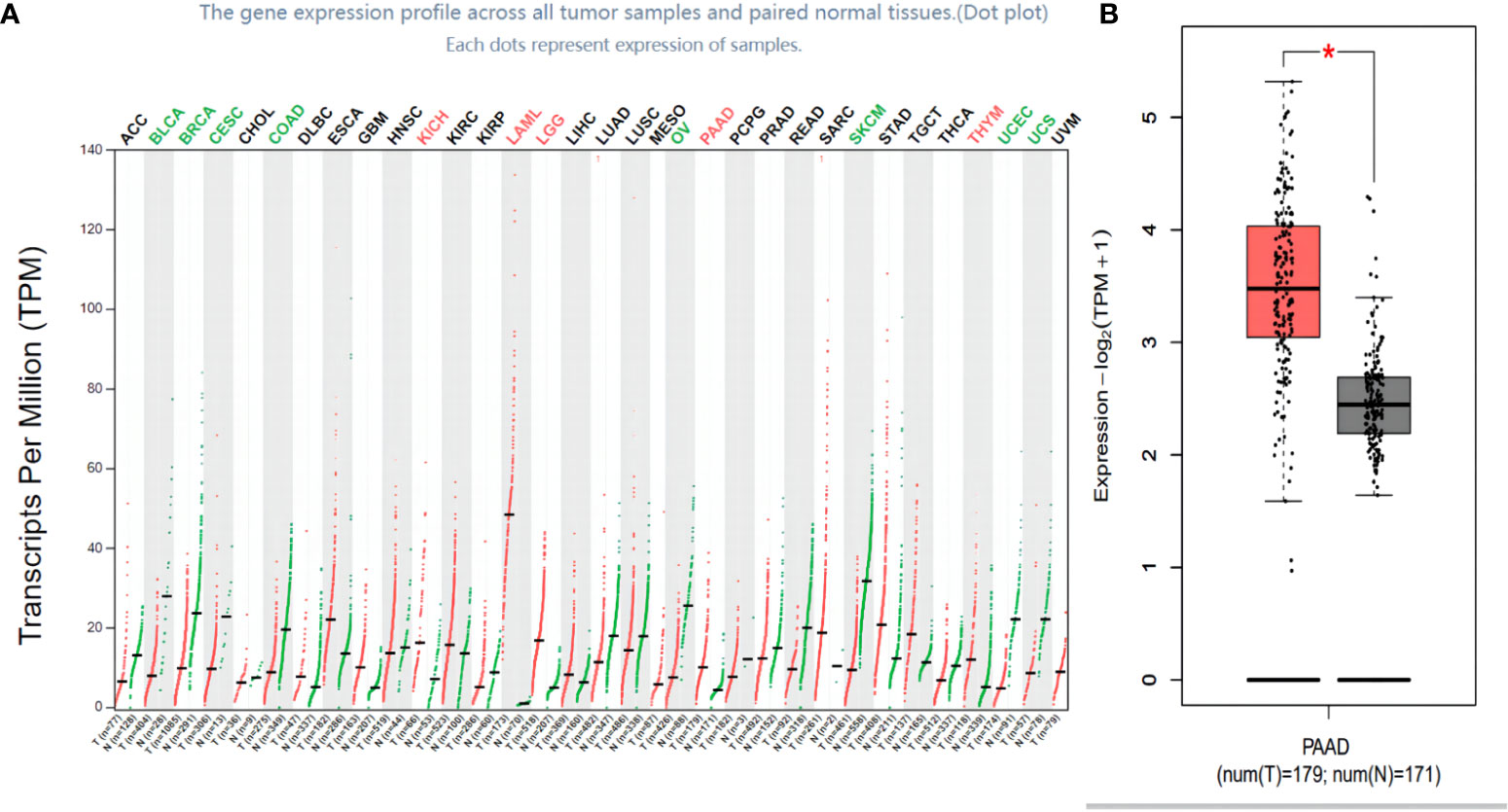
Figure 2 FOXN3 expression in carcinoma tissues. (A) FOXN3 expression in pan cancer in TCGA database. (B) FOXN3 expression in Pancreatic adenocarcinoma(PAAD) in TCGA database. *P < 0.05.
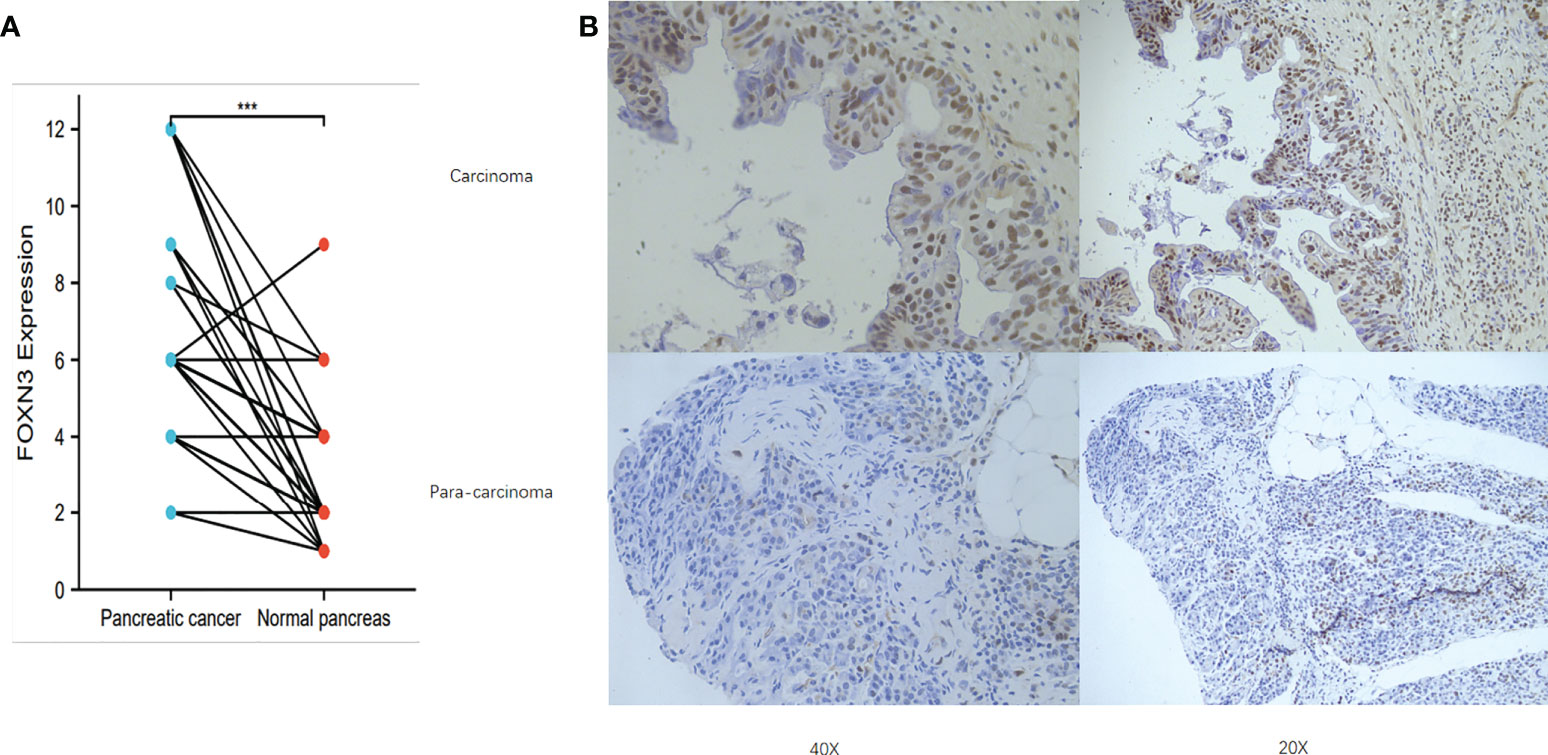
Figure 3 (A) FOXN3 expression in PC tissues and adjust tissues in clinical samples. ***P < 0.005. (B) Immunohistochemistry of FOXN3 expression in PC tissues and adjust tissues.
Relationship between FOXN3 expression and survival in patients with PDAC
Based on the optimal cut-off points provided by Kaplan-Meier Plotter, we divided PDAC patients into high expression and low expression groups for survival analysis. The results showed that high FOXN3 expression level was associated with poor recurrence free survival (p<0.05) but has no statistical significance for overall survival(p>0.05)(Figures 4A, B). In the clinical samples, Patients were divided into high expression(n=18) and low expression(n=18) groups using the median SI. Prognostic analysis revealed that patients with high FOXN3 expression had a worse disease-free survival and overall survival compared to those with low FOXN3 expression(p<0.05)(Figures 4C, D).
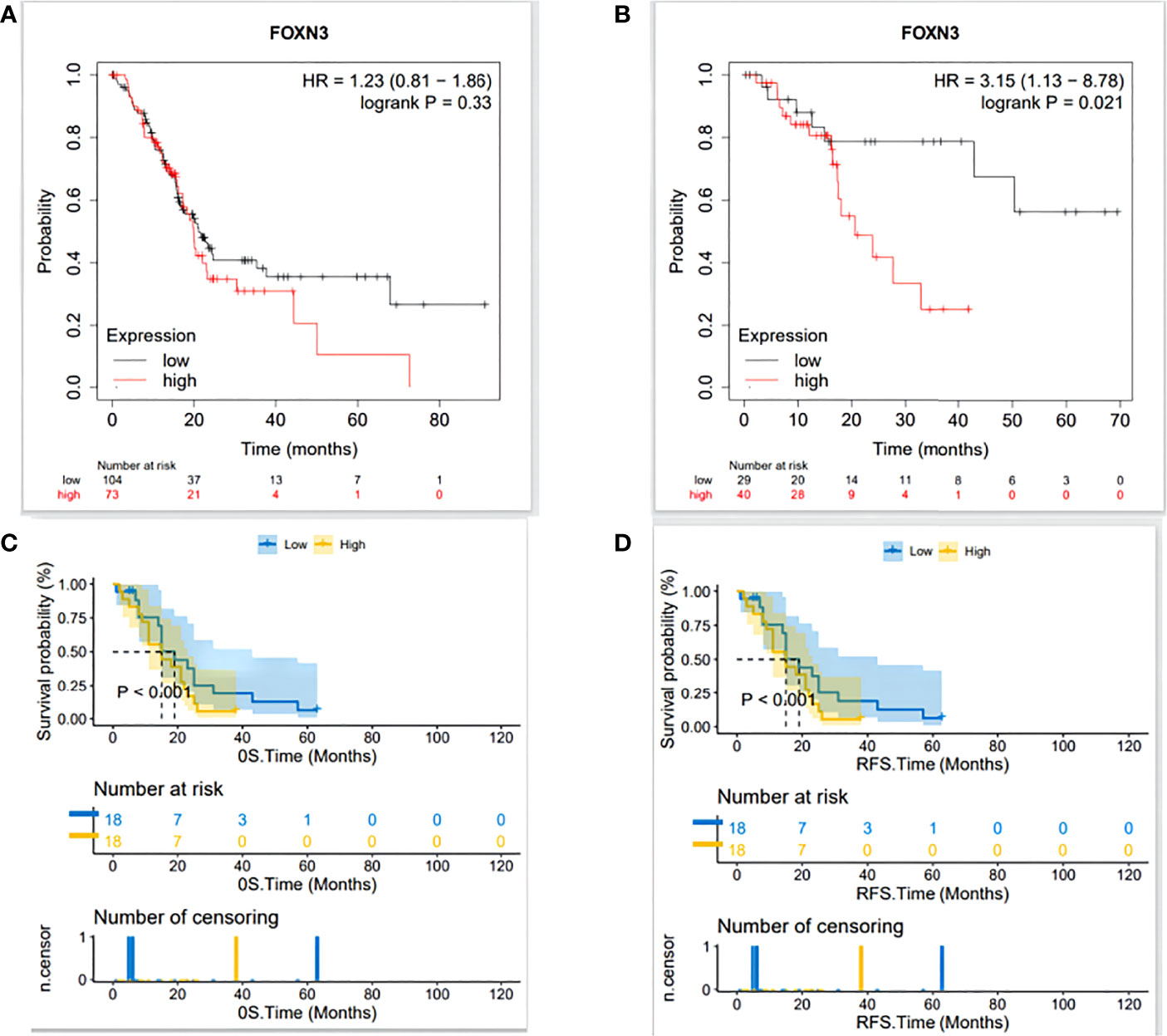
Figure 4 (A) The relationship between FOXN3 expression level and overall survival in TCGA database analyzed by Kaplan-Meier Plotter. (B) The relationship between FOXN3 expression level and disease-free survival in TCGA database by Kaplan-Meier Plotter. (C) The relationship between FOXN3 expression level and overall survival in clinical samples. (D) The relationship between FOXN3 expression level and disease-free survival in clinical samples.
GO, KEGG and GSEA analysis of DEGs
A total of 394 genes (|log FC| > 1.5) from the TCGA were screened using differential expression gene analysis in pancreatic cancer patients. Next, the related heat map and volcano map were constructed accordingly (Figure 5). The DEGs were used for gene set enrichment analysis (GSEA), revealing that they were significantly correlated with the regulation of the immune system process, adaptive immunity, and immune system development (P < 0.05) (Figure 6). Based on the TCGA database, the expression correlation between FOXN3 and all genes in PDAC was analyzed, and the genes with Pearson coefficient > 0.5 were defined as FOXN3-related genes, with a total of 98 identified genes. These FOXN3-related genes were then subjected to GO and KEGG analysis, demonstrating that these genes were significantly associated with BP, especially in the T cell activation, lymphocyte differentiation, mononuclear cell differentiation, activation of immune response, regulation of T cell activation, immune response-activating cell surface receptor signaling pathway, immune response-activating signal transduction, antigen receptor-mediated signaling pathway, immune response-regulating cell surface receptor signaling pathway and T cell differentiation, to name a few. Together, these results imply that FOXN3 is closely related to the occurrence and development of the tumor immune microenvironment in PDAC tissues. Based on KEGG’s analysis, FOXN3 was found to be associated with cell adhesion molecules, T cell receptor signaling pathway, Primary immunodeficiency, Chemokine signaling pathway, etc. (Figure 7). Most of which affect the immune process and are in line with the above conclusion.
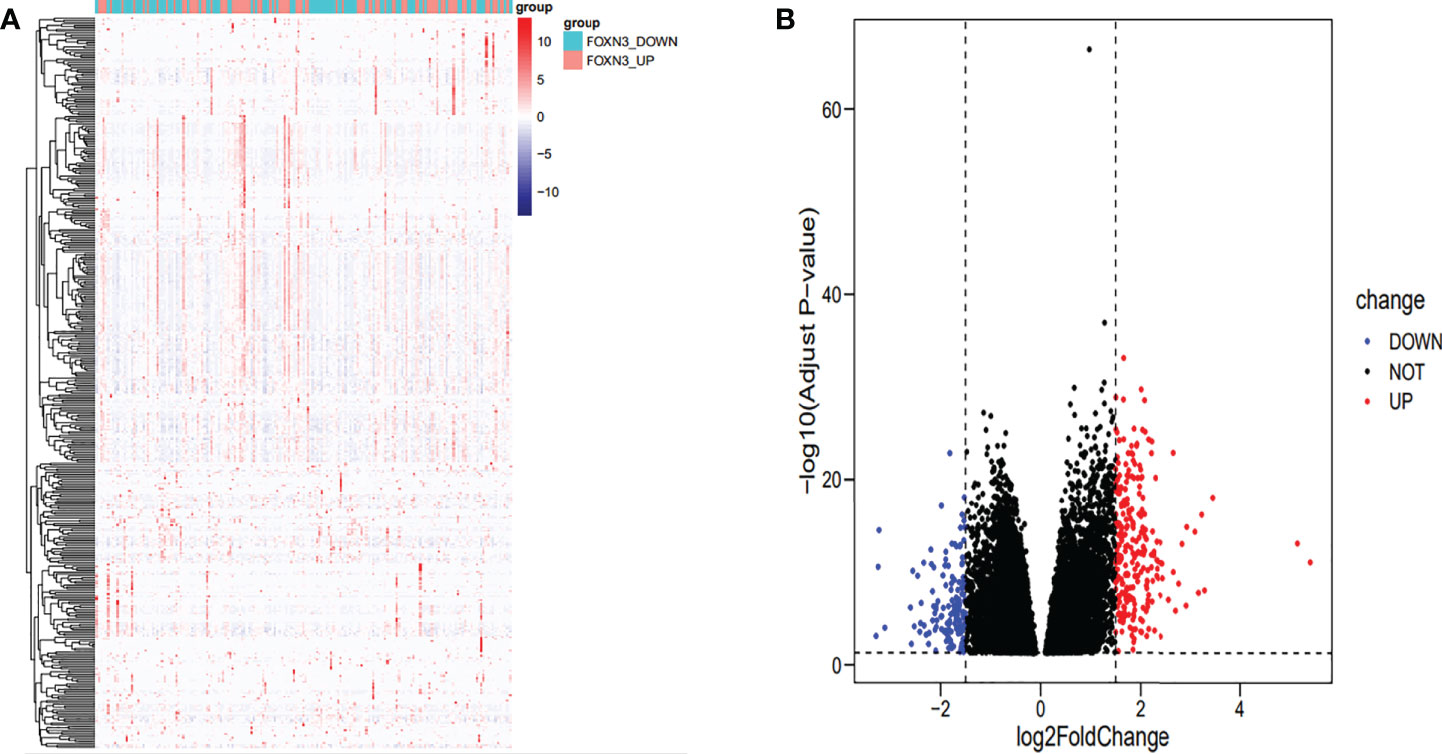
Figure 5 (A) Heat map of DEGs when FOXN3 is down-regulated and up-regulated in PDAC based on TCGA database. (B) Volcanic map of differential gene expression.
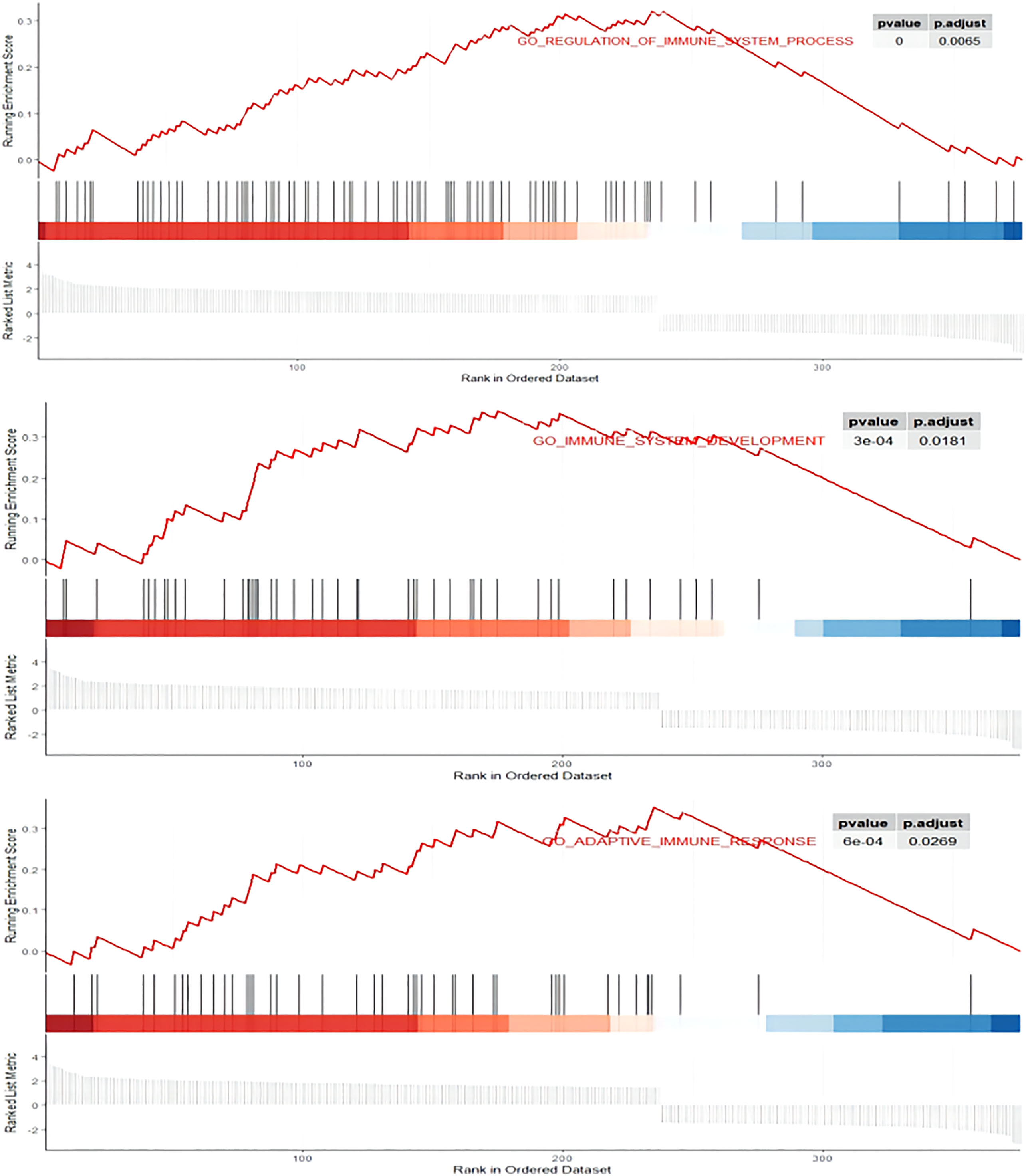
Figure 6 GSEA enrichment plots. DEGs was significantly correlated with the regulation of immune system process, adaptive immunity, and immune system development. P.adjust<0.05.
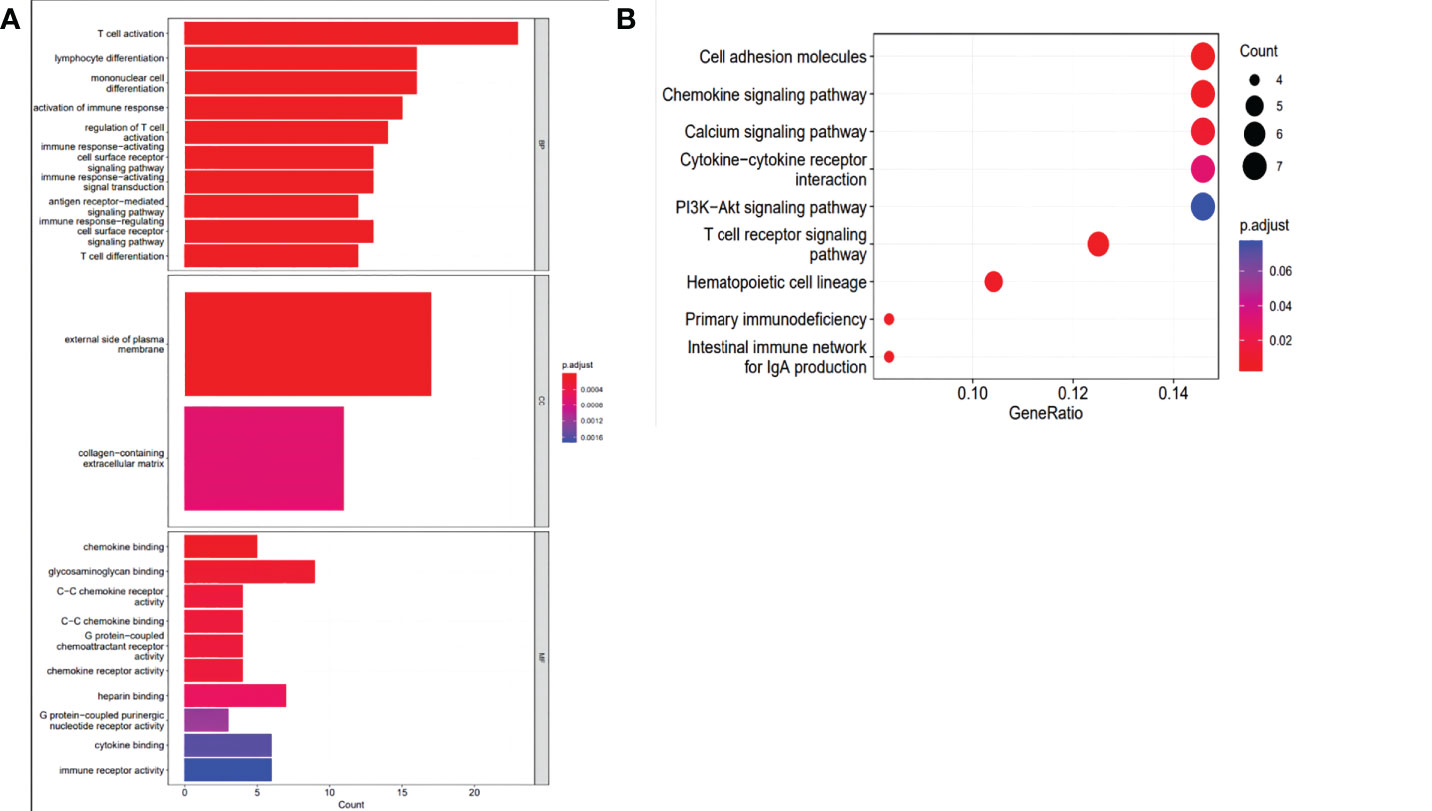
Figure 7 (A) GO analysis of the intersection of DEGs with related genes in PC biological process, cell components, and molecular function enrichment analyses. (B) KEGG pathway analysis of the intersection of DEGs with related genes.
The relationship between FOXN3 gene expression and immune infiltration in PDAC
Tumor-infiltrating immune cells (TIICs) include T cells, macrophages, NK cells, etc., which are important components of the tumor immune microenvironment (TIME). TISIDB was used to analyze the relationship between FOXN3 expression and TIICs in pan-cancer samples (Figure 8A). FOXN3 expression was found to be highly correlated with most TIICs (such as CD8T cells, CD4T cells, etc.) in PDAC. Therefore, FOXN3 may play an important role in the tumor immune microenvironment of PDAC (Figure 8B). To further understand how FOXN3 affects the occurrence and development of the PDAC immune process, Xcell was used to understand the relationship between FOXN3 and TIICs. Xcell is a ssGSEA-based method that calculates the abundance fractions of 64 immune cells. Herein, TCGA samples was classified into high and low groups using the median FOXN3 expression. The figure shows the differential expression of immune cells between the two groups. FOXN3 high expression group had higher immune cell expression (Figure 9). Moreover, using GEPIA2, we analyzed the correlation between main immunotherapy drug targets and FOXN3, including PDCD1, CTLA4 and PDL1, with a p-value less than 0.05 (Figure 10).
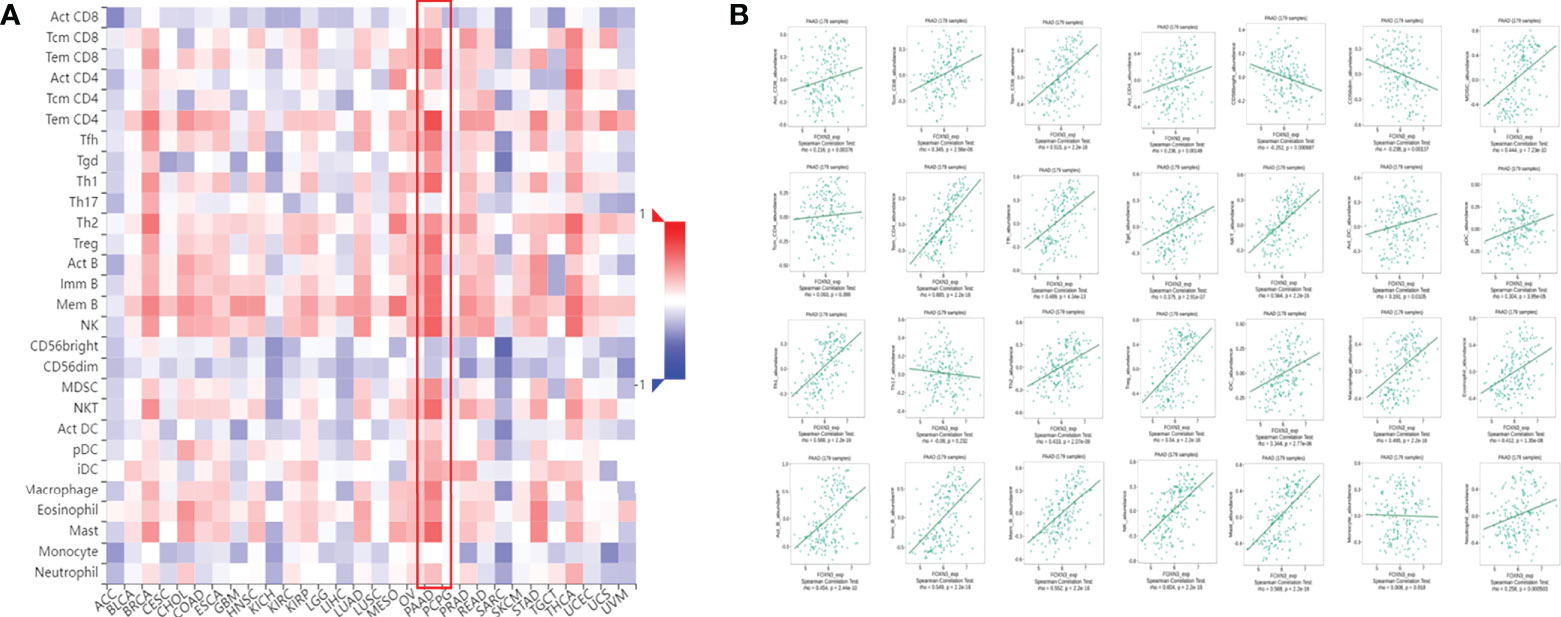
Figure 8 (A) The relationship between FOXN3 gene expression and TIICs in pan-cancer. (B) Relationship between FOXN3 expression and tumor-infiltrating immune cells in PDAC.
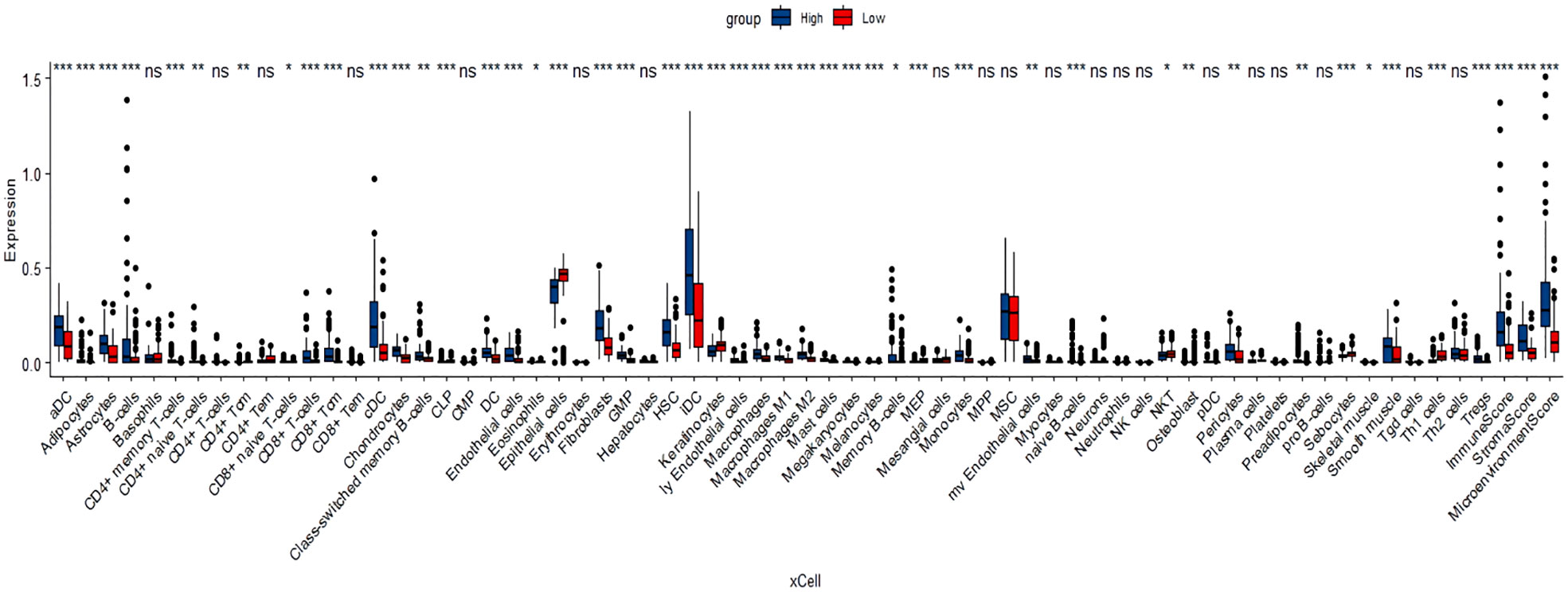
Figure 9 Xcell analyzed the relationship between FOXN3 and immune cell expression * means P < 0.05, ** means P < 0.005, *** means P < 0.005, “ns” means no significance.
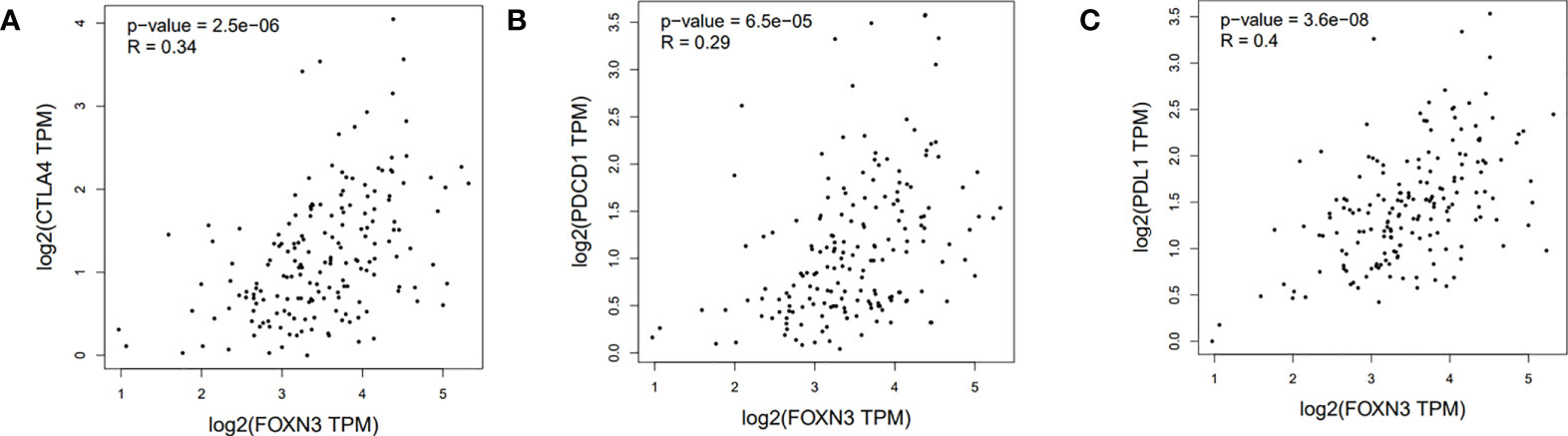
Figure 10 (A) Correlation between FOXN3 and CTLA4 in PDAC patients in TCGA database. (B) Correlation between FOXN3 and PDCD1 in PDAC patients in TCGA database. (C) Correlation between FOXN3 and PDL1 in PDAC patients in TCGA database.
Discussion
Since it is difficult to detect PDAC in the early stage, most PDAC patients are in advanced stages upon diagnosis, and systemic chemotherapy is their only treatment option (20). However, chemotherapy is frequently ineffective due to PDAC’s strong immunosuppressive environment (21). As a result, we urgently need to understand the immune microenvironment of PDAC to devise new treatment strategies.
During pancreatic cancer development, pancreatic stellate cells become activated and undergo fibrosis around the tumor, forming a dense stroma devoid of blood vessels that promotes immune escape by blocking lymphocyte invasion (22, 23). Therefore, pancreatic cancer is commonly infiltrated with immune cells, but the core of the tumor is relatively free of cytotoxic T lymphocytes (24), preventing adaptive immunity from accurately recognizing pathogens or tumor tissue to mount an effective response (25). Analysis of large PDAC genomic datasets showed that only a small number of PDAC were immunologically active (26). In PDAC, immunosuppressive cells, tumor-associated macrophages, myeloid suppressor cells, and Treg cells are initially prominent, forming an immunosuppressive environment (27).
FOXN3 is a member of the FOX protein family, which has been studied in the liver, thyroid, breast, and other cancers. Herein, we mainly focus on its relationship with PDAC. Based on an online database of gene differential expression, survival analysis and a combination of immunohistochemical staining results, we concluded that FOXN3 is highly expressed in PDAC and could potentially be used as a prognostic tool. To further understand the mechanism of FOXN3 in PDAC, GSEA analysis was performed using DEG, and pathway analysis revealed an enrichment in immune-related functions. Subsequently, immune infiltration analysis was performed on TISBD, and FOXN3 was found to be significantly associated with TIICs in PDAC when compared to other cancers. The expression of most TIICs increases with FOXN3 expression, implying that FOXN3 may influence the immune microenvironment in PDAC by regulating TIICs expression. Next, we searched for FOXN3-related genes and looked for intersecting DEGs. Subsequently, the final gene set was used for further GO and KEGG analysis, and the analysis results validated our above conjecture. We discovered that FOXN3’s functional role is closely related to the T cell receptor pathway, and T cells play an important role in the immunosuppressive microenvironment of PDAC.
PDAC is typically infiltrated by T cells with a low proportion of CD8T toxic cells and is often referred to immunologically as a “cold” tumor (21). Treg cells have a role in maintaining immune homeostasis in normal organisms by inhibiting inflammatory responses (28). FOXP3 positive Treg cells were observed to increase in number as PDAC progressed, leading to immune evasion (29). Taken together, an increase in the total T cell count in PDAC could contribute to tumor development and a worse prognosis (30).
Combined with our findings, it can be determined that FOXN3 plays a non-negligible role in the formation of the immunosuppressive microenvironment of PDAC by regulating T cells. In most cancers, T cells, the main force of the anti-tumor army in the immune system, are disabled by the specific immune cold environment of PDAC (31). However, if T cell immunity can be sufficiently induced, it will inhibit tumor development (32). Immune checkpoint blockers, including antibodies against cytotoxic T lymphocyte antigen-4(CTLA4), programmed cell death protein 1(PD-1) and its ligand PD-L1, enhance T cell anti-tumor immune function (33). After analyzing its relationship with FOXN3, we identified a statistically significant correlation, implying that FOXN3 could be a potential target for immunotherapy in PDAC.
Herein, the results of clinical samples and the TCGA and GTEx databases were used to validate each other in this study, but the total sample size was small, which may have resulted in significant bias. Further limitations include the lack of additional in vitro and in vivo evidence. Therefore, we look forward to future research to confirm the function of FOXN3 in PDAC.
Data availability statement
The original contributions presented in the study are included in the article/Supplementary Material. Further inquiries can be directed to the corresponding authors.
Ethics statement
The studies involving human participants were reviewed and approved by the ethics committee of Zhejiang Province People’s Hospital. The patients/participants provided their written informed consent to participate in this study.
Author contributions
JZ, DH and YS designed and conceptualized studies. WY and YD conducted experiments, process experimental data, and conduct bioinformatics analysis. WY, YD, KZ, XL, YZ, YS, WFY, and CZ participated in editing manuscripts and put forward valuable suggestions. All authors contributed to the article and approved the submitted version.
Funding
This work was supported by the Science and Technology Department Public Welfare Project of Zhejiang Province (GF22H168107), Zhejiang Provincial Science Foundation Committee of China (LQ19H160015) and the Medical and Health Science and Technology Project of Zhejiang Province (2022RC107, 2021KY486).
Conflict of interest
The authors declare that the research was conducted in the absence of any commercial or financial relationships that could be construed as a potential conflict of interest.
Publisher’s note
All claims expressed in this article are solely those of the authors and do not necessarily represent those of their affiliated organizations, or those of the publisher, the editors and the reviewers. Any product that may be evaluated in this article, or claim that may be made by its manufacturer, is not guaranteed or endorsed by the publisher.
Supplementary material
The Supplementary Material for this article can be found online at: https://www.frontiersin.org/articles/10.3389/fonc.2022.1008100/full#supplementary-material
Supplementary Figure 1 | Differences in FOXN3 mRNA expression between pancreatic cancer and normal pancreatic tissues.
References
1. Klein AP. Pancreatic cancer epidemiology: understanding the role of lifestyle and inherited risk factors. Nat Rev Gastroenterol Hepatol (2021) 18:493–502. doi: 10.1038/s41575-021-00457-x
2. Siegel RL, Miller KD, Fuchs HE, Jemal A. Cancer statistics, 2021. CA Cancer J Clin (2021) 71:7–33. doi: 10.3322/caac.21654
3. Frappart PO, Hofmann TG. Pancreatic ductal adenocarcinoma (PDAC) organoids: The shining light at the end of the tunnel for drug response prediction and personalized medicine. Cancers (Basel) (2020) 12(10):2750. doi: 10.3390/cancers12102750
4. Zhao Z, Liu W. Pancreatic cancer: A review of risk factors, diagnosis, and treatment. Technol Cancer Res Treat (2020) 19:1533033820962117. doi: 10.1177/1533033820962117
5. Ackson BC, Carpenter C, Nebert DW, Vasiliou V. Update of human and mouse forkhead box (FOX) gene families. Hum Genomics (2010) 4:345–52. doi: 10.1186/1479-7364-4-5-345
6. Gross DN, Wan M, Birnbaum MJ. The role of FOXO in the regulation of metabolism. Curr Diabetes Rep (2009) 9:208–14. doi: 10.1007/s11892-009-0034-5
7. Salih DA, Brunet A. FoxO transcription factors in the maintenance of cellular homeostasis during aging. Curr Opin Cell Biol (2008) 20:126–36. doi: 10.1016/j.ceb.2008.02.005
8. Miyara M, Wing K, Sakaguchi S. Therapeutic approaches to allergy and autoimmunity based on FoxP3+ regulatory T-cell activation and expansion. J Allergy Clin Immunol (2009) 123:749–55. doi: 10.1016/j.jaci.2009.03.001
9. Friedman JR, Kaestner KH. The foxa family of transcription factors in development and metabolism. Cell Mol Life Sci (2006) 63:2317–28. doi: 10.1007/s00018-006-6095-6
10. Pati D, Keller C, Groudine M, Plon SE. Reconstitution of a MEC1-independent checkpoint in yeast by expression of a novel human fork head cDNA. Mol Cell Biol (1997) 17:3037–46. doi: 10.1128/MCB.17.6.3037
11. Kong X, Zhai J, Yan C, Song Y, Wang J, Bai X, et al. Recent advances in understanding FOXN3 in breast cancer, and other malignancies. Front Oncol (2019) 9:234. doi: 10.3389/fonc.2019.00234
12. Schuff M, R?ssner A, Wacker SA, Donow C, Gessert S, Kn?chel W. FoxN3 is required for craniofacial and eye development of xenopus laevis. Dev Dyn (2007) 236:226–39. doi: 10.1002/dvdy.21007
13. Li W, Zhang Z, Liu X, Cheng X, Zhang Y, Han X, et al. The FOXN3-NEAT1-SIN3A repressor complex promotes progression of hormonally responsive breast cancer. J Clin Invest (2017) 127:3421–40. doi: 10.1172/JCI94233
14. Sun J, Li H, Huo Q, Cui M, Ge C, Zhao F, et al. The transcription factor FOXN3 inhibits cell proliferation by downregulating E2F5 expression in hepatocellular carcinoma cells. Oncotarget (2016) 7:43534–45. doi: 10.18632/oncotarget.9780
15. Zhang ZJ, Xiao Q, Li XY. MicroRNA-574-5p directly targets FOXN3 to mediate thyroid cancer progression via wnt/β-catenin signaling pathway. Pathol Res Pract (2020) 216:152939. doi: 10.1016/j.prp.2020.152939
16. Dai Y, Wang M, Wu H, Xiao M, Liu H, Zhang D. Loss of FOXN3 in colon cancer activates beta-catenin/TCF signaling and promotes the growth and migration of cancer cells. Oncotarget (2017) 8:9783–93. doi: 10.18632/oncotarget.14189
17. Tang Z, Li C, Kang B, Gao G, Li C, Zhang Z. GEPIA: a web server for cancer and normal gene expression profiling and interactive analyses. Nucleic Acids Res (2017) 45:W98–98W102. doi: 10.1093/nar/gkx247
18. Lánczky A, á N, Bottai G, Munkácsy G, Szabó A, Santarpia L, et al. miRpower: a web-tool to validate survival-associated miRNAs utilizing expression data from 2178 breast cancer patients. Breast Cancer Res Treat (2016) 160:439–46. doi: 10.1007/s10549-016-4013-7
19. Ru B, Wong CN, Tong Y, Zhong JY, Zhong S, Wu WC, et al. TISIDB: an integrated repository portal for tumor-immune system interactions. Bioinformatics (2019) 35:4200–2. doi: 10.1093/bioinformatics/btz210
20. Mizrahi JD, Surana R, Valle JW, Shroff RT. Pancreatic cancer. Lancet (2020) 395:2008–20. doi: 10.1016/S0140-6736(20)30974-0
21. Bear AS, Vonderheide RH, O’Hara MH. Challenges and opportunities for pancreatic cancer immunotherapy. Cancer Cell (2020) 38:788–802. doi: 10.1016/j.ccell.2020.08.004
22. Apte MV, Park S, Phillips PA, Santucci N, Goldstein D, Kumar RK, et al. Desmoplastic reaction in pancreatic cancer: role of pancreatic stellate cells. Pancreas (2004) 29:179–87. doi: 10.1097/00006676-200410000-00002
23. Johnson BA 3rd, Yarchoan M, Lee V, Laheru DA, Jaffee EM. Strategies for increasing pancreatic tumor immunogenicity. Clin Cancer Res (2017) 23:1656–69. doi: 10.1158/1078-0432.CCR-16-2318
24. Binnewies M, Roberts EW, Kersten K, Chan V, Fearon DF, Merad M, et al. Understanding the tumor immune microenvironment (TIME) for effective therapy. Nat Med (2018) 24:541–50. doi: 10.1038/s41591-018-0014-x
25. Evans RA, Diamond MS, Rech AJ, Chao T, Richardson MW, Lin JH, et al. Lack of immunoediting in murine pancreatic cancer reversed with neoantigen. JCI Insight (2016) 1(14):e88328. doi: 10.1172/jci.insight.88328
26. Bailey P, Chang DK, Nones K, Johns AL, Patch AM, Gingras MC, et al. Genomic analyses identify molecular subtypes of pancreatic cancer. Nature (2016) 531:47–52. doi: 10.1038/nature16965
27. Clark CE, Hingorani SR, Mick R, Combs C, Tuveson DA, Vonderheide RH. Dynamics of the immune reaction to pancreatic cancer from inception to invasion. Cancer Res (2007) 67:9518–27. doi: 10.1158/0008-5472.CAN-07-0175
28. Glasner A, Plitas G. Tumor resident regulatory T cells. Semin Immunol (2021) 52:101476. doi: 10.1016/j.smim.2021.101476
29. Wang X, Li X, Wei X, Jiang H, Lan C, Yang S, et al. PD-L1 is a direct target of cancer-FOXP3 in pancreatic ductal adenocarcinoma (PDAC), and combined immunotherapy with antibodies against PD-L1 and CCL5 is effective in the treatment of PDAC. Signal Transduct Target Ther (2020) 5:38. doi: 10.1038/s41392-020-0144-8
30. Ajina R, Weiner LM. T-Cell immunity in pancreatic cancer. Pancreas (2020) 49:1014–23. doi: 10.1097/MPA.0000000000001621
31. Stromnes IM, Hulbert A, Pierce RH, Greenberg PD, Hingorani SR. T-Cell localization, activation, and clonal expansion in human pancreatic ductal adenocarcinoma. Cancer Immunol Res (2017) 5:978–91. doi: 10.1158/2326-6066.CIR-16-0322
32. Soares KC, Rucki AA, Wu AA, Olino K, Xiao Q, Chai Y, et al. PD-1/PD-L1 blockade together with vaccine therapy facilitates effector T-cell infiltration into pancreatic tumors. J Immunother (2015) 38:1–11. doi: 10.1097/CJI.0000000000000062
Keywords: FOXN3, pancreatic adenocarcinoma, TCGA, prognosis, immune infiltration
Citation: Yu W, Diao Y, Zhang Y, Shi Y, Lv X, Zhang C, Zhang K, Yao W, Huang D and Zhang J (2022) Bioinformatic analysis of FOXN3 expression and prognostic value in pancreatic cancer. Front. Oncol. 12:1008100. doi: 10.3389/fonc.2022.1008100
Received: 31 July 2022; Accepted: 28 September 2022;
Published: 17 October 2022.
Edited by:
Ajay Singh, University of South Alabama, United StatesReviewed by:
Shan Lei, Guizhou Medical University, ChinaBo Wang, Peking University People’s Hospital, China
Copyright © 2022 Yu, Diao, Zhang, Shi, Lv, Zhang, Zhang, Yao, Huang and Zhang. This is an open-access article distributed under the terms of the Creative Commons Attribution License (CC BY). The use, distribution or reproduction in other forums is permitted, provided the original author(s) and the copyright owner(s) are credited and that the original publication in this journal is cited, in accordance with accepted academic practice. No use, distribution or reproduction is permitted which does not comply with these terms.
*Correspondence: Jungang Zhang, zjg2sy1@163.com; Dongsheng Huang, huangdszj@163.com
†These authors have contributed equally to this work