- 1Division of Medical Oncology, National Cancer Centre Singapore, Singapore, Singapore
- 2Duke-NUS Medical School, National University of Singapore, Singapore, Singapore
- 3Department of Radiation Oncology, University of Alabama at Birmingham School of Medicine, Birmingham, AL, United States
- 4Center for Neuro-Oncology, Dana-Farber Cancer Institute, Boston, MA, United States
- 5Department of Radiation Oncology, University of Michigan, Ann Arbor, MI, United States
- 6Department of Radiation Oncology, Miami Cancer Institute, Baptist Health South Florida, Miami, FL, United States
- 7Duke Cancer Institute, Duke University, Durham, NC, United States
Brain metastases cause significant morbidity and mortality in patients with advanced cancer. In the era of precision oncology and immunotherapy, there are rapidly evolving systemic treatment options. These novel therapies may have variable intracranial efficacy, and patients with brain metastases remain a population of special interest. Typically, only patients with stable, asymptomatic and/or treated brain metastases are enrolled in clinical trials, or may be excluded altogether, particularly in the setting of leptomeningeal carcinomatosis. Consequently, this leads to significant concerns on the external validity of clinical trial evidence to real-world clinical practice. Here we describe the current trends in cancer clinical trial eligibility for patients with brain metastases in both early and late phase trials, with a focus on targeted and immunotherapies. We evaluate recent newly FDA approved therapies and the clinical trial evidence base leading to approval. This includes analysis of inclusion and exclusion criteria, requirements for baseline screening for brain metastases, surveillance cerebral imaging and incorporation of trial endpoints for patients with brain metastases. Finally, the use of alternative sources of data such as real-world evidence with registries and collaborative studies will be discussed.
Introduction
Brain or central nervous system (CNS) metastases remain a significant cause of morbidity and mortality in patients with advanced cancers (1). The incidence of brain metastases may be increasing, in part due to greater detection through routine cerebral imaging and more effective systemic therapies allowing later manifestations of the disease to occur (2). Particularly in the era of precision oncology and immunotherapy, there are rapidly evolving systemic treatment options for many cancers. These novel therapies may have variable intracranial efficacy, and patients with brain metastases remain a population of special interest (3). Typically, only patients with stable, asymptomatic, and/or treated brain metastases are enrolled in clinical trials, or may be excluded altogether, particularly in the setting of leptomeningeal carcinomatosis. Consequently, this leads to significant concerns on the external validity of clinical trial evidence to real-world clinical practice (4).
In this review, we describe the current trends in cancer clinical trial eligibility for patients with brain metastases in both early and late phase trials, with a focus on recently approved targeted and immunotherapies. The United States (US) Food and Drug Administration (FDA) approved therapies from 2018-2020 and the clinical trial evidence base leading to approval are evaluated. Key recommendations previously published by the American Society of Clinical Oncology (ASCO)–Friends of Cancer Research (FCR) Brain Metastases Working Group for the inclusion of patients with brain metastases in clinical trials to improve generalizability of trial evidence are considered (5). This includes an analysis of inclusion and exclusion criteria, requirements for baseline screening for brain metastases, surveillance cerebral imaging and incorporation of trial endpoints for patients with brain metastases. Finally, the use of alternative sources of evidence such as real-world evidence with registries and collaborative studies are discussed.
Analysis of Recently FDA Approved Cancer Therapies
We conducted an analysis of newly FDA approved cancer therapies from 2018-2020 (6–8) as shown in Supplementary Table 1. For each cancer therapy, the registrational trial leading to regulatory approval was evaluated. The characteristics of the registrational trials are summarized in Supplementary Table 2. Trials conducted in the metastatic or late-stage cancer setting were initially assessed for eligibility for patients with brain metastases. Of 27 trials, 18 (67%) allowed enrollment of patients with stable and asymptomatic brain metastases (Figure 1A). Baseline screening for brain metastases with CT or MR imaging was required in 14/27 (52%) trials (Figure 1B). Surveillance cerebral imaging in patients without brain metastases at baseline was required in only 1/27 (4%) trials (Figure 1C). A prespecified trial endpoint evaluating patients with brain metastases was incorporated in 5/27 (19%) trials (Figure 1D).
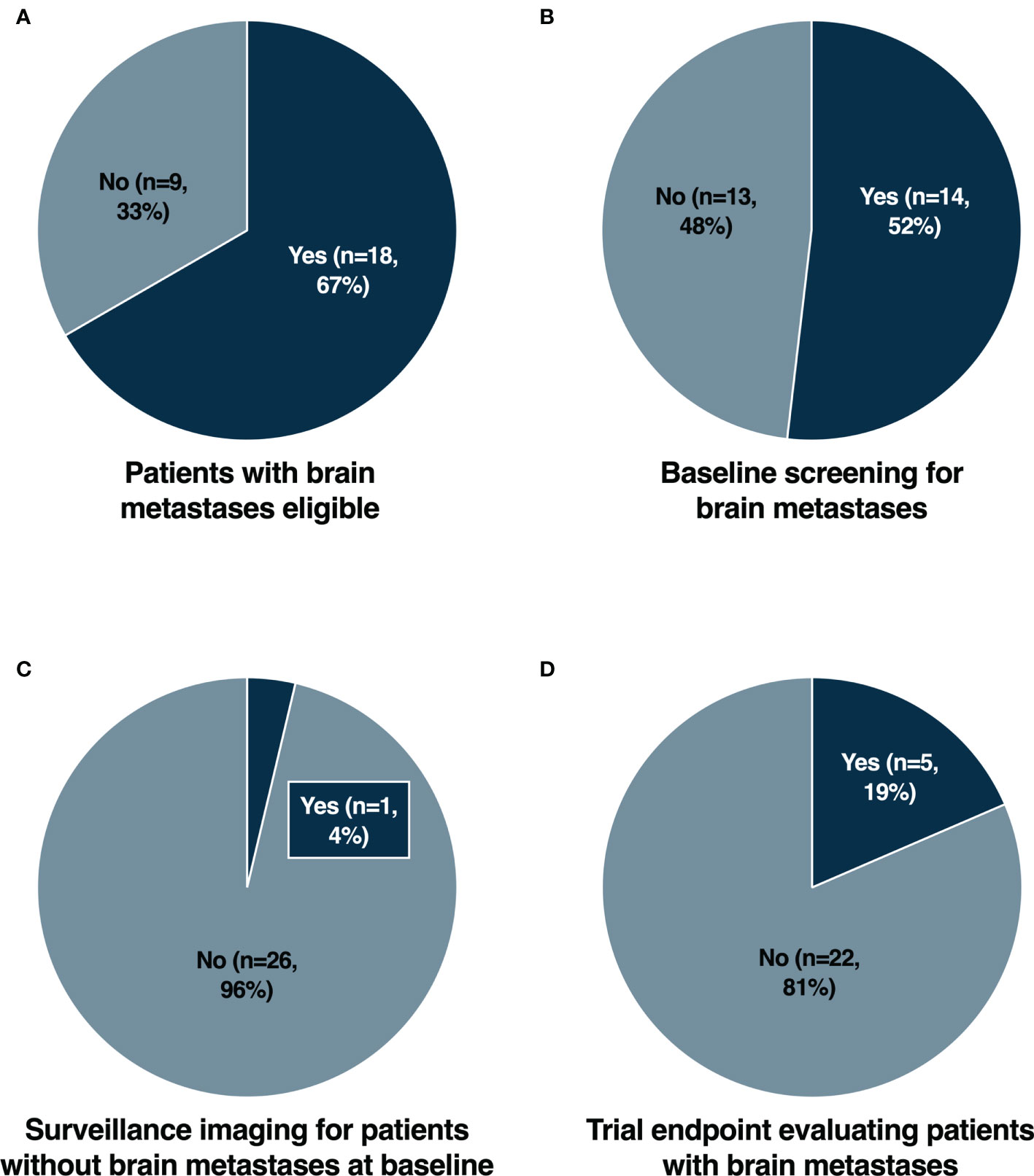
Figure 1 Eligibility for patients with brain metastases (A), baseline screening for brain metastases (B), surveillance imaging for patients without brain metastases at baseline (C) and trial endpoint evaluating patients with brain metastases (D) in registrational trials for newly FDA approved cancer therapies from 2018-2020.
Eligibility of Patients With Brain Metastases
Patients with brain metastases have historically been excluded from clinical trials due to concerns relating to overall greater risks of toxicity and poorer survival outcomes. With improved local therapeutic options for brain metastases and greater survival outcomes, assessment of intracranial efficacy and toxicity is becoming ever more important. The potential lack of blood-brain barrier (BBB) penetration for novel therapies is also often cited as a rationale for exclusion. However, for established brain metastases, tumors compromise the integrity of the BBB acquiring neovasculature and a resulting blood-tumor barrier (9). Consequently, increasingly there are trends to include patients with stable, treated and/or asymptomatic brain metastases (10). This approach would improve the generalizability of trial results, particularly in cancer types with a high prevalence of brain metastases. Nevertheless, from our analysis (Figure 1A), we found that a significant proportion of recently approved cancer therapies still exclude patients with brain metastases from the initial registrational trials. This included trials in tumor types with a low overall prevalence of brain metastases, such as NAVIGATOR (avapritinib) (11) and INVICTUS (ripretinib) (12) for gastrointestinal stromal tumors (GIST) and Study EZH-202 (tazemetostat) (13) for epithelioid sarcomas. In addition, there were cases in which CNS disease may represent a distinct clinical entity such as CNS lymphoma (14, 15). However, even in cancers with a high prevalence of brain metastases, there were examples of trials which completely excluded patients with brain metastases. Notably this included small cell lung cancer (SCLC) with Study B-005 (lurbinectedin) (16), EGFR mutated non-small cell lung cancer (NSCLC) with ARCHER 1050 (dacomitinib) (17) and triple negative breast cancer (TNBC) with IMMU-132-01 (sacituzumab govitecan) (18). The lack of evidence for intracranial efficacy of agents that have received regulatory approval represents a significant limitation for these compounds (19, 20). Particularly for EGFR mutated NSCLC and TNBC where the prevalence of brain metastases may be as high as 32% and 46% respectively (21–23). Whilst patients with brain metastases may have been subsequently allowed in larger phase 3 trials such as for lurbinectedin (24) and sacituzumab govitecan (25), the initial exclusion also necessitated the initiation of further trials to generate data for this important patient subpopulation (26, 27). Furthermore, the rationale for excluding patients with brain metastases was not elaborated upon in the primary publications. The lack of efficacy and safety data from early phase trials may have been a contributory factor. However, lurbinectedin (28, 29) and dacomitinib (30) for example had allowed patients with non-progressive or treated/stable brain metastases in earlier trials.
Baseline Screening for Brain Metastases and Surveillance Cerebral Imaging
Screening for brain metastases at baseline is a common cause of screen failure, particularly in early phase clinical trials (31). Consequently, unless mandated this may lead to hesitancy from clinicians to perform cerebral imaging for risk of jeopardizing a patient’s eligibility for trials (5). As trials increasingly allow patients with stable and treated brain metastases however, more completely characterizing the intracranial efficacy of novel therapies also becomes of heightened importance. From our analysis, nearly half (48%) of trials did not require mandatory cerebral imaging at baseline (Figure 1B), and only one (4%) trial required surveillance imaging for patients without brain metastases at baseline (Figure 1C). For many trials, cerebral imaging during screening was at least required with known or suspected brain metastases. However, there is increasing evidence supporting routine standard of care screening for brain metastases in many cancers, such as advanced breast cancer, melanoma and NSCLC, both at diagnosis and after initiation of palliative systemic therapy (32). Despite this, there remained trials such as SOLAR-1 (alpelisib) (33), SOPHIA (margetuximab) (34), ARROW (pralsetinib) (35) and LIBRETTO-001 (selpercatinib) (36), that did not mandate baseline cerebral imaging. The NAVIGATOR trial (11), a phase 1 study of avapritinib which included a dose expansion cohort for PDGFRA-mutated GIST patients, was the only study with regular surveillance cerebral imaging. However, this was performed due to safety concerns regarding an increased risk of intracranial bleeding, rather than generating data elucidating intracranial efficacy. In addition to intracranial response rates, measures of intracranial efficacy such as time to CNS progression, are also increasingly reported. Therefore, the role for routine surveillance cerebral imaging may become important in cancers with a propensity for the development of brain metastases.
Protocol Specific Management of Intracranial Progression
The treatment paradigm for brain metastases includes a multimodality approach including surgery, radiation therapy and systemic therapy (3). Consequently, for patients that experience isolated intracranial progression, local therapeutic approaches whilst continuing systemic therapy beyond progression represents a commonly adopted clinical approach. For clinical trials, specific protocol guidance in such instances is crucial to safely and effectively treat progressive brain metastases whilst collecting adequate data for CNS outcomes. For example, the COLUMBUS trial evaluating encorafenib plus binimetinib in patients with BRAF mutated melanoma (37), specified the potential for dosing beyond progression for new brain metastases treatable with stereotactic radiotherapy or surgery but not requiring whole brain radiotherapy. However, for a large majority of trials that allowed treatment beyond progression, there was no specific protocol guidance for the management of intracranial progression.
Incorporation of Trial Endpoints for Patients With Brain Metastases
With the improving intracranial efficacy of many novel targeted and immunotherapies compared with traditional chemotherapy, the prospective evaluation of CNS outcomes with prespecified endpoints is also becoming paramount. In our analysis, only a small number of registrational trials prespecified a trial endpoint evaluating patients with brain metastases (Figure 1D). This included Study B7461001 of lorlatinib for patients with ALK rearranged NSCLC (38), which included intracranial objective response rates as a co-primary endpoint. The remaining trials incorporated secondary endpoints assessing intracranial response and/or time to intracranial progression, including trials such as ARROW (pralsetinib) (35) and LIBRETTO-001 (selpercatinib) (36) for RET rearranged NSCLC, HER2CLIMB (tucatinib) (39) for HER2 positive breast cancer and ALKA/STARTRK-1/STARTRK-2 (entrectinib) (40, 41) for NTRK rearranged solid tumors and ROS1 rearranged NSCLC. Post hoc analyses describing outcomes for patients with brain metastases have subsequently been reported from many of the other registrational trials. However, without prospective plans to evaluate CNS response and progression, results may be more exploratory. For example, in the DESTINY-Breast01 trial of trastuzumab deruxtecan in HER2 positive breast cancer (42), there was a cap of patients with brain metastases allowed for enrolment. Intracranial efficacy from this trial has been shown to be promising (43), however further prospective trials for patients with brain metastases have been initiated to better characterize the CNS activity (27).
Alternative Sources of Data Such as Real-World Evidence
Despite increasing trends to include patients with brain metastases in clinical trials there remains subpopulations of patients that often remain excluded. This includes patients with leptomeningeal disease and symptomatic or active (new and/or progressive) brain metastases. Safety considerations or unsupportive pre-clinical evidence are potential reasons where exclusion from trials may still be appropriate (5). Therefore, there is heightened need for alternative sources of evidence in such populations, for which real-world evidence may provide an opportunity. Data sharing and collaboration through multi-center registries and trial networks are potential avenues to integrate supportive real-world evidence to clinical trial data (44). Particularly given the relative rarity of these subpopulations, pooled analyses may represent efficient methods of generating high quality prospective data. The Brain Metastases in Breast Cancer Network Germany is one such example (45), however future efforts need to be driven by both academia and industry. With rapid development of targeted and immunotherapies with unique mechanisms of action, greater reverse translation of our biological understanding of novel compounds from real-world evidence and clinical trial data to inform pre-clinical models and drug discovery pipelines is also paramount (46).
Discussion
In our analysis, a significant proportion of registrational trials for new recently FDA approved cancer therapies allowed patients with brain metastases. However, there remained prominent examples of trials which excluded even stable or asymptomatic brain metastases. These trials may have been conducted in tumor types with extremely rare incidence of brain metastases, such as GIST (47). Nevertheless, the relatively rarity of brain metastases in these tumor types may not represent sufficient rationale for automatic exclusion from clinical trials – particularly when baseline screening for brain metastases is mandated. Whilst most trials also required baseline screening for brain metastases, only a minority of trials required surveillance CNS imaging for patients without baseline brain metastases, had protocol specified guidelines for intracranial progression, or incorporated a trial endpoint for patients with brain metastases. This highlights important areas in which we can improve our understanding of the intracranial activity of novel therapies from clinical trials (Table 1).
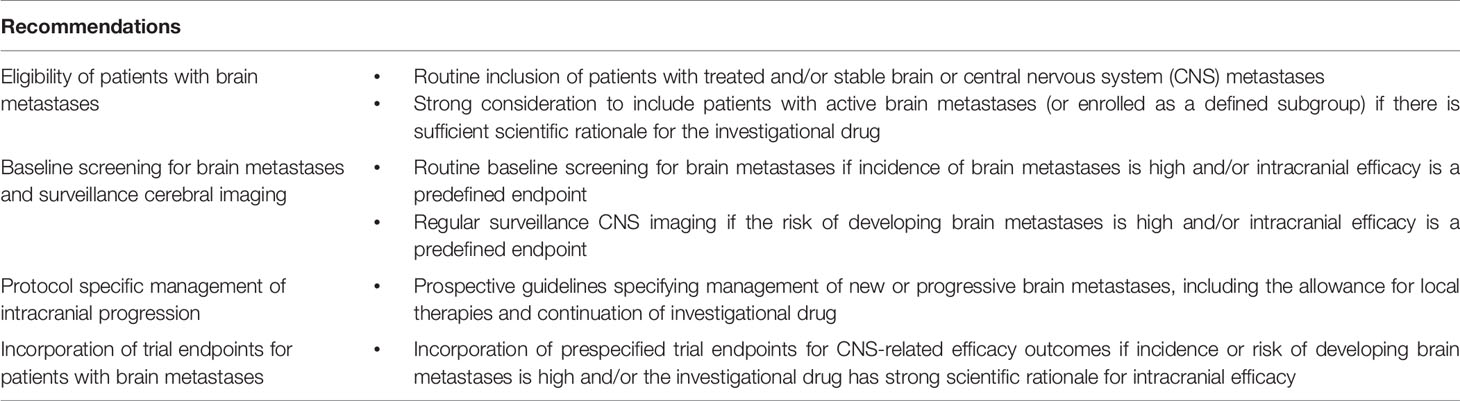
Table 1 Recommendations to improve the clinical trial eligibility criteria and evaluation of patients with brain metastases.
Recently, the FDA released specific guidance for industry, outlining recommendations for the inclusion of patients with brain metastases (48). It is emphasized that patients with active brain metastases or leptomeningeal disease should not be automatically excluded from trials. Eligibility in early drug development trials to inform criteria for later-phase trials, mitigation of uncertainties with separate subgroups within trials and the importance of CNS imaging at regular intervals are other key recommendations. Nevertheless, deeper considerations of risk benefit ratio with regards to cancer type, disease stage, known pre-clinical data and drug safety profile are all clearly influential in the development of clinical trial eligibility criteria and protocols. For novel therapies with unique mechanisms of action, such as newer immunotherapies, the potential for distinct toxicity profiles or adverse events due to CNS tumor inflammation and/or psuedoprogression is a relevant concern (49). However, from trials to date of immune checkpoint inhibitors in patients with brain metastases, the rates of toxicities and neurologic adverse events do not appear significantly different, and deaths due to neurologic complications remain rare (50). A greater molecular understanding of the development and progression of brain metastases within the unique brain microenvironment is also driving advances with more precise approaches for local and systemic therapies (51). This has broader implications for the data generated from the inclusion of patients with brain metastases on trials evaluating novel compounds, where combination approaches may enhance the intracranial efficacy. Costs and impacts on trial efficiency however, remain other practical considerations (5). Regular surveillance CNS imaging for example, particularly with MRI may be burdensome for patients with tumor types with low prevalence of brain metastases. Our analysis must therefore also be viewed in the context of a markedly heterogenous collation of therapies and registrational trials. In addition, given the length of time required for drug development from early to late phase trials and regulatory approval, the trials in our analysis may not be wholly representative of more contemporary practices in trial protocol design. Ultimately however, the drug development landscape is rapidly evolving with an increasing incidence of accelerated approvals from early phase trials. Consequently, the critical evaluation of clinical trial evidence and its generalizability across the patient population is of heightened relevance.
With an increasing prevalence of patients with brain metastases, understanding the intracranial efficacy of novel therapies is crucial. Expanding the eligibility of patients with brain metastases in registrational trials, or the incorporation of procedures or endpoints in trial design will generate important high-quality data in this patient population with significant unmet need. This will enhance our ability to integrate systemic therapies in the multimodality treatment of patients with brain metastases.
Authors Contributions
AT and MK contributed to conception and design of the study. All authors contributed to manuscript preparation, read, and approved the submitted version.
Conflict of Interest
AT reports consultant or advisory roles for Amgen; outside of the submitted work. DB reports honoraria from Varian Medical Systems; and research funding from Varian Medical Systems and Novocure; outside of the submitted work. EL reports honoraria from MedLink, prIME Oncology, and the American Academic of Neurology (Continuum); and royalties from Wolters Kluwer Health (UpToDate); outside of the submitted work. MMK reports research funding from Blue Earth Diagnostics; outside of the submitted work. MM reports consultant or advisory roles for Zap, Mevion, Karyopharm, Tocagen and Astra-Zeneca; and Board of Directors options from Oncoceutics; outside of the submitted work. MK reports consultant or advisory roles for Janssen, AbbVie, Ipsen, Pfizer Roche, and Jackson Laboratory for Genomic Medicine; and research funding from AbbVie, Bristol-Myers Squibb, and Specialized Therapeutics; outside of the submitted work.
Publisher’s Note
All claims expressed in this article are solely those of the authors and do not necessarily represent those of their affiliated organizations, or those of the publisher, the editors and the reviewers. Any product that may be evaluated in this article, or claim that may be made by its manufacturer, is not guaranteed or endorsed by the publisher.
Supplementary Material
The Supplementary Material for this article can be found online at: https://www.frontiersin.org/articles/10.3389/fonc.2021.780379/full#supplementary-material
References
1. Cagney DN, Martin AM, Catalano PJ, Redig AJ, Lin NU, Lee EQ, et al. Incidence and Prognosis of Patients With Brain Metastases at Diagnosis of Systemic Malignancy: A Population-Based Study. Neuro Oncol (2017) 19:1511–21. doi: 10.1093/neuonc/nox077
2. Arvold ND, Lee EQ, Mehta MP, Margolin K, Alexander BM, Lin NU, et al. Updates in the Management of Brain Metastases. Neuro-oncology (2016) 18:1043–65. doi: 10.1093/neuonc/now127
3. Suh JH, Kotecha R, Chao ST, Ahluwalia MS, Sahgal A, Chang EL. Current Approaches to the Management of Brain Metastases. Nat Rev Clin Oncol (2020) 17:279–99. doi: 10.1038/s41571-019-0320-3
4. Unger JM, Vaidya R, Hershman DL, Minasian LM, Fleury ME. Systematic Review and Meta-Analysis of the Magnitude of Structural, Clinical, and Physician and Patient Barriers to Cancer Clinical Trial Participation. J Natl Cancer Inst (2019) 111:245–55. doi: 10.1093/jnci/djy221
5. Lin NU, Prowell T, Tan AR, Kozak M, Rosen O, Amiri-Kordestani L, et al. Modernizing Clinical Trial Eligibility Criteria: Recommendations of the American Society of Clinical Oncology-Friends of Cancer Research Brain Metastases Working Group. J Clin Oncol (2017) 35:3760–73. doi: 10.1200/JCO.2017.74.0761
6. Mullard A. FDA Drug Approvals. Nat Rev Drug Discov (2019) 18:85–9. doi: 10.1038/d41573-019-00014-x
7. Mullard A. FDA Drug Approvals. Nat Rev Drug Discov (2020) 19:79–84. doi: 10.1038/d41573-020-00001-7
8. Mullard A. FDA Drug Approvals. Nat Rev Drug Discov (2021) 20:85–90. doi: 10.1038/d41573-021-00002-0
9. Arvanitis CD, Ferraro GB, Jain RK. The Blood-Brain Barrier and Blood-Tumour Barrier in Brain Tumours and Metastases. Nat Rev Cancer (2020) 20:26–41. doi: 10.1038/s41568-019-0205-x
10. Patel RR, Verma V, Miller AB, Lin TA, Jethanandani A, Espinoza AF, et al. Exclusion of Patients With Brain Metastases From Cancer Clinical Trials. Neuro Oncol (2020) 22:577–9. doi: 10.1093/neuonc/noz246
11. Heinrich MC, Jones RL, von Mehren M, Schöffski P, Serrano C, Kang Y-K, et al. Avapritinib in Advanced PDGFRA D842V-Mutant Gastrointestinal Stromal Tumour (NAVIGATOR): A Multicentre, Open-Label, Phase 1 Trial. Lancet Oncol (2020) 21:935–46. doi: 10.1016/S1470-2045(20)30269-2
12. Blay J-Y, Serrano C, Heinrich MC, Zalcberg J, Bauer S, Gelderblom H, et al. Ripretinib in Patients With Advanced Gastrointestinal Stromal Tumours (INVICTUS): A Double-Blind, Randomised, Placebo-Controlled, Phase 3 Trial. Lancet Oncol (2020) 21:923–34. doi: 10.1016/S1470-2045(20)30168-6
13. Gounder M, Schöffski P, Jones RL, Agulnik M, Cote GM, Villalobos VM, et al. Tazemetostat in Advanced Epithelioid Sarcoma With Loss of INI1/SMARCB1: An International, Open-Label, Phase 2 Basket Study. Lancet Oncol (2020) 21:1423–32. doi: 10.1016/S1470-2045(20)30451-4
14. Song Y, Zhou K, Zou D, Zhou J, Hu J, Yang H, et al. Treatment of Patients With Relapsed or Refractory Mantle-Cell Lymphoma With Zanubrutinib, a Selective Inhibitor of Bruton’s Tyrosine Kinase. Clin Cancer Res (2020) 26:4216–24. doi: 10.1158/1078-0432.CCR-19-3703
15. Salles G, Duell J, González Barca E, Tournilhac O, Jurczak W, Liberati AM, et al. Tafasitamab Plus Lenalidomide in Relapsed or Refractory Diffuse Large B-Cell Lymphoma (L-MIND): A Multicentre, Prospective, Single-Arm, Phase 2 Study. Lancet Oncol (2020) 21:978–88. doi: 10.1016/S1470-2045(20)30225-4
16. Trigo J, Subbiah V, Besse B, Moreno V, López R, Sala MA, et al. Lurbinectedin as Second-Line Treatment for Patients With Small-Cell Lung Cancer: A Single-Arm, Open-Label, Phase 2 Basket Trial. Lancet Oncol (2020) 21:645–54. doi: 10.1016/S1470-2045(20)30068-1
17. Wu Y-L, Cheng Y, Zhou X, Lee KH, Nakagawa K, Niho S, et al. Dacomitinib Versus Gefitinib as First-Line Treatment for Patients With EGFR-Mutation-Positive non-Small-Cell Lung Cancer (ARCHER 1050): A Randomised, Open-Label, Phase 3 Trial. Lancet Oncol (2017) 18:1454–66. doi: 10.1016/S1470-2045(17)30608-3
18. Bardia A, Mayer IA, Vahdat LT, Tolaney SM, Isakoff SJ, Diamond JR, et al. Sacituzumab Govitecan-Hziy in Refractory Metastatic Triple-Negative Breast Cancer. N Engl J Med (2019) 380:741–51. doi: 10.1056/NEJMoa1814213
19. Caruso C. Dacomitinib Approved, But Might Not Be Used. Cancer Discovery (2018) 8:1500. doi: 10.1158/2159-8290.CD-NB2018-138
20. Pacheco JM. Systemic Therapy Options Following First-Line Chemoimmunotherapy in Small-Cell Lung Cancer. J Thorac Dis (2020) 12:6264–74. doi: 10.21037/jtd.2020.03.67
21. Rangachari D, Yamaguchi N, VanderLaan PA, Folch E, Mahadevan A, Floyd SR, et al. Brain Metastases in Patients With EGFR-Mutated or ALK-Rearranged non-Small-Cell Lung Cancers. Lung Cancer (2015) 88:108–11. doi: 10.1016/j.lungcan.2015.01.020
22. Iuchi T, Shingyoji M, Itakura M, Yokoi S, Moriya Y, Tamura H, et al. Frequency of Brain Metastases in non-Small-Cell Lung Cancer, and Their Association With Epidermal Growth Factor Receptor Mutations. Int J Clin Oncol (2015) 20:674–9. doi: 10.1007/s10147-014-0760-9
23. Lin NU, Claus E, Sohl J, Razzak AR, Arnaout A, Winer EP. Sites of Distant Recurrence and Clinical Outcomes in Patients With Metastatic Triple-Negative Breast Cancer: High Incidence of Central Nervous System Metastases. Cancer (2008) 113:2638–45. doi: 10.1002/cncr.23930
24. Farago AF, Drapkin BJ, Lopez-Vilarino de Ramos JA, Galmarini CM, Núñez R, Kahatt C, et al. ATLANTIS: A Phase III Study of Lurbinectedin/Doxorubicin Versus Topotecan or Cyclophosphamide/Doxorubicin/Vincristine in Patients With Small-Cell Lung Cancer Who Have Failed One Prior Platinum-Containing Line. Future Oncol (2019) 15:231–9. doi: 10.2217/fon-2018-0597
25. Bardia A, Hurvitz SA, Tolaney SM, Loirat D, Punie K, Oliveira M, et al. Sacituzumab Govitecan in Metastatic Triple-Negative Breast Cancer. N Engl J Med (2021) 384:1529–41. doi: 10.1056/NEJMoa2028485
26. Tan A, Mok T, Kim D, Yang JC, Lee C, Soo R, et al. P2.14-20 ATORG-003: Dacomitinib With or Without Dose Titration as First-Line Therapy for Metastatic EGFR Mutant Non-Small Cell Lung Cancer (NSCLC). J Thorac Oncol (2019) 14:S836–7. doi: 10.1016/j.jtho.2019.08.1805
27. Adams E, Wildiers H, Neven P, Punie K. Sacituzumab Govitecan and Trastuzumab Deruxtecan: Two New Antibody-Drug Conjugates in the Breast Cancer Treatment Landscape. ESMO Open (2021) 6:100204. doi: 10.1016/j.esmoop.2021.100204
28. Calvo E, Moreno V, Flynn M, Holgado E, Olmedo ME, Lopez Criado MP, et al. Antitumor Activity of Lurbinectedin (PM01183) and Doxorubicin in Relapsed Small-Cell Lung Cancer: Results From a Phase I Study. Ann Oncol (2017) 28:2559–66. doi: 10.1093/annonc/mdx357
29. Elez ME, Tabernero J, Geary D, Macarulla T, Kang SP, Kahatt C, et al. First-In-Human Phase I Study of Lurbinectedin (PM01183) in Patients With Advanced Solid Tumors. Clin Cancer Res (2014) 20:2205–14. doi: 10.1158/1078-0432.CCR-13-1880
30. Janne PA, Ou SH, Kim DW, Oxnard GR, Martins R, Kris MG, et al. Dacomitinib as First-Line Treatment in Patients With Clinically or Molecularly Selected Advanced Non-Small-Cell Lung Cancer: A Multicentre, Open-Label, Phase 2 Trial. Lancet Oncol (2014) 15:1433–41. doi: 10.1016/s1470-2045(14)70461-9
31. Kempf E, Lemoine N, Tergemina-Clain G, Turpin A, Postel-Vinay S, Lanoy E, et al. Hollebecque A. A Case-Control Study Brings to Light the Causes of Screen Failures in Phase 1 Cancer Clinical Trials. PloS One (2016) 11:e0154895. doi: 10.1371/journal.pone.0154895
32. Cagney DN, Martin AM, Catalano PJ, Brown PD, Alexander BM, Lin NU, et al. Implications of Screening for Brain Metastases in Patients With Breast Cancer and Non-Small Cell Lung Cancer. JAMA Oncol (2018) 4:1001–3. doi: 10.1001/jamaoncol.2018.0813
33. André F, Ciruelos E, Rubovszky G, Campone M, Loibl S, Rugo HS, et al. Alpelisib for PIK3CA-Mutated, Hormone Receptor-Positive Advanced Breast Cancer. N Engl J Med (2019) 380:1929–40. doi: 10.1056/NEJMoa1813904
34. Rugo HS, Im S-A, Cardoso F, Cortés J, Curigliano G, Musolino A, et al. Efficacy of Margetuximab vs Trastuzumab in Patients With Pretreated ERBB2-Positive Advanced Breast Cancer: A Phase 3 Randomized Clinical Trial. JAMA Oncol (2021) 7:573–84. doi: 10.1001/jamaoncol.2020.7932
35. Gainor JF, Curigliano G, Kim D-W, Lee DH, Besse B, Baik CS, et al. Pralsetinib for RET Fusion-Positive Non-Small-Cell Lung Cancer (ARROW): A Multi-Cohort, Open-Label, Phase 1/2 Study. Lancet Oncol (2021) 22:959–69. doi: 10.1016/S1470-2045(21)00247-3
36. Drilon A, Oxnard GR, Tan DSW, Loong HHF, Johnson M, Gainor J, et al. Efficacy of Selpercatinib in RET Fusion-Positive Non-Small-Cell Lung Cancer. N Engl J Med (2020) 383:813–24. doi: 10.1056/NEJMoa2005653
37. Dummer R, Ascierto PA, Gogas HJ, Arance A, Mandala M, Liszkay G, et al. Encorafenib Plus Binimetinib Versus Vemurafenib or Encorafenib in Patients With BRAF-Mutant Melanoma (COLUMBUS): A Multicentre, Open-Label, Randomised Phase 3 Trial. Lancet Oncol (2018) 19:603–15. doi: 10.1016/S1470-2045(18)30142-6
38. Solomon BJ, Besse B, Bauer TM, Felip E, Soo RA, Camidge DR, et al. Lorlatinib in Patients With ALK-Positive non-Small-Cell Lung Cancer: Results From a Global Phase 2 Study. Lancet Oncol (2018) 19:1654–67. doi: 10.1016/S1470-2045(18)30649-1
39. Murthy RK, Loi S, Okines A, Paplomata E, Hamilton E, Hurvitz SA, et al. Tucatinib, Trastuzumab, and Capecitabine for HER2-Positive Metastatic Breast Cancer. N Engl J Med (2020) 382:597–609. doi: 10.1056/NEJMoa1914609
40. Drilon A, Siena S, Dziadziuszko R, Barlesi F, Krebs MG, Shaw AT, et al. Entrectinib in ROS1 Fusion-Positive Non-Small-Cell Lung Cancer: Integrated Analysis of Three Phase 1-2 Trials. Lancet Oncol (2020) 21:261–70. doi: 10.1016/S1470-2045(19)30690-4
41. Doebele RC, Drilon A, Paz-Ares L, Siena S, Shaw AT, Farago AF, et al. Entrectinib in Patients With Advanced or Metastatic NTRK Fusion-Positive Solid Tumours: Integrated Analysis of Three Phase 1-2 Trials. Lancet Oncol (2020) 21:271–82. doi: 10.1016/S1470-2045(19)30691-6
42. Modi S, Saura C, Yamashita T, Park YH, Kim S-B, Tamura K, et al. Trastuzumab Deruxtecan in Previously Treated HER2-Positive Breast Cancer. N Engl J Med (2020) 382:610–21. doi: 10.1056/NEJMoa1914510
43. Jerusalem GHM, Park YH, Yamashita T, Hurvitz SA, Modi S, Andre F, et al. Trastuzumab Deruxtecan (T-DXd) in Patients With HER2+ Metastatic Breast Cancer With Brain Metastases: A Subgroup Analysis of the DESTINY-Breast01 Trial. JCO (2021) 39:526–6. doi: 10.1200/JCO.2021.39.15_suppl.526
44. Spreafico A, Hansen AR, Abdul Razak AR, Bedard PL, Siu LL. The Future of Clinical Trial Design in Oncology. Cancer Discov (2021) 11:822–37. doi: 10.1158/2159-8290.CD-20-1301
45. Witzel I, Laakmann E, Weide R, Neunhöffer T, Park-Simon T-J, Schmidt M, et al. Treatment and Outcomes of Patients in the Brain Metastases in Breast Cancer Network Registry. Eur J Cancer (2018) 102:1–9. doi: 10.1016/j.ejca.2018.07.004
46. Shakhnovich V. It’s Time to Reverse Our Thinking: The Reverse Translation Research Paradigm. Clin Transl Sci (2018) 11:98–9. doi: 10.1111/cts.12538
47. Yang DY, Wang X, Yuan WJ, Chen ZH. Metastatic Pattern and Prognosis of Gastrointestinal Stromal Tumor (GIST): A SEER-Based Analysis. Clin Transl Oncol (2019) 21:1654–62. doi: 10.1007/s12094-019-02094-y
48. Food and Drug Administration. Cancer Clinical Trial Eligibility Criteria: Brain Metastases (2020). Available at: https://www.fda.gov/regulatory-information/search-fda-guidance-documents/cancer-clinical-trial-eligibility-criteria-brain-metastases (Accessed September 1, 2021).
49. Postow MA, Sidlow R, Hellmann MD. Immune-Related Adverse Events Associated With Immune Checkpoint Blockade. New Engl J Med (2018) 378:158–68. doi: 10.1056/NEJMra1703481
50. Wang DY, Johnson DB, Davis EJ. Toxicities Associated With PD-1/PD-L1 Blockade. Cancer J (2018) 24:36–40. doi: 10.1097/PPO.0000000000000296
Keywords: brain metastases, trial eligibility, intracranial efficacy, novel therapeutic agents, CNS metastases
Citation: Tan AC, Boggs DH, Lee EQ, Kim MM, Mehta MP and Khasraw M (2022) Clinical Trial Eligibility Criteria and Recently Approved Cancer Therapies for Patients With Brain Metastases. Front. Oncol. 11:780379. doi: 10.3389/fonc.2021.780379
Received: 21 September 2021; Accepted: 09 December 2021;
Published: 03 January 2022.
Edited by:
Gavin P. Dunn, Washington University School of Medicine in St. Louis, United StatesReviewed by:
Dan Qi, Baylor Scott and White Health, United StatesMariko Sato, The University of Iowa, United States
Copyright © 2022 Tan, Boggs, Lee, Kim, Mehta and Khasraw. This is an open-access article distributed under the terms of the Creative Commons Attribution License (CC BY). The use, distribution or reproduction in other forums is permitted, provided the original author(s) and the copyright owner(s) are credited and that the original publication in this journal is cited, in accordance with accepted academic practice. No use, distribution or reproduction is permitted which does not comply with these terms.
*Correspondence: Mustafa Khasraw, mustafa.khasraw@duke.edu