- Department of Pathology, University of Hong Kong, Hong Kong, Hong Kong SAR, China
Acute lymphoblastic leukaemia (ALL) is an aggressive haematolymphoid malignancy. The prognosis of ALL is excellent in paediatric population, however the outcome of relapse/refractory disease is dismal. Adult ALL has less favourable prognosis and relapse/refractory disease is not uncommonly encountered. Bortezomib is the first generation proteasome inhibitor licensed to treat plasma cell myeloma and mantle cell lymphoma with favourable side effect profile. Efficacy of bortezomib had been proven in other solid tumors. Clinical studies showed promising response for proteasome inhibitors in treating relapse/refractory ALL. Thus, proteasome inhibitors are attractive alternative agents for research in treating ALL. In the review article, we will introduce different proteasome inhibitors and their difference in pharmacological properties. Moreover, the mechanism of action of proteasome inhibitors on ALL will be highlighted. Finally, results of various clinical studies on proteasome inhibitors in both paediatric and adult ALL will be discussed. This review article provides the insights on the use of proteasome inhibitors in treating ALL with a summary of mechanism of action in ALL which facilitates future research on its use to improve the outcome of ALL.
Introduction
Acute lymphoblastic leukaemia (ALL) is an aggressive haematological malignancy. The prognosis of paediatric ALL is excellent with 90% of long-term survivor (1). However, a small proportion of them still die from relapse/refractory disease. The prognosis of the adult population is poor, with only 50-60% of long-term survivor (2). The prognosis of relapse/refractory disease is dismal.
Bortezomib is approved by Food and Drug Administration (FDA) for treatment of plasma cell myeloma and mantle cell lymphoma with reasonable side effect profile (3). Since then, bortezomib is an attractive novel agent for research in the treatment of other cancers, e.g., glioblastoma multiforme and colorectal cancer (4, 5). Bortezomib is also being researched for novel treatment of ALL with significant major discoveries made. Newer proteasome inhibitors are developed with improved efficacy and side effect profile. In this review article, it summarized the differences among various proteasome inhibitors and their mechanisms of action in ALL. Recent clinical studies to evaluate the role of proteasome inhibitors in ALL were highlighted. Finally, the future prospect of research about those agents in treating ALL will be proposed.
Differences in Pharmacological Properties of Various Proteasome Inhibitors
Bortezomib
Bortezomib is a first generation 26S proteasome reversible inhibitor which binds to β5 subunit of chymotryptic site of 20S subunit proteasome (6). It also binds to β1 and β2 subunits at lower affinity. Bortezomib can either be administered via intravenous or subcutaneous route with comparable amount of systemic concentration of drug and inhibitory action of proteasome. Bortezomib has haematological toxicities including thrombocytopenia and neutropenia which are not dose-limiting (7). Other common side effects are gastrointestinal side effects including diarrhoea, nausea, and vomiting which occur in 84% of patients. The most disabling adverse effect is peripheral neuropathy which is dose limiting and route-dependent, whereas subcutaneous administration has reduced incidence of this adverse effect (6).
Carfilzomib
Carfilzomib is a second generation proteasome inhibitor which belongs to epoxyketone group. This drug has irreversible binding towards β5 subunit with higher β5 to β2 selectivity compared with bortezomib. The binding of carfilzomib to both β5 and β2 subunit makes it effective in treating bortezomib-resistant plasma cell myeloma (6, 8). This drug can only be administrated via intravenous route (6).
Carfilzomib associates with less risk of peripheral neuropathy compared with bortezomib. However, carfilzomib has a higher incidence of serious cardiotoxicity (9). It could be due to reduced number of proteasome per unit of protein in cardiac muscle (6, 8). Moreover, the off-target effect of inhibiting autophagy due to activation of protein phosphatase 2A also contributes to carfilzomib-induced cardiotoxicity (10).
Ixazomib
Ixazomib is a boronate-based third generation proteasome inhibitor licensed to treat plasma cell myeloma and it can be administrated via oral route. It is a prodrug which will be hydrolyzed to form active metabolite and the active metabolite is reversibly bind to β5 subunit and to lesser extent to β1 and β2 subunits of proteasome. However, the time of dissociation from β5 subunit is shorter for ixazomib when compared with bortezomib (6, 11). Because the metabolite is similar to that of bortezomib, the incidence of grade 3 or more haematological and gastrointestinal side effects is comparable with bortezomib (6). However, the risk of peripheral neuropathy is lower than that of bortezomib (6, 12, 13).
Newer Classes of Proteasome Inhibitors
Some newer classes of proteasome inhibitors are developing, namely oprozomib, delanzomib and marizomib with more favorable side effects profile.
Delanzomib shows similar potency of proteasome inhibition with both β5 and β1 subunit binding, in contrast to bortezomib binds only β5 subunit (14). Delanzomib shows higher affinity of binding with 20 times slower rate of dissociation compared with bortezomib (15). A phase I/II study of delanzomib showed that grade 3 or above hematological side effects were common: anemia (15%), neutropenia (23%) and thrombocytopenia (54%) at a dose of 2.1 mg/m2. Other non-hematological side effects included rash, nausea and diarrhea. A small proportion of patients had grade 1 or 2 peripheral neuropathy (12%). No patients had grade 3 or above peripheral neuropathy (16).
Oprozomib is an epoxyketone similar to that of carfilzomib and it shows irreversible binding of β5 subunit. However, the affinity of bindings is higher than that of ixazomib (15). The most common grade 3 or above adverse events were nausea, vomiting, diarrhea and thrombocytopenia. Only rare occurrence of grade 2 or above peripheral neuropathy (17, 18).
Marizomib can bind β1, β2 and β5 subunits of 20S proteasome irreversibly with long duration (6, 19). Safety data from phase I clinical trials showed that hematological toxicities were milder than bortezomib and carfilzomib. The common non-hematological adverse events were nausea, diarrhea, fatigue. Occurrence of central nervous system toxicities including reversible hallucination and cognitive deterioration were seen. Cardiac events were much less common than bortezomib and carfilzomib. No patients had grade 3 or above peripheral neuropathy (20, 21).
Mechanism of Action and Pre-Clinical Studies in ALL
The mechanism of action of proteasome inhibitors on ALL are summarized in Figure 1.
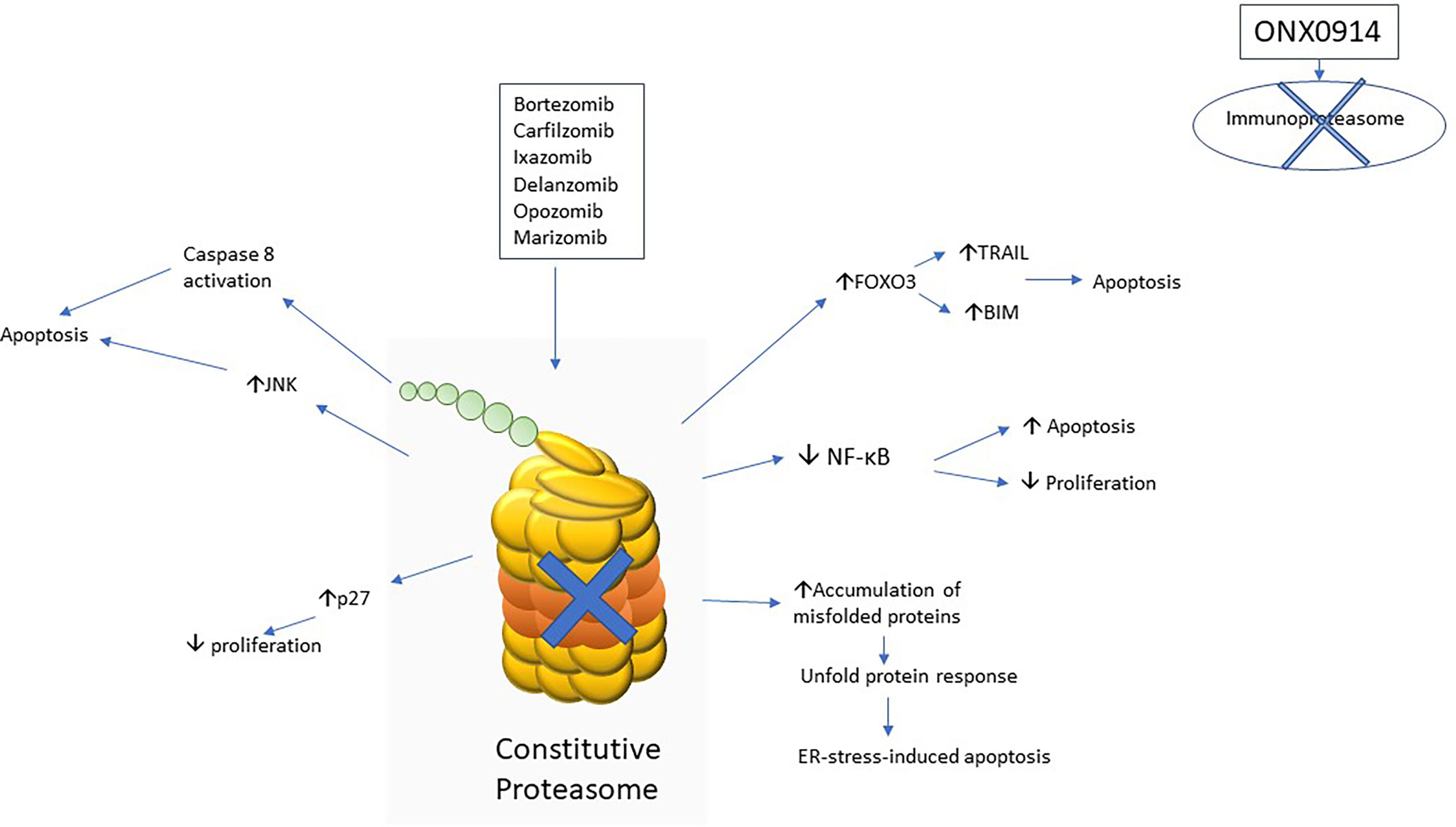
Figure 1 Schematic diagram to show various mechanisms of action of proteasome inhibitors implicated in acute lymphoblastic leukaemia.
NF-κB Inhibition
Study showed that bortezomib was able to stabilize IκB by preventing the degradation of IκB-α and thus inhibit NF-κB pathway in plasma cell myeloma (22). Inhibition of NF-κB pathway was found to be one of the mechanisms of therapeutic effect in ALL from a phase 1 clinical study in 2007 (23). Another earlier study in 2011 showed that bortezomib was active against relapse T lymphoblastic leukemia (T-ALL) by inhibiting the NF-κB activity in primary leukemic cells of patients (24).
NOTCH1 activating mutation accounts for 60% of T-ALL and it drives leukemogenesis by activating NF-κB pathway (25, 26). Therefore, it is reasonable to postulate that bortezomib is effective in NOTCH1-mutated T-ALL. Study showed that bortezomib enhanced degradation of transcription factor Sp-1 and thus reduced NOTCH1 transcription (27). Pre-clinical study showed that bortezomib was more effective in T-ALL than in B lymphoblastic leukaemia (B-ALL) which probably due to the action of NF-κB pathway inhibition by bortezomib (28). However, the NOTCH1 mutation status was unknown in that study.
It is uncertain that whether the treatment of proteasome inhibitors is also effective in NOTCH1 wild-type T-ALL, especially early T-cell precursor acute lymphoblastic leukemia (ETP-ALL) which confers a poor prognosis. More studies are needed to address this issue.
Targeting Immunoproteasome
Immunoproteasome is a special type of proteasome that is expressed in hematopoietic cells. In contrast to the usual proteasome, or namely constitutive proteasome, immunoproteasome processes specific subunits (β1i, β2i, β5i) in the active site. This results in an increased specificity to produce peptides with hydrophobic amino acid ends, which was hypothesized to facilitate antigen presentation (29).
In a recent study on proteasome subunit expression in leukemic cells from relapsed patients, it was found that immunoproteasome was more abundantly expressed in ALL than that in acute myeloid leukemia (AML). Thus, it accounted for increased sensitivity towards multiple proteasome inhibitors, including bortezomib, carfilzomib and ONX0914 (an irreversible β5i selective inhibitor) in ALL when compared with AML (30). Targeting immunoproteasome can achieve selective toxicities towards ALL and prevent those side effects resulting from constitutive proteasome inhibition of non-lymphoid tissue, e.g., cardiac toxicities. Pre-clinical study showed that B-ALL with KMT2A-AF4 fusion and T-ALL were highly sensitive to the treatment of ONX0914 (31).
Restoration of Forkhead Box O3 (FOXO3)
Forkhead transcription factor family (FOXO) comprises different sub-classes namely forkhead box O1 (FOXO1), forkhead box O3a (FOXO3a) and forkhead box O4 (FOXO4). This transcription factor family, FOXO is responsible for regulating gene transcription related to apoptosis and cell cycle control. The activation of Akt phosphorylates FOXO3a and leads to translocation form nucleus to cytoplasm, preventing its transcription function. Philadelphia-positive acute lymphoblastic leukemia (Ph-positive ALL) is characterized by the presence of BCR-ABL1 fusion protein resulting from translocation of chromosome 9 and 22 and PI3K-Akt pathway is constitutively activated in this subtype of B-ALL (32). Jagani, Z. et al. showed a reduced level of FOXO3a expression in a BCR-ABL1 transgenic mice model in 2009. The treatment of bortezomib restored the level of FOXO3a by preventing proteasome-mediated degradation and prolonged survival in BCR-ABL1 transgenic mice. The level of FOXO3a target proteins, namely TRAIL and BIM were increased upon bortezomib treatment resulting into apoptosis (33). These findings were also verified in patients with Ph-positive ALL. A study in 2011 showed that treatment with bortezomib induced complete hematological response with disappearance of BCR-ABL1 transcript and restoration of FOXO3a (34).
Philadelphia-like acute lymphoblastic leukemia (Ph-like ALL) is a provisional entity in World Health Organization (WHO) 2016 classification with genetic signature significantly overlapping with Ph-positive ALL and the prognosis is poor. Various kinases signaling pathway are hyperactivated, including PI3K-Akt pathway (35–37). It implies that FOXO3a will also be inactivated as a result and thus the treatment with proteasome inhibitors is potentially effective. However, no preclinical and clinical studies to verify this hypothesis.
C-Jun Pathway
C-Jun N-terminal kinase (JNK) has three isoforms (JNK1, JNK2 and JNK3) and it belongs to mitogen activated protein kinase (MAPK). JNK is activated by MAPK kinase and JNK phosphorylates c-Jun. Activation of c-Jun will in turn activate pro-apoptotic proteins, e.g., BAD and trigger subsequent apoptosis (38). Study showed that bortezomib activates c-Jun pathway resulting into apoptosis in plasma cell myeloma (39). The c-Jun activation by bortezomib was also demonstrated in B-ALL (40). However, no studies to evaluate the effect of proteasome inhibitors on c-Jun pathway in T-ALL so far.
Modulation of Proteostasis
Unfold protein response is triggered when there are stimuli to interfere protein folding in endoplasmic reticulum (ER) (41). If those misfolded proteins cannot be properly refolded again, they will be translocated out of ER and subjected to proteasome-mediated degradation via 26S subunit (42). Proteasome inhibition results into unfold protein response due to accumulation of misfolded proteins which triggered subsequent ER-stress-induced apoptosis (43). However, cells may restore proteostasis by upregulating molecular chaperones upon stimulation of unfold protein response triggered by ER stress. Therefore, maximal anti-leukemic effects can be achieved by inhibiting unfold protein response together with proteasome inhibition (44). A recent study utilized a casein kinase 2 (CK2) inhibitor, CX-4945 which inhibited the interaction between BIP (a sensor chaperone of ER stress) and Hsp90 (a molecular chaperone that stabilizes BIP). It resulted into impairment of BIP activities and thus inhibited the buffering function of unfold protein response against the ER stress induced by bortezomib pre-treatment. Similar effect was achieved by using a Hsp90 inhibitor, tanespimycin. The study also demonstrated synergistic effects of the above combination in various ALL cell lines (45).
When proteasome is inhibited, other pathways for protein haemostasis are activated to maintain cellular function which results into resistance to treatment with proteasome inhibitors. One such pathway is the activation of various molecular chaperones and deposition of misfolded protein aggregates in various deposition sites (46). Concurrent inhibition of molecular chaperones with the use of proteasome inhibitors will overcome the resistance and produce synergistic effect. Further studies on the modulation of proteostatic network by proteasome inhibitors and other chaperone inhibitors are needed in ALL.
Other Mechanism
Study showed that bortezomib was particularly active against KMT2A-rearranged ALL and increased the level of both KMT2A and KMT2A-fusion proteins. Bortezomib was able to trigger apoptosis via caspase 8 activation and lead to BID activation, with subsequent activation of BAX and BAD. Moreover, bortezomib was able to induce G2/M cell cycle arrest via induction of p27 transcription through promoting transcription activity of CDKN1B by accumulation of KMT2A-fusion proteins upon bortezomib treatment (47). Similar therapeutic effect towards KMT2A-rearranged ALL was observed upon the treatment of carfilzomib. However, the underlying mechanism did not explore in that study (48).
Resistance Mechanism to Proteasome Inhibitors
Multiple mechanisms which lead to resistance to proteasome inhibitors in hematological malignancies have been described (49). As β5 subunit of the proteasome is the target of most proteasome inhibitors, point mutations in the binding pocket of proteasome inhibitors are one of the mechanisms (50–52). In addition, upregulation of the β5 subunit by gene amplification leading to increased chymotrypsin activities and thus upregulation of NF-κB activities has also been suggested as a possible resistance mechanism in T-ALL cell lines (53).
Studies on reverting bortezomib resistance have been conducted. Interferon-γ (IFN-γ) is known to induce immunoproteasome expression (29). Immunoproteasomes was found to be reduced in bortezomib-resistant T-ALL cell lines. In an in vitro study using T-ALL cell lines, IFN-γ treated cells were able to demonstrate restored sensitivity towards proteasome inhibitors, including bortezomib, carfilzomib and ONX0914 (54). Therefore, combination of IFN-γ and bortezomib could sensitize bortezomib-resistance leukemic cells towards bortezomib.
Clinical Studies of Various Proteasome Inhibitors
A phase 1 study in 2004 recruited 3 relapse/refractory ALL patients and treated with single agent bortezomib. The toxicities were tolerable. Those patients either showed blood count improvement or reduction in blast count, but no complete remission (CR) documented (55). Another phase 1 study in 2007 also demonstrated acceptable toxicities in pediatric patients with refractory ALL, though no clinical response observed (23) (Table 1).
Single treatment of bortezomib had limited efficacy. Therefore, various clinical studies utilizing combined treatment strategy had been conducted. Study by Messinger et al. in 2010 recruited 10 pediatric patients with relapse/refractory ALL and treated with combination of bortezomib, dexamethasone and chemotherapy. The response rate was 67% and majority of the adverse events were grade 3-4 hematological toxicities. Only 2 patients had grade 1-2 peripheral neuropathy (56). Recent study by Iguchi et al. recruited 6 pediatric patients with relapse/refractory B-ALL and treated them with bortezomib plus induction chemotherapy. The overall response rate was 80%. However, 67% of them suffered from serious infective complications due to prolonged neutropenia. Five of them had grade 1-2 peripheral neuropathy and combination with vincristine could account for high rate of peripheral neuropathy (57). D. Hasegawa et al. recruited 3 Japanese pediatric relapse/refractory ALL patients and treated them with combined chemotherapy and bortezomib. All patients achieved CR and one patient achieved minimal residual disease (MRD) negativity after treatment. No fatal infective episodes occurred (58). Recent small-scale clinical studies also demonstrated excellent response to relapse/refractory ALL in pediatrics and young adults with CR rate around 60-80% and a substantial proportion of patients achieved MRD negativity. The side effects were acceptable (59–61).
Given the promising results of phase 1 studies, more large-scale clinical studies were conducted. Study by Messinger et al. in 2012 recruited 22 patients with relapse/refractory ALL (1-22 years old). The overall response rate was 73% with few patients developed peripheral neuropathy (62). Recent clinical studies also demonstrated excellent therapeutic efficacy and safety profile for bortezomib-based treatment. A. Bertaina et al. recruited 37 patients with relapse/refractory ALL aged 2–21-year-old. The overall response rate was 73% and 38% of them were MRD negative. Three patients died from infective complications and 5 patients had peripheral neuropathy (63). Horton et al. recruited 135 pediatrics and young adult patients (1-31 years old) with relapse/refractory ALL. They were treated with combined bortezomib and reinduction chemotherapy. The CR rate was 68% and 64% of patients were MRD negative after 3 cycles of treatment. The outcome of relapse/refractory T-ALL was excellent with CR rate of 68% (64). Combined bortezomib with reinduction chemotherapy also demonstrated improved event-free survival (EFS) and overall survival (OS) compared with supportive treatment in pediatric relapse/refractory ALL (65). Kaspers et al. showed 60% overall response rate in pediatric patients with relapse/refractory disease (66). Nachmias et al. showed 78% overall response rate in adult patients with relapse/refractory ALL with minimal toxicities when treated with combined chemotherapy and bortezomib (67). Jain et al. showed that combined pediatric-inspired regimen with rituximab and bortezomib was highly effective in treating newly diagnosed CD20-positive B-ALL in adolescent and adult patients. They showed that 71% of them was MRD negative after induction versus 52% when treated with chemotherapy alone (68).
Carfilzomib also showed to be active against B-ALL and T-ALL in pre-clinical studies (30, 47, 48, 71, 72). Pre-clinical study showed that ixazomib was active against ALL despite it was less potent than bortezomib (73). A phase 1 trial of carfilzomib conducted on adult patients showed acceptable side effects with only 11% of them had congestive heart failure and all of them had pre-existing heart disease (69). A recent phase 1 study recruited 10 adult patients with newly diagnosed ALL and they were treated with combined hyper-CVAD and carfilzomib. They achieved 80% of MRD negativity rate, compared with 53% when treated with hyper-CVAD alone. None of them had cardiac events (70).
The efficacy and safely of combined bortezomib and chemotherapy in treating relapse/refractory ALL had been proven in pediatric population. However, more studies are needed to evaluate this strategy in treating relapse/refractory disease in adult. The findings of high MRD negativity rate when combining proteasome inhibitors with chemotherapy in treating newly diagnosed ALL in adult is a promising finding, since MRD is crucial determinant of prognosis in adult ALL (74–76). This therapeutic strategy for newly diagnosed ALL can potentially improve outcome of ALL, particularly in adult patients.
Concluding Landmark and Future Direction
In conclusion, the safety and efficacy of bortezomib in treating paediatric relapse/refractory ALL had been demonstrated in clinical studies. Data from clinical studies of carfilzomib and ixazomib are emerging. Various mechanisms of action of proteasome inhibitors were implicated in ALL. The expression of immunoproteasome is increased in ALL and thus selective inhibition of immunoproteasome is a promising approach in achieving maximal therapeutic efficacy and minimizing undesirable side effects.
However, large-scale clinical trials of proteasome inhibitors in treating relapse/refractory adult ALL are lacking. Moreover, the use of proteasome inhibitors-based regimen in treating newly diagnosed ALL is under-investigated. Further clinical studies are needed to establish the role of proteasome inhibitors in treating newly diagnosed ALL and relapse/refractory ALL in adult. Moreover, clinical trials of newer generation of proteasome inhibitors are also needed.
Despites its therapeutic efficacy, the mechanism of action of proteasome inhibitors are not well understood in certain subtypes of ALL, particularly NOTCH1 wild-type T-ALL including ETP-ALL and Ph-like ALL which confer a poor prognosis (35, 77, 78). Further studies are needed to elucidate the underlying mechanism of action of proteasome inhibitors in those subtypes of ALL. Optimal combination therapeutic strategy can be developed after throughout understanding the mechanism of action. It can enhance the therapeutic efficacy of proteasome inhibitors and thus improve the prognosis of patients with ALL. Lastly, the mechanism of action of newer proteasome inhibitors is under-investigated and more mechanistic studies are needed.
Author Contributions
CS planned and conceptualized the article. Both CS and MM wrote the article. The article had been reviewed by both CS and MM before submission.
Conflict of Interest
The authors declare that the research was conducted in the absence of any commercial or financial relationships that could be construed as a potential conflict of interest.
Publisher’s Note
All claims expressed in this article are solely those of the authors and do not necessarily represent those of their affiliated organizations, or those of the publisher, the editors and the reviewers. Any product that may be evaluated in this article, or claim that may be made by its manufacturer, is not guaranteed or endorsed by the publisher.
References
1. Pui CH, Evans WE. Treatment of Acute Lymphoblastic Leukemia. N Engl J Med (2006) 354(2):166–78. doi: 10.1056/NEJMra052603
2. Rafei H, Kantarjian HM, Jabbour EJ. Recent Advances in the Treatment of Acute Lymphoblastic Leukemia. Leuk Lymphoma (2019) 60(11):2606–21. doi: 10.1080/10428194.2019.1605071
3. Raedler L. Velcade (Bortezomib) Receives 2 New FDA Indications For Retreatment of Patients With Multiple Myeloma and for First-Line Treatment of Patients With Mantle-Cell Lymphoma. Am Health Drug Benefits (2015) 8:135–40.
4. Yin D, Zhou H, Kumagai T, Liu G, Ong JM, Black KL, et al. Proteasome Inhibitor PS-341 Causes Cell Growth Arrest and Apoptosis in Human Glioblastoma Multiforme (GBM). Oncogene (2005) 24(3):344–54. doi: 10.1038/sj.onc.1208225
5. Hong YS, Hong SW, Kim SM, Jin DH, Shin JS, Yoon DH, et al. Bortezomib Induces G2-M Arrest in Human Colon Cancer Cells Through ROS-Inducible Phosphorylation of ATM-Chk1. Int J Oncol (2012) 41(1):76–82. doi: 10.3892/ijo.2012.1448
6. Fogli S, Galimberti S, Gori V, Del Re M, Danesi R. Pharmacology Differences Among Proteasome Inhibitors: Implications for Their Use in Clinical Practice. Pharmacol Res (2021) 167:105537. doi: 10.1016/j.phrs.2021.105537
7. Tan CRC, Abdul-Majeed S, Cael B, Barta SK. Clinical Pharmacokinetics and Pharmacodynamics of Bortezomib. Clin Pharmacokinet (2019) 58(2):157–68. doi: 10.1007/s40262-018-0679-9
8. Besse A, Besse L, Kraus M, Mendez-Lopez M, Bader J, Xin BT, et al. Proteasome Inhibition in Multiple Myeloma: Head-To-Head Comparison of Currently Available Proteasome Inhibitors. Cell Chem Biol (2019) 26(3):340–51 e3. doi: 10.1016/j.chembiol.2018.11.007
9. Waxman AJ, Clasen S, Hwang WT, Garfall A, Vogl DT, Carver J, et al. Carfilzomib-Associated Cardiovascular Adverse Events: A Systematic Review and Meta-Analysis. JAMA Oncol (2018) 4(3):e174519. doi: 10.1001/jamaoncol.2017.4519
10. Efentakis P, Kremastiotis G, Varela A, Nikolaou PE, Papanagnou ED, Davos CH, et al. Molecular Mechanisms of Carfilzomib-Induced Cardiotoxicity in Mice and the Emerging Cardioprotective Role of Metformin. Blood (2019) 133(7):710–23. doi: 10.1182/blood-2018-06-858415
11. Kupperman E, Lee EC, Cao Y, Bannerman B, Fitzgerald M, Berger A, et al. Evaluation of the Proteasome Inhibitor MLN9708 in Preclinical Models of Human Cancer. Cancer Res (2010) 70(5):1970–80. doi: 10.1158/0008-5472.CAN-09-2766
12. Li J, Bao L, Xia Z, Wang S, Zhou X, Ding K, et al. Ixazomib-Based Frontline Therapy in Patients With Newly Diagnosed Multiple Myeloma in Real-Life Practice Showed Comparable Efficacy and Safety Profile With Those Reported in Clinical Trial: A Multi-Center Study. Ann Hematol (2020) 99(11):2589–98. doi: 10.1007/s00277-020-04234-9
13. Richardson PG, Sonneveld P, Schuster MW, Irwin D, Stadtmauer EA, Facon T, et al. Safety and Efficacy of Bortezomib in High-Risk and Elderly Patients With Relapsed Multiple Myeloma. Br J Haematol (2007) 137(5):429–35. doi: 10.1111/j.1365-2141.2007.06585.x
14. Berkers CR, Leestemaker Y, Schuurman KG, Ruggeri B, Jones-Bolin S, Williams M, et al. Probing the Specificity and Activity Profiles of the Proteasome Inhibitors Bortezomib and Delanzomib. Mol Pharm (2012) 9(5):1126–35. doi: 10.1021/mp2004143
15. Hasinoff BB. Progress Curve Analysis of the Kinetics of Slow-Binding Anticancer Drug Inhibitors of the 20S Proteasome. Arch Biochem Biophys (2018) 639:52–8. doi: 10.1016/j.abb.2017.12.020
16. Vogl DT, Martin TG, Vij R, Hari P, Mikhael JR, Siegel D, et al. Phase I/II Study of the Novel Proteasome Inhibitor Delanzomib (CEP-18770) for Relapsed and Refractory Multiple Myeloma. Leuk Lymphoma (2017) 58(8):1872–9. doi: 10.1080/10428194.2016.1263842
17. Ghobrial IM, Vij R, Siegel D, Badros A, Kaufman J, Raje N, et al. A Phase Ib/II Study of Oprozomib in Patients With Advanced Multiple Myeloma and Waldenstrom Macroglobulinemia. Clin Cancer Res (2019) 25(16):4907–16. doi: 10.1158/1078-0432.CCR-18-3728
18. Hari P, Paba-Prada CE, Voorhees PM, Frye J, Chang YL, Moreau P, et al. Efficacy and Safety Results From a Phase 1b/2, Multicenter, Open-Label Study of Oprozomib and Dexamethasone in Patients With Relapsed and/or Refractory Multiple Myeloma. Leuk Res (2019) 83:106172. doi: 10.1016/j.leukres.2019.106172
19. Levin N, Spencer A, Harrison SJ, Chauhan D, Burrows FJ, Anderson KC, et al. Marizomib Irreversibly Inhibits Proteasome to Overcome Compensatory Hyperactivation in Multiple Myeloma and Solid Tumour Patients. Br J Haematol (2016) 174(5):711–20. doi: 10.1111/bjh.14113
20. Spencer A, Harrison S, Zonder J, Badros A, Laubach J, Bergin K, et al. A Phase 1 Clinical Trial Evaluating Marizomib, Pomalidomide and Low-Dose Dexamethasone in Relapsed and Refractory Multiple Myeloma (NPI-0052-107): Final Study Results. Br J Haematol (2018) 180(1):41–51. doi: 10.1111/bjh.14987
21. Richardson PG, Zimmerman TM, Hofmeister CC, Talpaz M, Chanan-Khan AA, Kaufman JL, et al. Phase 1 Study of Marizomib in Relapsed or Relapsed and Refractory Multiple Myeloma: NPI-0052-101 Part 1. Blood (2016) 127(22):2693–700. doi: 10.1182/blood-2015-12-686378
22. Hideshima T, Chauhan D, Richardson P, Mitsiades C, Mitsiades N, Hayashi T, et al. NF-Kappa B as a Therapeutic Target in Multiple Myeloma. J Biol Chem (2002) 277(19):16639–47. doi: 10.1074/jbc.M200360200
23. Horton TM, Pati D, Plon SE, Thompson PA, Bomgaars LR, Adamson PC, et al. A Phase 1 Study of the Proteasome Inhibitor Bortezomib in Pediatric Patients With Refractory Leukemia: A Children’s Oncology Group Study. Clin Cancer Res (2007) 13(5):1516–22. doi: 10.1158/1078-0432.CCR-06-2173
24. Hu X, Xu J, Sun A, Shen Y, He G, Guo F. Successful T-Cell Acute Lymphoblastic Leukemia Treatment With Proteasome Inhibitor Bortezomib Based on Evaluation of Nuclear Factor-kappaB Activity. Leuk Lymphoma (2011) 52(12):2393–5. doi: 10.3109/10428194.2011.593271
25. Belver L, Ferrando A. The Genetics and Mechanisms of T Cell Acute Lymphoblastic Leukaemia. Nat Rev Cancer (2016) 16(8):494–507. doi: 10.1038/nrc.2016.63
26. Vilimas T, Mascarenhas J, Palomero T, Mandal M, Buonamici S, Meng F, et al. Targeting the NF-kappaB Signaling Pathway in Notch1-Induced T-Cell Leukemia. Nat Med (2007) 13(1):70–7. doi: 10.1038/nm1524
27. Koyama D, Kikuchi J, Hiraoka N, Wada T, Kurosawa H, Chiba S, et al. Proteasome Inhibitors Exert Cytotoxicity and Increase Chemosensitivity via Transcriptional Repression of Notch1 in T-Cell Acute Lymphoblastic Leukemia. Leukemia (2014) 28(6):1216–26. doi: 10.1038/leu.2013.366
28. Szczepanek J, Konatkowska B, Juraszewska E, Badowska W, Olejnik I, Kuzmicz M, et al. Differential Ex Vivo Activity of Bortezomib in Newly Diagnosed Paediatric Acute Lymphoblastic and Myeloblastic Leukaemia. Anticancer Res (2010) 30(6):2119–21.
29. Murata S, Takahama Y, Kasahara M, Tanaka K. The Immunoproteasome and Thymoproteasome: Functions, Evolution and Human Disease. Nat Immunol (2018) 19(9):923–31. doi: 10.1038/s41590-018-0186-z
30. Niewerth D, Franke NE, Jansen G, Assaraf YG, van Meerloo J, Kirk CJ, et al. Higher Ratio Immune Versus Constitutive Proteasome Level as Novel Indicator of Sensitivity of Pediatric Acute Leukemia Cells to Proteasome Inhibitors. Haematologica (2013) 98(12):1896–904. doi: 10.3324/haematol.2013.092411
31. Jenkins TW, Downey-Kopyscinski SL, Fields JL, Rahme GJ, Colley WC, Israel MA, et al. Activity of Immunoproteasome Inhibitor ONX-0914 in Acute Lymphoblastic Leukemia Expressing MLL-AF4 Fusion Protein. Sci Rep (2021) 11(1):10883. doi: 10.1038/s41598-021-90451-9
32. Jagani Z, Singh A, Khosravi-Far R. FoxO Tumor Suppressors and BCR-ABL-Induced Leukemia: A Matter of Evasion of Apoptosis. Biochim Biophys Acta (2008) 1785(1):63–84. doi: 10.1016/j.bbcan.2007.10.003
33. Jagani Z, Song K, Kutok JL, Dewar MR, Melet A, Santos T, et al. Proteasome Inhibition Causes Regression of Leukemia and Abrogates BCR-ABL-Induced Evasion of Apoptosis in Part Through Regulation of Forkhead Tumor Suppressors. Cancer Res (2009) 69(16):6546–55. doi: 10.1158/0008-5472.CAN-09-0605
34. Dewar R, Chen ST, Yeckes-Rodin H, Miller K, Khosravi-Far R. Bortezomib Treatment Causes Remission in a Ph+ALL Patient and Reveals FoxO as a Theranostic Marker. Cancer Biol Ther (2011) 11(6):552–8. doi: 10.4161/cbt.11.6.14675
35. Tasian SK, Loh ML, Hunger SP. Philadelphia Chromosome-Like Acute Lymphoblastic Leukemia. Blood (2017) 130(19):2064–72. doi: 10.1182/blood-2017-06-743252
36. Shiraz P, Payne KJ, Muffly L. The Current Genomic and Molecular Landscape of Philadelphia-Like Acute Lymphoblastic Leukemia. Int J Mol Sci (2020) 21(6):1–17. doi: 10.3390/ijms21062193
37. Tasian SK, Doral MY, Borowitz MJ, Wood BL, Chen IM, Harvey RC, et al. Aberrant STAT5 and PI3K/mTOR Pathway Signaling Occurs in Human CRLF2-Rearranged B-Precursor Acute Lymphoblastic Leukemia. Blood (2012) 120(4):833–42. doi: 10.1182/blood-2011-12-389932
38. Verma G, Datta M. The Critical Role of JNK in the ER-Mitochondrial Crosstalk During Apoptotic Cell Death. J Cell Physiol (2012) 227(5):1791–5. doi: 10.1002/jcp.22903
39. Hideshima T, Mitsiades C, Akiyama M, Hayashi T, Chauhan D, Richardson P, et al. Molecular Mechanisms Mediating Antimyeloma Activity of Proteasome Inhibitor PS-341. Blood (2003) 101(4):1530–4. doi: 10.1182/blood-2002-08-2543
40. Saunders P, Cisterne A, Weiss J, Bradstock KF, Bendall LJ. The Mammalian Target of Rapamycin Inhibitor RAD001 (Everolimus) Synergizes With Chemotherapeutic Agents, Ionizing Radiation and Proteasome Inhibitors in Pre-B Acute Lymphocytic Leukemia. Haematologica (2011) 96(1):69–77. doi: 10.3324/haematol.2010.026997
41. Schroder M, Kaufman RJ. The Mammalian Unfolded Protein Response. Annu Rev Biochem (2005) 74:739–89. doi: 10.1146/annurev.biochem.73.011303.074134
42. Kostova Z, Wolf DH. For Whom the Bell Tolls: Protein Quality Control of the Endoplasmic Reticulum and the Ubiquitin-Proteasome Connection. EMBO J (2003) 22(10):2309–17. doi: 10.1093/emboj/cdg227
43. Obeng EA, Carlson LM, Gutman DM, Harrington WJ Jr., Lee KP, Boise LH. Proteasome Inhibitors Induce a Terminal Unfolded Protein Response in Multiple Myeloma Cells. Blood (2006) 107(12):4907–16. doi: 10.1182/blood-2005-08-3531
44. Hetz C, Zhang K, Kaufman RJ. Mechanisms, Regulation and Functions of the Unfolded Protein Response. Nat Rev Mol Cell Biol (2020) 21(8):421–38. doi: 10.1038/s41580-020-0250-z
45. Buontempo F, Orsini E, Lonetti A, Cappellini A, Chiarini F, Evangelisti C, et al. Synergistic Cytotoxic Effects of Bortezomib and CK2 Inhibitor CX-4945 in Acute Lymphoblastic Leukemia: Turning Off the Prosurvival ER Chaperone BIP/Grp78 and Turning on the Pro-Apoptotic NF-Kappab. Oncotarget (2016) 7(2):1323–40. doi: 10.18632/oncotarget.6361
46. Sontag EM, Samant RS, Frydman J. Mechanisms and Functions of Spatial Protein Quality Control. Annu Rev Biochem (2017) 86:97–122. doi: 10.1146/annurev-biochem-060815-014616
47. Liu H, Westergard TD, Cashen A, Piwnica-Worms DR, Kunkle L, Vij R, et al. Proteasome Inhibitors Evoke Latent Tumor Suppression Programs in Pro-B MLL Leukemias Through MLL-Af4. Cancer Cell (2014) 25(4):530–42. doi: 10.1016/j.ccr.2014.03.008
48. Cheung LC, de Kraa R, Oommen J, Chua GA, Singh S, Hughes AM, et al. Preclinical Evaluation of Carfilzomib for Infant KMT2A-Rearranged Acute Lymphoblastic Leukemia. Front Oncol (2021) 11:631594. doi: 10.3389/fonc.2021.631594
49. Niewerth D, Jansen G, Assaraf YG, Zweegman S, Kaspers GJ, Cloos J. Molecular Basis of Resistance to Proteasome Inhibitors in Hematological Malignancies. Drug Resist Update (2015) 18:18–35. doi: 10.1016/j.drup.2014.12.001
50. Franke NE, Niewerth D, Assaraf YG, van Meerloo J, Vojtekova K, van Zantwijk CH, et al. Impaired Bortezomib Binding to Mutant Beta5 Subunit of the Proteasome is the Underlying Basis for Bortezomib Resistance in Leukemia Cells. Leukemia (2012) 26(4):757–68. doi: 10.1038/leu.2011.256
51. Lu S, Yang J, Song X, Gong S, Zhou H, Guo L, et al. Point Mutation of the Proteasome Beta5 Subunit Gene is an Important Mechanism of Bortezomib Resistance in Bortezomib-Selected Variants of Jurkat T Cell Lymphoblastic Lymphoma/Leukemia Line. J Pharmacol Exp Ther (2008) 326(2):423–31. doi: 10.1124/jpet.108.138131
52. Lu S, Yang J, Chen Z, Gong S, Zhou H, Xu X, et al. Different Mutants of PSMB5 Confer Varying Bortezomib Resistance in T Lymphoblastic Lymphoma/Leukemia Cells Derived From the Jurkat Cell Line. Exp Hematol (2009) 37(7):831–7. doi: 10.1016/j.exphem.2009.04.001
53. Lu S, Chen Z, Yang J, Chen L, Gong S, Zhou H, et al. Overexpression of the PSMB5 Gene Contributes to Bortezomib Resistance in T-Lymphoblastic Lymphoma/Leukemia Cells Derived From Jurkat Line. Exp Hematol (2008) 36(10):1278–84. doi: 10.1016/j.exphem.2008.04.013
54. Niewerth D, Kaspers GJ, Assaraf YG, van Meerloo J, Kirk CJ, Anderl J, et al. Interferon-Gamma-Induced Upregulation of Immunoproteasome Subunit Assembly Overcomes Bortezomib Resistance in Human Hematological Cell Lines. J Hematol Oncol (2014) 7:7. doi: 10.1186/1756-8722-7-7
55. Cortes J, Thomas D, Koller C, Giles F, Estey E, Faderl S, et al. Phase I Study of Bortezomib in Refractory or Relapsed Acute Leukemias. Clin Cancer Res (2004) 10(10):3371–6. doi: 10.1158/1078-0432.CCR-03-0508
56. Messinger Y, Gaynon P, Raetz E, Hutchinson R, Dubois S, Glade-Bender J, et al. Phase I Study of Bortezomib Combined With Chemotherapy in Children With Relapsed Childhood Acute Lymphoblastic Leukemia (ALL): A Report From the Therapeutic Advances in Childhood Leukemia (TACL) Consortium. Pediatr Blood Cancer (2010) 55(2):254–9. doi: 10.1002/pbc.22456
57. Iguchi A, Cho Y, Sugiyama M, Terashita Y, Ariga T, Hosoya Y, et al. Bortezomib Combined With Standard Induction Chemotherapy in Japanese Children With Refractory Acute Lymphoblastic Leukemia. Int J Hematol (2017) 106(2):291–8. doi: 10.1007/s12185-017-2235-z
58. Hasegawa D, Yoshimoto Y, Kimura S, Kumamoto T, Maeda N, Hara J, et al. Bortezomib-Containing Therapy in Japanese Children With Relapsed Acute Lymphoblastic Leukemia. Int J Hematol (2019) 110(5):627–34. doi: 10.1007/s12185-019-02714-x
59. August KJ, Guest EM, Lewing K, Hays JA, Gamis AS. Treatment of Children With Relapsed and Refractory Acute Lymphoblastic Leukemia With Mitoxantrone, Vincristine, Pegaspargase, Dexamethasone, and Bortezomib. Pediatr Blood Cancer (2020) 67(3):e28062. doi: 10.1002/pbc.28062
60. Colunga-Pedraza JE, Gonzalez-Llano O, Gonzalez-Martinez CE, Gomez-Almaguer D, Yanez-Reyes JM, Jimenez-Antolinez V, et al. Outpatient Low Toxic Regimen With Bortezomib in Relapsed/Refractory Acute Lymphoblastic Leukemia in Pediatrics and AYA Patients: Single-Center Mexican Experience. Pediatr Blood Cancer (2020) 67(5):e28241. doi: 10.1002/pbc.28241
61. Yeo KK, Gaynon PS, Fu CH, Wayne AS, Sun W. Bortezomib, Dexamethasone, Mitoxantrone, and Vinorelbine (BDMV): An Active Reinduction Regimen for Children With Relapsed Acute Lymphoblastic Leukemia and Asparaginase Intolerance. J Pediatr Hematol Oncol (2016) 38(5):345–9. doi: 10.1097/MPH.0000000000000560
62. Messinger YH, Gaynon PS, Sposto R, van der Giessen J, Eckroth E, Malvar J, et al. Bortezomib With Chemotherapy Is Highly Active in Advanced B-Precursor Acute Lymphoblastic Leukemia: Therapeutic Advances in Childhood Leukemia & Lymphoma (TACL) Study. Blood (2012) 120(2):285–90. doi: 10.1182/blood-2012-04-418640
63. Bertaina A, Vinti L, Strocchio L, Gaspari S, Caruso R, Algeri M, et al. The Combination of Bortezomib With Chemotherapy to Treat Relapsed/Refractory Acute Lymphoblastic Leukaemia of Childhood. Br J Haematol (2017) 176(4):629–36. doi: 10.1111/bjh.14505
64. Horton TM, Whitlock JA, Lu X, O’Brien MM, Borowitz MJ, Devidas M, et al. Bortezomib Reinduction Chemotherapy in High-Risk ALL in First Relapse: A Report From the Children’s Oncology Group. Br J Haematol (2019) 186(2):274–85. doi: 10.1111/bjh.15919
65. Roy P, Islam R, Saha D, Gogoi M, Kumar Mishra D, Arora N, et al. Efficacy and Safety of a Bortezomib and Reduced-Intensity Cytarabine-Based Protocol, TMC ALLR1, for Relapsed Childhood ALL in India. Br J Haematol (2019) 186(6):861–5. doi: 10.1111/bjh.16005
66. Kaspers GJL, Niewerth D, Wilhelm BAJ, Scholte-van Houtem P, Lopez-Yurda M, Berkhof J, et al. An Effective Modestly Intensive Re-Induction Regimen With Bortezomib in Relapsed or Refractory Paediatric Acute Lymphoblastic Leukaemia. Br J Haematol (2018) 181(4):523–7. doi: 10.1111/bjh.15233
67. Nachmias B, Shaulov A, Gatt ME, Shapira M, Gural A. A Bortezomib-Based Protocol Induces a High Rate of Complete Remission With Minor Toxicity in Adult Patients With Relapsed/Refractory Acute Lymphoblastic Leukemia. Acta Haematol (2018) 140(4):209–14. doi: 10.1159/000493252
68. Jain H, Sengar M, Goli VB, Thorat J, Tembhare P, Shetty D, et al. Bortezomib and Rituximab in De Novo Adolescent/Adult CD20-Positive, Ph-Negative Pre-B-Cell Acute Lymphoblastic Leukemia. Blood Adv (2021) 5(17):3436–44. doi: 10.1182/bloodadvances.2020003368
69. Wartman LD, Fiala MA, Fletcher T, Hawkins ER, Cashen A, DiPersio JF, et al. A Phase I Study of Carfilzomib for Relapsed or Refractory Acute Myeloid and Acute Lymphoblastic Leukemia. Leuk Lymphoma (2016) 57(3):728–30. doi: 10.3109/10428194.2015.1076930
70. Jonas BA, Fisch SC, Rosenberg AS, Hoeg RT, Tuscano JM, Abedi M. Phase I Study of Escalating Doses of Carfilzomib With HyperCVAD in Patients With Newly Diagnosed Acute Lymphoblastic Leukemia. Am J Hematol (2021) 96(4):E114–E7. doi: 10.1002/ajh.26105
71. Hosseini MS, Mohammadi MH, Vahabpour Roudsari R, Jafari L, Mashati P, Gharehbaghian A. Proteasome Inhibition by Carfilzomib Induced Apotosis and Autophagy in a T-Cell Acute Lymphoblastic Leukemia Cell Line. Iran J Pharm Res (2019) 18(Suppl1):132–45. doi: 10.22037/ijpr.2020.112692.13898
72. Takahashi K, Inukai T, Imamura T, Yano M, Tomoyasu C, Lucas DM, et al. Anti-Leukemic Activity of Bortezomib and Carfilzomib on B-Cell Precursor ALL Cell Lines. PloS One (2017) 12(12):e0188680. doi: 10.1371/journal.pone.0188680
73. Roeten MSF, van Meerloo J, Kwidama ZJ, Ter Huizen G, Segerink WH, Zweegman S, et al. Pre-Clinical Evaluation of the Proteasome Inhibitor Ixazomib Against Bortezomib-Resistant Leukemia Cells and Primary Acute Leukemia Cells. Cells (2021) 10(3):1–14. doi: 10.3390/cells10030665
74. Chiaretti S, Foa R. Management of Adult Ph-Positive Acute Lymphoblastic Leukemia. Hematol Am Soc Hematol Educ Program (2015) 2015:406–13. doi: 10.1182/asheducation-2015.1.406
75. Nagafuji K, Miyamoto T, Eto T, Ogawa R, Okumura H, Takase K, et al. Prospective Evaluation of Minimal Residual Disease Monitoring to Predict Prognosis of Adult Patients With Ph-Negative Acute Lymphoblastic Leukemia. Eur J Haematol (2019) 103(3):164–71. doi: 10.1111/ejh.13268
76. Wang H, Zhou Y, Huang X, Zhang Y, Qian J, Li J, et al. Minimal Residual Disease Level Determined by Flow Cytometry Provides Reliable Risk Stratification in Adults With T-Cell Acute Lymphoblastic Leukaemia. Br J Haematol (2021) 193(6):1096–104. doi: 10.1111/bjh.17424
77. Coustan-Smith E, Mullighan CG, Onciu M, Behm FG, Raimondi SC, Pei D, et al. Early T-Cell Precursor Leukaemia: A Subtype of Very High-Risk Acute Lymphoblastic Leukaemia. Lancet Oncol (2009) 10(2):147–56. doi: 10.1016/S1470-2045(08)70314-0
Keywords: proteasome inhibitors, acute lymphoblastic leukaemia, bortezomib, carfilzomib, ixazomib
Citation: Sin C-f and Man P-hM (2021) The Role of Proteasome Inhibitors in Treating Acute Lymphoblastic Leukaemia. Front. Oncol. 11:802832. doi: 10.3389/fonc.2021.802832
Received: 27 October 2021; Accepted: 03 December 2021;
Published: 23 December 2021.
Edited by:
Anna Maria Testi, Sapienza University of Rome, ItalyReviewed by:
Qingbin Cui, University of Toledo, United StatesCopyright © 2021 Sin and Man. This is an open-access article distributed under the terms of the Creative Commons Attribution License (CC BY). The use, distribution or reproduction in other forums is permitted, provided the original author(s) and the copyright owner(s) are credited and that the original publication in this journal is cited, in accordance with accepted academic practice. No use, distribution or reproduction is permitted which does not comply with these terms.
*Correspondence: Chun-fung Sin, scf185@pathology.hku.hk