- 1Dipartimento di Scienze Radiologiche, Oncologiche ed Anatomo Patologiche, Università di Roma Sapienza, Rome, Italy
- 2UOSD Sperimentazioni Di Fase IV, Istituto di Ricerca e Cura a Carattere Scientifico (IRCCS) Regina Elena National Cancer Institute, Rome, Italy
- 3Gynecologic Oncology Program, European Institute of Oncology, Istituto di Ricerca e Cura a Carattere Scientifico (IRCCS), Milan, Italy
- 4Maternal and Child Department, Sapienza University of Rome, Rome, Italy
Triple negative tumors represent 15% of breast cancer and are characterized by the lack of estrogen receptors, progesterone receptor, and HER2 amplification or overexpression. Approximately 25% of patients diagnosed with triple negative breast cancer carry a germline BRCA1 or BRCA2 mutation. They have an aggressive biology, and chemotherapy has been the mainstay of treatment for a long time. Despite intensive therapies, prognosis is still poor, and many patients will eventually relapse or die due to cancer. Therefore, novel targeted agents that can increase the treatment options for this disease are urgently needed. Recently, a new class of molecules has emerged as a standard of care for patients with triple negative breast cancer and germline BRCA1 or BRCA2 mutation: poly (ADP-ribose) (PARP) inhibitors. In the first part of the review, we summarize and discuss evidence supporting the use of PARP inhibitors. Currently, two PARP inhibitors have been approved for triple negative metastatic breast cancer—olaparib and talazoparib—based on two phase III trials, which showed a progression-free survival benefit when compared to chemotherapy. Safety profile was manageable with supportive therapies and dose reductions/interruptions. In addition, other PARP inhibitors are currently under investigation, such as talazoparib, rucaparib, and veliparib. Subsequently, we will discuss the potential role of PARP inhibitors in the future. Clinical research areas are investigating PARP inhibitors in combination with other agents and are including patients without germline BRCA mutations: ongoing phase II/III studies are combining PARP inhibitors with immunotherapy, while phases I and II trials are combining PARP inhibitors with other targeted agents such as ATM and PIK3CA inhibitors. Moreover, several clinical trials are enrolling patients with somatic BRCA mutation or patients carrying mutations in genes, other than BRCA1/2, involved in the homologous recombination repair pathway (e.g., CHECK2, PALB2, RAD51, etc.).
Introduction
Although survival rates are constantly improving because of the current strategies of primary/secondary prevention and the availability of innovative and personalized therapeutic challenges, breast cancer (BC) is still the most frequent malignant neoplasia and the leading cause of cancer-related lethality among women worldwide today. Moreover, it is also the second most common cancer in the world (1–3). According to these data, BC constitutes one of the greatest health emergencies in Western countries today, pushing the health authorities to commit enormous resources to fight against this cancer. In 2020, an estimated 276,480 new cases of female breast cancer will be diagnosed in the US, and 42,170 metastatic BC patients are expected to die due to this disease. Some biological, epidemiological, and clinical aspects of BC deserve to be better investigated in order to explain the many differences occurring in clinical practice: geographic distribution of BC, reasons for the increasing early onset in young women, unexpected severe poor outcome in some patients with favorable prognostic factors, different levels of availability of targeted agents, and frequent occurrence of orphan drug diseases. In this context, a better understanding of the molecular portraits of BC in the last years has played a prominent role in order to improve our knowledge about a tailored BC clinical approach. Moreover, this speculative and investigative strategy could identify other novel molecular targets (beyond estrogen receptors, HER-2, and PIK3CA) that could better inhibit BC growth and diffusion, mainly in association with currently used drugs. BC is a heterogeneous disease, with different profiles of gene expression and amplifications determining great differences in prognosis and therapeutic strategies (4–6). In 2000, Perou et al. showed, by analyzing 8,102 different genes, that the phenotypic diversity of BC corresponded to specific gene expression profiles (4). This study identified four different molecular portraits that might be related to the specific molecular features of mammary epithelial biology: ER+/luminal-like, basal-like, Erb-B2 enriched, and normal breast.
Triple negative breast cancer (TNBC) represents about 14–16% of all BC patients and is characterized by lack of estrogen receptor (ER), progesterone receptor (PR), and HER2 expression. Lehmann et al. analyzed the gene expression profiles of BC patients by analyzing 21 databases and suggesting that a high and unexpected heterogeneity distinguishes TNBC from other BC tumors (7, 8). The authors suggested six different TNBC subtypes: two basal-like types (BL 1–2), a mesenchymal type (MES), an immunomodulatory, a mesenchymal stem-like, and a luminal androgen receptor (LAR). According to these gene analyses, TNBC shows partial correlations with basal-like type BC (discordance of about 20–30%). In a different study, other authors proposed four distinguished TNBC subtypes: LAR, MES, basal-like immunosuppressed, and basal-like immune-activated (9). However, despite the increasing knowledge of TNBC biology, there are no evidence to support their use in clinical practice for treatment selection. TNBC is an aggressive disease and frequently associated with early and distant recurrence, occurrence of visceral metastases, and higher risk of death compared to other BC types. Moreover, metastatic recurrence is constantly related with a short progressive disease and premature occurrence of death (usually, the median survival of advanced TNBC is not longer than 12 months) (10–14). TNBCs are usually basal-like, and they express a claudin-low condition and present high levels of cancer stem cells (15–18), which could explain their aggressive clinical behavior.
A chemotherapeutic approach has been considered for a long time as the most active and efficient systemic treatment for metastatic TNBC (19–24). Because TNBC frequently demonstrates an important immunogenic profile, a high number of tumor-infiltrating lymphocytes, and a high level of PD-L1 expression (25, 26), it has been possibly considered the most suitable BC subtype for immunotherapy. In fact, the combination of chemotherapy and immune-checkpoint inhibitors (ICIs) has shown superior efficacy in terms of progression-free survival (PFS) and overall survival (OS) when compared to chemotherapy in monotherapy and currently represents the standard of care for patients with PD-L1 positive metastatic TNBC (17, 27). In addition, TNBC could benefit also from other chemotherapeutic drugs, such as capecitabine and eribulin, in different settings (28–31).
The possibility to treat TNBC with other novel targeted agents have recently emerged in order to evaluate the relationship between this BC subtype and the occurrence of deleterious BRCA1 and BRCA2 mutations. On one hand, approximately 70% of BRCA1-2-mutated BC patients express TNBC subtype, and on the other hand, 10–20% of all TNBC are BRCA1/2 mutation carriers (32–34), regardless of family history (35).
According to these data and considering that some PARP inhibitors (PARPib) are FDA-approved (olaparib and talazoparib) for the treatment of BRCA-associated BC (36–39), an increased interest has emerged to evaluate their activity and safety specifically in TNBC patients with BRCA1/2 mutations. Moreover, other PARPib (niraparib, rucaparib, and veliparib) are being investigated in large randomized clinical trials in order to assess their activity as single agents or in combination with other drugs (chemotherapy, ICIs, and targeted molecules).
This review summarizes the current evidence supporting the use of PARPib in BRCA-mutated TNBC patients and focuses on new potential strategies to improve their outcomes and therapeutic opportunities.
The Rational Behind PARP Inhibitors: The Synthetic Lethality
DNA damage represents one of the leading processes of carcinogenesis and can occur through different mechanisms: single-strand breaks (SSB), helix-distorting damage, replication errors, and double-strand breaks (DSB). Specifically, DSB are considered one of the most cytotoxic types of DNA damage, so it is not a surprise that normal cells have developed multiple pathways to repair it. Among the DSB repair pathways, a key role is played by homologous recombination (HR) and nonhomologous end-joining (NHEJ) (40, 41). On the other hand, SSB, helix distorting damage, and replication errors are corrected by base excision repair, nucleotide excision repair, and mismatch repair, respectively.
Poly(ADP-ribose) polymerases (PARP) are a large family of multifunctional enzymes with a key role in base excision repair mechanism (42). Eighteen members have been identified, among which PARP-1 is the most important, while PARP2 and PARP3 are less involved. PARP-1 is essential for SSB repair, and it plays a dominant role in genome integrity (43). In particular, PARP-1 detects the damage of DNA and catalyzes the so-called PARylation, which is the addition of a poly-ADP-ribose (PAR) chain to target proteins in order to recruit additional repair factors on the damaged DNA (Figure 1A) (44, 45).
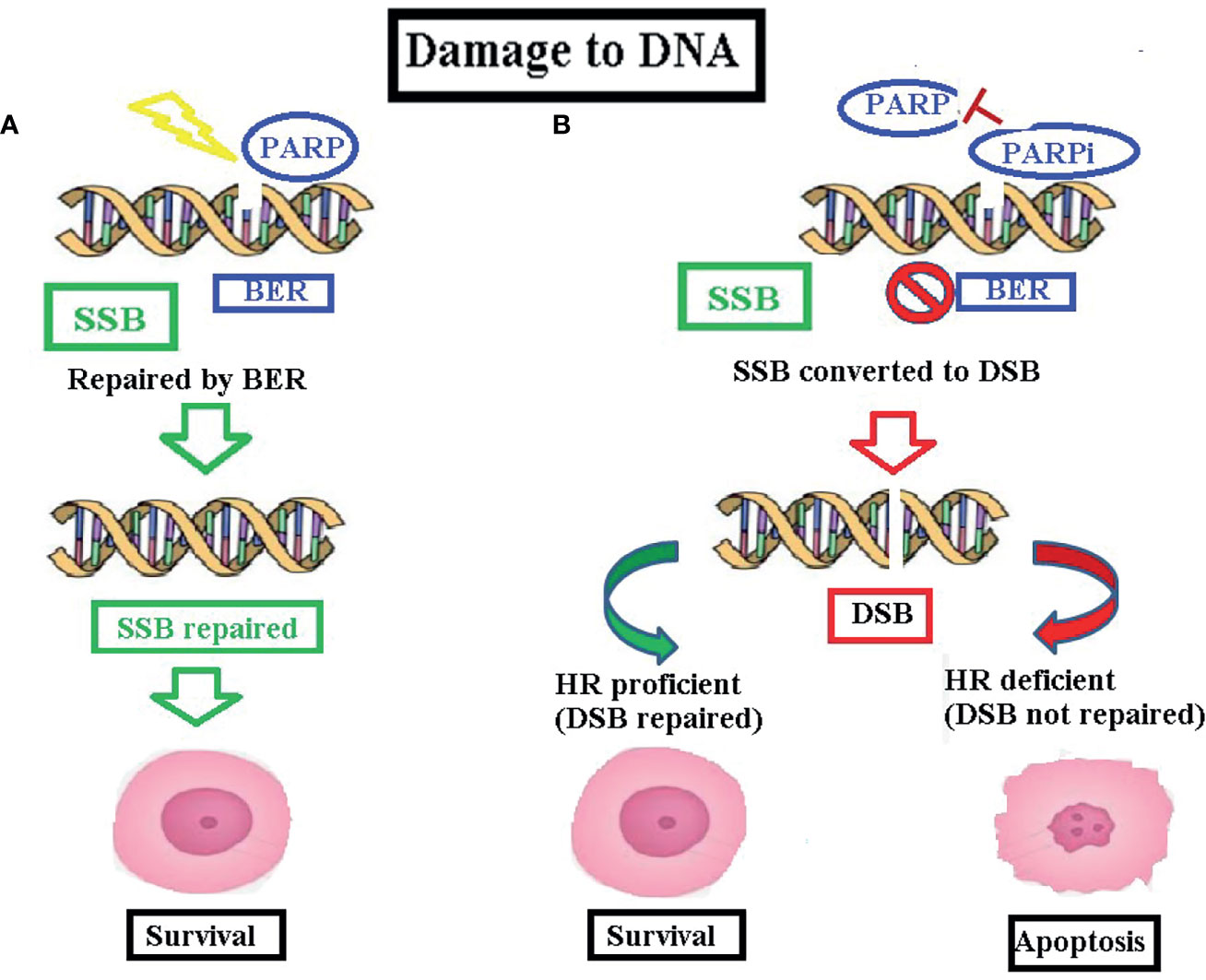
Figure 1 (A) PARP mechanism of action: PARP enzymes are key components in base excision repair, a DDR pathway which deals with SSB. In case of SSB DNA damage, PARP enzymes attach to the damaged DNA and allow NAD+ to bind to its active site. ADP-ribose moieties from NAD+ are transferred to target proteins (PARylation), which recruit single-strand DNA repair effectors. After the DNA damage has been repaired, PARP autoPARylates, returning to a catalytic state of inactivation. (B) PARP inhibitor mechanism of action: the synthetic lethality—PARPib are a class of molecules which prevent SSB repair. If SSB damage cannot be repaired, the immediate consequence is DSB formation. In cells with a proficient HRR pathway, HRR effectors (among which BRCA1 and BRCA2 play a crucial role) repair DSB, allowing cell survival. In tumor cells with HRR deficiency treated with PARPib, concomitant inhibition of base excision repair and HRR lack of function cause a progressive accumulation of DNA alterations which ultimately leads to cell apoptosis. DDR, damaged DNA repair; DSB, double-strand breaks; HRR, homologous recombination repair; PARPib, PARP inhibitors; SSB, single-strand breaks.
More recently, increasing evidences have shown that PARP can also be involved in DSB repair: PARP-1 recruits MRE11 and NS1 enzymes which are crucial in HR pathways (46) by opening the chromatin structure to give access to repair proteins.
Cancer cells affected by deleterious mutation in breast cancer susceptibility genes 1 or 2 (BRCA 1/2) are deficient in the DNA DSB repair. In fact, both BRCA1 and BRCA 2 are key components in the homologous recombination repair (HRR) pathway (47). BRCA 1 is a multifunctional enzyme with a direct involvement in HRR: with CHK2, it is initially responsible for signal transduction; after that, DNA double strand damage is recognized by ATM and ATR (47). Subsequently, it acts by forming a structure which organizes repair proteins at the DNA repair site (48, 49). BRCA 2, on the contrary, recruits RAD51 (a recombinase) at the DNA repair site (50). Therefore, tumors with BRCA 1 and BRCA2 inactivation are highly dependent on the repair pathway for SSB (51–53). Consequently, if other events occur that can impair DNA damage repair, the damage can lead to a progressive accumulation of DNA alterations which can ultimately lead to apoptosis (Figure 1B) (54, 55).
The aforementioned mechanism represents the core concept of synthetic lethality: an interaction between two genes in which the mutation of either gene alone is compatible with viability, while the simultaneous mutation of both genes causes death (56–58). PARPib are the first clinically approved drugs designed to exploit synthetic lethality, showing promising activity in patients with BRCA deficient tumors (53).
PARPib exert their functions through different systems: initially, it was believed that their principal mechanism of action consisted of “catalytic inhibition”: a competing bind to the PARP1 and PARP2 catalytic domains which displaces nicotinamide adenine ribonucleoside (NAD+) from its active site, thus preventing the recruitment of single-strand DNA repair effectors (59, 60). More recently, it has been demonstrated that PARPib act mostly by inhibiting the PARylation mechanism which induces the trapping at the site of DNA damage, the activation of effector genes, and consequently the interruption of the replication fork by leading to a DSB damage responsible for a cytotoxic effect (61). Accordingly, preclinical models showed that trapping DNA on PARP could be more effective in inducing cell death than catalytic enzyme alone (43, 60). Thus, in tumors harboring a defect in the HRR pathway, contemporary inhibition of PARP enzymes causes the accumulation of unpaired damages, leading to tumor cell death. On the contrary, healthy cells can be spared, thus providing a clinical benefit in patients with BRCA 1 or 2 mutation (62). The capacity of PARP trapping is different among PARPib and is independent from catalytic inhibition (43, 60, 63, 64). This difference can partially explain the different clinical activity and safety profile of PARPib.
At this time, two PARPib have been approved for the treatment of patients with TNBC in the metastatic setting: olaparib and talazoparib. Olaparib is a small molecule, which was initially described as a PARP-1 and PARP-2 inhibitor but for which recent data showed also a potent PARP-3 inhibition (65). Talazoparib, on the contrary, is a potent PARP inhibitor, with both strong catalytic inhibition and PARP trapping potential (preclinical models showed that the trapping potential of talazoparib is 100 times higher than the other PARPib) (63).
Clinical Evidence of PARP Inhibitors in Triple Negative Breast Cancer
Olaparib and talazoparib are currently approved as monotherapy for the treatment of metastatic TNBC harboring a germline BRCA (gBRCA) 1 or 2 mutation based on the results of two phase III trials: OlympiAD (37, 66) and EMBRACA (36).
OlympiAD Trial
The OlympiAD trial enrolled 302 metastatic breast cancer patients with both triple negative (TN) (49.8%) and hormone receptor positive (HR+) HER2 negative (50.2%) tumors. Not more than two lines of chemotherapy for metastatic disease were permitted. Pre-treatment with platinum was allowed, but the last dose should have been administered at least 12 months before randomization. The patients were randomized in a 2:1 ratio to receive olaparib, 300 mg bid, monotherapy or treatment physician’s choice (TPC) among capecitabine, eribulin, or vinorelbine. The primary endpoint was PFS. The secondary endpoint included OS, overall response rate (ORR), and health-related quality of life (HRQoL). The primary analysis showed that PFS was significantly longer in the olaparib arm than the standard chemotherapy (7.0 vs. 4.2 months; HR: 0.58; 95% CI: 0.43 to 0.80; p < 0.001). ORR was also higher in the olaparib group than in the standard chemotherapy group (59.8 vs. 29.8%). OS, on the contrary, did not differ from the two arms (HR for death, 0.90; 95% CI, 0.63 to 1.29; P = 0.57), but the trial was not powered to assess OS differences. In the forest plot, HR was lower in the TN subgroup than in the HR+ subgroup (0.43 vs. 0.82). Finally, olaparib had a good safety profile: there were fewer grade 3 events and fewer discontinuations related to an adverse event in the olaparib arm than in the chemotherapy arm. The side effects reported were comparable to previously published phase I and II trials with anemia, nausea, vomiting, fatigue, headache, and cough occurring more frequently in the olaparib group than in the standard therapy group (37).
The planned study final analysis with OS update has been recently published (66). Overall, OS was not improved by olaparib treatment compared to standard chemotherapy (19.3 months with olaparib versus 17.1 months with TPC HR: 0.90, 95% CI: 0.66–1.23; p = 0.513); however, when patients were stratified according to pre-defined subgroups, an OS benefit was observed in patients who had not received prior chemotherapy for metastatic disease (first-line treatment, 22.6 versus 14.7 months; HR: 0.51; 95% CI: 0.29–0.90). Safety data were also updated: no new findings were reported. Overall, the incidence of grade 3 adverse events was 38%, while 5% of patients discontinued olaparib because of toxicity.
Another important secondary endpoint of the OlympiAD trial was the quality of life (QoL) of the patients. Investigators employed the European Organisation for Research and Treatment of Cancer Quality-of-Life Questionnaire Core 30-item module (EORTC QLQ-C30) to assess patient global health status/QoL. The final results (67) showed a significant QoL improvement in the olaparib arm compared to the TPC arm with a mean change of 3.9 (standard deviation 1.2) versus -3.6 (2.2), a difference of 7.5 points (95% confidence interval, CI: 2.48, 12.44; p = 0.0035). In addition, for EORTC QLQ-C30 symptoms and functioning subscales, only the nausea/vomiting symptom score was worse in the olaparib arm than in the TPC arm (across all visits compared with baseline) (68).
An extended follow-up exploratory analysis of the OlympiAD trial was presented at the San Antonio Breast Cancer Symposium in December 2019 (69). The median follow-up was 18.9 vs. 15.5 months in the olaparib vs. the TPC arms, respectively. Median study treatment duration was 8.3 months in the olaparib arm vs. 3.5 months in the TPC arm, and in the olaparib arm, 8.8% of patients received the treatment for more than 3 years, while no one did in the TPC arm. The results of the extended follow-up confirmed previously published results: no OS differences were registered between the two arms in the overall population (19.3 months for olaparib vs. 17.1 months in the TPC arm, HR: 0.84, 95% CI: 0.63–1.12), but an OS benefit was detected in the subgroup of patients treated with olaparib who had not received chemotherapy for metastatic setting (first-line treatment: OS 22.6 month in the olaparib arm vs. 14.7 months in the TPC arm, HR: 0.54, 95% CI: 0.32–0.92). In first-line subgroup, 40.8% of patients in the olaparib arm were alive at 3 years compared with 12.8% of patients in the TPC arm. No new safety data and no cumulative toxicity occurred at the extended follow-up analysis, confirming good olaparib tolerability even in long-term exposure.
EMBRACA Trial
The phase III EMBRACA trial was an open-label, randomized trial, comparing talazoparib versus choice of standard chemotherapy of the physician (capecitabine, eribulin, gemcitabine, or vinorelbine) in pretreated locally advanced (not amenable to curative treatment) or metastatic BRCA1/2 mutated breast cancer (36). A total of 431 patients were randomized in a 2:1 ratio to receive talazoparib at a dose of 1.0 mg daily (n = 287) vs. standard chemotherapy (n = 144). Forty percent of the enrolled patients were TN. No more than three previous chemotherapy regimens were admitted. Patients must have had previously received anthracyclines and taxanes, unless clinically contraindicated. Previous platinum-based adjuvant or neoadjuvant chemotherapy was admitted only if the patients had a disease-free interval of at least 6 months from the last platinum dose. The primary endpoint was PFS by blinded independent central review. The secondary endpoints were OS and ORR. Safety and patient-reported outcomes were also assessed.
At a median follow-up of 11.2 months, the EMBRACA trial met its primary endpoint: the median PFS was significantly higher in the talazoparib arm (8.6 months; 95% CI, 7.2–9.3) than in the standard chemotherapy arm (5.6 months; CI, 4.2–6.7). The HR was 0.54 (95% CI, 0.41–0.71; p = 0.001), and it was confirmed by an independent radiologic review. The PFS HRs were consistent among subgroups, specifically, for HR+ and TN. The PFS HR was 0.47, 95% CI: = 0.32 to 0.71 for HR+/HER2− and 0.60, 95% CI: = 0.41 to 0.87 for TN (32, 33). The response rate by the investigators was 62.6% in the talazoparib arm compared with 27.2% in the chemotherapy arm.
At interim analysis, the median OS was longer in the talazoparib arm (22.3 months) than in the chemotherapy arm (19.5 months), but it did not reach statistical significance (HR: 0.76; 95% CI, 0.55–1.06, p = 0.11). Data about safety showed that the most common all-grade adverse events for talazoparib were anemia, fatigue, and nausea, while for chemotherapy nausea, fatigue, and neutropenia were more frequent. Grade 3 or 4 hematologic adverse events occurred in 55 vs. 36.1% of patients in the talazoparib and standard chemotherapy arms, respectively. Grade 3 non-hematologic adverse events occurred in 32 vs. 38% of patients in the talazoparib arm and in the standard chemotherapy arm, respectively. However, discontinuation rate due to an adverse event was low: 5.9% in the talazoparib group vs. 8.7% in the standard chemotherapy group. Moreover, talazoparib significantly delayed the onset of a clinically meaningful deterioration of global health status of QoL questionnaire, and it also significantly delayed deterioration according to breast symptom scale compared to chemotherapy (70).
In the final OS analysis, published after that 75% of the events occurred (324 patients), talazoparib showed no OS benefit compared to chemotherapy: median OS was 19.3 months (16.6–22.5 months) versus 19.5 months (17.4–22.4 months); HR: 0.848 (95% CI: 0.670–1.073; P = 0.17). A possible explanation for the lack of OS benefit relies on subsequent treatment that could have impaired the analysis: 32.6% of patients randomized to TPC received a PARP inhibitor in later lines of treatment (at the time of EMBRACA publication, olaparib had already been approved for metastatic breast cancer patients harboring gBRCA 1/2 mutation) (71).
More recently, a Cochrane metanalysis investigated the efficacy of PARPib in metastatic breast cancer patients with BRCA 1 or 2 mutations (72). The primary outcome was OS, while the secondary outcomes were PFS, tumor response rate, and safety. The authors included five trials involving 1,474 patients. PARPib showed a small OS benefit: HR: 0.87 (95% CI: 0.76 to 1.00; P = 0.05; high-certainty evidence), with no significant heterogeneity (I2 = 0%, P =0.81). Unfortunately, subgroup analysis could not be performed because data were not available for the included trials. On the contrary, PARPib significantly prolonged PFS with a HR of 0.63 (95% CI: 0.56 to 0.71; P < 0.00001; high-certainty evidence), with no significant heterogeneity (I2 = 2%, P = 0.39). For patients with TNBC (N = 664, four randomized controlled trials, RCTs), there was evidence of PFS benefit on pooling of studies (HR: 0.61, 95% CI: 0.47 to 0.80; P = 0.0003), with moderate heterogeneity (I2 = 44%, P = 0.15).
In addition to olaparib and talazoparib, other PARPib are currently under investigation in TNBC: rucaparib, niraparib, and veliparib. Of note is that the phase III Bravo trial, which investigated the role of niraparib versus TPC in BRCA mutated breast cancer, was prematurely closed because of high discontinuation rate in the control arm (the patients enrolled in the control arm did not continue the trial long enough to receive their first radiological scan, which is required to assess disease progression, resulting in an unusually high rate of censoring) (68). A complete list of other published trials (73) and monotherapy ongoing trials of PARPib in TNBC is summarized in Tables 1, 2 (74, 75, 80, 81).
Future Perspective and Ongoing Clinical Trials
Despite the fact that the role of PARPib as a therapeutic milestone is now confirmed in the management of BRCA-mutant TNBC, approximately 50% of patients progressed during treatment (76). From preclinical studies, four principal mechanisms of resistance have been identified (77): (i) the influence of cellular availability of the inhibitor, mainly by overexpression of drug-efflux transporter genes; (ii) direct impact on the activity and abundance of PAR chains due to PARP1 mutations that diminish trapping of the protein on DNA or the loss of PAR glycohydrolase, which is responsible for the degradation of PAR chains; (iii) the occurrence of “reversion mutations” that lead to the reactivation of both BRCA1/2 function and of HR by the activation of a specific protein complex (53BP1–RIF1–Shieldin axis); and (iv) influence of replication fork protection, mainly due to the attack by MRE11 and MUS81 nucleases.
Although the clinical relevance of this issue needs to be proven, some new drugs are engineered to target the acquired vulnerabilities of resistant tumors, thus restoring PARPib sensitivity.
Overall, PARPib showed improved PFS and response rate compared with standard chemotherapy, but no difference in OS was observed in those studies (72). Thus, the development of new agents and/or combination strategies are urgently needed to overcome PARPib resistance and to better understand TNBC molecular aspects. Several ongoing clinical trials aiming at evaluating the safety and efficacy of PARPib in combination with immune checkpoint inhibitors (78, 79, 82–90), chemotherapy (91–94), or target agents (95–100) for advanced BC (including TNBC) are summarized in Tables 3–5. Particularly promising are the data that emerged with combinations of PARPib and immunotherapy according to the durable response rates (101–103).
PARP Inhibitors in Combination With Immune Checkpoint Inhibitors
The combination between a PARPi and ICI is based on the evidence of the interaction between the abnormal presence of unrepaired DNA in the cytoplasm of TN tumor cells and the activation of the stimulator of interferon genes pathway which leads to the release of interferons and enhances T-cell infiltration inside the tumor (104). Thus, combining ICIs with a PARPib could be a great strategy to improve the antitumor immunity as well as response to treatment. Promising efficacy and safety findings have been reported in two single-armed phase 2 studies: TOPACIO and MEDIOLA for niraparib combined with pembrolizumab and for olaparib plus durvalumab, respectively (78, 79, 102, 103). The TOPACIO trial enrolled 55 patients of whom 15 were with BRCA mutations (103). Overall, an ORR of 21% (47% in patients with BRCA-mutated tumors) and a disease control rate (DCR) of 49% (80% in patients with BRCA mutated tumors) were reported. For the five patients harboring non-BRCA HRR pathway mutations, ORR was 20% (n = 1/5) and DCR was 80% (n = 4/5). In the overall population, ORR was higher in patients with PD-L1-positive TNBC (32%; n = 9/28) than in those with PD-L1-negative tumor (8%; n = 1/13). Despite the relatively small sample size (N = 47 for efficacy, N = 55 for safety), the combination of niraparib and pembrolizumab resulted to be active, regardless of BRCA mutation status, in patients with somatic or gBRCA-mutated and wild-type BRCA advanced/metastatic TNBC. Comparable results were obtained in the MEDIOLA trial where the combination of olaparib and durvalumab was associated with DCRs of 80 and 50% after 12 and 28 weeks, respectively, and a favorable tolerability in patients with gBRCA-mutated metastatic BC (101, 102).
PARP Inhibitors and Chemotherapy
PARPib are also being evaluated in combination with chemotherapeutic agents (91–94). In the phase 3 BROCADE3 trial (N = 509), addition of veliparib to carboplatin and paclitaxel resulted in a significant improvement in median PFS compared with placebo plus carboplatin and paclitaxel (14.5 vs. 12.6 months; HR: 0.71, 95% CI: 0.57–0.88; p = 0.002) in patients with gBRCA-mutated, HER2-negative, locally advanced or metastatic BC (105, 106). The PFS benefit was durable, and no additional toxicities were seen, although there was a high degree of toxicity in both treatment arms (105). However, veliparib appeared to be effective in terms of PFS benefit as monotherapy (HR: 0.49, 95% CI: 0.33–0.73) as well as in combination therapy (HR: 0.81, 95% CI: 0.62–1.06), regardless of the number of treatment cycles. Other ongoing phase II and phase III trials are reported in Table 4.
PARP Inhibitors and Targeted Therapy
Ongoing clinical trials are investigating PARPib in combination with new agents, including DDR molecules (ATR or Wee1 inhibitors). WEE1 is a kinase inhibitor which decreases kinases cyclin-dependent kinase1 (CDK1) expression, subsequently followed by activating replication firing and DSB repair (107). HR is scheduled but weakened by WEE1 inhibitor through phosphorylation of CDK1 in BRCA1/2-deficient tumor cells (108, 109). The combination of PARP and WEE1 inhibitors arrests G2 phase and results in chromosomal aberration and replication stress, which is proven to have an antitumor activity in numerous preclinical models (110). VIOLETTE is a global, multicenter, open-label, phase II study randomizing 1:1:1 450 patients with advanced TNBC to olaparib alone or in combination with AZD1775 (a WEE1 checkpoint inhibitor) or AZD6738 (an ataxia telangiectasia and Rad3-related protein inhibitor). Patients will be stratified in BRCA-mutated, non-BRCA HRR-mutated, and non-HRR mutated. The primary endpoint is PFS (95).
A two-part, open-label, non-randomized, phase 2, ongoing trial is testing the combination of ZEN003694 (a bromodomain inhibitor) with talazoparib in patients with TNBC without BRCA 1/2 germline mutations. The part 1 of this trial is a dose escalation study, with primary outcome incidence of treatment-related adverse events and treatment-related serious adverse events. The part 2 is a Simon 2-stage design, with primary outcome ORR (97).
The other group of agents that are interesting are the AKT inhibitors: previous research has shown that PI3K inhibitors (PI3Kib) lower nucleotide pools required for DNA synthesis and S-phase progression. Additionally, inhibition of PI3K/mTOR could inhibit PI3K interaction with the homologous recombination complex, increasing the dependency on PARP enzymes for DNA repair (111). Based on this data, the combination of PI3Kib and PARPib could potentially lead to a new, chemotherapy-free treatment option for BRCA wild-type TNBC as well as to improve the modest PFS/OS seen with the PARPib as single agents in BRCA1/2 mutant advanced setting. At the ASCO 2020, two randomized phase 2 studies, LOTUS and PAKT, reported the role of AKT inhibitors in combination with taxanes. Both trials demonstrated some improvement in PFS, with hints toward improvement in OS, in advanced TNBC. The results also showed some suggestions that PTEN loss or a PI3K-altered pathway could be a biomarker to predict who is going to benefit the most AKT inhibitors. Thus, a dual mTOR/PI3K inhibitor (gedatolisib) for metastatic or recurrent/unresectable TNBC could be a promising strategy in combination with talazoparib (98). Finally, the combination of olaparib plus trastuzumab for HER2-positive BC (OPHELIA trial) and a phase I trial with veliparib plus lapatinib are also under evaluation (99, 100). A complete list of clinical trials evaluating the combination of PARPib with other targeted therapies is summarized in Table 5.
PARP Inhibitors in Triple Negative Breast Cancer Beyond BRCA Mutations
Along with BRCA1 and BRCA2, multiple HRR genes, including ATM, BARD1, BRIP1, CHEK2 (encodes CHK2), MRE11A, PALB2, RAD50, RAD51C, and RAD51D, are also implicated in hereditary cancer risk and are recently considered new potential biomarkers in patients with non-gBRCA HRR gene mutations (112).
Clinical studies that showed positive findings for PARPib in settings other than gBRCA-mutated BC include single-arm phase 2 studies of olaparib (113), rucaparib (114), and talazoparib (75) monotherapy (Table 6).
In the olaparib expanded study, in 54 patients with metastatic BC and germline mutations in various non-BRCA DDR genes (cohort 1) or somatic mutations in DDR genes including BRCA (cohort 2), ORR was 33 and 31%, respectively (115). Overall, antitumor activity was reported in patients with somatic BRCA or gPALB2 mutations, but not in those with ATM or CHEK2 mutations. At the ASCO 2020 symposium, a study investigating the role of olaparib in women with HER2-negative breast cancer and a germline alteration in DDR pathway, such as PALB2, CHEK2, and ATM, or a somatic tumor mutation without a germline BRCA1/2 mutation was presented (115). Of the two cohorts, the first included patients with germline mutations other than BRCA. Olaparib demonstrated a high response rate, and the trial met its primary endpoint. Specifically, patients with a germline PALB2 mutation had 80% ORR, whereas in the somatic mutation cohort, patients with a somatic BRCA1/2 mutation reported 50% ORR.
In the RUBY trial, rucaparib monotherapy was investigated in 41 patients with HRD, including four patients harboring somatic BRCA mutations. Five patients (13.5%) demonstrated clinical benefit, comprising three patients with high loss of heterozygosity, one with a somatic BRCA1 mutation, and another patient with a somatic BRCA2 mutation (116).
In the phase 2 study of single-agent talazoparib, patients with BRCA wild-type, HER2-negative, advanced BC and non-BRCA HRR pathway mutations were enrolled. Based on 12 evaluable patients, the ORR and the clinical benefit rate were 25 and 50% after 6 months of treatment, respectively (117). In detail, two-thirds of the responders had gPALB2 mutations; the others had gCHEK2, gFANCA, and somatic PTEN mutations.
According to the above-mentioned reported data, PARPib demonstrated to have a role beyond BRCA2 germline mutation carriers, although the responses seem to be gene specific: the ATM and CHEK2 cohorts seemed not to respond, but the sample size was small.
Discussion
Olaparib and talazoparib are now approved for triple negative metastatic breast cancer patients harboring gBRCA 1 or 2 mutations. Both registered trials (OlympiAD and EMBRACA) showed a consistent PFS benefit when compared to chemotherapy (7.0 versus 4.2 months for olaparib, HR: 0.58; 95% CI: 0.43 to 0.80; p < 0.001; 8.6 versus 5.6 months for talazoparib, HR: 0.54; 95% CI, 0.41–0.71; p = 0.001) (36, 37). However, the PFS benefit did not translate in a significant OS benefit for either of the two trials (66, 71). In fact, findings from a final prespecified analysis showed no OS difference in the general population (19.3 versus 17.1 months; HR: 0.90; 95% CI: 0.66–1.23; P = 0.513 for olaparib; 19.3 versus 19.5 months for talazoparib, HR: 0.848; 95% CI, 0.670–1.073; p = 0.17) and in the TNBC subgroup (18.8 versus 17.2 months; HR: 1.13; 95% CI: 0.79–1.64; P = NS for olaparib; data not available for talazoparib). A possible reason that could explain the OS lack of benefit is that the sample was not powered to detect OS differences between the two arms, as it was in the OlympiAD trial, or crossover design: in the EMBRACA trial, approximately 35% of patients treated in the control arm received a PARPib in subsequent lines of therapy versus 8% of patients enrolled in the OlympiAD trial. Interestingly, in the OlympiAD trial, a 7.9-month OS benefit was observed in patients who had not received prior chemotherapy for metastatic breast cancer, confirming previously published data where olaparib seemed to be more active in less pretreated patients (38). However, the sample size was small. Therefore, a confounding process cannot be excluded. Furthermore, perspective data are needed to confirm this finding. The safety profile for both talazoparib and olaparib was manageable: drug discontinuation was low (<5% for olaparib and 5.9% for talazoparib), showing that supportive therapies and dose interruptions/reductions were sufficiently effective to manage tolerability (66, 118). Most grade 3 and 4 adverse events were hematological: 40% of patients in the talazoparib arm and 16% of patients in the olaparib arm experienced grade 3 anemia. Fortunately, no new side effects were recorded with extended follow-up, and the safety profile was consistent with the primary analysis, indicating the absence of cumulative toxicity with prolonged exposition to the molecules.
Recent results have demonstrated that PARP inhibitors could play an emerging role in the maintenance treatment of advanced ovarian cancer with long-term efficacy and improved PFS in patients with newly diagnosed disease experimenting CR or PR to platinum-based chemotherapy (119–121). According to these results and in the light of the emerging role of PARP inhibitors in the treatment of triple negative breast cancer, there is a solid scientific rationale for the use of these molecules as maintenance therapy even in patients with TNBC.
One of the most consequential risks associated with PARPib is the development of therapy-related myeloid neoplasms (t-MNs). Most of the data available about t-MNs come from ovarian cancer where incidence is estimated in 1–3% of patients (122, 123). The spectrum of t-MNs comprehends myelodysplastic syndrome (MDS) and acute myeloid leukemia (AML). Both are characterized by a complex karyotype and poor prognosis (124), but the mechanisms responsible for t-MNs onset have not yet been clarified. In fact, a recent metanalysis did not confirm the association between t-MNs and previously identified clinical risk factors such as gBRCA variants, recurrent disease, and exposure to specific antineoplastic agents (125). According to a recently published systematic review, which evaluated the safety profile of 31 RCTs comparing PARPib therapy versus control treatments in different settings and tumor types, PARPib therapy was associated with an increased risk of t-MNs, but all the cases of MDS or AML were reported in RCTs in ovarian cancer (126). This exclusivity for ovarian cancer might be explained by the difference in median follow-up, with ovarian cancer RCTs having the longest duration when compared to the other trials included in the analysis. Therefore, at this point, patients with metastatic TNBC treated with PARPib do not seem to be at a higher risk for t-MN development, but a longer follow-up is needed to confirm those findings.
Despite the established role of PARPib in the therapeutical armamentarium of TNBC treatment, almost all the patients will become eventually resistant to the therapy, thus the need to improve therapeutical opportunities for this class of patients. In recent years, precision medicine is rapidly evolving thanks to next-generation sequencing (NGS) advances. Genomically driven molecular interrogation revealed that TNBC is a complex and heterogeneous disease. Unfortunately, there is still lack of clinical data supporting a major benefit of PARPib therapy in specific TN molecular subtypes (e.g., immunomodulatory, basal-like, etc.), but recent evidences showed that approximately 20% of patients with basal-like tumors harbored genetic or somatic BRCA1/2 mutations which may confer sensitivity to PARPib or platinum compounds (127, 128).
To overcame drug resistance and take advantage from our better understanding of TN tumors, an optimized and effective strategy probably requires a treatment combination rather than monotherapies. In that sense, several ongoing trials are combining PARPib with other agents (Tables 3–5). At this time, major clinical evidences derive from the combination of PARPib with ICIs: two phase 2, single-arm studies (TOPACIO and MEDIOLA) showed comparable promising results in terms of ORR, safety, and tolerability with the combination of niraparib plus pembrolizumab (101) and durvalumab plus olaparib (102), respectively. Alongside this, several phase I and II trials are evaluating PARPib with other targeted agents according to the growing stratification and knowledge of TNBC chromosomal aberrations. Hopefully, in the near future, the role of PARPib for the treatment of TNBC will gradually evolve towards a more personalized approach with promising expectations.
Conclusion
PARPib now represent a standard of care for the treatment of patients with triple negative breast cancer and gBRCA mutations. The oral formulation and the improvement in QoL are responsible for the increasing adherence and awareness of the patients. The safety profile is manageable, but patients must be checked routinely. Future directions comprehend the association of PARPib with other agents such as immunotherapy and other targeted therapies and the inclusion of patients with somatic BRCA mutations or patients carrying mutations beyond BRCA1 and BRCA2 but always involved in HRR pathway.
Author Contributions
GB and MR wrote the manuscript. MV selected bibliographic contributes. PV corrected the manuscript. ST checked the introduction, the conclusions, and the tables. FT devised, designed, and supervised the paper. All authors contributed to the article and approved the submitted version.
Conflict of Interest
The authors declare that the research was conducted in the absence of any commercial or financial relationships that could be construed as a potential conflict of interest.
Publisher’s Note
All claims expressed in this article are solely those of the authors and do not necessarily represent those of their affiliated organizations, or those of the publisher, the editors and the reviewers. Any product that may be evaluated in this article, or claim that may be made by its manufacturer, is not guaranteed or endorsed by the publisher.
Abbreviations
AML, acute myeloid leukemia; CSC, cancer stem cells; DSB, double-strand breaks; HR, homologous recombination; HR+, hormone receptors—positive; HRR, homologous recombination repair; HRQoL, health-related quality of life; ICIs, immune checkpoint inhibitors; MDS, myelodysplastic syndrome; NGS, next-generation sequencing; NHEJ, non-homologous end-joining; ORR, overall response rate; OS, overall survival; PARPib, PARP inhibitors; PI3Kib, PI3K inhibitors; PFS, progression-free survival; RCTs, randomized clinical trials; SSB, single-strand breaks; t-MNs, therapy-related myeloid neoplasm; TN, triple negative; TNBC, triple negative breast cancer; TPC, treatment physician’s choice.
References
1. Maajani K, Jalali A, Alipour S, Khodadost M, Tohidinik HR, Yazdani K. The Global and Regional Survival Rate of Women With Breast Cancer: A Systematic Review and Meta-Analysis. Clin Breast Cancer (2019) 19:165–77. doi: 10.1016/j.clbc.2019.01.006
3. Ferlay J, Colombet M, Soerjomataram I, Parkin DM, Piñeros M, Znaor A, et al. Cancer Statistics for the Year 2020: An Overview. Int J Cancer (2021). doi: 10.1002/ijc.33588
4. Perou CM, Sørile T, Eisen MB, Van De Rijn M, Jeffrey SS, Ress CA, et al. Molecular Portraits of Human Breast Tumours. Nature (2000) 406:747–52. doi: 10.1038/35021093
5. Sørlie T, Tibshirani R, Parker J, Hastie T, Marron JS, Nobel A, et al. Repeated Observation of Breast Tumor Subtypes in Independent Gene Expression Data Sets. Proc Natl Acad Sci USA (2003) 100:8418–23. doi: 10.1073/pnas.0932692100
6. Sørlie T, Perou CM, Tibshirani R, Aas T, Geisler S, Johnsen H, et al. Gene Expression Patterns of Breast Carcinomas Distinguish Tumor Subclasses With Clinical Implications. Proc Natl Acad Sci USA (2001) 98:10869–74. doi: 10.1073/pnas.191367098
7. Lehmann BD, Bauer JA, Chen X, Sanders ME, Chakravarthy AB, Shyr Y, et al. Identification of Human Triple-Negative Breast Cancer Subtypes and Preclinical Models for Selection of Targeted Therapies. J Clin Invest (2011) 121:2750–67. doi: 10.1172/JCI45014
8. Lehmann BD, Pietenpol JA. Clinical Implications of Molecular Heterogeneity in Triple Negative Breast Cancer. Breast (2015) 24:S36–40. doi: 10.1016/j.breast.2015.07.009
9. Burstein MD, Tsimelzon A, Poage GM, Covington KR, Contreras A, Fuqua SAW, et al. Comprehensive Genomic Analysis Identifies Novel Subtypes and Targets of Triple-Negative Breast Cancer. Clin Cancer Res (2015) 21:1688–98. doi: 10.1158/1078-0432.CCR-14-0432
10. Sharma P. Biology and Management of Patients With Triple-Negative Breast Cancer. Oncologist (2016) 21:1050–62. doi: 10.1634/theoncologist.2016-0067
11. Bareche Y, Venet D, Ignatiadis M, Aftimos P, Piccart M, Rothe F, et al. Unravelling Triple-Negative Breast Cancer Molecular Heterogeneity Using an Integrative Multiomic Analysis. Ann Oncol (2018) 29:895–902. doi: 10.1093/annonc/mdy024
12. Borri F, Granaglia A. Pathology of Triple Negative Breast Cancer. Semin Cancer Biol (2021) 72:136–45. doi: 10.1016/j.semcancer.2020.06.005
13. Foulkes WD, Smith IE, Reis-Filho JS. Triple-Negative Breast Cancer. N Engl J Med (2010) 363:1938–48. doi: 10.1056/NEJMra1001389
14. Dent R, Trudeau M, Pritchard KI, Hanna WM, Kahn HK, Sawka CA, et al. Triple-Negative Breast Cancer: Clinical Features and Patterns of Recurrence. Clin Cancer Res (2007) 13:4429–34. doi: 10.1158/1078-0432.CCR-06-3045
15. Tsang JYS, Huang YH, Luo MH, Ni YB, Chan SK, Lui PCW, et al. Cancer Stem Cell Markers Are Associated With Adverse Biomarker Profiles and Molecular Subtypes of Breast Cancer. Breast Cancer Res Treat (2012) 136(2):407–17. doi: 10.1007/s10549-012-2271-6
16. Perrone G, Gaeta LM, Zagami M, Nasorri F, Coppola R, Borzomati D, et al. In Situ Identification of CD44+/CD24- Cancer Cells in Primary Human Breast Carcinomas. PloS One (2012) 7:43110. doi: 10.1371/journal.pone.0043110
17. Idowu MO, Kmieciak M, Dumur C, Burton RS, Grimes MM, Powers CN, et al. CD44 +/CD24 -/Low Cancer Stem/Progenitor Cells Are More Abundant in Triple-Negative Invasive Breast Carcinoma Phenotype and Are Associated With Poor Outcome. Hum Pathol (2012) 43:364–73. doi: 10.1016/j.humpath.2011.05.005
18. Ricardo S, Vieira AF, Gerhard R, Leitão D, Pinto R, Cameselle-Teijeiro JF, et al. Breast Cancer Stem Cell Markers CD44, CD24 and ALDH1: Expression Distribution Within Intrinsic Molecular Subtype. J Clin Pathol (2011) 64:937–44. doi: 10.1136/jcp.2011.090456
19. Bianchini G, Balko JM, Mayer IA, Sanders ME, Gianni L. Triple-Negative Breast Cancer: Challenges and Opportunities of a Heterogeneous Disease. Nat Rev Clin Oncol (2016) 13:674–90. doi: 10.1038/nrclinonc.2016.66
20. Schmid P, Adams S, Rugo HS, Schneeweiss A, Barrios CH, Iwata H, et al. Atezolizumab and Nab-Paclitaxel in Advanced Triple-Negative Breast Cancer. N Engl J Med (2018) 379:2108–21. doi: 10.1056/nejmoa1809615
21. Cardoso F, Senkus E, Costa A, Papadopoulos E, Aapro M, André F, et al. 4th ESO-ESMO International Consensus Guidelines for Advanced Breast Cancer (ABC 4). Ann Oncol (2018) 29:1634–57. doi: 10.1093/annonc/mdy192
22. Abraham J, Aft R, Agnese D, Allison KH, Anderson B, Blair SL, et al. NCCN Guidelines Version 4.2021 Breast Cancer. (2021).
23. Tomao F, Papa A, Zaccarelli E, Rossi L, Caruso D, Minozzi M, et al. Triple-Negative Breast Cancer: New Perspectives for Targeted Therapies. Onco Targets Ther (2015) 8:177–93. doi: 10.2147/OTT.S67673
24. Papa A, Caruso D, Tomao S, Rossi L, Zaccarelli E, Tomao F. Triple-Negative Breast Cancer: Investigating Potential Molecular Therapeutic Target. Expert Opin Ther Targets (2015) 19:55–75. doi: 10.1517/14728222.2014.970176
25. Stanton SE, Adams S, Disis ML. Variation in the Incidence and Magnitude of Tumor-Infiltrating Lymphocytes in Breast Cancer Subtypes: A Systematic Review. JAMA Oncol (2016) 2:1354–60. doi: 10.1001/jamaoncol.2016.1061
26. Safonov A, Jiang T, Bianchini G, Gyorffy B, Karn T, Hatzis C, et al. Immune Gene Expression Is Associated With Genomic Aberrations in Breast Cancer. Cancer Res (2017) 77:3317–24. doi: 10.1158/0008-5472.CAN-16-3478
27. Schmid P, Rugo HS, Adams S, Schneeweiss A, Barrios CH, Iwata H, et al. Atezolizumab Plus Nab-Paclitaxel as First-Line Treatment for Unresectable, Locally Advanced or Metastatic Triple-Negative Breast Cancer (Impassion130): Updated Efficacy Results From a Randomised, Double-Blind, Placebo-Controlled, Phase 3 Trial. Lancet Oncol (2020) 21:44–59. doi: 10.1016/S1470-2045(19)30689-8
28. Parsons HA, Burstein HJ. Adjuvant Capecitabine in Triple-Negative Breast Cancer: New Strategies for Tailoring Treatment Recommendations. JAMA - J Am Med Assoc (2021) 325:36–8. doi: 10.1001/jama.2020.23371
29. Wang X, Sen WS, Huang H, Cai L, Zhao L, Peng RJ, et al. Effect of Capecitabine Maintenance Therapy Using Lower Dosage and Higher Frequency vs Observation on Disease-Free Survival Among Patients With Early-Stage Triple-Negative Breast Cancer Who had Received Standard Treatment: The SYSUCC-001 Randomized Clinica. JAMA - J Am Med Assoc (2021) 325:50–8. doi: 10.1001/jama.2020.23370
30. Cortes J, O’Shaughnessy J, Loesch D, Blum JL, Vahdat LT, Petrakova K, et al. Eribulin Monotherapy Versus Treatment of Physician’s Choice in Patients With Metastatic Breast Cancer (EMBRACE): A Phase 3 Open-Label Randomised Study. Lancet (2011) 377:914–23. doi: 10.1016/S0140-6736(11)60070-6
31. Krasniqi E, Pizzuti L, Valerio MR, Capomolla E, Botti C, Sanguineti G, et al. Second-Line Eribulin in Triple Negative Metastatic Breast Cancer Patients. Multicentre Retrospective Study: The Tetris Trial. Int J Med Sci (2021) 18:2245–50. doi: 10.7150/ijms.54996
32. Tung N, Lin NU, Kidd J, Allen BA, Singh N, Wenstrup RJ, et al. Frequency of Germline Mutations in 25 Cancer Susceptibility Genes in a Sequential Series of Patients With Breast Cancer. J Clin Oncol (2016) 34:1460–8. doi: 10.1200/JCO.2015.65.0747
33. Winter C, Nilsson MP, Olsson E, George AM, Chen Y, Kvist A, et al. Targeted Sequencing of BRCA1 and BRCA2 Across a Large Unselected Breast Cancer Cohort Suggests That One-Third of Mutations Are Somatic. Ann Oncol (2016) 27:1532–8. doi: 10.1093/annonc/mdw209
34. Kuchenbaecker KB, Hopper JL, Barnes DR, Phillips KA, Mooij TM, Roos-Blom MJ, et al. Risks of Breast, Ovarian, and Contralateral Breast Cancer for BRCA1 and BRCA2 Mutation Carriers. JAMA - J Am Med Assoc (2017) 317:2402–16. doi: 10.1001/jama.2017.7112
35. Toss A, Molinaro E, Venturelli M, Domati F, Marcheselli L, Piana S, et al. Brca Detection Rate in an Italian Cohort of Luminal Early-Onset and Triple-Negative Breast Cancer Patients Without Family History: When Biology Overcomes Genealogy. Cancers (Basel) (2020) 12(5):1252. doi: 10.3390/cancers12051252
36. Litton JK, Rugo HS, Ettl J, Hurvitz SA, Gonçalves A, Lee K-H, et al. Talazoparib in Patients With Advanced Breast Cancer and a Germline BRCA Mutation. N Engl J Med (2018) 379:753–63. doi: 10.1056/nejmoa1802905
37. Robson M, Im S-A, Senkus E, Xu B, Domchek SM, Masuda N, et al. Olaparib for Metastatic Breast Cancer in Patients With a Germline BRCA Mutation. N Engl J Med (2017) 377:523–33. doi: 10.1056/nejmoa1706450
38. Kaufman B, Shapira-Frommer R, Schmutzler RK, Audeh MW, Friedlander M, Balmaña J, et al. Olaparib Monotherapy in Patients With Advanced Cancer and a Germline BRCA1/2 Mutation. J Clin Oncol (2015) 33:244–50. doi: 10.1200/JCO.2014.56.2728
39. Tutt A, Robson M, Garber JE, Domchek SM, Audeh MW, Weitzel JN, et al. Oral Poly(ADP-Ribose) Polymerase Inhibitor Olaparib in Patients With BRCA1 or BRCA2 Mutations and Advanced Breast Cancer: A Proof-of-Concept Trial. Lancet (2010) 376:235–44. doi: 10.1016/S0140-6736(10)60892-6
40. Chapman JR, Taylor MRG, Boulton SJ. Playing the End Game: DNA Double-Strand Break Repair Pathway Choice. Mol Cell (2012) 47:497–510. doi: 10.1016/j.molcel.2012.07.029
41. Jasin M, Rothstein R. Repair of Strand Breaks by Homologous Recombination. Cold Spring Harb Perspect Biol (2013) 5(11):a012740. doi: 10.1101/cshperspect.a012740
42. Hoeijmakers JHJ. Genome Maintenance Mechanisms for Preventing Cancer. Nature (2001) 411:366–74. doi: 10.1038/35077232
43. Rouleau M, Patel A, Hendzel MJ, Kaufmann SH, Poirier GG. PARP Inhibition: PARP1 and Beyond. Nat Rev Cancer (2010) 10:293–301. doi: 10.1038/nrc2812
44. Amé JC, Spenlehauer C, De Murcia G. The PARP Superfamily. BioEssays (2004) 26:882–93. doi: 10.1002/bies.20085
45. Wei H, Yu X. Functions of Parylation in DNA Damage Repair Pathways. Genomics Proteomics Bioinforma (2016) 14:131–9. doi: 10.1016/j.gpb.2016.05.001
46. Haince JF, McDonald D, Rodrigue A, Déry U, Masson JY, Hendzel MJ, et al. PARP1-Dependent Kinetics of Recruitment of MRE11 and NBS1 Proteins to Multiple DNA Damage Sites. J Biol Chem (2008) 283:1197–208. doi: 10.1074/jbc.M706734200
47. Walsh CS. Two Decades Beyond BRCA1/2: Homologous Recombination, Hereditary Cancer Risk and a Target for Ovarian Cancer Therapy? Gynecol Oncol (2015) 137:343–50. doi: 10.1016/j.ygyno.2015.02.017
48. Scully R, Anderson SF, Chao DM, Wei W, Ye L, Young RA, et al. BRCA1 Is a Component of the RNA Polymerase II Holoenzyme. Proc Natl Acad Sci USA (1997) 94(11):5605–10. doi: 10.1073/pnas.94.11.5605
49. Wang B, Matsuoka S, Ballif BA, Zhang D, Smogorzewska A, Gygi SP, et al. Abraxas and RAP80 Form a BRCA1 Protein Complex Required for the DNA Damage Response. Science (80-) (2007) 316:1194–8. doi: 10.1126/science.1139476
50. Roy R, Chun J, Powell SN. BRCA1 and BRCA2: Different Roles in a Common Pathway of Genome Protection. Nat Rev Cancer (2012) 12:68–78. doi: 10.1038/nrc3181
51. Helleday T. The Underlying Mechanism for the PARP and BRCA Synthetic Lethality: Clearing Up the Misunderstandings. Mol Oncol (2011) 5:387–93. doi: 10.1016/j.molonc.2011.07.001
52. Javle M, Curtin NJ. The Potential for Poly (ADP-Ribose) Polymerase Inhibitors in Cancer Therapy. Ther Adv Med Oncol (2011) 3:257–67. doi: 10.1177/1758834011417039
53. Lord CJ, Ashworth A. PARP Inhibitors: Synthetic Lethality in the Clinic. Science (2017) 355:1152–8. doi: 10.1126/science.aam7344
54. Featherstone C, Jackson SP. DNA Double-Strand Break Repair. Curr Biol (1999) 9(20):R759–61. doi: 10.1016/S0960-9822(00)80005-6
55. Wang JYJ. DNA Damage and Apoptosis. Cell Death Differ (2001) 8:1047–8. doi: 10.1038/sj.cdd.4400938
56. Hartman JL IV, Garvik B, Hartwell L. Cell Biology: Principles for the Buffering of Genetic Variation. Science (80-) (2001) 291:1001–4. doi: 10.1126/science.291.5506.1001
57. Friend SH, Oliff A. Emerging Uses for Genomic Information in Drug Discovery. N Engl J Med (1998) 338:125–6. doi: 10.1056/nejm199801083380211
58. Kamb A. Mutation Load, Functional Overlap, and Synthetic Lethality in the Evolution and Treatment of Cancer. J Theor Biol (2003) 223:205–13. doi: 10.1016/S0022-5193(03)00087-0
59. Farmer H, McCabe H, Lord CJ, Tutt AHJ, Johnson DA, Richardson TB, et al. Targeting the DNA Repair Defect in BRCA Mutant Cells as a Therapeutic Strategy. Nature (2005) 434:917–21. doi: 10.1038/nature03445
60. McCabe N, Turner NC, Lord CJ, Kluzek K, Białkowska A, Swift S, et al. Deficiency in the Repair of DNA Damage by Homologous Recombination and Sensitivity to Poly(ADP-Ribose) Polymerase Inhibition. Cancer Res (2006) 66:8109–15. doi: 10.1158/0008-5472.CAN-06-0140
61. Murai J, Huang SYN, Das BB, Renaud A, Zhang Y, Doroshow JH, et al. Trapping of PARP1 and PARP2 by Clinical PARP Inhibitors. Cancer Res (2012) 72:5588–99. doi: 10.1158/0008-5472.CAN-12-2753
62. Mateo J, Lord CJ, Serra V, Tutt A, Balmaña J, Castroviejo-Bermejo M, et al. De Bono JS. A Decade of Clinical Development of PARP Inhibitors in Perspective. Ann Oncol (2019) 30:1437–47. doi: 10.1093/annonc/mdz192
63. Murai J, Huang SYN, Renaud A, Zhang Y, Ji J, Takeda S, et al. Stereospecific PARP Trapping by BMN 673 and Comparison With Olaparib and Rucaparib. Mol Cancer Ther (2014) 13:433–43. doi: 10.1158/1535-7163.MCT-13-0803
64. Shen Y, Rehman FL, Feng Y, Boshuizen J, Bajrami I, Elliott R, et al. BMN673, a Novel and Highly Potent PARP1/2 Inhibitor for the Treatment of Human Cancers With DNA Repair Deficiency. Clin Cancer Res (2013) 19:5003–15. doi: 10.1158/1078-0432.CCR-13-1391
65. Menear KA, Adcock C, Boulter R, Cockcroft XL, Copsey L, Cranston A, et al. 4-[3-(4-Cyclopropanecarbonylpiperazine-1-Carbonyl)-4-Fluorobenzyl] -2H-Phthalazin-1-One: A Novel Bioavailable Inhibitor of Poly(ADP-Ribose) Polymerase-1. J Med Chem (2008) 51:6581–91. doi: 10.1021/jm8001263
66. Robson ME, Tung N, Conte P, Im SA, Senkus E, Xu B, et al. Olympiad Final Overall Survival and Tolerability Results: Olaparib Versus Chemotherapy Treatment of Physician’s Choice in Patients With a Germline BRCA Mutation and HER2-Negative Metastatic Breast Cancer. Ann Oncol (2019) 30:558–66. doi: 10.1093/annonc/mdz012
67. Robson M, Ruddy KJ IM, Senkus E, Xu B, Domchek SM, Masuda N, et al. Patient-Reported Outcomes in Patients With a Germline BRCA Mutation and HER2-Negative Metastatic Breast Cancer Receiving Olaparib Versus Chemotherapy in the Olympiad Trial. Eur J Cancer (2019) 120:20–30. doi: 10.1016/j.ejca.2019.06.023. SA.
68. Tryfonidis K, Bogaerts J, Martell RE, Sledge GW, Balmaña J, Audeh MW, et al. A Phase III Randomized Trial of Niraparib Versus Physician’s Choice in Previously Treated, HER2-Negative, Germline-BRCA Mutated Breast Cancer Patients: Intergroup Study EORTC-1307-BCG and BIG5-13. J Clin Oncol (2014) 32:TPS659–9. doi: 10.1200/jco.2014.32.15_suppl.tps659
69. Olympi AD. Extended Follow-Up for Overall Survival and Safety: Olaparib Versus Chemotherapy Treatment of Physician’s Choice in Patients With a Germline BRCA Mutation and HER2-Negative Metastatic Breast Cancer.
70. Rugo HS, Ettl J, Hurvitz SA, Gonçalves A, Lee KH, Fehrenbacher L, et al. Outcomes in Clinically Relevant Patient Subgroups From the EMBRACA Study: Talazoparib vs Physician’s Choice Standard-of-Care Chemotherapy. JNCI Cancer Spectr (2020) 4(1):pkz085. doi: 10.1093/jncics/pkz085
71. Litton JK, Hurvitz SA, Mina LA, Rugo HS, Lee KH, Gonçalves A, et al. Talazoparib Versus Chemotherapy in Patients With Germline BRCA1/2-Mutated HER2-Negative Advanced Breast Cancer: Final Overall Survival Results From the EMBRACA Trial. Ann Oncol (2020) 31:1526–35. doi: 10.1016/j.annonc.2020.08.2098
72. Taylor AM, Chan DLH, Tio M, Patil SM, Traina TA, Robson ME, et al. PARP (Poly ADP-Ribose Polymerase) Inhibitors for Locally Advanced or Metastatic Breast Cancer. Cochrane Database Syst Rev (2021) 2021. doi: 10.1002/14651858.CD011395.pub2
73. Turner NC, Telli ML, Rugo HS, Mailliez A, Ettl J, Grischke EM, et al. A Phase II Study of Talazoparib After Platinum or Cytotoxic Nonplatinum Regimens in Patients With Advanced Breast Cancer and Germline BRCA1/2 Mutations (ABRAZO). Clin Cancer Res (2019) 25:2717–24. doi: 10.1158/1078-0432.CCR-18-1891
74. A Feasibility Study of Niraparib for Advanced, BRCA1-Like, HER2-Negative Breast Cancer Patients - Full Text View - Clinicaltrials.Gov.
75. Phase II Trial of Talazoparib in BRCA1/2 Wild-Type HER2-Negative Breast Cancer and Other Solid Tumors - Full Text View - Clinicaltrials.Gov.
76. Han Y, Yu X, Li S, Tian Y, Liu C. New Perspectives for Resistance to PARP Inhibitors in Triple-Negative Breast Cancer. Front Oncol (2020) 10:578095. doi: 10.3389/fonc.2020.578095
77. Noordermeer SM, van Attikum H. PARP Inhibitor Resistance: A Tug-of-War in BRCA-Mutated Cells. Trends Cell Biol (2019) 29. doi: 10.1016/j.tcb.2019.07.008
78. Niraparib in Combination With Pembrolizumab in Patients With Triple-Negative Breast Cancer or Ovarian Cancer - Full Text View - Clinicaltrials.Gov.
79. A Phase I/II Study of MEDI4736 in Combination With Olaparib in Patients With Advanced Solid Tumors. - Full Text View - Clinicaltrials.Gov.
80. A Phase III Trial of Niraparib Versus Physician’s Choice in HER2 Negative, Germline BRCA Mutation-Positive Breast Cancer Patients - Full Text View - Clinicaltrials.Gov.
81. To Study Clinical Effectiveness and Safety of Olaparib Monotherapy in Metastatic Breast Cancer Patients. - Full Text View - Clinicaltrials.Gov.
82. A Trial of SHR-1210 (an Anti-PD-1 Inhibitor) in Combination With Apatinib and Fluzoparib in Patients With TNBC - Full Text View - Clinicaltrials.Gov.
83. Phase II Multicenter Study of Durvalumab and Olaparib in Platinum Treated Advanced Triple Negative Breast Cancer (DORA) - Full Text View - Clinicaltrials.Gov.
84. Phase I/II Study of the Anti-Programmed Death Ligand-1 Antibody MEDI4736 in Combination With Olaparib and/or Cediranib for Advanced Solid Tumors and Advanced or Recurrent Ovarian, Triple Negative Breast, Lung, Prostate and Colorectal Cancers - Full Text V.
85. Durvalumab, With Olaparib and Fulvestrant in Advanced ER+, HER2- Breast Cancer Patients. - Full Text View - Clinicaltrials.Gov.
86. Olaparib With or Without Atezolizumab in Treating Patients With Locally Advanced Unresectable or Metastatic Non-HER2-Positive Breast Cancer - Full Text View - Clinicaltrials.Gov.
87. A Study of Radiation Therapy With Pembrolizumab and Olaparib in Women Who Have Triple-Negative Breast Cancer - Full Text View - Clinicaltrials.Gov.
88. Javelin BRCA/ATM: Avelumab Plus Talazoparib in Patients With BRCA or ATM Mutant Solid Tumors - Full Text View - Clinicaltrials.Gov.
89. TALAVE: Induction Talazoparib Followed by Combination of Talazoparib and Avelumab in Advanced Breast Cancer - Full Text View - Clinicaltrials.Gov.
90. A Study to Evaluate Talazoparib, Radiotherapy and Atezolizumab in Gbrca 1/2 Negative Patients With PD-L1+ Metastatic Triple Negative Breast Cancer (TARA) - Full Text View - Clinicaltrials.Gov.
91. A Phase 3 Randomized, Placebo-Controlled Trial of Carboplatin and Paclitaxel With or Without Veliparib (ABT-888) in HER2-Negative Metastatic or Locally Advanced Unresectable BRCA-Associated Breast Cancer - Full Text View - Clinicaltrials.Gov.
92. Veliparib With or Without Carboplatin in Treating Patients With Stage IV Breast Cancer - Full Text View - Clinicaltrials.Gov.
93. ABT-888 and Temozolomide for Metastatic Breast Cancer and BRCA1/2 Breast Cancer - Full Text View - Clinicaltrials.Gov.
94. Cisplatin With or Without Veliparib in Treating Patients With Recurrent or Metastatic Triple-Negative and/or BRCA Mutation-Associated Breast Cancer With or Without Brain Metastases - Full Text View - Clinicaltrials.Gov.
95. To Assess Safety and Efficacy of Agents Targeting DNA Damage Repair With Olaparib Versus Olaparib Monotherapy. - Full Text View - Clinicaltrials.Gov.
96. A Study to Evaluate Rucaparib in Combination With Other Anticancer Agents in Patients With a Solid Tumor (SEASTAR) - Full Text View - Clinicaltrials.Gov.
97. A Study of ZEN003694 and Talazoparib in Patients With Triple Negative Breast Cancer - Full Text View - Clinicaltrials.Gov.
98. Gedatolisib Plus Talazoparib in Advanced Triple Negative or BRCA1/2 Positive, HER2 Negative Breast Cancers - Full Text View - Clinicaltrials.Gov.
99. Olaparib+Trastuzumab in HER2[+],Breast Cancer Susceptibility Gene (BRCA) Mutated Advanced Breast Cancer - Full Text View - Clinicaltrials.Gov.
100. Pilot Study of Veliparib (ABT-888) and Lapatinib (Tykerb) in Patients With Metastatic, Triple Negative Breast Cancer - Full Text View - Clinicaltrials.Gov.
101. Mitri ZI, Vuky J, Kemmer KA, Savin MA, Parmar S, Kolodzie AK, et al. A Phase II Trial of Olaparib and Durvalumab in Metastatic BRCA Wild Type Triple-Negative Breast Cancer. J Clin Oncol (2019) 37:TPS1111–TPS1111. doi: 10.1200/jco.2019.37.15_suppl.tps1111
102. Domchek S, Postel-Vinay S, Im S-A, Park YH, Delord J-P, Italiano A, et al. Phase II Study of Olaparib (O) and Durvalumab (D) (MEDIOLA): Updated Results in Patients (Pts) With Germline BRCA-Mutated (Gbrcam) Metastatic Breast Cancer (MBC). Ann Oncol (2019) 30:v477. doi: 10.1093/annonc/mdz253.017
103. Vinayak S, Tolaney SM, Schwartzberg L, Mita M, McCann G, Tan AR, et al. Open-Label Clinical Trial of Niraparib Combined With Pembrolizumab for Treatment of Advanced or Metastatic Triple-Negative Breast Cancer. JAMA Oncol (2019) 5:1132–40. doi: 10.1001/jamaoncol.2019.1029
104. Ding L, Kim HJ, Wang Q, Kearns M, Jiang T, Ohlson CE, et al. PARP Inhibition Elicits STING-Dependent Antitumor Immunity in Brca1-Deficient Ovarian Cancer. Cell Rep (2018) 25:2972–2980.e5. doi: 10.1016/j.celrep.2018.11.054
105. Arun BK, Han HS, Kaufman B, Wildiers H, Friedlander M, Ayoub J-P, et al. Abstract PD4-01: First-Line Veliparib Plus Carboplatin/Paclitaxel in Patients With HER2-Negative Advanced/Metastatic G BRCA -Associated Breast Cancer: Planned Subgroup Analysis From the Phase 3 BROCADE3 Trial. Cancer Res (American Assoc Cancer Res (AACR)) (2020) PD4–01-PD4-01. doi: 10.1158/1538-7445.sabcs19-pd4-01
106. Ayoub J-P, Friedlander ML, Dieras VC, Wildiers H, Arun B, Han HS, et al. 140O Veliparib Plus Carboplatin-Paclitaxel in Patients With HER2-Negative Advanced/Metastatic Gbrca-Associated Breast Cancer: Results in Hormone Receptor-Positive and Triple-Negative Breast Cancer Subgroups From the Phase III BROCADE3 Trial. Ann Oncol (2020) 31. doi: 10.1016/j.annonc.2020.03.241
107. Beck H, Nahse-Kumpf V, Larsen MSY, O’Hanlon KA, Patzke S, Holmberg C, et al. Cyclin-Dependent Kinase Suppression by WEE1 Kinase Protects the Genome Through Control of Replication Initiation and Nucleotide Consumption. Mol Cell Biol (2012) 32(20):4226–36. doi: 10.1128/mcb.00412-12
108. Bitler BG, Watson ZL, Wheeler LJ, Behbakht K. PARP Inhibitors: Clinical Utility and Possibilities of Overcoming Resistance. Gynecol Oncol (2017) 147:695–704. doi: 10.1016/j.ygyno.2017.10.003
109. Dréan A, Williamson CT, Brough R, Brandsma I, Menon M, Konde A, et al. Modeling Therapy Resistance in BRCA1/2-Mutant Cancers. Mol Cancer Ther (2017) 16:2022–34. doi: 10.1158/1535-7163.MCT-17-0098
110. Parsels LA, Karnak D, Parsels JD, Zhang Q, Velez-Padilla J, Reichert ZR, et al. PARP1 Trapping and DNA Replication Stress Enhance Radiosensitization With Combined WEE1 and PARP Inhibitors. Mol Cancer Res (2018) 16:222–32. doi: 10.1158/1541-7786.MCR-17-0455
111. Juvekar A, Hu H, Yadegarynia S, Lyssiotis CA, Ullas S, Lien EC, et al. Phosphoinositide 3-Kinase Inhibitors Induce DNA Damage Through Nucleoside Depletion. Proc Natl Acad Sci USA (2016) 113:E4338–47. doi: 10.1073/pnas.1522223113
112. Cortesi L, Rugo HS, Jackisch C. An Overview of PARP Inhibitors for the Treatment of Breast Cancer. Target Oncol (2021) 16(3):255–82. doi: 10.1007/s11523-021-00796-4
114. A Study to Assess the Efficacy of Rucaparib in Metastatic Breast Cancer Patients With a Brcaness Genomic Signature - Full Text View - Clinicaltrials.Gov.
115. Tung NM, Robson ME, Ventz S, Santa-Maria CA, Nanda R, Marcom PK, et al. TBCRC 048: Phase II Study of Olaparib for Metastatic Breast Cancer and Mutations in Homologous Recombination-Related Genes. J Clin Oncol (2020) 38:4274–82. doi: 10.1200/JCO.20.02151
116. Patsouris A, Tredan O, Nenciu D, Tran-Dien A, Campion L, Goncalves A, et al. RUBY: A Phase II Study Testing Rucaparib in Germline (G) BRCA Wild-Type Patients Presenting Metastatic Breast Cancer (Mbc) With Homologous Recombination Deficiency (HRD). J Clin Oncol (2019) 37:1092–2. doi: 10.1200/jco.2019.37.15_suppl.1092
117. Gruber JJ, Afghahi A, Hatton A, Scott D, McMillan A, Ford JM, et al. Talazoparib Beyond BRCA: A Phase II Trial of Talazoparib Monotherapy in BRCA1 and BRCA2 Wild-Type Patients With Advanced HER2-Negative Breast Cancer or Other Solid Tumors With a Mutation in Homologous Recombination (HR) Pathway Genes. J Clin Oncol (2019) 37:3006–6. doi: 10.1200/jco.2019.37.15_suppl.3006
118. Ettl J, Quek RGW, Lee KH, Rugo HS, Hurvitz S, Gonçalves A, et al. Quality of Life With Talazoparib Versus Physician’s Choice of Chemotherapy in Patients With Advanced Breast Cancer and Germline BRCA1/2 Mutation: Patient-Reported Outcomes From the EMBRACA Phase III Trial. Ann Oncol (2018) 29:1939–47. doi: 10.1093/annonc/mdy257
119. Tomao F, Bardhi E, Di Pinto A, Sassu CM, Biagioli E, Petrella MC, et al. Parp Inhibitors as Maintenance Treatment in Platinum Sensitive Recurrent Ovarian Cancer: An Updated Meta-Analysis of Randomized Clinical Trials According to BRCA Mutational Status. Cancer Treat Rev (2019) 80:101909. doi: 10.1016/j.ctrv.2019.101909
120. Xu Y, Ding L, Tian Y, Bi M, Han N, Wang L. Comparative Efficacy and Safety of PARP Inhibitors as Maintenance Therapy in Platinum Sensitive Recurrent Ovarian Cancer: A Network Meta-Analysis. Front Oncol (2021) 10:573801. doi: 10.3389/fonc.2020.573801
121. DiSilvestro P, Colombo N, Scambia G, Kim BG, Oaknin A, Friedlander M, et al. Efficacy of Maintenance Olaparib for Patients With Newly Diagnosed Advanced Ovarian Cancer With a BRCA Mutation: Subgroup Analysis Findings From the SOLO1 Trial. J Clin Oncol (2020) 38(30):3528–37. doi: 10.1200/JCO.20.00799
122. Gonzalez-Angulo AM, Litton JK, Broglio KR, Meric-Bernstam F, Rakkhit R, Cardoso F, et al. High Risk of Recurrence for Patients With Breast Cancer Who Have Human Epidermal Growth Factor Receptor 2–Positive, Node-Negative Tumors 1 Cm or Smaller. J Clin Oncol (2009) 27:5700–6. doi: 10.1200/JCO.2009.23.2025
123. Moore K, Colombo N, Scambia G, Kim B-G, Oaknin A, Friedlander M, et al. Maintenance Olaparib in Patients With Newly Diagnosed Advanced Ovarian Cancer. N Engl J Med (2018) 379(26):2495–505. doi: 10.1056/nejmoa1810858
124. Gillis NK, Ball M, Zhang Q, Ma Z, Zhao YL, Yoder SJ, et al. Clonal Haemopoiesis and Therapy-Related Myeloid Malignancies in Elderly Patients: A Proof-of-Concept, Case-Control Study. Lancet Oncol (2017) 18(1):112–21. doi: 10.1016/S1470-2045(16)30627-1
125. Nitecki R, Melamed A, Gockley AA, Floyd J, Krause KJ, Coleman RL, et al. Incidence of Myelodysplastic Syndrome and Acute Myeloid Leukemia in Patients Receiving Poly-ADP Ribose Polymerase Inhibitors for the Treatment of Solid Tumors: A Meta-Analysis of Randomized Trials. Gynecol Oncol (2021) 161(3):653–9. doi: 10.1016/j.ygyno.2021.03.011
126. Morice PM, Leary A, Dolladille C, Chrétien B, Poulain L, González-Martín A, et al. Myelodysplastic Syndrome and Acute Myeloid Leukaemia in Patients Treated With PARP Inhibitors: A Safety Meta-Analysis of Randomised Controlled Trials and a Retrospective Study of the WHO Pharmacovigilance Database. Lancet Haematol (2021) 8(2):e122–34. doi: 10.1016/S2352-3026(20)30360-4
127. Ahn SG, Kim SJ, Kim C, Jeong J. Molecular Classification of Triple-Negative Breast Cancer. J Breast Cancer (2016) 19:223–30. doi: 10.4048/JBC.2016.19.3.223
Keywords: triple negative, metastatic breast cancer, PARP inhibitors, olaparib, talazoparib, BRCA1/2
Citation: Barchiesi G, Roberto M, Verrico M, Vici P, Tomao S and Tomao F (2021) Emerging Role of PARP Inhibitors in Metastatic Triple Negative Breast Cancer. Current Scenario and Future Perspectives. Front. Oncol. 11:769280. doi: 10.3389/fonc.2021.769280
Received: 01 September 2021; Accepted: 05 November 2021;
Published: 25 November 2021.
Edited by:
Mariana Segovia, National Autonomous University of Mexico, MexicoReviewed by:
Alice Chen, National Cancer Institute (NCI), United StatesAngela Toss, University of Modena and Reggio Emilia, Italy
Copyright © 2021 Barchiesi, Roberto, Verrico, Vici, Tomao and Tomao. This is an open-access article distributed under the terms of the Creative Commons Attribution License (CC BY). The use, distribution or reproduction in other forums is permitted, provided the original author(s) and the copyright owner(s) are credited and that the original publication in this journal is cited, in accordance with accepted academic practice. No use, distribution or reproduction is permitted which does not comply with these terms.
*Correspondence: Silverio Tomao, c2lsdmVyaW8udG9tYW9AZ21haWwuY29t
†These authors have contributed equally to this work