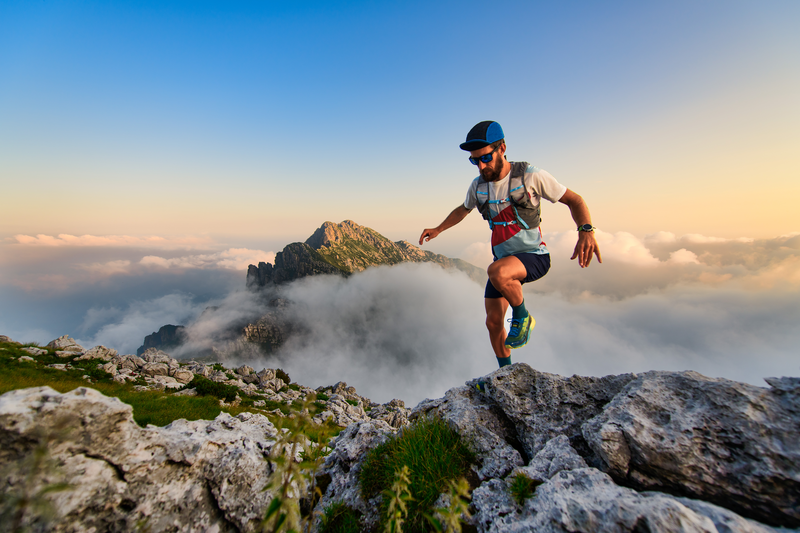
94% of researchers rate our articles as excellent or good
Learn more about the work of our research integrity team to safeguard the quality of each article we publish.
Find out more
REVIEW article
Front. Oncol. , 15 December 2021
Sec. Cancer Molecular Targets and Therapeutics
Volume 11 - 2021 | https://doi.org/10.3389/fonc.2021.801779
Tyrosine kinase inhibitors (TKIs) have revolutionized the treatment of patients with chronic myeloid leukemia (CML). However, continued use of these inhibitors has contributed to the increase in clinical resistance and the persistence of resistant leukemic stem cells (LSCs). So, there is an urgent need to introduce additional targeted and selective therapies to eradicate quiescent LSCs, and to avoid the relapse and disease progression. Here, we focused on emerging BCR-ABL targeted and non-BCR-ABL targeted drugs employed in clinical trials and on alternative CML treatments, including antioxidants, oncolytic virus, engineered exosomes, and natural products obtained from marine organisms that could pave the way for new therapeutic approaches for CML patients.
Chronic myeloid leukemia (CML) is caused by a t (9;22) (q34; q11) reciprocal translocation resulting in the fusion between the breakpoint cluster region (BCR) and Abelson leukemia (ABL1) genes (1). The resultant BCR-ABL fusion protein, is a hyper-activated tyrosine kinase. The target-therapy is mainly based on the use of tyrosine kinase inhibitors (TKIs) that can inhibit this fusion protein’s activity (1).
Currently, the annual incidence of CML varies from 0.7 to 1.0 cases per 100 000 inhabitants, it increases with age and is higher in men than women with a ratio ranging from 1.2- 1.7 (2). CML occurs in the chronic phase (CML-CP) associated with massive expansion of myeloid cells. Acquisition of genetic mutations in BCR-ABL1 and/or epigenetic alterations results in progression of the disease to an advanced phase classified as accelerated (CML-AP) or blastic (CML-BP) (3). In most classification systems, the following criteria are considered for a differential diagnosis between AP and BP: the percentage of blasts or basophils, white blood cells and splenomegaly unresponsive to therapy (4).
The development of TKIs has been crucial role in increasing the survival of patients in chronic-phase and limiting the disease progression. However, some limitations of TKIs treatment are related to the accumulation of BCR-ABL1 mutations, which leads to the initial and persistent resistance to TKIs dependent to BCR-ABL. Furthermore, TKI-therapy is not effective on quiescent leukemia stem cells (LSCs) as they exhibit independent BCR-ABL TKI resistance (5). It was demonstrated that there is a connection between the quiescent LSCs and the CML progression as their genetic instability favors the accumulation of mutations, stimulating the progression of the disease to accelerated and blastic-phase (6). The molecular pathways altered in CML, which include the JAK/STAT, NF-kB, WNT/β-catenin, PI3K/AKT/mTOR, Hedgehog and NOTCH pathways, are involved in LSCs maintenance and resistance to TKIs (7). In addition to the side effects of TKIs approach (8), this therapeutic strategy has the following disadvantages: the high costs of TKIs and healthcare, of molecular monitoring of patients during the therapy (9) and teratogenicity (10).
Several studies are focused on the achievement of the treatment-free remission (TFR), a condition aiming to the interruption of TKI therapy in CML patients without recurrence of the disease. The recent guidelines recommend TKI discontinuation in CML-CP patients pre-treated for at least 3 years who exhibited molecular response with BCR-ABL1 level of < 0.01% or a 4-log reduction in transcription level respect to the baseline (MR4) for at a minimum 2 years (11). The efficacy of TFR seems related both to the duration of treatment with TKIs and the persistent molecular response. During the first 6 months after TKIs discontinuation, a deeper monitoring of patients is required to identify molecular relapse (11). Conversely, it was observed that only 14% of the total molecular recurrences occurred in follow-up CML patients after 2 years from TKIs discontinuation (12). Although further investigations are required in order to better clarify the failure of TFR, the level of natural killer cells and the presence of factors that stimulate the resistance of LSCs could affect the time of TFR (13). However, it is necessary to restart with TKI-therapy when molecular recurrences are detectable in patients (11). Here, we discuss the mechanisms underlying TKIs-resistance and we provide an update on current and innovative therapeutic approaches as strategies for CML therapy, focusing on in vitro and in vivo findings.
Tyrosine kinase inhibitors (TKIs) have improved survival of patients with CML. Imatinib (Gleevec), a drug of first-line in the therapy of CML, was approved by Food and Drug Administration (FDA) in 2001 for accelerated phase and subsequently for newly diagnosed CML-CP patients. TKIs recognize the binding site for ATP in BCR-ABL kinase but with different affinity related to eventual mutations occured (Figure 1A). The missense and frameshift mutations in this site are related to Imatinib-resistance development (14, 15). For this reason, second and third-generation TKIs were developed: Dasatinib (Sprycel), Nilotinib (Tasigna), Bosutinib (Bosulif) and Ponatinib (Iclusig) (16, 17). In particular, European Medicines Agency (EMA) approved Dasatinib and Bosutinib in all three phases of CML while Nilotinib only during the chronic and accelerated phase (18–211–3). In the Table 1, we report the known BCR-ABL mutations responsible for drug resistance in patients. By evaluating the efficacy of second-generation TKIs versus Imatinib in peripheral blood leukocytes derived from newly diagnosed patients, these drugs significantly induced apoptosis more than Imatinib (43). In addition, these drugs were tested in clinical trials for newly diagnosed CML-CP patients. After a follow-up of 12 months with Dasatinib (44), Nilotinib (45) or Bosutinib (46), significantly higher rates of major molecular response (MMR) and complete cytogenetic response (CCyR) have been observed (NCT00481247; NCT00471497; NCT02130557). The most common side effects are pleural effusion occurring after Dasatinib treatment (47, 48), headache and skin rashes more frequent with Nilotinib compared to Imatinib (45). Increased transaminase levels and diarrhea are observed in patients treated with Bosutinib respect to Imatinib (46). According to guidelines, the use of second-generation TKIs is recommended for patients without comorbidities over Imatinib monotherapy (8) in order to obtain a faster response and thus make the patients eligible for TFR (11). It is necessary to re-initiate the therapy when molecular relapse occurs (11). A new selective TKI is Radotinib (IY5511HCl), currently approved in Korea for CML-CP patients with newly diagnosis or not responsive to other TKIs (49). The efficacy and safety of Radotinib were demonstrated in a phase II study (NCT01602952). After treatment with this TKI, 65% of enrolled patients had a major cytogenetic response (MCyR) and a CCyR in 47% of them. The most common hematological side effects are thrombocytopenia (24.7%) and anemia (5.2%); while the non-hematological ones are fatigue (3.9%), asthenia (3.9%) and nausea (2.6%) (50). Compared to Imatinib, Radotinib showed superior efficacy with 91% CCyR versus 77% and induced MMR in 52% of patients (NCT01511289) (51).
Figure 1 Current status of TKI therapy for CML. (A) Most approved drugs recognize the binding site for ATP in BCR-ABL, as: Imatinib, Dasatinib, Nilotinib, Bosutinib, and Ponatinib. Asciminib binds to the myristoyl pocket of the BCR-ABL kinase. The new molecules of third and fourth generation TKIs including: Olverembatinib (HQP1351), Vodobatinib (K0706) and PF-114. (B) Omacetaxine is an approved inhibitor that blocks protein synthesis.
Table 1 The main TKIs approved for CML patients during the various phases of the disease, their molecular targets and BCR-ABL mutations responsible for drug resistance in CML patients.
Ponatinib is a third-generation TKI employed for CML patients carrying the T315I mutation in BCR-ABL (BCR-ABLT315I) for which first and second-generation TKIs result inefficient (38). Unlike the other inhibitors mentioned above, Ponatinib inhibits the BCR-ABLT315I activity due to its major affinity to modified amino acid I315 (52). In a recent clinical trial, its efficacy was demonstrated with the achievement of prolonged MMR and MCyR in patients intolerant to Dasatinib/Nilotinib or having T315I mutation (NCT01207440) (53). Adverse effects seen after Ponatinib-treatment are rash, abdominal pain, dryness of the skin, thrombocytopenia and increased lipase levels (39), thrombosis and other cardiovascular problems (54). For this reason, several studies evidence the need to use low doses of Ponatinib to treat CML patients resistant to other TKIs without impairing its efficacy (55, 56).
Although TKIs have proven their clinical efficacy in the treatment of CML, the onset of resistance still represents a critical issue. In order to overcome the resistance, alternative therapies have been developed. For this reason, innovative BCR-ABL targeted or NON BCR-ABL targeted drugs can be considered as a valid alternative for CML patients resistant/intolerant to conventional treatment.
New approaches based on combination of drugs that act on different pathways can improve their effectiveness to obtain optimal response and reach TFR more quickly (57). We describe new specific drugs, focusing on the mechanisms of action, efficacy and safety. In detail, the molecules acting on BCR-ABL include Asciminib (ABL001), Olverembatinib (HQP1351), PF-114 and K0706 (58).
Non-BCR-ABL targeted therapies are employed to act on different pathways than those related to TKIs and/or promote LSCs eradication to overcome TKIs resistance in CML. Among these, we describe the action of inhibitors targeting farnesyl transferase (Lonafarnib and Tipifarnib), mammalian target of Rapamycin (mTOR, Rapamycin and Everolimus), Janus kinase 2 (JAK2), histone deacetylase (HDAC) and aurora kinase; peroxisome proliferator-activated receptor gamma (PPARγ) activators, hypomethylating agents and Omacetaxine. All drugs tested in clinical studies are summarized in Table 2.
Asciminib is a potent, specific BCR-ABL kinase inhibitor that recognizes the myristoyl pocket contrary to conventional TKIs that target the ATP binding site (Figure 1A). Asciminib binds to the BCR-ABL oncoprotein, induces a conformational change in the myristoyl pocket leading to the inactive state of the kinase and its regulation (59). Its different mechanism of action could increase the efficacy especially in patients resistant or with therapeutic failure to conventional TKIs.
A combined approach of TKIs with Asciminib is important, having a different target therapy site.
In phase I studies (NCT03595917 and NCT02081378), Asciminib monotherapy showed the achievement of MCyR in 82% of chronic phase CML-patients who failed with three or more TKIs (57, 60), and at 12 months the incidence of MMR and CCyR were 48% and 70%, respectively (61). Clinical trials are ongoing to evaluate the efficacy of the combination of Asciminib with Imatinib (phase II study: NCT03578367), Dasatinib (phase I study: NCT03595917), Nilotinib (phase 1 study: NCT02081378) and Bosutinib (phase III study: NCT03106779). However, preliminary data confirm that this drug has greater efficacy compared to Bosutinib and high tolerability in CML-CP patients resistant or intolerant to ≥2 prior TKIs (62). Recently, the FDA approved Asciminib (Scemblix, Novartis AG) for patients in the chronic phase with failure to ≥2 TKIs or carrying T315I mutation (634). In conclusion, the combination of Asciminib with ATP-competitive TKIs can be considered a valid therapeutic approach in CML patients with failure of more TKIs.
Olverembatinib (HQP1351) is a new third-generation TKI, active against both wild type (wt) and mutated BCR-ABL (mut) isoforms. Recently, Jiang et al. demonstrate, in a phase I study in patients with CML resistant to current TKIs therapies, a long-lasting antitumor activity associated with high tolerability, particularly in subjects with T315I mutation (64). Preliminary data on patients with mean follow-up of 12.8 months showed that the treatment with HQP1351 promoted the achievement of a complete haematological response (CHR) in 94.5% of CML-CP and 84.6% of CML-AP patients versus baseline. In 69.1% of chronic phase subjects, the treatment induced MCyR compared to 42.9% in accelerated phase. A notable increase in CCyR was instead observed in 60.5% of patients with CML-CP compared to baseline, in the corresponding CML-AP patients was only 35.7%. These results were also confirmed in patients carrying BCR-ABL T315I mutation (64). Two Phase II clinical studies are ongoing in China in patients with CML-CP and CML-AP harboring T315I mutation to evaluate the efficacy of HQP1351 both on the MCyR and major hematological response, as well as its safety profile (NCT03883087 and NCT03883100). Non-severe clinical effects, such as thrombocytopenia, anaemia and leukopenia, were found in CML-CP and CML-AP patients (65).
Vodobatinib (K0706) and PF-114 are innovative ATP-competitive TKIs belonging to third- and fourth-generation, respectively. K0706 is a novel BCR-ABL TKI direct to wild-type and mutated isoforms although with less affinity for T315I mutation (66). A phase I/II study (NCT02629692) is ongoing to evaluate the efficacy and safety of K0706 in patients with comorbidities precluding the use of conventional second-generation TKIs or after ≥ 3 TKIs failure. The initial phase I results of this study showed disease progression in patients carrying BCR-ABL T315I mutation, therefore the enrolment of patients with this mutation was stopped (67).
PF-114 is a TKI similar to Ponatinib due to its chemical structure, capable of acting on both wt and mutated BCR-ABL including T315I. Unlike Ponatinib, PF-114 was designed not to inhibit the vascular endothelial growth factor receptor (VEGFR) in order to reduce cardiovascular effects in patients (68). 65 patients, resistant to second-generation TKIs or carrying the BCR-ABLT315I mutation, were enrolled in a phase I/II clinical study to evaluate safety, pharmacokinetics and tolerability still ongoing (NCT02885766). In a phase I study, Turkina et al. achieved a complete hematologic response in 42.1% patients treated with PF-114 of which 37.5% were carriers of the T315I mutation. At different doses, mainly 200 and 300 mg, a complete MMR occurred in 11% of patients and a MCyR in 28.5% (69). However, the most effective dose was 300 mg with effective response in 41.6% of patients with BCR-ABLT315I. The most side effect of PF-114 is reversible grade 3 skin toxicity was found at 400 mg, in 11/12 patients (70).
In conclusion, PF-114 and K0706 have an acceptable safety profile in patients who are resistant and/or intolerant to ≥3 TKIs for K0706 and ≥ 2 for PF-114 (67, 70).
Farnesyl transferase inhibitors (FTIs), as Tipifarnib or Zarnestra (R115777) and Lonafarnib (SCH66336), inhibit the activity of farnesyl transferase, acting on downstream targets such as the oncogene RAS and resulting in cell growth arrest (71, 72).
In a first pilot study conducted with Tipifarnib as monotherapy, a complete or partial haematological response was detected in 7/22 patients with a minor transient cytogenetic response in 4 of these (73).
Co-treatment with Tipifarnib, after previous Imatinib failure, induced a cytogenetic and overall haematological response in 36% and 76% of patients, respectively (NCT00040105). The combination was also well tolerated and had a selective activity for mutated kinase domains (74).
Regarding Lonafarnib, its efficacy was evaluated as monotherapy in a pilotal study on 13 patients with chronic and accelerated phase CML, finding a haematological response in only 15.3% (NCT00038597) (75). Subsequently, the combination with Imatinib was tested on a larger court of CML patients, finding an increase in its efficacy in 35% of patients, based on haematological and cytogenetic responses (NCT00047502) (76).
Using Lonafarnib as monotherapy only small benefits are seen in patients, while the combination can be used in patients unresponsive to TKI therapy (57).
The specific target of mTOR inhibitors is a serine/threonine kinase which is over-activated in CML. Rapamycin and Everolimus belong to this family. To date, only one clinical trial is currently ongoing for Rapamycin in combination with DNA-damaging agents (etoposide) (NCT00776373) (57). Everolimus blocks the constitutive activation of mTOR and makes cells more sensitive to Imatinib in combination therapy. Two clinical trials are underway to prove its safety and efficacy both as monotherapy and in co-treatment (NCT00081874 and NCT00093639).
JAK2 inhibitors, such as Ruxolitinib, act on Janus kinase 2 (JAK2), an intracellular kinase commonly involved in phosphorylation and activation of STAT5 (77). There is considerable clinical interest in inhibiting JAK2 activity to deplete quiescent TKIs-resistant LSCs. The advantage of these inhibitors may be related to their ability to downregulate the JAK2/STAT5 pathway which plays a crucial role in the proliferation and survival of CML LSCs (78). A promising therapeutic approach given by the combination of Ruxolitinib and Nilotinib has been evaluated in adult CML-CP patients in a phase 1 study. Preliminary data confirm that the combination Ruxolitinib and Nilotinib is safe and well tolerated with encouraging molecular responses (NCT01702064; NCT02253277). However, these data need to be validated in a phase II study (79). An ongoing phase II study has the primary objective to evaluate the molecular responses after the combination of Ruxolitinib plus conventional TKIs (NCT03654768). The effectiveness on TFR of adding Ruxolitinib to TKIs is currently under a phase II study (NCT03610971).
In conclusion, the main advantage in the use of this therapeutic approach with JAK2 inhibitors may be associated with the eradication of LSCs responsible for BCR-ABL independent resistance.
Emerging alternative therapies are Histone deacetylase inhibitors (HDACis), involved in the epigenetic modifications that regulate the acetylation state of histones. Zhang et al. proved in CML cells that the co-treatment of HDACi with Imatinib downregulated the expression levels of genes involved in the maintenance of CML LSCs, such as Wnt/β-catenin (80). Panobinostat is a HDACi, whose efficacy was demonstrated both in vitro including on cells carrying BCR-ABLT315I and in vivo as reported in two phase 2 clinical trials (NCT00449761 and NCT00451035) (57). In patients CML-CP resistant to ≥ 2 TKIs, Panobinostat monotherapy didn’t induce MCyR but only in 3% a complete haematological response was observed (81). Finally, emerging evidence has shown that HDACis may have promising action as a combination therapy in patients with TKIs failure compared to the reduced efficacy in monotherapy (82).
Aurora kinase inhibitors act on the serine-threonine kinases of Aurora family proteins which are key regulators of cell division whose dysregulation results in DNA alterations and cellular transformation. Tozasertib and Danusertib are Aurora kinase inhibitors. The first drug is known to avoid CML progression and even lead to a change from advanced to chronic phase in CML patients, including those carrying BCR-ABLT315I. The second agent is characterized by a dual role, since it can inhibits both Aurora kinase family and BCR-ABL, including T315I (82). As monotherapy, a phase II study was performed in CML-CP patients with T315I mutation (NCT00405054). Overall, a major cytogenetic response was achieved in 8% of patients, unconfirmed complete or partial response in 6% and only 13.3% had a complete haematological response (83). While, the combination with Dasatinib was used in phase I study to determine safety and efficacy of Tozasertib (NCT00500006).
Danusertib is a drug with an acceptable safety profile, as demonstrated in a clinical trial (EudraCT number 2007-004070-18). When it was administered as a single drug, complete molecular and cytogenetic responses appeared to be constant over time in all patients carrying T315I BCR-ABL mutation (84).
Glitazones are PPARgamma (PPARγ) activators utilized for diabetic patients. However, they can be successfully employed in CML therapy, especially to counteract the persistence of CML LSCs. PPARγ reduce the expression of STAT5 and its downstream targets which are involved in the survival of quiescent CML LSCs. As shown in clinical trials (NCT02888964; EudraCT 2009‐011675‐79), the combination of Pioglitazone with Imatinib was well tolerated in CML patients with a better molecular response (MR 4.5) in 56% versus 23% found in patients treated with Imatinib alone (85). Finally, there is a current phase II study that aims to further characterize the use of Pioglitazone in patients with TKIs failure after first TFR to proceed with the second discontinuation attempt (NCT02889003). In summary, this combination constitutes a potential strategy for the eradication of quiescent LSCs.
High methylation levels in ABL play a critical role in the progression from CML-CP to CML-BP (86). Since DNA methylation correlates with the progression, the use of hypomethylating agents constitutes an alternative therapy in combination with TKIs in CML. Among these drugs, Decitabine in combination with Dasatinib induced complete haematological response in 48% of patients, achieving MCyR and MMR in 44% and 33% of patients, respectively (NCT01498445) (87). Severe myelosuppression was an adverse effect occurring in patients treated with this methylating agent (88). Two clinical trials of phase II are evaluating the efficacy of Decitabine both as monotherapy and in combination with Imatinib in patients who had no benefit from conventional therapy and disease progression (NCT00042016; NCT00054431). Venetoclax, a selective BCL2 inhibitor, is approved in combination with hypomethylating agents for newly diagnosed Acute Myeloid Leukemia (AML) (89). Preclinical studies demonstrated a synergism of Venetoclax in combination with BCR-ABL TKI in the eradication of LSCs in CML-BP (90). Indeed, the overall response rate (ORR) was 75% in CML-BP and 43% in AML patients (91).
A new drug relevant for the treatment of chronic or accelerated phase CML is Omacetaxine, considered a third generation TKI capable of inhibiting protein synthesis (Figure 1B). The efficacy of Omacetaxine has been evaluated in several clinical trials in patients resistant to more than 2 TKIs (NCT02078960, phase I/II and NCT00462943, phase II) and carriers of the T315I mutation (NCT00375219, phase II). FDA guidelines indicate this drug effective for patients intolerant/resistant to ≥2 TKI, including those with BCR-ABLT315I (68). Based on the results obtained by Cortes et al., 18% of patients with CP-CML achieved persistent MCyR for 12.5 months; while only 14% of CML-AP patients had a major haematological response for 4.7 months (92). Therefore, long-term administration of Omacetaxine results in prolonged benefits. On the other hand, the main adverse effect is the haematological toxicity induced in 10% of CML-CP patients and in 5% of CML-AP patients resulting in discontinuous treatment (92).
The activity of BCR-ABL oncoprotein is linked to ROS production leading to the onset of new mutations (93). This “self-mutagenesis” favors the progression of CML from CP to BP (94). Usually, alterated levels of antioxidant enzyme superoxide dismutase (SOD) (95), thiobarbituric acid reactive substances (TBARS), total lipid hydroperoxides (96) and higher plasmatic malondialdehyde levels are observed in CML patients compared to healthy subjects (97). Antioxidants could be a useful weapon to reduce oxidative stress and to hinder the onset of new BCR-ABL mutations, as demonstrated in vivo with xenograft mice (98). Ghalaut et al. detected a reduction in nitrosative stress (NO) levels following the addition of turmeric to the diet of patients treated with Imatinib (99) proposing the antioxidants as possible new therapeutic approach. The anti-proliferative and pro-apoptotic properties of curcumin are known, as demonstrated in K562 leukemia cells (100). It was also proved that curcumin was able to increase PTEN and inhibit BCR-ABL activity in vitro, while the tumor size was reduced in xenograft model (101). Wu et al. proposed two curcumin derivates, C086 and C817, as a potential therapeutic approach to counteract Imatinib-resistance (102, 103). Curcumin derivates are capable of promoting apoptosis in cells carrying the following mutations: Y253F, Q252H and, including, T315I. Moreover, a reduced survival of CML progenitor/stem cells, obtained from patients ‘bone marrow, was observed after the treatment with the same derivatives of curcumin (102, 103). These agents may constitute a new potential therapeutic approach to overcome the persistence of LSCs resistant to TKIs.
Although several antioxidants play an essential role in reducing oxidative damage, some of them at high concentrations show pro-oxidant action and induce death in cancer cells (104) such as resveratrol (RES) which has a dual effect in a dose-dependent manner (105). The latter is a compound that at low doses (20 µM) it is able to reduce oxidative stress (106) while at higher doses (60μmol/L) it induces apoptosis in K562 cells depending on the duration of the treatment (107).
Damiano et al. evaluated the antiproliferative effects of combining TKIs (Nilotinib or Dasatinib) with different antioxidants including RES, δ-tocotrienol (δ-TOCO) and a new recombinant mitochondrial manganese containing superoxide dismutase (rMnSOD) in K562 cells. The results suggested that the combination increased the action of inhibitors and induced the cytotoxic effect through the production of ROS confirming the potential use of antioxidants in CML (108).
The development of a specific target-therapy is a major focus of cancer research. In recent years, oncolytic virus (OV) therapy or virotherapy has become a promising alternative strategy through the use of wild type or genetically modified viruses (109).
In detail, the most exploited oncolytic viruses in virotherapy are: adenovirus, herpesvirus, herpes simplex (HSV), measles virus, parvovirus and reovirus (110–113). For human adenovirus and herpes simplex 1 (HSV-1), additional genetic modifications are required in order to both mitigate virulence and improve specificity and safety (114, 115). Non-human viruses don’t need to be modified due to their species-specific infection capacity (116–118).
In our previous studies, we demonstrated the efficacy of the Caprine herpesvirus type 1 (CpHV-1) in promoting the death of different tumor cell lines. However, it was not able to reduce the viability of K562 probably due to a lower permissiveness of these cells to this type of caprine virus (116).
To improve the efficacy of virotherapy, viruses are often engineered or modified with tumor suppressor or pro-apoptotic genes (119). As suggested by in vitro and in vivo studies, adenoviruses with chimeric Ad5/11 fiber expressing Beclin-1 could be a promising approach in CML therapy (120).
By overexpressing Beclin-1 protein in chimeric adenovirus 5/11 (SG511-BECN), Tong et al. induced autophagy in primary cells obtained from Imatinib-resistant CML patients.
Increased survival has also been shown in xenografted mice after treatment with SG511-BECN (121).
Li et al. used oncolytic viruses in combination with other anticancer drugs to demonstrate their efficacy in CML. The data confirmed a high antitumor action in multidrug- resistant CML cells after combined treatment with SG511-BECN and chemotherapy agents (122). In conclusion, these promising results reinforce the idea of employing this new type of therapeutic approach also for CML disease.
Exosomes are membrane-bound extracellular vesicles (EVs) that carry both RNA and other bio-molecules, such as lipids and proteins; they are often found in biological fluids, including blood, urine and cerebrospinal fluid (123).
The presence of BCR-ABL is now also known in exosomes obtained from CML cells (124), therefore these EVs could be useful for the identification of new biomarkers for CML. One of these is miR-140-3p, upregulated in CML patients and involved in the inflammatory process (125).
Exosomes can also be used as drug carriers in fact Bellavia et al. employed exosomes expressing Interleukin 3 (IL-3), whose receptor is usually overexpressed in CML blasts, to deliver Imatinib or BCR-ABL siRNA. These exosomes thus loaded were capable of inhibiting cancer cell growth both in vitro and in vivo (126). While these data are promising, further studies are needed to demonstrate and test the efficacy of exosomes as a vehicle for CML-specific drugs and siRNA.
Marine organisms are able to produce metabolites with bioactivities useful for the treatment or prevention of human pathologies (127–130). There are currently on the market fourteen compounds derived from marine species used for various types of cancer (such as multiple myeloma, leukemia, lymphoma, breast, ovarian, lung and urothelial cancer), hypertriglyceridemia, pain and infections (https://www.midwestern.edu/departments/marinepharmacology/clinical-pipeline.xml). In addition to those on the market, other marine compounds/extracts showed antiproliferative activities against various cancer cells (131–133). Anticancer is the most frequent activity identified for marine derived compounds, maybe because these molecules have defensive roles in the natural environments. In addition, there is great interest in marine natural products because of their novel chemical structures that often have no other equivalent in terrestrial habitats (~70% of their structural scaffolds are only found in marine organisms).
Most of the marine compounds active against chronic myelogenous leukemia cells derives from sponges. The National Cancer Institute screened about 90,000 extracts of terrestrial and marine plants, and invertebrates on its prescreen platform of 60 human cell lines including the K562 and the HL-60 cell lines (134). Data showed that different phyla, including Annelida, Bryozoa, Chlorophyta, Chordata, Cnidaria, Cyanophyta, Echinodermata, Mollusca, Phaeophyta, Porifera, Rhodophyta and Tracheophyta (Mangrove) and 620/9945 extracts had antileukemia properties. Among the screened organisms, Porifera showed selectivity against leukemia cell lines (134). Regarding the purified compounds from sponges with activity against CML, there are gombamide A, compound 1-8, Lembehyne B, Heteronemin, Smenospongine, Aaptamine, chujamides A and B (135–142).
In particular, gombamide A is a peptide obtained from Clathria gombawuiensis, a native sponge of Korean waters (135). It was proved that gombamide A had cytotoxicity effects against K562 cells with LC50 of 6.9 µM (135). Compound 1-8, extracted from the sponge Coscinoderma sp. of Chuuk Island (Micronesia) (136), showed to be cytotoxic against K562 leukemia cells with LC50 value of 0.9-5.5 µM. Lembehyne B, an acetylenic alcohol obtained from the sponge Haliclona sp. living in the Indonesian waters (137), exhibited cytotoxic properties against K562 cells showing an IC50 of 3 µM (138). In addition, Lembehyne B induced apoptosis and phosphatidylserine externalization on the plasmatic membrane after treatment. Heteronemin is a sesterterpene derivative isolated from the sponge Hippospongia sp (139). which showed strong anticancer activity against K562 cells with EC50 value of 0.41 ± 0.08 mg/mL. Smenospongine is a compound extracted from the marine sponge Dactylospongia elegans which was active against K562 cells and promoted cell cycle arrest when tested at 5-15 µM (140). In addition, Smenospongine was able to block the progression of cell cycle by increasing p21 levels and reducing Rb phosphorilation in K562 cells. Aaptamine is a marine-derived alkaloid isolated from the sponge Aaptos suberitoids (141) and able to inhibit CML K562 cell proliferation with a GI50 of 10 μM. Aaptamine also induced the arrest of cell cycle at G2/M phase and a higher p21 levels, as demonstrated by protein analyses in K562 cells. Finally, the isolation from the sponge Suberites waedoensis of chujamides A and B was reported by Song and co-authors (142). Chujamides A and B are cyclic cysteine bridged peptides, with cytotoxicity toward K562 cells with LC50 values of 37 µM and 55.6 µM, respectively.
Other promising activities derive from marine microalgae, more manageable to grow in small or large photobioreactors compared to sponges and representing an eco-friendly and eco-sustainable source of new compounds from marine environments still poorly explored (130, 133, 143–145). Carbenolide was isolated from the dinoflagellate Amphidinium sp. GA3P and was able to induce apoptosis in K562 cells by inhibiting DNA topoisomerase I and II showing an IC50 of 30 ng/mL (146). Additionally, it was shown that Carbenolide raised lifespan in mice implanted with P-388 leukemia cells (147). Recently, Atasever-Arslan and collaborators (148) tested a serious of microalgae for possible cytotoxicity against both HL60 and K562 cell lines. Stichococcus bacillaris, Phaeodactylum tricornutum, Microcystis aeruginosa and Nannochloropsis oculata extracts were found active against one or both cell lines when screened at 1-500 µg/mL. In particular, S. bacillaris, M. aeruginosa and N. oculata extracts were able to induce apoptosis in K562 cells as demonstrated by Annexin V, PI and DNA fragmentation analyses. S. bacillaris also increased phospho-p38 MAPK protein levels in K562 cells. Atasever-Arslan et al. (148) chemically characterized the composition of oils of these microalgal species by gas chromatography-mass spectrometry (GC-MS) and found 206 compounds. In the active extracts, twelve of these compounds were identified. Hence, these twelve molecules were further analyzed for docking analysis against various key intracellular proteins and results showed that five of them had in silico possible antileukemic activities (e.g. inhibitors of key proteins).
Targeted therapy using TKIs is considered the conventional treatment in CML patients. Some limitations of TKIs use are the high healthcare costs of the drugs, the continuous molecular monitoring of patients for the identification of new mutations and the persistence of LSCs that induce resistance to TKIs. At present, the development of new therapeutic approaches aims at increasing survival, improving quality of life and achieving successful TFR after TKIs discontinuation. BCR-ABL targeted and non BCR-ABL targeted therapies, alone or in combination with conventional inhibitors, constitute a new valid approach to achieve better molecular, haematological or cytogenetic responses and to attempt TFR. Preliminary data in vitro and in vivo results based on the use of oncolytic viruses and engineered exosomes as drugs carrier prove that they could be employed to selectively eradicate CML cells. The ability of antioxidants in combination with TKIs could be a future approach to be employed for CML therapy. Although further investigation is required to better understand the molecular mechanism of these new agents, they represent promising options that could pave the way for new therapeutic approaches for CML patients.
Conceptualization, EA, SD, CLo, CLa and RC; writing original draft preparation, EA and CC; supervision, AG, SM, MQ, CC and RC; project administration, RC and FP; funding acquisition, CC., SM and RC. All authors contributed to the article and approved the submitted version.
This research was supported by: The Italian Ministry of Health progetto di Ricerca Corrente (M4/7) “Identificazione di nuovi approcci per la diagnosi e terapia del mesotelioma pleurico” and grant from Italian Association Leukemia and Lymphoma (A.I.L.) - Caserta - ONLUS “Valentina Picazio”. Human Health Foundation Onlus (http://www.hhfonlus.org).
The authors declare that the research was conducted in the absence of any commercial or financial relationships that could be construed as a potential conflict of interest.
All claims expressed in this article are solely those of the authors and do not necessarily represent those of their affiliated organizations, or those of the publisher, the editors and the reviewers. Any product that may be evaluated in this article, or claim that may be made by its manufacturer, is not guaranteed or endorsed by the publisher.
1. Jabbour E, Kantarjian H. Chronic Myeloid Leukemia: 2020 Update on Diagnosis, Therapy and Monitoring. Am J Hematol (2020) 95:691–709. doi: 10.1002/ajh.25792
2. Höglund M, Sandin F, Simonsson B. Epidemiology of Chronic Myeloid Leukaemia: An Update. Ann Hematol (2015) 94:241–7. doi: 10.1007/s00277-015-2314-2
3. Perrotti D, Jamieson C, Goldman J, Skorski T. Chronic Myeloid Leukemia: Mechanisms of Blastic Transformation. J Clin Invest (2010) 120:2254–64. doi: 10.1172/JCI41246
4. Bonifacio M, Stagno F, Scaffidi L, Krampera M, Di Raimondo F. Management of Chronic Myeloid Leukemia in Advanced Phase. Front Oncol (2019) 9:1132. doi: 10.3389/fonc.2019.01132
5. Loscocco F, Visani G, Galimberti S, Curti A, Isidori A. BCR-ABL Independent Mechanisms of Resistance in Chronic Myeloid Leukemia. Front Oncol (2019) 9:939. doi: 10.3389/fonc.2019.00939
6. Skorski T. Chronic Myeloid Leukemia Cells Refractory/Resistant to Tyrosine Kinase Inhibitors Are Genetically Unstable and may Cause Relapse and Malignant Progression to the Terminal Disease State. Leuk Lymphoma (2011) 52:23–9. doi: 10.3109/10428194.2010.546912
7. Arrigoni E, Del Re M, Galimberti S, Restante G, Rofi E, Crucitta S, et al. Concise Review: Chronic Myeloid Leukemia: Stem Cell Niche and Response to Pharmacologic Treatment. Stem Cells Transl Med (2018) 7:305–14. doi: 10.1002/sctm.17-0175
8. Vener C, Banzi R, Ambrogi F, Ferrero A, Saglio G, Pravettoni G, et al. First-Line Imatinib vs Second- And Third-Generation TKIs for Chronic-Phase CML: A Systematic Review and Meta-Analysis. Blood Adv (2020) 4:2723–35. doi: 10.1182/bloodadvances.2019001329
9. Wilkes JJ, Lyman GH, Doody DR, Chennupati S, Becker LK, Morin PE, et al. Health Care Cost Associated With Contemporary Chronic Myelogenous Leukemia Therapy Compared With That of Other Hematologic Malignancies. JCO Oncol Pract (2021) 17:e406–15. doi: 10.1200/OP.20.00143
10. Abruzzese E, Mauro M, Apperley J, Chelysheva E. Tyrosine Kinase Inhibitors and Pregnancy in Chronic Myeloid Leukemia: Opinion, Evidence, and Recommendations. Ther Adv Hematol (2020) 11:204062072096612. doi: 10.1177/2040620720966120
11. Atallah E, Schiffer CA. Discontinuation of Tyrosine Kinase Inhibitors in Chronic Myeloid Leukemia: When and for Whom? Haematologica (2020) 105:2738–45. doi: 10.3324/haematol.2019.242891
12. Rousselot P, Loiseau C, Delord M, Cayuela JM, Spentchian M. Late Molecular Recurrences in Patients With Chronic Myeloid Leukemia Experiencing Treatment-Free Remission. Blood Adv (2020) 4:3034–40. doi: 10.1182/bloodadvances.2020001772
13. Kumar R, Krause DS. Recent Advances in Understanding Chronic Myeloid Leukemia: Where do We Stand? Fac Rev (2021) 10:35. doi: 10.12703/r/10-35
14. Chandrasekhar C, Kumar PS, Sarma PVGK. Novel Mutations in the Kinase Domain of BCR-ABL Gene Causing Imatinib Resistance in Chronic Myeloid Leukemia Patients. Sci Rep (2019) 9:2045–322. doi: 10.1038/s41598-019-38672-x
15. Roumiantsev S, Shah NP, Gorre ME, Nicoll J, Brasher BB, Sawyers CL, et al. Clinical Resistance to the Kinase Inhibitor STI-571 in Chronic Myeloid Leukemia by Mutation of Tyr-253 in the Abl Kinase Domain P-Loop. Proc Natl Acad Sci (2002) 99:10700–5. doi: 10.1073/pnas.162140299
16. Hochhaus A, Baccarani M, Silver RT, Schiffer C, Apperley JF, Cervantes F, et al. European LeukemiaNet 2020 Recommendations for Treating Chronic Myeloid Leukemia. Leukemia (2020) 34:966–84. doi: 10.1038/s41375-020-0776-2
17. Abruzzese E, Breccia M, Latagliata R. Second-Generation Tyrosine Kinase Inhibitors in First-Line Treatment of Chronic Myeloid Leukaemia (CML). BioDrugs (2014) 28:17–26. doi: 10.1007/s40259-013-0056-z
18. European Medicines Agency. Tasigna. Dublin: EMA (2007). Available at: https://www.ema.europa.eu/en/medicines/human/EPAR/tasigna#authorisation-details-section.
19. Hazarika M, Jiang X, Liu Q, Lee S-L, Ramchandani R, Garnett C, et al. Tasigna for Chronic and Accelerated Phase Philadelphia Chromosome–Positive Chronic Myelogenous Leukemia Resistant to or Intolerant of Imatinib. Clin Cancer Res (2008) 14:5325–31. doi: 10.1158/1078-0432.CCR-08-0308
20. European Medicines Agency. Sprycel. Dublin: EMA (2006). Available at: https://www.ema.europa.eu/en/medicines/human/EPAR/sprycel.
21. U.S. Food and Drug Administration. Drug Approval Package. Sprycel (Dasatinib) Tablets Company: Bristol-Myers Squibb Co (2006). Available at: https://www.accessdata.fda.gov/drugsatfda_docs/nda/2006/021986_022072_SprycelTOC.cfm.
22. Shah NP, Nicoll JM, Nagar B, Gorre ME, Paquette RL, Kuriyan J, et al. Multiple BCR-ABL Kinase Domain Mutations Confer Polyclonal Resistance to the Tyrosine Kinase Inhibitor Imatinib (STI571) in Chronic Phase and Blast Crisis Chronic Myeloid Leukemia. Cancer Cell (2002) 2:117–25. doi: 10.1016/S1535-6108(02)00096-X
23. Branford S, Rudzki Z, Walsh S, Parkinson I, Grigg A, Szer J, et al. Detection of BCR-ABL Mutations in Patients With CML Treated With Imatinib Is Virtually Always Accompanied by Clinical Resistance, and Mutations in the ATP Phosphate-Binding Loop (P-Loop) Are Associated With a Poor Prognosis. Blood (2003) 102:276–83. doi: 10.1182/blood-2002-09-2896
24. Hochhaus A, La Rosée P. Imatinib Therapy in Chronic Myelogenous Leukemia: Strategies to Avoid and Overcome Resistance. Leukemia (2004) 18:1321–31. doi: 10.1038/sj.leu.2403426
25. Waller CF. Imatinib Mesylate. Recent Results Cancer Res (2018) 212:1–27. doi: 10.1007/978-3-319-91439-8_1
26. European Medicines Agency. EMA/436309/2013 EMEA/H/C/000406 Glivec Imatinib. London: EMA (2013). Available at: https://www.ema.europa.eu/en/documents/overview/glivec-epar-summary-public_en.pdf.
27. Hughes T, Saglio G, Branford S, Soverini S, Kim D-W, Müller MC, et al. Impact of Baseline BCR-ABL Mutations on Response to Nilotinib in Patients With Chronic Myeloid Leukemia in Chronic Phase. J Clin Oncol (2009) 27:4204–10. doi: 10.1200/JCO.2009.21.8230
28. García-Gutiérrez V, Hernández-Boluda JC. Tyrosine Kinase Inhibitors Available for Chronic Myeloid Leukemia: Efficacy and Safety. Front Oncol (2019) 9:603. doi: 10.3389/fonc.2019.00603
29. Sacha T, Saglio G. Nilotinib in the Treatment of Chronic Myeloid Leukemia. Futur Oncol (2019) 15:953–65. doi: 10.2217/fon-2018-0468
30. U.S. Food and Drug Administration. Center for Drug Evaluation and Research. FDA (2007). Application Number: 22-068 Labeling Tasigna. Available at: https://www.accessdata.fda.gov/drugsatfda_docs/nda/2007/022068s000_LBL.pdf
31. Lindauer M, Hochhaus A. “Dasatinib”. In: Recent Results in Cancer Research 201:27–65. doi: 10.1007/978-3-642-54490-3_2
32. Hochhaus A, Rosée P, Müller MC, Ernst T, Cross NCP. Impact of BCR-ABL Mutations on Patients With Chronic Myeloid Leukemia. Cell Cycle (2011) 10:250–60. doi: 10.4161/cc.10.2.14537
33. Branford S, Melo JV, Hughes TP. Selecting Optimal Second-Line Tyrosine Kinase Inhibitor Therapy for Chronic Myeloid Leukemia Patients After Imatinib Failure: Does the BCR-ABL Mutation Status Really Matter? Blood (2009) 114:5426–35. doi: 10.1182/blood-2009-08-215939
34. Cortes JE, Kantarjian HM, Brümmendorf TH, Kim D-W, Turkina AG, Shen Z-X, et al. Safety and Efficacy of Bosutinib (SKI-606) in Chronic Phase Philadelphia Chromosome-Positive Chronic Myeloid Leukemia Patients With Resistance or Intolerance to Imatinib. Blood (2011) 118:4567–76. doi: 10.1182/blood-2011-05-355594
35. Gambacorti-Passerini C, Coutre P, Piazza R. The Role of Bosutinib in the Treatment of Chronic Myeloid Leukemia. Futur Oncol (2020) 16:4395–408. doi: 10.2217/fon-2019-0555
36. Food & Drug Administration. Highlights of Prescribing Information. Bosulif (Bosutinib) Tablets, for Oral Use. (2012), Reference ID: 4083804. Available at: https://www.accessdata.fda.gov/drugsatfda_docs/label/2017/20334s007s008lbl.pdf.
37. European Medicines Agency. EMA/178678/2018 EMEA/H/C/002373. Bosulif (Bosutinib) An Overview of Bosulif and Why It Is Authorised in the EU. London: EMA (2018). Available at: https://www.ema.europa.eu/en/documents/overview/bosulif-epar-medicine-overview_en.pdf.
38. Massaro F, Molica M, Breccia M. Ponatinib: A Review of Efficacy and Safety. Curr Cancer Drug Targets (2018) 18:847–56. doi: 10.2174/1568009617666171002142659
39. Hoy SM. Ponatinib: A Review of Its Use in Adults With Chronic Myeloid Leukaemia or Philadelphia Chromosome-Positive Acute Lymphoblastic Leukaemia. Drugs (2014) 74:793–806. doi: 10.1007/s40265-014-0216-6
40. Tan FH, Putoczki TL, Stylli SS, Luwor RB. Ponatinib: A Novel Multi-Tyrosine Kinase Inhibitor Against Human Malignancies. Onco Targets Ther (2019) 12:635–45. doi: 10.2147/OTT.S189391
41. Zabriskie MS, Eide CA, Tantravahi SK, Vellore NA, Estrada J, Nicolini FE, et al. BCR-ABL1 Compound Mutations Combining Key Kinase Domain Positions Confer Clinical Resistance to Ponatinib in Ph Chromosome-Positive Leukemia. Cancer Cell (2014) 26:428–42. doi: 10.1016/j.ccr.2014.07.006
42. Food & Drug Administration. Highlights of Prescribing Information. Iclusig (Ponatinib) Tablets for Oral Use. (2016), Reference ID: 4019587. Available at: https://www.accessdata.fda.gov/drugsatfda_docs/label/2016/203469s022lbl.pdf.
43. Ciarcia R, Damiano S, Puzio MV, Montagnaro S, Pagnini F, Pacilio C, et al. Comparison of Dasatinib, Nilotinib, and Imatinib in the Treatment of Chronic Myeloid Leukemia. J Cell Physiol (2016) 231:680–7. doi: 10.1002/jcp.25118
44. Kantarjian H, Shah NP, Hochhaus A, Cortes J, Shah S, Ayala M, et al. Dasatinib Versus Imatinib in Newly Diagnosed Chronic-Phase Chronic Myeloid Leukemia. N Engl J Med (2010) 362:2260–70. doi: 10.1056/NEJMoa1002315
45. Saglio G, Kim D-W, Issaragrisil S, le Coutre P, Etienne G, Lobo C, et al. Nilotinib Versus Imatinib for Newly Diagnosed Chronic Myeloid Leukemia. N Engl J Med (2010) 362:2251–9. doi: 10.1056/NEJMoa0912614
46. Cortes JE, Gambacorti-Passerini C, Deininger MW, Mauro MJ, Chuah C, Kim D-W, et al. Bosutinib Versus Imatinib for Newly Diagnosed Chronic Myeloid Leukemia: Results From the Randomized BFORE Trial. J Clin Oncol (2018) 36:231–7. doi: 10.1200/JCO.2017.74.7162
47. Cortes JE, Jimenez CA, Mauro MJ, Geyer A, Pinilla-Ibarz J, Smith BD. Pleural Effusion in Dasatinib-Treated Patients With Chronic Myeloid Leukemia in Chronic Phase: Identification and Management. Clin Lymphoma Myeloma Leuk (2017) 17:78–82. doi: 10.1016/j.clml.2016.09.012
48. Gora-Tybor J, Medras E, Calbecka M, Kolkowska-Leśniak A, Ponikowska-Szyba E, Robak T, et al. Real-Life Comparison of Severe Vascular Events and Other Non-Hematological Complications in Patients With Chronic Myeloid Leukemia Undergoing Second-Line Nilotinib or Dasatinib Treatment. Leuk Lymphoma (2015) 56:2309–14. doi: 10.3109/10428194.2014.994205
49. Eskazan AE, Keskin D. Radotinib and Its Clinical Potential in Chronic-Phase Chronic Myeloid Leukemia Patients: An Update. Ther Adv Hematol (2017) 8:237–43. doi: 10.1177/2040620717719851
50. Kim S-H, Menon H, Jootar S, Saikia T, Kwak J-Y, Sohn S-K, et al. Efficacy and Safety of Radotinib in Chronic Phase Chronic Myeloid Leukemia Patients With Resistance or Intolerance to BCR-ABL1 Tyrosine Kinase Inhibitors. Haematologica (2014) 99:1191–6. doi: 10.3324/haematol.2013.096776
51. Kwak J-Y, Kim S-H, Oh SJ, Zang DY, Kim H, Kim J-A, et al. Phase III Clinical Trial (RERISE Study) Results of Efficacy and Safety of Radotinib Compared With Imatinib in Newly Diagnosed Chronic Phase Chronic Myeloid Leukemia. Clin Cancer Res (2017) 23:7180–8. doi: 10.1158/1078-0432.CCR-17-0957
52. O’Hare T, Shakespeare WC, Zhu X, Eide CA, Rivera VM, Wang F, et al. AP24534, a Pan-BCR-ABL Inhibitor for Chronic Myeloid Leukemia, Potently Inhibits the T315I Mutant and Overcomes Mutation-Based Resistance. Cancer Cell (2009) 16:401–12. doi: 10.1016/j.ccr.2009.09.028
53. Cortes JE, Kim D-W, Pinilla-Ibarz J, le Coutre PD, Paquette R, Chuah C, et al. Ponatinib Efficacy and Safety in Philadelphia Chromosome-Positive Leukemia: Final 5-Year Results of the Phase 2 PACE Trial. Blood (2018) 132:393–404. doi: 10.1182/blood-2016-09-739086
54. Jain P, Kantarjian H, Boddu PC, Nogueras-González GM, Verstovsek S, Garcia-Manero G, et al. Analysis of Cardiovascular and Arteriothrombotic Adverse Events in Chronic-Phase CML Patients After Frontline TKIs. Blood Adv (2019) 3:851–61. doi: 10.1182/bloodadvances.2018025874
55. Iurlo A, Cattaneo D, Orofino N, Bucelli C, Molica M, Breccia M. Low-Dose Ponatinib in Intolerant Chronic Myeloid Leukemia Patients: A Safe and Effective Option. Clin Drug Investig (2018) 38:475–6. doi: 10.1007/s40261-018-0623-7
56. Tefferi A. Upfront Low-Dose Ponatinib (15 Mg/Day) for Multi-TKI Resistant Chronic Myeloid Leukemia. Hematol Oncol (2018) 36:718–20. doi: 10.1002/hon.2517
57. Özgür Yurttaş N, Eşkazan AE. Novel Therapeutic Approaches in Chronic Myeloid Leukemia. Leuk Res (2020) 91:106337. doi: 10.1016/j.leukres.2020.106337
58. Malik S, Hassan S, Eşkazan AE. Novel BCR-ABL1 Tyrosine Kinase Inhibitors in the Treatment of Chronic Myeloid Leukemia. Expert Rev Hematol (2021) 14:1–4. doi: 10.1080/17474086.2021.1990034
59. Wylie AA, Schoepfer J, Jahnke W, Cowan-Jacob SW, Loo A, Furet P, et al. The Allosteric Inhibitor ABL001 Enables Dual Targeting of BCR–Abl1. Nature (2017) 543:733–7. doi: 10.1038/nature21702
60. Ottmann OG, Alimena G, DeAngelo DJ, Goh Y-T, Heinrich MC, Hochhaus A, et al. ABL001, a Potent, Allosteric Inhibitor of BCR-ABL, Exhibits Safety and Promising Single- Agent Activity in a Phase I Study of Patients With CML With Failure of Prior TKI Therapy. Blood (2015) 126:138–8. doi: 10.1182/blood.V126.23.138.138
61. Hughes TP, Mauro MJ, Cortes JE, Minami H, Rea D, DeAngelo DJ, et al. Asciminib in Chronic Myeloid Leukemia After ABL Kinase Inhibitor Failure. N Engl J Med (2019) 381:2315–26. doi: 10.1056/NEJMoa1902328
62. Rea D, Mauro MJ, Boquimpani C, Minami Y, Lomaia E, Voloshin S, et al. A Phase 3, Open-Label, Randomized Study of Asciminib, a STAMP Inhibitor, vs Bosutinib in CML After ≥2 Prior TKIs. Blood (2021). doi: 10.1182/blood.2020009984
63. U.S. Food and Drug Administration. FDA Approves Asciminib for Philadelphia Chromosome-Positive Chronic Myeloid Leukemia. FDA (2021). Available at: https://www.fda.gov/drugs/resources-information-approved-drugs/fda-approves-asciminib-philadelphia-chromosome-positive-chronic-myeloid-leukemia.
64. Jiang Q, Huang X, Chen Z, Niu Q, Men L, Wang H, et al. An Updated Safety and Efficacy Results of Phase 1 Study of HQP1351, A Novel 3rd Generation of BCR-ABL Tyrosine Kinase Inhibitor (TKI), in Patients With TKI Resistant Chronic Myeloid Leukemia. Blood (2019) 134:493–3. doi: 10.1182/blood-2019-124295
65. Jiang Q, Huang X, Chen Z, Niu Q, Shi D, Li Z, et al. Novel BCR-ABL1 Tyrosine Kinase Inhibitor (TKI) HQP1351 (Olverembatinib) Is Efficacious and Well Tolerated in Patients With T315I-Mutated Chronic Myeloid Leukemia (CML): Results of Pivotal (Phase II) Trials. Blood (2020) 136:50–1. doi: 10.1182/blood-2020-142142
66. Antelope O, Vellore NA, Pomicter AD, Patel AB, Van Scoyk A, Clair PM, et al. BCR-ABL1 Tyrosine Kinase Inhibitor K0706 Exhibits Preclinical Activity in Philadelphia Chromosome-Positive Leukemia. Exp Hematol (2019) 77:36–40.e2. doi: 10.1016/j.exphem.2019.08.007
67. Cortes JE, Kim D-W, Nicolini FE, Saikia T, Charbonnier A, Apperley JF, et al. Phase 1 Trial of K0706, a Novel Oral BCR-ABL1 Tyrosine Kinase Inhibitor (TKI): In Patients With Chronic Myelogenous Leukemia (CML) and Phildelphia Positive Acute Lymphoblastic Leukemia (Ph+ ALL) Failing ≥ 3 Prior TKI Therapies: Initial Safety and Efficacy. Blood (2019) 134:4158–8. doi: 10.1182/blood-2019-129751
68. Cortes J, Lang F. Third-Line Therapy for Chronic Myeloid Leukemia: Current Status and Future Directions. J Hematol Oncol (2021) 14:44. doi: 10.1186/s13045-021-01055-9
69. Turkina AG, Vinogradova O, Lomaia E, Shatokhina E, Shukhov O, Chelysheva E, et al. Phase-1 Study of PF-114 Mesylate in CML Failing Prior Tyrosine Kinase-Inhibitor Therapy. Blood (2018) 132:790. doi: 10.1182/blood-2018-99-116803
70. Turkina AG, Vinogradova O, Lomaia E, Shatokhina E, Shukhov OA, Chelysheva EY, et al. PF-114: A 4th Generation Tyrosine Kinase-Inhibitor for Chronic Phase Chronic Myeloid Leukaemia Including BCRABL1T315I. Blood (2019) 134:1638–8. doi: 10.1182/blood-2019-127951
71. Appels NMGM, Beijnen JH, Schellens JHM. Development of Farnesyl Transferase Inhibitors: A Review. Oncologist (2005) 10:565–78. doi: 10.1634/theoncologist.10-8-565
72. Ahmed W, Van Etten RA. Signal Transduction in the Chronic Leukemias: Implications for Targeted Therapies. Curr Hematol Malig Rep (2013) 8:71–80. doi: 10.1007/s11899-012-0150-1
73. Cortes J, Albitar M, Thomas D, Giles F, Kurzrock R, Thibault A, et al. Efficacy of the Farnesyl Transferase Inhibitor R115777 in Chronic Myeloid Leukemia and Other Hematologic Malignancies. Blood (2003) 101:1692–7. doi: 10.1182/blood-2002-07-1973
74. Cortes J, Quintás-Cardama A, Garcia-Manero G, O’Brien S, Jones D, Faderl S, et al. Phase 1 Study of Tipifarnib in Combination With Imatinib for Patients With Chronic Myelogenous Leukemia in Chronic Phase After Imatinib Failure. Cancer (2007) 110:2000–6. doi: 10.1002/cncr.23006
75. Borthakur G, Kantarjian H, Daley G, Talpaz M, O’Brien S, Garcia-Manero G, et al. Pilot Study of Lonafarnib, a Farnesyl Transferase Inhibitor, in Patients With Chronic Myeloid Leukemia in the Chronic or Accelerated Phase That Is Resistant or Refractory to Imatinib Therapy. Cancer (2006) 106:346–52. doi: 10.1002/cncr.21590
76. Cortes J, Jabbour E, Daley GQ, O’Brien S, Verstovsek S, Ferrajoli A, et al. Phase 1 Study of Lonafarnib (SCH 66336) and Imatinib Mesylate in Patients With Chronic Myeloid Leukemia Who Have Failed Prior Single-Agent Therapy With Imatinib. Cancer (2007) 110:1295–302. doi: 10.1002/cncr.22901
77. Kiu H, Nicholson SE. Biology and Significance of the JAK/STAT Signalling Pathways. Growth Factors (2012) 30:88–106. doi: 10.3109/08977194.2012.660936
78. Valent P. Targeting the JAK2-STAT5 Pathway in CML. Blood (2014) 124:1386–8. doi: 10.1182/blood-2014-07-585943
79. Sweet K, Hazlehurst L, Sahakian E, Powers J, Nodzon L, Kayali F, et al. A Phase I Clinical Trial of Ruxolitinib in Combination With Nilotinib in Chronic Myeloid Leukemia Patients With Molecular Evidence of Disease. Leuk Res (2018) 74:89–96. doi: 10.1016/j.leukres.2018.10.002
80. Zhang B, Strauss AC, Chu S, Li M, Ho Y, Shiang K-D, et al. Effective Targeting of Quiescent Chronic Myelogenous Leukemia Stem Cells by Histone Deacetylase Inhibitors in Combination With Imatinib Mesylate. Cancer Cell (2010) 17:427–42. doi: 10.1016/j.ccr.2010.03.011
81. Zaritskey A, Alimena G, Konopka L, Shamsazar J, Bourquelot PM, Jalaluddin M, et al. A Phase II Study of Oral Panobinostat (LBH589) for Chronic Phase Chronic Myeloid Leukemia (CML) With Resistance to ≥2 BCR-ABL Tyrosine Kinase Inhibitors. Blood (2008) 112:4254–4. doi: 10.1182/blood.V112.11.4254.4254
82. Massimino M, Stella S, Tirrò E, Romano C, Pennisi MS, Puma A, et al. Non ABL-Directed Inhibitors as Alternative Treatment Strategies for Chronic Myeloid Leukemia. Mol Cancer (2018) 17:56. doi: 10.1186/s12943-018-0805-1
83. Seymour JF, Kim DW, Rubin E, Haregewoin A, Clark J, Watson P, et al. A Phase 2 Study of MK-0457 in Patients With BCR-ABL T315I Mutant Chronic Myelogenous Leukemia and Philadelphia Chromosome-Positive Acute Lymphoblastic Leukemia. Blood Cancer J (2014) 4:e238–8. doi: 10.1038/bcj.2014.60
84. Borthakur G, Dombret H, Schafhausen P, Brummendorf TH, Boissel N, Jabbour E, et al. A Phase I Study of Danusertib (PHA-739358) in Adult Patients With Accelerated or Blastic Phase Chronic Myeloid Leukemia and Philadelphia Chromosome-Positive Acute Lymphoblastic Leukemia Resistant or Intolerant to Imatinib and/or Other Second Generation C-. Haematologica (2015) 100:898–904. doi: 10.3324/haematol.2014.115279
85. Rousselot P, Prost S, Guilhot J, Roy L, Etienne G, Legros L, et al. Pioglitazone Together With Imatinib in Chronic Myeloid Leukemia: A Proof of Concept Study. Cancer (2017) 123:1791–9. doi: 10.1002/cncr.30490
86. Florean C, Schnekenburger M, Grandjenette C, Dicato M, Diederich M. Epigenomics of Leukemia: From Mechanisms to Therapeutic Applications. Epigenomics (2011) 3:581–609. doi: 10.2217/epi.11.73
87. Abaza Y, Kantarjian H, Alwash Y, Borthakur G, Champlin R, Kadia T, et al. Phase I/II Study of Dasatinib in Combination With Decitabine in Patients With Accelerated or Blast Phase Chronic Myeloid Leukemia. Am J Hematol (2020) 95:1288–95. doi: 10.1002/ajh.25939
88. Kantarjian HM, O’Brien S, Cortes J, Giles FJ, Faderl S, Issa J-P, et al. Results of Decitabine (5-Aza-2?Deoxycytidine) Therapy in 130 Patients With Chronic Myelogenous Leukemia. Cancer (2003) 98:522–8. doi: 10.1002/cncr.11543
89. Jonas BA, Pollyea DA. How We Use Venetoclax With Hypomethylating Agents for the Treatment of Newly Diagnosed Patients With Acute Myeloid Leukemia. Leukemia (2019) 33:2795–804. doi: 10.1038/s41375-019-0612-8
90. Carter BZ, Mak PY, Mu H, Zhou H, Mak DH, Schober W, et al. Combined Targeting of BCL-2 and BCR-ABL Tyrosine Kinase Eradicates Chronic Myeloid Leukemia Stem Cells. Sci Transl Med (2016) 8(355):355ra117. doi: 10.1126/scitranslmed.aag1180
91. Maiti A, Franquiz MJ, Ravandi F, Cortes JE, Jabbour EJ, Sasaki K, et al. Venetoclax and BCR-ABL Tyrosine Kinase Inhibitor Combinations: Outcome in Patients With Philadelphia Chromosome-Positive Advanced Myeloid Leukemias. Acta Haematol (2020) 143:567–73. doi: 10.1159/000506346
92. Cortes JE, Kantarjian HM, Rea D, Wetzler M, Lipton JH, Akard L, et al. Final Analysis of the Efficacy and Safety of Omacetaxine Mepesuccinate in Patients With Chronic- or Accelerated-Phase Chronic Myeloid Leukemia: Results With 24 Months of Follow-Up. Cancer (2015) 121:1637–44. doi: 10.1002/cncr.29240
93. Warsch W, Grundschober E, Sexl V. Adding a New Facet to STAT5 in CML: Multitasking for Leukemic Cells. Cell Cycle (2013) 12:1813–4. doi: 10.4161/cc.25116
94. Popp HD, Kohl V, Naumann N, Flach J, Brendel S, Kleiner H, et al. DNA Damage and DNA Damage Response in Chronic Myeloid Leukemia. Int J Mol Sci (2020) 21:1177. doi: 10.3390/ijms21041177
95. Ciarcia R, D’Angelo D, Pacilio C, Pagnini D, Galdiero M, Fiorito F, et al. Dysregulated Calcium Homeostasis and Oxidative Stress in Chronic Myeloid Leukemia (CML) Cells. J Cell Physiol (2010) 224:443–53. doi: 10.1002/jcp.22140
96. Singh RK, Tripathi AK, Tripathi P, Singh S, Singh R, Ahmad R. Studies on Biomarkers for Oxidative Stress in Patients With Chronic Myeloid Leukemia. Hematol Oncol Stem Cell Ther (2009) 2:285–8. doi: 10.1016/S1658-3876(09)50039-8
97. Ahmad R, Tripathi AK, Tripathi P, Singh R, Singh S, Singh RK. Oxidative Stress and Antioxidant Status in Patients With Chronic Myeloid Leukemia. Indian J Clin Biochem (2008) 23:328–33. doi: 10.1007/s12291-008-0072-9
98. Nieborowska-Skorska M, Hoser G, Hochhaus A, Stoklosa T, Skorski T. Anti-Oxidant Vitamin E Prevents Accumulation of Imatinib-Resistant BCR-ABL1 Kinase Mutations in CML-CP Xenografts in NSG Mice. Leukemia (2013) 27:2253–4. doi: 10.1038/leu.2013.123
99. Ghalaut VS, Sangwan L, Dahiya K, Ghalaut P, Dhankhar R, Saharan R. Effect of Imatinib Therapy With and Without Turmeric Powder on Nitric Oxide Levels in Chronic Myeloid Leukemia. J Oncol Pharm Pract (2012) 18:186–90. doi: 10.1177/1078155211416530
100. Martínez-Castillo M, Villegas-Sepúlveda N, Meraz-Rios MA, Hernández-Zavala A, Berumen J, Coleman MA, et al. Curcumin Differentially Affects Cell Cycle and Cell Death in Acute and Chronic Myeloid Leukemia Cells. Oncol Lett (2018) 15:6777–83. doi: 10.3892/ol.2018.8112
101. Taverna S, Giallombardo M, Pucci M, Flugy A, Manno M, Raccosta S, et al. Curcumin Inhibits In Vitro and In Vivo Chronic Myelogenous Leukemia Cells Growth: A Possible Role for Exosomal Disposal of miR-21. Oncotarget (2015) 6:21918–33. doi: 10.18632/oncotarget.4204
102. Wu L, Yu J, Chen R, Liu Y, Lou L, Wu Y, et al. Dual Inhibition of Bcr-Abl and Hsp90 by C086 Potently Inhibits the Proliferation of Imatinib-Resistant CML Cells. Clin Cancer Res (2015) 21:833–43. doi: 10.1158/1078-0432.CCR-13-3317
103. Wu L, Wu Y, Chen R, Liu Y, Huang L, Lou L, et al. Curcumin Derivative C817 Inhibits Proliferation of Imatinib-Resistant Chronic Myeloid Leukemia Cells With Wild-Type or Mutant Bcr-Abl In Vitro. Acta Pharmacol Sin (2014) 35:401–9. doi: 10.1038/aps.2013.180
104. Tong L, Chuang C-C, Wu S, Zuo L. Reactive Oxygen Species in Redox Cancer Therapy. Cancer Lett (2015) 367:18–25. doi: 10.1016/j.canlet.2015.07.008
105. de la Lastra CA, Villegas I. Resveratrol as an Antioxidant and Pro-Oxidant Agent: Mechanisms and Clinical Implications. Biochem Soc Trans (2007) 35:1156–60. doi: 10.1042/BST0351156
106. Chan W-H. Effect of Resveratrol on High Glucose-Induced Stress in Human Leukemia K562 Cells. J Cell Biochem (2005) 94:1267–79. doi: 10.1002/jcb.20408
107. Wu X, Xiong M, Xu C, Duan L, Dong Y, Luo Y, et al. Resveratrol Induces Apoptosis of Human Chronic Myelogenous Leukemia Cells In Vitro Through P38 and JNK-Regulated H2AX Phosphorylation. Acta Pharmacol Sin (2015) 36:353–61. doi: 10.1038/aps.2014.132
108. Damiano S, Montagnaro S, Puzio MV, Severino L, Pagnini U, Barbarino M, et al. Effects of Antioxidants on Apoptosis Induced by Dasatinib and Nilotinib in K562 Cells. J Cell Biochem (2018) 119:4845–54. doi: 10.1002/jcb.26686
109. Mavani HJ, Wick JY. Oncology’s Trojan Horse: Using Viruses to Battle Cancer. Consult Pharm (2016) 31:676–84. doi: 10.4140/TCP.n.2016.676
110. Mondal M, Guo J, He P, Zhou D. Recent Advances of Oncolytic Virus in Cancer Therapy. Hum Vaccin Immunother (2020) 16:2389–402. doi: 10.1080/21645515.2020.1723363
111. Müller L, Berkeley R, Barr T, Ilett E, Errington-Mais F. Past, Present and Future of Oncolytic Reovirus. Cancers (Basel) (2020) 12:3219. doi: 10.3390/cancers12113219
112. Rosewell Shaw A, Suzuki M. Recent Advances in Oncolytic Adenovirus Therapies for Cancer. Curr Opin Virol (2016) 21:9–15. doi: 10.1016/j.coviro.2016.06.009
113. Marchini A, Bonifati S, Scott EM, Angelova AL, Rommelaere J. Oncolytic Parvoviruses: From Basic Virology to Clinical Applications. Virol J (2015) 12:6. doi: 10.1186/s12985-014-0223-y
114. Shen Y, Nemunaitis J. Herpes Simplex Virus 1 (HSV-1) for Cancer Treatment. Cancer Gene Ther (2006) 13:975–92. doi: 10.1038/sj.cgt.7700946
115. Kirn D, Martuza RL, Zwiebel J. Replication-Selective Virotherapy for Cancer: Biological Principles, Risk Management and Future Directions. Nat Med (2001) 7:781–7. doi: 10.1038/89901
116. Montagnaro S, Damiano S, Ciarcia R, Puzio MV, Ferrara G, Iovane V, et al. Caprine Herpesvirus 1 (CpHV-1) as a Potential Candidate for Oncolytic Virotherapy. Cancer Biol Ther (2019) 20:42–51. doi: 10.1080/15384047.2018.1504722
117. Yurchenko KS, Zhou P, Kovner AV, Zavjalov EL, Shestopalova LV, Shestopalov AM. Oncolytic Effect of Wild-Type Newcastle Disease Virus Isolates in Cancer Cell Lines In Vitro and In Vivo on Xenograft Model. PLoS One (2018) 13:e0195425. doi: 10.1371/journal.pone.0195425
118. Rodrigues R, Cuddington B, Mossman K. Bovine Herpesvirus Type 1 as a Novel Oncolytic Virus. Cancer Gene Ther (2010) 17:344–55. doi: 10.1038/cgt.2009.77
119. Cody JJ, Douglas JT. Armed Replicating Adenoviruses for Cancer Virotherapy. Cancer Gene Ther (2009) 16:473–88. doi: 10.1038/cgt.2009.3
120. Innao V, Rizzo V, Allegra AG, Musolino C, Allegra A. Oncolytic Viruses and Hematological Malignancies: A New Class of Immunotherapy Drugs. Curr Oncol (2020) 28:159–83. doi: 10.3390/curroncol28010019
121. Tong Y, You L, Liu H, Li L, Meng H, Qian Q, et al. Potent Antitumor Activity of Oncolytic Adenovirus Expressing Beclin-1 via Induction of Autophagic Cell Death in Leukemia. Oncotarget (2013) 4:860–74. doi: 10.18632/oncotarget.1018
122. Li L, You L-S, Mao L-P, Jin S-H, Chen X-H, Qian W-B. Combing Oncolytic Adenovirus Expressing Beclin-1 With Chemotherapy Agent Doxorubicin Synergistically Enhances Cytotoxicity in Human CML Cells In Vitro. Acta Pharmacol Sin (2018) 39:251–60. doi: 10.1038/aps.2017.100
123. Raposo G, Stoorvogel W. Extracellular Vesicles: Exosomes, Microvesicles, and Friends. J Cell Biol (2013) 200:373–83. doi: 10.1083/jcb.201211138
124. Kang K-W, Jung J-H, Hur W, Park J, Shin H, Choi B, et al. The Potential of Exosomes Derived From Chronic Myelogenous Leukaemia Cells as a Biomarker. Anticancer Res (2018) 38:3935–42. doi: 10.21873/anticanres.12679
125. Asano M, Umezu T, Katagiri S, Kobayashi C, Tauchi T, Gotoh M, et al. Up-Regulated Exosomal miRNA-140-3p in CML Patients With Musculoskeletal Pain Associated With Discontinuation of Tyrosine Kinase Inhibitors. Int J Hematol (2017) 105:419–22. doi: 10.1007/s12185-017-2199-z
126. Bellavia D, Raimondo S, Calabrese G, Forte S, Cristaldi M, Patinella A, et al. Interleukin 3- Receptor Targeted Exosomes Inhibit In Vitro and In Vivo Chronic Myelogenous Leukemia Cell Growth. Theranostics (2017) 7:1333–45. doi: 10.7150/thno.17092
127. Blunt JW, Carroll AR, Copp BR, Davis RA, Keyzers RA, Prinsep MR. Marine Natural Products. Nat Prod Rep (2018) 35:8–53. doi: 10.1039/c7np00052a
128. Jaspars M, De Pascale D, Andersen JH, Reyes F, Crawford AD, Ianora A. The Marine Biodiscovery Pipeline and Ocean Medicines of Tomorrow. J Mar Biol Assoc United Kingdom (2016) 96:151–8. doi: 10.1017/S0025315415002106
129. Romano G, Costantini M, Sansone C, Lauritano C, Ruocco N, Ianora A. Marine Microorganisms as a Promising and Sustainable Source of Bioactive Molecules. Mar Environ Res (2017) 128:58–69. doi: 10.1016/j.marenvres.2016.05.002
130. Saide A, Martínez KA, Ianora A, Lauritano C. Unlocking the Health Potential of Microalgae as Sustainable Sources of Bioactive Compounds. Int J Mol Sci (2021) 22:4383. doi: 10.3390/ijms22094383
131. Riccio G, Nuzzo G, Zazo G, Coppola D, Senese G, Romano L, et al. Bioactivity Screening of Antarctic Sponges Reveals Anticancer Activity and Potential Cell Death via Ferroptosis by Mycalols. Mar Drugs (2021) 19:459. doi: 10.3390/md19080459
132. Lauritano C, Martínez KA, Battaglia P, Granata A, de la Cruz M, Cautain B, et al. First Evidence of Anticancer and Antimicrobial Activity in Mediterranean Mesopelagic Species. Sci Rep (2020) 10:4929. doi: 10.1038/s41598-020-61515-z
133. Martínez Andrade K, Lauritano C, Romano G, Ianora A. Marine Microalgae With Anti-Cancer Properties. Mar Drugs (2018) 16:165. doi: 10.3390/md16050165
134. Cragg GM, Newman DJ, Yang SS. Natural Product Extracts of Plant and Marine Origin Having Antileukemia Potential. The NCI Experience. J Nat Prod (2006) 69:488–98. doi: 10.1021/np0581216
135. Woo J-K, Jeon J, Kim C-K, Sim CJ, Oh D-C, Oh K-B, et al. Gombamide A, a Cyclic Thiopeptide From the Sponge Clathria Gombawuiensis. J Nat Prod (2013) 76:1380–3. doi: 10.1021/np4003367
136. Kim C-K, Song I-H, Park HY, Lee Y-J, Lee H-S, Sim CJ, et al. Suvanine Sesterterpenes and Deacyl Irciniasulfonic Acids From a Tropical Coscinoderma Sp. Sponge. J Nat Prod (2014) 77:1396–403. doi: 10.1021/np500156n
137. Aoki S, Matsui K, Wei H, Murakami N, Kobayashi M. Structure–activity Relationship of Neuritogenic Spongean Acetylene Alcohols, Lembehynes. Tetrahedron (2002) 58:5417–22. doi: 10.1016/S0040-4020(02)00519-7
138. Dzhemileva LU, D’yakonov VA, Makarov AA, Andreev EN, Yunusbaeva MM, Dzhemilev UM. The First Total Synthesis of the Marine Acetylenic Alcohol, Lembehyne B – A Selective Inducer of Early Apoptosis in Leukemia Cancer Cells. Org Biomol Chem (2017) 15:470–6. doi: 10.1039/C6OB02346K
139. Chen Y-C, Lu M-C, El-Shazly M, Lai K-H, Wu T-Y, Hsu Y-M, et al. Breaking Down Leukemia Walls: Heteronemin, a Sesterterpene Derivative, Induces Apoptosis in Leukemia Molt4 Cells Through Oxidative Stress, Mitochondrial Dysfunction and Induction of Talin Expression. Mar Drugs (2018) 16:212. doi: 10.3390/md16060212
140. Kong D, Aoki S, Sowa Y, Sakai T, Kobayashi M. Smenospongine, a Sesquiterpene Aminoquinone From a Marine Sponge, Induces G1 Arrest or Apoptosis in Different Leukemia Cells. Mar Drugs (2008) 6:480–8. doi: 10.3390/md6030480
141. Jin M, Zhao W, Zhang Y, Kobayashi M, Duan H, Kong D. Antiproliferative Effect of Aaptamine on Human Chronic Myeloid Leukemia K562 Cells. Int J Mol Sci (2011) 12:7352–9. doi: 10.3390/ijms12117352
142. Song J, Jeon J, Won T, Sim C, Oh D-C, Oh K-B, et al. New Cyclic Cystine Bridged Peptides From the Sponge Suberites Waedoensis. Mar Drugs (2014) 12:2760–70. doi: 10.3390/md12052760
143. Lauritano C, Ianora A. Grand Challenges in Marine Biotechnology: Overview of Recent EU-Funded Projects. In: Grand Challenges in Biology and Biotechnology. Cham: Springer (2018). doi: 10.1007/978-3-319-69075-9_11
144. Brillatz T, Lauritano C, Jacmin M, Khamma S, Marcourt L, Righi D, et al. Zebrafish-Based Identification of the Antiseizure Nucleoside Inosine From the Marine Diatom Skeletonema Marinoi. PLoS One (2018) 13:e0196195. doi: 10.1371/journal.pone.0196195
145. Saide A, Lauritano C, Ianora A. Pheophorbide a: State of the Art. Mar Drugs (2020) 18:257. doi: 10.3390/md18050257
146. Folmer F, Jaspars M, Dicato M, Diederich M. Photosynthetic Marine Organisms as a Source of Anticancer Compounds. Phytochem Rev (2010) 9:557–79. doi: 10.1007/s11101-010-9200-2
147. Kerr RG, Kerr SS. Marine Natural Products as Therapeutic Agents. Expert Opin Ther Pat (1999) 9:1207–22. doi: 10.1517/13543776.9.9.1207
148. Atasever-Arslan B, Yilancioglu K, Kalkan Z, Timucin AC, Gür H, Isik FB, et al. Screening of New Antileukemic Agents From Essential Oils of Algae Extracts and Computational Modeling of Their Interactions With Intracellular Signaling Nodes. Eur J Pharm Sci (2016) 83:120–31. doi: 10.1016/j.ejps.2015.12.001
Keywords: chronic myeloid leukemia, tyrosine kinase inhibitors, quiescent leukemia stem cells, Non-BCR-ABL targeted drugs, oncolytic therapy, antioxidants, exosomes, marine organisms
Citation: Andretta E, Costa C, Longobardi C, Damiano S, Giordano A, Pagnini F, Montagnaro S, Quintiliani M, Lauritano C and Ciarcia R (2021) Potential Approaches Versus Approved or Developing Chronic Myeloid Leukemia Therapy. Front. Oncol. 11:801779. doi: 10.3389/fonc.2021.801779
Received: 25 October 2021; Accepted: 12 November 2021;
Published: 15 December 2021.
Edited by:
Thomas Ollila, Warren Alpert Medical School of Brown University, United StatesReviewed by:
Ahmet Emre Eskazan, Istanbul University-Cerrahpasa, TurkeyCopyright © 2021 Andretta, Costa, Longobardi, Damiano, Giordano, Pagnini, Montagnaro, Quintiliani, Lauritano and Ciarcia. This is an open-access article distributed under the terms of the Creative Commons Attribution License (CC BY). The use, distribution or reproduction in other forums is permitted, provided the original author(s) and the copyright owner(s) are credited and that the original publication in this journal is cited, in accordance with accepted academic practice. No use, distribution or reproduction is permitted which does not comply with these terms.
*Correspondence: Caterina Costa, Yy5jb3N0YUBpc3RpdHV0b3R1bW9yaS5uYS5pdA==; Roberto Ciarcia, cmNpYXJjaWFAdW5pbmEuaXQ=
†These authors share first authorship
‡These authors share last authorship
Disclaimer: All claims expressed in this article are solely those of the authors and do not necessarily represent those of their affiliated organizations, or those of the publisher, the editors and the reviewers. Any product that may be evaluated in this article or claim that may be made by its manufacturer is not guaranteed or endorsed by the publisher.
Research integrity at Frontiers
Learn more about the work of our research integrity team to safeguard the quality of each article we publish.