- 1Department of Internal Medicine I, University Hospital Dresden, TU Dresden, Dresden, Germany
- 2German Cancer Consortium (DKTK) partner site Dresden, Dresden, Germany
- 3German Cancer Research Center (DKFZ), Heidelberg, Germany
- 4Institute of Pathology, University Hospital Dresden, TU Dresden, Dresden, Germany
- 5DKMS Clinical Trials Unit, Dresden, Germany
- 6AgenDix GmbH, Dresden, Germany
- 7National Center for Tumor Diseases (NCT) Dresden, Dresden, Germany
In patients who have undergone allogeneic hematopoietic cell transplantation (HCT), myeloid mixed donor chimerism (MC) is a risk factor for disease relapse. In contrast, several studies found favorable outcome in patients with lymphoid MC. Thus far, most studies evaluating MC focused on a short-term follow-up period. Here, we report the first case series of long-term survivors with MC. We screened 1,346 patients having undergone HCT for myeloid neoplasms at our center from 1996 to 2016; 443 patients with data on total peripheral blood mononuclear cells (PBMC)/CD4+/CD34+ short tandem repeat (STR) donor chimerism (DC) and follow-up ≥24 months post-HCT were included. We identified 10 patients with long-term MC (PBMC DC <95% at ≥12 months post-HCT). Median follow-up was 11 years. All patients had received combined ex vivo/in vivo T cell-depleted (TCD) peripheral blood stem cells; none experienced ≥grade 2 acute graft-versus-host disease (GVHD). The mean total PBMC, CD4+, and CD34+ DC of all patients were 95.88%, 85.84%, and 90.15%, respectively. Reduced-intensity conditioning (RIC) was associated with a trend to lower mean total DC. Of note, two patients who experienced relapse had lower CD34+ DC but higher CD4+ DC as compared with patients in continuous remission. Bone marrow evaluation revealed increased CD4+/FOXP3+ cells in patients with MC, which might indicate expansion of regulatory T cells (Tregs). Our results support known predictive factors associated with MC such as RIC and TCD, promote the value of CD34+ MC as a potential predictor of relapse, highlight the potential association of CD4+ MC with reduced risk of GVHD, and indicate a possible role of Tregs in the maintenance of immune tolerance post-HCT.
Introduction
The rationale of allogeneic hematopoietic cell transplantation (HCT) for hematologic malignancies is complete eradication of malignant clones and subsequent replacement of the recipients by the donor hematopoiesis together with development of full donor chimerism (FDC). However, some patients—transplanted for either malignant or non-malignant hematologic disorders—retain recipient hematopoietic cells and develop a mixture of both donor- and recipient-derived hematopoiesis, referred to as mixed donor chimerism (MC). Several factors for the establishment of MC have been identified, e.g., the underlying disease [inherited bone marrow failure syndromes (IBMFS) vs. hematologic malignancies, myeloid vs. lymphoid neoplasms)]; conditioning regimen [reduced-intensity conditioning (RIC) vs. myeloablative conditioning (MAC)]; use of T-cell depletion (TCD) (ex vivo, e.g., CD34+ selection; or in vivo, e.g., anti-thymocyte globulin (ATG) or alemtuzumab); and the dose of CD34+ cells infused (1–6). In patients with IBMFS, such as thalassemia, presence of MC is a common event after successful transplantation. Here, the outcome of patients transplanted for IBMFS with persistent MC seems to be comparable with that with FDC (7, 8). In contrast, in hematologic malignancies, MC is a risk factor for graft failure and relapse (9). For example, in a study conducted by Lamba and colleagues, patients with day +90 peripheral blood mononuclear cells (PBMC) MC had significantly higher relapse rates as compared with patients with FDC at 6 months post-HCT (23% vs. 7%; p = .03) (10). Similar, Koreth et al. found that patients with day +100 PBMC MC had higher 2-year relapse rates (53% vs. 30%; p <.0001) and worse 2-year progression-free survival (PFS) (37% vs. 59%; p <.0001) as compared with patients with day +100 PBMC FDC (11). In particular, a decline in DC/MC of the myeloid lineage (CD33+/CD34+) is associated with disease relapse: in patients transplanted for acute myeloid leukemia (AML) and myelodysplastic syndrome (MDS), CD34+ PBMC MC was found to be an independent predictor of relapse and inferior survival (12–14). Here, the incidence of relapse in patients with CD34+ MC was 57% as compared with 18% in patients with CD34+ FDC (12). The impact of lymphoid MC is still under discussion. While Koreth et al. found CD3+ MC to be associated with increased rates of relapse and unfavorable PFS and overall survival (OS) in patients with hematological malignancies undergoing T cell-replete HCT, Deeg and colleagues reported improved PFS and OS in patients with CD3+ MC who underwent HCT for primary or secondary myelofibrosis (11, 15). However, there is evidence that low-grade MC—in particular MC affecting the lymphoid lineage (CD3+/CD4+)—is associated with lower non-relapse mortality (NRM): in line with previous reports, Baron et al. showed that lymphoid MC is associated with a lower rate of grade 2–4 acute graft-versus-host disease (GVHD) in patients who underwent RIC (9, 16, 17). Here, Sykes and colleagues evaluated the intentional induction of MC aiming for the induction of immune tolerance in patients undergoing HCT: their novel RIC/TCD (ATG/thymic irradiation) regimen was associated with a low rate of NRM and grade 2–4 acute GVHD and enabled safe subsequent administration of donor lymphocytes (18, 19). Of note, most studies evaluating chimerism focus on a short-term follow-up period (≤12–24 months post-HCT). Accordingly, little is known about patients with long-term MC. Here, we aimed to evaluate incidence, risk factors, and associated patient outcome of long-term MC in patients who underwent HCT for myeloid neoplasms.
Methods
For this retrospective analysis, all patients who underwent allogeneic HCT for myeloid neoplasms at our center from 1996 to 2016 (n = 1,346) were screened for long-term MC. Supplementary Figure 1 summarizes the patient selection and composition. Four hundred forty-three patients with myeloid neoplasms with follow-up ≥24 months post-HCT and data on short tandem repeat (STR)-based PBMC, lymphoid (CD4+), and myeloid (CD34+) subset DC at various timepoints post-HCT were included in the present analysis. Patients with evidence of molecular (other than MC) or hematological relapse within 12 months post-HCT were excluded. Long-term MC was defined as PBMC DC <95% at ≥12 months post-HCT. All patients provided written informed consent; forms were approved by the Ethics Committee of the TU Dresden (EK98032010) in accordance with the Declaration of Helsinki.
STR-based DC analysis had been performed on PBMC and/or bone marrow mononuclear cells (BMMC) on a regular basis according to institutional standards (PBMC-DC: every 14 days for the first 4 months, monthly for eight additional months, and then every 3 months and as indicated/needed (e.g., new blood cytopenia); BMMC-DC: around day +90 and day +180 post-HCT and as indicated/needed (e.g., new blood cytopenia)). DC was determined using the AmpFlSTR Profiler PCR Amplification Kit (Applied Biosystems, Darmstadt, Germany) (20). From 2004 on, the Mentype® Chimera® Kit (Biotype, Dresden, Germany), which uses STR loci (D2S1360, D3S1744, D4S2366, D5S2500, D6S474, D7S1517, D8S1132, D10S2325, D12S391, D18S51, D21S2055, and SE33 (ACTBP2)) and the amelogenin locus (on the X and Y chromosomes), was used as previously reported (21).
Patients with relapse, measurable residual disease (MRD)-positive patients, and/or patients with MC were evaluated for donor lymphocyte infusion (DLI) on a regular basis according to institutional standards.
Immunohistochemistry (IHC) in formalin-fixed paraffin-embedded (FFPE) bone marrow trephine biopsies were performed as previously described (22). Bone marrow biopsies obtained >12 months post-HCT were available for three patients (UPN01, UPN04, and UPN06), bone marrow biopsies from three patients in continuous complete remission (CR) and with FDC >12 months post-HCT served as control. The following primary antibodies were used: CD4 (clone SP35, Ventana/Roche Diagnostics), FOXP3 (clone SP97, Abcam), PDCD1 [PD1] (clone NAT105, Ventana/Roche Diagnostics), CD274 [PD-L1] (clone SP263, Ventana/Roche Diagnostics), and HAVCR2 [TIM3] (clone EPR22241, Abcam).
Standard statistical methods were used for descriptive analyses, the Mann–Whitney U test was used for non-parametric data, and the Shapiro–Wilk test was used to test for normal distribution; analyses were done with R version 3.5.3, RStudio version 1.2.1335 and GraphPad Prism version 9.0.0.
Results
We identified 10 patients [0.74% of all patients who have undergone HCT for myeloid neoplasms (n = 1,346) and 2.26% of patients with valid records and follow-up ≥24 months (n = 443)] with long-term MC (<95% DC at ≥12 months post-HCT). Patient demographics and transplantation characteristics are summarized in Table 1 and Figure 1A. Of these patients, one was diagnosed with MDS, five patients (50%) were diagnosed with AML, and four patients (40%) were diagnosed with chronic myeloid leukemia (CML). The time from diagnosis to allogeneic HCT ranged from 4 to 27 months (median, 11 months). The median age at transplantation was 39 years (range, 19–66 years). The median follow-up was 11 years (range, 2.2–21 years). Six patients (60%) had a human leukocyte antigen (HLA)-matched unrelated donor (MUD), three patients (30%) had an HLA-haploidentical donor (HAPLO), and one patient had an HLA-identical sibling who served as donor (SIB). All patients were cytomegalovirus (CMV) seronegative, and only one patient received a CMV-seropositive graft. The conditioning regimen was RIC/non-myeloablative in two patients (20%). Myeloablative conditioning was used in eight patients (80%); amongst these, five patients (45%) received fractionated total body irradiation (TBI) to a total dose of 12 Gy. Of note, all patients received combined ex vivo (CD34-selection or CD3/CD19-depletion)/in vivo (ATG, alemtuzumab, muromonab-CD3) TCD peripheral blood stem cells (PBSC). The median CD3+ and CD34+ cell dose was 3.11 × 103/kg and 6.54 × 106/kg, respectively. For GVHD prophylaxis, all patients received in vivo TCD (ATG, alemtuzumab, or muromonab-CD3) and calcineurin inhibitors with or without mycophenolate mofetil. All patients transplanted for CML received DLIs based on the institutional standards. None of the patients experienced ≥grade 2 acute GVHD or moderate/extensive chronic GVHD. At the last follow-up, eight patients (80%) were alive in continuous complete remission (CR), while two patients (UPN 09, UPN 10) deceased due to relapse of the underlying malignancy 26 and 45 months post-HCT.
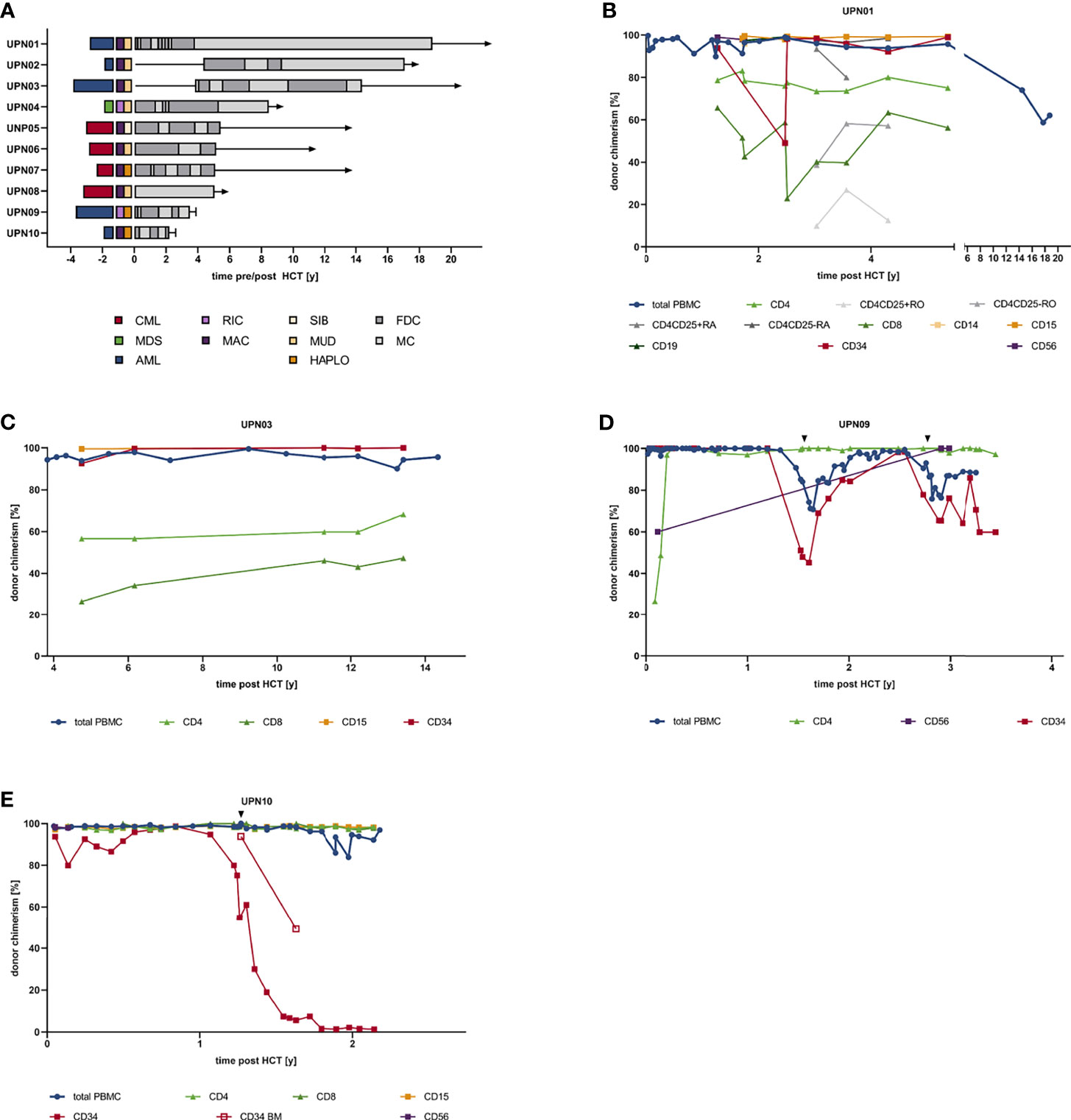
Figure 1 Patient and transplant characteristics and individual donor chimerism kinetics. (A) Swimmer plot indicating diagnosis, transplant characteristics, and chimerism kinetics. (B) Chimerism kinetics of UPN01. (C) Chimerism kinetics of UPN03. (D) Chimerism kinetics of UPN09. (E) Chimerism kinetics of UPN10. AML, acute myeloid leukemia; CML, chronic myeloid leukemia; FDC, full donor chimerism; HAPLO, haploidentical donor; HCT, hematopoietic cell transplantation; MAC, myeloablative conditioning; MC, mixed chimerism; MDS, myelodysplastic syndrome; MUD, matched unrelated donor; RIC, reduced-intensity conditioning; SIB, sibling donor; UPN, unique patient number.
Patients with >10% mixed CD4/CD34+ DC (UPN01, UPN03, UPN09, and UPN10) and the management of these patients were assessed in detail. In UPN01, the diagnosis of acute promyelocytic leukemia [APL with t (15, 17) (q22;q21); PML–RARA fusion (bcr3)] was established. Intensive induction and consolidation therapy were performed according to the APL93 trial protocol (NCT00599937). Two years after achieving a CR, the patient experienced relapse, and re-induction therapy and subsequent allogeneic HCT with an ex vivo/in vivo TCD graft (CD34 selection + ATG) from a matched unrelated donor was performed. Follow-up examinations showed complete hematological and molecular remission for the last 20 years but repeatedly revealed MC affecting the lymphoid as well as myeloid lineage (Figures 1B, 2). UPN03 (Figures 1C, 2) was diagnosed with therapy-related AML (prior radiotherapy for testicular germ cell tumor; normal male karyotype) in 1994. After induction therapy, the patient achieved CR and received consolidation and maintenance therapy. However, shortly thereafter, the patient experienced relapse, received re-induction therapy, and underwent subsequent allogeneic HCT with an ex vivo/in vivo TCD graft (CD34 selection + ATG) from a MUD in CR2. Notwithstanding, with regard MC, especially affecting the lymphoid lineage, the patient is still in complete hematological remission. UPN09 (Figures 1D, 2) was diagnosed with secondary AML (prior diagnosis of myeloproliferative neoplasm (MPN); AML not otherwise specified (NOS), AML without maturation, normal male karyotype, no BCR–ABL1 fusion, no CEBPA, FLT3, or NPM1 mutation) in 2008. The patient received intensive induction therapy and underwent haploidentical HCT with an ex vivo/in vivo TCD graft (CD3/CD19 depletion + muromonab-CD3) in CR1. Eighteen months later, the patient relapsed and received a total of 23 courses of azacytidine over 2 years, which resulted in a CR with incomplete hematologic recovery (CRi). In 2012, he relapsed again and received intensive re-induction chemotherapy and underwent second haploidentical HCT, which was complicated by pneumonia with fatal outcome during aplasia post-HCT. Both relapses were accompanied by prior decline in CD34+ DC, while full CD4+ DC was observed. UPN10 (Figures 1E, 2) was diagnosed with AML (AML with inv (3)(q21.3q26.2); GATA2, MECOM; ELN adverse risk category) in 2002 and received intensive induction therapy and subsequently underwent allogeneic haploidentical HCT with an ex vivo/in vivo TCD graft (CD34 selection + ATG) in CR1 3 months later. Fifteen months later, the patient relapsed; under salvage chemotherapy, morphologic leukemia-free state (MLFS) was achieved. However, the patient died due to recurring infectious complications under best supportive care measures 27 months post-HCT. Again, relapse was associated with CD34+ PBMC/BMMC MC, while in CD4/CD8+ subsets, FDC could be observed.
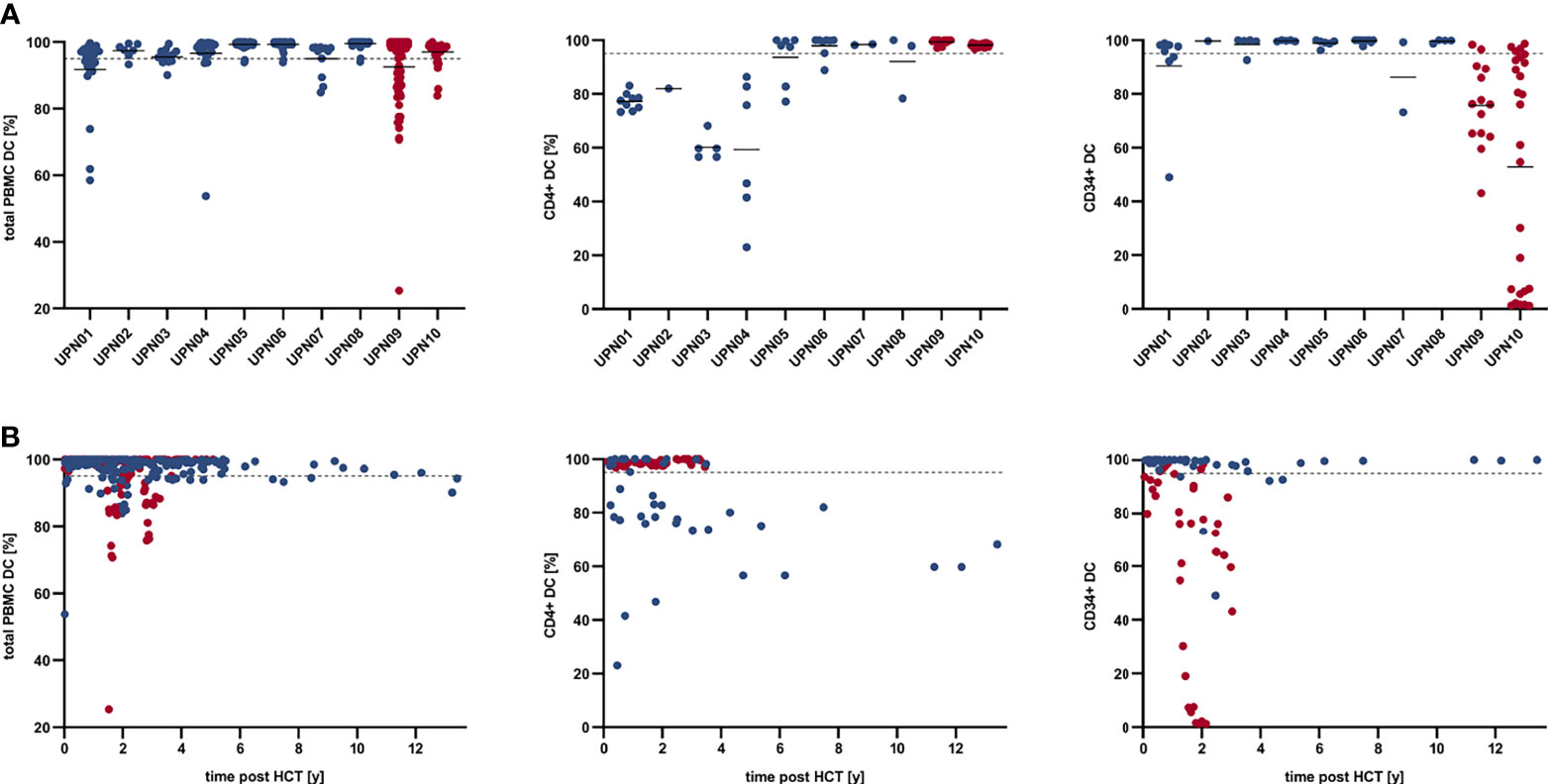
Figure 2 Total PBMC, CD4+, and CD34+ donor chimerism kinetics in MC patients who are alive in continuous CR (blue) and MC patients who deceased due to relapse (red). (A) Total PBMC, CD4+, and CD34+ donor chimerism per patient; solid lines indicate mean total PBMC, CD4+, and CD34+ DC per UPN. (B) Total PBMC, CD4+, and CD34+ donor chimerism over time post HCT. Dashed line indicates 95% DC. CR, complete remission; DC, donor chimerism; HCT, hematopoietic cell transplantation; MC, mixed chimerism; PBMC, peripheral blood mononuclear cells; UPN, unique patient number.
Overall, patients with CD4+ MC and CD34+ FDC (n = 6), CD4+ FDC and CD34+ MC (n = 2), and CD4+ and CD34+ MC (n = 2) were identified. Table 2 and Figure 2 summarize the results of individual chimerism determinations for total DC, CD4+ DC, and CD34+ DC. The mean total PBMC DC, CD4+ DC, and CD34+ DC of all patients was 95.88% (range, 90.56%–99.52%), 85.84% (range, 59.40%–99.38%), and 90.15% (range, 52.84%–99.76%), respectively. RIC was associated with a trend to lower mean total PBMC DC as compared with MAC (91.57% vs. 98.71%, p = .0714). Patients with haploidentical donors demonstrated lower mean CD34+ DC (71.63% vs. 98.08% p = .0176) but higher mean CD4+ DC (98.63% vs. 80.36%, p = .0176). Of note, the two patients who experienced relapse (UPN09 and UPN10) had lower mean CD34+ DC (64.32% vs. 96.61%, p = .0444) but higher CD4+ DC (98.78% vs. 82.61%, p = .0444) as compared with patients in continuous CR. Patient age, patient gender, myeloid neoplasm (AML vs. CML), time from initial diagnosis to allogeneic HCT, and CD3+/CD34+ cell dose had no significant impact on the extent of MC in either CD4+ or CD34+ cells. To address the underlying mechanisms of MC without evidence of relapse, we performed IHC studies in bone marrow trephine biopsies obtained >12 months post-HCT, which were available for three patients (UPN01, UPN04, and UPN06); eight patients in CR with FDC > 12 months post-HCT served as control. IHC showed more CD4+ (14.41% vs. 6.66%, p = .0391), FOXP3+ (2.06% vs. 0.36%, p = .0474) and PDCD1 [PD1]+ (2.82% vs. 0.54%, p =.0292) cells in patients with MC as compared with patients with FDC; in contrast, no differences in CD274 [PDL1]+ (0.08% vs. 0.09%, p = .9792) or HAVCR2 [TIM3]+ (1.35% vs. 2.34%, p = .2301) cells were observed (Figure 3).
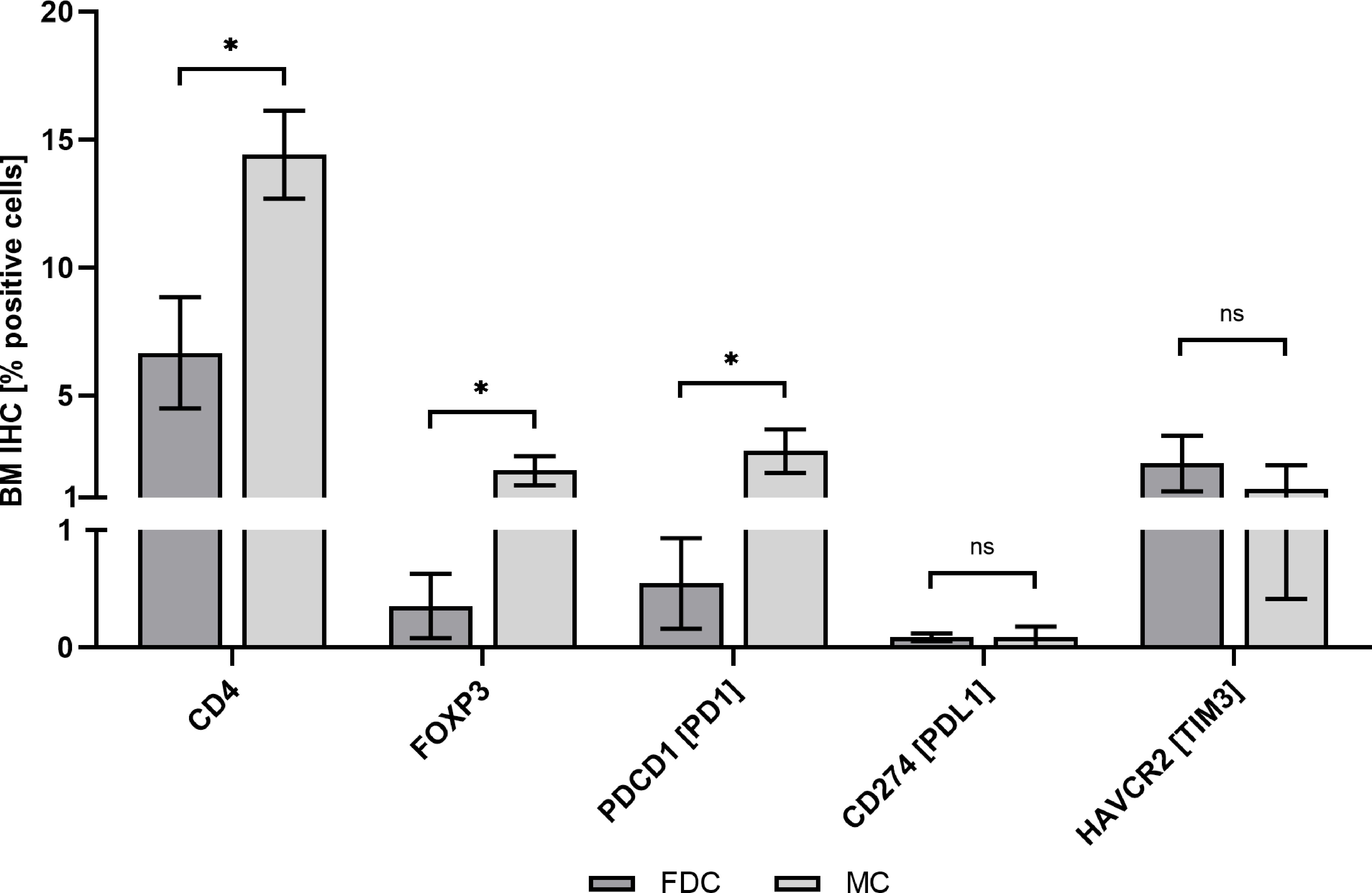
Figure 3 Bone marrow immunohistochemistry assessment. Patients with FDC (n = 8) vs. patients with MC (n = 3). BM, bone marrow; FDC, full donor chimerism; IHC, immunohistochemistry; MC, mixed chimerism; ns, non-significant; *p < .05.
Discussion
The rationale of HCT for hematologic malignancies is the complete eradication of malignant clones and subsequent replacement of the recipients by the donor hematopoiesis, achieving FDC. Several studies found favorable outcome in patients with low-grade MC—in particular lymphoid (CD3+/CD4+) MC after allogeneic HCT (9, 16, 17). In contrast, myeloid (CD33+/CD34+) MC is associated with disease relapse and poor prognosis (12–14). Of note, most studies evaluating chimerism focus on a short-term follow-up period (≤12–24 months post-HCT); therefore, little is known about patients with long-term MC. Here, we aimed to evaluate incidence, risk factors, and associated patient outcome of long-term MC in patients who underwent HCT for myeloid neoplasms. While screening 1,346 patients who underwent HCT for myeloid neoplasms at our center, 10 patients with long-term MC (0.74% of all patients undergoing HCT for myeloid neoplasms; 2.26% of patients with valid records and follow-up ≥24 months post-HCT) were identified. In contrast to our cohort, evaluating 231 patients transplanted for hematologic malignancies and severe aplastic anemia (SAA), Schaap et al. identified 19 patients (8.26%) with long-term MC. However, most patients evaluated by Schaap and coworkers were diagnosed with acute lymphoblastic leukemia (ALL), donors were HLA-A, HLA-B, HLA-DRB1, and HLA-DQB1-identical siblings, and all patients who were evaluated received TCD grafts (2). In contrast, we focused on patients with myeloid neoplasms, and all but one patient with evidence of MC had matched-unrelated or haploidentical donors, which might explain different incidences of MC. In accordance with Schaap et al. and others, all patients with MC received ex vivo as well as in vivo TCD (2, 25, 26). For example, Grimaldi et al. reported prolonged CD4+ MC in patients transplanted for SAA after TCD with alemtuzumab; furthermore, several other authors found TCD with ATG or alemtuzumab to be associated with MC (2, 26–28). In accordance with previous reports, TCD and subsequent CD4+ MC were associated with a low incidence of GVHD: none of our patients experienced ≥grade 2 acute GVHD or extensive chronic GVHD (2, 9, 26). Furthermore, conditioning regimen had an impact on DC: patients with RIC were more likely to show mixed total PBMC DC than patients with MAC; in contrast to previous reports, RIC was associated with mixed total PBMC DC but not mixed CD4+/CD34+ DC; however, a small number of cases caution against overinterpretation of these data (9, 29). In our analysis, patients with haploidentical donors had lower total and CD34+, but higher CD4+ DC. In contrast, Federmann et al. reported achievement of FDC in all patients after haploidentical HCT with RIC and ex vivo TCD (30). Both patients who deceased due to relapse showed recurrent/persistent decline in CD34+ DC, which is in line with previous studies identifying CD34+ MC as an independent risk factor for subsequent relapse and inferior survival (12, 14). Here, our findings support CD34+ DC monitoring, in particular since preemptive intervention might prevent/delay hematologic relapse in patients after HCT (13). Patient age, patient gender, and CD3+/CD34+ cell dose had no impact on the extent of MC. To address possible mechanisms of immune tolerance in MC long-term survivors, we performed IHC in BM trephine biopsies. As compared with FDC patients, IHC showed increased CD4+ and FOXP3+ cells in patients with MC, which might indicate expansion of regulatory T cells (Tregs) (31, 32). Here, our results are in line with previous reports: Kinsella et al. found increased levels of Tregs in MC patients as compared with patients with DC evaluating 69 patients with AML undergoing reduced-intensity conditioned allogeneic HCT with ex vivo TCD grafts (33). Of note, several studies indicate that ATG might induce Treg expansion and that Treg might preserve graft-versus-leukemia (GVL) effect while inhibiting GVHD (34–37). Similarly, in patients who have undergone combined kidney and bone marrow transplantation (CKBMT), donor-specific Tregs expanded within 6 months after CKBMT in those patients who achieved allograft tolerance, suggesting that early expansion of donor-specific Tregs is involved in tolerance induction following CKBMT (38). Here, we report one of the first case series of long-term survivors with MC with a follow-up >10 years post HCT. Our results support RIC and TCD as risk factors for MC and highlight the value of CD34+ PBMC MC as a potential independent predictor of relapse and inferior survival. While CD4+ MC seem to be associated with reduced risk of GVHD, the role of Tregs in immune tolerance induction needs to be appreciated. Since little is known about the mechanisms of MC, further research addressing mechanisms maintaining GVL while suppressing GVHD as well as the origin and function of maintained autologous non-malignant lymphohematopoietic cells is warranted.
Data Availability Statement
The original contributions presented in the study are included in the article/Supplementary Material. Further inquiries can be directed to the corresponding author.
Ethics Statement
The studies involving human participants were reviewed and approved by the Ethics Committee of the TU Dresden (EK98032010). The patients/participants provided their written informed consent to participate in this study.
Author Contributions
LR, FS, MB, and CT conceived the presented idea. FS, MvB, CR, KS, JM, and CR took care of the patients. LR, FS, UO, MvB, KJ, and CT performed the research. LR, FS, JS, and CT performed the data analysis. LR wrote the manuscript with help from FS, UO, CR, JS, MB, and CT. All authors contributed to the article and approved the submitted version.
Conflict of Interest
Author CT was employed by AgenDix GmbH.
The remaining authors declare that the research was conducted in the absence of any commercial or financial relationships that could be construed as a potential conflict of interest.
Publisher’s Note
All claims expressed in this article are solely those of the authors and do not necessarily represent those of their affiliated organizations, or those of the publisher, the editors and the reviewers. Any product that may be evaluated in this article, or claim that may be made by its manufacturer, is not guaranteed or endorsed by the publisher.
Supplementary Material
The Supplementary Material for this article can be found online at: https://www.frontiersin.org/articles/10.3389/fonc.2021.776946/full#supplementary-material
Supplementary Figure 1 | Patient selection and composition. AML, acute myeloid leukemia; CML, chronic myeloid leukemia; FU, follow-up; HCT, hematopoietic cell transplantation; MDS, myelodysplastic syndrome; MPN, myeloproliferative neoplasms; PBMC, peripheral blood mononuclear cells; STR, short tandem repeats.
References
1. Schaap N, Schattenberg A, Bär B, Preijers F, Kessel AG, Maazen RVD, et al. Outcome of Transplantation for Standard-Risk Leukaemia With Grafts Depleted of Lymphocytes After Conditioning With an Intensified Regimen. Br J Haematol (1997) 98(3):750–9. doi: 10.1046/j.1365-2141.1997.d01-3499.x
2. Schaap N, Schattenberg A, Mensink E, Preijers F, Hillegers M, Knops R, et al. Long-Term Follow-Up of Persisting Mixed Chimerism After Partially T Cell-Depleted Allogeneic Stem Cell Transplantation. Leukemia (2002) 16(1):13–21. doi: 10.1038/sj.leu.2402343
3. Bär BM, Schattenberg A, De Man AJ, Hoogenhout MJ, Boezeman J, de Witte T. Influence of the Conditioning Regimen on Erythrocyte Chimerism, Graft-Versus-Host Disease and Relapse After Allogeneic Transplantation With Lymphocyte Depleted Marrow. Bone Marrow Transpl (1992) 10(1):45–52.
4. Briones J, Urbano-Ispizua A, Lawler M, Rozman C, Gardiner N, Marín P, et al. High Frequency of Donor Chimerism After Allogeneic Transplantation of CD34+-Selected Peripheral Blood Cells. Exp Hematol (1998) 26(5):415–20.
5. Bertheas MF, Lafage M, Levy P, Blaise D, Stoppa AM, Viens P, et al. Influence of Mixed Chimerism on the Results of Allogeneic Bone Marrow Transplantation for Leukemia. Blood (1991) 78(11):3103–6. doi: 10.1182/blood.V78.11.3103.3103
6. Martin PJ. Determinants of Engraftment After Allogeneic Marrow Transplantation. Blood (1992) 79(7):1647–50. doi: 10.1182/blood.V79.7.1647.1647
7. Andreani M, Manna M, Lucarelli G, Tonucci P, Agostinelli F, Ripalti M, et al. Persistence of Mixed Chimerism in Patients Transplanted for the Treatment of Thalassemia. Blood (1996) 87(8):3494–9. doi: 10.1182/blood.V87.8.3494.bloodjournal8783494
8. Andreani M, Nesci S, Lucarelli G, Tonucci P, Rapa S, Angelucci E, et al. Long-Term Survival of Ex-Thalassemic Patients With Persistent Mixed Chimerism After Bone Marrow Transplantation. Bone Marrow Transpl (2000) 25(4):401–4. doi: 10.1038/sj.bmt.1702151
9. Baron F, Sandmaier BM. Chimerism and Outcomes After Allogeneic Hematopoietic Cell Transplantation Following Nonmyeloablative Conditioning. Leukemia (2006) 20(10):1690–700. doi: 10.1038/sj.leu.2404335
10. Lamba R, Abella E, Kukuruga D, Klein J, Savasan S, Abidi MH, et al. Mixed Hematopoietic Chimerism at Day 90 Following Allogenic Myeloablative Stem Cell Transplantation Is a Predictor of Relapse and Survival. Leukemia (2004) 18(10):1681–6. doi: 10.1038/sj.leu.2403468
11. Koreth J, Kim HT, Nikiforow S, Milford EL, Armand P, Cutler C, et al. Donor Chimerism Early After Reduced-Intensity Conditioning Hematopoietic Stem Cell Transplantation Predicts Relapse and Survival. Biol Blood Marrow Transpl (2014) 20(10):1516–21. doi: 10.1016/j.bbmt.2014.05.025
12. Bornhäuser M, Oelschlaegel U, Platzbecker U, Bug G, Lutterbeck K, Kiehl MG, et al. Monitoring of Donor Chimerism in Sorted CD34+ Peripheral Blood Cells Allows the Sensitive Detection of Imminent Relapse After Allogeneic Stem Cell Transplantation. Haematologica (2009) 94(11):1613–7. doi: 10.3324/haematol.2009.007765
13. Platzbecker U, Wermke M, Radke J, Oelschlaegel U, Seltmann F, Kiani A, et al. Azacitidine for Treatment of Imminent Relapse in MDS or AML Patients After Allogeneic HSCT: Results of the RELAZA Trial. Leukemia (2012) 26(3):381–9. doi: 10.1038/leu.2011.234
14. Rosenow F, Berkemeier A, Krug U, Müller-Tidow C, Gerss J, Silling G, et al. CD34(+) Lineage Specific Donor Cell Chimerism for the Diagnosis and Treatment of Impending Relapse of AML or Myelodysplastic Syndrome After Allo-SCT. Bone Marrow Transpl (2013) 48(8):1070–6. doi: 10.1038/bmt.2013.2
15. Deeg HJ, Salit RB, Monahan T, Schoch G, McFarland C, Scott BL, et al. Early Mixed Lymphoid Donor/Host Chimerism Is Associated With Improved Transplant Outcome in Patients With Primary or Secondary Myelofibrosis. Biol Blood Marrow Transplant J Am Soc Blood Marrow Transpl (2020) 26(12):2197–203. doi: 10.1016/j.bbmt.2020.07.013
16. McSweeney PA, Niederwieser D, Shizuru JA, Sandmaier BM, Molina AJ, Maloney DG, et al. Hematopoietic Cell Transplantation in Older Patients With Hematologic Malignancies: Replacing High-Dose Cytotoxic Therapy With Graft-Versus-Tumor Effects. Blood (2001) 97(11):3390–400. doi: 10.1182/blood.V97.11.3390
17. Maris MB, Niederwieser D, Sandmaier BM, Storer B, Stuart M, Maloney D, et al. HLA-Matched Unrelated Donor Hematopoietic Cell Transplantation After Nonmyeloablative Conditioning for Patients With Hematologic Malignancies. Blood (2003) 102(6):2021–30. doi: 10.1182/blood-2003-02-0482
18. Spitzer TR, McAfee S, Sackstein R, Colby C, Toh HC, Multani P, et al. Intentional Induction of Mixed Chimerism and Achievement of Antitumor Responses After Nonmyeloablative Conditioning Therapy and HLA-Matched Donor Bone Marrow Transplantation for Refractory Hematologic Malignancies. Biol Blood Marrow Transplant J Am Soc Blood Marrow Transplant (2000) 6(3A):309–20. doi: 10.1016/S1083-8791(00)70056-5
19. Sachs DH, Kawai T, Sykes M. Induction of Tolerance Through Mixed Chimerism. Cold Spring Harb Perspect Med (2014) 4(1):a015529. doi: 10.1101/cshperspect.a015529
20. Thiede C, Bornhäuser M, Oelschlägel U, Brendel C, Leo R, Daxberger H, et al. Sequential Monitoring of Chimerism and Detection of Minimal Residual Disease After Allogeneic Blood Stem Cell Transplantation (BSCT) Using Multiplex PCR Amplification of Short Tandem Repeat-Markers. Leukemia (2001) 15(2):293–302. doi: 10.1038/sj.leu.2401953
21. Thiede C, Bornhäuser M, Ehninger G. Evaluation of STR Informativity for Chimerism Testing–Comparative Analysis of 27 STR Systems in 203 Matched Related Donor Recipient Pairs. Leukemia (2004) 18(2):248–54. doi: 10.1038/sj.leu.2403212
22. Scheel AH, Dietel M, Heukamp LC, Jöhrens K, Kirchner T, Reu S, et al. Harmonized PD-L1 Immunohistochemistry for Pulmonary Squamous-Cell and Adenocarcinomas. Mod Pathol (2016) 29(10):1165–72. doi: 10.1038/modpathol.2016.117
23. Harris AC, Young R, Devine S, Hogan WJ, Ayuk F, Bunworasate U, et al. International, Multicenter Standardization of Acute Graft-versus-Host Disease Clinical Data Collection: A Report from the Mount Sinai Acute GVHD International Consortium. Biol Blood Marrow Transpl (2016) 22(1):4–10.
24. Jagasia MH, Greinix HT, Arora M, Williams KM, Wolff D, Cowen EW, et al. National Institutes of Health Consensus Development Project on Criteria for Clinical Trials in Chronic Graft-Versus-Host Disease: I. The 2014 Diagnosis and Staging Working Group Report. Biol Blood Marrow Transpl (2015) 21(3):389–401.e1.
25. Görner M, Kordelas L, Thalheimer M, Luft T, Pfeiffer S, Ustaoglu F, et al. Stable Mixed Chimerism After T Cell-Depleted Allogeneic Hematopoietic Stem Cell Transplantation Using Conditioning With Low-Dose Total Body Irradiation and Fludarabine. Bone Marrow Transpl (2002) 29(7):621–4. doi: 10.1038/sj.bmt.1703427
26. Grimaldi F, Potter V, Perez-Abellan P, Veluchamy JP, Atif M, Grain R, et al. Mixed T Cell Chimerism After Allogeneic Hematopoietic Stem Cell Transplantation for Severe Aplastic Anemia Using an Alemtuzumab-Containing Regimen Is Shaped by Persistence of Recipient CD8 T Cells. Biol Blood Marrow Transplant J Am Soc Blood Marrow Transpl (2017) 23(2):293–9. doi: 10.1016/j.bbmt.2016.11.003
27. Spinner MA, Kennedy VE, Tamaresis JS, Lavori PW, Arai S, Johnston LJ, et al. Nonmyeloablative TLI-ATG Conditioning for Allogeneic Transplantation: Mature Follow-Up From a Large Single-Center Cohort. Blood Adv (2019) 3(16):2454–64. doi: 10.1182/bloodadvances.2019000297
28. Juliusson G, Theorin N, Karlsson K, Frödin U, Malm C. Subcutaneous Alemtuzumab vs ATG in Adjusted Conditioning for Allogeneic Transplantation: Influence of Campath Dose on Lymphoid Recovery, Mixed Chimerism and Survival. Bone Marrow Transpl (2006) 37(5):503–10. doi: 10.1038/sj.bmt.1705263
29. Soiffer RJ, LeRademacher J, Ho V, Kan F, Artz A, Champlin RE, et al. Impact of Immune Modulation With Anti–T-Cell Antibodies on the Outcome of Reduced-Intensity Allogeneic Hematopoietic Stem Cell Transplantation for Hematologic Malignancies. Blood (2011) 117(25):6963–70. doi: 10.1182/blood-2011-01-332007
30. Federmann B, Bornhauser M, Meisner C, Kordelas L, Beelen DW, Stuhler G, et al. Haploidentical Allogeneic Hematopoietic Cell Transplantation in Adults Using CD3/CD19 Depletion and Reduced Intensity Conditioning: A Phase II Study. Haematologica (2012) 97(10):1523–31. doi: 10.3324/haematol.2011.059378
31. Fontenot JD, Gavin MA, Rudensky AY. Foxp3 Programs the Development and Function of CD4+CD25+ Regulatory T Cells. Nat Immunol (2003) 4(4):330–6. doi: 10.1038/ni904
32. Walker MR, Kasprowicz DJ, Gersuk VH, Benard A, Van Landeghen M, Buckner JH, et al. Induction of FoxP3 and Acquisition of T Regulatory Activity by Stimulated Human CD4+CD25- T Cells. J Clin Invest (2003) 112(9):1437–43. doi: 10.1172/JCI19441
33. Kinsella FAM, Zuo J, Inman CF, Pearce H, Maggs L, Eldershaw SE, et al. Mixed Chimerism Established by Hematopoietic Stem Cell Transplantation Is Maintained by Host and Donor T Regulatory Cells. Blood Adv (2019) 3(5):734–43. doi: 10.1182/bloodadvances.2018025502
34. Lopez M, Clarkson MR, Albin M, Sayegh MH, Najafian N. A Novel Mechanism of Action for Anti-Thymocyte Globulin: Induction of CD4+CD25+Foxp3+ Regulatory T Cells. J Am Soc Nephrol (2006) 17(10):2844–53. doi: 10.1681/ASN.2006050422
35. Shimony O, Nagler A, Gellman YN, Refaeli E, Rosenblum N, Eshkar-Sebban L, et al. Anti-T Lymphocyte Globulin (ATG) Induces Generation of Regulatory T Cells, at Least Part of Them Express Activated CD44. J Clin Immunol (2012) 32(1):173–88. doi: 10.1007/s10875-011-9599-2
36. Servais S, Menten-Dedoyart C, Beguin Y, Seidel L, Gothot A, Daulne C, et al. Impact of Pre-Transplant Anti-T Cell Globulin (ATG) on Immune Recovery After Myeloablative Allogeneic Peripheral Blood Stem Cell Transplantation. PloS One (2015) 10(6):e0130026. doi: 10.1371/journal.pone.0130026
37. Edinger M, Hoffmann P, Ermann J, Drago K, Fathman CG, Strober S, et al. CD4+CD25+ Regulatory T Cells Preserve Graft-Versus-Tumor Activity While Inhibiting Graft-Versus-Host Disease After Bone Marrow Transplantation. Nat Med (2003) 9(9):1144–50. doi: 10.1038/nm915
Keywords: chimerism, mixed donor chimerism, allogeneic hematopoietic cell transplantation, myeloid neoplasms, AML, CML
Citation: Ruhnke L, Stölzel F, Oelschlägel U, von Bonin M, Sockel K, Middeke JM, Röllig C, Jöhrens K, Schetelig J, Thiede C and Bornhäuser M (2021) Long-Term Mixed Chimerism After Ex Vivo/In Vivo T Cell-Depleted Allogeneic Hematopoietic Cell Transplantation in Patients With Myeloid Neoplasms. Front. Oncol. 11:776946. doi: 10.3389/fonc.2021.776946
Received: 14 September 2021; Accepted: 08 November 2021;
Published: 07 December 2021.
Edited by:
Cyrus Khandanpour, University Hospital Münster, GermanyReviewed by:
Everett Meyer, Stanford University, United StatesNatasha A. Jain, Deaconess Henderson Clinic, United States
Shigeo Fuji, Osaka International Cancer Institute, Japan
Copyright © 2021 Ruhnke, Stölzel, Oelschlägel, von Bonin, Sockel, Middeke, Röllig, Jöhrens, Schetelig, Thiede and Bornhäuser. This is an open-access article distributed under the terms of the Creative Commons Attribution License (CC BY). The use, distribution or reproduction in other forums is permitted, provided the original author(s) and the copyright owner(s) are credited and that the original publication in this journal is cited, in accordance with accepted academic practice. No use, distribution or reproduction is permitted which does not comply with these terms.
*Correspondence: Leo Ruhnke, bGVvLnJ1aG5rZUB1a2RkLmRl