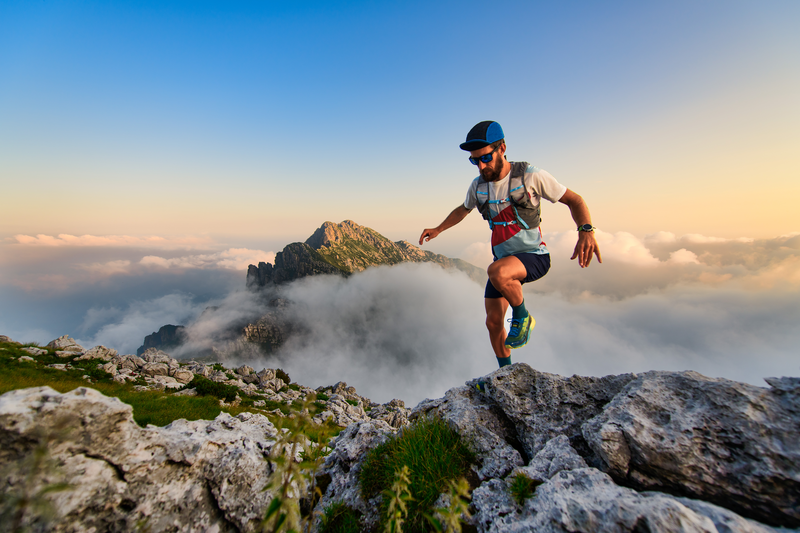
95% of researchers rate our articles as excellent or good
Learn more about the work of our research integrity team to safeguard the quality of each article we publish.
Find out more
SYSTEMATIC REVIEW article
Front. Oncol. , 06 December 2021
Sec. Radiation Oncology
Volume 11 - 2021 | https://doi.org/10.3389/fonc.2021.745262
Aim: Pelvic radiation therapy (RT) can impact the gut microbiome in patients with cancer and result in gastrointestinal (GI) toxicities. The purpose of this systematic review was to describe the effects of RT on the gut microbiome and the associations between the gut microbiome and GI toxicities in patients treated with pelvic RT.
Methods: PubMed, Embase, and Web of Science databases were searched from their earliest records to August 2020. The articles screening process adhered to the Preferred Reporting Items for Systematic Reviews and Meta-Analyses guidelines. The Mixed Method Assessment Tool was used to assess the methodological quality for each included study. All study findings were synthesized and presented in narrative format. Thirteen studies were included. The gut microbiome of fecal samples was analyzed using 16S rRNA sequencing approaches.
Results: There were disparities in alpha and beta diversities that existed across the studies. Divergent results were found among various phyla, including Firmicutes, Bacteroidetes, Proteobacteria, Actinobacteria, Cyanobacteria, Fusobacteria, and Deinococcus-Thermus. Moreover, alteration in the gut microbiome diversity and abundance related to cancer treatment was associated with pelvic toxicities, specifically diarrhea. Following treatment, increases in the abundance of Bacteroides was associated with diarrhea and radiation enteritis.
Conclusions: Pelvic RT can disrupt the diversity and abundance of commensal gut microorganisms. A dysbiotic gut microbiome showed a promising association with radiation enteritis through alterations of the intestinal barrier function, innate immunity, and intestinal repair mechanisms; however, confounders, such as diet, were not thoroughly addressed.
Cancer is a major public health problem across the world, and it is the second leading cause of death in the United States (1, 2). In 2020, a total of 1,806,590 new cancer cases and 606,520 cancer deaths were estimated to occur in the United States (2). Pelvic cancers, such as gynecological cancer, prostate cancer, and colorectal cancer, are among the most frequently diagnosed cancers (1, 2). Pelvic radiation therapy (RT) is a core modality primarily used to treat pelvic cancers (3, 4), with the purpose of reducing tumor size to allow surgical resection of tumors or killing tumor cells that cannot be removed via surgeries (5). With the advancement of cancer treatment modalities, including pelvic RT, the cancer mortality rate has continuously decreased, with an estimated 16.9 million cancer survivors currently alive in the United States (2, 6, 7). However, the wide use of RT has resulted in a high incidence of radiation‐induced symptoms and toxicities (8, 9). Specifically, 90% of cancer patients with pelvic RT develop a permanent change in their bowel habits (4, 10), and they may develop pelvic radiation toxicities such as radiation enteritis, radiation proctitis, and radiation cystitis post treatment (4, 11). As these patients with pelvic cancers live longer, an increasing number of them suffer for decades with RT-related gastrointestinal (GI) symptoms and toxicities, all of which can significantly decrease the patients’ quality of life (QOL).
Current knowledge has delineated the microbiome-host interactions as an integrative point in the pathogenesis of GI symptoms and toxicities among cancer patients receiving RT (12, 13). The human microbiotas and their genomes in the GI tract are collectively called the gut microbiome (14), which varies among different hosts and across different parts of the GI tract within a single host (15, 16). The gut microbiome is a dynamic ecosystem and is highly susceptible to a variety of environmental factors (e.g., diet and physical activity) and host-driven factors (e.g., cancer diagnosis and tolerance of RT) (17, 18). The pathogenesis of the gut microbiome for cancer treatment-related symptoms and GI toxicities may be related to the following pathways: inflammatory cytokines, intestinal permeability, bacteria translocation; changes in the epithelial surface microbiota pattern, intestinal protection from noxious stimuli, epithelial repair mechanisms; and the release of immune cells and molecules (19). Thus, pelvic RT can lead to a dysbiotic gut microbiome, potentially increasing intestinal permeability, thereby causing GI symptoms and toxicities.
Recently, research has started to investigate the interaction of the gut microbiome with pelvic RT and its associations with RT-related GI toxicities. Both animal models and clinical studies have corroborated decreases in diversity and disturbance of the gut microbial communities across cancer treatments (12, 13). Based on male C57/Bl6 mice, Johnson et al. found that radiation led to significant decreases in anaerobic Enterobacteriaceae and Lactobacillus genera (20); Kim and colleagues found a significant increase in Alistipes and a decrease in Mucispirillum genera when characterizing the mouse gut microbiome during radiation (21). Among human cancer populations, gynecological cancer patients with pelvic RT showed a higher abundance of Actinobacteria but a lower abundance of Fusobacteria compared to healthy individuals (22). Patients with pelvic tumors who experienced acute post-RT diarrhea showed a decrease in microbial diversity compared to both healthy volunteers and patients who received pelvic RT but without diarrhea; patients with diarrhea exhibited an increased abundance of Actinobacteria and Bacilli but a decreased abundance of Clostridia compared to patients without diarrhea (23). All these studies seem to indicate that patients receiving RT exhibit marked changes in their gut microbiome, and these changes may be associated with GI symptoms and toxicities, such as diarrhea and radiation enteritis.
Although evidence has shown the potential role of the gut microbiome in GI symptoms and toxicities during cancer treatments, current literature has primarily focused on the impact of chemotherapy and immunotherapy on the gut microbiome (12, 13). Some animal studies have proposed the potential role of the gut microbiome as a protection against RT toxicities, suggesting a higher abundance of Lachnospiraceae and Enterococcaceae associated with less RT side effects in mice models (24). Although some evidence has shown the potential associations of the gut microbiome with symptoms and GI toxicities in cancer patients, findings of studies are inconsistent, and the role of pelvic RT on the gut microbiome and resulted symptoms remain obscure (12, 13).
Radiation enteritis and symptoms experienced by cancer patients treated with RT is a global challenge. Understanding the impact of pelvic RT on the gut microbiome and its associations with symptoms and GI toxicities may provide a precise target to relieve symptoms and decrease patients’ suffering. The current literature reviews primarily focused on preclinical and clinical studies to elucidate the underlying mechanisms of gut microbiome dysbiosis in relation to RT (8, 25). Also, to date, no systematic review was conducted with a robust methodology to evaluate the quality of the included studies using a valid tool (25). Thus, a systematic review was needed to synthesize current research regarding the impact of pelvic RT on the gut microbiome and its relationship with symptoms and GI toxicities in adult cancer patients with pelvic cancers.
A systematic review was conducted to examine: 1) the influence of pelvic RT on the gut microbiome in adult patients with pelvic cancers receiving RT; and 2) the associations between the gut microbiome and clinician-reported and patient-reported GI toxicities and symptoms among adult patients treated with pelvic RT.
With the guidance of the Preferred Reporting Items for Systematic Reviews and Meta-Analyses (PRISMA) framework (26), a systematic review was conducted by searching three databases: PubMed, Embase, and Web of Science. Key terms used to search these databases included GI microbiome, gut microbiome, gut microflora, intestinal bacterial, microbiota composition, radiation, radiation injury, radiation toxicities, radiotherapy, pelvic radiotherapy, pelvic irradiation, and brachytherapy. All three databases were searched from their earliest records to August 2020. Detailed information for the searching process is displayed in supplementary Table S1. The literature search process was overseen by a health science librarian from Emory University in Atlanta, Georgia, United States.
All the articles identified from the database searches were initially screened via title and abstract by one author (CR) and subsequently confirmed by another author (ZB) from this study. Then, all full texts for the remaining articles were retrieved and independently reviewed by two reviewers (CR and ZB) to determine study eligibility. Ambiguous studies were discussed and reviewed by the independent reviewers (CR and ZB) together with a third author (JB). Eligibility criteria for articles to be included were as follows: 1) studied the gut microbiome with next generation sequencing (e.g., 16S rRNA gene amplicon) in patients receiving pelvic RT; 2) focused on pelvic cancer populations; and 3) published in English. Articles were excluded from this study if they: 1) studied an animal model or other non-human models; 2) did not focus on pelvic cancer; 3) did not study the gut microbiome or gut microbiota with next generation sequencing approaches; or 4) were supplementary information, patents, abstracts without available full texts, or unpublished reports. When multiple studies with similar results were found to be authored by the same research team, only the most recent publication was included in this review. A secondary search of reference lists was conducted to decrease the likelihood of omitting eligible articles from this review.
A standard data extraction form was developed by our team to extract data from eligible studies. Information that was extracted from each study included: 1) characteristics of the studies according to the Johns Hopkins evidence level and quality guide (27) (e.g., authors, year of publication, origin of the study, research purpose, study design, and sample size); 2) participant information (e.g., demographics, clinical information, inclusion and exclusion criteria); 3) variables, measures, and data collection strategies; 4) data analysis; and 5) research findings and related interpretations. All study data were extracted by two authors (CR and ZB), and one author (JB) confirmed the accuracy of data extraction.
The Mixed Methods Assessment Tool (MMAT) is a scoring system designed to evaluate complex systematic literature reviews, including qualitative studies, quantitative studies (e.g., randomized controlled trials [RCTs], quasi-experimental studies, and descriptive studies), and mixed methods studies (28). Studies eligible for this review represent many types of research, so the MMAT was appropriate for quality assessment. The MMAT was updated in 2018, and the updated version of this tool was utilized for this review. This tool includes two screening criteria (applicable to all types of studies) and five domain criteria (specific to each study design), resulting in seven criteria for each study. All these questions have three response options (i.e., “yes”, “no”, or “can’t tell”) (28). An overall quality score ranges between 20% (one criterion met) and 100% (all criteria met), which is calculated using the number of domain criteria met divided by five. This tool has moderate to high reliability and has been widely used for the critical evaluation of more than 50 systematic reviews (29). The quality assessment of the included studies attempted to provide a comprehensive overview of their value and to address any potential weaknesses.
Descriptive statistics were used to describe all the eligible studies. All study findings were synthesized and presented in tables and figures using a narrative format.
We initially identified 3,402 reports for possible inclusion in this systematic review by searching three databases: PubMed (n = 92), Web of Science (n = 1905), and Embase (n = 1405). Eleven additional records were identified via the reference articles of related review papers. After reviewing the articles’ titles and abstracts, duplicate articles and other irrelevant reports (n = 3358) were removed, resulting in 55 reports requiring full text review. Among these 55 full texts, we excluded studies of animal models (n = 3), reports not including the gut microbiome (n = 10) or RT (n = 1), conference abstracts or poster presentations (n = 13), full articles in a language other than English (n = 10), and articles in which the study population did not include adults (n = 2), leading to 16 studies left for study quality review. Three articles were further excluded due to outdated methods in the gut microbiome sequencing. Thus, a total of 13 studies were included in this systematic review (Figure 1).
Figure 1 PRISMA flow diagram of the studies of the gut microbiome and RT-related gastrointestinal toxicities.
Among these 13 included studies, 11 of them were quantitative descriptive studies, one was a quantitative non-randomized study, and one was a RCT. The sample sizes of the included studies varied from 5 to 134 participants. These studies were conducted between 2008 and 2020, primarily in the United States (n = 4), China (n = 3), South Korea (n = 2), Malaysia (n = 1), Finland (n = 1), France (n=1) and the United Kingdom (n = 1). All the included studies collected stool samples. In addition, two of the studies also collected blood samples, and one study collected gut biopsies. The gut microbiome data were sequenced using 16S rRNA targeting various gene regions. Radiation-induced GI clinician reported toxicities or patient reported symptoms were reported by seven studies, with most studies primarily focusing on diarrhea. Table 1 describes characteristics and sampling of these included studies.
Based on the MMAT quality scoring system, most of the included studies had an overall quality score of 80% or above: 80% (n = 6) and 100% (n = 5). Two studies had a quality score of 60% due to the small sample size and potential risk of inappropriate measures. Of the studies with an overall quality score of 80%, the criterion that was not met was primarily associated with the sampling strategy relevant to address the research question. Based on this assessment, none of the eligible studies were excluded from this review. Supplementary Tables S2, S3 display the quality scores of these quantitative non-randomized studies (n = 12) and RCTs (n = 1), respectively.
A total of 11 studies were reviewed to show the impact of pelvic RT on changes in gut microbiome diversity (alpha diversity and/or beta diversity) and taxa abundance of the gut microbiome (Table 2). These studies focused on a variety of pelvic cancers, including gynecological cancers only (n = 3), colorectal cancers only (n = 4), prostate cancer only (n = 1), and mixed pelvic cancers, such as gynecological, colon, and prostate cancers (n = 3). Most of the included studies reported the gut microbiome composition pre- to post-RT or chemoradiation therapy (CRT) (30–32, 34, 36, 37, 40); two studies evaluated the gut microbiome changes pre-RT, during RT, and post-RT (22, 33); two studies assessed the gut microbiome pre-RT and during RT (38, 39); and one study assessed the composition of the gut microbiome only post-CRT (37).
Dynamic changes of the gut microbial diversity were inconsistent with respect to pelvic RT. Some studies reported lower alpha diversity and different beta diversity during and at the end of RT (22, 38). One study showed significantly lower alpha diversity over time, up to one year after RT (33). Furthermore, other studies found lower alpha diversity and differences in beta diversity post-RT or post-CRT (30, 31, 37, 40). In contrast, some studies did not find significant changes in alpha and beta diversity post-RT or post-CRT (31, 32, 36, 37).
Significant gut microbial taxa were found to be associated with gut microbiome changes during and following pelvic RT compared to pre-RT. At the phylum level, a lower ratio of Firmicutes to Bacteroidetes, a decrease of Firmicutes, and an increase of Fusobacteria were reported during and post-RT compared to pre-RT (22, 30). At the order level, a decrease in the abundance of Clostridiales was observed over the course of RT (38). At the family level, an increase in the abundance of Fusobacteriaceae and Streptococcaceae and a decrease in the abundance of Eubacteriaceae were reported during and post-RT (22). At the genus level, a decrease in Bacteroides, Prevotella_9, Coprococcus, Desulfovibri, Faecalibacterium, Lachnospiracea, Oscillibacter, Roseburia, and Streptococcus and an increase in the abundance of Sutterella, Prevotella_2, Serratia, Pseudomonas, Veillonella, Megamonas, Bacteroides, and Clostridium_XIVa were observed pre- to post-RT (30, 34). In contrast, Sze et al. observed post-treatment samples more closely resembled those of a normal colon (31).
In addition, some studies reported various taxa by comparing different patient groups at post-RT/post-CRT. At the genus level, González-Mercado et al. found higher abundance of Muribaculaceae, Prevotella, Gemella, Bacillales Family XI, Catenibacterium, Sutterella, Pasteurellales, and Pasteurellaceae in Hispanic Puerto Ricans compared to non-Hispanic whites; however, non-Hispanic whites were enriched in Turicibacter and Eubacteriaceae compared to the Hispanic Puerto Ricans at the end of CRT (36). Jang et al. reported higher abundance of Corynebacteriales and Acholeplasmatales at the order level; of Corynebacteriaceae, Deinococcaceae, and Clostridiaceae at the family level; and of Acholeplasma, Oribacterium at the genus level in the complete response group compared to the non-complete response group (37). Also, Youssef et al. reported higher abundance of Lactobacillaceae at the family level and Lactobacillus at the genus level in patients treated with either chemotherapy and/or RT compared with the non-treated control group (32).
A total of seven studies were reviewed to show the associations between the gut microbiome and symptoms/GI toxicities among cancer patients with pelvic RT (Table 3). These studies primarily focused on GI-related symptoms including diarrhea (n = 5), radiation enteritis (n = 1), and GI and urinary toxicities (n = 1).
Studies reported contradictory results on the gut microbiome diversity in patients who were treated with RT and developed GI toxicities. Lower alpha diversity and significant dissimilarities of beta diversity were observed in patients with diarrhea/radiation enteritis post-RT compared to healthy controls and/or patients without diarrhea/radiation enteritis before RT (23, 30, 34). However, one study found that patients with higher GI toxicity had higher alpha diversity over time. Also, significant dissimilar microbiome communities (i.e., beta-diversity) were found between patients with low and high toxicity at week 5 after RT (38).
Various gut microbial taxa were found to be associated with diarrhea, bowel and urinary toxicities, and radiation enteritis. At the phylum level, a higher abundance of Firmicutes, Actinobacteria and Proteobacteria was reported in patients with diarrhea/radiation enteritis after RT compared to patients without diarrhea/radiation enteritis (23, 34). At the family level, cancer patients who developed at least two out of three symptoms (fatigue, depression, and sleep disturbance) had lower Ruminococcaceae (40). At the genus level, the relative abundance of Alistipes, Bacteroides, Clostridium_XI, Erysipelotrichaceae, Escherichia, Lachnospiracea, Roseburia, Megamonas, Clostridium IV, and Phascolarctobacterium were significantly higher, whereas Clostridium_XIVa and Sutterella were lower in patients with diarrhea compared to patients without diarrhea during and after RT (30, 33). Regarding bowel and urinary toxicities, an increase in the abundance of Phascolarctobacterium, Lachnospiraceae, Veillonella, Erysipelotrichaceae, and Fecalitalea was found in patients with high toxicity after RT compared to before RT. Meanwhile, a high abundance of Clostridiales and Desulfovibrio in patients with low toxicity and a high abundance of Sutterella, Finegoldia, and Peptococcaceae (Clostridia) in patients with high toxicity was reported from pre-RT to post-RT (38). González-Mercado et al. showed that cancer patients who developed at least two out of three symptoms had a higher abundance of Bacteroides, Blautia1, Oscillibacter, Lactobacillus, and Blautia2 after CRT (40). In terms of radiation enteritis, the abundances of the of Bacteroidaceae family, Bacteroides genus, and Plebeius species were higher in the non-radiation enteritis group compared to patients with radiation enteritis (34). Furthermore, patients with Grade 1 radiation enteritis had higher abundance of genera Virgibacillus, Alcanivorax, and Phenybacterium; and patients with Grade 2 radiation enteritis were enriched in Coprococcus, Collinsella, and rc4_4 (34).
Two intervention studies were assessed in this review. Ding et al. evaluated the efficacy of fecal microbiome transplant (FMT) on the gut microbiome pattern and symptoms in 5 women with endometrial and cervical cancers. Findings revealed that FMT increased alpha diversity and changed the composition of the microbiome in every recipient (35). Also, FMT can lower the Radiation Therapy Oncology Group/European Organization for Research and Treatment of Cancer (RTOG/EORTC) grade and improve diarrhea, rectal hemorrhage, abdominal/rectal pain, fecal incontinence, and cirrhosis (35). Rosli et al. reported that consumption of 10 g partially hydrolyzed guar gum (PHGG) twice daily from 14 days before RT increased the relative abundance of Bifidobacterium species at the initiation of RT; however, its abundance decreased during RT (39). The impact of PHGG on GI toxicities is unclear (39).
Thirteen studies were systematically synthesized to describe the role pelvic RT plays in influencing the gut microbiome and its associations with symptoms and GI toxicities among patients with various pelvic cancers. These studies indicated that pelvic RT could lead to dysbiosis by altering the diversity and abundance of the gut microbiome. A lower alpha diversity, dissimilarity in beta diversity, and decreased relative abundance of the healthy-associated gut microbes seemed to be associated with GI toxicities such as diarrhea and radiation enteritis. However, these studies have very specific limitations, including small sample size, using different tools to measure toxicities, diversity of patient populations, combination of multiple pelvic cancers at different stages, RT dose, use of concurrent chemotherapy as another systematic treatment, the use of diverse DNA sequencing approaches (e.g., 16S rRNA V1-V9 gene regions), and using different methodologies to describe the gut microbiome diversity and composition (i.e., definition of operational taxonomic unit (OTU), and sequencing read processing), all of which further complicate the interpretation of inconsistent findings. Moreover, cofounding factors such as diet, body mass index (BMI), physical activity, changes in BMI pre- to post-treatment, and use of antibiotics, were not systematically measured in the included studies, which may affect the results.
RT is a core modality used to treat pelvic cancers (41). Pelvic RT can disrupt the gut microbiome and lead to marked changes (e.g., promoting dysbiosis) in the gut microbiome (8, 13). Pre-clinical studies (via animal models) found that RT causes significant changes in the diversity and abundance of the gut microbiome (21), including a significant decrease in Enterobacteriaceae and Lactobacillus groups (20). Current findings were inconsistent in human populations. This systematic review found a lower alpha diversity (e.g., Chao1 index, Shannon index, and observed OTUs) among cancer patients with pelvic RT (22, 30, 33, 38) and significant differences in beta diversity (e.g., Jaccard distance metric) between patients with and without pelvic RT (37, 38); however, these findings were inconsistent with other studies (31, 32, 37). These differences in results can be explained due to small sample size (with a range between 5 and 134), studying various different pelvic cancers (e.g., gynecologic cancers, colorectal cancers, prostate cancer, and other GI cancers), and the use of multiple treatment modalities (e.g., RT alone and CRT). Importantly, the use of various 16S rRNA gene regions (i.e., V1-V9) for the microbiome data sequencing may further explain the discrepancies in these results.
Pelvic RT can lead to a dysbiosis of gut microbiome taxa, with a larger average variation of the relative abundance during RT compared to before RT and after RT (22). Specifically, cancer patients with pelvic RT showed decreased Firmicutes (the phylum level) (22), Eubacteriaceae (the family level) (22), Clostridiales (the order level) (38), Faecalibacterium (the genus level) (30), and increased Fusobacteria (the phylum level) (22), Fusobacteriaceae (the family level) (22), Streptococcaceae (the family level) (22), Pseudomonas (the genus level) (34), Veillonella (the genus level) (34), Megamonas (the genus level) (34), Bacteroides (the genus level) (30), Clostridium_XIVa (the genus level) (30), and Lactobacillus (the genus level) (32) across pelvic RT. These significant changes in the gut microbiome are associated with cytotoxic chemotherapy or RT (8). For example, pelvic RT could lead to a significant change in the health status of the GI tract, including chronic inflammation or abnormal function of the epithelial cells, which might directly affect the gut microbial community. Although previous study has concluded that pelvic RT leads to increases in Bacteroides and Enterobacteriaceae, and decreases in Bifidobacterium, Faecalibacterium prausnitzii, and Clostridium cluster XIVa (13), this study suggested a variety of gut microbial taxa are disrupted during pelvic RT, such as Bifidobacterium, Lactobacillus, and Faecalibacterium prausnitzii, all of which have been examined as probiotics to treat a dysbiotic gut microbiome (8, 42, 43). Due to the limitations of study methodology and study design for this review, these probiotic studies would benefit from a future review.
RT-induced diarrhea affects more than 80% of cancer patients with pelvic RT (44), and this can cause significant treatment delays or dose reductions and diminish patients’ QOL (45, 46). The gut microbiome can impact RT-associated GI toxicity and diarrhea through two primary mechanisms: microbial translocation and dysbiosis (8). Specifically, a dysbiotic gut microbiome can promote the pathogenesis of radiation-induced GI mucositis (inflammation) (13) via modulating the oxidative stress and inflammatory processes, intestinal permeability, mucus layer composition, epithelial repair and ability to resist harmful stimuli, and expression and release of immune effector molecules in the intestine (19). In this study, our findings showed a lower alpha diversity (e.g., Shannon index) of the gut microbiome to be associated with worse diarrhea and radiation enteritis before and after RT (30, 34), as well as a significant difference in beta diversity between patients with low and high GI toxicities after RT (38). Findings of our study further indicated that a higher abundance of Bacteroides, Phascolarctobacterium, and Roseburia was associated with higher GI toxicities while Clostridium XI, XIVa and XVIII, and Faecalibacterium were associated with lower GI toxicities (30, 33, 38). Phascolarctobacterium and Roseburia are short chain fatty acid (SCFA) producers that promote GI homeostasis and whose depletion has been associated with irritable bowel disease; this seems to contradict the findings of our review. One potential hypothesis is that these bacteria are part of an intestinal mucosa–associated community, and increased competition by potentially pathogenic bacteria leads to increased shedding in the stools of patients at risk of RT-induced GI toxicities (33, 47). Clostridia cluster groups (P. 48, 49) and Faecalibacterium genus (50) are potential probiotics with leading roles in the maintenance of gut homeostasis. For example, Clostridia cluster XVIII was linked to promotion of regulatory T-cell expansion and protection from colitis and allergic diarrhea, and this group was significantly less abundant in patients who developed diarrhea (51). Similarly, Faecalibacterium genus includes a protective commensal bacteria, such as Faecalibacterium prausnitzii (50). Therefore, depletion of protective commensal bacteria during pelvic RT can promote much more severe GI toxicities among patients with pelvic cancers.
Pelvic RT for pelvic cancers has frequently been found to increase the risk of radiation enteritis, and this may lead to longer hospitalizations and interruptions to the treatment plans of cancer patients (52). Previous work has examined the impact of RT on the gut microbiome by assuming that changes in the gut microbiome may affect the developmental course of radiation enteropathy (20, 53). Particularly, a linkage between the gut microbiome and RT-induced enteritis has been reported in germ-free animals (54). It is known that inflammatory pathways such as the NF-kB signaling pathway could be activated in response to RT (55), and commensal bacteria may regulate inflammatory responses as well. For instance, Bacteroides thetaiotaomicron and Bifidobacterium infantis are able to suppress NF-kB activation, whereas gut microbes in the Clostridium XIVa group have been reported to attenuate inflammation of the gut epithelium (56, 57). With respect to these promising microbial relationships, strategies such as FMT (35), prebiotics (39), and probiotics (58–60), used for increasing bacterial diversity and promoting the protective commensal bacteria in patients at risk for radiation enteritis, should be tested to understand whether they modify the course of RT-induced enteropathy.
This study has several limitations. According to our results, it is not possible to causatively link any of those significantly identified taxa to the etiology of RT-induced enteropathy. The current study synthesized data related to both acute and late toxicities related to RT. These promising findings should be further examined in a large sample size by considering confounding factors, such as diet, and using standard methods to measure symptoms and gut microbiome profiles so that confirmed findings can be used to improve the clinical practice among patients with cancer receiving pelvic RT. Secondly, radiation enteropathy has multiple causes, which are likely to have differential contributions from the gut microbiome. So far, no objective markers of radiation enteropathy have been defined, and there is no option but to rely on abnormal outcomes, primarily measured by clinician-reported toxicities or patient-reported symptoms. Finally, this study systematically reviewed all the articles using next generation sequencing and published in English only, which may lead to some data selection biases.
Pelvic RT can disrupt the diversity and abundance of commensal gut microorganisms. A dysbiotic gut microbiome showed promising associations with pelvic radiation enteropathy and toxicities through alterations of intestinal barrier function, innate immunity, and intestinal repair mechanisms. The conflicting reports on microbial diversity and abundance may be attributed to cofounding factors within the complicated relationship between the gut microbiome and RT enteropathy. This may limit our ability to clearly define the unique contribution of the gut microbiome in RT enteropathy, and this important relationship warrants further investigations. A better understanding of the role of the gut microbiome in RT-induced symptoms and GI toxicities may lead to new therapeutic approaches and the identification of predictive markers of RT-induced GI toxicities.
The original contributions presented in the study are included in the article/Supplementary Material. Further inquiries can be directed to the corresponding author.
JB: conception of the study, methodology, developing the search strategy, supervision, drafting and editing the article, and funding acquisition. ZB: conception of the study, methodology, screening and extracting data, developing the search strategy, drafting, and editing the article. CR: methodology, screening and extracting data, developing the search strategy, drafting and editing the article. RM: drafting and editing the article. DT: methodology, screening and extracting data, assessing the risk of bias, and completing data synthesis. DB: conception of the study, drafting and editing the article. All authors contributed to the article and approved the submitted version.
This work was partially supported by the National Institutes of Health/National Institute of Nursing Research (1K99NR017897-01 and 4R00NR017897-03).
The authors declare that the research was conducted in the absence of any commercial or financial relationships that could be construed as a potential conflict of interest.
All claims expressed in this article are solely those of the authors and do not necessarily represent those of their affiliated organizations, or those of the publisher, the editors and the reviewers. Any product that may be evaluated in this article, or claim that may be made by its manufacturer, is not guaranteed or endorsed by the publisher.
The authors acknowledge the support from the Emory University, Woodruff Health Science Library in assisting with the literature search.
The Supplementary Material for this article can be found online at: https://www.frontiersin.org/articles/10.3389/fonc.2021.745262/full#supplementary-material
Supplementary Table 1 | Search Strategies.
Supplementary Table 2 | Study Quality Assessment Based on MMAT Methodological Quality Criteria Checklist for Quantitative Studies.
Supplementary Table 3 | Study Quality Assessment Based on MMAT Methodological Quality Criteria Checklist for Randomized Control Trials.
1. Jemal A, Bray F, Center MM, Ferlay J, Ward E, Forman D. Global Cancer Statistics. CA Cancer J Clin (2011) 61(2):69–90. doi: 10.3322/caac.20107
2. Siegel RL, Miller KD, Jemal A. Cancer Statistics 2020. CA Cancer J Clin (2020) 70(1):7–30. doi: 10.3322/caac.21590
3. Hogan NM, Kerin MJ, Joyce MR. Gastrointestinal Complications of Pelvic Radiotherapy: Medical and Surgical Management Strategies. Curr Problems Surg (2013) 50(9):395–407. doi: 10.1067/j.cpsurg.2013.04.004
4. Morris KA, Haboubi NY. Pelvic Radiation Therapy: Between Delight and Disaster. World J Gastrointest Surg (2015) 7(11):279–88. doi: 10.4240/wjgs.v7.i11.279
5. Shah JP, Patel SG, Singh B, Wong R. Jatin Shah’s Head and Neck Surgery and Oncology. Elsevier Health Sciences. New York, NY (2020).
6. American Cancer Society. Cancer Facts & Figures 2019. Atlanta, GA: American Cancer Society (2019). Retrieved Jan 22 from 6. https://www.cancer.org/research/cancer-facts-statistics/all-cancer-facts-figures/cancer-facts-figures-2019.html.
7. Miller KD, Nogueira L, Mariotto AB, Rowland JH, Yabroff KR, Alfano CM, et al. Cancer Treatment and Survivorship Statistics 2019. CA Cancer J Clin (2019) 69(5):363–85. doi: 10.3322/caac.21565
8. Liu J, Liu C, Yue J. Radiotherapy and the Gut Microbiome: Facts and Fiction. Radiat Oncol (2021) 16(1):9. doi: 10.1186/s13014-020-01735-9
9. Zimmerer T, Böcker U, Wenz F, Singer MV. Medical Prevention and Treatment of Acute and Chronic Radiation Induced Enteritis–Is There Any Proven Therapy? A Short Review. Z Gastroenterol (2008) 46(5):441–8. doi: 10.1055/s-2008-1027150
10. Wang Y, Kong W, Lv N, Li F, Chen J, Jiao S, et al. Incidence of Radiation Enteritis in Cervical Cancer Patients Treated With Definitive Radiotherapy Versus Adjuvant Radiotherapy. J Cancer Res Ther (2018) 14(Supplement):S120–s124. doi: 10.4103/0973-1482.163762
11. Suresh U, Smith V, Lupton E, Haboubi N. Radiation Disease of the Urinary Tract: Histological Features of 18 Cases. J Clin Pathol (1993) 46(3):228–31. doi: 10.1136/jcp.46.3.228
12. Muls A, Andreyev J, Lalondrelle S, Taylor A, Norton C, Hart A. Systematic Review: The Impact of Cancer Treatment on the Gut and Vaginal Microbiome in Women With a Gynecological Malignancy. Int J Gynecol Cancer (2017) 27(2):1550–9. doi: 10.1097/igc.0000000000000999
13. Touchefeu Y, Montassier E, Nieman K, Gastinne T, Potel G, Bruley des Varannes S, et al. Systematic Review: The Role of the Gut Microbiota in Chemotherapy- or Radiation-Induced Gastrointestinal Mucositis - Current Evidence and Potential Clinical Applications. Aliment Pharmacol Ther (2014) 40(5):409–21. doi: 10.1111/apt.12878
14. Ursell LK, Metcalf JL, Parfrey LW, Knight R. Defining the Human Microbiome. Nutr Rev (2012) 70 Suppl 1:S38–44. doi: 10.1111/j.1753-4887.2012.00493.x
15. Morgan XC, Huttenhower C. Chapter 12: Human Microbiome Analysis. PLoS Comput Biol (2012) 8(12):e1002808. doi: 10.1371/journal.pcbi.1002808
16. Spor A, Koren O, Ley R. Unravelling the Effects of the Environment and Host Genotype on the Gut Microbiome [10.1038/Nrmicro2540]. Nat Rev: Microbiol (2011) 9(4):279–90. doi: 10.1038/nrmicro2540
17. Bai J, Behera M, Bruner DW. The Gut Microbiome, Symptoms, and Targeted Interventions in Children With Cancer: A Systematic Review. Support Care Cancer (2018) 26(2):427–39. doi: 10.1007/s00520-017-3982-3
18. Bai J, Hu Y, Bruner DW. Composition of Gut Microbiota and Its Association With Body Mass Index and Lifestyle Factors in a Cohort of 7-18 Years Old Children From the American Gut Project. Pediatr Obes (2019) 14(4):e12480. doi: 10.1111/ijpo.12480
19. van Vliet MJ, Harmsen HJM, de Bont ESJM, Tissing WJE. The Role of Intestinal Microbiota in the Development and Severity of Chemotherapy-Induced Mucositis. PLoS Pathog (2010) 6(5):e1000879. doi: 10.1371/journal.ppat.1000879
20. Johnson LB, Riaz AA, Adawi D, Wittgren L, Bäck S, Thornberg C, et al. Radiation Enteropathy and Leucocyte-Endothelial Cell Reactions in a Refined Small Bowel Model. BMC Surg (2004) 4(1):10. doi: 10.1186/1471-2482-4-10
21. Kim YS, Kim J, Park S-J. High-Throughput 16S rRNA Gene Sequencing Reveals Alterations of Mouse Intestinal Microbiota After Radiotherapy. Anaerobe (2015) 33:1–7. doi: 10.1016/j.anaerobe.2015.01.004
22. Nam YD, Kim HJ, Seo JG, Kang SW, Bae JW. Impact of Pelvic Radiotherapy on Gut Microbiota of Gynecological Cancer Patients Revealed by Massive Pyrosequencing. PLoS One (2013) 8(12):e82659. doi: 10.1371/journal.pone.0082659
23. Manichanh C, Varela E, Martinez C, Antolin M, Llopis M, Doré J, et al. The Gut Microbiota Predispose to the Pathophysiology of Acute Postradiotherapy Diarrhea. Am J Gastroenterol (2008) 103(7):1754–61. doi: 10.1111/j.1572-0241.2008.01868.x
24. Guo H, Chou W-C, Lai Y, Liang K, Tam JW, Brickey WJ, et al. Multi-Omics Analyses of Radiation Survivors Identify Radioprotective Microbes and Metabolites. Science (2020) 370(6516):eaay9097. doi: 10.1126/science.aay9097
25. Tonneau M, Elkrief A, Pasquier D, Del Socorro TP, Chamaillard M, Bahig H, et al. The Role of the Gut Microbiome on Radiation Therapy Efficacy and Gastrointestinal Complications: A Systematic Review. Radiother Oncol (2020) 156:1–9. doi: 10.1016/j.radonc.2020.10.033
26. Moher D, Liberati A, Tetzlaff J, Altman DG. Preferred Reporting Items for Systematic Reviews and Meta-Analyses: The PRISMA Statement. BMJ (2009) 339:b2535. doi: 10.1136/bmj.b2535
27. Haussler SC. The Johns Hopkins Nursing Evidence-Based Practice Model and Guidelines. The Journal of Continuing Education in Nursing (2008) 39(9):432. doi: 10.3928/00220124-20080901-08
28. Pluye P, Gagnon M-P, Griffiths F, Johnson-Lafleur J. A Scoring System for Appraising Mixed Methods Research, and Concomitantly Appraising Qualitative, Quantitative and Mixed Methods Primary Studies in Mixed Studies Reviews. Int J Nurs Stud (2009) 46(4):529–46. doi: 10.1016/j.ijnurstu.2009.01.009
29. Pace R, Pluye P, Bartlett G, Macaulay AC, Salsberg J, Jagosh J, et al. Testing the Reliability and Efficiency of the Pilot Mixed Methods Appraisal Tool (MMAT) for Systematic Mixed Studies Review. Int J Nurs Stud (2012) 49(1):47–53. doi: 10.1016/j.ijnurstu.2011.07.002
30. Wang A, Ling Z, Yang Z, Kiela PR, Wang T, Wang C, et al. Gut Microbial Dysbiosis may Predict Diarrhea and Fatigue in Patients Undergoing Pelvic Cancer Radiotherapy: A Pilot Study. PLoS One (2015) 10(5):e0126312. doi: 10.1371/journal.pone.0126312
31. Sze MA, Baxter NT, Ruffin MT, Rogers MA, Schloss PD. Normalization of the Microbiota in Patients After Treatment for Colonic Lesions. Microbiome (2017) 5(1):1–10. doi: 10.1186/s40168-017-0366-3
32. Youssef O, Lahti L, Kokkola A, Karla T, Tikkanen M, Ehsan H, et al. Stool Microbiota Composition Differs in Patients With Stomach, Colon, and Rectal Neoplasms. Dig Dis Sci (2018) 63(11):2950–8. doi: 10.1007/s10620-018-5190-5
33. Ferreira MR, Andreyev HJN, Mohammed K, Truelove L, Gowan SM, Li J, et al. Microbiota-And Radiotherapy-Induced Gastrointestinal Side-Effects (MARS) Study: A Large Pilot Study of the Microbiome in Acute and Late-Radiation Enteropathy. Clin Cancer Res (2019) 25(21):6487–500. doi: 10.1158/1078-0432.CCR-19-0960
34. Wang Z, Wang Q, Wang X, Zhu L, Chen J, Zhang B, et al. Gut Microbial Dysbiosis Is Associated With Development and Progression of Radiation Enteritis During Pelvic Radiotherapy. J Cell Mol Med (2019) 23(5):3747–56. doi: 10.1111/jcmm.14289
35. Ding X, Li Q, Li P, Chen X, Xiang L, Bi L, et al. Fecal Microbiota Transplantation: A Promising Treatment for Radiation Enteritis? Radiother Oncol (2020) 143:12–8. doi: 10.1016/j.radonc.2020.01.011
36. González-Mercado VJ, Lim J, Berk L, Esele M, Rodríguez CS, Colón-Otero G. Gut Microbiota Differences in Island Hispanic Puerto Ricans and Mainland Non-Hispanic Whites During Chemoradiation for Rectal Cancer: A Pilot Study. Curr Probl Cancer (2020) 44(4):100551. doi: 10.1016/j.currproblcancer.2020.100551
37. Jang B-S, Chang JH, Chie EK, Kim K, Park JW, Kim MJ, et al. Gut Microbiome Composition Is Associated With a Pathologic Response After Preoperative Chemoradiation in Patients With Rectal Cancer. Int J Radiat Oncol Biol Phys (2020) 107(4):736–46. doi: 10.1016/j.ijrobp.2020.04.015
38. Mitra A, Biegert GWG, Delgado AY, Karpinets TV, Solley TN, Mezzari MP, et al. Microbial Diversity and Composition Is Associated With Patient-Reported Toxicity During Chemoradiation Therapy for Cervical Cancer. Int J Radiat Oncol Biol Phys (2020) 107(1):163–71. doi: 10.1016/j.ijrobp.2019.12.040
39. Rosli D, Shahar S, Manaf ZA, Lau HJ, Yusof NYM, Haron MR, et al. Randomized Controlled Trial on the Effect of Partially Hydrolyzed Guar Gum Supplementation on Diarrhea Frequency and Gut Microbiome Count Among Pelvic Radiation Patients. J Parenter Enteral Nutr (2020) 45(2):277–86. doi: 10.1002/jpen.1987
40. González-Mercado VJ, Henderson WA, Sarkar A, Lim J, Saligan LN, Berk L, et al. Changes in Gut Microbiome Associated With Co-Occurring Symptoms Development During Chemo-Radiation for Rectal Cancer: A Proof of Concept Study. Biol Res For Nurs (2021) 23(1):31–41. doi: 10.1177/1099800420942830
41. Ahmad SS, Duke S, Jena R, Williams MV, Burnet NG. Advances in Radiotherapy. BMJ Br Med J (2012) 345:e7765. doi: 10.1136/bmj.e7765
42. Mohania D, Kansal VK, Kruzliak P, Kumari A. Probiotic Dahi Containing Lactobacillus Acidophilus and Bifidobacterium Bifidum Modulates the Formation of Aberrant Crypt Foci, Mucin-Depleted Foci, and Cell Proliferation on 1, 2-Dimethylhydrazine-Induced Colorectal Carcinogenesis in Wistar Rats. Rejuvenation Res (2014) 17(4):325–33. doi: 10.1089/rej.2013.1537
43. Pandey KR, Naik SR, Vakil BV. Probiotics, Prebiotics and Synbiotics-A Review. J Food Sci Technol (2015) 52(12):7577–87. doi: 10.1007/s13197-015-1921-1
44. Demers M, Dagnault A, Desjardins J. A Randomized Double-Blind Controlled Trial: Impact of Probiotics on Diarrhea in Patients Treated With Pelvic Radiation. Clin Nutr (2014) 33(5):761–7. doi: 10.1016/j.clnu.2013.10.015
45. Benson AB, Ajani JA, Catalano RB, Engelking C, Kornblau SM, Martenson JA Jr, et al. Recommended Guidelines for the Treatment of Cancer Treatment-Induced Diarrhea. J Clin Oncol (2004) 22(14):2918–26. doi: 10.1200/jco.2004.04.132
46. Dalsania RM, Shah KP, Stotsky-Himelfarb E, Hoffe S, Willingham FF. Management of Long-Term Toxicity From Pelvic Radiation Therapy. American Society of Clinical Oncology Educational Book (2021) 41:147–57. doi: 10.1200/edbk_323525
47. Machiels K, Joossens M, Sabino J, De Preter V, Arijs I, Eeckhaut V, et al. A Decrease of the Butyrate-Producing Species Roseburia Hominis and Faecalibacterium Prausnitzii Defines Dysbiosis in Patients With Ulcerative Colitis. Gut (2014) 63(8):1275–83. doi: 10.1136/gutjnl-2013-304833
48. Guo P, Zhang K, Ma X, He P. Clostridium Species as Probiotics: Potentials and Challenges. J Anim Sci Biotechnol (2020) 11(1):24. doi: 10.1186/s40104-019-0402-1
49. Lopetuso LR, Scaldaferri F, Petito V, Gasbarrini A. Commensal Clostridia: Leading Players in the Maintenance of Gut Homeostasis. Gut Pathog (2013) 5(1):23–3. doi: 10.1186/1757-4749-5-23
50. Sokol H, Thomas M, Wells J, Langella P. Faecalibacterium Prausnitzii and Human Intestinal Health S Miquel, R Martın, O Rossi 3, LG Bermu Dez-Humara N, JM Chatel. Curr Opin Microbiol (2013) 16:1–7. doi: 10.1016/j.mib.2013.06.003
51. Atarashi K, Tanoue T, Oshima K, Suda W, Nagano Y, Nishikawa H, et al. T Reg Induction by a Rationally Selected Mixture of Clostridia Strains From the Human Microbiota. Nature (2013) 500(7461):232–6. doi: 10.1038/nature12331
52. Bismar MM, Sinicrope FA. Radiation Enteritis. Curr Gastroenterol Rep (2002) 4(5):361–5. doi: 10.1007/s11894-002-0005-3
53. Husebye E, Skar V, Høverstad T, Iversen T, Melby K. Abnormal Intestinal Motor Patterns Explain Enteric Colonization With Gram-Negative Bacilli in Late Radiation Enteropathy. Gastroenterology (1995) 109(4):1078–89. doi: 10.1016/0016-5085(95)90565-0
54. Crawford PA, Gordon JI. Microbial Regulation of Intestinal Radiosensitivity. Proc Natl Acad Sci (2005) 102(37):13254–9. doi: 10.1073/pnas.0504830102
55. Sonis ST. The Biologic Role for Nuclear Factor-kappaB in Disease and its Potential Involvement in Mucosal Injury Associated With Anti-Neoplastic Therapy. Crit Rev Oral Biol Med (2002) 13(5):380–9. doi: 10.1177/154411130201300502
56. Beg AA. ComPPARtmentalizing NF-κb in the Gut. Nat Immunol (2004) 5(1):14–6. doi: 10.1038/ni0104-14
57. Matsumoto M, Benno Y. The Relationship Between Microbiota and Polyamine Concentration in the Human Intestine: A Pilot Study. Microbiol Immunol (2007) 51(1):25–35. doi: 10.1111/j.1348-0421.2007.tb03887.x
58. Blanarova C, Galovicova A, Petrasova D. Use of Probiotics for Prevention of Radiation-Induced Diarrhea. Bratisl Lek Listy (2009) 110(2):98–104.
59. Chitapanarux I, Chitapanarux T, Traisathit P, Kudumpee S, Tharavichitkul E, Lorvidhaya V. Randomized Controlled Trial of Live Lactobacillus Acidophilus Plus Bifidobacterium Bifidum in Prophylaxis of Diarrhea During Radiotherapy in Cervical Cancer Patients. Radiat Oncol (2010) 5:31. doi: 10.1186/1748-717x-5-31
60. Giralt J, Regadera JP, Verges R, Romero J, de la Fuente I, Biete A, et al. Effects of Probiotic Lactobacillus Casei DN-114 001 in Prevention of Radiation-Induced Diarrhea: Results From Multicenter, Randomized, Placebo-Controlled Nutritional Trial. Int J Radiat Oncol Biol Phys (2008) 71(4):1213–9. doi: 10.1016/j.ijrobp.2007.11.009
Keywords: cancer, gut microbiome, gastrointestinal side effects, treatment toxicity, pelvic radiation therapy
Citation: Bai J, Barandouzi ZA, Rowcliffe C, Meador R, Tsementzi D and Bruner DW (2021) Gut Microbiome and Its Associations With Acute and Chronic Gastrointestinal Toxicities in Cancer Patients With Pelvic Radiation Therapy: A Systematic Review. Front. Oncol. 11:745262. doi: 10.3389/fonc.2021.745262
Received: 21 July 2021; Accepted: 22 November 2021;
Published: 06 December 2021.
Edited by:
Christina Tsien, Johns Hopkins Medicine, United StatesReviewed by:
Adam C. Olson, University of Pittsburgh Medical Center, United StatesCopyright © 2021 Bai, Barandouzi, Rowcliffe, Meador, Tsementzi and Bruner. This is an open-access article distributed under the terms of the Creative Commons Attribution License (CC BY). The use, distribution or reproduction in other forums is permitted, provided the original author(s) and the copyright owner(s) are credited and that the original publication in this journal is cited, in accordance with accepted academic practice. No use, distribution or reproduction is permitted which does not comply with these terms.
*Correspondence: Jinbing Bai, amluYmluZy5iYWlAZW1vcnkuZWR1
Disclaimer: All claims expressed in this article are solely those of the authors and do not necessarily represent those of their affiliated organizations, or those of the publisher, the editors and the reviewers. Any product that may be evaluated in this article or claim that may be made by its manufacturer is not guaranteed or endorsed by the publisher.
Research integrity at Frontiers
Learn more about the work of our research integrity team to safeguard the quality of each article we publish.