- 1Department of Oral and Maxillofacial Surgery, The Affiliated Hospital of Qingdao University, Qingdao, China
- 2School of Stomatology of Qingdao University, Qingdao, China
- 3Key Lab of Oral Clinical Medicine, The Affiliated Hospital of Qingdao University, Qingdao, China
Oral squamous cell carcinoma (OSCC) is a kind of malignant tumors with low survival rate and prone to have early metastasis and recurrence. Cisplatin is an alkylating agent which induces DNA damage through the formation of cisplatin-DNA adducts, leading to cell cycle arrest and apoptosis. In the management of advanced OSCC, cisplatin-based chemotherapy or chemoradiotherapy has been considered as the first-line treatment. Unfortunately, only a portion of OSCC patients can benefit from cisplatin treatment, both inherent resistance and acquired resistance greatly limit the efficacy of cisplatin and even cause treatment failure. Herein, this review outline the underlying mechanisms of cisplatin resistance in OSCC from the aspects of DNA damage and repair, epigenetic regulation, transport processes, programmed cell death and tumor microenvironment. In addition, this review summarizes the strategies applicable to overcome cisplatin resistance, which can provide new ideas to improve the clinical therapeutic outcome of OSCC.
1 Introduction
Oral cancer is the sixth leading cause of global cancer-related deaths (1), with the most common type being oral squamous cell carcinoma (OSCC). OSCC usually presents in smokers and drinkers aged 40 to 70 years, and in recent years human papillomavirus (HPV) infection has also been identified as a major cause (2, 3). OSCC tends to have early, extensive lymph node metastases and is among the malignancies with low survival rates. Despite advances in diagnostic and therapeutic approaches for OSCC in the past decades, its five-year survival rate remains suboptimal (4). At present, early stage non-metastatic OSCC (stages I and II) can be largely cured by surgery alone, but for advanced OSCC (stages III and IV), besides the standard surgical treatment and external radiotherapy, supportive treatment with a combination of chemotherapeutic agents is required (5).
Cisplatin (cis-diaminedichloroplatinum, CDDP), a chemotherapeutic agent with high antitumor activity against many cancers in clinical application, is the first-line and most widely used chemotherapeutic drug for OSCC. In 1968, cisplatin was first discovered to have the ability to lead to tumor regression in a tumor-bearing mouse model (6), and its antitumor activity was confirmed in a variety of solid tumors over the next decade (7–9). The cytotoxic effect of cisplatin is mainly manifested as forming adducts with genomic DNA, which directly damages DNA and inhibits DNA replication, thereby arresting the cell cycle and eventually leading to cell death (Figure 1) (10). In a large-scale randomized clinical trial, a treatment regimen of postoperative radiotherapy combined with cisplatin chemotherapy significantly reduced local and regional recurrences and prolonged disease-free survival (DFS) in patients with advanced OSCC (11). Meanwhile, preoperative intraarterial induction chemotherapy with cisplatin partially reduced tumor volume and improved overall prognosis (12). Considering the side effects and chemoresistance of cisplatin, researchers have developed thousands of cisplatin analogues (13), but only carboplatin and oxaliplatin are currently approved for clinical use. And these platinum-based agents, such as carboplatin, are less effective than cisplatin at the same dose, although they reduce side effects to some extent (14). In addition, other anti-cancer drugs included targeted drugs also do not have the absolute advantages. A phase III clinical trial conducted in Sweden reported that cetuximab plus radiotherapy (RT) produced an overall toxicity comparable to cisplatin plus RT in patients with locoregionally advanced head and neck squamous cell carcinoma (HNSCC), but showed inferiority in terms of local tumor control and treatment prognosis (15). Therefore, cisplatin remains the cornerstone of OSCC chemotherapy.
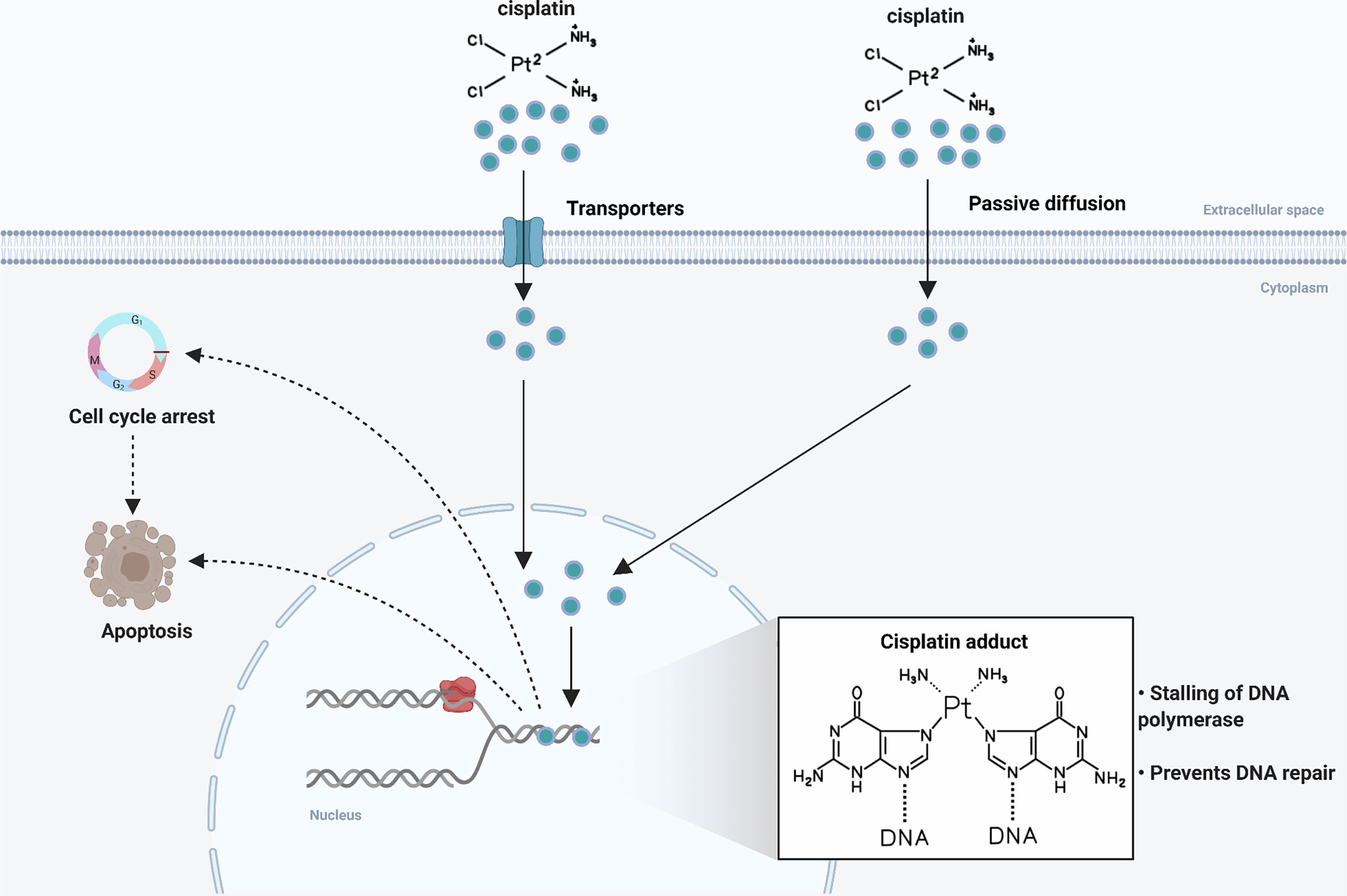
Figure 1 Mechanism of action of cisplatin. Following entry into tumor cells by active transport or passive diffusion, cisplatin forms DNA adducts in the nucleus, leading to cell cycle arrest and cell apoptosis.
In recent years, with the widespread use of platinum-based chemotherapeutic agents, especially cisplatin, many patients have experienced serious toxic side effects. In particular, the development of chemoresistance has severely attenuated the efficacy of cisplatin in the treatment of OSCC. To our knowledge, no review has systematically summarized the mechanisms of cisplatin resistance in OSCC. This paper provides an overview regarding the mechanisms of cisplatin resistance in OSCC based on now-available research findings and summarizes promising strategies to overcome cisplatin resistance in OSCC.
2 Molecular Basis of Cisplatin Resistance in OSCC
2.1 DNA Damage Response and Cisplatin Resistance in OSCC
The combination of DNA damage tolerance and DNA damage repair is critical for tumor cells to counteract cisplatin-induced DNA damage. The major forms of DNA damage repair are mis-match repair (MMR), interstrand cross-link repair (ICR), nucleotide excision repair (NER), base-excision repair (BER), homologous recombination repair (HR), trans-damaged DNA synthesis (TLS) and non-homologous end joining (NHEJ) (16). Enhanced NER has been reported to be associated with cisplatin-resistant phenotypes in a variety of cancers (17). ERCC1 is an important member of the NER pathway, and its expression has been demonstrated to be negatively correlate with the efficacy of platinum-based chemotherapy and the prognosis of several cancers (18–20), including OSCC. For example, ERCC1 expression was enhanced in advanced HNSCC patients who responded poorly to cisplatin-based chemoradiotherapy and had the habit of chewing areca nuts (21); Snail-mediated upregulation of ERCC1 led to cisplatin resistance in OSCC (22). Furthermore, not only the expression level of ERCC1 can regulate the response of tumor cells to cisplatin, but ERCC1 gene polymorphisms have also been proved to make sense. Avinash Tejasvi et al. reported that ERCC1 C118T genotype were more frequently detected in OSCC specimens and that patients carrying this genotype had a worse response to cisplatin (23).
TLS has also been found involved in cisplatin resistance in OSCC. Polη is known to be a DNA polymerase that functions in the TLS pathway, which can bypass the cisplatin-DNA adducts formed during cisplatin chemotherapy and maintain DNA synthesis, thus resisting cisplatin-induced DNA damage. Therefore, endogenous Polη levels may be a potent marker of the efficacy of cisplatin (24). Chen et al. reported that in OSCC, prolonged endoplasmic reticulum (ER) stress upregulated Polη expression and induced the development of cisplatin resistance, whereas the presence or absence of ER stress had little effect on the response to cisplatin of cells lacking POLH, the gene encoding Polη. Interestingly, this study also found that p53 nuclear translocation occurred in endoplasmic reticulum stress-adapted cells and that intracellular cisplatin uptake was not significantly different from control cells, but the accumulation of cisplatin-induced DNA damage was dramatically reduced. Once p53 was knocked down, the cells could hardly tolerate prolonged ER stress and cisplatin treatment (25). This suggested that prolonged ER stress might counteract the effects of cisplatin by repairing DNA damage through the Polη-dependent TLS pathway, and inducing p53 nuclear translocation to make cells tolerate DNA damage. In summary, targeting DNA repair pathways may be a promising approach to enhance chemotherapy sensitivity. However, these findings have not been validated in clinical practice.
2.2 Epigenetic Mechanisms and Cisplatin Resistance in OSCC
The epigenetic modifications include several different forms such as methylation, histone modification, and regulation of non-coding RNAs. Genetic mechanisms and epigenetic mechanisms influence each other and work together to obtain the characteristics of cancer (26). Many pieces of evidence indicate that epigenetic mechanisms are also involved in the occurrence and development of OSCC, including cisplatin resistance in OSCC (Table 1).
2.2.1 Non-Coding RNA-Based Mechanisms
Non-coding RNAs participate in extensive physiological and pathological processes. Increasing evidences indicate that non-coding RNAs, including circular non-coding RNAs (circRNAs), long non-coding RNAs (lncRNAs) and microRNAs (miRNAs), play a vital role in cisplatin resistance of OSCC.
Circ_0109291 had higher expressions in CDDP-resistant OSCC tissues and cells compared with CDDP-sensitive OSCC tissues and cells, and mechanistically promoted proliferation but inhibited the apoptosis of OSCC cells via miR-188-3p/ABCB1 axis (27). LncRNAs act in a similar form to circRNAs. For instance, long non-coding RNA Opa-interacting protein 5 antisense RNA 1 (OIP5-AS1) sponged miR-27b-3p and then influenced the expression of TRIM14, and knockdown of OIP5-AS1 restored the CDDP sensitivity of resistant OSCC cells (29). Futhermore, lncRNAs can modulate chemoresistance of OSCC via influencing epithelial mesenchymal transition (EMT). LncRNA MALAT1 functionally reduced apoptotic cell death by promoting EMT process and activating the PI3K/AKT/m-TOR signaling pathway in cisplatin-resistant OSCC cells (30). Studies of differentially expressed circRNAs or lncRNAs associated with OSCC cisplatin resistance have been emerging. However, these findings seemed to be cell-specific and could not be validated in other OSCC cell lines or clinical samples.
MicroRNAs (miRNAs) can negatively regulate gene expression. Yu et al. reported the difference of miRNAs profile between the cisplatin-resistant TSCC cell lines and their parental cell lines for the first time. Compared with parental cells, there were 19 differential miRNAs in resistant cells, among which 17 were upregulated and 2 were downregulated. In further studies, researchers found that silence of miR-214 and miR-23a or overexpression of miR-21 could reverse chemoresistance against cisplatin in cisplatin-resistant subline (55). MiR-5787 was found downregulated in cisplatin-resistant TSCC cells and functionally target mitochondrial cytochrome c oxidase subunit 3 (MT-CO3). Upregulation of miR-5787 in cisplatin-resistant cells and knockdown its expression in parental cells could regulate the responses to cisplatin of two cell lines respectively, and these results were verified in further in vivo experiments (41).
Previous studies on the non-coding RNAs involved in OSCC cisplatin resistance have mainly focused on function of circRNAs and lncRNAs as sponges for miRNAs and the regulatory role of miRNAs on target genes (Figure 2). Since non-coding RNAs have other complex functions that have been less studied so far, their association with cisplatin resistance of OSCC should be further revealed in the future.
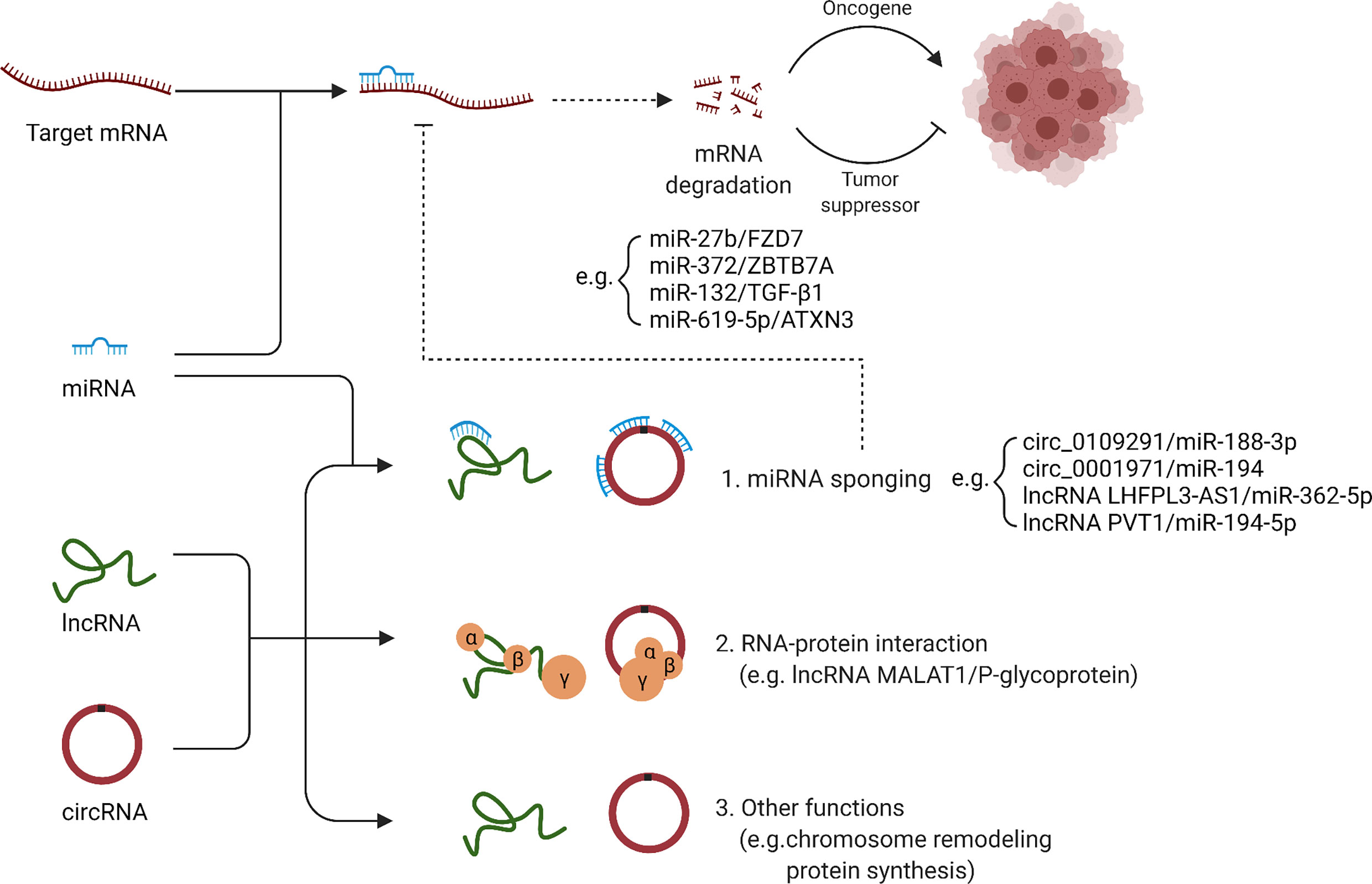
Figure 2 Regulation of cisplatin resistance by ncRNAs: a molecular mechanism. LncRNAs and circRNAs can function as sponges for miRNAs, and thus affect the regulatory role of miRNAs on target genes. In addition, lncRNAs and circRNAs can also interact with proteins, remodel chromosome structure and affect protein synthesis.
2.2.2 Methylation
Methylation is one of the components of epigenetic regulation and the most common methylation modifications are DNA methylation, RNA methylation and histone methylation. Methylation can cause genome instability and mutation, and then make the cancer cells acquire malignant characteristics (56). Wang et al. found the expression level of ten-eleven translocation 1 (TET1) was associated with cisplatin resistance, stem cell properties and o6-methylguanine-DNA methyltransferase (MGMT) methylation in OSCC. The use of TET1-siRNA induced MGMT promoter methylation and cell apoptosis, thereby enhancing the cisplatin sensitivity of OSCC cells with stemness (57). DDX3, a human DEAD-box RNA helicase associated with lymph node metastasis, cell migration and invasion, was found upregulated in cisplatin-resistant OSCC cells and chemotherapy-non-responder OSCC patients. Knockdown of DDX3 restored cisplatin-induced cell death in chemotherapy-resistant cell lines and reduced the proportion of cells with cancer stem cells (CSCs)-like features via suppressing the expression of FOXM1 and NANOG. What’s more, DDX3 could regulate m6A demethylase ALKBH5 directly, which resulted in decreased m6A methylation in FOXM1 and NANOG nascent transcript that contribute to cisplatin resistance in OSCC (52). Therefore, DDX3 was expected to be an effective therapeutic target to overcome cisplatin resistance in OSCC. Together, these studies suggest that cisplatin resistance in OSCC may be associated with methylation of cancer-related genes, and further studies of methylation may be valuable for tracing chemoresistance.
2.3 Programmed Cell Death (PCD) and Cisplatin Resistance in OSCC
Cell death is an indispensable process for maintaining the normal state of living organisms, and can be divided into accidental cell death (ACD) and regulatory cell death (RCD), while RCD is also called programmed cell death (PCD). At present, more and more new forms of PCD other than apoptosis have been discovered, and they are found to be involved in various pathological processes. Emerging evidence suggests that PCD, especially apoptosis and autophagy, is involved in cisplatin resistance in OSCC.
2.3.1 Autophagy
Over the past few decades, researchers have made remarkable breakthroughs with regard to comprehension on what role autophagy plays in systemic health and disease, in particular the recognition that autophagy could inhibit or promote tumor growth and respond to anti-cancer treatments (58–60). Therefore, studies about the role of autophagy will contribute to the development of innovative therapies.
It has been shown that DNA-damaging chemotherapeutic agents such as cisplatin, carboplatin, and 5-fluorouracil could induce autophagy, thereby reducing apoptosis and making tumor cells resistant to chemotherapy. Li et al. found high autophagic flux in cisplatin-resistant OSCC cells, which showed as increased conversion rate of autophagic protein LC3, decreased expression of p62, and increased autophagosomes that were visible by transmission electron microscope (61). High positive rates of CD44, ABCB1, ADAM17 were observed in clinical OSCC specimens and cisplatin-resistant OSCC cells. Interestingly, enhanced autophagy and mitophagy were also detected. After the use of autophagy inhibitors, decreased expression of CD44, ABCB1, ADAM17 and increased sensitivity of cisplatin-resistant OSCC cells to cisplatin could be detected (62). Reactive Oxygen Species (ROS) and cancer-associated fibroblasts (CAFs) have been reported to be associated with tumor progression in OSCC, and studies showed that these two factors also contributed to cisplatin resistance through the induction of autophagy (63, 64).
These results have demonstrated that enhanced autophagy led to cisplatin resistance of OSCC, and inhibition of autophagy might be an effective method to reverse chemotherapy resistance. However, autophagy plays as a double-edged sword in the development of cancer (65, 66). In terms of drug resistance, a study showed that inhibition of autophagy led to cisplatin resistance in laryngeal squamous cell carcinoma (LSCC) (67). Whether autophagy is negatively associated with sisplatin resistance in OSCC remains unknown and requires further investigation.
2.3.2 Apoptosis
Apoptosis is a form of PCD regulated by intrinsic and extrinsic pathways and activated when cells are attacked by special factors like DNA damage (68). During tumorigenesis and progression, cancer cells have to carry anti-apoptotic proteins to maintain cell viability. Nowadays, chemotherapeutic agents mostly rely on triggering apoptosis to induce cell death. Alternations in apoptosis related molecules and pathways would make anti-cancer drugs could not function at conventional concentrations, which means drug resistance.
The Bcl-2 family consists of anti-apoptotic proteins (e.g., Bcl-2 and Bcl-xL) and pro-apoptotic proteins (e.g., Bax, Bak, and Bad). It has been demonstrated that the expressions of Bcl-2-family members have a non-negligible effect on the response of cancers to chemotherapy (69). For example, Bcl-xL was overexpressed in cisplatin-resistant OSCC cell lines and suppression on its upstream regulators to attenuate Bcl-xL expression could promote apoptosis (70). Mcl-1 is also an important member of the Bcl-2 anti-apoptotic family. A survey about the expression of Bcl-2 anti-apoptotic proteins in 68 human cancer cell lines showed that in many solid tumors, the expression level of Mcl-1 was much higher than that of other Bcl-2 anti-apoptotic members (71). Maji et al. found that the Mcl-1 expression was upregulated in both chemoresistant OSCC lines and chemoresistant tumors. Mechanistically, STAT3 and AKT-mediated GSK3β both functioned in regulating the expression of Mcl-1. After blocking Mcl-1 expression by applying siRNA or chemical inhibitors, a significant increase in apoptosis could be observed, suggesting that overexpressed Mcl-1 was related to multiple drug resistance in OSCC (72). Beyond that, reduced expression of pro-apoptotic proteins also contributed to chemoresistance. Bax expression was reduced in cancer tissues of OSCC patients who were resistant to cisplatin-based chemoradiatherapy and two cisplatin-resistant OSCC cells lines. Bax was a downstream gene in p53/Akt pathway, the application of Akt inhibitors released the inhibition of Bax and increased the proportion of apoptosis among cisplatin-resistant OSCC cells (53). In summary, restoration of the inhibited apoptotic process may be an effective strategy to induce OSCC cell death.
Survivin is a member of the inhibitor of apoptosis protein (IAP) family, and recognized as a potential predictive biomarker for cancers for its high expression (73, 74). Münscher et al. found survivin was expressed at high levels in HNSCC, and positively correlated with the malignant characteristics (75). Another study reported that nicotine reduced cisplatin-induced apoptosis in oral cancer cells, while this protective effect could be attenuated when survivin was inhibited (76). YM155 is a small molecule inhibitor of survivin that has been found to be effective and selective in recent years. Experiments showed that, YM155 induced apoptosis and autophagic cell death in OSCC cells by effectively downregulating survivin in the cytoplasm and upregulating Beclin1 through the Akt/mTOR pathway (77). Meanwhile, the combination of YM155 with cisplatin yielded better anti-cancer effects than monotherapy (78). More recently, an antagonist of IAPs, Debio 1143, has shown potential to enhance the antitumor activity of cisplatin and radiotherapy in HNSCC, and a phase III clinical trial will be conducted to demonstrate its efficacy (79).
In general, targeting anti-apoptotic molecules or promoting the expression of pro-apoptotic molecules may be an effective mechanism to reverse chemoresistance in OSCC.
2.4 The Tumor Microenvironment (TME) and Cisplatin Resistance in OSCC
The tumor microenvironment consists of the tumor cells themselves, multiple stromal cells and extracellular matrix, and is usually characterized by hypoxia, and immunosuppression (80–82). The interactions of various cells and cytokines form a complex network of mechanisms (83), and recent studies have revealed that TME is responsible for the induction of multidrug resistance in OSCC (Figure 3).
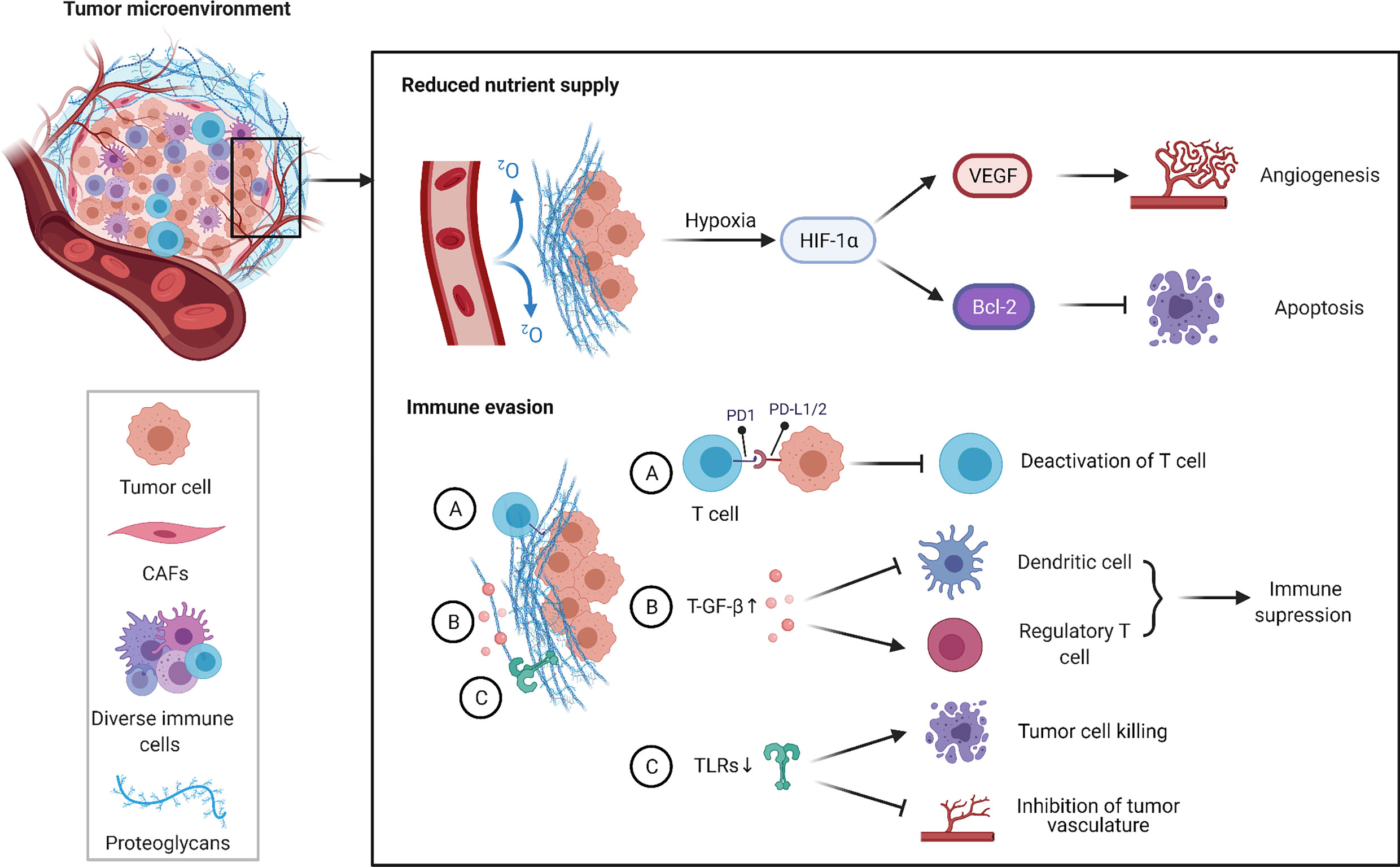
Figure 3 TME and cisplatin resistance in OSCC. TME is typically characterized by hypoxia and immunosuppression. Hypoxia induces HIF-1α-mediated signaling cascades, including induction of VEGF expression to promote tumor angiogenesis in hypoxic conditions and inhibition of apoptosis by regulating the expression of Bcl-2 anti-apoptotic family. In addition, a large number of cellular factors released by cancer cells and various bystander cells cause immune evasion by regulating the production and function of immune cells. Overall, the above pathways contribute to the cisplatin resistance of OSCC.
2.4.1 Hypoxic Microenvironment
OSCC is a solid tumor with a high hypoxia degree inside and this hypoxic condition may cause patients respond poorly to anticancer treatments (84). Hypoxia can activate the expression of HIFs to maintain cell viability, especially HIF-1α, which is widely expressed in different cancer cells (85). High expression of HIF-1α has been demonstrated to be associated with the malignant features of OSCC, and patients with high-expression levels of HIF-1α tend to have a shorter overall survival (86). HIF-1α can activate the transcription of multiple genes under hypoxic conditions, including vascular endothelial growth factor (VEGF) (87). To some extent, HIF-1α promotes angiogenesis in tumors under hypoxic environment by inducing the expression of VEGF, thereby regulating the balance of oxygen supply and metabolism within the tumor, and improving the survival of tumor cells (88). Moreover, HIF-1α participates in the regulation of apoptosis. For example, HIF-1α inhibited cell apoptosis by promoting the expression of the anti-apoptotic protein Bcl-2 while suppressing the expression of the pro-apoptotic proteins Bax and Bak, and the application of siRNA to downregulate HIF-1α expression restored sensitivity to chemoradiotherapy of OSCC cells (89, 90). Qi et al. reported that under hypoxic conditions, the synergistic application of metformin and cisplatin suppressed the expression of the upstream transcription factor NK-κB of HIF-1α, thereby blocking the synthesis of HIF-1α to enable cisplatin to exert its anticancer effects without difficulty (91). These studies validate the relationship between HIF-1α and resistance to cisplatin, suggesting that hypoxia significantly affects cisplatin therapy and further investigation of potential molecular mechanisms in hypoxia-induced chemoresistance will provide new promising directions for the treatment of OSCC.
2.4.2 Immune Microenvironment
In recent years, most HNSCC have been shown to have highly immunosuppressive TME which allows the tumor to escape the host immune response. What’s more, tumor immune escape is also a crucial contributor for the formation of multi drug resistance, because drug-resistant cells are more likely to evade recognition and killing by the immune system than drug-sensitive cells (92). Programmed death 1 (PD-1) is widely expressed on the surface of immune cells such as T cells, B cells and macrophages, whereas programmed death ligand 1 (PD-L) is expressed in a variety of tumor cells. The combination of PD-L and PD-1 on the surface of T cells can inhibit the activity of killer T cells and even induce their apoptosis, thus allowing tumor cells to gain immune escape (93). A global proteomic profiling of cisplatin-sensitive and cisplatin-resistant OSCC cell lines showed CMTM6 was the top-ranked upregulated protein in cisplatin-resistant cells (94). What’s more, it had been demonstrated that in HNSCC, CMTM6 positively correlated with PD-L1 expression and both of CMTM6 and PD-L1 were associated with a worse prognosis. Knockdown of CMTM6 resulted in decreased PD-L1 expression and increased tumor-infiltrating CD4+CD8+ T cells (Tregs), which consequently improved antitumor immunity and cisplatin-induced apoptosis; whereas overexpression of CMTM6 resulted in increased PD-L1 expression and decreased sensitivity of OSCC cells to cisplatin (95, 96). The relevance of PD-L1, another ligand of PD-1, to cisplatin resistance in OSCC has also been reported. Sudo, et al. established a cisplatin-resistant OSCC cell line, HSC-2, and found that cisplatin upregulated the gene expression levels of PD-L2, ABCG2 in HSC-2 in a time-dependent manner. In addition, STAT1/3 mediated the induction of PD-L2 by cisplatin, and PD-L2-positive cells have higher metastatic and invasive potential compared to PD-L2-negative cells (97). These studies suggested that anti-PD-L immunotherapy may be valuable for cisplatin-resistant OSCC patients.
TGF-β is an important regulator of immune homeostasis in tumor microenvironment, and it can create an immunosuppressive TME by regulating the production and function of multiple immune cells (such as regulatory T cells and dendritic cells) (98). In OSCC, the application of miRNAs mimics targeting TGF-β1 effectively reduced the gene expression of TGF-β1, inhibited growth, invasion, and metastasis of tumor cells and increased the sensitivity of drug-resistant cells to cisplatin (42). Based on this study, we can speculate that the high expression of TGF-β1 may lead to cisplatin resistance in OSCC by suppressing immune cell function.
In the tumor microenvironment, aberrant expression of Toll-like receptors (TLRs) not only gives tumor cells the opportunity to escape the host immune response, but also contributes to tumor development by promoting proliferation and angiogenesis and resisting apoptosis (99, 100). Current preclinical trials have shown that monotherapy or combination therapeutic strategies of applying TLRs modulators can effectively improve tumor prognosis (101). In OSCC, the application of Poly(I:C), an agonist of TLR3, reduced drug efflux by inhibiting ABC transporters in tumor cells, activated immune cells in TME, induced delayed tumor cell apoptosis that based on caspase3 pathway, and enhanced the anti-cancer effect of low-dose cisplatin with reduced adverse side effects (102). This chemoimmunotherapeutic strategy of sequential application of the TLR3 agonist Poly(I:C) and low-dose cisplatin was suitable for long-term treatment of OSCC patients with poor physical status and bad response to conventional chemotherapy drugs.
Understanding the role played by immune checkpoints and the tumor microenvironment in anticancer therapy could help improve single-drug-based chemotherapy regimens and thus facilitate the development of a novel chemotherapy strategy that incorporates immunotherapy.
2.5 Transport and Cisplatin Resistance in OSCC
Anti-cancer drugs must reach an appropriate concentration at the target site in order to exert their killing effect in tumor cells. Predictably, if the concentration in cells does not reach the concentration required for treatment for some reason, the anti-cancer drugs will not function, in which case drug resistance will occur. ATP binding cassette (ABC) transporters are well known for their role to enable drug efflux in multidrug resistance (MDR). They serve as transmembrane proteins that facilitate the efflux of anticancer drugs from cancer cells hence reducing intracellular drug levels. In addition, extracellular vesicles also play a key role in cancer chemoresistance as natural carriers of signals in the organism. For example, exosomes can wrap therapeutic drugs and export them out of the cell, or transport drug efflux pumps into the cell (103). The mechanisms of cisplatin resistance based on transport process in OSCC are reviewed below (Figure 4).
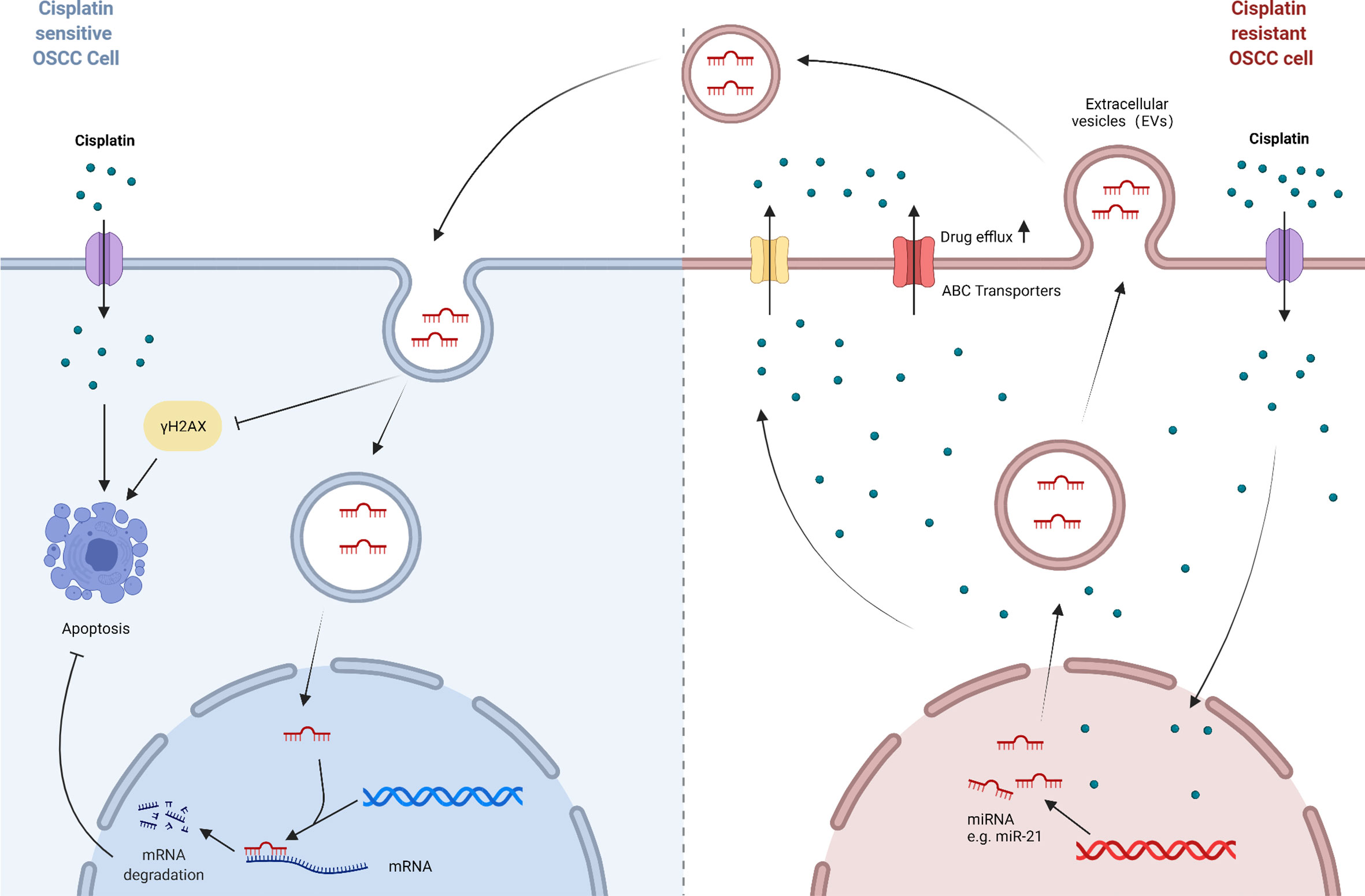
Figure 4 A model of transport process-mediated cisplatin resistance in OSCC. ABC transporters act as transmembrane proteins that result in the efflux of cisplatin from OSCC cells and reduce intracellular drug levels. And extracellular vesicles act as natural carriers of biological signals that can deliver substances such as functional RNAs from cisplatin-resistant OSCC cells to parental cells, thus enabling the propagation of cisplatin resistance in OSCC cells.
2.5.1 ABC Transporters
Currently, the most studied ABC transporters associated with drug resistance include ABCB1 (P-glycoprotein/P-gp/MDR1), ABCG2 (Breast Cancer Resistance Protein/BCRP) and ABCC1 (Multidrug Resistance Protein1/MRP1) (104). However, the regulatory roles of ABC transporters in cisplatin-resistant OSCC are still poorly understood.
Choi, et al. established three cisplatin-resistant OSCC cell lines and found elevated gene expression of BCRP and MDR1 in these three cell lines (105). Lu et al. found higher expression of ABCC1 and ABCG2 in specimens from OSCC patients who had poor differentiation and received chemotherapy compared to those from patients with well differentiation and was not treated with chemotherapy. In addition, in vitro experiments revealed that the Hedgehog signaling pathway was activated in OSCC cells resistant to cisplatin and 5-FU. Overexpression of SHH, a key gene in the Hh pathway, resulted in increased ABCG2 expression, which suggested that the Hh pathway promoted multidrug resistance in OSCC by modulating the ABC transporters (106). For decades, small molecule transport inhibitors based on strategies targeting ABC transporters have been developed to avoid drug efflux. Unfortunately, these inhibitors have been proven to have only a limited effect in clinical trials (107, 108). The reason for the failure might be that the inhibitors was targeted and compensatory up-regulation of other ABC transporters could still lead to the efflux of chemotherapeutic drugs. Therefore, a comprehensive study of the ABC transporter family is necessary to understand the function of other proteins in this family. In the meantime, agents that directly target the upstream regulators should be developed to avoid the compensatory mediation between downstream ABC transporters.
2.5.2 Extracellular Vesicles
Extracellular vesicles (Evs) can be subdivided into exosomes (30–100 nm), microvesicles (MVs) (100–1,000 nm), and the oncosomes (1–10 μm), but the way to divide these three by size is not absolute, a more reasonable method is to distinguish them by their origin and function. Extracellular vesicles can deliver cellular cargoes such as proteins, nucleic acids, lipids and other biomolecules or pharmacological compounds to recipient cells, thus affecting the biological functions of recipient cells (109, 110). Currently, exosomes and microvesicles are more studied in OSCC chemoresistance (111, 112). It has been investigated that miR-21 is involved in multidrug resistance of various cancers (113, 114). Liu, et al. found that culturing cisplatin-sensitive OSCC cells with conditioned media obtained from cisplatin-resistant OSCC cells resulted in decreased sensitivity of parental cells to cisplatin and reduced expression of γH2AX, a protein marker characterizing DNA damage. However, application of conditioned media obtained from resistant cells treated with the exosome inhibitor GW4869 reversed this phenomenon. Further mechanistic studies showed that exosomes derived from resistant cells delivered miR-21 to parental cells. MiR-21 targeted PTEN and PDCD4 in cispaltin-sensitive cells, with a resultant inhibition of cisplatin-induced apoptosis (115). Not coincidentally, Chen et al. reported that miR-21 was the most abundantly expressed miRNA in CSC_EVs derived from OSCC cells (43). The application of Ovatodiolide (OV), a newly discovered bioactive component with anti-cancer activity, reduced the expression of oncogenic markers like PI3K, STAT3 and miR-21, and effectively inhibited CSC_EVs mediating cisplatin resistance and tumorigenesis (116). Thus, extracellular vesicles and the cellular cargoes they carry, such as miR-21, can be used as candidate targets for counteracting drug resistance. Moreover, researchers have developed innovative anti-cancer therapies that utilize the biological functions of extracellular vesicles, such as exosomes drug delivery systems (117).
2.6 Other Possible Mechanisms of Cisplatin Resistance in OSCC
Epithelial-mesenchymal transition (EMT). Many studies have found that chemo-resistant tumor cells often exhibit a transition from epithelial to mesenchymal, with the disrupted intercellular adhesion, resulting in a greater migratory and invasive capacity of tumor cells and correlating with worse prognosis of patients (105, 118–120). Therefore, blocking the EMT process may improve the antitumor efficacy of chemotherapeutic agents (121, 122). Chen, et al. reported that in OSCC, high expression of FOXD1 induced EMT and resistance to cisplatin via the CYTOR/miR-1252-5p/miR-3148/LPP signaling axis, whereas blocking FOXD1 expression could interfere with CYTOR-dependent signaling events, ultimately reducing the EMT phenotype and restoring sensitivity of drug-resistant cell lines to cisplatin (36).
Cancer stem cells (CSCs). According to the cancer stem cell hypothesis, the presence of cancer stem-like cells contributes to tumor recurrence and drug resistance, and there is a correlation between stemness-related genes and poor prognosis (123, 124). CD10 is a newly identified surface marker of cancer-associated fibroblasts, which maintains cancer stemness and facilitates cancer therapy resistance. It was experimentally demonstrated that CD10-positive OSCC cells exhibited enhanced self-renewal ability, tumorigenicity, and poor response to cisplatin. And further studies revealed that CD10 might act through the Hedgehog pathway to enhance cell stemness and cisplatin resistance (125). Naik et al. reported that, in OSCC, cisplatin could induce FaDu cells to exhibit a remarkable stemness feature, and the positive rate of the CSC marker CD44 in cisplatin-resistant FaDu cells reached a peak of 90%. Mechanistically, enhanced autophagy and mitochondrial phagocytosis promoted the accumulation of β-catenin in OSCC cells to activate CSC properties, and thus leading to cisplatin resistance (62).
p53. As a well-known tumor suppressor gene, p53 serves as a hub for cellular stress response by regulating the transcription of multiple downstream targets. p53 mutations are common in human cancers, and mutant p53 not only leads to tumor aggressiveness but also to therapeutic resistance (126, 127). Temam et al. reported that p53 mutations were closely associated with reduced efficiency of platinum- and fluorouracil-based induction chemotherapy in advanced HNSCC (128). In another study, HNSCC cells with cytoplasmic mutant p53 were generally more resistant to cisplatin than cells with nuclear mutant p53. As a result, cytoplasmic p53 mutant proteins promoted upregulation of intracellular ABC transporter proteins (ABCC2 and ABCG2) and increased metabolic activity (129).
Furthermore, several diverse studies about cisplatin resistance in OSCC also merit mention, but the underlying mechanisms do not fall into the aforementioned categories (Table 2). For example, a specific region on the endoplasmic reticulum (ER) membrane protein p22phox could bind to cisplatin directly, thus blocking cisplatin entering into the nucleus and inducing DNA damage in OSCC cells (130, 142, 143). Downregulated cylindromatosis (CYLD) contributed to cisplatin resistance of OSCC cells via excessive activation of NF-κB (131). Although these findings are mostly inferred from basic experiments, they provide motivation and corroboration for researchers to improve clinical treatment outcomes for OSCC.
3 Strategies to Reverse Cisplatin Resistance in OSCC and Novel Therapeutic Opportunities
3.1 Enhancing Drug Influx: Drug Delivery Systems
Appropriate drug concentration is an important prerequisite to ensure anticancer efficacy. Recently, researchers have attempted to use nanoparticles (NPs) as carriers for chemotherapeutic drug delivery. Owing to ultra-small size and potent adsorption properties, on the one hand, NPs can directly target tumor sites, which results in the localized accumulation of drugs and prolonged drug release time; on the other hand, the targeting of NPs reduces the toxicity of loaded drugs towards normal tissues (144, 145).
Novel N-vinylpyrrolidone (NVP)/acrylic acid (AA) nanoparticles have been revealed to bind and deliver cisplatin into cells via coordination bonds. Cisplatin bound to NPs showed higher uptake rate in OSCC cells compared to free cisplatin. This drug delivery system was non-toxic to normal oral epithelial cells, but induced a higher percentage of early apoptosis in OSCC cells and reduced the localized inflammatory response elicited by apoptosis (146). Wang et al. synthesized CDDP loaded and ligand-modified PLGA-PEG/NR7 nanoparticles, which were capable of targeting OSCC cells highly expressing epidermal growth factor receptor (EGFR). Due to the hydrophilic PEG shell and hydrophobic PLGA core of the polymeric NPs, the CDDP loaded therein were allowed for a slow and stable release. In addition, the modified NPs had a higher cellular uptake rate compared to the non-targeting nanoparticles and significantly enhanced the anticancer effect by actively delivering more cisplatin to the tumor site (147).
Overall, nanomedicine delivery systems are promising in enhancing the efficacy of cisplatin and reversing cisplatin resistance in OSCC. Unfortunately, cisplatin-loaded nanoparticles have not yet gained clinical approval.
3.2 Combination Therapeutic Regimens
3.2.1 Combination With Cytotoxic Chemotherapeutic Agents
Novel combination regimens involving cisplatin and other cytotoxic agents have been investigated to improve therapeutic outcome of advanced OSCC. Arsenic trioxide (ATO) is one of the few FDA-approved commercially available inorganic non-radioactive anticancer drugs (148), and studies have demonstrated that arsenic trioxide has broad anticancer activity against multiple tumors (149–151). More importantly, the combination of ATO and cisplatin exhibited remarkable synergistic effects (152–154). In OSCC, Kotowski et al. reported that the combination of ATO and CDDP exhibited stronger cytotoxic effects compared to monotherapy (155). Nakaoka et al. found that ATO/CDDP increased the proportion of cells that underwent apoptosis and cell cycle arrest. The potential mechanism could be that ATO/CDDP led to a decrease in mitochondrial membrane potential by stimulating the production of ROS in tumor cells, which subsequently activated the caspase-3/7 signaling pathway, and resulted in cell apoptosis. The experimental results of downregulated cytochrome c, anti-apoptotic Bcl-2 and XIAP also provided a support for this hypothesis (156). However, these results were derived from in vitro experiments, and no publications or clinical trials have reported in the subsequent nearly decade that ATO might benefit as a chemosensitizer for cisplatin-based chemotherapy in OSCC, and its efficacy still requires further validation.
With the increasing availability of new cytotoxic agents, investigators have tried a wide range of cisplatin-based combination chemotherapy regimens in clinical trials of OSCC, such as cisplatin plus 5-fluorouracil (PF) combination, cisplatin plus paclitaxel (PP) combination (157), and docetaxel, cisplatin plus 5-FU (TPF) combination (158). However, PP and PF regimens have been proven to show no difference in survival (158). Although TPF regimen has shown superiority over PF regimen and to be favorable in reducing tumor-induced dysphagia and distant metastasis (159), the superiority has been questioned in recent years and there is no definitive evidence that it is superior to concomitant treatment in terms of survival (160–162). In addition, TPF regimens carry a high risk of toxicity, which can be fatal for many patients with co-morbidities, with a treatment-related mortality rate of up to 6% (163). Therefore, the exact role of doublet or triplet chemotherapy regimens requires further exploration, and certain criteria should be established to identify the patient subgroup that would benefit from them, thereby improving treatment outcomes and reducing treatment risks.
3.2.2 Combination With Molecular Targeted Agents
In studies of OSCC tumor heterogeneity, a number of genes and molecules associated with poor prognosis have been identified to be commonly altered, and researchers view them as potential targets for OSCC treatment.
EGFR is a member of the human epidermal growth factor receptor (HER) family. Currently, there are two main types of medications that target EGFR in clinical practice, which are monoclonal antibodies represented by cetuximab and tyrosine kinase inhibitors (TKIs) (164). In a multi‐centric phase III clinical trial, a regimen of cetuximab combined with platinum–fluorouracil improved treatment response rate, PFS and OS in patients with recurrent and/or metastatic squamous cell carcinoma of the head and neck (R/M-SCCHN) compared with platinum–fluorouracil chemotherapy alone (165). However, the benefits of the combined regime was not absolute, in another phase III clinical trial, the addition of cetuximab failed to improve PFS or OS in patients with stage III-IV OSCC receiving cisplatin and radiation therapy (166). When it comes to EGFR-TKIs, regrettably, there was insufficient evidence showing the addition of EGFR-TKIs to standard therapies could provide overall therapeutic benefits. Additionally, cetuximab resistance has been reported, and the use of EGFR-mAb and EGFR-TKIs may lead to increased skin toxicity (167, 168).
Apart from EGFR, targeting other molecules can also benefit the anticancer treatment. Stathmin is a downstream molecule in the PI3K/AKT/mTOR signaling pathway, and OSCC patients with high stathmin expression responded poorly to TPF chemotherapy regimens. The combination of PI3K inhibitor, BKM-120, with TPF decreased the expression and phosphorylation of stathmin, and induced cell cycle arrest, thereby increasing the proportion of apoptotic tumor cells and inhibiting the growth of xenografts (169). The combination of Bruton’s tyrosine kinase (BTK) inhibitors, ibrutinib, and cisplatin were capable of suppressing stemness characteristics and promoting apoptosis in OSCC cells (170).
Since the above findings are mostly obtained from prospective studies, the simultaneous or sequential combination of targeted agents and conventional chemotherapeutic agents remains controversial, and the combination strategies need further clinical study.
3.2.3 Combination With Immunotherapeutic Drugs
Recent studies have shown that early immunotherapy is of great benefit to the prognosis of OSCC patients. New generation of immunotherapy applies immune checkpoint inhibitors to alter the immunosuppressive tumor microenvironment and reactivate the host immune response to tumor cells, thereby inhibiting tumor cell proliferation and even triggering apoptosis (171). In 2016, two immune checkpoint inhibitors, pembrolizumab and nivolumab, were approved by FDA for advanced R/M-SCCHN patients who had disease progression during or following treatment with cisplatin (172–174). Following that, multiple immune checkpoint inhibitors (ICIs) have been applied to the treatment of OSCC, among which PD-1/PD-L1 targeted are the most numerous. In a systematic review of eight clinical trials applying ICIs, the authors reported that the standard therapy had poor efficacy in PD-L1-positive R/M SCCHN patients, while the immunotherapy had a better performance. The overall survival (OS) of R/M SCCHN patients using PD-1 checkpoint inhibitors ranged from 7.5 to 14.9 months, which was significantly longer compared to those receiving standard therapy, and the life quality of patients was significantly improved due to immunotherapy producing fewer systemic toxicities (175). Immune checkpoint inhibitors and cisplatin exert anticancer activity through different mechanisms, and immunochemotherapy combining the two may be beneficial in the treatment of advanced OSCC. However, Tringale et al. reported that the overall efficiency of current immune checkpoint inhibitors for R/M SCCHN is not favorable due to the high cost of treatment, and further research is needed to minimize treatment costs and improve treatment outcomes (176).
3.2.4 Combination With Potential Anti-Cancer Drugs: Natural Products
Since a long time, natural products have served as one of the most important sources of anti-cancer drugs. Some anticancer drugs that possess definite anticancer activity and have been widely used, such as hydroxycamptothecin and paclitaxel (PTX), are natural products, secondary metabolites and/or their structural analogues. Recently, combination therapies of natural products and traditional anticancer drugs have shown potential on improving therapeutic effect.
Sulforaphane (SF) and curcumin (CRM) are both natural compounds derived from plants and commonly employed as antioxidants in anticancer therapy (177). The combination of SF and CDDP inhibited the growth of SCCHN cells and made the cytotoxicity of CDDP 2 times magnified (178). While the combination therapy with liposomal curcumin and cisplatin also had an enhanced growth inhibitory effect on HNSCC compared to monotherapy (179). In addition, several natural products, ursolic acid (180), plumbagin (181) and epigallocatechin gallate (182) are also worth mentioning, which exhibit chemosensitizing function in cisplatin-resistant OSCC through similar mechanisms. Although the potential of natural products in anticancer therapy has been demonstrated in many preclinical trials, there are still many hurdles to overcome before formal clinical application, such as the low water solubility, poor stability, and low oral availability. Therefore, the physicochemical characterization of natural products needs to be further improved in the future to achieve effective clinical application.
4 Conclusion and Perspectives
Resistance to cisplatin remains a major challenge that hinders the success of OSCC treatment. According to current knowledge, multiple factors such as DNA damage and repair, transport process, and programmed cell death are involved in initial or acquired resistance to platinum-based drugs in OSCC. Recently, new factors such as epigenetic biological processes and tumor microenvironment have also received increasing attention in the study of the mechanisms of chemoresistance in OSCC. Increased understanding of the underlying molecular mechanisms of cisplatin resistance is essential to predict, prevent, and reverse chemoresistance. Drug resistance is essentially a form of tumor heterogeneity, and the molecules that have been reported to be associated with OSCC cisplatin resistance may not be representative of the entire resistant subset due to small sample size or lack of clinical validation. In the future, a large-scale screening may be needed to identify biomarkers associated with cisplatin resistance in OSCC and validate them prospectively before treatment, thus identifying cases that may benefit from cisplatin-based treatment and providing more rational personalized treatment regimens for resistant patients, which could improve treatment outcomes and increase survival in refractory OSCC patients.
Author Contributions
SML and LG gathered the related literature. YLC drafted the first manuscript and created the graphs. KQZ and WHR designed this review and contributed to critical revision of the manuscript. All authors contributed to the article and approved the submitted version.
Funding
This work was supported by the Postdoctoral Science Foundation of China (2017M622145), Natural Science Foundation of Shandong Province (ZR2018BH021), Science and Technology Project of Qingdao West Coast New Area (2019–60).
Conflict of Interest
The authors declare that the research was conducted in the absence of any commercial or financial relationships that could be construed as a potential conflict of interest.
Publisher’s Note
All claims expressed in this article are solely those of the authors and do not necessarily represent those of their affiliated organizations, or those of the publisher, the editors and the reviewers. Any product that may be evaluated in this article, or claim that may be made by its manufacturer, is not guaranteed or endorsed by the publisher.
Acknowledgments
We acknowledge support from the Department of Oral and Maxillofacial Surgery, the Affiliated Hospital of Qingdao University, Qingdao, Shandong, China. Figures created with BioRender (https://biorender.com/).
References
1. Sung H, Ferlay J, Siegel RL, Laversanne M, Soerjomataram I, Jemal A, et al. Global Cancer Statistics 2020: GLOBOCAN Estimates of Incidence and Mortality Worldwide for 36 Cancers in 185 Countries. Ca Cancer J Clin (2021) 71(3):209–49. doi: 10.3322/caac.21660
2. Sarradin V, Siegfried A, Uro-Coste E, Delord J-P. WHO Classification of Head and Neck Tumours 2017: Main Novelties and Update of Diagnostic Methods. Bull Cancer (2018) 105(6):596–602. doi: 10.1016/j.bulcan.2018.04.004
3. Fauzi FH, Hamzan NI, Rahman NA, Suraiya S, Mohamad S. Detection of Human Papillomavirus in Oropharyngeal Squamous Cell Carcinoma. J Zhejiang Univ Sci B (2020) 21(12):961–76. doi: 10.1631/jzus.B2000161
4. Zanoni DK, Montero PH, Migliacci JC, Shah JP, Wong RJ, Ganly I, et al. Survival Outcomes After Treatment of Cancer of the Oral Cavity (1985-2015). Oral Oncol (2019) 90:115–21. doi: 10.1016/j.oraloncology.2019.02.001
5. Gharat SA, Momin M, Bhavsar C. Oral Squamous Cell Carcinoma: Current Treatment Strategies and Nanotechnology-Based Approaches for Prevention and Therapy. Crit Rev Ther Drug Carrier Syst (2016) 33(4):363–400. doi: 10.1615/CritRevTherDrugCarrierSyst.2016016272
6. Rosenberg B, VanCamp L, Trosko JE, Mansour VH. Platinum Compounds: A New Class of Potent Antitumour Agents. Nature (1969) 222(5191):385–6. doi: 10.1038/222385a0
7. Prestayko AW, D'Aoust JC, Issell BF, Crooke ST. Cisplatin (Cis-Diamminedichloroplatinum II). Cancer Treat Rev (1979) 6(1):17–39. doi: 10.1016/S0305-7372(79)80057-2
8. Einhorn LH. Treatment of Testicular Cancer: A New and Improved Model. J Clin Oncol (1990) 8(11):1777–81. doi: 10.1200/JCO.1990.8.11.1777
9. Gralla RJ, Casper ES, Kelsen DP, Braun DW Jr, Dukeman ME, Martini N, et al. Cisplatin and Vindesine Combination Chemotherapy for Advanced Carcinoma of the Lung: A Randomized Trial Investigating Two Dosage Schedules. Ann Internal Med (1981) 95(4):414–20. doi: 10.7326/0003-4819-95-4-414
10. Sancho-Martínez SM, Prieto-García L, Prieto M, López-Novoa JM, López-Hernández FJ. Subcellular Targets of Cisplatin Cytotoxicity: An Integrated View. Pharmacol Ther (2012) 136(1):35–55. doi: 10.1016/j.pharmthera.2012.07.003
11. Cooper JS, Pajak TF, Forastiere AA, Jacobs J, Campbell BH, Saxman SB, et al. Postoperative Concurrent Radiotherapy and Chemotherapy for High-Risk Squamous-Cell Carcinoma of the Head and Neck. N Engl J Med (2004) 350(19):1937–44. doi: 10.1056/NEJMoa032646
12. Kovács AF. Response to Intraarterial Induction Chemotherapy: A Prognostic Parameter in Oral and Oropharyngeal Cancer. Head Neck (2006) 28(8):678–88. doi: 10.1002/hed.20388
13. Johnstone TC, Suntharalingam K, Lippard SJ. The Next Generation of Platinum Drugs: Targeted Pt(II) Agents, Nanoparticle Delivery, and Pt(IV) Prodrugs. Chem Rev (2016) 116(5):3436–86. doi: 10.1021/acs.chemrev.5b00597
14. Go RS, Adjei AA. Review of the Comparative Pharmacology and Clinical Activity of Cisplatin and Carboplatin. J Clin Oncol (1999) 17(1):409–22. doi: 10.1200/JCO.1999.17.1.409
15. Gebre-Medhin M, Brun E, Engström P, Haugen Cange H, Hammarstedt-Nordenvall L, Reizenstein J, et al. ARTSCAN III: A Randomized Phase III Study Comparing Chemoradiotherapy With Cisplatin Versus Cetuximab in Patients With Locoregionally Advanced Head and Neck Squamous Cell Cancer. J Clin Oncol (2021) 39(1):38–47. doi: 10.1200/JCO.20.02072
16. Tian H, Gao Z, Li H, Zhang B, Wang G, Zhang Q, et al. DNA Damage Response–A Double-Edged Sword in Cancer Prevention and Cancer Therapy. Cancer Lett (2015) 358: (1):8–16. doi: 10.1016/j.canlet.2014.12.038
17. Duan M, Ulibarri J, Liu KJ, Mao P. Role of Nucleotide Excision Repair in Cisplatin Resistance. Int J Mol Sci (2020) 21(23):9248. doi: 10.3390/ijms21239248
18. Li W, Jie Z, Li Z, Liu Y, Gan Q, Mao Y, et al. ERCC1 siRNA Ameliorates Drug Resistance to Cisplatin in Gastric Carcinoma Cell Lines. Mol Med Rep (2014) 9(6):2423–8. doi: 10.3892/mmr.2014.2112
19. Du P, Zhang X, Liu H, Chen L. Lentivirus-Mediated RNAi Silencing Targeting ERCC1 Reverses Cisplatin Resistance in Cisplatin-Resistant Ovarian Carcinoma Cell Line. DNA Cell Biol (2015) 34(7):497–502. doi: 10.1089/dna.2015.2805
20. He Y, Chen D, Yi Y, Zeng S, Liu S, Li P, et al. Histone Deacetylase Inhibitor Sensitizes ERCC1-High Non-Small-Cell Lung Cancer Cells to Cisplatin via Regulating miR-149. Mol Ther Oncolytics (2020) 17:448–59. doi: 10.1016/j.omto.2020.05.001
21. Chiu TJ, Chen CH, Chien CY, Li SH, Tsai HT, Chen YJ. High ERCC1 Expression Predicts Cisplatin-Based Chemotherapy Resistance and Poor Outcome in Unresectable Squamous Cell Carcinoma of Head and Neck in a Betel-Chewing Area. J Trans Med (2011) 9:31. doi: 10.1186/1479-5876-9-31
22. Hsu DS, Lan HY, Huang CH, Tai SK, Chang SY, Tsai TL, et al. Regulation of Excision Repair Cross-Complementation Group 1 by Snail Contributes to Cisplatin Resistance in Head and Neck Cancer. Clin Cancer Res (2010) 16: (18):4561–71. doi: 10.1158/1078-0432.CCR-10-0593
23. Avinash Tejasvi ML, Maragathavalli G, Putcha UK, Ramakrishna M, Vijayaraghavan R, Anulekha Avinash CK. Impact of ERCC1 Gene Polymorphisms on Response to Cisplatin Based Therapy in Oral Squamous Cell Carcinoma (OSCC) Patients. Indian J Pathol Microbiol (2020) 63(4):538–43. doi: 10.4103/IJPM.IJPM_964_19
24. Ummat A, Rechkoblit O, Jain R, Roy Choudhury J, Johnson RE, Silverstein TD, et al. Structural Basis for Cisplatin DNA Damage Tolerance by Human Polymerase η During Cancer Chemotherapy. Nat Struct Mol Biol (2012) 19(6):628–32. doi: 10.1038/nsmb.2295
25. Chen CY, Kawasumi M, Lan TY, Poon CL, Lin YS, Wu PJ, et al. Adaptation to Endoplasmic Reticulum Stress Enhances Resistance of Oral Cancer Cells to Cisplatin by Up-Regulating Polymerase η and Increasing DNA Repair Efficiency. Int J Mol Sci (2020) 22(1):355. doi: 10.3390/ijms22010355
26. Hanahan D, Weinberg RA. Hallmarks of Cancer: The Next Generation. Cell (2011) 144(5):646–74. doi: 10.1016/j.cell.2011.02.013
27. Gao F, Han J, Wang Y, Jia L, Luo W, Zeng Y. Circ_0109291 Promotes the Cisplatin Resistance of Oral Squamous Cell Carcinoma by Sponging miR-188-3p to Increase ABCB1 Expression. Cancer Biother Radiopharm (2020). doi: 10.1089/cbr.2020.3928
28. Tan X, Zhou C, Liang Y, Lai YF, Liang Y. Circ_0001971 Regulates Oral Squamous Cell Carcinoma Progression and Chemosensitivity by Targeting miR-194/miR-204 In Vitro and In Vivo. Eur Rev Med Pharmacol Sci (2020) 24(5):2470–81. doi: 10.26355/eurrev_202003_20515
29. Xiao Z, Li J, Jin Q, Liu D. Long Non-Coding RNA OIP5-AS1 Contributes to Cisplatin Resistance of Oral Squamous Cell Carcinoma Through the miR-27b-3p/TRIM14 Axis. Exp Ther Med (2021) 21(4):408. doi: 10.3892/etm.2021.9839
30. Wang R, Lu X, Yu R. lncRNA MALAT1 Promotes EMT Process and Cisplatin Resistance of Oral Squamous Cell Carcinoma via PI3K/AKT/m-TOR Signal Pathway. Onco Targets Ther (2020) 13:4049–61. doi: 10.2147/OTT.S251518
31. Li J, Xu X, Zhang D, Lv H, Lei X. LncRNA LHFPL3-AS1 Promotes Oral Squamous Cell Carcinoma Growth and Cisplatin Resistance Through Targeting miR-362-5p/CHSY1 Pathway. Onco Targets Ther (2021) 14:2293–300. doi: 10.2147/OTT.S298679
32. Wang X, Hao R, Wang F, Wang F. ZFAS1 Promotes Cisplatin Resistance via Suppressing miR-421 Expression in Oral Squamous Cell Carcinoma. Cancer Manag Res (2020) 12:7251–62. doi: 10.2147/CMAR.S248869
33. Wang J, Jia J, Zhou L. Long Non-Coding RNA CASC2 Enhances Cisplatin Sensitivity in Oral Squamous Cell Cancer Cells by the miR-31-5p/KANK1 Axis. Neoplasma (2020) 67(6):1279–92. doi: 10.4149/neo_2020_191029N1102
34. Wang F, Ji X, Wang J, Ma X, Yang Y, Zuo J, et al. LncRNA PVT1 Enhances Proliferation and Cisplatin Resistance via Regulating miR-194-5p/HIF1a Axis in Oral Squamous Cell Carcinoma. Onco Targets Ther (2020) 13:243–52. doi: 10.2147/OTT.S232405
35. Ma SQ, Wang YC, Li Y, Li XY, Yang J, Sheng YM. LncRNA XIST Promotes Proliferation and Cisplatin Resistance of Oral Squamous Cell Carcinoma by Downregulating miR-27b-3p. J Biol Regul Homeost Agents (2020) 34(6):1993–2001. doi: 10.23812/20-222-A
36. Chen S, Yang M, Wang C, Ouyang Y, Chen X, Bai J, et al. Forkhead Box D1 Promotes EMT and Chemoresistance by Upregulating lncRNA CYTOR in Oral Squamous Cell Carcinoma. Cancer Lett (2021) 503:43–53. doi: 10.1016/j.canlet.2020.11.046
37. Wang X, Li H, Shi J. LncRNA HOXA11-AS Promotes Proliferation and Cisplatin Resistance of Oral Squamous Cell Carcinoma by Suppression of miR-214-3p Expression. BioMed Res Int (2019) 2019:8645153. doi: 10.1155/2019/8645153
38. Wang X, Liu W, Wang P, Li S. RNA Interference of Long Noncoding RNA HOTAIR Suppresses Autophagy and Promotes Apoptosis and Sensitivity to Cisplatin in Oral Squamous Cell Carcinoma. J Oral Pathol Med (2018) 47(10):930–7. doi: 10.1111/jop.12769
39. Zhang D, Ding L, Li Y, Ren J, Shi G, Wang Y, et al. Midkine Derived From Cancer-Associated Fibroblasts Promotes Cisplatin-Resistance via Up-Regulation of the Expression of lncRNA ANRIL in Tumour Cells. Sci Rep (2017) 7(1):16231. doi: 10.1038/s41598-017-13431-y
40. Fang Z, Zhao J, Xie W, Sun Q, Wang H, Qiao B. LncRNA UCA1 Promotes Proliferation and Cisplatin Resistance of Oral Squamous Cell Carcinoma by Sunppressing miR-184 Expression. Cancer Med (2017) 6(12):2897–908. doi: 10.1002/cam4.1253
41. Chen W, Wang P, Lu Y, Jin T, Lei X, Liu M, et al. Decreased Expression of Mitochondrial miR-5787 Contributes to Chemoresistance by Reprogramming Glucose Metabolism and Inhibiting MT-CO3 Translation. Theranostics (2019) 9(20):5739–54. doi: 10.7150/thno.37556
42. Chen L, Zhu Q, Lu L, Liu Y. MiR-132 Inhibits Migration and Invasion and Increases Chemosensitivity of Cisplatin-Resistant Oral Squamous Cell Carcinoma Cells via Targeting TGF-β1. Bioengineered (2020) 11(1):91–102. doi: 10.1080/21655979.2019.1710925
43. Chen JH, Wu ATH, Bamodu OA, Yadav VK, Chao TY, Tzeng YM, et al. Ovatodiolide Suppresses Oral Cancer Malignancy by Down-Regulating Exosomal Mir-21/Stat3/β-Catenin Cargo and Preventing Oncogenic Transformation of Normal Gingival Fibroblasts. Cancers (2019) 12(1):56. doi: 10.3390/cancers12010056
44. Lu M, Wang C, Chen W, Mao C, Wang J. miR-654-5p Targets GRAP to Promote Proliferation, Metastasis, and Chemoresistance of Oral Squamous Cell Carcinoma Through Ras/MAPK Signaling. DNA Cell Biol (2018) 37(4):381–8. doi: 10.1089/dna.2017.4095
45. Lin SS, Peng CY, Liao YW, Chou MY, Hsieh PL, Yu CC. miR-1246 Targets CCNG2 to Enhance Cancer Stemness and Chemoresistance in Oral Carcinomas. Cancers (2018) 10(8):272. doi: 10.3390/cancers10080272
46. Lin XJ, He CL, Sun T, Duan XJ, Sun Y, Xiong SJ. hsa-miR-485-5p Reverses Epithelial to Mesenchymal Transition and Promotes Cisplatin-Induced Cell Death by Targeting PAK1 in Oral Tongue Squamous Cell Carcinoma. Int J Mol Med (2017) 40(1):83–9. doi: 10.3892/ijmm.2017.2992
47. Lin J, Lin Y, Fan L, Kuang W, Zheng L, Wu J, et al. miR-203 Inhibits Cell Proliferation and Promotes Cisplatin Induced Cell Death in Tongue Squamous Cancer. Biochem Biophys Res Commun (2016) 473(2):382–7. doi: 10.1016/j.bbrc.2016.02.105
48. Yeh L-Y, Yang C-C, Wu H-L, Kao S-Y, Liu C-J, Chen Y-F, et al. The miR-372-ZBTB7A Oncogenic Axis Suppresses TRAIL-R2 Associated Drug Sensitivity in Oral Carcinoma. Front Oncol (2020) 10. doi: 10.3389/fonc.2020.00047
49. Liu B, Cao G, Dong Z, Guo T. Effect of microRNA-27b on Cisplatin Chemotherapy Sensitivity of Oral Squamous Cell Carcinoma via FZD7 Signaling Pathway. Oncol Lett (2019) 18(1):667–73. doi: 10.3892/ol.2019.10347
50. Chen Y-F, Wei Y-Y, Yang C-C, Liu C-J, Yeh L-Y, Chou C-H, et al. miR-125b Suppresses Oral Oncogenicity by Targeting the Anti-Oxidative Gene PRXL2A. Redox Biol (2019) 22:101140. doi: 10.1016/j.redox.2019.101140
51. Jiang F, Zhao W, Zhou L, Liu Z, Li W, Yu D. MiR-222 Targeted PUMA to Improve Sensitization of UM1 Cells to Cisplatin. Int J Mol Sci (2014) 15(12):22128–41. doi: 10.3390/ijms151222128
52. Shriwas O, Priyadarshini M, Samal SK, Rath R, Panda S, Das Majumdar SK, et al. DDX3 Modulates Cisplatin Resistance in OSCC Through ALKBH5-Mediated M(6)A-Demethylation of FOXM1 and NANOG. Apoptosis (2020) 25(3-4):233–46. doi: 10.1007/s10495-020-01591-8
53. Alam M, Kashyap T, Mishra P, Panda AK, Nagini S, Mishra R. Role and Regulation of Proapoptotic Bax in Oral Squamous Cell Carcinoma and Drug Resistance. Head Neck (2019) 41(1):185–97. doi: 10.1002/hed.25471
54. Huang Z, Zhang Y, Li H, Zhou Y, Zhang Q, Chen R, et al. Vitamin D Promotes the Cisplatin Sensitivity of Oral Squamous Cell Carcinoma by Inhibiting LCN2-Modulated NF-κb Pathway Activation Through RPS3. Cell Death Dis (2019) 10(12):936. doi: 10.1038/s41419-019-2177-x
55. Yu ZW, Zhong LP, Ji T, Zhang P, Chen WT, Zhang CP. MicroRNAs Contribute to the Chemoresistance of Cisplatin in Tongue Squamous Cell Carcinoma Lines. Oral Oncol (2010) 46(4):317–22. doi: 10.1016/j.oraloncology.2010.02.002
56. Shen H, Laird PW. Interplay Between the Cancer Genome and Epigenome. Cell (2013) 153(1):38–55. doi: 10.1016/j.cell.2013.03.008
57. Wang W, Li X, Wang F, Sun XY. Effect of TET1 Regulating MGMT on Chemotherapy Resistance of Oral Squamous Cell Carcinoma Stem Cells. J Cell Biochem (2018) 119(1):723–35. doi: 10.1002/jcb.26236
58. Saha S, Panigrahi DP, Patil S, Bhutia SK. Autophagy in Health and Disease: A Comprehensive Review. Biomed Pharmacother (2018) 104:485–95. doi: 10.1016/j.biopha.2018.05.007
59. Levine B, Kroemer G. Biological Functions of Autophagy Genes: A Disease Perspective. Cell (2019) 176(1-2):11–42. doi: 10.1016/j.cell.2018.09.048
60. Amaravadi R, Kimmelman AC, White E. Recent Insights Into the Function of Autophagy in Cancer. Genes Dev (2016) 30(17):1913–30. doi: 10.1101/gad.287524.116
61. Li S, Wu Y, Ding Y, Yu M, Ai Z. CerS6 Regulates Cisplatin Resistance in Oral Squamous Cell Carcinoma by Altering Mitochondrial Fission and Autophagy. J Cell Physiol (2018) 233(12):9416–25. doi: 10.1002/jcp.26815
62. Naik PP, Mukhopadhyay S, Panda PK, Sinha N, Das CK, Mishra R, et al. Autophagy Regulates Cisplatin-Induced Stemness and Chemoresistance via the Upregulation of CD44, ABCB1 and ADAM17 in Oral Squamous Cell Carcinoma. Cell Prolif (2018) 51(1):e12411. doi: 10.1111/cpr.12411
63. Liao JK, Zhou B, Zhuang XM, Zhuang PL, Zhang DM, Chen WL. Cancer-Associated fibroblasts Confer Cisplatin Resistance of Tongue Cancer via Autophagy Activation. Biomed Pharmacother (2018) 97:1341–8. doi: 10.1016/j.biopha.2017.11.024
64. Xu Z, Huang CM, Shao Z, Zhao XP, Wang M, Yan TL, et al. Autophagy Induced by Areca Nut Extract Contributes to Decreasing Cisplatin Toxicity in Oral Squamous Cell Carcinoma Cells: Roles of Reactive Oxygen Species/AMPK Signaling. Int J Mol Sci (2017) 18(3):524. doi: 10.3390/ijms18030524
65. Shintani T, Klionsky DJ. Autophagy in Health and Disease: A Double-Edged Sword. Science (New York NY) (2004) 306(5698):990–5. doi: 10.1126/science.1099993
66. White E, DiPaola RS. The Double-Edged Sword of Autophagy Modulation in Cancer. Clin Cancer Res (2009) 15(17):5308–16. doi: 10.1158/1078-0432.CCR-07-5023
67. Gao W, Guo H, Niu M, Zheng X, Zhang Y, Xue X, et al. Circpard3 Drives Malignant Progression and Chemoresistance of Laryngeal Squamous Cell Carcinoma by Inhibiting Autophagy Through the PRKCI-Akt-mTOR Pathway. Mol Cancer (2020) 19(1):166. doi: 10.1186/s12943-020-01279-2
68. D'Arcy MS. Cell Death: A Review of the Major Forms of Apoptosis, Necrosis and Autophagy. Cell Biol Int (2019) 43(6):582–92. doi: 10.1002/cbin.11137
69. Kontos CK, Christodoulou MI, Scorilas A. Apoptosis-Related BCL2-Family Members: Key Players in Chemotherapy. Anticancer Agents Med Chem (2014) 14(3):353–74. doi: 10.2174/18715206113139990091
70. Alam M, Mishra R. Bcl-xL Expression and Regulation in the Progression, Recurrence, and Cisplatin Resistance of Oral Cancer. Life Sci (2021) 280:119705. doi: 10.1016/j.lfs.2021.119705
71. Placzek WJ, Wei J, Kitada S, Zhai D, Reed JC, Pellecchia M. A Survey of the Anti-Apoptotic Bcl-2 Subfamily Expression in Cancer Types Provides a Platform to Predict the Efficacy of Bcl-2 Antagonists in Cancer Therapy. Cell Death Dis (2010) 1(5):e40. doi: 10.1038/cddis.2010.18
72. Maji S, Shriwas O, Samal SK, Priyadarshini M, Rath R, Panda S, et al. STAT3- and GSK3β-Mediated Mcl-1 Regulation Modulates TPF Resistance in Oral Squamous Cell Carcinoma. Carcinogenesis (2019) 40(1):173–83. doi: 10.1093/carcin/bgy135
73. Jaiswal PK, Goel A, Mittal RD. Survivin: A Molecular Biomarker in Cancer. Indian J Med Res (2015) 141(4):389–97. doi: 10.4103/0971-5916.159250
74. Altieri DC. Survivin, Cancer Networks and Pathway-Directed Drug Discovery. Nat Rev Cancer (2008) 8(1):61–70. doi: 10.1038/nrc2293
75. Münscher A, Prochnow S, Gulati A, Sauter G, Lörincz B, Blessmann M, et al. Survivin Expression in Head and Neck Squamous Cell Carcinomas Is Frequent and Correlates With Clinical Parameters and Treatment Outcomes. Clin Oral Invest (2019) 23(1):361–7. doi: 10.1007/s00784-018-2444-8
76. Lima CF, Crastechini E, Dos Santos NC, Prado Ribeiro AC, Brandão TB, Castro FCF, et al. Smoking Cessation Leads to Changes in Survivin Expression in Oral Mucosa. J Oral Pathol Med (2018) 47(3):293–8. doi: 10.1111/jop.12664
77. Zhang L, Zhang W, Wang YF, Liu B, Zhang WF, Zhao YF, et al. Dual Induction of Apoptotic and Autophagic Cell Death by Targeting Survivin in Head Neck Squamous Cell Carcinoma. Cell Death Dis (2015) 6(5):e1771. doi: 10.1038/cddis.2015.139
78. Kumar B, Yadav A, Lang JC, Cipolla MJ, Schmitt AC, Arradaza N, et al. YM155 Reverses Cisplatin Resistance in Head and Neck Cancer by Decreasing Cytoplasmic Survivin Levels. Mol Cancer Ther (2012) 11(9):1988–98. doi: 10.1158/1535-7163.MCT-12-0167
79. Sun XS, Tao Y, Le Tourneau C, Pointreau Y, Sire C, Kaminsky MC, et al. Debio 1143 and High-Dose Cisplatin Chemoradiotherapy in High-Risk Locoregionally Advanced Squamous Cell Carcinoma of the Head and Neck: A Double-Blind, Multicentre, Randomised, Phase 2 Study. Lancet Oncol (2020) 21(9):1173–87. doi: 10.1016/S1470-2045(20)30327-2
80. Liotta LA, Kohn EC. The Microenvironment of the Tumour-Host Interface. Nature (2001) 411(6835):375–9. doi: 10.1038/35077241
81. Hinshaw DC, Shevde LA. The Tumor Microenvironment Innately Modulates Cancer Progression. Cancer Res (2019) 79(18):4557–66. doi: 10.1158/0008-5472.CAN-18-3962
82. Yang L, Lin PC. Mechanisms That Drive Inflammatory Tumor Microenvironment, Tumor Heterogeneity, and Metastatic Progression. Semin Cancer Biol (2017) 47:185–95. doi: 10.1016/j.semcancer.2017.08.001
83. Hernández-Camarero P, López-Ruiz E, Marchal JA, Perán M. Cancer: A Mirrored Room Between Tumor Bulk and Tumor Microenvironment. J Exp Clin Cancer Res (2021) 40(1):217. doi: 10.1186/s13046-021-02022-5
84. Rohwer N, Cramer T. Hypoxia-Mediated Drug Resistance: Novel Insights on the Functional Interaction of HIFs and Cell Death Pathways. Drug Resist Updat (2011) 14(3):191–201. doi: 10.1016/j.drup.2011.03.001
85. Zhong H, De Marzo AM, Laughner E, Lim M, Hilton DA, Zagzag D, et al. Overexpression of Hypoxia-Inducible Factor 1alpha in Common Human Cancers and Their Metastases. Cancer Res (1999) 59(22):5830–5.
86. Pérez-Sayáns M, Suárez-Peñaranda JM, Pilar GD, Barros-Angueira F, Gándara-Rey JM, García-García A. Hypoxia-Inducible Factors in OSCC. Cancer Lett (2011) 313(1):1–8. doi: 10.1016/j.canlet.2011.08.017
87. Forsythe JA, Jiang BH, Iyer NV, Agani F, Leung SW, Koos RD, et al. Activation of Vascular Endothelial Growth Factor Gene Transcription by Hypoxia-Inducible Factor 1. Mol Cell Biol (1996) 16(9):4604–13. doi: 10.1128/MCB.16.9.4604
88. Zimna A, Kurpisz M. Hypoxia-Inducible Factor-1 in Physiological and Pathophysiological Angiogenesis: Applications and Therapies. BioMed Res Int (2015) 2015:549412. doi: 10.1155/2015/549412
89. Sasabe E, Tatemoto Y, Li D, Yamamoto T, Osaki T. Mechanism of HIF-1alpha-Dependent Suppression of Hypoxia-Induced Apoptosis in Squamous Cell Carcinoma Cells. Cancer Sci (2005) 96(7):394–402. doi: 10.1111/j.1349-7006.2005.00065.x
90. Sasabe E, Zhou X, Li D, Oku N, Yamamoto T, Osaki T. The Involvement of Hypoxia-Inducible Factor-1alpha in the Susceptibility to Gamma-Rays and Chemotherapeutic Drugs of Oral Squamous Cell Carcinoma Cells. Int J Cancer (2007) 120(2):268–77. doi: 10.1002/ijc.22294
91. Qi X, Xu W, Xie J, Wang Y, Han S, Wei Z, et al. Metformin Sensitizes the Response of Oral Squamous Cell Carcinoma to Cisplatin Treatment Through Inhibition of NF-κb/HIF-1α Signal Axis. Sci Rep (2016) 6:35788. doi: 10.1038/srep35788
92. Mandal R, Şenbabaoğlu Y, Desrichard A, Havel JJ, Dalin MG, Riaz N, et al. The Head and Neck Cancer Immune Landscape and Its Immunotherapeutic Implications. JCI Insight (2016) 1(17):e89829. doi: 10.1172/jci.insight.89829
93. Shen B, Huang D, Ramsey AJ, Ig-Izevbekhai K, Zhang K, Lajud SA, et al. PD-L1 and MRN Synergy in Platinum-Based Chemoresistance of Head and Neck Squamous Cell Carcinoma. Br J Cancer (2020) 122(5):640–7. doi: 10.1038/s41416-019-0697-x
94. Mohapatra P, Shriwas O, Mohanty S, Ghosh A, Smita S, Kaushik SR, et al. CMTM6 Drives Cisplatin Resistance by Regulating Wnt Signaling Through the ENO-1/AKT/Gsk3β Axis. JCI Insight (2021) 6(4):e143643. doi: 10.1172/jci.insight.143643
95. Burr ML, Sparbier CE, Chan YC, Williamson JC, Woods K, Beavis PA, et al. CMTM6 Maintains the Expression of PD-L1 and Regulates Anti-Tumour Immunity. Nature (2017) 549(7670):101–5. doi: 10.1038/nature23643
96. Mezzadra R, Sun C, Jae LT, Gomez-Eerland R, de Vries E, Wu W, et al. Identification of CMTM6 and CMTM4 as PD-L1 Protein Regulators. Nature (2017) 549(7670):106–10. doi: 10.1038/nature23669
97. Sudo S, Kajiya H, Okano S, Sasaki M, Katsumata Y, Ohno J, et al. Cisplatin-Induced Programmed Cell Death Ligand-2 Expression Is Associated With Metastasis Ability in Oral Squamous Cell Carcinoma. Cancer Sci (2020) 111(4):1113–23. doi: 10.1111/cas.14336
98. Batlle E, Massagué J. Transforming Growth Factor-β Signaling in Immunity and Cancer. Immunity (2019) 50(4):924–40. doi: 10.1016/j.immuni.2019.03.024
99. Pradere JP, Dapito DH, Schwabe RF. The Yin and Yang of Toll-Like Receptors in Cancer. Oncogene (2014) 33(27):3485–95. doi: 10.1038/onc.2013.302
100. Keshavarz A, Pourbagheri-Sigaroodi A, Zafari P, Bagheri N, Ghaffari SH, Bashash D. Toll-Like Receptors (TLRs) in Cancer; With an Extensive Focus on TLR Agonists and Antagonists. IUBMB Life (2021) 73(1):10–25. doi: 10.1002/iub.2412
101. Kashani B, Zandi Z, Karimzadeh MR, Bashash D, Nasrollahzadeh A, Ghaffari SH. Blockade of TLR4 Using TAK-242 (Resatorvid) Enhances Anti-Cancer Effects of Chemotherapeutic Agents: A Novel Synergistic Approach for Breast and Ovarian Cancers. Immunol Res (2019) 67(6):505–16. doi: 10.1007/s12026-019-09113-8
102. Ding L, Ren J, Zhang D, Li Y, Huang X, Ji J, et al. The TLR3 Agonist Inhibit Drug Efflux and Sequentially Consolidates Low-Dose Cisplatin-Based Chemoimmunotherapy While Reducing Side Effects. Mol Cancer Ther (2017) 16(6):1068–79. doi: 10.1158/1535-7163.MCT-16-0454
103. Mostafazadeh M, Samadi N, Kahroba H, Baradaran B, Haiaty S, Nouri M. Potential Roles and Prognostic Significance of Exosomes in Cancer Drug Resistance. Cell Biosci (2021) 11(1):1. doi: 10.1186/s13578-020-00515-y
104. Fletcher JI, Williams RT, Henderson MJ, Norris MD, Haber M. ABC Transporters as Mediators of Drug Resistance and Contributors to Cancer Cell Biology. Drug Resist Updat (2016) 26:1–9. doi: 10.1016/j.drup.2016.03.001
105. Choi HS, Kim YK, Yun PY. Upregulation of MDR- and EMT-Related Molecules in Cisplatin-Resistant Human Oral Squamous Cell Carcinoma Cell Lines. Int J Mol Sci (2019) 20(12):3034. doi: 10.3390/ijms20123034
106. Lu X, Wang Z, Huang H, Wang H. Hedgehog Signaling Promotes Multidrug Resistance by Regulation of ABC Transporters in Oral Squamous Cell Carcinoma. J Oral Pathol Med (2020) 49(9):897–906. doi: 10.1111/jop.13050
107. Amiri-Kordestani L, Basseville A, Kurdziel K, Fojo AT, Bates SE. Targeting MDR in Breast and Lung Cancer: Discriminating Its Potential Importance From the Failure of Drug Resistance Reversal Studies. Drug Resist Updat (2012) 15(1-2):50–61. doi: 10.1016/j.drup.2012.02.002
108. Shaffer BC, Gillet JP, Patel C, Baer MR, Bates SE, Gottesman MM. Drug Resistance: Still a Daunting Challenge to the Successful Treatment of AML. Drug Resist Updat (2012) 15(1-2):62–9. doi: 10.1016/j.drup.2012.02.001
109. Wolf P. The Nature and Significance of Platelet Products in Human Plasma. Br J Haematol (1967) 13(3):269–88. doi: 10.1111/j.1365-2141.1967.tb08741.x
110. Li I, Nabet BY. Exosomes in the Tumor Microenvironment as Mediators of Cancer Therapy Resistance. Mol Cancer (2019) 18(1):32. doi: 10.1186/s12943-019-0975-5
111. Khoo XH, Paterson IC, Goh BH, Lee WL. Cisplatin-Resistance in Oral Squamous Cell Carcinoma: Regulation by Tumor Cell-Derived Extracellular Vesicles. Cancers (2019) 11(8):1166. doi: 10.3390/cancers11081166
112. Milman N, Ginini L, Gil Z. Exosomes and Their Role in Tumorigenesis and Anticancer Drug Resistance. Drug Resist Updat (2019) 45:1–12. doi: 10.1016/j.drup.2019.07.003
113. Wei X, Wang W, Wang L, Zhang Y, Zhang X, Chen M, et al. MicroRNA-21 Induces 5-Fluorouracil Resistance in Human Pancreatic Cancer Cells by Regulating PTEN and PDCD4. Cancer Med (2016) 5(4):693–702. doi: 10.1002/cam4.626
114. Ren W, Wang X, Gao L, Li S, Yan X, Zhang J, et al. MiR-21 Modulates Chemosensitivity of Tongue Squamous Cell Carcinoma Cells to Cisplatin by Targeting PDCD4. Mol Cell Biochem (2014) 390(1-2):253–62. doi: 10.1007/s11010-014-1976-8
115. Liu T, Chen G, Sun D, Lei M, Li Y, Zhou C, et al. Exosomes Containing miR-21 Transfer the Characteristic of Cisplatin Resistance by Targeting PTEN and PDCD4 in Oral Squamous Cell Carcinoma. Acta Biochim Biophys Sin (2017) 49(9):808–16. doi: 10.1093/abbs/gmx078
116. Su YK, Bamodu OA, Tzeng YM, Hsiao M, Yeh CT, Lin CM. Ovatodiolide Inhibits the Oncogenicity and Cancer Stem Cell-Like Phenotype of Glioblastoma Cells, as Well as Potentiate the Anticancer Effect of Temozolomide. Phytomedicine (2019) 61:152840. doi: 10.1016/j.phymed.2019.152840
117. Pérez-Herrero E, Fernández-Medarde A. Advanced Targeted Therapies in Cancer: Drug Nanocarriers, the Future of Chemotherapy. Eur J Pharm Biopharm (2015) 93:52–79. doi: 10.1016/j.ejpb.2015.03.018
118. Ashrafizadeh M, Zarrabi A, Hushmandi K, Kalantari M, Mohammadinejad R, Javaheri T, et al. Association of the Epithelial-Mesenchymal Transition (EMT) With Cisplatin Resistance. Int J Mol Sci (2020) 21(11):4002. doi: 10.3390/ijms21114002
119. Harada K, Ferdous T, Ueyama Y. Establishment of 5-Fluorouracil-Resistant Oral Squamous Cell Carcinoma Cell Lines With Epithelial to Mesenchymal Transition Changes. Int J Oncol (2014) 44(4):1302–8. doi: 10.3892/ijo.2014.2270
120. Ghosh RD, Ghuwalewala S, Das P, Mandloi S, Alam SK, Chakraborty J, et al. MicroRNA Profiling of Cisplatin-Resistant Oral Squamous Cell Carcinoma Cell Lines Enriched With Cancer-Stem-Cell-Like and Epithelial-Mesenchymal Transition-Type Features. Sci Rep (2016) 6:23932. doi: 10.1038/srep23932
121. Wang J, Wei Q, Wang X, Tang S, Liu H, Zhang F, et al. Transition to Resistance: An Unexpected Role of the EMT in Cancer Chemoresistance. Genes Dis (2016) 3(1):3–6. doi: 10.1016/j.gendis.2016.01.002
122. Erin N, Grahovac J, Brozovic A, Efferth T. Tumor Microenvironment and Epithelial Mesenchymal Transition as Targets to Overcome Tumor Multidrug Resistance. Drug Resist Updat (2020) 53:100715. doi: 10.1016/j.drup.2020.100715
123. Atashzar MR, Baharlou R, Karami J, Abdollahi H, Rezaei R, Pourramezan F, et al. Cancer Stem Cells: A Review From Origin to Therapeutic Implications. J Cell Physiol (2020) 235(2):790–803. doi: 10.1002/jcp.29044
124. Garcia-Mayea Y, Mir C, Masson F, Paciucci R, ME LL. Insights Into New Mechanisms and Models of Cancer Stem Cell Multidrug Resistance. Semin Cancer Biol (2020) 60:166–80. doi: 10.1016/j.semcancer.2019.07.022
125. Wang Y, Li Q, Xu L, Chen J, Pu Y, Wang L, et al. Cancer Stemness of CD10-Positive Cells Regulated by Hedgehog Pathway Promotes the Resistance to Cisplatin in Oral Squamous Cell Carcinoma. Oral Dis (2020) 27(6):1403–11. doi: 10.1111/odi.13673
126. Henriksson E, Baldetorp B, Borg A, Kjellen E, Akervall J, Wennerberg J, et al. P53 Mutation and Cyclin D1 Amplification Correlate With Cisplatin Sensitivity in Xenografted Human Squamous Cell Carcinomas From Head and Neck. Acta Oncol (Stockholm Sweden) (2006) 45(3):300–5. doi: 10.1080/02841860600547380
127. El-Deiry WS. The Role of P53 in Chemosensitivity and Radiosensitivity. Oncogene (2003) 22(47):7486–95. doi: 10.1038/sj.onc.1206949
128. Temam S, Flahault A, Périé S, Monceaux G, Coulet F, Callard P, et al. P53 Gene Status as a Predictor of Tumor Response to Induction Chemotherapy of Patients With Locoregionally Advanced Squamous Cell Carcinomas of the Head and Neck. J Clin Oncol (2000) 18(2):385–94. doi: 10.1200/JCO.2000.18.2.385
129. Tonigold M, Rossmann A, Meinold M, Bette M, Märken M, Henkenius K, et al. A Cisplatin-Resistant Head and Neck Cancer Cell Line With Cytoplasmic P53(Mut) Exhibits ATP-Binding Cassette Transporter Upregulation and High Glutathione Levels. J Cancer Res Clin Oncol (2014) 140(10):1689–704. doi: 10.1007/s00432-014-1727-y
130. Hung CC, Li FA, Liang SS, Wang LF, Lin IL, Chiu CC, et al. Direct Binding of Cisplatin to P22phox, an Endoplasmic Reticulum (ER) Membrane Protein, Contributes to Cisplatin Resistance in Oral Squamous Cell Carcinoma (OSCC) Cells. Molecules (Basel Switzerland) (2020) 25(17):3815. doi: 10.3390/molecules25173815
131. Suenaga N, Kuramitsu M, Komure K, Kanemaru A, Takano K, Ozeki K, et al. Loss of Tumor Suppressor CYLD Expression Triggers Cisplatin Resistance in Oral Squamous Cell Carcinoma. Int J Mol Sci (2019) 20(20):5194. doi: 10.3390/ijms20205194
132. Shriwas O, Arya R, Mohanty S, Mohapatra P, Kumar S, Rath R, et al. RRBP1 Rewires Cisplatin Resistance in Oral Squamous Cell Carcinoma by Regulating Hippo Pathway. Br J Cancer (2021) 124(12):2004–16. doi: 10.1038/s41416-021-01336-7
133. Wang LM, Gan YH. Cancer-Derived IgG Involved in Cisplatin Resistance Through PTP-BAS/Src/PDK1/AKT Signaling Pathway. Oral Dis (2021) 27(3):464–74. doi: 10.1111/odi.13583
134. Sun L, Wang K, Peng L, Zhang J, Yang J, Zhao J, et al. Naa10p Enhances Chemosensitivity to Cisplatin in Oral Squamous Cell Carcinoma Cells. Cancer Manag Res (2021) 13:1843–51. doi: 10.2147/CMAR.S296783
135. Peng B, Gu Y, Xiong Y, Zheng G, He Z. Microarray-Assisted Pathway Analysis Identifies MT1X & NFκB as Mediators of TCRP1-Associated Resistance to Cisplatin in Oral Squamous Cell Carcinoma. PloS One (2012) 7(12):e51413. doi: 10.1371/journal.pone.0051413
136. Gu Y, Fan S, Xiong Y, Peng B, Zheng G, Yu Y, et al. Cloning and Functional Characterization of TCRP1, a Novel Gene Mediating Resistance to Cisplatin in an Oral Squamous Cell Carcinoma Cell Line. FEBS Lett (2011) 585(6):881–7. doi: 10.1016/j.febslet.2010.12.045
137. Peng B, Yi S, Gu Y, Zheng G, He Z. Purification and Biochemical Characterization of a Novel Protein-Tongue Cancer Chemotherapy Resistance-Associated Protein1 (TCRP1). Protein Expression Purif (2012) 82(2):360–7. doi: 10.1016/j.pep.2012.02.002
138. Hsu T-N, Huang C-M, Huang C-S, Huang M-S, Yeh C-T, Chao T-Y, et al. Targeting FAT1 Inhibits Carcinogenesis, Induces Oxidative Stress and Enhances Cisplatin Sensitivity Through Deregulation of LRP5/WNT2/GSS Signaling Axis in Oral Squamous Cell Carcinoma. Cancers (2019) 11(12):1883. doi: 10.3390/cancers11121883
139. Li L, Liu HC, Wang C, Liu X, Hu FC, Xie N, et al. Overexpression of β-Catenin Induces Cisplatin Resistance in Oral Squamous Cell Carcinoma. BioMed Res Int (2016) 2016:5378567. doi: 10.1155/2016/5378567
140. Li R, Li W, He F, Zhang M, Luo H, Tang H. Systematic Screening Identifies a TEAD4-S100A13 Axis Modulating Cisplatin Sensitivity of Oral Squamous Cell Carcinoma Cells. J Oral Pathol Med (2021) p. 1–9. doi: 10.1111/jop.13224
141. Kashyap T, Nath N, Mishra P, Jha A, Nagini S, Mishra R. Pluripotency Transcription Factor Nanog and Its Association With Overall Oral Squamous Cell Carcinoma Progression, Cisplatin-Resistance, Invasion and Stemness Acquisition. Head Neck (2020) 42(11):3282–94. doi: 10.1002/hed.26373
142. Hung CC, Chien CY, Chiang WF, Lin CS, Hour TC, Chen HR, et al. P22phox Confers Resistance to Cisplatin, by Blocking Its Entry Into the Nucleus. Oncotarget (2015) 6(6):4110–25. doi: 10.18632/oncotarget.2893
143. Hung CC, Chien CY, Chu PY, Wu YJ, Lin CS, Huang CJ, et al. Differential Resistance to Platinum-Based Drugs and 5-Fluorouracil in P22phox-Overexpressing Oral Squamous Cell Carcinoma: Implications of Alternative Treatment Strategies. Head Neck (2017) 39(8):1621–30. doi: 10.1002/hed.24803
144. Kashyap D, Tuli HS, Yerer MB, Sharma A, Sak K, Srivastava S, et al. Natural Product-Based Nanoformulations for Cancer Therapy: Opportunities and Challenges. Semin Cancer Biol (2021) 69:5–23. doi: 10.1016/j.semcancer.2019.08.014
145. Zhu Y, Wen LM, Li R, Dong W, Jia SY, Qi MC. Recent Advances of Nano-Drug Delivery System in Oral Squamous Cell Carcinoma Treatment. Eur Rev Med Pharmacol Sci (2019) 23(21):9445–53. doi: 10.26355/eurrev_201911_19438
146. Pornpitchanarong C, Rojanarata T, Opanasopit P, Ngawhirunpat T, Patrojanasophon P. Synthesis of Novel N-Vinylpyrrolidone/Acrylic Acid Nanoparticles as Drug Delivery Carriers of Cisplatin to Cancer Cells. Colloids Surf B Biointerfaces (2020) 185:110566. doi: 10.1016/j.colsurfb.2019.110566
147. Wang ZQ, Liu K, Huo ZJ, Li XC, Wang M, Liu P, et al. A Cell-Targeted Chemotherapeutic Nanomedicine Strategy for Oral Squamous Cell Carcinoma Therapy. J Nanobiotechnology (2015) 13:63. doi: 10.1186/s12951-015-0116-2
148. Hoonjan M, Jadhav V, Bhatt P. Arsenic Trioxide: Insights Into Its Evolution to an Anticancer Agent. J Biol Inorg Chem (2018) 23(3):313–29. doi: 10.1007/s00775-018-1537-9
149. Jing Y, Dai J, Chalmers-Redman RM, Tatton WG, Waxman S. Arsenic Trioxide Selectively Induces Acute Promyelocytic Leukemia Cell Apoptosis via a Hydrogen Peroxide-Dependent Pathway. Blood (1999) 94(6):2102–11. doi: 10.1182/blood.V94.6.2102
150. Chang KJ, Yang MH, Zheng JC, Li B, Nie W. Arsenic Trioxide Inhibits Cancer Stem-Like Cells via Down-Regulation of Gli1 in Lung Cancer. Am J Trans Res (2016) 8(2):1133–43.
151. Ji H, Li Y, Jiang F, Wang X, Zhang J, Shen J, et al. Inhibition of Transforming Growth Factor Beta/SMAD Signal by MiR-155 Is Involved in Arsenic Trioxide-Induced Anti-Angiogenesis in Prostate Cancer. Cancer Sci (2014) 105(12):1541–9. doi: 10.1111/cas.12548
152. Miodragović Ð, Swindell EP, Waxali ZS, Bogachkov A, O'Halloran TV. Beyond Cisplatin: Combination Therapy With Arsenic Trioxide. Inorganica Chim Acta (2019) 496:119030. doi: 10.1016/j.ica.2019.119030
153. Muenyi CS, Pinhas AR, Fan TW, Brock GN, Helm CW, States JC. Sodium Arsenite ± Hyperthermia Sensitizes P53-Expressing Human Ovarian Cancer Cells to Cisplatin by Modulating Platinum-DNA Damage Responses. Toxicol Sci (2012) 127(1):139–49. doi: 10.1093/toxsci/kfs085
154. Nasrollahzadeh A, Bashash D, Kabuli M, Zandi Z, Kashani B, Zaghal A, et al. Arsenic Trioxide and BIBR1532 Synergistically Inhibit Breast Cancer Cell Proliferation Through Attenuation of NF-κb Signaling Pathway. Life Sci (2020) 257:118060. doi: 10.1016/j.lfs.2020.118060
155. Kotowski U, Heiduschka G, Brunner M, Erovic BM, Martinek H, Thurnher D. Arsenic Trioxide Enhances the Cytotoxic Effect of Cisplatin in Head and Neck Squamous Cell Carcinoma Cell Lines. Oncol Lett (2012) 3(6):1326–30. doi: 10.3892/ol.2012.643
156. Nakaoka T, Ota A, Ono T, Karnan S, Konishi H, Furuhashi A, et al. Combined Arsenic Trioxide-Cisplatin Treatment Enhances Apoptosis in Oral Squamous Cell Carcinoma Cells. Cell Oncol (Dordrecht) (2014) 37(2):119–29. doi: 10.1007/s13402-014-0167-7
157. Gibson MK, Li Y, Murphy B, Hussain MH, DeConti RC, Ensley J, et al. Randomized Phase III Evaluation of Cisplatin Plus Fluorouracil Versus Cisplatin Plus Paclitaxel in Advanced Head and Neck Cancer (E1395): An Intergroup Trial of the Eastern Cooperative Oncology Group. J Clin Oncol (2005) 23(15):3562–7. doi: 10.1200/JCO.2005.01.057
158. Vermorken JB, Remenar E, van Herpen C, Gorlia T, Mesia R, Degardin M, et al. Cisplatin, Fluorouracil, and Docetaxel in Unresectable Head and Neck Cancer. N Engl J Med (2007) 357(17):1695–704. doi: 10.1056/NEJMoa071028
159. Blanchard P, Bourhis J, Lacas B, Posner MR, Vermorken JB, Cruz Hernandez JJ, et al. Taxane-Cisplatin-Fluorouracil as Induction Chemotherapy in Locally Advanced Head and Neck Cancers: An Individual Patient Data Meta-Analysis of the Meta-Analysis of Chemotherapy in Head and Neck Cancer Group. J Clin Oncol (2013) 31(23):2854–60. doi: 10.1200/JCO.2012.47.7802
160. Zhong LP, Zhang CP, Ren GX, Guo W, William WN Jr., Sun J, et al. Randomized Phase III Trial of Induction Chemotherapy With Docetaxel, Cisplatin, and Fluorouracil Followed by Surgery Versus Up-Front Surgery in Locally Advanced Resectable Oral Squamous Cell Carcinoma. J Clin Oncol (2013) 31(6):744–51. doi: 10.1200/JCO.2012.43.8820
161. Hitt R, Grau JJ, López-Pousa A, Berrocal A, García-Girón C, Irigoyen A, et al. A Randomized Phase III Trial Comparing Induction Chemotherapy Followed by Chemoradiotherapy Versus Chemoradiotherapy Alone as Treatment of Unresectable Head and Neck Cancer. Ann Oncol (2014) 25(1):216–25. doi: 10.1093/annonc/mdt461
162. Takácsi-Nagy Z, Hitre E, Remenár É, Oberna F, Polgár C, Major T, et al. Docetaxel, Cisplatin and 5-Fluorouracil Induction Chemotherapy Followed by Chemoradiotherapy or Chemoradiotherapy Alone in Stage III-IV Unresectable Head and Neck Cancer: Results of a Randomized Phase II Study. Strahlenther Onkol (2015) 191(8):635–41. doi: 10.1007/s00066-015-0829-z
163. Bernadach M, Lapeyre M, Dillies AF, Miroir J, Casile M, Moreau J, et al. Predictive Factors of Toxicity of TPF Induction Chemotherapy for Locally Advanced Head and Neck Cancers. BMC Cancer (2021) 21(1):360. doi: 10.1186/s12885-021-08128-5
164. Liu L, Chen J, Cai X, Yao Z, Huang J. Progress in Targeted Therapeutic Drugs for Oral Squamous Cell Carcinoma. Surg Oncol (2019) 31:90–7. doi: 10.1016/j.suronc.2019.09.001
165. Vermorken JB, Mesia R, Rivera F, Remenar E, Kawecki A, Rottey S, et al. Platinum-Based Chemotherapy Plus Cetuximab in Head and Neck Cancer. N Engl J Med (2008) 359(11):1116–27. doi: 10.1056/NEJMoa0802656
166. Ang KK, Zhang Q, Rosenthal DI, Nguyen-Tan PF, Sherman EJ, Weber RS, et al. Randomized Phase III Trial of Concurrent Accelerated Radiation Plus Cisplatin With or Without Cetuximab for Stage III to IV Head and Neck Carcinoma: RTOG 0522. J Clin Oncol (2014) 32(27):2940–50. doi: 10.1200/JCO.2013.53.5633
167. Chang JW, Kang SU, Shin YS, Seo SJ, Kim YS, Yang SS, et al. Combination of NTP With Cetuximab Inhibited Invasion/Migration of Cetuximab-Resistant OSCC Cells: Involvement of NF-κb Signaling. Sci Rep (2015) 5:18208. doi: 10.1038/srep18208
168. Chan KK, Glenny AM, Weldon JC, Furness S, Worthington HV, Wakeford H. Interventions for the Treatment of Oral and Oropharyngeal Cancers: Targeted Therapy and Immunotherapy. Cochrane Database Syst Rev (2015) 12:Cd010341. doi: 10.1002/14651858.CD010341.pub2
169. Ju WT, Ma HL, Zhao TC, Liang SY, Zhu DW, Wang LZ, et al. Stathmin Guides Personalized Therapy in Oral Squamous Cell Carcinoma. Cancer Sci (2020) 111(4):1303–13. doi: 10.1111/cas.14323
170. Liu SC, Wu YC, Huang CM, Hsieh MS, Huang TY, Huang CS, et al. Inhibition of Bruton's Tyrosine Kinase as a Therapeutic Strategy for Chemoresistant Oral Squamous Cell Carcinoma and Potential Suppression of Cancer Stemness. Oncogenesis (2021) 10(2):20. doi: 10.1038/s41389-021-00308-z
171. Perri F, Ionna F, Longo F, Della Vittoria Scarpati G, De Angelis C, Ottaiano A, et al. Immune Response Against Head and Neck Cancer: Biological Mechanisms and Implication on Therapy. Transl Oncol (2020) 13(2):262–74. doi: 10.1016/j.tranon.2019.11.008
172. Larkins E, Blumenthal GM, Yuan W, He K, Sridhara R, Subramaniam S, et al. FDA Approval Summary: Pembrolizumab for the Treatment of Recurrent or Metastatic Head and Neck Squamous Cell Carcinoma With Disease Progression on or After Platinum-Containing Chemotherapy. Oncologist (2017) 22(7):873–8. doi: 10.1634/theoncologist.2016-0496
173. Ferris RL, Blumenschein G Jr., Fayette J, Guigay J, Colevas AD, Licitra L, et al. Nivolumab for Recurrent Squamous-Cell Carcinoma of the Head and Neck. N Engl J Med (2016) 375(19):1856–67. doi: 10.1056/NEJMoa1602252
174. Cohen EEW, Bell RB, Bifulco CB, Burtness B, Gillison ML, Harrington KJ, et al. The Society for Immunotherapy of Cancer Consensus Statement on Immunotherapy for the Treatment of Squamous Cell Carcinoma of the Head and Neck (HNSCC). J Immunother Cancer (2019) 7(1):184. doi: 10.1186/s40425-019-0662-5
175. Ghanizada M, Jakobsen KK, Grønhøj C, von Buchwald C. The Effects of Checkpoint Inhibition on Head and Neck Squamous Cell Carcinoma: A Systematic Review. Oral Oncol (2019) 90:67–73. doi: 10.1016/j.oraloncology.2019.01.018
176. Tringale KR, Carroll KT, Zakeri K, Sacco AG, Barnachea L, Murphy JD. Cost-Effectiveness Analysis of Nivolumab for Treatment of Platinum-Resistant Recurrent or Metastatic Squamous Cell Carcinoma of the Head and Neck. J Natl Cancer Inst (2018) 110(5):479–85. doi: 10.1093/jnci/djx226
177. Salehi B, Martorell M, Arbiser JL, Sureda A, Martins N, Maurya PK, et al. Antioxidants: Positive or Negative Actors? Biomolecules (2018) 8(4):11. doi: 10.3390/biom8040124
178. Elkashty OA, Tran SD. Broccoli Extract Increases Drug-Mediated Cytotoxicity Towards Cancer Stem Cells of Head and Neck Squamous Cell Carcinoma. Br J Cancer (2020) 123(9):1395–403. doi: 10.1038/s41416-020-1025-1
179. Duarte VM, Han E, Veena MS, Salvado A, Suh JD, Liang LJ, et al. Curcumin Enhances the Effect of Cisplatin in Suppression of Head and Neck Squamous Cell Carcinoma via Inhibition of Ikkβ Protein of the NFκB Pathway. Mol Cancer Ther (2010) 9(10):2665–75. doi: 10.1158/1535-7163.MCT-10-0064
180. Chen CF, Yang JS, Chen WK, Lu CC, Chiang JH, Chiu HY, et al. Ursolic Acid Elicits Intrinsic Apoptotic Machinery by Downregulating the Phosphorylation of AKT/BAD Signaling in Human Cisplatin−Resistant Oral Cancer CAR Cells. Oncol Rep (2018) 40(3):1752–60. doi: 10.3892/or.2018.6530
181. Xue D, Pan ST, Zhou X, Ye F, Zhou Q, Shi F, et al. Plumbagin Enhances the Anticancer Efficacy of Cisplatin by Increasing Intracellular ROS in Human Tongue Squamous Cell Carcinoma. Oxid Med Cell Longev (2020) 2020:5649174. doi: 10.1155/2020/5649174
182. Yuan CH, Horng CT, Lee CF, Chiang NN, Tsai FJ, Lu CC, et al. Epigallocatechin Gallate Sensitizes Cisplatin-Resistant Oral Cancer CAR Cell Apoptosis and Autophagy Through Stimulating AKT/STAT3 Pathway and Suppressing Multidrug Resistance 1 Signaling. Environ Toxicol (2017) 32(3):845–55. doi: 10.1002/tox.22284
Keywords: oral squamous cell carcinoma, cisplatin, chemoresistance, molecular mechanisms, cancer therapy
Citation: Cheng Y, Li S, Gao L, Zhi K and Ren W (2021) The Molecular Basis and Therapeutic Aspects of Cisplatin Resistance in Oral Squamous Cell Carcinoma. Front. Oncol. 11:761379. doi: 10.3389/fonc.2021.761379
Received: 19 August 2021; Accepted: 04 October 2021;
Published: 22 October 2021.
Edited by:
Nerina Denaro, Azienda Sanitaria Ospedaliera S.Croce e Carle Cuneo, ItalyReviewed by:
Maria Cossu Rocca, European Institute of Oncology (IEO), ItalyGaurisankar Sa, Bose Institute, India
Copyright © 2021 Cheng, Li, Gao, Zhi and Ren. This is an open-access article distributed under the terms of the Creative Commons Attribution License (CC BY). The use, distribution or reproduction in other forums is permitted, provided the original author(s) and the copyright owner(s) are credited and that the original publication in this journal is cited, in accordance with accepted academic practice. No use, distribution or reproduction is permitted which does not comply with these terms.
*Correspondence: Wenhao Ren, herohao@163.com; Keqian Zhi, zhikeqian@sina.com
†These authors have contributed equally to this work and share first authorship